- 1Department of Basic Nursing Science, College of Nursing, Korea University, Seoul, Republic of Korea
- 2BK21 FOUR Program of Transdisciplinary Major in Learning Health Systems, Graduate School, Korea University, Seoul, Republic of Korea
Mild cognitive impairment (MCI) is a major public health challenge with an increasing prevalence. Although the mechanisms underlying the development of MCI remain unclear, MCI has been reported to be associated with oxidative stress, inflammatory responses, and endothelial dysfunction, suggesting that agents that reduce these factors may be key to preventing MCI. Currently, no agents have been approved for the treatment of MCI, with the efficacy of commonly prescribed cholinesterase inhibitors remaining unclear. Relatively safe natural products that can prevent the development of MCI are of great interest. Linalyl acetate (LA), the major component of clary sage and lavender essential oils, has been shown to have a variety of pharmacological effects, including anti-hypertensive, anti-diabetic, neuroprotective, anti-inflammatory, and antioxidant properties, which may have the potential for the prevention of MCI. The present review briefly summarizes the pathogenesis of MCI related to oxidative stress, inflammatory responses, and endothelial dysfunction as well as the benefits of LA against these MCI-associated factors. The PubMed and Google Scholar databases were used to search the relevant literature. Further clinical research may lead to the development of new strategies for preventing MCI, particularly in high-risk populations with oxidative stress, inflammatory responses, and endothelial dysfunction (e.g., patients with hypertension and/or diabetes mellitus).
1 Introduction
Mild cognitive impairment (MCI) is a condition in which individual experiences a moderate cognitive decline greater than that expected during normal aging (Anderson, 2019). The global prevalence of MCI in community-dwelling adults has been estimated to be about 15.6% (Bai et al., 2022). The number of people living with MCI in 2020 in the United States was 12.2 million, which has been estimated to increase to 21.6 million by 2060 (Rajan et al., 2021). Individuals with MCI have a poorer quality of life and a greater cost burden than healthy individuals (Tahami Monfared et al., 2022), with a large proportion of caregivers of individuals with MCI reporting caregiver burden (Connors et al., 2019). Moreover, approximately one-third of people with MCI progress to dementia (Glynn et al., 2021). Preventing the development of MCI may therefore prevent possible progression to dementia in aging populations.
Although the exact mechanisms underlying the development of MCI remain unclear, oxidative stress is frequently observed in MCI patients. Modifications of protein structures (e.g., α-enolase, glucose-regulated protein precursor) induced by oxidative stress were found to be more frequent in MCI brains than in control brains (Butterfield, 2023), and oxidative stress and nitrosative stress/antioxidant ratio were reported to be higher in individuals with MCI than in healthy controls (Nantachai et al., 2022). MCI patients showed significantly increased serum malondialdehyde (MDA) levels compared with age-matched healthy controls (Padurariu et al., 2010). In addition, nicotinamide adenine dinucleotide phosphate oxidase (NOX) 2 expression in the brain vascular fraction was significantly elevated in patients with vascular cognitive impairment compared to control cases suggesting vascular contribution to cognitive decline (Alfieri et al., 2022). In addition, oxidative stress was found to be the mechanistic link between age-related disorders and MCI. For example, hypertension-induced reactive oxygen species (ROS) cause oxidative damage to cerebral endothelial cells, resulting in blood-brain barrier (BBB) disruption (Ungvari et al., 2021). Moreover, in patients with the common age-related metabolic disorder diabetes mellitus (Natarajan et al., 2020), peripheral metabolic alterations increase oxidative stress and neuro-inflammation, which negatively affect cognitive function (Dutta et al., 2022).
Although several studies have reported that oxidative stress plays an important role in MCI, clinical studies of antioxidants in MCI patients have yielded inconsistent results (Alavi Naeini et al., 2014; Rita Cardoso et al., 2016). The discrepancies among these studies may stem from the multifactorial characteristics of MCI. For example, one study found that brachial flow-mediated dilatation was significantly related to MCI in an elderly population, suggesting a pivotal role of endothelial dysfunction in MCI (Vendemiale et al., 2013). Another study, however, found that serum inflammatory markers such as interleukin (IL)-6 and high-sensitivity C-reactive protein levels were associated with the risk of MCI in patients with type 2 diabetes mellitus (Zheng et al., 2019). And a third study reported that MCI patients with high plasma matrix metalloproteinase (MMP)-9 levels show a faster rate of cognitive decline (Abe et al., 2020). Another consideration when exploring the factors giving rise to MCI is that oxidative stress, inflammation, and endothelial dysfunction are closely linked (Higashi, 2022). Given the multifactorial nature of MCI, in this review we explore the notion that reducing oxidative stress, inflammation, and endothelial dysfunction, not just oxidative stress, may be key to preventing MCI.
To date, no drugs have been found that cure MCI (Alvi et al., 2022). Cholinesterase inhibitors are commonly used to delay progression of MCI or to improve cognition in MCI patients, but their efficacy remains inconclusive. For example, although donepezil showed limited efficacy in improving the cognitive function of individuals with MCI, it could not delay MCI progression (Zhang et al., 2022). Moreover, donepezil was associated with significantly higher rates of adverse effects, such as diarrhea and vomiting, than placebo (Zhang et al., 2022). Efforts are underway to develop complementary and integrative therapeutic regimens using natural products for cognitive disorders as such products are perceived to constitute a safer and more natural option than conventional medicine (Nguyen et al., 2022). For instance, mangiferin, a natural glucoxilxanthone, has been found to show protective effects against memory impairment in animals and humans, without any side effects at the selected doses (Lum et al., 2021). Also, previous studies have suggested that the naturally occurring compounds genistein and celastrol are promising molecules for the development of neuroprotective drugs (Fuloria et al., 2022; Amir Yusri et al., 2023).
Linalyl acetate (3,7-dimethyl-1,6-octadien-3-yl acetate; LA) is a major volatile component of the essential oils of Salvia sclarea (clary sage) and Lavandula angustifolia (lavender) (Seol et al., 2013). LA is used as a fragrance ingredient in shampoos, detergents, and cosmetic products (Letizia et al., 2003). Humans are exposed to LA not only through fragrances but also through the consumption of flavored teas such as Earl Grey tea, a cup of which contains 0.2 mg of LA (Orth et al., 2014). Repeated-dose and reproductive toxicity studies showed that exposure to 36 mg/kg/day and 200 mg/kg/day LA, respectively, did not have any adverse effects (Api et al., 2015). Computational analyses showed that LA was neither a substrate nor an inhibitor of cytochromes involved in the metabolism of neuropsychiatric drugs (Avram et al., 2021). In addition, the intake of lavender essential oil (0.03 mL/kg), which contains a significant amount of LA, for 2 weeks did not have any disruptive effects on lipid profiles or liver enzymes in healthy athletes (Maral et al., 2022).
LA has been reported to have various therapeutic properties, including analgesic (Yu and Seol, 2017; Scuteri et al., 2022), antispasmodic (Rombolà et al., 2022), anti-psoriatic (Rai et al., 2020) and antibacterial (Mirzaei-Najafgholi et al., 2017; Ramić et al., 2021) effects. We have previously reported that LA is effective in preventing hypertension (Hsieh et al., 2018; Hsieh et al., 2019; Shin et al., 2022) and diabetes mellitus (Shin et al., 2018; Shin et al., 2020), which are risk factors for MCI. Moreover, LA has shown antioxidant and anti-inflammatory properties, as well as the ability to treat endothelial dysfunction (see Sections 3–5), suggesting that LA may have potential in preventing MCI. The present review briefly summarizes the roles of oxidative stress, inflammation, and endothelial dysfunction in MCI, as well as the benefits of LA in treating these MCI-associated factors. Additionally, because anxiety and mood disorders such as depression can affect cognition (Gulpers et al., 2019; Yuan et al., 2023), this review also considers the effects of LA on mood and cognition.
