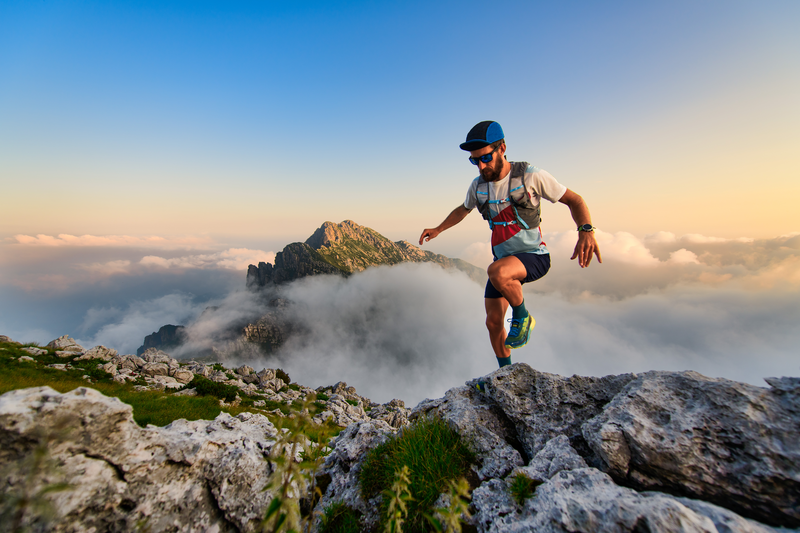
94% of researchers rate our articles as excellent or good
Learn more about the work of our research integrity team to safeguard the quality of each article we publish.
Find out more
ORIGINAL RESEARCH article
Front. Pharmacol. , 16 June 2023
Sec. Ethnopharmacology
Volume 14 - 2023 | https://doi.org/10.3389/fphar.2023.1204641
This article is part of the Research Topic Therapeutic potential of natural products in oxidative and metabolic diseases View all 16 articles
Background: Type 2 diabetes (T2D) is a metabolic disorder characterized by insulin resistance (IR) and hyperglycemia. Plants are valuable sources of therapeutic agents for the management of T2D. Euphorbia peplus has been widely used as a traditional medicine for the treatment of various diseases, but its beneficial role in T2D has not been fully explored.
Methods: The anti-diabetic efficacy of E. peplus extract (EPE) was studied using rats with T2D induced by high-fat diet (HFD) and streptozotocin (STZ). The diabetic rats received 100, 200, and 400 mg/kg EPE for 4 weeks.
Results: Phytochemical fractionation of the aerial parts of E. peplus led to the isolation of seven known flavonoids. Rats with T2D exhibited IR, impaired glucose tolerance, decreased liver hexokinase and glycogen, and upregulated glycogen phosphorylase, glucose-6-phosphatase (G-6-Pase), and fructose-1,6-bisphosphatase (F-1,6-BPase). Treatment with 100, 200, and 400 mg/kg EPE for 4 weeks ameliorated hyperglycemia, IR, liver glycogen, and the activities of carbohydrate-metabolizing enzymes. EPE attenuated dyslipidemia, serum transaminases, tumor necrosis factor (TNF)-α, interleukin (IL)-1β and liver lipid accumulation, nuclear factor (NF)-κB p65, and lipid peroxidation, nitric oxide and enhanced antioxidants. All EPE doses upregulated serum adiponectin and liver peroxisome proliferator-activated receptor γ (PPARγ) in HFD/STZ-induced rats. The isolated flavonoids showed in silico binding affinity toward hexokinase, NF-κB, and PPARγ.
Conclusion: E. peplus is rich in flavonoids, and its extract ameliorated IR, hyperglycemia, dyslipidemia, inflammation and redox imbalance, and upregulated adiponectin and PPARγ in rats with T2D.
Diabetes mellitus (DM) is a common metabolic disorder associated with several complications, including nephropathy, neuropathy, and cardiomyopathy. This disorder includes type 1 (T1DM) and type 2 (T2DM) forms of the disease where T1DM is characterized by insulin insufficiency, whereas insulin resistance (IR) is the characteristic feature of T2DM. Both insulin deficiency and IR lead to the accumulation of glucose in the blood (hyperglycemia) (American Diabetes Association, 2021). DM is a fast-increasing disease worldwide, and the number of patients with diabetes is expected to reach 700 million by 2045 (Saeedi et al., 2019). T2DM is the most common form of the disease characterized by hyperglycemia and IR (Kahn et al., 2014). IR increases the risk of hypertension, dyslipidemia, and atherosclerosis (Guzik and Cosentino, 2018). Oxidative stress (OS) and inflammation mediated by excess reactive oxygen species (ROS) and inflammatory mediators produced under hyperglycemic conditions are implicated in the pathophysiology of DM and its complications (Mahmoud et al., 2012). Excess ROS can damage cellular macromolecules and work in concert with inflammatory mediators to provoke cell death. ROS and inflammatory mediators impair insulin signaling by provoking β-cell death, alter peripheral glucose uptake, and increase gluconeogenesis (Jheng et al., 2012). Therefore, mitigation of OS and inflammation could be beneficial to prevent IR and hyperglycemia in T2DM.
Plants of the genus Euphorbia include numerous known species with chemical diversity and multiple biological and commercial uses (Shi et al., 2008). The latex of plants of the family Euphorbiaceae was acknowledged for its various phytoconstituents that possess both commercial and pharmacological importance such as triterpene alcohols (Giner and Schroeder, 2015). Because of its toxic nature and unpleasantness, the latex protects the plants against the attack of animals (Al-Sultan and Hussein, 2006). Steroids, flavonoids, sesquiterpenoids, glycerols, and cerebrosides are among the phytoconstituents reported in plants of the genus Euphorbia (Shi et al., 2008; Kamel et al., 2022). With this rich content, Euphorbia plants found their way to be employed in folkloric medicine to treat migraine, intestinal parasites, gonorrhea, and skin disorders (Singla and Kamla, 1990), and studies have reported their wound-healing potential (Pattanaik et al., 2014; Ahmed et al., 2016). Recent work from our laboratory revealed the inhibitory activity of E. peplus on xanthine oxidase (XO) and hyperuricemia in rats (Kamel et al., 2022). Other studies showed the possible beneficial effects of E. royleana stem extract (Zafar et al., 2021) and E. hirta flower extract (Kumar et al., 2010) in rats with streptozotocin (STZ)- and alloxan-induced diabetes, respectively. These studies revealed the ability of E. royleana and E. hirta to ameliorate hyperglycemia and oxidative damage. Another recent study highlighted the anti-hyperglycemic effect of E. helioscopia methanolic extract in sucrose-supplemented rats (Mustafa et al., 2022). Owing to the promising therapeutic value of plants of this genus, this study explored the phytochemical constituents and the effect of E. peplus extract (EPE) on hyperglycemia, IR, OS, and inflammation in rats with T2D induced by high-fat diet (HFD) and STZ.
Proton nuclear magnetic resonance (1HNMR) and 13CNMR (500 MHz and 125 MHz, respectively) spectra were recorded on the Bruker AV-500 spectrometer using TMS as an internal standard. The optical rotation of isolated flavonoids was obtained using a Rudolph Autopol III polarimeter. Ultraviolet (UV) spectral data were measured using the Shimadzu UV-vis 160i spectrophotometer, and the HREIMS and EIMS spectral data were recorded using the Finnigan MAT TSQ 700 mass spectrometer. Infrared spectral data were obtained through KBr pellets on the Shimadzu FTIR-8400 instrument.
