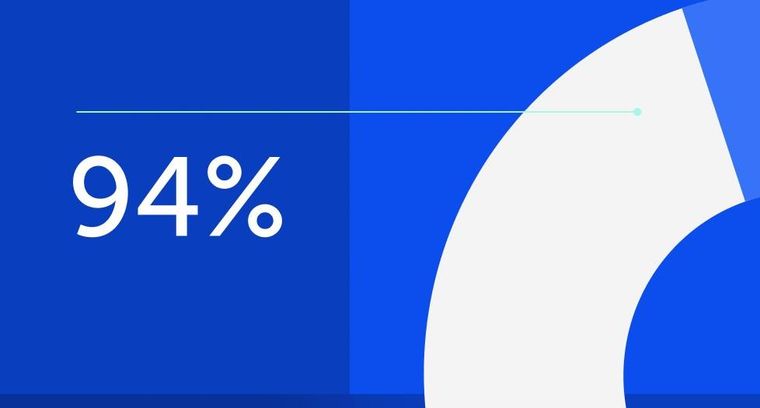
94% of researchers rate our articles as excellent or good
Learn more about the work of our research integrity team to safeguard the quality of each article we publish.
Find out more
MINI REVIEW article
Front. Pharmacol., 13 July 2023
Sec. Neuropharmacology
Volume 14 - 2023 | https://doi.org/10.3389/fphar.2023.1222158
G protein-coupled receptors (GPCRs) are the target of hundreds of approved drugs. Although these drugs were designed to target individual receptors, it is becoming increasingly apparent that GPCRs interact with each other to form heteromers. Approved drug targets are often part of a GPCR heteromer, and therefore new drugs can be developed with heteromers in mind. This review presents several strategies to selectively target GPCRs in heteromeric contexts, namely, taking advantage of i) heteromer-mediated biased agonism/signalling, ii) discovery of drugs with higher affinity for the receptor if it is part of a heteromer (heteromer selective drugs), iii) allosteric compounds directed against the interacting transmembrane domains and, eventually, iv) antagonists that block both GPCRs in a heteromer. Heteromers provide unique allosteric sites that should help designing a new type of drug that by definition would be a heteromer selective drug. The review also provides examples of rhodopsin-like class A receptors in heteromers that could be targeted to neuroprotect and/or delay the progression of diseases such as Parkinson’s and Alzheimer’s. GPCRs in heteromers (GriH) with the potential to address dyskinesias, a common complication of dopaminergic replacement therapy in parkinsonian patients, are also described.
G protein-coupled receptors (GPCRs) are the target of 30%–40% of approved drugs. Early functionality studies related to neurotransmission/neuromodulation led to the hypothesis of receptor-receptor interactions (Agnati et al., 1982). Among the major GPCR families (Alexander et al., 2021), taste receptors form macromolecular complexes made up of at least two different receptors (Nelson et al., 2002). Class C GPCRs, such as metabotropic glutamate receptors do form dimers, both homo- and heterodimers. Dimer formation in class C GPCRs has been mainly addressed by studying the interactions established by large extracellular N-terminal domains. The first crystal structure of two interacting extracellular N-terminal domains of a class C receptor was reported in 2000 (Kunishima et al., 2000). Pharmacological studies performed in parallel to structural determination suggested that class C GPCR heteromerization “is required to obtain a functional dimer as one of the subunits contains the endogenous ligand binding site, whilst the other mediates signal transduction heteromerization” (Kniazeff et al., 2011). This view opened the question of whether GPCR/G protein stoichiometry was 1:1 or 2:1 (Cordomí et al., 2015).
With few exceptions, rhodopsin-like class A GPCRs do not have the huge N-terminal extracellular domains of class C GPCRs; hence, they form dimers through transmembrane and/or C-terminal domains (Stenkamp, 2018).
The first experimental evidence for class A heterodimer formation was provided for δ/κ opioid receptors (Jordan and Devi, 1999), and adenosine A1/dopamine D1 receptors (Gines et al., 2000). Heterodimerization of class A receptors has been questioned despite huge biochemical, pharmacological and biophysical evidence (Franco et al., 2016). The recent resolution of the structure of the homodimer of apelin receptor (Yue et al., 2022) has both confirmed that class A receptors may interact and that structure is important for signalling via the G proteins.
Adrenoceptors are the targets of several medicines. Are these medicines targeting monomeric adrenoceptors? It is reasonable to speculate that these medicines are targeting (cell surface) adrenoceptors that are expressed as monomers, as homodimers and as heteromers. For example, betaxolol is a selective antagonist of ß1 adrenoceptors, which can be expressed on the cell surface as homodimers or as complexes (heteromers) with ß2 adrenoceptors (Zhu et al., 2005).
A nice example of functional GPCR-containing macromolecular complex is the heterotetramer formed by two adenosine A2A receptors and two adenosine A1 receptors coupled to one Gs and one Gi protein (Navarro et al., 2016; 2018b); the stoichiometry is two GPCRs:one G protein. There may be exceptions as deduced from the structure of a class D fungal receptor, Ste2, that reveals a homodimer coupled to two G proteins (Velazhahan et al., 2021). The structure and the stoichiometry of GPCR-G protein in oligomeric complexes is important for function (Yue et al., 2022). While it would be equivalent to target monomers or homomers, targeting heteromers makes possible to specifically target those cells that express them. On the one hand, targeting only cells expressing a given heteromer would reduce unwanted side effects and probably increase drug delivery options. On the other hand, targeting the GPCRs in heteromers (GRiH) of heteromers themselves can be achieved by quite different approaches, thus raising new possibilities for drug development.
Once a given heteromer is formed and appears on the cell surface, drug discovery must consider the properties of GriHs. The following properties should be considered: i) altered pharmacology, ii) cross-antagonism, iii) “altered” coupling to G proteins and iv) “altered” signaling.