2 Oxidative stress, inflammation and endothelial dysfunction related to MCI
Epidemiological studies have suggested that hypertension and diabetes mellitus are risk factors for MCI. For example, cognitive performance was significantly lower in participants with uncontrolled hypertension than in other subjects (Lespinasse et al., 2022). In addition, the risk of MCI was significantly lower in hypertensive patients who did than did not effectively control their blood pressure (Yan et al., 2022). Mechanisms by which hypertension and diabetes mellitus can contribute to MCI include oxidative stress, inflammatory processes, and endothelial dysfunction (Ungvari et al., 2021). Hypertension has been found to increase NOX activity in vascular endothelial cells, thereby promoting ROS formation (Drummond and Sobey, 2014), and hyperglycemia due to diabetes mellitus stimulates ROS production via the advanced glycation end product, polyol, hexosamine, and protein kinase C pathways (Ighodaro, 2018). Increased oxidative stress can lead to MMP activity in brain tissue, which ultimately disrupts BBB integrity (Nath et al., 2019), and increased BBB permeability induces the activation of microglia (Ju et al., 2018). Activated microglia, in turn, produce inflammatory mediators, which further affect neuro-inflammation and neurodegeneration (Shabab et al., 2017).
Oxidative stress can also interact with inflammatory responses, creating a vicious cycle that increases vascular endothelial dysfunction (Higashi, 2022). Oxidative stress due to an imbalance between ROS production and NO bioavailability results in endothelial dysfunction, ultimately leading to cardiovascular complications, including arterial stiffness (Burgos-Morón et al., 2019). Increased arterial stiffness contributes to the development of cerebrovascular dysfunction (Barnes and Corkery, 2018), which ultimately leads to cognitive impairment. In addition, inflammation mediated by ROS can increase BBB permeability, which increases the infiltration of immune cells, glial activation, and neuronal damage, further promoting neuro-inflammation (Van Dyken and Lacoste, 2018). Moreover, endothelial dysfunction can lead to endothelial activation (Liao, 2013). Proinflammatory cytokines, chemokines, and adhesion molecules are upregulated in activated endothelial cells, leading to inflammatory processes in blood vessels (Sun et al., 2020). Inflammation-induced oxidative stress increases ROS production in endothelial cells (Scioli et al., 2020). These findings indicate that oxidative stress and inflammation lead to endothelial dysfunction, which in turn enhances inflammatory responses and oxidative stress, forming a vicious cycle that may be associated with MCI (Figure 1).
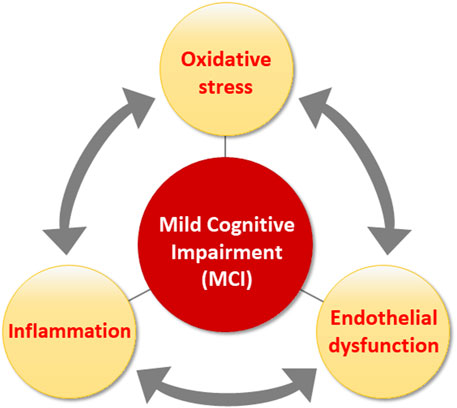
FIGURE 1. Overview of the relationships among oxidative stress, inflammation and endothelial dysfunction, factors involved in the pathology of MCI.
3 Effects of LA on oxidative stress
Several in vivo and in vitro studies have shown that LA has antioxidant properties. Specifically, LA has been shown to have high peroxyl radical scavenger ability in vitro (Cutillas et al., 2018); to exert antioxidant effects in a rat model of combined hypertension and chronic obstructive pulmonary disease (COPD) by reducing MDA and lactate dehydrogenase (LDH) levels in serum (Hsieh et al., 2019); to inhibit cardiovascular disruption in rats treated with acute nicotine by restoring abnormally decreased heart rate and by reducing serum nitrite and LDH levels (Kim et al., 2017); to reduce systolic blood pressure in a rat model of hypertension-ischemia injury, a model that included the attenuation of p47phox overexpression, ROS overproduction, and LDH release in the aorta (Hsieh et al., 2018); and to improve oxidative damage by reducing MDA levels in the liver tissue of diabetic rats (Shin et al., 2020). Moreover, under conditions mimicking Ca2+-related ischemic injury, LA was found to decrease NOX2 expression, ROS generation, and LDH release in microglial cells and to reduce p47phox expression and LDH release in neuron-like cells, suggesting the protective roles of LA on the neurovascular unit (Hsieh et al., 2021).
Ca2+ is an intracellular second messenger that plays an important role in regulating cellular functions (Bootman and Bultynck, 2020). Agonist-induced Ca2+ entry into vascular endothelial cells induces nitric oxide (NO) production by endothelial nitric oxide synthase (eNOS) (Wei et al., 2018), and intracellular Ca2+ contributes to the contraction of vascular smooth muscle cells (Chen Y. L. et al., 2022). Neuronal processes, including the release of neurotransmitters and synaptic plasticity, are dependent on the fine-tuned regulation of intracellular Ca2+ levels (Brini et al., 2014). However, high concentrations of intracellular Ca2+ increase the activities of respiratory chain complexes, leading to excessive ROS formation, which further increases Ca2+ release from the endoplasmic reticulum (Görlach et al., 2015). LA has been reported to inhibit Ca2+ influx into human umbilical vein endothelial cells, indicating that it may have possible protective effects against endothelial dysfunction (You et al., 2013). Taken together, these findings demonstrating the antioxidant properties of LA suggest that LS has the potential to prevent MCI.
4 Effects of LA on inflammation
LA has also been found to have significant anti-inflammatory effects. LA has been shown to reduce skin levels of IL-1β and tumor necrosis factor (TNF)-α in mice with psoriasis-like skin lesions (Rai et al., 2020); to inhibit the activation of caspase-1 and nuclear factor-κB (NF-κB) in a human mast cell line exposed to inflammatory stimuli (Moon et al., 2018); to inhibit the expression of cell adhesion molecules and NF-κB activation in murine brain endothelial cells stimulated with TNF-α (Aoe et al., 2017); and to exert anti-inflammatory effects in a rat model of combined COPD and hypertension by reducing TNF-α, IL-6, and MMP-9 levels in bronchoalveolar lavage fluid (Hsieh et al., 2019). Moreover, LA was effective in reducing systolic and diastolic blood pressure in rats with repeatedly stressed-ulcerative colitis, as well as reducing serum IL-6 concentrations (Shin et al., 2022). LA also significantly reduced serum glucose levels in rats with streptozotocin-induced diabetes exposed to chronic immobilization stress, which resulted from increased liver AMP-activated protein kinase expression, decreased liver NF-κB expression, and excessive amounts of serum nitrite (Shin et al., 2018).
A sustained increase in intracellular Ca2+ levels has been associated with the activation of microglia (Brawek and Garaschuk, 2013), further emphasizing the importance of maintaining intracellular Ca2+ homeostasis. In this regard, LA has been shown to decrease store-operated Ca2+ entry elevation in microglial cells under the conditions of muscarinic receptor blockade and inflammatory stimulus (Kim et al., 2022).
