- 1Key Laboratory of Characteristic Chinese Medicine Resources in Southwest China, College of Pharmacy, Chengdu University of Traditional Chinese Medicine, Chengdu, China
- 2College of Medical Technology, Chengdu University of Traditional Chinese Medicine, Chengdu, China
- 3Chongqing Key Laboratory of Sichuan-Chongqing Co Construction for Diagnosis and Treatment of Infectious Diseases Integrated Traditional Chinese and Western Medicine, Chongqing, China
Diabetes mellitus (DM) is a group of metabolic diseases characterized by hyperglycemia that can occur in children, adults, elderly people, and pregnant women. Oxidative stress is a significant adverse factor in the pathogenesis of DM, especially type 2 diabetes mellitus (T2DM), and metabolic syndrome. Natural polysaccharides are macromolecular compounds widely distributed in nature. Some polysaccharides derived from edible plants and microorganisms were reported as early as 10 years ago. However, the structural characterization of polysaccharides and their therapeutic mechanisms in diabetes are relatively shallow, limiting the application of polysaccharides. With further research, more natural polysaccharides have been reported to have antioxidant activity and therapeutic effects in diabetes, including plant polysaccharides, microbial polysaccharides, and polysaccharides from marine organisms and animals. Therefore, this paper summarizes the natural polysaccharides that have therapeutic potential for diabetes in the past 5 years, elucidating their pharmacological mechanisms and identified primary structures. It is expected to provide some reference for the application of polysaccharides, and provide a valuable resource for the development of new diabetic drugs.
1 Introduction
Diabetes mellitus (DM) is a metabolic disease with symptoms of hyperglycemia. It can be caused by the deficiency of insulin secretion or insulin action in islet β cells (Galicia-Garcia et al., 2020). In 2015, approximately 415 million people worldwide had diabetes; of them, more than 90% had type 2 DM (T2DM). This number is projected to increase to 642 million in 2040 (Chen, 2015; Chatterjee et al., 2017). In addition, the onset of T2DM is often associated with conditions such as chronic hyperglycemia, hyperlipidemia and hypertension, placing a great burden on the healthcare system (Gerich, 2003; Taylor, 2013; Chatterjee et al., 2017). Physical activity has been reported to improve symptoms in diabetic patients with OS (Leeuwenburgh et al., 1994; Venkatasamy et al., 2013), but people seem to lack the time to exercise. Nowadays, unhealthy lifestyles and dietary factors are aggravating this condition (Dos Santos et al., 2019; Salazar-Garcia and Corona, 2021). Diabetes is still a key topic worthy of continued attention of scholars.
Insulin resistance is a major feature in T2DM development (Gerich, 2003) and may be caused by an insulin signalling defect, inflammatory cytokines (Halim and Halim, 2019), lipotoxicity (Kelly et al., 2019; Poznyak et al., 2020), glucose transporter defect, amyloid formation for β-cell dysfunction (Eizirik et al., 2020), OS (Dos Santos et al., 2019), mitochondrial dysfunction, excess fatty acid, or lack of the cretin effect (Galicia-Garcia et al., 2020). At present, according to the manifestations of diabetes, many treatment methods are available for T2DM. In addition to injectable preparations such as insulin and insulin analogs, glucagon-like peptide 1and oral hypoglycemic drugs, such as peptidyl peptidase-4 (DPP-4) inhibitors and sodium-glucose co-transporter 2 (SGLT2) inhibitor, are commonly used to treat diabetes (Chatterjee et al., 2017). New methods have recently been used to treat diabetes, including islet transplantation, gene therapy (Green et al., 2018), and combination therapy. Islet transplantation can effectively control blood sugar and prevent long-term complications by increasing the number of islet cells in patients, to reduce their dependence on exogenous insulin and rebuild their physiological regulation of blood sugar (Bertuzzi et al., 2018; Rickels and Robertson, 2019). However, reducing inflammation and adverse effects around transplantation is a problem associated with this method that needs to be urgently resolved. Stem cell transplantation can help increase β cells content. However, the medical evidence of the efficacy and safety of stem cell therapy to support its routine use for T2DM is insufficient (Chatterjee et al., 2017). Moreover, when single drug therapy is insufficient for controlling T2DM, drug combinations can be used to achieve rapid improvement in blood glucose levels. For example, better outcomes can be achieved with a combination of the DPP-4 inhibitor and metformin or TZD pioglitazone, and insulin and metformin, glyburide, and pioglitazone (Cersosimo et al., 2018). Combination therapy appears to be more effective than single-agent therapy, however, complex multi-agent regimens, higher costs, and reduced patient compliance make it difficult to determine the efficacy of combination therapies (Miccoli et al., 2011). Meanwhile, many adverse reactions associated with these drugs, including hypoglycemia and gastrointestinal problems, can cause extremely painful experiences for patients (Ganesan and Xu, 2019). The development of safer and more economical hypoglycemic drugs has become the need of the hour urgent problem for researchers.
As the advantages of traditional Chinese medicine (TCM) in chronic diseases continue to manifest, several TCMs are used in the treatment of diabetes with mild side effects (Ganesan and Xu, 2019; Zheng et al., 2019). Polysaccharide is a type of bioactive macromolecule present in almost every TCM and food, and plays crucial role in new drug development. It have been found to have antioxidant, antitumor, and immune, regulatory properties and can improve intestinal microorganisms and hypoglycemic effects (Wang et al., 2016; Zheng et al., 2019). Several studies have reported that polysaccharides from natural products may improve diabetes symptoms mainly through regulating the OS (Dos Santos et al., 2019; Zheng et al., 2019), inhibiting α-amylase and α-glucosidase (Qiu et al., 2022), enhancing insulin secretion and improving glucose metabolism in a non-insulin-dependent manner. OS has been recognized as a potential causative factor for diabetes (Maritim et al., 2003; Thakur et al., 2018; Kang and Yang, 2020), and therefore, we tried to summarize natural polysaccharides that can act on T2DM through the OS pathway, hoping to provide a data reference for developing of new therapeutic agents for diabetes.
2 Relationship between oxidative stress and diabetes mellitus
Hyperglycemia is a chronic T2DM manifestation, prolonged hyperglycaemia and hyperlipidaemia in T2DM can lead to OS (Nishikawa et al., 2000; Giacco and Brownlee, 2010). OS may aggravate insulin resistance in diabetic patients (Dos Santos et al., 2019). Impaired insulin signalling disrupts the glucose flow into fat cells (Yaribeygi et al., 2019). As the result reactive oxygen species (ROS) and reactive nitrogen species (RNS) overproduction in the cytosol or mitochondria, which counteract the cellular redox balance and induces more OS (Petersen et al., 2003; Ceriello et al., 2009; Salazar-García and Corona, 2021; Liu et al., 2022). Simultaneously, ROS overproduction can damage lipids, proteins, and DNA, leading to the deterioration of β cell function (Omolola et al., 2014; Ezraty et al., 2017). Another momentous reason for OS is the overproduction of free radicals due to mitochondrial dysfunction (Schieber and Chandel, 2014). Furthermore, the levels of plasma free fatty acids (FFAs) are higher in T2DM patients. The increased FFAs levels also affects mitochondrial function, resulting in impaired insulin signalling pathways and lower nitric oxide (NO) levels (Ghosh et al., 2017), and higher ROS contents. Eventually, at high glucose levels, OS induction through regulating the protein kinase C (PKC), glycolysis, hexosamine, polyols pathway, and advanced glycation end products (AGE). In summary, OS plays a pivotal role in the mechanism of DM and related complications (Maritim et al., 2003; Hu et al., 2018). The mechanism of OS in diabetic patients is presented in Figure 1.
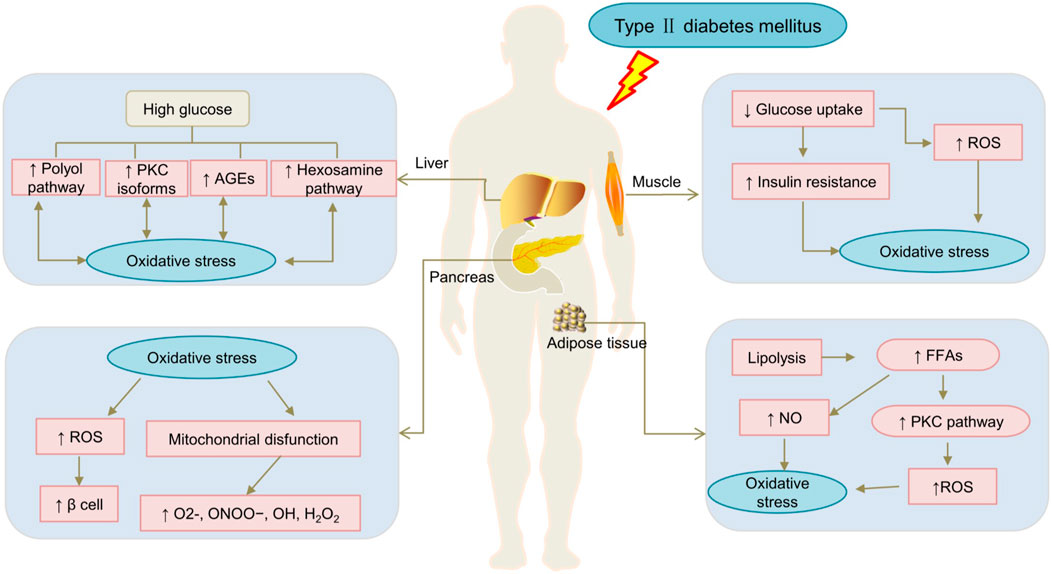
FIGURE 1. The mechanism of oxidative stress in diabetic patients. AGE, advanced glycosylation end products; FFAs, Free fatty acids. NO, nitric oxide; PKC, protein kinase C; ROS, reactive oxygen species; ↓: downregulated; ↑:upregulated.
2.1 Hyperglycemic condition-induced oxidative stress in diabetes
Constant high glucose levels activate the polyol pathway, and formation of advanced glycosylation end products (AGEs); upregulate the hexosamine pathway (Kang and Yang, 2020); and activate PKC isoforms, leading to OS and eventually worsening of T2DM (Ighodaro, 2018; Thakur et al., 2018). Hyperglycemia influx to the glycolytic pathway and activation of the polyol pathway result in NADH overproduction and higher ROS levels (Mima, 2016). Furthermore, NADPH overproduced leads to a decrease in the intracellular concentration of glutathione (GSH), an antioxidant (Giannini et al., 2011). Moreover, NADPH plays an important role in AGEs-RAGE activation and OS (Ceriello et al., 2009). AGEs are associated with several molecules that enhance oxidative activity, and the activated AGE pathway also ultimately results in increased ROS production (Yamagishi and Matsui, 2010). The higher NADH/NAD+ ratio also leads to low-density lipoprotein (LDL) oxidation, cytotoxic effects, decrease in NO levels, and increase of O2− in cells (Eftekharpour and Fernyhough, 2022) (Kessler et al., 1998; Giannini et al., 2011). Excessive production of free radicals damages the body’s antioxidant defense system, and has toxic effects, which cause OS (Halim and Halim, 2019) (Spinelli and Haigis, 2018) (Sergi et al., 2019). The activated polyol pathway also leads to immoderate and continuous activation of several PKC isoforms, which prevents NO release and thus a reduction in NO levels (Goncalves et al., 2022). The mechanism of hyperglycemic condition-induced OS in diabetes is shown in Figure 2.
