- 1Department of Pharmacology, University of the Free State, Bloemfontein, South Africa
- 2Department of Biochemistry, University of KwaZulu-Natal, Durban, South Africa
- 3Laser Research Centre, Faculty of Health Sciences, University of Johannesburg, Doornfontein, South Africa
Introduction: Hepatic oxidative injury is one of the pathological mechanisms that significantly contributes to the development of several liver diseases. In the present study, the hepatoprotective effect of Lippia javanica herbal tea was investigated in Fe2+- mediated hepatic oxidative injury.
Methods: Using an in vitro experimental approach, hepatic oxidative injury was induced by co-incubating 7 mM FeSO4 with Chang liver cells that have been pre-incubated with or without different concentrations (15–240 μg/mL) of L. javanica infusion. Gallic acid and ascorbic acid served as the standard antioxidants.
Results: The infusion displayed a reducing antioxidant activity in ferric-reducing antioxidant power (FRAP) assay and a potent scavenging activity on 2,2-diphenyl-2- picrylhydrazyl (DPPH) radical. Pretreatment with L. javanica infusion significantly elevated the levels of reduced glutathione and non-protein thiol, and the activities of superoxide dismutase (SOD) and catalase, with concomitant decrease in hepatic malondialdehyde levels, acetylcholinesterase, glucose-6-phosphatase, fructose-1,6-bisphosphatase, glycogen phosphorylase and lipase activities. The infusion showed the presence of phytoconstituents such as phenolic compounds, tannins, phenolic glycosides and terpenoids when subjected to liquid chromatography—mass spectrometry analysis. Molecular docking revealed a strong binding affinity of dihydroroseoside and obacunone with both SOD and catalase compared to other phytoconstituents.
Conclusion: These results portray a potent antioxidant and hepatoprotective effect of L. javanica, which may support the local usage of the herbal tea as a prospective therapeutic agent for oxidative stress-related liver diseases.
Introduction
About two million cases of global mortality are attributed to liver diseases, with liver cirrhosis and liver cancer being the most common causes of these deaths (Asrani et al., 2019). Besides increased risks of mortality, chronic liver diseases cause several extrahepatic morbidities which contribute notably to low quality of life. Thus, liver diseases, though underestimated, pose a high economic burden which is a major concern (Stepanova et al., 2017; Asrani et al., 2019).
Regardless of the cause, most chronic liver diseases are typified by oxidative stress (Cichoż-Lach and Michalak, 2014). Excessive reactive oxygen species (ROS) cause disturbances in redox homeostasis which results in oxidative stress, a major pathological mechanism involved in the development and progression of several liver diseases. Oxidative stress induces dire alterations in liver proteins, lipids and DNA components as well as impair pathways involved in normal biological functions of the liver (Li et al., 2014; Li et al., 2015). The liver is the main organ usually attacked by ROS, as the parenchymal cells, hepatic stellate cells, Kupffer cells and endothelial cells of the liver are all vulnerable to oxidative injury, causing damages to each cell types (Cichoż-Lach and Michalak, 2014). Several risk factors including drugs, alcohol, irradiation and environmental pollutants such as heavy metals may mediate hepatic oxidative stress. Damages induced by oxidative stress significantly contribute to impairment of gene expression and progression of liver diseases as well as apoptosis and necrosis (Cichoż-Lach and Michalak, 2014; Li et al., 2015).
Severe disturbances in hepatic glucose and lipid metabolism homeostasis have been recognized as some of the major mechanisms involved in liver diseases such as liver cirrhosis, liver steatosis and fatty liver, with oxidative stress being a key contributor (Miksztowicz et al., 2012; Ding et al., 2018). Excess cellular levels of glucose and lipids can serve as substrates for the generation of glucotoxic and lipotoxic species, respectively, which can cause damage to biomolecules, induce metabolic stress and eventual cell death (Mota et al., 2016; Chen et al., 2020). Additionally, altered cholinergic enzyme activities have been implicated in the pathogenesis of liver diseases and studies have reported oxidative stress as facilitator of cholinergic dysfunction (Garcia-Ayllon et al., 2012; Erukainure et al., 2021a). These corroborates the use of antioxidants as therapies for targeting oxidative stress in the management of liver diseases (Upadhyay et al., 2022).
Several medicinal plants, including herbal infusions have been globally used over the decades for the treatment of several chronic liver diseases due to their availability, curative effects and minute adverse effects. These therapeutic characteristics have been ascribed to the phytochemical components of the plants (Hong et al., 2015; Chukwuma et al., 2019). Studies have indicated that medicinal plants and their phytochemicals exhibit their hepatoprotective effects in several ways including mitigation of oxidative stress, blockage of fibrogenesis and suppression of tumorigenesis (Dhiman et al., 2012; Hong et al., 2015).
Lippia javanica (Burm.f.) (Family: Verbenaceae) is a multi-stemmed, woody, drought-resistant shrub that is naturally distributed in central, eastern and southern Africa including South Africa, Malawi, Botswana, Kenya, Zambia, Angola, Zanzibar, Tanzania and Mozambique, as well as tropical Indian sub-continent (Germishuizen et al., 2006; Shahriar et al., 2014). In South Africa, it is widely distributed in different provinces which include KwaZulu-Natal, Gauteng, Free State, Limpopo, Eastern Cape and Northwest. It is known as one of the aromatic indigenous shrubs in South Africa. The common names of L. javanica include fever tree, wild sage, wild tea and lemon bush. The Xhosa community of South Africa call it inzinzinba while the Zulus call it umwazi (Maroyi, 2017). Traditionally, L. javanica has been used from time immemorial as herbal tea or as either root or leave decoction to treat fever, malaria, cough, cold, chest pain, asthma, bronchitis, and diarrhea. The Zulus in South Africa use the herbal tonic as an immune booster. In Zimbabwe and South Africa, the burnt whole plant or leaves are used as mosquito repellant (Lukwa et al., 2009; Maroyi, 2017). The reported pharmacological activities of the plant include antioxidant, antimalarial, antidiabetic, anticancer, antiviral and antimicrobial activities (Fouché et al., 2008; Mujovo et al., 2008; Shikanga et al., 2010; Maroyi, 2017). The neuroprotective effect of its herbal tea infusion on lead-induced brain oxidative damage in Wistar rats was also reported by Suleman et al. (2022). Despite its numerous documented medicinal properties, there is limited information on the effect of L. javanica on liver diseases.
The purpose of the present study was to investigate the potential hepatoprotective effect of L. javanica tea infusion on iron-induced oxidative hepatic injury in Chang liver cells by assessing its effect on oxidative stress, cholinergic dysfunction, altered carbohydrate metabolism and lipase activities.
Materials and methods
Plant material
Plant collection and verification
Lippia javanica (Burm.f.) Spreng leaves were collected from Langenhoven Park, Bloemfontein, Free State Province, South Africa (GPS Coordinates: 29°05′32.2″S 26°09′25.6″E) by Prof. M.G. Matsabisa. The plant sample was deposited at the Geo Potts Herbarium, University of the Free State, Bloemfontein 9,300, South, where it was identified, authenticated and assigned a voucher specimen number (BLFU/MGM005).
Plant infusion preparation
After air-drying at room temperature, the L. javanica leaves were pulverized into powder. Extraction was done by boiling 50 g of the leave powder in 500 mL of distilled water for 10 min. The mixture was allowed to cool and then filtered into a pre-weighed glass beaker using a Whatman filter (Whatman, England). The extract was concentrated in a water bath at 50°C. The dry plant infusion was scrapped and transferred into a glass vial and stored at −20°C.
The infusion was re-constituted in distilled water by preparing a stock solution of 1 mg/mL from which various working concentrations ranging from 15 to 240 μg/mL were prepared for different assays. Similarly, the same working concentrations were prepared for two antioxidant standards, ascorbic acid and gallic acid from a stock solution of 1 mg/mL.
Phytochemical characterization and quantification
Total phenolic content
The total phenolic content of the infusion was determined using the Folin-Ciocalteu’s phenol reagent as described by McDonald et al. (2001). In brief, 40 µL of 240 μg/mL plant extract was incubated in the dark with 200 µL of 10% Folin Ciocalteau reagent and 160 µL of 0.7 M Na2CO3 for 30 min at room temperature. The absorbance of the triplicates were measured at 765 nm, using a Multiskan ascent plate reader (Thermo scientific, S.A). The total phenolic content was estimated from a gallic acid standard curve and results were expressed as gallic acid equivalents (GAE) in milligrams per gram of dry weight.
Total flavonoid content
The total flavonoid content of the infusion was estimated by utilizing the aluminum chloride colorimetric method described by Chang et al. (2002), with slight modification. Briefly, 100 μL of the infusion (240 μg/mL) was added to a mixture of 100 μL of methanol, 10 μL of aluminum chloride, 10 μL of 1 mol/L potassium chloride and 200 μL of distilled water. The mixture was allowed to stand for 30 min at room temperature. The absorbance of the independent triplicates were measured at 415 nm and the total flavonoid content was estimated from a quercetin calibration standard curve. The results were expresses as quercetin equivalent (QE) in milligrams per gram of dry weight.