The plant was collected from Beni Suef Governorate in March 2021 and identified by a taxonomist and a voucher specimen (EP-038021-2) was stored. The aerial parts (2.75 kg) were extracted four times using 70% acetone followed by the removal of the solvent under reduced pressure, resulting in 904 g of extract. Thereafter, the extract was dissolved in water and successively partitioned using chloroform, ethyl acetate (EA), and n-butanol (3L x 2, each). The EA fraction (69.7 g) was subjected to chromatographic fractionation over a silica gel column (120 × 4 cm, 1.1 kg) and eluted with dichloromethane (DCM)/acetone mixture of increasing polarity. To track the movement of the bands along the column and to regulate the collection of fractions, a UV lamp was employed. A total of 22 fractions were collected and combined into seven main subfractions (F1–F7) according to their similar thin-layer chromatography (TLC) profiles. Subfraction F3 was chromatographed over silica gel using chloroform–EA of gradient elution to afford nine subfractions (F3.1–F3.9). Subfractions F3.3–F3.7 were combined and applied to the Sephadex LH-20 column eluted with methanol (MeOH):water (50:50→100:0) to give seven TLC-monitored subfractions (E1–E7). Sub-subfractions (E2–E5) were combined and purified over a Sephadex LH-20 column eluted with MeOH to yield the purified compounds 2 (22 mg), 3 (17 mg), and 4 (14 mg). Subfraction F4 was fractionated over a polyamide 6S column eluted with the MeOH–water solvent mixture of increasing polarity to afford eleven subfractions (F4.1–F4.11). Subfraction F4.6 was purified over the Sephadex LH-20 column eluted with MeOH to yield purified compound 1 (23 mg). Subfractions F4.8–F4.10 were combined and re-chromatographed using the Sephadex LH-20 column eluted with MeOH to give compound 5 (19 mg). Subfraction F5 was partitioned by means of the Sephadex LH-20 column using MeOH–water (2:8, 3:7→10:0) to afford six subfractions (F5.1–F5.6). Compound 6 (23 mg) was obtained from the chromatographic fractionation of F5.3–F5.5 over two consecutive Sephadex LH-20 columns using 30% MeOH as an eluent. Subfraction F7 was subjected to silica gel column chromatography eluted with the solvent system chloroform–MeOH–water (lower layer, 28:9:6 and 6:3:1) to yield five subfractions (F7.1–F7.5). Compound 7 (24 mg) was obtained from the recombination and purification of subfractions F7.1–F7.3 over Sephadex LH-20 and eluted with MeOH (Supplementary Figure S1).
The RSA activity of EPE was measured using 2,2-diphenyl-1-picrylhydrazyl (DPPH) and 2,2-azinobis (3-ethylbenzothiazoline-6-sulfonic acid) (ABTS) assays following the methods of Brand-Williams et al. (1995) and Re et al. (1999), respectively, using ascorbic acid as a standard.
Male Wistar rats weighing 170–190 g were included in this investigation. The rats were maintained under standard conditions of temperature (23°C ± 1°C) and humidity (50%–60%) on a 12-h light/dark cycle with free access to food and water. The animal study protocol was approved by the Research Ethics Committee of Al-Azhar University (ZA-AS/PH/18/C/2023). The rats received a normal diet and a single intraperitoneal (i.p.) injection of freshly prepared citrate buffer (pH 4.5) to serve as a control. Other rats were fed a HFD (58% fat, 17% carbohydrate, and 25% protein) for 28 days and received a single i.p. dose of STZ (35 mg/kg; Sigma, United States) dissolved in freshly prepared citrate buffer (pH 4.5) to induce T2D (Germoush et al., 2019). After 7 days, T2D was confirmed by measuring blood glucose (BG) for 2 h after supplementing the overnight fasted rats with 3 g/kg glucose orally. The rats exhibited BG higher than 250 mg/kg were included in the investigation.
To investigate the antidiabetic effects of the EA fraction of E. peplus extract (EPE; dissolved in 0.5% carboxymethyl cellulose (CMC) as a vehicle), 30 diabetic and 12 normal rats were allocated into seven groups (n = 6) as follows:
Group I (control): received 0.5% CMC.
Group II (EPE): received 400 mg/kg EPE.
Group III (diabetic): received 0.5% CMC.
Group IV (diabetic + 100 mg/kg EPE): received 100 mg/kg EPE.
Group V (diabetic + 200 mg/kg EPE): received 200 mg/kg EPE.
Group VI (diabetic + 400 mg/kg EPE): received 400 mg/kg EPE.
Group VII (diabetic + PIO): received 10 mg/kg of the antidiabetic pioglitazone (PIO) (Abd El-Twab et al., 2016).
EPE, 0.5% CMC, and PIO were supplemented orally for 4 weeks. A day before the end of the experiment, the rats were fasted overnight and then supplemented with 3 g/kg glucose solution, and the blood was collected from the tail vein over 2 h for the determination of BG using a Spinreact (Spain) kit (Trinder, 1969). At the end of treatments, the animals were euthanized under ketamine anesthesia (100 mg/kg i.p.), and blood and liver samples were collected. Serum was separated following centrifugation of blood, and samples from the liver were homogenized in Tris-HCl buffer (pH = 7.4). Other samples were fixed in 10% neutral buffered formalin (NBF) or stored at −80°C.
Serum insulin, transaminases (ALT and AST), adiponectin and cytokines (TNF-α and IL-1β) were determined using kits from RayBiotech (United States), Spinreact (Spain), and R&D Systems (United States), respectively. NF-kB p65 in liver homogenate was determined using the kit from R&D Systems (United States). All assays were performed according to the manufacturers’ instructions.
The homeostasis model assessment of IR (HOMA-IR) was calculated as previously described by Haffner (2000) using the following equation:
Liver glycogen content was determined as previously described (Seifter and Dayton, 1950). Liver homogenate was centrifuged, and the clear supernatant was used to assess the activities of hexokinase (Brandstrup et al., 1957), G-6-Pase (Koide and Oda, 1959), F-1,6-BPase (Freedland and Harper, 1959), and glycogen phosphorylase (Stalmans and Hers, 1975). Malondialdehyde (MDA) (Ohkawa et al., 1979), nitric oxide (NO) (Green et al., 1982), reduced glutathione (GSH) (Beutler et al., 1963), and the activities of superoxide dismutase (SOD) (Marklund and Marklund, 1974), catalase (CAT) (Aebi, 1984), and glutathione peroxidase (GPx) (Flohé and Günzler, 1984) were determined in the supernatant of the homogenized liver. Liver triglycerides (TGs) and cholesterol were assayed using Spinreact (Spain) kits after extracting the lipids using chloroform/MeOH mixture (2:1, v/v) as descried by Folch et al (1957). Serum TG, total cholesterol (TC), and high-density lipoprotein (HDL)-C were assayed using kits from Spinreact (Spain). Low-density lipoprotein (LDL)-C, very low-density lipoprotein (vLDL)-C, and atherogenic index of plasma (AIP) were calculated according to the following equations:
Samples from the liver fixed in 10% NBF for 24 h were dehydrated, cleared, and embedded in paraffin wax. Sections of 5 μm thickness were cut for routine staining with hematoxylin and eosin (H&E) (Bancroft and Gamble, 2008) and examined under a light microscope.
To determine the changes in PPARγ mRNA, RNA was isolated from the frozen liver using TRIzol and quantified, and samples with OD260/280 ≥ 1.8 were reverse-transcribed into cDNA using a cDNA synthesis kit (Thermo Scientific, United States). cDNA amplification was carried out using SYBR Green Master Mix (Thermo Scientific, United States), and the primers used in the qRT-PCR experiment are listed in Supplementary Table S1. The Ct values were analyzed by using the 2−ΔΔCt method (Livak and Schmittgen, 2001).
The binding of E. peplus compounds with hexokinase II (PDB ID: 2NZT), NF-κB–DNA complex (PDB ID 1LE9), and PPARγ (PDB ID: 2PRG) was investigated as previously reported (Supplementary Material) (Sami et al., 2022; Abduh et al., 2023).
The obtained results are presented as mean ± standard deviation (SD), and all statistical comparisons were made using one-way ANOVA followed by post hoc Tukey’s test on GraphPad Prism 8 software. p-values < 0.05 were considered statistically significant.
