- 1School of Public Health and Management, Binzhou Medical University, Yantai, Shandong, China
- 2Key Laboratory of Molecular Pharmacology and Drug Evaluation, School of Pharmacy, Ministry of Education, Collaborative Innovation Center of Advanced Drug Delivery System and Biotech Drugs in Universities of Shandong, Yantai University, Yantai, Shandong, China
- 3School of Pharmacy, Binzhou Medical University, Yantai, China
- 4Medicine and Pharmacy Research Center, Binzhou Medical University, Yantai, Shandong, China
- 5WestChina-Frontier PharmaTech Co., Ltd., Chengdu, Sichuan, China
Opiates produce analgesia via G-protein signaling, and adverse effects, such as respiratory depression and decreased bowel motility, by β-arrestin pathway. Oliceridine, a G protein-biased MOR agonist, only presents modest safety advantages as compared to other opiates in clinical trials, possibly due to its limited bias. Our previous study shown that LPM3480392, a full MOR biased agonist, is selective for the Gi pathway over the β-arrestin-2. In the present article, we evaluated the subacute toxicity of LPM3480392 in rats. The rats were administered with control article or LPM3480392 0.6, 1.2 or 2.4 mg/kg/day for 4 consecutive weeks followed by a 4-week recovery phase. Intravenous infusion was conducted at tail vein at 0.2, 0.4 or 0.8 mg/kg/day with a dosing volume of 10 mL/kg and 5 min/rat/dose, three times a day with an interval of approximately 4 h. The concomitant toxicokinetics study was conducted. Two unscheduled rats at 2.4 mg/kg/day died with no clear cause. For the scheduled necropsy, the major effects were associated with the MOR agonist-related pharmacodynamic properties of LPM3480392 (e.g., increased activity, increased muscle tone; decreased food consumption and body weight gain; and clinical chemistry changes related with decreased food consumption) in three LPM3480392 groups. In addition, LPM3480392 at 2.4 mg/kg/day also induced deep respiration and histopathology changes in testis and epididymis in sporadic individual rats. However, different from other opiates, LPM3480392 presents weak/no immunosuppression and the decreased adrenal gland weight, which may be due to LPM3480392’ full MOR bias. At the end of recovery phase, all findings were recovered to some extent or completely. In the toxicokinetics study, the dose-dependent elevation of drug exposure was observed, which partly explained the toxicity of high dose. In summary, LPM3480392 has exhibited good safety characteristics in this subacute toxicity study in rats.
1 Introduction
Acute postoperative pain is experienced in more than 80% of all surgical patients, with the moderate to severe intensity in at least 75% of these patients (Gan et al., 2014; Gan, 2017). Opiates, especially µ-opioid receptor (MOR) agonists such as morphine and fentanyl, are first-line treatment options for the treatment of moderate to severe pain (Bostrom et al., 1997; Tan and Habib, 2021). Although opiates are effective analgesics, they also induced severe adverse effects (SAE) such as addiction, respiratory suppression, and constipation, thereby limiting their clinical utilization (Del Vecchio et al., 2017; Zhuang et al., 2022). Opiate-induced side effects result in significant morbidity and mortality. For instance, opiate-induced respiratory depression is associated with 15,000 deaths per year, the majority of which are related to illicit and recreational opiate use (Mattson et al., 2021; Tan and Habib, 2021; Zhuang et al., 2022).
As a G protein-coupled receptor (GPCR), MOR has been revealed as the main receptor for both the analgesic and adverse effects of morphine (Matthes et al., 1996). Signaling of MOR is primarily transduced through Gi to inhibit cAMP production. MOR can also signal through β-arrestin upon the receptor activation (Zhuang et al., 2022). The activation of the Gi signaling is responsible for analgesia, while the activation of the β-arrestin pathway contributes to unwanted effects of MOR activation such as respiratory depression and constipation (Bohn et al., 1999; Bohn et al., 2000; Raehal et al., 2005). Therefore, by selectively acting on the Gi signaling pathway in preference to the β-arrestin pathway, there is an interesting drug development strategy with preserving the analgesic activity and avoiding unwanted MOR-associated opiate side effects.
Biased agonism refers to the ability of compounds to drive preferred signaling pathways and avoid adverse signaling pathways in a ligand-dependent manner for some GPCRs (Chan et al., 2017; Daugvilaite et al., 2017; Yang et al., 2022). Some studies have indicated that MOR agonists that are functionally selective for the Gi pathway over the β-arrestin-2, which may elicit analgesia while reducing some side-effects of opiates. Several MOR agonists with negligible β-arrestin activities and potentially with better therapeutic windows than morphine and fentanyl have been identified and characterized, including oliceridine (TRV130), PZM21, and SR17018 (DeWire et al., 2013; Manglik et al., 2016; Schmid et al., 2017; Zhuang et al., 2022). Oliceridine (Olinvyk® Trevena, PA, United States) was approved by the US Food and Drug Administration (FDA) for the treatment of moderate to severe pain on 8 Aug 2020 (Goudra, 2022), which is a G protein-biased MOR agonist that preferentially activates the inhibitory Gi signaling pathway over β-arrestin-2 (Chen et al., 2013). However, in phase III clinical trials, TRV130 did not present its superiority to morphine in terms of the respiratory depression in humans (Kliewer et al., 2020). Recently, a question was generated that the degree of bias will impact the separation of analgesia and respiratory depression side effects (Schmid et al., 2017). Therefore, full MOR biased agonists need to be designed for obtaining higher MOR bias with enough safety margin.
In the previous study, we rationally designed and synthesized a series of potent highly biased MOR agonists through the modification and structure-activity relationship study of TRV130 (Yang et al., 2022). Among these compounds, LPM3480392 demonstrated improved in vitro biased agonism (EC50 = 0.35 nM, Emax = 91.4%) without β-arrestin-2 recruitment activity (EC50 > 30,000 nM, Emax = 1.6%), good brain penetration, a favorable pharmacokinetic profile and produced potent antinociceptive effect with reduced respiratory suppression as compared to TRV130.
Previously, we have completed a series of toxicity studies in order to support the clinical trials in agreement with the 21 CFR Part 58 of US FDA, Good Laboratory Practice (GLP) regulations. Until now, LPM3480392 has completed phase I clinical trials (CTR20210370; CTR20212865) and is currently under phase II clinical trial (CTR20222358) as an analgesic for the treatment of moderate to severe pain. In this article, we present the subacute toxicity study for LPM3480392 in rats.
2 Materials and methods
2.1 Materials
LPM3480392 maleate injection (6.45 mg/5 mL) with a chemical purity of >97.9% was provided by the State Key Laboratory of Long-acting and Targeting Drug Delivery Technologies (Yantai, China) (Figure 1).
2.2 Experimental animals
The Sprague Dawley (SD) rats of SPF grade (76 females and 76 males) were from Beijing Vital River Laboratory Animal Co., Ltd. (Production license No.: SCXK (Jing) 016–0011), with the body weight of 132.8–174.6 g and the ages of 6–7 weeks. The rats were supplied with rat/mouse breeding diet ad libitum manufactured by Beijing Keao Xieli Feed Co., Ltd (Production License Number: Jingsi license (2018) 06,073). The animals were housed under 12 h light/dark cycles at 21.24°C–25.55°C in 45.23%–72.01% relative humidity environment. All animals had free access to water and food during the experimental periods. This subacute toxicity study has been conducted in WestChina-Frontier PharmaTech Co., Ltd. (WCFP). Animal experiments complied with the relevant regulations in Institutional Animal Care and Use Committee (IACUC) in WCFP.