Liquid chromatography-mass spectrometry (LC-MS) analysis of L. javanica
The pharmacologically active chemical constituents present in the L. javanica infusion were characterized via direct-loop injection into Shimadzu LC/MS-2020 Single Quadrupole Liquid Chromatograph Mass Spectrometer (LCMS) equipped with an electrospray ionization (ESI) source. The analysis data acquisition duration was set at 50 min at a low-pressure gradient, while the LC photodiode array (PDA) sampling frequency was kept at 1.5625 Hz. The oven temperature range was maintained between 40°C–50°C, and the pump flow rate was kept at 300 μL/min. The mobile phase solvent system contained 0.1% formic acid in water (phase A) and methanol: acetonitrile (1:1) (phase B). Scanning was done at positive and negative polarities with other operating parameters which include, Start Time: 0.0 min; End Time: 50.0 min; Event Time: 0.25 s; Cell Temperature: 40°C; Threshold: 0; Start Wavelength: 220 nm; End Wavelength: 400 nm; Detector Voltage: +0.00 kV; Scan Speed: 5,000 u/s; Start and End m/z: 100.0 and 1,000.0, respectively. The compounds were identified by direct comparison of the generated spectra containing the relative abundance and the m/z fragmentation patterns with those in the m/z cloud database at https://www.mzcloud.org/.
In vitro antioxidant activities of the infusion
2,2-Diphenyl-1-picrylhydrazyl (DPPH) scavenging activity
The free radical (DPPH) scavenging activity of the infusion was determined by using a previously established protocol (Changlian et al., 2000). In brief, 50 μL of 0.3 mM DPPH in methanol was mixed with 100 μL of different concentrations (15–240 μg/mL) of the infusions or the standards ascorbic acid and gallic acid in a 96-well plate. The plate with the samples was incubated in the dark at room temperature for 30 min. The absorbance was measured against a blank solution at 517 nm, and the percentage scavenging activity was calculated.
Ferric reducing antioxidant power (FRAP)
The ferric-reducing capacity of the infusion was determined by the potassium ferricyanide method according to Benzie and Strain (1996) with slight modifications. 20 μL of different concentrations (15–240 μg/mL) of the herbal infusion was incubated with 20 µL of 0.2 M sodium phosphate buffer (pH 6.6) and 20 µL of 1% potassium ferricyanide at 50°C for 30 min 10% trichloroacetic acid (20 µL) was then added to the reaction mixture to acidify it. An aliquot of the acidified sample was added to 20 µL of distilled water and 20 µL of 10% FeCl3. The absorbance was read at 700 nm. The results were expressed as a percentage of the absorbance of the sample to the absorbance of gallic acid.
Cell lines
Chang liver cells (ATCC® CCL-13™) were procured from the American Type Culture Collection (ATCC®), Manassas, Virginia, United States.
Cytotoxicity screening
Chang liver cells were seeded in a 96-well plate (Nunc, Thermofischer Scientific) at a cell density of 10,000 cells/well (100 µL/well) and left to attach overnight at 37°C in humidified atmosphere with a 5% CO2 concentration. The cells were then treated with various concentrations of L. javanica (15–240 μg/mL) and incubated for 48 h at 37°C. Doxorubicin (3 μg/mL) was used as a positive control. Dimenthylsulfoxide (DMSO) (0.5%) was used as a vehicle control. The cytotoxicity of the cells were evaluated using MTT [3-(4,5-dimethylthiazol-2-yl)-2,5-diphenyltetrazolium bromide. After treatment, the spent media was aspirated and replaced with 0.5 mg/mL MTT solution (100 µL/well) dissolved in fresh media followed by an incubation at 37°C for 2 h. After the incubation, the MTT solution was removed and 100 µL of dimenthylsulfoxide was added. Subsequently, the absorbance was read at 550 nm using the Multiskan GO spectrophotometer (Thermo Scientific). The results were expressed as mean ± SD the percentage cell viability of biological repeats.
Induction of oxidative stress in Chang liver cells
Oxidative stress was induced in Chang liver cells using a modified method from a previous protocol (Chukwuma et al., 2019). Briefly, the cells were seeded into 6-well plates (TPP®, Merck) at 160,000 cells/well (2 mL/well) and left to attach overnight at 37°C. After attachment, the cells were incubated with various concentrations of L. javanica extract or the standards, ascorbic acid and gallic acid (15–240 μg/mL) at 37°C for 25 min. Thereafter, 600 µL of 7 mM iron sulphate (FeSO4) was added to each well and the cells further incubated for 30 min at 37°C. Positive controls and negative controls were included and comprised of untreated cells with and without the addition of FeSO4. After incubation, the treatment was removed, and the cells washed twice with PBS (1 mL/well). The PBS was aspirated and the cells were dissociated using 200 µL of trypsin/EDTA and incubated at 37°C until the cells were completely dissociated. The trypsin was neutralized using 700 µL of complete media (DMEM with 10% FBS). The cell suspension was collected in 1.5 mL microcentrifuge tubes respectively and centrifuged at 17,000 × g for 12 min using the Hettich ® MIKRO 120 centrifuge. The supernatant collected and stored at −80°C until further use.
Determination of oxidative stress biomarkers
Oxidative stress biomarkers were determined in the cell by analyzing the level of reduced glutathione (GSH), activities of superoxide dismutase (SOD) and catalase, as well as and malondialdehyde (MDA) level.
Reduced glutathione (GSH) level
GSH levels were determined in the hepatic cells by utilizing Ellman’s spectrophotometric method (Ellman, 1959) with slight modification. Briefly, 0.2 mL of the cell’s supernatant was mixed with 0.6 mL of trichloroacetic acid (10%) and centrifuged for 5 min at 1,000 × g. 0.2 mL aliquot of the supernatant (deproteinized solution) and 0.05 mL of Ellman’s reagent were placed in a 96 well microplate and incubated for 10 min at room temperature. Absorbance was read at 415 nm, and GSH protein level was estimated from a glutathione standard curve.
Superoxide dismutase (SOD) activity
The SOD activity in the cells were determined by utilizing a modified procedure of Kakkar et al. (1984). Briefly, a 96- well microplate containing the supernatant (15 μL), 100 µM diethylenetriaminepentaacetic acid (DETAPAC; 170 μL) and 15 μL of 1.6 mM hydroxydopamine (6-HD) was gently swirled before the absorbance was immediately measured at 492 nm thrice at 1 min interval.
Catalase activity
The hepatic catalase activity was measured in the supernatant by adopting the method of Hadwan and Abed (2016). Briefly, 100 μL of the supernatant was added to 1,000 μL of 0.065 μM H2O2 and incubated for 2 min at 37°C. 100 μL of 32.4 mM ammonium molybdate was used to terminate the reaction and the absorbance was read at 347 nm against the blank containing only H2O2.
Malondialdehyde (MDA) levels
Lipid peroxidation levels was determined by analyzing for MDA concentration according to previous method (Chowdhury and Soulsby, 2002). Briefly, 100 μL of the cell’s supernatant was added to a mixture containing 100 μL of 8.1% sodium dodecyl sulfate solution, 375 μL of 20% pure acetic acid and 1,000 μL of 0.25% thiobarbituric acid. The reaction mixture boiled for 1 h in water bath, and the absorbance was read at 532 nm after cooling, to estimate MDA levels.
Determination of non-protein thiol (NPSH) content
Hepatic non-protein thiol level was estimated spectrophotometrically using Ellman’s method (Ellman, 1959). Briefly, a mixture of 300 μL of cell supernatant and the same volume of 10% trichloroacetic acid were centrifuged at 1,000 × g for 5 min. 50 μL of the resulting supernatant, 150 μL of Ellman’s reagent and 50 μL of 0.1 M phosphate buffer were mixed and incubated for 10 min at 37°C. The absorbance was read at 412 nm and non-protein thiol content were calculate from standard curve of cysteine.
Determination of nitric oxide (NO) level
Hepatic nitric oxide level was determined based on Greiss method according to Tsikas (2005). Briefly a solution made up of 100 μL of the cell supernatant and equal volume of Greiss reagent in a 96 well-plate was incubated in the dark for 30 min at room at 25°C, using the same volume of distilled water as the blank. After incubation, absorbance of the solution was measured at 548 nm.
Determination of acetylcholinesterase activity
Acetylcholinesterase activity was determined in the hepatic cells by employing a previously established method (Ellman et al., 1961). Briefly, a solution containing 100 μL of the supernatant was added to 50 μL of 3.3 mM Ellman’s reagent (pH 7.0) and 250 μL of 0.1 M phosphate buffer (pH 8) was incubated for 20 min at 25°C. 50 μL of 0.05 M acetylcholine iodide was then added. The absorbance was immediately measured at 412 nm at 3 min intervals.