The analysis of the EA fraction of E. peplus led to the isolation of seven known flavonoids. Structures of isolated compounds (1-7) were elucidated based on spectroscopic data (Supplementary Figures S2–15) and by comparison with those previously reported. The isolated flavonoids (Figure 1A) were identified as isoquercetin (1) (Han et al., 2004), myricitrin (2) (Fu et al., 2013), astragalin (3) (Wei et al., 2011), quercitrin (4) (Tatsis et al., 2007), quercetin (5) (Mabry et al., 1970), kaempferol (6) (Mabry et al., 1970; Elsayed et al., 2020), and methyl gallate (7) (Ma et al., 2005). The in vitro RSA showed a concentration-dependent antioxidant activity of EPE against DPPH (Figure 1C) and ABTS (Figure 1D) radicals with IC50 values of 51.30 and 27.78 μg/ml, respectively.
FIGURE 1. (A) Chemical structure of the isolated compounds. (B, C) DPPH and ABTS radical-scavenging activities of EPE. Data are mean ± SD (N = 3).
OGTT was performed, and insulin was measured to determine the anti-hyperglycemic effect of EPE. The HFD/STZ-induced diabetic rats exhibited significant elevation in BG (Figure 2A, B). Treatment with EPE and PIO effectively ameliorated BG levels in diabetic rats (p < 0.001). Insulin was declined in diabetic rats (p < 0.001; Figure 2C), and the value of HOMA-IR was elevated (Figure 2D). All doses of EPE effectively alleviated insulin and HOMA-IR (p < 0.001). EPE didn’t alter glucose and insulin in normal animals.
FIGURE 2. EPE ameliorated glucose intolerance (A and B), serum insulin (C), and HOMA-IR (D) in diabetic rats. Data are mean ± SD (n = 6). *p < 0.05 and ***p < 0.001 vs. control. #p < 0.05, ##p < 0.01, and ###p < 0.001 vs. diabetic.
The activity of hexokinase (Figure 3A) was decreased, and G-6-Pase (Figure 3B), F-1,6-BPase (Figure 3C), and glycogen phosphorylase (Figure 3D) were activated in the diabetic rat liver (p < 0.001). Liver glycogen was decreased in diabetic rats as compared to the non-diabetic animals (p < 0.001; Figure 3E). EPE remarkably increased hexokinase and glycogen and suppressed other enzymes in diabetic rats.
FIGURE 3. EPE increased hexokinase (A), decreased G-6-Pase (B), F-1,6-BPase (C), and glycogen phosphorylase (D) and enhanced glycogen (E) in the liver of diabetic rats. Data are mean ± SD (n = 6). *p < 0.05, **p < 0.01, and ***p < 0.001 vs. control. ##p < 0.01 and ###p < 0.001 vs. diabetic.
MD simulations showed the binding affinity of EPE flavonoids with hexokinase as shown in Table 1 and Figure 4 and Supplementary Figure S16. Compounds 3, 4, 5, and 6 exhibited the lowest binding energy (−7.4, −7.3, −8.1, and −7.8 kcal/mol, respectively) and formed multiple polar bonding and hydrophobic interactions with different amino acid residues (Table 1).
TABLE 1. Binding affinities, interacting polar residues, and hydrophobic interactions of the compounds isolated from E. peplus with hexokinase.
TG, TC, LDL-C, and vLDL-C were increased in the serum of diabetic rats (p < 0.001) as shown in Figures 5A–D. HDL-C was decreased (p < 0.01; Figure 5E), and AIP was elevated (p < 0.001; Figure 5F) in diabetic animals. All doses of EPE decreased serum lipids and AIP and increased HDL-C in diabetic rats. Dyslipidemia was associated with increased liver TG (Figure 6A) and cholesterol (Figure 6B). Likewise, the stained sections of the liver of diabetic rats revealed the deposition of lipids (Figure 6C) along with increased circulating transaminases (Figures 6D,E; p < 0.001). All doses of EPE decreased liver lipids and serum transaminases in diabetic rats.
FIGURE 5. EPE decreased serum TG (A), TC (B), LDL-C (C), vLDL-C (D), and AIP (F) and increased HDL-C (E) in diabetic rats. Data are mean ± SD (n = 6). *p < 0.05, **p < 0.01, and ***p < 0.001 vs. control. ##p < 0.01 and ###p < 0.001 vs. diabetic.
FIGURE 6. EPE decreased liver TG (A) and cholesterol (B), prevented lipid deposition (C), and ameliorated serum ALT (D) and AST (E) in diabetic rats. Data are mean ± SD (n = 6). *p < 0.05, **p < 0.01, and ***p < 0.001 vs. control. ###p < 0.001 vs. diabetic.
MDA and NO were elevated in HFD/STZ-induced rats (p < 0.001) as compared to the control rats (Figures 7A,B). In contrast, GSH (Figure 7C), SOD (Figure 7D), CAT (Figure 7E), and GPx (Figure 7F) were decreased in diabetic animals. EPE decreased MDA and NO and increased antioxidants effectively in diabetic rats while showing no effect on normal animals.
FIGURE 7. EPE decreased liver MDA (A) and NO (B) and increased GSH (C), SOD (D), CAT (E), and GPx (F) in diabetic rats. Data are mean ± SD (n = 6). *p < 0.05, **p < 0.01, and ***p < 0.001 vs. control. #p < 0.05, ##p < 0.01, and ###p < 0.001 vs. diabetic.
Liver NF-kB p65 and serum TNF-α and IL-1β were upregulated in diabetic rats as depicted in Figures 8A–C. Treatment with EPE noticeably decreased the assayed inflammatory markers in rats with diabetes. The binding affinity of the isolated flavonoids toward NF-kB was investigated with MD (Table 2; Figure 9 and Supplementary Figure S17). All compounds showed binding affinity marked by the polar bonding and hydrophobic interactions, and compounds 2, 3, 4, and 5 showed the lowest binding energy (−9.5, −10.6, −9.8, and −9.6 kcal/mol, respectively).
FIGURE 8. EPE decreased liver NF-kB p65 (A) and serum TNF-α (B) and IL-1β (C) in diabetic rats. Data are mean ± SD (n = 6). *p < 0.05, **p < 0.01, and ***p < 0.001 vs. control. ###p < 0.001 vs. diabetic.
TABLE 2. Binding affinities, interacting polar residues, and hydrophobic interactions of the compounds isolated from E. peplus with the NF-κB–DNA complex.
FIGURE 9. Molecular docking showing the binding modes of compounds 2, 3, 4, and 5 with the NF-κB–DNA complex.
Circulating adiponectin was declined in rats with diabetes, and all EPE doses effectively restored its levels (Figure 10A). The effect of EPE on PPARγ and the binding affinity of the isolated flavonoids were determined using qRT-PCR and MD, respectively. As shown in Figure 10B, diabetic rats exhibited significant downregulation of liver PPARγ, an effect that was reversed following treatment with all doses of EPE and the PPARγ agonist PIO. MD revealed the affinity of E. peplus flavonoids toward PPARγ, and compounds 3, 4, and 5 exhibited the lowest binding energy (−8.7, −8.0, and −8.0 kcal/mol, respectively) (Table 3; Figure 10C and Supplementary Figure S18I).
FIGURE 10. EPE increased serum adiponectin (A) and upregulated liver PPARγ mRNA (B) in diabetic rats. Data are mean ± SD (n = 6). *p < 0.05 and ***p < 0.001 vs. control. ##p < 0.01 and ###p < 0.001 vs. diabetic. (C) Molecular docking showing the binding modes of compounds 2, 3, and 4 with PPARγ.
TABLE 3. Binding affinities, interacting polar residues, and hydrophobic interactions of the compounds isolated from E. peplus with PPARγ.
