- 1Department of Pharmaceutical Sciences, Dibrugarh University, Dibrugarh, Assam, India
- 2Faculty of Pharmaceutical Science, Assam Down Town University, Guwahati, Assam, India
Obesity is fast growing as a global pandemic and is associated with numerous comorbidities like cardiovascular disease, hypertension, diabetes, gastroesophageal reflux disease, sleep disorders, nephropathy, neuropathy, as well as asthma. Studies stated that obese asthmatic subjects suffer from an increased risk of asthma, and encounter severe symptoms due to a number of pathophysiology. It is very vital to understand the copious relationship between obesity and asthma, however, a clear and pinpoint pathogenesis underlying the association between obesity and asthma is scarce. There is a plethora of obesity-asthma etiologies reported viz., increased circulating pro-inflammatory adipokines like leptin, resistin, and decreased anti-inflammatory adipokines like adiponectin, depletion of ROS controller Nrf2/HO-1 axis, nucleotide-binding domain, leucine-rich-containing family, pyrin domain-containing-3 (NLRP3) associated macrophage polarization, hypertrophy of WAT, activation of Notch signaling pathway, and dysregulated melanocortin pathway reported, however, there is a very limited number of reports that interrelates these pathophysiologies. Due to the underlying complex pathophysiologies exaggerated by obese conditions, obese asthmatics respond poorly to anti-asthmatic drugs. The poor response towards anti-asthmatic drugs may be due to the anti-asthmatics approach only that ignores the anti-obesity target. So, aiming only at the conventional anti-asthmatic targets in obese-asthmatics may prove to be futile until and unless treatment is directed towards ameliorating obesity pathogenesis for a holistic approach towards amelioration of obesity-associated asthma. Herbal medicines for obesity as well as obesity-associated comorbidities are fast becoming safer and more effective alternatives to conventional drugs due to their multitargeted approach with fewer adverse effects. Although, herbal medicines are widely used for obesity-associated comorbidities, however, a limited number of herbal medicines have been scientifically validated and reported against obesity-associated asthma. Notable among them are quercetin, curcumin, geraniol, resveratrol, β-Caryophyllene, celastrol, tomatidine to name a few. In view of this, there is a dire need for a comprehensive review that may summarize the role of bioactive phytoconstituents from different sources like plants, marine as well as essential oils in terms of their therapeutic mechanisms. So, this review aims to critically discuss the therapeutic role of herbal medicine in the form of bioactive phytoconstituents against obesity-associated asthma available in the scientific literature to date.
1 Introduction
Obesity affects more than one billion people worldwide, including 650 million adults, 340 million adolescents, and 39 million children, and the number is alarmingly rising (World Health Organization, 2022). Hippocrates, the Greek Physician in the classical Greek era quoted that “Corpulence is not only a disease itself but the harbinger of others,” which holds true in today’s modern world. Obesity is associated with numerous comorbidities like cardiovascular disease, hypertension, diabetes, gastroesophageal reflux disease, sleep disorders, nephropathy, neuropathy, as well as asthma. As per the latest World Health Organization statistics, ischemic heart disease (IHC) tops the leading causes of death globally, however, reports stated that obesity is one of the prominent causes of IHC (World Health Organization, 2021). Studies stated that obese asthmatic subjects suffer from an increased risk of asthma, and encounter severe symptoms that result in the excessive release of white adipose-derived pro-inflammatory adipokines producing severe oxidative stress in the respiratory system (Global Initiative for Asthma, 2020; Pathak et al., 2021). Obesity and asthma have both increased in the United States over the last several decades with nearly 60% of adults with severe asthma being obese. Asthma prevalence is prominent in obese adult women and children. Obesity has a copious effect on the risk of asthma, the higher the body mass index (BMI), the higher the risk of asthma (Peters et al., 2018; Koebnick et al., 2016). There is a plethora of reported obesity-asthma pathophysiologies, namely, modulation of adipose tissue (Lumeng and Saltiel, 2011), increased circulating pro-inflammatory adipokines like leptin, and resistin, and decreased anti-inflammatory adipokines like adiponectin (Fu et al., 2017; Salvator et al., 2021; Palma et al., 2022), depletion of ROS controller Nrf2/HO-1 axis (Yuan et al., 2018; Pathak et al., 2021), NLRP3 associated macrophage polarization (Russo and Lumeng, 2018), activation of the Notch signaling pathway (Zeng et al., 2019), downregulation of Ucp1 in BAT (Cannon and Nedergaard, 2004) followed by downregulated AMPKα (Yamauchi et al., 2003) and melanocortin pathway (Raap et al., 2003) are reported (Figure 1). Asthma is worsened in obese patients and they become less responsive to conventional anti-asthmatic drugs. Inhaled corticosteroids (ICTs) are the common and most-effective drugs that provide fast relief by acting on the airways. Although effective in non-obese asthmatics, ICT does not provide relief to obese asthmatics, and in fact, increased ICT doses lead to poorer asthma control in the absence of eosinophilic inflammation in the sputum or presence of bronchial neutrophilia (Peerboom et al., 2020). Humankind is bestowed with the greatest gift of nature in the form of herbal medicine which comes with a multi-targeted pathway and fewer side effects. There has been an increase in scientific studies evaluating the value of compounds acquired from herbal medicine, which has traditionally been used in folk medicine. Plant-based compounds such as flavonoids, alkaloids, and terpenoids have been reported to show promising in-vitro and in-vivo anti-obesity effects through a repertoire of mechanisms, namely, appetite suppression, triglyceride reduction, metabolic rate increase, pancreatic lipase inhibition, etc. Recently piperine, found in Piper species, has been reported to ameliorate obesity via the alteration of the gut microbiota (He et al., 2022a) as well as found to combat pancreatic β-Cell apoptosis in obese diabetic mice (He et al., 2022b). Similarly, there have been reports of the therapeutic role of plant extract and their bioactive phytoconstituents in allergic asthma. Solasodine, found in Solanaceae family, is reported to suppress ovalbumin (OVA) induced allergic asthma through modulation of different inflammatory markers (Arora et al., 2022a); piperine is also reported to suppress inflammatory markers and oxidative stress in cigarette smoke-exposed experimental mice (Arora et al., 2022b). Likewise, stamen extract of Mesua ferrea L. (Calophyllaceae) from the Calophyllaceae family and root extract of Clerodendrum serratum of the genus Clerodendrum is reported to ameliorate allergic asthma through the modulation of few asthma-associated cytokines like TNF-α, IL-5, and IL-4. The authors speculated that the ameliorative effect of M. ferrea L. may be due to the presence of bioactive phytoconstituents like rhusflavanone, mesuaferrone B, α-Amyrin or β-Amyrin (Arora et al., 2021) and of C. serratum due to compounds like scutellarein, icosahydropicenic acid, oleanolic acid, ursolic acid, and queretaroic acid (Arora et al., 2022a). Similarly, the root extract of Withania somnifera demonstrated potential anti-asthmatic properties. The study reported that treatment with root extract of W. somnifera downregulated pro-inflammatory cytokines like immunoglobulin E (IgE), IL-4), and TNF-α as well as Histone deacetylase 2 (HDAC2) in the blood and bronchoalveolar lavage fluid (BALF) of the treated animals (Ali et al., 2023). From the above published reports, it is observed that bioactive phytoconstituents like piperine possess both the anti-asthmatic as well as anti-obesity properties separately, however, very few studies are undertaken to study both the properties in the same animal model. Although, herbal medicines are widely used for obesity-associated comorbidities nowadays, however, a very smaller number of herbal medicines in the form of bioactive phytoconstituents have been scientifically validated and reported against obesity-associated asthma. Notable among them are resveratrol, β-Caryophyllene, celastrol, and tomatidine. In view of this, there is a dire need for a comprehensive review that may summarize the role of herbal medicines targeting obesity-associated asthma in terms of their therapeutic mechanisms. So, this review aims to critically discuss the therapeutic role of herbal medicine in the form of bioactive phytoconstituents against obesity-associated asthma available in the scientific literature to date.
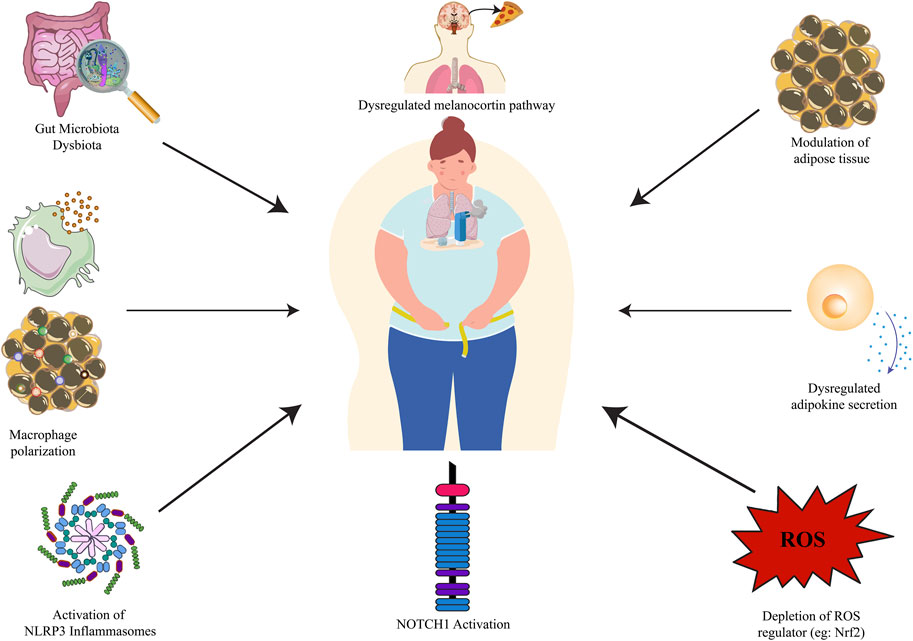
FIGURE 1. Obesity-asthma pathophysiology. NLRP3: nucleotide-binding domain, leucine-rich-containing family, pyrin domain-containing-3; NOTCH1: Neurogenic locus notch homolog protein 1.
2 Obesity-asthma pathophysiology
2.1 Modulation of adipose tissue
The adipose tissue produces cytokines and hormones that regulate metabolism and immunity (Sideleva et al., 2012). Adipose tissue can be divided into 2 major types: white adipose tissue (WAT) and brown adipose tissue (BAT). The largest portion of adipose tissue in most organisms is WAT, which is used for energy storage, whereas BAT plays a major role in thermogenesis in small mammals and neonates (Curat et al., 2004; Fantuzzi, 2005). Lean adipose tissue secretes low levels of proinflammatory cytokines [e.g., IL-6, IL-8, tumor necrosis factor (TNF)-α] and adipokines (e.g., leptin), while producing high levels of the anti-inflammatory adipokine adiponectin. Adipose tissue hypertrophies in obese individuals and becomes infiltrated with macrophages that promote inflammation (Lumeng and Saltiel, 2011) and may exaggerate asthmatic conditions in obese individuals (Shim et al., 2023). Consequently, proinflammatory adipokines are speculated to enhance asthmatic airway inflammation in obesity. A recent report suggests that two significant depots of white adipose tissue, i.e., visceral fat are responsible for the narrowing of bronchial lumina and subcutaneous fat for bronchial wall thickening (Yang et al., 2018).
In metabolic syndrome, mitochondrial dysfunction and defective mitochondrial biogenesis are observed in various organs such as adipose tissue, muscle, liver, and beta islet cells in the pancreas (Kim et al., 2008; Luptak et al., 2019). Factors affecting mitochondrial function include hereditary, environmental, and lifestyle influences. Overeating and high-fat consumption are two of the most common causes of obesity, resulting in nutritional overload, excess electron flux, increased oxidative stress, accumulated partially oxidized substrates, and eventually, damage to the body (Koves et al., 2008). Researchers have found that mitochondrial dysfunction in the airway epithelium was a major contributor to allergic asthma models in mice (Mabalirajan et al., 2008). In a similar model, Aguilera-Aguirre et al. (2009) found that pre-existing mitochondrial dysfunction increased asthma severity. Pattnaik et al. (2016) showed that the levels of asymmetric dimethylarginine (ADMA) in allergically inflamed lungs were extremely high, and IL-4 increased ADMA synthesis via protein arginine methyl transferases and inhibited its degradation by dimethylarginine dimethylaminohydrolase 2. In airway epithelial cells, IL-4 and ADMA worked together to fragment mitochondria, increase mitochondrial ROS release, and reduce mitochondrial mass. As a result of this, obesity and asthma, both of which have high ADMA and IL-4 levels, could potentiate each other by causing mitochondrial dysfunction to become more severe (Bhatraju and Agrawal, 2017). There is increasing evidence that energy metabolism plays a significant role in the development and maintenance of inflammatory conditions such as asthma. Energy metabolism depends heavily on adipose tissue. White adipocytes store energy in the form of triglycerides, and beige/brown adipocytes are involved in heat metabolism (Wu et al., 2012; Harms and Seale, 2013). Several studies have indicated that brown adipose tissue (BAT) can be used as an obesity therapeutic target. BATs are specialized for metabolic thermogenesis mediated by mitochondrial uncoupling protein 1 (UCP1), which uncouples respiration from ATP synthesis (Cannon and Nedergaard, 2004). UCP1 is an inner mitochondrial membrane protein that uncouples oxidative phosphorylation from ATP synthesis through FA/H+ symports (Chouchani et al., 2019). UCP1 expression is mainly driven through β3-adrenoreceptors (β3-AR) stimulation by sympathetically and non-sympathetically produced norepinephrine in thermogenically active adipocytes (Alzaim et al., 2020). Thermogenesis in BAT is due to UCP1, also called thermogenin or SLC25A7 (Dalgaard and Pedersen, 2001). Brown adipose tissue-induced thermogenesis is reported to activate the AMPK pathway which is responsible for the amelioration of asthma in obese subjects (Liu et al., 2023). In cold environments and during the postpartum period, UCP1-mediated thermogenesis is critical for thermoregulation (Tsubota et al., 2019) as well as in regulating adiposity. In both rodents and humans, the activation of UCP1-mediated thermogenesis raises total body energy expenditure and reduces fat mass (Okamatsu-Ogura et al., 2020; Saito et al., 2020). In BAT, UCP1 is highly expressed and oversees adaptive thermogenesis. When UCP1 is stimulated, the respiratory chain is activated. The combustion of readily available substrates produces heat, which the circulatory system then transfers to the other parts of the body (Ricquier, 2011; Jorge et al., 2017). The UCP1 protein helps the body burn energy and lose weight through a process called thermogenesis. It is also involved in maintaining healthy levels of glucose and lipids in the body (Kozak and Anunciado-Koza, 2008; Stanford et al., 2013).
2.2 Dysregulated adipokine secretion
Adipokines are substances produced by fat cells that play a crucial role in the relationship between metabolism and immune system function. However, when they are not regulated properly, as is often the case in obesity, they can cause persistent, low-level inflammation that can lead to various health problems (Taylor, 2021). Adipose tissue has functions beyond just storing energy. It also produces and releases various substances called adipokines, which can act on the fat tissue itself as well as other organs like the heart and lungs to regulate their function. Adipokines act in both local and systemic ways (McGillis, 2005). In addition, adipokines can influence the production of cytokines, which are signaling molecules that can have either pro-inflammatory or anti-inflammatory effects, depending on the specific circumstances (Fu et al., 2017; Salvator et al., 2021; Palma et al., 2022). In general, the production of most adipokines increases in obesity, and the pro-inflammatory ones tend to contribute to the development of health problems associated with obesity as well as in lean subjects, such as asthma where adipokines play an important role in the pathogenesis (Sood and Shore, 2013).