2.3 Study design
The rats were assigned to 4 groups, including control group, and three LPM3480392 injection groups, with 19 rats/sex in each group. The rats were administered with control article (0.9% sodium chloride injection) or LPM3480392 injection 0.6, 1.2 or 2.4 mg/kg/day for 4 consecutive weeks followed by a 4-week recovery phase. Intravenous infusion was conducted at tail vein at 0.2, 0.4 or 0.8 mg/kg with a dosing volume of 10 mL/kg and 5 min/rat/dose using micro-injection pump, three times a day with an interval of approximately 4 h. The first dosing day was defined as Day 1, and the day after the last dosing was defined as Recovery day 1. At 1 day after the last dosing, 10 rats per sex in each group (9 males and 10 females in 2.4 mg/kg/day group) were anesthetized with pentobarbital sodium (i.p., 60 mg/kg) followed by abdominal aorta exsanguination for a gross necropsy based on AVMA Guidelines for the Euthanasia of Animals: 2020 Edition (the American Veterinary Medical Association, 2020). At the end of recovery phase, the 5 animals per sex in each group (5 males and 4 females in 2.4 mg/kg/day group) were sacrificed. For the toxicokinetics study, 4 rats/sex/group were used in three LPM3480392 injection groups.
2.4 Preparation and analysis of dose formulation
LPM3480392 injection was diluted with 0.9% NaCl injection to the required concentration under the aseptic condition. The dose formulations were prepared at least once every 8 days. The prepared dose formulations were used within 192 h when stored at 15°C–25°C. Dose formulations in control group and LPM3480392 groups were analyzed for the first and last dosing. The sample (0.100 mL) was collected from the middle layer of control formulation, or LPM3480392 injection dose formulations at each concentration. The absence of LPM3480392 should be confirmed in control formulations. The relative error (RE%) between detected result and nominal value should be within ±10% for LPM3480392 injection dose formulations at different concentrations.
2.5 Clinical observation
For all surviving animals, a morning and an afternoon observation every day were conducted for the moribundity and death. Daily observation was carried out once within 1 h after each dosing in dosing phase, and at least once daily in recovery phase, including mental status, food and water consumption, hair, discharge, mortality and other toxic reactions. Detailed observation was performed once every week, including appearance, movement, mental status, gland secretions, skin and mucosa color, respiration, genitals, mortality and other toxic reactions.
2.6 Body weight
Body weight was recorded twice a week during the dosing phase and then once a week during the recovery phase. Terminal body weight was recorded before each necropsy for calculating the organ weight ratios.
2.7 Food consumption
Food consumption of all surviving animals was recorded twice a week during the dosing phase and then once a week in last 2 weeks of recovery phase.
2.8 Ophthalmic examination
The examination was conducted on rats in control and 2.4 mg/kg/day groups at the end of dosing phase (Day 27) and at the end of recovery phase (Recovery day 27). The mydriasis (eye instillation, 1-2 drops/eye) was conducted using Mydrin-P (compound tropicamide eye drops) before examination. Binocular indirect ophthalmoscope was used to examine conjunctiva, cornea, iris, lens, anterior chamber, posterior chamber and fundus.
2.9 Clinical pathology
The blood samples were collected via abdominal aorta after anaesthetization with pentobarbital sodium (i.p., 60 mg/kg) on the scheduled necropsy days of dosing phase and recovery phase. Hematological examination was performed using ADVIA 2120i hematology analyzer (Germany) and CS-5100 automatic coagulaiton analyzer (Japan) (Table 1). The clinical chemistry examination was assessed using Roche cobas 6000/c501 automatic biochemistry analyzer (Switzerland) (Table 1).
Urine samples were directly collected from the metabolic cage at the end of dosing phase (Day 26) and at the end of recovery phase (Recovery Day 28), respectively. Urinalysis parameters in Table 1 were evaluated using CLINITEK Atlas automatic urine analyzer (Germany).
2.10 Necropsy
Complete gross necropsies were conducted on all surviving animals at the end of treatment (the day after the last dosing) and recovery periods. The following selected organs were weighed and evaluated in terms of organ/body or organ/brain weight ratios: brain, kidneys, adrenals, testes, ovaries, uterus, epididymides, thymus, spleen, heart and liver. Paired organs were weighed together. Organ-to-body weight ratio = Organ weight(g)/Body weight(g) × 100%, and Organ-to-brain weight ratio = Organ weight(g)/Brain weight(g) × 100%.
2.11 Histopathology
The tissues in the Table 2 were preserved in 10% neutral phosphate-buffered formalin. Bilateral eyes, optic nerves, testes, epididymides and Harderian glands were fixed in modified Davidson’s solution. For all the above tissues in all groups, sampling, paraffin embedding, sectioning and hematoxylin-eosin staining was performed, followed by histopathology examination microscopically with light microscopy.
2.12 Handling of dead animals
The animals found dead were recorded for the observation or death time, and weighed followed by a complete postmortem examination to explore the cause of death. All required organs and tissue as listed in the Section 2.11 and other tissues with gross lesions were performed with histopathology examination. Animals found dead which could not be examined immediately were temporarily refrigerated.
2.13 Toxicokinetics
Blood samples from animals in LPM3480392 groups were collected at predose and 2 min, 30 min, 1 h and 4 h postdose of the 1st dosing on Days 1 and 28; at 2 min and 4 h postdose of the 2nd dosing on Days 1 and 28; at 2 min, 1 h and 4 h postdose the 3rd dosing on Days 1 and 28. Animals in control group were sampled at predose and 2 min postdose of the 1st dosing on Days 1 and 28. Approximately 0.36 mL of blood was collected from the jugular vein of surviving TK animals at each time point. Whole blood samples were anticoagulated with EDTA-K2 and centrifuged at 2°C–8°C and 1800 × g for 10 min. The harvested plasma was stored below −66°C before the analysis. LC-MS/MS methods (liquid chromatograph/mass spectrometer) were used to detect concentrations of LPM3480392 at each time point. TK parameters (AUC, Cmax, Tmax) were calculated by Phoenix WinNonlin (Pharsight) 8.2. The detection instruments include ultra-performance liquid chromatography (Waters, United States ACQUITY UPLC I -CLASS) and triple quadrupole tandem mass spectrometer (Waters, United States, Xevo TQ-S).
2.14 Statistical analysis
Quantitative parameters were described as means ± standard deviation (X ± SD). The parameters included body weight, food consumption, hematology, clinical chemistry, urinary specific gravity (only at the end of recovery phase), organ weight and ratios, et al. Qualitative data (binomial category, unordered multi-category and ordinal multi-category) of urinalysis (including urinary specific gravity at the end of dosing phase) were presented as observed counts (frequency). When sample size was less than three, raw data of that group were directly presented without statistical comparison.
Statistical analysis was performed between each LPM3480392 group and the control group. Levene’s test was used to analyse the variance homogeneity of quantitative data. In the case of homogeneity of variance (p > 0.05), they were evaluated using one-way analysis of variance (ANOVA); in the case of heterogeneity of variances (p ≤ 0.05), Kruskal-Wallis (K-W) H test was used. And if ANOVA was with significant difference (p ≤ 0.05), Dunnett’s t-test (Dunnett) was used for pairwise comparisons; if ANOVA was not significant (p > 0.05), the statistical analysis was completed. When K-W H test was significant (p ≤ 0.05), Mann-Whitney (M-W) U test was used for pairwise comparisons; if K-W H test was not significant (p > 0.05), the statistical analysis was completed. Ordinal multi-category data were analysed by K-W H test. In the case of finding significant difference (p ≤ 0.05), M-W U test was used for pairwise comparison. Binomial category data were analysed by Fisher’s exact probabilities test (EXACT). If there was significant difference (p ≤ 0.05), Fisher EXACT was used for pairwise comparisons. Analyses were performed according to sex at two-tailed probability level (α = 0.05). PRISTIMA 7.2.0 was used for statistical analysis of body weight, hematology, clinical chemistry, organ weight and ratios. Stata/IC 15.0 for Windows was adopted for the statistical analysis of the rest data.