Plants of the genus Euphorbia showed a very promising anti-diabetic effect in STZ-, alloxan-, and sucrose-induced DM in rats (Kumar et al., 2010; Zafar et al., 2021; Mustafa et al., 2022), and the LD50 of most Euphorbia species was estimated to exceed 5,000 mg/kg (Abd-Elhakim et al., 2019). Herein, we explored the ameliorative effect of the flavonoid-rich fraction of E. peplus on hyperglycemia, IR, OS, and inflammation in HFD/STZ-induced T2D rats. The in vitro assays showed that EPE scavenged DPPH radicals in a concentration-dependent manner. Previous studies showed the DPPH radical-scavenging efficacy of plants of the genus Euphorbia such as E. royleana (Zafar et al., 2021). The DPPH assay data were supported by the ability of EPE to scavenge ABTS radicals, demonstrating its powerful RSA. ABTS assay is more reliable and accurate for the evaluation of RSA of phytoconstituents than DPPH (Floegel et al., 2011). The RSA of EPE could be directly related to the rich content of flavonoids that possess potent scavenging properties against free radicals (Kamel et al., 2016; Elsayed et al., 2020).
The effect of EPE on glucose intolerance and IR was investigated in vivo in rats with HFD/STZ-induced diabetes. HFD and STZ were employed to induce T2D as this model showed similarities to the disease in humans. Feeding a HFD results in IR, and STZ decreases insulin release by damaging β-cells (Breyer et al., 2005; Lee et al., 2011), leading to hyperglycemia. Together with IR, hyperglycemia is a characteristic feature of T2D and should be managed to prevent complications in different organs (Jellinger, 2007). Here, HFD/STZ-challenged animals showed hyperglycemia marked by glucose intolerance and IR. The developed T2D was consistent with our previous investigations, showing IR and hyperglycemia in HFD/STZ-induced rats (Mahmoud et al., 2012; Germoush et al., 2019; Elsayed et al., 2020; Abduh et al., 2023). The chronic hyperglycemia in this model was supported by the values of HbA1c%, a reliable marker for both diagnosis and prognosis of DM (American Diabetes Association, 2014) reported in our recent work (Abduh et al., 2023). Hyperglycemia was associated with hypoinsulinemia, and the development of IR as the value of HOMA-IR was revealed. Similar to these findings, elevated glucose, HbA1c%, and HOMA-IR along with decreased insulin were reported in HFD/STZ-challenged rats (Abduh et al., 2023). The declined insulin is due to damage caused to the pancreatic islets induced by STZ-mediated ROS generation and DNA damage (Lenzen, 2008). Although the early phase of damage is associated with increased insulin release as a compensatory mechanism, prolonged hyperglycemia and ROS release deteriorate the pancreatic islets and promote more β-cell damage and ultimately reduced insulin release (Ntimbane et al., 2016). The effects of excessive ROS include enhanced lipid peroxidation (LPO), massively increased cytosolic Ca2+, and diminished pancreatic antioxidants, effects that enhance the destruction of β-cells (Nahdi et al., 2017).
Treatment with EPE effectively ameliorated glucose intolerance and HOMA-IR, denoting its anti-hyperglycemic and insulin-sensitizing effects. These effects added support to the previously reported anti-hyperglycemic activity of plants of the same genus. For instance, E. royleana stem extract decreased fasting BG (FBG) and ameliorated glucose intolerance in diabetic rats (Zafar et al., 2021), and E. helioscopia alleviated BG and insulin in sucrose-fed rats (Mustafa et al., 2022). The ameliorative effect of EPE on hyperglycemia is a direct result of increased insulin secretion. Impaired insulin release and IR increase hepatic glucose output due to suppressed glycolysis and glycogenesis. Impaired insulin release and IR can also impair peripheral glucose uptake and hepatic gluconeogenesis, resulting in hyperglycemia (Nordlie et al., 1999). By alleviating insulin release and IR, EPE effectively ameliorated hyperglycemia possibly by modulating enzymes involved in glycogenesis and gluconeogenesis. This notion was supported by the findings of this study where EPE increased hexokinase and suppressed F-1,6-BPase, G-6-Pase, and glycogen phosphorylase, resulting in increased liver glycogen content. Hexokinase is involved in glucose oxidation and suppressed by IR and insulin deficiency. Suppressed hexokinase activity decreases glycolysis, and hence glucose accumulates in the blood (Gupta et al., 1999). Along with hexokinase suppression, insulin insufficiency activates G-6-Pase, F-1,6-BPase, and glycogen phosphorylase, resulting in enhanced gluconeogenesis and glycogenolysis (Roden and Bernroider, 2003). The improved insulin sensitivity and levels of EPE decreased glycogenolysis and gluconeogenesis and enhanced liver glycogen by modulating the activity of the involved enzymes. In addition to the determined enzymes, insulin activates glycogen synthase and suppresses glycogen phosphorylase (Postic et al., 2004), and this explains the alleviated glycogen levels following treatment with EPE. Owing to its role in glucose oxidation, the ameliorated FBG following EPE supplementation is a result of enhanced hexokinase activity. To further explore the effect of EPE on hexokinase activity, we carried out MD simulations of the binding affinity of the contained flavonoids toward the enzyme. All flavonoids revealed binding affinity marked by polar bonding toward important residues in the active site and dense hydrophobic interactions. Recent findings showed improvements in glycemic status and insulin sensitivity by plant extracts that modulate the carbohydrate-metabolizing enzymes (Germoush et al., 2019; Elsayed et al., 2020). In this context, Mustafa et al. (2022) related the anti-hyperglycemic effect of E. helioscopia in sucrose-fed rats to its ability to modulate the activities of pyruvate kinase, glucokinase, and phosphofructokinase.
In addition to hyperglycemia, dyslipidemia is found in T2D and can increase atherogenicity and the risk of cardiovascular disease (Reaven, 2005). Elevated serum lipids and decreased HDL-C in this study represent an atherogenic profile as previously described (Germoush et al., 2019). AIP, a marker of lipoprotein particle size that possesses a predictive value beyond that of the assayed lipids (Dobiásová, 2006), was increased in diabetic rats. The observed dyslipidemia is a direct result of IR and the enhanced lipolysis and decreased lipogenesis (Carpentier, 2021). Increased lipolysis provokes liver lipid accumulation which is also promoted by increased synthesis of free fatty acids (FFAs) that provoke lipogenesis within hepatocytes (Mohamed et al., 2016). Lipid accumulation in hepatocytes causes cell injury, thereby aggravating IR, hyperglycemia, and dyslipidemia (Levinthal and Tavill, 1999). Herein, lipids were increased in the liver, and circulating transaminases were elevated in diabetic rats as previously reported (Elsayed et al., 2020; Abduh et al., 2023). EPE effectively ameliorated serum and liver lipids, effects that were directly related to the enhanced insulin release and sensitivity.
Owing to the involvement of OS and inflammation in provoking IR and the complications of DM (Mahmoud et al., 2012; Mahmoud, 2017), we explored the ability of EPE to suppress these pathological processes. Diabetic rats showed OS and inflammatory reactions marked by elevated MDA, NO, NF-kB, TNF-α, and IL-1β and declined antioxidants. OS, defined by excess ROS and decreased antioxidants, is a key mechanism in IR and can damage cells and alter multiple signaling pathways. Hyperglycemia can increase the production of ROS and lead to OS by activating NADPH oxidases and promoting mitochondrial dysfunction (Jimenez et al., 2018). Excess ROS can activate pathways related to increased pro-inflammatory cytokines, and both can impair insulin signaling, leading to IR and glucose accumulation in the blood (Rösen et al., 2001). The altered insulin levels shift the signaling where PI3K phosphorylates Rac, resulting in increased NADPH oxidase 4-mediated ROS generation (Campa et al., 2015). Excess ROS activates casein kinase-2 followed by retromer that alters glucose transporter-4 membrane translocation and impair glucose uptake (Ma et al., 2014). ROS can also increase mitochondrial fission that stimulates stress responses and impairs insulin signaling and has been linked to IR as well as apoptosis (Jheng et al., 2012). Pro-inflammatory cytokines trigger IR by altering insulin signaling and many kinases. The elevated IL-1β and TNF-α reported in this study can impair insulin-stimulated uptake of glucose, stimulate lipolysis and gluconeogenesis, and inhibit tyrosine phosphorylation of insulin receptor substrate-1 and protein kinase B activation (Green et al., 1994; Del Aguila et al., 1999; Jager et al., 2007). Therefore, attenuation of OS and pro-inflammatory cytokines can attenuate IR and increase insulin signaling, activity, and stimulated glucose uptake.
