- 1Department of Pulmonary and Critical Care Medicine, Shengjing Hospital of China Medical University, Shenyang, China
- 2Department of Neurology, Shengjing Hospital of China Medical University, Shenyang, Liaoning, China
Obstructive sleep apnea syndrome (OSAS) refers to the current apnea during sleep caused by upper airway collapse. Meanwhile, epilepsy is a common neurological disorder with a tendency for spontaneous and persistent seizures. Accumulating evidence indicates that OSAS was not independent of epilepsy. Patients with OSAS were observed to be susceptible to epilepsy, while OSAS could decrease the seizure threshold in epilepsy. However, the mechanisms underlying the association of OSAS with epilepsy have not been fully understood. In this study, we propose that intermittent hypoxia, common among OSAS patients due to upper airway collapse, is the linkage between OSAS and epilepsy. Intermittent hypoxia induces elevated levels of oxidative stress and inflammation, potentially causing excessive inflammatory and endoplasmic reticulum stress in brain tissue, which might ultimately lead to the development of epilepsy. Therapeutic approaches targeting inflammation and oxidative stress may provide novel insights into the treatment of OSAS and epilepsy.
1 Introduction
Obstructive sleep apnea syndrome (OSAS) is a common sleep-related breathing disorder which is characterized by recurrent upper airway collapse during sleep causing apnea, followed by arousal that re-establishes the airway (Lv et al., 2023). The severity of OSAS is differentiated clinically by the number of apnea-hypopnea events per hour of sleep and the apnea-hypopnea index (AHI) (Sateia, 2014; Kapur et al., 2017). Apnea is characterized by a 90% reduction in airflow lasting for at least 10 s, whereas Hypopnea is defined as a decrease in airflow of at least 50%, coupled with a 3% drop in blood oxygen saturation, lasting for a minimum of 10 s (Louter et al., 2012; Virk and Kotecha, 2016). OSAS arises from a mix of structural and neuromotor factors causing upper airway narrowing and collapse during sleep, disrupting sleep patterns (Schwab, 2003; Ruttanaumpawan et al., 2009; Marcus et al., 2017). Among OSAS patients, inadequate ventilation caused by apnea leads to insufficient air exchange, resulting in hypercapnia (PaCO2 > 45 mmHg), which prompt the patients to awaken, and facilitate the restoration of airway patency (Kaur et al., 2020). However, the resulting sleep fragmentation could contribute to various cognitive disorders such as concentration issues, excessive daytime sleepiness (EDS), and fatigue (Ferguson et al., 2017). In addition, it is common for OSAS patients to experience symptoms such as morning bloating, headaches, emotional numbness, depression, irritability, mood fluctuations, memory problems, and erectile dysfunction during the daytime (Azagra-Calero et al., 2012; Lévy et al., 2015). OSAS is a highly prevalent disorder around the world with considerable morbidity and mortality (Batool-Anwar et al., 2014). In the United States, about 12% of men and 7% of women aged 30–70 suffer from moderate to severe OSAS (Peppard et al., 2013). It was also observed in Brazil that 24.8% of men had OSAS (Tufik et al., 2010). In addition, OSAS is also common in China and Spanish, with prevalence of 9.5% and 14.2% correspondingly (Durán et al., 2001; Li et al., 2005). Recent studies also estimated that about 936 million people between 30 and 60 years old are affected by OSAS (Benjafield et al., 2019). Obstructive events predominantly occur during the rapid eye movement (REM) sleep stage (Haba-Rubio et al., 2005). During REM sleep, the hypoglossal nerve is inhibited through cholinergic neurons, resulting in the suppression of genioglossus muscle tone and an elevated risk of upper airway collapse (Mokhlesi et al., 2014). Collapse of upper airway further exacerbates the severity of OSAS and lead to blood hypoxemia, hypercapnia, sleep fragmentation, enhanced respiratory effort, which subsequently result in increased risks of intermittent hypoxia during sleep (Rosenzweig et al., 2014; Xing et al., 2014; Xanthopoulos et al., 2015). Long-term intermittent hypoxia could further result in a series of changes in the body, including oxidative stress, obvious inflammation, apoptosis, and neural activation (Huang et al., 2018; Patke et al., 2020; Mishra et al., 2021). Meanwhile, increased sympathetic activity during REM sleep also results in multiple systems such as cardiovascular system and nervous systems (Mokhlesi et al., 2014; Grigg-Damberger and Foldvary-Schaefer, 2021; Lehner et al., 2022). Therefore, OSAS patients might also have vario`us other medical conditions concomitantly.
Epilepsy is a common neurological disorder with a tendency of spontaneous and persistent seizures characterized by recurrent epilepsy, which will bring adverse effects on patients’ cognitive, psychological, and social life (Engel, 1995; Fisher et al., 2014). The defining feature of epilepsy is the presence of spontaneous recurrent seizures, which at a cellular level manifest as aberrant discharges of neuronal clusters leading to hyperexcitability and hypersynchrony (Ibhazehiebo et al., 2018). More than 70 million people around the world suffer from epilepsy (Thijs et al., 2019). The prevalence of epilepsy varies globally, with rates of approximately 0.4% in China, 0.39% in India, 0.3% in Europe, and 0.93% in Africa (Forsgren et al., 2005; Mac et al., 2007; Ba-Diop et al., 2014). In addition, it was also observed in France in 2020 that the incidence of epilepsy in France is about 1.02% (Coste et al., 2023). A seizure is a temporary sign or symptom triggered by abnormal, excessive, or synchronized neuronal activity in the brain. It can potentially cause permanent neuronal damage, leading to lifelong neurological problems. (Fisher et al., 2005; Parfenova et al., 2012). According to the latest classification methods of epilepsy, epilepsy is mainly divided into the following types: generalized epilepsy syndromes, with polygenic etiologies; self-limited focal epilepsy syndromes with presumed complex inheritance; self-limited focal epilepsy syndromes with presumed complex inheritance; a combined generalized and focal epilepsy syndrome with polygenic etiology, epilepsy syndromes with developmental encephalopathy (Riney et al., 2022).
More and more studies have shown that OSAS and epilepsy often coexist (Ebben et al., 2008; Parhizgar et al., 2011; Shaki et al., 2011). As is mentioned above, recurrent apnea during sleep and sleep fragmentation in OSAS patients could result in intermittent hypoxia in various tissues especially the brain. Indeed, OSAS patients frequently undergo abnormal physiological consequences in brain tissues due to prolonged oxygen deficiency (Burnsed et al., 2019; Li et al., 2021). As the brain is more sensitive to hypoxia injuries, intermittent hypoxia can cause more serious brain damage compared with other pathological changes (Rodgers et al., 2016). It was further demonstrated by Wang et al. that activities of GABAA receptor is modified by hypoxia, which further exacerbates brain injuries and increased the risk of epilepsy (Wang and Greenfield, 2009). When hypoxia is corrected through continuous positive airway pressure ventilation, epileptic seizures are controlled in patients with OSAS, further confirming the connection between intermittent hypoxia and epilepsy (van Golde et al., 2011). In addition, intermittent hypoxia, especially during REM stage, could also lead to high levels of inflammation and oxidative stress (Drum et al., 2016; Cronstein and Sitkovsky, 2017). Inflammation and oxidative stress in brain tissues could contribute to neuronal cell damage, which subsequently increased the risks of epileptic seizures. Moreover, it was also demonstrated by Lehner J et al. that sleep deprivation, common among OSAS patients, exhibited as a strong trigger for seizures (Lehner et al., 2022). Thus, it is possible that intermittent hypoxia caused by OSAS may play a significant role during the pathogenesis of epilepsy. In this study, we illustrate the mechanisms of intermittent hypoxia caused by OSAS, and further elucidate that inflammation and oxidative stress induced by intermittent hypoxia act as a bridge linking OSAS and epilepsy (Kong et al., 2012; Na et al., 2020).
2 Methods and materials
2.1 Objectives
The aim of the present study was to investigate the relationship between epilepsy and further identify underlying mechanisms.
2.2 Hypotheses
Intermittent hypoxia caused by OSAS, especially during REM sleep, might play crucial role in the pathogenesis of epilepsy.
2.3 Methods, key words, and search strategy
PubMed, PubMed Central (PMC), Embase, and Web of Science databases were searched for this study, using the following keyword: “obstructive sleep apnea syndrome or OSAS,” “REM sleep,” “epilepsy,” “intermittent hypoxia,” “oxidative stress or ROS,” “inflammation,” “TNF-α or IL-1β or IL-6,” “endoplasmic reticulum stress or ER stress” and “treatment.” The search was limited to English-language publications. Searches of published papers were conducted up to 18 October 2023. In addition, references from relevant articles were also examined to identify additional eligible studies.
3 OSAS and epilepsy
Common clinical manifestations of OSAS include symptoms of upper airway obstruction, insomnia, and sleep-related apnea. The occurrence of sleep apnea in patients with OSAS is due to the collapse of the upper airway during sleep, and airway stenosis is an anatomical factor for sleep apnea (Kim et al., 2012; Schloss et al., 2020). However, OSAS is not only a respiratory disease, but also a long-term disease process that can affect the circulatory system, nervous system and even psychology of patients (Milagro et al., 2019). It was noted that OSAS and epilepsy often coexist and influence each other. Many studies have shown that the incidence of OSAS is higher in patients with epilepsy (Maurousset et al., 2017). The prevalence of OSAS in patients with epilepsy is higher than that in the general population (van Golde et al., 2011; Shaheen et al., 2012). A cohort study published in 2018 revealed that patients with refractory epilepsy and OSAS experienced more frequent focal seizures and a longer duration of epilepsy, indicating a stronger association between OSAS and focal seizures (McCarter et al., 2018). Furthermore, it was also noted that the treatment for OSAS can alleviate symptoms of epilepsy. In a case report in 1994, continuous positive nasal pressure (CPAP) was given to 7 patients with both refractory epilepsy and sleep apnea syndrome, showing that 4/5 of the patients significantly reduced the frequency of seizures after positive pressure ventilation (Devinsky et al., 1994). It was further confirmed in a recent studies including 503 participants that OSAS frequently coexisted with epilepsy, and CPAP could reduce the frequency of seizures (Jo et al., 2022). It was also observed in 2008 that after left frontal lobectomy was performed in patients with epilepsy complicated with OSAS, the seizure almost disappeared due to resolution of obstructive sleep apnea (Foldvary-Schaefer et al., 2008). Meanwhile, epilepsy patients often suffer from various sleep disorders, and treatment for OSAS can significantly improve epilepsy seizures in patients (van Golde et al., 2011). However, there is insufficient evidence regarding the mechanism of epilepsy’s impact on OSAS at present, which needs to be further discovered.
4 Intermittent hypoxia: linkage between OSAS and epilepsy
Recurrent upper respiratory tract obstruction in sleep apnea syndrome can lead to hypoxia of arterial microcirculation and proliferation of capillary endothelial cells, resulting in autonomic nervous dysfunction and increased the risk of epilepsy (Valli et al., 1984). It was noted that patients with epilepsy with or without OSAS will have a state of hypoxia. Whether epileptic patients are complicated with OSAS or not, the causes of hypoxia will be different. Patients with simple epilepsy will have a state of hypoxia during seizures, but patients with both epilepsy and OSAS will not only have hypoxia during seizures, but also have intermittent repeated hypoxia due to sleep apnea (Xanthopoulos et al., 2015; Tereshchenko et al., 2019; Han et al., 2020). It was observed that mice developed shortness of breath during seizures, followed by shallow breathing and hyperventilation (Trosclair et al., 2020). Central and obstructive apnea can also occur during seizures (Nakase et al., 2016). These symptoms may lead to epilepsy-related hypoxemia asphyxia and even sudden death in severe cases, which suggests a potential connection between epilepsy and OSAS (Bateman et al., 2008). It was also demonstrated in a meta-analysis that 33.4% of patients with moderate to severe epilepsy had co-existing OSAS, and patients with epilepsy were more likely to develop OSAS than healthy controls (Lin et al., 2017). At the same time, there is also evidence indicating that OSAS can trigger epilepsy. In a clinical study involving 25 pediatric patients with OSAS, overnight EEG monitoring revealed epileptiform brain discharges coinciding with OSAS onset in 2 children, and seizures in 2 other children, indicating that there may be a two-way relationship between OSAS and epilepsy (Miano et al., 2010; Brunetti et al., 2017).
Hypoxia may also lead to seizures. Current international academic research on hypoxia and epilepsy susceptibility is insufficient. However, a study indicates that closing a patent foramen ovale improves migraine and epilepsy by reducing hypoxia induced by right-to-left shunts (Dong et al., 2023). Another epidemiological study revealed that hypoxia in middle-aged adults with sleep apnea significantly increased the risk of late-onset epilepsy (Grigg-Damberger and Foldvary-Schaefer, 2023). Currently, the primary research on hypoxia-induced epilepsy is focused in the field of children. Neonatal rat models of mild hypoxia causing epilepsy without significant brain damage have been established (Justice and Sanchez, 2018). Researchers have attempted to control hypoxic-induced neonatal epilepsy in rats through a variety of approaches, and it was demonstrated that seizure susceptibility is associated with brain damages in male mice treated with hypothermia after neonatal hypoxia ischemia (McNally et al., 2019). Another study demonstrated that administering fingolimod, a sphingosine 1-phosphate receptor (S1PR) modulator known for its anti-inflammatory effects in the central nervous system (CNS), after hypoxia-induced neonatal seizures, successfully reversed cognitive impairment and reduced seizure severity in both male and female adult rats (Mehling et al., 2011; Najafian et al., 2021). Meanwhile, some medications can reduce the susceptibility to epilepsy by inhibiting hypoxia. It was demonstrated that bumetanide can reduce the susceptibility to epilepsy by inhibiting abnormal hippocampal neurogenesis in newborn rats after hypoxia ischemia (Hu et al., 2017). Taurine deoxycholic acid (TUDCA) improves the pathological changes of epileptic seizures in rats by reducing inflammation and hypoxia in autonomic nervous tissue (Üner et al., 2023). Minocycline inhibits the activation of microglia induced by hypoxia and reduces the onset of epilepsy (Fukushi et al., 2023). Therefore, there might be a causal relationship between OSAS and epilepsy.
4.1 Different types of hypoxia and brain excitability
Different types of hypoxia have different effects on brain excitability. Studies have shown that chronic and persistent hypoxia triggers the activation of microglia, and enhances glutamatergic neurotransmission in the NTS at the same time (Lima-Silveira et al., 2019). It was demonstrated by Wais M et al. that repeated hypoxic episode could induce seizures with alterations in hippocampal network activities (Wais et al., 2009). Another study showed that chronic, persistent hypoxia enhances neuroplasticity (Kuloglu et al., 2021). However, chronic intermittent hypoxia in the brain led to low-grade neuroinflammation in the hippocampus of mice, involving early but temporary cytokine elevation and delayed but long-term microglial changes. These changes may lead to intermittent hypoxia-induced cognitive impairment and pathological brain aging (Sapin et al., 2015). Compared with chronic hypoxia, acute hypoxia can cause severe and irreversible damage to brain function and eventually lead to cortical neuron death (Zhang et al., 2023).
Hypoxia has different effects on brain excitability at different ages. It has been demonstrated that specific groups of neurons and regions in the developing brain (cortex, thalamus, and putamen) are particularly sensitive to hypoxia during the neonatal period, a phenomenon known as selective vulnerability (Johnston, 1998; Johnston and Hoon, 2000). Damages caused by hypoxia can last for months or even years, causing mitochondrial failure, acute inflammation, oxidative stress and increased seizure activity (Fleiss and Gressens, 2012). It was noted by Rakhade SN et al. that hypoxia-induced neonatal seizures could result in the development of epilepsy in later life (Rakhade et al., 2011). In adults, hypoxia is a cause of neurological damage, including triggers for Alzheimer’s, Parkinson’s, and other age-related neurodegenerative diseases, and can also be a neuroprotector, a potential therapeutic application for neurodegenerative diseases (Burtscher et al., 2021).
