- Ministry of Education Key Laboratory of Molecular and Cellular Biology, Hebei Key Laboratory of Animal Physiology, Biochemistry, and Molecular Biology, College of Life Sciences, Hebei Normal University, Shijiazhuang, Hebei, China
Oxidative stress is caused by an imbalance in oxidant/antioxidant processes and is a critical process in pulmonary diseases. As no truly effective therapies exist for lung cancer, lung fibrosis and chronic obstructive pulmonary disease (COPD), at present, it is important to comprehensively study the relationship between oxidative stress and pulmonary diseases to identify truly effective therapeutics. Since there is no quantitative and qualitative bibliometric analysis of the literature in this area, this review provides an in-depth analysis of publications related to oxidative stress and pulmonary diseases over four periods, including from 1953 to 2007, 2008 to 2012, 2013 to 2017, and 2018 to 2022. Interest in many pulmonary diseases has increased, and the mechanisms and therapeutic drugs for pulmonary diseases have been well analyzed. Lung injury, lung cancer, asthma, COPD and pneumonia are the 5 most studied pulmonary diseases related to oxidative stress. Inflammation, apoptosis, nuclear factor erythroid 2 like 2 (NRF2), mitochondria, and nuclear factor-κB (NF-κB) are rapidly becoming the most commonly used top keywords. The top thirty medicines most studied for treating different pulmonary diseases were summarized. Antioxidants, especially those targeting reactive oxygen species (ROS) in specific organelles and certain diseases, may be a substantial and necessary choice in combined therapies rather than acting as a single “magic bullet” for the effective treatment of refractory pulmonary diseases.
Introduction
Oxidative stress is an upstream event leading to multiple diseases, including cancer, cardiovascular diseases, neuron degenerative diseases and pulmonary diseases (Klaunig, 2018; van der Vliet et al., 2018; Singh et al., 2019; Steven et al., 2019). In organisms, the production of free radicals, including reactive oxygen species (ROS) and reactive nitrogen species (RNS), is mediated by extrinsic factors (drugs, radiation, inorganic particles, and tobacco smoking (Tostes et al., 2008; Helmig et al., 2018; Owusu et al., 2020; Uchiyama et al., 2020)) or endogenous sources (mitochondria, oxidative enzymes, and peroxisomes) (Dan Dunn et al., 2015; Forrester et al., 2018). ROS include superoxide radical anions (O2−), hydroxyl radicals (OH), hydrogen peroxide (H2O2) and hypohalite radicals (HOO.). NO is the main RNS synthesized by numerous cells and is central to the formation of other RNS (Martinez and Andriantsitohaina, 2009). The antioxidant system, including antioxidative enzymes (such as SOD, catalase, glutathione peroxidase and glutathione S-transferase) (Margis et al., 2008; Weydert and Cullen, 2009; Chatterjee and Gupta, 2018) and nonenzymatic antioxidants (such as N-acetylcysteine, glutathione, and vitamin E) (Schmitt et al., 2015; Lee and Han, 2018), helps to remove excessive free radicals by acting on them directly or indirectly by consuming substances that produce free radicals. Excess free radicals can cause oxidative stress if their levels exceed the antioxidation limits both in vivo and in vitro (Pizzino et al., 2017).
Pulmonary diseases are one of the main factors contributing to the morbidity and mortality of older individuals, who experience these diseases at a higher prevalence. Due to smoking, second-hand smoke or air pollution, the occurrence of pulmonary diseases in young persons is increasing annually. Unfortunately, no truly effective interventions are currently available for some lung diseases, such as lung cancer, chronic obstructive pulmonary disease (COPD), and lung fibrosis. Therefore, it is important to elucidate their mechanisms to identify better therapeutic strategies to cure patients with these diseases.
Most pathogenicity factors, including viral and bacterial infections, air pollution, occupational exposure, smoking, and aging, contribute to pulmonary diseases through oxidative stress (Cheresh et al., 2013). For instance, exposure to excess fine particulate matter, respirable fibers, inhalable quartz and metal powders, ozone, and vehicle exhaust from the environment causes oxidative stress, which initiates pulmonary inflammation, carcinogenesis, and fibrosis (Valavanidis et al., 2013; Han et al., 2023). Oxidative stress induced by cigarette smoke leads to mitochondrial dysfunction of human bronchial epithelial cells, increased risk of emphysema through upregulation of MMP-9 activity, and EGFR activation, which results in the decrease of lung functions and hypersecretory diseases of the airways (Malinska et al., 2018; Muratani et al., 2023; Staitieh et al., 2023). This study aims to provide the first in-depth quantitative and qualitative bibliometric analysis of publications related to oxidative stress and pulmonary disease over time from 1953 to 2022 and to identify the major contributors, emerging mainstream research themes, and therapeutic drugs. The authors, institutions, countries, and journals with the highest output for each period were analyzed; the keywords with occurrences in the titles and abstracts of most publications were identified and provide important information on the major mechanisms underlying the function of oxidative stress in pulmonary diseases. Furthermore, the developing trend of publications in major research categories and annual publications on major pulmonary diseases were analyzed. Moreover, potential therapeutic drugs are summarized. Hopefully, by presenting a comprehensive view of recent research accomplishments on the relationship between oxidative stress and pulmonary diseases, this paper provides novel insights into the development of truly effective therapies for refractory pulmonary diseases.
Materials and methods
An advanced search in the Web of Science (WOS) electronic databases was performed by “Topic search” to obtain the whole body of publications concerning both oxidative stress and pulmonary diseases (33 kinds in total) from 1953 to 2022 or four separate time periods, including from 1953 to 2007, 2008 to 2012, 2013 to 2017 and 2018 to 2022. The retrieval pattern was as follows: TS = (“Oxidative Stress*”) AND TS=(“corpulmonale” OR “lung cancer” OR “lung carcinoma” OR “pulmonary carcinoma” OR “carcinoma of lung*” OR “carcinoma of the lung*” OR “pulmonary sarcoidosis” OR “lung sarcoidosis” OR “pulmonary artery hypertension” OR “pulmonary arterial hypertension” OR “pulmonary hypertension” OR “lung artery hypertension” OR “lung arterial hypertension” OR “lung hypertension” OR “pulmonary embolism” OR “lung embolism” OR “pulmonary thromboembolism” OR “lung thromboembolism” OR “COPD” OR “chronic obstructive pulmonary disease*” OR “lung infection*” OR “pulmonary infection*” OR “infectious lung disease*” OR “infectious pulmonary disease*” OR “pulmonary mycosis” OR “lung mycosis” OR “pulmonary candidiasis” OR “lung candidiasis” OR “pulmonary aspergillosis” OR “lung aspergillosis” OR “pulmonary cryptococcosis” OR “lung cryptococcosis” OR “pulmonary tuberculosis” OR “lung tuberculosis” OR “pneumonia” OR “pulmonary abscess” OR “lung abscess” OR “bronchitis” OR “acute tracheobronchitis*” OR “alveolitis” OR “asthma” OR “bronchiectasis” OR “emphysema” OR “pulmonary bullae” OR “lung bullae” OR “pneumothorax” OR “pleural effusion” OR “hypoxemia” OR “pulmonary lymphangioleiomyomatosis” OR “lung lymphangioleiomyomatosis” OR “pulmonary edema” OR “lung edema” OR “alveolar proteinosis” OR “lung injury*” OR “pulmonary injury*” OR “dyspnea” OR “ventilation dysfunction” OR “acute respiratory distress syndrome*” OR “respiratory failure” OR “sleep apnea” OR “pulmonary fibrosis” OR “pulmonary fibrogene*” OR “lung fibrosis” OR “lung fibrogene*” OR “Cystic fibrosis” OR “idiopathic pulmonary fibrosis” OR “pneumoconiosis” OR “BOS” OR “bronchiolitis obliterans syndrome*” OR “lung disease*” OR “pulmonary disease*”). After the retrieval results were shown, by clicking on “Create Citation Report” and then “Analyze”, the top productive authors, institutions, countries/regions, and journals were displayed.
The keywords over four periods from 1953 to 2007, 2008 to 2012, 2013 to 2017 and 2018 to 2022 were obtained by importing all the publications on oxidative stress and pulmonary diseases during the corresponding period from WOS electronic databases into VOSviewer1.6.18, and the most frequent 25 keywords during each period were identified.
Bubble diagrams were generated by importing the publications on oxidative stress and pulmonary diseases during different time periods into VOSviewer1.6.18 for all keyword analyses, and those appearing in at least 2% of publications were included in the bubble diagrams.
The numbers of publications related to iron, mitochondria, or inflammation in four time periods were obtained by adding the retrieval conditions of TS = (“inflammat*”), TS = (“iron” or “Ferroptosis”), or TS = (“mitochondria” or “mitochondrial” or “mitochondrion”), respectively, to the previous retrieval patterns in the advanced search through the WOS electronic databases.
The publication number on each kind of pulmonary disease related to oxidative stress was obtained through the advanced search in the WOS electronic databases by using the retrieval pattern of TS = (“Oxidative Stress*”) and TS = (“the corresponding disease”).
After importing all the publications on oxidative stress and pulmonary diseases into VOSviewer1.6.18, the drugs that appeared as keywords in more than 20 publications in VOSviewer1.6.18 were selected and ordered by their publication numbers. The numbers of publications on the top thirty drugs on eight common pulmonary diseases were analyzed in VOSviewer1.6.18 by the retrievals of TS = (“Oxidative Stress*") and TS = (“the corresponding disease”) through the advanced search in the WOS electronic databases.
Results
Publications on oxidative stress and pulmonary diseases from each time period were analyzed
First, we searched all publications concerning both oxidative stress and lung diseases from 1953 to 2022 to analyze the roles of oxidative stress in pulmonary diseases. As a result, a total of 31,373 publications from 1953 to 2022 were obtained. Among them, the top twenty authors, institutions, countries/regions, and journals with the highest productivity were retrieved, and this information is shown in Table 1. In the retrieval record, the first names and middle names of authors were initialized by WOS; therefore, some of the author’s name abbreviations may represent multiple author identities. It was found that the most prolific author name “Wang Y” by the original search data corresponds to multiple author identities, such as “Wang Yan”, “Wang Ye” and “Wang Yong”. By excluding the names corresponding to multiple identities, we found that the most prolific author was BARNES PJ in the National Heart, Lung, and Blood Institute, University of London, United Kingdom [n = 179/0.57%, citation per publication (CPP) = 130.56]. Table 1 shows that the most productive institution, countries/region, and journal are the University of California System (n = 716/2.28%, CPP = 59.48), the United States (n = 8,800/28.04%, CPP = 56.02), and Free Radical Biology and Medicine (n = 571/1.82%, CPP = 56.91).