EPE enhanced antioxidants and prevented OS and inflammation in diabetic rats in this investigation. In addition to its in vitro RSA, EPE prevented LPO, enhanced antioxidants, and suppressed NF-kB and cytokines in diabetic rats. The suppression of inflammation following EPE supplementation was supported by in silico investigations that showed the ability of flavonoids to bind strongly with NF-kB through multiple polar bonding and hydrophobic interactions. The attenuation of these pathological processes contributed to the anti-hyperglycemic and insulin-sensitizing effects of EPE. Numerous studies showed the beneficial effects of antioxidants and plant extracts that are rich in antioxidant phytochemicals against hyperglycemia and IR (Mahmoud, 2013; Mahmoud et al., 2017; Germoush et al., 2019). The antioxidant and anti-inflammatory role of EPE is related to its content of flavonoids which possess potent RSA and showed benefits against DM (Mahmoud, 2013; Mahmoud et al., 2017; Germoush et al., 2019; Abukhalil et al., 2021). In diabetic patients, the supplementation of flavonoids improved glycemic and lipidemic statuses and antioxidants and decreased inflammatory markers (Li et al., 2015). In obese patients, the consumption of flavonoids positively affected the metabolic status by lowering systemic oxidation and enhancing insulin sensitivity (Suliburska et al., 2012).
The beneficial effects of EPE could also be linked to the upregulation of adiponectin and PPARγ. EPE increased serum adiponectin that participated, at least in part, in the amelioration of hyperglycemia. Adiponectin exerts insulin-sensitizing effects and possesses anti-inflammatory activity, and experimental evidence revealed that it ameliorated hyperglycemia in HFD-fed rodents (Fruebis et al., 2001; Yamauchi et al., 2001). Despite its ameliorated hyperglycemia in T1D and T2D in rodents, high adiponectin doses didn’t affect BG in normal animals. These findings suggested that the downregulation of glycogenolysis and gluconeogenesis mediated its anti-hyperglycemic effects. Accordingly, adiponectin decreased glucose production in rat hepatocytes and G-6-Pase mRNA abundance in mice (Berg et al., 2001; Combs et al., 2001). It can also upregulate liver CD36, PPARα, and UCP-2, effects that were related to the increase in insulin sensitivity (Yamauchi et al., 2001). EPE upregulated liver PPARγ, and its flavonoids were shown to dock into the PPARγ active site through polar bonding and hydrophobic interactions. The activation of PPARγ is a key mechanism for ameliorating hyperglycemia, and IR and PPARγ agonists, such as PIO, increase insulin sensitivity and ameliorate hyperglycemia, dyslipidemia, OS, and inflammation (Tontonoz and Spiegelman, 2008). PPARγ suppresses OS and inflammation by enhancing antioxidant enzymes (Okuno et al., 2010), inhibiting the activation of NF-κB both directly and indirectly (Kersten et al., 2000; Remels et al., 2009), and preventing ROS generation from NADPH oxidases (Hwang et al., 2005). However, the lack of PPARγ protein expression data could be considered a limitation to this study.
This investigation introduces new information that E. peplus is rich in flavonoids and possesses potent radical-scavenging and anti-diabetic efficacies. EPE ameliorated hyperglycemia, IR, OS, dyslipidemia, and inflammation in rats with T2D. In addition, EPE modulated carbohydrate-metabolizing enzymes and enhanced antioxidants, adiponectin, and PPARγ. In silico findings revealed the binding affinity of E. peplus constituents toward hexokinase, NF-kB, and PPARγ. Therefore, E. peplus could be a promising candidate for the development of a potent anti-hyperglycemic and insulin-sensitizing agent. However, further investigations to determine other molecular mechanism(s) of action are needed.
The original contributions presented in the study are included in the article/Supplementary Material; further inquiries can be directed to the corresponding author.
The animal study was reviewed and approved by the Research Ethics Committee of Al-Azhar University.
Conceptualization, AM, EK, and RA; methodology, AM, RA, MA, GM-H, AB-A, and EK; software, AM and EK; validation, AM; formal analysis, RA, MA, AM, and EK; investigation, RA, AM, GM-H, MA, AB-A, and EK; resources, RA, GM-H, MA, and EH; data curation, AM, EK, and RA; writing—original draft and preparation, AM, GM-H, and EK; writing—review and editing, AM; visualization, AM; supervision, AM; project administration, RA and AM; funding acquisition, RA. All authors contributed to the article and approved the submitted version.
This research was funded by the Nourah Bint Abdulrahman University Researchers Supporting Project (grant number PNURSP 2023R381), Princess Nourah Bint Abdulrahman University, Riyadh, Saudi Arabia.
Princess Nourah bint Abdulrahman University Researchers Supporting Project number (PNURSP2023R381), Princess Nourah bint Abdulrahman University, Riyadh, Saudi Arabia.
The authors declare that the research was conducted in the absence of any commercial or financial relationships that could be construed as a potential conflict of interest.
All claims expressed in this article are solely those of the authors and do not necessarily represent those of their affiliated organizations, or those of the publisher, the editors, and the reviewers. Any product that may be evaluated in this article, or claim that may be made by its manufacturer, is not guaranteed or endorsed by the publisher.
The Supplementary Material for this article can be found online at: https://www.frontiersin.org/articles/10.3389/fphar.2023.1204641/full#supplementary-material
Abd El-Twab, S. M., Mohamed, H. M., and Mahmoud, A. M. (2016). Taurine and pioglitazone attenuate diabetes-induced testicular damage by abrogation of oxidative stress and up-regulation of the pituitary-gonadal axis. Can. J. Physiol. Pharmacol. 94, 651–661. doi:10.1139/cjpp-2015-0503
Abd-Elhakim, Y. M., Abdo Nassan, M., Salem, G. A., Sasi, A., Aldhahrani, A., Ben Issa, K., et al. (2019). Investigation of the in-vivo cytotoxicity and the in silico-prediction of MDM2-p53 inhibitor potential of Euphorbia peplus methanolic extract in rats. Toxins (Basel) 11, 642. doi:10.3390/toxins11110642
Abduh, M. S., Alzoghaibi, M. A., Alzoghaibi, A. M., Bin-Ammar, A., Alotaibi, M. F., Kamel, E. M., et al. (2023). Arbutin ameliorates hyperglycemia, dyslipidemia and oxidative stress and modulates adipocytokines and PPARγ in high-fat diet/streptozotocin-induced diabetic rats. Life Sci. 321, 121612. doi:10.1016/j.lfs.2023.121612
Abukhalil, M. H., Althunibat, O. Y., Aladaileh, S. H., Al-Amarat, W., Obeidat, H. M., Al-Khawalde, A. a. A., et al. (2021). Galangin attenuates diabetic cardiomyopathy through modulating oxidative stress, inflammation and apoptosis in rats. Biomed. Pharmacother. 138, 111410. doi:10.1016/j.biopha.2021.111410
Aebi, H. (1984). “[13] catalase in vitro,” in Methods in enzymology (Cambridge: Academic Press), 121–126.