At first it was assumed that the interaction of receptor A with receptor B could affect, even in the absence of a ligand of A, the ligand binding properties of B, or vice versa. There are allosteric modulations occurring when a heteromer is formed and, accordingly, the ligand binding affinity is, by definition, different between monomers/homodimers and heteromers (even for the same receptor in different heteromeric contexts). By the same token potency may be affected; the activation of a given pathway can even by blunted by heteromerization. After demonstrating that adenosine A2A and A3 receptors do interact, we noticed that the Gi-mediated signalling in response to A3 agonists does not occur unless the A2A is blocked by an antagonists (Lillo et al., 2020).
The occurrence of heteromers in natural cells/environments can be identified by an imprint. The cross-antagonism imprint consists of blocking the function of a receptor by using an antagonist targeting the partner receptor in the heteromer. In other words, cross-antagonism for the AB heteromer, means that B function is blocked by the antagonist of A. The imprint occurring when cannabinoid CB1 and GPR55 interact consists of the blockade by a selective CB1 antagonist of the link of GPR55 to the mitogen-activated protein kinase signaling pathway. This imprint was observed in co-transfected cells and in striatal rat brain slices; further confirmation of the expression of the heteromer in striatal neurons was provided by an imaging technique using sections from Macaca fascicularis primates (Martínez-Pinilla et al., 2014).
Another possibility is differential G protein coupling. This property was described for dopamine D1-D2 receptor heteromers. While the D1 couples to Gs and the D2 couples to Gi, the D1-D2 receptor heteromer couples to Gq and signals via Ca2+ instead of via cAMP (Rashid et al., 2007; Hasbi et al., 2009; Perreault et al., 2010; Perreault et al., 2015; Verma et al., 2010). Dopamine D2 and cannabinoid CB1 receptors when expressed individually couple to Gi, but when they interact, the heteromer couples to Gs (Faron-Górecka et al., 2019). Co-activation of receptors leads to an increase in cytosolic cAMP levels, i.e., a Gs-mediated effect; in cells where these heteromers are expressed, cannabinoids or D2 agonists increase rather than decrease cAMP levels (Caballero-Florán et al., 2016; Yu et al., 2021). Activation of a receptor in a heteromer may also affect regulation of ion fluxes and/or activation of the mitogen-activated protein kinase (MAPK) signaling pathway in a different way than if the receptor is expressed as a monomer/homodimer.
A type of functional selectivity known as biased signalling is also affected by the heteromeric context in which the GRiH is expressed. One of the advantages of targeting heteromers is that a given receptor may interact with several other receptors. An online tool allows finding the GPCR heteromers that have been reported to date (Borroto-Escuela et al., 2014). Accordingly, heteromeric-targeted drug discovery programs could take advantage of the possibility that there are compounds that have a higher affinity for binding to GRiH and/or that provide the most desirable signaling bias for therapeutic success. For instance, coactivation in a heteromer context of both cannabinoid CB2 and orexin OX1 receptors, for instance by dual drugs, results in a negative crosstalk at the level of the MAPK signaling pathway, favouring the G protein-dependent signalling (Raïch et al., 2022).
Heteromer selective drugs are those that only interact with the structures arising from the interaction. As shown in Figure 1, a drug with a specific structure that cannot bind with high affinity to A or B receptors, could bind with high affinity to the AB heteromer. The design of selective heteromeric drugs with the potential to allosterically affect the binding of agonists to orthosteric centers or agonist potency is an attractive approach in future drug discovery.
FIGURE 1. Heteromer-selective compounds. Panel (A). The heteromer selective compound (orange) interacts with low affinity with the two GPCRs (blue and red). Panel (B). The compound (orange) interacts with high affinity with the blue/red receptor heteromer and may lead to conformational changes.
Bivalent ligands that interact with two receptors in a heterodimer have been instrumental in detecting heteromers in the brain (Soriano et al., 2009; Kuhhorn et al., 2011). They are high-affinity probes with therapeutic potential, but they likely won't be developed to combat CNS diseases because chances of degradation before entering the brain. An alternative is the use of interfering peptides. A peptide containing both part of the sequence of an HIV-1 protein, transactivator of transcription (TAT), and the sequence of a GPCR transmembrane domain can be inserted into the plasma membrane. This type of peptide was already used in 2011 to demonstrate that disruption of the µ and ∂ opioid receptor heteromer affects analgesic effects of µ opioid receptor agonists (He et al., 2011). The question is whether these peptides able to modify the functionality of GRiH will be proposed and approved to enter clinical trials to assess efficacy in neurological diseases. A recent article provides clues on how to target transmembrane domains of GRiHs to obtain successful therapeutic drugs (Cai et al., 2023).
Considering the information presented above, altering the function of a given GRiH to meet a specific therapeutic requirement can be accomplished in at least four ways:
- Taking advantage of heteromer-mediated biased agonism/signalling (see previous section).
- Discovering heteromer selective drugs, i.e., drugs that only have high affinity for a GRiH.
There are few reported heteromer selective drugs and such paucity is mainly because selection is made for “monomers” rather than using heteromers. Early on, the laboratory of Susan George reported a selective agonist of a GRiH of the two forming the dopamine D1-D2 receptor heteromer (Verma et al., 2010). Subsequently, we reported A2A receptor (A2AR) antagonists with differential binding/potency when tested on presynaptic A2AR heteromers in comparison with postsynaptic A2AR heteromers (Orru et al., 2011). Accordingly, it is suggested that drug screening be performed using the actual target, which in the case of GCPRs, would often be a GRiH.
- Altering the interaction interfaces in such a way that the GRiH function is altered to provide therapeutic benefits (see next section)
- Taking advantage of the cross-antagonism heteromer imprint (see next section)
A detailed description of all heteromers with potential in neurodegeneration is beyond the scope of this article; we have selected examples that illustrate both the therapeutic potential and the underlying mechanisms.
