- 1Department of Japanese Oriental Medicine Graduate School of Medicine and Pharmaceutical Sciences, University of Toyama, Toyama, Japan
- 2Aizu Medical Center, Department of Neuropsychiatry, Fukushima Medical University, Aizuwakamatsu, Japan
- 3Department of Research and Development, Division of Complex Biosystem Research (CBR), Institute of National Medicine (INM), University of Toyama, Toyama, Japan
- 4Department of Neurology, School of Medicine, Fukuoka University, Fukuoka, Japan
- 5Department of Geriatric Medicine, Kanazawa Medical University, Ishikawa, Japan
- 6Translational Neuroscience Center, Graduate School of Medicine, International University of Health and Welfare, Tochigi, Japan
- 7Department of Neuropsychiatry, Graduate School of Medical Sciences, Kyushu University, Fukuoka, Japan
- 8Department of Neurology, Graduate School of Medicine, Chiba University, Chiba, Japan
- 9Department of Psychiatry, Jichi Medical University, Tochigi, Japan
- 10Second Department of Internal Medicine, Division of Neurology, Faculty of Medical Sciences, University of Fukui, Fukui, Japan
- 11Department of Medicine, Division of Neurology, Showa University School of Medicine, Tokyo, Japan
- 12Department of Neurology, Wakayama Medical University, Wakayama, Japan
- 13Department of General Medicine, Oita University Faculty of Medicine, Oita, Japan
- 14Department of Internal Medicine, Wakayama Medical University Kihoku Hospital, Wakayama, Japan
- 15Department of Dementia Medicine, Kawasaki Medical School, Okayama, Japan
- 16Department of Neuropsychiatry, Graduate School of Medicine and Pharmaceutical Sciences, University of Toyama, Toyama, Japan
- 17Department of General Internal Medicine, Kyushu University Hospital, Fukuoka, Japan
- 18Department of Clinical Research Promotion, Kyushu University Hospital, Fukuoka, Japan
- 19Department of Rehabilitation, Fukui Health Science University, Fukui, Japan
- 20Department of Neurology, Kanazawa University Graduate School of Medical Sciences, Ishikawa, Japan
Background: Alzheimer’s disease (AD), the most prevalent form of dementia, is a debilitating, progressive neurodegeneration. Amino acids play a wide variety of physiological and pathophysiological roles in the nervous system, and their levels and disorders related to their synthesis have been related to cognitive impairment, the core feature of AD. Our previous multicenter trial showed that hachimijiogan (HJG), a traditional Japanese herbal medicine (Kampo), has an adjuvant effect for Acetylcholine estelase inhibitors (AChEIs) and that it delays the deterioration of the cognitive dysfunction of female patients with mild AD. However, there are aspects of the molecular mechanism(s) by which HJG improves cognitive dysfunction that remain unclear.
Objectives: To elucidate through metabolomic analysis the mechanism(s) of HJG for mild AD based on changes in plasma metabolites.
Methods: Sixty-seven patients with mild AD were randomly assigned to either an HJG group taking HJG extract 7.5 g/day in addition to AChEI or to a control group treated only with AChEI (HJG:33, Control:34). Blood samples were collected before, 3 months, and 6 months after the first drug administration. Comprehensive metabolomic analyses of plasma samples were done by optimized LC-MS/MS and GC-MS/MS methods. The web-based software MetaboAnalyst 5.0 was used for partial least square-discriminant analysis (PLS-DA) to visualize and compare the dynamics of changes in the concentrations of the identified metabolites.
Results: The VIP (Variable Importance in Projection) score of the PLS-DA analysis of female participants revealed a significantly higher increase in plasma metabolite levels after HJG administration for 6 months than was seen in the control group. In univariate analysis, the aspartic acid level of female participants showed a significantly higher increase from baseline after HJG administration for 6 months when compared with the control group.
Conclusion: Aspartic acid was a major contributor to the difference between the female HJG and control group participants of this study. Several metabolites were shown to be related to the mechanism of HJG effectiveness for mild AD.
Introduction
The cognitive and memory impairment of dementia patients has been shown to lead to negative changes in behavior and activities of daily life (Cipriani et al., 2020). Currently, more than 55 million people suffer from dementia worldwide, with an incidence of about 10 million each year. Alzheimer’s disease (AD), the most prevalent form of dementia, is a debilitating, progressive form of neurodegeneration that accounts for 60%–70% of this disease that creates an enormous burden on public health systems around the world (World health Organization, 2023).
Numerous therapies have been developed in an attempt to alleviate or cure symptoms (Jeon et al., 2019). Notably, aducanumab was recently approved for mild AD (Billy et al., 2021); however, there is currently no available treatment that is an effective cure. The discovery and introduction of new therapeutic agents and strategies for AD are urgently needed.
“Kidney[™] deficiency” is one of the key pathologies of Kampo medicine, defined as a pattern characterized by an insufficient amount of essential qi of kidney. Hachimijiogan (HJG) is a traditional Japanese (herbal) medicine (Kampo) composed of eight herbs that is prescribed for the treatment of “Kidney[™] deficiency” (Terasawa, 1993; Kainuma et al., 2022). It is effective for various symptoms common to members of the older population, such as numbness, nocturia, lower back pain and coldness in the legs, and is used for cases of impotence, nephritis, diabetes, sciatica, bladder catarrh, lumbago, prostatic hypertrophy, and hypertension (Nakae et al., 2018). In Kampo, cognitive impairment is considered to be a sign of “Kidney[™] deficiency”. We previously demonstrated the effectiveness of HJG for AD both in vivo and in vitro: improvement was seen in the spatial memory impairment of AD model rats and in cognitive function via cAMP response element binding protein (CREB) (Kubota et al., 2017; Moriyama et al., 2017). Although not conclusive, we reported in a clinical trial that HJG has an adjuvant effect for acetylcholinesterase inhibitors (AChEIs) and that it delays the deterioration of the cognitive dysfunction of mild AD patients (Kainuma et al., 2022). However, there are aspects of the molecular mechanism(s) by which HJG improves cognitive dysfunction that remain unclear.