2.2.1 Proinflammatory adipokines
2.2.1.1 Leptin
Leptin is the most well-known proinflammatory adipokine, and it was originally described as a satiation hormone (Friedman and Halaas, 1998). Leptin stimulates the production of chemokine (C-C motif) ligand 2 (CCL2) and vascular endothelial growth factor in human hepatic stellate cells and activates monocytes and macrophages to produce pro-inflammatory interleukin-6 (IL-6), tumor necrosis factor-alpha (TNF-α), and interleukin-12 (IL-12) (Gainsford et al., 1996; Aleffi et al., 2005). Other inflammation signals, such as TNF-α and lipopolysaccharides (LPS), increase leptin and leptin receptor expression (Grunfeld et al., 1996; Gan et al., 2012). In CD4+ T cells, leptin also increases the production of pro-inflammatory Th1 cytokines while suppressing the release of anti-inflammatory Th2 cytokines such as interleukin-4 (IL-4) (Lord et al., 1998). Increased expression of leptin is reported to be associated with obesity-asthma phenotype through the activation of the STAT3 signaling pathway (Chong et al., 2019). Several clinical studies reported an increased level of leptin in obese asthmatics as compared to the non-obese population (Sánchez-Ortega et al., 2022). As reported in human normal BEAS-2 bronchial epithelial cells, leptin promotes exacerbation in obese asthmatic patients by upregulating the mitochondrial reactive oxygen species/NOD-, LRR-, and pyrin domain-containing protein 3 (mostly known as mtROS/NLRP3) inflammasome signaling pathways (Chong et al., 2021). A recent systematic review and meta-analysis also confirmed the pathological role of leptin in obesity-associated asthma where leptin was reported to hold responsible for activating the signaling pathways of inflammation and parasympathetic system that lead to the disease severity (Sánchez-Ortega et al., 2022).
2.2.1.2 Interleukin-6
In adult adipose tissue, IL-6 is highly expressed and favorably linked with obesity (Kwon and Pessin, 2013). One of the most researched factors linked to poor adipogenesis and insulin resistance is IL-6, a pro-inflammatory and immunomodulatory cytokine. IL-6 suppresses the synthesis of adiponectin and impairs insulin signaling, which together contribute to inflammation in obesity (Tzanavari et al., 2010). C-reactive protein (CRP) is also produced as a result, which intensifies the inflammatory response (Bahceci et al., 2007). Additionally, IL-6 participates in the early and late phases of the asthma response and is correlated with the severity of the condition (Sideleva et al., 2012). Plasma IL-6 exerts its pathological aspects in obese-asthmatics by targeting several lung cells, i.e., endothelial cells, epithelial cells, and other airway cells as well as immune cells like regulatory T cells and Th17 cells. The same study reveals that IL-6 high subset of obese asthmatics suffering from other metabolic dysfunctions like hypertension has the highest incidence of asthma as compared with obese asthmatics without any metabolic dysfunctions. This finding reveals a contentious relationship between high plasma IL-6 and obese asthmatics with other metabolic dysfunctions that warrant further studies (Peters et al., 2016).
2.2.1.3 Tumor necrosis factor
Tumour necrosis factor-alpha (TNF-α) is a multi-functional cytokine that can regulate many cellular and biological processes such as immune function, cell differentiation, proliferation, apoptosis, and energy metabolism. It is synthesized as a 26-kDa transmembrane monomer (mTNF-α). In the pulmonary environment, alveolar macrophages can create significant levels of TNF-α, which plays an important role in the pathogenesis of asthma (Kim et al., 2012). The TNF-α receptor 1 (TNFR1) mediates the anti-adipogenic effects of TNF-α (Cawthorn and Sethi, 2008). The number of preadipocytes undergoing differentiation in the abdomen subcutaneous tissue is decreased due to elevated levels of mitogen-activated protein kinase kinase 4 (MAP4K4), which is engaged in the TNF-signaling pathway, leading to hypertrophic fat cells in conjunction with obesity. This implies that fat accumulation and proinflammatory capability are inversely correlated (Cawthorn et al., 2007; Isakson et al., 2009; Al-Mansoori et al., 2022). A study conducted on diet-induced obese (DIO)-mice revealed that controlling obesity through exercise and diet control was found to be an effective means decrease the pulmonary TNF-α levels which result in decreased asthma severity in obesity-associated asthma (Kim et al., 2015). Extracellular matrix (ECM) protects the airways by preventing them from collapsing during expiration, and metalloproteinase (MMPs) enzymes are responsible for ECM renewal, making them important in pulmonary biology. In obese mice, intraperitoneal administration of anti-TNF- monoclonal antibody significantly decreased lung matrix metallopeptidase 9 (MMP-9) activity, demonstrating a link between TNF- and increased asthma severity in obese subjects (Vieira et al., 2020).
2.2.1.4 Resistin
Resistin, a 10 kDa polypeptide of 114 amino acids in rodents, is known to produce pulmonary inflammation and insulin resistance (Holcomb et al., 2000; Steppan et al., 2001). Peripheral blood inflammatory cells, monocytes, and macrophages are the principal producers of resistin. Resistin has also been found in the cells of the bone marrow, lungs, placenta, pancreatic islet tissue, and adipose tissue (Le and Pang, 2006). Resistin expression in human macrophages is induced by inflammatory cytokines such IL-1, IL-6, TNF-α, and LPS. Through the NF-κB signalling pathway, resistin induces human peripheral mononuclear cells to release IL-6 and TNF-α, whereas rosiglitazone, a peroxisome proliferator-activated receptor (PPAR) agonist, inhibits resistin expression in adipose tissues, reducing inflammatory responses (Bokarewa et al., 2005). As per a recent study, serum resistin levels were found to be elevated in obese asthmatics especially in females as compared to males which further confirms the role of resistin in the exaggeration of asthma in obese (Hosny et al., 2021). The hResistin:adiponectin ratio is found to be much higher in obese asthmatics, supporting the idea that hResistin may make a significant contribution to increased severity in the obese population which encourages the idea that this ratio could be a possible therapeutic avenue for managing the obese asthma phenotype (Lin and Johns, 2020).
There are several other proinflammatory adipokines that have been suggested to contribute to the inflammation associated with obesity, although they have not been studied as much as others. These include chemerin, retinol binding protein 4 (RBP4), CC-Chemokine Ligand 2 and CC-Chemokine Receptor Type 5, Angiopoietin-Like Protein 2, and lipocalin 2 (LCN2).
2.2.2 Anti-inflammatory adipokines
2.2.2.1 Adiponectin
Adipocytes strongly express the potent anti-inflammatory molecule adiponectin. A complex molecule, adiponectin can aggregate into complexes with low, intermediate, and high molecular weights in the blood. The AdipoR1 and AdipoR2 receptors, which are involved in immune cells and tissues, activate AMP-activated protein kinase (AMPK) to produce its effects (Yamauchi et al., 2003). The high-molecular-weight complex possesses anti-inflammatory effects that are known to suppress inflammation by preventing NF-κB activation and decreasing cytokines including TNFα, IL-6, and IL-18 (Yokota et al., 2000; Ouchi et al., 2011). Increased inflammatory responses are seen in adiponectin-knockout mice, pointing to the crucial role of adiponectin in reducing tissue and systemic inflammation (Summer et al., 2008; Yoshida et al., 2014). Many clinical investigations have discovered lower serum adiponectin levels in adult obese-asthmatic patients when compared to non-obese asthmatics or controls, with a statistically significant connection between BMI and adiponectin (Bianco et al., 2017). Low adiponectin level is especially found in female obese asthmatics as compared to men which imply that adiponectin responds differently in both sexes having a common type of asthma in obese phenotype (Sood et al., 2008). It has recently been revealed that a high BMI and a low level of adiponectin in children may suggest severe asthma (Machado et al., 2022). Although there have been reports of the protective effect of adiponectin on obese asthmatics, however, there are also reports of the negative impacts of adiponectin in the same condition. As per reports, an elevated level of serum adiponectin is linked to adverse clinical outcomes of asthma in men but not in women, however, the study ruled out the association of BMI with the change in absolute forced expiratory volume in 1 s (FEV1) (Sood et al., 2011). The controversies surrounding adiponectin may be due to polymorphisms in the ADIPOQ gene, where rs822396 and the T allele of rs1063537 have been linked to an increased risk of asthma, whereas variants of rs11760956, rs11763517, and rs2167270 have been linked to a protective nature in obese-asthmatics. There is an urgent need to clarify the diverse role played by adiponectin in different asthma and obese phenotypes before targeting adiponectin for therapeutic implications.
2.2.2.2 C1q/TNF-related protein (CTRP) family
A highly conserved family of adiponectin paralogs known as the C1q/TNF-related proteins (CTRP3) is reported to play an important role in obesity-associated comorbidities (Wong et al., 2004). Similarly, to adiponectin, some CTRPs such as CTRP6, CTRP9, and CTRP12 are mainly expressed in adipose tissue (Wong et al., 2008). CTRP3 is reported to inhibit PS-induced expression of macrophage migration inhibitory factor (MIF), MCP-1, and C-C motif chemokine ligand4 (CCL4) in human monocyte-derived macrophages (Weigert et al., 2005). CTRP6 is reported to increase the expression of anti-inflammatory cytokine IL-10 in human monocyte-derived macrophages through the p42/44 mitogen-activated protein kinase (MAPK)-dependent pathway (Kim et al., 2010). Plasma CTRP9 levels are reported to decrease in mouse models of obesity. Additionally, CTRP9 forms heterotrimers with adiponectin and shares the receptor Adipo R1 with adiponectin in vascular endothelial cells and cardiac myocytes (Kim et al., 2010; Kambara et al., 2012; Ohashi et al., 2014). CTRP12 or adipolin is an insulin-sensitizing adipokine that is abundantly produced by AT and whose expression levels decrease in rodent models of obesity (Enomoto et al., 2011). Additionally, adipolin treatment reduced macrophage infiltration and the expression of pro-inflammatory genes in the adipose tissue of obese mice (Alzaim et al., 2020). In a clinical study involving chronic obstructive pulmonary disease (COPD) patients with a BMI of 22.88 ± 2.78 kg/m2, CTRP-3 and CTRP-5 were found to be elevated in COPD patients however only CTRP-5 was inversely related to FEV1/FVC ratio. The study discovered that CTRPs were statistically unrelated to adiponectin levels, however, like CTRPS, adiponectin was found to be elevated in COPD patients (Li et al., 2015). The elevated level of adiponectin might be due to COPD-induced hyperinflation, necessitating chronic respiratory muscle exercise to restore normal lung function (Pathak et al., 2020) but the reason behind the elevated expressions of the CTRPs is yet to divulge. Although, there are reports of the effect of CTRPs in obese conditions and in pulmonary conditions like COPD, however, there has been a minimal report on the role of CTRPs in modulating obesity-associated asthma.
2.2.2.3 Omentin
Omentin is a type of adipokine whose levels tend to be lower in obese individuals and are negatively correlated with a measure of arterial stiffness (Shibata et al., 2011; Yoo et al., 2011). Additionally, coronary artery disease prevalence and angiographic severity are adversely correlated with omentin expression (Askin et al., 2020). Omentin increases eNOS activity and reduces TNFα-induced endothelial COX2 expression (Zhong et al., 2012). Omentin-1 has been demonstrated to reduce inflammatory responses in endothelial cells in culture by inhibiting JNK activation via the AMPK/eNOS signalling pathway (Yamawaki et al., 2011). Reports on the role played by omentin in respiratory diseases are inconsistent. Omentin was found to be elevated in the airway epithelial sample of asthma as well as in the circulatory sample of obstructive sleep apnea syndrome and decreased in the airway epithelium sample of COPD (Zhou et al., 2017). A significantly reduced level of circulating omentin was found in a group of none-obese (BMI 23.81 ± 3.57 kg/m2) patients with severe persistent asthma as compared to the control group which revealed the inverse relationship of omentin to asthma (Zhou et al., 2018). There has been reporting on the decreased level of omentin in obese subjects as well as in non-obese asthmatics, however, a study on the level of omentin in obese asthmatics is not missing in the scientific literature which is the need of the hour to ascertain the role of omentin the later condition.
2.3 Activation of NLRP3 inflammasomes and macrophage polarization
Innate immunity depends on pathogen-associated molecular patterns (PAMPs) and danger-associated molecular patterns (DAMPs), which activate a variety of immune cells including dendritic cells and the inflammasome (Lee et al., 2014). Inflammasomes are multi-protein complexes that are essential for innate immunity and play a role in controlling inflammatory responses that are triggered by infection or cellular injury. There are numerous different types of inflammasomes, including NLRC4, NLRP1, NLRP6, AIM2, and IFI16 (Rathinam et al., 2012), NLRP3, however, is the inflammasome that has received the most attention. The NLRP3 inflammasome is a group of proteins found in immune cells such as macrophages and dendritic cells, as well as other types of cells. The activation of NLRP3 has also been linked to various metabolic and inflammatory conditions such as gout, diabetes, insulin resistance, and obesity. The activation of NLRP3 plays a crucial role in various metabolic conditions however, too much activation of the inflammasome can cause excessive inflammation and damage to tissues, contributing to the development of chronic inflammatory diseases such as asthma (Williams et al., 2021). The activation of inflammasome-mediated reactions is necessary for an appropriate immunological reaction; however, excessive activation can lead to elevated inflammation and tissue damage, affecting the pathogenic mechanisms of chronic inflammatory diseases such as asthma. In a recent study, activation of NLRP3 inflammasome gene expression along with other inflammatory markers like TLR4, and IL-1β were found to exaggerate the asthmatic episode in obese asthmatic patients as compared to non-obese asthmatics which confirms the negative role of NLRP3 in obesity-associated asthma (Wood et al., 2019). The relationship between NLRP3 and asthma was further cemented when the activation of NLRP3 was inhibited by some novel NLRP3 inhibitors or by silencing of NLRP3. There have been reports of reduction of airway hyperresponsiveness (AHR) and airway inflammation in murine models of ovalbumin (OVA)-induced respiratory infections model by NLRP3 inhibitors like MCC950 and AC-YVAD-cho (Kim et al., 2017). Similarly, silencing the NLRP3 gene through short hairpin RNA is reported to reduce NLRP3 mediated IL-1β secretions in human bronchial epithelial cells (Theofani et al., 2019). Macrophages are immune cells that play a role in inflammation and the immune response. The activation state of macrophages, or their “polarization,” has been found to significantly influence the development of asthma. When macrophages are recruited to a specific area of the body, they can be activated in one of two ways: a “classically activated” or M1 state, or an “alternatively activated” or M2 state, depending on the local environment (Saradna et al., 2018). Weisberg et al. (2003) and Xu et al. (2003) first described the infiltration of a significant number of macrophages into the adipose tissues of obese persons. M1 macrophages typically produce inflammation through the activation of receptors for TNF-α, IL-1β, and CD36, which leads to the activation of NF-κB and the production of NLRP3 and other pro-inflammatory cytokines such as IL-1β and IL-18. This process, which is known as “priming,” sets the stage for further inflammation (Russo and Lumeng, 2018). However, the NLRP3 inflammasome requires a second “hit” or stimulus to assemble and become active. This second stimulus is usually provided by substances such as ceramides, fatty acids, oxidized low-density lipoproteins, and cholesterol crystals, which bind to TLR 2/4 receptors (Rajamaki et al., 2010; Camell et al., 2015; Karasawa et al., 2018; Okla et al., 2018). The claim of the second “hit” by substances for NLRP3 activation was further cemented when microarray data demonstrated a significant increase of ceramide accumulation during M1 macrophage polarization in obese asthmatics. In the same study, M1 macrophage polarization as well as an elevated level of airway hyperresponsiveness (AHR) and C18:0 ceramide levels were observed in obese mice which confirms the role of ceramide or similar substances in the deterioration of obesity-associated asthma (Choi et al., 2020). Finally, sustained activation of NLRP3 leads to the formation of GSDMD pores in the membrane of macrophages (Liu et al., 2016). This process, called “pyroptosis,” causes the cell to die and release pro-inflammatory molecules into the surrounding area. It does this by disrupting the osmotic potential inside the cell (Evavold et al., 2018; López-Reyes et al., 2020). M1 macrophages are believed to be beneficial in asthma and make a significant contribution to the pathogenesis of obesity, whereas M2 macrophages are responsible for asthma pathology but restrict the obesity comorbidities (Sharma et al., 2018). T2 cytokines and the NLRP3 inflammasome, which is responsible for obesity-related asthma, were recently discovered to have a link, making them targets for therapeutic applications (Pinkerton et al., 2022).