In what concerns hypoxia/ischemia/stroke, heteromers containing adenosine or serotonin receptors are involved in neuroprotective mechanisms. Preclinical experiments carried out years ago suggest the benefits of cannabidiol (CBD) in neonatal hypoxia (Castillo et al., 2010; Lafuente et al., 2011; Pazos et al., 2012). The mode of action is, at least in part, mediated by serotonin-HT1A/cannabinoid CB2 receptor heteromers, whose expression increases in a model of hypoxic-ischemic brain damage (Pazos et al., 2013; Franco et al., 2019). CBD is an allosteric modulator of the CB2 receptor that affects biased agonism depending on whether the receptor is expressed alone or it interacts with the most abundant cannabinoid receptor in the central nervous system (CNS), the CB1 (Martínez-Pinilla et al., 2017; Navarro et al., 2018c). In scenarios of increased expression of the CB2 receptor, for example, under neurodegenerative and/or ischemic conditions, CBD treatment attenuates brain damage (Campos et al., 2016). CBD also has beneficial effects on attenuating psychotic-, anxiety- and depressive behaviors. In summary, CBD itself or the derivatives recently reported (Navarro et al., 2021) have neuroprotective potential via cannabinoid GRiHs.
Drug addiction is one cause of neuronal cell death and attempts to minimize neurodegeneration have focused on GPCRs for neurotransmitters and neuromodulators expressed in reward circuits in the brain. Interestingly, in vitro disruption of a heteromer formed by two dopamine receptors, D1 and D2, promotes, increases, and accelerates locomotor activity, also enhancing the motivational effects induced by cocaine (Perreault et al., 2016). Consequently, a heteromer-selective agonist acting on dopamine GRiHs attenuates the action of the drug of abuse and the reinstatement of seeking behaviour (Hasbi et al., 2018). Thought to be segregated in different neuronal types, the D1 and the D2 receptors are coexpressed in a population of GABAergic neurons where they form heteromers (Rico et al., 2016); it is likely that the benefit on preventing cocaine noxious effects of activating dopamine receptors in the D1-D2 heteromer context is explained by key neuronal events that involve key proteins in dopaminergic neurotransmission, among others, extracellular signal-regulated kinase (ERK), ΔFosB and 32 kDa dopamine- and cAMP-regulated phosphoprotein (DARPP-32) (Hasbi et al., 2018). Another of the 5 existing dopamine receptor types, the D3, can form dimers with D1, D2 and other class A GPCRs. Apart from neuroprotection itself, D3 GRiHs are promising to manage dyskinesia, which is a common side effect of levodopa medication in Parkinson’s disease (PD) and that, at present, can only be treated by deep brain stimulation approaches (Scarselli et al., 2001; Fiorentini et al., 2003; Fiorentini et al., 2008b; Fiorentini et al., 2008a; Fiorentini et al., 2008c; Fiorentini et al., 2010; Fiorentini et al., 2013; Torvinen et al., 2005; Marcellino et al., 2008; Maggio et al., 2010; Maggio and Millan, 2010; Mutti et al., 2020).
Further examples of dopamine heteromers with potential in neuroprotection are those formed with adenosine receptors (ARs). These were among the first heteromers identified for receptors of two different endogenous agonists. In the basal ganglia neurons, the adenosine A1 interacts with the D1 receptor and the adenosine A2AR interacts with the D2 receptor. The therapeutic potential of targeting ARs in PD, disclosed several years ago, was based on the adenosine-dopamine antagonism at the CNS level. The lack of dopamine production in the disease would be partially reverted by using AR antagonists; AR blockade would potentiate the effect of dopamine produced by patients and/or the dopamine-replacement medication. After several attempts by different pharmaceutical companies and several clinical trials, a first in class AR antagonist, istradefylline, has been approved for human use (Jenner et al., 2009; Saki et al., 2013; Jenner, 2014; Mori et al., 2022) (marketed as Nouriast® in Japan and as Nourianz® in US; 20 mg tablets). Istradefylline targets the receptor in the striatum where it interacts with the dopamine D2 receptor and with other GPCRs. Istradefylline has been approved as adjuvant therapy in combination with levodopa, i.e., it has not been approved as a neuroprotective drug. Then the second relevant possibility is that this drug may have neuroprotective, i.e., disease-modifying, potential. On the one hand, the FDA does not have useful biomarkers for assaying neuroprotective potential. On the other hand, longitudinal studies that compare the progression of the disease in patients who take the drug versus those who do not (it is not approved in Europe), can confirm whether the neuroprotective potential shown by A2AR antagonists in vitro (Dall’lgna et al., 2003; Agnati et al., 2004; Armentero et al., 2011; Jenner, 2014) also occurs in patients with neurodegenerative diseases.
Much of the research aimed at providing neuroprotection in neurodegenerative diseases focuses on neurons even though microglia play a key role in neurodegenerative diseases, such as Parkinson’s (PD), Alzheimer’s (AD) and Huntington’s, which have a neuroinflammatory component. About two decades ago we reported that activation of the A2AR increases the nitric oxide release by activated primary microglia (Saura et al., 2005) and we discovered that the A2AR was upregulated in the microglia surrounding the plaques found in post-mortem brain samples from patients with AD (Angulo et al., 2003). We became interested since then in literature showing how pharmacological manipulation of A2A would affect the proinflammatory/neuroprotective balance of microglia. Strong evidence comes from the detection of a limitation of the proinflammatory action of lipopolysaccharide after intracerebroventricular administration of a highly selective A2AR antagonist, SCH-58261 (Rebola et al., 2011). In a rodent model of PD, administration of A2AR antagonists reverses neuroinflammation accompanying 1-methyl-4-phenyl-1,2,3,6-tetrahydropyridine injury (Frau et al., 2011). Equivalent results, i.e., A2AR antagonists reversing the microglial inflammatory response, have been reported in another rodent model of striatal neurodegeneration (Minghetti et al., 2007). Based on these and other complementary results, whose description) is beyond the scope of this article, we recently noted that evidence for the neuroprotective potential of A2AR antagonists needs to be addressed both in microglia and in terms of targeting A2AR-containing heteromers. Another AR that is present in the microglia is the A3, which in the presence of the A2A cannot signal through G proteins. These adenosine GRiHs expressed in the same cell are potential targets to prevent neuroinflammation. An interesting finding related to the A2A-A3 heteromer is that it is upregulated in primary microglia of the APPSw,Ind transgenic model of AD. The receptor heteromer functionality in primary microglia from the transgenic and from the control animals was similar (Lillo et al., 2022). Since the animal model does not present cognitive alterations until an advanced age, there must be protection that lasts for months until the system becomes unbalanced. Are microglia involved in brain circuit protection despite the expression of a noxious mutated amyloid precursor protein? Could activation or blockade of GRiHs in microglia protect neurons from severe impairment?