Determination of glucogenic enzymes activities
Glucose 6-phosphatase activity
Glucose 6-phosphatase activity was estimated in the hepatic cells according to a modified method of Balogun and Ashafa (2017). Briefly, 200 μL of the cell supernatant, 100 μL of 0.1 M glucose 6-phosphate, 200 μL of 5 mM KCl, and 1.3 mL of 0.1 M Tris-HCl buffer mixture was incubated at 37°C for 20 min. An addition of 1,000 μL 10% trichloroacetic acid was used to terminate the reaction, after which it was allowed to stand on ice for 10 min before centrifuging for 10 min at 5,000 g. 250 μL aliquot of the supernatant was placed in a 96-well plate and the absorbance was read at 340 nm.
Fructose-1-6-bisphosphatase activity
Fructose-1-6-bisphosphatase activity of the cells were determined according to a modified method by Balogun and Ashafa (2017). Briefly, 100 μL of the cell supernatant was transferred to tube containing 0.05 M 100 μL of fructose (0.05 M), 100 μL of potassium chloride (0.1 M), 250 μL of magnesium chloride (0.1 M), 1.2 mL of Tris–HCl buffer (0.1 M, pH 7.0) and 250 μL of Ethylenediaminetetraacetic acid (1 mM) and incubated for 15 min at 37°C. 10% trichloroacetic acid was used to halt the reaction and further centrifuged for 10 min at 5,000 g (4°C). Thereafter, 50 μL of 1.25% ammonium molybdate and 9% ascorbic acid was included in the reaction, it was allowed to stand for 20 min at room temperature. The absorbance was read at 680 nm.
Glycogen phosphorylase activity
The glycogen phosphorylase activity of cells were evaluated based on the procedure of Balogun and Ashafa (2017). Briefly, 200 µL of the cell supernatant, 100 µL solution of 64 mM glucose-1-phosphate and 100 µL 4% glycogen were mixed and incubated for 10 min at 30°C. 20% ammonium molybdate in concentrated sulfuric acid was used to terminate the reaction. Thereafter, Elon reducer and distilled water was added to the mixture and further incubated for 45 min at 30°C. The absorbance was measured at 340 nm.
Determination of lipase activity
Hepatic lipase activity in the cells was estimated according to a previous method with slight modifications (Kim et al., 2010). Briefly, 200 µL of the cell supernatant was added 390 µL of Tris buffer (pH 7.0) and incubated at 37°C for 15 min 100 μL of p-nitrophenyl butyrate in dimethylformamide (p-NPB) was then added to the mixture before incubating for another 15 min. The absorbance was measured at 405 nm at 1 min interval. Lipase activity of the hepatic cells was expressed as the rate of reaction (ΔA/min).
Molecular docking
This computer analysis was employed to determine the binding affinities of the phytochemicals of L. javanica infusion with catalase and SOD antioxidant enzymes. The x-ray diffraction structure of the respective proteins (1F4J and 2C9V) with 2.40 Å and 1.07 Å resolutions were retrieved from the Protein Data Bank (https://www.rcsb.org/). Non-proteins and water molecules co-crystalized with the proteins were removed using the Dock prep tool algorithm of the Chimera software (V.1.16). Next, the automated program also added hydrogen atoms and gasteiger charges, as described according to Wang et al. (2006). Subsequently, the 3D structure of the LC-MS identified chemical compounds in the L. javanica infusion were similarly downloaded from the Zinc database (https://zinc15.docking.org/substances/home/) and prepared using the previous software employed for the antioxidant enzymes. The catalytic pocket of the proteins were determined using the algorithm of the CASTp online server (http://cast.engr.uic.edu/) before molecular docking was carried out within a search volume covering X, Y, and Z dimension of 13 × 13 × 13 for SOD and 18 × 16 × 14 for catalase. Then, the calculated binding energies of the most stable ligand-protein complexes were recorded, and the 2D images of the various interactions involved were visualized using with BIOVIA Discovery Studio application.
Statistical analysis
The experiments were carried out in triplicate (n = 3) and results were presented as mean ± SD. Analysis of data was achieved by utilizing SPSS (Windows V25) and statistically significant difference between test groups was established at p < 0.05 using a one-way analysis of variance (ANOVA), followed by the use of Dunnett and Tukey’s HSD multiple range Post-hoc tests for comparison of experimental mean values.
Results
The infusion extract of L. javanica revealed a total phenolic content of 25.95 ± 0.74 mg GAE/g and a total flavonoid content of 73.57 ± 2.12 mg QE/g of dry weight of extract. The extract showed a higher amount of flavonoid than phenolics.
As indicated in Figure 1A, L. javanica infusion significantly (p < 0.05) scavenged DPPH free radical at increasing concentrations, with a low IC50 value of 1.22 μg/mL (Table 1) and compared favorably with the standards, ascorbic acid (IC50: values of 0.11 μg/mL) and gallic acid (IC50: values of 0.61 μg/mL). However, the infusion only exhibited slight increase in Fe3+ reducing activity (Figure 1B) with IC50 value of ˃1,000 μg/mL) as compared to ascorbic acid at 120 and 240 μg/mL doses (IC50: values of 975.73 μg/mL) and gallic acid at all tested concentrations (IC50: values of 63.81 μg/mL).
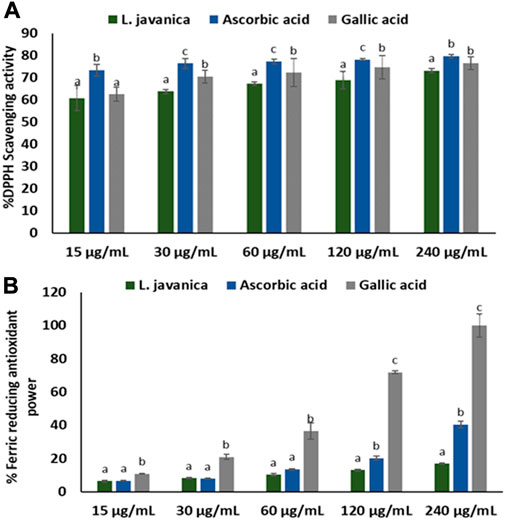
FIGURE 1. (A) DPPH scavenging and (B) FRAP activities of L. javanica. Data presented as mean ± standard deviation. Different unique alphabetical letters (a-c) above the bars for a given concentration illustrate the statistical significance of difference (p < 0.05), Tukey’s-HSD multiple range post hoc test).
As portrayed in Figure 2, there was no significant difference in % cell viability between the cells treated with L. Javanica infusion and the normal Chang liver cells. Treatment with doxorubicin significantly (p < 0.05) reduced the cell viability when compared to the normal control.
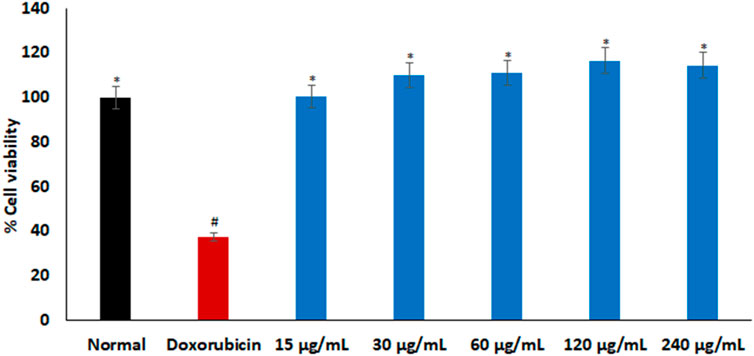
FIGURE 2. Cytotoxic effect of L. javanica on Chang liver cells. Value = mean ± SD; n = 3. *Statistically significant compared to doxorubicin group; #statistically significant compared to the normal control cell (p < 0.05, Dunnett’s multiple range post hoc test).
As depicted in Figures 3A–E, the induction of oxidative injury in Chang liver cells using ferrous sulphate led to significant (p < 0.05) depletion in the levels of GSH and NPSH, activities of SOD and catalase, with a concurrent increase in MDA level when compared with the normal control. Pretreatment of the cells with L. javanica significantly (p < 0.05) reversed these levels and activities and compared favorably with the two standard antioxidants, ascorbic acid and gallic acid. However, there was no significant difference in the catalase activity and NPSH level of the untreated cells and L. javanica-treated cells at lower concentrations.