2.4 Dysregulated melanocortin pathway
Eosinophil infiltration into the airways, a characteristic of bronchial asthma, is crucial to the pathophysiology of the disease (De Monchy et al., 1985; Bousquet et al., 1990). Although there have been significant advancements in our understanding of the aetiology of this disease over the past 20 years, the therapy of choice is still the same as it was 30 years ago: inhaled 2-adrenoceptor agonists and glucocorticoids. As a result, there is still a very real medical need for treating the disease’s symptoms and underlying pathology. Melanocortins may be used to suppress airway inflammation, according to one study. In an OVA-challenged model of airway inflammation, α-Melanocyte-stimulating hormone (α-MSH) reduced eosinophil migration and subsequently IgE, IL-4, and IL-13 levels, but had no effect on IL-5. Although it may appear odd that eosinophils are inhibited but not IL-5, α-MSH inhibits VCAM-1, which is regulated by IL-4 and IL-13, which may account for the decrease in migratory cells. α-MSH can also promote the anti-inflammatory cytokine IL-10, as was previously mentioned with the production of the anti-inflammatory protein heme oxygenase-1 (HO-1) (Lam et al., 2005). Given that α-MSH is inactive in IL-10 null animals, this would seem to be a crucial mechanism for the anti-inflammatory effects of the melanocortin. Very few substances can treat the underlying pathophysiology of bronchial hyperresponsiveness, even though many have been demonstrated to block certain components of the inflammatory response associated with asthma. Since α-MSH suppressed the inflammation as well as cellular migration and cytokine production, it had steroid-like effects (Montague and O’Rahilly, 2000). The central melanocortin system is reported to play important role in obesity and diabetes. Aside from its effects on food intake and body weight, α-MSH has anti-inflammatory activities in both immune cells and non-immune cells (e.g., adipocytes) that express melanocortin receptors which may be a potential target for obesity-associated asthma (Goit et al., 2022).
2.5 Depletion of ROS regulator
The rise in free fatty acid levels boosts adipocyte ROS production by activating NADPH oxidase and decreasing the expression of enzymatic antioxidants, which is correlated with fat adipocyte fat storage (Hattori et al., 2005; Johnson et al., 2007; Devaraj et al., 2008). Anti-inflammatory adiponectin levels decrease and the concentration of pro-inflammatory adipocytokines increases in adipocytes with OS (Soares et al., 2005; Chen et al., 2009). Because there are no accurate ways to measure oxidative stress in vivo, most of the evidence indicating the action of ROS in asthma is indirect or circumstantial. The analysis of lipid peroxidation based on diene conjugate and thiobarbituric acid (TBA), measurement of increases in nitric oxide (NO), H2O2, and pentane in exhaled gas or breath condensates, and evaluation of substrate oxidisability (or spin trapping) of free radical adducts ex vivo all have low sensitivity and specificity. However, utilizing these techniques, it was discovered that adults and children with different asthma severity and acute asthma exacerbations had an increased generation of ROS (Jarjour et al., 1992; Dohlman et al., 1993; Antczak et al., 1997; Olopade et al., 1997; Saleh et al., 1998). Nrf2 is a key regulator in the transcriptional activation of antioxidation and biosynthesis of endogenous antioxidants (Kasai et al., 2020). Nrf2 signals the nucleus during oxidative stress to promote the expression of HO-1, which in turn, protects the lung from oxidative stress (Pathak et al., 2021). Both Nrf2 and HO-1 are reported to be downregulated in obese conditions.
2.6 Notch signaling pathway
Currently, Notch signaling pathway has gained considerable attention as a therapeutic target for obesity-associated asthma. Notch signaling activation is reported to inhibit adipocyte differentiation by suppressing the expression of CCAAT/enhancer binding protein alpha (C/EBPα) and peroxisome proliferator-activated receptor gamma (PPARγ) in the 3T3-L1 preadipocytes (Ross et al., 2004). In obese mice, inactivating Notch1 causes the browning of white adipose tissue and increased expression of UCP1, a key regulator of thermogenesis (Bi et al., 2014). Notch signaling pathway having CD4+ T cell (Th17 cell) is reported to express increased interleukin (IL)-17 in obese asthmatic individuals. In a preclinical study, γ-Secretase inhibitor (GSI) is reported to reduce the Notch1, Th17 cell proportion and serum IL-17 in a diet-induced-obesity-ovalbumin (DIO-OVA) C57BL/6 mice model of asthma (Zeng et al., 2019). Pre-clinical and clinical studies involving Notch signaling pathway reveals that targeting Notch signaling pathway therapeutically may open new avenues in the treatment of obesity-associated asthma in recent times.
2.7 Gut microbiota dysbiosis
Investigating how gut microbiota affects asthma has gained popularity in recent years. Children at risk for developing asthma exhibited considerably reduced relative abundances of the taxa Faecalibacterium, Lachnospira, Rothia, and Veillonella (FLVR) in their gut microbiomes at 3 months of age. Increased risk of asthma was linked to higher Veillonella abundance and lower Roseburia, Alistipes, and Flavonifractor abundances at 1-year-old age for children with asthma at age 5 (n = 60), albeit the association was only significant for kids whose mothers also had asthma (Stokholm et al., 2018). According to various research, people with asthma had higher concentrations of Streptococcus pneumoniae, Haemophilus influenzae, Moraxella catarrhalis, and Haemophilus species in their airway microbiomes (Bisgaard et al., 2007; Hilty et al., 2010; Green et al., 2014). Diet can cause fast microbial alterations in the gut microbiome. According to one study, lifetime vitamin D administration increased the number of Acinetobacter operational taxonomic units (OTUs) in the lungs of female mice. The lung microbiota was significantly more altered by allergic airway illness that was brought on by ovalbumin, suggesting that vitamin D’s ability to modulate the microbiota may be constrained (Roggenbuck et al., 2016). According to recent studies, food, gut microbiota, and airway inflammation interact via short chain fatty acids (SCFAs) and pro- or anti-inflammatory cytokines. Additionally, because observational human studies are associative, creating possible prebiotic or probiotic treatments might benefit from employing mouse models that focus on the causative relationships between microbiome dysbiosis and airway pathobiology. Obesity is linked to changes in the gut microbiota (Yuan et al., 2018). According to several studies, obesity in humans is linked to a higher Firmicutes/Bacteroidetes (F/B) ratio at the phylum level (Rahat-Rozenbloom et al., 2014; Serena et al., 2018). Which bacteria are most impacted by obesity has been the subject of widely varying studies. Lachnospiraceae, Prevotellaceae, Megamonas spp., Roseburia sp., Veillonellaceae, Lactobacillus spp., Bacteroides fragilis, Faecalibacterium sp., and a Prevotella spp. -dominated enterotype are more prevalent in people with obesity who have microbiota dysbiosis. Additionally, obesity was linked to decreased gene richness, decreased microbiota diversity, and decreased levels of Oscillospiraceae, Succinivibrio sp., Odoribacteraceae, Clostridaceae, and Oscillospira sp. (Murugesan et al., 2015; Liu et al., 2017). Significant correlations between changes in metabolic syndrome markers and gut microbial dysbiosis have been found in several human cohort studies. After adjusting for age and sex, Fu et al. (2015) found a negative correlation between gut bacterial richness and both BMI and serum triglyceride levels and a positive correlation between gut bacterial richness and serum HDL levels. According to this study’s estimates, the makeup of the gut microbiota may account for up to 6% of the variation in independent of age, genetic variables, blood lipid levels, and sex. The gut microbiome affects not only obesity and metabolic syndrome, but also airway inflammation mostly through the production of short-chain fatty acid and cytokine levels and triggers asthma (Kim, 2021). The administration of prebiotics and probiotics are reported to play an important role in the amelioration of obesity-associated asthma through modulation of the gut microbiota. In a recent study, dietary fiber pectin, a prebiotic, demonstrated a promising effect on the O3-exposed db/db mice model of AHR indicating the importance of targeting gut microbiota in the therapeutic strategy of obesity-associated asthma (Tashiro et al., 2019).
3 Bioactive phytoconstituents in obesity-associated asthma
Obese patients with asthma experience poor asthma control for many reasons, but a crucial one appears to be that their reaction to controller medication is impaired. Many studies have indicated that obese individuals do not respond as effectively to conventional control regimens. It appears that inhaled corticosteroids and combination inhalation corticosteroid-long-acting agonists are superior to montelukast for treating asthma in obese persons (Park et al., 2009). All existing approved asthma drugs were developed using lean animal models of asthma and tested on patient populations that were far slimmer than the typical patient population seen today (Halliwell et al., 1992). Herbs have always been a crucial and valuable treatment option for many persistent health problems, including obesity. Herbs generally have fewer side effects than single-compound drugs, except for allergic reactions in those particularly sensitive to them (Park et al., 2009). There has been a rise in scientific studies assessing the value of substances obtained from herbal medicine, which have traditionally been employed in folk medicine. Plant-based compounds, such as flavonoids, alkaloids, and terpenoids, have been shown to have biological effects in vitro and in vivo. Tripterygium wilfordii, Hibiscus sabdariffa, Ilex paraguariensis, Coffea arabica, Caralluma fimbriata, Panax ginseng, plants from solanaceae family, and many others have all been shown to have positive effects on obesity through various mechanisms, such as appetite suppression, triglyceride reduction, metabolic rate increase, pancreatic lipase inhibition, and so on. Therefore, they aid in the process of losing weight (MacNee, 2001; Kannaiyan et al., 2011).
Here we have emphasized the effects of some important bioactive compounds like celastrol, tomatidine, resveratrol, quercetin, ascorbic acid, β-Carotene, chrysophanol, etc. (Figure 2), for treatment of obesity-associated asthma in experimental models and in humans and pointing out some possible mechanisms of action are emphasized.
3.1 Celastrol
Celastrol is an effective natural bioactive component isolated from T. wilfordii’s roots. It has proven highly beneficial in anti-inflammatory, anti-cancer, anti-rheumatic, and autoimmune illness (Kannaiyan et al., 2011). In addition, it has been shown to have a remarkable effect on body mass reduction in mice (Lee et al., 2006). Celastrol was reported to cause significant weight loss in a hyperleptinemic DIO mice model through the reduction of food intake, increasing energy expenditure, and leptin sensitivity (Liu et al., 2015). Celastrol has also been shown to lower airway inflammation and AHR in allergic asthma, which is interesting. On a model of obesity and asthma, celastrol therapy reduced the frequency of Th17 cell growth and IL-17A production in the lung and serum. The research study’s findings suggested that Th17 and its cytokine, as assessed in the spleen and lung, were strongly linked to AHR. In addition, celastrol inhibits Th17 in obese asthmatic mice, which has been demonstrated to decrease AHR. Celastrol reduces AHR and inhibits Th17 responses in obese asthmatic mice (Zhang et al., 1990).
3.2 Tomatidine
Tomatidine is a steroidal alkaloid under the Solanaceae family is obtained from leaves of eggplant, potato, and tomato. Solanaceae plants release tomatidine and α-Tomatine to combat fungal infections (Norton, 1998). Tomatidine has demonstrated promising anti-obesity in animal models. In male C57BL/6 mice, tomatidine significantly reduces body weight, fat weight, and regulates other metabolic parameters such as total cholesterol, fasting blood glucose, low-density lipoprotein, and triglyceride levels in serum (Wu et al., 2021). Tomatidine suppresses the reproduction of Staphylococcus aureus, according to a previous study. Additionally, tomatidine reduces the invasion activity of A549 human lung adenocarcinoma cells and promotes death in HL60 human myeloid leukemia cells. Tomatidine inhibits iNOS and COX-2 production by blocking the NF-κB pathway in LPS-stimulated RAW 264.7 cells, according to a study conducted (Steel and Drysdale, 1988). The research found that tomatidine ameliorates the allergic inflammatory response in the airways in asthma by reducing the levels of Th2-associated cytokines, goblet cell hyperplasia, eosinophil infiltration, and AHR. Tomatidine inhibited the generation of Th2 cytokines in bronchoalveolar lavage fluid. In lung tissue, tomatidine inhibited the expression of inflammatory and Th2 cytokine genes. Tomatidine decreased the generation of proinflammatory cytokines and CCL11 in inflammatory BEAS-2B bronchial epithelial cells in vitro. These findings suggest that tomatidine ameliorates AHR and eosinophil infiltration in asthmatic mice by inhibiting the inflammatory response and Th2 cell activity (Kuo et al., 2017). The above reports showcase the ameliorative action of tomatidine against both obesity and respiratory diseases like asthma and AHR by targeting various pathways which support the possible therapeutic efficacy of tomatidine against obesity-associated asthma which is yet to explore.
3.3 Curcumin (diferuloylmethane)
Curcumin, the active phytoconstituent in turmeric, is a polyphenol having anti-inflammatory, anti-amyloid, antibacterial, anti-tumor, anti-allergic, and anti-oxidative activities (Kurup and Barrios, 2008). Curcumin has been shown to have potent anti-obesity properties. Curcumin reportedly interacts with white adipose tissue to reduce chronic inflammation, macrophage infiltration, and nuclear factor B (NF-κB) activation. Curcumin also reduces the proinflammatory adipokines tumour necrosis factor (TNF), monocyte chemoattractant protein-1 (MCP-1), and plasminogen activator inhibitor type-1 (PAI-1) and increases adiponectin expression (Bradford, 2013). Curcumin acts as an HDAC activator or inhibits histamine release from mast cells, which reduces the production and level of cytokines like IL-2 and IL-5 in lung tissue (Biswas and Rahman, 2008). Curcumin’s ability to regulate asthma phenotypes indicates that it can alleviate asthma symptoms, decrease eosinophil recruitment to the airway, and improve airway hyper-responsiveness, all of which contribute to weight loss. These results suggest curcumin could be an effective adjunct treatment for obesity-related asthma (Brouet and Ohshima, 1995; Mortellini et al., 2000).