Another homotropic heteromer, formed by adenosine A1 and A2A receptors seems to be involved in the in vitro neuroprotective potential of an endogenous nucleoside, guanosine (Massari et al., 2021). The in vitro benefits of guanosine, consists of reducing both mitochondrial alterations and oxidative stress. It would be relevant to know how guanosine exerts its effects to address the possibility of design novel ligands targeting adenosine GRiH. It is relevant to i) select the right heteromer and the right GRiH(s) to be targeted to obtain a given therapeutic outcome and ii) be sure that the heteromer is expressed in the cells that must be targeted in the intended therapeutic benefit.
Cannabinoid CB1 and CB2 receptors forming CB1-CB2 heteromers in microglia have potential as targets for AD therapy. They are upregulated in both activated microglia and in the microglia isolated from the hippocampus from a rodent model of the disease (Navarro et al., 2018a). Other receptors that are targeted by phytocannabinoids although they are not considered as cannabinoid receptors, GPR18 and GPR55, can form heteromers with cannabinoid receptors. Their expression in brain cells and in animal models of neurodegenerative diseases indicate that those GRiH must be considered in disease-modifying neuroprotective approaches to combat PD and/or AD (Reyes-Resina et al., 2018; Martínez-Pinilla et al., 2019; Pérez-Olives et al., 2021).
The bradykinin B2 receptor and the κ opioid receptor, which are co-expressed in several brain regions, can form heteromers whose potential as a neuroprotective target stems from the fact that the major endogenous κ-opioid receptor agonist, dynorphin, is involved in the regulation of neuronal fate (Ji et al., 2017). Finally, the 2 types of angiotensin II receptors, AT1 and AT2, are expressed in various brain regions and are of special interest in relation to the pathophysiology of Parkinson’s disease. The two receptors can form heteromers that are upregulated in the unilateral 6-hydroxydopamine lesioned Parkinson’s disease model. Boosting the neuroprotective potential of AT2 receptors may be more successful when heteromers and GRiHs are considered (Rivas-Santisteban et al., 2020).
All authors listed have made a substantial, direct, and intellectual contribution to the work and approved it for publication.
This work was supported by grants PID 2020-113430RB-I00 and PID 2021-126600OB-I00 funded by Spanish MCIN/AEI/10.13039/501100011033 and, as appropriate, by “ERDF A way of making Europe,” by the “European Union” or by the “European Union Next- Generation EU/PRTR.” The research group of the University of Barcelona is considered of excellence (grup consolidat #2021 SGR 00304) by the Regional Catalonian Government.
The authors declare that the research was conducted in the absence of any commercial or financial relationships that could be construed as a potential conflict of interest.
All claims expressed in this article are solely those of the authors and do not necessarily represent those of their affiliated organizations, or those of the publisher, the editors and the reviewers. Any product that may be evaluated in this article, or claim that may be made by its manufacturer, is not guaranteed or endorsed by the publisher.
AR, Adenosine receptor; CBD, Cannabidiol; CNS, central nervous system; GPCR, G protein-coupled receptor; GRiH, GPCRs in heteromers; TAT, transactivator of transcription (TAT); TM, transmembrane domain.
Agnati, L. F., Fuxe, K., Zoli, M., Rondanini, C., and Ogren, S. O. (1982). New vistas on synaptic plasticity: The receptor mosaic hypothesis of the engram. Med. Biol. 60, 183–190.
Agnati, L. F. L. F., Leo, G., Vergoni, A.-V. V. A.-V., Martínez, E., Hockemeyer, J., Lluis, C., et al. (2004). Neuroprotective effect of L-DOPA co-administered with the adenosine A2A receptor agonist CGS 21680 in an animal model of Parkinson’s disease. Brain Res. Bull. 64, 155–164. doi:10.1016/j.brainresbull.2004.06.003
Alexander, S. P., Christopoulos, A., Davenport, A. P., Kelly, E., Mathie, A., Peters, J. A., et al. (2021). The concise guide to pharmacology 2021/22: G protein-coupled receptors. Br. J. Pharmacol. 178, S27–S156. doi:10.1111/BPH.15538
Angulo, E., Casadó, V., Mallol, J., Canela, E. I., Viñals, F., Ferrer, I., et al. (2003). A1 adenosine receptors accumulate in neurodegenerative structures in Alzheimer disease and mediate both amyloid precursor protein processing and tau phosphorylation and translocation. Brain Pathol. 13, 440–451. doi:10.1111/j.1750-3639.2003.tb00475.x
Armentero, M. T., Pinna, A., Ferré, S., Lanciego, J. L., Müller, C. E., and Franco, R. (2011). Past, present and future of A2A adenosine receptor antagonists in the therapy of Parkinson’s disease. Pharmacol. Ther. 132, 280–299. doi:10.1016/j.pharmthera.2011.07.004
Borroto-Escuela, D. O., Brito, I., Romero-Fernandez, W., Di Palma, M., Oflijan, J., Skieterska, K., et al. (2014). The G protein-coupled receptor heterodimer network (GPCR-HetNet) and its hub components. Int. J. Mol. Sci. 15, 8570–8590. doi:10.3390/ijms15058570