Metabolomics is a system-biology technology that can be used to monitor the dynamic changes of endogenous small-molecule metabolites (Nicholson et al., 2002). Several blood metabolic biomarker studies of AD have reported that changes in phospholipids, polyamines, and amino acids have potential for use in diagnosis (Chouraki et al., 2017; Huo et al., 2020; Lin et al., 2019b; Olazarán et al., 2015; Orešič et al., 2011; Ozaki et al., 2022; Peña-Bautista et al., 2019; Shao et al., 2020; Trushina and Mielke, 2014; Wan et al., 2020; Wilkins and Trushina, 2018). Kampo medicines are multi-component, multi-targeted drugs that act on various targets in the body. To understand the complex actions of Kampo medicines, it is important to extensively study the biological reactions they induce and the relation of the components to these reactions. Because it has been shown to be a useful tool for elucidating complex pharmacological actions and evaluating the effects of Kampo medicines on the living body (Kitagawa et al., 2018; Yamashita et al., 2021; Kobayashi et al., 2022), comprehensive metabolomic analysis was used in this study to determine the mechanism(s) of the effectiveness of HJG for mild AD.
Methods
The data analyzed in this study was from our previous open-label, randomized, multicenter, control trials (Kainuma et al., 2020; Kainuma et al., 2022).
Ethics
The study was done in accordance with the principles of the Declarations of Helsinki and Tokyo and approved by the Kyushu University Hospital Clinical Research Review Board (CRB) (Fukuoka, Japan) (CRB approval number: KD 2019001). All participants gave written, informed consent.
Study design
This study started 2 August 2019 at three sites in Japan. Unfortunately, recruitment was delayed because of the COVID-19 pandemic, but we adjusted and were able to add 11 institutions by January of 2021. The study finished on 31 March 2022. In brief, our protocol was done with mild AD patients who met all the inclusion criteria:
1) Age: ≥50 to <85 years old, 2) Mild Alzheimer’s disease (MMSE ≥21), 3) Taking the same dose of Donepezil, Galantamine, or Rivastigmine for more than 3 months, 4) Not taking Memantine, and 5) Written informed consent. Patients were excluded who were taking Kampo Medicine other than HJG for more than 3 months or who had a change in drug dosage that could affect the progression of cognitive function during the 3 months. The other major exclusion criteria are as follows: 1) Kidney dysfunction (eGFR<30 mL/min/1.73 m2); 2) AST or ALT>100 IU/L; 3) Complication with gastric ulcer, bronchial asthma, or epilepsy; and 4) Judged by doctors not to be suited for study, such as having serious complications.
Participants were divided into an AChEI plus HJG group and an AChEI alone group to compare the effectiveness and safety of the addition of HJG. In addition to AChEI, the HJG group took 2.5 g of HJG extract 3 times/day (TSUMURA hachimijiogan Extract Granules for Ethical Use: TJ-7, HJG, Tokyo Japan), the usual adult daily dose. Randomization was done with the Randomization Module of Research Electronical Data Capture (RED Cap). A computer-generated list of random numbers was transferred to RED Cap for block randomization by age and sex, then the participants were randomly assigned at a 1:1 ratio. Participants, physicians and data evaluators were aware of the allocation group after randomization, but outcome evaluators were blinded.
The primary outcome was the change of the Cognitive Component of the Alzheimer’s Disease Assessment Scale—Japanese Version (ADAS-Jcog) from baseline to 6 months, with assessments done at baseline and after 3 and 6 months. Blood tests were done at the same time points for the analyses of the metabolome, with samples collected at least 4 hours after breakfast. Plasma was separated by centrifugation at 2000 f for 25 min at 4°C, immediately stored at −80°C, and kept frozen until use.
Metabolomics analysis
We analyzed the plasma metabolome using gas chromatography-tandem mass spectrometry (GC-MS/MS; GCMS-TQ8040, Shimadzu, Kyoto, Japan) and liquid chromatography-tandem mass spectrometry (LC-MS/MS; Nexera X2 system connected with LCMS-8050, Shimadzu). We extracted, measured, and analyzed both hydrophilic and hydrophobic metabolites, including lipid mediators and phospholipids, in accordance with previously reported methods (Kitagawa et al., 2018; Yamashita et al., 2021).
Statistical analysis
The search for the HJG biomarkers was done through analysis of covariance of the change in metabolites from baseline, with the treatment group and baseline values as covariates. Continuous variables are expressed as the mean value and standard deviation.
A two-tailed test at the 5% level of significance was done for the statistical analyses. Two-tailed interval estimation was done with a 95% confidence interval (CI) and corresponding p values (with significance defined as p < 0.05). A t-test was used for comparison of the metabolomics of the HJG and control groups at each visit, with p < 0.05 representing a significant difference. JMP (Ver. 16, SAS Institute Japan Ltd.) was used for these analyses. In addition, to maximize class discrimination necessary for the identification discovery of potential metabolic biomarkers of between the HJG and Control groups, a supervised model of potential least squares discriminant analysis (PLS-DA) was applied. Variable importance in the projection (VIP) values were used in the PLS-DA model to estimate the discriminatory power of each variable for separation of the groups. The features, and variables with VIP values > 1 were considered important. The validation of all PLS-DA models was based on the leave-one-out cross validation method via parameters Q2 and R2. The significance of these models was demonstrated by permutation test with 2000 iterations using separation distance and p-value < 0.05.
Results
From among the 77 enrollees, the data of 67 (33 HJG and 34 control) were available for analysis: 2 of the 69 patients analyzed for the primary endpoint were not available for analysis due to hemolysis (Figure 1). No difference was found in the background factors between the HJG and control groups (Table 1).