3.4 Resveratrol
Resveratrol (3,4,5-trihydroxystilbene) is a phytoalexin present in plant sources, such as grapes, berries, and peanuts as well as known as a component in red wine. Resveratrol is one of the polyphenolic molecules comprised of two phenol rings joined by a 2-carbon methylene bridge with multiple effects on cardiovascular protection, anticancer impact, and as a positive regulator of several areas of metabolism. Resveratrol can eliminate intracellular reactive oxygen species by activating and stabilizing antioxidant enzymes such as catalase, SOD, and glutathione peroxidase hemoxygenase (Das and Das, 2007; Shankar et al., 2007). In addition to its reducing properties, resveratrol has been shown to reduce inflammation by inhibiting prostaglandin production and decreasing the phosphorylation of ERK1/2, cyclooxygenase-2 activity, and the activity of various transcription factors, including NF-B, STAT3, HIF-1α, and β-Catenin (Shankar et al., 2007; Pirola and Fröjdö, 2008). Resveratrol is reported to exert its anti-obesity effect by the browning of WAT as well as by targeting several intracellular components such as sirtuin-1 (SIRT-1), adenosine monophosphate-activated protein kinase (AMPK), and the peroxisome proliferator-activated receptor coactivator-1 (PGC-1) (Price et al., 2012). Resveratrol can also modulate the innate immune response by inhibiting the expression of costimulatory molecules (CD80 and CD86) and major histocompatibility complex classes I and II in bone marrow-derived dendritic cells and by inhibiting angiogenesis pathway mediated by the expression of MMPs, VEGF, cathepsin D, ICAM-1, and E-selectin. Resveratrol has demonstrated potent ameliorative in obesity-associated asthma effect in experimental animal model. Resveratrol was effective in combating ovalalbumin and obesity-induced oxidative stress in obese asthmatic rat models by inhibiting Keap1 and activating the Nrf2 antioxidant defense system (Li et al., 2018).
3.5 Carotenoids
Carotenoids are a group of pigmented compounds produced by fruits, vegetables, and microorganisms. Carotenoids, which are found as micro-components in fruits and vegetables, are responsible for their yellow, orange, and red colours (Rao and Rao, 2007). β-Carotene, α-Carotene, lycopene, β-Cryptoxanthin and lutein are some of the major dietary carotenoids. In several observational studies, low level of α-Carotene, β-Carotene, and β-Cryptoxanthin has been linked to both asthma as well as obesity (Tobias et al., 2019). Total vitamin A is composed of preformed vitamin A (retinol) and provitamin A molecules, known as carotenoids, with beta-carotene being the most essential. Plants and microbes have carotenoids as pigments (Rao and Rao, 2007). Various studies have revealed that carotenoids may protect or inhibit some types of cancer, atherosclerosis, immunological problems, asthma, and other diseases (Gallicchio et al., 2008; Young et al., 2008). Carotenoids may influence the activation of many transcription factors (Niles 2004). β-Carotene inhibits oxidative stress-induced activation of NF-κB and generation of IL-6, TNF-α, and inflammatory cytokines in cells treated with β-Carotene. Carotenoids may impact the apoptotic process in healthy cells. β-Carotene can boost the expression of the anti-apoptotic protein Bcl-2 in normal cells but the pro-apoptotic protein Bax is downregulated when external stimuli are induced. It has also been demonstrated that lycopene regulates transcription factors. Lycopene inhibits AP-1 binding and reduces the activation of insulin-like growth factor-I in mammary cancer cells (Sharoni et al., 2004). These combined functions of vitamin A and dietary carotenoids make carotenoids an excellent anti-inflammatory agent in the treatment of obesity-associated asthma.
3.6 Vitamin C
Vitamin C (ascorbic acid) is a vital and effective antioxidant in the body’s aqueous environment. It exists in two interconvertible biologically active forms, ascorbic acid and its oxidized derivative, dehydro-ascorbic acid (Romieu and Trenga, 2001). As a hydrogen donor, vitamin C reverses oxidation and shields membranes from oxidation (i.e., reducing agent). Vitamin C, also known as ascorbic acid, has been shown to inhibit adipocyte lipolysis, regulate glucocorticoid release from the adrenal glands, inhibit glucose metabolism, modulate glucose metabolism, decrease glycosylation in obese-diabetic models, and reduce the inflammatory response (Garcia-Diaz et al., 2014). A deficiency in vitamin C is related to an increased risk of asthma in obese individuals, who have significantly lower levels of ascorbic acid; conversely, treatment with vitamin C is advantageous for asthmatic adults who smoke, lowering cough and wheezing (Allen et al., 2009). Vitamin C can also regulate factors that affect gene expression, apoptosis, and other cellular processes. It protects against cell death induced by a variety of stressors, and much of the protection is due to its antioxidant capacity (Romieu and Trenga, 2001). Vitamin C controls the AP-1 complex, which consists of the Fos and Jun superfamilies. Vitamin C treatment of cells exposed to UV-B resulted in a 50% decrease in JNK phosphorylation, which activates AP-1 and inhibits the JNK/AP-1 signaling pathways (Catani et al., 2001). Currently, however, the evidence from randomized controlled trials is insufficient to suggest a specific role for vitamin C in the treatment of asthma due to the various study design and typically flawed reporting systems (Kaur et al., 2009).
3.7 Vitamin E
There are eight distinct forms of fat-soluble vitamin E. It consists of a group of chemicals belonging to two closely related families, the tocopherols and tocotrienols, each of which exists in multiple isomeric forms (Riccioni and D’Orazio, 2005). Alpha-tocopherol is the most active form of vitamin E in humans and its primary antioxidant activity is lipid peroxidation prevention (Moriguchi and Muraga, 2000; Devarah et al., 2002). Vitamin E is also reported to ameliorative role in the obese condition. Vitamin E administration reduced oxidative stress, deposition of collagen in the visceral adipose tissue of obese mice, which resulted in a reduction in circulating cytokines and hepatic steatosis, hypertriglyceridemia, and followed by improvement of insulin sensitivity (Alcalá et al., 2015). Vitamin E is well known for playing a potent role in curbing the generation of free radicals which is due to its high concentration in the body among all lipid-soluble vitamins. Vitamin E is associated with a low incidence of asthma in infants as well as in adults. Vitamin E administration during pregnancy is associated with a lower chance of exacerbation of asthma in children (Miyazawa et al., 2019). Vit-E alleviated AHR by lowering Th2 responses, namely, as IL-4, IL-5, IL-13, and OVA-specific IgE, eotaxin, transforming growth factor-1, airway inflammation, lipid peroxidation, and nitric oxide metabolites, and by restoring mitochondrial dysfunction in the lungs (Mabalirajan et al., 2009). Besides, several epidemiological studies have demonstrated the effectiveness of vitamin E supplementation in treating bronchial asthma in obese and non-obese patients (Picado et al., 2001; Troisi et al., 1995; Trenga et al., 2001; Pearson et al., 2004; Pletsityi et al., 1995). However, the exact mechanism underlying the ameliorative role of vitamin E in obese asthmatics is yet to be unearth.
3.8 Alpha-lipoic acid
α-Lipoic acid (ALA), an organosulfur chemical produced from octanoic acid, is also known as thiothic acid. ALA is quickly absorbed from the diet and swiftly transformed into dihydrolipoic acid (DHLA), its reduced dithiol form. LA and DHLA are both potent antioxidants (Prior and Cao, 2000). Spinach, broccoli, and tomatoes are high in lipoyllysine -containing plant sources. ALA has been demonstrated to drastically lower serum IgE concentration, attenuate Th2 cytokines, IL-4, IL-5, IL-13, and IL-18, and reduce NF-B activation by decreasing intracellular ROS levels in a mouse model of asthma with allergic airway inflammation (Bustamante et al., 1998). In addition, ALA activation connected to VEGF expression, a crucial asthma factor, suppresses the pathogenesis of HIF-1 (Bustamante et al., 1998). These studies demonstrate that ALA is a multifunctional molecule in airway inflammatory illnesses, acting as a potent antioxidant and a regulator of gene expression that induces inflammatory cascades and plasma exudation and promotes the management of obesity (Cao and Phillis, 1995). ALA has demonstrated a marked effect in targeting obesity clinically by reducing body weight and BMI significantly without any effect on waist circumference (Namazi et al., 2018; Vajdi and Abbasalizad Farhangi, 2020). As observed in the animal models, ALA exerted its anti-obesity effect by modulating oxidative stress, and inflammation and by increasing insulin sensitivity in the subcutaneous and visceral adipose tissue of high-fat diet rats (Dajnowicz-Brzezika et al., 2022; Sztolsztener et al., 2022). ALA is reported to play a positive role in the antioxidant level of pulmonary tissue of obese asthmatic mice. The study reported that the administration of ALA combined with L-ascorbic acid to obese C57BL6 mice resulted in the upregulation of the endogenous antioxidant enzymes superoxide dismutase and catalase which demonstrated the antioxidant property of ALA. However, the effect of a single administration of ALA in obese asthmatic animals is reported to date.
3.9 Chrysophanol
Chrysophanol is an anthraquinone reported to found in many plants, insects, and microbes. In plants, chrysophanol was first reported in Rheum rhabarbarum, in fungi it was first reported in Penicillium islandicum Sopp, however, in insects, chrysophanol was reported to use as a defensive chemical (Yusuf et al., 2019). In animal models, chysophanol has demonstrated potential anti-obesity efficacy. Chrysophenol has been shown to reduce lipid accumulation in HFD rats’ primary hepatocytes, reduce inflammation by significantly lowering IL-6 and IL-1 levels, and increase IL-10 levels. Furthermore, the same study found that chrysophanol decreased the expression of lipogenic genes by activating AMP-activated protein kinase (AMPK)/Sirtuin 1. (SIRT1). Chrysophanol has also demonstrated ameliorative effects in asthma by inhibiting the activation of nuclear factor-kappa B (NF-kB) signaling pathway in animal models (Song et al., 2019). In a recent study involving the systematic pharmacological and bioinformatics analysis, chrysophanol was found to a potential candidate for combating obesity asthma/childhood asthma comorbidities. The study found that chrysophanol It was discovered that chrysophanol regulates inflammation by being involved in the resistance of EGFR tyrosine kinase inhibitor, the HIF-1 signaling pathway, and the trapping of extracellular neutrophils. However, the study emphasized the importance of experimental verification of their claim using in-vitro, in-vivo, and OMICS technologies (Liu et al., 2023).
3.10 Flavonoids
With more than 8,000 molecules reported, flavonoids are the most important group of low molecular weight polyphenolic secondary plant metabolites. They are found in fruits, vegetables, nuts, seeds, stems, flowers, roots, tea, wine, and coffee, and are prevalent in our diet (Hollman and Katan, 1999). Flavonoids limit the release of histamine and other granule-associated mediators by reducing the activation of basophils and mast cells (Harborne and Williams, 2000). Furthermore, flavonoids suppress the synthesis of IL-4, IL-13, and CD40 ligand, whereas they stimulate the production of novel phospholipid-derived mediators. Quercetin, one of the well-studied flavonoids, inhibits concentration-dependently the eosinophilic secretion of Charcot-Leyden crystal protein and eosinophil cationic protein (Balsano and Alisi, 2009). In addition, quercetin’s inhibitory effect on other inflammatory cells appears to be superior to that of any other clinically accessible drug (Middleton et al., 2000). Li et al. (2010) have proven that apigenin displays anti-inflammatory effects in a mouse asthma model and can modify the immune response to allergens toward the T-helper type 1 cell (Th1) profile. These results imply that flavonoids are excellent anti-allergenic and anti-inflammatory medicines for the treatment and prevention of asthma. Vascular alterations are one of the primary factors in the pathophysiology of asthma (Li et al., 2010). The alterations include a rise in vascular permeability, vascular dilation/engorgement, and vasculogenesis/angiogenesis (Williams and Grayer, 2004). Flavonoids and their related chemicals influence the production of HIF-1, VEGF, matrix metalloproteinases (MMPs), and the epidermal growth factor receptor, but also inhibit the NF-B, PI3K/Akt, and ERK1/2 signaling pathways (Hollman and Katan, 1999). Observations indicate that flavonoids and their related substances block some phases of angiogenesis, including cell migration, microcapillary tube formation, and matrix metallopeptidase (MMP) production.
3.11 Bioactive compounds from marine sources
Fucosterol, a phytosterol derived from the marine brown algae Padina boryana, exhibited anti-inflammatory properties via dose-dependently down regulating pro-inflammatory cytokines (IL-1, IL-6, and TNF) and the Nrf2/HO-1 pathway and aid in the process of losing weight in obesity associated asthma (Sohn et al., 2021). Mojabanchromanol (MC), a chromanol isolated from the brown alga Sargassum horneri, exhibited anti-oxidant effects via the attenuation of particulate matter-induced oxidative stress, the reduction of the ROS-mediated phosphorylation of MAPK extracellular signal-regulated kinase 1/2 (Erk1/2) and of c-JNK, and the inhibition of the secretion of pro-inflammatory cytokines (The authors proposed that mojabanchromanol be developed as a treatment for airway inflammation caused by particulate matter (Herath et al., 2020). Sargachromenol, isolated from S. horneri, exhibited anti-inflammatory effects in LPS-stimulated RAW 264.7 macrophages, by reducing nitric oxide (NO); and in intracellular reactive oxygen species (ROS), by reducing the mRNA expression levels of inflammatory cytokines (IL-1, IL-6, and TNF-) and by inhibiting the activation of NFκB and MAPK signaling (Herath et al., 2020). Pyrenocine A, which is derived from the marine-derived fungus Penicillium paxilli, suppresses pro-inflammatory mediators (TNF- and PGE2) and NFB-related gene expression in LPS-stimulated macrophages (Toledo et al., 2014). Spirulina extract (Immulina®), a high-molecular-weight polysaccharide extract from the Cyanobacterium Arthrospira platensis (Spirulina), exhibited anti-inflammatory and inhibitory effects on histamine release from RBL-2H3 mast cells and an induced allergic inflammatory response. Moreover, it has the potential to block the IgE-antigen-complex-induced generation of TNF-, IL-4, leukotrienes, and histamine, and it exhibited promising results in relieving allergic rhinitis symptoms (Appel et al., 2018).
3.12 Bioactive compounds from essential oils
Essential oils from Citrus paradisi, Cymbopogon nardus L., Pogostemon cablin Benth, Hamelia patens, Citrus sinensis and many more are reported to have promising anti-obesity effects. The anti-obesity effects in those essential oils might be due to the presence of a myriad of bioactive phytoconstituents like α-Pinene, β-Myrcene, geraniol, β-Citronellol, citronellol, α-Patchoulene, β-Patchoulene, carvacrol, linalool, D-limonene (De Blasio et al., 2021; Miya et al., 2021). Along with their anti-obesity potential, these essential oil isolated bioactive phytoconstituents has demonstrated significant efficacy in respiratory diseases like asthma and AHR. Geraniol is reported to ameliorate allergic asthma by reducing eosinophils, Th2 cytokines like IL-4, 5 and 3 and increasing Th1 cytokine like interferon γ, Nrf2 protein expression, and reduced glutathione (Xue et al., 2016). β-Caryophyllene (BCP), a dietary bicyclic sesquiterpene cannabinoid-2 (CB2) receptor-ligand is repoeted to found mainly in the essential oils of Syzygium aromaticum (Prashar et al., 2006). BCP suppresses obesity-related AHR by its effects on macrophage polarization (inhibited by activation of AMPK, Nrf2/HO-1 and AdipoR1 and AdipoR2 signaling pathway, upregulation of adiponectin, glucagon-like peptide-1 (GLP-1), interferon-gamma (IFN-γ), superoxide dismutases (SOD), catalase, and downregulation of NF-κ-B, leptin, IL-4, TNF-α, and IL-1). The browning of eWAT through the promotion of thermogenesis and activation of the melanocortin pathway also had a role in the reduction of obesity-related AHR. The findings of the study demonstrated that BCP reduced obesity-related AHR by inhibiting macrophage polarisation, activating AMPK, activating Nrf2/HO-1, increasing AdipoR1 and AdipoR2 expression, and decreasing NFB expression in the lungs of animals (Pathak et al., 2021).