3 Results
3.1 Dose formulation analysis
For LPM3480392 dose formulations at 0.02 mg/mL, 0.04 mg/mL and 0.08 mg/mL prepared before the first dosing, the mean measured concentrations were 0.021 mg/mL, 0.041 mg/mL and 0.078 mg/mL, respectively. The RE% between measured value and nominal value were 5.0%, 2.5% and −2.5%, respectively. For LPM3480392 dose formulations at 0.02 mg/mL, 0.04 mg/mL and 0.08 mg/mL prepared before the last dosing, the mean measured concentrations were 0.019 mg/mL, 0.039 mg/mL and 0.078 mg/mL, respectively. The RE% between measured value and nominal value were −5.0%, −2.5% and −2.5%, respectively. No LPM3480392 injection was detected in control formulations.
3.2 Mortality
Two rats (1 male and 1 female, 2/30 animals) at 2.4 mg/kg/day were found dead on Days 3 and 15, respectively. They showed decreased activity and/or increased muscle tone, sternal recumbent posture after dosing, with no drug-related abnormalities in gross necropsy or histopathology.
3.3 Clinical observation
The rats in 0.6, 1.2 and 2.4 mg/kg/day groups displayed mild to severe increased activity, starting from Days 7, 4 and 1 during the dosing phase, respectively. And the animals in 2.4 mg/kg/day showed decreased activity (18 males, 8 females, 26/36 animals) only a few time points during the dosing phase. Muscle tone was also increased in 1.2 mg/kg/day group (17 males, 16 females, 33/38 animals) during the early stage of dosing phase in the first 5 days, and 2.4 mg/kg/day group (18 males, 18 females, 36/36 animals) during the mostly complete dosing phase, following by the gradually decreased incidence with time.
The rats in 2.4 mg/kg/day group occasionally showed hunchback (1 male, 1/36 animals), piloerection (1 male, 1/36 animals) and deep respiration or rale (4 males, 4/36 animals). During the middle and late stage of dosing phase, sporadic individual rats in 1.2 and 2.4 mg/kg/day groups had fester at the application site of tail or broken tail, with no drug-related abnormalities in histopathology examination, which was maybe caused by mechanical stimulation of long-term operation.
LPM3480392-treated rats showed good general health, normal spontaneous activity, with no other toxic reaction during the recovery phase.
3.4 Body weight
During the dosing phase, body weight gains were dose-dependently decreased in males from Day 16 to Day 27 at 0.6 mg/kg/day, and from Day 6 to Day 27 at 1.2 and 2.4 mg/kg/day. Interestingly, during the recovery phase, at the four time points, body weight gains were deeply reduced in the three LPM3480392 groups (Table 3).
However, in females, body weight gains were increased at every time point during the dosing phase, while the increased degree of body weight gains seem to be less during the recovery phase (Table 4).
3.5 Food consumption
During the dosing phase, at every time points, food consumption in males was dose-dependently reduced. Especially, from Day 10 to Day 17, food consumption was deeply decreased compared with other time points. And, during the recovery phase, decreased food consumption obtained the partial recovery (Table 5).
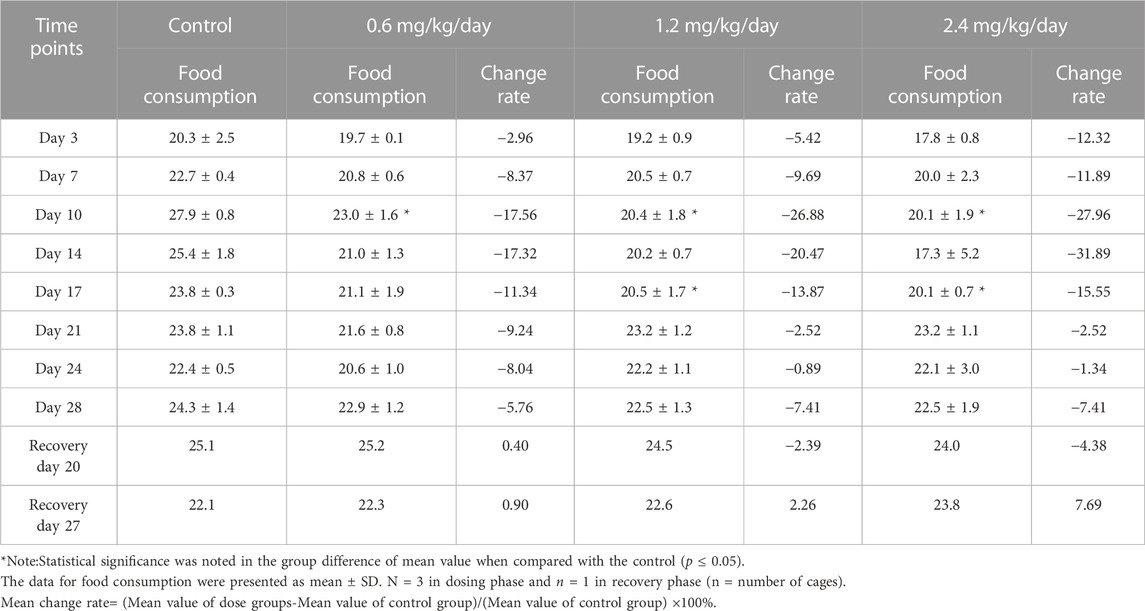
TABLE 5. Effects of LPM3480392 on food consumption (g/animal/cage/day) and mean change rate (%) in male rats.
However, in females, during the dosing phase or the recovery phase, the food consumption was decreased at some time points while increased at the other time points, which did not present obvious pattern (Table 6).
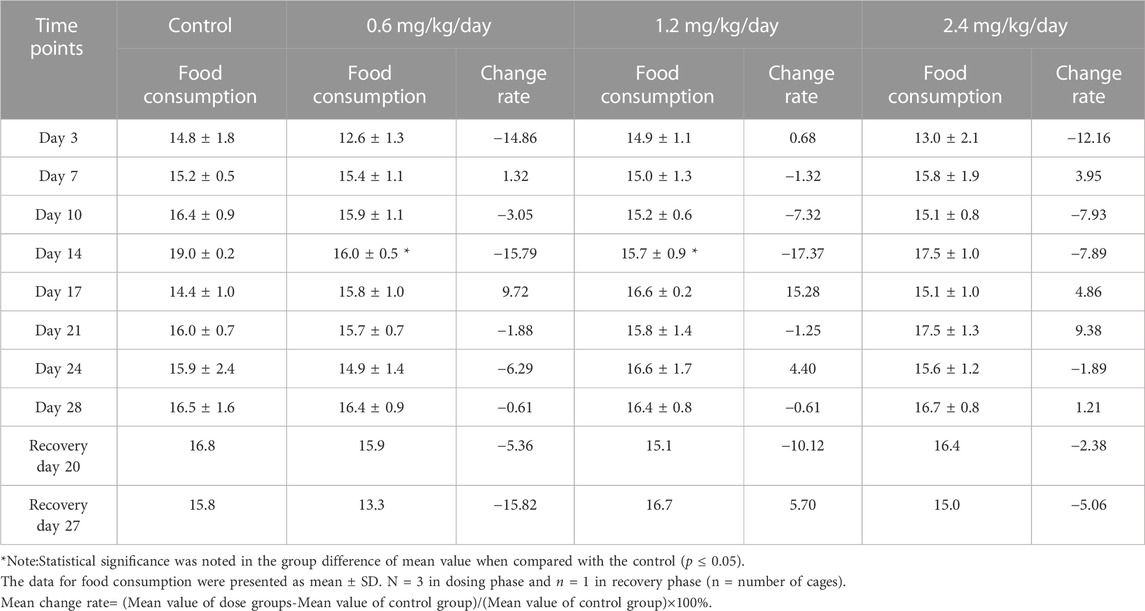
TABLE 6. Effects of LPM3480392 on food consumption (g/animal/cage/day) and mean change rate (%) in female rats.