Differential metabolite analysis
We conducted a comprehensive analysis of plasma metabolites to search for HJG biomarkers. The results for the plasma metabolites of male participants at 3 months in the HJG group showed significant change in hippuric acid, DHA, EPA, and phosphatidylcholine (PC) (36_1) and PC (36_2). At 6 months, hippuric acid, phenylacetic acid, suberic acid, tartaric acid, 2-Aminooctanoic acid, and 3-phosphoglyceric acid also showed more significant change in the HJG group than in the control group.
For the female participants of the HJG group at 3 months, suberic acid, tartaric acid and 1,5-anhydro-glucitol showed significant change. They also had significantly greater change of aspartic acid, 1-hexadecanol, 2-hydroxyisovaleric acid, 3-hydroxybutyric acid, and 3-hydroxyisobutyric acid at 6 months.
In the comparison of the metabolomics data for which more significant change from baseline was seen in the HJG group than in the control group at each testing point (day0, 3 M, and 6 M), the 3-phosphoglyceric acid of male participants at 6 months was significantly higher in the HJG group (Table 2). For the female participants, aspartic acid was significantly higher in the HJG group than in the control group (Table 3). In the analysis of aspartic acid, the difference in the change from baseline to 6 months of the HJG and control groups was 0.0099 (95% CI, 0.0015 to 0.0184 p = 0.0223) (Figure 2).
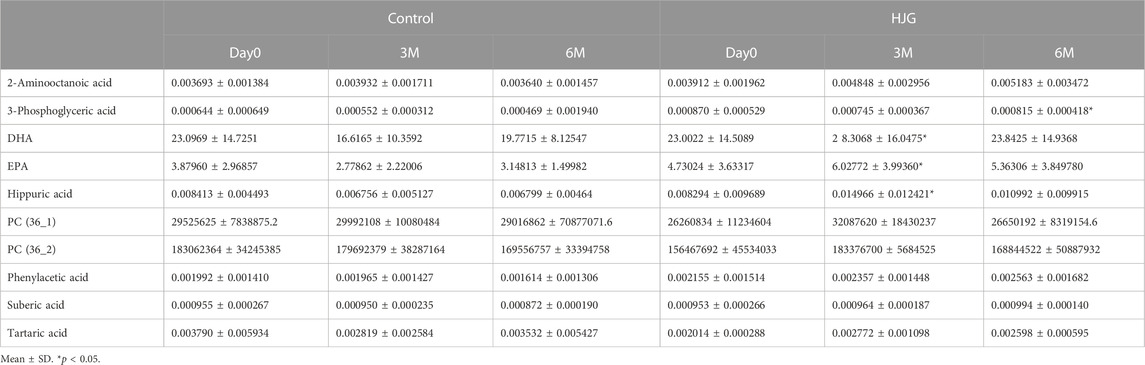
TABLE 2. Comparison of metabolites that had a significant difference in change from baseline (Male).
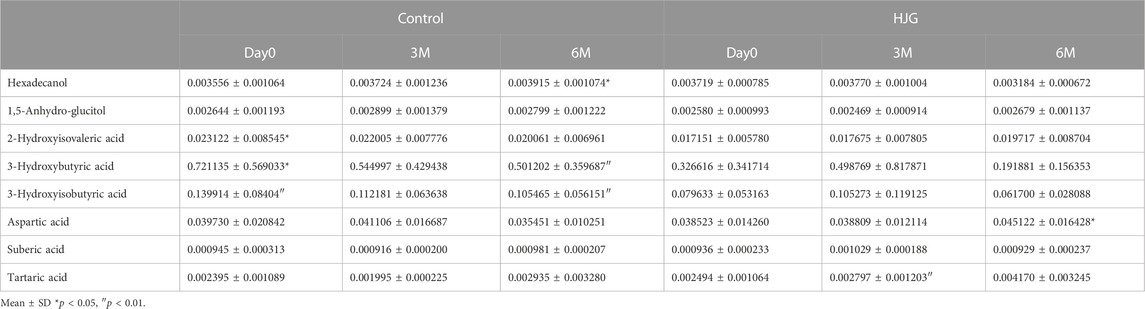
TABLE 3. Comparison of metabolites that had a significant difference in change from baseline (Female).
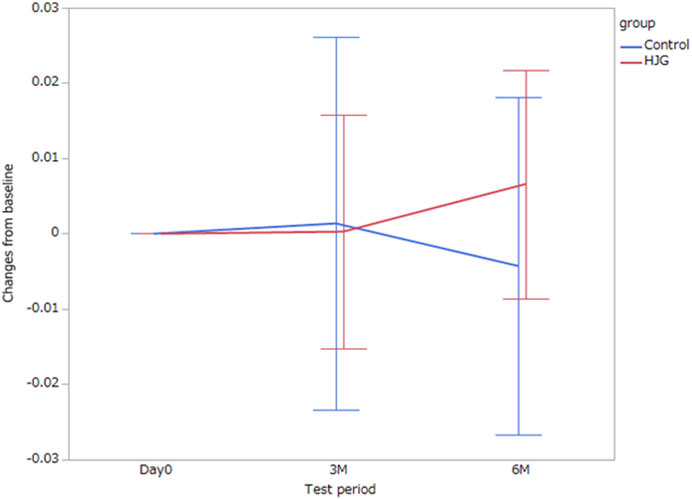
FIGURE 2. Difference between the HJG and control group female participants in the change of aspartic acid from baseline to 3 and 6 months.