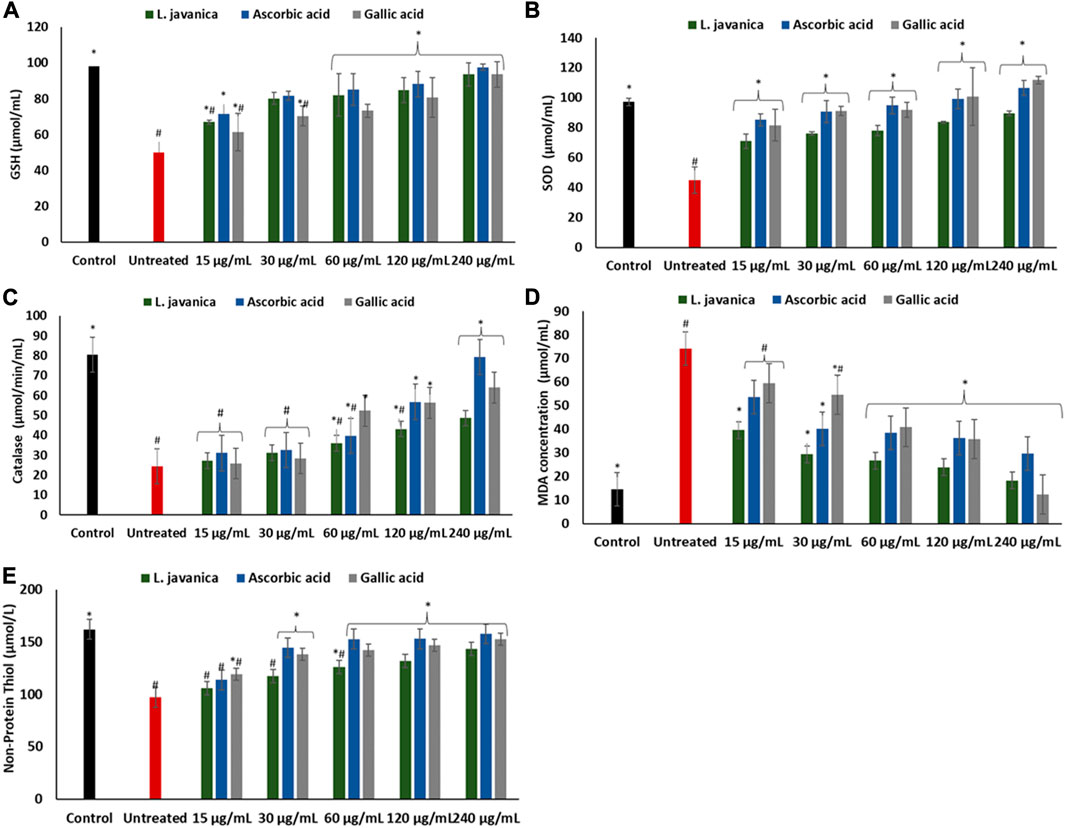
FIGURE 3. Effect of L. javanica on (A) GSH level (B) SOD activity, (C) catalase activity, (D) MDA level, and (E) non-protein thiol level in oxidative hepatic injury. Value = mean ± SD; n = 3. *Statistically significant compared to untreated hepatic cells; #statistically significant compared to the control cells (p < 0.05, Dunnett’s range post hoc test). GSH = Reduced glutathione, SOD = superoxide dismutase, MDA = Malondialdehyde.
Induction of oxidative stress led to a significant (p < 0.05) elevation in hepatic nitric oxide (NO) level (Figure 4). Pretreatment of cells with L. javanica infusion led to remarkable lower levels of NO in a dose-dependent trend. The infusion presented a better effect on NO level than both ascorbic acid and gallic acid.
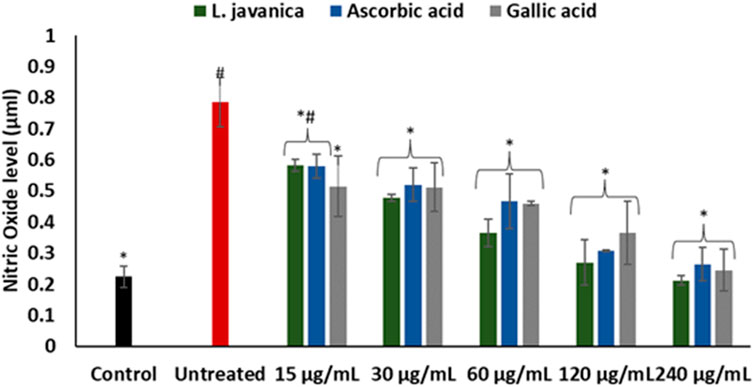
FIGURE 4. Effect of L. javanica on nitric oxide level in oxidative hepatic injury. Value = mean ± SD; n = 3. *Statistically significant compared to untreated hepatic cells; #statistically significant compared to the control cells (p < 0.05, Dunnett’s multiple range post hoc test).
There was a significant (p < 0.05) elevation in acetylcholinesterase activity on incubation of Chang liver cells with FeSO4 (Figure 5). Except at the lowest dose, pretreatment with L. javanica infusion led to a significant (p < 0.05) dose-dependent suppression of acetylcholinesterase activity and was competitive with those of the standards, ascorbic acid and gallic acid.
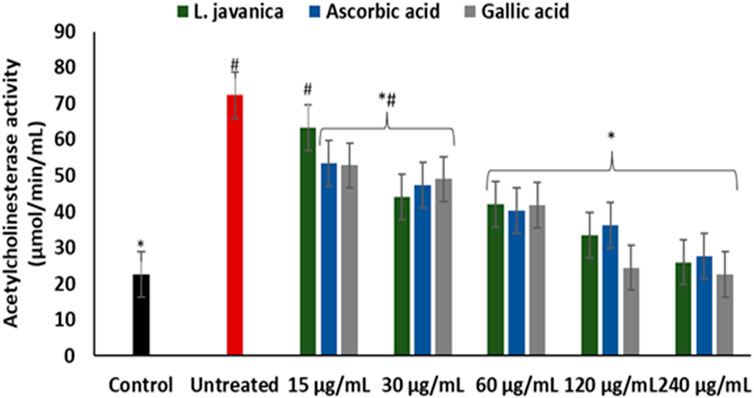
FIGURE 5. Effect of L. javanica on acetylcholinesterase activity in oxidative hepatic injury. Value = mean ± SD; n = 3. *Statistically significant compared to untreated hepatic cells; #statistically significant compared to the control cells (p < 0.05, Dunnett’s multiple range post hoc test).
Induction of oxidative injury caused significant (p < 0.05) elevation of glucose-6-phosphatase, fructose-1,6-bisphosphatase and glycogen phosphorylase activities as depicted in Figures 6A–C. Pretreatment with L. javanica led to significant suppression of the enzyme’s activities in a dose-dependent trend to levels indistinguishable from the normal control.
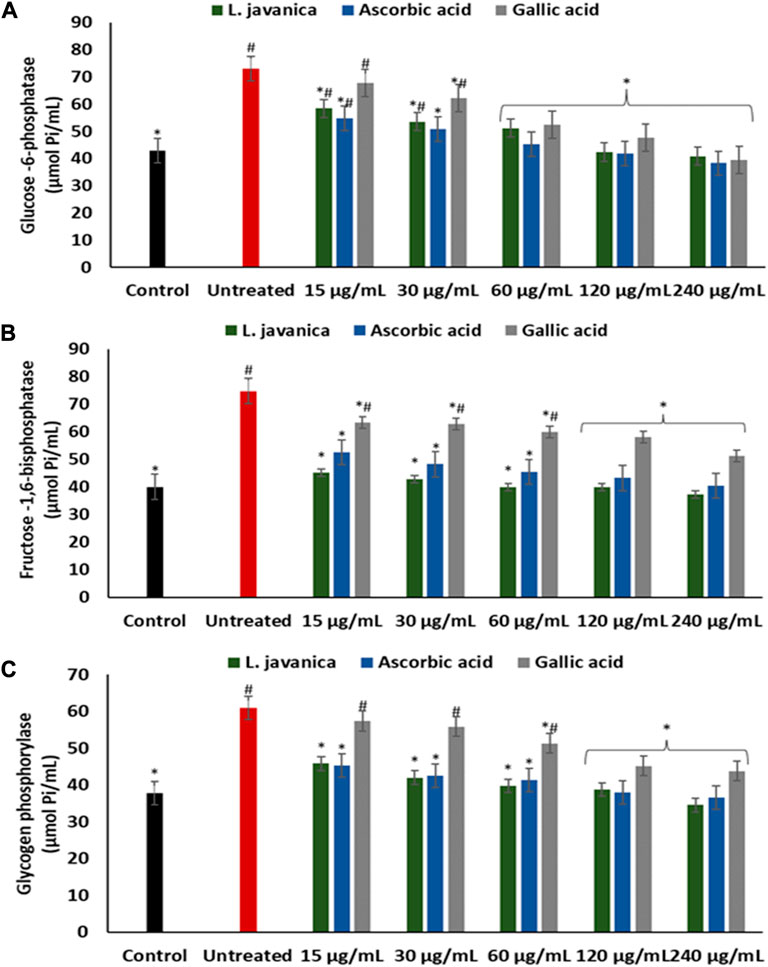
FIGURE 6. Effect of L. javanica on (A) Glucose 6 phosphatase, (B) Fructose 1,6 bisphosphatase and (C) Glycogen phosphorylase activities in oxidative hepatic injury. Value = mean ± SD; n = 3. *Statistically significant compared to untreated hepatic cells; #statistically significant compared to the control cells (p < 0.05, Dunnett’s multiple range post hoc test).
As presented in Figure 7, induction of hepatic oxidative injury significantly (p < 0.05) elevated the activity of lipase. Incubation of the cells with L. javanica significantly (p < 0.05) depleted the activity of the enzyme which outperformed the activities of ascorbic acid and gallic acid.