Ahmed, S., Yousaf, M., Mothana, R. A., and Al-Rehaily, A. J. (2016). Studies on wound healing activity of some EUPHORBIA species on experimental rats. Afr. J. Tradit. Complement. Altern. Med. 13, 145–152. doi:10.21010/ajtcam.v13i5.19
Al-Sultan, S., and Hussein, Y. A. (2006). Acute toxicity of Euphorbia heliscopia in rats. Pak. J. Nutr. 5, 135–140. doi:10.3923/pjn.2006.135.140
American Diabetes Association (2021). 2. Classification and diagnosis of diabetes: Standards of medical care in diabetes-2021. Diabetes Care 44, S15–S33. doi:10.2337/dc21-S002
American Diabetes Association (2014). Standards of medical care in diabetes-2014. Diabetes Care 37, S14–S80. doi:10.2337/dc14-S014
Bancroft, J. D., and Gamble, M. (2008). Theory and practice of histological techniques. Netherlands: Elsevier health sciences.
Berg, A. H., Combs, T. P., Du, X., Brownlee, M., and Scherer, P. E. (2001). The adipocyte-secreted protein Acrp30 enhances hepatic insulin action. Nat. Med. 7, 947–953. doi:10.1038/90992
Beutler, E., Duron, O., and Kelly, B. M. (1963). Improved method for the determination of blood glutathione. J. laboratory Clin. Med. 61, 882–888.
Brand-Williams, W., Cuvelier, M. E., and Berset, C. (1995). Use of a free radical method to evaluate antioxidant activity. LWT - Food Sci. Technol. 28, 25–30. doi:10.1016/s0023-6438(95)80008-5
Brandstrup, N., Kirk, J. E., and Bruni, C. (1957). The hexokinase and phosphoglucoisomerase activities of aortic and pulmonary artery tissue in individuals of various ages. J. Gerontol. 12, 166–171. doi:10.1093/geronj/12.2.166
Breyer, M. D., Böttinger, E., Brosius, F. C., Coffman, T. M., Harris, R. C., Heilig, C. W., et al. (2005). Mouse models of diabetic nephropathy. J. Am. Soc. Nephrol. 16, 27–45. doi:10.1681/ASN.2004080648
Campa, C. C., Ciraolo, E., Ghigo, A., Germena, G., and Hirsch, E. (2015). Crossroads of PI3K and rac pathways. Small GTPases 6, 71–80. doi:10.4161/21541248.2014.989789
Carpentier, A. C. (2021). 100th anniversary of the discovery of insulin perspective: Insulin and adipose tissue fatty acid metabolism. Am. J. Physiology-Endocrinology Metabolism 320, E653–E670. doi:10.1152/ajpendo.00620.2020
Combs, T. P., Berg, A. H., Obici, S., Scherer, P. E., and Rossetti, L. (2001). Endogenous glucose production is inhibited by the adipose-derived protein Acrp30. J. Clin. Invest. 108, 1875–1881. doi:10.1172/JCI14120
Del Aguila, L. F., Claffey, K. P., and Kirwan, J. P. (1999). TNF-alpha impairs insulin signaling and insulin stimulation of glucose uptake in C2C12 muscle cells. Am. J. Physiology - Endocrinol. Metabolism 276, E849–E855. doi:10.1152/ajpendo.1999.276.5.E849
Dobiásová, M. (2006). AIP-atherogenic index of plasma as a significant predictor of cardiovascular risk: From research to practice. Vnitr Lek. 52, 64–71.
Elsayed, R. H., Kamel, E. M., Mahmoud, A. M., El-Bassuony, A. A., Bin-Jumah, M., Lamsabhi, A. M., et al. (2020). Rumex dentatus L. phenolics ameliorate hyperglycemia by modulating hepatic key enzymes of carbohydrate metabolism, oxidative stress and PPARγ in diabetic rats. Food Chem. Toxicol. 138, 111202. doi:10.1016/j.fct.2020.111202
Floegel, A., Kim, D.-O., Chung, S.-J., Koo, S. I., and Chun, O. K. (2011). Comparison of ABTS/DPPH assays to measure antioxidant capacity in popular antioxidant-rich US foods. J. food Compos. analysis 24, 1043–1048. doi:10.1016/j.jfca.2011.01.008
Flohé, L., and Günzler, W. A. (1984). Assays of glutathione peroxidase. Methods Enzymol. 105, 114–121. doi:10.1016/s0076-6879(84)05015-1
Folch, J., Lees, M., and Sloane Stanley, G. H. (1957). A simple method for the isolation and purification of total lipides from animal tissues. J. Biol. Chem. 226, 497–509. doi:10.1016/s0021-9258(18)64849-5
Freedland, R. A., and Harper, A. E. (1959). Metabolic adaptations in higher animals. J. Biol. Chem. 234, 1350–1354. doi:10.1016/s0021-9258(18)70010-0
Fruebis, J., Tsao, T. S., Javorschi, S., Ebbets-Reed, D., Erickson, M. R., Yen, F. T., et al. (2001). Proteolytic cleavage product of 30-kDa adipocyte complement-related protein increases fatty acid oxidation in muscle and causes weight loss in mice. Proc. Natl. Acad. Sci. U. S. A. 98, 2005–2010. doi:10.1073/pnas.041591798
Fu, Y., Li, Z., Si, J., Chang, Q., Li, Z., and Pan, R. (2013). Separation and purification of myricitrin from bayberry tree bark by high-speed counter-current chromatography. J. Liq. Chromatogr. Relat. Technol. 36, 1503–1512. doi:10.1080/10826076.2012.692147
Germoush, M. O., Elgebaly, H. A., Hassan, S., Kamel, E. M., Bin-Jumah, M., and Mahmoud, A. M. (2019). Consumption of terpenoids-rich padina pavonia extract attenuates hyperglycemia, insulin resistance and oxidative stress, and upregulates PPARγ in a rat model of type 2 diabetes. Antioxidants (Basel) 9, 22. doi:10.3390/antiox9010022
Giner, J. L., and Schroeder, T. N. (2015). Polygonifoliol, a new tirucallane triterpene from the latex of the seaside sandmat Euphorbia polygonifolia. Chem. Biodivers. 12, 1126–1129. doi:10.1002/cbdv.201400426
Green, A., Dobias, S. B., Walters, D. J. A., and Brasier, A. R. (1994). Tumor necrosis factor increases the rate of lipolysis in primary cultures of adipocytes without altering levels of hormone-sensitive lipase. Endocrinology 134, 2581–2588. doi:10.1210/endo.134.6.8194485
Green, L. C., Wagner, D. A., Glogowski, J., Skipper, P. L., Wishnok, J. S., and Tannenbaum, S. R. (1982). Analysis of nitrate, nitrite, and [15N]nitrate in biological fluids. Anal. Biochem. 126, 131–138. doi:10.1016/0003-2697(82)90118-x
Gupta, D., Raju, J., Prakash, J., and Baquer, N. Z. (1999). Change in the lipid profile, lipogenic and related enzymes in the livers of experimental diabetic rats: Effect of insulin and vanadate. Diabetes Res. Clin. Pract. 46, 1–7. doi:10.1016/s0168-8227(99)00067-4