Visualization of metabolites
A PLS-DA model with unit variance scaling was used to visualize the differences in the metabolites of the HJG and Control groups and to determine which metabolites can be used to distinguish between the two groups, As shown in Figures 3, 4, plots of the PLS-DA score values of components 1 and 2 demonstrated visible clustering and clear separation between the HJG and control groups. Moreover, the VIP (Variable Importance in Projection) score of the PLS-DA analysis of male participants revealed significantly greater increases in 17,18-DHETE, EPA, DHA, and hippuric acid levels after HJG administration for 3 months than were seen in the control group. 3-phosphoglyce, O-phosphoethan, suberic acid, 17,18-DHETE, phenylacetic acid, hypotaurine, and aspartic acid had a significantly greater increase at 6 months.
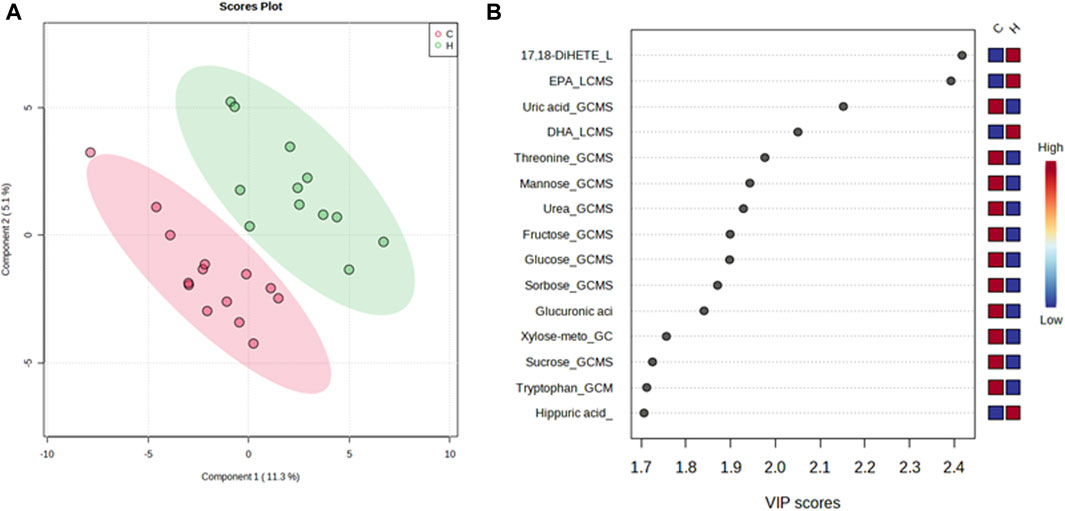
FIGURE 3. Partial least squares-discriminate analysis (PLS-DA) plot of the differential metabolites of the HJG and control group male participants and their importance in the projection (VIP) values at 3 months. (A) Score plot for PLS-DA of the HJG (H, green dots) (n = 12) and control groups (C, red dots) (n = 14). (B) The highest VIP values for the 1st component of PLS-DA. The blue to red color shift denotes low to high plasma metabolite concentrations.
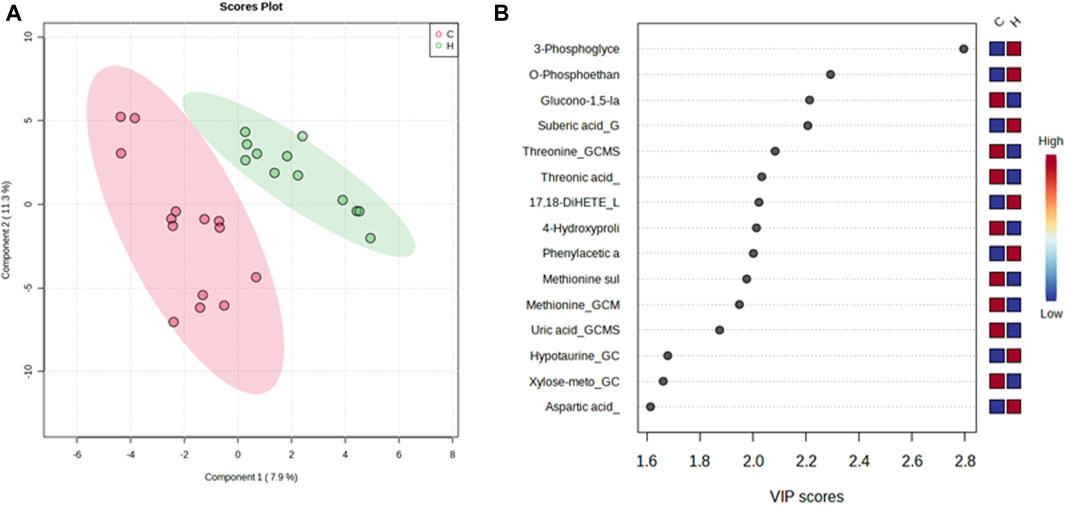
FIGURE 4. PLS-DA plot of differential metabolites and their corresponding VIP values for male participants at 6 months. (A) Score plot of the PLS-DA of the HJG (H, green dots) (n = 12) and control groups (C, red dots) (n = 14). (B) The highest VIP values for the 1st component of PLS-DA. The blue to red color shift denotes low to high plasma metabolite concentrations.
In contrast, the VIP score of the PLS-DA analysis of female participants revealed a significantly greater increase in tartaric acid, tryptophan, suberic acid, pyridoxal, cysteine, and hippuric acid after HJG administration for 3 months, compared with the control group, and that proline, tartaric acid, alanine, cystathionine, and aspartic acid had a significantly greater increase at 6 months (Figures 5, 6).
