- 1Department of Cardiology, Longhua Hospital, Shanghai University of Traditional Chinese Medicine, Shanghai, China
- 2Longhua Hospital, Shanghai University of Traditional Chinese Medicine, Shanghai, China
Cancer represents one of the leading causes of mortality worldwide. Conventional clinical treatments include radiation therapy, chemotherapy, immunotherapy, and targeted therapy. However, these treatments have inherent limitations, such as multidrug resistance and the induction of short- and long-term multiple organ damage, ultimately leading to a significant decrease in cancer survivors’ quality of life and life expectancy. Paeonol, a nature active compound derived from the root bark of the medicinal plant Paeonia suffruticosa, exhibits various pharmacological activities. Extensive research has demonstrated that paeonol exhibits substantial anticancer effects in various cancer, both in vitro and in vivo. Its underlying mechanisms involve the induction of apoptosis, the inhibition of cell proliferation, invasion and migration, angiogenesis, cell cycle arrest, autophagy, regulating tumor immunity and enhanced radiosensitivity, as well as the modulation of multiple signaling pathways, such as the PI3K/AKT and NF-κB signaling pathways. Additionally, paeonol can prevent adverse effects on the heart, liver, and kidneys induced by anticancer therapy. Despite numerous studies exploring paeonol’s therapeutic potential in cancer, no specific reviews have been conducted. Therefore, this review provides a systematic summary and analysis of paeonol’s anticancer effects, prevention of side effects, and the underlying mechanisms involved. This review aims to establish a theoretical basis for the adjunctive strategy of paeonol in cancer treatment, ultimately improving the survival rate and enhancing the quality of life for cancer patients.
1 Introduction
In recent times, the global incidence and mortality rates of cancer have exhibited a rapid upsurge, owing to several factors, including the progress of human society, economic development, and other multifarious factors. According to data, cancer is currently the second leading cause of death globally, following ischemic heart disease, and is projected to become the primary cause of death by 2060 (Bray et al., 2018; Mattiuzzi and Lippi, 2019). In particular, high-income countries experience high incidence rates of certain cancers, such as lung, breast, and prostate. At the same time, it also has a high incidence in middle and low-income countries due to the influence of many of the same risk factors, such as smoking and obesity (Torre et al., 2016). Currently, the primary treatment modalities for cancer include surgery, radiation therapy, and systemic therapy, such as chemotherapy, targeted therapy, hormone therapy, and immunotherapy (Miller et al., 2019). However, modern treatment strategies that improve the chances of surviving cancer come with a corresponding cost (Curigliano et al., 2016). Cancer patients may experience complications in multiple organs, such as the heart, liver, kidney, lung, and gastrointestinal tract, associated with cancer treatment, severely impacting their quality of life and reducing life expectancy (Kennedy and Salama, 2020; Yazbeck et al., 2022). Additionally, the rapid development of multidrug resistance to anticancer drugs by cancer cells presents a significant limitation to cancer treatment (Kolodny et al., 2018). Therefore, searching for effective antitumor drugs with fewer toxic side effects is a crucial area of focus in developing oncology drugs.
Over the past few years, there has been growing interest in natural products as potential alternatives for combating cancer progression (Zhang et al., 2022). Among them, paeonol, a phenolic compound derived from the root bark of the medicinal plant peony, has demonstrated promising pharmacological activities, including anti-inflammatory, analgesic, anti-cardiovascular disease, and neuroprotective effects (Zhang et al., 2019). In addition, an increasing number of studies have paid attention to the extensive anti-tumor activity of paeonol, which has a significant inhibitory effect on various types of cancer cells through multiple pathways and multiple targets (Chen et al., 2022b; Ding et al., 2022). Simultaneously, paeonol plays a certain protective role against multiorgan damage in addition to its anticancer activity (Ding et al., 2022). Thus, paeonol is considered a potentially safe and effective antitumor agent that could be used as an alternative or supplement to conventional cancer therapy (Wan et al., 2008; Wu et al., 2016a). This study comprehensively reviews the pharmacological mechanisms underlying paeonol’s antitumor effects and its potential for reducing multiorgan toxicity induced by cancer therapy. Consequently, this research contributes to a better understanding of the potential benefits of paeonol in tumor therapy and its synergistic reducing-toxicity effects to provide a reference for the further development and clinical application of paeonol in anticancer therapy.
2 Pharmacological properties of paeonol
2.1 Chemical properties of paeonol
Natural products possess unique, diverse, and complex structures, chemical properties, and a wide range of biological activities, making them increasingly attractive as potential drugs for treating various clinical diseases (Chen and Kirchmair, 2020). Paeonol is a natural active substance derived from the traditional Chinese medicine Cortex Moutan (CM). Modern analytical techniques have identified paeonol as the most abundant compound in CM and the key to its various pharmacological effects (Yang et al., 2020). Paeonol, chemically known as 2-hydroxy-4-methoxy acetophenone, and the chemical structures are presented in Figure 1. It has a low molecular weight of 166.17 g/mol and a melting point of 52°C (Latif et al., 2022). It has been found to exert antitumor effects through various molecular mechanisms (Fu et al., 2018; Cai et al., 2020; Zhang et al., 2020a). In addition, several derivatives of paeonol have been discovered and synthesized, which also exhibit high anticancer potential and provide structural templates for the design and development of new anticancer agents (Wang et al., 2012; Tsai et al., 2016).
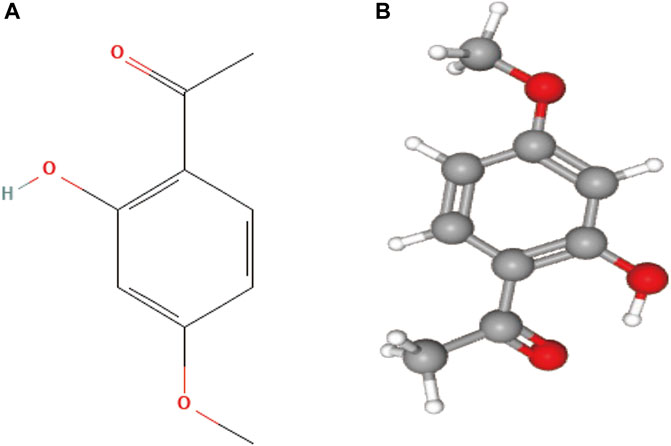
FIGURE 1. (A) 2D structure of paeonol, the molecular formula is C9H10O3, and PubChem CID is 11092; (B) 3D structure of paeonol.
2.2 Pharmacokinetics and new drug delivery systems of paeonol
The pharmacokinetics of paeonol in rats were found to be unsatisfactory after various routes of administration, exhibiting short peak concentration (Tmax) and elimination half-life (t1/2), as well as low oral bioavailability (only 16%) (Wu et al., 2003; Ma et al., 2008; Xie et al., 2008). The lower bioavailability of gavage administration compared to intravenous administration of paeonol may be attributed to incomplete absorption or a more pronounced first-pass effect (Ma et al., 2009). The rapid metabolism of paeonol in the heart, liver, kidney, and other tissues in the body seems to guarantee its safety because of the absence of long-term accumulation, but it also restricts its clinical utility (Hu et al., 2020). Moreover, paeonol exhibits drawbacks, such as low aqueous solubility, poor stability, and high volatility at room temperature. Therefore, extensive efforts have been made to develop various paeonol formulations and novel drug delivery systems, such as tablets, hydrogels, microparticles, microsponges, nanocapsules, polymer nanoparticles, nanospheres, microemulsions, and liposomes. These formulations not only enhance the solubility, chemical stability, and bioavailability of paeonol but also improve its pharmacological activity. Several studies have reported significant improvements in the efficacy and therapeutic potential of paeonol-based formulations (Ajazuddin and Saraf, 2010; Adki and Kulkarni, 2020).
A study reported the preparation of methoxy PEG-PCL (mPEG-PCL) nanoparticles as drug carriers to load paeonol (Pae-NPs), which showed more potent antitumor effects than free paeonol in both in vitro and in vivo experiments (Chen et al., 2017). Another study explored PEGylated liposome-mediated drug delivery systems for paeonol (Pae-PEG-NISVs) that effectively stabilize the delivery of the drug to cancer cells. This system has the potential to serve as an efficient carrier for paeonol, and a significant synergistic effect was observed with low concentrations of Pae-PEG-NISVs and 5-fluorouracil (5-Fu) (He et al., 2017). These results suggest that the novel drug delivery system of paeonol not only addresses the pharmacological limitations of paeonol but also enhances its antitumor efficacy, providing potential for the widespread application of paeonol.
3 Paeonol anticancer effects
3.1 Inhibition of cancer cell growth and proliferation
Dysregulation of cell proliferation is considered a significant contributing factor to various diseases. In particular, uncontrolled cell proliferation is a hallmark of cancer, and targeting this process is essential to controlling tumor progression (Loftus et al., 2022). There is considerable evidence to suggest that paeonol exhibits inhibitory effects on cancer cell growth and proliferation in a variety of cell lines, including cervix cancer HeLa cells (Du et al., 2022), human bladder cancer T24 and 5,637 cells (Zhang et al., 2021a), non-small-cell lung cancer A549 cells, hepatocellular carcinoma Hep3B, Huh-7, BEL-7404, SMMC-7721, MHCC97-H cells (Chunhu et al., 2008; Cai et al., 2020; Liu and Zhang, 2020), colorectal cancer HT-29 cells (Ye et al., 2009), pancreatic cancer Panc-1, Capan-1 cells (Cheng et al., 2020), osteosarcoma Saos-2, MG-63 cells (Zhou et al., 2020), ovarian cancer A2780, SKOV3, OVCAR-3 cells (Zhou et al., 2017; Gao et al., 2019), gastric cancer SGC-7901, BGC823, HGC-27 cells (Lyu et al., 2017; Fu et al., 2018), breast cancer MDA-MB-231 cells (Saahene et al., 2018), prostate cancer DU145, PC-3 cells (Xu et al., 2017), fibrosarcoma HT-1080 cells, esophageal cancer SEG-1, Eca-109 cells (Sun et al., 2008), glioma U87MG cells, U251 cells (Hao et al., 2023). These data indicate that paeonol has broad-spectrum antitumor properties, inhibiting tumor cell proliferation in a dose/time-dependent manner in various tumor cell lines. It was also found that paeonol exhibited more potent proliferation inhibition with 5-FU in a certain concentration range, while at higher concentrations, they did not show more significant synergistic effects (Chunhu et al., 2008). This interesting phenomenon shows that paeonol may exhibit synergistic or antagonistic effects with other chemotherapeutics at different concentrations, which may be related to the activation of the intrinsic defense mechanism of cells under the action of high concentrations of compounds to regulate and reduce the cytotoxicity of compounds. However, its mechanism needs to be further elucidated. In conclusion, paeonol has inhibitory effects on the growth of a variety of cancer cells in vitro and in vivo, and its effects are associated with the activation of cell apoptotic pathways and the regulation of the cell cycle.