Skeletal muscle releases several myokines, including insulin-like growth factor (IGF)-1, which have pleiotropic effects. IGF-1 is responsible for maintaining skeletal muscle mass (Bian et al., 2020), and has been shown to have anti-inflammatory and antioxidant properties (Sukhanov et al., 2007). IGF-1 deficient mice exhibited significantly reduced expression of Nrf2 in the aorta, which makes the vasculature susceptible to oxidative stress (Bailey-Downs et al., 2012). Also, mice with IGF-1 deficiency showed increased Tnfa and Il1b mRNAs in the retina indicating persistent inflammation (Arroba et al., 2016). In addition, activation of the IGF-1/PI3K/AKT/GSK-3β pathway was shown to attenuate neuro-inflammation and cognitive impairment after sleep deprivation (Wan et al., 2022). LA was shown to prevent losses in body weight and gastrocnemius muscle weight in rats with rheumatoid arthritis exposed to chronic nicotine treatment. Mechanistically, LA increased muscle fiber cross-sectional area and serum IGF-1 levels in these rats and decreased serum IL-6 levels and mitochondrial membrane potential in the gastrocnemius muscle. Moreover, LA was more potent than lavender essential oil in enhancing serum IGF-1 concentrations (Seo et al., 2021). These findings indicate that LA is an effective anti-inflammatory agent, which may attenuate the cascade of events leading to MCI.
5 Effects of LA on endothelial dysfunction
LA has also been shown to reduce endothelial dysfunction. For example, LA reduced ROS-induced eNOS suppression in the aorta in a rat model of hypertension-ischemia injury (Hsieh et al., 2018); improved endothelial function by increasing eNOS expression and acetylcholine (ACh)-induced vasorelaxation in the aortas of diabetic rats (Shin et al., 2018); and increased NO and decreased MMP-9 expression, a key marker of BBB disruption, under conditions mimicking Ca2+-related ischemic injury in a mouse brain endothelial cell line (Hsieh et al., 2021). Taken together, these findings suggest that LA may prevent MCI associated with muscle wasting. Overall, the findings presented in Sections 3–5 suggest that LA has the potential to prevent the development of MCI by reducing oxidative stress, inflammation, and endothelial dysfunction (Table 1).
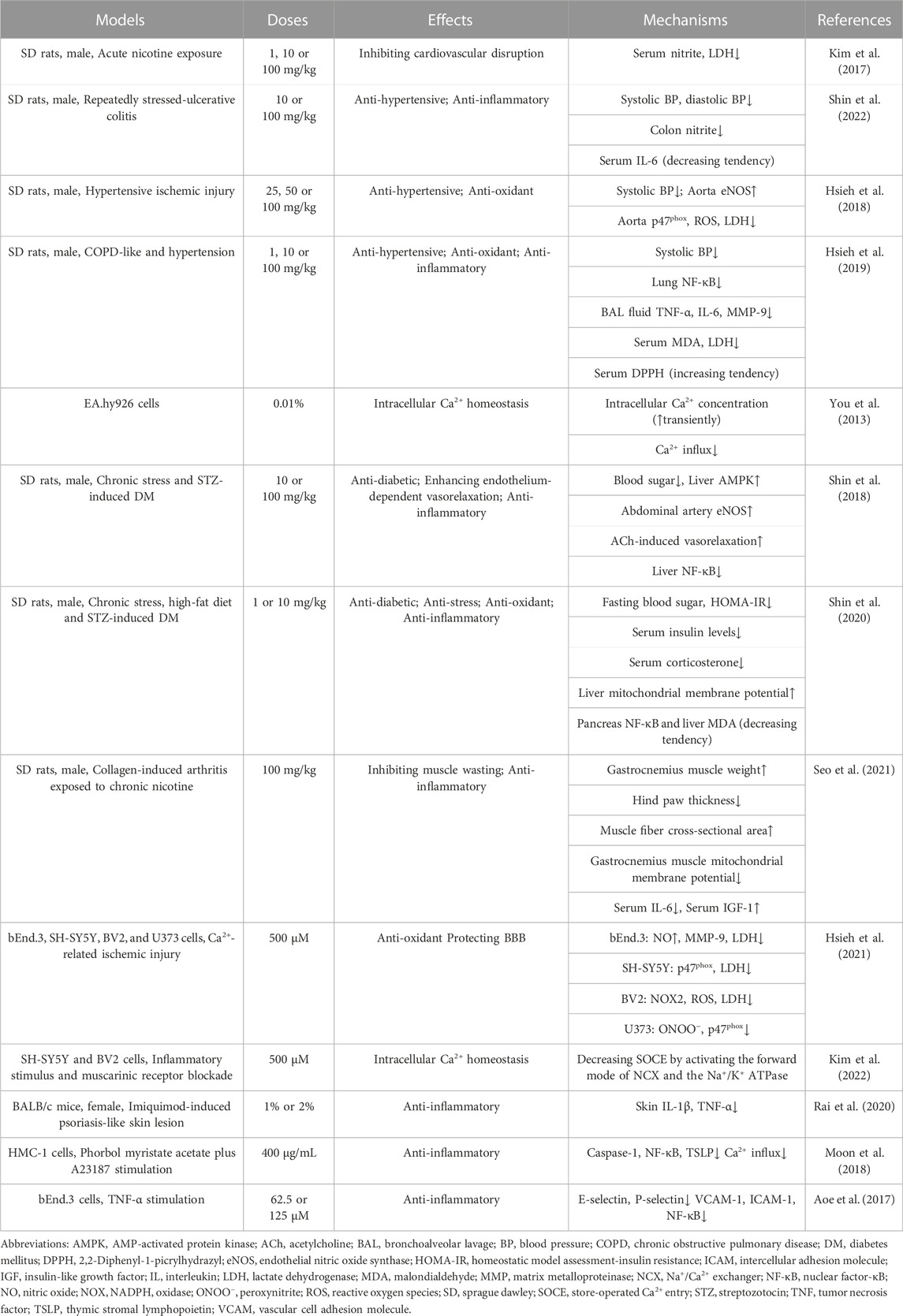
TABLE 1. Summary of the effects of LA on oxidative stress, inflammation and endothelial dysfunction.
6 Effects of LA on mood and cognition
Anxiety and depression are common in individuals with MCI, thereby increasing the risk of progression to dementia (Ma, 2020). Moreover, psychological distress and depression have been identified as longitudinal predictors of cognitive decline in older adults (Freire et al., 2017).
LA has been shown to have positive effects on mood. Inhalation of LA by cancer patients prior to chemotherapy decreased the anxiety-visual analogue scale (VAS) and the stress-VAS scores in cancer patients, suggesting that LA has anti-anxiety and anti-stress effects (Kim et al., 2021). Inhalation of lavender, petitgrain, or bergamot essential oil containing large amounts of LA was found to reduce the Anger-Hostility and the Tension-Anxiety scores in pregnant women (Igarashi, 2013). Lavender essential oil showed anxiolytic effects in mice by increasing the time spent in the open arm of the elevated plus maze test (Schuwald et al., 2013). Clary sage essential oil containing LA as its major bioactive component showed an antidepressant-like effect by reducing immobility time in the forced swimming test, an effect blocked by a dopamine antagonist (Seol et al., 2010). A study using bioinformatics tools found that LA appeared to modulate serotonin transporters and to have a strong affinity to serotonin 1A and dopamine D2 receptors, indicating that LA may be promising as an antidepressant (Avram et al., 2021). In addition, the abilities of LA to increase parasympathetic activity (Igarashi, 2013), inhibit Ca2+ influx (Schuwald et al., 2013), and inhibit sedative activity (Buchbauer et al., 1991) may be related to its mood-enhancing effects.