3.6 Ophthalmic examination
At the end of dosing phase and recovery phase, both genders in 2.4 mg/kg/day group were observed with clear dioptric media and optic disk. The diameter of retinal artery was even. Veins were without apparent dilation. No abnormalities were noted in retina, optic disk, conjunctiva, cornea, iris, lens, anterior chamber or posterior chamber.
3.7 Hematology
At the end of dosing phase, for the males, 2.4 mg/kg/day of LPM3480392 induced the significantly increased RET/RET% and the significantly decreased EOS/EOS% and MONO, and 1.2 mg/kg/day significantly increased RET/RET% and decreased ESO. At the end of recovery phase, 2.4 mg/kg/day significantly increased RET% (Table 7).
At the end of dosing phase, for the females, the significantly decreased MONO% or MONO in 0.6, 1.2 and 2.4 mg/kg/day groups, the significantly decreased RBC in 1.2 and 2.4 mg/kg/day groups, the increased MCV in 1.2 mg/kg/day group and the decreased PLT in 2.4 mg/kg/day group, were observed. At the end of recovery phase, the significantly increased LYM%, and the significantly decreased NEU/NEU%, and MONO% were noted in all LPM3480392 groups (Table 8).
With the exception of the above changes, no significant differences were observed in other parameters in males or females treated with LPM3480392 at the end of dosing phase and recovery phase as compared with the control (p > 0.05).
3.8 Clinical chemistry
At the end of dosing phase, for the males, 0.6, 1.2 and 2.4 mg/kg/day induced the significantly decreased ALB and TP; 1.2 and 2.4 mg/kg/day significantly decreased TG; and 2.4 mg/kg/day significantly increased GLU. At the end of recovery phase, 1.2 and 2.4 mg/kg/day significantly increased A/G, and decreased GLU (Table 9).
At the end of dosing phase, for the females, 0.6, 1.2 and 2.4 mg/kg/day induced the significantly decreased ALB, TP, TG, A/G; 1.2 and 2.4 mg/kg/day significantly decreased TBIL; and 2.4 mg/kg/day significantly decreased CREA, and increased AST, GLU and ALP (Table 10).
With the exception of the above, no significant differences were observed in other clinical chemistry parameters for males or females treated with LPM3480392 at the end of dosing phase and recovery phase as compared with the control (p > 0.05).
3.9 Urinalysis
For the male rats, the grade distribution of NIT at 1.2 and 2.4 mg/kg/day, and grade distribution of KET at 2.4 mg/kg/day were significantly decreased compared with the concurrent control (p ≤ 0.05) at the end of dosing phase (Table 11).
For the female rats, the grade distribution of pH at 2.4 mg/kg/day at end of dosing phase, and at 0.6 mg/kg/day at end of recovery phase were significantly increased compared with the concurrent control (p ≤ 0.05) (Table 11).
With the exception of the above, there were no significant differences in urinary parameters for the males or females treated with LPM3480392 at the end of dosing phase and recovery phase as compared with the control (p > 0.05).
3.10 Organ weight and ratios
For the male rats, at the end of dosing phase, the significantly increased organ-to-body weight ratio of heart at 0.6, 1.2 and 2.4 mg/kg/day, the significantly decreased absolute weight of liver and adrenal gland at 1.2 and 2.4 mg/kg/day, and the significantly decreased absolute weight of epididymides and the organ-to-brain weight ratio of liver at 2.4 mg/kg/day were observed. At the end of recovery phase, only the significantly increased absolute weight of thymus was noted in 0.6 mg/kg/day group (Table 12).
For the female rats, at the end of dosing phase, the significantly decreased organ-to-body weight ratio of brain at 0.6 and 1.2 mg/kg/day, and the significantly decreased organ-to-body weight ratio of adrenal gland at 1.2 mg/kg/day were observed (Table 13).
With the exception of above changes, no significant differences were noted in the absolute weight and ratios of other organs of LPM3480392-treated males or females at the end of dosing phase and recovery phase as compared with the control (p > 0.05).
3.11 Gross necropsy
There were no LPM3480392-related abnormalities in the size, morphology, texture or color of main organs such as brain, heart, liver, spleen, kidney, adrenal gland, gastrointestinal tract, uterus, testes, epididymides at the end of dosing phase and recovery phase.
3.12 Histopathology
Sporadic individual males at 2.4 mg/kg/day were observed with the mild tubular degeneration of the testis (1/9), the marked decreased sperm in the lumen and the minimal to moderate cell debris in the lumen of the epididymis (1/9) at the end of dosing phase. The above changes were not identified at the end of recovery phase (Table 14; Figure 2).
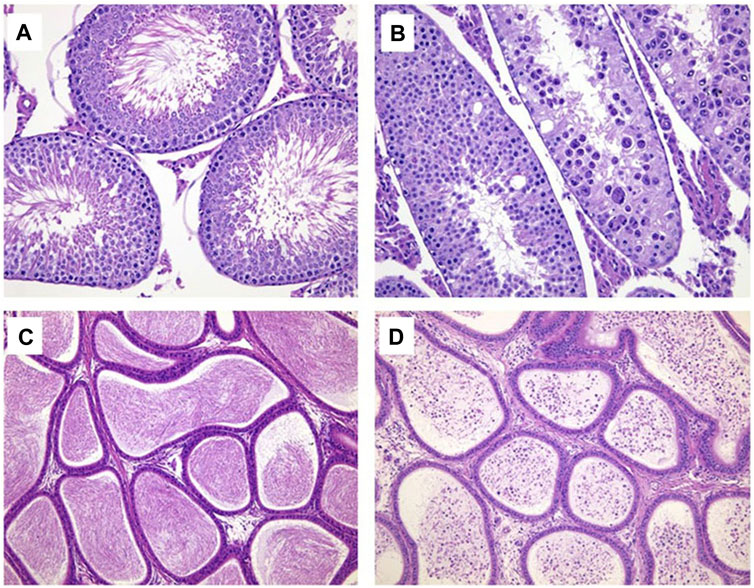
FIGURE 2. Hematoxylin and eosin-stained histologic sections for testis (× 200) and epididymis (× 100). (A) Normal testis in control group; (B) Tubule degeneration of testis at 2.4 mg/kg/day. (C) Normal epididymis in control group; (D) Cell debris and decreased sperm in the lumen of epididymis at 2.4 mg/kg/day.
With the exception of the above, no microscopic changes were observed in brain, heart, liver, spleen, lungs, kidney, adrenal gland, thymus, lymph node, and gastrointestinal tract of LPM3480392 -treated rats at the end of dosing phase and recovery phase.
3.13 Toxicokinetics
The concomitant toxicokinetics study was conducted for LPM3480392. Over the dose range from 0.6–2.4 mg/kg/day, the mean AUC0–12h of LPM3480392 in plasma had no apparent gender difference, and was increased generally in a dose-proportional manner on Day 1 and Day 28. No accumulation of LPM3480392 was noted after the 28-day repeated dosing.