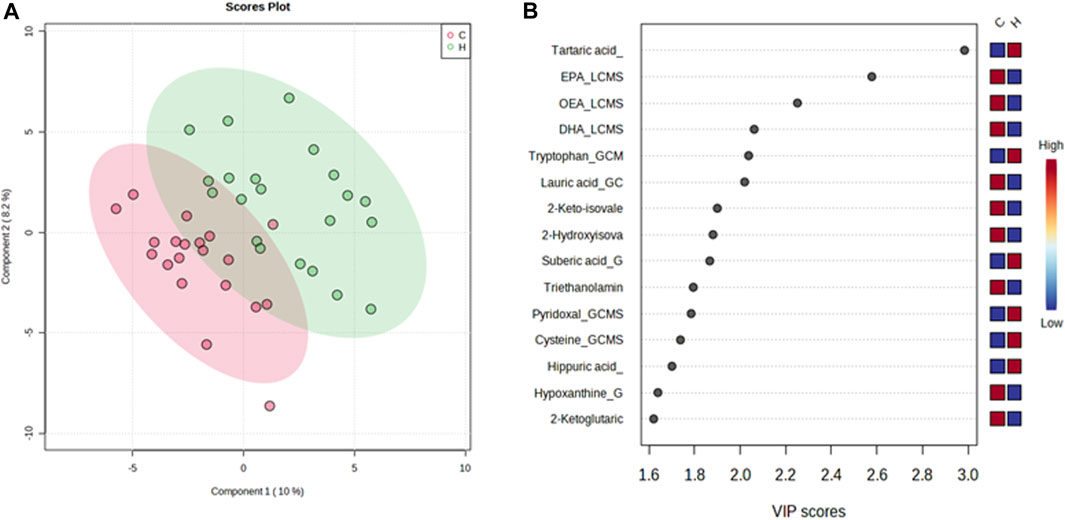
FIGURE 5. PLS-DA plot of differential metabolites and their corresponding VIP values for the female participants at 3 months. (A) Score plot of the PLS-DA of the HJG (H, green dots) (n = 21) and control groups (C, red dots) (n = 20). (B) The highest VIP values for the 1st component of PLS-DA. The blue to red color shift denotes low to high plasma metabolite concentrations.
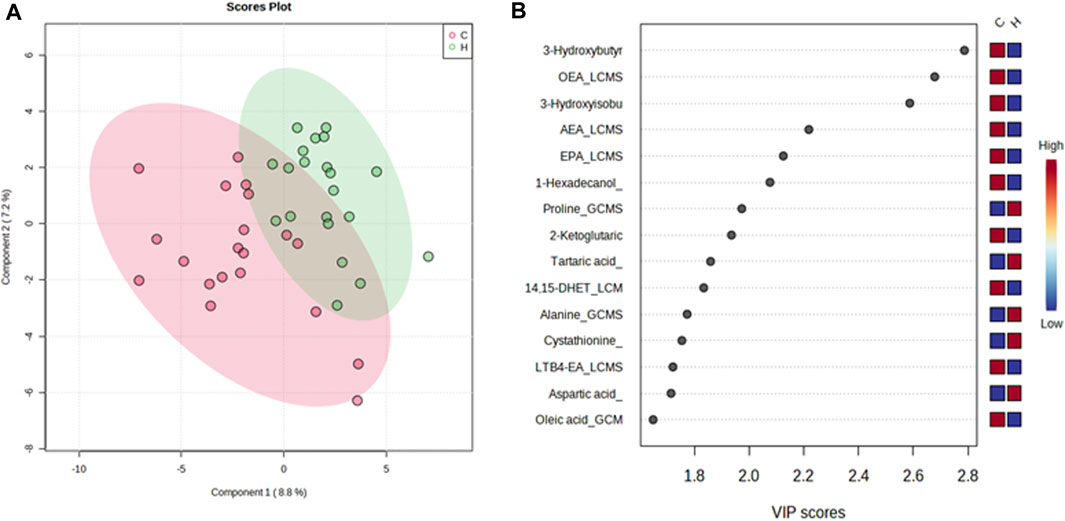
FIGURE 6. PLS-DA plot of differential metabolites and their corresponding VIP values for the female participants at 6 months. (A) Score plot of the PLS-DA of the HJG (H, green dots) (n = 21) and control groups (C, red dots) (n = 20). (B) The highest VIP values for the 1st component of PLS-DA. The blue to red color shift denotes low to high plasma metabolite concentrations.
Discussion
This is the first study to use metabolomic analysis to investigate the mechanism(s) of the action of HJG in a clinical study of dementia. The results of univariate and metabolomic analysis showed a significantly greater elevation of aspartic acid after 6 months of HJG in female subjects when compared to controls. These results indicate that an increase in aspartic acid is an important mechanism in the action of HJG on cognitive function. Because this part of the analysis used the same female patients as were used for the primary endpoint, the significantly greater improvement in cognitive function at 6 months of these female patients treated with HJG (the difference in change from baseline at 6 months for female participants was 2.90 (90% CI, 0.09 to 5.71, p = 0.090) was shown not to be due to a synergistic effect with AchEI: it was the effect of HJG alone (Kainuma et al., 2022).
Aspartic acid is involved in the development of nerves and their activity in the central nervous system. It has been reported that the concentration of aspartic acid in blood and cerebrospinal fluid was decreased in an AD group compared to a normal group (D'Aniello et al., 2005; Olazarán et al., 2015). In their AD group, aspartic acid was also decreased in the brain, and a decrease in aspartic acid was reported to be correlated with the severity of AD (Kwo-On-Yuen et al., 1994). Of the many reports that use metabolome analysis of early stage AD (Chouraki et al., 2017; Lin et al., 2019a; Orešič, et al., 2011; Shao et al., 2020; Ozaki et al., 2022; Peña-Bautista et al., 2019), some have concluded that aspartic acid is useful for diagnosis (Huo et al., 2020; Olazarán et al., 2015; Trushina and Mielke, 2014; Wilkins and Trushina, 2018). These findings indicate that aspartic acid plays an important role in the maintenance of cognitive function. The results of our study indicate that HJG causes an increase of aspartic acid in the blood and a subsequent increase in aspartic acid in the brain, thereby suppressing the progress of cognitive function.