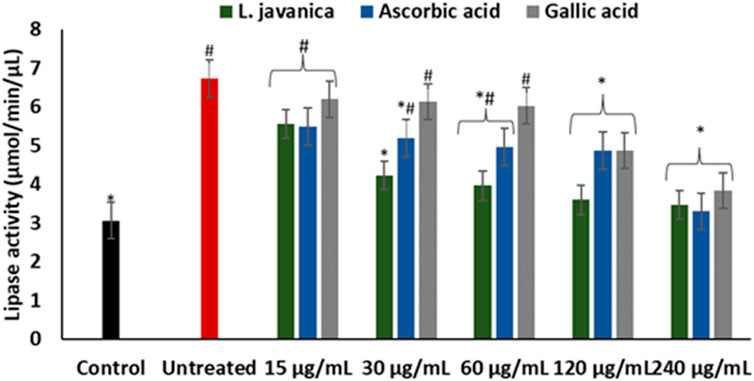
FIGURE 7. Effect of L. javanica on lipase activity in oxidative hepatic injury. Value = mean ± SD; n = 3. *Statistically significant compared to untreated hepatic cells; #statistically significant compared to the control cells (p < 0.05, Dunnett’s multiple range post hoc test).
LC-MS analysis of L. javanica infusion revealed the presence of phenolic compound such as amino phenol; tannins such as corilagin; phenolic glycosides such as coniferin and dihydroroseoside and terpenoids such as obacunone and radicol. [1-(3,4-Dihydroxy-5-methoxyphenyl)-7-(3,4-dihydroxyphenyl) heptan-3-yl] acetate and 3-Ethyl-4-hydroxy-1-phenylquinolin-2(1H)-one were also identified in the infusion (Table 2; Figure 8).
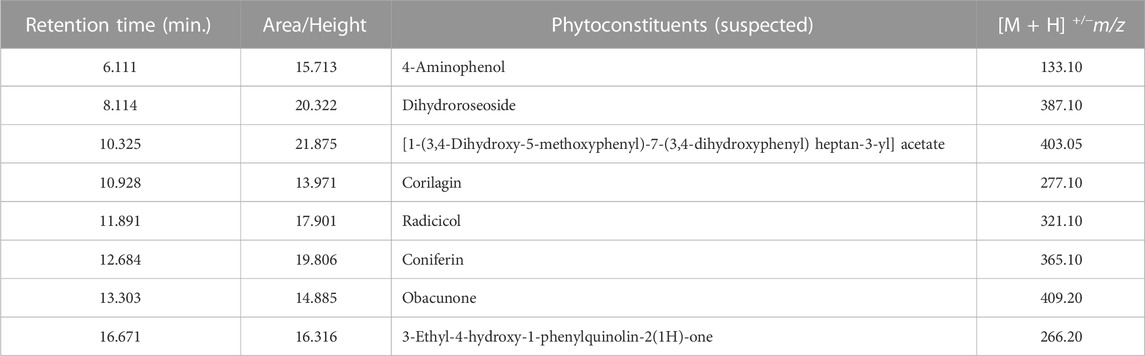
TABLE 2. Liquid Chromatography Mass Spectrometry (LC-MS) identified chemical compounds of L. javanica.
Table 3 shows the free binding energies of the molecular docking of the phytoconstituents of L. javanica and antioxidant standards (ascorbic acid and gallic acid) with catalase and SOD, which indicates that dihydroroseoside and obacunone had the highest binding affinity. Figure 8 gives a representation of 3D and 2D images of the molecular interaction of the active site of catalase with dihydroroseoside (Figure 9A), SOD with dihydroroseoside (Figure 9B) and catalase with obacunone (Figure 9C), and SOD with obacunone (Figure 9D). The compounds molecular interacted with the amino acid residues of the binding pocket of the proteins via hydrogen bonds (H-bond), carbon hydrogen bonds, and vanda Waal forces. Dihydroroseoside shared multiple H-bond with both catalase and SOD, while obacunone shared a single and double H-bond with catalase and SOD, respectively.
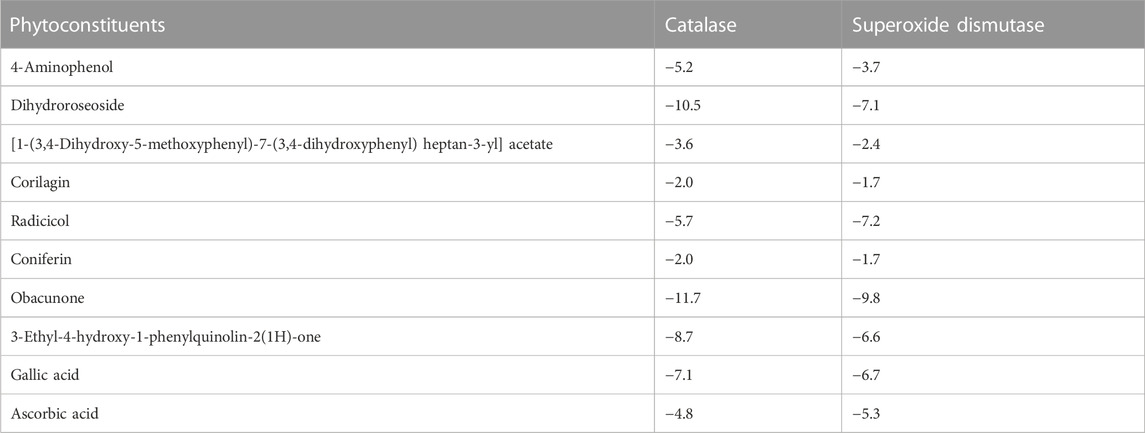
TABLE 3. Binding energies (Kcal/mol) of the phytochemical constituents of L. javanica with antioxidant enzymes.
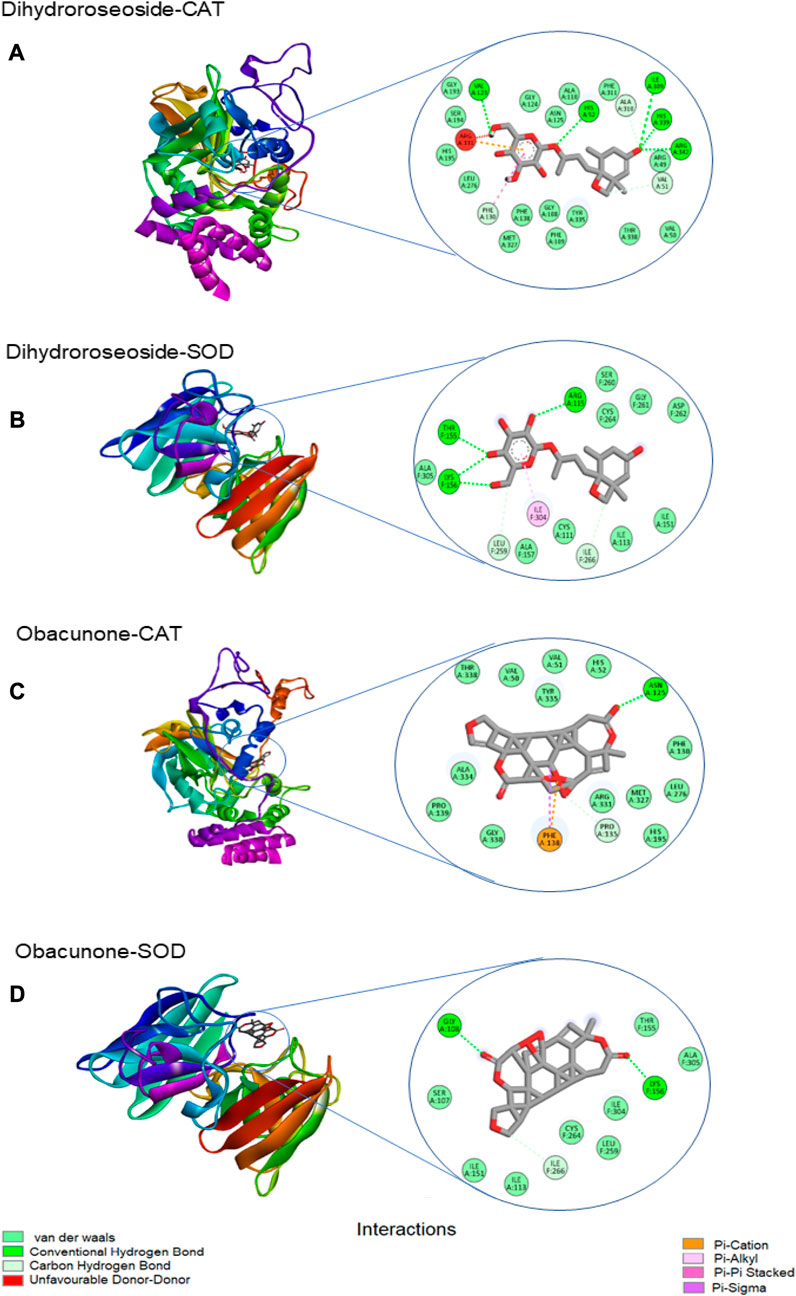
FIGURE 9. The 3D and 2D images of the molecular interaction of dihydroroseoside with the amino active site of (A) catalase and (B) SOD; and 3D and 2D images of the molecular interaction of obacunone with active sites of (C) catalase, and (D) SOD. SOD = superoxide dismutase.