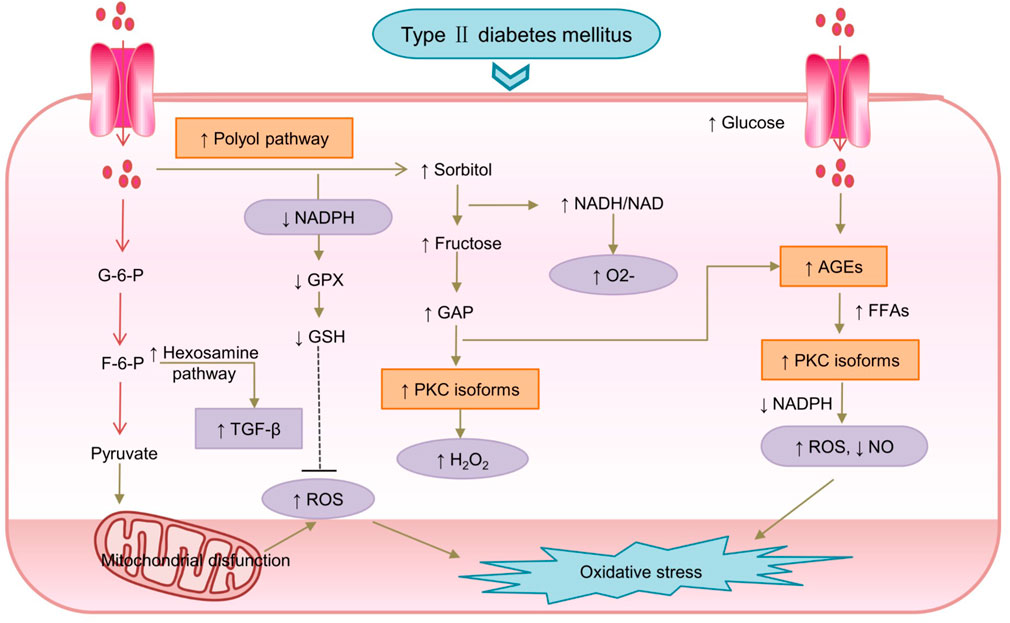
FIGURE 2. The mechanism of hyperglycemic conditions induced-oxidative stress in diabetes.F-6-P, Fructose-6-P; G-6-P, Glucose-6-P; GSH, glutathione; NO, nitric oxide; TGF-β, transforming growth factor beta; ↓: downregulated; ↑:upregulated.
2.2 Mitochondrial dysfunction caused by oxidative stress in diabetes
Mitochondria, also known as the “energy house” [39], are highly dynamic double-membrane organelles that produce adenosine triphosphate (ATP), the energy that the body requires (Newmeyer and Ferguson-Miller, 2003). Cellular energy production mainly occurs through two pathways: glycolysis and oxidative phosphorylation. Abnormal metabolism in diabetes can lead to excess production of mitochondrial superoxide in endothelial cells (Kaneto et al., 2016), thus damaging the mitochondrial respiratory chain (Maritim et al., 2003; Hu et al., 2018). Mitochondrial dysfunction that occurs in T2DM is inseparable from the glycolytic pathway, presumably it involves increased ROS production and energy expenditure (Qi et al., 2017). It also leads to the production of more free radicals (O2−, ONOO−, OH, and H2O2) (Erejuwa, 2012), ROS production (Wu et al., 2018), and ultimately decrease glucose homeostasis and insulin sensitivity in the liver (Yang et al., 2010). In addition to their role in energy production, mitochondria are responsible for FFA synthesis, which is an important consideration in DM (Yao L. et al., 2022). Especially in some obese diabetic patients, FFAs increased significantly (Ghosh et al., 2017). Excessive exposure to FFAs results in mitochondrial dysfunction and the increased formation of the toxic product MDA (Wu et al., 2018). In conclusion, mitochondrial dysfunction accelerates T2DM the development as shown in Figure 3.
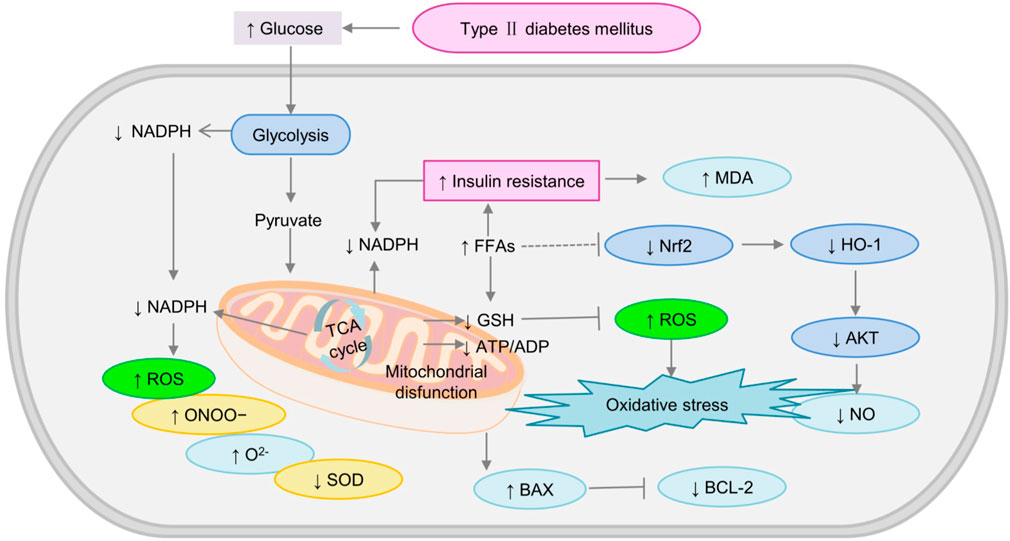
FIGURE 3. The mechanism of mitochondrial dysfunction induced-oxidative stress in diabetes. AKT, Phosphorylated protein kinase B; BAX, Bcl-2-associated X protein (BAX); HO-1, heme oxygenase-1; MDA, malondialdehyde; Nrf2, nuclear factor erythroid-2; SOD, superoxide dismutase; ↓: downregulated; ↑: upregulated.
3 Application of natural polysaccharides in treating diabetes by reducing oxidative stress
As a kind of complex biological macromolecule, polysaccharides have many structural characteristics, such as molecular weight, monosaccharide composition, glucoside bond, branching degree and high chain conformation, which are important factors affecting their functional properties. Due to their near non-toxic properties (Ganesan and Xu, 2019), the activity of natural polysaccharides has received more attention. With the deepening of the research, the chemical structure of polysaccharide and its mechanism of action in the treatment of diabetes have been reported more. It can control diabetes and reduce its complications through various mechanisms. For example, polysaccharides purified from saffron, curcumin, cinnamon and garlic have clear antioxidant properties, and those purified from pumpkin, wolfberry, sea cucumber, mushroom, tea, and beans have good effects on glucose homeostasis, reduce diabetic complications, and ultimately improve insulin sensitivity through the anti-OS damage defense mechanism (Liu et al., 2016). Thus, natural polysaccharides have great potential in improving OS and treating diabetes. In order to accelerate the study of the structure and therapeutic mechanism of natural polysaccharides, we classified the antioxidant polysaccharides with the potential to treat diabetes into 4 categories according to the sources of natural polysaccharides, such as plant polysaccharides, microbial polysaccharides, Marine polysaccharides, and animal polysaccharides.
3.1 Polysaccharides derived from plants
3.1.1 Medicinal plant
The Abelmoschus esculentus polysaccharides (AEP) were reported to exhibit anti-oxidative and hypoglycemic activities. On the one hand, AEP can significantly increase the content of glutathione peroxidase (GSH-Px), superoxide dismutase (SOD), SOD2, GSH, and catalase (CAT), whereas decreases the content of ROS and malondialdehyde (MDA). In particular, It may alleviate T2DM by improving the phosphoinositide 3-kinase (PI3K)/Phosphorylated protein kinase B (AKT)/glycogen synthase kinase 3 beta (GSK3β) pathway, and activating nuclear factor erythroid-2/heme oxygenase-1(Nrf2/HO-1) pathway. Furthermore, AEP regulated mitochondrial dysfunction by inhibiting NOX2 activation in HFD and STZ treated mice (Liao et al., 2019a). Moreover, AEP obviously increased adenosine monophosphate-activated protein kinase (p-AMPK/AMPK), and exhibited anti-apoptotic effects by diminishing the levels of cleaved caspase-3, and bcl-2-associated X protein (Bax), and enhanced Bcl-2 expression in the same models (Liao et al., 2019b). These indicate that AEP can not only improve the content of antioxidant enzymes, but also improve mitochondrial function and oxidation-related pathways to relieve diabetic.
The Cyclocarya paliurus polysaccharide (CCPP) could alleviate T2DM symptoms by boosting GSH-px, SOD, GSH, and CAT levels in diabetic rats (Zhang et al., 2021). In addition, compared with T2DM group, CCPP could upregulate SOD, CAT, GSH-Px, and GSH levels, whereas downregulated AGE and transforming growth factor-beta1 (TGF-β1) levels (Xia et al., 2020; Lin C. et al., 2021). Besides, CCPP significantly improved pathways closely with the nutrition metabolism (amino acids and purine) and energy metabolism (TCA cycle) to improve mitochondrial function (Li et al., 2021). Therefore, CCPP may improve the OS of diabetic patients by regulating AGE pathway to increase the content of antioxidant enzymes. In addition, in the same article, it was also reported to have a role in regulating inflammation and mitochondrial function.
Polysaccharides from the Chinese medicine Fructus Corni (FCPs) showed hypoglycemic, hypolipidemic and antioxidative effects in the STZ-induced diabetic rats by increased insulin secretion and promoted pancreatic β cell proliferation. In same study, FCPs have been found to increase the levels of antioxidant enzymes, such as SOD and GSH in STZ injected rats to alleviate diabetic (Wang D. et al., 2019). In a word, the potential mechanism of the hypoglycemic and hypolipidemic activities of FCP in diabetic related to OS. FCPC might have potential applications in regulating OS and T2DM.
The Astragalus membranaceus polysaccharide (AMP), as a main bioactive macromolecules of A. membranaceus (Fisch.), alleviates OS to relieve liver damage in T2DM mice (Chen et al., 2022). One possible therapeutic mechanism was observed in experimental mice that exhibited augmented SOD, GSH, and CAT levels, and decreased MDA levels after AMP treatment. In STZ-treated mice and heterozygous (SOD2+/−) knockout mice, astragalus polysaccharides (APS) improved the damage of the cardiac stem and progenitor cells by inhibiting of apoptosis (Chen et al., 2018). In particular, APS has a beneficial effect on SOD2 enzyme activities. Notably, APS reduced cellular ROS levels, and inhibited OS injury indicators, that are 8-Hydroxy-2′-deoxyguanosine (8-OH-dG) and nitrotyrosine levels, in high glucose-induced H9C2 cells (Sun et al., 2019). Moreover, APS can promote SOD, and GSH-Px levels and diminish ROS, MDA, and NO levels in an AGE-induced H9C2 cell model, thereby performing the anti-oxidative function (Chang et al., 2018). Otherwise, APS may display a protective function by activating NGR1/ErbB signalling and the downstream AKT/PI3K signalling pathway (Chang et al., 2018). APS can regulate multiple pathways to improve the etiology and complications of DM (Zhang Z. et al., 2019).
Polysaccharides from Hedysarum alpinum (HPS) are an active ingredient for DM treatment. HPS markedly revises OS damage in DM mouse models and cell model. Mechanistically, it inhibits Keap1 signalling and upregulates Nrf2 signalling pathway. Besides, HPS significantly suppressed MDA concentration and enhanced the concentration of antioxidant enzymes in HG-induced Schwann cells. Moreover, HPS stimulated Nrf2 signalling, whereas decreased Keap1 expression in SCs (He et al., 2022). Therefore, HPS has been demonstrated to have antioxidant activity both in vivo and in vitro and can be considered as a potential diabetes treatment.