4.2 Intermittent hypoxia: primary outcomes of OSAS
As is mentioned above, OSAS is a severe sleep-disordered breathing disorder in which patients are intermittently hypoxic due to repeated apneas and hypoventilations during sleep (Benjafield et al., 2019). More and more studies have shown that OSAS patients often experience long-term intermittent hypoxia due to changes in blood gas balance caused by upper airway collapse and apnea/hypopnea events (Andaku et al., 2015). The pathogenesis of OSAS is multifactorial with multiple mechanisms, including oxidative stress, inflammation, endothelial dysfunction, and altered metabolism (Aherne and Hull, 1966; Salman et al., 2020). Intermittent hypoxia is a major pathophysiological feature of OSAS, which could trigger a variety of pathological states including oxidative stress, over inflammation, apoptosis, and increased neural activation (Patke et al., 2020) (Figure 1).
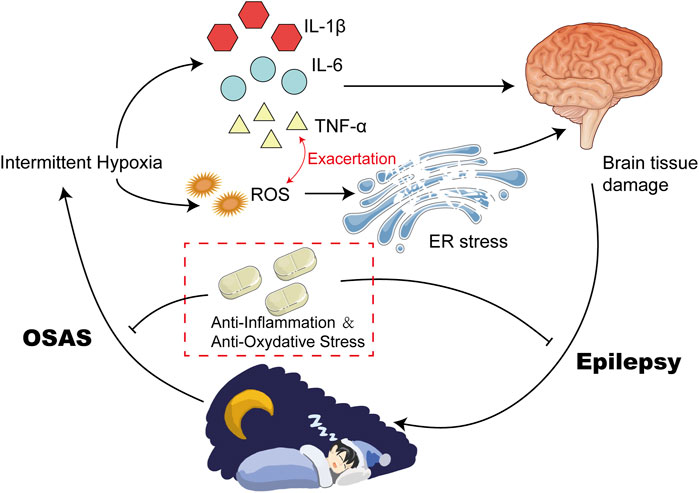
FIGURE 1. Intermittent Hypoxia Could Result in High Levels of Inflammatory and ROS. Intermittent Hypoxia could upregulate the expression of HIF-α via increasing its posttranslational stabilization. Elevated HIF-α induced the transcription of various inflammatory cytokines, such as IL-6 and TNF-α. In addition, hypoxia can also disrupt the balance of antioxidants between the defense system and the oxidative system, leading to upregulation of ROS. Elevated IL-6 and TNF-α exacerbate each other, and finally contribute to high levels of inflammation and oxidative stress.
4.3 Intermittent hypoxia results in oxidative stress
Studies have shown that oxidative stress plays an important role in neurological injury caused by intermittent hypoxia (Zhang and Veasey, 2012; Lavie, 2015). Oxidative stress is a state of imbalance between pro-oxidant and anti-aging agents caused by various endogenous or exogenous stimuli or stressors, which is induced by overproduction of reactive oxygen species (ROS) and reactive nitrogen or a reduction of antioxidants (van der Pol et al., 2019). ROS is a double-edged sword. Mild levels of ROS can facilitate the activation of signaling pathways associated with repair and survival, while excessive levels can lead to adverse effects and inflammation (Tang et al., 2019). Hypoxia-induced elevated production of ROS can be attributed to several factors, including mitochondrial dysfunction, activation of NADPH oxidase (NOX) and xanthine oxidase, and uncoupling of nitric oxide synthase (NOS) (Lavie, 2003; Lavie, 2015). A large number of signaling pathways and transcription factors are regulated by ROS, the most notable of which are the Hypoxia-Inducible Factor 1α (HIF-1α) and nuclear factor-κB (NF-κB) pathways in OSAS (Reuter et al., 2010). NF-κB is a typical pro-inflammatory signaling molecule (Lawrence, 2009). NF-κB serves as an important regulator of various inflammatory processes by activating molecules that induce the production of pro-inflammatory cytokines such as tumor necrosis factor-α (TNF-α), IL-1β, and IL-6, along with chemokines and adhesion molecules (Shih et al., 2015).
Hypoxia in patients with OSAS can cause an imbalance of antioxidants between the defense system and the oxidative system, leading to oxidative stress, which in turn activates and accelerates peroxidative damage (Lavie, 2015). It was observed that circulating markers of protein nutrition and lipid peroxidation are elevated in OSAS patients, and the severity is related to the apnea-hypopnea index (Klein et al., 2010; Vatansever et al., 2011). Biomarker studies in OSAS patients have shown that ROS could reduce plasma nitrite and nitrate levels, increase lipid peroxidation, and reduce antioxidant capacity (Kanbayashi et al., 2009). Furthermore, oxidative stress in OSAS is associated with surrogate markers of cardiovascular disease, such as endothelial function, intima-media thickness, and hypertension (Eisele et al., 2015). In addition, OSAS patients exhibited alterations in metabolomic results which appear to be attributable to oxidative stress induced by OSAS (Xu et al., 2016) (Figure 1).
4.4 Intermittent hypoxia results in inflammation
Inflammation is the body biological response to injury, infection, or other stimuli, which is controlled by the body immune system and is designed to protect the body from harm (Gopal, 2020; Shen et al., 2020). The brain consumes a greater amount of oxygen and energy compared to the rest of the body, and is highly susceptible to hypoxia (Wang et al., 2010). Inflammation triggered by hypoxia can result in endothelial dysfunction and atherosclerosis in the brain, leading to a drop in cerebral blood flow and decreased neuronal metabolic function and oxygen consumption, which subsequently result in nerve cell apoptosis and necrosis (Zhang et al., 2016). Inflammation occurred in the peripheral regions of the body could reach the central nervous system through either blood-brain barrier penetration or vagal afferent stimulation (Dilger and Johnson, 2008). High levels of inflammation in the central nervous system further upregulate the activity of glial cells (microglia and astrocytes), inducing and exacerbating neuroinflammatory responses. At the same time, intermittent hypoxia can directly activate microglia and astrocytes, and promote the release of inflammatory cytokines in the central nervous system (Kiernan et al., 2016). Elevated levels of inflammation in the central nervous system can cause an increase in the activity of glial cells such as microglia and astrocytes, leading to the aggravation of neuroinflammatory responses (Neher et al., 2012; Dang et al., 2021).
Additionally, intermittent hypoxia can directly stimulate microglia and astrocytes, resulting in the release of inflammatory factors within the central nervous system (Hong et al., 2016). Inflammatory factors are molecules that mediate and regulate inflammatory responses during inflammation, including cytokines, chemokines, inflammatory mediators, et al. Inflammatory factors can promote or inhibit inflammatory responses by regulating the activity and proliferation of inflammatory cells (Shen et al., 2020). Excessive production of inflammatory factors under inflammatory conditions can lead to adverse effects, such as tissue damage and disease development (Jia et al., 2016). Intermittent hypoxia affects the occurrence and development of inflammation by regulating the production and differentiation of neutrophils, macrophages, and T cells (Hafner et al., 2017). Hypoxia could upregulate HIF1-α via increasing posttranslational stabilization (Meretoja et al., 2013). HIF1-α is a transcription factor which induces the transcription of multiple inflammatory factors via binding to hypoxia-response element (HRE) of target region, such as TNF-α and IL-6 (Wang et al., 2012; Supriya et al., 2016; Peek et al., 2017; Li et al., 2020). In addition, HIF1-α could also promote the expression of TNF-α and IL-6 via upregulation of NF-κB pathway (Bradshaw et al., 2020).
Increasing evidence supports OSAS as a low-grade chronic inflammatory disease. Many studies have confirmed that intermittent hypoxia and sleep deprivation are associated with inflammatory activation and progression in patients with OSAS (Biddlestone et al., 2015; Colgan et al., 2016; Nunes et al., 2018). There is a strong link between hypoxia and inflammation. It was observed that the levels of inflammatory factors in patients with OSAS were significantly higher than those in the control group (Kohler et al., 2010; Imani et al., 2020). Among these inflammatory mediators and pro-inflammatory cytokines, the most documented are IL-6 and TNF-α (Imani et al., 2020) (Figure 1).
4.4.1 TNF-α
Tumor necrosis factor-α (TNF-α) is a widely studied inflammatory factor. TNF-α leads to activation of the NF-κB pathway and further activates nitric oxide synthase, cyclooxygenase and other receptors, participating in sleep regulation (Ramesh et al., 2012). Elevated levels of TNF- α were observed in OSAS patients, which were demonstrated to be associated with poor outcomes (Xin et al., 2022). In addition, it was noted by Olszewska E et al. that high levels of TNF- α were declined in OSAS patients after surgery (Olszewska et al., 2022). It was further reinforced in an animal experiment that chronic intermittent hypoxia induced by OSAS on mice result in high expression of TLR4-NFκB pathway and the increase of TNF- α level under hypoxia (Ramesh et al., 2012).
4.4.2 IL-6
IL-6 is part of a group of cytokines known as the IL-6 family, which includes other members such as cardiotrophin-1, inhibin M, and leukemia inhibitory factor (Morris et al., 2018). Studies have shown that intermittent hypoxia induces macrophage polarization and increased IL-6 production (Li et al., 2017). Biopsies of adipose tissue and blood samples from obese patients with and without OSAS found that significantly increased tissue expression and circulating levels of IL-6, which was significantly attenuated after 6 months of continuous positive airway pressure treatment (Baessler et al., 2013). A systematic review examining inflammation and sleep disorders noted that two inflammatory factors, C-reactive protein (CRP), and IL-6, are strongly associated with sleep disorders, which upregulate the expression of NF-κB and increase the levels of intercellular adhesion molecules (Devaraj et al., 2006). At the same time, the combination of TNF-α and tumor necrosis factor receptor protein 1 (TNF-r1) can also increase the activity of NF-κB, resulting in increased expression of vascular cell adhesion molecule-1 and monocyte chemoattractant protein-1 in endothelial cells (Feng et al., 2018).
4.4.3 IL-1β
IL-1β, one of the first interleukins discovered, produces a similar pro-inflammatory response through signaling of the interleukin-1 receptor type 1 (IL-1R1), which is involved in regulating the innate immune response of the host (Dinarello, 2011). The central role of IL-1β in mediating neuroinflammation has been recognized in most central nervous system-related diseases, activating microglia and astrocytes, and leading to downstream synthesis of other pro-inflammatory and chemoattractants within the CNS (Shaftel et al., 2008). IL-1β activates the IL-1R1-TLR4 signaling pathway by binding to IL-1R1, which can decrease the expression of GABA receptor and increase the expression of N-methyl-D-aspartate (NMDA) receptor in neurons and glial cells (Dheer et al., 2018; Cramer et al., 2019; Sanz and Garcia-Gimeno, 2020; Semple et al., 2020). One study shows that activation of IL-1R1-TLR4 signaling enhances the function of NMDA receptors in cultured hippocampal neurons by non-transcriptional mechanisms, thereby promoting NMDA-induced calcium influx and the release of excitatory neurotransmitters such as glutamate (Vezzani et al., 2011a). This suggests that the activation of this signaling pathway disrupts the homeostasis of various neurotransmitters and the excitability of neurons (Lai et al., 2006). Interestingly, IL-1β was found to enhance the releases of both glutamate and γ-aminobutyric acid (GABA) in the hippocampus through a mechanism engaging the toxic overload response of Ca2+ influx and Ca2+-induced Ca2+ release revealing that the IL-1β hyperactivation might cause an imbalance between excitatory and inhibitory neurotransmission (Zhu et al., 2006). Indeed, IL-1β was able to enhance the glutamatergic NMDA receptor through facilitating the Ca2+ influx, which was followed by increased neuronal cell death as a consequence of excitotoxicity (Viviani et al., 2003). These findings together suggest that IL-1β signaling via IL-1R contributes to the CNS hyperexcitability and excitotoxicity under neuropathophysiological conditions such as seizures likely through disrupting the balance between glutamatergic and GABAergic neurotransmission.
4.5 Oxidative stress and inflammation are mutually reinforcing
Oxidative stress and inflammation are two prominent potential mechanisms explaining various complications of OSAS. The former refers to a state where there is an unequal balance between pro-oxidative and anti-oxidative systems, resulting in the excessive production of ROS. The latter is response to various external and internal damages including oxidative stress. Inflammation is a response to oxidative stress, while oxidative stress and inflammation can mutually amplify each other (Wang et al., 2019). Oxidative stress sets off a harmful cycle, which triggers sympathetic activation and inflammation, thereby augmenting oxidative stress. ROS-activated PI3K/PKB signaling pathway mediates NF-kB activation, which is responsible for the release of inflammatory factors (Xu et al., 2004; Ravenna et al., 2014). In addition, NF-κB can also activate the transcriptional reaction of HIF-1α mRNA and serves as a prominent transcriptional activator of HIF-1α (Chou et al., 2016). Activation of HIF1-α could also stimulate expression of many proinflammatory molecules and exacerbate inflammation (Preston et al., 2019) (Figure 1).
5 Oxidative stress and inflammation could induce seizures
Epilepsy is a severe and persistent neurological disorder that is distinguished by the recurring, spontaneous onset of epileptic seizures. The primary cause of seizures is an imbalance between excitation and inhibition in the brain (Noam et al., 2013). This imbalance can result in the transition from normal brain function to an epileptic state. Epileptic activity can be promptly induced by the inhibition of synaptic and voltage-gated inhibitory conductances. Alternatively, it can be stimulated by the activation of synaptic and voltage-gated excitatory conductance (Matsumoto and Ajmonemarsan, 1964). A broad spectrum of theories has emerged from existing evidence to elucidate the pathogenic mechanisms of epilepsy. These theories encompass a range of factors such as modifications to ion channels, aberrant neurotransmitter release or uptake, metabolic dysfunction, inflammatory responses, neuronal loss, structural abnormalities, genetic factors, and more (Treiman, 2001; Kovac et al., 2017; Schmeiser et al., 2017; Terrone et al., 2017; Wei et al., 2017). Despite the numerous theories proposed, our understanding of the fundamental pathogenesis of epilepsy remains incomplete.
5.1 Inflammation induced by OSAS: a potent mechanism for epilepsy development
As is mentioned above, chronic inflammatory conditions caused by chronic intermittent hypoxia is common in brain tissues among patients with OSAS. In the last decade, a growing amount of both clinical and experimental data has bolstered the idea that inflammatory mechanisms occurring in the brain may play a crucial and ubiquitous role in the development and progression of seizures and epilepsy (Vezzani and Granata, 2005; Vezzani and Baram, 2007; Riazi et al., 2010). In the case of febrile seizures, it has been noted that an increase in the concentrations of pro-inflammatory agents is frequently observed during epileptic events (Dinarello, 2004). Moreover, research has shown that certain drug-resistant forms of epilepsy may respond positively to treatment with anti-inflammatory agents like steroids, suggesting that they possess anticonvulsant properties (Wirrell et al., 2005; Wheless et al., 2007). Therefore, inflammatory processes within the brain, which was induced by intermittent hypoxia among patients with, maybe a fundamental and critical mechanism involved in the pathophysiology of seizures and epilepsy (Figure 2).