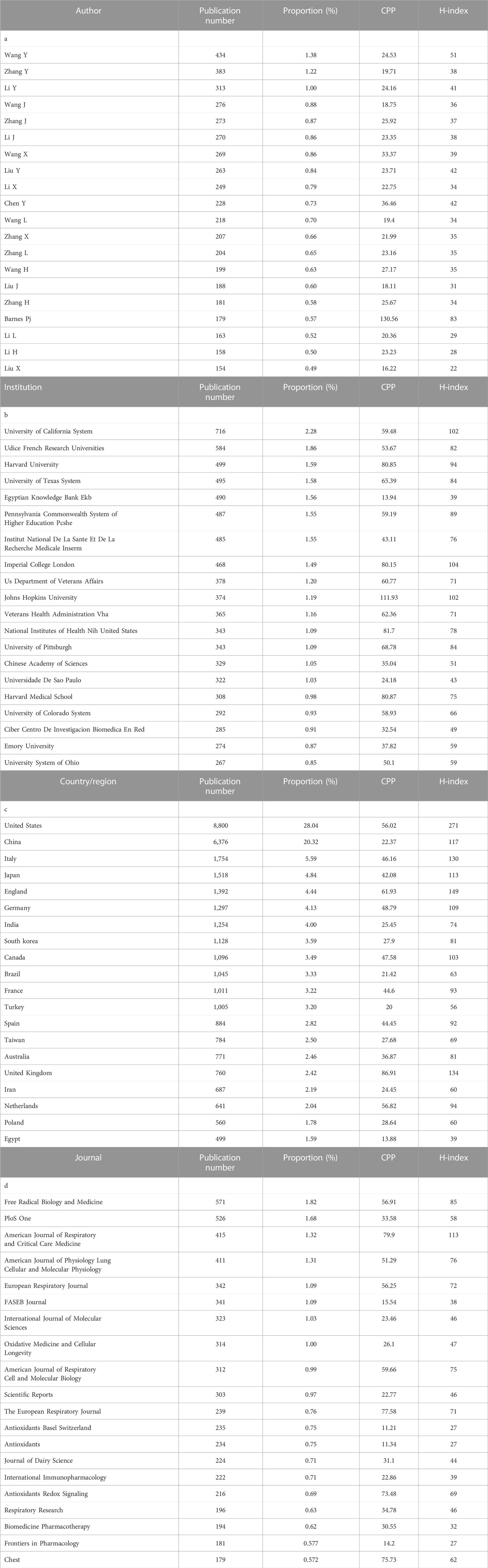
TABLE 1. The top 20 authors, institutions, countries/regions, and journals with the highest productivity on publications on oxidative stress and pulmonary diseases from 1953 to 2022. CPP, citation per publication.
Among the 31,373 retrieved publications, 29,619 (94.41%) were articles, and 30,738 (97.98%) were written in English. The annual count of these publications shown in Figure 1 indicates that the annual publications in 2009 exceeded 1,000 for the first time. To show the developing trend of the vast amount of research from 1953 to 2022, we separated these 31,373 publications into four groups based on the publication time periods from 1953 to 2007, 2008 to 2012, 2013 to 2017 and 2018 to 2022, which include 4,254, 5,673, 8,471, and 12,975 publications, respectively. Then, we reanalyzed these publications, and the number of papers, English publications, and average citations per publication; the most referenced publication and the top prolific author; and the top five institutions, countries/regions, and journals with the highest productivity are summarized in Table 2.
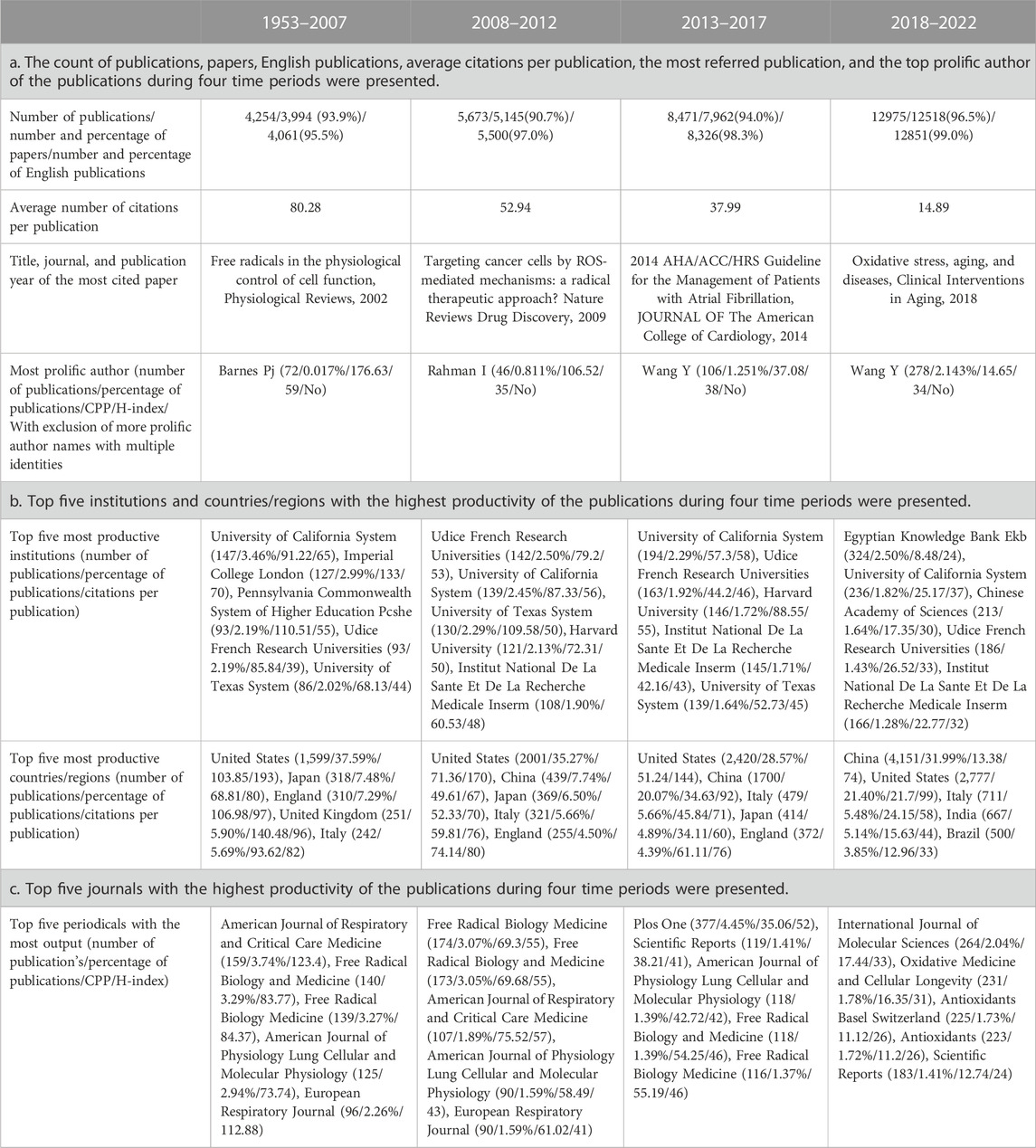
TABLE 2. The information of the publications on oxidative stress and pulmonary diseases during four time periods were shown. CPP, citation per publication.
Top keywords with the most occurrences in the publications provided main mechanisms underlying the effects of oxidative stress on pulmonary diseases
To identify the important molecular events regarding oxidative stress in pulmonary diseases, we searched for the keywords by importing the publications into VOSviewer1.6.18. The most frequent 25 keywords in the publications over five periods from 1953 to 2022, 1953 to 2007, 2008 to 2012, 2013 to 2017 and 2018 to 2022 are indicated in Table 3. Most of the 25 keywords can be sorted into 3 categories. The first category includes oxidative stress or molecules that are closely related, such as oxidative damage, ROS, lipid peroxidation, SOD, MDA, glutathione, glutathione peroxidase, nitric oxide, and catalase. The second category comprises lung diseases or factors that are closely connected, including COPD, lung injury, asthma, lung cancer, obstructive sleep apnea, cystic fibrosis, hypertension, cigarette smoke, particle, lipopolysaccharide (LPS), biomarker, bronchoalveolar lavage fluid, and age. The third category includes molecules, organelles, or cell functions that play a role in both oxidative stress and pulmonary diseases, including NRF2 (nuclear factor, erythroid 2 like 2), nuclear factor-κB (NF-κB), TNF-alpha, cytokines, DNA damage, macrophages, heme oxygenase, endothelial dysfunction, inflammation, apoptosis, cell death, and hypoxia. Among the top 25 keywords of all four time periods, ROS, COPD, asthma, lung injury, cancer, biomarker, smoke, inflammation, apoptosis, caspase, catalase, glutathione, MDA, SOD, and TNF-alpha appeared during at least three periods. In a comparison of the top 25 keywords during different time periods, NRF2, NF-κB, and LPS first arose from 2013 to 2017 and have been reported after, indicating that research on these three terms involves a comparatively novel mechanism, new interests or reagents used by scientists conducting research on oxidative stress and pulmonary diseases starting in 2013.