4 Conclusion and prospects
The state of being obese is the reason behind many diseases where obesity is either primarily responsible or exaggerates the ongoing diseased condition. The pathogenesis of asthma in obese individuals is different from the normal weight individuals due to different genotypes and so responds poorly to the conventional asthma treatment. Although several mechanisms have been proposed, however, the exact mechanism behind the exaggeration of asthma in obese asthmatics is yet to unfurl. Studies found that for a better therapeutic outcome in obesity-associated asthma, pathogenesis related to obesity should also be targeted along with the asthma for a better outcome. Herbal medicines are known to possess a myriad of therapeutic approach together with low adverse effects. Although several bioactive phytoconstituents has demonstrated potential efficacy against obesity-associated asthma in pre-clinical models, however their number is less. In depth studies are warranted to decipher the pinpoint mechanisms to design effective therapeutic approach targeting the obesity-associated asthma. Similarly, it is high time to explore promising bioactive phytoconstituents having proven anti-obesity efficacy for amelioration of obesity-associated asthma in clinical setup for better understanding of their mechanism of actions.
Author contributions
MP, KP, and AD: Conception and design. MP, KP, AD, RS, and UG: Writing and revising the manuscript. All authors have contributed to and approved this work.
Acknowledgments
The authors are thankful to Dibrugarh University, Dibrugarh, Assam, India, and Assam Down Town University, Guwahati, Assam, India, for providing all necessary facilities and support to carry out this work.
Conflict of interest
The authors declare that the research was conducted in the absence of any commercial or financial relationships that could be construed as a potential conflict of interest.
Publisher’s note
All claims expressed in this article are solely those of the authors and do not necessarily represent those of their affiliated organizations, or those of the publisher, the editors and the reviewers. Any product that may be evaluated in this article, or claim that may be made by its manufacturer, is not guaranteed or endorsed by the publisher.
References
Aguilera-Aguirre, L., Bacsi, A., Saavedra-Molina, A., Kurosky, A., Sur, S., and Boldogh, I. (2009). Mitochondrial dysfunction increases allergic airway inflammation. J. Immunol. 183 (8), 5379–5387. doi:10.4049/JIMMUNOL.0900228
Al-Mansoori, L., Al-Jaber, H., Prince, M. S., and Elrayess, M. A. (2022). Role of inflammatory cytokines, growth factors and adipokines in adipogenesis and insulin resistance. Inflammation 45, 31–44. doi:10.1007/s10753-021-01559-z
Alcalá, M., Sánchez-Vera, I., Sevillano, J., Herrero, L., Serra, D., Ramos, M. P., et al. (2015). Vitamin E reduces adipose tissue fibrosis, inflammation, and oxidative stress and improves metabolic profile in obesity. Obesity 23 (8), 1598–1606. doi:10.1002/oby.21135
Aleffi, S., Petrai, I., Bertolani, C., Parola, M., Colombatto, S., Novo, E., et al. (2005). Upregulation of proinflammatory and proangiogenic cytokines by leptin in human hepatic stellate cells. Hepatology 42, 1339–1348. doi:10.1002/HEP.20965
Ali, N. H., Rehman, S., Naqvi, M., Reshi, M. R., Gulati, K., and Ray, A. (2023). Withania somnifera extract ameliorates airway inflammation and oxidative stress in ovalbumin-induced bronchial asthma in rats. S. Afr. J. Bot. 155, 310–317. doi:10.1016/j.sajb.2023.02.003
Allen, S., Britton, J. R., and Leonardi-Bee, J. A. (2009). Association between antioxidant vitamins and asthma outcome measures: Systematic review and meta-analysis. Thorax 64, 610–619. doi:10.1136/thx.2008.101469
Alzaim, I., Hammoud, S. H., Al-Koussa, H., Ghazi, A., Eid, A. H., and El-Yazbi, A. F. (2020). Adipose tissue immunomodulation: A novel therapeutic approach in cardiovascular and metabolic diseases. Front. Cardiovasc. Med. 7, 602088. doi:10.3389/fcvm.2020.602088
Antczak, A., Nowak, D., Shariati, B., Król, M., Piasecka, G., and Kurmanowska, Z. (1997). Increased hydrogen peroxide and thiobarbituric acid-reactive products in expired breath condensate of asthmatic patients. Eur. Respir. J. 10, 1235–1241. doi:10.1183/09031936.97.10061235
Appel, K., Munoz, E., Navarrete, C., Cruz-Teno, C., Biller, A., and Thiemann, E. (2018). Immunomodulatory and inhibitory effect of Immulina®, and Immunloges® in the ig-E mediated activation of RBL-2H3 cells. A new role in allergic inflammatory responses. Plants (Basel) 7 (1), 13. doi:10.3390/plants7010013
Arora, P., Ansari, S. H., and Nainwal, L. M. (2021). Mesua ferrea L. (Calophyllaceae) exerts therapeutic effects in allergic asthma by modulating cytokines production in asthmatic rats. Turk. J. Bot. 45 (8), 820–832. doi:10.3906/bot-2111-22
Arora, P., Athari, S. S., and Nainwal, L. M. (2022b). Piperine attenuates production of inflammatory biomarkers, oxidative stress and neutrophils in lungs of cigarette smoke-exposed experimental mice. Food Biosci. 49, 101909. doi:10.1016/j.fbio.2022.101909
Arora, P., Nainwal, L. M., Gupta, G., Singh, S. K., Chellappan, D. K., Oliver, B. G., et al. (2022a). Orally administered solasodine, a steroidal glycoalkaloid, suppresses ovalbumin-induced exaggerated Th2-immune response in rat model of bronchial asthma. Chem. Biol. Interact. 366, 110138. doi:10.1016/j.cbi.2022.110138
Askin, L., Duman, H., Ozyıldız, A., Tanriverdi, O., and Turkmen, S. (2020). Association between omentin-1 and coronary artery disease: Pathogenesis and clinical research. Curr. Cardiol. Rev. 16, 198–201. doi:10.2174/1573403X16666200511085304
Bahceci, M., Gokalp, D., Bahceci, S., Tuzcu, A., Atmaca, S., and Arikan, S. (2007). The correlation between adiposity and adiponectin, tumor necrosis factor α, interleukin-6 and high sensitivity C-reactive protein levels. Is adipocyte size associated with inflammation in adults? J. Endocrinol. Invest. 30, 210–214. doi:10.1007/BF03347427
Balsano, C., and Alisi, A. (2009). Antioxidant effects of natural bioactive compounds. Curr. Pharmaceu. Des. 15, 3063–3073. doi:10.2174/138161209789058084
Bhatraju, N. K., and Agrawal, A. (2017). Mitochondrial dysfunction linking obesity and asthma. Ann. Am. Thorac. Soc. 14 (5), S368–S373. doi:10.1513/ANNALSATS.201701-042AW
Bi, P., Shan, T., Liu, W., Yue, F., Yang, X., Liang, X. R., et al. (2014). Inhibition of Notch signaling promotes browning of white adipose tissue and ameliorates obesity. Nat. Med. 20 (8), 911–918. doi:10.1038/nm.3615
Bianco, A., Nigro, E., Monaco, M. L., Matera, M. G., Scudiero, O., Mazzarella, G., et al. (2017). The burden of obesity in asthma and COPD: Role of adiponectin. Pulm. Pharmacol. Ther. 43, 20–25. doi:10.1016/j.pupt.2017.01.004
Bisgaard, H., Hermansen, M. N., Buchvald, F., Loland, L., Halkjaer, L. B., Bønnelykke, K., et al. (2007). Childhood asthma after bacterial colonization of the airway in neonates. N. Engl. J. Med. 357 (15), 1487–1495. doi:10.1056/NEJMoa052632
Biswas, S., and Rahman, I. (2008). Modulation of steroid activity in chronic inflammation: A novel antiinflammatory role for curcumin. Mol. Nutr. Food Res. 52, 987–994. doi:10.1002/mnfr.200700259
Bokarewa, M., Nagaev, I., Dahlberg, L., Smith, U., and Tarkowski, A. (2005). Resistin, an adipokine with potent proinflammatory properties. J. Immunol. 174, 5789–5795. doi:10.4049/JIMMUNOL.174.9.5789
Bousquet, J., Chanez, P., Lacoste, J. Y., Barneon, G., Ghavanian, N., Enander, I., et al. (1990). Eosinophilic inflammation in asthma. N. Engl. J. Med. 323, 1033–1039. doi:10.1056/NEJM199010113231505
Brouet, I., and Ohshima, H. (1995). Curcumin, an anti-tumour promoter and anti-inflammatory agent, inhibits induction of nitric oxide synthase in activated macrophages. Biochem. Biophys. Res. Commun. 206, 533–540. doi:10.1006/bbrc.1995.1076
Bustamante, J., Lodge, J. K., Marcocci, L., Tritschler, H. J., Packer, L., and Rihn, B. H. (1998). Alpha-lipoic acid in liver metabolism and disease. Free Radic. Biol. Med. 24, 1023–1039. doi:10.1016/s0891-5849(97)00371-7
Camell, C. D., Nguyen, K. Y., Jurczak, M. J., Christian, B. E., Shulman, G. I., Shadel, G. S., et al. (2015). Macrophage-specific de Novo synthesis of ceramide is dispensable for inflammasome-driven inflammation and insulin resistance in obesity. J. Biol. Chem. 290, 29402–29413. doi:10.1074/jbc.M115.680199
Cannon, B., and Nedergaard, J. (2004). Brown adipose tissue: Function and physiological significance. Physiol. Rev. 84, 277–359. doi:10.1152/physrev.00015.2003
Cao, X., and Phillis, J. W. (1995). The free radical scavenger, alpha-lipoic acid, protects against cerebral ischemia-reperfusion injury in gerbils. Free Radic. Res. 23, 365–370. doi:10.3109/10715769509065257
Catani, M. V., Rossi, A., Costanzo, A., Sabatini, S., Levrero, M., Melino, G., et al. (2001). Induction of gene expression via activator protein-1 in the ascorbate protection against UVinduced damage. Biochem. J. 356, 77–85. doi:10.1042/0264-6021:3560077
Cawthorn, W. P., Heyd, F., Hegyi, K., and Sethi, J. K. (2007). Tumour necrosis factor-alpha inhibits adipogenesis via a beta-catenin/TCF4(TCF7L2)-dependent pathway. Cell Death Differ. 147, 1361–1373. doi:10.1038/sj.cdd.4402127
Cawthorn, W. P., and Sethi, J. K. (2008). TNF-alpha and adipocyte biology. FEBS Lett. 582, 117–131. doi:10.1016/J.FEBSLET.2007.11.051
Chen, B., Wei, J., Wang, W., Cui, G., Zhao, Y., Zhu, X., et al. (2009). Identification of signaling pathways involved in aberrant production of adipokines in adipocytes undergoing oxidative stress. Arch. Med. Res. 40, 241–248. doi:10.1016/j.arcmed.2009.03.007
Choi, Y., Kim, M., Kim, S. J., Yoo, H. J., Kim, S. H., and Park, H. S. (2020). Metabolic shift favoring C18: 0 ceramide accumulation in obese asthma. Allergy 75 (11), 2858–2866. doi:10.1111/all.14366
Chong, L., Li, H., Zhu, L., and Yu, G. (2021). Regulatory effect of mitoQ on the mtROS-NLRP3 inflammasome pathway in leptin-pretreated BEAS-2 cells. Exp. Ther. Med. 21 (5), 466–469. doi:10.3892/etm.2021.9897
Chong, L., Liu, L., Zhu, L., Li, H., Shao, Y., Zhang, H., et al. (2019). Expression levels of predominant adipokines and activations of STAT3, STAT6 in an experimental mice model of obese asthma. Iran. J. Allergy, Asthma Immunol. 18, 62–71. doi:10.18502/ijaai.v18i1.631
Chouchani, E. T., Kazak, L., and Spiegelman, B. M. (2019). New advances in adaptive thermogenesis: UCP1 and beyond. Cell Metab. 29 (1), 27–37. doi:10.1016/J.CMET.2018.11.002
Curat, C. A., Miranville, A., Sengenes, C., Diehl, M., Tonus, C., Busse, R., et al. (2004). From blood monocytes to adipose tissue-resident macrophages. Diabetes 53 (5), 12851292. doi:10.2337/diabetes.53.5.1285
Dajnowicz-Brzezika, P., Żebrowskaa, E., Maciejczykb, M., Zalewskac, A., and Chabowskia, A. (2022). The effect of α-lipoic acid on oxidative stress in adipose tissue of rats with obesity-induced insulin resistance. Cell Physiol. Biochem. 56, 239–253. doi:10.33594/000000528
Dalgaard, L. T., and Pedersen, O. (2001). Uncoupling proteins: Functional characteristics and role in the pathogenesis of obesity and Type II diabetes. Diabetologia 44, 946–965. doi:10.1007/s001250100596
Das, S., and Das, D. K. (2007). Resveratrol: A therapeutic promise for cardiovascular diseases. Recent pat.cardiovasc. Drug Discov. 2, 133–138. doi:10.2174/157489007780832560
De Blasio, A., D’Anneo, A., Lauricella, M., Emanuele, S., Giuliano, M., Pratelli, G., et al. (2021). The beneficial effects of essential oils in anti-obesity treatment. Int. J. Mol. Sci. 22 (21), 11832. doi:10.3390/ijms222111832
De Monchy, J. G., Kauffman, H. F., Venge, P., Koeter, G. H., Jansen, H. M., Sluiter, H. J., et al. (1985). Bronchoalveolar eosinophilia during allergeninduced late asthmatic reactions. Am. Rev. Respir. Dis. 131, 373–376. doi:10.1164/arrd.1985.131.3.373
Devarah, S., Harris, A., and Jialal, I. (2002). Modulation of monocyte-macrophage function with alpha-tocopherol: Implications for atherosclerosis. Nutr. Rev. 60, 8–14. doi:10.1301/002966402760240381
Devaraj, S., Wang-Polagruto, J., Polagruto, J., Keen, C. L., and Jialal, I. (2008). High-fat, energydense, fast-food-style breakfast results in an increase in oxidative stress in metabolic syndrome. Metab. Clin. Exp. 57, 867–870. doi:10.1016/j.metabol.2008.02.016
Dohlman, A. W., Black, H. R., and Royall, J. A. (1993). Expired breath hydrogen peroxide is a marker of acute airway inflammation in pediatric patients with asthma. Am. Rev. Respir. 148, 955–960. doi:10.1164/ajrccm/148.4_Pt_1.955
Enomoto, T., Ohashi, K., Shibata, R., Higuchi, A., Maruyama, S., Izumiya, Y., et al. (2011). Adipolin/C1qdc2/CTRP12 protein functions as an adipokine that improves glucose metabolism. J. Biol. Chem. 286, 34552–34558. doi:10.1074/jbc.M111.277319
Evavold, C. L., Ruan, J., Tan, Y., Xia, S., Wu, H., and Kagan, J. C. (2018). The pore-forming protein gasdermin D regulates interleukin-1 secretion from living macrophages. Immunity 48, 35–44. doi:10.1016/J.IMMUNI.2017.11.013
Fantuzzi, G. (2005). Adipose tissue, adipokines, and inflammation. J. Allergy Clin. Immunol. 115 (5), 911–919. doi:10.1016/J.JACI.2005.02.023
Friedman, J. M., and Halaas, J. L. (1998). Leptin and the regulation of body weight in mammals. Nat 395, 763–770. doi:10.1038/27376
Fu, J., Bonder, M. J., Cenit, M. C., Tigchelaar, E. F., Maatman, A., Dekens, J. A. M., et al. (2015). The gut microbiome contributes to a substantial proportion of the variation in blood lipids. Circ. Res. 117 (9), 817–824. doi:10.1161/CIRCRESAHA.115.306807
Fu, S., Liu, L., Han, L., and Yu, Y. (2017). Leptin promotes IL-18 secretion by activating the NLRP3 inflammasome in RAW 264.7 cells. Mol. Med. Rep. 16 (6), 9770–9776. doi:10.3892/mmr.2017.7797
Gainsford, T., Willson, T. A., Metcalf, D., Handman, E., Mcfarlane, C., Ng, A., et al. (1996). Leptin can induce proliferation, differentiation, and functional activation of hemopoietic cells. Proc. Natl. Acad. Sci. U. S. A. 93, 14564–14568. doi:10.1073/PNAS.93.25.14564
Gallicchio, L., Boyd, K., Matanoski, G., Tao, X. G., Chen, L., Lam, T. K., et al. (2008). Carotenoids and the risk of developing lung cancer: A systematic review. Am. J. Clin. Nutr. 88, 372–383. doi:10.1093/ajcn/88.2.372
Gan, L., Guo, K., Cremona, M. L., McGraw, T. E., Leibel, R. L., and Zhang, Y. (2012). TNF-α up-regulates protein level and cell surface expression of the leptin receptor by stimulating its export via a PKC-dependent mechanism. Endocrinology 153, 5821–5833. doi:10.1210/EN.2012-1510
Garcia-Diaz, D. F., Lopez-Legarrea, P., Quintero, P., and Martinez, J. A. (2014). Vitamin C in the treatment and/or prevention of obesity. J. Nutri. Sci. Vitaminol. 60 (6), 367–379. doi:10.3177/jnsv.60.367
Global Initiative for Asthma (2020). Global strategy for asthma management and prevention. Available at: www.ginasthma.org (Accessed January 27, 2023).