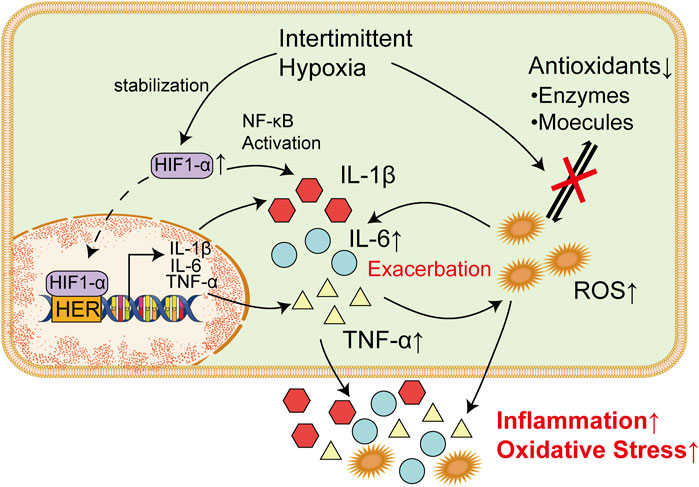
FIGURE 2. Inflammatory factors and ROS Are Responsible for Damages in Nervous Systems. Increased TNF-α could bind to corresponding receptor in microglia cells and trigger the release of glutamate via upregulating intracellular ATP/ADP and Ca2+. Elevated glutamate could thereby promote synaptic activity and lead to epilepsy. Meanwhile, IL-6 could increase the excitation of synapses when it binds to IL-6R through the JAK/STAT pathway. In endoplasmic reticulum, excessive ROS could lead to the production of a massive amount of unfolded or misfolded proteins, which result in the dissociation of GRP78 from ER transmembrane proteins (PERK, IRE1, and ATF6). Overactivation of these proteins induce unfolded protein response and subsequently lead to ER stress. ROS-mediated UPR could finally induce the apoptosis of neurons and thereby increase incidence of epilepsy.
5.1.1 Influence of TNF-α on epilepsy
Previous studies have shown that inflammatory factors represented by IL-6 and TNF-α play an important role in the mechanism of epileptic seizure (Dey et al., 2016). Animal evidence suggests that there may be an interaction between increased levels of TNF-α during seizures and, in turn, increased levels of TNF-α that lead to seizures, and this interaction seems to be a vicious cycle (Shandra et al., 2002). Transgenic mice that constitutively overexpress TNF-α showed multiple symptoms including seizures, ataxia, and paresis, along with inflammation-associated pathological alterations within the brain (Probert et al., 1995). Intriguingly, computational modeling of neuron-glia interactions also showed that TNF-α overexpression led to seizure-like activity patterns (Savin et al., 2009). Similarly, rats that received TNF-α prior to amygdala kindling showed prolonged seizure-like discharges and increased power of β and γ bands when compared to control animals (Shandra et al., 2002). TNF-α plays its role in vivo by interacting with TNF receptor type 1 (TNFR1) and TNF receptor type 2 (TNFR2) (Salazar-Montes et al., 2006; Hop et al., 2017; Sun, 2017). Interestingly, seizures lead to expression of TNF-α in the hippocampus and increase seizure sensitivity through activation of TNFR1, while activation of TNFR2 plays an antiepileptic role in vivo (Weinberg et al., 2013), Lack of TNFR2 or both led to increased epilepsy sensitivity in mice (Balosso et al., 2005). This suggests that the role of TNF-α in the course of seizures may be bidirectional.
Studies have shown that TNF-α can cause the activation of microglia cells and accelerate the death of peripheral neurons, which plays an important role in the occurrence and progression of epilepsy (Zhao et al., 2018; Henning et al., 2023). TNFR1 in microglia cells is activated after binding with TNF-α, which leads to increases in intracellular ATP/ADP and Ca2+ and subsequently trigger the release of glutamate. Increased levels of glutamate could promote synaptic activity and leads to epilepsy (Bedner and Steinhäuser, 2019). Another study also supports this claim. To prevent the harmful effects of TNF-a, some authors have used anti-TNF-α monoclonal antibodies (Etanercepp) and non-specific TNF-α inhibitors such as dihydrothalidomide, nilotinib, and cannabinoids. All of these treatments have been shown to be successful in improving seizures in animal models of epilepsy (Ravizza and Vezzani, 2018). Another study showed that TNF-α converting enzyme can reduce the incidence of seizures and play a neuroprotective role (Meli et al., 2004). It was also noted by Ljiljana Nikolic et al. that P2Y1 receptor inhibitors, which is known to block the activities of TNF-α, could restore abnormal neural activity in the hippocampus and thereby reduce epilepsy seizures (Nikolic et al., 2018). Targeting to cut off the purine metabolism of TNF-α and microglia may be a new strategy for the treatment of epilepsy.
5.1.2 Influence of IL-6 on epilepsy
IL-6 is a convulsion-promoting and neurotoxic cytokine that regulates neuronal and synaptic function in the central nervous system (Gruol, 2015). High levels of IL-6 was observed in patients with epilepsy, suggesting that IL-6 is important for the development of epilepsy (Sallmann et al., 2000; Liimatainen et al., 2009). When it binds to IL-6R and gp130, it acts either through the JAK/STAT trans-signaling pathway (Leo et al., 2020). Meanwhile, IL-6 could disrupt the balance between the inhibition and excitation of synapses via excessive activation of synapses (Garcia-Oscos et al., 2012). Intranasal administration of IL-6 could increase the severity of epilepsy induced by pentylenenetrazole (Kalueff et al., 2004). It was also observed in IL-6-deficient mice that significantly fewer behavioral seizures was developed compared with wild mice when they are infected with neurotropic virus (Libbey et al., 2011). Therefore, IL-6 system may also act as a novel target for anticonvulsant drugs. Some researchers have suggested that the use of anti-IL-6 monoclonal antibodies or the use of specific STAT3 inhibitors such as WP1066 may help reduce seizures (Cerri et al., 2017). These results suggest that a certain baseline level of IL-6 appears to be necessary to maintain the seizure threshold, and that higher expression of IL-6 may exacerbate seizures and associated neuropathological changes.
5.1.3 Influence of IL-1β on epilepsy
IL-1β serves as the archetypal inflammatory cytokine. Its binding to the IL-1β receptor (IL-1R) activates NF-κB and three mitogen-activated protein kinase (MAPK) signaling pathways, which play a crucial role in cytokine production, upregulation of inflammatory genes, and the generation of reactive oxygen species (Dey et al., 2016; Patel et al., 2017). IL-1R rises sharply in epilepsy (Allan et al., 2005; Ravizza and Vezzani, 2006; Maroso et al., 2010). IL-1β is commonly elevated in the serum and cerebrospinal fluid (CSF) of patients with epilepsy (Bauer et al., 2009; Shi et al., 2017). Similarly, in animal models of kainic acid (KA)-induced seizures, upregulation of IL-1β mRNA was observed in multiple brain regions including hippocampus, cerebral cortex, thalamus, hypothalamus, and striatum (Minami et al., 1991). One study showed that high doses of IL-1β induced seizures in mice expressing the IL-1β receptor (Dubé et al., 2005). Meanwhile, exogenous IL-1β can increase the proportion of epilepsy in rats after prolonged febrile convulsion (Fukuda et al., 2014). Intrahippocampal administration of IL-1β before KA injection in rodents significantly enhanced seizure severity and duration (Vezzani et al., 2002), suggesting a convulsive role of IL-1β in acute seizures. Therefore, IL-β system may also act as a novel target for anticonvulsant drugs. One study uses an antagonist of the IL-1β receptor IL-1R to prevent susceptibility to chronic seizures (Fukuda et al., 2014). Several studies have used anakinra (an antagonist of the IL-1R1 receptor) or anti-IL-1β monoclonal antibodies in animal models, both of which have been shown to reduce the occurrence of epilepsy (Vezzani, 2015; Dey et al., 2016). Interestingly, the combination of IL-1β and IL-6 antibodies is effective in preventing chronic epilepsy (Pineda et al., 2013).
5.1.4 ROS exacerbates inflammation in brain tissues
Oxidative stress can induce inflammation, and inflammation can also promote the production of ROS (Lv et al., 2016). Brain tissue is vulnerable to oxidative stress (Freitas, 2009). It was demonstrated that oxidative stress-related seizures change the activity of antioxidant enzymes and the ability of receptor binding (Freitas et al., 2005). TNF-α is the key to coordinate oxidative stress and inflammation in the central nervous system (Gustafson et al., 2012). When the oxygen supply to the brain is insufficient, a large amount of ROS was produced, which then promotes the release of TNF-α (Wall et al., 2015). As an inflammatory factor in the brain, TNF-α can also rapidly increase the production of ROS and cause apoptosis mediated by oxidative stress in brain microvascular endothelial cells (Basuroy et al., 2011). In addition to TNF-α, oxidative stress can also upregulate the expression of IL-6 (Cho et al., 2005). IL-6 can aggravate the inflammatory response of premature infants and aggravate hypoxia-induced brain damage (Dammann and Leviton, 2000). Increased expression of IL-6 is involved in various neurological or mental diseases such as stroke, depression, epilepsy, Alzheimer’s disease, autism, et al (Wei et al., 2013; Ting et al., 2020; Rahman et al., 2021; Soltani Khaboushan et al., 2022; Zhu et al., 2022).
5.1.5 Recent perspectives on neuroinflammatory factors and epilepsy
The latest view is that neuroinflammation is not a direct cause of epilepsy, but a modulator of epilepsy and a disease-inducing factor. Studies have shown that an increase in inflammatory molecules in the brain alone does not cause epilepsy (Iori et al., 2016; Villasana-Salazar and Vezzani, 2023), and epilepsy does not go into remission after treatment with anti-inflammatory drugs (Villasana-Salazar and Vezzani, 2023) There is a lot of evidence that inflammation contributes to seizures and seizures (Choi and Koh, 2008; Chiang and Serhan, 2017; Mukhtar, 2020). Signaling downstream of inflammation can lead to neuronal damage, which can affect the clinical manifestations of pathology. Patients with autoimmune diseases and encephalitis characterized by severe neuroinflammatory responses often exhibit a high incidence of seizures (Michael and Solomon, 2012; Sakamoto et al., 2022). The hyperexcitability that leads to seizures is the result of an imbalance in glutamatergic and GABAergic signaling. Many inflammatory mediators released by activated microglia and astrocytes lower the seizure threshold by regulating glutamatergic and GABAergic signaling. For instance, increase in TNF-α leads to upregulation of AMPA receptors and endocytosis of GABAergic receptors. IL-1β upregulates NMDA receptors, enhances glutamate release, and inhibits GABA release. Similarly, IL-6 also can promote glutamate release to enhance neuronal excitability. Therefore, the reduced seizure threshold and uninduced seizures are caused by a number of pathological changes, rather than directly induced by neuroinflammatory factors (Chen et al., 2023).
5.2 Inflammation and oxidative stress induced by OSAS: potential links to epilepsy via ER stress
The endoplasmic reticulum (ER) is a vital organelle in eukaryotic cells that performs several functions such as protein folding and quality control, cellular calcium ion homeostasis, and lipid biosynthesis (Anelli and Sitia, 2008; Song et al., 2017). However, various intracellular and extracellular factors such as hypoxia, oxidative injury, and mutant protein expression can disrupt ER function, leading to the accumulation of unfolded or misfolded proteins, which is termed as ER stress (Iurlaro and Muñoz-Pinedo, 2016; Hang et al., 2022). The degradation of misfolded proteins can start in the ER with the unfolded protein response (UPR) activation, which controls the stability of RNAs and the rate of protein synthesis, and activates the transcription of large amounts of genes (Yoshida, 2007; Cornejo et al., 2013; Coleman and Haller, 2019).
The UPR is a signal transduction pathway that involves stress sensors at the ER membrane and downstream transcription factors reprogramming gene expression towards either stress mitigation or the induction of proapoptotic programs (Chow et al., 2015). The three main ER transmembrane proteins, namely protein kinase R-like ER kinase (PERK), inositol-requiring enzyme 1 (IRE1), and activating transcription factor 6 (ATF6), initiate the UPR process (Hashimoto and Saido, 2018; Fu et al., 2020; He et al., 2020). PERK, IRE1, and ATF6 have both pro-apoptotic and protective functions, and there is an overlap amongst their activities (Fu et al., 2018; Balsa et al., 2019; Di Conza and Ho, 2020). Under normal conditions, the ER chaperone 78-kDa glucose-regulated protein (GRP78), inhibits the activation of these ER transmembrane proteins by binding to their lumenal domains (Bertolotti et al., 2000). However, under ER stress, the accumulation of misfolded and unfolded proteins could result in the dissociation of GRP78 from the lumenal domains, which subsequently lead to their activation (Lebeaupin et al., 2018). There is increasing evidence to suggest the crucial role of the ER stress and UPR in the pathogenesis of epilepsy (Yamamoto et al., 2006; Liu et al., 2019; Nowakowska et al., 2020). Moreover, interventions aimed at mitigating ER stress have demonstrated both antiepileptic and neuroprotective effects in epilepsy (Zhang et al., 2019; Fu et al., 2020). Thus, ER stress is considered as one of the predominant mechanisms in the pathogenesis of epilepsy (Figure 2).
5.2.1 Role of ROS in exacerbating ER stress
As is mentioned above, inflammation and high levels of ROS is common among patients with OSAS due to intermittent hypoxia. Inflammation and ROS are also involved in the induction of ER stress, which contribute to epilepsy (Wang et al., 2014; Li et al., 2019).
Oxidative stress has the potential to disrupt endoplasmic reticulum (ER) function and trigger ER stress (Wang et al., 2017). In ER, ROS stimulate the activation of PERK, ATF-6α, and IRE1α, which increased abnormal protein folding and subsequently triggers endoplasmic reticulum (ER) stress (Zhang and Kaufman, 2008; Zhang et al., 2019). ROS-mediated UPR could lead to apoptosis through the activation of PERK (Hong et al., 2015). Meanwhile, upregulation of ATF-6α and IRE1α could also induce neuronal apoptosis (Li et al., 2013; Zhu et al., 2020). Neuronal apoptosis is a common outcome induced by seizures, which could lead to epileptogenesis and cognitive impairment (Bengzon et al., 2002; Henshall and Simon, 2005) (Figure 2).
5.2.2 Role of inflammation in exacerbating ER stress
Numerous studies have shown that inflammation is connected with ER stress, which is triggered by the activation of the NF-κB signaling pathway and the release of various proinflammatory cytokines (Dong et al., 2016). It was also noted in vivo. That exposure to IL-6 could reduce calcium storage of ER, activate UPR and thereby increased cell death (O'Neill et al., 2013). Notably, ER stress and inflammation are two-way interactions (Liu et al., 2012). Activated IRE-1 caused by UPR could recruit TRAF2, which result in activation of downstream signaling of kinases and upregulate the expression of various inflammatory cytokines, such as TNF-α and IL-6 (Amen et al., 2019). These inflammatory cytokines could in turn exacerbate ER stress and result in neuronal apoptosis (Figure 2).
6 Discussion
Emerging studies suggest that patients with OSAS are predisposed to injuries in nervous system. It is proposed in this study that intermittent hypoxia is the linkage between OSAS and epilepsy. Long-term intermittent hypoxia is common among patients with OSAS during sleep due to upper-airway collapsibility, which lead to high levels of oxidative stress and inflammation. ROS and inflammatory factors, primarily TNF-α and IL-6, result in nervous system insults via induction of inflammation and ER stress in brain tissues, which subsequently increase the risks of epilepsy (Figure 3).
