- 1Department of Pharmacology and Toxicology, Faculty of Medicine, American University of Beirut, Beirut, Lebanon
- 2Department of Pharmaceutical Biochemistry, Faculty of Pharmacy, Alexandria University, Alexandria, Egypt
- 3Translational and Clinical Research Institute, Medical School, Newcastle University, Newcastle upon Tyne, United Kingdom
- 4Institute of Experimental and Clinical Pharmacology, University Hospital Schleswig-Holstein, Kiel, Germany
Considerable efforts have been exerted to implement Pharmacogenomics (PGx), the study of interindividual variations in DNA sequence related to drug response, into routine clinical practice. In this article, we first briefly describe PGx and its role in improving treatment outcomes. We then propose an approach to initiate clinical PGx in the hospital setting. One should first evaluate the available PGx evidence, review the most relevant drugs, and narrow down to the most actionable drug-gene pairs and related variant alleles. This is done based on data curated and evaluated by experts such as the pharmacogenomics knowledge implementation (PharmGKB) and the Clinical Pharmacogenetics Implementation Consortium (CPIC), as well as drug regulatory authorities such as the US Food and Drug Administration (FDA) and European Medicinal Agency (EMA). The next step is to differentiate reactive point of care from preemptive testing and decide on the genotyping strategy being a candidate or panel testing, each of which has its pros and cons, then work out the best way to interpret and report PGx test results with the option of integration into electronic health records and clinical decision support systems. After test authorization or testing requirements by the government or drug regulators, putting the plan into action involves several stakeholders, with the hospital leadership supporting the process and communicating with payers, the pharmacy and therapeutics committee leading the process in collaboration with the hospital laboratory and information technology department, and healthcare providers (HCPs) ordering the test, understanding the results, making the appropriate therapeutic decisions, and explaining them to the patient. We conclude by recommending some strategies to further advance the implementation of PGx in practice, such as the need to educate HCPs and patients, and to push for more tests’ reimbursement. We also guide the reader to available PGx resources and examples of PGx implementation programs and initiatives.
1 Introduction
Interindividual variability in drug response is driven by several extrinsic and intrinsic factors, with genetic variations being increasingly recognized among these factors that lead to changes in the activity or availability of drug metabolizing enzymes (DMEs), receptors, channels, and other proteins involved in drug pharmacokinetics (PK) and pharmacodynamics (PD) (Thummel and Lin, 2014). Consequently, the term Pharmacogenomics (PGx), the study of interindividual variations in DNA sequence related to drug efficacy and toxicity, was coined. In this sense, PGx has become an effective tool to fulfill the promise of personalized medicine, while allowing patients to be treated based on their genetic makeup (Mitri et al., 2010; Bartlett et al., 2012).
Despite all emerging evidence and efforts enabling PGx, its clinical implementation has been suboptimal worldwide, especially in the developing world (Abou Diwan et al., 2019; Zgheib et al., 2020; El Shamieh and Zgheib, 2022). For instance, and in addition to global challenges such as the perceived lack of clinical utility and worries of disrupting the usual clinical pathways, other barriers may be attributed to local circumstances such as absence of national regulations for PGx testing, suboptimal infrastructure for the PGx integration into healthcare providers’ (HCP) workflow, lagging insurance plans for coverage of PGx testing, and lack of resources including national PGx data, guidelines, and necessary funds (Caraballo et al., 2017; Rigter et al., 2020; Pirmohamed, 2023).
Considerable efforts have been exerted to implement PGx into routine clinical practice. These efforts relied upon studies providing robust evidence for the benefit of PGx-guided therapeutic strategies. For instance, it has been reported that approximately 91%–99% of patients have at least one genotype that is associated with PGx actionable drugs, and that these drugs constitute up to 18% of all prescribed medications (Krebs and Milani, 2019). Moreover, a recently published study from the European Ubiquitous Pharmacogenomics (U-PGx) clinical implementation project showed that patients with PGx actionable test results, when treated according to Royal Dutch Association for the Advancement of Pharmacy - Pharmacogenetics Working Group (DPWG) recommendations, resulted in a lower percentage (21%) of clinically relevant adverse drug reactions (ADRs) compared to the control group (27.7%) that received standard treatment, though this difference was also seen for patients in the case group receiving nonactionable drugs (Swen et al., 2023). Further evaluations can be pursued in this study to address the influence of several genes, specific adverse reactions related to individual drugs, and phenoconversion caused by polypharmacy (Penas, 2023). In addition, it has been shown that ADRs and hospitalization resulting from drug toxicity can be better controlled by applying PGx. Cost savings from PGx-guided therapy can reach up to 3962 USD per patient per year even when test costs are considered (Luzum et al., 2021). More specifically, a systematic review that evaluated PGx-guided treatment of antidepressants and antipsychotic medications showed that 50% and 39% of the included studies revealed cost-effectiveness and cost-saving of PGx testing, respectively (Karamperis et al., 2021).
Considering the extensive evidence on the benefits of PGx and the availability of a myriad of resources enabling its clinical implementation, herein we propose an approach for initiating clinical PGx in the hospital setting, while acknowledging that implementation depends on local circumstances such as resources available, differences in insurance plans, and peculiarities of the health service’s organization, etc .,… To begin with, we introduce the stakeholders engaged in the implementation, evaluation, and improvement of the program. Next, we propose steps to be followed for developing and applying PGx in hospital clinical practice. We then discuss strategies to address the PGx awareness and training needs of HCPs and patients, and elaborate on the necessity of test reimbursement and how it can be enhanced. We also guide the reader to available PGx resources, and examples of PGx implementation programs and initiatives.
2 Stakeholders engaged in the clinical pgx design and implementation process
At least eight main stakeholders are involved in the PGx design and implementation process in the hospital setting (Figure 1; Box 1). These include drug regulators authorizing or requiring specific PGx tests, hospital leadership supporting the process and communicating with payers, pharmacy and therapeutics (P&T) committee leading the process in collaboration with the hospital, molecular laboratory and information technology (IT), and HCPs ordering the test, understanding the results, making the appropriate therapeutic decisions, and explaining them to the patients.
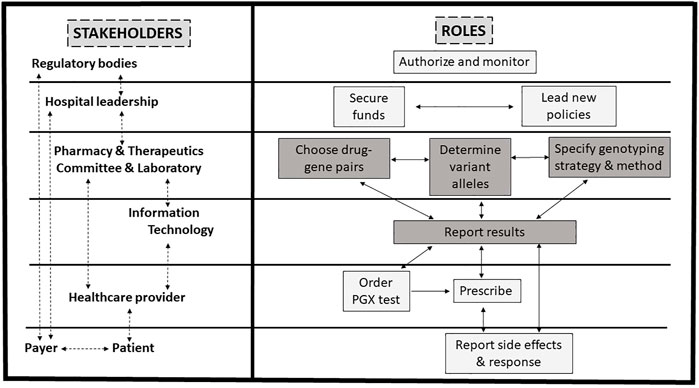
FIGURE 1. Proposed framework for pharmacogenomics (PGx) implementation in practice. PGx implementation in practice involves several stakeholders. See Box 1 for details. Briefly, after test authorization or requirements for testing by drug regulators, the hospital leadership supports the process and communicates with payers, while the pharmacy and therapeutics committee leads the process in collaboration with the hospital laboratory and information technology department. Healthcare providers order the test, make the appropriate therapeutic decisions, and explain them to the patient whom reports to the healthcare provider and payer. Steps for the design of the clinical PGx are highlighted in grey and detailed in Figure 2.
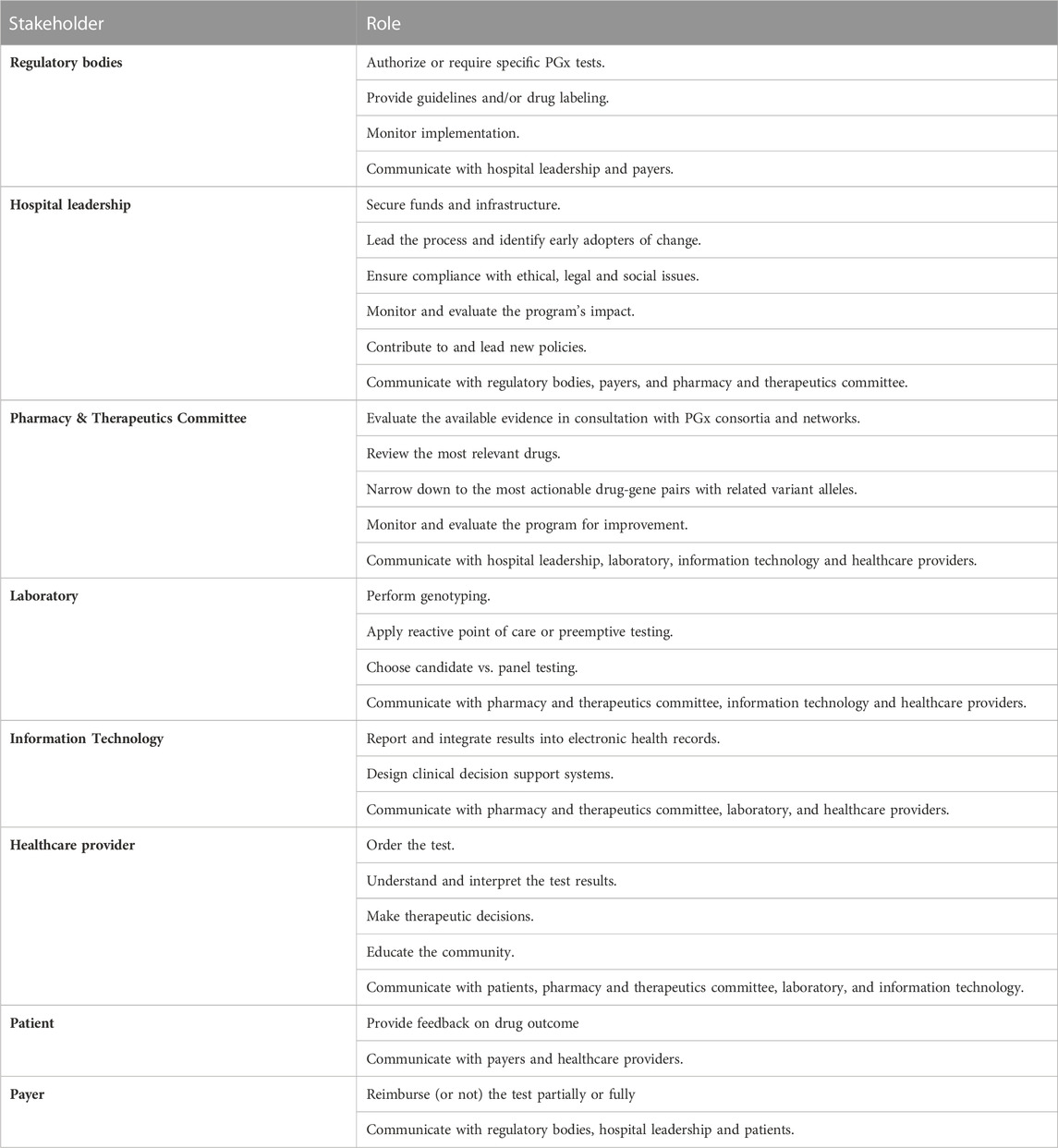
BOX 1. List and role of main stakeholders involved in the pharmacogenomics (PGx) implementation process in a hospital setting
2.1 Regulatory bodies
The FDA and EMA regulatory bodies in the US and Europe, respectively, are responsible for regulating the addition of PGx information and assessing the level of PGx labeling, be it required, recommended, actionable or informative (Ehmann et al., 2014; Mehta et al., 2020). Their recommendations are available through PharmGKB website, where HCPs can access all corresponding prescribing information and recommendations. In addition, guidelines on PGx data usage in drug development and labeling were established by the FDA and EMA. According to the FDA (United States Food and Drug Administration, 2005), the sponser may choose to submit with an investigational or marketing application PGx data that have not yet reached the status of a valid biomarker to, for example, correlate specific toxicities with genetic data, or inform the design of clinical trials. However, when the PGx data are known to affect safety in animals or efficacy or safety in humans, it is recommended that such data are submitted with the application. As for labeling, the PGx data may be included in an informational or actionable manner. Concerning the EMA (European Medicines Agency, 2010), and in the case of co-development of PGx biomarkers or assays, guidelines are put in place while reflecting on key scientific principles that need to be met to ensure compliance with good laboratory standards resulting in optimal reliability of the PGx assay.