Goit, R. K., Taylor, A. W., and Lo, A. C. Y. (2022). The central melanocortin system as a treatment target for obesity and diabetes: A brief overview. Eur. J. Pharmacol. 924, 174956. doi:10.1016/j.ejphar.2022.174956
Green, B. J., Wiriyachaiporn, S., Grainge, C., Rogers, G. B., Kehagia, V., Lau, L., et al. (2014). Potentially pathogenic airway bacteria and neutrophilic inflammation in treatment resistant severe asthma. PLoS One 9 (6), e100645. doi:10.1371/journal.pone.0100645
Grunfeld, C., Zhao, C., Fuller, J., Pollock, A., Moser, A., Friedman, J., et al. (1996). Endotoxin and cytokines induce expression of leptin, the ob gene product, in hamsters. J. Clin. Invest. 97, 2152–2157. doi:10.1172/JCI118653
Halliwell, B., Gutteridge, J. M., and Cross, C. E. (1992). Free radicals, antioxidants, and human disease: Where are we now? J. Lab. Clin. Med. 119, 598–620.
Harborne, J. B., and Williams, C. A. (2000). Advances in flavonoid research since 1992. Phytochemistry 55, 481–504. doi:10.1016/s0031-9422(00)00235-1
Harms, M., and Seale, P. (2013). Brown and beige fat: Development, function and therapeutic potential. Nat. Med. 19 (10), 1252–1263. doi:10.1038/nm.3361
Hattori, Y., Akimoto, K., Gross, S. S., Hattori, S., and Kasai, K. (2005). Angiotensin-II-induced oxidative stress elicits hypoadiponectinaemia in rats. Diabetologia 48, 1066–1074. doi:10.1007/s00125-005-1766-7
He, J., Le, Q., Wei, Y., Yang, L., Cai, B., Liu, Y., et al. (2022a). Effect of piperine on the mitigation of obesity associated with gut microbiota alteration. Curr. Res. Nutr. Food Sci. 5, 1422–1432. doi:10.1016/j.crfs.2022.08.018
He, J., Xu, J.-Y., Gu, J., Tong, X., Wan, Z., Gu, Y., et al. (2022b). Piperine is capable of improving pancreatic β-cell apoptosis in high fat diet and streptozotocin induced diabetic mice. J. Funct. Foods 88, 104890. doi:10.1016/j.jff.2021.104890
Herath, K., Kim, H. J., Jang, J. H., Kim, H. S., Kim, H. J., Jeon, Y. J., et al. (2020). Mojabanchromanol isolated from Sargassum horneri attenuates particulate matter induced inflammatory responses via suppressing TLR2/4/7-MAPK signaling in MLE-12 cells. Mar. Drugs 18 (7), 355. doi:10.3390/md18070355
Hilty, M., Burke, C., Pedro, H., Cardenas, P., Bush, A., Bossley, C., et al. (2010). Disordered microbial communities in asthmatic airways. PLoS One 5 (1), e8578. doi:10.1371/journal.pone.0008578
Holcomb, I. N., Kabakoff, R. C., Chan, B., Baker, T. W., Gurney, A., Henzel, W., et al. (2000). FIZZ1, a novel cysteine-rich secreted protein associated with pulmonary inflammation, defines a new gene family. EMBO J. 19, 4046–4055. doi:10.1093/EMBOJ/19.15.4046
Hollman, P. C., and Katan, M. B. (1999). Health effects and bioavailability of dietary flavonols. Free Rad. Res. 31, S75–S80. doi:10.1080/10715769900301351
Hosny, S. S., Farres, M. N., Melek, N. A., Kamal, S. T., El Najjar, M. R., Abou El Fotoh, R. H., et al. (2021). Assessment of serum levels of adiponectin and resistin in adult patients with asthma: Relation to obesity and disease severity. Egypt. J. Chest Dis. Tuberc. 70 (2), 223.
Isakson, P., Hammarstedt, A., Gustafson, B., and Smith, U. (2009). Impaired preadipocyte differentiation in human abdominal obesity: Role of wnt, tumor necrosis factor-alpha, and inflammation. Diabetes 58, 1550–1557. doi:10.2337/DB08-1770
Jarjour, N. N., Busse, W. W., and Calhoun, W. J. (1992). Enhanced production of oxygen radicals in nocturnal asthma. Am. Rev. Respir. Dis. 146, 905–911. doi:10.1164/ajrccm/146.4.905
Johnson, J. B., Summer, W., Cutler, R. G., Martin, B., Hyun, D-H., Dixit, V. D., et al. (2007). Alternate day calorie restriction improves clinical findings and reduces markers of oxidative stress and inflammation in overweight adults with moderate asthma. Free Radic. Biol. Med. 42, 665–674. doi:10.1016/j.freeradbiomed.2006.12.005
Jorge, A. S. B., Jorge, G. C. B., Paraíso, A. F., Franco, R. M. P., Vieira, L. J. T., Hilzenderger, A. M., et al. (2017). Brown and white adipose tissue expression of IL6, UCP1 and SIRT1 are associated with alterations in clinical, metabolic and anthropometric parameters in obese humans. Exp. Clin. Endocrinol. Diabetes 125 (3), 163–170. doi:10.1055/s-0042-119525
Kambara, T., Ohashi, K., Shibata, R., Ogura, Y., Maruyama, S., Enomoto, T., et al. (2012). CTRP9 protein protects against myocardial injury following ischemia-reperfusion through AMP-activated protein kinase (AMPK)-dependent mechanism. J. Biol. Chem. 287, 18965–18973. doi:10.1074/JBC.M112.357939
Kannaiyan, R., Shanmugam, M. K., and Sethi, G. (2011). Molecular targets of celastrol derived from thunder of god vine: Potential role in the treatment of inflammatory disorders and cancer. Cancer Lett. 303 (1), 9–20. doi:10.1016/j.canlet.2010.10.025
Karasawa, T., Kawashima, A., Usui-Kawanishi, F., Watanabe, S., Kimura, H., Kamata, R., et al. (2018). Saturated fatty acids undergo intracellular crystallization and activate the NLRP3 inflammasome in macrophages. Arterioscler. Thromb. Vasc. Biol. 38, 744–756. doi:10.1161/ATVBAHA.117.310581
Kasai, S., Shimizu, S., Tatara, Y., Mimura, J., and Itoh, K. (2020). Regulation of Nrf2 by mitochondrial reactive oxygen species in physiology and pathology. Biomolecules 10 (2), 320. doi:10.3390/biom10020320
Kaur, B., Rowe, B. H., and Arnold, E. (2009). Vitamin C supplementation for asthma. Cochrane Database syst.rev. 1, CD000993. doi:10.1002/14651858.CD000993
Kim, C. H. (2021). Control of lymphocyte functions by gut microbiota-derived short-chain fatty acids. Cell. Mol. Immunol. 18 (5), 1161–1171. doi:10.1038/s41423-020-00625-0
Kim, J. A., Wei, Y., and Sowers, J. R. (2008). Role of mitochondrial dysfunction in insulin resistance. Circ. Res. 102 (4), 401–414. doi:10.1161/CIRCRESAHA.107.165472
Kim, J. Y., Sohn, J. H., Choi, J. M., Lee, J. H., Hong, C. S., Lee, J. S., et al. (2012). Alveolar macrophages play a key role in cockroach-induced allergic inflammation via TNF-α pathway. PLoS One 7, e47971. doi:10.1371/JOURNAL.PONE.0047971
Kim, J. Y., Sohn, J. H., Lee, J. H., and Park, J. W. (2015). Obesity increases airway hyperresponsiveness via the TNF-α pathway and treating obesity induces recovery. PLoS One 10 (2), e0116540. doi:10.1371/journal.pone.0116540
Kim, M. J., Lee, W., Park, E. J., and Park, S. Y. (2010). C1qTNF-Related protein-6 increases the expression of interleukin-10 in macrophages. Mol. Cells 30, 59–64. doi:10.1007/S10059-010-0088-X
Kim, R. Y., Pinkerton, J. W., Essilfie, A. T., Robertson, A. A., Baines, K. J., Brown, A. C., et al. (2017). Role for NLRP3 inflammasome–mediated, IL-1β–dependent responses in severe, steroid-resistant asthma. Am. J. Respir. Crit. Care Med. 196 (3), 283–297. doi:10.1164/rccm.201609-1830OC
Koebnick, C., Fischer, H., Daley, M. F., Ferrara, A., Horberg, M. A., Waitzfelder, B., et al. (2016). Interacting effects of obesity, race, ethnicity and sex on the incidence and control of adult-onset asthma. Allergy Asthma Clin. Immunol. 12 (1), 50. doi:10.1186/s13223-016-0155-8
Koves, T. R., Ussher, J. R., Noland, R. C., Slentz, D., Mosedale, M., Ilkayeva, O., et al. (2008). Mitochondrial overload and incomplete fatty acid oxidation contribute to skeletal muscle insulin resistance. Cell Metab. 7 (1), 45–56. doi:10.1016/J.CMET.2007.10.013
Kozak, L. P., and Anunciado-Koza, R. (2008). UCP1: Its involvement and utility in obesity. Int. J. Obes. (Lond). 32, S32–S38. doi:10.1038/IJO.2008.236
Kuo, C. Y., Huang, W. C., Liou, C. J., Chen, L. C., Shen, J. J., and Kuo, M. L. (2017). Tomatidine attenuates airway hyperresponsiveness and inflammation by suppressing Th2 cytokines in a mouse model of asthma. Mediat. Inflamm. 2017, 5261803. doi:10.1155/2017/5261803
Kurup, V. P., and Barrios, C. S. (2008). Immunomodulatory effects of curcumin in allergy. Mol. Nutr. Food Res. 52, 1031–1039. doi:10.1002/mnfr.200700293
Kwon, H., and Pessin, J. E. (2013). Adipokines mediate inflammation and insulin resistance. Front. Endocrinol. (Lausanne). 4, 71. doi:10.3389/fendo.2013.00071
Lam, C. W., Getting, S. J., and Perretti, M. (2005). In vitro and in vivo induction of heme oxygenase 1 in mouse macrophages following melanocortin receptor activation. J. Immunol. 174, 2297–2304. doi:10.4049/jimmunol.174.4.2297
Le, Y., and Pang, S. (2006). The role of FAM3B in turmorgenesis view project role of resistin in inflammation and inflammation-related diseases. Available at: https://www.researchgate.net/publication/7230027 (Accessed December 29, 2022).
Lee, J. H., Koo, T. H., Yoon, H., Jung, H. S., Jin, H. Z., Lee, K., et al. (2006). Inhibition of NF-kappa B activation through targeting I kappa B kinase by celastrol, a quinone methide triterpenoid. Biochem. Pharmacol. 72 (10), 1311–1321. doi:10.1016/j.bcp.2006.08.014
Lee, T.-H., Song, H. J., and Park, C.-S. (2014). Role of inflammasome activation in development and exacerbation of asthma. Asia Pac. Allergy 4, 187–196. doi:10.5415/APALLERGY.2014.4.4.187
Li, D., Wu, Y., Tian, P., Zhang, X., Wang, H., Wang, T., et al. (2015). Adipokine CTRP-5 as a potential novel inflammatory biomarker in chronic obstructive pulmonary disease. Medicine 94 (36), e1503. doi:10.1097/md.0000000000001503
Li, R. R., Pang, L. L., Du, Q., Shi, Y., Dai, W. J., and Yin, K. S. (2010). Apigenin inhibits allergen-induced airway inflammation and switches immune response in a murine model of asthma.Immunopharmacol. Immunotoxicology 32, 364–370. doi:10.3109/08923970903420566
Li, X. N., Ma, L. Y., Ji, H., Qin, Y. H., Jin, S. S., and Xu, L. X. (2018). Resveratrol protects against oxidative stress by activating the Keap-1/Nrf2 antioxidant defense system in obese-asthmatic rats. Exp. Ther. Med. 16 (6), 4339–4348. doi:10.3892/etm.2018.6747
Lin, Q., and Johns, R. A. (2020). Resistin family proteins in pulmonary diseases. Am. J. Physiol. Lung Cell Mol. Physiol. 319 (3), L422–L434. doi:10.1152/ajplung.00040.2020
Liu, J., Lee, J., Hernandez, M. A. S., Mazitschek, R., and Ozcan, U. (2015). Treatment of obesity with celastrol. Cell 161 (5), 999–1011. doi:10.1016/j.cell.2015.05.011
Liu, R., Hong, J., Xu, X., Feng, Q., Zhang, D., Gu, Y., et al. (2017). Gut microbiome and serum metabolome alterations in obesity and after weight-loss intervention. Nat. Med. 23 (7), 859–868. doi:10.1038/nm.4358
Liu, X., Bai, L., Li, L., Piao, X., and Yu, J. (2023). A practical strategy for exploring the pharmacological mechanism of chrysophanol against obesity asthma/childhood asthma comorbidity. United States: Wiley.