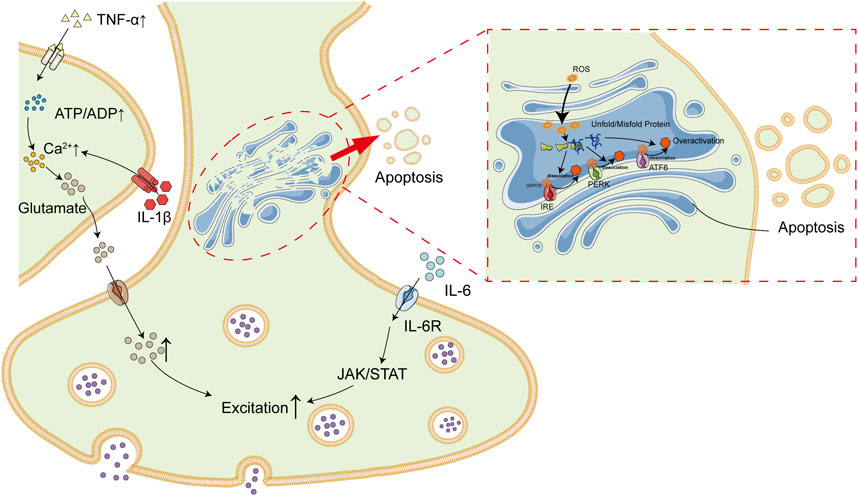
FIGURE 3. Association Between OSAS and Epilepsy. Patients with OSAS are in a state of long-term intermittent hypoxia due to upper airway collapse. Intermittent hypoxia result in high levels of inflammatory cytokines (e.g., IL-6 and TNF-α) and ROS, which subsequently lead to inflammatory and ER stress in brain tissues. In addition, IL-6 and TNF-α could also exacerbate each other. All of these deregulations finally contribute to high incidence of epilepsy among patients with OSAS.
Various drugs targeting inflammation and oxidative exhibit therapeutic effects on OSAS and epilepsy. Melatonin, which have broad effects in anti-inflammatory and anti-oxidative stress, was confirmed to have antiepileptic effects as an antioxidant and neuroprotective drug (Park et al., 2015; Khan et al., 2020). In addition, anti-inflammatory therapy is also an effective treatment to reduce seizures and also improve the prognosis (Gershen et al., 2015; Fan et al., 2019). ACTH, steroids and intravenous immunoglobulin (IVIg) have been used in the treatment of seizures (Vezzani et al., 2011b). It was also observed that various anti-inflammatory drugs have therapeutic effects on drug-resistant epilepsy through specific inflammatory pathways in epilepsy (Terrone et al., 2017). In addition, non-steroidal anti-inflammatory drugs have also been used in 2013 as a feasible choice of antiepileptic drugs (Yu et al., 2013).
Recently, there has been an increasing interest in the nervous system impairments among OSAS patients. OSAS could decrease the seizure threshold in epilepsy patients and thereby increase the incidence of epilepsy seizures (van Golde et al., 2011). In this study, we proposed that intermittent hypoxia caused by OSAS is a critical factor in the pathogenesis of epilepsy via upregulation of oxidative stress and inflammation levels. However, there have been relatively few studies focusing on the role of intermittent hypoxia in the relationship of OSAS and epilepsy. Therefore, greater focus on the status of hypoxia maybe warranted in those with OSAS to prevent and reduce damage to the nervous system. Researchers should first pay more attention to the sleep quality of epilepsy patients to confirm the existence of hypoxia. Next, it is necessary to investigate whether resolving intermittent hypoxia in epilepsy patients during sleep could result in a reduction of epilepsy symptoms. In addition, more studies are also required to further understand mechanisms linking OSAS and epilepsy, such as oxidative stress, inflammation, and ER stress, which might provide novel therapeutic targets on epilepsy.
Author contributions
YM conceived the project, wrote the manuscript, and produced figures. QW supervised the draft, revised the manuscript and figures, and obtained the funding. All authors contributed to the article and approved the submitted version.
Funding
This work was sponsored by Project 82101525 supported by the National Natural Science Foundation of China, and Project LJKMZ20221199 supported by Education Foundation of Liaoning Province.
Conflict of interest
The authors declare that the research was conducted in the absence of any commercial or financial relationships that could be construed as a potential conflict of interest.
Publisher’s note
All claims expressed in this article are solely those of the authors and do not necessarily represent those of their affiliated organizations, or those of the publisher, the editors and the reviewers. Any product that may be evaluated in this article, or claim that may be made by its manufacturer, is not guaranteed or endorsed by the publisher.
References
Aherne, W., and Hull, D. (1966). Brown adipose tissue and heat production in the newborn infant. J. pathology Bacteriol. 91, 223–234. doi:10.1002/path.1700910126
Allan, S. M., Tyrrell, P. J., and Rothwell, N. J. (2005). Interleukin-1 and neuronal injury. Nat. Rev. Immunol. 5, 629–640. doi:10.1038/nri1664
Amen, O. M., Sarker, S. D., Ghildyal, R., and Arya, A. (2019). Endoplasmic reticulum stress activates unfolded protein response signaling and mediates inflammation, obesity, and cardiac dysfunction: therapeutic and molecular approach. Front. Pharmacol. 10, 977. doi:10.3389/fphar.2019.00977
Andaku, D. K., D'Almeida, V., Carneiro, G., Hix, S., Tufik, S., and Togeiro, S. M. (2015). Sleepiness, inflammation and oxidative stress markers in middle-aged males with obstructive sleep apnea without metabolic syndrome: a cross-sectional study. Respir. Res. 16, 3. doi:10.1186/s12931-015-0166-x
Anelli, T., and Sitia, R. (2008). Protein quality control in the early secretory pathway. EMBO J. 27, 315–327. doi:10.1038/sj.emboj.7601974
Azagra-Calero, E., Espinar-Escalona, E., Barrera-Mora, J. M., Llamas-Carreras, J. M., and Solano-Reina, E. (2012). Obstructive sleep apnea syndrome (OSAS). Review of the literature. Med. oral, Patol. oral cirugia bucal 17, e925–e929. doi:10.4317/medoral.17706
Ba-Diop, A., Marin, B., Druet-Cabanac, M., Ngoungou, E. B., Newton, C. R., and Preux, P. M. (2014). Epidemiology, causes, and treatment of epilepsy in sub-Saharan Africa. Neurology 13, 1029–1044. doi:10.1016/S1474-4422(14)70114-0
Baessler, A., Nadeem, R., Harvey, M., Madbouly, E., Younus, A., Sajid, H., et al. (2013). Treatment for sleep apnea by continuous positive airway pressure improves levels of inflammatory markers - a meta-analysis. J. Inflamm. Lond. Engl. 10, 13. doi:10.1186/1476-9255-10-13
Balosso, S., Ravizza, T., Perego, C., Peschon, J., Campbell, I. L., De Simoni, M. G., et al. (2005). Tumor necrosis factor-alpha inhibits seizures in mice via p75 receptors. Ann. neurology 57, 804–812. doi:10.1002/ana.20480
Balsa, E., Soustek, M. S., Thomas, A., Cogliati, S., García-Poyatos, C., Martín-García, E., et al. (2019). ER and nutrient stress promote assembly of respiratory chain supercomplexes through the PERK-eIF2α Axis. Mol. Cell. 74, 877–890.e6. doi:10.1016/j.molcel.2019.03.031
Basuroy, S., Tcheranova, D., Bhattacharya, S., Leffler, C. W., and Parfenova, H. (2011). Nox4 NADPH oxidase-derived reactive oxygen species, via endogenous carbon monoxide, promote survival of brain endothelial cells during TNF-α-induced apoptosis. Am. J. Physiol. Cell. Physiol. 300, C256–C265. doi:10.1152/ajpcell.00272.2010
Bateman, L. M., Li, C. S., and Seyal, M. (2008). Ictal hypoxemia in localization-related epilepsy: analysis of incidence, severity and risk factors. Brain 131, 3239–3245. doi:10.1093/brain/awn277
Batool-Anwar, S., Kales, S. N., Patel, S. R., Varvarigou, V., DeYoung, P. N., and Malhotra, A. (2014). Obstructive sleep apnea and psychomotor vigilance task performance. Nat. Sci. sleep 6, 65–71. doi:10.2147/NSS.S53721
Bauer, S., Cepok, S., Todorova-Rudolph, A., Nowak, M., Köller, M., Lorenz, R., et al. (2009). Etiology and site of temporal lobe epilepsy influence postictal cytokine release. Epilepsy Res. 86, 82–88. doi:10.1016/j.eplepsyres.2009.05.009
Bedner, P., and Steinhäuser, C. (2019). Tnfα-Driven astrocyte purinergic signaling during epileptogenesis. Trends Mol. Med. 25, 70–72. doi:10.1016/j.molmed.2018.12.001
Bengzon, J., Mohapel, P., Ekdahl, C. T., and Lindvall, O. (2002). Neuronal apoptosis after brief and prolonged seizures. Prog. Brain Res. 135, 111–119. doi:10.1016/S0079-6123(02)35011-8
Benjafield, A. V., Ayas, N. T., Eastwood, P. R., Heinzer, R., Ip, M. S. M., Morrell, M. J., et al. (2019). Estimation of the global prevalence and burden of obstructive sleep apnoea: a literature-based analysis. Respir. Med. 7, 687–698. doi:10.1016/S2213-2600(19)30198-5
Bertolotti, A., Zhang, Y., Hendershot, L. M., Harding, H. P., and Ron, D. (2000). Dynamic interaction of BiP and ER stress transducers in the unfolded-protein response. Nat. Cell. Biol. 2, 326–332. doi:10.1038/35014014
Biddlestone, J., Bandarra, D., and Rocha, S. (2015). The role of hypoxia in inflammatory disease (review). Int. J. Mol. Med. 35, 859–869. doi:10.3892/ijmm.2015.2079
Bradshaw, P. C., Seeds, W. A., Miller, A. C., Mahajan, V. R., and Curtis, W. M. (2020). COVID-19: proposing a ketone-based metabolic therapy as a treatment to blunt the cytokine storm. Oxidative Med. Cell. Longev. 2020, 6401341. doi:10.1155/2020/6401341
Brunetti, V., Ferilli, M. A. N., Vollono, C., and Della Marca, G. (2017). Sleep apneas trigger epilepsy. Sleep. Med. 39, 23–24. doi:10.1016/j.sleep.2017.08.014
Burnsed, J., Skwarzyńska, D., Wagley, P. K., Isbell, L., and Kapur, J. (2019). Neuronal circuit activity during neonatal hypoxic-ischemic seizures in mice. Ann. Neurol. 86, 927–938. doi:10.1002/ana.25601
Burtscher, J., Mallet, R. T., Burtscher, M., and Millet, G. P. (2021). Hypoxia and brain aging: neurodegeneration or neuroprotection? Ageing Res. Rev. 68, 101343. doi:10.1016/j.arr.2021.101343
Cerri, C., Caleo, M., and Bozzi, Y. (2017). Chemokines as new inflammatory players in the pathogenesis of epilepsy. Epilepsy Res. 136, 77–83. doi:10.1016/j.eplepsyres.2017.07.016
Chen, Y., Nagib, M. M., Yasmen, N., Sluter, M. N., Littlejohn, T. L., Yu, Y., et al. (2023). Neuroinflammatory mediators in acquired epilepsy: an update. Inflamm. Res. 72, 683–701. doi:10.1007/s00011-023-01700-8
Chiang, N., and Serhan, C. N. (2017). Structural elucidation and physiologic functions of specialized pro-resolving mediators and their receptors. Mol. Asp. Med. 58, 114–129. doi:10.1016/j.mam.2017.03.005
Cho, I. J., Lee, A. K., Lee, S. J., Lee, M. G., and Kim, S. G. (2005). Repression by oxidative stress of iNOS and cytokine gene induction in macrophages results from AP-1 and NF-kappaB inhibition mediated by B cell translocation gene-1 activation. Free Radic. Biol. Med. 39, 1523–1536. doi:10.1016/j.freeradbiomed.2005.07.017
Choi, J., and Koh, S. (2008). Role of brain inflammation in epileptogenesis. Yonsei Med. J. 49, 1–18. doi:10.3349/ymj.2008.49.1.1
Chou, T. F., Chuang, Y. T., Hsieh, W. C., Chang, P. Y., Liu, H. Y., Mo, S. T., et al. (2016). Tumour suppressor death-associated protein kinase targets cytoplasmic HIF-1α for Th17 suppression. Nat. Commun. 7, 11904. doi:10.1038/ncomms11904
Chow, C. Y., Wang, X., Riccardi, D., Wolfner, M. F., and Clark, A. G. (2015). The genetic architecture of the genome-wide transcriptional response to ER stress in the mouse. PLoS Genet. 11, e1004924. doi:10.1371/journal.pgen.1004924
Coleman, O. I., and Haller, D. (2019). ER stress and the UPR in shaping intestinal tissue homeostasis and immunity. Front. Immunol. 10, 2825. doi:10.3389/fimmu.2019.02825
Colgan, S. P., Campbell, E. L., and Kominsky, D. J. (2016). Hypoxia and mucosal inflammation. Annu. Rev. pathology 11, 77–100. doi:10.1146/annurev-pathol-012615-044231
Cornejo, V. H., Pihán, P., Vidal, R. L., and Hetz, C. (2013). Role of the unfolded protein response in organ physiology: lessons from mouse models. IUBMB life 65, 962–975. doi:10.1002/iub.1224
Coste, J., Mandereau-Bruno, L., Carcaillon-Bentata, L., Mikaeloff, Y., and Bouilleret, V. (2023). Prevalence, demographic and spatial distribution of treated epilepsy in France in 2020: a study based on the French national health data system. J. neurology. doi:10.1007/s00415-023-11953-2
Cramer, N. P., Korotcov, A., Bosomtwi, A., Xu, X., Holman, D. R., Whiting, K., et al. (2019). Neuronal and vascular deficits following chronic adaptation to high altitude. Exp. Neurol. 311, 293–304. doi:10.1016/j.expneurol.2018.10.007
Cronstein, B. N., and Sitkovsky, M. (2017). Adenosine and adenosine receptors in the pathogenesis and treatment of rheumatic diseases. Nat. Rev. Rheumatol. 13, 41–51. doi:10.1038/nrrheum.2016.178
Dammann, O., and Leviton, A. (2000). Brain damage in preterm newborns: biological response modification as a strategy to reduce disabilities. J. Pediatr. 136, 433–438. doi:10.1016/s0022-3476(00)90004-0
Dang, J., Tiwari, S. K., Agrawal, K., Hui, H., Qin, Y., and Rana, T. M. (2021). Glial cell diversity and methamphetamine-induced neuroinflammation in human cerebral organoids. Mol. psychiatry 26, 1194–1207. doi:10.1038/s41380-020-0676-x
Devaraj, S., Davis, B., Simon, S. I., and Jialal, I. (2006). CRP promotes monocyte-endothelial cell adhesion via Fcgamma receptors in human aortic endothelial cells under static and shear flow conditions. Am. J. physiology. Heart circulatory physiology 291, H1170–H1176. doi:10.1152/ajpheart.00150.2006
Devinsky, O., Ehrenberg, B., Barthlen, G. M., Abramson, H. S., and Luciano, D. (1994). Epilepsy and sleep apnea syndrome. Neurology 44, 2060–2064. doi:10.1212/wnl.44.11.2060
Dey, A., Kang, X., Qiu, J., Du, Y., and Jiang, J. (2016). Anti-inflammatory small molecules to treat seizures and epilepsy: from bench to bedside. Trends Pharmacol. Sci. 37, 463–484. doi:10.1016/j.tips.2016.03.001