3.2 Induction of apoptosis of cancer cells
Apoptosis is widely recognized as a form of programmed cell death critical in maintaining the normal growth and development of eukaryotes and homeostasis. Additionally, eliminating harmful or unnecessary cells in organisms is an essential mechanism for regulating cell death (Kaczanowski, 2016; Qian et al., 2022). Therefore, the disruption of apoptosis can lead to an imbalance of cell division and cell death, resulting in various diseases. In cancer, malignant cells can promote tumor growth and development by decreasing apoptosis or reducing resistance to apoptosis through multiple mechanisms (Wong, 2011). In several studies related to paeonol’s inhibition of tumors, significant apoptotic changes in tumor cells were clearly observed, which are typically characterized by cell shrinkage, chromatin accumulation and condensation, irregular nucleic shapes, and fragmentation of cells into membrane-wrapped apoptotic bodies, suggesting that the antitumor activity of paeonol is related to the mechanism of inducing apoptosis (Sun et al., 2008; Yin et al., 2013; Ou et al., 2014). There are two classical pathways of apoptosis, including the intrinsic (also known as the mitochondrial pathway) and extrinsic pathways (death receptor pathway), triggered by cellular stress, DNA damage, and immune surveillance mechanisms (Carneiro and El-Deiry, 2020). In the intrinsic apoptotic pathway, proapoptotic proteins (BAX and BAK) of the Bcl-2 family members promote cytochrome c release through mitochondrial membrane permeabilization and form apoptosomes with cysteine 9, which then activates the executioner cysteine 3 to promote apoptosis. In the extrinsic pathway, the death ligand binds to the TNF receptor superfamily to form a death-inducing signaling complex (DISC) composed of Fas-associated death domain (FADD) protein and proteinase 8, 10, thereby activating downstream effector caspases (caspase-3, 6, and 7), they are responsible for the execution phase of apoptosis (Su et al., 2015; Carneiro and El-Deiry, 2020). Previous studies have shown that paeonol significantly reduces the expression of Bcl-2, increases the expression of Bax, and reduces the ratio of Bcl-2/Bax, accompanied by the activation of caspase 3, 8 and 9 to promote apoptosis (Ou et al., 2014). When paeonol was combined with cisplatin, it showed a more substantial apoptosis-inducing effect (Wan et al., 2008). (Du et al., 2022; Li et al., 2014) reported that paeonol induces mitochondrial dysfunction, including the inducing of mitochondrial membrane potential (MMP), reactive oxygen species (ROS) production, and the release of cytochrome c. These results further suggest that paeonol mediates apoptosis through intrinsic and extrinsic pathways. In addition to the well-known apoptotic pathway, it is increasingly recognized that in different tumors concomitant disruption of endoplasmic reticulum (ER) homeostasis causes persistent ER stress (Chen et al., 2022b). Qin Niu demonstrated that paeonol promoted the expression of apoptotic proteins GRP78, CHOP, Caspase-12, and Caspase-3. It also promotes the release of Ca2+ from the endoplasmic reticulum into the cytoplasm, causing mitochondrial dysfunction and subsequently inducing apoptosis (Niu et al., 2020). However, it has been found that tumor cells can adapt to endoplasmic reticulum stress and thus evade apoptosis (Zhang et al., 2015b). In Lulu Fan’s study, it was found that increased expression of cyclooxygenase-2 (COX-2) may be critical for apoptosis resistance to endoplasmic reticulum stress, while paeonol downregulated COX-2 in hepatocellular carcinoma cells and reversed this effect (Fan et al., 2018).
Further research found that paeonol-mediated apoptosis involves multiple targets and pathways. Chen Yajing et al., based on a network pharmacology study, found that the expected targets in the biological process of paeonol and renal cell carcinoma (RCC) are mainly enriched in the positive regulation of cell death and apoptosis. The key signaling pathways mainly include the TNF signaling pathway, PI3K-AKT signaling pathway, and MAPK signaling pathway (Chen et al., 2022a). Activation of the PI3K-AKT signaling pathway and its downstream effector mTOR, leads to a cascade of cellular responses that promote cancer development. As a tumor suppressor, PTEN was found to play a crucial role in the negative regulation of the PI3K/AKT pathway (El-Tanani et al., 2023). The PTEN/PI3K/AKT/mTOR pathway is considered to be the key to the paeonol-induced apoptosis mechanism. Studies have found that paeonol can inhibit the phosphorylation expression of the PI3K/AKT pathway (Zhang et al., 2021; Du et al., 2022) and can inhibit the abnormal activation of this pathway by upregulating the level of PTEN (Zhang et al., 2006). MicroRNAs (miRNAs) are essential regulators of gene expression, and therefore miRNAs influence cancer progression by regulating the expression of oncogenes and oncogenes (Lai et al., 2019). A study found that miR-106a-5p is a critical upstream target for the regulation of PTEN and that paeonol can inhibit the expression of miR-106a-5p and negatively regulate PTEN expression, exerting proapoptotic effect (Zhang et al., 2021c). Nuclear factor κB (NF-κB) is also considered an essential target against cancer, and its activation promotes cancer cell proliferation and apoptosis inhibition (Chauhan et al., 2022). Similarly, paeonol has been shown to mediate apoptosis by inhibiting the NF-κB signaling pathway (Li et al., 2019). Jun Fu found that ERBB2 can activate the PI3K/AKT pathway, activating the downstream NF-κB, which was reversed by paeonol (Fu et al., 2018). The Wnt/β-catenin signal transduction pathway has been identified as a significant regulator of apoptosis. Paeonol can significantly reduce β-catenin and c-Myc protein expression, inhibiting the Wnt/β-catenin signaling pathway to play a proapoptotic role (Li et al., 2017). The chemokine CXCR3-B has also been found to induce proliferation inhibition and apoptosis. Studies have demonstrated that paeonol can modulate the expression of BACH1 and Nrf2 by mediating the CXCL4/CXCR3-B signaling pathway, resulting in the down-regulation of HO-1 and the promotion of cell apoptosis (Saahene et al., 2018). Nrf2 and HO-1 are recognized as essential antioxidants, and thus, paeonol may provide a novel idea for antitumor by modulating oxidative stress by inhibiting Nrf2/HO-1 expression (Mao et al., 2020). The above research shows that paeonol can play a role in promoting apoptosis through multiple pathways. Among these pathways, the intrinsic pathway appears to be particularly important for paeonol-induced apoptosis.
3.3 Inhibition of cancer cell invasion and metastasis
Secondary tumors, rather than primary tumors, are responsible for 90% of cancer deaths. This is because malignant cells have metastatic properties (Lambert et al., 2017). Primary malignant tumor cells can invade adjacent tissues, enter the vascular system, and spread throughout the body, causing severe secondary lesions (Novikov et al., 2021). This intricate process, known as the invasion-metastasis cascade, comprises five fundamental stages: invasion, intravasation, circulation, extravasation, and colonization (Fares et al., 2020; Babaei et al., 2021). The epithelial-mesenchymal transition (EMT) is widely recognized as the vital process of metastasis, which promotes the acquisition of malignant traits by cancer cells, while EMT is closely affected by extracellular matrix (EMC) hardness (Tian et al., 2022). Cell scratch-wound healing and transwell invasion assays provided the initial evidence that paeonol markedly suppressed cancer cell migration and invasion, which was associated with the inhibition of MMP2 and MMP9 of the matrix metalloproteinase (MMP) family because MMP can decompose EMC (Lyu et al., 2017). Moreover, the study revealed that paeonol effectively inhibited 5-lipoxygenase (5-LO), which stimulates cell invasion and migration by upregulating MMP2 and MMP9 expression (Sheng et al., 2021). MiRNAs participate in the regulation of various biological processes, including cell proliferation, differentiation, and apoptosis. Recently, miRNAs have also been discovered to directly target the core factors involved in epithelial-mesenchymal transition (EMT), contributing to cancer progression and metastasis (Chan and Wang, 2015). The study found that paeonol inhibited the EMT of cancer cells (E-cadherin expression was upregulated, while N-cadherin expression was downregulated), which was related to the regulation of miR-126–5p by paeonol and dependent on miR-126–5p to regulate its target genes ZEB2, a potential inducer of EMT, acts to suppress cell metastasis (Lv et al., 2022). Another study confirmed that paeonol upregulates miR-141 via the PKCδ and c-Src pathways to inhibit migration and invasion of cancer cells (Horng et al., 2014). Furthermore, paeonol’s inhibition of cell migration is inseparable from inhibiting the PI3K/AKT signaling pathway, and this effect can be reversed by upregulating miR-21 and downregulating miR-424–3p (Sun et al., 2020; Yang et al., 2022). Inflammatory cytokines and active substances in the tumor inflammatory microenvironment can activate various pathways that induce EMT and promote cancer cell migration (Tan et al., 2021). Several studies have shown that paeonol can reduce inflammation in the tumor microenvironment and inhibit cell migration by suppressing the STAT3/NF-κB and TGF-β1/Smad signaling pathways (Zhang et al., 2015a; Cheng et al., 2020; Zhang et al., 2020a). In summary, paeonol inhibits cell invasion and migration primarily by suppressing EMT, which involves the co-regulation of specific miRNAs and inflammatory signaling pathways.
3.4 Induce cancer cell cycle arrest
The progression of cancer is the result of cell division, and the cell cycle is a critical mechanism that regulates this process. By targeting cell cycle progression, it may be possible to inhibit cell proliferation and tumor growth (Matthews et al., 2022). The cell cycle is composed of four phases: Gap 1 (G1), DNA synthesis (S), Gap 2 (G2), and mitosis (M), and the progression of each phase is regulated by the activity of cyclins, cyclin-dependent kinases (CDK) (Goel et al., 2018; Suski et al., 2021). Studies have found that paeonol can arrest the cell cycle in the G0/G1 phase, affecting the DNA replication and synthesis of tumor cells and inhibiting cell proliferation (Li et al., 2013). Aberrant activation of the WNT/β-catenin signaling pathway has been reported to induce transcription of LEF/TCF-responsive genes (such as c-Myc, cyclin D1) involved in the cell cycle. It was further found that paeonol downregulates cyclin D1 and CDK4 and upregulates p21Cip1 to arrest the cell cycle in G0/G1 phase by inhibiting the activation of WNT/β-catenin signaling (Liu et al., 2020). In addition, paeonol has been found to stimulate the movement of gastric cancer and esophageal cancer cells from the G0/G1 phase to the S phase, increasing the proportion of cells in the S phase and fractions decreasing in the G0/G1 and G2/M phases, this effectively prevents the cells from progressing into the M phase (Sun et al., 2008a; Li et al., 2010). The G2/M phase is an essential phase of cell mitosis. In human melanoma cells, paeonol-induced cell arrest in the G2/M phase is dose-dependent (Tao et al., 2014). These results indicate that paeonol can arrest cancer cells in different cell cycles and thus inhibit cell proliferation. However, the relevant mechanism of paeonol’s cell specificity to the cell cycle remains unclear and needs further study.