We previously reported in an in vitro study that HJG improves cognitive function through the phosphorylation of CREB (Kubota et al., 2017). Aspartic acid acts as a neurotransmitter and increases cAMP as a second messenger after stimulating receptors (D'Aniello et al., 2011.). Because cAMP is upstream of CREB phosphorylation, an aspartic acid-induced cAMP increase may induce CREB phosphorylation. The results of our study indicate that the increase in aspartic acid by HJG is involved in the phosphorylation of CREB. On the other hand, because aspartic acid in PC12 cells has been reported to increase during neuronal differentiation of PC12 cells (Sano et al., 2018), the increase in aspartic acid may be a product of HJG-induced neurite outgrowth.
Metabolomic analysis of donepezil has been done to examine lipid metabolism, but no significant findings were obtained, and there are no reports of amino acid changes (Wan et al., 2020). There are also no reports on the use of metabolomics for responder analysis of dementia drugs other than donepezil. In this respect, responder analysis of HJG based on metabolome analysis, as used in this study is important, thus our results indicate that aspartic acid would be useful as a therapeutic drug for dementia.
The drugs used in Kampo medicine are combinations of two or more herbs, thus it is difficult to identify the causative agent in the same way as can be done for Western medicines. Although not detected in the univariate analysis in the present study, metabolomic analysis has shown that, in addition to aspartic acid, proline, tartaric acid, alanine, and cystathionine had significantly greater elevation after 6 months than was seen in our control group. Further, proline, alanine, and cystathionine has been correlated with cognitive decline associated with dementia (Xie et al., 2021; Lin et al., 2019b; Zhang et al., 2022). Therefore, it is possible that these substances may interact with each other to improve cognitive function.
The univariate metabolome analysis of the data of male participants showed significantly higher 3-phosphoglyceric acid in the HJG group. There are no reports of correlation between a change of 3-phosphoglyceric acid and cognitive impairment, but our data indicate that this may be related to the effect of HJG. On the other hand, although AD is more common in female patients and gender differences have been reported in the development of AD (Alzheimer’s Association, 2023; Altmann et al., 2014; Tao et al., 2018), in the metabolome analysis of this study a significantly greater increase in aspartic acid was found in our male participants than in the controls after 6 months of HJG. This indicates that the results for men might be similar to those of women if we had had more male patients. Our experience has shown that patients who have signs of “Kidney[TM] deficiency” other than cognitive impairment who take HJG long term have less AD than patients who take it for shorter periods of time. Further studies are needed to confirm this observation.
There are several limitations to the present study. The sample size available for analysis was small, especially that of men. Also, the peripheral blood-brain relation of amino acid remains unknown. Finally, only Japanese populations were recruited for this study.
Conclusion
Aspartic acid was the main contributor to the difference between female patients treated with HJG in addition to AChEI and a control group treated with only AChEI. A combination of several metabolites was shown to be related to the mechanism of the effectiveness of HJG for mild AD.
Data availability statement
The raw data supporting the conclusion of this article will be made available by the authors, without undue reservation.
Ethics statement
The studies involving human participants were reviewed and approved by the Kyushu University Hospital Clinical Research Review Board (CRB) (Fukuoka, Japan) (CRB approval number: KD 2019001). The patients/participants provided their written informed consent to participate in this study.
Author contributions
Conceptualization: MK, SO, K-IY, TO, AY, MM, and YT. Investigation: SO, SK, OI, K-IY, TO, ShH, SSu, TH, SoH, MY, AY, SSh, MH, K-IW, DS, KH, NS, HS, YK, YM, MS, HI, KO, and YT, project administration: MK, methodology: J-DK, KF, and MM, writing-original draft: MK, writing-review and editing: SK, J-DK, and YT. All authors contributed to the article and approved the submitted version.
Funding
This study was supported by a grant from the Japan Agency for Medical Research and Development (AMED) (grant number: JP19lk0310056).
Acknowledgments
Metabolomic analysis was outsourced to Tsumura & Co. (Tokyo, Japan). We also deeply thank Kiyoko Momota and Wakako Hiraoka for their efforts and support for our study.
Conflict of interest
The authors declare that the research was conducted in the absence of any commercial or financial relationships that could be construed as a potential conflict of interest.
Tsumura & Co. (Tokyo, Japan) provided TSUMURA hachimijiogan (TJ-7) Extract Granules for Ethical Use free of charge.
Publisher’s note
All claims expressed in this article are solely those of the authors and do not necessarily represent those of their affiliated organizations, or those of the publisher, the editors and the reviewers. Any product that may be evaluated in this article, or claim that may be made by its manufacturer, is not guaranteed or endorsed by the publisher.
Abbreviations
AChEI; acetylcholinesterase inhibitor, AD; Alzheimer’s disease, ADAS-Jcog; Alzheimer’s Disease Assessment Scale-cognitive component-Japanese version, CREB; cAMP response element binding prote, HJG; Hachimijiogan, RED Cap; Research electronical data capture.
References
Altmann, A., Tian, L., Henderson, V. W., and Greicius, M. D.Alzheimer's Disease Neuroimaging Initiative Investigators (2014). Sex modifies the APOE-related risk of developing Alzheimer disease. Ann. Neurol. 75, 563–573. doi:10.1002/ana.24135
Altzheimer‘s Association (2023). Altzheimer’s disease a facts and Figures. Available at: https://www.alz.org/media/Documents/alzheimers-facts-and-figures (Accessed March 21, 2023).