The Morus alba polysaccharide (MLP) can ameliorate diabetes by improving OS injury and the mitochondrial function of islet cells in HFD- and STZ-induced diabetic rats. Furthermore, MLP inhibits MDA production, but promotes SOD and SDH activities (Liu et al., 2017). In 2015, MLP was found to activate the PI3K/AKT pathway and mitigate OS in HFD- and STZ-induced rats (Ren et al., 2015). These results suggest that MLP has the potential to treat diabetes. MLP could improve OS by reducing MDA content, enhancing SOD, CAT, and GPx activities, and regulating amino acid and lipid metabolism by decreasing TG and TC levels. Additionally, blood urea nitrogen (BUN), albumin (ALB), Creatinine (CRE), and UA (uric acid) levels, and the expression of PI3K of insulin receptor substrate 2 (IRS2) and the PKB/AKT pathway relative to insulin signalling were significantly increased with the MLPs. Finally, glucose levels were restored in T2DM rats (Li et al., 2022).
The Dendrobium officinale polysaccharide (DOP) can ameliorate hyperglycemia, improve β-cell function inflammation, regulate lipid concentration, inhibit insulin resistance, and regulate OS to ameliorate diabetes (Yang J. et al., 2020). Specifically, compared with normal C57BL/6 male mice, SOD and CAT concentrations in the liver were significantly increased after DOP administration in T2DM rats (Yang J. et al., 2020). DOP can also improve AKT signalling pathways (Liu et al., 2020b).
Polygonatum sibiricum polysaccharides (PSP), as an antioxidant activity component, have been found to have anti-diabetic effects. PSP attenuates diabetes by decreasing ROS production and MDA levels, and augmenting SOD and GPx activities. PSP also scavenged the activation of the Nrf2/HO-1 pathway in HG-induced ARPE-19 cells. Moreover, PSP significantly regulated apoptosis by diminishing bax and caspase-3 expression, and promoting Bcl-2 production (Wang et al., 2022). In another in vivo study, PSP was advantageous in controlling polydipsia, polyuria, polyphagia, and weight loss in DM rats. With PSP, MDA content was lowered, and cataract progression was delayed. Thus, PSP may alleviate the progression of diabetes by alleviating OS (Wang et al., 2017d). Simultaneously, PSP pre-treatment significantly alleviated the IR effect in IR-3T3-L1 adipocytes by inhibiting IL-6, IL-1β, and TNF-α levels and promoting Nrf2 and HO-1 expression (Cai et al., 2019).
The polysaccharide (CLP) of Codonopsis lanceolata, as an important bio-active, has been used to treat diabetes. It improves insulin sensitivity by regulating Akt pathway and hyperphosphorylation of IRS-1, decrease MDA levels, elevates SOD, and CAT levels, and the GSH/oxidized glutathione ratio, and activates Nrf2 signalling in chronic high fat/high sucrose (HFHS) diet-fed mice (Zhang et al., 2020). CLP through activating Nrf2 signaling pathway and increased antioxidant oxidase content regulated HFHS diet-induced insulin resistance, offers the possibility that the CLP can be used as a treatment for diabetes.
The Polysaccharide (DAP) from the roots of Dipsacus asper has been used in STZ-induced diabetic rats. After administration with DAP, the fasting blood glucose and glycosylated haemoglobin (HbA1c) levels were decreased, but the body weight increase in STZ-induced diabetic rats. Moreover, advanced glycation end products-receptor accumulation were nearly reversed to the normal levels along with inhibition of AGE-RAGE expression in STZ-treated diabetic rat by DAP (Li T. et al., 2019).
The Ophiopogon japonicus polysaccharide (OJP) exhibited antioxidant effects by efficiently diminishing the levels of free radicals (DPPH and ABTS), markedly enhancing the NO production, and diminishing the mRNA expressions of iNOS, COX2 in nickel-induced RAW264.7 cells (Chen et al., 2013; Lin et al., 2020). Moreover, with OJP treatment, the levels of TG, TC, HDL-C and LDL-C were obviously reversed in diabetic rats. Altogether, these results suggest that OJP could be a natural antioxidant and can improve diabetes (Chen et al., 2013).
The polysaccharide isolated from Rehmannia glutinosa (RGP), as a main active ingredient exhibited hypoglycemic effects (Zhou et al., 2015a). In STZ-induced mice, RGP dispiayed anti-oxidative activities by elevating serum GPx and SOD levels, whereas decreasing blood levels of IL-6, MDA, TNF-α, and monocyte chemoattractant protein-1 (MCP-1) (Zhou et al., 2015a). Moreover, with RGP administration, high levels of TG, TC, LDL-C were reversed and HDL-C increased in diabetic mice.
Polysaccharides obtained from guava (Psidium guajava L.) Leaves (GLP) showed antioxidant and antidiabetic activities. In a STZ + HFD induced diabetic mice. These polysaccharides exhibited good OH, ABTS free-radical, and DPPH scavenging abilities, and significantly ameliorated fasting blood sugar, Total cholesterol (TC), MDA, creatinine, and Triglycerides (TG) levels. Meanwhile, it significantly increased SOD enzyme activity (Luo et al., 2019). Thus, GLP might be a promising candidate for the development of a new anti-diabetic or antioxidant agents. However, its clinical efficacy needs to be further verified.
Holothurian glycosaminoglycan from Apostichopus japonicus (AHG) can suppress hepatic glucose production for the development of an anti-hyperglycemic agent. In HFD-fed mice, AHG supplementation apparently reduced conventional index, such as body weight, blood glucose level, and serum insulin content. In same model, AHG elevated phosphorylation levels of insulin receptor substrate-1(IRS1), Akt, and AMPK levels that were decreased by HFD treatment (Chen Y. et al., 2019).
The Pueraria lobata polysaccharide (PLP) improved diabetic by scavenging blood glucose levels and alleviating the lipid metabolic function of db/db mice. In particular, PLP boosted the expression of G6PC, FOXO1, PEPCK, SREBP-1, and ACC, whereas diminished the mRNA expression of antioxidant-related indicators, such as glycogen synthase, phosphatidylinositol-3-kinase, Akt2, PPARa, and glucose transporter 2 (GLUT2) in the liver of db/db mice (Luo et al., 2021).
Lycium barbarum polysaccharides (LBPs) extracted from L. barbarum, markedly increased insulin secretion and insulin sensitizing activities to improve insulin resistance. They also regulated glucose metabolism through hypoglycemic and antioxidative activities to relieve diabetes (Li, 2007; Cheng et al., 2015; Shi et al., 2017). Compared with the diabetic group, LBPs promoted the production of antioxidant enzymes such as SOD, CAT, and GPx, while down-regulating Caspase-3 expression and up-regulating the ratio of Bcl-2/Bax in STZ-included diabetes mice (Shi et al., 2017). Simultaneously, LBPs activated the PI3K/Akt/mTOR pathway, and Nrf2/HO-1 pathway, whereas inhibited the AGEs-RAGE signalling pathway to mitigate OS (Li, 2007; Tian et al., 2019).
Euryale ferox polysaccharides (EFP) exhibited protective effects against alloxan-induced oxidative injury in mice. Furthermore, EFPPs can enhance CAT, SOD and GSH-Px expression and scavenging of MDA content in the liver and kidney of alloxan-induced hyperglycemic mice. In addition, EFPP was beneficial enhancing oral glucose tolerance, and hepatic glycogen content, but reversed blood glucose levels and relieved diabetes (Wu C. Y. et al., 2017).
Physalis alkekengi polysaccharides (PAP) have been reported to have an antidiabetic effect in mice. The PAP intervention decreased blood glucose and glycated serum protein levels. Moreover, PAP protected and reversed β-cell necrosis and upregulated the mRNA expression of PI3K, GLUT4, and Akt in alloxan-induced diabetic mice (Guo et al., 2017). Consequently, PAP could be explored as a potential for the development of a new treatment diabetic agent.
Schisandra sphenanthera polysaccharides (SSPs) regulated hyperglycemia, improved the glucose tolerance, reduced FBG, and elevated the levels of FINS and the value of ISI in STZ induced rats. After SSPs administration, MDA levels were also repressed and SOD, GSH-PX and CAT activities were enhanced (Niu et al., 2017).
The polysaccharide from Moutan Cortex (MC-P) ameliorates diabetes by down-regulating the AGEs pathway. It also decreased ROS production induced by AGEs in HG- and STZ-induced rats and the AGEs-stimulated human umbilical vein endothelial cells. Moreover, MC-P can regulate the production of inflammatory factors (VCAM-1 and ICAM-1) and improve TGF-β1 levels (Lian et al., 2021).
Arctium lappa polysaccharides (ALP) displayed anti-diabetes effect by regulating lipid metabolism and OS through the PKC/NF-κB pathway in diabetic rats. Administration with these polysaccharides ALP in vivo, led to upregulation of antioxidant enzymes such as SOD and GSH-Px, whereas a decrease in MDA levels decreased in the rat livers. Moreover, the expression of P-select, PKC-α, and PKC-β was obviously decreased. Meanwhile, the PKC/NF-κB signalling pathway was inhibited (Li X. et al., 2019).
The Anoectochilus roxburghii polysaccharide (ARP) has been reported to regulate OS. After the ARP intervention in HFD-induced male C57BL/6 mice for 12 weeks, the fatty acid pathway regulating lipogenesis was inhibited, the levels of antioxidant enzymes, such as GPX, glutathione S-transferase (GST) and SOD were increased, and MDA levels were decreased. Furthermore, the Nrf2-mediated phase II enzyme system (Nrf2/HO-1/NQO1) was activated to improve OS. Moreover, the mitochondrial complex and PGC-1α were activated to promote mitochondrial function. Furthermore, ARP also had some effects on reducing ROS levels and increasing ATP levels, thereby significantly improving diet-induced OS (Chen et al., 2021).
Buddleja officinalis (BOP) polysaccharides could improve diabetes by improving levels of blood glucose, blood lipids, and insulin and further improving CD34 expression. Furthermore, BOP diminished ROS levels, whereas promoted SOD, NQO1, Nrf2, and HO-1 expression levels in db/db mice to ameliorate diabetes (Zhu et al., 2022).
Opuntia milpa alta polysaccharides (MAPs) contain xylose (23%), arabinose (36%), and galactose (15%) and have been reported exhibit anti-diabetic effects. In vitro research, treatment with MAPs restored oxidative enzyme activities and cell viability improved NO, MDA and ROS, and regulated antioxidant PARP and Nrf2 pathways in alloxan-induced INS-1 cell. Moreover, MAPs regulated proteins related to apoptosis, such as the expression of Bcl-2, Bax, caspase-3, and caspase-9 (Li W. et al., 2020). These findings indicated that MAPs has the potential to regulate OS.
Astragalus mongholicus Bunge polysaccharides (APS) also repressed apoptosis by enhancing Bcl-2 levels and diminished Bax, caspase-3, and caspase-9 levels (Chang et al., 2018). In both STZ-induced diabetic mice and nondiabetic SOD2+/−mice, APS reduced the apoptosis and enhanced the proliferation of cardiac stem and progenitor cells (CSPCs). Furthermore, APS enhanced SOD2 protein levels and enzyme activities, and downregulated ROS formation and oxidative damage of CSPCs (Chen et al., 2018). In HG-induced or SOD2-silenced H9C2 cells, APS improved cell apoptosis and higher levels of ROS, and regulated the levels of oxidative stress injury indicators 8-OH-dG and nitrotyrosine (Sun et al., 2019). More polysaccharides from medicinal plant with antioxidation and anti-diabetic effects show in Table 1.
3.1.2 Food
The Siraitia grosvenorii polysaccharide (SGP-1) alleviated inflammation by reducing the cytokines IL-6 and TNF-α. Moreover, it regulated oxidation by stimulating SOD production of and diminished MDA in DN mouse models (Gong et al., 2021).