3.5 Inhibition of angiogenesis
The process of developing from existing blood vessels to new capillaries is called angiogenesis, which is beneficial for wound healing and embryo development. However, when angiogenesis is abnormally accelerated, it provides essential oxygen and nutrients for tumor growth and metastasis, and this process is activated during tumor progression (Nishida et al., 2006; Rajabi and Mousa, 2017). This complex process is jointly regulated by its activator and inhibitor, and vascular endothelial growth factor (VEGF) is considered a crucial angiogenesis activator. Much experimental evidence indicates that VEGF is highly expressed in growing tumor tissue, promoting its growth, invasion, and metastasis compared to healthy tissue (Yang and Cao, 2022). Targeting VEGF may be a feasible approach to block tumor progression. Tang et al. (2014) discovered that paeonol inhibits VEGF expression in human glioblastoma cells in a time-dependent manner. Basic fibroblast growth factor (bFGF) also acts as an activator of angiogenesis and promotes angiogenesis by directly or indirectly acting to upregulate VEGF in endothelial cells (Przybylski, 2009). It was found that paeonol could inhibit the migration and angiogenesis of human umbilical vein endothelial cells (HUVECs) treated with bFGF. This effect was further found to be associated with the downregulation of Akt phosphorylation in bFGF-stimulated HUVECs by paeonol and a decrease in matrix metalloproteinase-2 and -9 expression in HT1080 human fibrosarcoma cells (Kim et al., 2009). These findings suggest that paeonol can target tumor angiogenesis by regulating PI3K/AKT signaling pathway and MMPs activity, thereby inhibiting cancer cell invasion and migration. The research team found that paeonol oxime (PO), a derivative of paeonol, also inhibited the angiogenesis of fibrosarcoma cells. Interestingly, PO was more lethal to tumor cells than normal cells, indicating that paeonol and its derivatives can target tumor cells to exert antitumor effects (Lee et al., 2010). However, the clinical application of angiogenesis inhibition is currently controversial, and few studies investigate the effects of paeonol on tumor angiogenesis. Therefore, further research is needed to clarify the complex mechanisms involved in tumor therapy and the angiogenesis of angiogenesis in tumor therapy.
3.6 Induction of autophagy of cancer cells
As we all know, autophagy is a conservative process of capturing and degrading damaged proteins and organelles. Autophagy delivers cytoplasmic components to lysosomes for degradation to maintain cell metabolism and cell homeostasis (Yun and Lee, 2018). It has been demonstrated that autophagy inhibits tumor growth by preventing tissue damage and inducing cell death through self-degradation pathways, particularly in the early stages of cancer (Li et al., 2020). In contrast, in advanced stages of cancer, accompanied by tremendous intrinsic tumor cell stress, autophagy is activated by cancer cells to maintain survival and regeneration (Onorati et al., 2018). Therefore, autophagy plays a dynamic role in tumors under the influence of multiple factors. In studies on the regulation of autophagy by paeonol, it exerted anticancer effects by upregulating autophagy-related genes Atg5, LC3-II, and Beclin1 proteins in colorectal cancer LoVo cells and inducing autophagy (Cheng et al., 2019). In another study, the antiproliferative and apoptosis-promoting effects of paeonol in ovarian cancer may be related to paeonol-induced cytoprotective autophagy, as evidenced by a significant increase in the expression of the autophagy marker LC3-II and a decrease in the expression of p62, accompanied by the accumulation of autophagosomes and autolysosomes, while inhibition of the Akt/mTOR pathway is paeonol-induced autophagy in ovarian cancer cells and induction of apoptosis key. The study further found that combining paeonol and autophagy inhibitors enhanced the antitumor activities in a xenograft nude mouse model (Gao et al., 2019). The regulation mechanism of autophagy activity suggests that combining autophagy inducers and autophagy inhibitors could be an effective strategy against cancer. Therefore, paeonol may be a promising candidate for inducing autophagy when combined with autophagy inhibitors for cancer treatment.
3.7 Modulation of tumor immunity
We know that conventional radiotherapy treatment regimens kill tumor cells while potentially destroying adjacent normal cells, affecting patient prognosis. Cancer immunotherapy harnesses the cytotoxic potential of the human immune system as an important strategy for treating malignant tumors (Zhang and Zhang, 2020). T cells are activated by binding T cell receptors (TCR) to major histocompatibility complex (MHC) molecules or short tumor antigen peptides presented by human leukocyte antigens, thus exerting a powerful tumor-killing capacity. Programmed cell death protein 1 (PD1) is an immune checkpoint receptor that inhibits T cell activation through its ligand 1 (PD-L1) and ligand 2 (PD-L2) interactions, leading to tumor cell escape from T cell-mediated induction (Sun et al., 2018). In the study of Xianjie Chen et al., the effect of paeonol on tumor immunomodulation was observed for the first time. It was found that tumor progression was accompanied by accelerated thymic atrophy and apoptosis of thymocytes (differentiated CD4+ and CD8+ T cells) and that paeonol increased the mRNA levels of granzyme B in thymocytes and inhibited melanoma growth. Paeonol targets miR-139e5p to downregulate PD1 expression in the thymus to abolish immune dysfunction in melanoma (Chen et al., 2023). This result suggests that paeonol has some immunomodulatory effects in combating tumor growth. However, the mechanisms involved in tumor immunity are relatively complex, and more research is still needed to explore whether paeonol also has immunomodulatory effects on other tumors and its underlying mechanism.
3.8 Reversing cancer chemotherapy resistance and enhancing radiosensitivity
Currently, there are various cancer treatment means, and chemotherapy as the first choice for cancer therapy has remarkable efficacy. However, cancer patients still have poor prognoses and high recurrence and metastasis rates. One of the main reasons is that tumor cells can produce phenotype resistance to drugs with different structures and functions (Gatti and Zunino, 2005). Cancer cells can act individually or in combination through various mechanisms such as DNA repair, enhanced drug inactivation and efflux, cell death inhibition, EMT, and tumor microenvironment (TM), leading to chemoresistance (Senthebane et al., 2017; Hasan et al., 2018). Almost all chemotherapy drugs used to treat cancer will produce drug resistance. In vitro and in vivo experiments have demonstrated that paeonol can reverse the resistance to chemotherapy drugs like paclitaxel, doxorubicin (DOX), apatinib, and cisplatin. Studies have found that paeonol reverses drug resistance by regulating drug efflux, specifically by targeting adenosine triphosphate binding cassette (ABC) transporter proteins and p-glycoprotein (P-gp), which may be associated with inhibition of SET-mediated PI3K/AKT signal pathway activity (Zhang et al., 2015b; Sun et al., 2016). Additionally, paeonol can also induce PTEN expression, further contributing to the reversal of drug resistance (Han et al., 2018). In addition, the downregulation of transgelin 2 expression by paeonol may be an additional target for reversing paclitaxel resistance (Cai et al., 2014). It is gradually recognized that the tumor microenvironment can promote chemoresistance by mediating endoplasmic reticulum stress (ERS). The research reveals that paeonol can rectify ER-induced drug resistance by targeting COX-2-mediated PI3K/AKT/CHOP pathway inactivation (Fan et al., 2013). In addition to being resistant to chemotherapy drugs, tumor cells often resist radiation, leading to tumor recurrence. Research has found that paeonol can promote cell apoptosis and enhance the sensitivity of cancer cells to radiation by inhibiting the expression of the PI3K/Akt pathway and its downstream proteins COX-2 and Survivin (Lei et al., 2013; Wu et al., 2014; Ren and Zhang, 2017). In response to the radiotherapy resistance induced by tumor hypoxia, paeonol plays a role by inhibiting hypoxia-inducible factor-1 (HIF-1) and its downstream target gene VEGF (Zhou et al., 2017). Thus, paeonol reverses chemoresistance and enhances radiosensitivity by promoting apoptosis, mediating ERS, inhibiting angiogenesis, and regulating drug efflux, which is closely related to the regulation of PI3K/AKT. Based on these findings, we concluded that paeonol could reverse chemoresistance and act as a safe radiosensitizer. In clinical application, the combination of paeonol with radiotherapy and chemotherapy may further enhance the anticancer efficacy.
4 Organ protective effect of paeonol on anticancer treatment-induced toxicity
4.1 Protective effects of paeonol on cardiotoxicity
Cancer treatments have undergone significant advancements in recent years, leading to improved patient survival rates. However, these treatments have been accompanied by adverse effects. In addition to conventional chemotherapy and radiotherapy, targeted therapy and immunotherapy may also result in short-term or long-term cardiac damage, such as cardiomyopathy, heart failure, arrhythmia, and hypertension, which have emerged as the second most common cause of morbidity and mortality among cancer survivors, after recurrent malignant tumors (Curigliano et al., 2016). DOX is an established chemotherapeutic drug for treating several types of cancers. However, its use is limited by its potential to induce cardiotoxicity in a dose-dependent and cumulative manner, which is linked to increased oxidative stress and lipid peroxidation, disruption of iron regulatory protein, perturbation of Ca2+ homeostasis, alterations in autophagy and apoptosis pathways, and the release of nitric oxide and inflammatory mediators (Shabalala et al., 2017; Rawat et al., 2021). A study was conducted to establish a model of DOX-induced cardiotoxicity using H9c2 cells in vitro and the adult zebrafish heart in vivo. The results showed that paeonol could inhibit apoptosis, hypertrophy, and fibrosis through activation of Notch1 signaling, thereby attenuating adriamycin-induced pathological cardiac remodeling to reverse cardiotoxicity (Thabassum Akhtar Iqbal et al., 2020). Mitochondria are responsible for providing most of the energy for cardiomyocytes, and mitochondrial fusion and fission regulate the dynamic formation and remodeling of the mitochondrial network (Varga et al., 2015). DOX was found to inhibit mitochondrial fusion and accelerate mitochondrial fission, promoting ROS production and mitochondrial dysfunction and inducing cardiac myocardial injury, which was reversed by paeonol. Therefore, a deeper investigation of the mechanism revealed that paeonol exerts cardioprotective effects by restoring Mfn2-mediated mitochondrial fusion and function, and PKCε may act as a direct target of paeonol to activate Stat3-Mfn2 signaling pathway and promote mitochondrial fusion (Ding et al., 2023). The role of oxidative and nitrosative stress and inflammatory responses has been demonstrated in the methotrexate (MTX)-induced cardiotoxicity model. Administration of paeonol treatment reduced NOX-2, MAD, and NO levels and increased GSH and SOD expression. Simultaneous inhibition of the TLR4/NF-KB pathway to attenuate the inflammatory response to improve oxidative stress has also been suggested as a possible mechanism for paeonol to attenuate MTX-induced cardiotoxicity (Al-Taher et al., 2020). MiRNAs play a crucial role in the pathogenesis of various heart diseases. Evidence suggests that paeonol improves cardiac function in a rat model of DOX-induced chronic heart failure by inhibiting miR-21-5P and upregulating the expression of miR-21-5P downstream target S-phase kinase-associated protein 2 (SKP2) to inhibit apoptosis (Chen et al., 2022a). In epirubicin (EPI)-induced cardiotoxicity, LC3-II/I, Beclin-1, and Atg 5 levels were significantly decreased, indicating that autophagic activity was significantly inhibited, whereas paeonol reversed EPI-induced inhibition of autophagy. Further study revealed that miR-1 was downregulated in EPI-induced myocardial injury, while paeonol could inhibit the activation of PI3K/AKT/mTOR and its downstream NF-kB signaling pathway by increasing miR-1 expression, thus promoting autophagy and ameliorating myocardial injury (Wu et al., 2018). Our findings indicate that paeonol modulates oxidative stress, mitochondrial function, autophagy, apoptosis, and other mechanisms to regulate cardiotoxicity induced by anticancer treatments while synergistically enhancing anticancer effectiveness.