Billy, D. B., Stein, P., and Cavazzoni, P. (2021). Approval of aducanumab for alzheimer disease—the FDA’s perspective. JAMA Intern Med. 181, 1276–1278. doi:10.1001/jamainternmed.2021.4607
Chouraki, V., Preis, S. R., Yang, Q., Beiser, A., Li, S., Larson, M. G., et al. (2017). Association of amine biomarkers with incident dementia and Alzheimer's disease in the Framingham Study. Alzheimers Dement. 13, 1327–1336. doi:10.1016/j.jalz.2017.04.009
Cipriani, G., Danti, S., Picchi, L., Nuti, A., and Fiorino, M. D. (2020). Daily functioning and dementia. Dement. Neuropsychol. 14, 93–102. doi:10.1590/1980-57642020dn14-020001
D'Aniello, A., Fisher, G., Migliaccio, N., Cammisa, G., D'Aniello, E., and Patrizia Spinelli, P. (2005). Amino acids and transaminases activity in ventricular CSF and in brain of normal and Alzheimer patients. Neurosci. Lett. 388, 49–53. doi:10.1016/j.neulet.2005.06.030
D'Aniello, S., Somorjai, I., Jordi, G. F., Topo, E., and D'Aniello, A. (2011). D-Aspartic acid is a novel endogenous neurotransmitter. FASEB J. 25, 1014–1027. doi:10.1096/fj.10-168492
Honig, L. S., Vellas, B., Woodward, M., Boada, M., Bullock, R., Borrie, M., et al. (2018). Trial of solanezumab for mild dementia due to Alzheimer's disease. N. Engl. J. Med. 378, 321–330. doi:10.1056/NEJMoa1705971
Huo, Z., Yu, L., Yang, J., Zhu, Y., Bennett, D. A., and Zhao, J. (2020). Brain and blood metabolome for Alzheimer's dementia: Findings from a targeted metabolomics analysis. Neurobiol. Aging 86, 123–133. doi:10.1016/j.neurobiolaging.2019.10.014
Jeon, S. G., Song, E. J., Lee, D., Park, J., Nam, Y., Kim, J. I., et al. (2019). Traditional oriental medicines and Alzheimer's disease. Aging Dis. 10, 307–328. doi:10.14336/AD.2018.0328
Kainuma, M., Funakoshi, K., Ouma, S., Yamashita, K. I., Ohara, T., Yoshiiwa, A., et al. (2020). The efficacy and safety of hachimijiogan for mild alzheimer disease in an exploratory, open standard treatment controlled, randomized allocation, multicenter trial: A study protocol. Med. Baltim. 99 (38), e22370. doi:10.1097/MD.0000000000022370
Kainuma, M., Ouma, S., Kawakatsu, S., Iritani, O., Yamashita, K. I., Ohara, T., et al. (2022). An exploratory, open-label, randomized, multicenter trial of hachimijiogan for mild Alzheimer’s disease. Front. Pharmacol. 13, 991982. Published online 2022 Oct 14. doi:10.3389/fphar.2022.991982
Kitagawa, H., Ohbuchi, K., Munekage, M., Fujisawa, K., Kawanishi, Y., Namikawa, T., et al. (2018). Data on metabolic profiling of healthy human subjects' plasma before and after administration of the Japanese Kampo medicine maoto. Data Brief. 3, 359–364. doi:10.1016/j.dib.2018.11.116
Kobayashi, A., Nagashima, K., Hu, A., Harada, Y., and Kobayashi, H. (2022). Effectiveness and safety of kamikihito, a traditional Japanese medicine, in managing anxiety among female patients with intractable chronic constipation. Complement. Ther. Clin. Pract. 46, 101526. doi:10.1016/j.ctcp.2021.101526
Kubota, K., Fukue, H., Sato, H., Hashimoto, K., Fujikane, A., Moriyama, H., et al. (2017). The traditional Japanese herbal medicine hachimijiogan elicits neurite outgrowth effects in PC12 cells and improves cognitive in AD model rats via phosphorylation of CREB. Front. Pharmacol. 8, 850. doi:10.3389/fphar.2017.00850
Kwo-On-Yuen, P. F., Newmark, R. D., Budinger, T. F., Kaye, J. A., M J Ball, M. J., and W Jagust, W. J. (1994). Brain N-acetyl-L-aspartic acid in Alzheimer's disease: A proton magnetic resonance spectroscopy study. Brain Res. 667, 167–174. doi:10.1016/0006-8993(94)91494-x
Lin, C. H., Yang, H. T., and Lane, H. Y. (2019a). D-glutamate, D-serine, and D-alanine differ in their roles in cognitive decline in patients with Alzheimer's disease or mild cognitive impairment. Pharmacol. Biochem. Behav. 185, 172760. doi:10.1016/j.pbb.2019.172760
Lin, C. N., Huang, C. C., Huang, K. L., Lin, K. J., Yen, T. C., and Kuo, H. C. (2019b). A metabolomic approach to identifying biomarkers in blood of Alzheimer's disease. Ann. Clin. Transl. Neurol. 27, 537–545. doi:10.1002/acn3.726
Mintun, M. A., Lo, A. C., Evans, C. D., Wessels, A. M., Ardayfio, P. A., Andersen, S. W., et al. (2021). Donanemab in early Alzheimer's disease. N. Engl. J. Med. 384, 1691–1704. doi:10.1056/NEJMoa2100708
Moriyama, H., Mishima, T., Kainuma, M., Watanabe, T., Nagao, M., Takasaki, K., et al. (2017). Effect of hachimijiogan on memory impairment induced by beta-amyloid combined with cerebral ischemia in rats. Traditional Kampo Med. 4, 51–54. doi:10.1002/tkm2.1069
Nakae, H., Hiroshima, Y., and Hebiguchi, M. (2018). Kampo medicines for frailty in locomotor disease. Front. Nutr. 26 (5), 31. doi:10.3389/fnut.2018.00031