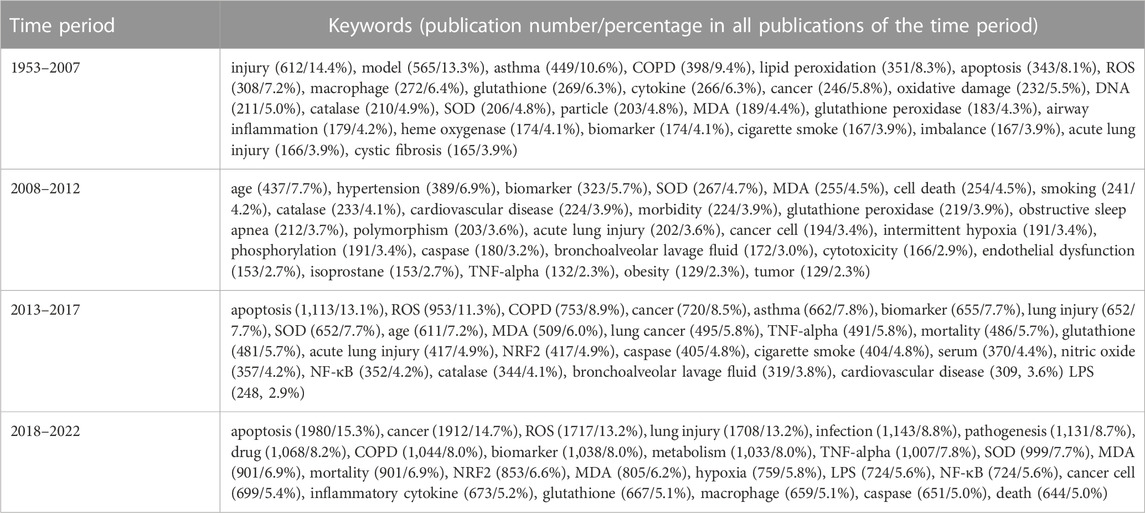
TABLE 3. The 25 most frequent keywords with publication number and the proportion of the publications on oxidative stress and pulmonary diseases in the corresponding time period.
The developing trend of publications in major research categories was demonstrated
VOSviewer identified 258 keywords that appeared in more than 2% (n = 260) of 12,975 publications between 2018 and 2022. Among them, except for those of oxidative stress and a few lung diseases, eight keywords on mechanism emerged, which are inflammation, apoptosis, NRF2, antioxidant, hypoxia, lipid peroxidation, mitochondria, NF-κB and autophagy. To explore the trend of scientists’ research focus, we analyzed the occurrence of these keywords in the publications on oxidative stress and pulmonary diseases during different time periods. As shown in Figure 2, except for antioxidant, hypoxia, and lipid peroxidation, the occurrences of these keywords during 2018–2022 increased by more than 1.5 times compared with those in 1953–2007. The occurrence of three keywords, including inflammation, NRF2 and NF-κB, increased dramatically. Obviously, inflammation remains a focus of interest, and the latter two keywords have emerged as hot spots of pulmonary research.
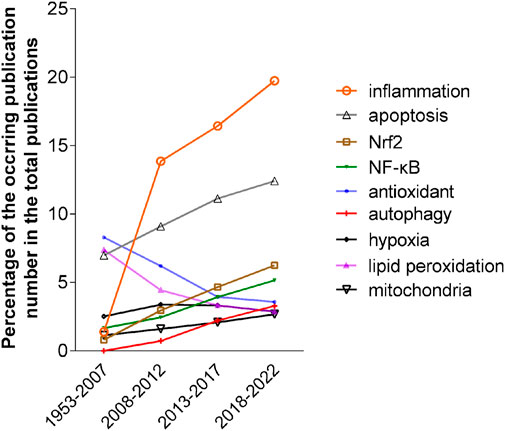
FIGURE 2. The occurrence trend of eight keywords in four periods, 1953–2007, 2008–2012, 2013–2017 and 2018–2022. The occurrences were calculated as the percentage of the number of publications of each keyword in the total number of publications in the corresponding periods.
Bubble diagrams demonstrate the frequency, connection, and citation numbers of the keywords visually during different time periods
Furthermore, to visualize the frequency and connection of the keywords (words or phrases appearing in the titles or abstracts of the publications), the publications during four periods from 1953 to 2007, 2008 to 2012, 2013 to 2017, and 2018 to 2022 were imported into VOSviewer1.6.18 for all keyword analyses. As shown in Figures 3A–D, each keyword appearing in at least 2% of publications during the period was presented as a bubble in the bubble diagrams during the four time periods. The bubble size represents the frequency of its appearance in different publications during the time period (multiple occurrences of the word or phrase in one publication do not have repeat counts). The bubble color represents the overlay score among the keywords. In the bubble diagrams in Figures 3A–D, 228, 200, 227 and 258 terms appeared in the titles or abstracts of at least 2% of the publications on oxidative stress and pulmonary diseases from 1953 to 2007, 2008 to 2012, 2013 to 2017 and 2018 to 2022, respectively.
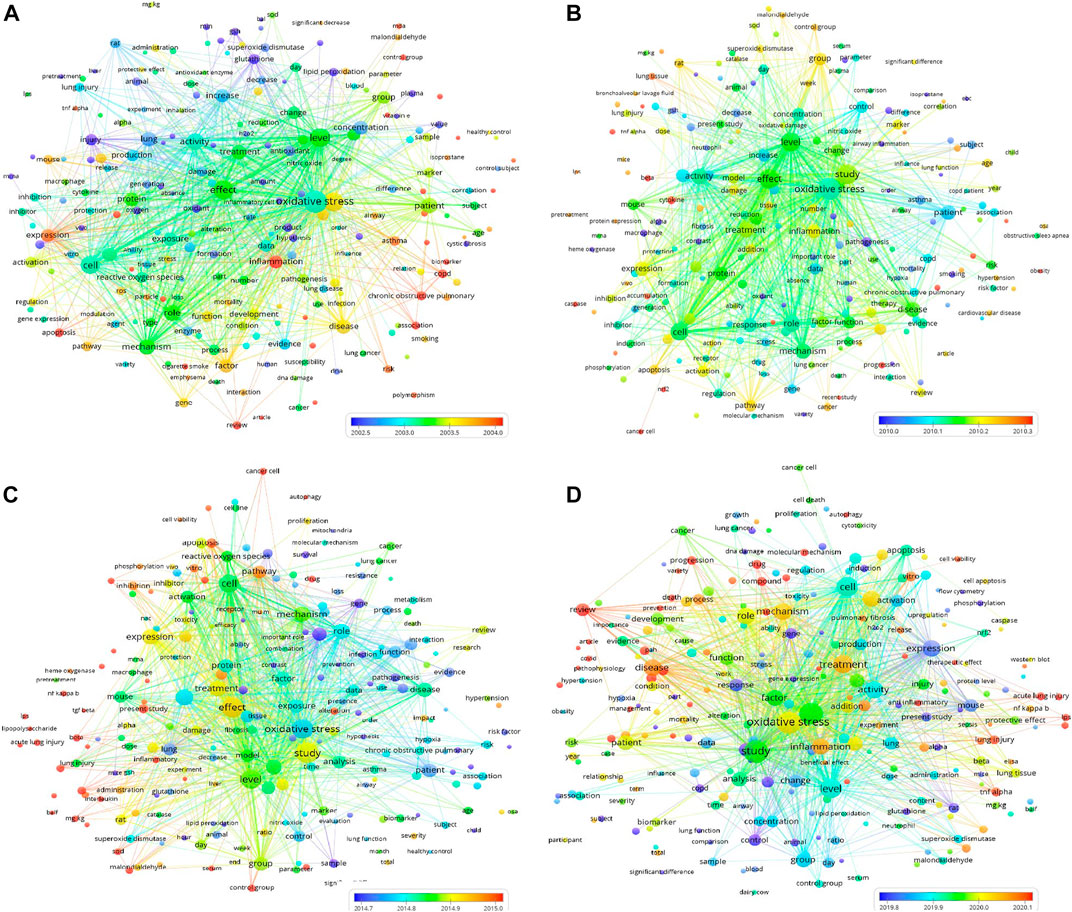
FIGURE 3. Four bubble diagrams representing the keywords appearing in the titles or abstracts of at least 2% publications on oxidative stress and lung diseases and their co-occurrence from 1953 to 2007, 2008 to 2012, 2013 to 2017, and 2018 to 2022, respectively. During the four periods, there are 228 [(A) from 1953 to 2007], 200 [(B), from 2008 to 2012], 227 [(C) from 2013 to 2017] and 258 [(D) 2018–2022] bubbles in 3 clusters, respectively. The overlay score was demonstrated by rainbow color style as indicated. The above data can be viewed and downloaded from VOSviewer1.6.18 and this bubble diagram does not show all the terms and required data.
As shown in Figures 3A–D, the top 2% keywords with the highest average citation rates during all four time periods can be sorted into several clusters. The center words of the clusters include oxidative stress, lung, disease, injury, patients, factor, study, effect, mechanism, mouse, cell, inflammation, apoptosis, level, and expression. In Figure 3D, it is noted that COVID (n = 17, 2018–2022) appeared in the titles or abstracts of at least 2% of the publications, suggesting a rapid increase in the number of publications during this period. Moreover, N-acetylcysteine (n = 107, 1953–2007) and vitamin E (n = 128, 1953–2007) appeared as keywords in at least 2% of the publications, suggesting their acknowledged therapeutic effects in some pulmonary diseases. However, no therapeutic agent appeared in at least 2% of publications during 2008–2022.
The number of publications on major pulmonary diseases is increasing annually
It is well known that excess oxidative stress can cause inflammation (Duecker et al., 2018; Wiegman et al., 2020). It has also been reported that iron homeostasis is disrupted and mitochondrial structure and function are defective in some pulmonary diseases (Ghio et al., 2003; Reid et al., 2007; Philippot et al., 2014; Rangarajan et al., 2018; Yu et al., 2018). Therefore, we analyzed the number of publications that are related to iron, mitochondria, or inflammation by adding the retrieval conditions of TS = (“inflammat*”), TS=(“iron” or “Ferroptosis”), and TS=(“mitochondria” or “mitochondrial” or “mitochondrion”), respectively, to the retrieval pattern of the previously retrieved 31,373 publications in the WOS electronic databases.
As shown in Figure 4, both the total number (1710/1953–2007, 2,419/2008–2012, 3,932/2013–2017, 6,923/2018–2022) and the proportion (40.2%/1953–2007, 42.6%/2008–2012, 46.4%/2013–2017, 53.4%/2018–2022) of publications related to inflammation showed obvious increasing trends during each time period. Similarly, publications related to mitochondria demonstrated an elevation in both the number (248/1953–2007, 479/2008–2012, 1,040/2013–2017, 1884/2018–2022) and the proportion (5.8%/1953–2007, 8.4%/2008–2012, 12.3%/2013–2017, 14.5%/2018–2022) of publications during each time period. In addition, the proportion (6.0%/1953–2007, 3.6%/2008–2012, 3.2%/2013–2017, 3.6%/2018–2022) of iron-related publications first decreased and then increased during the four periods.