On Day 1, mean AUC0–12h were 315, 663 and 1250 h ng/mL for rats (female + male) in each group, with the between-group ratio of 1:2.1:4.0. On Day 28, mean AUC0–12h were 349, 680 and 1490 h ng/mL for rats (female + male) in each group, with the between-group ratio of 1:1.9:4.3. Compared with Day 28, mean AUC0–12h ratios of LPM3480392 in plasma were 1.1, 1.0 and 1.2 for rats (female + male) in each group on Day 1, respectively, (Table 15; Figure 3).
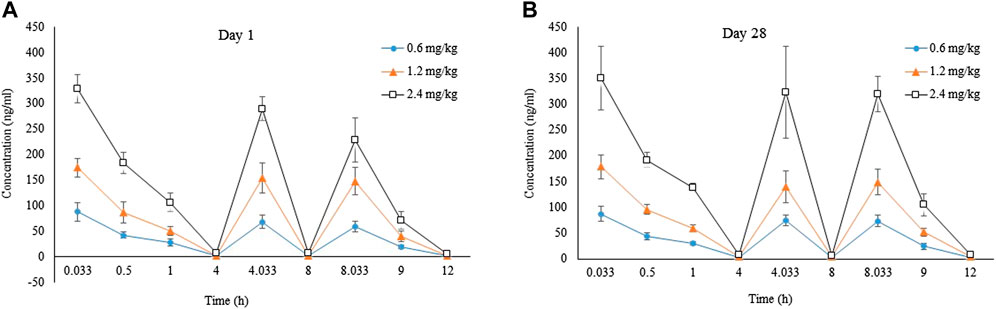
FIGURE 3. Tokicokinetics profile for LPM3480392. Mean plasma LPM4870059 concentration-time curve in rats (F + M) intravenously administered with LPM3480392 on Day 1 (A) and Day 28 (B).
4 Discussion
LPM3480392, a full MOR biased agonist, demonstrates in vitro biased agonism without β-arrestin-2 recruitment activity, which induces potent antinociceptive effect with reduced respiratory suppression (Yang et al., 2022). In the present study, we evaluated the subacute toxicity of LPM3480392 in rats. Two rats (1 male and 1 female) at 2.4 mg/kg/day were found dead on Days 3 and 15, respectively. They showed decreased activity and/or increased muscle tone, sternal recumbent posture after dosing, with no drug-related gross necropsy or histopathology changes. There is no clear death cause found.
Opiates-induced locomotor hyperactivity in rodents involves activation of the mesolimbic dopamine system and serves as one behavioural consequence of enhanced mesolimbic dopamine signalling (Botz-Zapp et al., 2021; Liu et al., 2021; Paul et al., 2021; Santos et al., 2023). However, at a higher dose, morphine induced the decreased locomotor activity(Paul et al., 2021). Opiates, such as morphine can induce dose-dependent muscular activity (rigidity), which was explained by decreased striatal dopaminergic activity (Wand et al., 1973; Havemann et al., 1982). Traditional opiates can induce severe adverse effects (SAE) such as respiratory suppression by activating both Gi and β-arrestin signaling pathway (Bohn et al., 1999; Bohn et al., 2000; Raehal et al., 2005; Del Vecchio et al., 2017; Zhuang et al., 2022). In rodent studies, TRV130, LPM3480392 and other G-protein-biased MOR agonists produce less respiratory depressions than traditional opioids (Bossert et al., 2020; Yang et al., 2022). In this study, during the dosing phase, the rats in 0.6, 1.2 and 2.4 mg/kg/day groups mostly displayed increased activity while 2.4 mg/kg/day also induced the decreased activity only a few time points. LPM3480392 at 1.2 and 2.4 mg/kg/day increased muscle tone. In addition, the sporadic individual rats occasionally had deep respiration or rale, hunched back and piloerection in 2.4 mg/kg/day group. Only the rats (4/36) in 2.4 mg/kg/day group were observed with deep respiration or rale. LPM3480392-treated rats showed no activity abnormalities during the recovery phase.
The opiates-induced body weight decrease could be due to increased energy expenditure (Levine et al., 1988). And, the body weight decrease induced by opiates withdrawal may be mediated by the activation of stress-related brain circuits (Bobzean et al., 2019; Jimenez-Romero et al., 2020). LPM3480392 treatment resulted in the decreased body weight gains and food consumption in males during the dosing phase, which are typically observed with opiate drugs (Mitzelfelt et al., 2011). Especially, body weight gains were pronounced reduced in the three LPM3480392 groups during the recovery phase, compared with the dosing phase, which is a typical predictive factor of opiates withdrawal (Glick et al., 1975; Pinelli and Trivulzio, 1997; Binsack et al., 2006; Mitzelfelt et al., 2011; Le et al., 2014; Townsend et al., 2022). However, though the decreased food consumption in females were observed in several time points during dosing phase and recovery phase, the body weight gains were increased. No obvious effects on body weight gain in females were observed in other opiates studies (Bobzean et al., 2019; Jimenez-Romero et al., 2020; Trevena, Inc, 2020; Taylor et al., 2023), such as the 4-week continuous IV infusion toxicity study of TRV130 (Trevena, Inc, 2020) and prolonged morphine administration (Taylor et al., 2023). The sex difference of the effects on body weight especially after drug withdrawal might reflect sex difference in cellular activation (phosphorylated CREB) in the GABAergic neurons of the ventral tegmental area (Bobzean et al., 2019).
In this study, we regret that we did not carefully observe the withdrawal behaviour. However, we have conducted the spontaneous withdrawal test in rats in 2019 at WCFP (data not shown in this manuscript). The rats were intravenously injected with LPM3480392 injection from 0.3 to 1.8 mg/kg/day for 30 consecutive days. Physical dependence was induced by LPM3480392 injection in rats after the spontaneous withdrawal. Increased scores of the withdrawal behaviours such as wet dog shaking, writhing, chew, teeth chattering and eyelid ptosis were noted in LPM3480392 injection groups after the drug withdrawal. These withdrawal behaviours had a gradually recovery trend, which had reserved to normal on Recovery day 6 for rats administered with LPM3480392 injection. After the withdrawal, body weight of male rats in LPM3480392 injection group significantly decreased and then recovered to normal within the withdrawal recovery phase. There were no apparent abnormalities in body weights of female rats in LPM3480392 injection groups during dosing and recovery phases. The effects of LPM3480392 on body weight in males or females in the spontaneous withdrawal test are similar to those in the subacute toxicity study in rats.