Discussion
Oxidative hepatic injury is a culprit in the pathological mechanisms that leads to the initiation and development of various liver diseases. Liver diseases have been reported to contribute significantly to socio-economic burden, low quality of life and mortality (Li et al., 2015; Asrani et al., 2019). Medicinal plants constitute a variety of secondary metabolites with inherent antioxidant properties that has been widely reported for the prevention and treatment of hepatic oxidative injury and numerous liver diseases (Stickel and Schuppan, 2007; Chukwuma et al., 2019). In this study, the protective effect of L. javanica herbal tea was investigated in iron-induced oxidative hepatic cells injury.
When surplus ROS are generated in the body, they deplete antioxidant levels, leading to a failure to counteract ROS deleterious activities which then result in cellular injury (Pham-Huy et al., 2008). Medicinal plants, including herbal teas are well noted for their rich antioxidant competence, which are ascribed to the presence of prominent phytoconstituents such as phenolics, terpenes, flavonoids, tannins, glycosides, alkaloids and saponins (Mathivha et al., 2020). The antioxidant capacity of these plant constituents are attributed to their ability to act as reducing agents, metal ion chelators, quenchers of singlet oxygen and free radical scavengers (Bendary et al., 2013; Salau et al., 2021). The potent DPPH scavenging activity of L. javanica infusion (Figure 1A), coupled with its ability to reduce Fe3+ to Fe2+ (Figure 1B) indicate the antioxidant pharmacological property of this plant. This can be linked to the high phenolic and flavonoid contents of the plant as well as the presence of LC-MS identified bioactive compounds which include terpenoids, tannins, phenols and glycosides (Figure 8; Table 2). These results corroborates with the previous study of Osunsanmi et al. (2019) who reported that the crude extract of L. javanica’s leaves are embedded with varieties of phytochemicals that are a source of natural antioxidants for treating and managing oxidative stress related diseases.
To further buttress the antioxidant and other possible pharmacological potential of L. javanica as attributed to its phytoconstituents, its effect on iron induced oxidative hepatic injury were assessed. The liver is an organ that is constantly exposed to oxidative attack due to its critical and numerous physiological roles in the body that also involves generation of ROS as by-products and involvement in ROS-generation reactions (Li et al., 2015; Conde de la Rosa et al., 2022). This includes the liver being the major site of iron storage which makes it a major target for iron toxicity (Pietrangelo, 2016). The ability of iron to exist in dual forms: Fe2+ and Fe3+, presents it as a major cofactor in many enzymatic redox reactions as well as a potent prooxidant (Erukainure et al., 2017). Thus, hepatic iron overload and/or iron toxicity leads to the generation of reactive oxygen species and reactive nitrogen species, which are responsible for lipid, protein and nucleic acid peroxidation and proinflammation that ultimately contributes the promotion of hepatic oxidative injury and numerous liver diseases (Videla et al., 2003; Milic et al., 2016). The suppressed levels of GSH and NPSH, SOD and catalase activities and increased levels of MDA and NO on induction of hepatic injury (Figures 3A–E; Figure 4) indicate an occurrence of oxidative stress and proinflammation. This corroborates previous study on Fe2+ induced oxidative injury in Chang liver cells (Chukwuma et al., 2019). Treatment with the L. javanica infusion demonstrated an antioxidant and anti-proinflammatory effect by significantly elevating the levels of GSH and NSPH, SOD and catalase activities and suppressing MDA and NO levels. Thus, indicating a protective effect of L. javanica on oxidative hepatic injury. The strong molecular interaction formed between the LC-MS identified phytoconstituents of L. javanica and the amino residue active sites of catalase and SOD (Figure 9; Table 3) further indicates the antioxidant potency of the infusion. These properties may be attributed to the synergistic activities of the identified phytochemical constituents.
Cholinergic dysfunction typified by elevated cholinesterase activities has been reported as one of the instigators of hepatotoxicity as it incites hepatic cells inflammation (Erukainure et al., 2021b), Altered acetylcholinesterase level has been reported as a useful biomarker for liver disease (García-Ayllón et al., 2006). Studies have linked oxidative stress and proinflammation to a rise in acetylcholinesterase activities (Bondok et al., 2013; Rodríguez-Fuentes et al., 2015). In the present study, the elevated activity of hepatic acetylcholinesterase (Figure 5) on induction of hepatic injury conforms with oxidative stress occurrence (Figure 3) and increased NO level (Figure 4). Cholinesterase inhibitors are targets for protecting against and treating liver diseases (Steinebrunner et al., 2014). The depleted activity of acetylcholinesterase on treatment with L. javanica infusion insinuates a protective effect of the herbal tea against cholinergic dysfunction in oxidative hepatic injury.
The liver plays a pivotal function in the maintenance of glucose homeostasis and metabolism. Perturbations in hepatic glucose level is implicated in development of several liver diseases (Ding et al., 2018; Zhang et al., 2019). Oxidative stress contributes to disturbances in carbohydrate metabolism, which can lead to perturbed energy metabolism (Yazdi et al., 2019). Increased activities of enzymes involved in hepatic gluconeogenesis and glycogenolysis leads to the generation of excess glucose. Glucose toxicity characterised by hepatic glucose accumulation promotes hepatic oxidative stress which results in severe liver oxidative injury and eventual hepatic cell death (Chandrasekaran et al., 2012; Mota et al., 2016). Reports have shown the protective property of various plant-based antioxidants in scavenging free radical and the improvement of liver carbohydrate metabolism (Yazdi et al., 2019; Erukainure et al., 2021a; Olofinsan et al., 2022). This is in accordance with the ability of L. javanica herbal infusion to significantly reduce the activities of glucose-6-phosphatase, fructose-1,6-bisphosphatase and glycogen phosphorylase in oxidative hepatic injury (Figures 6A–C), suggesting a hepatoprotective and hepatic metabolic function-improving potential of the herbal tea infusion.
The damaging effect of oxidative stress in the development of liver diseases cannot be overemphasized. ROS can induce lipid peroxidation by attacking the polyunsaturated fatty acids of lipid membrane and impair cellular functions (Barrera, 2012). Increased level of lipase activity in the liver has been reported in hepatotoxicity due to its catalyzed excessive breakdown of triglycerides, which causes accumulation of hepatic free fatty acids (Erukainure et al., 2021a). Thus, oxidative attack of vulnerable fatty acids progresses oxidative injury. Cytotoxic products generated from hepatic lipid peroxidation such as malondialdehyde (MDA) have been implicated in hepatic fibrogenesis (Koruk et al., 2004). The elevation of lipase activity on induction of oxidative injury (Figure 7) with concomitant increased MDA level (Figure 3D) may indicate progressive oxidative hepatic injury and development of liver disease. The ability of L. javanica infusion to significantly suppress hepatic lipase activity may further suggest the protective effect of the herbal infusion against oxidative hepatic injury.
Phytopharmaceuticals play a key role in medical practice for various diseases treatment and management. However, in addition to their therapeutic properties, many medicinal herbs may contain potential toxic properties that may cause more harm to human health (Lombardi et al., 2017). Cytotoxicity studies is thus a crucial step in determining the safety and therapeutic properties of plants and plant-derived compounds for oral pharmacological agent development (Beseni et al., 2022). The cell viability effect of L. javanica infusion (Figure 2) portrays a non-cytotoxic effect on liver cells. Thus, implying the safety of the L. javanica herbal tea on hepatic cells.
Conclusion
Results from this study demonstrated that L. javanica conferred hepatoprotection against oxidative hepatic injury by mitigating oxidative stress and cholinergic dysfunction as well as improved impaired glucogenic and lipase enzymes activities. These biological activities may be attributed to the synergistic effect of the identified phytoconstituents. Thus, these results may support the ingestion of L. javanica herbal tea as potential curative agent for liver diseases. Further in vivo and molecular studies are required to unravel the mechanisms by which L. javanica brings about its hepatoprotective effect in hepatic oxdative injury. The limitation of the present study is the non-use of commercial standard for the LC-MS analysis. We therefore propose the use commercially available standards for future characterization of L. javanica phytoconstituents.
Data availability statement
The original contributions presented in the study are included in the article/Supplementary material, further inquiries can be directed to the corresponding author.
Author contributions
VS: conceptualization; data curation; investigation; methodology; writing-original draft. OE: formal analysis; methodology; writing-review and editing. KO: data curation; methodology; writing-review and editing. RS: methodology; writing-review and editing. MM: resources; supervision; writing-review and editing. All authors contributed to the article and approved the submitted version.
Acknowledgments
VS acknowlegdes University of the Free State, Bloemfontein, South Africa for Postdoctoral Fellowship Grant (2022162164). MM acknowledges the funding support from the IK-based Technology Innovations at the Department of Science and Innovation.
Conflict of interest
The authors declare that the research was conducted in the absence of any commercial or financial relationships that could be construed as a potential conflict of interest.