Dheer, A., Jain, V., Kushwah, N., Kumar, R., Prasad, D., and Singh, S. B. (2018). Temporal and spatial changes in glial cells during chronic hypobaric hypoxia: role in neurodegeneration. Neuroscience 383, 235–246. doi:10.1016/j.neuroscience.2018.04.026
Di Conza, G., and Ho, P. C. (2020). ER stress responses: an emerging modulator for innate immunity. Cells 9, 695. doi:10.3390/cells9030695
Dilger, R. N., and Johnson, R. W. (2008). Aging, microglial cell priming, and the discordant central inflammatory response to signals from the peripheral immune system. J. Leukoc. Biol. 84, 932–939. doi:10.1189/jlb.0208108
Dinarello, C. A. (2004). Infection, fever, and exogenous and endogenous pyrogens: some concepts have changed. J. endotoxin Res. 10, 201–222. doi:10.1179/096805104225006129
Dinarello, C. A. (2011). Interleukin-1 in the pathogenesis and treatment of inflammatory diseases. Blood 117, 3720–3732. doi:10.1182/blood-2010-07-273417
Dong, B., Lu, Y., He, S., Li, B., Li, Y., Lai, Q., et al. (2023). Multisite and multitimepoint proteomics reveal that patent foramen ovale closure improves migraine and epilepsy by reducing right-to-left shunt-induced hypoxia. MedComm (2020) 4, e334. doi:10.1002/mco2.334
Dong, Y. F., Chen, Z. Z., Zhao, Z., Yang, D. D., Yan, H., Ji, J., et al. (2016). Potential role of microRNA-7 in the anti-neuroinflammation effects of nicorandil in astrocytes induced by oxygen-glucose deprivation. J. neuroinflammation 13, 60. doi:10.1186/s12974-016-0527-5
Drum, B. M., Yuan, C., Li, L., Liu, Q., Wordeman, L., and Santana, L. F. (2016). Oxidative stress decreases microtubule growth and stability in ventricular myocytes. J. Mol. Cell. Cardiol. 93, 32–43. doi:10.1016/j.yjmcc.2016.02.012
Dubé, C., Vezzani, A., Behrens, M., Bartfai, T., and Baram, T. Z. (2005). Interleukin-1beta contributes to the generation of experimental febrile seizures. Ann. Neurol. 57, 152–155. doi:10.1002/ana.20358
Durán, J., Esnaola, S., Rubio, R., and Iztueta, A. (2001). Obstructive sleep apnea-hypopnea and related clinical features in a population-based sample of subjects aged 30 to 70 yr. Am. J. Respir. Crit. care Med. 163, 685–689. doi:10.1164/ajrccm.163.3.2005065
Ebben, M. R., Sethi, N. K., Conte, M., Pollak, C. P., and Labar, D. (2008). Vagus nerve stimulation, sleep apnea, and CPAP titration. J. Clin. Sleep. Med. 4, 471–473. doi:10.5664/jcsm.27284
Eisele, H. J., Markart, P., and Schulz, R. (2015). Obstructive sleep apnea, oxidative stress, and cardiovascular disease: evidence from human studies. Oxidative Med. Cell. Longev. 2015, 608438. doi:10.1155/2015/608438
Engel, J. (1995). Concepts of epilepsy. Epilepsia 36 (Suppl. 1), S23–S29. doi:10.1111/j.1528-1157.1995.tb01648.x
Fan, J. J., Shan, W., Wu, J. P., and Wang, Q. (2019). Research progress of vagus nerve stimulation in the treatment of epilepsy. CNS Neurosci. Ther. 25, 1222–1228. doi:10.1111/cns.13209
Feng, Y. M., Thijs, L., Zhang, Z. Y., Yang, W. Y., Huang, Q. F., Wei, F. F., et al. (2018). Glomerular function in relation to circulating adhesion molecules and inflammation markers in a general population. Nephrol. Dial. Transplant. 33, 426–435. doi:10.1093/ndt/gfx256
Ferguson, M. S., Magill, J. C., and Kotecha, B. T. (2017). Narrative review of contemporary treatment options in the care of patients with obstructive sleep apnoea. Ther. Adv. Respir. Dis. 11, 411–423. doi:10.1177/1753465817736263
Fisher, R. S., Acevedo, C., Arzimanoglou, A., Bogacz, A., Cross, J. H., Elger, C. E., et al. (2014). ILAE official report: a practical clinical definition of epilepsy. Epilepsia 55, 475–482. doi:10.1111/epi.12550
Fisher, R. S., van Emde Boas, W., Blume, W., Elger, C., Genton, P., Lee, P., et al. (2005). Epileptic seizures and epilepsy: definitions proposed by the international league against epilepsy (ILAE) and the international bureau for epilepsy (IBE). Epilepsia 46, 470–472. doi:10.1111/j.0013-9580.2005.66104.x
Fleiss, B., and Gressens, P. (2012). Tertiary mechanisms of brain damage: a new hope for treatment of cerebral palsy? Lancet Neurol. 11, 556–566. doi:10.1016/S1474-4422(12)70058-3
Foldvary-Schaefer, N., Stephenson, L., and Bingaman, W. (2008). Resolution of obstructive sleep apnea with epilepsy surgery? Expanding the relationship between sleep and epilepsy. Epilepsia 49, 1457–1459. doi:10.1111/j.1528-1167.2008.01677.x
Forsgren, L., Beghi, E., Oun, A., and Sillanpää, M. (2005). The epidemiology of epilepsy in Europe - a systematic review. Eur. J. neurology 12, 245–253. doi:10.1111/j.1468-1331.2004.00992.x
Freitas, R. M. (2009). Investigation of oxidative stress involvement in hippocampus in epilepsy model induced by pilocarpine. Neurosci. Lett. 462, 225–229. doi:10.1016/j.neulet.2009.07.037
Freitas, R. M., Vasconcelos, S. M., Souza, F. C., Viana, G. S., and Fonteles, M. M. (2005). Oxidative stress in the hippocampus after pilocarpine-induced status epilepticus in Wistar rats. Febs J. 272, 1307–1312. doi:10.1111/j.1742-4658.2004.04537.x
Fu, J., Tao, T., Li, Z., Chen, Y., Li, J., and Peng, L. (2020). The roles of ER stress in epilepsy: molecular mechanisms and therapeutic implications. Biomed. Pharmacother. = Biomedecine Pharmacother. 131, 110658. doi:10.1016/j.biopha.2020.110658
Fu, Y. L., Han, D. Y., Wang, Y. J., Di, X. J., Yu, H. B., and Mu, T. W. (2018). Remodeling the endoplasmic reticulum proteostasis network restores proteostasis of pathogenic GABAA receptors. PloS one 13, e0207948. doi:10.1371/journal.pone.0207948
Fukuda, M., Hino, H., Suzuki, Y., Takahashi, H., Morimoto, T., and Ishii, E. (2014). Postnatal interleukin-1β enhances adulthood seizure susceptibility and neuronal cell death after prolonged experimental febrile seizures in infantile rats. Acta Neurol. Belg 114, 179–185. doi:10.1007/s13760-013-0246-y
Fukushi, I., Ikeda, K., Takeda, K., Yoshizawa, M., Kono, Y., Hasebe, Y., et al. (2023). Minocycline prevents hypoxia-induced seizures. Front. Neural Circuits 17, 1006424. doi:10.3389/fncir.2023.1006424
Garcia-Oscos, F., Salgado, H., Hall, S., Thomas, F., Farmer, G. E., Bermeo, J., et al. (2012). The stress-induced cytokine interleukin-6 decreases the inhibition/excitation ratio in the rat temporal cortex via trans-signaling. Biol. psychiatry 71, 574–582. doi:10.1016/j.biopsych.2011.11.018
Gershen, L. D., Zanotti-Fregonara, P., Dustin, I. H., Liow, J. S., Hirvonen, J., Kreisl, W. C., et al. (2015). Neuroinflammation in temporal lobe epilepsy measured using positron emission tomographic imaging of translocator protein. JAMA neurol. 72, 882–888. doi:10.1001/jamaneurol.2015.0941
Gopal, S. (2020). Syndecans in inflammation at a glance. Front. Immunol. 11, 227. doi:10.3389/fimmu.2020.00227
Grigg-Damberger, M., and Foldvary-Schaefer, N. (2021). Bidirectional relationships of sleep and epilepsy in adults with epilepsy. Epilepsy & Behav. E&B 116, 107735. doi:10.1016/j.yebeh.2020.107735
Grigg-Damberger, M., and Foldvary-Schaefer, N. (2023). Hypoxia not AHI in Adults with Sleep Apnea Midlife Markedly Increases Risk of Late Onset Epilepsy Carosella CM et al Sleep apnea, hypoxia, and late-onset epilepsy: the Atherosclerosis Risk in Communities study SLEEP-2023-0175.R1. Sleep. doi:10.1093/sleep/zsad252
Gruol, D. L. (2015). IL-6 regulation of synaptic function in the CNS. Neuropharmacology 96, 42–54. doi:10.1016/j.neuropharm.2014.10.023
Gustafson, S. J., Dunlap, K. L., McGill, C. M., and Kuhn, T. B. (2012). A nonpolar blueberry fraction blunts NADPH oxidase activation in neuronal cells exposed to tumor necrosis factor-α. Oxid. Med. Cell. Longev. 2012, 768101. doi:10.1155/2012/768101
Haba-Rubio, J., Janssens, J. P., Rochat, T., and Sforza, E. (2005). Rapid eye movement-related disordered breathing: clinical and polysomnographic features. Chest 128, 3350–3357. doi:10.1378/chest.128.5.3350
Hafner, C., Wu, J., Tiboldi, A., Hess, M., Mitulovic, G., Kaun, C., et al. (2017). Hyperoxia induces inflammation and cytotoxicity in human adult cardiac myocytes. Shock 47, 436–444. doi:10.1097/SHK.0000000000000740
Han, C. L., Liu, Y. P., Guo, C. J., Du, T. T., Jiang, Y., Wang, K. L., et al. (2020). The lncRNA H19 binding to let-7b promotes hippocampal glial cell activation and epileptic seizures by targeting Stat3 in a rat model of temporal lobe epilepsy. Cell. Prolif. 53, e12856. doi:10.1111/cpr.12856
Hang, J., Wang, J., Lu, M., Xue, Y., Qiao, J., and Tao, L. (2022). Protein O-mannosylation across kingdoms and related diseases: from glycobiology to glycopathology. Biomed. Pharmacother. = Biomedecine Pharmacother. 148, 112685. doi:10.1016/j.biopha.2022.112685
Hashimoto, S., and Saido, T. C. (2018). Critical review: involvement of endoplasmic reticulum stress in the aetiology of Alzheimer's disease. Open Biol. 8, 180024. doi:10.1098/rsob.180024
He, Y. X., Shen, Q. Y., Tian, J. H., Wu, Q., Xue, Q., Zhang, G. P., et al. (2020). Zonisamide ameliorates cognitive impairment by inhibiting ER stress in a mouse model of type 2 diabetes mellitus. Front. aging Neurosci. 12, 192. doi:10.3389/fnagi.2020.00192
Henning, L., Antony, H., Breuer, A., Müller, J., Seifert, G., Audinat, E., et al. (2023). Reactive microglia are the major source of tumor necrosis factor alpha and contribute to astrocyte dysfunction and acute seizures in experimental temporal lobe epilepsy. Glia 71, 168–186. doi:10.1002/glia.24265
Henshall, D. C., and Simon, R. P. (2005). Epilepsy and apoptosis pathways. J. Int. Soc. Cereb. Blood Flow Metabolism 25, 1557–1572. doi:10.1038/sj.jcbfm.9600149
Hong, S., Beja-Glasser, V. F., Nfonoyim, B. M., Frouin, A., Li, S., Ramakrishnan, S., et al. (2016). Complement and microglia mediate early synapse loss in Alzheimer mouse models. Sci. (New York, N.Y.) 352, 712–716. doi:10.1126/science.aad8373
Hong, Y. H., Uddin, M. H., Jo, U., Kim, B., Song, J., Suh, D. H., et al. (2015). ROS accumulation by PEITC selectively kills ovarian cancer cells via UPR-mediated apoptosis. Front. Oncol. 5, 167. doi:10.3389/fonc.2015.00167
Hop, H. T., Reyes, A. W. B., Huy, T. X. N., Arayan, L. T., Min, W., Lee, H. J., et al. (2017). Activation of NF-kB-Mediated TNF-induced antimicrobial immunity is required for the efficient Brucella abortus clearance in RAW 264.7 cells. Front. Cell. Infect. Microbiol. 7, 437. doi:10.3389/fcimb.2017.00437
Hu, J. J., Yang, X. L., Luo, W. D., Han, S., Yin, J., Liu, W. H., et al. (2017). Bumetanide reduce the seizure susceptibility induced by pentylenetetrazol via inhibition of aberrant hippocampal neurogenesis in neonatal rats after hypoxia-ischemia. Brain Res. Bull. 130, 188–199. doi:10.1016/j.brainresbull.2017.01.022
Huang, T., Lin, B. M., Markt, S. C., Stampfer, M. J., Laden, F., Hu, F. B., et al. (2018). Sex differences in the associations of obstructive sleep apnoea with epidemiological factors. Eur. Respir. J. 51, 1702421. doi:10.1183/13993003.02421-2017
Ibhazehiebo, K., Gavrilovici, C., de la Hoz, C. L., Ma, S. C., Rehak, R., Kaushik, G., et al. (2018). A novel metabolism-based phenotypic drug discovery platform in zebrafish uncovers HDACs 1 and 3 as a potential combined anti-seizure drug target. Brain J. Neurology 141, 744–761. doi:10.1093/brain/awx364
Imani, M. M., Sadeghi, M., Khazaie, H., Emami, M., Sadeghi Bahmani, D., and Brand, S. (2020). Evaluation of serum and plasma interleukin-6 levels in obstructive sleep apnea syndrome: a meta-analysis and meta-regression. Front. Immunol. 11, 1343. doi:10.3389/fimmu.2020.01343
Iori, V., Frigerio, F., and Vezzani, A. (2016). Modulation of neuronal excitability by immune mediators in epilepsy. Curr. Opin. Pharmacol. 26, 118–123. doi:10.1016/j.coph.2015.11.002
Iurlaro, R., and Muñoz-Pinedo, C. (2016). Cell death induced by endoplasmic reticulum stress. FEBS J. 283, 2640–2652. doi:10.1111/febs.13598
Jia, Y., Zhao, J., Liu, M., Li, B., Song, Y., Li, Y., et al. (2016). Brazilin exerts protective effects against renal ischemia-reperfusion injury by inhibiting the NF-κB signaling pathway. Int. J. Mol. Med. 38, 210–216. doi:10.3892/ijmm.2016.2616
Jo, H., Choi, S., Kim, D., and Joo, E. (2022). Effects of obstructive sleep apnea on epilepsy, and continuous positive airway pressure as a treatment option. J. Clin. Med. 11, 2063. doi:10.3390/jcm11072063
Johnston, M. V. (1998). Selective vulnerability in the neonatal brain. Ann. Neurol. 44, 155–156. doi:10.1002/ana.410440202
Johnston, M. V., and Hoon, A. H. (2000). Possible mechanisms in infants for selective basal ganglia damage from asphyxia, kernicterus, or mitochondrial encephalopathies. J. Child. Neurol. 15, 588–591. doi:10.1177/088307380001500904
Justice, J. A., and Sanchez, R. M. (2018). A rat model of perinatal seizures provoked by global hypoxia. Methods Mol. Biol. 1717, 155–159. doi:10.1007/978-1-4939-7526-6_13