Studies have reported that LA may play a role in cognitive function. Because proper Ca2+ signaling is important in regulating neuronal function, such as synaptic plasticity, abnormal Ca2+ signaling can lead to synaptic loss in neurodegenerative diseases (Pchitskaya et al., 2018). Exposure of mice to lipopolysaccharide (LPS) decreased learning and memory functions, through mechanisms associated with increased ROS production and NOX2 expression in brain tissue, as well as neuronal inflammation (Dong et al., 2021). Moreover, exposure to LPS significantly increased intracellular Ca2+ overload in a murine hippocampal cell line showing Ca2+ dyshomeostasis (Dong et al., 2021). LA also reduced store-operated Ca2+ entry elevation induced by muscarinic receptor inhibition and inflammatory stimuli by activating the forward mode of Na+/Ca2+ exchanger and Na+/K+ pump in neuron-like and microglial cells (Kim et al., 2022). Under conditions mimicking Ca2+-related ischemic injury, LA decreased p47phox expression and LDH release in neuron-like cells and reduced NOX2 expression, ROS generation, and LDH release in microglial cells (Hsieh et al., 2021). These findings indicate that LA can protect neurons and microglia against oxidative stress and inflammatory responses.
Acetylcholine is a cholinergic neurotransmitter that plays an important role in cognitive function (Chen Z. R. et al., 2022). However, acetylcholinesterase (AChE), an enzyme whose primary function is to degrade acetylcholine, inhibits postsynaptic signal transmission (Singh and Gupta, 2017). In the absence of AChE, butyrylcholinesterase (BChE) can compensate, by hydrolyzing acetylcholine (Mesulam et al., 2002). LA has been reported to have AChE and BChE inhibitory activities, with IC50 values of 82 μg/mL (Miyazawa et al., 1998) and 169 μg/mL (Bonesi et al., 2010), respectively. Molecular docking simulations also showed that LA had a high affinity for the binding site of BChE (Lobine et al., 2021), further suggesting that LA may be useful for improving cognitive function in subjects with MCI.
7 Overall effects of LA against MCI-associated factors
In summary, LA has proved to have antioxidant effect by reducing NOX2 (Hsieh et al., 2021), p47phox (Hsieh et al., 2018; Hsieh et al., 2021), ROS (Hsieh et al., 2018; Hsieh et al., 2021), MDA (Hsieh et al., 2019), peroxynitrite (ONOO−) (Hsieh et al., 2021), and intracellular Ca2+ levels (You et al., 2013; Moon et al., 2018; Kim et al., 2022). Also, LA has been reported to have anti-inflammatory activity by reducing NF-κB activation (Aoe et al., 2017; Moon et al., 2018; Shin et al., 2018; Hsieh et al., 2019), TNF-α production (Hsieh et al., 2019; Rai et al., 2020), IL-6 production (Hsieh et al., 2019; Seo et al., 2021), IL-1β production (Rai et al., 2020), MMP-9 production (Hsieh et al., 2019; Hsieh et al., 2021), and E-selectin, P-selectin, vascular cell adhesion molecule-1, and intercellular adhesion molecule-1 expression (Aoe et al., 2017). In addition, it has been reported that LA has the ability to reverse endothelial dysfunction by increasing eNOS expression (Hsieh et al., 2018; Shin et al., 2018), NO production (Hsieh et al., 2021), and ACh-induced vasorelaxation (Shin et al., 2018). Therefore, LA may prevent the development of MCI by mitigating MCI-associated factors such as oxidative stress, inflammation, and endothelial dysfunction (Figure 2). Additionally, LA is effective in reducing anxiety and depressive symptoms related to cognitive decline.
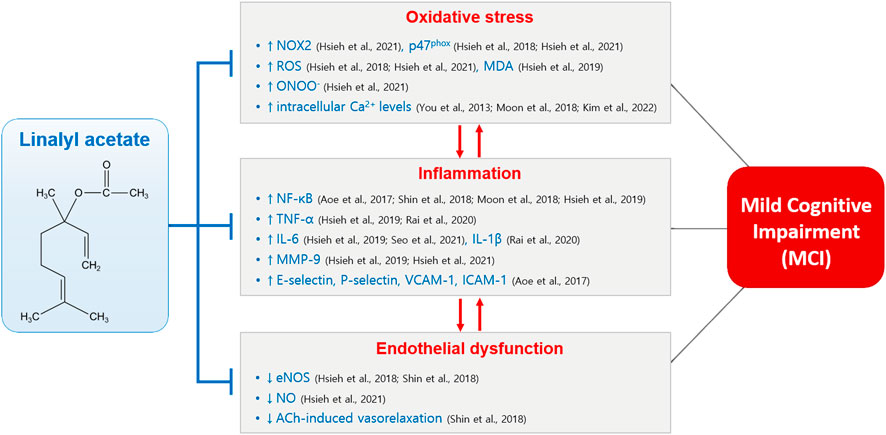
FIGURE 2. Possible methods of action of LA in preventing MCI by reducing oxidative stress, inflammation and endothelial dysfunction. Abbreviations: ACh, acetylcholine; eNOS, endothelial nitric oxide synthase; ICAM, intercellular adhesion molecule; IL, interleukin; MDA, malondialdehyde; MMP, matrix metalloproteinase; NF-κB, nuclear factor-κB; NO, nitric oxide; NOX, NADPH oxidase; ONOO−, peroxynitrite; ROS, reactive oxygen species; TNF, tumor necrosis factor; VCAM, vascular cell adhesion molecule.
8 Conclusion and future perspectives
In conclusion, numerous in vivo and in vitro studies have shown that LA is an effective antioxidant and anti-inflammatory agent, and that it reduces endothelial dysfunction, suggesting that LA can prevent the development of MCI. Importantly, LA does not have genotoxic, phototoxic, photoallergenic, or skin-sensitization properties (Api et al., 2015). However, efforts to develop LA as a drug molecule must overcome some challenges. Main issues are that the pharmacokinetic and pharmacodynamic properties of LA are insufficient for clinical applications. For example, although LA penetrated through the skin of healthy male subjects after massage with LA-containing lavender essential oil, the maximum concentration of LA in blood was only 100 ng/mL, which is insufficient to have a clinical effect (Jäger et al., 1992). In addition, the blood levels of LA in mice that inhaled LA at a concentration of 5 mg/L air were found to be only 1–2 ng/mL (Jirovetz et al., 1991). Moreover, when LA is taken orally, the compound is metabolized to linalool and α-terpineol by carboxylesterase in gastric juice, following which both linalool and α-terpineol are conjugated and oxidized to more polar metabolites and excreted (Bickers et al., 2003). Another problem is the low solubility of LA in water (0.054 mg/mL) (Cal, 2006). Additional studies, therefore, are needed to investigate the pharmacokinetic and pharmacodynamic properties of LA, and to verify the effects of LA in patients at risk of developing MCI. Collectively, the results summarized in the present review provide a basis for the development of new strategies for preventing MCI using LA.