Moreover, in the USA, the National Human Genome Research Institute encourages research conducted on health benefits and cost-effectiveness of genetic testing to promote genomic medicine (Vozikis et al., 2016). It also supports payers to enable reimbursement of genetic tests. On the other hand, in Europe, there are national regulatory bodies for each country, such as the Gemeinsamer Bundesausschuss in Germany, the Medicines and Healthcare Regulatory Agency in the United Kingdom, and La Haute Autorité de Santé in France (Vozikis et al., 2016). Depending on specific national regulations, they are responsible for authorizing, marketing, and/or monitoring the quality and safety of medicinal products. Their role is tuned by the government to ensure cooperation between various stakeholders -regulatory authorities, medical device manufacturers, payer organizations, academic/research institutes, wholesalers, laboratories, pharmaceutical companies, and HCPs-to ensure availability and affordability of medical supplies including genetic tests. Tests that were shown to improve healthcare were proposed to be included in a “positive medical device” list to enforce its use and reimbursement by public and private insurance companies (Vozikis et al., 2016).
2.2 Hospital leadership
The leadership group is the initial sponsor of the program. It is responsible for securing funds and infrastructure, ensuring compliance with ethical legal social issues (ELSI), and monitoring and evaluating the program’s impact. The leadership is fully engaged in the whole process and should be sensitive to the hospital culture and climate, including the readiness for change. It should identify early adopters of change or implementation champions (Tuteja et al., 2022). It may also present evidence to national officials to suggest amending regulations in favor of promoting the practice of personalized medicine, and developing reimbursement policies and educational programs (Hartzler et al., 2013; Cicali et al., 2022).
2.3 Developers: pharmacy and therapeutics committee, laboratory and information technology
Then comes the role of the program developers being the P&T committee in collaboration with the hospital’s laboratory and IT department. The P&T is a multidisciplinary committee that is responsible for all matters related to the use of medications in the institution, including the development and maintenance of the hospital formulary. For the sake of the proposed PGx program, we suggest the P&T committee, while in constant communication with expert PGx consortia and networks, to be responsible for evaluating the available PGx evidence, reviewing the most relevant drugs, and narrowing down to the most actionable drug-gene pairs with related variant alleles to be tested. P&T members also discuss and decide with laboratory experts whether to apply reactive point of care or preemptive testing and on the genotyping strategy being a candidate or panel testing. The P&T committee also collaborates with IT to find the best way to report and interpret PGx test results with the option of integration into electronic health records (EHRs) coupled with clinical decision support (CDS) systems. It follows the program’s progress for improvement (Hartzler et al., 2013; Cicali et al., 2022).
2.4 Users: healthcare providers and patients
After that comes the role of the program users, being HCPs and patients. HCPs order the PGx test paired with the drug they plan to prescribe for a specific therapeutic need, interpret test results, communicate with patients, prescribe the personalized dose, or choose an alternative medication as applicable. HCPs also have an essential role in educating the community and patients on PGx and how it can impact their treatment. They can help monitor the general attitude toward PGx implementation and propose strategies to increase PGx awareness. These include educational workshops and conferences, TV and social media talks, and billboard and brochure advertisements that introduce the program to the public. HCPs also must provide feedback to the P&T, the molecular laboratory, and IT personnel regarding the process of including CDS in order to enhance the efficiency and efficacy of the implemented system (Hartzler et al., 2013; Cicali et al., 2022). Patients’ attitudes towards the PGx testing should also be taken into consideration. They should be informed about regulations that protect them from genetic discrimination by insurance companies and employers. They also should be informed regarding reimbursement policies and whether testing is entirely, partially, or not covered (Hartzler et al., 2013; Cicali et al., 2022).
2.5 Payers
Finally comes the role of payers, which may be public or private health insurance plans, research grants, laboratory reimbursement plans, out-of-pocket, or others. Although many potential payers are still reluctant to reimburse the PGx implementation or test, the growing evidence on the clinical utility of PGx testing is pushing toward fulfilling the right of patients to receive individualized treatment and to be protected by public policies and regulations that are integrated into national public or private health plans (Tuteja et al., 2022). Establishing or updating well-defined regulations will ultimately force insurance companies to revise their coverage plans to enable PGx testing. A success story is the experience of genotyping for DPYD variant alleles upon fluoropyrimidines prescribing whereby the resulting clinical and economic benefits led to securing governmental financial support in Ontario, with further evidence of cost-effectiveness probably leading to expansion of the experience to other medical institutions (Brooks et al., 2022; Medwid and Kim, 2022; Varughese et al., 2022). Moreover, the EMA recommended testing for DPYD, but concerns regarding the economic benefit and cost-effectiveness of testing resulted in slow adoption of the recommendation. Thus, studies were initiated in some countries to address cost-effectiveness and potential for improvement of quality of life as a result of DPYD genotyping prior to fluoropyrimidine-based chemotherapy (Deenen et al., 2016; Brooks et al., 2022). One study showed that DPYD screening is a cost-effective strategy and improves survival by 0.0038 quality adjusted life years (Brooks et al., 2022). Another study showed that genotype-guided dosing reduces grade 3 and above toxicity from 73% to 28%, drug-induced death from 10% to 0%, and average treatment cost per patient (Deenen et al., 2016). The EMA recommendation resulted in a requirement for DPYD genotyping in the UK (Tsiachristas et al., 2022). Also, guidelines for DPYD testing have been issued in other European countries such as The Netherlands, Italy, Germany and France (Tsiachristas et al., 2022) with a mandatory character.
3 Design of clinical pgx in the hospital setting
As shown in Figures 1, 2, designing a clinical PGx program in the hospital setting entails several steps. The P&T committee should evaluate the available PGx evidence, review the most relevant drugs, and narrow down to the most actionable drug-gene pairs and related variant alleles based on data curated and evaluated by experts and drug regulatory authorities. The next step is to decide with the molecular laboratory on the genotyping strategies and methods. Then is to work out with the IT team the best way to interpret and report PGx test results, ideally into patients’ EHRs if available, and with CDS systems if feasible.
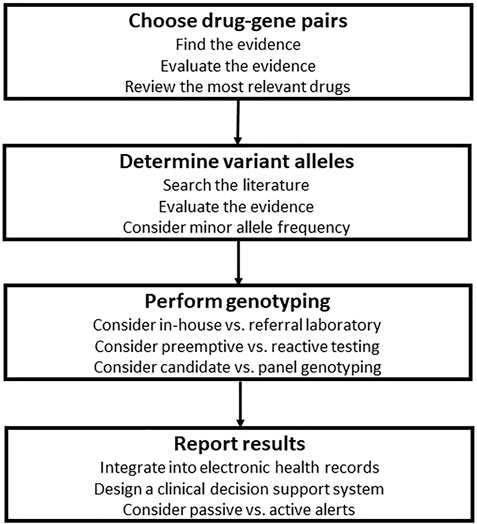
FIGURE 2. Steps for the design of clinical pharmacogenomics (PGx) in the hospital setting. The first step is to evaluate the available PGx evidence, review the most relevant drugs, and narrow down to the most actionable drug-gene pairs, then is to choose variant alleles based on population specific minor allele frequencies and data curated and evaluated by experts and drug regulatory authorities. Next is to decide with the molecular laboratory on the genotyping strategies and methods. Then is to work out with the information technology team the best way to interpret and report PGx test results, ideally into patients’ electronic health records if available, and with clinical decision support systems if feasible.
3.1 Choosing the top drug-gene pairs
In order to choose the top drug-gene pairs to be initially implemented, the P&T committee should first consult external expert sources such as PGx consortia and networks to find and evaluate the most substantial evidence for PGx testing. Then, the list of drugs and related genes can be narrowed down based on the reviewed evidence.
3.1.1 Finding the evidence
The implementation of PGx programs requires high-quality and consistent evidence that can be translated into regulations and guidelines (Luzum et al., 2021). These can be compiled from available PGx resources such as consortia, networks, societies, and regulatory agencies.
Several consortia and networks, some of which are listed and described in Table 1, were launched in the attempt to increase awareness, facilitate adoption, and provide the guidance necessary for integration of PGx programs into clinical practice. The Pharmacogenomics Global Research Network (PGRN) (Pharmacogenomics Research Network PGRN, 1998) is one of the first professional communities to work on PGx implementation. It has been heading several projects to include the recruitment and genotyping of people as part of a research protocol for the evaluation of the utility of the PGx endeavor on drug response. In addition, and as part of the Electronic Medical Records and Genomics Network (e-MERGE) (Electronic Medical Records and Genomics Network e-MERGE, 2007), the PGRN has been working on and proposing ways to upgrade EHR systems to be compatible with genetic results storage, as well as designing CDS for drug-gene pairs to guide HCPs in test ordering, interpretation, and drug prescription. Similarly, the Implementing Genomics in Practice (IGNITE) (Implementing Genomics in Practice IGNITE, 2013) network provides guidance for genomic implementation in healthcare, and provides a guiding toolbox for clinicians. Moving forward, professional PGx communities and programs progressed to provide improved PGx implementation models, clinical utility evidence, and comprehensive resources. All these efforts produced a set of valuable databases and tools that allow getting information on the drug and genes affecting its response, such as with the Pharmacogenomics Knowledge Base (PharmGKB) (Pharmacogenomics Knowledge Base PharmGKB, 2001; Whirl-Carrillo et al., 2012; Whirl-Carrillo et al., 2021), the genes, variants, frequencies and their phenotype with the Pharmacogene Variation Consortium (PharmVar) (Pharmacogene Variation Consortium PharmVar, 2000; Gaedigk et al., 2018; Gaedigk et al., 2020; Gaedigk et al., 2021), recommendations on what genetic variants should be tested to get interpretable results by the Association of Molecular Pathology (AMP) (Association of Molecular Pathology AMP, 1995), templates for creating genotyping result reports by the Pharmacogenomics Clinical Annotation Tool (PharmCat) (Sangkuhl et al., 2020; Pharmacogenomics Clinical Annotation Tool PharmCat, 2022), and recommendations on what to do when a PGx drug is prescribed by the Clinical Pharmacogenetics Implementation Consortium (CPIC) (Clinical pharmacogenetics implemetation Consortium CPIC, 2009).