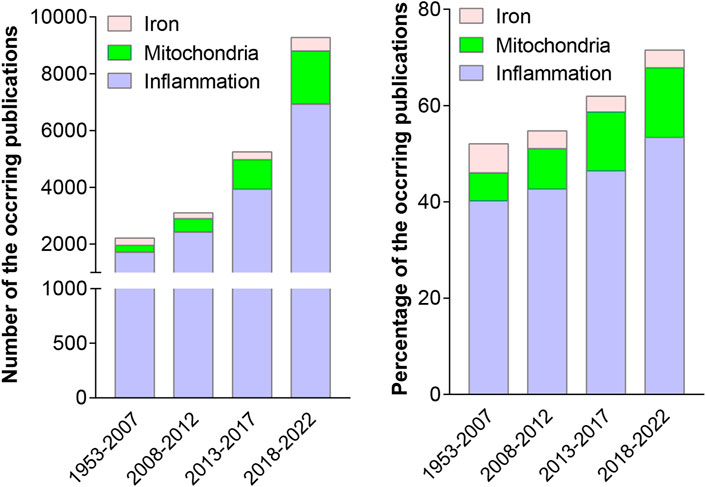
FIGURE 4. Numbers of the retrieved publications on oxidative stress and lung diseases that are related to iron, mitochondria, and inflammation (the left panel), and their percentages (the right panel) were analyzed during the four periods, from 1953 to 2007, 2008 to 2012, 2013 to 2017 and 2018 to 2022.
To explore the numbers of publications on each kind of pulmonary disease related to oxidative stress during 1953–2022, we performed another advanced search in the WOS electronic databases by using the retrieval words of TS = (“Oxidative Stress*”) AND TS = (“the corresponding disease”). As shown in Table 4, among the 33 lung diseases, the top 8 diseases with the most publications were lung injury (6,285/20.0%), lung cancer (5,725/18.2%), asthma (4,034/12.9%), COPD (3,311/10.6%), sleep apnea (2,278/7.3%), pneumonia (2,106/6.7%), pulmonary hypertension (1,606/5.1%), and emphysema (1,156/3.7%). Figure 5 shows that between 1988 and 2021, the annual number of publications regarding these pulmonary diseases has been continuously growing rapidly in general except for those on emphysema, and the number of publications on lung injury showed the fastest growing trend, followed by those on lung cancer and asthma. There was a drop in 2022, which may have been caused by shifted scientist interests to COVID-19 and the prevalence of COVID-19.
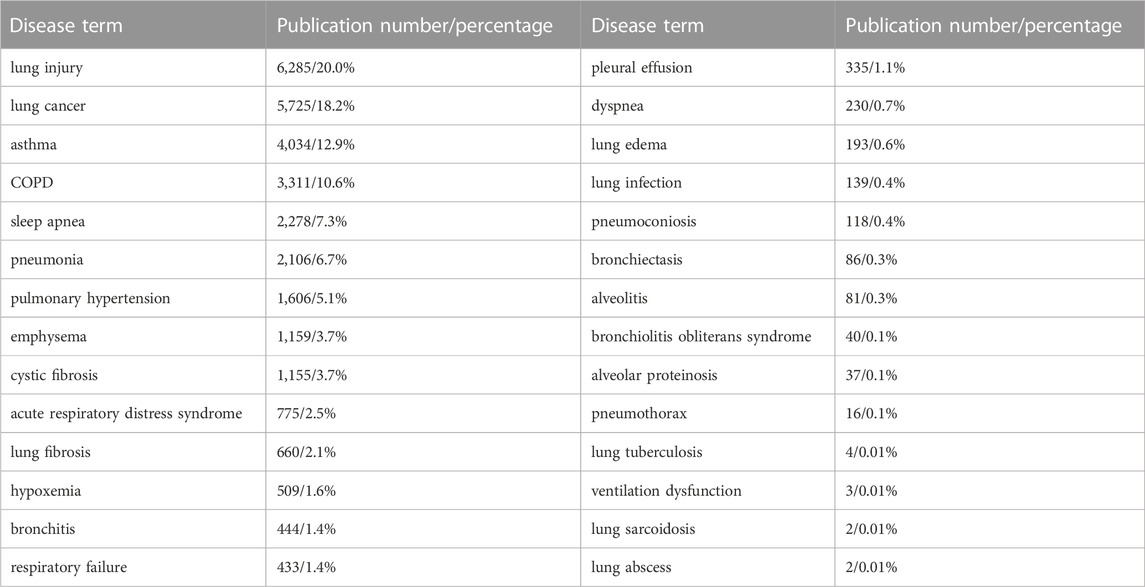
TABLE 4. The number of publications on oxidative stress and different categories of lung diseases from 1953 to 2022.
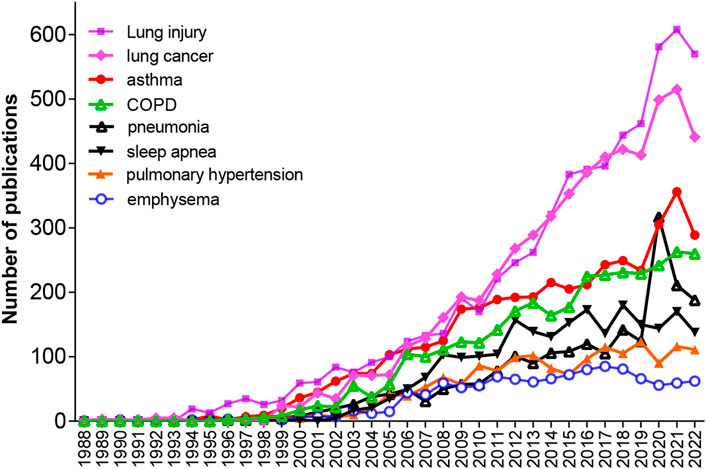
FIGURE 5. Annual publications on eight pulmonary diseases, including lung injury, lung cancer, asthma, COPD, pneumonia, sleep apnea, pulmonary hypertension and emphysema, respectively, from 1953 to 2022.
Potential therapeutic drugs for pulmonary diseases are summarized
By keyword analysis of the 31,373 publications on oxidative stress and pulmonary diseases between 1953 and 2022, the top 30 most frequent drugs are shown in Table 5. The top 10 medicines included N-acetylcysteine (n = 458/1.46%), vitamin E (n = 382/1.22%), vitamin C (n = 303/0.97%), dexamethasone (n = 225/0.72%), curcumin (n = 216/0.69%), β-carotene (n = 180/0.57%), polyphenols (n = 149/0.47%), carotenoid (n = 118/0.38%), vitamin D (n = 100/0.32%) and sulforaphane (n = 89/0.28%), indicating their close associations with oxidative stress and their potential use as therapeutics for pulmonary diseases. Furthermore, we analyzed the number of publications on each of the top eight pulmonary diseases (Table 5). The appearance of N-acetylcysteine had the most publications related to COPD (n = 81/2.45%), followed by lung cancer (n = 62/1.08%) and lung injury (n = 53/0.84%). Vitamin E appeared most in publications related to asthma (n = 66/1.64%), followed by lung cancer (n = 64/1.12%) and lung injury (n = 22/0.35%). Vitamin C has the most appearances in publications related to lung cancer (n = 70/1.22%), followed by asthma (n = 50/1.24%) and pneumonia (n = 30/1.43%). Dexamethasone appeared most in publications related to asthma (n = 100/2.48%). Notably, N-acetylcysteine appeared the most frequently in publications on lung injury, COPD, pneumonia, sleep apnea and emphysema; beta-carotene, dexamethasone, and sildenafil appeared the most frequently in publications on lung cancer, asthma and lung hypertension, respectively.
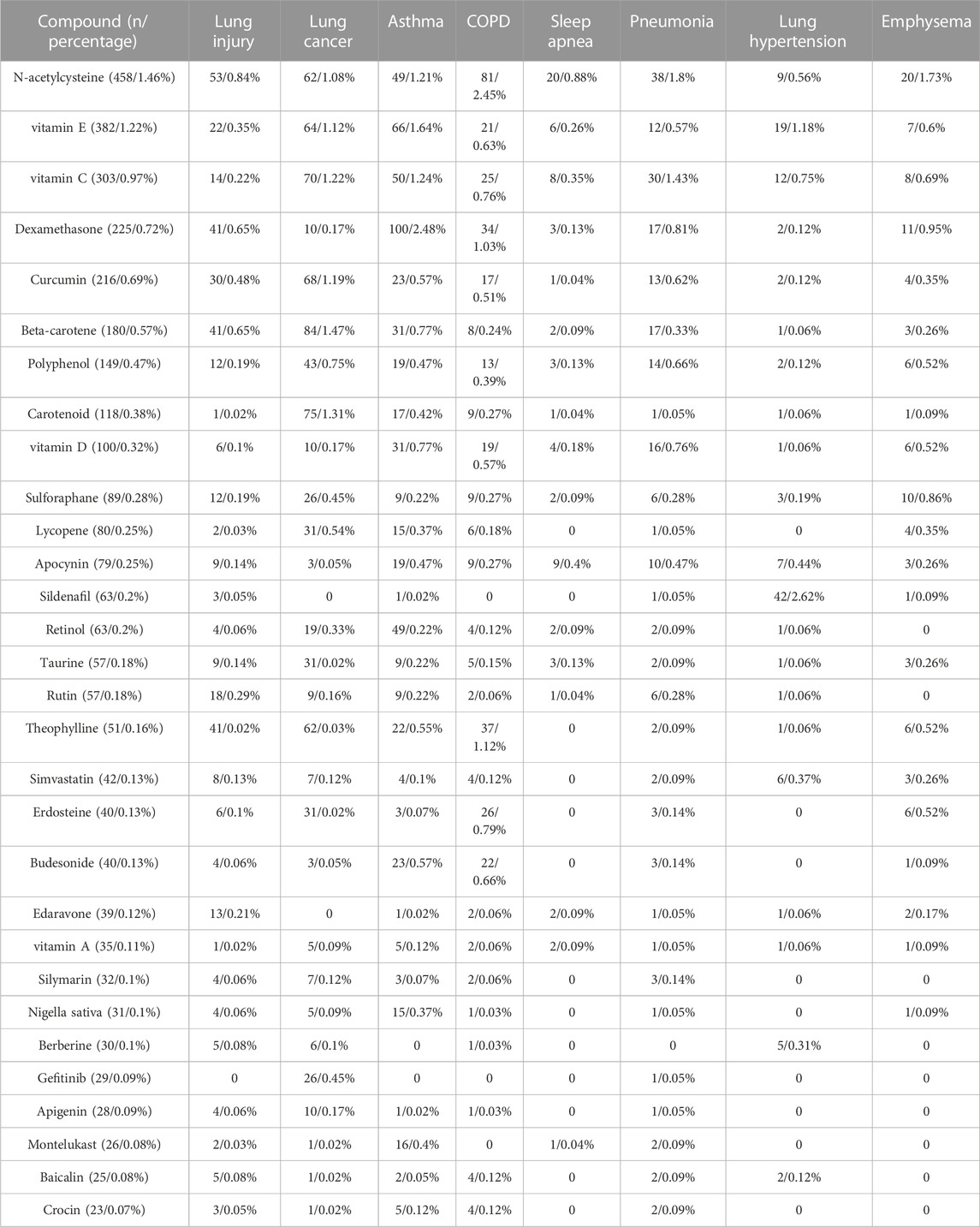
TABLE 5. The top thirty drugs appearing most in 31,373 publications on oxidative stress and pulmonary diseases as keywords from 1953 to 2022 were presented, and the number of their occurring publications and percentages on oxidative stress and each of the eight lung diseases were shown, respectively. Eight pulmonary diseases include lung injury, lung cancer, asthma, COPD, sleep apnea, pneumonia, pulmonary hypertension and emphysema.