Publisher’s note
All claims expressed in this article are solely those of the authors and do not necessarily represent those of their affiliated organizations, or those of the publisher, the editors and the reviewers. Any product that may be evaluated in this article, or claim that may be made by its manufacturer, is not guaranteed or endorsed by the publisher.
References
Asrani, S. K., Devarbhavi, H., Eaton, J., and Kamath, P. S. (2019). Burden of liver diseases in the world. J. hepatology 70, 151–171. doi:10.1016/j.jhep.2018.09.014
Balogun, F., and Ashafa, A. (2017). Aqueous root extracts of Dicoma anomala (Sond) extenuates postprandial hyperglycaemia in vitro and its modulation on the activities of carbohydrate-metabolizing enzymes in streptozotocin-induced diabetic Wistar rats. South Afr. J. Bot. 112, 102–111. doi:10.1016/j.sajb.2017.05.014
Barrera, G. 2012. Oxidative stress and lipid peroxidation products in cancer progression and therapy. China, International Scholarly Research Notices,
Bendary, E., Francis, R., Ali, H., Sarwat, M., and el Hady, S. (2013). Antioxidant and structure–activity relationships (SARs) of some phenolic and anilines compounds. Ann. Agric. Sci. 58, 173–181. doi:10.1016/j.aoas.2013.07.002
Benzie, I. F., and Strain, J. J. (1996). The ferric reducing ability of plasma (FRAP) as a measure of “antioxidant power”: The FRAP assay. Anal. Biochem. 239, 70–76. doi:10.1006/abio.1996.0292
Beseni, B. K., Olofinsan, K. A., Salau, V. F., Erukainure, O. L., and Islam, M. S. (2022). Rhus longipes (Engl) infusions improve glucose metabolism and mitigate oxidative biomarkers in ferrous sulfate-induced renal injury. Asian Pac. J. Trop. Biomed. 12, 453. doi:10.4103/2221-1691.360561
Bondok, R. S., Ahmed, M. A., Soliman, N. B. E., el-Shokry, M. H., Ali, R. M., Fahmy, H. F., et al. (2013). The effect of cholinesterase inhibition on liver dysfunction in experimental acute liver failure. Egypt. J. Crit. Care Med. 1, 51–59. doi:10.1016/j.ejccm.2013.05.002
Chandrasekaran, K., Swaminathan, K., Mathan Kumar, S., Clemens, D. L., and Dey, A. (2012). In vitro evidence for chronic alcohol and high glucose mediated increased oxidative stress and hepatotoxicity. Alcohol. Clin. Exp. Res. 36, 1004–1012. doi:10.1111/j.1530-0277.2011.01697.x
Chang, C.-C., Yang, M.-H., Wen, H.-M., and Chern, J.-C. (2002). Estimation of total flavonoid content in propolis by two complementary colometric methods. J. food drug analysis 10. doi:10.38212/2224-6614.2748
Changlian, P., Shaowei, C., Zhifang, L., and Guizhu, L. (2000). Detection of antioxidative capacity in plants by scavenging organic free radical DPPH. Sheng wu hua xue yu Sheng wu wu li jin Zhan 27, 658–661.
Chen, Z., Tian, R., She, Z., Cai, J., and Li, H. (2020). Role of oxidative stress in the pathogenesis of nonalcoholic fatty liver disease. Free Radic. Biol. Med. 152, 116–141. doi:10.1016/j.freeradbiomed.2020.02.025
Chowdhury, P., and Soulsby, M. (2002). Lipid peroxidation in rat brain is increased by simulated weightlessness and decreased by a soy-protein diet. Ann. Clin. Laboratory Sci. 32, 188–192.
Chukwuma, C. I., Matsabisa, M. G., Rautenbach, F., Rademan, S., Oyedemi, S. O., Chaudhary, S. K., et al. (2019). Evaluation of the nutritional composition of Myrothamnus flabellifolius (Welw) herbal tea and its protective effect against oxidative hepatic cell injury. J. food Biochem. 43, e13026. doi:10.1111/jfbc.13026
Cichoż-Lach, H., and Michalak, A. (2014). Oxidative stress as a crucial factor in liver diseases. World J. gastroenterology WJG 20, 8082–8091. doi:10.3748/wjg.v20.i25.8082
Conde De la Rosa, L., Goicoechea, L., Torres, S., Garcia-Ruiz, C., and Fernandez-Checa, J. C. (2022). Role of oxidative stress in liver disorders. Livers 2, 283–314. doi:10.3390/livers2040023
Dhiman, A., Nanda, A., and Ahmad, S. (2012). A recent update in research on the antihepatotoxic potential of medicinal plants. Zhong xi yi jie he xue bao= J. Chin. Integr. Med. 10, 117–127. doi:10.3736/jcim20120201
Ding, H.-R., Wang, J.-L., Ren, H.-Z., and Shi, X.-L. 2018. Lipometabolism and glycometabolism in liver diseases. BioMed Res. Int., 2018, 1287127, doi:10.1155/2018/1287127
Ellman, G. L., Courtney, K. D., Andres, V., and Featherstone, R. M. (1961). A new and rapid colorimetric determination of acetylcholinesterase activity. Biochem. Pharmacol. 7, 88–95. doi:10.1016/0006-2952(61)90145-9
Ellman, G. L. (1959). Tissue sulfhydryl groups. Archives Biochem. biophysics 82, 70–77. doi:10.1016/0003-9861(59)90090-6
Erukainure, O. L., Matsabisa, M. G., Salau, V. F., Oyedemi, S. O., Oyenihi, O. R., Ibeji, C. U., et al. (2021a). Cannabis sativa L(var Indica) exhibits hepatoprotective effects by modulating hepatic lipid profile and mitigating gluconeogenesis and cholinergic dysfunction in oxidative hepatic injury. Front. Pharmacol 12, 705402. doi:10.3389/fphar.2021.705402
Erukainure, O. L., Mopuri, R., Oyebode, O. A., Koorbanally, N. A., and Islam, M. S. (2017). Dacryodes edulis enhances antioxidant activities, suppresses DNA fragmentation in oxidative pancreatic and hepatic injuries; and inhibits carbohydrate digestive enzymes linked to type 2 diabetes. Biomed. Pharmacother. 96, 37–47. doi:10.1016/j.biopha.2017.09.106
Erukainure, O. L., Sanni, O., Salau, V. F., Koorbanally, N. A., and Islam, M. S. (2021b). Cola nitida (kola nuts) attenuates hepatic injury in type 2 diabetes by improving antioxidant and cholinergic dysfunctions and dysregulated lipid metabolism. Endocr. Metabolic Immune Disorders-Drug Targets (Formerly Curr. Drug Targets-Immune, Endocr. Metabolic Disord. 21, 688–699. doi:10.2174/1871530320666200628030138
Fouché, G., Cragg, G., Pillay, P., Kolesnikova, N., Maharaj, V., and Senabe, J. (2008). In vitro anticancer screening of South African plants. J. Ethnopharmacol. 119, 455–461. doi:10.1016/j.jep.2008.07.005
Garcia-Ayllon, M.-S., Millan, C., Serra-Basante, C., Bataller, R., and Sáez-Valero, J. (2012). Readthrough acetylcholinesterase is increased in human liver cirrhosis. PLoS One 7, e44598. doi:10.1371/journal.pone.0044598
García-Ayllón, M. S., Silveyra, M. X., Candela, A., Compañ, A., Clària, J., Jover, R., et al. (2006). Changes in liver and plasma acetylcholinesterase in rats with cirrhosis induced by bile duct ligation. Hepatology 43, 444–453. doi:10.1002/hep.21071
Germishuizen, G., Meyer, N., Steenkamp, Y., and Keith, M. (2006). A checklist of South African plants. Southern african botanical diversity network report No. 41. Pretoria: SABONET.
Hadwan, M. H., and Abed, H. N. (2016). Data supporting the spectrophotometric method for the estimation of catalase activity. Data brief 6, 194–199. doi:10.1016/j.dib.2015.12.012
Hong, M., Li, S., Tan, H. Y., Wang, N., Tsao, S.-W., and Feng, Y. (2015). Current status of herbal medicines in chronic liver disease therapy: The biological effects, molecular targets and future prospects. Int. J. Mol. Sci. 16, 28705–28745. doi:10.3390/ijms161226126
Kakkar, P., Das, B., and Viswanathan, P. (1984). A modified spectrophotometric assay of superoxide dismutase. Indian J. Biochem. Biophys. 21, 130–132.
Kim, Y. S., Lee, Y. M., Kim, H., Kim, J., Jang, D. S., Kim, J. H., et al. (2010). Anti-obesity effect of morus bombycis root extract: Anti-lipase activity and lipolytic effect. J. Ethnopharmacol. 130, 621–624. doi:10.1016/j.jep.2010.05.053
Koruk, M., Taysi, S., Savas, M. C., Yilmaz, O., Akcay, F., and Karakok, M. (2004). Oxidative stress and enzymatic antioxidant status in patients with nonalcoholic steatohepatitis. Ann. Clin. Laboratory Sci. 34, 57–62.