In addition to the above-described CPIC, few other professional societies have established guidelines for PGx practice (Pharmacogenomics Knowledge Base PharmGKB, 2017), including the DPWG (The Dutch Pharmacogenetics Working Group DPWG, 2005), the Canadian Pharmacogenomics Network for Drug Safety (CPNDS) (Canadian Pharmacogenomics Network for Drug Safety CPNDS, 2004) and the French National Network of Pharmacogenetics (RNPGx) (Picard et al., 2017), among others. In addition, drug regulatory agencies (Pharmacogenomics Knowledge Base PharmGKB, 2017) including the EMA (European Medicinal Agency EMA, 1995) and the US FDA, have incorporated PGx information and prescribing tags in the approved drug labels, with the FDA allocating a specific and regularly updated link to all approved drugs with PGx label annotations (Mehta et al., 2020; United States Food and Drug Administration, 2022a).
3.1.2 Evaluating the evidence
The P&T may choose to build on guidelines or regulations established in one’s country if available. For many countries, however, such regulations are not available, institutions would hence have to compare and contrast various resources and choose what is most applicable to their local context. The Office of Public Health Genomics at the US Center for Disease Control and Prevention (CDC) proposes a model for the evaluation and integration of genomic tests based on four components, A, C, C, and E, with A being analytical validity that addresses the accuracy and reliability of genetic testing, C being clinical validity that looks at the accuracy and reliability by which the test predicts the associated drug outcome, C being the clinical utility as the risks and benefits resulting from introducing genetic tests into clinical practice on the community, and E for ELSI being the associated ethical and regulatory policies (Centers for Disease Control and Prevention, 2000).
Similar frameworks have been applied by the FDA and EMA regulatory authorities and the PharmGKB to come up with evidence-based annotation levels, and the CPIC for their implementation guidelines. All provide recommendations on whether to use a drug, adjust the dose or switch to an alternative based on rigorously evaluated evidence. The PharmGKB curates and analyzes available studies to provide annotations for drugs and gene variants, while assigning a level of evidence based on an elaborate scoring system that depends on two main factors. First, the variant annotation score is calculated by a stepwise process that considers all aspects of the evaluated studies including phenotype category, p-value, cohort and effect size, study type and the presence of a significant association. Second is the presence of a clinical guideline and or a drug label. Level of evidence for drug-gene variants ranges from 1 to 4, with 1A being supported by solid and non-conflicting data, while pairs assigned a level of evidence of 4 lack supporting data (Whirl-Carrillo et al., 2012; Whirl-Carrillo et al., 2021). As for CPIC, A, B, C, and D levels are designated such that A and B imply that evidence favors changing the drug prescription to a genetically safer one. In contrast, C and D imply that evidence did not reach a level to suggest a genetically-based prescription. CPIC also applies a framework to rank its recommendations as strong, moderate, or optional based on supporting studies such as randomized clinical trials (RCTs) and in vivo PK/PD studies (Caudle et al., 2016).
3.1.3 Narrowing down to a list of top drug-gene pairs
We propose that institutions that do not have country-specific guidelines or regulations compile all available PGx data from the PharmGKB, CPIC, FDA, and EMA. See Supplementary Table S1 as an example. We tabulated all drugs that are either listed in the PharmGKB’s Drug Label Annotations table under FDA or the Clinical Guideline Annotations table under CPIC. We also added the PharmGKB level of evidence for clinical annotations and all CPIC recommendations, and FDA and EMA PGx levels and drug labels when available. Of note that some discordance can be noted among the various resources despite being derived from the same evidence base (Koutsilieri et al., 2020; Shekhani et al., 2020; United States Food and Drug Administration, 2022b; Pirmohamed, 2023). For instance, many of the drugs labeled as “testing required” by the FDA are not mentioned in EMA labels. Moreover, many drug-gene associations listed by the FDA are neither listed by EMA nor by CPIC (See Supplementary Table S1). More specifically for clopidogrel prescription, for example, CPIC (Scott et al., 2013) and FDA (United States Food and Drug Administration, 1997) recommend the use -or consideration of the use-of alternative drugs in CYP2C19 poor metabolizers. At the same time, the EMA label (European Medicines Agency, 1998) does not make such a specific recommendation. Hence the role of the P&T committee to assess these inconsistencies and to make an informed decision on what model to follow and what drugs to include.
Two methods can be applied concomitantly or independently to narrow down the list of drug-gene pairs to be initially implemented. First, one can evaluate and choose the most frequently prescribed drugs in one’s setting. For example, a program initiated in Africa should include drugs like chloroquine, HIV-protease inhibitors, and isoniazid used to treat malaria, HIV, and tuberculosis, respectively (Greenwood, 2004; Marais et al., 2013; Dandara et al., 2019), while recognizing that non-communicable diseases are also an important cause of morbidity and mortality in developing countries (Grant and De Cock, 1998; Kennedy et al., 2007; Dean et al., 2020). If such data are unavailable, one can refer to the World Health Organization (WHO) list of essential drugs (World Health Organization WHO, 2021). The second method is to review available literature in other institutions or countries on the most commonly prescribed drugs supported by solid evidence of clinical utility for PGx. Several studies were conducted on multiple populations (Schildcrout et al., 2012; Samwald et al., 2016; Caraballo et al., 2017; Chanfreau-Coffinier et al., 2019; Hicks et al., 2021). Samwald et al. (2016) classified the most frequently used PGx drugs in the USA within different age groups based on a model whereby PGx drug exposure data were collected from insurance databases, the drugs that had CPIC or DWPG guidelines were then highlighted, followed by the selection of the most frequently used drugs, while considering different ethnic groups. This analysis showed that opioids (codeine, oxycodone, and please inset here: groups. This analysis showed that opioids (codeine, oxycodone, and tramadol) were primarily used in the younger population, while cardiovascular drugs (simvastatin, clopidogrel, and warfarin) were frequently prescribed for older people (Samwald et al., 2016; Hicks et al., 2021). Similar results appeared from a survey conducted by IGNITE group with 11 healthcare systems, whereby each site provided the available e-prescription records of adults above 18 years for in and outpatient settings (Hicks et al., 2021). A third study conducted at Vanderbilt University Medical Center reached a similar conclusion, adding some oncology drugs to the top 25 PGx most prescribed drugs (Schildcrout et al., 2012). In this study, drugs having FDA PGx prescribing information were considered. Consequently, a list of drugs was suggested by several investigators as ready to be implemented. This list includes but is not limited to: statins, clopidogrel, and warfarin for cardiovascular diseases, codeine and tramadol as opioid analgesics, ondansetron and proton pump inhibitors for gastrointestinal illnesses, fluoropyrimidines, tamoxifen, and thiopurines for cancer, and antidepressants such as the selective serotonin reuptake inhibitors (Weitzel et al., 2019; Rollinson et al., 2020; Medwid and Kim, 2022). Finally, a fourth study evaluated the longitudinal exposure in primary care in the United Kingdom of a list of 63 drugs identified from the PharmGKB database to be associated with 19 pharmacogenes. The authors showed that most of the prescribed PGx drugs were for pain relief, gastrointestinal protection, and psychiatric and cardiovascular conditions, and that more than 95% of these drugs are affected by three pharmacogenes: CYP2C19, CYP2D6, and SLCO1B1 (Kimpton et al., 2019).
Based on these two methods, we narrowed down the list of 499 drugs from Supplementary Table S1 into 59 drugs with related pharmacogenes (Table 2). We limited the list to drugs associated with germline non-somatic PGx tests. As such, we did not include genes for targeted anticancer drugs or immunotherapy. We also checked the Tier 1 Very Important Pharmacogenes (VIP) list from PharmGKB (Pharmacogenomics Knowledge Base PharmGKB, 2020), and kept some drug-gene pairs such as TPMT and NUDT15 for thioguanine despite being non-level 1A per PharmGKB level of evidence. We chose so for the mere fact that both VIP are also associated with more substantial evidence with other drugs such as mercaptopurine and azathioprine. We excluded VIP for drugs not commonly used in the community worldwide such as CFTR, F5, and RYR1 related to cystic fibrosis treatment, thrombopoeitin receptor agonists, and anesthetic drugs, respectively. We also did not include NAT2 for isoniazid as the drug-gene pair has so far not been addressed by the CPIC.
3.2 Choosing the variants to be tested
After determining the drug-gene pairs, the P&T committee should specify the variants that should be tested in coordination with Laboratory experts and personnel. We describe in Table 3 a list of variants associated with the genes proposed in Table 2. This list is non-exhaustive and laid out for illustrative purposes only. It is based on the most commonly proposed variants and genes in the literature (Samwald et al., 2016; van der Wouden et al., 2017; Chanfreau-Coffinier et al., 2019; van der Wouden et al., 2019; Rollinson et al., 2020; Medwid and Kim, 2022) and supported with high quality evidence, clinical guidelines and/or drug labels with genetic information. We also chose variants that are relatively common in Africans, Asians or Europeans. Each genetic variant has a unique identifier (rsID) as per the NCBI dbSNP database (National Center for Biotechnology Information NCBI, 1999). The *allele nomenclature and genotype-phenotype relation can be extracted from the PharmGKB and/or PharmVar resources.
The most crucial consideration for the choice of the target variants is the Minor Allele Frequency (MAF) of the local population or ethnicities (Van Driest et al., 2014). For instance, while in East Asians, HLA-B*15:02 allele is relatively common, and its PGx testing for carbamazepine is required to prevent Stevens–Johnson syndrome (SJS) and toxic epidermal necrolysis (TEN), the same test is not required in other populations where the allele is quite rare (United States Food and Drug Administration, 2009). Another example is mercaptopurine, whereby only TPMT genotyping is recommended in Caucasians, while both TPMT and NUDT15 genotyping should be performed for East Asians who may carry some common actionable NUDT15 variants (Yang et al., 2015). A third example comprises CYP2C9 genotyping test before prescribing warfarin to African Americans, whereby the test should include CYP2C9 *2, *3, *5, *6, *8, and *11 versus only *2 and *3 for Europeans (Johnson et al., 2017). Another important consideration to be kept in mind is how well the variants reflect the phenotype of the enzyme activity. For this purpose, the AMP guides on the basic variants that should be tested together to allow accurate interpretation of the gene’s phenotype. For example, at least the *2, *3, and *17 should be included when genotyping for CYP2C19, while other additional variants are optional. This classification was based on the prevalence of these variants in different ethnic groups and their functional effect on enzyme activity and drug response (Pratt et al., 2018). Of note that one has to constantly review the literature for emergent variants or haplotypes such as the common CYP2C:TG haplotype, defined by rs2860840T and rs11188059G co-occurrence, that is associated with CYP2C19 increased enzyme activity, hence affecting metabolism of drugs such as sertraline and escitalopram (Braten et al., 2021; Braten et al., 2022).
3.3 Specifying genotyping strategies and methods
Herein, two strategic decisions should be made by the P&T committee in coordination with the molecular laboratory. The first decision is whether to genotype for the selected drug-gene pairs preemptively or reactively. The second is whether to test for one or only a few candidate gene variants or perform more extensive panel genotyping. The points to be considered include, in addition to funds or reimbursement matters, availability of in-house or reference laboratories, IT expertise, EHR interfaces capable of holding and interpreting genetic test results, and well-trained HCPs able to deal with genetic data.