Discussion
Oxidative stress has been reported to play a critical role in the pathology of multiple pulmonary diseases (Cheresh et al., 2013; Kirkham and Barnes, 2013; van der Vliet et al., 2018). Since 1983, the number of publications on oxidative stress and pulmonary diseases have continued to increase in general, and the cumulative number of publications is 31,373 until 2022. Unfortunately, quantitative and qualitative bibliometric analyses of the literature on oxidative stress and pulmonary diseases are lacking. This review presented a comprehensive and quantitative analysis of all the literature on oxidative stress and pulmonary disease. The United states, China, Italy, Japan, and England are the top five countries with the most publications from 1953 to 2022. The information published during four separate periods, 1953–2007, 2008–2012, 2013–2017, and 2018–2022, was also shown to demonstrate the developing trend. The increasing trend in the annual number of publications from 1983 to 2022, in addition to the large volume, demonstrates the close relationship between oxidative stress and pulmonary diseases, which is leading to growing scientific interest.
The common symptoms of lung diseases are cough, sputum, hemoptysis, and dyspnea. The causes of lung diseases vary and include air pollution, smoking, and viral and bacterial infections, which trigger or are closely related to oxidative stress in the lung. Notably, electronic cigarettes and heated tobacco products also showed harmful effects, including impaired pulmonary function and airway remodeling, and increased risk of cardiac and neurological diseases (Bahl et al., 2012; Lippi et al., 2014; Bhatt et al., 2020; Gu et al., 2023). As the lung is in direct contact with ultrafine particles in the air, it is challenging and critical to maintain redox homeostasis in the lung. In this study, it was demonstrated that oxidative stress has broad associations with the pathology of most pulmonary diseases, with the most publications in lung injury, lung cancer, asthma, COPD, and pneumonia, demonstrating the close connections between oxidative stress and these five diseases and the strong interest of scientists in these diseases. Mechanistically, oxidative stress causes cell signaling changes, organelle dysfunction, and alterations in the functions and fate of multiple cell types and leads to pathological conditions in the lung.
Among the keywords and the frequent keywords in the bubble diagrams, inflammation, mitochondria, autophagy, ER (endoplasmic reticulum) stress, apoptosis, DNA damage, hypoxia, vascular endothelial growth factor (VEGF), NRF2, and NF-κB demonstrate the most studied mechanisms of oxidative stress and pulmonary diseases. Inflammation appeared in 40%–60% of publications from 4 time periods as keywords. Continuous oxidative stress is acknowledged to cause chronic inflammation, which mediates most chronic diseases, including cancer, diabetes, cardiovascular and neurological diseases (Reuter et al., 2010; Hussain et al., 2016). In the lung, oxidative stress increases inflammation, at least partly through attenuated macrophage function (Flohe et al., 1997; Mokhtari-Zaer et al., 2020).
Mitochondria play a critical role in maintaining normal cell function. Excessive mitochondrial ROS increase the permeability of the mitochondrial membrane, oxidize the sulfhydryl group of enzymes, and cause irreversible damage to mitochondrial DNA, resulting in electron transport chain impairment and mitochondrial membrane potential loss (Liu and Chen, 2017). Mitochondrial dysfunction then contributes to cell abnormalities, such as hyperproliferation in airway smooth muscle cells or apoptosis in alveolar epithelial cells (Wiegman et al., 2015; Yu et al., 2018).
Iron imbalance and especially iron accumulation have been reported in several pulmonary diseases, including chronic obstructive pulmonary disease, cystic fibrosis, pulmonary alveolar proteinosis, and lung fibrosis (Ghio et al., 2008; Neves et al., 2019). The iron chelator deferoxamine alleviates bleomycin-induced lung fibrosis in a mouse model (Ali et al., 2020), indicating that pulmonary iron accumulation contributes to the pathology of pulmonary fibrosis. Iron can produce excessive hydroxyl radicals through the Fenton reaction (Lu and Koppenol, 2005), and the accumulation of excessive ROS promotes mitochondrial dysfunction (Gu et al., 2019). In addition, when free radicals attack unsaturated fatty acids on cell membranes, lipid peroxidation occurs. Severe lipid peroxidation can initiate ferroptosis, which is characterized by iron-dependent lipid peroxidation, metabolic constraints and abnormal mitochondrial morphology, including condensed mitochondrial membrane densities, smaller mitochondrial volume, diminished or vanished mitochondrial cristae, and ruptured mitochondrial outer membrane, and is regulated by glutathione peroxidase 4 (Seibt et al., 2019; Wang et al., 2020). Ferroptosis of cells was reported to play a role in the pathology of COPD, lung injury and lung cancer, and a ferrostatin inhibitor alleviated LPS-induced acute lung injury (Alvarez et al., 2017; Li et al., 2019; Yoshida et al., 2019; Liu et al., 2020).
Autophagy is a catabolic intracellular pathway that plays a crucial role in maintaining cellular homeostasis. Autophagy is associated with allergen-induced oxidative stress and regulates cell senescence (Sachdeva et al., 2019). Mitophagy was reported to reduce EMT (epithelial-mesenchymal transition) induced by TGF-β1, thus inhibiting the progression of pulmonary fibrosis (Prashanth Goud et al., 2019). Additionally, oxidative stress and endoplasmic reticulum stress interact with each other directly and indirectly in respiratory disease (Chen et al., 2018).
Oxidative stress can trigger DNA damage and lung epithelial cell death in pulmonary fibrosis (Cheresh et al., 2013), and mediates lung damage caused by intermittent hypoxia (Tuleta et al., 2016). During the oxidative stress induced by cerebral ischemia/reperfusion injury, the hypoxia inducible factor 1 subunit alpha (HIF-1α)/VEGF signaling pathway is activated, enabling repair of the endothelial barrier in the lung; additionally, the NRF2/heme oxygenase-1 (HO-1) pathway is activated to upregulate antioxidative stress (Fan et al., 2019).
NRF2 is a member of the Cap “n” Collar (CNC) family ofbasic leucine zipper transcription factors and is a “master regulator” of the antioxidant response in the cell. NRF2 regulates the expression of hundreds of genes, including antioxidant enzymes (SOD, catalase, and phase II detoxifying enzymes), genes participating in immune and inflammatory responses, tissue remodeling and fibrosis and carcinogenesis and metastasis (Martin et al., 1998; Itoh et al., 1999; Hybertson et al., 2011). Kelch-like ECH-associated protein 1 (KEAP1) negatively regulates NRF2 by targeting NRF2 for proteasomal degradation; conversely, NRF2 can drive KEAP1 transcription (Singh et al., 2006; Tian et al., 2020). The NRF2-KEAP1 system is an evolutionarily conserved intracellular defense mechanism that counteracts oxidative stress (Bellezza et al., 2018). HO-1 is a phase II detoxifying enzyme transcriptionally regulated by NRF2 and participates in the degradation of heme into biliverdin, carbon monoxide (CO), and Fe2+. Through these metabolites, the NRF2/HO-1 axis exerts protective roles against oxidation, apoptosis and ferroptosis in an organism (Zhang et al., 2018; Fan et al., 2019; Dong et al., 2020; Huang et al., 2020). NRF2 was reported to attenuate the progression of pulmonary fibrosis by inhibiting EMT mediated by suppressing snail expression and upregulating Numb (Zhou et al., 2016; Zhang et al., 2018). Moreover, NRF2 has been suggested as a therapeutic target for lung oxidative injuries, cancer, Alzheimer’s disease, and diabetic cardiomyopathy (Lu et al., 2016; Dianat et al., 2018; Bahn and Jo, 2019; Ge et al., 2019).
As a transcription factor, NF-κB plays essential roles in inflammatory responses. Oxidative status activates NF-κB activity by causing the dissociation of NF-κB from its inhibitor, IκB (inhibitor of NF-κB), and facilitating its nuclear translocation (Flohe et al., 1997). NF-κB overexpression is related to COPD, and it regulates the release of inflammatory mediators such as interleukin 1 beta (IL1β), C-X-C motif chemokine ligand 8 (CXCL8) and cytochrome c oxidase subunit II (COX2) in COPD (Zhou et al., 2018).
The generation of oxidative stress and the pathological processes resulting in pulmonary diseases are summarized in Figure 6. It should be noted that oxidative stress leads to different lung diseases with some similarities and distinct features. For example, most of the pathological mechanisms of pulmonary diseases involve inflammation. While COPD is characterized by excessive airway epithelial cell death and lung injury, lung cancer is caused by uncontrolled epithelial cell proliferation (Goldkorn et al., 2014). Some patients with asthma and COPD show overlapping features, which may be because of the cooccurrence of the two diseases or the different phenotypes of each disease (Barnes, 2017). In addition, for patients with age-related fibrotic diseases such as COPD and lung fibrosis, targeting autophagy and mitochondrial dysfunction that are related to metabolic homeostasis may offer better interventions than other strategies. Collectively, targeting the specific mechanisms underlying a particular pulmonary disease is necessary for developing truly effective therapeutics.