Author contributions
GS: Conceptualization, Methodology, Visualization, Writing—original draft, Funding acquisition, Supervision, Writing—review and editing. YS: Investigation, Visualization, Writing—original draft. All authors contributed to the article and approved the submitted version.
Funding
This work was supported by the National Research Foundation of Korea (NRF) grant funded by the Korea government (MSIT) (No. 2021R1A2C2004118).
Conflict of interest
The authors declare that the research was conducted in the absence of any commercial or financial relationships that could be construed as a potential conflict of interest.
Publisher’s note
All claims expressed in this article are solely those of the authors and do not necessarily represent those of their affiliated organizations, or those of the publisher, the editors and the reviewers. Any product that may be evaluated in this article, or claim that may be made by its manufacturer, is not guaranteed or endorsed by the publisher.
References
Abe, K., Chiba, Y., Hattori, S., Yoshimi, A., Asami, T., Katsuse, O., et al. (2020). Influence of plasma matrix metalloproteinase levels on longitudinal changes in Alzheimer's disease (AD) biomarkers and cognitive function in patients with mild cognitive impairment due to AD registered in the Alzheimer's Disease Neuroimaging Initiative database. J. Neurol. Sci. 416, 116989. doi:10.1016/j.jns.2020.116989
Alavi Naeini, A., Elmadfa, I., Djazayery, A., Barekatain, M., Aghaye Ghazvini, M., Djalali, M., et al. (2014). The effect of antioxidant vitamins E and C on cognitive performance of the elderly with mild cognitive impairment in isfahan, Iran: A double-blind, randomized, placebo-controlled trial. Eur. J. Nutr. 53, 1255–1262. doi:10.1007/s00394-013-0628-1
Alfieri, A., Koudelka, J., Li, M., Scheffer, S., Duncombe, J., Caporali, A., et al. (2022). Nox2 underpins microvascular inflammation and vascular contributions to cognitive decline. J. Cereb. Blood Flow. Metab. 42, 1176–1191. doi:10.1177/0271678X221077766
Alvi, A. M., Siuly, S., Wang, H., Wang, K., and Whittaker, F. (2022). A deep learning based framework for diagnosis of mild cognitive impairment. Knowl. Based Syst. 248, 108815. doi:10.1016/j.knosys.2022.108815
Amir Yusri, M. A., Sekar, M., Wong, L. S., Gan, S. H., Ravi, S., Subramaniyan, V., et al. (2023). Celastrol: A potential natural lead molecule for new drug design, development and therapy for memory impairment. Drug Des. devel. Ther. 17, 1079–1096. doi:10.2147/DDDT.S389977
Anderson, N. D. (2019). State of the science on mild cognitive impairment (MCI). CNS Spectr. 24, 78–87. doi:10.1017/S1092852918001347
Aoe, M., Ueno-Iio, T., Shibakura, M., Shinohata, R., Usui, S., Arao, Y., et al. (2017). Lavender essential oil and its main constituents inhibit the expression of TNF-α-induced cell adhesion molecules in endothelial cells. Acta Med. Okayama 71, 493–503. doi:10.18926/AMO/55586
Api, A., Belsito, D., Bhatia, S., Bruze, M., Calow, P., Dagli, M., et al. (2015). RIFM fragrance ingredient safety assessment, Linalyl acetate, CAS Registry Number 115-95-7. Food Chem. Toxicol. 82, S39–S48. doi:10.1016/j.fct.2015.01.010
Arroba, A. I., Rodríguez-de la Rosa, L., Murillo-Cuesta, S., Vaquero-Villanueva, L., Hurlé, J. M., Varela-Nieto, I., et al. (2016). Autophagy resolves early retinal inflammation in Igf1-deficient mice. Dis. Model. Mech. 9, 965–974. doi:10.1242/dmm.026344
Avram, S., Stan, M. S., Udrea, A. M., Buiu, C., Boboc, A. A., and Mernea, M. (2021). 3D-ALMOND-QSAR models to predict the antidepressant effect of some natural compounds. Pharmaceutics 13, 1449. doi:10.3390/pharmaceutics13091449
Bai, W., Chen, P., Cai, H., Zhang, Q., Su, Z., Cheung, T., et al. (2022). Worldwide prevalence of mild cognitive impairment among community dwellers aged 50 years and older: A meta-analysis and systematic review of epidemiology studies. Age Ageing 51, afac173–14. doi:10.1093/ageing/afac173
Bailey-Downs, L. C., Mitschelen, M., Sosnowska, D., Toth, P., Pinto, J. T., Ballabh, P., et al. (2012). Liver-specific knockdown of IGF-1 decreases vascular oxidative stress resistance by impairing the nrf2-dependent antioxidant response: A novel model of vascular aging. A Biol. Sci. Med. Sci. 67, 313–329. doi:10.1093/gerona/glr164
Barnes, J. N., and Corkery, A. T. (2018). Exercise improves vascular function, but does this translate to the brain? Brain Plast. 4, 65–79. doi:10.3233/BPL-180075
Bian, A., Ma, Y., Zhou, X., Guo, Y., Wang, W., Zhang, Y., et al. (2020). Association between sarcopenia and levels of growth hormone and insulin-like growth factor-1 in the elderly. BMC Musculoskelet. Disord. 21, 214–219. doi:10.1186/s12891-020-03236-y
Bickers, D., Calow, P., Greim, H., Hanifin, J., Rogers, A., Saurat, J., et al. (2003). A toxicologic and dermatologic assessment of linalool and related esters when used as fragrance ingredients. Food Chem. Toxicol. 41, 919–942. doi:10.1016/s0278-6915(03)00016-4
Bonesi, M., Menichini, F., Tundis, R., Loizzo, M. R., Conforti, F., Passalacqua, N. G., et al. (2010). Acetylcholinesterase and butyrylcholinesterase inhibitory activity of Pinus species essential oils and their constituents. J. Enzyme Inhib. Med. Chem. 25, 622–628. doi:10.3109/14756360903389856
Bootman, M. D., and Bultynck, G. (2020). Fundamentals of cellular calcium signaling: A primer. Cold Spring Harb. Perspect. Biol. 12, a038802. doi:10.1101/cshperspect.a038802