Kalueff, A. V., Lehtimaki, K. A., Ylinen, A., Honkaniemi, J., and Peltola, J. (2004). Intranasal administration of human IL-6 increases the severity of chemically induced seizures in rats. Neurosci. Lett. 365, 106–110. doi:10.1016/j.neulet.2004.04.061
Kanbayashi, T., Kodama, T., Kondo, H., Satoh, S., Inoue, Y., Chiba, S., et al. (2009). CSF histamine contents in narcolepsy, idiopathic hypersomnia and obstructive sleep apnea syndrome. Sleep 32, 181–187. doi:10.1093/sleep/32.2.181
Kapur, V. K., Auckley, D. H., Chowdhuri, S., Kuhlmann, D. C., Mehra, R., Ramar, K., et al. (2017). Clinical practice guideline for diagnostic testing for adult obstructive sleep apnea: an American academy of sleep medicine clinical practice guideline. J. Clin. sleep Med. 13, 479–504. doi:10.5664/jcsm.6506
Kaur, S., De Luca, R., Khanday, M. A., Bandaru, S. S., Thomas, R. C., Broadhurst, R. Y., et al. (2020). Role of serotonergic dorsal raphe neurons in hypercapnia-induced arousals. Nat. Commun. 11, 2769. doi:10.1038/s41467-020-16518-9
Khan, S., Khurana, M., Vyas, P., and Vohora, D. (2020). The role of melatonin and its analogues in epilepsy. Rev. Neurosci. 32, 49–67. doi:10.1515/revneuro-2019-0088
Kiernan, E. A., Smith, S. M., Mitchell, G. S., and Watters, J. J. (2016). Mechanisms of microglial activation in models of inflammation and hypoxia: implications for chronic intermittent hypoxia. J. physiology 594, 1563–1577. doi:10.1113/JP271502
Kim, K. S., Kim, J. H., Park, S. Y., Won, H. R., Lee, H. J., Yang, H. S., et al. (2012). Smoking induces oropharyngeal narrowing and increases the severity of obstructive sleep apnea syndrome. J. Clin. Sleep. Med. 8, 367–374. doi:10.5664/jcsm.2024
Klein, C., Martinez, D., Hackenhaar, F. S., Medeiros, T. M., Marcolin, M. L., Silveira, F. S., et al. (2010). Carbonyl groups: bridging the gap between sleep disordered breathing and coronary artery disease. Free Radic. Res. 44, 907–912. doi:10.3109/10715762.2010.489112
Kohler, M. J., Lushington, K., and Kennedy, J. D. (2010). Neurocognitive performance and behavior before and after treatment for sleep-disordered breathing in children. Nat. Sci. sleep 2, 159–185. doi:10.2147/NSS.S6934
Kong, Q., Liu, K., Wu, L., and Wang, L. (2012). Heme oxygenase-1 inhibits neuropathic pain in rats with diabetic mellitus. Neural Regen. Res. 7, 2305–2311. doi:10.3969/j.issn.1673-5374.2012.29.010
Kovac, S., Dinkova Kostova, A. T., Herrmann, A. M., Melzer, N., Meuth, S. G., and Gorji, A. (2017). Metabolic and homeostatic changes in seizures and acquired epilepsy-mitochondria, calcium dynamics and reactive oxygen species. Int. J. Mol. Sci. 18, 1935. doi:10.3390/ijms18091935
Kuloglu, N., Basaran, K. E., and Yakan, B. (2021). Investigation of vascular endothelial growth factor receptor-dependent neuroplasticity on rat nucleus tractus solitarius and phrenic nerve after chronic sustained hypoxia. J. Biochem. Mol. Toxicol. 35, e22918. doi:10.1002/jbt.22918
Lai, A. Y., Swayze, R. D., El-Husseini, A., and Song, C. (2006). Interleukin-1 beta modulates AMPA receptor expression and phosphorylation in hippocampal neurons. J. Neuroimmunol. 175, 97–106. doi:10.1016/j.jneuroim.2006.03.001
Lavie, L. (2003). Obstructive sleep apnoea syndrome--an oxidative stress disorder. Sleep. Med. Rev. 7, 35–51. doi:10.1053/smrv.2002.0261
Lavie, L. (2015). Oxidative stress in obstructive sleep apnea and intermittent hypoxia--revisited--the bad ugly and good: implications to the heart and brain. Sleep. Med. Rev. 20, 27–45. doi:10.1016/j.smrv.2014.07.003
Lawrence, T. (2009). The nuclear factor NF-kappaB pathway in inflammation. Cold Spring Harb. Perspect. Biol. 1, a001651. doi:10.1101/cshperspect.a001651
Lebeaupin, C., Vallée, D., Hazari, Y., Hetz, C., Chevet, E., and Bailly-Maitre, B. (2018). Endoplasmic reticulum stress signalling and the pathogenesis of non-alcoholic fatty liver disease. J. hepatology 69, 927–947. doi:10.1016/j.jhep.2018.06.008
Lehner, J., Frueh, J. S., and Datta, A. N. (2022). Sleep quality and architecture in Idiopathic generalized epilepsy: a systematic review and meta-analysis. Sleep. Med. Rev. 65, 101689. doi:10.1016/j.smrv.2022.101689
Leo, A., Nesci, V., Tallarico, M., Amodio, N., Gallo Cantafio, E. M., De Sarro, G., et al. (2020). IL-6 receptor blockade by tocilizumab has anti-absence and anti-epileptogenic effects in the WAG/rij rat model of absence epilepsy. J. Am. Soc. Exp. Neurother. 17, 2004–2014. doi:10.1007/s13311-020-00893-8
Lévy, P., Kohler, M., McNicholas, W. T., Barbé, F., McEvoy, R. D., Somers, V. K., et al. (2015). Obstructive sleep apnoea syndrome. Nat. Rev. Dis. Prim. 1, 15015. doi:10.1038/nrdp.2015.15
Li, C., Li, L., Chen, K., Wang, Y., Yang, F., and Wang, G. (2019). UFL1 alleviates lipopolysaccharide-induced cell damage and inflammation via regulation of the TLR4/NF-κB pathway in bovine mammary epithelial cells. Oxidative Med. Cell. Longev. 2019, 6505373. doi:10.1155/2019/6505373
Li, C., Ma, W., Zhao, Y., and Wang, H. (2021). Changes in the expression of endothelial monocyte-activating polypeptide II in the rat hippocampus following status epilepticus. Int. J. Mol. Med. 47, 699–707. doi:10.3892/ijmm.2020.4808
Li, M. X., Wang, Y., Hua, S. C., Li, C. M., Wang, M. P., Liu, Y., et al. (2005). The prevalence of obstructive sleep apnea-hypopnea syndrome in adults aged over 20 years in Changchun city. Zhonghua jie he he hu xi za zhi = Zhonghua jiehe he huxi zazhi = Chin. J. Tuberc. Respir. Dis. 28, 833–835.
Li, S., Yang, L., Selzer, M. E., and Hu, Y. (2013). Neuronal endoplasmic reticulum stress in axon injury and neurodegeneration. Ann. neurology 74, 768–777. doi:10.1002/ana.24005
Li, X., Zhao, Y., Chen, C., Yang, L., Lee, H. H., Wang, Z., et al. (2020). Critical role of matrix metalloproteinase 14 in adipose tissue remodeling during obesity. Mol. Cell. Biol. 40, e00564-19. doi:10.1128/MCB.00564-19
Li, Y., Vgontzas, A. N., Fernandez-Mendoza, J., Kritikou, I., Basta, M., Pejovic, S., et al. (2017). Objective, but not subjective, sleepiness is associated with inflammation in sleep apnea. Sleep 40, zsw033. doi:10.1093/sleep/zsw033
Libbey, J. E., Kennett, N. J., Wilcox, K. S., White, H. S., and Fujinami, R. S. (2011). Interleukin-6, produced by resident cells of the central nervous system and infiltrating cells, contributes to the development of seizures following viral infection. J. virology 85, 6913–6922. doi:10.1128/JVI.00458-11
Liimatainen, S., Fallah, M., Kharazmi, E., Peltola, M., and Peltola, J. (2009). Interleukin-6 levels are increased in temporal lobe epilepsy but not in extra-temporal lobe epilepsy. J. neurology 256, 796–802. doi:10.1007/s00415-009-5021-x
Lima-Silveira, L., Accorsi-Mendonça, D., Bonagamba, L. G. H., Almado, C. E. L., da Silva, M. P., Nedoboy, P. E., et al. (2019). Enhancement of excitatory transmission in NTS neurons projecting to ventral medulla of rats exposed to sustained hypoxia is blunted by minocycline. J. Physiol. 597, 2903–2923. doi:10.1113/JP277532
Lin, Z., Si, Q., and Xiaoyi, Z. (2017). Obstructive sleep apnoea in patients with epilepsy: a meta-analysis. Sleep Breath. = Schlaf Atmung 21, 263–270. doi:10.1007/s11325-016-1391-3
Liu, D. C., Eagleman, D. E., and Tsai, N. P. (2019). Novel roles of ER stress in repressing neural activity and seizures through Mdm2-and p53-dependent protein translation. PLoS Genet. 15, e1008364. doi:10.1371/journal.pgen.1008364
Liu, J., Ren, F., Cheng, Q., Bai, L., Shen, X., Gao, F., et al. (2012). Endoplasmic reticulum stress modulates liver inflammatory immune response in the pathogenesis of liver ischemia and reperfusion injury. Transplantation 94, 211–217. doi:10.1097/TP.0b013e318259d38e
Louter, M., Aarden, W. C., Lion, J., Bloem, B. R., and Overeem, S. (2012). Recognition and diagnosis of sleep disorders in Parkinson's disease. J. neurology 259, 2031–2040. doi:10.1007/s00415-012-6505-7
Lv, H., Yu, Z., Zheng, Y., Wang, L., Qin, X., Cheng, G., et al. (2016). Isovitexin exerts anti-inflammatory and anti-oxidant activities on lipopolysaccharide-induced acute lung injury by inhibiting MAPK and NF-κB and activating HO-1/Nrf2 pathways. Int. J. Biol. Sci. 12, 72–86. doi:10.7150/ijbs.13188
Lv, R., Liu, X., Zhang, Y., Dong, N., Wang, X., He, Y., et al. (2023). Pathophysiological mechanisms and therapeutic approaches in obstructive sleep apnea syndrome. Signal Transduct. Target. Ther. 8, 218. doi:10.1038/s41392-023-01496-3
Mac, T. L., Tran, D. S., Quet, F., Odermatt, P., Preux, P. M., and Tan, C. T. (2007). Epidemiology, aetiology, and clinical management of epilepsy in Asia: a systematic review. Lancet. Neurology 6, 533–543. doi:10.1016/S1474-4422(07)70127-8
Marcus, C. L., Keenan, B. T., Huang, J., Yuan, H., Pinto, S., Bradford, R. M., et al. (2017). The obstructive sleep apnoea syndrome in adolescents. Thorax 72, 720–728. doi:10.1136/thoraxjnl-2016-208660
Maroso, M., Balosso, S., Ravizza, T., Liu, J., Aronica, E., Iyer, A. M., et al. (2010). Toll-like receptor 4 and high-mobility group box-1 are involved in ictogenesis and can be targeted to reduce seizures. Nat. Med. 16, 413–419. doi:10.1038/nm.2127
Matsumoto, H., and Ajmonemarsan, C. (1964). CELLULAR MECHANISMS IN EXPERIMENTAL EPILEPTIC SEIZURES. Sci. (New York, N.Y.) 144, 193–194. doi:10.1126/science.144.3615.193
Maurousset, A., De Toffol, B., Praline, J., Biberon, J., and Limousin, N. (2017). High incidence of obstructive sleep apnea syndrome in patients with late-onset epilepsy. Neurophysiol. Clin. = Clin. Neurophysiol. 47, 55–61. doi:10.1016/j.neucli.2016.11.002
McCarter, A. R., Timm, P. C., Shepard, P. W., Sandness, D. J., Luu, T., McCarter, S. J., et al. (2018). Obstructive sleep apnea in refractory epilepsy: a pilot study investigating frequency, clinical features, and association with risk of sudden unexpected death in epilepsy. Epilepsia 59, 1973–1981. doi:10.1111/epi.14548
McNally, M. A., Chavez-Valdez, R., Felling, R. J., Flock, D. L., Northington, F. J., and Stafstrom, C. E. (2019). Seizure susceptibility correlates with brain injury in male mice treated with hypothermia after neonatal hypoxia-ischemia. Dev. Neurosci. 40, 576–585. doi:10.1159/000496468
Mehling, M., Johnson, T. A., Antel, J., Kappos, L., and Bar-Or, A. (2011). Clinical immunology of the sphingosine 1-phosphate receptor modulator fingolimod (FTY720) in multiple sclerosis. Neurology 76, S20–S27. doi:10.1212/WNL.0b013e31820db341
Meli, D. N., Loeffler, J. M., Baumann, P., Neumann, U., Buhl, T., Leppert, D., et al. (2004). In pneumococcal meningitis a novel water-soluble inhibitor of matrix metalloproteinases and TNF-alpha converting enzyme attenuates seizures and injury of the cerebral cortex. J. Neuroimmunol. 151, 6–11. doi:10.1016/j.jneuroim.2004.01.026
Meretoja, V. V., Dahlin, R. L., Wright, S., Kasper, F. K., and Mikos, A. G. (2013). The effect of hypoxia on the chondrogenic differentiation of co-cultured articular chondrocytes and mesenchymal stem cells in scaffolds. Biomaterials 34, 4266–4273. doi:10.1016/j.biomaterials.2013.02.064
Miano, S., Bachiller, C., Gutiérrez, M., Salcedo, A., Villa, M. P., and Peraita-Adrados, R. (2010). Paroxysmal activity and seizures associated with sleep breathing disorder in children: a possible overlap between diurnal and nocturnal symptoms. Seizure 19, 547–552. doi:10.1016/j.seizure.2010.07.015
Michael, B. D., and Solomon, T. (2012). Seizures and encephalitis: clinical features, management, and potential pathophysiologic mechanisms. Epilepsia 53 (Suppl. 4), 63–71. doi:10.1111/j.1528-1167.2012.03615.x
Milagro, J., Deviaene, M., Gil, E., Lázaro, J., Buyse, B., Testelmans, D., et al. (2019). Autonomic dysfunction increases cardiovascular risk in the presence of sleep apnea. Front. physiology 10, 620. doi:10.3389/fphys.2019.00620
Minami, M., Kuraishi, Y., and Satoh, M. (1991). Effects of kainic acid on messenger RNA levels of IL-1 beta, IL-6, TNF alpha and LIF in the rat brain. Biochem. Biophys. Res. Commun. 176, 593–598. doi:10.1016/s0006-291x(05)80225-6
Mishra, P., Jaseja, H., and Goyal, M. (2021). A critical analysis of the purported role of hypoxaemia in the comorbidity of obstructive sleep apnoea and epilepsy. Clin. physiology Funct. imaging 41, 4–9. doi:10.1111/cpf.12672
Mokhlesi, B., Finn, L. A., Hagen, E. W., Young, T., Hla, K. M., Van Cauter, E., et al. (2014). Obstructive sleep apnea during REM sleep and hypertension. results of the Wisconsin Sleep Cohort. Am. J. Respir. Crit. care Med. 190, 1158–1167. doi:10.1164/rccm.201406-1136OC
Morris, R., Kershaw, N. J., and Babon, J. J. (2018). The molecular details of cytokine signaling via the JAK/STAT pathway. Protein Sci. 27, 1984–2009. doi:10.1002/pro.3519