Nicholson, J. K., Connelly, J., Lindon, J. C., and Holmes, E. (2002). Metabonomics: A platform for studying drug toxicity and gene function. Nat. Rev. Drug. Discov. 1, 153–161. doi:10.1038/nrd728
Olazarán, J., Gil-de-Gómez, L., Rodríguez-Martín, A., Valentí-Soler, M., Frades-Payo, B., Marín-Muñoz, J., et al. (2015). A blood-based, 7-metabolite signature for the early diagnosis of Alzheimer's disease. J. Alzheimers Dis. 45, 1157–1173. doi:10.3233/JAD-142925
Orešič, M., Hyötyläinen, T., Herukka, S-K., Sysi-Aho, M., Mattila, I., Seppänan-Laakso, T., et al. (2011). Metabolome in progression to Alzheimer's disease. H.Transl Psychiatry 1 (12), e57. doi:10.1038/tp.2011.55
Ozaki, T., Yoshino, Y., Tachibana, A., Shimizu, H., Mori, T., Nakayama, T., et al. (2022). Metabolomic alterations in the blood plasma of older adults with mild cognitive impairment and Alzheimer's disease (from the Nakayama Study). Sci. Rep. 12, 15205. doi:10.1038/s41598-022-19670-y
Peña-Bautista, C., Roca, M., Hervás, D., Cuevas, A., López-Cuevas, R., Vento, M., et al. (2019). Plasma metabolomics in early Alzheimer's disease patients diagnosed with amyloid biomarker. J. Proteomics 30, 144–152. doi:10.1016/j.jprot.2019.04.008
Sano, A., Shi, H., Suzuki, R., Shirataki, Y., and Sakagami, H. (2018). Change in amino acid pools during neuronal differentiation of PC12 cells. Vivo 32, 1403–1408. doi:10.21873/invivo.11392
Shao, Y., Ouyang, Y., Li, T., Liu, X., Xu, X., Li, S., et al. (2020). Alteration of metabolic profile and potential biomarkers in the plasma of Alzheimer's disease. Aging Dis. 11, 1459–1470. doi:10.14336/AD.2020.0217
Tao, Y., Peters, M. E., Drye, L. T., Devanand, D. P., Mintzer, J. F., Pollock, B. G., et al. (2018). Sex differences in the neuropsychiatric symptoms of patients with Alzheimer's disease. Am. J. Alzheimers Dis. Other Demen 33, 450–457. doi:10.1177/1533317518783278
Trushina, E., and Mielke, M. M. (2014). Recent advances in the application of metabolomics to Alzheimer's Disease. Biochim. Biophys. Acta 1842, 1232–1239. doi:10.1016/j.bbadis.2013.06.014
Wan, L., Lu, J., Huang, J., Huo, Y., Jiang, S., and Guo, C. (2020). Association between peripheral adiponectin and lipids levels and the therapeutic response to donepezil treatment in han Chinese patients with Alzheimer's disease. Front. Aging Neurosci. 12, 532386. doi:10.3389/fnagi.2020.532386
Wilkins, J. M., and Trushina, E. (2018). Application of metabolomics in Alzheimer's disease. Front. Neurol. 12 (8), 719. doi:10.3389/fneur.2017.00719
World health Organization (2018). World health organization. Available at: https://www.who.int/news-room/fact-sheets/detail/dementia (Accessed March 19, 2023).
Xie, K., Qin, Q., Long, Z., Yang, Y., Peng, C., Xi, C., et al. (2021). High-throughput metabolomics for discovering potential biomarkers and identifying metabolic mechanisms in aging and Alzheimer's disease. Front. Cell Dev. Biol. 9, 602887. doi:10.3389/fcell.2021.602887
Yamashita, H., Ohbuchi, K., Nagino, M., Ebata, T., Tsuchiya, K., Kushida, H., et al. (2021). Comprehensive metabolome analysis for the pharmacological action of inchinkoto, a hepatoprotective herbal medicine. Metabolomics 17, 106. doi:10.1007/s11306-021-01824-0
Keywords: metabolomic analysis, Alzheimer’s disease, hachimijiogan, aspartic acid, kampo
Citation: Kainuma M, Kawakatsu S, Kim J-D, Ouma S, Iritani O, Yamashita K-I, Ohara T, Hirano S, Suda S, Hamano T, Hieda S, Yasui M, Yoshiiwa A, Shiota S, Hironishi M, Wada-Isoe K, Sasabayashi D, Yamasaki S, Murata M, Funakoshi K, Hayashi K, Shirafuji N, Sasaki H, Kajimoto Y, Mori Y, Suzuki M, Ito H, Ono K and Tsuboi Y (2023) Metabolic changes in the plasma of mild Alzheimer’s disease patients treated with Hachimijiogan. Front. Pharmacol. 14:1203349. doi: 10.3389/fphar.2023.1203349
Received: 10 April 2023; Accepted: 30 May 2023;
Published: 12 June 2023.
Edited by:
Woon-Man Kung, Chinese Culture University, TaiwanReviewed by:
Juexian Song, Capital Medical University, ChinaPriyanka Baloni, Purdue University, United States
Copyright © 2023 Kainuma, Kawakatsu, Kim, Ouma, Iritani, Yamashita, Ohara, Hirano, Suda, Hamano, Hieda, Yasui, Yoshiiwa, Shiota, Hironishi, Wada-Isoe, Sasabayashi, Yamasaki, Murata, Funakoshi, Hayashi, Shirafuji, Sasaki, Kajimoto, Mori, Suzuki, Ito, Ono and Tsuboi. This is an open-access article distributed under the terms of the Creative Commons Attribution License (CC BY). The use, distribution or reproduction in other forums is permitted, provided the original author(s) and the copyright owner(s) are credited and that the original publication in this journal is cited, in accordance with accepted academic practice. No use, distribution or reproduction is permitted which does not comply with these terms.
*Correspondence: Mosaburo Kainuma, a2FpbnVtYUBtZWQudS10b3lhbWEuYWMuanA=