Brawek, B., and Garaschuk, O. (2013). Microglial calcium signaling in the adult, aged and diseased brain. Cell Calcium 53, 159–169. doi:10.1016/j.ceca.2012.12.003
Brini, M., Calì, T., Ottolini, D., and Carafoli, E. (2014). Neuronal calcium signaling: Function and dysfunction. Cell. Mol. Life Sci. 71, 2787–2814. doi:10.1007/s00018-013-1550-7
Buchbauer, G., Jirovetz, L., Jäger, W., Dietrich, H., and Plank, C. (1991). Aromatherapy: Evidence for sedative effects of the essential oil of lavender after inhalation. Z. Naturforsch., C. J. Biosci. 46, 1067–1072. doi:10.1515/znc-1991-11-1223
Burgos-Morón, E., Abad-Jiménez, Z., Martínez de Marañón, A., Iannantuoni, F., Escribano-López, I., López-Domènech, S., et al. (2019). Relationship between oxidative stress, ER stress, and inflammation in type 2 diabetes: The battle continues. J. Clin. Med. 8, 1385. doi:10.3390/jcm8091385
Butterfield, D. A. (2023). Oxidative stress in brain in amnestic mild cognitive impairment. Antioxidants 12, 462. doi:10.3390/antiox12020462
Cal, K. (2006). Aqueous solubility of liquid monoterpenes at 293 K and relationship with calculated log P value. Yakugaku Zasshi 126, 307–309. doi:10.1248/yakushi.126.307
Chen, Y. L., Daneva, Z., Kuppusamy, M., Ottolini, M., Baker, T. M., Klimentova, E., et al. (2022a). Novel smooth muscle Ca2+-signaling nanodomains in blood pressure regulation. Circulation 146, 548–564. doi:10.1161/CIRCULATIONAHA.121.058607
Chen, Z. R., Huang, J. B., Yang, S. L., and Hong, F. F. (2022b). Role of cholinergic signaling in Alzheimer’s disease. Molecules 27, 1816. doi:10.3390/molecules27061816
Connors, M. H., Seeher, K., Teixeira-Pinto, A., Woodward, M., Ames, D., and Brodaty, H. (2019). Mild cognitive impairment and caregiver burden: A 3-year-longitudinal study. Am. J. Geriatr. Psychiatry 27, 1206–1215. doi:10.1016/j.jagp.2019.05.012
Cutillas, A. B., Carrasco, A., Martinez-Gutierrez, R., Tomas, V., and Tudela, J. (2018). Thymus mastichina L. essential oils from Murcia (Spain): Composition and antioxidant, antienzymatic and antimicrobial bioactivities. PloS One 13, e0190790. doi:10.1371/journal.pone.0190790
Dong, X., Li, L., Zhang, D., Su, Y., Yang, L., Li, X., et al. (2021). Ginsenoside Rg1 attenuates LPS-induced cognitive impairments and neuroinflammation by inhibiting NOX2 and Ca2+–CN–NFAT1 signaling in mice. J. Funct. Foods 87, 104791. doi:10.1016/j.jff.2021.104791
Drummond, G. R., and Sobey, C. G. (2014). Endothelial NADPH oxidases: Which NOX to target in vascular disease? Trends Endocrinol. Metab. 25, 452–463. doi:10.1016/j.tem.2014.06.012
Dutta, B. J., Singh, S., Seksaria, S., Gupta, G. D., and Singh, A. (2022). Inside the diabetic brain: Insulin resistance and molecular mechanism associated with cognitive impairment and its possible therapeutic strategies. Pharmacol. Res. 182, 106358. doi:10.1016/j.phrs.2022.106358
Freire, A. C. C., Pondé, M. P., Liu, A., and Caron, J. (2017). Anxiety and depression as longitudinal predictors of mild cognitive impairment in older adults. Can. J. Psychiatry 62, 343–350. doi:10.1177/0706743717699175
Fuloria, S., Yusri, M. A. A., Sekar, M., Gan, S. H., Rani, N. N. I. M., Lum, P. T., et al. (2022). Genistein: A potential natural lead molecule for new drug design and development for treating memory impairment. Molecules 27, 265. doi:10.3390/molecules27010265
Glynn, K., O'Callaghan, M., Hannigan, O., Bruce, I., Gibb, M., Coen, R., et al. (2021). Clinical utility of mild cognitive impairment subtypes and number of impaired cognitive domains at predicting progression to dementia: A 20-year retrospective study. Int. J. Geriatr. Psychiatry 36, 31–37. doi:10.1002/gps.5385
Görlach, A., Bertram, K., Hudecova, S., and Krizanova, O. (2015). Calcium and ROS: A mutual interplay. Redox Biol. 6, 260–271. doi:10.1016/j.redox.2015.08.010
Gulpers, B. J., Voshaar, R. C. O., van Boxtel, M. P., Verhey, F. R., and Köhler, S. (2019). Anxiety as a risk factor for cognitive decline: A 12-year follow-up cohort study. Am. J. Geriatr. Psychiatry 27, 42–52. doi:10.1016/j.jagp.2018.09.006
Higashi, Y. (2022). Roles of oxidative stress and inflammation in vascular endothelial dysfunction-related disease. Antioxidants 11, 1958. doi:10.3390/antiox11101958
Hsieh, Y. S., Kwon, S., Lee, H. S., and Seol, G. H. (2018). Linalyl acetate prevents hypertension-related ischemic injury. PLoS One 13, e0198082. doi:10.1371/journal.pone.0198082
Hsieh, Y. S., Shin, Y. K., Han, A. Y., Kwon, S., and Seol, G. H. (2019). Linalyl acetate prevents three related factors of vascular damage in COPD-like and hypertensive rats. Life Sci. 232, 116608. doi:10.1016/j.lfs.2019.116608
Hsieh, Y. S., Shin, Y. K., and Seol, G. H. (2021). Protection of the neurovascular unit from calcium-related ischemic injury by linalyl acetate. Chin. J. Physiol. 64, 88–96. doi:10.4103/cjp.cjp_94_20
Igarashi, T. (2013). Physical and psychologic effects of aromatherapy inhalation on pregnant women: A randomized controlled trial. J. Altern. Complement. Med. 19, 805–810. doi:10.1089/acm.2012.0103
Ighodaro, O. M. (2018). Molecular pathways associated with oxidative stress in diabetes mellitus. Biomed. Pharmacother. 108, 656–662. doi:10.1016/j.biopha.2018.09.058
Jäger, W., Buchbauer, G., Jirovetz, L., and Fritzer, M. (1992). Percutaneous absorption of lavender oil from a massage oil. J. Soc. Cosmet. Chem. 43, 49–54.