3.3.1 Laboratory considerations
The medical institution may either establish, already have a certified genotyping laboratory, or refer to an outside reference laboratory to perform the PGx test. Such decisions mainly depend on availability of expertise, resources and funds as well as the extent of demand for PGx testing. The Genetic Testing Registry website (National Center for Biotechnology Information NCBI, 2012; Rubinstein et al., 2013) presents information on already available genetic and PGx tests in the United States. Also, the National genomic test directory (National genomic test directory, 2022) provides genomic tests commissioned by the National Health Services in the United Kingdom. One can search for a specific gene of interest and get a list of tests with details on purpose and coverage, validity, genotyping methodology, associated evidence for effectiveness, and contact laboratories with credentials.
Regardless of whether the lab is in-house or contracted, four primary standards must be considered (Vo et al., 2017). First, and as noted above, the pharmacogene(s)’ selection should be relevant to the tested population, and feasible with the available technology being a candidate or panel genotyping. Second, there must be documented evidence of good laboratory practices such as the College of American Pathologists accreditation and Clinical Laboratory Improvement Amendments certification for the USA, the European co-operation for Accreditation for Europe (European co-operation for Accreditation, 2022), and the International Laboratory Accreditation Cooperation for the international level (International Laboratory Accreditation Cooperation, 2022), to ensure accuracy, reproducibility, sensitivity, and specificity of the assay performed with reference and reportable ranges (Bristol, 2002; Endrullat et al., 2016). Third, the format of lab reports should be designed to include simple stand-alone gene results with or without interpretative comments. These are integrated into the EHR or an online portal, if available, or simple paper reports. Finally, it is important to negotiate with reference laboratories outside the institution on cost or reimbursement models with the possibility for financial assistance or partnerships as applicable (Tuteja et al., 2022). In the USA, genetic testing companies may facilitate the process through contracting payers to reimburse patients who are required to undergo the test, or making special discounts for out-of-pocket payers. In addition, the growing body of genetic testing companies increases the competition among them leading to better offers for the consumer (Wolff and Wolff, 2018). In Europe, pricing policies are developed to restrict manufacturers’ power in controlling genetic testing prices to ensure availability and protect consumers from exaggerated charges (Vozikis et al., 2016).
3.3.2 Preemptive versus reactive testing
Genes are stable, and genetic data remain unchanged with time; hence it is a practical call to genotype high-risk gene variants and store them in EHRs before a PGx drug is needed. This preemptive genetic testing approach saves critical time and allows HCPs to prescribe PGx medications directly when required. This approach protects the patient from trial and error with unwanted ADRs (Roden et al., 2018). Nevertheless, this strategy comes at a higher cost, necessitates complex technologies and EHR integration, and is typically not reimbursed as payers may not see the value of the test at the time of the request (Keeling et al., 2019; Haidar et al., 2022a). The other approach, the reactive point of care testing, is to order the genotyping test when a PGx drug is to be prescribed. Although less costly, this approach may not be timely since a drug prescription cannot be postponed in many cases, and the patient may suffer ADRs due to the wrong dosage or drug choice until the results are out (Nicholson et al., 2021; Haidar et al., 2022a). It is up to the institution to decide on which strategy is most suitable depending on funding and feasibility in the local context.
3.3.3 Candidate versus panel genotyping
As noted above, the medical institution may perform the planned PGx test in-house or refer to an outer reference laboratory. It should also strategize on choosing the candidate instead of panel genetic testing. Conversely, candidate genetic tests cover one or few specific gene variants related to a particular PK or PD pathway. The advantage of this type of test is that it is more accessible, less time-consuming, and less challenging to perform and interpret. It is mostly Polymerase Chain Reaction (PCR)-based or performed on small microarrays (Krebs and Milani, 2019; van der Lee et al., 2020; Verlouw et al., 2021). On the other hand, panel and genome-wide genotyping allow coverage of a more significant number of variants (Krebs and Milani, 2019; van der Lee et al., 2020; Verlouw et al., 2021). These tests may be ordered in patients taking multiple PGx actionable drugs or suspected to be prescribed various drugs based on age, comorbidities, or family history. This method is considered cost-effective since several genes can be genotyped together as one pool which decreases the cost per gene. For example, one may choose a panel of variants for drug transporters and CYP enzymes for a patient suffering from hypercholesterolemia to decide on the actionable rosuvastatin prescription. Since hypercholesterolemia is a risk factor for myocardial infarction, the panel can also cover variants for genes involved in the PK pathway of other cardiovascular drugs such as the antiplatelet clopidogrel. Panel tests are more challenging to deal with due to the large amounts of data they generate. In addition, this approach may not be practical in cases where the patient requires imminent treatment. Note that although panel testing includes many variants, one can decide to report only those associated with the prescribed drugs into the EHR. At the same time, the remaining results are stored in a separate database. This approach decreases the amount of data the HCP has to deal with, but at the same time, the data are readily available to be dispatched when a new PGx drug is added.
3.4 Reporting of results
Ideally, genotyping results should be reported in the patients’ EHR. In case EHRs are available but are not compliant with genetic data, the IT team should upgrade the system to have the necessary features to receive such data. Yet, paper reports may also be considered (Cicali et al., 2022). Another important consideration is the language used to report genotyping data. For example, a report for CYP2C19 may state the result, such as that the genotype is CYP2C19*2, or may describe all evaluated genetic variants. The reporting discrepancies may lead to confusion in interpreting results from different laboratories. With the aim of standardising terms for allele functional status and inferred phenotype for the CPIC guidelines, Caudle et al. (2017) surveyed experts with diverse involvement in at least one area of PGx, and agreed on the following consensus terms: increased, normal, decreased, no, unknown, and uncertain function for allele functional status of all genes; ultrarapid, rapid, normal, intermediate, and poor metabolizer for drug-metabolizing enzymes; increased, normal, decreased, and poor function for the phenotype of transporters; and positive or negative for high-risk genotype status such as for HLA-B. It is hence advisable to include an interpretation of the phenotype associated with the resultant genotype, coupled with a recommendation on what to do next with the drug to be prescribed using language from CPIC or other guidelines. In addition, and if possible, CDS systems should be incorporated to guide the HCP into an informed decision based on genetic data (Caraballo et al., 2017; O'Donnell et al., 2014).
CDS systems can be passive or active. The first generates alerts that are stored in the patient’s EHR for use when needed, while the latter spontaneously delivers alerts pre- and/or post-PGx testing (Hicks et al., 2016; Haidar et al., 2022a). Passive CDS systems require the HCP to remember at the point of care to look for the potential drug-gene interaction, and find the PGx test results in the EHR if available (Haidar et al., 2022a). For active CDS systems, the pre- PGx test alert is triggered when an HCP prescribes an actionable drug at the point of care, and the patient lacks previous genetic test results (Bell et al., 2014). The alert is either displayed directly on the computer screen or by email to direct the HCP to order a genotyping test (Bell et al., 2014). The post- PGx test alert is triggered when a HCP prescribes an actionable drug that coincides with already available high-risk gene data (Bell et al., 2014; Haidar et al., 2022a). The alert provides phenotype interpretation, possible drug-gene interaction, if any, and recommended actions, such as changing the drug or dose, or monitoring (Bell et al., 2014; Haidar et al., 2022a).
4 Recommendations for the further enhancement of pgx implementation in clinical practice
Some strategies should be put in place to frequently evaluate emerging evidence, continuously audit and evaluate the progress and performance of the program, and integrate research for ethical, legal and social issues (Pirmohamed, 2023). In addition, there is a need to educate HCPs and patients, and to push for more tests’ reimbursement as detailed below.
4.1 Education
HCPs and patients are on the receiving end of the clinical PGx implementation process. HCPs, be they physicians or pharmacists, are responsible for the return of results to patients. HCPs and patients are both accountable for reporting back on the outcome of the PGx-guided prescription. They need education to enhance their use and understanding of the whole practice.
4.1.1 Healthcare providers
Survey data have consistently shown that HCPs, despite being generally aware of the importance of PGx and having a positive attitude towards PGx’s ability to improve drug therapy and reduce side effects, few have ordered or recommended PGx testing (Stanek et al., 2012; Abou Diwan et al., 2019; Algahtani, 2020). This lag in PGx clinical implementation is primarily related to the lack of formal education about PGx testing in medical school and postgraduate studies (Stanek et al., 2012; Algahtani, 2020). For instance, a survey among HCPs on PGx education in Europe showed that 83.3% of the participants still lack PGx expertise (Just et al., 2017). Another global survey study was recently done to evaluate the current status of PGx education in medical and pharmacy study programs (Karas Kuzelicki et al., 2019). Results showed that 13.4% had no PGx education among the recruited participants, 19.6% took PGx as an independent elective, and only 10.3% had PGx as a mandatory subject. These results were congruent with survey data collected over a decade ago (2005) and led to the recommendation that PGx should be taught as an integral part of pharmacology curricula (Gurwitz et al., 2005; Karas Kuzelicki et al., 2019). These recommendations are crucial, knowing that education and training increase physicians’ confidence to request PGx tests or use such test results if already available before prescribing drugs (Luzum and Luzum, 2016).
Undergraduate education that considers PGx as foundational content can hence contribute to the education of HCP graduates for the integration of PGx into clinical care. Also, training during fellowships or residencies, graduate and postgraduate programs, or certificates can address the PGx knowledge gap. As such, accreditation standards have been developed by the American Society of Health-System Pharmacists for postgraduate year two residence pharmacists to include clinical PGx training (Haidar et al., 2022b).
Besides, continuous education activities are essential to staying up to date. These include just in-time education such as active CDS systems, and PGx programs that provide on-site services, dedicated webpages, and messages to the clinician’s inbox (Freimuth et al., 2017; Williams, 2019). Also, one can build on the already available educational resources, such as those by CPIC. In addition, the National Human Genome Research Institute has supported an Inter-Society Coordinating Committee to develop educational resources aiming to improve the PGx education of HCPs (National Human Genome Research Institute, 2021; Haidar et al., 2022a).
4.1.2 Patients
Patients’ awareness and education are essential drivers for the success of PGx implementation. Available studies suggest that patients have a positive attitude toward PGx implementation and believe in its ability to predict the correct dose, medication efficacy, mild or serious side effects, and explain the family history of medication toxicity (Nielsen and Moldrup, 2007). The general public can also understand specific genetic terminology, yet people cannot comprehend underlying concepts and how this may affect their health (Lea et al., 2011; Haga et al., 2014).
To address this gap, PGx education may be provided through technological tools such as interactive webpages, educational videos, and telehealth sessions (Hoffman et al., 2014; Dunnenberger et al., 2015). Moreover, written reports should be user-friendly. They can include summaries of genetic results in a tabular or graphical format such as infographics (e.g., human icon drawn as running motion representing rapid metabolizer) and icon arrays (e.g., shaded figures reflecting the proportion of affected out of total) (Galesic et al., 2009; Sinayev et al., 2015). Also, simple drawings of pie charts, risk labels, and tri-color-coding systems for risk assessment (red, yellow/orange, and green for high, moderate, and average risk, respectively) (Haga, 2017) are examples of patient-friendly formats. These methods can generate more confidence in patients toward PGx implementation. Finally, adequate counseling should be provided through written reports or in-person contact by well-trained HCPs or genetic counselors if available (Haga, 2017; Haidar et al., 2022a).
4.2 Reimbursement considerations
The cost of PGx testing varies between companies and platforms, with the cost of single-gene tests ranging from 100$ to 500$, while the price of a multigene panel test may reach double that of the single-gene test (Anderson et al., 2020; Haidar et al., 2022a). Although single-gene test costs less, patients may require the prescription of several actionable drugs, whereby in this case, multigene panel testing becomes more cost-effective. Nevertheless, most payers are still reluctant to reimburse multigene panel tests due to the lack of evidence of clinical utility for preemptive panel testing (Keeling et al., 2019). Even though the clinical utility of reactive testing using single-gene tests is easier to obtain compared to preemptive multigene panel testing, its reimbursement is still a barrier (Haidar et al., 2022a).