Guzik, T. J., and Cosentino, F. (2018). Epigenetics and immunometabolism in diabetes and aging. Antioxid. Redox Signal 29, 257–274. doi:10.1089/ars.2017.7299
Haffner, S. M. (2000). Coronary heart disease in patients with diabetes. N. Engl. J. Med. 342, 1040–1042. doi:10.1056/NEJM200004063421408
Han, J.-T., Bang, M.-H., Chun, O.-K., Kim, D.-O., Lee, C.-Y., and Baek, N.-I. (2004). Flavonol glycosides from the aerial parts ofAceriphyllum rossii and their antioxidant activities. Archives Pharmacal Res. 27, 390–395. doi:10.1007/BF02980079
Hwang, J., Kleinhenz, D. J., Lassegue, B., Griendling, K. K., Dikalov, S., and Hart, C. M. (2005). Peroxisome proliferator-activated receptor-gamma ligands regulate endothelial membrane superoxide production. Am. J. Physiol. Cell Physiol. 288, C899–C905. doi:10.1152/ajpcell.00474.2004
Jager, J., Grémeaux, T., Cormont, M., Le Marchand-Brustel, Y., and Tanti, J. F. (2007). Interleukin-1beta-induced insulin resistance in adipocytes through down-regulation of insulin receptor substrate-1 expression. Endocrinology 148, 241–251. doi:10.1210/en.2006-0692
Jellinger, P. S. (2007). Metabolic consequences of hyperglycemia and insulin resistance. Clin. Cornerstone 8, S30–S42. doi:10.1016/s1098-3597(07)80019-6
Jheng, H. F., Tsai, P. J., Guo, S. M., Kuo, L. H., Chang, C. S., Su, I. J., et al. (2012). Mitochondrial fission contributes to mitochondrial dysfunction and insulin resistance in skeletal muscle. Mol. Cell Biol. 32, 309–319. doi:10.1128/MCB.05603-11
Jimenez, R., Toral, M., Gómez-Guzmán, M., Romero, M., Sanchez, M., Mahmoud, A. M., et al. (2018). The role of Nrf2 signaling in pparβ/δ-mediated vascular protection against hyperglycemia-induced oxidative stress. Oxid. Med. Cell Longev. 2018, 5852706. doi:10.1155/2018/5852706
Kahn, S. E., Cooper, M. E., and Del Prato, S. (2014). Pathophysiology and treatment of type 2 diabetes: Perspectives on the past, present, and future. Lancet 383, 1068–1083. doi:10.1016/S0140-6736(13)62154-6
Kamel, E. M., Ahmed, N. A., El-Bassuony, A. A., Hussein, O. E., Alrashdi, B., Ahmed, S. A., et al. (2022). Xanthine oxidase inhibitory activity of Euphorbia peplus L. Phenolics. Comb. Chem. High. Throughput Screen 25, 1336–1344. doi:10.2174/1386207324666210609104456
Kamel, E. M., Mahmoud, A. M., Ahmed, S. A., and Lamsabhi, A. M. (2016). A phytochemical and computational study on flavonoids isolated from Trifolium resupinatum L. and their novel hepatoprotective activity. Food Funct. 7, 2094–2106. doi:10.1039/c6fo00194g
Kersten, S., Desvergne, B., and Wahli, W. (2000). Roles of PPARs in health and disease. Nature 405, 421–424. doi:10.1038/35013000
Koide, H., and Oda, T. (1959). Pathological occurrence of glucose-6-phosphatase in serum in liver diseases. Clin. Chim. Acta 4, 554–561. doi:10.1016/0009-8981(59)90165-2
Kumar, S., Malhotra, R., and Kumar, D. (2010). Antidiabetic and free radicals scavenging potential of Euphorbia hirta flower extract. Indian J. Pharm. Sci. 72, 533–537. doi:10.4103/0250-474X.73921
Lee, Y. S., Li, P., Huh, J. Y., Hwang, I. J., Lu, M., Kim, J. I., et al. (2011). Inflammation is necessary for long-term but not short-term high-fat diet-induced insulin resistance. Diabetes 60, 2474–2483. doi:10.2337/db11-0194
Lenzen, S. (2008). The mechanisms of alloxan- and streptozotocin-induced diabetes. Diabetologia 51, 216–226. doi:10.1007/s00125-007-0886-7
Levinthal, G. N., and Tavill, A. S. (1999). Liver disease and diabetes mellitus. Clin. DIABETES 17, 73–81.
Li, D., Zhang, Y., Liu, Y., Sun, R., and Xia, M. (2015). Purified anthocyanin supplementation reduces dyslipidemia, enhances antioxidant capacity, and prevents insulin resistance in diabetic patients. J. Nutr. 145, 742–748. doi:10.3945/jn.114.205674
Livak, K. J., and Schmittgen, T. D. (2001). Analysis of relative gene expression data using real-time quantitative PCR and the 2(-Delta Delta C(T)) Method. Methods 25, 402–408. doi:10.1006/meth.2001.1262
Ma, J., Nakagawa, Y., Kojima, I., and Shibata, H. (2014). Prolonged insulin stimulation down-regulates GLUT4 through oxidative stress-mediated retromer inhibition by a protein kinase CK2-dependent mechanism in 3T3-L1 adipocytes. J. Biol. Chem. 289, 133–142. doi:10.1074/jbc.M113.533240
Ma, X., Wu, L., Ito, Y., and Tian, W. (2005). Application of preparative high-speed counter-current chromatography for separation of methyl gallate from Acer truncatum Bunge. J. Chromatogr. A 1076, 212–215. doi:10.1016/j.chroma.2005.04.077
Mabry, T. J., Markham, K., Thomas, M., Mabry, T. J., Markham, K., and Thomas, M. (1970). “The NMR spectra of flavonoids,” in The systematic identification of flavonoids (Berlin, Heidelberg: Springer), 274–343.
Mahmoud, A. M., Abd El-Twab, S. M., and Abdel-Reheim, E. S. (2017). Consumption of polyphenol-rich morus alba leaves extract attenuates early diabetic retinopathy: The underlying mechanism. Eur. J. Nutr. 56, 1671–1684. doi:10.1007/s00394-016-1214-0
Mahmoud, A. M., Ashour, M. B., Abdel-Moneim, A., and Ahmed, O. M. (2012). Hesperidin and naringin attenuate hyperglycemia-mediated oxidative stress and proinflammatory cytokine production in high fat fed/streptozotocin-induced type 2 diabetic rats. J. Diabetes its Complicat. 26, 483–490. doi:10.1016/j.jdiacomp.2012.06.001
Mahmoud, A. M. (2017). “Exercise amaliorates metabolic disturbances and oxidative stress in diabetic cardiomyopathy: Possible underlying mechanisms,” in Exercise for cardiovascular disease prevention and treatment: From molecular to clinical, Part 1. Editor J. Xiao (Singapore: Springer Singapore), 207–230.
Mahmoud, A. M. (2013). Hematological alterations in diabetic rats - role of adipocytokines and effect of citrus flavonoids. Excli J. 12, 647–657.