Jirovetz, L., Jäger, W., Buchbauer, G., Nikiforov, A., and Raverdinno, V. (1991). Investigations of animal blood samples after fragrance drug inhalation by gas chromatography/mass spectrometry with chemical ionization and selected ion monitoring. Biol. Mass Spectrom. 20, 801–803. doi:10.1002/bms.1200201210
Ju, F., Ran, Y., Zhu, L., Cheng, X., Gao, H., Xi, X., et al. (2018). Increased BBB permeability enhances activation of microglia and exacerbates loss of dendritic spines after transient global cerebral ischemia. Front. Cell. Neurosci. 12, 236. doi:10.3389/fncel.2018.00236
Kim, J. R., Kang, P., Lee, H. S., Kim, K. Y., and Seol, G. H. (2017). Cardiovascular effects of linalyl acetate in acute nicotine exposure. Environ. Health Prev. Med. 22, 42–47. doi:10.1186/s12199-017-0651-6
Kim, M., Shin, Y. K., and Seol, G. H. (2021). Inhalation of clary sage oil before chemotherapy alleviates anxiety and stress without changing blood pressure: A randomized controlled trial. J. Korean Biol. Nurs. Sci. 23, 267–268. doi:10.3393/ac.2021.00864.0123
Kim, Y. J., Shin, Y. K., Seo, E., and Seol, G. H. (2022). Astrocytes reduce store-operated Ca2+ entry in microglia under the conditions of an inflammatory stimulus and muscarinic receptor blockade. Pharmaceuticals 15, 1521. doi:10.3390/ph15121521
Lespinasse, J., Chêne, G., Mangin, J. f., Dubois, B., Blanc, F., Paquet, C., et al. (2022). Associations among hypertension, dementia biomarkers, and cognition: The MEMENTO cohort. Alzheimers Demen 19, 2332–2342. doi:10.1002/alz.12866
Letizia, C., Cocchiara, J., Lalko, J., and Api, A. (2003). Fragrance material review on linalyl acetate. Food Chem. Toxicol. 41, 965–976. doi:10.1016/s0278-6915(03)00014-0
Liao, J. K. (2013). Linking endothelial dysfunction with endothelial cell activation. J. Clin. Invest. 123, 540–541. doi:10.1172/JCI66843
Lobine, D., Pairyanen, B., Zengin, G., Yılmaz, M. A., Ouelbani, R., Bensari, S., et al. (2021). Chemical composition and pharmacological evaluation and of toddalia asiatica (rutaceae) extracts and essential oil by in vitro and in silico approaches. Chem. Biodivers. 18, e2000999. doi:10.1002/cbdv.202000999
Lum, P. T., Sekar, M., Gan, S. H., Pandy, V., and Bonam, S. R. (2021). Protective effect of mangiferin on memory impairment: A systematic review. Saudi J. Biol. Sci. 28, 917–927. doi:10.1016/j.sjbs.2020.11.037
Ma, L. (2020). Depression, anxiety, and apathy in mild cognitive impairment: Current perspectives. Front. Aging Neurosci. 12, 9. doi:10.3389/fnagi.2020.00009
Maral, H., Ulupınar, S., Türk Baydır, A., Özbay, S., Altınkaynak, K., Şebin, E., et al. (2022). Effect of Origanum dubium, Origanum vulgare subsp. hirtum, and Lavandula angustifolia essential oils on lipid profiles and liver biomarkers in athletes. Z. Naturforsch., C. J. Biosci. 77, 177–187. doi:10.1515/znc-2021-0142
Mesulam, M. M., Guillozet, A., Shaw, P., Levey, A., Duysen, E., and Lockridge, O. (2002). Acetylcholinesterase knockouts establish central cholinergic pathways and can use butyrylcholinesterase to hydrolyze acetylcholine. Neuroscience 110, 627–639. doi:10.1016/s0306-4522(01)00613-3
Mirzaei-Najafgholi, H., Tarighi, S., Golmohammadi, M., and Taheri, P. (2017). The effect of citrus essential oils and their constituents on growth of Xanthomonas citri subsp. citri. Molecules 22, 591. doi:10.3390/molecules22040591
Miyazawa, M., Watanabe, H., Umemoto, K., and Kameoka, H. (1998). Inhibition of acetylcholinesterase activity by essential oils of Mentha species. J. Agric. Food Chem. 46, 3431–3434. doi:10.1021/jf9707041
Moon, P. D., Han, N. R., Lee, J. S., Kim, H. M., and Jeong, H. J. (2018). Effects of linalyl acetate on thymic stromal lymphopoietin production in mast cells. Molecules 23, 1711. doi:10.3390/molecules23071711
Nantachai, G., Vasupanrajit, A., Tunvirachaisakul, C., Solmi, M., and Maes, M. (2022). Oxidative stress and antioxidant defenses in mild cognitive impairment: A systematic review and meta-analysis. Ageing Res. Rev. 101639, 101639. doi:10.1016/j.arr.2022.101639
Natarajan, V., Chawla, R., Mah, T., Vivekanandan, R., Tan, S. Y., Sato, P. Y., et al. (2020). Mitochondrial dysfunction in age-related metabolic disorders. Proteomics 20, 1800404. doi:10.1002/pmic.201800404
Nath, N., Prasad, H. K., and Kumar, M. (2019). Cerebroprotective effects of hydrogen sulfide in homocysteine-induced neurovascular permeability: Involvement of oxidative stress, arginase, and matrix metalloproteinase-9. J. Cell. Physiol. 234, 3007–3019. doi:10.1002/jcp.27120
Nguyen, S. A., Oughli, H. A., and Lavretsky, H. (2022). Complementary and integrative medicine for neurocognitive disorders and caregiver health. Curr. Psychiatry Rep. 24, 469–480. doi:10.1007/s11920-022-01355-y
Orth, A. M., Poplacean, I., Fastowski, O., and Engel, K. H. (2014). Assessment of dietary exposure to flavouring substances via consumption of flavoured teas. Part II: Transfer rates of linalool and linalyl esters into Earl Grey tea infusions. Food Addit. Contam. Part A 31, 207–217. doi:10.1080/19440049.2013.866717
Padurariu, M., Ciobica, A., Hritcu, L., Stoica, B., Bild, W., and Stefanescu, C. (2010). Changes of some oxidative stress markers in the serum of patients with mild cognitive impairment and Alzheimer's disease. Neurosci. Lett. 469, 6–10. doi:10.1016/j.neulet.2009.11.033
Pchitskaya, E., Popugaeva, E., and Bezprozvanny, I. (2018). Calcium signaling and molecular mechanisms underlying neurodegenerative diseases. Cell Calcium 70, 87–94. doi:10.1016/j.ceca.2017.06.008
Rai, V. K., Sinha, P., Yadav, K. S., Shukla, A., Saxena, A., Bawankule, D. U., et al. (2020). Anti-psoriatic effect of Lavandula angustifolia essential oil and its major components linalool and linalyl acetate. J. Ethnopharmacol. 261, 113127. doi:10.1016/j.jep.2020.113127
Rajan, K. B., Weuve, J., Barnes, L. L., McAninch, E. A., Wilson, R. S., and Evans, D. A. (2021). Population estimate of people with clinical Alzheimer's disease and mild cognitive impairment in the United States (2020–2060). Alzheimers Dement. 17, 1966–1975. doi:10.1002/alz.12362
Ramić, D., Bucar, F., Kunej, U., Dogša, I., Klančnik, A., and Smole Možina, S. (2021). Antibiofilm potential of Lavandula preparations against Campylobacter jejuni. Appl. Environ. Microbiol. 87, e0109921. doi:10.1128/AEM.01099-21
Rita Cardoso, B., Apolinário, D., da Silva Bandeira, V., Busse, A. L., Magaldi, R. M., Jacob-Filho, W., et al. (2016). Effects of Brazil nut consumption on selenium status and cognitive performance in older adults with mild cognitive impairment: A randomized controlled pilot trial. Eur. J. Nutr. 55, 107–116. doi:10.1007/s00394-014-0829-2
Rombolà, L., Straface, M., Scuteri, D., Sakurada, T., Sakurada, S., Corasaniti, M. T., et al. (2022). Antispasmodic effect of bergamot essential oil on rat isolated gut tissues. Pharmaceutics 14, 775. doi:10.3390/pharmaceutics14040775
Schuwald, A. M., Nöldner, M., Wilmes, T., Klugbauer, N., Leuner, K., and Müller, W. E. (2013). Lavender oil-potent anxiolytic properties via modulating voltage dependent calcium channels. PloS One 8, e59998. doi:10.1371/journal.pone.0059998