Polysaccharides obtained from the dried pumpkin pulp (PPs) have hypoglycemic effects (Wang S. et al., 2017). After PPs treatment OS was improved through the upregulation of the Nrf2, HO-1, NOS, MDA and PI3K levels (Chen X. et al., 2019). They also can reduce TG, TC, and LDL-C levels and improve HDL-C, SOD, PI3K levels in the hypoglycemic mechanism (Chen X. et al., 2019).
The Ficus pumila Linn polysaccharide (FPLP) improved glycogen metabolism by activating the phosphoenolpyruvate carboxykinase and glucose-6-phosphatase expression, regulating the IRS-1/PI3K/Akt/GSK3β/GS and AMPK/GSK3β/GS signalling pathway and glucokinase in C57BL/KsJ db/db mice (Wu J. et al., 2017). Mechanistically, the PI3K/Akt pathway and AMPK signalling pathway are closely related to the oxidation reaction process of the body. This suggests that FPLP may have the potential to ameliorate diabetes by regulating OS (Wu J. et al., 2017).
The peach [Prunus persica (L.)] tic gum polysaccharide (PGP) could restore the postprandial blood glucose levels in STZ-induced diabetic mice by recovering pancreaislets, and activating the expression of HO-1 and insulin, which are all beneficial for improving diabetes (Wang et al., 2017c).
The mulberry fruit polysaccharide (MFP) also possesses numerous bioactivities. In STZ induced diabetic mice deal with STZ, MFP can promote pancreatic β cells proliferation and thus increase insulin secretion. Moreover, MFP can improve diabetes by regulating OS, which is mainly manifested in inhibiting MDA content, and increasing SOD, GPx, and CAT levels (Chen et al., 2017).
The polysaccharide from the sweet potato (SPP) significantly alterated the lipid metabolism index (TC, TG, and LDL), while remarkably decreasing HDL levels in STZ-induced rats. Compared with the diabetic rats, the level of MDA decreased along with an increase in SOD, GSH-Px, GSH and CAT levels after SPP treatment (Yuan et al., 2017).
Momordica charantia L. has been reported to exhibit antiobesity and antidiabetes activities (Zhang et al., 2019). In an in vitro study, this bitter gourd polysaccharide improved diabetes mice by enhancing the concentration of CAT, SOD, and GSH-px antioxidant enzymes and decreasing the content of MDA in mice (Zhang et al., 2019).
The Dipsacusa sper polysaccharide (DAP) regulated hyperlipidemia, downregulated the formation of the advanced glycated end-product receptor (AGE-RAGE), and improved OS in STZ-treated diabetic rats (Li et al., 2019).
A novel polysaccharide (RTFP-3), extracted from Rosa roxburghii fruit, has been found have antioxidant activity. In H2O2-induced INS-1 cells, RTFP-3 possessed higher protective and suppressive activities against H2O2-induced apoptosis of INS-1 cells in comparison with normal. Additional, after treatment with RTFP-3, ROS was decreased in INS-1 cells, which indicated that RTFP-3 via attenuating oxidative stress to prevent dibaetes (Wang et al., 2019). More polysaccharides from foods with antioxidation and anti-diabetic effects show in Table 2.
3.2 Polysaccharides derived from Microorganisms
The Agrocybe Cylindracea polysaccharide (ACP) can decrease blood glucose levels, heal liver and colon injuries, and repress inflammation (Wu L. et al., 2022). It also can increase SOD, GSH-Px, and CAT levels and improve lipid metabolism in HFD combined STZ induced mice (Sun et al., 2022).
Cordyceps militaris (CM), as a TCM, has been reported have anti-diabetes effects (Chen, 2015). In animal experiments, it was found that CM polysaccharide (CMP) could downregulate the contents of lipid peroxidation, blood lipid and blood sugar. Besides, it could improve insulin resistance and blood glucose levels. In particular, CMP increased the activities of antioxidant enzymes, such as glutathione peroxidase (GSH-px), superoxide dismutase (SOD), and catalase (CAT) in HFD and STZ induced mice (Zhao et al., 2018). Similarly, in another research, a polysaccharide-enriched fraction obtained from CM also produced hypoglycemic effects in STZ-induced rats (Zhang et al., 2006).The anti-diabetic effects of C. cicadae polysaccharide (SHF) were evaluated in alloxan-induced diabetic rats. SHF administered repressed the body weights of rats. Additionally, GSH, SOD, and HDL levels were upregulated, whereas MDA, urea, LDL, TG, CREA, TC, ALP, AST, and ALT levels were downregulated with SHF treatment (Zhang et al., 2018).
Mushroom (Cynomorium coccineum L.) polysaccharides (MPs), derived from the mycelium and the fermentation broth, have a role in anti-diabetes treatment (Zhao et al., 2014; Chen, 2015; Liu et al., 2022). MP can enhance the levels of antioxidant enzymes such as SOD, GSH-Px, and CAT in diabetes models in vivo (Chen, 2015; Zhang et al., 2015; Zhang et al., 2017; Liu et al., 2022). The polysaccharide hispidin from Phellinus linteus (Berk. and M.A. Curtis) improved the β-cell activities by inhibiting H2O2 induced apoptosis and improving insulin levels (Maity et al., 2021).
Ganoderma lingzhi polysaccharides (GLPs) exhibited outstanding antioxidant, hypoglycemic, and hypolipidemic activities in STZ-induced T2DM rats (Liu, 2019; Xu et al., 2019; Chen et al., 2020). More data on the role of MP in diabetes treatment can be obtained from another review (Arunachalam et al., 2022). Furthermore, GLP improved the OS and inhibited apoptosis by increasing the expression of TGF-β1, GSH, SOD, and NOS and suppressing the phosphorylation of MDA, JNK, eNOS and ERK in DM rats (Yao et al., 2022). In addition, GLP-treatment reversed the levels of fasting plasma lipids, hepatic lipid accumulation, and higher blood glucose levels (Li et al., 2020), increased SOD, CAT, and GSH-Px expression, as well as promoted Nrf2 and HO-1expression in db/db mice, by contrast, the content of MDA and TNF-a decreased in these mice.
Auricularia auricular (L.et Hook.) Underw polytricha (AAP) are popular edible fungi. At a low dose (100 mg/kg/day), AAP generally increased the vitality of antioxidant enzymes in STZ-induced diabetes mice, and might regulate the NF-κB pathway and the associated signalling pathway to alleviate diabetes (Xiang et al., 2021).
Lentinus edodes polysaccharides (LEPs) have been found to exhibit hypoglycemic ability by improving the Nrf2/HO-1 pathway. The LEP can increase SOD and GSH activity, as well as reduce MDA levels in STZ induced DM mice (Gong et al., 2022). Furthermore, in an vivo study, LEP reduced cell damage by increasing SOD activity, decreasing ROS content, and revising HG-induced MDA levels in MIN6 cells (Cao et al., 2019). In addition, LEP increased the viability of HG-induced human umbilical vein endothelial cells, decreased ROS production, and reversed the inhibition of α-glucosidase activity. It especially inhibited AGE formation in cells (Cao et al., 2020).
The polysaccharide from the caps of Suillellus luridus (SLP) exhibited excellent antidiabetic activities by improving Nrf2/HO-1-mediated OS (Liu et al., 2020a) in the STZ-induced diabetic mice. SLP was composed of galactose (gal), glucose (glu), arabinose (ara), and mannose (man). It had a backbone principally composed of 1,3 linked α-D-Galp, 1,3 linked β-D-Glcp and 1,6 linked β-D-Glcp with the branches mainly composed of T-linked α-D-Galp, 1,3 linked α-L-Arap, 1,3 linked β-D-Glcp, and 1,3 linked α-D-Manp. Moreover, SLP regulated the mRNA levels and protein expression in NF-kB signaling pathways. In short, SLP can be treated as a potential agent for preventing and treating diabetes via regulating oxidative stress (Liu et al., 2020a).
Polysaccharides from Armillariella tabescens mycelia diminished the levels of blood glucose, and repressed OS cytokine. They increased SOD and GSH concentrations, and decreased MDA production in diabetic mice to improve T2DM (Yang et al., 2020).
Polysaccharides separated from Inonotus obliquusvia (IOP) modulated OS in STZ-induced mice (Wang et al., 2017). Simultaneously, it remarkably regulated DPPH scavenging activity and ferric-reducing power in H2O2-induced hepatic L02 cells (Wang et al., 2018).
Polysaccharides from the mycelia of Coprinus comatus (CMP) reduced insulin resistance in STZ-induced mice models. It is mainly manifested in the many aspects, they improved energy metabolism, suppressed OS and inflammation, and modulated the Wnt-1/β-catenin and PTEN/PI3K/Akt pathways (Gao et al., 2021).
Extracellular polysaccharides (EPS) from Pleurotus eryngii SI-04 exhibited anti-glycated activities in STZ-regulated diabetic mice. EPS significantly suppressed GLU levels; decreased BUN, ALB, CRE and UA levels; downregulated serum lipid levels; modified the content of antioxidant enzymes, such as CAT, GSH-Px, SOD, and MDA (Zhang et al., 2018).
Paecilomyces hepiali polysaccharides (PHP) exerted anti-diabetic properties by regulating Nrf2-meadited NF-κB signalling in db/db mice. They not only decreased ROS and MDA concentrations, but also increased GSH-Px, CAT, and SOD contents in the serum and kidney (Hu et al., 2020).
Grifolafrondosa frondosa polysaccharides exert hypoglycemic, anti-diabetic, and nephritic properties by modulating the content of antioxidant enzymes in HFD- and STZ-induced diabetic rats. When CAT, SOD, and GSH-px levels increased, ROS levels decreased in the serum (Kou et al., 2019).
Pholiota nameko polysaccharides (PNPs) exhibited antiglycation ability by improving methylglyoxal -induced Hs68 cell damage. Preprocessing of Hs68 cells with PNPs increased the cell survival rate and inhibited intracellular ROS content. Furthermore, PNPs significantly decreased AGEs and inhibited ROS production, thus alleviating cell damage and OS (Lin et al., 2021). Representative polysaccharides from microorganisms with antioxidation and anti-diabetic effects show in Table 3.
3.3 Polysaccharides derived from marine life.
Sargassum thunbergii polysaccharides (STP), as a promising natural antioxidant and hypoglycemic agent, can be used to improve diabetes. They not only exhibited strong antioxidant and α-glucosidase inhibitory activities, but also improved the glucose uptake and radical-scavenging (DPPH, ROS) rates in insulin-resistant HepG2 cells (Ren et al., 2017).
The polysaccharide from Laminaria japonica (LGP) displays various pharmacological functions, especially in oxidative stress. LGP significantly improved mitochondrial dysfunction, leading to higher ATP and SOD content and lower ROS content. Furthermore, LGP upregulated Nrf2 pathway and repaired dysfunction in H2O2-induced β-cell (Wu et al., 2022).
3.4 Polysaccharides derived from animal
Field cricket (Gryllus bimaculatus), as an edible insect, has been reported to have multiple effects (Table 3). Field cricket glycosaminoglycan has regulated diabetes by significantly increased CAT, SOD and GSH-Px content in db mice (Ahn et al., 2020).
The Misgurnus anguillicaudatus polysaccharide (MAP) has a potential hyperglycemic effect (Table 3). It repressed the levels of inflammatory factor, such as IL-6, TNF-α, MDA, and MCP-1, whereas boosted SOD and GPx activities in STZ-induced mice (Zhou et al., 2015b). Representative polysaccharides from marine life and animal with antioxidation and anti-diabetic effects show in Table 4.