4.2 Protective effects of paeonol on hepatotoxicity
The liver is the primary organ responsible for drug metabolism. Therefore, chemotherapy treatments can potentially cause reversible or permanent damage to the liver, such as steatosis, steatohepatitis, and sinusoidal obstruction (White et al., 2016). Additionally, patients with pre-existing liver disease or liver cancer may experience aggravated hepatotoxicity (Thatishetty et al., 2013). In vivo and in vitro studies, EPI can cause hepatocyte death, while co-treatment of EPI and paeonol can decrease hepatocytes death and reverse the increased levels of hepatic functional enzymes (ALT, AST, ALP, and GGT) while attenuating EPI-induced oxidative stress injury (SOD, CAT, and GSH) and inflammatory response (IL-2, IL-6, TNF-α, IFN-α), which is related to the inhibition of PI3K/AKT/NF-kB pathway (Wu et al., 2016b). The research team utilized metabonomics research to discover that paeonol effectively inhibits autophagy induced by the AMPK/mTOR signaling pathway. Its metabolic mechanism involves intricate interactions with lipid metabolism (specifically palmitic acid and oleic acid), amino acid metabolism (serine, valine, leucine, and glycine), and energy metabolism (succinate and malate) (Jing et al., 2019). In MTX-induced hepatotoxicity model in mice, paeonol has been shown to promote the expression of drug efflux transporters, specifically P-gp and multidrug resistance-associated protein 2 (Mrp-2). This mechanism may be vital in reversing the hepatotoxicity caused by MTX hepatic accumulation (Morsy et al., 2022a). These findings offer significant contributions to the comprehension of the hepatoprotective properties of paeonol via the regulation of metabolic pathways. These outcomes emphasize the clinical potential of paeonol in preventing and treating hepatotoxicity.
4.3 Protective effects of paeonol on nephrotoxicity
The kidney serves as the primary pathway for eliminating both anticancer drugs and their metabolites, leading to damage of glomeruli and renal tubules during the excretion of chemotherapy drugs. In addition to conventional cytotoxic drugs, molecularly targeted drugs have also been implicated in causing nephrotoxicity, manifesting as a range of kidney diseases and electrolyte imbalances (Małyszko et al., 2016). Cisplatin is a widely used chemotherapeutic drug that is known to cause nephrotoxicity. A mouse model of cisplatin-induced acute renal failure showed signs of renal tubular injury with elevated serum creatinine and urea levels. In comparison, paeonol reversed these results and attenuated cisplatin-induced high levels of pro-inflammatory cytokines (TNF-α and IL-1β) and nitrite. These results suggest that the protective effect of paeonol against cisplatin-induced nephrotoxicity may be mediated by inhibiting inflammation and nitrosative stress (Lee et al., 2013). (Wu et al., 2017) further discovered that paeonol protects against DOX-induced nephrotoxicity by activating the Nrf2/HO-1 pathway to mitigate oxidative stress and nitrosative stress and by suppressing the inflammatory response through the NF-κB pathway. Moreover, paeonol attenuating the nephrotoxicity associated with renal accumulation of MTX by promoting the expression of renal efflux transport proteins is an important mechanism (Morsy et al., 2022b). A recent study has revealed that overexpression of the long non-coding RNA (lncRNA) maternally expressed gene 3 (lnc-MEG3) can lead to the inhibition of miRNA-126 and the subsequent activation of the AKT/TSC/mTOR pathway, which in turn suppresses autophagy and aggravates cisplatin-induced nephrotoxicity. Instead, paeonol can target and inhibit lnc-MEG3 and its related signaling pathways to regulate autophagy, exerting nephroprotective effects (Jing et al., 2021). These findings suggest that lncRNA or miRNA may be novel targets for preventing cancer treatment-induced nephrotoxicity, and the underlying mechanisms need to be thoroughly investigated.
4.4 Protective effects of paeonol on testicular injury
The administration of cytotoxic chemotherapy and radiotherapy as therapeutic interventions in malignancies, such as lymphoma, testicular cancer, osteosarcoma, and lung cancer, can elicit testicular dysfunction in male patients as a prevalent and significant long-term adverse effect. The resultant dysfunction manifests as either temporary or permanent oligospermia and azoospermia, owing to the detrimental effects of these therapies on the germinal epithelium (Howell and Shalet, 2005). The study revealed that paeonol significantly ameliorated MTX-induced testicular damage, as evidenced by the augmentation of testicular weight, elevation of testosterone levels, and improvement in pathological changes within the seminiferous tubules. The underlying mechanisms of paeonol’s protective effects are attributed to its ability to upregulate testicular P-gp levels and downregulate TNF-α and caspase 3 expression, concurrent with a decline in MDA and NOx levels and a rise in GSH levels and SOD activity. It can be surmised that paeonol protects against MTX-induced testicular injury by mitigating oxidative stress, inflammation, and apoptosis. Furthermore, the upregulation of P-gp expression mediated by paeonol is a promising and effective protective mechanism against MTX-induced testicular injury (Morsy et al., 2020).
5 Discussion and future perspectives
With the continuous progress of medical diagnosis and treatment, the mortality rate of cancer is showing a downward trend year by year (Siegel et al., 2023). However, the acute or chronic toxic side effects caused by various anticancer therapies seriously affect patients’ quality of life (Schirrmacher, 2019). Therefore, exploring natural products with abundant anticancer potential has become the focus of cancer drug development (Huang et al., 2021). Paeonol, a bioactive substance found in CM, has demonstrated excellent effectiveness against malignant tumors in both in vitro and in vivo experiments. Its anticancer functions include inhibiting tumor growth, protecting against multiorgan damage caused by cancer treatment, and demonstrating broad-spectrum antitumor effects that inhibit a wide range of tumor cells (including lung cancer, stomach cancer, breast cancer, prostate cancer, and many other cancers). Paeonol’s numerous pharmacological activities enable it to act through various mechanisms, including the induction of cancer cell apoptosis, autophagy, cell cycle arrest, and inhibition of cancer cell migration and angiogenesis. These mechanisms implicate the participation of various molecular targets and signaling pathways, among which the PI3K/AKT/mTOR and NF-κB pathways are significant. Furthermore, miRNAs can potentially be a promising target for studying the anticancer effects of paeonol. (Figure 2).
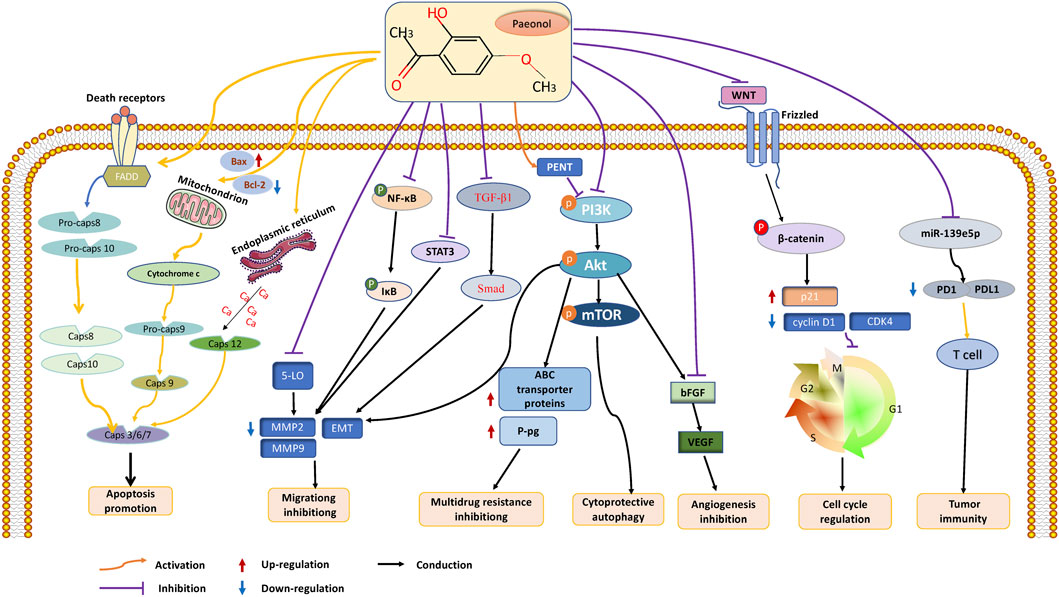
FIGURE 2. The anticancer effects and mechanisms of paeonol. Paeonol can exert anticancer effects through various mechanisms, including 1. Inhibiting the proliferation of various tumor cells; 2. Inhibit cell invasion and migration by inhibiting EMT of tumor cells; 3. Promote intracellular apoptosis through intrinsic and extrinsic pathways; 3. Induce cell cycle arrest, including G1, S, G2, and M phases; 4. Inhibit angiogenesis by targeting VEGF; 5. Promote the formation of autophagosomes and autophagy of cancer cells; 6. Regulating tumor immunity; 7. Reverse multidrug resistance and increase radiosensitivity.
The multiorgan toxicities associated with cancer therapeutics, including those affecting the heart, liver, kidneys, and testes, represent a significant impediment to the clinical application of cancer therapeutics and can ultimately impact the prognosis of cancer patients. Paeonol was found to have a protective effect on multiorgan damage induced by anticancer treatment. Research has revealed that paeonol can ameliorate heart, liver, kidney, and testicular injury caused by chemotherapy drugs such as DOX, EPI, and MTX. This is accomplished by regulating oxidative stress, mitochondrial function, inflammatory reaction, autophagy, and cell apoptosis. (Figure 3). Combined with the existing studies, we found that paeonol has significant anticancer activity and certain organ protective effects, which may be related to the fact that paeonol exerts different regulatory mechanisms in different cell types. For example, in tumor cells, paeonol can induce apoptosis and inhibit tumor growth, while under the intervention of chemotherapeutic drugs, cardiomyocytes, hepatocytes, and renal cells showed noticeable apoptotic changes, at which time paeonol can exert organ-protective effects by inhibiting apoptosis. In addition, paeonol plays an essential role in reversing chemotherapy drug resistance and organ damage induced by anticancer treatment by modulating drug efflux. However, it was found that paeonol could reverse paclitaxel resistance by down-regulating P-gp expression. Still, it can protect against organ damage by upregulating P-gp expression in chemotherapeutic drug-induced liver toxicity and testicular injury. This suggests that regulating P-gp levels by paeonol in malignant and non-malignant cells may be multifactorial, and the mechanism needs further investigation. Gastrointestinal toxicity is another common side effect associated with anticancer treatment, and in severe cases, it can be life-threatening (Huang et al., 2021). However, to date, no research has been conducted on the potential benefits of paeonol in combating gastrointestinal toxicity, indicating a promising avenue for future investigation.