Liu, X., Zhang, Z., Ruan, J., Pan, Y., Magupalli, V. G., Wu, H., et al. (2016). Inflammasome-activated gasdermin D causes pyroptosis by forming membrane pores. Nat 535, 153–158. doi:10.1038/nature18629
López-Reyes, A., Martinez-Armenta, C., Espinosa-Velázquez, R., Vázquez-Cárdenas, P., Cruz-Ramos, M., Palacios-Gonzalez, B., et al. (2020). NLRP3 inflammasome: The stormy link between obesity and COVID-19. Front. Immunol. 11, 570251. doi:10.3389/fimmu.2020.570251
Lord, G. M., Matarese, G., Howard, J. K., Baker, R. J., Bloom, S. R., and Lechler, R. I. (1998). Leptin modulates the T-cell immune response and reverses starvation-induced immunosuppression. Nat 394, 897–901. doi:10.1038/29795
Lumeng, C. N., and Saltiel, A. R. (2011). Inflammatory links between obesity and metabolic disease. J. Clin. Investig. 121 (6), 2111–2117. doi:10.1172/jci57132
Luptak, I., Qin, F., Sverdlov, A. L., Pimentel, D. R., Panagia, M., Croteau, D., et al. (2019). Energetic dysfunction is mediated by mitochondrial reactive oxygen species and precedes structural remodeling in metabolic heart disease. Antioxid. Redox Signal. 31 (7), 539–549. doi:10.1089/ARS.2018.7707
Mabalirajan, U., Aich, J., Leishangthem, G. D., Sharma, S. K., Dinda, A. K., and Ghosh, B. (2009). Effects of vitamin E on mitochondrial dysfunction and asthma features in an experimental allergic murine model. J. Appl. Physiology 107 (4), 1285–1292. doi:10.1152/japplphysiol.00459.2009
Mabalirajan, U., Dinda, A. K., Kumar, S., Roshan, R., Gupta, P., Sharma, S. K., et al. (2008). Mitochondrial structural changes and dysfunction are associated with experimental allergic asthma. J. Immunol. 181 (5), 3540–3548. doi:10.4049/JIMMUNOL.181.5.3540
Machado, M. E., Porto, L. C., Alves Galvão, M. G., Sant’Anna, C. C., and Lapa e Silva, J. R. (2022). SNPs, adipokynes and adiposity in children with asthma. J. Asthma 60 (3), 446–457. doi:10.1080/02770903.2022.2077218
MacNee, W. (2001). Oxidative stress and lung inflammation in airways disease. Eur. J. Pharmacol. 429, 195–207. doi:10.1016/s0014-2999(01)01320-6
McGillis, J. P. (2005). White adipose tissue, inert No more. Endocrinology 146, 2154–2156. doi:10.1210/EN.2005-0248
Middleton, E. J., Kandaswami, C., and Theoharides, T. C. (2000). The effects of plant flavonoids on mammalian cells: Implications for inflammation, heart disease, and cancer. Pharmacol. Rev. 52, 673–751.
Miya, G., Nyalambisa, M., Oyedeji, O., Gondwe, M., and Oyedeji, A. (2021). Chemical profiling, toxicity and anti-inflammatory activities of essential oils from three grapefruit cultivars from KwaZulu-Natal in South Africa. Molecules 26 (11), 3387. doi:10.3390/molecules26113387
Miyazawa, T., Burdeos, G. C., Itaya, M., Nakagawa, K., and Miyazawa, T. (2019). Vitamin E: Regulatory redox interactions. IUBMB life 71 (4), 430–441. doi:10.1002/iub.2008
Montague, C. T., and O’Rahilly, S. (2000). The perils of portliness: Causes and consequences of visceral adiposity. Diabetes 49, 883–888. doi:10.2337/diabetes.49.6.883
Moriguchi, S., and Muraga, M. (2000). Vitamin E and immunity. Vitam. Horm. 59, 305–336. doi:10.1016/s0083-6729(00)59011-6
Mortellini, R., Foresti, R., Bassi, R., and Green, C. J. (2000). Curcumin, an antioxidant and anti-inflammatory agent, induces heme oxygenase-1 and protects endothelial cells against oxidative stress. Free Radic. Biol. Med. 28, 1301–1312.
Murugesan, S., Ulloa-Martínez, M., Martínez-Rojano, H., Galván-Rodríguez, F. M., Miranda-Brito, C., Romano, M. C., et al. (2015). Study of the diversity and short-chain fatty acids production by the bacterial community in overweight and obese Mexican children. Eur. J. Clin. Microbiol. Infect. Dis. 34 (7), 1337–1346. doi:10.1007/s10096-015-2355-4
Namazi, N., Larijani, B., and Azadbakht, L. (2018). Alpha-lipoic acid supplement in obesity treatment: A systematic review and meta-analysis of clinical trials. Clin. Nutr. 37 (2), 419–428. doi:10.1016/j.clnu.2017.06.002
Norton, S. A. (1998). Useful plants of dermatology. III. Corticosteroids, strophanthus, and dioscorea. J. Am. Acad. Dermatol. 38, 256–259. doi:10.1016/s0190-9622(98)70245-2
Ohashi, K., Shibata, R., Murohara, T., and Ouchi, N. (2014). Role of anti-inflammatory adipokines in obesity-related diseases. Trends Endocrinol. Metab. 25, 348–355. doi:10.1016/j.tem.2014.03.009
Okamatsu-Ogura, Y., Kuroda, M., Tsutsumi, R., Tsubota, A., Saito, M., Kimura, K., et al. (2020). UCP1-dependent and UCP1-independent metabolic changes induced by acute cold exposure in Brown adipose tissue of mice. Metabolism 113, 154396. doi:10.1016/j.metabol.2020.154396
Okla, M., Zaher, W., Alfayez, M., and Chung, S. (2018). Inhibitory effects of toll-like receptor 4, NLRP3 inflammasome, and interleukin-1β on white adipocyte browning. Inflammation 41, 626–642. doi:10.1007/s10753-017-0718-y
Olopade, C. O., Zakkar, M., Swedler, W. I., and Rubinstein, I. (1997). Exhaled pentane levels in acute asthma. Chest 111, 862–865. doi:10.1378/chest.111.4.862
Ouchi, N., Parker, J. L., Lugus, J. J., and Walsh, K. (2011). Adipokines in inflammation and metabolic disease. Nat. Rev. Immunol. 112, 85–97. doi:10.1038/nri2921
Palma, G., Sorice, G. P., Genchi, V. A., Giordano, F., Caccioppoli, C., D’Oria, R., et al. (2022). Adipose tissue inflammation and pulmonary dysfunction in obesity. Int. J. Mol. Sci. 23 (13), 7349. doi:10.3390/ijms23137349
Park, H. S., Kim, S. R., and Lee, Y. C. (2009). Impact of oxidative stress on lung diseases. Respirology 14, 27–38. doi:10.1111/j.1440-1843.2008.01447.x
Pathak, M. P., Das, A., Patowary, P., and Chattopadhyay, P. (2020). Contentious role of ‘Good Adiponectin’in pulmonary and cardiovascular diseases: Is adiponectin directed therapy a boon or a bane? Biochimie 175, 106–119. doi:10.1016/j.biochi.2020.05.008
Pathak, M. P., Patowary, P., Goyary, D., Das, A., and Chattopadhyay, P. (2021). β-caryophyllene ameliorated obesity-associated airway hyperresponsiveness through some non-conventional targets. Phytomedicine 89, 153610. doi:10.1016/j.phymed.2021.153610
Pattnaik, B., Bodas, M., Bhatraju, N. K., Ahmad, T., Pant, R., Guleria, R., et al. (2016). IL-4 promotes asymmetric dimethylarginine accumulation, oxo-nitrative stress, and hypoxic response–induced mitochondrial loss in airway epithelial cells. J. Allergy Clin. Immunol. 138 (1), 130–141. doi:10.1016/J.JACI.2015.11.036
Pearson, P. J., Lewis, S. A., Britton, J., and Fogarty, A. (2004). Vitamin E supplements in asthma: A parallel group randomised placebo controlled trial. Thorax 59, 652–656. doi:10.1136/thx.2004.022616
Peerboom, S., Graff, S., Seidel, L., Paulus, V., Henket, M., Sanchez, C., et al. (2020). Predictors of a good response to inhaled corticosteroids in obesity-associated asthma. Biochem. Pharmacol. 179, 113994. doi:10.1016/j.bcp.2020.113994
Peters, M. C., McGrath, K. W., Hawkins, G. A., Hastie, A. T., Levy, B. D., Israel, E., et al. (2016). Plasma interleukin-6 concentrations, metabolic dysfunction, and asthma severity: A cross-sectional analysis of two cohorts. Lancet Resp. medi. 4 (7), 574–584. doi:10.1016/S2213-2600(16)30048-0
Peters, U., Dixon, A. E., and Forno, E. (2018). Obesity and asthma. J. Allergy Clin. Immunol. 141 (4), 1169–1179. doi:10.1016/j.jaci.2018.02.004
Picado, C., Deulofeu, R., Lleonart, R., Agusti, M., Mullol, J., Quintó, L., et al. (2001). Dietary.micronutrients/antioxidants and their relationship with bronchial asthma severity. Allergy 56, 43–49. doi:10.1034/j.1398-9995.2001.00793.x
Pinkerton, J. W., Kim, R. Y., Brown, A. C., Rae, B. E., Donovan, C., Mayall, J. R., et al. (2022). Relationship between type 2 cytokine and inflammasome responses in obesity-associated asthma. J. Allergy Clin. Immunol. 149 (4), 1270–1280. doi:10.1016/j.jaci.2021.10.003
Pirola, L., and Fröjdö, S. (2008). Resveratrol: One molecule, many targets. IUBMB Life 60, 323–332. doi:10.1002/iub.47
Pletsityi, K. D., Vasipa, S. B., Daydova, T. V., and Fomina, V. G. (1995). Vitamin E: Immunocorrecting effect in bronchial asthma patients. Vopr. Med. Khim. 41, 33–36.
Prashar, A., Locke, I. C., and Evans, C. S. (2006). Cytotoxicity of clove (Syzygium aromaticum) oil and its major components to human skin cells. Cell Prolif. 39 (4), 241–248. doi:10.1111/j.1365-2184.2006.00384.x
Price, N. L., Gomes, A. P., Ling, A. J., Duarte, F. V., Martin-Montalvo, A., North, B. J., et al. (2012). SIRT1 is required for AMPK activation and the beneficial effects of resveratrol on mitochondrial function. Cell metab. 15 (5), 675–690. doi:10.1016/j.cmet.2012.04.003
Prior, R. L., and Cao, G. (2000). Antioxidant phytochemicals in fruits and vegetables: Diet and health implications. HortScience 35, 588–592. doi:10.21273/hortsci.35.4.588
Raap, U., Brzoska, T., Sohl, S., Path, G., Emmel, J., Herz, U., et al. (2003). Alpha-melanocyte-stimulating hormone inhibits allergic airway inflammation. J. Immunol. 171 (1), 353–359. doi:10.4049/jimmunol.171.1.353
Rahat-Rozenbloom, S., Fernandes, J., Gloor, G. B., and Wolever, T. M. S. (2014). Evidence for greater production of colonic short-chain fatty acids in overweight than lean humans. Int. J. Obes. (Lond). 38 (12), 1525–1531. doi:10.1038/ijo.2014.46
Rajamaki, K., Lappalainen, J., Öörni, K., Välimäki, E., Matikainen, S., Kovanen, P. T., et al. (2010). Cholesterol crystals activate the NLRP3 inflammasome in human macrophages: A novel link between cholesterol metabolism and inflammation. PLoS One 5, e11765. doi:10.1371/JOURNAL.PONE.0011765
Rao, A. V., and Rao, L. G. (2007). Carotenoids and human health. Pharmacol. Res. 55, 207–216. doi:10.1016/j.phrs.2007.01.012
Rathinam, V. A. K., Vanaja, S. K., and Fitzgerald, K. A. (2012). Regulation of inflammasome signaling. Nat. Immunol. 134 13, 333–342. doi:10.1038/ni.2237
Riccioni, G., and D’Orazio, N. (2005). The role of selenium, zinc and antioxidant vitamin supplementation in the treatment of bronchial asthma: Adjuvant therapy or not? Expert Opin. Investig. Drugs 14, 1145–1155. doi:10.1517/13543784.14.9.1145
Ricquier, D. (2011). Uncoupling protein 1 of Brown adipocytes, the only uncoupler: A historical perspective. Front. Endocrinol. (Lausanne) 2, 85. doi:10.3389/fendo.2011.00085
Roggenbuck, M., Anderson, D., Barfod, K. K., Feelisch, M., Geldenhuys, S., Sørensen, S. J., et al. (2016). Vitamin D and allergic airway disease shape the murine lung microbiome in a sex-specific manner. Respir. Res. 17 (1), 116. doi:10.1186/s12931-016-0435-3
Romieu, I., and Trenga, C. (2001). Diet and obstructive lung diseases. Epidemiol. Rev. 23 (2), 268–287. doi:10.1093/oxfordjournals.epirev.a000806
Ross, D. A., Rao, P. K., and Kadesch, T. (2004). Dual roles for the Notch target gene Hes-1 in the differentiation of 3T3-L1 preadipocytes. Mol. Cell. Biol. 24 (8), 3505–3513. doi:10.1128/mcb.24.8.3505-3513.2004
Russo, L., and Lumeng, C. N. (2018). Properties and functions of adipose tissue macrophages in obesity. Immunology 155 (4), 407–417. doi:10.1111/imm.13002
Saito, M., Matsushita, M., Yoneshiro, T., and Okamatsu-Ogura, Y. (2020). Brown adipose tissue, diet-induced thermogenesis, and thermogenic food ingredients: From mice to men. Front. Endocrinol. (Lausanne) 11, 222. doi:10.3389/fendo.2020.00222
Saleh, D., Ernst, P., Lim, S., Barnes, P. J., and Giaid, A. (1998). Increased formation of the potent oxidant peroxynitrite in the airways of asthmatic patients is associated with induction of nitric oxide synthase: Effect of inhaled glucocorticoid. FASEB J. 12, 929–937. doi:10.1096/fasebj.12.11.929
Salvator, H., Grassin-Delyle, S., Brollo, M., Couderc, L.-J., Abrial, C., Victoni, T., et al. (2021). Adiponectin inhibits the production of TNF-α, IL-6 and chemokines by human lung macrophages. Front. Pharmacol. 12, 718929. doi:10.3389/fphar.2021.718929
Sánchez-Ortega, H., Jiménez-Cortegana, C., Novalbos-Ruiz, J. P., Gómez-Bastero, A., Soto-Campos, J. G., and Sánchez-Margalet, V. (2022). Role of leptin as a link between asthma and obesity: A systematic review and meta-analysis. Int. J. Mol. Sci. 24 (1), 546. doi:10.3390/ijms24010546
Saradna, A., Do, D. C., Kumar, S., Fu, Q. L., and Gao, P. (2018). Macrophage polarization and allergic asthma. Transl. Res. 191, 1–14. doi:10.1016/J.TRSL.2017.09.002
Serena, C., Ceperuelo-Mallafré, V., Keiran, N., Queipo-Ortuño, M. I., Bernal, R., Gomez-Huelgas, R., et al. (2018). Elevated circulating levels of succinate in human obesity are linked to specific gut microbiota. ISME J. 12 (7), 1642–1657. doi:10.1038/s41396-018-0068-2
Shankar, S., Singh, G., and Srivastava, R. K. (2007). Chemoprevention by resveratrol: Molecular mechanisms and therapeutic potential. Front. Biosci. 12, 4839–4854. doi:10.2741/2432
Sharma, N., Akkoyunlu, M., and Rabin, R. L. (2018). Macrophages-common culprit in obesity and asthma. Allergy 73 (6), 1196–1205. doi:10.1111/all.13369
Shibata, R., Takahashi, R., Kataoka, Y., Ohashi, K., Ikeda, N., Kihara, S., et al. (2011). Association of a fat-derived plasma protein omentin with carotid artery intima-media thickness in apparently healthy men. Hypertens. Res. 34, 1309–1312. doi:10.1038/hr.2011.130
Shim, J.-S., Lee, H.-S., Kwon, H., Kim, M.-H., Cho, Y.-J., and Park, H.-W. (2023). Inhibition of glutaminase 1 activity reverses airway hyperresponsiveness and decreases IL-1β+ M1s and IL-17 producing ILC3s in high-fat diet-fed obese mice. Am. J. Physiol. Lung Cell Mol. Physiol. 324 (5), L625–L638. doi:10.1152/ajplung.00181.2022