Mukhtar, I. (2020). Inflammatory and immune mechanisms underlying epileptogenesis and epilepsy: from pathogenesis to treatment target. Seizure 82, 65–79. doi:10.1016/j.seizure.2020.09.015
Na, H., Gan, Q., McParland, L., Yang, J. B., Yao, H., Tian, H., et al. (2020). Characterization of the effects of calcitonin gene-related peptide receptor antagonist for Alzheimer's disease. Neuropharmacology 168, 108017. doi:10.1016/j.neuropharm.2020.108017
Najafian, S. A., Farbood, Y., Sarkaki, A., and Ghafouri, S. (2021). FTY720 administration following hypoxia-induced neonatal seizure reverse cognitive impairments and severity of seizures in male and female adult rats: the role of inflammation. Neurosci. Lett. 748, 135675. doi:10.1016/j.neulet.2021.135675
Nakase, K., Kollmar, R., Lazar, J., Arjomandi, H., Sundaram, K., Silverman, J., et al. (2016). Laryngospasm, central and obstructive apnea during seizures: defining pathophysiology for sudden death in a rat model. Epilepsy Res. 128, 126–139. doi:10.1016/j.eplepsyres.2016.08.004
Neher, J. J., Neniskyte, U., and Brown, G. C. (2012). Primary phagocytosis of neurons by inflamed microglia: potential roles in neurodegeneration. Front. Pharmacol. 3, 27. doi:10.3389/fphar.2012.00027
Nikolic, L., Shen, W., Nobili, P., Virenque, A., Ulmann, L., and Audinat, E. (2018). Blocking TNFα-driven astrocyte purinergic signaling restores normal synaptic activity during epileptogenesis. Glia 66, 2673–2683. doi:10.1002/glia.23519
Noam, Y., Raol, Y. H., and Holmes, G. L. (2013). Searching for new targets for treatment of pediatric epilepsy. Epilepsy & Behav. 26, 253–260. doi:10.1016/j.yebeh.2012.09.022
Nowakowska, M., Gualtieri, F., von Rüden, E. L., Hansmann, F., Baumgärtner, W., Tipold, A., et al. (2020). Profiling the expression of endoplasmic reticulum stress associated heat shock proteins in animal epilepsy models. Neuroscience 429, 156–172. doi:10.1016/j.neuroscience.2019.12.015
Nunes, J. O. F., Apostolico, J. S., Andrade, D. A. G., Ruiz, F. S., Fernandes, E. R., Andersen, M. L., et al. (2018). Sleep deprivation predisposes allergic mice to neutrophilic lung inflammation. J. allergy Clin. Immunol. 141, 1018–1027. doi:10.1016/j.jaci.2017.06.025
Olszewska, E., Pietrewicz, T. M., Świderska, M., Jamiołkowski, J., and Chabowski, A. (2022). A case-control study on the changes in high-sensitivity C-reactive protein and tumor necrosis factor-alpha levels with surgical treatment of OSAS. Int. J. Mol. Sci. 23, 14116. doi:10.3390/ijms232214116
O’Neill, C. M., Lu, C., Corbin, K. L., Sharma, P. R., Dula, S. B., Carter, J. D., et al. (2013). Circulating levels of IL-1B+IL-6 cause ER stress and dysfunction in islets from prediabetic male mice. Endocrinology 154, 3077–3088. doi:10.1210/en.2012-2138
Parfenova, H., Leffler, C. W., Basuroy, S., Liu, J., and Fedinec, A. L. (2012). Antioxidant roles of heme oxygenase, carbon monoxide, and bilirubin in cerebral circulation during seizures. J. Cereb. Blood Flow. Metab. 32, 1024–1034. doi:10.1038/jcbfm.2012.13
Parhizgar, F., Nugent, K., and Raj, R. (2011). Obstructive sleep apnea and respiratory complications associated with vagus nerve stimulators. J. Clin. Sleep. Med. 7, 401–407. doi:10.5664/JCSM.1204
Park, Y. S., Chung, S. H., Lee, S. K., Kim, J. H., Kim, J. B., Kim, T. K., et al. (2015). Melatonin improves experimental colitis with sleep deprivation. Int. J. Mol. Med. 35, 979–986. doi:10.3892/ijmm.2015.2080
Patel, D. C., Wilcox, K. S., and Metcalf, C. S. (2017). Novel targets for developing antiseizure and, potentially, antiepileptogenic drugs. Epilepsy Curr. 17, 293–298. doi:10.5698/1535-7597.17.5.293
Patke, A., Young, M. W., and Axelrod, S. (2020). Molecular mechanisms and physiological importance of circadian rhythms. Nat. Rev. Mol. Cell. Biol. 21, 67–84. doi:10.1038/s41580-019-0179-2
Peek, C. B., Levine, D. C., Cedernaes, J., Taguchi, A., Kobayashi, Y., Tsai, S. J., et al. (2017). Circadian clock interaction with HIF1α mediates oxygenic metabolism and anaerobic glycolysis in skeletal muscle. Cell. metab. 25, 86–92. doi:10.1016/j.cmet.2016.09.010
Peppard, P. E., Young, T., Barnet, J. H., Palta, M., Hagen, E. W., and Hla, K. M. (2013). Increased prevalence of sleep-disordered breathing in adults. Am. J. Epidemiol. 177, 1006–1014. doi:10.1093/aje/kws342
Pineda, E., Shin, D., You, S. J., Auvin, S., Sankar, R., and Mazarati, A. (2013). Maternal immune activation promotes hippocampal kindling epileptogenesis in mice. Ann. Neurol. 74, 11–19. doi:10.1002/ana.23898
Preston, K. J., Rom, I., Vrakas, C., Landesberg, G., Etwebi, Z., Muraoka, S., et al. (2019). Postprandial activation of leukocyte-endothelium interaction by fatty acids in the visceral adipose tissue microcirculation. FASEB J. official Publ. Fed. Am. Soc. Exp. Biol. 33, 11993–12007. doi:10.1096/fj.201802637RR
Probert, L., Akassoglou, K., Pasparakis, M., Kontogeorgos, G., and Kollias, G. (1995). Spontaneous inflammatory demyelinating disease in transgenic mice showing central nervous system-specific expression of tumor necrosis factor alpha. Proc. Natl. Acad. Sci. U. S. A. 92, 11294–11298. doi:10.1073/pnas.92.24.11294
Rahman, M. A., Islam, K., Rahman, S., and Alamin, M. (2021). Neurobiochemical cross-talk between COVID-19 and Alzheimer's disease. Mol. Neurobiol. 58, 1017–1023. doi:10.1007/s12035-020-02177-w
Rakhade, S. N., Klein, P. M., Huynh, T., Hilario-Gomez, C., Kosaras, B., Rotenberg, A., et al. (2011). Development of later life spontaneous seizures in a rodent model of hypoxia-induced neonatal seizures. Epilepsia 52, 753–765. doi:10.1111/j.1528-1167.2011.02992.x
Ramesh, V., Nair, D., Zhang, S. X., Hakim, F., Kaushal, N., Kayali, F., et al. (2012). Disrupted sleep without sleep curtailment induces sleepiness and cognitive dysfunction via the tumor necrosis factor-α pathway. J. neuroinflammation 9, 91. doi:10.1186/1742-2094-9-91
Ravenna, L., Principessa, L., Verdina, A., Salvatori, L., Russo, M. A., and Petrangeli, E. (2014). Distinct phenotypes of human prostate cancer cells associate with different adaptation to hypoxia and pro-inflammatory gene expression. PLoS One 9, e96250. doi:10.1371/journal.pone.0096250
Ravizza, T., and Vezzani, A. (2006). Status epilepticus induces time-dependent neuronal and astrocytic expression of interleukin-1 receptor type I in the rat limbic system. Neuroscience 137, 301–308. doi:10.1016/j.neuroscience.2005.07.063
Ravizza, T., and Vezzani, A. (2018). Pharmacological targeting of brain inflammation in epilepsy: therapeutic perspectives from experimental and clinical studies. Epilepsia Open 3, 133–142. doi:10.1002/epi4.12242
Reuter, S., Gupta, S. C., Chaturvedi, M. M., and Aggarwal, B. B. (2010). Oxidative stress, inflammation, and cancer: how are they linked? Free Radic. Biol. Med. 49, 1603–1616. doi:10.1016/j.freeradbiomed.2010.09.006
Riazi, K., Galic, M. A., and Pittman, Q. J. (2010). Contributions of peripheral inflammation to seizure susceptibility: cytokines and brain excitability. Epilepsy Res. 89, 34–42. doi:10.1016/j.eplepsyres.2009.09.004
Riney, K., Bogacz, A., Somerville, E., Hirsch, E., Nabbout, R., Scheffer, I. E., et al. (2022). International League against Epilepsy classification and definition of epilepsy syndromes with onset at a variable age: position statement by the ILAE Task Force on Nosology and Definitions. Epilepsia 63, 1443–1474. doi:10.1111/epi.17240
Rodgers, Z. B., Leinwand, S. E., Keenan, B. T., Kini, L. G., Schwab, R. J., and Wehrli, F. W. (2016). Cerebral metabolic rate of oxygen in obstructive sleep apnea at rest and in response to breath-hold challenge. J. Cereb. Blood Flow. Metab. 36, 755–767. doi:10.1177/0271678X15605855
Rosenzweig, I., Williams, S. C., and Morrell, M. J. (2014). The impact of sleep and hypoxia on the brain: potential mechanisms for the effects of obstructive sleep apnea. Curr. Opin. Pulm. Med. 20, 565–571. doi:10.1097/MCP.0000000000000099
Ruttanaumpawan, P., Logan, A. G., Floras, J. S., and Bradley, T. D.CANPAP Investigators (2009). Effect of continuous positive airway pressure on sleep structure in heart failure patients with central sleep apnea. Sleep 32, 91–98. doi:10.5665/sleep/32.1.91
Sakamoto, M., Matsumoto, R., Shimotake, A., Togawa, J., Takeyama, H., Kobayashi, K., et al. (2022). Diagnostic value of an algorithm for autoimmune epilepsy in a retrospective cohort. Front. Neurol. 13, 902157. doi:10.3389/fneur.2022.902157
Salazar-Montes, A., Ruiz-Corro, L., Sandoval-Rodriguez, A., Lopez-Reyes, A., and Armendariz-Borunda, J. (2006). Increased DNA binding activity of NF-kappaB, STAT-3, SMAD3 and AP-1 in acutely damaged liver. World J. Gastroenterol. 12, 5995–6001. doi:10.3748/wjg.v12.i37.5995
Sallmann, S., Jüttler, E., Prinz, S., Petersen, N., Knopf, U., Weiser, T., et al. (2000). Induction of interleukin-6 by depolarization of neurons. J. Neurosci. 20, 8637–8642. doi:10.1523/JNEUROSCI.20-23-08637.2000
Salman, L. A., Shulman, R., and Cohen, J. B. (2020). Obstructive sleep apnea, hypertension, and cardiovascular risk: epidemiology, pathophysiology, and management. Curr. Cardiol. Rep. 22, 6. doi:10.1007/s11886-020-1257-y
Sanz, P., and Garcia-Gimeno, M. A. (2020). Reactive glia inflammatory signaling pathways and epilepsy. Int. J. Mol. Sci. 21, 4096. doi:10.3390/ijms21114096
Sapin, E., Peyron, C., Roche, F., Gay, N., Carcenac, C., Savasta, M., et al. (2015). Chronic intermittent hypoxia induces chronic low-grade neuroinflammation in the dorsal Hippocampus of mice. Sleep 38, 1537–1546. doi:10.5665/sleep.5042
Sateia, M. J. (2014). International classification of sleep disorders-third edition: highlights and modifications. Chest 146, 1387–1394. doi:10.1378/chest.14-0970
Savin, C., Triesch, J., and Meyer-Hermann, M. (2009). Epileptogenesis due to glia-mediated synaptic scaling. J. R. Soc. Interface 6, 655–668. doi:10.1098/rsif.2008.0387
Schloss, M. J., Swirski, F. K., and Nahrendorf, M. (2020). Modifiable cardiovascular risk, hematopoiesis, and innate immunity. Circ. Res. 126, 1242–1259. doi:10.1161/CIRCRESAHA.120.315936
Schmeiser, B., Zentner, J., Prinz, M., Brandt, A., and Freiman, T. M. (2017). Extent of mossy fiber sprouting in patients with mesiotemporal lobe epilepsy correlates with neuronal cell loss and granule cell dispersion. Epilepsy Res. 129, 51–58. doi:10.1016/j.eplepsyres.2016.11.011
Schwab, R. J. (2003). Pro: sleep apnea is an anatomic disorder. Am. J. Respir. Crit. care Med. 168, 270–271. doi:10.1164/rccm.2305014
Semple, B. D., Dill, L. K., and O'Brien, T. J. (2020). Immune challenges and seizures: how do early life insults influence epileptogenesis? Front. Pharmacol. 11, 2. doi:10.3389/fphar.2020.00002
Shaftel, S. S., Griffin, W. S., and O'Banion, M. K. (2008). The role of interleukin-1 in neuroinflammation and Alzheimer disease: an evolving perspective. J. Neuroinflammation 5, 7. doi:10.1186/1742-2094-5-7
Shaheen, H. A., Abd El-Kader, A. A., El Gohary, A. M., El-Fayoumy, N. M., and Afifi, L. M. (2012). Obstructive sleep apnea in epilepsy: a preliminary Egyptian study. Sleep. Breath. 16, 765–771. doi:10.1007/s11325-011-0573-2
Shaki, D., Goldbart, A., Daniel, S., Fraser, D., and Shorer, Z. (2011). Pediatric epilepsy and parental sleep quality. J. Clin. Sleep. Med. 7, 502–506. doi:10.5664/JCSM.1318
Shandra, A. A., Godlevsky, L. S., Vastyanov, R. S., Oleinik, A. A., Konovalenko, V. L., Rapoport, E. N., et al. (2002). The role of TNF-alpha in amygdala kindled rats. Neurosci. Res. 42, 147–153. doi:10.1016/s0168-0102(01)00309-1
Shen, Y., Zhang, Q., Huang, Z., Zhu, J., Qiu, J., Ma, W., et al. (2020). Isoquercitrin delays denervated soleus muscle atrophy by inhibiting oxidative stress and inflammation. Front. physiology 11, 988. doi:10.3389/fphys.2020.00988
Shi, L. M., Chen, R. J., Zhang, H., Jiang, C. M., and Gong, J. (2017). Cerebrospinal fluid neuron specific enolase, interleukin-1β and erythropoietin concentrations in children after seizures. Childs Nerv. Syst. 33, 805–811. doi:10.1007/s00381-017-3359-4
Shih, R. H., Wang, C. Y., and Yang, C. M. (2015). NF-kappaB signaling pathways in neurological inflammation: a mini review. Front. Mol. Neurosci. 8, 77. doi:10.3389/fnmol.2015.00077
Soltani Khaboushan, A., Yazdanpanah, N., and Rezaei, N. (2022). Neuroinflammation and proinflammatory cytokines in epileptogenesis. Mol. Neurobiol. 59, 1724–1743. doi:10.1007/s12035-022-02725-6
Song, S., Tan, J., Miao, Y., Li, M., and Zhang, Q. (2017). Crosstalk of autophagy and apoptosis: involvement of the dual role of autophagy under ER stress. J. Cell. physiology 232, 2977–2984. doi:10.1002/jcp.25785
Sun, S. C. (2017). The non-canonical NF-κB pathway in immunity and inflammation. Nat. Rev. Immunol. 17, 545–558. doi:10.1038/nri.2017.52