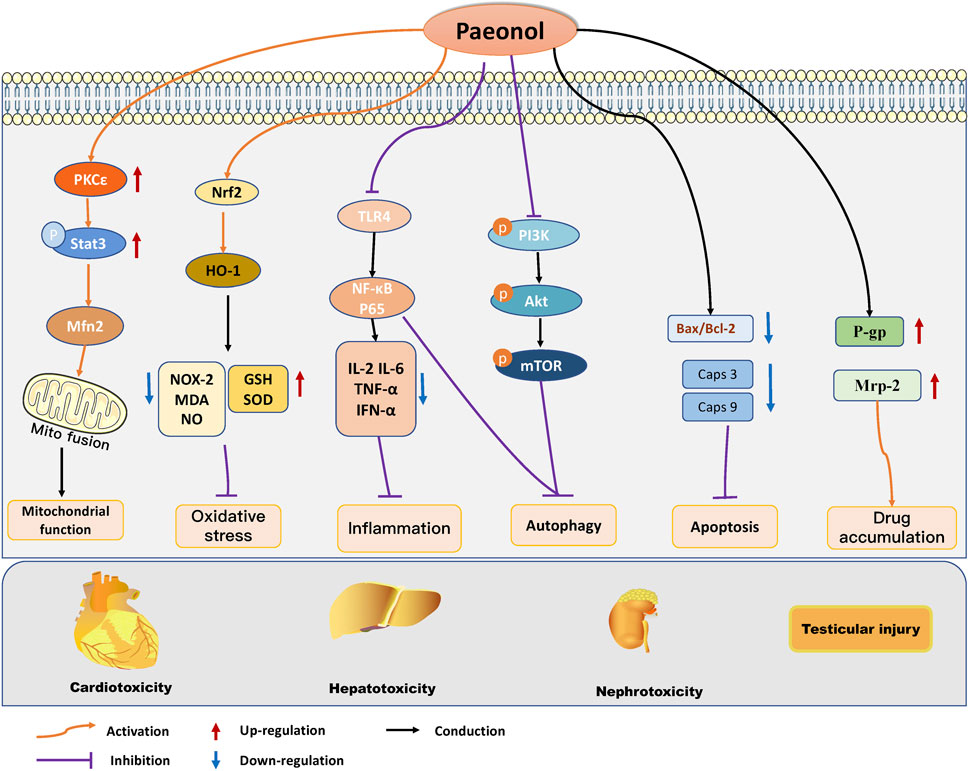
FIGURE 3. Molecular protective mechanism of paeonol against cancer therapy-induced side effects, mainly including cardiotoxicity, hepatotoxicity, nephrotoxicity, and testicular damage. Paeonol modulates oxidative stress, mitochondrial function, autophagy, apoptosis, inflammation, and apoptosis to regulate multiple organ injuries induced by anticancer treatments.
To overcome the limitations associated with the poor physical properties of paeonol, including limited solubility, poor stability, rapid metabolism, and low bioavailability, various drug delivery systems have been developed, such as nanocarriers, liposomes, and microemulsions. These drug delivery systems have not only effectively improved the pharmacokinetic deficiencies of paeonol but also enhanced its target specificity, resulting in increased bioavailability and anticancer efficacy of paeonol, thereby addressing the practical limitations of its application.
In conclusion, paeonol exerts antitumor activity by inhibiting cell proliferation, inducing apoptosis, inhibiting metastasis and angiogenesis, regulating tumor immunity, reversing drug resistance, and protecting against cancer treatment-induced multiorgan damage by regulating oxidative stress, apoptosis, mitochondrial function, and autophagy. It is a promising natural active medicine. Combined with the dual regulatory effects of paeonol in tumors, we have reason to believe that paeonol may have a wide range of prospects as an adjuvant strategy or even as a primary therapy in clinical treatment. However, the current research on the effects of paeonol on tumor prevention and therapy mainly comprises basic experimental studies and lacks clinical evidence to support these findings. Therefore, large-scale, high-quality, randomized controlled trials are necessary to evaluate the safety and efficacy of paeonol in clinical tumor patients and provide the clinical data needed to support the widespread use of paeonol.
Author contributions
XC was a major contributor to writing the manuscript. PL and YW conceived and designed the study, XF critically revised the paper. MD, SL, and JW amended the paper. All authors contributed to the article and approved the submitted version.
Funding
This research was funded by the National Natural Science Foundation of China (82204849, 82074200, 81873117).
Acknowledgments
We thank all authors for their suggestions and comments on this article.
Conflict of interest
The authors declare that the research was conducted in the absence of any commercial or financial relationships that could be construed as a potential conflict of interest.
Publisher’s note
All claims expressed in this article are solely those of the authors and do not necessarily represent those of their affiliated organizations, or those of the publisher, the editors and the reviewers. Any product that may be evaluated in this article, or claim that may be made by its manufacturer, is not guaranteed or endorsed by the publisher.
References
Adki, K. M., and Kulkarni, Y. A. (2020). Chemistry, pharmacokinetics, pharmacology and recent novel drug delivery systems of paeonol. Life Sci. 250, 117544. doi:10.1016/j.lfs.2020.117544
Ajazuddin, , and Saraf, S. (2010). Applications of novel drug delivery system for herbal formulations. Fitoterapia 81 (7), 680–689. doi:10.1016/j.fitote.2010.05.001
Al-Taher, A. Y., Morsy, M. A., Rifaai, R. A., Zenhom, N. M., and Abdel-Gaber, S. A. (2020). Paeonol attenuates methotrexate-induced cardiac toxicity in rats by inhibiting oxidative stress and suppressing TLR4-induced NF-κB inflammatory pathway. Mediat. Inflamm. 2020, 8641026. doi:10.1155/2020/8641026
Babaei, G., Aziz, S. G., and Jaghi, N. Z. Z. (2021). EMT, cancer stem cells and autophagy; the three main axes of metastasis. Biomed. Pharmacother. 133, 110909. doi:10.1016/j.biopha.2020.110909
Bray, F., Ferlay, J., Soerjomataram, I., Siegel, R. L., Torre, L. A., and Jemal, A. (2018). Global cancer statistics 2018: GLOBOCAN estimates of incidence and mortality worldwide for 36 cancers in 185 countries. Ca-a Cancer J. Clin. 68 (6), 394–424. doi:10.3322/caac.21492
Cai, J., Chen, S., Zhang, W., Hu, S., Lu, J., Xing, J., et al. (2014). Paeonol reverses paclitaxel resistance in human breast cancer cells by regulating the expression of transgelin 2. Phytomedicine 21 (7), 984–991. doi:10.1016/j.phymed.2014.02.012
Cai, M., Shao, W., Yu, H., Hong, Y., and Shi, L. (2020). Paeonol inhibits cell proliferation, migration and invasion and induces apoptosis in hepatocellular carcinoma by regulating miR-21-5p/KLF6 Axis. Cancer Manag. Res. 12, 5931–5943. doi:10.2147/cmar.S254485
Carneiro, B. A., and El-Deiry, W. S. (2020). Targeting apoptosis in cancer therapy. Nature Reviews Clinical Oncology 17 (7), 395–417. doi:10.1038/s41571-020-0341-y
Chan, S. H., and Wang, L. H. (2015). Regulation of cancer metastasis by microRNAs. J. Biomed. Sci. 22 (1), 9. doi:10.1186/s12929-015-0113-7
Chauhan, A., Islam, A. U., Prakash, H., and Singh, S. (2022). Phytochemicals targeting NF-κB signaling: Potential anti-cancer interventions. J. Pharm. Anal. 12 (3), 394–405. doi:10.1016/j.jpha.2021.07.002
Chen, C., Jia, F., Hou, Z., Ruan, S., and Lu, Q. (2017). Delivery of paeonol by nanoparticles enhances its in vitro and in vivo antitumor effects. Int. J. Nanomedicine 12, 6605–6616. doi:10.2147/ijn.S143938
Chen, Y., and Kirchmair, J. (2020). Cheminformatics in natural product-based drug discovery. Mol. Inf. 39 (12), e2000171. doi:10.1002/minf.202000171
Chen, X., and Cubillos-Ruiz, J. R. (2021). Endoplasmic reticulum stress signals in the tumour and its microenvironment. Nat Rev Cancer 21 (2), 71–88. doi:10.1038/s41568-020-00312-2
Chen, C., Liu, S., Cao, G., Hu, Y., Wang, R., Wu, M., et al. (2022a). Cardioprotective effect of paeonol on chronic heart failure induced by doxorubicin via regulating the miR-21-5p/S-phase kinase-associated protein 2 Axis. Front. Cardiovasc Med. 9, 695004. doi:10.3389/fcvm.2022.695004
Chen, Y., Jia, Y., Li, Y., Zheng, Y., Chen, G., and Shi, Y. (2022b). Investigation on the antitumor effects of paeonol against renal cell carcinoma based on network pharmacology and experimental validation. J. Ethnopharmacol. 285, 114857. doi:10.1016/j.jep.2021.114857
Chen, X., Xu, Z., Lu, M., Ding, W., Zhong, J., Deng, S., et al. (2023). Paeonol inhibits melanoma growth by targeting PD1 through upregulation of miR-139-5p. Biochem Biophys Res Commun 656, 86–96. doi:10.1016/j.bbrc.2023.03.005
Cheng, C. S., Chen, J. X., Tang, J., Geng, Y. W., Zheng, L., Lv, L. L., et al. (2020). Paeonol inhibits pancreatic cancer cell migration and invasion through the inhibition of TGF-β1/smad signaling and epithelial-mesenchymal-transition. Cancer Manag. Res. 12, 641–651. doi:10.2147/cmar.S224416
Cheng, Y., Li, M., Tan, S., and Zhou, Z. (2019). Effects of paeonol on cell proliferation, apoptosis and autophagy in colorectal cancer cells. J. Med. Res. 48 (10), 144–147. (in Chinese)
Chunhu, Z., Suiyu, H., Meiqun, C., Guilin, X., and Yunhui, L. (2008). Antiproliferative and apoptotic effects of paeonol on human hepatocellular carcinoma cells. Anticancer Drugs 19 (4), 401–409. doi:10.1097/CAD.0b013e3282f7f4eb
Curigliano, G., Cardinale, D., Dent, S., Criscitiello, C., Aseyev, O., Lenihan, D., et al. (2016). Cardiotoxicity of anticancer treatments: Epidemiology, detection, and management. CA Cancer J. Clin. 66 (4), 309–325. doi:10.3322/caac.21341
Ding, M., Shi, R., Fu, F., Li, M., De, D., Du, Y., et al. (2023). Paeonol protects against doxorubicin-induced cardiotoxicity by promoting Mfn2-mediated mitochondrial fusion through activating the PKCε-Stat3 pathway. J. Adv. Res. 47, 151–162. doi:10.1016/j.jare.2022.07.002
Du, J., Song, D., Li, J., Li, Y., Li, B., and Li, L. (2022). Paeonol triggers apoptosis in HeLa cervical cancer cells: The role of mitochondria-related caspase pathway. Psychopharmacol. Berl. 239 (7), 2083–2092. doi:10.1007/s00213-021-05811-0
El-Tanani, M., Nsairat, H., Aljabali, A. A., Serrano-Aroca, Á., Mishra, V., Mishra, Y., et al. (2023). Role of mammalian target of rapamycin (mTOR) signalling in oncogenesis. Life Sciences 323, 121662. doi:10.1016/j.lfs.2023.121662
Fan, L., Song, B., Sun, G., Ma, T., Zhong, F., and Wei, W. (2013). Endoplasmic reticulum stress-induced resistance to doxorubicin is reversed by paeonol treatment in human hepatocellular carcinoma cells. PLoS One 8 (5), e62627. doi:10.1371/journal.pone.0062627