3.5 Characteristics of polysaccharides in improving oxidative stress in diabetes mellitus
As we all know, excessive sugar intake is a contraindication in diabetic patients, which will cause increased insulin secretion, resulting in damage to islet beta cells, causing elevated blood sugar. But natural polysaccharides, as a special class of compounds, have been found not only to improve high blood sugar, but also to treat diabetes through other routes. Acting as an antioxidant, natural polysaccharides may control OS-induced diabetes mainly in two ways. They improved type 2 diabetes by inhibiting the production of reactive oxygen species, improving mitochondrial function impairment, and regulating signalling pathways such as oxidative pathway AGE, which is closely related to hyperglycemia patients. They may ameliorate OS by controlling the production of ROS, improving mitochondrial dysfunction of β cells, scavenge free radicals, or enhance antioxidant defense enzymes and their related pathways, such as Nrf2 pathway, oxygenase-1 (HO-1) pathway, Kelch-like ECH-associated protein 1 (Keap1) pathway, and antioxidant response elements (ARE) pathway to improve OS-induced insulin resistance (Nishikawa et al., 2000; Giacco and Brownlee, 2010; Yaribeygi et al., 2020). In addition, polysaccharides may ameliorate diabetes by regulating the cascade of molecular events in different metabolic pathways, such as glycolysis, hexosamine, PKC, polyols, and advanced AGE pathway. The mechanism of representative polysaccharides underlying diabetes treatment by OS is given in Figure 4.
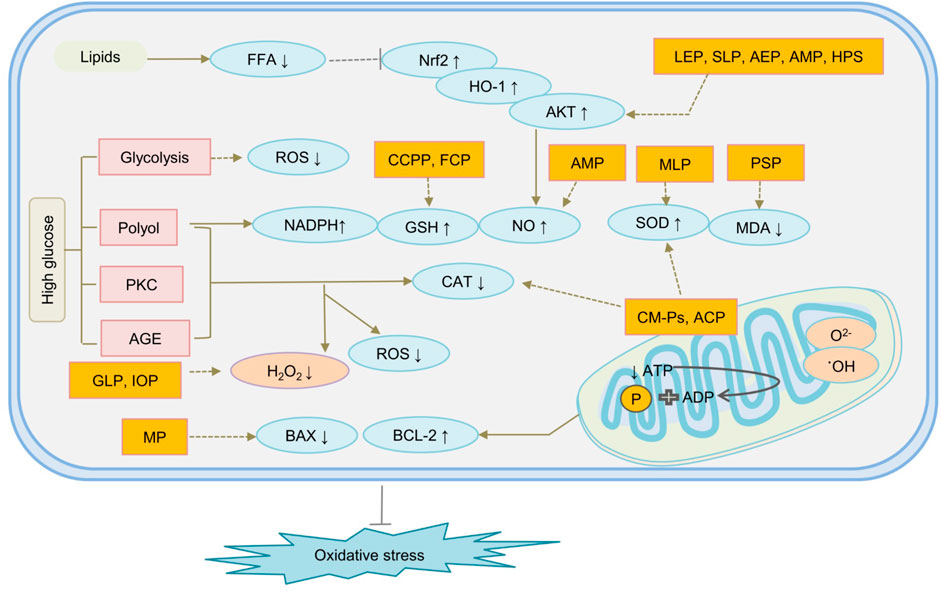
FIGURE 4. The mechanism of natural polysaccharides treat diabetes through oxidative stress is shown follows. ACP, Agrocybe Cylindracea Polysaccharide; AEP, Abelmoschus esculentus polysaccharide; AMP, Astragalus membranaceus polysaccharide; AMP, Astragalus membranaceus polysaccharide; CCPP, Cyclocarya paliurus polysaccharide; CM-Ps, acidic-extractable polysaccharides; FCP, Fructus Corni Polysaccharides; GLP, Ganoderma lucidum polysaccharide; HPS, Hedysarum polysaccharide; IOP, Polysaccharides separated from Inonotus obliquusvia; LEP, Lentinus edodes polysaccharides; MLP, mulberry leaf polysaccharide; MP, Mushroom polysaccharides; PSP, Polygonatum sibiricum polysaccharides; SLP, Suillellus luridus Polysaccharide; STP, Sargassum thunbergii Polysaccharides.
3.6 Boundedness of polysaccharides in diabetes improvement
Although numerous natural polysaccharides have been shown to have a therapeutic effect on diabetes and oxidative stress inhibition, their pharmacological effects is closely linked to the structure of these compounds. Currently, the primary structure analysis is the main method used to determine the structure of natural polysaccharides, and the structure-activity relationship of natural polysaccharides cannot be accurately predicted. Moreover, the structural analysis of natural polysaccharides from food, marine organisms, and animals is relatively limited. The characterization of the advanced structure of natural polysaccharides is still in the exploration stage, and there are few studies on the functional fragments of natural polysaccharides that can clarify the activity orientation. Although many scholars have investigated the efficacy of natural polysaccharides in improving diabetic oxidative stress and attempted to speculate on the possible mechanism of action, there is a lack of innovation and breakthrough, and few in-depth studies have been conducted. Again, because the precise structure of polysaccharides is not clear, the application of polysaccharides is still only in animal or cell models, and there is not much clinical trial evidence. Whether the role of polysaccharides in vivo and in vitro models can fully reflect the efficacy of polysaccharides in diabetic patients in the future is a question worthy of consideration for every scholar.
4 Conclusion and future perspectives
More than 50 natural polysaccharides have been documented to improve oxidative stress, by regulating mitochondrial function, free radical levels, oxidase content, and Nrf2/HO-1, and AGEs/RAGE pathways in diabetes. However, intervention in diabetes and its complications is the result of multi-target, multi-pathway synergies. For example, polysaccharides extracted from fermented Momordica charantia significantly increased intestinal flora diversity and elevated the levels of short-chain fatty acids (SCFAs), thus exhibiting antidiabetic effects in HFD + STZ-induced rats (Gao et al., 2018). Furthermore, polysaccharides obtained from L. barbarum increased SCFAs levels, elevated gut microbiota diversities, and improved the intestinal barrier in HFD mice to mitigate diabetes (Yang et al., 2021). Bupleurum polysaccharides not only decreased blood creatinine, blood glucose, and urine albumin levels but also repaired the gut barrier and regulated inflammatory responses (Feng et al., 2019). Natural polysaccharides may also improve diabetes by regulating the function of glucose receptors. For example, SSP could promote GLUT-4 levels in BRL cells (Jin et al., 2016). Natural polysaccharides may have many potential antidiabetic activities waiting for us to explore. At the same time, we should also consider how to identify the key signalling pathways and core targets involved in the regulation of natural polysaccharides. It is worth exploring whether network pharmacology can be used to predict potential core targets of natural polysaccharides, and if the signalling pathway of significant enrichment of core targets can be used as the research pathway. Finally, animal experiments or in vitro cell experiments can be conducted to provide evidence.
The study of natural polysaccharides is currently limited to animal and in vitro cell experiments. Animal and cellular models have been used to explore mechanisms of oxidative damage in diabetes, such as streptozotocin, high-fat diets, and alloxan induced mice or rat, ob/ob mice, zucker diabetic fatty rat, as well as otsuka long-evans tokushima fatty rats (King, 2012). A review of polysaccharide applications for diabetes treatment for the past 5 years, revealed that the dose of polysaccharides ranged from 1 to 1,500 mg/kg/d in STZ or HFD induced diabetic models. Moreover, the polysaccharide and was mostly administered through intragastric administration. For example, the polysaccharide from C. paliurus was used as of 1, 10, and 100 mg/kg/d in STZ-induced mice (Zhang et al., 2021). After the administration of 1,500 mg/kg polysaccharide from M. charantia L (Zhang et al., 2019), diabetes symptoms improved. In vivo experiments of mice, polysaccharide doses were mainly distributed in the range of 100–800 mg/kg/d. How dosing is determined is unclear in some papers, but ultimately the results of these non-clinical trials seem to be very promising. We found that the dose of polysaccharides administered in vitro and in vivo seems to differ greatly, which seems to make it difficult to explain the specific effective dose of polysaccharides. In order to develop new treatments for diabetes with natural product characteristics that meet international standards, further clinical studies and evaluation of natural products, as well as structural and mechanism of action, as well as the relationship between biological outcomes and therapeutic effects, are needed. Using modern technology to develop more effective and economical methods to extract and separate polysaccharides, and expand the application of polysaccharides.
Author contributions
L-YH, conceived and designed the study and wrote the manuscript, YL and JB, collected data, S-QN and S-JL, revised the manuscript. J-LG supervised the project. All authors contributed to the article and approved the submitted version.
Funding
This work was supported by the National Natural Sciences Foundation of China (Nos. 81872959, 81373920, 30801522, 82003900). “Xinglin Scholar” Talent Research Promotion Plan of Chengdu University of Traditional Chinese Medicine (No. ZYTS2019022). Young Science and Technology Innovation Team of Sichuan Province (No. 2019CXTD0055).
Acknowledgments
The authors would like to thank MJEditor (www.mjeditor.com) for its linguistic assistance during the preparation of this manuscript.
Conflict of interest
The authors declare that the research was conducted in the absence of any commercial or financial relationships that could be construed as a potential conflict of interest.
Publisher’s note
All claims expressed in this article are solely those of the authors and do not necessarily represent those of their affiliated organizations, or those of the publisher, the editors and the reviewers. Any product that may be evaluated in this article, or claim that may be made by its manufacturer, is not guaranteed or endorsed by the publisher.