Caballero-Florán, R. N., Conde-Rojas, I., Oviedo Chávez, A., Cortes-Calleja, H., Lopez-Santiago, L. F., Isom, L. L., et al. (2016). Cannabinoid-induced depression of synaptic transmission is switched to stimulation when dopaminergic tone is increased in the globus pallidus of the rodent. Neuropharmacology 110, 407–418. doi:10.1016/J.NEUROPHARM.2016.08.002
Cai, X., Wang, D., Zhang, R., Chen, Y., and Chen, J. (2023). The transmembrane domains of GPCR dimers as targets for drug development. Drug Discov. Today 28, 103419. doi:10.1016/J.DRUDIS.2022.103419
Campos, A. C., Fogaça, M. V., Sonego, A. B., and Guimarães, F. S. (2016). Cannabidiol, neuroprotection and neuropsychiatric disorders. Pharmacol. Res. 112, 119–127. doi:10.1016/J.PHRS.2016.01.033
Castillo, A., Tolón, M. R., Fernández-Ruiz, J., Romero, J., and Martinez-Orgado, J. (2010). The neuroprotective effect of cannabidiol in an in vitro model of newborn hypoxic-ischemic brain damage in mice is mediated by CB2and adenosine receptors. Neurobiol. Dis. 37, 434–440. doi:10.1016/j.nbd.2009.10.023
Choi, I.-Y., Lee, J.-C., Ju, C., Hwang, S., Cho, G.-S., Lee, H. W., et al. (2011). A3 adenosine receptor agonist reduces brain ischemic injury and Inhibits inflammatory cell Migration in rats. Am. J. Pathol. 179, 2042–2052. doi:10.1016/j.ajpath.2011.07.006
Cordomí, A., Navarro, G., Aymerich, M. S., and Franco, R. (2015). Structures for G-protein-coupled receptor Tetramers in complex with G proteins. Trends biochem. Sci. 40, 548–551. doi:10.1016/j.tibs.2015.07.007
Dall’lgna, O. P., Porciúncula, L. O., Souza, D. O., Cunha, R. A., and Lara, D. R. (2003). Neuroprotection by caffeine and adenosine A2A receptor blockade of beta-amyloid neurotoxicity. Br. J. Pharmacol. 138, 1207–1209. doi:10.1038/SJ.BJP.0705185
Faron-Górecka, A., Szlachta, M., Kolasa, M., Solich, J., Górecki, A., Kuśmider, M., et al. (2019). Understanding GPCR dimerization. Methods Cell Biol. 149, 155–178. doi:10.1016/BS.MCB.2018.08.005
Fiorentini, C., Busi, C., Gorruso, E., Gotti, C., Spano, P. F., and Missale, C. (2008a). Reciprocal regulation of dopamine D1 and D3 receptor function and trafficking by heterodimerization. Mol. Pharmacol. 74, 59–69. doi:10.1124/mol.107.043885
Fiorentini, C., Busi, C., Gorruso, E., Gotti, C., Spano, P. F., and Missale, C. (2008b). Reciprocal regulation of dopamine D1 and D3 receptor function and trafficking by heterodimerization. Mol. Pharmacol. 74, 59–69. doi:10.1124/MOL.107.043885
Fiorentini, C., Busi, C., Spano, P. F., and Missale, C. (2010). Dimerization of dopamine D1 and D3 receptors in the regulation of striatal function. Curr. Opin. Pharmacol. 10, 87–92. doi:10.1016/J.COPH.2009.09.008
Fiorentini, C., Busi, C., Spano, P., and Missale, C. (2008c). Role of receptor heterodimers in the development of l-dopa-induced dyskinesias in the 6-hydroxydopamine rat model of Parkinson’s disease. Park. Relat. Disord. 14, S159–S164. doi:10.1016/j.parkreldis.2008.04.022
Fiorentini, C., Gardoni, F., Spano, P., Di Luca, M., and Missale, C. (2003). Regulation of dopamine D1 receptor trafficking and Desensitization by Oligomerization with glutamate N-Methyl-D-aspartate receptors. J. Biol. Chem. 278, 20196–20202. doi:10.1074/jbc.M213140200
Fiorentini, C., Savoia, P., Savoldi, D., and Missale, C. (2013). Receptor heteromers in Parkinson’s disease and L-DOPA-induced dyskinesia. CNS Neurol. Disord. Drug Targets 12, 1101–1113.
Franco, R., Martínez-Pinilla, E., Lanciego, J. L., and Navarro, G. (2016). Basic pharmacological and structural evidence for class A G-protein-coupled receptor heteromerization. Front. Pharmacol. 7, 76–10. doi:10.3389/fphar.2016.00076
Franco, R., Villa, M., Morales, P., Reyes-Resina, I., Gutiérrez-Rodríguez, A., Jiménez, J., et al. (2019). Increased expression of cannabinoid CB2 and serotonin 5-HT1A heteroreceptor complexes in a model of newborn hypoxic-ischemic brain damage. Neuropharmacology 152, 58–66. doi:10.1016/j.neuropharm.2019.02.004
Frau, L., Borsini, F., Wardas, J., Khairnar, A. S., Schintu, N., and Morelli, M. (2011). Neuroprotective and anti-inflammatory effects of the adenosine A(2A) receptor antagonist ST1535 in a MPTP mouse model of Parkinson’s disease. Synapse 65, 181–188. doi:10.1002/SYN.20833
Galvao, J., Elvas, F., Martins, T., Cordeiro, M. F., Ambrósio, A. F., and Santiago, A. R. (2015). Adenosine A3 receptor activation is neuroprotective against retinal neurodegeneration. Exp. Eye Res. 140, 65–74. doi:10.1016/J.EXER.2015.08.009
Gines, S., Hillion, J., Torvinen, M., Le Crom, S., Casado, V., Canela, E. I., et al. (2000). Dopamine D1 and adenosine A1 receptors form functionally interacting heteromeric complexes. Proc. Natl. Acad. Sci. 97, 8606–8611. doi:10.1073/pnas.150241097
Hasbi, A., Fan, T., Alijaniaram, M., Nguyen, T., Perreault, M. L., O’Dowd, B. F., et al. (2009). Calcium signaling cascade links dopamine D1-D2 receptor heteromer to striatal BDNF production and neuronal growth. Proc. Natl. Acad. Sci. U. S. A. 106, 21377–21382. doi:10.1073/pnas.0903676106