However, reimbursement of PGx in the US may be forthcoming (Empey et al., 2021). Lately, local coverage determinations for the Molecular Diagnostic Services program were established based on earlier decisions made by payers (public and private). They expanded the coverage for some Medicare Administrative Contractors that cover molecular diagnostic tests (US Centers for Medicare and Medicaid Services, 2020). Accordingly, for Medicare patients, local coverage determinations are indicated for PGx tests related to medications that are medically necessary, appropriate, and approved for the patient’s condition and have clinically actionable drug-gene interaction defined by the FDA and CPIC (US Centers for Medicare and Medicaid Services, 2020). Moreover, payer reimbursement policies are evolving, and the availability of specific criteria for PGx testing may increase the probability of coverage (Keeling et al., 2019). For example, the American Medical Association has created several Current Procedure Terminology codes for single-gene PGx tests to detect specific gene variants that impact drug therapy. These codes result in more specific documentation that may increase the chance of PGx test coverage (Hefti and Blanco, 2016). Also, the establishment and demonstration of evidence that PGx improves clinical outcomes, and finding value for PGx testing, as reflected by helping HCPs to decide on therapy for a specific population, may increase the possibility of PGx test reimbursement (Weitzel et al., 2019).
In Europe, the reimbursement systems differ among individual countries (Payne and Annemans, 2013). For the sake of illustration in the Netherlands, all citizens should have a basic healthcare insurance that includes coverage for PGx tests that are ordered to explore causes of ADRs. Moreover, optional reimbursement packages for PGx screening are provided by some healthcare insurers (van der Wouden et al., 2020). In addition, The “G-standaard” Dutch drug database offers information, guidance and standards that are used by different parties in healthcare including health insurers to enhance the infrastructure for national testing programs (Thornley et al., 2021). Finally in England, the National Health Services Genomics Medicine Service that aims to provide genetic services equally among patients is leading innovative projects to allow integration of genetic testing into routine healthcare through supporting research, adequate planning, and reimbursement (Robinson, 2022). Interestingly, a system for reimbursement of PGx testing was suggested whereby it is proposed for medical authorities to develop a “positive medical device” list to control the cost in the market, and impose reimbursement by insurance companies (Vozikis et al., 2016).
Besides coverage by payers, some commercial laboratories provide reimbursement on their panel-based testing by income-based sliding scale payment method, patient assistance programs, or help the patient navigate the reimbursement process. Also, the PGx test can be initially covered or supplemented by institutional support or research funding (Luzum et al., 2017; Cicali et al., 2022).
5 Conclusion
This article proposed an approach to designing and implementing clinical PGx in the hospital setting. After test authorization or requirements for testing by the government or drug regulators, putting the plan into action involves several stakeholders, with the hospital leadership supporting the process and communicating with payers, the P&T committee leading the process in collaboration with the hospital laboratory and IT department, and HCPs ordering the test, understanding the results, making the appropriate therapeutic decisions, and explaining them to the patient. We concluded by recommending strategies to further advance the implementation of PGx in practice, such as the need to educate HCPs and patients and to push for more tests’ reimbursement. The reader can refer to Table 4 and learn from the experience of other institutions that have been implementing PGx for years for clinical and or research purposes and adapt some of their approaches concerning the choice of drug-gene pairs, genotyping strategies and methods, integration of results and reports, and educational practices. Several barriers and schemes should be considered before implementing clinical PGx on a big scale (Swift, 2022; Tuteja et al., 2022; Pirmohamed, 2023).
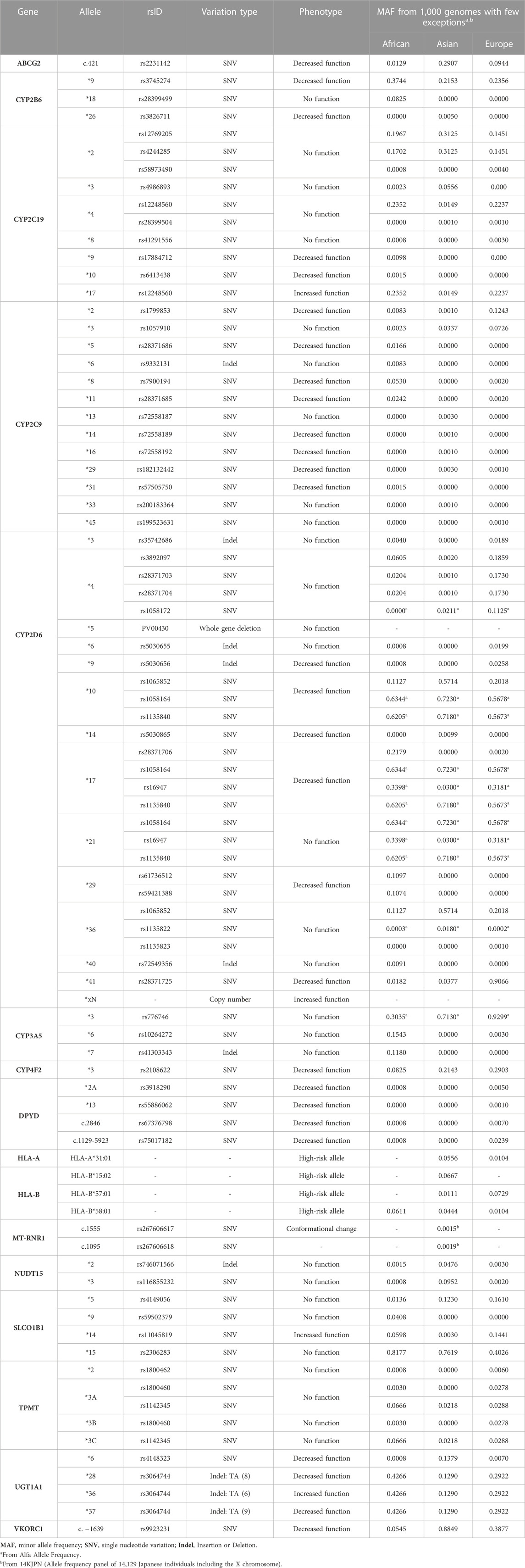
TABLE 3. Proposed non-exhaustive list and description of genetic allele variants for the clinical implementation of pharmacogenomics (PGx).
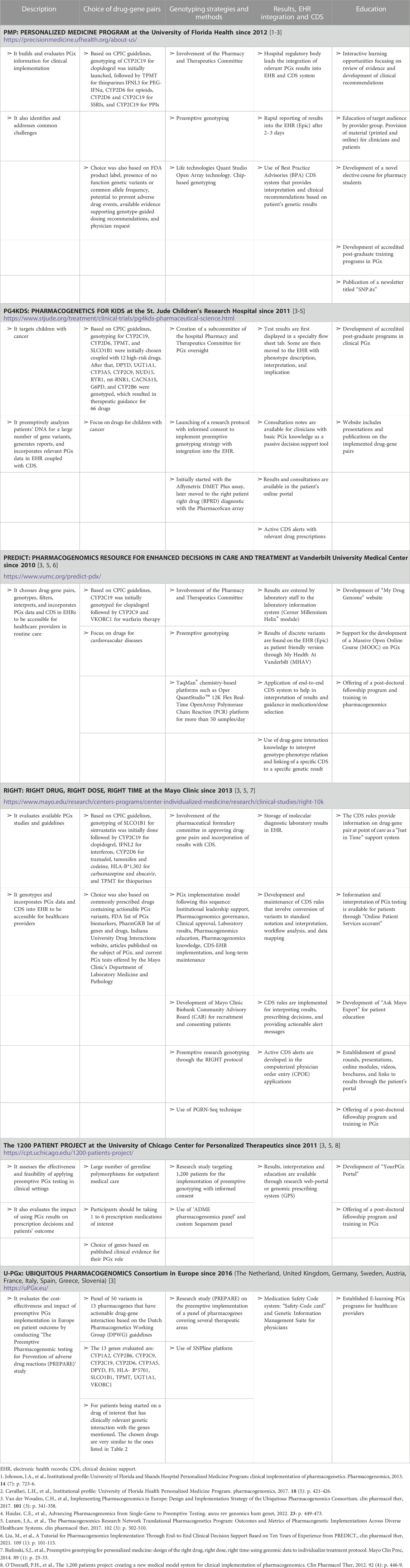
TABLE 4. List and description of few pharmacogenomic (PGx) clinical implementation programs and initiatives.
Author contributions
DK, RA and NKZ conceptualized and wrote the first draft of the manuscript. DK gathered and tabulated data from the guidelines and drug labels. AW, AD and IC commented on the concepts and content, and contributed to the writing. All authors approved the final version of the submitted work. All authors read and approved the final manuscript. All authors listed have made a substantial, direct, and intellectual contribution to the work and approved it for publication.
Funding
This work is funded by the ERASMUS + Programme of the European Union: 617303-EPP-1-2020-1-EG-EPPKA2-CBHE-JP. The European Union’s support for the production of this publication does not constitute an endorsement of the contents, which reflect the views only of the authors, and the Commission cannot be held responsible for any use which may be made of the information contained herein.
Conflict of interest
The authors declare that the research was conducted in the absence of any commercial or financial relationships that could be construed as a potential conflict of interest.
Publisher’s note
All claims expressed in this article are solely those of the authors and do not necessarily represent those of their affiliated organizations, or those of the publisher, the editors and the reviewers. Any product that may be evaluated in this article, or claim that may be made by its manufacturer, is not guaranteed or endorsed by the publisher.
Supplementary material
The Supplementary Material for this article can be found online at: https://www.frontiersin.org/articles/10.3389/fphar.2023.1189976/full#supplementary-material
References
Abou Diwan, E., Zeitoun, R. .I., Abou Haidar, L., Cascorbi, I., and Khoueiry Zgheib, N. (2019). Implementation and obstacles of pharmacogenetics in clinical practice: An international survey. Br. J. Clin. Pharmacol. 85 (9), 2076–2088. doi:10.1111/bcp.13999
Algahtani, M. (2020). Knowledge, perception, and application of pharmacogenomics among hospital pharmacists in Saudi arabia. Risk Manag. Healthc. Policy 13, 1279–1291. doi:10.2147/RMHP.S267492
Anderson, H. .D., Crooks, K. .R., Kao, D. .P., and Aquilante, C. .L. (2020). The landscape of pharmacogenetic testing in a US managed care population. Genet. Med. 22 (7), 1247–1253. doi:10.1038/s41436-020-0788-3
Association of Molecular Pathology (AMP) (1995). Association of molecular Pathology (AMP). Available at: https://www.amp.org/.