Li, A.-N., Li, S., Zhang, Y.-J., Xu, X.-R., Chen, Y.-M., and Li, H.-B. (2014). Resources and biological activities of natural polyphenols. Nutrients 6, 6020–6047. doi:10.3390/nu6126020
Li, S., Tan, H.-Y., Wang, N., Zhang, Z.-J., Lao, L., Wong, C.-W., et al. (2015). The role of oxidative stress and antioxidants in liver diseases. Int. J. Mol. Sci. 16, 26087–26124. doi:10.3390/ijms161125942
Lombardi, V. R., Carrera, I., and Cacabelos, R. 2017. In vitro screening for cytotoxic activity of herbal extracts. Germany, Evidence-Based Complementary and Alternative Medicine.
Lukwa, N., Molgaard, P., Furu, P., and Bogh, C. (2009). Lippia javanica (burm F) Spreng: Its general constituents and bioactivity on mosquitoes. Trop. Biomed. 26, 85–91.
Maroyi, A. 2017. Lippia javanica (burm F) Spreng.: Traditional and commercial uses and phytochemical and pharmacological significance in the african and indian subcontinent. Evidence-based complementary Altern. Med., 2017, 1.doi:10.1155/2017/6746071
Mathivha, P. L., Msagati, T. A., Thibane, V. S., and Mudau, F. N. (2020). Herbal medicine in India: Indigenous knowledge, practice, innovation and its value, 281–301.Phytochemical analysis of herbal teas and their potential health, and food safety benefits: A review
Mcdonald, S., Prenzler, P. D., Antolovich, M., and Robards, K. (2001). Phenolic content and antioxidant activity of olive extracts. Food Chem. 73, 73–84. doi:10.1016/s0308-8146(00)00288-0
Miksztowicz, V., Lucero, D., Zago, V., Cacciagiú, L., Lopez, G., Gonzalez Ballerga, E., et al. (2012). Hepatic lipase activity is increased in non-alcoholic fatty liver disease beyond insulin resistance. Diabetes/metabolism Res. Rev. 28, 535–541. doi:10.1002/dmrr.2312
Milic, S., Mikolasevic, I., Orlic, L., Devcic, E., Starcevic-Cizmarevic, N., Stimac, D., et al. (2016). The role of iron and iron overload in chronic liver disease. Med. Sci. Monit. Int. Med. J. Exp. Clin. Res. 22, 2144–2151. doi:10.12659/msm.896494
Mota, M., Banini, B. A., Cazanave, S. C., and Sanyal, A. J. (2016). Molecular mechanisms of lipotoxicity and glucotoxicity in nonalcoholic fatty liver disease. Metabolism 65, 1049–1061. doi:10.1016/j.metabol.2016.02.014
Mujovo, S. F., Hussein, A. A., Meyer, J. M., Fourie, B., Muthivhi, T., and Lall, N. (2008). Bioactive compounds from Lippia javanica and Hoslundia opposita. Nat. Prod. Res. 22, 1047–1054. doi:10.1080/14786410802250037
Olofinsan, K. A., Erukainure, O. L., Brian, B. K., and Islam, M. S. (2022). Harpephyllum caffrum stimulates glucose uptake, abates redox imbalance and modulates purinergic and glucogenic enzyme activities in oxidative hepatic injury. Asian Pac. J. Trop. Biomed. 12, 9. doi:10.4103/2221-1691.333209
Osunsanmi, F. O., Zharare, G. E., and Opoku, A. R. (2019). Phytochemical constituents and antioxidant potential of crude extracts from Lippia Javanica (Burm. f.) Spreng Leaves Pharmacogn. J. 11, 803–807. doi:10.5530/pj.2019.11.128
Pham-Huy, L. A., He, H., and Pham-Huy, C. (2008). Free radicals, antioxidants in disease and health. Int. J. Biomed. Sci. IJBS 4, 89–96.
Rodríguez-Fuentes, G., Rubio-Escalante, F. J., Noreña-Barroso, E., Escalante-Herrera, K. S., and Schlenk, D. (2015). Impacts of oxidative stress on acetylcholinesterase transcription, and activity in embryos of zebrafish (Danio rerio) following Chlorpyrifos exposure. Comp. Biochem. Physiology Part C Toxicol. Pharmacol. 172, 19–25. doi:10.1016/j.cbpc.2015.04.003
Salau, V. F., Erukainure, O. L., Koorbanally, N. A., and Islam, M. S. (2021). Ferulic acid promotes muscle glucose uptake and modulate dysregulated redox balance and metabolic pathways in ferric-induced pancreatic oxidative injury. J. Food Biochem. 46, e13641. doi:10.1111/jfbc.13641
Shahriar, M., Chowdhury, A., Rahman, M., Uddin, M., Al-Amin, M., Rahman, M., et al. (2014). Scientific validation of medicinal plants used by a folk medicinal practitioner of Chuadanga district, Bangladesh. World J. Pharm. Pharm. Sci. (WJPPS) 3, 13–24.
Shikanga, E., Combrinck, S., and Regnier, T. (2010). South African Lippia herbal infusions: Total phenolic content, antioxidant and antibacterial activities. South Afr. J. Bot. 76, 567–571. doi:10.1016/j.sajb.2010.04.010
Steinebrunner, N., Mogler, C., Vittas, S., Hoyler, B., Sandig, C., Stremmel, W., et al. (2014). Pharmacologic cholinesterase inhibition improves survival in acetaminophen-induced acute liver failure in the mouse. BMC Gastroenterol. 14, 148–8. doi:10.1186/1471-230X-14-148
Stepanova, M., De Avila, L., Afendy, M., Younossi, I., Pham, H., Cable, R., et al. (2017). Direct and indirect economic burden of chronic liver disease in the United States. Clin. Gastroenterology Hepatology 15, 759–766. e5. doi:10.1016/j.cgh.2016.07.020
Stickel, F., and Schuppan, D. (2007). Herbal medicine in the treatment of liver diseases. Dig. liver Dis. 39, 293–304. doi:10.1016/j.dld.2006.11.004
Suleman, Z., Engwa, G. A., Shauli, M., Musarurwa, H. T., Katuruza, N. A., and Sewani-Rusike, C. R. (2022). Neuroprotective effects of Lippia javanica (Burm. F) Spreng. Herbal tea infusion on Lead-induced oxidative brain damage in Wistar rats. BMC Complementary Med. Ther. 22, 4–10. doi:10.1186/s12906-021-03471-3
Tsikas, D. (2005). Methods of quantitative analysis of the nitric oxide metabolites nitrite and nitrate in human biological fluids. Free Radic. Res. 39, 797–815. doi:10.1080/10715760500053651
Upadhyay, J., Tiwari, N., Durgapal, S., and Farzaei, M. H. (2022). Antioxidants and liver diseases. Antioxidants effects in health. Germany: Elsevier.
Videla, L. A., Fernández, V., Tapia, G., and Varela, P. (2003). Oxidative stress-mediated hepatotoxicity of iron and copper: Role of kupffer cells. Biometals 16, 103–111. doi:10.1023/a:1020707811707
Wang, J., Wang, W., Kollman, P. A., and Case, D. A. (2006). Automatic atom type and bond type perception in molecular mechanical calculations. J. Mol. Graph. Model. 25, 247–260. doi:10.1016/j.jmgm.2005.12.005
Yazdi, H. B., Hojati, V., Shiravi, A., Hosseinian, S., Vaezi, G., and Hadjzadeh, M. A. R. (2019). Liver dysfunction and oxidative stress in streptozotocin-induced diabetic rats: Protective role of artemisia turanica. J. pharmacopuncture 22, 109–114. doi:10.3831/KPI.2019.22.014
Keywords: oxidative stress, hepatotoxicity, gluconeogenesis, antioxidants, cholinergic enzyme
Citation: Salau VF, Erukainure OL, Olofinsan KA, Schoeman RLS and Matsabisa MG (2023) Lippia javanica (Burm. F.) Herbal Tea: Modulation of Hepatoprotective Effects in Chang Liver Cells via Mitigation of Redox Imbalance and Modulation of Perturbed Metabolic Activities. Front. Pharmacol. 14:1221769. doi: 10.3389/fphar.2023.1221769
Received: 12 May 2023; Accepted: 27 July 2023;
Published: 07 August 2023.
Edited by:
Mansour Sobeh, Mohammed VI Polytechnic University, MoroccoReviewed by:
Sultan Ayesh Mohammed Saghir, Al Hussein Bin Talal University, JordanOluwafemi Adeleke Ojo, Bowen University, Nigeria
Rogers Mwakalukwa, Muhimbili University of Health and Allied Sciences, Tanzania
Copyright © 2023 Salau, Erukainure, Olofinsan, Schoeman and Matsabisa. This is an open-access article distributed under the terms of the Creative Commons Attribution License (CC BY). The use, distribution or reproduction in other forums is permitted, provided the original author(s) and the copyright owner(s) are credited and that the original publication in this journal is cited, in accordance with accepted academic practice. No use, distribution or reproduction is permitted which does not comply with these terms.
*Correspondence: Motlalepula G. Matsabisa, bWF0c2FiaXNhbWdAdWZzLmFjLnph