Fan, L., Sun, G., and Cha, L. (2018). Paeonol increases HepG2 cells to endoplasmic reticulum stress-induced apoptosis resistance. Acta Universitatis Medicinalis Anhui 53 (1), 45–50. doi:10.19405/j.cnki.issn1000-1492.2018.01.010
Fares, J., Fares, M. Y., Khachfe, H. H., Salhab, H. A., and Fares, Y. (2020). Molecular principles of metastasis: A hallmark of cancer revisited. Signal Transduct. Target Ther. 5 (1), 28. doi:10.1038/s41392-020-0134-x
Fu, J., Yu, L., Luo, J., Huo, R., and Zhu, B. (2018). Paeonol induces the apoptosis of the SGC-7901 gastric cancer cell line by downregulating ERBB2 and inhibiting the NF-κB signaling pathway. Int. J. Mol. Med. 42 (3), 1473–1483. doi:10.3892/ijmm.2018.3704
Gao, L., Wang, Z., Lu, D., Huang, J., Liu, J., and Hong, L. (2019). Paeonol induces cytoprotective autophagy via blocking the Akt/mTOR pathway in ovarian cancer cells. Cell Death Dis. 10 (8), 609. doi:10.1038/s41419-019-1849-x
Gatti, L., and Zunino, F. (2005). Overview of tumor cell chemoresistance mechanisms. Methods Mol. Med. 111, 127–148. doi:10.1385/1-59259-889-7:127
Goel, S., DeCristo, M. J., McAllister, S. S., and Zhao, J. J. (2018). CDK4/6 inhibition in cancer: Beyond cell cycle arrest. Trends Cell Biol. 28 (11), 911–925. doi:10.1016/j.tcb.2018.07.002
Han, L., Guo, X., Chen, Z., and Bian, H. (2018). The mechanisms of paeonol reversing multidrug resistance in ovarian cancer SKOV3/DDP cells. Acta Pharm. Sin. B 53 (09), 1511–1517. doi:10.16438/j.0513-4870.2018-0407
Hao, M., Zhang, K., Wang, H., Wang, J., Li, J., Cheng, R., et al. (2023). Paeonol Inhibits Glioma Growth In Vivo and In Vitro by Inducing Apoptosis and Cell Cycle Arrest. Revista Brasileira de Farmacognosia 33 (3), 534–542. doi:10.1007/s43450-023-00380-z
Hasan, S., Taha, R., and Omri, H. E. (2018). Current opinions on chemoresistance: An overview. Bioinformation 14 (2), 80–85. doi:10.6026/97320630014080
He, R. X., Ye, X., Li, R., Chen, W., Ge, T., Huang, T. Q., et al. (2017). PEGylated niosomes-mediated drug delivery systems for paeonol: Preparation, pharmacokinetics studies and synergistic anti-tumor effects with 5-FU. J. Liposome Res. 27 (2), 161–170. doi:10.1080/08982104.2016.1191021
Horng, C. T., Shieh, P. C., Tan, T. W., Yang, W. H., and Tang, C. H. (2014). Paeonol suppresses chondrosarcoma metastasis through up-regulation of miR-141 by modulating PKCδ and c-Src signaling pathway. Int. J. Mol. Sci. 15 (7), 11760–11772. doi:10.3390/ijms150711760
Howell, S. J., and Shalet, S. M. (2005). Spermatogenesis after cancer treatment: Damage and recovery. J. Natl. Cancer Inst. Monogr. 34, 12–17. doi:10.1093/jncimonographs/lgi003
Hu, X., Ding, L., Cao, S., Cheng, L., Wang, K., Guang, C., et al. (2020). Pharmacokinetics, tissue distribution and excretion of paeonol and its major metabolites in rats provide a further insight into paeonol effectiveness. Front. Pharmacol. 11, 190. doi:10.3389/fphar.2020.00190
Huang, M., Lu, J.-J., Ding, J., Yang, Y., Jiang, W., Wang, F., et al. (2021). Natural products in cancer therapy: Past, present and future. Nat. Prod. bioprospecting 11, 5–13. doi:10.1007/s13659-020-00293-7
Jing, X., Han, J., Zhang, J., Chen, Y., Yuan, J., Wang, J., et al. (2021). Long non-coding RNA MEG3 promotes cisplatin-induced nephrotoxicity through regulating AKT/TSC/mTOR-mediated autophagy. Int. J. Biol. Sci. 17 (14), 3968–3980. doi:10.7150/ijbs.58910
Jing, X., Sun, C., Chen, H., Sun, J., Zhang, Y., and Wu, J. (2019). Protection of paeonol against epirubicin-induced hepatotoxicity: A metabolomic study. Biosci. Trends 13 (3), 253–260. doi:10.5582/bst.2019.01105
Kaczanowski, S. (2016). Apoptosis: Its origin, history, maintenance and the medical implications for cancer and aging. Phys. Biol. 13 (3), 031001. doi:10.1088/1478-3975/13/3/031001
Kennedy, L. B., and Salama, A. K. S. (2020). A review of cancer immunotherapy toxicity. CA Cancer J. Clin. 70 (2), 86–104. doi:10.3322/caac.21596
Kim, S. A., Lee, H. J., Ahn, K. S., Lee, H. J., Lee, E. O., Ahn, K. S., et al. (2009). Paeonol exerts anti-angiogenic and anti-metastatic activities through downmodulation of Akt activation and inactivation of matrix metalloproteinases. Biol. Pharm. Bull. 32 (7), 1142–1147. doi:10.1248/bpb.32.1142
Kolodny, G., Li, X., and Balk, S. (2018). Addressing cancer chemotherapeutic toxicity, resistance, and heterogeneity: Novel theranostic use of DNA-encoded small molecule libraries. Bioessays 40 (10), e1800057. doi:10.1002/bies.201800057
Lai, X., Eberhardt, M., Schmitz, U., and Vera, J. (2019). Systems biology-based investigation of cooperating microRNAs as monotherapy or adjuvant therapy in cancer. Nucleic Acids Research 47 (15), 7753–7766. doi:10.1093/nar/gkz638
Lambert, A. W., Pattabiraman, D. R., and Weinberg, R. A. (2017). Emerging biological principles of metastasis. Cell 168 (4), 670–691. doi:10.1016/j.cell.2016.11.037
Latif, S., Choi, S. H., Gyawali, A., Hyeon, S. J., Kang, Y. S., and Ryu, H. (2022). Antioxidant and neuroprotective effects of paeonol against oxidative stress and altered carrier-mediated transport system on NSC-34 cell lines. Antioxidants (Basel) 11 (7), 1392. doi:10.3390/antiox11071392
Lee, H. J., Kim, S. A., Lee, H. J., Jeong, S. J., Han, I., Jung, J. H., et al. (2010). Paeonol oxime inhibits bFGF-induced angiogenesis and reduces VEGF levels in fibrosarcoma cells. PLoS One 5 (8), e12358. doi:10.1371/journal.pone.0012358
Lee, H., Lee, G., Kim, H., and Bae, H. (2013). Paeonol, a major compound of moutan cortex, attenuates Cisplatin-induced nephrotoxicity in mice. Evid. Based Complement. Altern. Med. 2013, 310989. doi:10.1155/2013/310989
Lei, Y., Li, H. X., Jin, W. S., Peng, W. R., Zhang, C. J., Bu, L. J., et al. (2013). The radiosensitizing effect of Paeonol on lung adenocarcinoma by augmentation of radiation-induced apoptosis and inhibition of the PI3K/Akt pathway. Int. J. Radiat. Biol. 89 (12), 1079–1086. doi:10.3109/09553002.2013.825058
Li, M., Tan, S. Y., and Wang, X. F. (2014). Paeonol exerts an anticancer effect on human colorectal cancer cells through inhibition of PGE₂ synthesis and COX-2 expression. Oncol. Rep. 32 (6), 2845–2853. doi:10.3892/or.2014.3543
Li, M., Tan, S. Y., Zhang, J., and You, H. X. (2013). Effects of paeonol on intracellular calcium concentration and expression of RUNX3 in LoVo human colon cancer cells. Mol. Med. Rep. 7 (5), 1425–1430. doi:10.3892/mmr.2013.1372
Li, N., Fan, L. L., Sun, G. P., Wan, X. A., Wang, Z. G., Wu, Q., et al. (2010). Paeonol inhibits tumor growth in gastric cancer in vitro and in vivo. World J. Gastroenterol. 16 (35), 4483–4490. doi:10.3748/wjg.v16.i35.4483
Li, Q., Wang, L., Tang, J., and Hong, L. (2017). Anticancer activity of paeonol on human ovarian cancer A2780 cells by inhibiting Wnt/β-catenin signal pathway. J. Chin. Pract. Diagnosis Ther. 31 (11), 1062–1066. doi:10.13507/j.issn.1674-3474.2017.11.006 (in Chinese)
Li, Q., Zhang, Y., Sun, J., and Bo, Q. (2019). Paeonol-mediated apoptosis of hepatocellular carcinoma cells by NF-κB pathway. Oncol. Lett. 17 (2), 1761–1767. doi:10.3892/ol.2018.9730
Li, X., He, S., and Ma, B. (2020). Autophagy and autophagy-related proteins in cancer. Mol. Cancer 19 (1), 12. doi:10.1186/s12943-020-1138-4
Liu, H., and Zhang, C. (2020). Paeonol induces antitumor effects in hepatocellular carcinoma cells through survivin via the cyclooxygenase-2/prostaglandin E2 signaling pathway. Transl. Cancer Res. 9 (11), 7183–7195. doi:10.21037/tcr-20-322A
Liu, L. H., Shi, R. J., and Chen, Z. C. (2020). Paeonol exerts anti-tumor activity against colorectal cancer cells by inducing G0/G1 phase arrest and cell apoptosis via inhibiting the Wnt/β-catenin signaling pathway. Int. J. Mol. Med. 46 (2), 675–684. doi:10.3892/ijmm.2020.4629
Loftus, L. V., Amend, S. R., and Pienta, K. J. (2022). Interplay between cell death and cell proliferation reveals new strategies for cancer therapy. Int. J. Mol. Sci. 23 (9), 4723. doi:10.3390/ijms23094723
Lv, J., Zhu, S., Chen, H., Xu, Y., Su, Q., Yu, G., et al. (2022). Paeonol inhibits human lung cancer cell viability and metastasis in vitro via miR-126-5p/ZEB2 axis. Drug Dev. Res. 83 (2), 432–446. doi:10.1002/ddr.21873
Lyu, Z. K., Li, C. L., Jin, Y., Liu, Y. Z., Zhang, X., Zhang, F., et al. (2017). Paeonol exerts potential activities to inhibit the growth, migration and invasion of human gastric cancer BGC823 cells via downregulating MMP-2 and MMP-9. Mol. Med. Rep. 16 (5), 7513–7519. doi:10.3892/mmr.2017.7576
Ma, L., Miao, J., Xu, X., Tang, B., and Zhang, Q. (2009). Pharmacokinetic features and absolute bioavailability of paeonol in conscious rat. Lishizhen Med. Materia Medica 20 (02), 413–414. (in Chinese)