Hasbi, A., Perreault, M. L., Shen, M. Y. F., Fan, T., Nguyen, T., Alijaniaram, M., et al. (2018). Activation of dopamine D1-D2 receptor complex attenuates cocaine reward and reinstatement of cocaine-seeking through inhibition of DARPP-32, ERK, and ΔFosB. Front. Pharmacol. 8, 924. doi:10.3389/fphar.2017.00924
He, S. Q., Zhang, Z. N., Guan, J. S., Liu, H. R., Zhao, B., Wang, H. B., et al. (2011). Facilitation of μ-opioid receptor activity by preventing δ-opioid receptor-mediated codegradation. Neuron 69, 120–131. doi:10.1016/J.NEURON.2010.12.001
Jenner, P. (2014). An Overview of adenosine A2A receptor antagonists in Parkinson’s disease. Int. Rev. Neurobiol. 119, 71–86. doi:10.1016/B978-0-12-801022-8.00003-9
Jenner, P., Mori, A., Hauser, R., Morelli, M., Fredholm, B. B., and Chen, J. F. (2009). Adenosine, adenosine A 2A antagonists, and Parkinson’s disease. Park. Relat. Disord. 15, 406–413. doi:10.1016/j.parkreldis.2008.12.006
Ji, B., Liu, H., Zhang, R., Jiang, Y., Wang, C., Li, S., et al. (2017). Novel signaling of dynorphin at κ-opioid receptor/bradykinin B2 receptor heterodimers. Cell. Signal. 31, 66–78. doi:10.1016/J.CELLSIG.2017.01.005
Jordan, B. A., and Devi, L. A. (1999). G-protein-coupled receptor heterodimerization modulates receptor function. Nature 399, 697–700. doi:10.1038/21441
Kniazeff, J., Prézeau, L., Rondard, P., Pin, J.-P., and Goudet, C. (2011). Dimers and beyond: The functional puzzles of class C GPCRs. Pharmacol. Ther. 130, 9–25. doi:10.1016/j.pharmthera.2011.01.006
Kuhhorn, J., Hubner, H., Gmeiner, P., Kühhorn, J., Hübner, H., Gmeiner, P., et al. (2011). Bivalent dopamine D2 receptor ligands: Synthesis and binding properties. J. Med. Chem. 54, 4896–4903. doi:10.1021/jm2004859
Kunishima, N., Shimada, Y., Tsuji, Y., Sato, T., Yamamoto, M., Kumasaka, T., et al. (2000). Structural basis of glutamate recognition by a dimeric metabotropic glutamate receptor. Nature 407, 971–977. doi:10.1038/35039564
Lafuente, H., Alvarez, F. J., Pazos, M. R., Alvarez, A., Rey-Santano, M. C., Mielgo, V., et al. (2011). Cannabidiol reduces brain damage and Improves functional Recovery after Acute hypoxia-ischemia in newborn pigs. Pediatr. Res. 70, 272–277. doi:10.1203/PDR.0b013e3182276b11
Li, P., Li, X., Deng, P., Wang, D., Bai, X., Li, Y., et al. (2020). Activation of adenosine A3 receptor reduces early brain injury by alleviating neuroinflammation after subarachnoid hemorrhage in elderly rats. Aging (Albany. NY) 13, 694–713. doi:10.18632/AGING.202178
Lillo, A., Martínez-Pinilla, E., Reyes-Resina, I., Navarro, G., and Franco, R. (2020). Adenosine A2a and A3 receptors are able to interact with each other. A further piece in the puzzle of adenosine receptor-mediated signaling. Int. J. Mol. Sci. 21, 1–13. doi:10.3390/ijms21145070
Lillo, A., Raïch, I., Lillo, J., Pérez-Olives, C., Navarro, G., and Franco, R. (2022). Expression of the adenosine A2A-A3 receptor heteromer in different brain regions and marked upregulation in the microglia of the transgenic APPSw,Ind Alzheimer’s disease model. Biomedicines 10, 214. doi:10.3390/BIOMEDICINES10020214
Maggio, R., Aloisi, G., Silvano, E., Rossi, M., and Millan, M. J. (2010). Heterodimerization of dopamine receptors: New insights into functional and therapeutic significance. Park. Relat. Disord. 15, S2–S7. doi:10.1016/S1353-8020(09)70826-0
Maggio, R., and Millan, M. J. (2010). Dopamine D2-D3 receptor heteromers: Pharmacological properties and therapeutic significance. Curr. Opin. Pharmacol. 10, 100–107. doi:10.1016/J.COPH.2009.10.001
Marcellino, D., Ferré, S., Casadó, V., Cortés, A., Le Foll, B., Mazzola, C., et al. (2008). Identification of dopamine D1-D3 receptor heteromers: Indications for a role of synergistic D1-D3 receptor interactions in the striatum. J. Biol. Chem. 283, 26016–26025. doi:10.1074/jbc.M710349200
Martínez-Pinilla, E., Reyes-Resina, I., Oñatibia-Astibia, A., Zamarbide, M., Ricobaraza, A., Navarro, G., et al. (2014). CB1 and GPR55 receptors are co-expressed and form heteromers in rat and monkey striatum. Exp. Neurol. 261, 44–52. doi:10.1016/j.expneurol.2014.06.017
Martínez-Pinilla, E., Aguinaga, D., Navarro, G., Rico, A. J., Oyarzábal, J., Sánchez-Arias, J. A., et al. (2019). Targeting CB 1 and GPR55 endocannabinoid receptors as a potential neuroprotective approach for Parkinson’s disease. Mol. Neurobiol. 56, 5900–5910. doi:10.1007/S12035-019-1495-4
Martínez-Pinilla, E., Varani, K., Reyes-Resina, I., Angelats, E., Vincenzi, F., Ferreiro-Vera, C., et al. (2017). Binding and signaling studies disclose a potential allosteric site for cannabidiol in cannabinoid CB2 receptors. Front. Pharmacol. 8, 744. doi:10.3389/fphar.2017.00744
Massari, C. M., Constantino, L. C., and Tasca, C. I. (2021). Adenosine A1 and A2A receptors are involved on guanosine protective effects against oxidative burst and mitochondrial dysfunction induced by 6-OHDA in striatal slices. Purinergic Signal 17, 247–254. doi:10.1007/S11302-021-09765-Y