Bartlett, G., Antoun, J., and Zgheib, N. .K. (2012). Theranostics in primary care: Pharmacogenomics tests and beyond. Expert Rev. Mol. Diagn 12 (8), 841–855. doi:10.1586/erm.12.115
Bell, G. .C., Crews, K. .R., Wilkinson, M. .R., Haidar, C. .E., Hicks, J. .K., Baker, D. .K., et al. (2014). Development and use of active clinical decision support for preemptive pharmacogenomics. J. Am. Med. Inf. Assoc. 21 (1), e93–e99. doi:10.1136/amiajnl-2013-001993
Braten, L. .S., Haslemo, T., Jukic, M. .M., Ivanov, M., Ingelman-Sundberg, M., Molden, E., et al. (2021). A novel CYP2C-haplotype associated with ultrarapid metabolism of escitalopram. Clin. Pharmacol. Ther. 110 (3), 786–793. doi:10.1002/cpt.2233
Braten, L. .S., Ingelman-Sundberg, M., Jukic, M. .M., Molden, E., and Kringen, M. .K. (2022). Impact of the novel CYP2C:TG haplotype and CYP2B6 variants on sertraline exposure in a large patient population. Clin. Transl. Sci. 15 (9), 2135–2145. doi:10.1111/cts.13347
Bristol, L. .A. (2002). A regulatory protocol for pharmacogenomics services. Pharmacogenomics J. 2 (2), 83–86. doi:10.1038/sj.tpj.6500079
Brooks, G. .A., Tapp, S., Daly, A. .T., Busam, J. .A., and Tosteson, A. .N. .A. (2022). Cost-effectiveness of DPYD genotyping prior to fluoropyrimidine-based adjuvant chemotherapy for colon cancer. Clin. Colorectal Cancer 21 (3), e189–e195. doi:10.1016/j.clcc.2022.05.001
Canadian Pharmacogenomics Network for Drug Safety (CPNDS) (2004). Canadian pharmacogenomics network for drug safety (CPNDS). Available at: https://cpnds.ubc.ca/.
Caraballo, P. .J., Hodge, L. .S., Bielinski, S. .J., Stewart, A. .K., Farrugia, G., Schultz, C. .G., et al. (2017). Multidisciplinary model to implement pharmacogenomics at the point of care. Genet. Med. 19 (4), 421–429. doi:10.1038/gim.2016.120
Caudle, K. .E., Dunnenberger, H. .M., Freimuth, R. .R., Peterson, J. .F., Burlison, J. .D., Whirl-Carrillo, M., et al. (2017). Standardizing terms for clinical pharmacogenetic test results: Consensus terms from the clinical pharmacogenetics implementation Consortium (CPIC). Genet. Med. 19 (2), 215–223. doi:10.1038/gim.2016.87
Caudle, K. .E., Gammal, R. .S., Whirl-Carrillo, M., Hoffman, J. .M., Relling, M. .V., and Klein, T. .E. (2016). Evidence and resources to implement pharmacogenetic knowledge for precision medicine. Am. J. Health Syst. Pharm. 73 (23), 1977–1985. doi:10.2146/ajhp150977
Centers for Disease Control and Prevention (2000). ACCE model process for evaluating genetic tests. Available at: https://www.cdc.gov/genomics/gtesting/acce/index.htm.
Chanfreau-Coffinier, C., Hull, L. .E., Lynch, J. .A., DuVall, S. .L., Damrauer, S. .M., Cunningham, F. .E., et al. (2019). Projected prevalence of actionable pharmacogenetic variants and level A drugs prescribed among US veterans health administration pharmacy users. JAMA Netw. Open 2 (6), e195345. doi:10.1001/jamanetworkopen.2019.5345
Cicali, E. .J., Lemke, L., Al Alshaykh, H., Nguyen, K., Cavallari, L. .H., and Wiisanen, K. (2022). How to implement a pharmacogenetics service at your institution. J. Am. Coll. Clin. Pharm. 5 (11), 1161–1175. doi:10.1002/jac5.1699
Clinical pharmacogenetics implemetation Consortium (CPIC).(2009) Clinical pharmacogenetics implemetation Consortium (CPIC) Available at: https://cpicpgx.org/.
Dandara, C., Masimirembwa, C., Haffani, Y. .Z., Ogutu, B., Mabuka, J., Aklillu, E., et al. (2019). African Pharmacogenomics Consortium: Consolidating pharmacogenomics knowledge, capacity development and translation in Africa: Consolidating pharmacogenomics knowledge, capacity development and translation in Africa. AAS Open Res. 2, 19. doi:10.12688/aasopenres.12965.1
Dean, A. .S., Zignol, M., Cabibbe, A. .M., Falzon, D., Glaziou, P., Cirillo, D. .M., et al. (2020). Prevalence and genetic profiles of isoniazid resistance in tuberculosis patients: A multicountry analysis of cross-sectional data. PLoS Med. 17 (1), e1003008. doi:10.1371/journal.pmed.1003008
Deenen, M. .J., Meulendijks, D., Cats, A., Sechterberger, M. .K., Severens, J. .L., Boot, H., et al. (2016). Upfront genotyping of DPYD*2A to individualize fluoropyrimidine therapy: A safety and cost analysis. J. Clin. Oncol. 34 (3), 227–234. doi:10.1200/JCO.2015.63.1325
Dunnenberger, H. .M., Crews, K. .R., Hoffman, J. .M., Caudle, K. .E., Broeckel, U., Howard, S. .C., et al. (2015). Preemptive clinical pharmacogenetics implementation: Current programs in five US medical centers. Annu. Rev. Pharmacol. Toxicol. 55, 89–106. doi:10.1146/annurev-pharmtox-010814-124835
Ehmann, F., Caneva, L., and Papaluca, M. (2014). European Medicines Agency initiatives and perspectives on pharmacogenomics. Br. J. Clin. Pharmacol. 77 (4), 612–617. doi:10.1111/bcp.12319
El Shamieh, S., and Zgheib, N. .K. (2022). Pharmacogenetics in developing countries and low resource environments. Hum. Genet. 141 (6), 1159–1164. doi:10.1007/s00439-021-02260-9
Electronic Medical Records and Genomics Network (e-MERGE) (2007). Electronic medical records and genomics (eMERGE) network. Available at: https://www.genome.gov/Funded-Programs-Projects/Electronic-Medical-Records-and-Genomics-Network-eMERGE.
Empey, P. .E., Pratt, V. .M., Hoffman, J. .M., Caudle, K. .E., and Klein, T. .E. (2021). Expanding evidence leads to new pharmacogenomics payer coverage. Genet. Med. 23 (5), 830–832. doi:10.1038/s41436-021-01117-w
Endrullat, C., Glokler, J., Franke, P., and Frohme, M. (2016). Standardization and quality management in next-generation sequencing. Appl. Transl. Genom 10, 2–9. doi:10.1016/j.atg.2016.06.001
European co-operation for Accreditation (2022). European co-operation for Accreditation. Available at: https://european-accreditation.org/.
European Medicinal Agency (Ema), (1995). [Available from: https://www.ema.europa.eu/en.
European Medicines Agency (2010). Co-development of pharmacogenomic biomarkers and assays in the context of drug development - scientific guideline. Available at: https://www.ema.europa.eu/en/co-development-pharmacogenomic-biomarkers-assays-context-drug-development-scientific-guideline.
European Medicines Agency (1998). Plavix. Available at: https://www.ema.europa.eu/en/medicines/human/EPAR/plavix.
Freimuth, R. .R., Formea, C. .M., Hoffman, J. .M., Matey, E., Peterson, J. .F., and Boyce, R. .D. (2017). Implementing genomic clinical decision support for drug-based precision medicine. CPT Pharmacometrics Syst. Pharmacol. 6 (3), 153–155. doi:10.1002/psp4.12173
Gaedigk, A., Casey, S. .T., Whirl-Carrillo, M., Miller, N. .A., and Klein, T. .E. (2021). Pharmacogene variation Consortium: A global resource and repository for pharmacogene variation. Clin. Pharmacol. Ther. 110 (3), 542–545. doi:10.1002/cpt.2321
Gaedigk, A., Ingelman-Sundberg, M., Miller, N. .A., Leeder, J. .S., Whirl-Carrillo, M., Klein, T. .E., et al. (2018). The pharmacogene variation (PharmVar) Consortium: Incorporation of the human cytochrome P450 (CYP) allele nomenclature database. Clin. Pharmacol. Ther. 103 (3), 399–401. doi:10.1002/cpt.910
Gaedigk, A., Whirl-Carrillo, M., Pratt, V. .M., Miller, N. .A., and Klein, T. .E. (2020). PharmVar and the landscape of pharmacogenetic resources. Clin. Pharmacol. Ther. 107 (1), 43–46. doi:10.1002/cpt.1654
Galesic, M., Garcia-Retamero, R., and Gigerenzer, G. (2009). Using icon arrays to communicate medical risks: Overcoming low numeracy. Health Psychol. 28 (2), 210–216. doi:10.1037/a0014474
Grant, A. .D., and De Cock, K. .M. (1998). The growing challenge of HIV/AIDS in developing countries. Br. Med. Bull. 54 (2), 369–381. doi:10.1093/oxfordjournals.bmb.a011694
Greenwood, B. (2004). Treating malaria in Africa. BMJ 328 (7439), 534–535. doi:10.1136/bmj.328.7439.534
Gurwitz, D., Lunshof, J. .E., Dedoussis, G., Flordellis, C. .S., Fuhr, U., Kirchheiner, J., et al. (2005). Pharmacogenomics education: International Society of Pharmacogenomics recommendations for medical, pharmaceutical, and health schools deans of education. Pharmacogenomics J. 5 (4), 221–225. doi:10.1038/sj.tpj.6500312
Haga, S. .B. (2017). Educating patients and providers through comprehensive pharmacogenetic test reports. Pharmacogenomics 18 (11), 1047–1050. doi:10.2217/pgs-2017-0088
Haga, S. .B., Mills, R., and Bosworth, H. (2014). Striking a balance in communicating pharmacogenetic test results: Promoting comprehension and minimizing adverse psychological and behavioral response. Patient Educ. Couns. 97 (1), 10–15. doi:10.1016/j.pec.2014.06.007
Haidar, C. .E., Crews, K. .R., Hoffman, J. .M., Relling, M. .V., and Caudle, K. .E. (2022). Advancing pharmacogenomics from single-gene to preemptive testing. Annu. Rev. Genomics Hum. Genet. 23, 449–473. doi:10.1146/annurev-genom-111621-102737
Haidar, C. .E., Petry, N., Oxencis, C., Douglas, J. .S., and Hoffman, J. .M. (2022). ASHP statement on the pharmacist's role in clinical pharmacogenomics. Am. J. Health Syst. Pharm. 79 (8), 704–707. doi:10.1093/ajhp/zxab339
Hartzler, A., McCarty, C. .A., Rasmussen, L. .V., Williams, M. .S., Brilliant, M., Bowton, E. .A., et al. (2013). Stakeholder engagement: A key component of integrating genomic information into electronic health records. Genet. Med. 15 (10), 792–801. doi:10.1038/gim.2013.127
Hefti, E., and Blanco, J. .G. (2016). Documenting pharmacogenomic testing with CPT codes. J. AHIMA 87 (1), 56–59.
Hicks, J. .K., Dunnenberger, H. .M., Gumpper, K. .F., Haidar, C. .E., and Hoffman, J. .M. (2016). Integrating pharmacogenomics into electronic health records with clinical decision support. Am. J. Health Syst. Pharm. 73 (23), 1967–1976. doi:10.2146/ajhp160030
Hicks, J. .K., El Rouby, N., Ong, H. .H., Schildcrout, J. .S., Ramsey, L. .B., Shi, Y., et al. (2021). Opportunity for genotype-guided prescribing among adult patients in 11 US health systems. Clin. Pharmacol. Ther. 110 (1), 179–188. doi:10.1002/cpt.2161
Hoffman, J. .M., Haidar, C. .E., Wilkinson, M. .R., Crews, K. .R., Baker, D. .K., Kornegay, N. .M., et al. (2014). PG4KDS: A model for the clinical implementation of pre-emptive pharmacogenetics. Am. J. Med. Genet. C Semin. Med. Genet. 166C (1), 45–55. doi:10.1002/ajmg.c.31391
Implementing Genomics in Practice (IGNITE) (2013). Implementing genomics in practice (IGNITE). Available at: https://www.genome.gov/Funded-Programs-Projects/Implementing-Genomics-in-Practice-IGNITE.