Supriya, R., Tam, B. T., Pei, X. M., Lai, C. W., Chan, L. W., Yung, B. Y., et al. (2016). Doxorubicin induces inflammatory modulation and metabolic dysregulation in diabetic skeletal muscle. Front. physiology 7, 323. doi:10.3389/fphys.2016.00323
Tang, D., Kang, R., Berghe, T. V., Vandenabeele, P., and Kroemer, G. (2019). The molecular machinery of regulated cell death. Cell. Res. 29, 347–364. doi:10.1038/s41422-019-0164-5
Tereshchenko, A., Magnotta, V., Epping, E., Mathews, K., Espe-Pfeifer, P., Martin, E., et al. (2019). Brain structure in juvenile-onset Huntington disease. Neurology 92, e1939–e1947. doi:10.1212/WNL.0000000000007355
Terrone, G., Salamone, A., and Vezzani, A. (2017). Inflammation and epilepsy: preclinical findings and potential clinical translation. Curr. Pharm. Des. 23, 5569–5576. doi:10.2174/1381612823666170926113754
Thijs, R. D., Surges, R., O'Brien, T. J., and Sander, J. W. (2019). Epilepsy in adults. Lancet (London, Engl. 393, 689–701. doi:10.1016/S0140-6736(18)32596-0
Ting, E. Y., Yang, A. C., and Tsai, S. J. (2020). Role of interleukin-6 in depressive disorder. Int. J. Mol. Sci. 21, 2194. doi:10.3390/ijms21062194
Treiman, D. M. (2001). GABAergic mechanisms in epilepsy. Epilepsia 42 (Suppl. 3), 8–12. doi:10.1046/j.1528-1157.2001.042suppl.3008.x
Trosclair, K., Dhaibar, H. A., Gautier, N. M., Mishra, V., and Glasscock, E. (2020). Neuron-specific Kv1.1 deficiency is sufficient to cause epilepsy, premature death, and cardiorespiratory dysregulation. Neurobiol. Dis. 137, 104759. doi:10.1016/j.nbd.2020.104759
Tufik, S., Santos-Silva, R., Taddei, J. A., and Bittencourt, L. R. (2010). Obstructive sleep apnea syndrome in the sao paulo epidemiologic sleep study. Sleep. Med. 11, 441–446. doi:10.1016/j.sleep.2009.10.005
Üner, A. K., Okan, A., Akyüz, E., Köklü, B., Eroğlu, E., Yilmaz, S., et al. (2023). Tauroursodeoxycholic acid (TUDCA) regulates inflammation and hypoxia in autonomic tissues of rats with seizures. Cell. Mol. Biol. (Noisy-le-grand) 68, 104–111. doi:10.14715/cmb/2022.68.12.19
Valli, G., Barbieri, S., Sergi, P., Fayoumi, Z., and Berardinelli, P. (1984). Evidence of motor neuron involvement in chronic respiratory insufficiency. J. Neurol. Neurosurg. Psychiatry 47, 1117–1121. doi:10.1136/jnnp.47.10.1117
van der Pol, A., van Gilst, W. H., Voors, A. A., and van der Meer, P. (2019). Treating oxidative stress in heart failure: past, present and future. Eur. J. heart Fail. 21, 425–435. doi:10.1002/ejhf.1320
van Golde, E. G., Gutter, T., and de Weerd, A. W. (2011). Sleep disturbances in people with epilepsy; prevalence, impact and treatment. Sleep. Med. Rev. 15, 357–368. doi:10.1016/j.smrv.2011.01.002
Vatansever, E., Surmen-Gur, E., Ursavas, A., and Karadag, M. (2011). Obstructive sleep apnea causes oxidative damage to plasma lipids and proteins and decreases adiponectin levels. Sleep Breath. = Schlaf Atmung 15, 275–282. doi:10.1007/s11325-010-0378-8
Vezzani, A. (2015). Anti-inflammatory drugs in epilepsy: does it impact epileptogenesis? Expert Opin. Drug Saf. 14, 583–592. doi:10.1517/14740338.2015.1010508
Vezzani, A., and Baram, T. Z. (2007). New roles for interleukin-1 Beta in the mechanisms of epilepsy. Epilepsy Curr. 7, 45–50. doi:10.1111/j.1535-7511.2007.00165.x
Vezzani, A., French, J., Bartfai, T., and Baram, T. Z. (2011b). The role of inflammation in epilepsy. Nat. Rev. Neurol. 7, 31–40. doi:10.1038/nrneurol.2010.178
Vezzani, A., and Granata, T. (2005). Brain inflammation in epilepsy: experimental and clinical evidence. Epilepsia 46, 1724–1743. doi:10.1111/j.1528-1167.2005.00298.x
Vezzani, A., Maroso, M., Balosso, S., Sanchez, M. A., and Bartfai, T. (2011a). IL-1 receptor/Toll-like receptor signaling in infection, inflammation, stress and neurodegeneration couples hyperexcitability and seizures. Brain Behav. Immun. 25, 1281–1289. doi:10.1016/j.bbi.2011.03.018
Vezzani, A., Moneta, D., Richichi, C., Aliprandi, M., Burrows, S. J., Ravizza, T., et al. (2002). Functional role of inflammatory cytokines and antiinflammatory molecules in seizures and epileptogenesis. Epilepsia 43 (Suppl. 5), 30–35. doi:10.1046/j.1528-1157.43.s.5.14.x
Villasana-Salazar, B., and Vezzani, A. (2023). Neuroinflammation microenvironment sharpens seizure circuit. Neurobiol. Dis. 178, 106027. doi:10.1016/j.nbd.2023.106027
Virk, J. S., and Kotecha, B. (2016). Otorhinolaryngological aspects of sleep-related breathing disorders. J. Thorac. Dis. 8, 213–223. doi:10.3978/j.issn.2072-1439.2016.01.39
Viviani, B., Bartesaghi, S., Gardoni, F., Vezzani, A., Behrens, M. M., Bartfai, T., et al. (2003). Interleukin-1beta enhances NMDA receptor-mediated intracellular calcium increase through activation of the Src family of kinases. J. Neurosci. 23, 8692–8700. doi:10.1523/JNEUROSCI.23-25-08692.2003
Wais, M., Wu, C., Zahid, T., Sheppy, E., Gillis, J., Del Campo, M., et al. (2009). Repeated hypoxic episodes induce seizures and alter hippocampal network activities in mice. Neuroscience 161, 599–613. doi:10.1016/j.neuroscience.2009.03.036
Wall, A. M., Mukandala, G., Greig, N. H., and O'Connor, J. J. (2015). Tumor necrosis factor-α potentiates long-term potentiation in the rat dentate gyrus after acute hypoxia. J. Neurosci. Res. 93, 815–829. doi:10.1002/jnr.23540
Wang, J., Zhang, J., Chen, L., Cai, J., Li, Z., Zhang, Z., et al. (2019). Combination of broccoli sprout extract and zinc provides better protection against intermittent hypoxia-induced cardiomyopathy than monotherapy in mice. Oxidative Med. Cell. Longev. 2019, 2985901. doi:10.1155/2019/2985901
Wang, L., and Greenfield, L. J. (2009). Post-hypoxic changes in rat cortical neuron GABA A receptor function require L-type voltage-gated calcium channel activation. Neuropharmacology 56, 198–207. doi:10.1016/j.neuropharm.2008.07.004
Wang, L., Zeng, X., Ryoo, H. D., and Jasper, H. (2014). Integration of UPRER and oxidative stress signaling in the control of intestinal stem cell proliferation. PLoS Genet. 10, e1004568. doi:10.1371/journal.pgen.1004568
Wang, Y., Alam, G. N., Ning, Y., Visioli, F., Dong, Z., Nör, J. E., et al. (2012). The unfolded protein response induces the angiogenic switch in human tumor cells through the PERK/ATF4 pathway. Cancer Res. 72, 5396–5406. doi:10.1158/0008-5472.CAN-12-0474
Wang, Y., Gu, Y. H., Liu, M., Bai, Y., Liang, L. Y., and Wang, H. L. (2017). TBHQ alleviated endoplasmic reticulum stress-apoptosis and oxidative stress by PERK-nrf2 crosstalk in methamphetamine-induced chronic pulmonary toxicity. Oxidative Med. Cell. Longev. 2017, 4310475. doi:10.1155/2017/4310475
Wang, Z., Ying, Z., Bosy-Westphal, A., Zhang, J., Schautz, B., Later, W., et al. (2010). Specific metabolic rates of major organs and tissues across adulthood: evaluation by mechanistic model of resting energy expenditure. Am. J. Clin. Nutr. 92, 1369–1377. doi:10.3945/ajcn.2010.29885
Wei, F., Yan, L. M., Su, T., He, N., Lin, Z. J., Wang, J., et al. (2017). Ion Channel genes and epilepsy: functional alteration, pathogenic potential, and mechanism of epilepsy. Neurosci. Bull. 33, 455–477. doi:10.1007/s12264-017-0134-1
Wei, H., Alberts, I., and Li, X. (2013). Brain IL-6 and autism. Neuroscience 252, 320–325. doi:10.1016/j.neuroscience.2013.08.025
Weinberg, M. S., Blake, B. L., and McCown, T. J. (2013). Opposing actions of hippocampus TNFα receptors on limbic seizure susceptibility. Exp. Neurol. 247, 429–437. doi:10.1016/j.expneurol.2013.01.011
Wheless, J. W., Clarke, D. F., Arzimanoglou, A., and Carpenter, D. (2007). Treatment of pediatric epilepsy: European expert opinion, 2007. Epileptic Disord. 9, 353–412. doi:10.1684/epd.2007.0144
Wirrell, E., Farrell, K., and Whiting, S. (2005). The epileptic encephalopathies of infancy and childhood. Le J. Can. des Sci. neurologiques 32, 409–418. doi:10.1017/s0317167100004388
Xanthopoulos, M. S., Gallagher, P. R., Berkowitz, R. I., Radcliffe, J., Bradford, R., and Marcus, C. L. (2015). Neurobehavioral functioning in adolescents with and without obesity and obstructive sleep apnea. Sleep 38, 401–410. doi:10.5665/sleep.4498
Xin, Y., Li, S., Liu, H., and Liu, B. (2022). Periostin and TNF-α expression levels in peripheral blood of patients with acute cerebral infarction combined with obstructive sleep apnea syndrome and their predictive value for clinical prognosis. BMC Neurol. 22, 358. doi:10.1186/s12883-022-02885-x
Xing, T., Pilowsky, P. M., and Fong, A. Y. (2014). Mechanism of sympathetic activation and blood pressure elevation in humans and animals following acute intermittent hypoxia. Prog. Brain Res. 209, 131–146. doi:10.1016/B978-0-444-63274-6.00007-2
Xu, H., Zheng, X., Qian, Y., Guan, J., Yi, H., Zou, J., et al. (2016). Metabolomics profiling for obstructive sleep apnea and simple snorers. Sci. Rep. 6, 30958. doi:10.1038/srep30958
Xu, L., Pathak, P. S., and Fukumura, D. (2004). Hypoxia-induced activation of p38 mitogen-activated protein kinase and phosphatidylinositol 3'-kinase signaling pathways contributes to expression of interleukin 8 in human ovarian carcinoma cells. Clin. Cancer Res. 10, 701–707. doi:10.1158/1078-0432.ccr-0953-03
Yamamoto, A., Murphy, N., Schindler, C. K., So, N. K., Stohr, S., Taki, W., et al. (2006). Endoplasmic reticulum stress and apoptosis signaling in human temporal lobe epilepsy. J. neuropathology Exp. neurology 65, 217–225. doi:10.1097/01.jnen.0000202886.22082.2a
Yoshida, H. (2007). ER stress and diseases. FEBS J. 274, 630–658. doi:10.1111/j.1742-4658.2007.05639.x
Yu, N., Liu, H., and Di, Q. (2013). Modulation of immunity and the inflammatory response: a new target for treating drug-resistant epilepsy. Curr. Neuropharmacol. 11, 114–127. doi:10.2174/157015913804999540
Zhang, J., Han, Y., Zhao, Y., Li, Q., Jin, H., and Qin, J. (2019a). Inhibition of TRIB3 protects against neurotoxic injury induced by kainic acid in rats. Front. Pharmacol. 10, 585. doi:10.3389/fphar.2019.00585
Zhang, J., and Veasey, S. (2012). Making sense of oxidative stress in obstructive sleep apnea: mediator or distracter? Front. neurology 3, 179. doi:10.3389/fneur.2012.00179
Zhang, J. Q., Wang, X. W., Chen, J. F., Bai, X. X., Wang, J., Gao, B. W., et al. (2019b). Grape seed procyanidin B2 protects porcine ovarian granulosa cells against oxidative stress-induced apoptosis by upregulating let-7a expression. Oxidative Med. Cell. Longev. 2019, 1076512. doi:10.1155/2019/1076512
Zhang, K., and Kaufman, R. J. (2008). From endoplasmic-reticulum stress to the inflammatory response. Nature 454, 455–462. doi:10.1038/nature07203
Zhang, W., Han, B., Zhang, H., Fu, R., Lu, Y., and Zhang, G. (2023). Integrated transcriptomic and metabolomic analysis of cortical neurons reveals dysregulated lipid metabolism, enhanced glycolysis and activated HIF-1 signaling pathways in acute hypoxia. Heliyon 9, e14949. doi:10.1016/j.heliyon.2023.e14949
Zhang, Y., Yang, Y., Zhang, G. Z., Gao, M., Ge, G. Z., Wang, Q. Q., et al. (2016). Stereotactic administration of edaravone ameliorates collagenase-induced intracerebral hemorrhage in rat. CNS Neurosci. Ther. 22, 824–835. doi:10.1111/cns.12584
Zhao, H., Zhu, C., and Huang, D. (2018). Microglial activation: an important process in the onset of epilepsy. Am. J. Transl. Res. 10, 2877–2889.
Zhu, G., Okada, M., Yoshida, S., Mori, F., Ueno, S., Wakabayashi, K., et al. (2006). Effects of interleukin-1beta on hippocampal glutamate and GABA releases associated with Ca2+-induced Ca2+ releasing systems. Epilepsy Res. 71, 107–116. doi:10.1016/j.eplepsyres.2006.05.017
Zhu, H., Hu, S., Li, Y., Sun, Y., Xiong, X., Hu, X., et al. (2022). Interleukins and ischemic stroke. Front. Immunol. 13, 828447. doi:10.3389/fimmu.2022.828447
Keywords: OSAS, epilepsy, intermittent hypoxia, inflammation, ER stress
Citation: Ma Y and Wu Q (2023) Intermittent hypoxia: linkage between OSAS and epilepsy. Front. Pharmacol. 14:1230313. doi: 10.3389/fphar.2023.1230313
Received: 28 May 2023; Accepted: 08 November 2023;
Published: 23 November 2023.
Edited by:
Frederic Roche, Université Jean Monnet, FranceReviewed by:
Benjamín Villasana-Salazar, Mario Negri Institute for Pharmacological Research (IRCCS), ItalyFlorian Chouchou, Université de la Réunion, France
Copyright © 2023 Ma and Wu. This is an open-access article distributed under the terms of the Creative Commons Attribution License (CC BY). The use, distribution or reproduction in other forums is permitted, provided the original author(s) and the copyright owner(s) are credited and that the original publication in this journal is cited, in accordance with accepted academic practice. No use, distribution or reproduction is permitted which does not comply with these terms.
*Correspondence: Qiong Wu, d3VxaW9uZ19jbXVAMTYzLmNvbQ==