Scioli, M. G., Storti, G., D’Amico, F., Rodríguez Guzmán, R., Centofanti, F., Doldo, E., et al. (2020). Oxidative stress and new pathogenetic mechanisms in endothelial dysfunction: Potential diagnostic biomarkers and therapeutic targets. J. Clin. Med. 9, 1995. doi:10.3390/jcm9061995
Scuteri, D., Rombolà, L., Crudo, M., Watanabe, C., Mizoguchi, H., Sakurada, S., et al. (2022). Translational value of the transdermal administration of bergamot essential oil and of its fractions. Pharmaceutics 14, 1006. doi:10.3390/pharmaceutics14051006
Seo, E., Shin, Y. K., Hsieh, Y. S., Lee, J. M., and Seol, G. H. (2021). Linalyl acetate as a potential preventive agent against muscle wasting in rheumatoid arthritis rats chronically exposed to nicotine. J. Pharmacol. Sci. 147, 27–32. doi:10.1016/j.jphs.2021.05.003
Seol, G. H., Lee, Y. H., Kang, P., You, J. H., Park, M., and Min, S. S. (2013). Randomized controlled trial for Salvia sclarea or Lavandula angustifolia: Differential effects on blood pressure in female patients with urinary incontinence undergoing urodynamic examination. J. Altern. Complement. Med. 19, 664–670. doi:10.1089/acm.2012.0148
Seol, G. H., Shim, H. S., Kim, P. J., Moon, H. K., Lee, K. H., Shim, I., et al. (2010). Antidepressant-like effect of Salvia sclarea is explained by modulation of dopamine activities in rats. J. Ethnopharmacol. 130, 187–190. doi:10.1016/j.jep.2010.04.035
Shabab, T., Khanabdali, R., Moghadamtousi, S. Z., Kadir, H. A., and Mohan, G. (2017). Neuroinflammation pathways: A general review. Int. J. Neurosci. 127, 624–633. doi:10.1080/00207454.2016.1212854
Shin, Y. K., Hsieh, Y. S., Han, A. Y., Kwon, S., Kang, P., and Seol, G. H. (2020). Sex-specific susceptibility to type 2 diabetes mellitus and preventive effect of linalyl acetate. Life Sci. 260, 118432. doi:10.1016/j.lfs.2020.118432
Shin, Y. K., Hsieh, Y. S., Kwon, S., Lee, H. S., and Seol, G. H. (2018). Linalyl acetate restores endothelial dysfunction and hemodynamic alterations in diabetic rats exposed to chronic immobilization stress. J. Appl. Physiol. 124, 1274–1283. doi:10.1152/japplphysiol.01018.2017
Shin, Y. K., Kwon, S., Hsieh, Y. S., Han, A. Y., and Seol, G. H. (2022). Linalyl acetate restores colon contractility and blood pressure in repeatedly stressed-ulcerative colitis rats. Environ. Health Prev. Med. 27, 27. doi:10.1265/ehpm.22-00041
Singh, S. P., and Gupta, D. (2017). Discovery of potential inhibitor against human acetylcholinesterase: A molecular docking and molecular dynamics investigation. Comput. Biol. Chem. 68, 224–230. doi:10.1016/j.compbiolchem.2017.04.002
Sukhanov, S., Higashi, Y., Shai, S. Y., Vaughn, C., Mohler, J., Li, Y., et al. (2007). IGF-1 reduces inflammatory responses, suppresses oxidative stress, and decreases atherosclerosis progression in ApoE-deficient mice. Arterioscler. Thromb. Vasc. Biol. 27, 2684–2690. doi:10.1161/ATVBAHA.107.156257
Sun, H. J., Wu, Z. Y., Nie, X. W., and Bian, J. S. (2020). Role of endothelial dysfunction in cardiovascular diseases: The link between inflammation and hydrogen sulfide. Front. Pharmacol. 10, 1568. doi:10.3389/fphar.2019.01568
Tahami Monfared, A. A., Byrnes, M. J., White, L. A., and Zhang, Q. (2022). The humanistic and economic burden of alzheimer's disease. Neurol. Ther. 11, 525–551. doi:10.1007/s40120-022-00335-x
Ungvari, Z., Toth, P., Tarantini, S., Prodan, C. I., Sorond, F., Merkely, B., et al. (2021). Hypertension-induced cognitive impairment: From pathophysiology to public health. Nat. Rev. Nephrol. 17, 639–654. doi:10.1038/s41581-021-00430-6
Van Dyken, P., and Lacoste, B. (2018). Impact of metabolic syndrome on neuroinflammation and the blood–brain barrier. Front. Neurosci. 12, 930. doi:10.3389/fnins.2018.00930
Vendemiale, G., Romano, A. D., Dagostino, M., de Matthaeis, A., and Serviddio, G. (2013). Endothelial dysfunction associated with mild cognitive impairment in elderly population. Aging Clin. Exp. Res. 25, 247–255. doi:10.1007/s40520-013-0043-8
Wan, Y., Gao, W., Zhou, K., Liu, X., Jiang, W., Xue, R., et al. (2022). Role of IGF-1 in neuroinflammation and cognition deficits induced by sleep deprivation. Neurosci. Lett. 776, 136575. doi:10.1016/j.neulet.2022.136575
Wei, R., Lunn, S. E., Gust, S. L., Kerr, P. M., and Plane, F. (2018). “The endothelium: The vascular information exchange,” in Calcium and signal transduction (London: IntechOpen), 43–64. doi:10.5772/intechopen.79897
Yan, X., Meng, T., Liu, H., Liu, J., Du, J., and Chang, C. (2022). The association between the duration, treatment, control of hypertension and lifestyle risk factors in middle-aged and elderly patients with mild cognitive impairment: A case-control study. Neuropsychiatr. Dis. Treat. 18, 585–595. doi:10.2147/NDT.S353164
You, J. H., Kang, P., Min, S. S., and Seol, G. H. (2013). Bergamot essential oil differentially modulates intracellular Ca2+ levels in vascular endothelial and smooth muscle cells: A new finding seen with fura-2. J. Cardiovasc. Pharmacol. 61, 324–328. doi:10.1097/FJC.0b013e3182834681
Yu, S. H., and Seol, G. H. (2017). Lavandula angustifolia mill. Oil and its active constituent linalyl acetate alleviate pain and urinary residual sense after colorectal cancer surgery: A randomised controlled trial. Evid. Based Complement. Altern. Med. 2017, 3954181. doi:10.1155/2017/3954181
Yuan, J., Wang, Y., and Liu, Z. (2023). Temporal relationship between depression and cognitive decline in the elderly: A two-wave cross-lagged study in a Chinese sample. Aging Ment. Health, 1–8. doi:10.1080/13607863.2023.2225432
Zhang, X., Lian, S., Zhang, Y., and Zhao, Q. (2022). Efficacy and safety of donepezil for mild cognitive impairment: A systematic review and meta-analysis. Clin. Neurol. Neurosurg. 213, 107134. doi:10.1016/j.clineuro.2022.107134
Keywords: linalyl acetate, prevention, oxidative stress, inflammation, endothelial dysfunction, mild cognitive impairment
Citation: Shin YK and Seol GH (2023) Effects of linalyl acetate on oxidative stress, inflammation and endothelial dysfunction: can linalyl acetate prevent mild cognitive impairment?. Front. Pharmacol. 14:1233977. doi: 10.3389/fphar.2023.1233977
Received: 03 June 2023; Accepted: 21 July 2023;
Published: 28 July 2023.
Edited by:
Ginpreet Kaur, SVKM’s Narsee Monjee Institute of Management Studies, IndiaReviewed by:
Alina Arulsamy, Monash University, MalaysiaMahendran Sekar, Monash University Malaysia, Malaysia
Copyright © 2023 Shin and Seol. This is an open-access article distributed under the terms of the Creative Commons Attribution License (CC BY). The use, distribution or reproduction in other forums is permitted, provided the original author(s) and the copyright owner(s) are credited and that the original publication in this journal is cited, in accordance with accepted academic practice. No use, distribution or reproduction is permitted which does not comply with these terms.
*Correspondence: Geun Hee Seol, ghseol@korea.ac.kr
†These authors have contributed equally to this work and share first authorship