International Laboratory Accreditation Cooperation (2022). International laboratory accreditation cooperation. Available at: https://ilac.org/.
Johnson, J. .A., Caudle, K. .E., Gong, L., Whirl-Carrillo, M., Stein, C. .M., Scott, S. .A., et al. (2017). Clinical pharmacogenetics implementation Consortium (CPIC) guideline for pharmacogenetics-guided warfarin dosing: 2017 update. Clin. Pharmacol. Ther. 102 (3), 397–404. doi:10.1002/cpt.668
Just, K. .S., Steffens, M., Swen, J. .J., Patrinos, G. .P., Guchelaar, H. .J., and Stingl, J. .C. (2017). Medical education in pharmacogenomics-results from a survey on pharmacogenetic knowledge in healthcare professionals within the European pharmacogenomics clinical implementation project Ubiquitous Pharmacogenomics (U-PGx). Eur. J. Clin. Pharmacol. 73 (10), 1247–1252. doi:10.1007/s00228-017-2292-5
Karamperis, K., Koromina, M., Papantoniou, P., Skokou, M., Kanellakis, F., Mitropoulos, K., et al. (2021). Economic evaluation in psychiatric pharmacogenomics: A systematic review. Pharmacogenomics J. 21 (4), 533–541. doi:10.1038/s41397-021-00249-1
Karas Kuzelicki, N., Prodan Zitnik, I., Gurwitz, D., Llerena, A., Cascorbi, I., Siest, S., et al. (2019). Pharmacogenomics education in medical and pharmacy schools: Conclusions of a global survey. Pharmacogenomics 20 (9), 643–657. doi:10.2217/pgs-2019-0009
Keeling, N. .J., Rosenthal, M. .M., West-Strum, D., Patel, A. .S., Haidar, C. .E., and Hoffman, J. .M. (2019). Preemptive pharmacogenetic testing: Exploring the knowledge and perspectives of US payers. Genet. Med. 21 (5), 1224–1232. doi:10.1038/gim.2017.181
Kennedy, C., O'Reilly, K., Medley, A., and Sweat, M. (2007). The impact of HIV treatment on risk behaviour in developing countries: A systematic review. AIDS Care 19 (6), 707–720. doi:10.1080/09540120701203261
Kimpton, J. .E., Carey, I. .M., Threapleton, C. .J. .D., Robinson, A., Harris, T., Cook, D. .G., et al. (2019). Longitudinal exposure of English primary care patients to pharmacogenomic drugs: An analysis to inform design of pre-emptive pharmacogenomic testing. Br. J. Clin. Pharmacol. 85 (12), 2734–2746. doi:10.1111/bcp.14100
Koutsilieri, S., Tzioufa, F., Sismanoglou, D. .C., and Patrinos, G. .P. (2020). Unveiling the guidance heterogeneity for genome-informed drug treatment interventions among regulatory bodies and research consortia. Pharmacol. Res. 153, 104590. doi:10.1016/j.phrs.2019.104590
Krebs, K., and Milani, L. (2019). Translating pharmacogenomics into clinical decisions: Do not let the perfect be the enemy of the good. Hum. Genomics 13 (1), 39. doi:10.1186/s40246-019-0229-z
Lea, D. .H., Kaphingst, K. .A., Bowen, D., Lipkus, I., and Hadley, D. .W. (2011). Communicating genetic and genomic information: Health literacy and numeracy considerations. Public Health Genomics 14 (4-5), 279–289. doi:10.1159/000294191
Luzum, J. .A., and Luzum, M. .J. (2016). Physicians' attitudes toward pharmacogenetic testing before and after pharmacogenetic education. Per Med. 13 (2), 119–127. doi:10.2217/pme.15.57
Luzum, J. .A., Pakyz, R. .E., Elsey, A. .R., Haidar, C. .E., Peterson, J. .F., Whirl-Carrillo, M., et al. (2017). The pharmacogenomics research network translational pharmacogenetics program: Outcomes and Metrics of pharmacogenetic implementations across diverse healthcare systems. Clin. Pharmacol. Ther. 102 (3), 502–510. doi:10.1002/cpt.630
Luzum, J. .A., Petry, N., Taylor, A. .K., Van Driest, S. .L., Dunnenberger, H. .M., and Cavallari, L. .H. (2021). Moving pharmacogenetics into practice: It's all about the evidence. Clin. Pharmacol. Ther. 110 (3), 649–661. doi:10.1002/cpt.2327
Marais, B. .J., Lonnroth, K., Lawn, S. .D., Migliori, G. .B., Mwaba, P., Glaziou, P., et al. (2013). Tuberculosis comorbidity with communicable and non-communicable diseases: Integrating health services and control efforts. Lancet Infect. Dis. 13 (5), 436–448. doi:10.1016/S1473-3099(13)70015-X
Medwid, S., and Kim, R. .B. (2022). Implementation of pharmacogenomics: Where are we now? Br. J. Clin. Pharmacol. doi:10.1111/bcp.15591
Mehta, D., Uber, R., Ingle, T., Li, C., Liu, Z., Thakkar, S., et al. (2020). Study of pharmacogenomic information in FDA-approved drug labeling to facilitate application of precision medicine. Drug Discov. Today 25 (5), 813–820. doi:10.1016/j.drudis.2020.01.023
Mitri, Z., Esmerian, M. .O., Simaan, J. .A., Sabra, R., and Zgheib, N. .K. (2010). Pharmacogenetics and personalized medicine: The future for drug prescribing. J. Med. Liban. 58 (2), 101–104.
National Center for Biotechnology Information (NCBI) (1999). dbSNP database. [Available from: https://www.ncbi.nlm.nih.gov/snp/.
National Center for Biotechnology Information (NCBI) (2012). Genetic testing Registry (GTR). Available at: https://www.ncbi.nlm.nih.gov/gtr/.
National genomic test directory (2022). National genomic test directory. Available at: https://www.england.nhs.uk/publication/national-genomic-test-directories/.
National Human Genome Research Institute (2021). Educational resources. Available at: https://www.genome.gov/About-Genomics/Educational-Resources.
Nicholson, W. .T., Formea, C. .M., Matey, E. .T., Wright, J. .A., Giri, J., and Moyer, A. .M. (2021). Considerations when applying pharmacogenomics to your practice. Mayo Clin. Proc. 96 (1), 218–230. doi:10.1016/j.mayocp.2020.03.011
Nielsen, L. .F., and Moldrup, C. (2007). The diffusion of innovation: Factors influencing the uptake of pharmacogenetics. Community Genet. 10 (4), 231–241. doi:10.1159/000106562
O'Donnell, P. .H., Danahey, K., Jacobs, M., Wadhwa, N. .R., Yuen, S., Bush, A., et al. (2014). Adoption of a clinical pharmacogenomics implementation program during outpatient care--initial results of the University of Chicago "1,200 Patients Project. Am. J. Med. Genet. C Semin. Med. Genet. 166C (1), 68–75. doi:10.1002/ajmg.c.31385
Payne, K., and Annemans, L. (2013). Reflections on market access for personalized medicine: Recommendations for Europe. Value Health 16 (6), S32–S38. doi:10.1016/j.jval.2013.06.010
Penas, L. .E. (2023). Clinical use of pre-emptive pharmacogenetic programmes. Lancet 401 (10374), 320–321. doi:10.1016/S0140-6736(22)02461-8
Pharmacogene Variation Consortium (PharmVar) (2000). Pharmacogene variation Consortium (PharmVar). Available at: https://www.pharmvar.org/.
Pharmacogenomics Clinical Annotation Tool (PharmCat) (2022). Pharmacogenomics clinical annotation tool (PharmCat). Available at: https://pharmcat.org/.
Pharmacogenomics Knowledge Base (PharmGKB).(2022) Clinical guideline annotations. Available at: https://www.pharmgkb.org/guidelineAnnotations.
Pharmacogenomics Knowledge Base (PharmGKB) (2017). Drug label annotations. Available at: https://www.pharmgkb.org/labelAnnotations.
Pharmacogenomics Knowledge Base (PharmGKB) (2001). Pharmacogenomics knowledge base (PharmGKB). Available at: https://www.pharmgkb.org/.
Pharmacogenomics Knowledge Base (PharmGKB) (2020). VIPs: Very important pharmacogenes. Available at: https://www.pharmgkb.org/vips.
Pharmacogenomics Research Network (PGRN) (1998). [Available from: https://www.pgrn.org/.
Picard, N., Boyer, J. .C., Etienne-Grimaldi, M. .C., Barin-Le Guellec, C., Thomas, F., Loriot, M. .A., et al. (2017). Pharmacogenetics-based personalized therapy: Levels of evidence and recommendations from the French Network of Pharmacogenetics (RNPGx). Therapie 72 (2), 185–192. doi:10.1016/j.therap.2016.09.014
Pirmohamed, M. (2023). Pharmacogenomics: Current status and future perspectives. Nat. Rev. Genet. doi:10.1038/s41576-022-00572-8
Pratt, V. .M., Del Tredici, A. .L., Hachad, H., Ji, Y., Kalman, L. .V., Scott, S. .A., et al. (2018). Recommendations for clinical CYP2C19 genotyping allele selection: A report of the association for molecular Pathology. J. Mol. Diagn 20 (3), 269–276. doi:10.1016/j.jmoldx.2018.01.011
Rigter, T., Jansen, M. .E., de Groot, J. .M., Janssen, S. .W. .J., Rodenburg, W., and Cornel, M. .C. (2020). Implementation of pharmacogenetics in primary care: A multi-stakeholder perspective. Front. Genet. 11, 10. doi:10.3389/fgene.2020.00010
Robinson, J. (2022). Everything you need to know about the NHS genomic medicine service. Available at: https://pharmaceutical-journal.com/article/feature/everything-you-need-to-know-about-the-nhs-genomic-medicine-service.