Minghetti, L., Greco, A., Potenza, R. L., Pezzola, A., Blum, D., Bantubungi, K., et al. (2007). Effects of the adenosine A2A receptor antagonist SCH 58621 on cyclooxygenase-2 expression, glial activation, and brain-derived neurotrophic factor availability in a rat model of striatal neurodegeneration. J. Neuropathol. Exp. Neurol. 66, 363–371. doi:10.1097/nen.0b013e3180517477
Mori, A., Chen, J.-F., Uchida, S., Durlach, C., King, S. M., and Jenner, P. (2022). The pharmacological potential of adenosine A 2A receptor antagonists for treating Parkinson’s disease. Molecules 27, 2366. doi:10.3390/MOLECULES27072366
Mutti, V., Fiorentini, C., Missale, C., and Bono, F. (2020). Dopamine D3 receptor heteromerization: Implications for Neuroplasticity and neuroprotection. Biomolecules 10 (7), 1016. doi:10.3390/BIOM10071016
Navarro, G., Borroto-Escuela, D., Angelats, E., Etayo, I., Reyes-Resina, I., Pulido-Salgado, M., et al. (2018a). Receptor-heteromer mediated regulation of endocannabinoid signaling in activated microglia. Role of CB1 and CB2 receptors and relevance for Alzheimer’s disease and levodopa-induced dyskinesia. Brain. Behav. Immun. 67, 139–151. doi:10.1016/j.bbi.2017.08.015
Navarro, G., Cordomí, A., Brugarolas, M., Moreno, E., Aguinaga, D., Pérez-Benito, L., et al. (2018b). Cross-communication between Gi and Gs in a G-protein-coupled receptor heterotetramer guided by a receptor C-terminal domain. BMC Biol. 16 (24), 24–15. doi:10.1186/s12915-018-0491-x
Navarro, G., Cordomí, A., Zelman-Femiak, M., Brugarolas, M., Moreno, E., Aguinaga, D., et al. (2016). Quaternary structure of a G-protein-coupled receptor heterotetramer in complex with Gi and Gs. BMC Biol. 14, 26. doi:10.1186/s12915-016-0247-4
Navarro, G., Gonzalez, A., Sánchez-Morales, A., Casajuana-Martin, N., Gómez-Ventura, M., Cordomí, A., et al. (2021). Design of negative and Positive allosteric modulators of the cannabinoid CB2 receptor derived from the natural product cannabidiol. J. Med. Chem. 64, 9354–9364. doi:10.1021/ACS.JMEDCHEM.1C00561
Navarro, G., Reyes-Resina, I., Rivas-Santisteban, R., Sánchez de Medina, V., Morales, P., Casano, S., et al. (2018c). Cannabidiol skews biased agonism at cannabinoid CB1 and CB2 receptors with smaller effect in CB1-CB2 heteroreceptor complexes. Biochem. Pharmacol. 157, 148–158. doi:10.1016/j.bcp.2018.08.046
Nelson, G., Chandrashekar, J., Hoon, M. A., Feng, L., Zhao, G., Ryba, N. J. P., et al. (2002). An amino-acid taste receptor. Nature 416, 199–202. doi:10.1038/NATURE726
Orru, M., Bakešová, J., Brugarolas, M., Quiroz, C., Beaumont, V., Goldberg, S. R., et al. (2011). Striatal pre- and postsynaptic profile of adenosine A2A receptor antagonists. PLoS One 6, e16088. doi:10.1371/journal.pone.0016088
Pazos, M. R., Cinquina, V., Gómez, A., Layunta, R., Santos, M., Fernández-Ruiz, J., et al. (2012). Cannabidiol administration after hypoxia–ischemia to newborn rats reduces long-term brain injury and restores neurobehavioral function. Neuropharmacology 63, 776–783. doi:10.1016/j.neuropharm.2012.05.034
Pazos, M. R. R., Mohammed, N., Lafuente, H., Santos, M., Martínez-Pinilla, E., Moreno, E., et al. (2013). Mechanisms of cannabidiol neuroprotection in hypoxic-ischemic newborn pigs: Role of 5HT1A and CB2 receptors. Neuropharmacology 71, 282–291. doi:10.1016/j.neuropharm.2013.03.027
Pérez-Olives, C., Rivas-Santisteban, R., Lillo, J., Navarro, G., and Franco, R. (2021). “Recent Advances in the potential of cannabinoids for neuroprotection in Alzheimer’s, Parkinson’s, and Huntington’s diseases,” in Advances in experimental medicine and Biology (Berlin, Germany: Springer), 81–92. doi:10.1007/978-3-030-57369-0_6
Perreault, M. L., Hasbi, A., Alijaniaram, M., Fan, T., Varghese, G., Fletcher, P. J., et al. (2010). The dopamine D1-D2 receptor heteromer localizes in dynorphin/enkephalin neurons: Increased high affinity state following amphetamine and in schizophrenia. J. Biol. Chem. 285, 36625–36634. doi:10.1074/jbc.M110.159954
Perreault, M. L., Hasbi, A., Shen, M. Y. F. F. M. Y. F., Fan, T., Navarro, G., Fletcher, P. J. P. J., et al. (2016). Disruption of a dopamine receptor complex amplifies the actions of cocaine. Eur. Neuropsychopharmacol. 26, 1366–1377. doi:10.1016/j.euroneuro.2016.07.008
Perreault, M. L., Shen, M. Y. F., Fan, T., and George, S. R. (2015). Regulation of c-fos expression by the dopamine D1-D2 receptor heteromer. Neuroscience 285, 194–203. doi:10.1016/j.neuroscience.2014.11.017
Raïch, I., Rebassa, J. B., Lillo, J., Cordomi, A., Rivas-Santisteban, R., Lillo, A., et al. (2022). Antagonization of OX1 receptor potentiates CB2 receptor function in microglia from APPSw/Ind mice model. Int. J. Mol. Sci. 23, 12801. doi:10.3390/IJMS232112801
Rashid, A. J., So, C. H., Kong, M. M. C., Furtak, T., El-Ghundi, M., Cheng, R., et al. (2007). D1-D2 dopamine receptor heterooligomers with unique pharmacology are coupled to rapid activation of Gq/11 in the striatum. Proc. Natl. Acad. Sci. U. S. A. 104, 654–659. doi:10.1073/pnas.0604049104
Rebola, N., Simões, A. P., Canas, P. M., Tomé, A. R., Andrade, G. M., Barry, C. E., et al. (2011). Adenosine A2A receptors control neuroinflammation and consequent hippocampal neuronal dysfunction. J. Neurochem. 117, 100–111. doi:10.1111/j.1471-4159.2011.07178.x