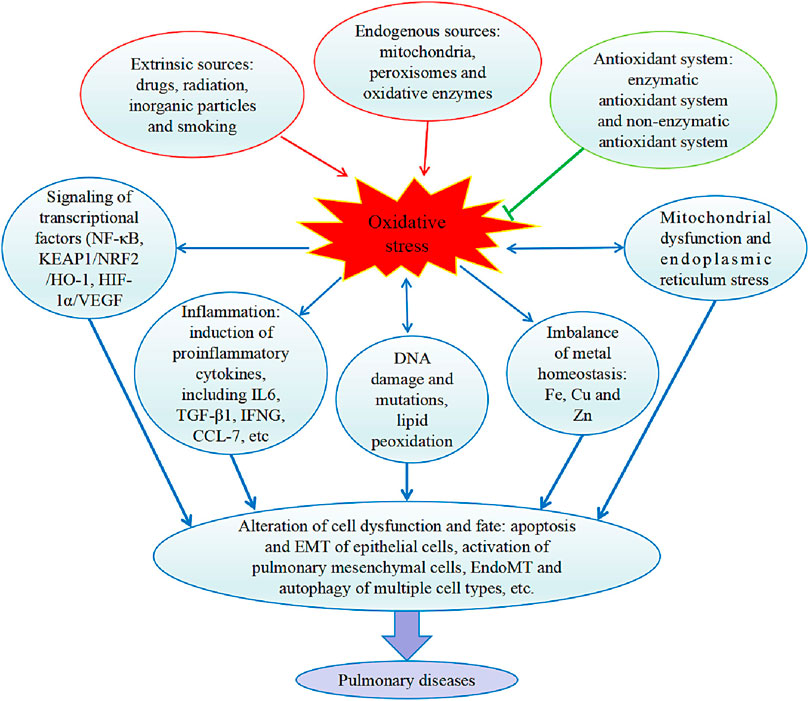
FIGURE 6. Internal and external causes of oxidative stress and effects of oxidative stress on the pathology of pulmonary diseases. In the lung, production of ROS (reactive oxygen species) by endogenous factors and extrinsic factors enhances oxidative stress and antioxidant system suppresses oxidative stress. Oxidative stress triggers the cell signaling pathways mediated by activation of transcriptional factors, and inflammatory cytokines were induced. Oxidative stress also damages DNA and lipids, and disturbs homeostatic balance of metals. Then, the resulted organelle normal function was defected, e.g., mitochondrial dysfunction and ER (endoplasmic reticulum) stress occur. Collectively, a series of cell malfunctions was induced, including apoptosis and EMT (epithelial-mesenchymal transition) of epithelial cells, activation of pulmonary mesenchymal cells, EndoMT (endothelial to mesenchymal transition) and autophagy of multiple cell types. IL6, interleukin 6. TGF-β1, transforming growth factor beta 1. IFNG, Interferon-gamma. CCL-7, chemokine C-C motif ligands 7. NF-κB, nuclear factor-κB. KEAP1, Kelch-like ECH-associated protein. NRF2, nuclear factor, erythroid 2 like 2. HO-1, heme oxygenase-1. HIF-1α, hypoxia inducible factor 1 subunit alpha. VEGF, vascular endothelial growth factor.
Conclusions and future directions
In this review, thirty potential therapeutic drugs are discussed. Vitamin E helps remove free radicals and regulates the immune system and inflammation (Lewis et al., 2019). In addition to being an antioxidant, N-acetylcysteine is a mucolytic agent and can be used to treat respiratory diseases caused by excessive secretion of thick mucus (Raghu et al., 2020). Polyphenols inhibit the expression of inducible nitric oxide synthase and protect against oxidative and nitroxidative lung injury (Impellizzeri et al., 2015). Several polyphenol compounds, including resveratrol, curcumin, isorhamnetin, tannic acid, and honokiol, can attenuate pulmonary fibrosis (Conte et al., 2015; Amini et al., 2018; Zheng et al., 2019; Pulivendala et al., 2020; Rajasekar et al., 2020; Wang et al., 2022). The effects of these potential therapeutic agents have been determined mainly in experimental animals; however, confirmation of their effects requires more evidence from the bedside.
At present, there is no truly effective medicine that can be used to cure several common pulmonary diseases, such as lung cancer, COPD, and lung fibrosis. It is evident that some kinds of pulmonary diseases share similarities in pathways, and therapeutics, although each lung disease is unique. It is well recognized that ROS and RNS are both deleterious and beneficial species for physiological processes (Qu et al., 2011). Therefore, in the light of the origin, targeting harmful ROS or RNS species in specific organelles with nanotechniques, in combination with regulation of critical upstream signaling pathways and improvement of important downstream pathological processes, such as mitochondrial dysfunction, ferroptosis and inflammation through combined medication, in a particular pulmonary disease, will be a promising direction to cure these diseases.
Author contributions
Conceptualization, XL, XW, and PC; methodology and software, XW, JC, and HZ; writing—original draft preparation, XL; writing—review and editing, PC; supervision, PC; funding acquisition, PC. All authors contributed to the article and approved the submitted version.
Funding
This study were supported by the National Natural Science Foundation of China (31900817 and 32071119) for open access publication fee.
Conflict of interest
The authors declare that the research was conducted in the absence of any commercial or financial relationships that could be construed as a potential conflict of interest.
Publisher’s note
All claims expressed in this article are solely those of the authors and do not necessarily represent those of their affiliated organizations, or those of the publisher, the editors, and the reviewers. Any product that may be evaluated in this article, or claim that may be made by its manufacturer, is not guaranteed or endorsed by the publisher.
References
Ali, M. K., Kim, R. Y., Brown, A. C., Donovan, C., Vanka, K. S., Mayall, J. R., et al. (2020). Critical role for iron accumulation in the pathogenesis of fibrotic lung disease. J. Pathol. 251, 49–62. doi:10.1002/path.5401
Alvarez, S. W., Sviderskiy, V. O., Terzi, E. M., Papagiannakopoulos, T., Moreira, A. L., Adams, S., et al. (2017). NFS1 undergoes positive selection in lung tumours and protects cells from ferroptosis. Nature 551, 639–643. doi:10.1038/nature24637
Amini, P., Saffar, H., Nourani, M. R., Motevaseli, E., Najafi, M., Ali Taheri, R., et al. (2018). Curcumin mitigates radiation-induced lung pneumonitis and fibrosis in rats. Int. J. Mol. Cell. Med. 7, 212–219. doi:10.22088/IJMCM.BUMS.7.4.212
Bahl, V., Lin, S., Xu, N., Davis, B., Wang, Y. H., and Talbot, P. (2012). Comparison of electronic cigarette refill fluid cytotoxicity using embryonic and adult models. Reprod. Toxicol. 34, 529–537. doi:10.1016/j.reprotox.2012.08.001
Bahn, G., and Jo, D. G. (2019). Therapeutic approaches to Alzheimer's disease through modulation of NRF2. Neuromolecular Med. 21, 1–11. doi:10.1007/s12017-018-08523-5
Barnes, P. J. (2017). Cellular and molecular mechanisms of asthma and COPD. Clin. Sci. 131, 1541–1558. doi:10.1042/CS20160487
Bellezza, I., Giambanco, I., Minelli, A., and Donato, R. (2018). Nrf2-Keap1 signaling in oxidative and reductive stress. Biochim. Biophys. Acta Mol. Cell Res. 1865, 721–733. doi:10.1016/j.bbamcr.2018.02.010
Bhatt, J. M., Ramphul, M., and Bush, A. (2020). An update on controversies in e-cigarettes. Paediatr. Respir. Rev. 36, 75–86. doi:10.1016/j.prrv.2020.09.003
Chatterjee, A., and Gupta, S. (2018). The multifaceted role of glutathione S-transferases in cancer. Cancer Lett. 433, 33–42. doi:10.1016/j.canlet.2018.06.028
Chen, A. C., Burr, L., and McGuckin, M. A. (2018). Oxidative and endoplasmic reticulum stress in respiratory disease. Clin. Transl. Immunol. 7, e1019. doi:10.1002/cti2.1019
Cheresh, P., Kim, S. J., Tulasiram, S., and Kamp, D. W. (2013). Oxidative stress and pulmonary fibrosis. Biochim. Biophys. Acta 1832, 1028–1040. doi:10.1016/j.bbadis.2012.11.021
Conte, E., Fagone, E., Fruciano, M., Gili, E., Iemmolo, M., and Vancheri, C. (2015). Anti-inflammatory and antifibrotic effects of resveratrol in the lung. Histology Histopathol. 30, 523–529. doi:10.14670/HH-30.523
Dan Dunn, J., Alvarez, L. A., Zhang, X., and Soldati, T. (2015). Reactive oxygen species and mitochondria: A nexus of cellular homeostasis. Redox Biol. 6, 472–485. doi:10.1016/j.redox.2015.09.005
Dianat, M., Radan, M., Badavi, M., Mard, S. A., Bayati, V., and Ahmadizadeh, M. (2018). Crocin attenuates cigarette smoke-induced lung injury and cardiac dysfunction by anti-oxidative effects: The role of Nrf2 antioxidant system in preventing oxidative stress. Respir. Res. 19, 58. doi:10.1186/s12931-018-0766-3
Dong, H., Qiang, Z., Chai, D., Peng, J., Xia, Y., Hu, R., et al. (2020). Nrf2 inhibits ferroptosis and protects against acute lung injury due to intestinal ischemia reperfusion via regulating SLC7A11 and HO-1. Aging 12, 12943–12959. doi:10.18632/aging.103378
Duecker, R., Baer, P., Eickmeier, O., Strecker, M., Kurz, J., Schaible, A., et al. (2018). Oxidative stress-driven pulmonary inflammation and fibrosis in a mouse model of human ataxia-telangiectasia. Redox Biol. 14, 645–655. doi:10.1016/j.redox.2017.11.006
Fan, J., Lv, H., Li, J., Che, Y., Xu, B., Tao, Z., et al. (2019). Roles of Nrf2/HO-1 and HIF-1α/VEGF in lung tissue injury and repair following cerebral ischemia/reperfusion injury. J. Cell Physiol. 234, 7695–7707. doi:10.1002/jcp.27767
Flohe, L., Brigelius-Flohe, R., Saliou, C., Traber, M. G., and Packer, L. (1997). Redox regulation of NF-kappa B activation. Free Radic. Biol. Med. 22, 1115–1126. doi:10.1016/s0891-5849(96)00501-1
Forrester, S. J., Kikuchi, D. S., Hernandes, M. S., Xu, Q., and Griendling, K. K. (2018). Reactive oxygen species in metabolic and inflammatory signaling. Circulation Res. 122, 877–902. doi:10.1161/CIRCRESAHA.117.311401