Ma, L. Y., Xu, X. D., Zhang, Q., Miao, J. H., and Tang, B. L. (2008). Paeonol pharmacokinetics in the rat following i.m. administration. Eur. J. Drug Metab. Pharmacokinet. 33 (3), 133–136. doi:10.1007/bf03191109
Małyszko, J., Kozłowska, K., Kozłowski, L., and Małyszko, J. (2016). Nephrotoxicity of anticancer treatment. Nephrol. Dial. Transplant. 32 (6), 924–936. doi:10.1093/ndt/gfw338
Mao, X., Wang, M., Wang, J., Jiang, G., and Yuan, S. (2020). The mechanism of Nrf2/HO-1 signaling involved in the inhibitory effect of paeonol on the growth of cervical carcinoma hela cells. Guangdong Chem. Ind. 47 (01), 21–22. (in Chinese)
Matthews, H. K., Bertoli, C., and de Bruin, R. A. M. (2022). Cell cycle control in cancer. Nat. Rev. Mol. Cell Biol. 23 (1), 74–88. doi:10.1038/s41580-021-00404-3
Mattiuzzi, C., and Lippi, G. (2019). Current cancer epidemiology. J. Epidemiol. Glob. Health 9 (4), 217–222. doi:10.2991/jegh.k.191008.001
Miller, K. D., Nogueira, L., Mariotto, A. B., Rowland, J. H., Yabroff, K. R., Alfano, C. M., et al. (2019). Cancer treatment and survivorship statistics, 2019. CA Cancer J. Clin. 69 (5), 363–385. doi:10.3322/caac.21565
Morsy, M. A., Abdel-Aziz, A. M., Abdel-Hafez, S. M. N., Venugopala, K. N., Nair, A. B., and Abdel-Gaber, S. A. (2020). The possible contribution of P-glycoprotein in the protective effect of paeonol against methotrexate-induced testicular injury in rats. Pharm. (Basel) 13 (9), 223. doi:10.3390/ph13090223
Morsy, M. A., Abdel-Latif, R., Hafez, S., Kandeel, M., and Abdel-Gaber, S. A. (2022a). Paeonol protects against methotrexate hepatotoxicity by repressing oxidative stress, inflammation, and apoptosis-the role of drug efflux transporters. Pharm. (Basel) 15 (10), 1296. doi:10.3390/ph15101296
Morsy, M. A., El-Sheikh, A. A. K., Abdel-Hafez, S. M. N., Kandeel, M., and Abdel-Gaber, S. A. (2022b). Paeonol protects against methotrexate-induced nephrotoxicity via upregulation of P-gp expression and inhibition of TLR4/NF-κB pathway. Front. Pharmacol. 13, 774387. doi:10.3389/fphar.2022.774387
Nishida, N., Yano, H., Nishida, T., Kamura, T., and Kojiro, M. (2006). Angiogenesis in cancer. Vasc. Health Risk Manag. 2 (3), 213–219. doi:10.2147/vhrm.2006.2.3.213
Niu, Q., Niu, H., and Zhai, J. (2020). Paeonol promotes apoptosis of ovarian cancer cells through EndoplasmicReticulum stress and mitochondrial damage pathways. J. Guangzhou Univ. Traditional Chin. Med. 37 (10), 1991–1998. doi:10.13359/j.cnki.gzxbtcm.2020.10.028 (in Chinese)
Novikov, N. M., Zolotaryova, S. Y., Gautreau, A. M., and Denisov, E. V. (2021). Mutational drivers of cancer cell migration and invasion. Br. J. Cancer 124 (1), 102–114. doi:10.1038/s41416-020-01149-0
Onorati, A. V., Dyczynski, M., Ojha, R., and Amaravadi, R. K. (2018). Targeting autophagy in cancer. Cancer 124 (16), 3307–3318. doi:10.1002/cncr.31335
Ou, Y., Li, Q., Wang, J., Li, K., and Zhou, S. (2014). Antitumor and apoptosis induction effects of paeonol on mice bearing EMT6 breast carcinoma. Biomol. Ther. Seoul. 22 (4), 341–346. doi:10.4062/biomolther.2013.106
Przybylski, M. (2009). A review of the current research on the role of bFGF and VEGF in angiogenesis. J Wound Care 18 (12), 516–519. doi:10.12968/jowc.2009.18.12.45609
Qian, S., Wei, Z., Yang, W., Huang, J., Yang, Y., and Wang, J. (2022). The role of BCL-2 family proteins in regulating apoptosis and cancer therapy. Front. Oncol. 12, 985363. doi:10.3389/fonc.2022.985363
Rajabi, M., and Mousa, S. A. (2017). The role of angiogenesis in cancer treatment. Biomedicines 5 (2), 34. doi:10.3390/biomedicines5020034
Rawat, P. S., Jaiswal, A., Khurana, A., Bhatti, J. S., and Navik, U. (2021). Doxorubicin-induced cardiotoxicity: An update on the molecular mechanism and novel therapeutic strategies for effective management. Biomed. Pharmacother. 139, 111708. doi:10.1016/j.biopha.2021.111708
Ren, Z., and Zhang, F. (2017). Influence of paeonol combined with γ ray on glioma cell apoptosis and apoptosis gene expressiona. J. Hainan Med. Univ. 23 (23), 3191–3194+3198. doi:10.13210/j.cnki.jhmu.20171130.002 (in Chinese)
Saahene, R. O., Wang, J., Wang, M. L., Agbo, E., and Pang, D. (2018). The antitumor mechanism of paeonol on CXCL4/CXCR3-B signals in breast cancer through induction of tumor cell apoptosis. Cancer Biother Radiopharm. 33 (6), 233–240. doi:10.1089/cbr.2018.2450
Schirrmacher, V. (2019). From chemotherapy to biological therapy: A review of novel concepts to reduce the side effects of systemic cancer treatment (review). Int. J. Oncol. 54 (2), 407–419. doi:10.3892/ijo.2018.4661
Senthebane, D. A., Rowe, A., Thomford, N. E., Shipanga, H., Munro, D., Mazeedi, M. A. M. A., et al. (2017). The role of tumor microenvironment in chemoresistance: To survive, keep your enemies closer. Int. J. Mol. Sci. 18 (7), 1586. doi:10.3390/ijms18071586
Shabalala, S., Muller, C. J. F., Louw, J., and Johnson, R. (2017). Polyphenols, autophagy and doxorubicin-induced cardiotoxicity. Life Sci. 180, 160–170. doi:10.1016/j.lfs.2017.05.003
Sheng, S. Q., Yu, L. Y., Zhou, X. W., Pan, H. Y., Hu, F. Y., and Liu, J. L. (2021). Paeonol prevents migration and invasion, and promotes apoptosis of cervical cancer cells by inhibiting 5-lipoxygenase. Mol. Med. Rep. 23 (6), 401. doi:10.3892/mmr.2021.12040
Siegel, R. L., Miller, K. D., Wagle, N. S., and Jemal, A. (2023). Cancer statistics, 2023. CA Cancer J. Clin. 73 (1), 17–48. doi:10.3322/caac.21763
Su, Z., Yang, Z., Xu, Y., Chen, Y., and Yu, Q. (2015). Apoptosis, autophagy, necroptosis, and cancer metastasis. Mol. Cancer 14, 48. doi:10.1186/s12943-015-0321-5
Sun, A., Chen, J., Guan, H., and Li, P. (2016). Blood examination is necessary for oral mucosal disease patients. Shandong Med. J. 56 (01), 1–2. doi:10.1016/j.jfma.2015.08.010
Sun, C., Mezzadra, R., and Schumacher, T. N. (2018). Regulation and Function of the PD-L1 Checkpoint. Immunity 48 (3), 434–452. doi:10.1016/j.immuni.2018.03.014
Sun, G. P., Wan, X., Xu, S. P., Wang, H., Liu, S. H., and Wang, Z. G. (2008). Antiproliferation and apoptosis induction of paeonol in human esophageal cancer cell lines. Dis. Esophagus 21 (8), 723–729. doi:10.1111/j.1442-2050.2008.00840.x
Sun, G. P., Wang, H., Xu, S. P., Shen, Y. X., Wu, Q., Chen, Z. D., et al. (2008). Anti-tumor effects of paeonol in a HepA-hepatoma bearing mouse model via induction of tumor cell apoptosis and stimulation of IL-2 and TNF-alpha production. Eur. J. Pharmacol. 584 (2-3), 246–252. doi:10.1016/j.ejphar.2008.02.016
Sun, X., Han, Z., Feng, W., Ma, W., Zhang, W., Xie, M., et al. (2020). Comparison of short-term outcomes following minimally invasive versus open Sweet esophagectomy for Siewert type II adenocarcinoma of the esophagogastric junction. Chin. J. Pathophysiol. 36 (08), 1487–1494. doi:10.1111/1759-7714.13415
Suski, J. M., Braun, M., Strmiska, V., and Sicinski, P. (2021). Targeting cell-cycle machinery in cancer. Cancer Cell 39 (6), 759–778. doi:10.1016/j.ccell.2021.03.010
Tan, Z., Xue, H., Sun, Y., Zhang, C., Song, Y., and Qi, Y. (2021). The role of tumor inflammatory microenvironment in lung cancer. Front. Pharmacol. 12, 688625. doi:10.3389/fphar.2021.688625
Tang, H., Chu, C., Wen, Q., and Zhang, B. (2014). Paeonol reduces vascular endothelial growth factor expression in human glioblastoma cells in vitro. Acta Med. Univ. Sci. Technol. Huazhong 43 (05), 537–540+549. (in Chinese)
Tao, Y., Zhang, M., Ma, F., and Sun, J. (2014). Effects of paeonol on the proliferation and apoptosis in human melan cell line in A375 and M14. China J. Lepr. Skin Dis. 30 (09), 519–523. (in Chinese)
Thabassum Akhtar Iqbal, S., Tirupathi Pichiah, P. B., Raja, S., and Arunachalam, S. (2020). Paeonol reverses adriamycin induced cardiac pathological remodeling through Notch1 signaling reactivation in H9c2 cells and adult zebrafish heart. Chem. Res. Toxicol. 33 (2), 312–323. doi:10.1021/acs.chemrestox.9b00093
Thatishetty, A. V., Agresti, N., and O'Brien, C. B. (2013). Chemotherapy-induced hepatotoxicity. Clin. Liver Dis. 17 (4), 671–686. ix-x. doi:10.1016/j.cld.2013.07.010
Tian, H., Shi, H., Yu, J., Ge, S., and Ruan, J. (2022). Biophysics role and biomimetic culture systems of ECM stiffness in cancer EMT. Glob. Chall. 6 (6), 2100094. doi:10.1002/gch2.202100094
Torre, L. A., Siegel, R. L., Ward, E. M., and Jemal, A. (2016). Global cancer incidence and mortality rates and trends--an update. Cancer Epidemiol. Biomarkers Prev. 25 (1), 16–27. doi:10.1158/1055-9965.Epi-15-0578