Reyes-Resina, I., Navarro, G., Aguinaga, D., Canela, E. I., Schoeder, C. T., Załuski, M., et al. (2018). Molecular and functional interaction between GPR18 and cannabinoid CB2 G-protein-coupled receptors. Relevance in neurodegenerative diseases. Biochem. Pharmacol. 157, 169–179. doi:10.1016/j.bcp.2018.06.001
Rico, A. J., Dopeso-Reyes, I. G., Martínez-Pinilla, E., Sucunza, D., Pignataro, D., Roda, E., et al. (2016). Neurochemical evidence supporting dopamine D1–D2 receptor heteromers in the striatum of the long-tailed macaque: Changes following dopaminergic manipulation. Brain Struct. Funct. 222, 1767–1784. doi:10.1007/s00429-016-1306-x
Rivas-Santisteban, R., Rodriguez-Perez, A. I., Muñoz, A., Reyes-Resina, I., Labandeira-García, J. L., Navarro, G., et al. (2020). Angiotensin AT1 and AT2 receptor heteromer expression in the hemilesioned rat model of Parkinson’s disease that increases with levodopa-induced dyskinesia. J. Neuroinflammation 17, 243. doi:10.1186/s12974-020-01908-z
Saki, M., Yamada, K., Koshimura, E., Sasaki, K., and Kanda, T. (2013). In vitro pharmacological profile of the A2A receptor antagonist istradefylline. Naunyn. Schmiedeb. Arch. Pharmacol. 386, 963–972. doi:10.1007/s00210-013-0897-5
Saura, J., Angulo, E., Ejarque, A., Casado, V., Tusell, J. M., Moratalla, R., et al. (2005). Adenosine A2A receptor stimulation potentiates nitric oxide release by activated microglia. J. Neurochem. 95, 919–929. doi:10.1111/j.1471-4159.2005.03395.x
Scarselli, M., Novi, F., Schallmach, E., Lin, R., Baragli, A., Colzi, A., et al. (2001). D2/D3 dopamine receptor heterodimers Exhibit unique functional properties. J. Biol. Chem. 276, 30308–30314. doi:10.1074/jbc.M102297200
Soriano, A., Ventura, R., Molero, A., Hoen, R., Casado, V., Corte, A., et al. (2009). Adenosine A2A receptor-antagonist/dopamine D2 receptor-agonist bivalent ligands as pharmacological tools to detect A2A-D2 receptor heteromers. J. Med. Chem. 52, 5590–5602. doi:10.1021/jm900298c
Stenkamp, R. E. (2018). Identifying G protein-coupled receptor dimers from crystal packings. Acta Crystallogr. Sect. D. Struct. Biol. 74, 655–670. doi:10.1107/S2059798318008136
Torvinen, M., Marcellino, D., Canals, M., Agnati, L. F., Lluis, C., Franco, R., et al. (2005). Adenosine A2A receptor and dopamine D3 receptor interactions: Evidence of functional A2A/D3 heteromeric complexes. Mol. Pharmacol. 67, 400–407. doi:10.1124/mol.104.003376
Velazhahan, V., Ma, N., Pándy-Szekeres, G., Kooistra, A. J., Lee, Y., Gloriam, D. E., et al. (2021). Structure of the class D GPCR Ste2 dimer coupled to two G proteins. Nature 589, 148–153. doi:10.1038/S41586-020-2994-1
Verma, V., Hasbi, A., O'Dowd, B. F., George, S. R., O’Dowd, B. F., and George, S. R. (2010). Dopamine D1-D2 receptor heteromer-mediated calcium release is desensitized by D1 receptor occupancy with or without signal activation: Dual functional regulation by G protein-coupled receptor kinase. J. Biol. Chem. 2, 35092–35103. doi:10.1074/jbc.M109.088625
Yu, J. J., Xue, Y., Wang, Y., Liu, C., and Chen, L. (2021). In vivo Bidirectional modulation of cannabinoid on the activity of globus pallidus in rats. Neuroscience 468, 123–138. doi:10.1016/J.NEUROSCIENCE.2021.06.012
Yue, Y., Liu, L., Wu, L. J., Wu, Y., Wang, L., Li, F., et al. (2022). Structural insight into apelin receptor-G protein stoichiometry. Nat. Struct. Mol. Biol. 297, 688–697. doi:10.1038/s41594-022-00797-5
Keywords: heteromer imprint, heteromer selective drug, GPCR, neuronal death, neurodegeneration, drug discovery, biased agonism
Citation: Franco R and Navarro G (2023) Neuroprotection afforded by targeting G protein-coupled receptors in heteromers and by heteromer-selective drugs. Front. Pharmacol. 14:1222158. doi: 10.3389/fphar.2023.1222158
Received: 13 May 2023; Accepted: 03 July 2023;
Published: 13 July 2023.
Edited by:
Mahmoud Iravani, University of Hertfordshire, United KingdomReviewed by:
Meng Cui, Northeastern University, United StatesCopyright © 2023 Franco and Navarro. This is an open-access article distributed under the terms of the Creative Commons Attribution License (CC BY). The use, distribution or reproduction in other forums is permitted, provided the original author(s) and the copyright owner(s) are credited and that the original publication in this journal is cited, in accordance with accepted academic practice. No use, distribution or reproduction is permitted which does not comply with these terms.
*Correspondence: Rafael Franco, cmZyYW5jbzEyM0BnbWFpbC5jb20=
Disclaimer: All claims expressed in this article are solely those of the authors and do not necessarily represent those of their affiliated organizations, or those of the publisher, the editors and the reviewers. Any product that may be evaluated in this article or claim that may be made by its manufacturer is not guaranteed or endorsed by the publisher.
Research integrity at Frontiers
Learn more about the work of our research integrity team to safeguard the quality of each article we publish.