Sideleva, O., Suratt, B. T., Black, K. E., Tharp, W. G., Pratley, R. E., Forgione, P., et al. (2012). Obesity and asthma: An inflammatory disease of adipose tissue not the airway. Am. J. Respir. Crit. Care Med. 186 (7), 598–605. doi:10.1164/rccm.201203-0573oc
Soares, A. F., Guichardant, M., Cozzone, D., Bernoud-Hubac, N., Bouzaidi-Tiali, N., Lagarde, M., et al. (2005). Effects of oxidative stress on adiponectin secretion and lactate production in 3T3-L1 adipocytes. Free Radic. Biol. Med. 38, 882–889. doi:10.1016/j.freeradbiomed.2004.12.010
Sohn, S. I., Rathinapriya, P., Balaji, S., Jaya Balan, D., Swetha, T. K., Durgadevi, R., et al. (2021). Phytosterols in seaweeds: An overview on biosynthesis to biomedical applications. Int. J. Mol. Sci. 22 (23), 12691. doi:10.3390/ijms222312691
Song, G., Zhang, Y., Yu, S., Lv, W., Guan, Z., Sun, M., et al. (2019). Chrysophanol attenuates airway inflammation and remodeling through nuclear factor-kappa B signaling pathway in asthma. Phytotherapy Res. 33 (10), 2702–2713. doi:10.1002/ptr.6444
Sood, A., Cui, X., Qualls, C., Beckett, W. S., Gross, M. D., Steffes, M. W., et al. (2008). Association between asthma and serum adiponectin concentration in women. Thorax 63 (10), 877–882. doi:10.1136/thx.2007.090803
Sood, A., Dominic, E., Qualls, C., Steffes, M. W., Thyagarajan, B., Smith, L. J., et al. (2011). Serum adiponectin is associated with adverse outcomes of asthma in men but not in women. Front. Pharmacol. 2, 55. doi:10.3389/fphar.2011.00055
Sood, A., and Shore, S. A. (2013). Adiponectin, leptin, and resistin in asthma: Basic mechanisms through population studies. J. Allergy 2013. doi:10.1155/2013/785835
Stanford, K. I., Middelbeek, R. J. W., Townsend, K. L., An, D., Nygaard, E. B., Hitchcox, K. M., et al. (2013). Brown adipose tissue regulates glucose homeostasis and insulin sensitivity. J. Clin. Invest. 123, 215–223. doi:10.1172/JCI62308
Steel, C. C., and Drysdale, R. B. (1988). Electrolyte leakage from plant and fungal tissues and disruption of liposome membranes by α-tomatine. Phytochemistry 27, 1025–1030. doi:10.1016/0031-9422(88)80266-8
Steppan, C. M., Bailey, S. T., Bhat, S., Brown, E. J., Banerjee, R. R., Wright, C. M., et al. (2001). The hormone resistin links obesity to diabetes. Nat 409, 307–312. doi:10.1038/35053000
Stokholm, J., Blaser, M. J., Thorsen, J., Rasmussen, M. A., Waage, J., Vinding, R. K., et al. (2018). Maturation of the gut microbiome and risk of asthma in childhood. Nat. Commun. 9 (1), 141. doi:10.1038/s41467-017-02573-2
Summer, R., Little, F. F., Ouchi, N., Takemura, Y., Aprahamian, T., Dwyer, D., et al. (2008). Alveolar macrophage activation and an emphysema-like phenotype in adiponectin-deficient mice. Am. J. Physiol. - Lung Cell. Mol. Physiol. 294, 1035–1042. doi:10.1152/AJPLUNG.00397.2007
Sztolsztener, K., Hodun, K., and Chabowski, A. (2022). α-lipoic acid ameliorates inflammation state and oxidative stress by reducing the content of bioactive lipid derivatives in the left ventricle of rats fed a high-fat diet. Biochimica Biophysica Acta (BBA)-Molecular Basis Dis. 1868 (9), 166440. doi:10.1016/j.bbadis.2022.166440
Tashiro, H., Cho, Y., Kasahara, D. I., Brand, J. D., Bry, L., Yeliseyev, V., et al. (2019). Microbiota contribute to obesity-related increases in the pulmonary response to ozone. Am. J. Respir. Cell Mol. Biol. 61(6), 702–712. doi:10.1165/rcmb.2019-0144OC
Taylor, E. B. (2021). The complex role of adipokines in obesity, inflammation, and autoimmunity. Clin. Sci. (Lond). 135, 731–752. doi:10.1042/CS20200895
Theofani, E., Semitekolou, M., Morianos, I., Samitas, K., and Xanthou, G. (2019). Targeting NLRP3 inflammasome activation in severe asthma. J. Clin. Med. 8 (10), 1615. doi:10.3390/jcm8101615
Tobias, T. A., Wood, L. G., and Rastogi, D. (2019). Carotenoids, fatty acids and disease burden in obese minority adolescents with asthma. Clin. Exp. Allergy 49 (6), 838–846. doi:10.1111/cea.13391
Toledo, T. R., Dejani, N. N., Monnazzi, L. G., Kossuga, M. H., Berlinck, R. G., Sette, L. D., et al. (2014). Potent anti-inflammatory activity of pyrenocine A isolated from the marine-derived fungus Penicillium paxilli Ma(G)K. Mediat. Inflamm. 2014, 767061. doi:10.1155/2014/767061
Trenga, C., Koenig, J. Q., and Williams, P. V. (2001). Dietary antioxidants and ozone-induced bronchial hyperresponsiveness in adults with asthma. Arch. Environ. Health. 56, 242–249. doi:10.1080/00039890109604448
Troisi, R. J., Willett, W. C., Weiss, S. T., Trichopoulos, D., Rosner, B., and Speizer, F. E. (1995). A prospective study of diet and adult-onset asthma. Am. J. Respir. Crit. Care Med. 151, 1401–1408. doi:10.1164/ajrccm.151.5.7735592
Tsubota, A., Okamatsu-Ogura, Y., Bariuan, J. V., Mae, J., Matsuoka, S., Nio-Kobayashi, J., et al. (2019). Role of Brown adipose tissue in body temperature control during the early postnatal period in Syrian hamsters and mice. J. Vet. Med. Sci. 81 (10), 1461–1467. doi:10.1292/JVMS.19-0371
Tzanavari, T., Giannogonas, P., and Karalis, K. P. (2010). TNF-alpha and obesity. Curr. Dir. Autoimmun. 11, 145–156. doi:10.1159/000289203
Vajdi, M., and Abbasalizad Farhangi, M. (2020). Alpha-lipoic acid supplementation significantly reduces the risk of obesity in an updated systematic review and dose response meta-analysis of randomised placebo-controlled clinical trials. Int. J. Clin. Pract. 74 (6), e13493. doi:10.1111/ijcp.13493
Vieira, C. P., de Oliveira, L. P., Da Silva, M. B., Andre, D. M., Tavares, E. B. G., Pimentel, E. R., et al. (2020). Role of metalloproteinases and TNF-α in obesity-associated asthma in mice. Life Sci. 259, 118191. doi:10.1016/j.lfs.2020.118191
Weigert, J., Neumeier, M., Schäffler, A., Fleck, M., Schölmerich, J., Schütz, C., et al. (2005). The adiponectin paralog CORS-26 has anti-inflammatory properties and is produced by human monocytic cells. FEBS Lett. 579, 5565–5570. doi:10.1016/J.FEBSLET.2005.09.022
Weisberg, S. P., McCann, D., Desai, M., Rosenbaum, M., Leibel, R. L., and Ferrante, A. W. (2003). Obesity is associated with macrophage accumulation in adipose tissue. J. Clin. Invest. 112, 1796–1808. doi:10.1172/JCI19246
Williams, C. A., and Grayer, R. J. (2004). Anthocyanins and other flavonoids. Nat. Prod. Rep. 21, 539–573. doi:10.1039/b311404j
Williams, E. J., Negewo, N. A., and Baines, K. J. (2021). Role of the NLRP3 inflammasome in asthma: Relationship with neutrophilic inflammation, obesity, and therapeutic options. J. Allergy Clin. Immunol. 147, 2060–2062. doi:10.1016/j.jaci.2021.04.022
Wong, G. W., Krawczyk, S. A., Kitidis-Mitrokostas, C., Revett, T., Gimeno, R., and Lodish, H. F. (2008). Molecular, biochemical and functional characterizations of C1q/TNF family members: Adipose-tissue-selective expression patterns, regulation by PPAR-γ agonist, cysteine-mediated oligomerizations, combinatorial associations and metabolic functions. Biochem. J. 416, 161–177. doi:10.1042/BJ20081240
Wong, G. W., Wang, J., Hug, C., Tsao, T. S., and Lodish, H. F. (2004). A family of Acrp30/adiponectin structural and functional paralogs. Proc. Natl. Acad. Sci. U. S. A. 101, 10302–10307. doi:10.1073/PNAS.0403760101
Wood, L. G., Li, Q., Scott, H. A., Rutting, S., Berthon, B. S., Gibson, P. G., et al. (2019). Saturated fatty acids, obesity, and the nucleotide oligomerization domain–like receptor protein 3 (NLRP3) inflammasome in asthmatic patients. J. Allergy Clin. Immunol. 143 (1), 305–315. doi:10.1016/j.jaci.2018.04.037
World Health Organization (2021). Cardiovascular diseases (CVDs). Available at: https://www.who.int/news-room/fact-sheets/detail/cardiovascular-diseases (Accessed December 27, 2023).
World Health Organization (2022). World obesity day 2022 – accelerating action to stop obesity. Available at: https://www.who.int/news/item/04-03-2022-world-obesity-day-2022-accelerating-action-to-stop-obesity (Accessed January 3, 2023).
Wu, J., Boström, P., Sparks, L. M., Ye, L., Choi, J. H., Giang, A. H., et al. (2012). Beige adipocytes are a distinct type of thermogenic fat cell in mouse and human. Cell 150 (2), 366–376. doi:10.1016/J.CELL.2012.05.016
Wu, S. J., Huang, W. C., Yu, M. C., Chen, Y. L., Shen, S. C., Yeh, K. W., et al. (2021). Tomatidine ameliorates obesity-induced nonalcoholic fatty liver disease in mice. J. Nutr. Biochem. 91, 108602. doi:10.1016/j.jnutbio.2021.108602
Xu, H., Barnes, G. T., Yang, Q., Tan, G., Yang, D., Chou, C. J., et al. (2003). Chronic inflammation in fat plays a crucial role in the development of obesity-related insulin resistance. J. Clin. Invest. 112, 1821–1830. doi:10.1172/JCI19451
Xue, Z., Zhang, X. G., Wu, J., Xu, W. C., Li, L. Q., Liu, F., et al. (2016). Effect of treatment with geraniol on ovalbumin-induced allergic asthma in mice. Ann. Allergy, Asthma & Immunol. 116 (6), 506–513. doi:10.1016/j.anai.2016.03.029
Yamauchi, T., Kamon, J., Ito, Y., Tsuchida, A., Yokomizo, T., Kita, S., et al. (2003). Cloning of adiponectin receptors that mediate antidiabetic metabolic effects. Nature 423 (6941), 762–769. doi:10.1038/nature01705
Yamawaki, H., Kuramoto, J., Kameshima, S., Usui, T., Okada, M., and Hara, Y. (2011). Omentin, a novel adipocytokine inhibits TNF-induced vascular inflammation in human endothelial cells. Biochem. Biophys. Res. Commun. 408, 339–343. doi:10.1016/J.BBRC.2011.04.039
Yang, M. S., Choi, S., Choi, Y., and Jin, K. N. (2018). Association between airway parameters and abdominal fat measured via computed tomography in asthmatic patients. Allergy Asthma Immunol. Res. 10 (5), 503–515. doi:10.4168/aair.2018.10.5.503
Yokota, T., Oritani, K., Takahashi, I., Ishikawa, J., Matsuyama, A., Ouchi, N., et al. (2000). Adiponectin, a new member of the family of soluble defense collagens, negatively regulates the growth of myelomonocytic progenitors and the functions of macrophages. Blood 96, 1723–1732. doi:10.1182/BLOOD.V96.5.1723
Yoo, H. J., Hwang, S. Y., Hong, H. C., Choi, H. Y., Yang, S. J., Seo, J. A., et al. (2011). Association of circulating omentin-1 level with arterial stiffness and carotid plaque in type 2 diabetes. Cardiovasc. Diabetol. 10, 103–108. doi:10.1186/1475-2840-10-103
Yoshida, S., Fuster, J. J., and Walsh, K. (2014). Adiponectin attenuates abdominal aortic aneurysm formation in hyperlipidemic mice. Atherosclerosis 235, 339–346. doi:10.1016/J.ATHEROSCLEROSIS.2014.05.923
Young, C. Y., Yuan, H. Q., He, M. L., and Zhang, J. Y. (2008). Carotenoids and prostate cancer risk. Mini Rev.Med. Chem. 8, 529–537. doi:10.2174/138955708784223495
Yuan, Y., Ran, N., Xiong, L., Wang, G., Guan, X., Wang, Z., et al. (2018). Obesity-related asthma: Immune regulation and potential targeted therapies. J. Immunol. Res. 2018. doi:10.1155/2018/1943497
Yusuf, M. A., Singh, B. N., Sudheer, S., Kharwar, R. N., Siddiqui, S., Abdel-Azeem, A. M., et al. (2019). Chrysophanol: A natural anthraquinone with multifaceted biotherapeutic potential. Biomolecules 9 (2), 68. doi:10.3390/biom9020068
Zeng, Z., Wang, L., Ma, W., Zheng, R., Zhang, H., Zeng, X., et al. (2019). Inhibiting the Notch signaling pathway suppresses Th17-associated airway hyperresponsiveness in obese asthmatic mice. Lab. Invest. 99 (12), 1784–1794. doi:10.1038/s41374-019-0294-x
Zhang, L. X., Yu, F. K., Zheng, Q. Y., Fang, Z., and Pan, D. J. (1990). Immunosuppressive and antiinflammatory activities of tripterine. Yao Xue Xue Bao 25 (8), 573–577.
Zhong, X., Li, X., Liu, F., Tan, H., and Shang, D. (2012). Omentin inhibits TNF-α-induced expression of adhesion molecules in endothelial cells via ERK/NF-κB pathway. Biochem. Biophys. Res. Commun. 425, 401–406. doi:10.1016/J.BBRC.2012.07.110
Zhou, Q., Fu, Y., Hu, L., Li, Q., Jin, M., and Jiang, E. (2018). Relationship of circulating chemerin and omentin levels with Th17 and Th9 cell immune responses in patients with asthma. J. Asthma 55 (6), 579–587. doi:10.1080/02770903.2017.1355378
Keywords: obesity-associated asthma, herbal medicine, bioactive phytoconstituents, adiponectin, macrophage polarization, NLRP3, NOTCH1
Citation: Das A, Pathak MP, Pathak K, Saikia R and Gogoi U (2023) Herbal medicine for the treatment of obesity-associated asthma: a comprehensive review. Front. Pharmacol. 14:1186060. doi: 10.3389/fphar.2023.1186060
Received: 14 March 2023; Accepted: 25 April 2023;
Published: 11 May 2023.
Edited by:
Lalit Mohan Nainwal, GD Goenka University, IndiaReviewed by:
Manoj Kumar Sharma, Apeejay Stya University, IndiaNeelkant Prasad, Shree Guru Gobind Singh Tricentenary University, India
Copyright © 2023 Das, Pathak, Pathak, Saikia and Gogoi. This is an open-access article distributed under the terms of the Creative Commons Attribution License (CC BY). The use, distribution or reproduction in other forums is permitted, provided the original author(s) and the copyright owner(s) are credited and that the original publication in this journal is cited, in accordance with accepted academic practice. No use, distribution or reproduction is permitted which does not comply with these terms.
*Correspondence: Aparoop Das, YXBhcm9vcGRhc0BkaWJydS5hYy5pbg==
†These authors have contributed equally to this work and share first authorship