Tsai, C. Y., Kapoor, M., Huang, Y. P., Lin, H. H., Liang, Y. C., Lin, Y. L., et al. (2016). Synthesis and evaluation of aminothiazole-paeonol derivatives as potential anticancer agents. Molecules 21 (2), 145. doi:10.3390/molecules21020145
Varga, Z. V., Ferdinandy, P., Liaudet, L., and Pacher, P. (2015). Drug-induced mitochondrial dysfunction and cardiotoxicity. Am J Physiol Heart Circ Physiol 309 (9), H1453–1467. doi:10.1152/ajpheart.00554.2015
Wan, X. A., Sun, G. P., Wang, H., Xu, S. P., Wang, Z. G., and Liu, S. H. (2008). Synergistic effect of paeonol and cisplatin on oesophageal cancer cell lines. Dig. Liver Dis. 40 (7), 531–539. doi:10.1016/j.dld.2008.01.012
Wang, X. J., Liu, J. L., and Wang, J. K. (2012). Synthesis and anti-tumor activity of paeonol and its derivatives. Yao Xue Xue Bao 47 (1), 72–76. (in Chinese)
White, M. A., Fong, Y., and Singh, G. (2016). Chemotherapy-associated hepatotoxicities. Surg. Clin. North Am. 96 (2), 207–217. doi:10.1016/j.suc.2015.11.005
Wong, R. S. (2011). Apoptosis in cancer: From pathogenesis to treatment. J. Exp. Clin. Cancer Res. 30 (1), 87. doi:10.1186/1756-9966-30-87
Wu, J., Sun, C., Wang, R., Li, J., Zhou, M., Yan, M., et al. (2018). Cardioprotective effect of paeonol against epirubicin-induced heart injury via regulating miR-1 and PI3K/AKT pathway. Chem. Biol. Interact. 286, 17–25. doi:10.1016/j.cbi.2018.02.035
Wu, J., Xu, L., Sun, C., Zhang, B., Li, J., Sun, J., et al. (2017). Paeonol alleviates epirubicin-induced renal injury in mice by regulating Nrf2 and NF-κB pathways. Eur. J. Pharmacol. 795, 84–93. doi:10.1016/j.ejphar.2016.12.005
Wu, J., Xue, X., Zhang, B., Cao, H., Kong, F., Jiang, W., et al. (2016a). Enhanced antitumor activity and attenuated cardiotoxicity of Epirubicin combined with Paeonol against breast cancer. Tumour Biol. 37 (9), 12301–12313. doi:10.1007/s13277-016-5088-9
Wu, J., Xue, X., Zhang, B., Jiang, W., Cao, H., Wang, R., et al. (2016b). The protective effects of paeonol against epirubicin-induced hepatotoxicity in 4T1-tumor bearing mice via inhibition of the PI3K/Akt/NF-kB pathway. Chem. Biol. Interact. 244, 1–8. doi:10.1016/j.cbi.2015.11.025
Wu, S., Quan, X., Sun, G., and Jin, W. (2014). Mechanism of radiosensitization effect of paeonol on eca-109 in vitro. Acta Univ. Med. Anhui 49 (10), 1418–1422. doi:10.19405/j.cnki.issn1000-1492.2014.10.016 (in Chinese)
Wu, X., Chen, H., Chen, X., and Hu, Z. (2003). Determination of paeonol in rat plasma by high-performance liquid chromatography and its application to pharmacokinetic studies following oral administration of Moutan cortex decoction. Biomed. Chromatogr. 17 (8), 504–508. doi:10.1002/bmc.259
Xie, Y., Zhou, H., Wong, Y. F., Xu, H. X., Jiang, Z. H., and Liu, L. (2008). Study on the pharmacokinetics and metabolism of paeonol in rats treated with pure paeonol and an herbal preparation containing paeonol by using HPLC-DAD-MS method. J. Pharm. Biomed. Anal. 46 (4), 748–756. doi:10.1016/j.jpba.2007.11.046
Xu, Y., Zhu, J. Y., Lei, Z. M., Wan, L. J., Zhu, X. W., Ye, F., et al. (2017). Anti-proliferative effects of paeonol on human prostate cancer cell lines DU145 and PC-3. J. Physiol. Biochem. 73 (2), 157–165. doi:10.1007/s13105-016-0537-x
Yang, J., Lin, Y., Zheng, X., and Cheng, M. (2022). Paeonol inhibits the invasion,metastasis and proliferation of Hela cells by down-regulating miR-21 and inhibiting PI3K/AKT pathway. J. Mod. Oncol. 30 (14), 2508–2515. (in Chinese)
Yang, L. C., Xia, Y. Q., Zhang, M., Zhu, M. M., Feng, L., and Jia, X. B. (2020). Practice and development of structural characteristics and quality control of multi-dimensional structure of basic components of genuine material of Moutan Cortex. Zhongguo Zhong Yao Za Zhi 45 (14), 3340–3350. doi:10.19540/j.cnki.cjcmm.20200622.301
Yang, Y., and Cao, Y. (2022). The impact of VEGF on cancer metastasis and systemic disease. Semin. Cancer Biol. 86 (3), 251–261. doi:10.1016/j.semcancer.2022.03.011
Yazbeck, V., Alesi, E., Myers, J., Hackney, M. H., Cuttino, L., and Gewirtz, D. A. (2022). An overview of chemotoxicity and radiation toxicity in cancer therapy. Adv. Cancer Res. 155, 1–27. doi:10.1016/bs.acr.2022.03.007
Ye, J. M., Deng, T., and Zhang, J. B. (2009). Influence of paeonol on expression of COX-2 and p27 in HT-29 cells. World J. Gastroenterol. 15 (35), 4410–4414. doi:10.3748/wjg.15.4410 (in Chinese)
Yin, J., Wu, N., Zeng, F., Cheng, C., Kang, K., and Yang, H. (2013). Paeonol induces apoptosis in human ovarian cancer cells. Acta histochem. 115 (8), 835–839. doi:10.1016/j.acthis.2013.04.004
Yun, C. W., and Lee, S. H. (2018). The roles of autophagy in cancer. Int. J. Mol. Sci. 19 (11), 3466. doi:10.3390/ijms19113466
Zhang, C., Hu, S., Li, Y., and Cao, M. (2006). Anti-tumor effect and mechanism of Paeonol on the hepatocellular carcinoma cell line Bel-7404. J. Central South Univ. Sci. 31 (05), 682–695. (in Chinese)
Zhang, J. J., Cai, L. J., Pang, K., Dong, Y., Zhang, Z. G., Li, B. B., et al. (2021a). Paeonol inhibits proliferation and induces cell apoptosis of human T24 and 5637 bladder cancer cells in vitro and in vivo. Clin. Transl. Oncol. 23 (3), 601–611. doi:10.1007/s12094-020-02455-y
Zhang, L., Chen, W. X., Li, L. L., Cao, Y. Z., Geng, Y. D., Feng, X. J., et al. (2020a). Paeonol suppresses proliferation and motility of non-small-cell lung cancer cells by disrupting STAT3/NF-κB signaling. Front. Pharmacol. 11, 572616. doi:10.3389/fphar.2020.572616
Zhang, L., Li, D. C., and Liu, L. F. (2019). Paeonol: Pharmacological effects and mechanisms of action. Int. Immunopharmacol. 72, 413–421. doi:10.1016/j.intimp.2019.04.033
Zhang, L., Tao, L., Shi, T., Zhang, F., Sheng, X., Cao, Y., et al. (2015a). Paeonol inhibits B16F10 melanoma metastasis in vitro and in vivo via disrupting proinflammatory cytokines-mediated NF-κB and STAT3 pathways. IUBMB Life 67 (10), 778–788. doi:10.1002/iub.1435
Zhang, W., Cai, J., Chen, S., Zheng, X., Hu, S., Dong, W., et al. (2015b). Paclitaxel resistance in MCF-7/PTX cells is reversed by paeonol through suppression of the SET/phosphatidylinositol 3-kinase/Akt pathway. Mol. Med. Rep. 12 (1), 1506–1514. doi:10.3892/mmr.2015.3468
Zhang, W., Liu, C., Li, J., Lu, Y., Li, H., Zhuang, J., et al. (2022). Tanshinone IIA: New perspective on the anti-tumor mechanism of A traditional natural medicine. Am. J. Chin. Med. 50 (1), 209–239. doi:10.1142/s0192415x22500070
Zhang, Y., Gao, D., Yi, W., and Zhao, R. (2021c). The effect of paeonol on the proliferation and apoptosis of leukemia cells by down-regulation miR-106a-5p/PENT. Curr. Immunol. 41 (01), 14–23. (in Chinese)
Zhang, Y., and Zhang, Z. (2020). The history and advances in cancer immunotherapy: understanding the characteristics of tumor-infiltrating immune cells and their therapeutic implications. Cell Mol Immunol 17 (8), 807–821. doi:10.1038/s41423-020-0488-6
Zhou, H. M., Sun, Q. X., and Cheng, Y. (2017). Paeonol enhances the sensitivity of human ovarian cancer cells to radiotherapy-induced apoptosis due to downregulation of the phosphatidylinositol-3-kinase/Akt/phosphatase and tensin homolog pathway and inhibition of vascular endothelial growth factor. Exp. Ther. Med. 14 (4), 3213–3220. doi:10.3892/etm.2017.4877
Zhou, J., Liu, Q., Qian, R., Liu, S., Hu, W., and Liu, Z. (2020). Paeonol antagonizes oncogenesis of osteosarcoma by inhibiting the function of TLR4/MAPK/NF-κB pathway. Acta histochem. 122 (1), 151455. doi:10.1016/j.acthis.2019.151455
Glossary
Keywords: paeonol, cancer, side effects, mechanism, pharmacological effects
Citation: Chang X, Feng X, Du M, Li S, Wang J, Wang Y and Liu P (2023) Pharmacological effects and mechanisms of paeonol on antitumor and prevention of side effects of cancer therapy. Front. Pharmacol. 14:1194861. doi: 10.3389/fphar.2023.1194861
Received: 27 March 2023; Accepted: 08 June 2023;
Published: 20 June 2023.
Edited by:
Defang Li, Binzhou Medical University, ChinaReviewed by:
Imran Shair Mohammad, University of North Carolina at Chapel Hill, United StatesKun Pang, Xuzhou Central Hospital, China
Copyright © 2023 Chang, Feng, Du, Li, Wang, Wang and Liu. This is an open-access article distributed under the terms of the Creative Commons Attribution License (CC BY). The use, distribution or reproduction in other forums is permitted, provided the original author(s) and the copyright owner(s) are credited and that the original publication in this journal is cited, in accordance with accepted academic practice. No use, distribution or reproduction is permitted which does not comply with these terms.
*Correspondence: Ping Liu, liuping0207@yeah.net