References
Ahn, M. Y., Kim, B. J., Kim, H. J., Jin, J. M., Yoon, H. J., Hwang, J. S., et al. (2020). Anti-diabetic activity of field cricket glycosaminoglycan by ameliorating oxidative stress. Bmc. Complement. Med. Ther. 20 (1), 232. doi:10.1186/s12906-020-03027-x
Arunachalam, K., Sreeja, P. S., and Yang, X. (2022). The antioxidant properties of mushroom polysaccharides can potentially mitigate oxidative stress, beta-cell dysfunction and insulin resistance. Front. Pharmacol. 13, 874474. doi:10.3389/fphar.2022.874474
Bertuzzi, F., Colussi, G., Lauterio, A., and De Carlis, L. (2018). Intramuscular islet allotransplantation in type 1 diabetes mellitus. Eur. Rev. Med. Pharmacol. Sci. 22, 1731–1736. doi:10.26355/eurrev_201803_14588
Cai, J., Zhu, Y., Zuo, Y., Tong, Q., Zhang, Z., Yang, L., et al. (2019). Polygonatum sibiricum polysaccharide alleviates inflammatory cytokines and promotes glucose uptake in high glucose and high insulin induced 3T3 L1 adipocytes by promoting Nrf2 expression. Mol. Med. Rep. 20, 3951–3958. doi:10.3892/mmr.2019.10626
Cao, X., Liu, D., Xia, Y., Cai, T., He, Y., and Liu, J. (2019). A novel polysaccharide from Lentinus edodes mycelia protects MIN6 cells against high glucose-induced damage via the MAPKs and Nrf2 pathways. Food. Nutr. Res. 63. doi:10.29219/fnr.v63.1598
Cao, X., Xia, Y., Liu, D., He, Y., Mu, T., Huo, Y., et al. (2020). Inhibitory effects of Lentinus edodes mycelia polysaccharide on α-glucosidase, glycation activity and high glucose-induced cell damage. Carbohydr. Polym. 246, 116659. doi:10.1016/j.carbpol.2020.116659
Ceriello, A., Ihnat, M. A., and Thorpe, J. E. (2009). Clinical review 2: The "metabolic memory": Is more than just tight glucose control necessary to prevent diabetic complications? J. Clin. Endocrinol. Metab. 94, 410–415. doi:10.1210/jc.2008-1824
Cersosimo, E., Johnson, E. L., Chovanes, C., and Skolnik, N. (2018). Initiating therapy in patients newly diagnosed with type 2 diabetes: Combination therapy vs a stepwise approach. Diabetes. Obes. Metab. 20, 497–507. doi:10.1111/dom.13108
Chang, X., Lu, K., Wang, L., Lv, M., and Fu, W. (2018). Astraglaus polysaccharide protects diabetic cardiomyopathy by activating NRG1/ErbB pathway. Biosci. Trends. 12, 149–156. doi:10.5582/bst.2018.01027
Chatterjee, S., Khunti, K., and Davies, M. J. (2017). Type 2 diabetes. Lancet 389, 2239–2251. doi:10.1016/S0140-6736(17)30058-2
Chen, C., Huang, Q., Li, C., and Fu, X. (2017). Hypoglycemic effects of a Fructus mori polysaccharide in vitro and in vivo. Food & Funct. 8, 2523–2535. doi:10.1039/c7fo00417f
Chen, C., Kang, M., Wang, Q., Liu, W., Yang, M., Liang, S., et al. (2021). Combination of Anoectochilus roxburghii polysaccharide and exercise ameliorates diet-induced metabolic disorders in obese mice. Front. Nutr. 8, 735501. doi:10.3389/fnut.2021.735501
Chen, M., Xiao, D., Liu, W., Song, Y., Zou, B., Li, L., et al. (2020). Intake of Ganoderma lucidum polysaccharides reverses the disturbed gut microbiota and metabolism in type 2 diabetic rats. Int. J. Biol. Macromol. 155, 890–902. doi:10.1016/j.ijbiomac.2019.11.047
Chen, P. H., Weng, Y. M., Lin, S. M., Yu, Z. R., and Wang, B. J. (2015). Molecular weight affected antioxidant, hypoglycemic and hypotensive activities of cold water extract from Pleurotus citrinopileatus. J. Food. Sci. 82 (10), 2456–2461. doi:10.1111/1750-3841.13851
Chen, W., Ju, J., Yang, Y., Wang, H., Chen, W., Zhao, X., et al. (2018). Astragalus polysaccharides protect cardiac stem and progenitor cells by the inhibition of oxidative stress-mediated apoptosis in diabetic hearts. Drug. Des. devel. Ther. 12, 943–954. doi:10.2147/DDDT.S155686
Chen, X., Chen, C., and Fu, X. (2022). Hypoglycemic activity in vitro and vivo of a water-soluble polysaccharide from Astragalus membranaceus. Food & Funct. 13 (21), 11210–11222. doi:10.1039/d2fo02298b
Chen, X., Qian, L., Wang, B., Zhang, Z., Liu, H., Zhang, Y., et al. (2019a). Synergistic hypoglycemic effects of pumpkin polysaccharides and puerarin on type II diabetes mellitus mice. Molecules 24 (5), 955. doi:10.3390/molecules24050955
Chen, X., Tang, J., Xie, W., Wang, J., Jin, J., Ren, J., et al. (2013). Protective effect of the polysaccharide from Ophiopogon japonicus on streptozotocin-induced diabetic rats. Carbohydr. Polym. 94 (1), 378–385. doi:10.1016/j.carbpol.2013.01.037
Chen, Y., Wang, Y., Yang, S., Yu, M., Jiang, T., and Lv, Z. (2019b). Glycosaminoglycan from Apostichopus japonicus improves glucose metabolism in the liver of insulin resistant mice. Mar. Drugs. 18 (1), 1. doi:10.3390/md18010001
Cheng, J., Zhou, Z. W., Sheng, H. P., He, L. J., Fan, X. W., He, Z. X., et al. (2015). An evidence-based update on the pharmacological activities and possible molecular targets of Lycium barbarum polysaccharides. Drug Des. devel. Ther. 9, 33–78. doi:10.2147/DDDT.S72892
Cho, E. J., Hwang, H. J., Kim, S. W., Oh, J. Y., Baek, Y. M., Choi, J. W., et al. (2007). Hypoglycemic effects of exopolysaccharides produced by mycelial cultures of two different mushrooms Tremella fuciformis and Phellinus baumii in ob/ob mice. Appl. Microbiol. Biotechnol. 75, 1257–1265. doi:10.1007/s00253-007-0972-2
Dos Santos, J. M., Tewari, S., and Mendes, R. H. (2019). The role of oxidative stress in the development of diabetes mellitus and its complications. J. Diabetes. Res. 2019, 4189813. doi:10.1155/2019/4189813
Eftekharpour, E., and Fernyhough, P. (2022). Oxidative stress and mitochondrial dysfunction associated with peripheral neuropathy in type 1 diabetes. Antioxid. Redox. Signal. 37, 578–596. doi:10.1089/ars.2021.0152
Eizirik, D. L., Pasquali, L., and Cnop, M. (2020). Pancreatic β-cells in type 1 and type 2 diabetes mellitus: Different pathways to failure. Endocrinology. 16, 349–362. doi:10.1038/s41574-020-0355-7
Erejuwa, O. O. (2012). Oxidative stress in diabetes mellitus: Is there a role for hypoglycemic drugs and/or antioxidants? (Oxidative stress and diseases). doi:10.5772/32741
Ezraty, B., Gennaris, A., Barras, F., and Collet, J. F. (2017). Oxidative stress, protein damage and repair in bacteria. Microbiology. 15, 385–396. doi:10.1038/nrmicro.2017.26
Feng, Y., Weng, H., Ling, L., Zeng, T., Zhang, Y., Chen, D., et al. (2019). Modulating the gut microbiota and inflammation is involved in the effect of Bupleurum polysaccharides against diabetic nephropathy in mice. Int. J. Biol. Macromol. 132, 1001–1011. doi:10.1016/j.ijbiomac.2019.03.242
Galicia-Garcia, U., Benito-Vicente, A., Jebari, S., Larrea-Sebal, A., Siddiqi, H., Uribe, K. B., et al. (2020). Pathophysiology of type 2 diabetes mellitus. Int. J. Mol. Sci. 21, 6275. doi:10.3390/ijms21176275
Ganesan, K., and Xu, B. (2019). Anti-diabetic effects and mechanisms of dietary polysaccharides. Molecules 24 (14), 2556. doi:10.3390/molecules24142556
Gao, H., Wen, J. J., Hu, J. L., Nie, Q. X., Chen, H. H., Xiong, T., et al. (2018). Polysaccharide from fermented Momordica charantia L. with Lactobacillus plantarum NCU116 ameliorates type 2 diabetes in rats. Carbohydr. Polym. 201, 624–633. doi:10.1016/j.carbpol.2018.08.075
Gao, Z., Kong, D., Cai, W., Zhang, J., and Jia, L. (2021). Characterization and anti-diabetic nephropathic ability of mycelium polysaccharides from Coprinus comatus. Carbohydr. Polym. 251, 117081. doi:10.1016/j.carbpol.2020.117081
Gerich, J. E. (2003). Contributions of insulin-resistance and insulin-secretory defects to the pathogenesis of type 2 diabetes mellitus. Mayo. Clin. Proc. 78, 447–456. doi:10.4065/78.4.447
Ghosh, A., Gao, L., Thakur, A., Siu, P. M., and Lai, C. W. K. (2017). Role of free fatty acids in endothelial dysfunction. J. Biomed. Sci. 24, 50. doi:10.1186/s12929-017-0357-5
Giacco, F., and Brownlee, M. (2010). Oxidative stress and diabetic complications. Circulation. Res. 107, 1058–1070. doi:10.1161/CIRCRESAHA.110.223545
Giannini, C., Mohn, A., Chiarelli, F., and Kelnar, C. J. (2011). Macrovascular angiopathy in children and adolescents with type 1 diabetes. Metab. Res. Rev. 27, 436–460. doi:10.1002/dmrr.1195
Goncalves, J. S., Seica, R. M., Laranjinha, J., and Lourenco, C. F. (2022). Impairment of neurovascular coupling in the hippocampus due to decreased nitric oxide bioavailability supports early cognitive dysfunction in type 2 diabetic rats. Free. Radic. Biol. Med. 193, 669–675. doi:10.1016/j.freeradbiomed.2022.11.009
Gong, P., Cui, D., Guo, Y., Wang, M., Wang, Z., Huang, Z., et al. (2021). A novel polysaccharide obtained from Siraitia grosvenorii alleviates inflammatory responses in a diabetic nephropathy mouse model via the TLR4-NF-κB pathway. Food & Funct. 12, 9054–9065. doi:10.1039/d1fo01182k
Gong, P., Wang, X., Liu, M., Wang, M., Wang, S., Guo, Y., et al. (2022). Hypoglycemic effect of a novel polysaccharide from Lentinus edodes on STZ-induced diabetic mice via metabolomics study and Nrf2/HO-1 pathway. Food & Funct. 13, 3036–3049. doi:10.1039/d1fo03487a
Green, A. D., Vasu, S., and Flatt, P. R. (2018). Cellular models for beta-cell function and diabetes gene therapy. Acta. Physiol. (Oxf). 222, e13012. doi:10.1111/apha.13012
Guo, Y., Li, S., Li, J., Ren, Z., Chen, F., and Wang, X. (2017). Anti-hyperglycemic activity of polysaccharides from calyx of Physalis alkekengi var. franchetii Makino on alloxan-induced mice. Int. J. Biol. Macromol. 99, 249–257. doi:10.1016/j.ijbiomac.2017.02.086
Halim, M., and Halim, A. (2019). The effects of inflammation, aging and oxidative stress on the pathogenesis of diabetes mellitus (type 2 diabetes). Diabetes. Metab. Syndr. 13, 1165–1172. doi:10.1016/j.dsx.2019.01.040
He, L., Huan, P., Xu, J., Chen, Y., Zhang, L., Wang, J., et al. (2022). Hedysarum polysaccharide alleviates oxidative stress to protect against diabetic peripheral neuropathy via modulation of the keap1/Nrf2 signaling pathway. J. Chem. Neuroanat. 126, 102182. doi:10.1016/j.jchemneu.2022.102182
Hu, J. L., Nie, S. P., and Xie, M. Y. (2018). Antidiabetic mechanism of dietary polysaccharides based on their gastrointestinal functions. J. Agric. Food. Chem. 66, 4781–4786. doi:10.1021/acs.jafc.7b05410
Hu, W., Wang, J., Guo, W., Liu, Y., Guo, Z., Miao, Y., et al. (2020). Studies on characteristics and anti-diabetic and -nephritic effects of polysaccharides isolated from Paecilomyces hepiali fermentation mycelium in db/db mice. Carbohydr. Polym. 232, 115766. doi:10.1016/j.carbpol.2019.115766
Ighodaro, O. M. (2018). Molecular pathways associated with oxidative stress in diabetes mellitus. Biomed. Pharmacother. 108, 656–662. doi:10.1016/j.biopha.2018.09.058
Jin, D., Zhao, T., Feng, W. W., Mao, G. H., Zou, Y., Wang, W., et al. (2016). Schisandra polysaccharide increased glucose consumption by up-regulating the expression of GLUT-4. Int. J. Biol. Macromol. 87, 555–562. doi:10.1016/j.ijbiomac.2016.03.028
Kaneto, H., Matsuoka, T. A., Kimura, T., Obata, A., Shimoda, M., Kamei, S., et al. (2016). Appropriate therapy for type 2 diabetes mellitus in view of pancreatic beta-cell glucose toxicity: "the earlier, the better. J. Diabetes. 8, 183–189. doi:10.1111/1753-0407.12331
Kang, Q., and Yang, C. (2020). Oxidative stress and diabetic retinopathy: Molecular mechanisms, pathogenetic role and therapeutic implications. Redox. Biol. 37, 101799. doi:10.1016/j.redox.2020.101799
Kelly, M. S., Lewis, J., Huntsberry, A. M., Dea, L., and Portillo, I. (2019). Efficacy and renal outcomes of SGLT2 inhibitors in patients with type 2 diabetes and chronic kidney disease. Postgrad. Med. 131, 31–42. doi:10.1080/00325481.2019.1549459
Kessler, L., Wiesel, M. L., Attali, P., Mossard, J. M., Cazenave, J. P., and Pinget, M. (1998). Von Willebrand factor in diabetic angiopathy. Diabetes. Metab. 24, 327–336.