Opiates have been conventionally considered immunosuppressive. The µ-opioid receptor is essential to the immunosuppressive effects observed after morphine administration (Plein and Rittner, 2018; Franchi et al., 2019; Ackerman et al., 2021). Not all opiates yield immunosuppression (Franchi et al., 2019; Ackerman et al., 2021). Morphine and fentanyl have been found to impair the function of macrophages, natural killer cells and T-cells in animal studies (Plein and Rittner, 2018; Franchi et al., 2019). However, The immunosuppressive effects has not been observed with oxycodone or buprenorphine (Plein and Rittner, 2018; Franchi et al., 2019; Ackerman et al., 2021). Though the impact of G-protein vs. β-arrestin activation in immunosuppression has never been explored, buprenorphine does not recruit β-arrestin to the receptor and has been reported to be devoid of immunosuppressive properties (Franchi et al., 2019). In our study, no histopathological changes were observed in spleen, thymus and lymph nodes in both males and females after LPM3480392 treatment. Also, there are no significant decreases in the absolute/relative organ weight of spleen and thymus in males or females. In the male rats, the mildly lowered MONO and EOS/EOS% was observed at the end of dosing phase. In the female rats, the mildly decreased MONO/MONO% at the end of dosing phase; and the mildly increased LYM%, and the decreased NEU/NEU% and MONO% were observed at the end of recovery phase. However, the absolute WBC were not significantly changed in males or females at the end of dosing phase or recovery phase. All the changes about WBC were considered not toxicologically significant because of evidence of the in-house background range, and absence of dose dependence and time dependence, and the related microscopic correlation. Importantly, in the concomitant toxicokinetics study, the mean AUC0–12h and Cmax of LPM3480392 were increased generally in a dose-proportional manner on Day 1 and Day 28. No accumulation of LPM3480392 was noted after the 28-day repeated dosing. After every LPM3480392 treatment, the drug was eliminated fast in the body (Table 15; Figure 3). Therefore, LPM3480392 cannot produce long-lasting hematologic issues. Based on the above results, LPM3480392 did not display obvious immunosuppressive effects maybe because of this compound’s full µ-opioid receptor bias.
A few LPM3480392-related minor changes in other haematology results were observed. At the end of dosing phase, only small magnitude of increases in RET/RET% in the male rats were noted without the abnormalities in peripheral blood erythroid series or histopathology of bone marrow, and the changes of MCV, RBC and PLT in the female rats were with small magnitude (less than 5%, 6% and 15%, respectively). All the changes were considered not toxicologically significant because of their small magnitude of changes, evidence of reversibility, and absence of microscopic correlation.
The effects of decreased food intake on a series of toxicological parameters in rats have been widely understood, including haematology, clinical chemistry, urinalysis, organ weight, and histopathology (Levin et al., 1993; Moriyama et al., 2008). The reduced TG, ALB, TG and GLU were attributable to the nutritional deficiency secondary to food intake reduction (Levin et al., 1993; Moriyama et al., 2008). In this study, in all LPM3480392 groups, the decreased TG (<49%), GLU (<20%), ALB (<10%) and TP (<10%) in the males, and decreased ALB (<18%) and TP (<18%) in the females were observed, which could be related to the decreased food consumption resulted from LPM3480392 treatment. The decreased TBIL in females could be induced by LPM3480392, which aren’t generally a cause for concern as a liver function index. In the female rats, the increased AST and GLU and decreased CREA at all LPM3480392 groups were observed with small magnitude (<18%). All the changes in clinical chemistry parameters were considered not toxicologically significant, because of their small magnitude of changes, evidence of reversibility, absence of microscopic correlation, and no direct target organs toxicity.
In urinalysis, the decreased grade distribution of NIT and KET in the males of 1.2 and/or 2.4 mg/kg/day groups, and the increased grade distribution of pH in the females of 2.4 mg/kg/day group were observed at the end of dosing phase; and PH changes in 0.6 mg/kg/day group were also noted at the end of recovery phase. The above changes had no mostly toxicologically significant, as no abnormalities were noted in serum renal function related parameters or histopathology of kidney and urinary system.
It is generally accepted that µ-opioid agonists such as morphine and fentanyl activate the hypothalamus-pituitary-adrenal (HPA) axis in rodents (Tanabe and Cafruny, 1958; Franchi et al., 2007), and chronic administration of morphine or TRV130 produces adrenal cortical hypertrophy(Tanabe and Cafruny, 1958; Trevena, Inc, 2020;). However, in our study, the decreased absolute weight in adrenal gland of males, and the decreased organ-to-body weight ratio of adrenal gland of females were noted in LPM3480392 groups, but there were no histopathology changes. We did not determine if a full µ-opioid receptor bias produce this difference compared with other opiates. At the end of dosing phase, the increase in organ-to-body weight ratio of heart but not heart weight was observed in all LPM3480392-treated male rats, which is due to the reduced body weight gain. The slightly decreased absolute weight or organ-to-brain weight ratio of liver in LPM3480392-treated male rats were observed, while no significant live-related clinical chemistry and histopathology changes. The decreased organ-to-body weight ratio of brain of females were presented, but no absolute brain weight changes at the end of dosing phase, which was related to the increased body weight gain. At the end of recovery phase, the increased thymus weight of males in 0.6 mg/kg/day group was noted without toxicological significance, because of no changes at the end of dosing phase, no dose-dependency or microscopic abnormalities. Therefore, the changes of heart, liver, brain, thymus and adrenal gland were considered not toxicologically significant, because of their small magnitude of changes, evidence of reversibility, no dose-dependency, and absence of histopathology changes.
At the end of recovery phase, the decreased absolute weight and organ-to-brain weight ratio of epididymides were observed in 2.4 mg/kg/day group. Sporadic individual males at 2.4 mg/kg/day were observed with the mild tubular degeneration of the testis, the marked decreased sperm in the lumen and the minimal to moderate cell debris in the lumen of the epididymis. The changes of testis and epididymis were maybe target organ toxicity for LPM3480392. However, the above microscopic changes of testis and epididymis were recovered at the end of recovery phase.
The toxicokinetics study shown that typical linear kinetics characteristics were presented on Day 1 and Day 28 after LPM3480392 administration, and the mean AUC0–12h was increased generally in a dose-proportional manner. No accumulation of LPM3480392 was noted after the 28-day repeated dosing. The highest drug exposure was observed at 2.4 mg/kg/day, which partly explained the toxicity, including the unscheduled death, the clinical signs of increased activity, increased muscle tone, sternal recumbent posture, deep respiration; microscopic changes of tubular degeneration of the testis, and decreased sperm in the lumen and cell debris in the lumen of the epididymis.
In this study, there are several limitations: (1) We missed the observation time points for the opiates withdrawal such as the decreased body weight and food consumption, and wet-dog shaking during the first days of recovery phase. (2) Morphine can induce mydriasis in rats (Klemfuss et al., 1979; Adler et al., 1981). However, we did not observe if LPM3480392 induced the mydriasis in the ophthalmic examination during the dosing and recovery phases. We will carry out the related experiments in order to observe the opiates withdrawal behaviours and mydriasis.
In summary, LPM3480392 presents weak/no immunosuppression and the decreased adrenal gland weight, which is different from other opiates. The main reason may be that LPM3480392 is a full MOR biased agonist. Further experiments need to be warranted in order to study the effects of LPM3480392 on immune system and HPA axis.
Data availability statement
The original contributions presented in the study are included in the article/Supplementary material, further inquiries can be directed to the corresponding authors.
Ethics statement
The animal study was reviewed and approved by This subacute toxicity study has been conducted in WestChina-Frontier PharmaTech Co., Ltd. (WCFP). Animal experiments complied with the relevant regulations in Institutional Animal Care and Use Committee (IACUC) in WCFP.
Author contributions
YY synthesized the compound. LY, CL, WJ, and XC designed the research work. WW, HZ, NL, and ZH analyzed the data. LY and CL drafted the manuscript. HW and JT revised and edited the content of the paper. All authors contributed to the article and approved the submitted version.
Funding
This work was partially supported by the Natural Science Foundation of Shandong Province, China (No. ZR2022MH186), National Natural Science Foundation of China (No. 81902161), and Taishan Scholar Project.
Conflict of interest
Author XC was employed by WestChina-Frontier PharmaTech Co., Ltd.
The remaining authors declare that the research was conducted in the absence of any commercial or financial relationships that could be construed as a potential conflict of interest.
Publisher’s note
All claims expressed in this article are solely those of the authors and do not necessarily represent those of their affiliated organizations, or those of the publisher, the editors and the reviewers. Any product that may be evaluated in this article, or claim that may be made by its manufacturer, is not guaranteed or endorsed by the publisher.