Roden, D. .M., Van Driest, S. .L., Mosley, J. .D., Wells, Q. .S., Robinson, J. .R., Denny, J. .C., et al. (2018). Benefit of preemptive pharmacogenetic information on clinical outcome. Clin. Pharmacol. Ther. 103 (5), 787–794. doi:10.1002/cpt.1035
Rollinson, V., Turner, R., and Pirmohamed, M. (2020). Pharmacogenomics for primary care: An overview. Genes. (Basel). 11 (11), 1337. doi:10.3390/genes11111337
Rubinstein, W. .S., Maglott, D. .R., Lee, J. .M., Kattman, B. .L., Malheiro, A. .J., Ovetsky, M., et al. (2013). The NIH genetic testing registry: A new, centralized database of genetic tests to enable access to comprehensive information and improve transparency. Nucleic Acids Res. 41 (2012), D925–D935. doi:10.1093/nar/gks1173
Samwald, M., Xu, H., Blagec, K., Empey, P. .E., Malone, D. .C., Ahmed, S. .M., et al. (2016). Incidence of exposure of patients in the United States to multiple drugs for which pharmacogenomic guidelines are available. PLoS One 11 (10), e0164972. doi:10.1371/journal.pone.0164972
Sangkuhl, K., Whirl-Carrillo, M., Whaley, R. .M., Woon, M., Lavertu, A., Altman, R. .B., et al. (2020). Pharmacogenomics clinical annotation tool (PharmCAT). Clin. Pharmacol. Ther. 107 (1), 203–210. doi:10.1002/cpt.1568
Schildcrout, J. .S., Denny, J. .C., Bowton, E., Gregg, W., Pulley, J. .M., Basford, M. .A., et al. (2012). Optimizing drug outcomes through pharmacogenetics: A case for preemptive genotyping. Clin. Pharmacol. Ther. 92 (2), 235–242. doi:10.1038/clpt.2012.66
Scott, S. .A., Sangkuhl, K., Stein, C. .M., Hulot, J. .S., Mega, J. .L., Roden, D. .M., et al. (2013). Clinical pharmacogenetics implementation Consortium guidelines for CYP2C19 genotype and clopidogrel therapy: 2013 update. Clin. Pharmacol. Ther. 94 (3), 317–323. doi:10.1038/clpt.2013.105
Shekhani, R., Steinacher, L., Swen, J. .J., and Ingelman-Sundberg, M. (2020). Evaluation of current regulation and guidelines of pharmacogenomic drug labels: Opportunities for improvements. Clin. Pharmacol. Ther. 107 (5), 1240–1255. doi:10.1002/cpt.1720
Sinayev, A., Peters, E., Tusler, M., and Fraenkel, L. (2015). Presenting numeric information with percentages and descriptive risk labels: A randomized trial. Med. Decis. Mak. 35 (8), 937–947. doi:10.1177/0272989X15584922
Stanek, E. .J., Sanders, C. .L., Taber, K. .A., Khalid, M., Patel, A., Verbrugge, R. .R., et al. (2012). Adoption of pharmacogenomic testing by US physicians: Results of a nationwide survey. Clin. Pharmacol. Ther. 91 (3), 450–458. doi:10.1038/clpt.2011.306
Swen, J. .J., van der Wouden, C. .H., Manson, L. .E., Abdullah-Koolmees, H., Blagec, K., Blagus, T., et al. (2023). A 12-gene pharmacogenetic panel to prevent adverse drug reactions: An open-label, multicentre, controlled, cluster-randomised crossover implementation study. Lancet 401 (10374), 347–356. doi:10.1016/S0140-6736(22)01841-4
Swift, C. .G. (2022). Personalised future prescribing using pharmacogenomics: A resume of a joint royal College of physicians/British pharmacological society working party report. Future Healthc. J. 9 (2), 174–178. doi:10.7861/fhj.rev-9.2.1
The Dutch Pharmacogenetics Working Group (DPWG) (2005). The Dutch pharmacogenetics working group (DPWG). Available at: https://www.knmp.nl/.
Thornley, T., Esquivel, B., Wright, D. .J., Dop, H. .V. .D., Kirkdale, C. .L., and Youssef, E. (2021). Implementation of a pharmacogenomic testing service through community pharmacy in The Netherlands: Results from an early service evaluation. Pharm. (Basel) 9 (1), 38. doi:10.3390/pharmacy9010038
Thummel, K. .E., and Lin, Y. .S. (2014). Sources of interindividual variability. Methods Mol. Biol. 1113, 363–415. doi:10.1007/978-1-62703-758-7_17
Tsiachristas, A., Vallance, G., Koleva-Kolarova, R., Taylor, H., Solomons, L., Rizzo, G., et al. (2022). Can upfront DPYD extended variant testing reduce toxicity and associated hospital costs of fluoropyrimidine chemotherapy? A propensity score matched analysis of 2022 UK patients. BMC Cancer 22 (1), 458. doi:10.1186/s12885-022-09576-3
Tuteja, S., Salloum, R. .G., Elchynski, A. .L., Smith, D. .M., Rowe, E., Blake, K. .V., et al. (2022). Multisite evaluation of institutional processes and implementation determinants for pharmacogenetic testing to guide antidepressant therapy. Clin. Transl. Sci. 15 (2), 371–383. doi:10.1111/cts.13154
United States Food and Drug Administration (2005). Pharmacogenomic data submissions. Available at: https://www.fda.gov/regulatory-information/search-fda-guidance-documents/pharmacogenomic-data-submissions.
United States Food and Drug Administration (1997). PLAVIX® (clopidogrel bisulfate). Available at: https://www.accessdata.fda.gov/drugsatfda_docs/label/2018/020839s070lbl.pdf.
United States Food and Drug Administration (2022). Table of pharmacogenetic associations. Available at: https://www.fda.gov/medical-devices/precision-medicine/table-pharmacogenetic-associations.
United States Food and Drug Administration (2022). Table of pharmacogenomic biomarkers in drug labeling. Available at: https://www.fda.gov/drugs/science-and-research-drugs/table-pharmacogenomic-biomarkers-drug-labeling.
United States Food and Drug Administration (2009). Tegretol® (carbamazepine). Available at: https://www.accessdata.fda.gov/drugsatfda_docs/label/2009/016608s101,018281s048lbl.pdf.
US Centers for Medicare & Medicaid Services (2020). MolDX: Pharmacogenomics testing. Local cover. Available at: https://www.cms.gov/medicare-coverage-database/view/lcd.aspx?LCDId=38294&ver=16.
van der Lee, M., Kriek, M., Guchelaar, H. .J., and Swen, J. .J. (2020). Technologies for pharmacogenomics: A review. Genes. (Basel). 11 (12), 1456. doi:10.3390/genes11121456
van der Wouden, C. .H., Cambon-Thomsen, A., Cecchin, E., Cheung, K. .C., Davila-Fajardo, C. .L., Deneer, V. .H., et al. (2017). Implementing pharmacogenomics in Europe: Design and implementation strategy of the ubiquitous pharmacogenomics Consortium. Clin. Pharmacol. Ther. 101 (3), 341–358. doi:10.1002/cpt.602
van der Wouden, C. .H., Paasman, E., Teichert, M., Crone, M. .R., Guchelaar, H. .J., and Swen, J. .J. (2020). Assessing the implementation of pharmacogenomic panel-testing in primary care in The Netherlands utilizing a theoretical framework. J. Clin. Med. 9 (3), 814. doi:10.3390/jcm9030814
van der Wouden, C. .H., van Rhenen, M. .H., Jama, W. .O. .M., Ingelman-Sundberg, M., Lauschke, V. .M., Konta, L., et al. (2019). Development of the PGx-passport: A panel of actionable germline genetic variants for pre-emptive pharmacogenetic testing. Clin. Pharmacol. Ther. 106 (4), 866–873. doi:10.1002/cpt.1489
Van Driest, S. .L., Shi, Y., Bowton, E. .A., Schildcrout, J. .S., Peterson, J. .F., Pulley, J., et al. (2014). Clinically actionable genotypes among 10,000 patients with preemptive pharmacogenomic testing. Clin. Pharmacol. Ther. 95 (4), 423–431. doi:10.1038/clpt.2013.229
Varughese, L. .A., Bhupathiraju, M., Hoffecker, G., Terek, S., Harr, M., Hakonarson, H., et al. (2022). Implementing pharmacogenetic testing in gastrointestinal cancers (IMPACT-GI): Study protocol for a pragmatic implementation trial for establishing DPYD and UGT1A1 screening to guide chemotherapy dosing. Front. Oncol. 12, 859846. doi:10.3389/fonc.2022.859846
Verlouw, J. .A. .M., Clemens, E., de Vries, J. .H., Zolk, O., Verkerk, A., Am Zehnhoff-Dinnesen, A., et al. (2021). A comparison of genotyping arrays. Eur. J. Hum. Genet. 29 (11), 1611–1624. doi:10.1038/s41431-021-00917-7
Vo, T. .T., Bell, G. .C., Owusu Obeng, A., Hicks, J. .K., and Dunnenberger, H. .M. (2017). Pharmacogenomics implementation: Considerations for selecting a reference laboratory. Pharmacotherapy 37 (9), 1014–1022. doi:10.1002/phar.1985
Vozikis, A., Cooper, D. .N., Mitropoulou, C., Kambouris, M. .E., Brand, A., Dolzan, V., et al. (2016). Test pricing and reimbursement in genomic medicine: Towards a general strategy. Public Health Genomics 19 (6), 352–363. doi:10.1159/000449152
Weitzel, K. .W., Duong, B. .Q., Arwood, M. .J., Owusu-Obeng, A., Abul-Husn, N. .S., Bernhardt, B. .A., et al. (2019). A stepwise approach to implementing pharmacogenetic testing in the primary care setting. Pharmacogenomics 20 (15), 1103–1112. doi:10.2217/pgs-2019-0053
Whirl-Carrillo, M., Huddart, R., Gong, L., Sangkuhl, K., Thorn, C. .F., Whaley, R., et al. (2021). An evidence-based framework for evaluating pharmacogenomics knowledge for personalized medicine. Clin. Pharmacol. Ther. 110 (3), 563–572. doi:10.1002/cpt.2350
Whirl-Carrillo, M., McDonagh, E. .M., Hebert, J. .M., Gong, L., Sangkuhl, K., Thorn, C. .F., et al. (2012). Pharmacogenomics knowledge for personalized medicine. Clin. Pharmacol. Ther. 92 (4), 414–417. doi:10.1038/clpt.2012.96
Williams, M. .S. (2019). Early lessons from the implementation of genomic medicine programs. Annu. Rev. Genomics Hum. Genet. 20, 389–411. doi:10.1146/annurev-genom-083118-014924
Wolff, N. .D., and Wolff, J. .A. (2018). A commentary on commercial genetic testing and the future of the genetic counseling profession. J. Genet. Couns. 27 (3), 521–527. doi:10.1007/s10897-018-0244-6
World Health Organization (WHO) (2021). Essential medicines list. Available at: https://www.who.int/groups/expert-committee-on-selection-and-use-of-essential-medicines/essential-medicines-lists.
Yang, J. .J., Landier, W., Yang, W., Liu, C., Hageman, L., Cheng, C., et al. (2015). Inherited NUDT15 variant is a genetic determinant of mercaptopurine intolerance in children with acute lymphoblastic leukemia. J. Clin. Oncol. 33 (11), 1235–1242. doi:10.1200/JCO.2014.59.4671
Keywords: guidelines, implementation, pharmacogenomics, practice, pharmacogenetics
Citation: Kabbani D, Akika R, Wahid A, Daly AK, Cascorbi I and Zgheib NK (2023) Pharmacogenomics in practice: a review and implementation guide. Front. Pharmacol. 14:1189976. doi: 10.3389/fphar.2023.1189976
Received: 20 March 2023; Accepted: 03 May 2023;
Published: 18 May 2023.
Edited by:
Miriam Saiz-Rodríguez, Hospital Universitario de Burgos, SpainReviewed by:
Julio Benitez, University of Extremadura, SpainMagnus Ingelman-Sundberg, Karolinska Institutet (KI), Sweden
Copyright © 2023 Kabbani, Akika, Wahid, Daly, Cascorbi and Zgheib. This is an open-access article distributed under the terms of the Creative Commons Attribution License (CC BY). The use, distribution or reproduction in other forums is permitted, provided the original author(s) and the copyright owner(s) are credited and that the original publication in this journal is cited, in accordance with accepted academic practice. No use, distribution or reproduction is permitted which does not comply with these terms.
*Correspondence: Nathalie Khoueiry Zgheib, nk16@aub.edu.lb