King, A. J. (2012). The use of animal models in diabetes research. Br. J. Pharmacol. 166 (3), 877–894. doi:10.1111/j.1476-5381.2012.01911.x
Kou, L., Du, M., Liu, P., Zhang, B., Zhang, Y., Yang, P., et al. (2019). Anti-diabetic and anti-nephritic activities of grifola frondosa mycelium polysaccharides in diet-streptozotocin-induced diabetic rats via modulation on oxidative stress. Appl. Biochem. And. Biotechnol. 187, 310–322. doi:10.1007/s12010-018-2803-6
Leeuwenburgh, C., Fiebig, R., Chandwaney, R., and Ji, L. L. (1994). Aging and exercise training in skeletal muscle: Responses of glutathione and antioxidant enzyme systems. Am. J. Physiol. 267, R439–R445. doi:10.1152/ajpregu.1994.267.2.R439
Li, H. N., Zhao, L. L., Zhou, D. Y., and Chen, D. Q. (2020a). Ganoderma lucidum polysaccharides ameliorates hepatic steatosis and oxidative stress in db/db mice via targeting nuclear factor E2 (Erythroid-Derived 2)-related factor-2/heme oxygenase-1 (HO-1) pathway. Med. Sci. Monit. 26, e921905. doi:10.12659/MSM.921905
Li, J. S., Ji, T., Su, S. L., Zhu, Y., Chen, X. L., Shang, E. X., et al. (2022). Mulberry leaves ameliorate diabetes via regulating metabolic profiling and AGEs/RAGE and p38 MAPK/NF-κB pathway. J. Ethnopharmacol. 283, 114713. doi:10.1016/j.jep.2021.114713
Li, Q., Hu, J., Nie, Q., Chang, X., Fang, Q., Xie, J., et al. (2021). Hypoglycemic mechanism of polysaccharide from Cyclocarya paliurus leaves in type 2 diabetic rats by gut microbiota and host metabolism alteration. Sci. China. Life. Sci. 64, 117–132. doi:10.1007/s11427-019-1647-6
Li, T., Hua, Q., Li, N., Cui, Y., and Zhao, M. (2019a). Protective effect of a polysaccharide from Dipsacus asper Wall on streptozotocin (STZ)-induced diabetic nephropathy in rat. Int. J. Biol. Macromol. 133, 1194–1200. doi:10.1016/j.ijbiomac.2019.04.069
Li, W., Lin, K., Zhou, M., Xiong, Q., Li, C., and Ru, Q. (2020b). Polysaccharides from Opuntia milpa alta alleviate alloxan-induced INS-1 cells apoptosis via reducing oxidative stress and upregulating Nrf2 expression. Nutr. Res. 77, 108–118. doi:10.1016/j.nutres.2020.02.004
Li, X. M. (2007). Protective effect of Lycium barbarum polysaccharides on streptozotocin-induced oxidative stress in rats. Int. J. Biol. Macromol. 40, 461–465. doi:10.1016/j.ijbiomac.2006.11.002
Li, X., Zhao, Z., Kuang, P., Shi, X., Wang, Z., and Guo, L. (2019b). Regulation of lipid metabolism in diabetic rats by Arctium lappa L. polysaccharide through the PKC/NF-κB pathway. Int. J. Biol. Macromol. 136, 115–122. doi:10.1016/j.ijbiomac.2019.06.057
Lian, Y., Zhu, M., Chen, J., Yang, B., Lv, Q., Wang, L., et al. (2021). Characterization of a novel polysaccharide from Moutan Cortex and its ameliorative effect on AGEs-induced diabetic nephropathy. Diabetes. Metab. J. 176, 589–600. doi:10.1016/j.ijbiomac.2021.02.062
Liao, Z., Zhang, J., Liu, B., Yan, T., Jia, Y. J. M., Xiao, F., et al. (2019a). Polysaccharide from okra (Abelmoschus esculentus (L) moench) improves antioxidant capacity via PI3K/AKT pathways and Nrf2 translocation in a type 2 diabetes model. Molecules 24, 1906. doi:10.3390/molecules24101906
Liao, Z., Zhang, J., Wang, J., Yan, T., Xu, F., Wu, B., et al. (2019b). The anti-nephritic activity of a polysaccharide from okra (Abelmoschus esculentus (L) Moench) via modulation of AMPK-Sirt1-PGC-1α signaling axis mediated anti-oxidative in type 2 diabetes model mice. Int. J. Biol. Macromol. 140, 568–576. doi:10.1016/j.ijbiomac.2019.08.149
Lin, C., Kuo, T. C., Lin, J. C., Ho, Y. C., and Mi, F. L. (2020). Delivery of polysaccharides from Ophiopogon japonicus (OJPs) using OJPs/chitosan/whey protein co-assembled nanoparticles to treat defective intestinal epithelial tight junction barrier. Int. J. Biol. Macromol. 160, 558–570. doi:10.1016/j.ijbiomac.2020.05.151
Lin, C., Lin, Y., Xiao, J., Lan, Y., Cao, Y., and Chen, Y. (2021a). Effect of Momordica saponin- and Cyclocarya paliurus polysaccharide-enriched beverages on oxidative stress and fat accumulation in Caenorhabditis elegans. J. Sci. Food. Agric. 101, 3366–3375. doi:10.1002/jsfa.10966
Lin, H., Lin, T. Y., Lin, J. A., Cheng, K. C., Santoso, S. P., Chou, C. H., et al. (2021b). Effect of pholiota nameko polysaccharides inhibiting methylglyoxal-induced glycation damage in vitro. Antioxidants. (Basel) 10, 1589. doi:10.3390/antiox10101589
Liu, C. G., Ma, Y. P., and Zhang, X. J. (2017). Effects of mulberry leaf polysaccharide on oxidative stress in pancreatic β-cells of type 2 diabetic rats. Eur. Rev. Med. Pharmacol. Sci. 21, 2482–2488.
Liu, C., Song, J., Teng, M., Zheng, X., Li, X., Tian, Y., et al. (2016). Antidiabetic and antinephritic activities of aqueous extract of cordyceps militaris fruit body in diet-streptozotocin-induced diabetic sprague dawley rats. Oxid. Med. Cell. Longev. 2016, 9685257. doi:10.1155/2016/9685257
Liu, M., Li, Y., Zhang, W., Sun, M., and Zhang, Z. (2019). Hypoglycemic effect of inulin combined with ganoderma lucidum polysaccharides in T2DM rats. J. Funct.Foods. 55, 381–390. doi:10.1016/j.jff.2019.02.036
Liu, X., Luo, D., Guan, J., Chen, J., and Xu, X. (2022). Mushroom polysaccharides with potential in anti-diabetes: Biological mechanisms, extraction, and future perspectives: A review. A Rev. Front. Nutr. 9, 1087826. doi:10.3389/fnut.2022.1087826
Liu, Y., Liu, Y., Zhang, M., Li, C., Zhang, Z., Liu, A., et al. (2020a). Structural characterization of a polysaccharide from Suillellus luridus and its antidiabetic activity via Nrf2/HO-1 and NF-κB pathways. Int. J. Biol. Macromol. 162, 935–945. doi:10.1016/j.ijbiomac.2020.06.212
Liu, Y., Yang, L., Zhang, Y., Liu, X., Wu, Z., Gilbert, R. G., et al. (2020b). Dendrobium officinale polysaccharide ameliorates diabetic hepatic glucose metabolism via glucagon-mediated signaling pathways and modifying liver-glycogen structure. J. Ethnopharmacol. 248, 112308. doi:10.1016/j.jep.2019.112308
Luo, D., Dong, X., Huang, J., Huang, C., Fang, G., and Huang, Y. (2021). Pueraria lobata root polysaccharide alleviates glucose and lipid metabolic dysfunction in diabetic db/db mice. Pharm. Biol. 59, 382–390. doi:10.1080/13880209.2021.1898648
Luo, Y., Peng, B., Wei, W., Tian, X., and Wu, Z. (2019). Antioxidant and anti-diabetic activities of polysaccharides from guava Leaves. Molecules. Basel, Switzerland, 24. doi:10.3390/molecules24071343
Maity, P., Sen, I. K., Chakraborty, I., Mondal, S., Bar, H., Bhanja, S. K., et al. (2021). Biologically active polysaccharide from edible mushrooms: A review. Int. J. Biol. Macromol. 172, 408–417. doi:10.1016/j.ijbiomac.2021.01.081
Maritim, A. C., Sanders, R. A., and Watkins, J. B. (2003). Diabetes, oxidative stress, and antioxidants: A review. J. Biochem. Mol. Toxicol. 17, 24–38. doi:10.1002/jbt.10058
Miccoli, R., Penno, G., and Del Prato, S. (2011). Multidrug treatment of type 2 diabetes: A challenge for compliance. Diabetes Care 34 (2), S231–S235. doi:10.2337/dc11-s235
Mima, A. (2016). Incretin-Based therapy for prevention of diabetic vascular complications. J. Diabetes. Res. 2016, 1379274. doi:10.1155/2016/1379274
Newmeyer, D. D., and Ferguson-Miller, S. J. C. (2003). Mitochondria: Releasing power for life and unleashing the machineries of death. Cell 112, 481–490. doi:10.1016/s0092-8674(03)00116-8
Nie, C., Zhu, P., Wang, M., Ma, S., and Wei, Z. (2017). Optimization of water-soluble polysaccharides from stem lettuce by response surface methodology and study on its characterization and bioactivities. Int. J. Biol. Macromol. 105, 912–923. doi:10.1016/j.ijbiomac.2017.07.125
Nishikawa, T., Edelstein, D., Du, X. L., Yamagishi, S., Matsumura, T., Kaneda, Y., et al. (2000). Normalizing mitochondrial superoxide production blocks three pathways of hyperglycaemic damage. Nature 404, 787–790. doi:10.1038/35008121
Niu, J., Xu, G., Jiang, S., Li, H., and Yuan, G. (2017). In vitro Antioxidant activities and anti-diabetic effect of a polysaccharide from Schisandra sphenanthera in rats with type 2 diabetes. Int. J. Biol. Macromol. 94, 154–160. doi:10.1016/j.ijbiomac.2016.10.015
Omolola, R., Nicole, L., and Oluwafemi, O. (2014). Oxidative stress and diabetic complications: The role of antioxidant vitamins and flavonoids. doi:10.5772/57282
Petersen, K. F., Befroy, D., Dufour, S., Dziura, J., Ariyan, C., Rothman, D. L., et al. (2003). Mitochondrial dysfunction in the elderly: Possible role in insulin resistance. Sci. N. Y. N.Y.). 300, 1140–1142. doi:10.1126/science.1082889
Poznyak, A., Grechko, A. V., Poggio, P., Myasoedova, V. A., Alfieri, V., and Orekhov, A. N. (2020). The diabetes mellitus-atherosclerosis connection: The role of lipid and glucose metabolism and chronic inflammation. Int. J. Mol. Sci. 21, 1835. doi:10.3390/ijms21051835
Qi, W., Keenan, H. A., Li, Q., Ishikado, A., Kannt, A., Sadowski, T., et al. (2017). Pyruvate kinase M2 activation may protect against the progression of diabetic glomerular pathology and mitochondrial dysfunction. Nat. Med. 23, 753–762. doi:10.1038/nm.4328
Qiu, A., Wang, Y., Zhang, G., and Wang, H. (2022). Natural polysaccharide-based nanodrug delivery systems for treatment of diabetes. Polym. (Basel) 14, 3217. doi:10.3390/polym14153217
Ren, B., Chen, C., Li, C., Fu, X., You, L., and Liu, R. H. (2017). Optimization of microwave-assisted extraction of Sargassum thunbergii polysaccharides and its antioxidant and hypoglycemic activities. Carbohydr. Polym. 173, 192–201. doi:10.1016/j.carbpol.2017.05.094