Ge, Z. D., Lian, Q., Mao, X., and Xia, Z. (2019). Current status and challenges of NRF2 as a potential therapeutic target for diabetic cardiomyopathy. Int. heart J. 60, 512–520. doi:10.1536/ihj.18-476
Ghio, A. J., Carter, J. D., Richards, J. H., Richer, L. D., Grissom, C. K., and Elstad, M. R. (2003). Iron and iron-related proteins in the lower respiratory tract of patients with acute respiratory distress syndrome. Crit. Care Med. 31, 395–400. doi:10.1097/01.CCM.0000050284.35609.97
Ghio, A. J., Stonehuerner, J. G., Richards, J. H., Crissman, K. M., Roggli, V. L., Piantadosi, C. A., et al. (2008). Iron homeostasis and oxidative stress in idiopathic pulmonary alveolar proteinosis: A case-control study. Respir. Res. 9, 10. doi:10.1186/1465-9921-9-10
Goldkorn, T., Filosto, S., and Chung, S. (2014). Lung injury and lung cancer caused by cigarette smoke-induced oxidative stress: Molecular mechanisms and therapeutic opportunities involving the ceramide-generating machinery and epidermal growth factor receptor. Antioxid. Redox Signal 21, 2149–2174. doi:10.1089/ars.2013.5469
Gu, J., Gong, D., Wang, Y., Feng, T., Zhang, J., Hu, S., et al. (2023). Chronic exposure to IQOS results in impaired pulmonary function and lung tissue damage in mice. Toxicol. Lett. 374, 1–10. doi:10.1016/j.toxlet.2022.11.022
Gu, L., Larson Casey, J. L., Andrabi, S. A., Lee, J. H., Meza-Perez, S., Randall, T. D., et al. (2019). Mitochondrial calcium uniporter regulates PGC-1α expression to mediate metabolic reprogramming in pulmonary fibrosis. Redox Biol. 26, 101307. doi:10.1016/j.redox.2019.101307
Han, B. H., Jang, S. H., Jang, Y. J., Na, S. W., Yoon, J. J., Moon, H. G., et al. (2023). Diesel vehicles-derived PM2.5 induces lung and cardiovascular injury attenuates by Securiniga suffruticosa: Involvement of NF-κB-mediated NLRP3 inflammasome activation pathway. Biomed. Pharmacother. 162, 114637. doi:10.1016/j.biopha.2023.114637
Helmig, S., Walter, D., Putzier, J., Maxeiner, H., Wenzel, S., and Schneider, J. (2018). Oxidative and cytotoxic stress induced by inorganic granular and fibrous particles. Mol. Med. Rep. 17, 8518–8529. doi:10.3892/mmr.2018.8923
Huang, C. Y., Deng, J. S., Huang, W. C., Jiang, W. P., and Huang, G. J. (2020). Attenuation of lipopolysaccharide-induced acute lung injury by hispolon in mice, through regulating the TLR4/PI3K/Akt/mTOR and keap1/nrf2/HO-1 pathways, and suppressing oxidative stress-mediated ER stress-induced apoptosis and autophagy. Nutrients 12, 1742. doi:10.3390/nu12061742
Hussain, T., Tan, B., Yin, Y., Blachier, F., Tossou, M. C., and Rahu, N. (2016). Oxidative stress and inflammation: What polyphenols can do for us? Oxid. Med. Cell Longev. 2016, 7432797. doi:10.1155/2016/7432797
Hybertson, B. M., Gao, B., Bose, S. K., and McCord, J. M. (2011). Oxidative stress in health and disease: The therapeutic potential of Nrf2 activation. Mol. Asp. Med. 32, 234–246. doi:10.1016/j.mam.2011.10.006
Impellizzeri, D., Talero, E., Siracusa, R., Alcaide, A., Cordaro, M., Maria Zubelia, J., et al. (2015). Protective effect of polyphenols in an inflammatory process associated with experimental pulmonary fibrosis in mice. Br. J. Nutr. 114, 853–865. doi:10.1017/S0007114515002597
Itoh, K., Ishii, T., Wakabayashi, N., and Yamamoto, M. (1999). Regulatory mechanisms of cellular response to oxidative stress. Free Radic. Res. 31, 319–324. doi:10.1080/10715769900300881
Kirkham, P. A., and Barnes, P. J. (2013). Oxidative stress in COPD. Chest 144, 266–273. doi:10.1378/chest.12-2664
Klaunig, J. E. (2018). Oxidative stress and cancer. Curr. Pharm. Des. 24, 4771–4778. doi:10.2174/1381612825666190215121712
Lee, G. Y., and Han, S. N. (2018). The role of vitamin E in immunity. Nutrients 10, 1614. doi:10.3390/nu10111614
Lewis, E. D., Meydani, S. N., and Wu, D. (2019). Regulatory role of vitamin E in the immune system and inflammation. IUBMB life 71, 487–494. doi:10.1002/iub.1976
Li, X., Zhuang, X., and Qiao, T. (2019). Role of ferroptosis in the process of acute radiation-induced lung injury in mice. Biochem. Biophys. Res. Commun. 519, 240–245. doi:10.1016/j.bbrc.2019.08.165
Lippi, G., Favaloro, E. J., Meschi, T., Mattiuzzi, C., Borghi, L., and Cervellin, G. (2014). E-Cigarettes and cardiovascular risk: Beyond science and mysticism. Semin. Thromb. Hemost. 40, 60–65. doi:10.1055/s-0033-1363468
Liu, P., Feng, Y., Li, H., Chen, X., Wang, G., Xu, S., et al. (2020). Ferrostatin-1 alleviates lipopolysaccharide-induced acute lung injury via inhibiting ferroptosis. Cell Mol. Biol. Lett. 25, 10. doi:10.1186/s11658-020-00205-0
Liu, X., and Chen, Z. (2017). The pathophysiological role of mitochondrial oxidative stress in lung diseases. J. Transl. Med. 15, 207. doi:10.1186/s12967-017-1306-5
Lu, C., and Koppenol, W. H. (2005). Inhibition of the Fenton reaction by nitrogen monoxide. J. Biol. Inorg. Chem. 10, 732–738. doi:10.1007/s00775-005-0019-z
Lu, M. C., Ji, J. A., Jiang, Z. Y., and You, Q. D. (2016). The keap1-nrf2-ARE pathway as a potential preventive and therapeutic target: An update. Med. Res. Rev. 36, 924–963. doi:10.1002/med.21396
Malinska, D., Szymanski, J., Patalas-Krawczyk, P., Michalska, B., Wojtala, A., Prill, M., et al. (2018). Assessment of mitochondrial function following short- and long-term exposure of human bronchial epithelial cells to total particulate matter from a candidate modified-risk tobacco product and reference cigarettes. Food Chem. Toxicol. 115, 1–12. doi:10.1016/j.fct.2018.02.013
Margis, R., Dunand, C., Teixeira, F. K., and Margis-Pinheiro, M. (2008). Glutathione peroxidase family - an evolutionary overview. FEBS J. 275, 3959–3970. doi:10.1111/j.1742-4658.2008.06542.x
Martin, F., van Deursen, J. M., Shivdasani, R. A., Jackson, C. W., Troutman, A. G., and Ney, P. A. (1998). Erythroid maturation and globin gene expression in mice with combined deficiency of NF-E2 and nrf-2. Blood 91, 3459–3466. doi:10.1182/blood.v91.9.3459.3459_3459_3466
Martinez, M. C., and Andriantsitohaina, R. (2009). Reactive nitrogen species: Molecular mechanisms and potential significance in health and disease. Antioxid. Redox Signal 11, 669–702. doi:10.1089/ars.2007.1993
Mokhtari-Zaer, A., Norouzi, F., Askari, V. R., Khazdair, M. R., Roshan, N. M., Boskabady, M., et al. (2020). The protective effect of Nigella sativa extract on lung inflammation and oxidative stress induced by lipopolysaccharide in rats. J. Ethnopharmacol. 253, 112653. doi:10.1016/j.jep.2020.112653
Muratani, S., Ichikawa, S., Erami, K., and Ito, S. (2023). Oxidative stress-mediated epidermal growth factor receptor activation by cigarette smoke or heated tobacco aerosol in human primary bronchial epithelial cells from multiple donors. J. Appl. Toxicol. Online ahead of print. doi:10.1002/jat.4469
Neves, J., Haider, T., Gassmann, M., and Muckenthaler, M. U. (2019). Iron homeostasis in the lungs-A balance between health and disease. Pharm. (Basel) 12, 5. doi:10.3390/ph12010005
Owusu, S. B., Hudik, E., Ferard, C., Dupre-Crochet, S., Addison, E., Preko, K., et al. (2020). Radiation-induced reactive oxygen species partially assemble neutrophil NADPH oxidase. Free Radic. Biol. Med. 164, 76–84. doi:10.1016/j.freeradbiomed.2020.12.233
Philippot, Q., Deslee, G., Adair-Kirk, T. L., Woods, J. C., Byers, D., Conradi, S., et al. (2014). Increased iron sequestration in alveolar macrophages in chronic obstructive pulmonary disease. PLoS One 9, e96285. doi:10.1371/journal.pone.0096285
Pizzino, G., Irrera, N., Cucinotta, M., Pallio, G., Mannino, F., Arcoraci, V., et al. (2017). Oxidative stress: Harms and benefits for human health. Oxidative Med. Cell. Longev. 2017, 8416763–8416813. doi:10.1155/2017/8416763
Prashanth Goud, M., Bale, S., Pulivendala, G., and Godugu, C. (2019). Therapeutic effects of Nimbolide, an autophagy regulator, in ameliorating pulmonary fibrosis through attenuation of TGF-β1 driven epithelial-to-mesenchymal transition. Int. Immunopharmacol. 75, 105755. doi:10.1016/j.intimp.2019.105755
Pulivendala, G., Bale, S., and Honokiol, G. C. (2020). Honokiol: A polyphenol neolignan ameliorates pulmonary fibrosis by inhibiting TGF-β/smad signaling, matrix proteins and IL-6/CD44/STAT3 axis both in vitro and in vivo. Toxicol. Appl. Pharmacol. 391, 114913. doi:10.1016/j.taap.2020.114913