Marklund, S., and Marklund, G. (1974). Involvement of the superoxide anion radical in the autoxidation of pyrogallol and a convenient assay for superoxide dismutase. FEBS Eur. J. Biochem. 47, 469–474. doi:10.1111/j.1432-1033.1974.tb03714.x
Mohamed, J., Nazratun Nafizah, A. H., Zariyantey, A. H., and Budin, S. B. (2016). Mechanisms of Diabetes-Induced Liver Damage: The role of oxidative stress and inflammation. Sultan Qaboos Univ. Med. J. 16, e132–e141. doi:10.18295/squmj.2016.16.02.002
Mustafa, I., Anwar, H., Irfan, S., Muzaffar, H., and Ijaz, M. U. (2022). Attenuation of carbohydrate metabolism and lipid profile by methanolic extract of Euphorbia helioscopia and improvement of beta cell function in a type 2 diabetic rat model. BMC Complementary Med. Ther. 22, 23. doi:10.1186/s12906-022-03507-2
Nahdi, A. M. T. A., John, A., and Raza, H. (2017). Elucidation of molecular mechanisms of streptozotocin-induced oxidative stress, apoptosis, and mitochondrial dysfunction in rin-5F pancreatic β-cells. Oxidative Med. Cell. Longev. 2017, 7054272. doi:10.1155/2017/7054272
Nordlie, R. C., Foster, J. D., and Lange, A. J. (1999). Regulation of glucose production by the liver. Annu. Rev. Nutr. 19, 379–406. doi:10.1146/annurev.nutr.19.1.379
Ntimbane, T., Mailhot, G., Spahis, S., Rabasa-Lhoret, R., Kleme, M. L., Melloul, D., et al. (2016). CFTR silencing in pancreatic β-cells reveals a functional impact on glucose-stimulated insulin secretion and oxidative stress response. Am. J. Physiol. Endocrinol. Metab. 310, E200–E212. doi:10.1152/ajpendo.00333.2015
Ohkawa, H., Ohishi, N., and Yagi, K. (1979). Assay for lipid peroxides in animal tissues by thiobarbituric acid reaction. Anal. Biochem. 95, 351–358. doi:10.1016/0003-2697(79)90738-3
Okuno, Y., Matsuda, M., Miyata, Y., Fukuhara, A., Komuro, R., Shimabukuro, M., et al. (2010). Human catalase gene is regulated by peroxisome proliferator activated receptor-gamma through a response element distinct from that of mouse. Endocr. J. 57, 303–309. doi:10.1507/endocrj.k09e-113
Pattanaik, S., Si, S. C., Pal, A., Panda, J., and Nayak, S. S. (2014). Wound healing activity of methanolic extract of the leaves of Crataeva magna and Euphorbia nerifolia in rats. J. Appl. Pharm. Sci. 4, 046–049. doi:10.7324/japs.2014.40310
Postic, C., Dentin, R., and Girard, J. (2004). Role of the liver in the control of carbohydrate and lipid homeostasis. Diabetes Metabolism 30, 398–408. doi:10.1016/s1262-3636(07)70133-7
Re, R., Pellegrini, N., Proteggente, A., Pannala, A., Yang, M., and Rice-Evans, C. (1999). Antioxidant activity applying an improved ABTS radical cation decolorization assay. Free Radic. Biol. Med. 26, 1231–1237. doi:10.1016/s0891-5849(98)00315-3
Reaven, G. M. (2005). Compensatory hyperinsulinemia and the development of an atherogenic lipoprotein profile: The price paid to maintain glucose homeostasis in insulin-resistant individuals. Endocrinol. Metab. Clin. North Am. 34, 49–62. doi:10.1016/j.ecl.2004.12.001
Remels, A. H., Langen, R. C., Gosker, H. R., Russell, A. P., Spaapen, F., Voncken, J. W., et al. (2009). PPARgamma inhibits NF-kappaB-dependent transcriptional activation in skeletal muscle. Am. J. Physiol. Endocrinol. Metab. 297, E174–E183. doi:10.1152/ajpendo.90632.2008
Roden, M., and Bernroider, E. (2003). Hepatic glucose metabolism in humans-its role in health and disease. Best. Pract. Res. Clin. Endocrinol. Metab. 17, 365–383. doi:10.1016/s1521-690x(03)00031-9
Rösen, P., Nawroth, P. P., King, G., Möller, W., Tritschler, H. J., and Packer, L. (2001). The role of oxidative stress in the onset and progression of diabetes and its complications: Asummary of a congress series sponsored byUNESCO-MCBN, the American diabetes association and the German diabetes society. Diabetes/Metabolism Res. Rev. 17, 189–212. doi:10.1002/dmrr.196
Saeedi, P., Petersohn, I., Salpea, P., Malanda, B., Karuranga, S., Unwin, N., et al. (2019). Global and regional diabetes prevalence estimates for 2019 and projections for 2030 and 2045: Results from the international diabetes federation diabetes atlas, 9th edition. Diabetes Res. Clin. Pract. 157, 107843. doi:10.1016/j.diabres.2019.107843
Sami, D. H., Soliman, A. S., Khowailed, A. A., Hassanein, E. H., Kamel, E. M., and Mahmoud, A. M. J. L. S. (2022). 7-hydroxycoumarin modulates Nrf2/HO-1 and microRNA-34a/SIRT1 signaling and prevents cisplatin-induced oxidative stress, inflammation, and kidney injury in rats. Life Sci. 310, 121104. doi:10.1016/j.lfs.2022.121104
Seifter, S., Dayton, S., et al. (1950). The estimation of glycogen with the anthrone reagent. Arch. Biochem. 25, 191–200.
Shi, Q. W., Su, X. H., and Kiyota, H. (2008). Chemical and pharmacological research of the plants in genus Euphorbia. Chem. Rev. 108, 4295–4327. doi:10.1021/cr078350s
Stalmans, W., and Hers, H. G. (1975). The stimulation of liver phosphorylase b by AMP, fluoride and sulfate. A technical note on the specific determination of the a and b forms of liver glycogen phosphorylase. Eur. J. Biochem. 54, 341–350. doi:10.1111/j.1432-1033.1975.tb04144.x
Suliburska, J., Bogdanski, P., Szulinska, M., Stepien, M., Pupek-Musialik, D., and Jablecka, A. (2012). Effects of green tea supplementation on elements, total antioxidants, lipids, and glucose values in the serum of obese patients. Biol. Trace Elem. Res. 149, 315–322. doi:10.1007/s12011-012-9448-z
Tatsis, E. C., Boeren, S., Exarchou, V., Troganis, A. N., Vervoort, J., and Gerothanassis, I. P. (2007). Identification of the major constituents of Hypericum perforatum by LC/SPE/NMR and/or LC/MS. Phytochemistry 68, 383–393. doi:10.1016/j.phytochem.2006.11.026
Tontonoz, P., and Spiegelman, B. M. (2008). Fat and beyond: The diverse biology of PPARgamma. Annu. Rev. Biochem. 77, 289–312. doi:10.1146/annurev.biochem.77.061307.091829
Trinder, P. (1969). Determination of glucose in blood using glucose oxidase with an alternative oxygen acceptor. Ann. Clin. Biochem. An Int. J. Biochem. laboratory Med. Ann. Clin. Biochem. An Int. J. Biochem. laboratory Med. 6, 24–27. doi:10.1177/000456326900600108
Wei, Y., Xie, Q., Fisher, D., and Sutherland, I. A. (2011). Separation of patuletin-3-O-glucoside, astragalin, quercetin, kaempferol and isorhamnetin from Flaveria bidentis (L) Kuntze by elution-pump-out high-performance counter-current chromatography. J. Chromatogr. A 1218, 6206–6211. doi:10.1016/j.chroma.2011.01.058
Yamauchi, T., Kamon, J., Waki, H., Terauchi, Y., Kubota, N., Hara, K., et al. (2001). The fat-derived hormone adiponectin reverses insulin resistance associated with both lipoatrophy and obesity. Nat. Med. 7, 941–946. doi:10.1038/90984
Keywords: Euphorbia, diabetes, insulin resistance, oxidative stress, inflammation
Citation: Alruhaimi RS, Mostafa-Hedeab G, Abduh MS, Bin-Ammar A, Hassanein EHM, Kamel EM and Mahmoud AM (2023) A flavonoid-rich fraction of Euphorbia peplus attenuates hyperglycemia, insulin resistance, and oxidative stress in a type 2 diabetes rat model. Front. Pharmacol. 14:1204641. doi: 10.3389/fphar.2023.1204641
Received: 12 April 2023; Accepted: 01 June 2023;
Published: 16 June 2023.
Edited by:
Chika Ifeanyi Chukwuma, Central University of Technology, South AfricaReviewed by:
Jude Akinyelu, Federal University Oye-Ekiti, NigeriaCopyright © 2023 Alruhaimi, Mostafa-Hedeab, Abduh, Bin-Ammar, Hassanein, Kamel and Mahmoud. This is an open-access article distributed under the terms of the Creative Commons Attribution License (CC BY). The use, distribution or reproduction in other forums is permitted, provided the original author(s) and the copyright owner(s) are credited and that the original publication in this journal is cited, in accordance with accepted academic practice. No use, distribution or reproduction is permitted which does not comply with these terms.
*Correspondence: Ayman M. Mahmoud, YXltYW4ubWFobW91ZEBzY2llbmNlLmJzdS5lZHUuZWc=, YS5tYWhtb3VkQG1tdS5hYy51aw==
Disclaimer: All claims expressed in this article are solely those of the authors and do not necessarily represent those of their affiliated organizations, or those of the publisher, the editors and the reviewers. Any product that may be evaluated in this article or claim that may be made by its manufacturer is not guaranteed or endorsed by the publisher.
Research integrity at Frontiers
Learn more about the work of our research integrity team to safeguard the quality of each article we publish.