References
Ackerman, R. S., Luddy, K. A., Icard, B. E., Pineiro Fernandez, J., Gatenby, R. A., and Muncey, A. R. (2021). The effects of anesthetics and perioperative medications on immune function: A narrative review. Anesth. Analg. 133, 676–689. doi:10.1213/ANE.0000000000005607
Adler, C. H., Robin, M., and Adler, M. W. (1981). Tolerance to morphine-induced mydriasis in the rat pupil. Life Sci. 28, 2469–2475. doi:10.1016/0024-3205(81)90588-9
Binsack, R., Zheng, M. L., Zhang, Z. S., Yang, L., and Zhu, Y. P. (2006). Chronic morphine drinking establishes morphine tolerance, but not addiction in Wistar rats. J. Zhejiang Univ. Sci. B 7, 892–898. doi:10.1631/jzus.2006.B0892
Bobzean, S. M., Kokane, S. S., Butler, B. D., and Perrotti, L. I. (2019). Sex differences in the expression of morphine withdrawal symptoms and associated activity in the tail of the ventral tegmental area. Neurosci. Lett. 705, 124–130. doi:10.1016/j.neulet.2019.04.057
Bohn, L. M., Gainetdinov, R. R., Lin, F. T., Lefkowitz, R. J., and Caron, M. G. (2000). Mu-opioid receptor desensitization by beta-arrestin-2 determines morphine tolerance but not dependence. Nature 408, 720–723. doi:10.1038/35047086
Bohn, L. M., Lefkowitz, R. J., Gainetdinov, R. R., Peppel, K., Caron, M. G., and Lin, F. T. (1999). Enhanced morphine analgesia in mice lacking beta-arrestin 2. Science 286, 2495–2498. doi:10.1126/science.286.5449.2495
Bossert, J. M., Kiyatkin, E. A., Korah, H., Hoots, J. K., Afzal, A., Perekopskiy, D., et al. (2020). In a rat model of opioid maintenance, the G protein-biased mu opioid receptor agonist TRV130 decreases relapse to oxycodone seeking and taking and prevents oxycodone-induced brain hypoxia. Biol. Psychiatry 88, 935–944. doi:10.1016/j.biopsych.2020.02.014
Bostrom, B. M., Ramberg, T., Davis, B. D., and Fridlund, B. (1997). Survey of post-operative patients' pain management. J. Nurs. Manag. 5, 341–349. doi:10.1046/j.1365-2834.1997.00031.x
Botz-Zapp, C. A., Foster, S. L., Pulley, D. M., Hempel, B., Bi, G. H., Xi, Z. X., et al. (2021). Effects of the selective dopamine D(3) receptor antagonist PG01037 on morphine-induced hyperactivity and antinociception in mice. Behav. Brain Res. 415, 113506. doi:10.1016/j.bbr.2021.113506
Chan, H. C. S., Mccarthy, D., Li, J., Palczewski, K., and Yuan, S. (2017). Designing safer analgesics via mu-opioid receptor pathways. Trends Pharmacol. Sci. 38, 1016–1037. doi:10.1016/j.tips.2017.08.004
Chen, X. T., Pitis, P., Liu, G., Yuan, C., Gotchev, D., Cowan, C. L., et al. (2013). Structure-activity relationships and discovery of a G protein biased μ opioid receptor ligand, [(3-methoxythiophen-2-yl)methyl]({2-[(9R)-9-(pyridin-2-yl)-6-oxaspiro-[4.5]decan-9-yl]ethyl})amine (TRV130), for the treatment of acute severe pain. J. Med. Chem. 56, 8019–8031. doi:10.1021/jm4010829
Daugvilaite, V., Madsen, C. M., Luckmann, M., Echeverria, C. C., Sailer, A. W., Frimurer, T. M., et al. (2017). Biased agonism and allosteric modulation of G protein-coupled receptor 183 - a 7TM receptor also known as Epstein-Barr virus-induced gene 2. Br. J. Pharmacol. 174, 2031–2042. doi:10.1111/bph.13801
Del Vecchio, G., Spahn, V., and Stein, C. (2017). Novel opioid analgesics and side effects. ACS Chem. Neurosci. 8, 1638–1640. doi:10.1021/acschemneuro.7b00195
Dewire, S. M., Yamashita, D. S., Rominger, D. H., Liu, G., Cowan, C. L., Graczyk, T. M., et al. (2013). A G protein-biased ligand at the mu-opioid receptor is potently analgesic with reduced gastrointestinal and respiratory dysfunction compared with morphine. J. Pharmacol. Exp. Ther. 344, 708–717. doi:10.1124/jpet.112.201616
Franchi, S., Moschetti, G., Amodeo, G., and Sacerdote, P. (2019). Do all opioid drugs share the same immunomodulatory properties? A review from animal and human studies. Front. Immunol. 10, 2914. doi:10.3389/fimmu.2019.02914
Franchi, S., Panerai, A. E., and Sacerdote, P. (2007). Buprenorphine ameliorates the effect of surgery on hypothalamus-pituitary-adrenal axis, natural killer cell activity and metastatic colonization in rats in comparison with morphine or fentanyl treatment. Brain Behav. Immun. 21, 767–774. doi:10.1016/j.bbi.2007.01.001
Gan, T. J., Habib, A. S., Miller, T. E., White, W., and Apfelbaum, J. L. (2014). Incidence, patient satisfaction, and perceptions of post-surgical pain: Results from a US national survey. Curr. Med. Res. Opin. 30, 149–160. doi:10.1185/03007995.2013.860019
Gan, T. J. (2017). Poorly controlled postoperative pain: Prevalence, consequences, and prevention. J. Pain Res. 10, 2287–2298. doi:10.2147/JPR.S144066
Glick, S. D., Cox, R. S., and Crane, A. M. (1975). Changes in morphine self-administration and morphine dependence after lesions of the caudate nucleus in rats. Psychopharmacologia 41, 219–224. doi:10.1007/BF00428927
Goudra, B. (2022). Oliceridine- opioid of the 21(st) century. Saudi J. Anaesth. 16, 69–75. doi:10.4103/sja.sja_510_21
Havemann, U., Turski, L., and Kuschinsky, K. (1982). Role of GABAergic mechanisms in the substantia nigra pars reticulata in modulating morphine-induced muscular rigidity in rats. Neurosci. Lett. 31, 25–30. doi:10.1016/0304-3940(82)90048-9
Jimenez-Romero, F., Bis-Humbert, C., and Garcia-Fuster, M. J. (2020). Adolescent morphine induces emotional signs of withdrawal paired with neurotoxicity selectively in male rats: Female resilience. Neurosci. Lett. 715, 134625. doi:10.1016/j.neulet.2019.134625
Klemfuss, H., Tallarida, R. J., Adler, C. H., and Adler, M. W. (1979). Morphine-induced mydriasis and fluctuation in the rat: Time and dose relationships. J. Pharmacol. Exp. Ther. 208, 91–95.