Qu, M., Zhou, Z., Chen, C., Li, M., Pei, L., Chu, F., et al. (2011). Lycopene protects against trimethyltin-induced neurotoxicity in primary cultured rat hippocampal neurons by inhibiting the mitochondrial apoptotic pathway. Neurochem. Int. 59, 1095–1103. doi:10.1016/j.neuint.2011.10.005
Raghu, G., Berk, M., Campochiaro, P. A., Jaeschke, H., Marenzi, G., Richeldi, L., et al. (2020). The multifaceted therapeutic role of N-acetylcysteine (NAC) in disorders characterized by oxidative stress. Curr. Neuropharmacol. 19, 1202–1224. doi:10.2174/1570159x19666201230144109
Rajasekar, N., Sivanantham, A., Kar, A., Mahapatra, S. K., Ahirwar, R., Thimmulappa, R. K., et al. (2020). Tannic acid alleviates experimental pulmonary fibrosis in mice by inhibiting inflammatory response and fibrotic process. Inflammopharmacology 28, 1301–1314. doi:10.1007/s10787-020-00707-5
Rangarajan, S., Bone, N. B., Zmijewska, A. A., Jiang, S., Park, D. W., Bernard, K., et al. (2018). Metformin reverses established lung fibrosis in a bleomycin model. Nat. Med. 24, 1121–1127. doi:10.1038/s41591-018-0087-6
Reid, D. W., Carroll, V., O'May, C., Champion, A., and Kirov, S. M. (2007). Increased airway iron as a potential factor in the persistence of Pseudomonas aeruginosa infection in cystic fibrosis. Eur. Respir. J. 30, 286–292. doi:10.1183/09031936.00154006
Reuter, S., Gupta, S. C., Chaturvedi, M. M., and Aggarwal, B. B. (2010). Oxidative stress, inflammation, and cancer: How are they linked? Free Radic. Biol. Med. 49, 1603–1616. doi:10.1016/j.freeradbiomed.2010.09.006
Sachdeva, K., Do, D. C., Zhang, Y., Hu, X., Chen, J., and Gao, P. (2019). Environmental exposures and asthma development: Autophagy, mitophagy, and cellular senescence. Front. Immunol. 10, 2787. doi:10.3389/fimmu.2019.02787
Schmitt, B., Vicenzi, M., Garrel, C., and Denis, F. M. (2015). Effects of N-acetylcysteine, oral glutathione (gsh) and a novel sublingual form of gsh on oxidative stress markers: A comparative crossover study. Redox Biol. 6, 198–205. doi:10.1016/j.redox.2015.07.012
Seibt, T. M., Proneth, B., and Conrad, M. (2019). Role of GPX4 in ferroptosis and its pharmacological implication. Free Radic. Biol. Med. 133, 144–152. doi:10.1016/j.freeradbiomed.2018.09.014
Singh, A., Kukreti, R., Saso, L., and Kukreti, S. (2019). Oxidative stress: A key modulator in neurodegenerative diseases. Molecules 24, 1583. doi:10.3390/molecules24081583
Singh, A., Misra, V., Thimmulappa, R. K., Lee, H., Ames, S., Hoque, M. O., et al. (2006). Dysfunctional KEAP1-NRF2 interaction in non-small-cell lung cancer. PLoS Med. 3, e420. doi:10.1371/journal.pmed.0030420
Staitieh, B. S., Malik, S., Auld, S. C., Wigger, G. W., Fan, X., Roth, A. T., et al. (2023). HIV increases the risk of cigarette smoke-induced emphysema through MMP-9. J. Acquir Immune Defic. Syndr. 92, 263–270. doi:10.1097/QAI.0000000000003125
Steven, S., Frenis, K., Oelze, M., Kalinovic, S., Kuntic, M., Bayo Jimenez, M. T., et al. (2019). Vascular inflammation and oxidative stress: Major triggers for cardiovascular disease. Oxidative Med. Cell. Longev. 2019, 7092151–7092226. doi:10.1155/2019/7092151
Tian, Y., Liu, Q., Yu, S., Chu, Q., Chen, Y., Wu, K., et al. (2020). NRF2-Driven KEAP1 transcription in human lung cancer. Mol. Cancer Res. 18, 1465–1476. doi:10.1158/1541-7786.MCR-20-0108
Tostes, R. C., Carneiro, F. S., Lee, A. J., Giachini, F. R., Leite, R., Osawa, Y., et al. (2008). Cigarette smoking and erectile dysfunction: Focus on NO bioavailability and ROS generation. J. Sex. Med. 5, 1284–1295. doi:10.1111/j.1743-6109.2008.00804.x
Tuleta, I., Stockigt, F., Juergens, U. R., Pizarro, C., Schrickel, J. W., Kristiansen, G., et al. (2016). Intermittent hypoxia contributes to the lung damage by increased oxidative stress, inflammation, and disbalance in protease/antiprotease system. Lung 194, 1015–1020. doi:10.1007/s00408-016-9946-4
Uchiyama, N., Yukawa, T., Dragan, Y. P., Wagoner, M. P., and Naven, R. T. (2020). New phenotypic cytotoxicity assay for ROS-inducing compounds using rat renal epithelial cells. Toxicol. Lett. 331, 227–234. doi:10.1016/j.toxlet.2020.06.005
Valavanidis, A., Vlachogianni, T., Fiotakis, K., and Loridas, S. (2013). Pulmonary oxidative stress, inflammation and cancer: Respirable particulate matter, fibrous dusts and ozone as major causes of lung carcinogenesis through reactive oxygen species mechanisms. Int. J. Environ. Res. Public Health 10, 3886–3907. doi:10.3390/ijerph10093886
van der Vliet, A., Janssen-Heininger, Y. M. W., and Anathy, V. (2018). Oxidative stress in chronic lung disease: From mitochondrial dysfunction to dysregulated redox signaling. Mol. Asp. Med. 63, 59–69. doi:10.1016/j.mam.2018.08.001
Wang, H., Liu, C., Zhao, Y., and Gao, G. (2020). Mitochondria regulation in ferroptosis. Eur. J. Cell Biol. 99, 151058. doi:10.1016/j.ejcb.2019.151058
Wang, L., Shao, M., Jiang, W., and Huang, Y. (2022). Resveratrol alleviates bleomycin-induced pulmonary fibrosis by inhibiting epithelial-mesenchymal transition and down-regulating TLR4/NF-κB and TGF-β1/smad3 signalling pathways in rats. Tissue Cell 79, 101953. doi:10.1016/j.tice.2022.101953
Weydert, C. J., and Cullen, J. J. (2009). Measurement of superoxide dismutase, catalase and glutathione peroxidase in cultured cells and tissue. Nat. Protoc. 5, 51–66. doi:10.1038/nprot.2009.197
Wiegman, C. H., Li, F., Ryffel, B., Togbe, D., and Chung, K. F. (2020). Oxidative stress in ozone-induced chronic lung inflammation and emphysema: A facet of chronic obstructive pulmonary disease. Front. Immunol. 11, 1957. doi:10.3389/fimmu.2020.01957
Wiegman, C. H., Michaeloudes, C., Haji, G., Narang, P., Clarke, C. J., Russell, K. E., et al. (2015). Oxidative stress–induced mitochondrial dysfunction drives inflammation and airway smooth muscle remodeling in patients with chronic obstructive pulmonary disease. J. Allergy Clin. Immunol. 136, 769–780. doi:10.1016/j.jaci.2015.01.046
Yoshida, M., Minagawa, S., Araya, J., Sakamoto, T., Hara, H., Tsubouchi, K., et al. (2019). Involvement of cigarette smoke-induced epithelial cell ferroptosis in COPD pathogenesis. Nat. Commun. 10, 3145. doi:10.1038/s41467-019-10991-7
Yu, G., Tzouvelekis, A., Wang, R., Herazo-Maya, J. D., Ibarra, G. H., Srivastava, A., et al. (2018). Thyroid hormone inhibits lung fibrosis in mice by improving epithelial mitochondrial function. Nat. Med. 24, 39–49. doi:10.1038/nm.4447
Zhang, Z., Qu, J., Zheng, C., Zhang, P., Zhou, W., Cui, W., et al. (2018). Nrf2 antioxidant pathway suppresses Numb-mediated epithelial-mesenchymal transition during pulmonary fibrosis. Cell death Dis. 9, 83. doi:10.1038/s41419-017-0198-x
Zheng, Q., Tong, M., Ou, B., Liu, C., Hu, C., and Yang, Y. (2019). Isorhamnetin protects against bleomycin-induced pulmonary fibrosis by inhibiting endoplasmic reticulum stress and epithelial-mesenchymal transition. Int. J. Mol. Med. 43, 117–126. doi:10.3892/ijmm.2018.3965
Zhou, L., Liu, Y., Chen, X., Wang, S., Liu, H., Zhang, T., et al. (2018). Over-expression of nuclear factor-κB family genes and inflammatory molecules is related to chronic obstructive pulmonary disease. Int. J. chronic Obstr. Pulm. Dis. 13, 2131–2138. doi:10.2147/COPD.S164151
Keywords: oxidative stress, inflammation, mitochondria, antioxidants, pulmonary disease
Citation: Liu X, Wang X, Chang J, Zhang H and Cao P (2023) Landscape analysis and overview of the literature on oxidative stress and pulmonary diseases. Front. Pharmacol. 14:1190817. doi: 10.3389/fphar.2023.1190817
Received: 21 March 2023; Accepted: 15 May 2023;
Published: 26 May 2023.
Edited by:
Sabina Antonela Antoniu, Grigore T. Popa University of Medicine and Pharmacy, RomaniaReviewed by:
Stefan Hadzic, University of Giessen, GermanyAntigona Trofor, Grigore T. Popa University of Medicine and Pharmacy, Romania
Copyright © 2023 Liu, Wang, Chang, Zhang and Cao. This is an open-access article distributed under the terms of the Creative Commons Attribution License (CC BY). The use, distribution or reproduction in other forums is permitted, provided the original author(s) and the copyright owner(s) are credited and that the original publication in this journal is cited, in accordance with accepted academic practice. No use, distribution or reproduction is permitted which does not comply with these terms.
*Correspondence: Pengxiu Cao, caopx@mail.hebtu.edu.cn
†These authors have contributed equally to this work