Kliewer, A., Gillis, A., Hill, R., Schmiedel, F., Bailey, C., Kelly, E., et al. (2020). Morphine-induced respiratory depression is independent of beta-arrestin2 signalling. Br. J. Pharmacol. 177, 2923–2931. doi:10.1111/bph.15004
Le, T., Xia, M., Jia, M., Sarkar, N., Chen, J., Li, H., et al. (2014). Association between initial morphine intake and body weight change, acoustic startle reflex and drug seeking in rats. Psychopharmacology 231, 4569–4577. doi:10.1007/s00213-014-3606-2
Levin, S., Semler, D., and Ruben, Z. (1993). Effects of two weeks of feed restriction on some common toxicologic parameters in sprague-dawley rats. Toxicol. Pathol. 21, 1–14. doi:10.1177/019262339302100101
Levine, A. S., Grace, M., Billington, C. J., Gosnell, B. A., Krahn, D. D., Brown, D. M., et al. (1988). Effect of morphine and nalmefene on energy balance in diabetic and non-diabetic rats. Pharmacol. Biochem. Behav. 29, 495–500. doi:10.1016/0091-3057(88)90010-x
Liu, S. X., Gades, M. S., Swain, Y., Ramakrishnan, A., Harris, A. C., Tran, P. V., et al. (2021). Repeated morphine exposure activates synaptogenesis and other neuroplasticity-related gene networks in the dorsomedial prefrontal cortex of male and female rats. Drug Alcohol Depend. 221, 108598. doi:10.1016/j.drugalcdep.2021.108598
Manglik, A., Lin, H., Aryal, D. K., Mccorvy, J. D., Dengler, D., Corder, G., et al. (2016). Structure-based discovery of opioid analgesics with reduced side effects. Nature 537, 185–190. doi:10.1038/nature19112
Matthes, H. W., Maldonado, R., Simonin, F., Valverde, O., Slowe, S., Kitchen, I., et al. (1996). Loss of morphine-induced analgesia, reward effect and withdrawal symptoms in mice lacking the mu-opioid-receptor gene. Nature 383, 819–823. doi:10.1038/383819a0
Mattson, C. L., Tanz, L. J., Quinn, K., Kariisa, M., Patel, P., and Davis, N. L. (2021). Trends and geographic patterns in drug and synthetic opioid overdose deaths - United States, 2013-2019. MMWR Morb. Mortal. Wkly. Rep. 70, 202–207. doi:10.15585/mmwr.mm7006a4
Mitzelfelt, J. D., Dupree, J. P., Seo, D. O., Carter, C. S., and Morgan, D. (2011). Effects of chronic fentanyl administration on physical performance of aged rats. Exp. Gerontol. 46, 65–72. doi:10.1016/j.exger.2010.10.004
Moriyama, T., Tsujioka, S., Ohira, T., Nonaka, S., Ikeda, H., Sugiura, H., et al. (2008). Effects of reduced food intake on toxicity study parameters in rats. J. Toxicol. Sci. 33, 537–547. doi:10.2131/jts.33.537
Paul, A. K., Gueven, N., and Dietis, N. (2021). Profiling the effects of repetitive morphine administration on motor behavior in rats. Molecules 26, 4355. doi:10.3390/molecules26144355
Pinelli, A., and Trivulzio, S. (1997). Quantitative evaluation of opioid withdrawal signs in rats repeatedly treated with morphine and injected with naloxone, in the absence or presence of the antiabstinence agent clonidine. J. Pharmacol. Toxicol. Methods 38, 117–131. doi:10.1016/s1056-8719(97)00050-6
Plein, L. M., and Rittner, H. L. (2018). Opioids and the immune system - friend or foe. Br. J. Pharmacol. 175, 2717–2725. doi:10.1111/bph.13750
Raehal, K. M., Walker, J. K., and Bohn, L. M. (2005). Morphine side effects in beta-arrestin 2 knockout mice. J. Pharmacol. Exp. Ther. 314, 1195–1201. doi:10.1124/jpet.105.087254
Santos, E. J., Nassehi, N., Bow, E. W., Chambers, D. R., Gutman, E., Jacobson, A. E., et al. (2023). Role of efficacy as a determinant of locomotor activation by mu-opioid receptor (MOR) ligands in female and male mice. II. Effects of novel MOR-selective phenylmorphans with high-to-low MOR efficacy. Pharmacol. Res. Perspect. 11, e01111. doi:10.1002/prp2.1111
Schmid, C. L., Kennedy, N. M., Ross, N. C., Lovell, K. M., Yue, Z., Morgenweck, J., et al. (2017). Bias factor and therapeutic window correlate to predict safer opioid analgesics. Cell 171, 1165–1175.e13. doi:10.1016/j.cell.2017.10.035
Tan, H. S., and Habib, A. S. (2021). Oliceridine: A novel drug for the management of moderate to severe acute pain - a review of current evidence. J. Pain Res. 14, 969–979. doi:10.2147/JPR.S278279
Tanabe, T., and Cafruny, E. J. (1958). Adrenal hypertrophy in rats treated chronically with morphine. J. Pharmacol. Exp. Ther. 122, 148–153.
Taylor, M., Cheng, A. B., Hodkinson, D. J., Afacan, O., Zurakowski, D., and Bajic, D. (2023). Body size and brain volumetry in the rat following prolonged morphine administration in infancy and adulthood. Front. Pain Res. (Lausanne) 4, 962783. doi:10.3389/fpain.2023.962783
Townsend, E. A., Blough, B. E., Epstein, D. H., Negus, S. S., Shaham, Y., and Banks, M. L. (2022). Effect of TRV130 and methadone on fentanyl-vs.-food choice and somatic withdrawal signs in opioid-dependent and post-opioid-dependent rats. Neuropsychopharmacology 47, 2132–2139. doi:10.1038/s41386-022-01393-3
Trevena, Inc (2020). Pharmacology/toxicology NDA review and evalution in multi-discipline review for Oliceridine (NDA 210730). Available at: https://www.accessdata.fda.gov/drugsatfda_docs/nda/2020/210730Orig1s000MultidisciplineR.pdf.
Wand, P., Kuschinsky, K., and Sontag, K. H. (1973). Morphine-induced muscular rigidity in rats. Eur. J. Pharmacol. 24, 189–193. doi:10.1016/0014-2999(73)90071-x
Yang, Y., Wang, Y., Zuo, A., Li, C., Wang, W., Jiang, W., et al. (2022). Synthesis, biological, and structural explorations of a series of mu-opioid receptor (MOR) agonists with high G protein signaling bias. Eur. J. Med. Chem. 228, 113986. doi:10.1016/j.ejmech.2021.113986
Keywords: LPM3480392, a full μ-opioid receptor biased agonist, pain, subacute toxicity, rats
Citation: Ye L, Li C, Jiang W, Yang Y, Wang W, Zhu H, Hu Z, Li N, Cen X, Wang H and Tian J (2023) Subacute toxicity evaluations of LPM3480392 in rats, a full µ-opioid receptor biased agonist. Front. Pharmacol. 14:1218380. doi: 10.3389/fphar.2023.1218380
Received: 07 May 2023; Accepted: 20 July 2023;
Published: 04 August 2023.
Edited by:
Swaran J. S. Flora, National Institute of Pharmaceutical Education and Research, IndiaReviewed by:
Jan Willem Van Der Laan, Medicines Evaluation Board, NetherlandsChristopher Cunningham, Concordia University (Wisconsin), United States
Copyright © 2023 Ye, Li, Jiang, Yang, Wang, Zhu, Hu, Li, Cen, Wang and Tian. This is an open-access article distributed under the terms of the Creative Commons Attribution License (CC BY). The use, distribution or reproduction in other forums is permitted, provided the original author(s) and the copyright owner(s) are credited and that the original publication in this journal is cited, in accordance with accepted academic practice. No use, distribution or reproduction is permitted which does not comply with these terms.
*Correspondence: Liang Ye, cHJvamVjdDAwODhAaG90bWFpbC5jb20=; Jingwei Tian, cHJvamVjdDAwODFAaG90bWFpbC5jb20=
†These authors have contributed equally to this work