Ren, C., Zhang, Y., Cui, W., Lu, G., Wang, Y., Gao, H., et al. (2015). A polysaccharide extract of mulberry leaf ameliorates hepatic glucose metabolism and insulin signaling in rats with type 2 diabetes induced by high fat-diet and streptozotocin. Int. J. Biol. Macromol. 72, 951–959. doi:10.1016/j.ijbiomac.2014.09.060
Rickels, M. R., and Robertson, R. P. (2019). Pancreatic islet transplantation in humans: Recent progress and future directions. Endocr. Rev. 40, 631–668. doi:10.1210/er.2018-00154
Salazar-Garcia, M., and Corona, J. C. (2021). The use of natural compounds as a strategy to counteract oxidative stress in animal models of diabetes mellitus. Int. J. Mol. Sci. 22 (13), 7009. doi:10.3390/ijms22137009
Schieber, M., and Chandel, N. S. (2014). ROS function in redox signaling and oxidative stress. Curr. Biol. CB 24, R453–R462. doi:10.1016/j.cub.2014.03.034
Sergi, D., Naumovski, N., Heilbronn, L. K., Abeywardena, M., O'Callaghan, N., Lionetti, L., et al. (2019). Mitochondrial (Dys)function and insulin resistance: From pathophysiological molecular mechanisms to the impact of diet. Front. physiology. 10, 532. doi:10.3389/fphys.2019.00532
Shi, G. J., Zheng, J., Wu, J., Qiao, H. Q., Chang, Q., Niu, Y., et al. (2017). Beneficial effects of Lycium barbarum polysaccharide on spermatogenesis by improving antioxidant activity and inhibiting apoptosis in streptozotocin-induced diabetic male mice. Food & Funct. 8, 1215–1226. doi:10.1039/c6fo01575a
Spinelli, J. B., and Haigis, M. C. (2018). The multifaceted contributions of mitochondria to cellular metabolism. Nat. Cell. Biol. 20, 745–754. doi:10.1038/s41556-018-0124-1
Sun, Q., Wu, X., Wang, H., Chen, W., Zhao, X., Yang, Y., et al. (2019). Protective effects of Astragalus polysaccharides on oxidative stress in high glucose-induced or SOD2-silenced H9C2 cells based on PCR array analysis. Diabetes. Metab. Syndr. Obes. 12, 2209–2220. doi:10.2147/DMSO.S228351
Sun, W., Zhang, Y., and Jia, L. J. F. B. (2022). Polysaccharides from Agrocybe cylindracea residue alleviate type 2-diabetes-induced liver and colon injuries by p38 MAPK signaling pathway. Food. Biosci. 47, 101690. doi:10.1016/j.fbio.2022.101690
Taylor, R. (2013). Type 2 diabetes: Etiology and reversibility. Diabetes. Care. 36, 1047–1055. doi:10.2337/dc12-1805
Thakur, P., Kumar, A., and Kumar, A. (2018). Targeting oxidative stress through antioxidants in diabetes mellitus. J. Drug. Target. 26, 766–776. doi:10.1080/1061186X.2017.1419478
Ti, Y., Wang, W., Wang, X., Ban, Y., Wang, P., Zhang, Y., et al. (2022). Pumpkin polysaccharide extracted by subcritical water: Physicochemical characterization and anti-diabetic effects in T2DM rats. Mol. Nutr. Food. Res. 66, e2200160. doi:10.1002/mnfr.202200160
Tian, X., Liang, T., Liu, Y., Ding, G., Zhang, F., and Ma, Z. (2019). Extraction, structural characterization, and biological functions of lycium barbarum polysaccharides: A review. Biomolecules 9 (9), 389. doi:10.3390/biom9090389
Venkatasamy, V. V., Pericherla, S., Manthuruthil, S., Mishra, S., and Hanno, R. (2013). Effect of physical activity on insulin resistance, inflammation and oxidative stress in diabetes mellitus. JCDR 7, 1764–1766. doi:10.7860/JCDR/2013/6518.3306
Wang, C., Gao, X., Santhanam, R. K., Chen, Z., Chen, Y., Xu, L., et al. (2018). Effects of polysaccharides from Inonotus obliquus and its chromium (III) complex on advanced glycation end-products formation, α-amylase, α-glucosidase activity and H2O2-induced oxidative damage in hepatic L02 cells. Food. Chem. Toxicol. 116, 335–345. doi:10.1016/j.fct.2018.04.047
Wang, D., Li, C., Fan, W., Yi, T., Wei, A., and Ma, Y. (2019a). Hypoglycemic and hypolipidemic effects of a polysaccharide from Fructus Corni in streptozotocin-induced diabetic rats. Int. J. Biol. Macromol. 133, 420–427. doi:10.1016/j.ijbiomac.2019.04.160
Wang, J., Hu, W., Li, L., Huang, X., Liu, Y., Wang, D., et al. (2017a). Antidiabetic activities of polysaccharides separated from Inonotus obliquus via the modulation of oxidative stress in mice with streptozotocin-induced diabetes. PloS. one . 12, e0180476. doi:10.1371/journal.pone.0180476
Wang, L., Li, C., Huang, Q., and Fu, X. (2019b). Biofunctionalization of selenium nanoparticles with a polysaccharide from Rosa roxburghii fruit and their protective effect against H(2)O(2)-induced apoptosis in INS-1 cells. Food & Funct. 10, 539–553. doi:10.1039/c8fo01958d
Wang, P. C., Zhao, S., Yang, B. Y., Wang, Q. H., and Kuang, H. X. (2016). Anti-diabetic polysaccharides from natural sources: A review. Carbohydr. Polym. 148, 86–97. doi:10.1016/j.carbpol.2016.02.060
Wang, S., Lu, A., Zhang, L., Shen, M., Xu, T., Zhan, W., et al. (2017b). Extraction and purification of pumpkin polysaccharides and their hypoglycemic effect. Int. J. Biol. Macromol. 98, 182–187. doi:10.1016/j.ijbiomac.2017.01.114
Wang, W., Li, S., and Song, M. (2022). Polygonatum sibiricum polysaccharide inhibits high glucose-induced oxidative stress, inflammatory response, and apoptosis in RPE cells. J. Recept. Signal. Transduct. Res. 42, 189–196. doi:10.1080/10799893.2021.1883061
Wang, Y., Lin, D., Wang, X., Zhu, W., Ye, J., Li, G., et al. (2017c). The impact of a novel peach gum-derived polysaccharide on postprandial blood glucose control in streptozotocin-induced diabetic mice. Int. J. Biol. Macromol. 98, 379–386. doi:10.1016/j.ijbiomac.2017.01.085
Wang, Y., Qin, S., Pen, G., Chen, D., Han, C., Miao, C., et al. (2017d). Original Research: Potential ocular protection and dynamic observation of Polygonatum sibiricum polysaccharide against streptozocin-induced diabetic rats' model. Exp. Biol. Med. (Maywood). 242, 92–101. doi:10.1177/1535370216663866
Wu, C. Y., Wang, H., He, X. X., Wu, D. W., Yue, W., and Wu, Q. N. (2017a). The hypoglycemic and antioxidant effects of polysaccharides from the petioles and pedicels of Euryale ferox Salisb. on alloxan-induced hyperglycemic mice. Food & Funct. 8, 3803–3813. doi:10.1039/c7fo01035d
Wu, J., Chen, M., Shi, S., Wang, H., Li, N., Su, J., et al. (2017b). Hypoglycemic effect and mechanism of a pectic polysaccharide with hexenuronic acid from the fruits of Ficus pumila L. in C57BL/KsJ db/db mice. Carbohydr. Polym. 178, 209–220. doi:10.1016/j.carbpol.2017.09.050
Wu, L., Liu, X., Hu, R., Chen, Y., Xiao, M., Liu, B., et al. (2022a). Prebiotic Agrocybe cylindracea crude polysaccharides combined with Lactobacillus rhamnosus GG postpone aging-related oxidative stress in mice. Food & Funct. 13, 1218–1231. doi:10.1039/d1fo02079j
Wu, M. Y., Yiang, G. T., Lai, T. T., and Li, C. J. (2018). The oxidative stress and mitochondrial dysfunction during the pathogenesis of diabetic retinopathy. Oxid. Med. Cell. Longev. 2018, 3420187. doi:10.1155/2018/3420187
Wu, N., Jin, W., Zhao, Y., Wang, H., He, S., Zhang, W., et al. (2022b). Sulfated fucogalactan from Laminaria japonica ameliorates β-cell failure by attenuating mitochondrial dysfunction via SIRT1-PGC1-α signaling pathway activation. Front. Endocrinol. (Lausanne). 13, 881256. doi:10.3389/fendo.2022.881256
Xia, X., Mao, D., Dai, H., Wu, X., Zhang, Z., Wang, H., et al. (2020). Effect of Cyclocarya paliurus polysaccharides on streptozotocin-induced diabetic nephropathy in rats. J. Tradit. Chin. Med. 40, 956–964. doi:10.19852/j.cnki.jtcm.2020.06.007
Xiang, H., Sun-Waterhouse, D., and Cui, C. J. (2021). Hypoglycemic polysaccharides from Auricularia auricula and Auricularia polytricha inhibit oxidative stress, NF-κB signalling and proinflammatory cytokine production in streptozotocin-induced diabetic mice. Food. Sci. Hum. Health. 001,010.
Xu, Y., Zhang, X., Yan, X. H., Zhang, J. L., Wang, L. Y., Xue, H., et al. (2019). Characterization, hypolipidemic and antioxidant activities of degraded polysaccharides from Ganoderma lucidum. Int. J. Biol. Macromol. 135, 706–716. doi:10.1016/j.ijbiomac.2019.05.166
Yamagishi, S., and Matsui, T. (2010). Advanced glycation end products, oxidative stress and diabetic nephropathy. Oxid. Med. Cell. Longev. 3, 101–108. doi:10.4161/oxim.3.2.11148
Yang, J., Chen, H., Nie, Q., Huang, X., and Nie, S. (2020a). Dendrobium officinale polysaccharide ameliorates the liver metabolism disorders of type II diabetic rats. Int. J. Biol. Macromol. 164, 1939–1948. doi:10.1016/j.ijbiomac.2020.08.007
Yang, L., Li, P., Fu, S., Calay, E. S., and Hotamisligil, G. S. (2010). Defective hepatic autophagy in obesity promotes ER stress and causes insulin resistance. Cell. Metab. 11, 467–478. doi:10.1016/j.cmet.2010.04.005
Yang, R., Li, Y., Mehmood, S., Yan, C., Huang, Y., Cai, J., et al. (2020b). Polysaccharides from Armillariella tabescens mycelia ameliorate renal damage in type 2 diabetic mice. Int. J. Biol. Macromol. 162, 1682–1691. doi:10.1016/j.ijbiomac.2020.08.006
Yang, Y., Chang, Y., Wu, Y., Liu, H., Liu, Q., Kang, Z., et al. (2021). A homogeneous polysaccharide from Lycium barbarum: Structural characterizations, anti-obesity effects and impacts on gut microbiota. Int. J. Biol. Macromol. 183, 2074–2087. doi:10.1016/j.ijbiomac.2021.05.209
Yao, L., Liang, X., Qiao, Y., Chen, B., Wang, P., and Liu, Z. (2022a). Mitochondrial dysfunction in diabetic tubulopathy. Metabolism 131, 155195. doi:10.1016/j.metabol.2022.155195
Yao, X., Yuan, Y., Jing, T., Ye, S., Wang, S., and Xia, D. (2022b). Ganoderma lucidum polysaccharide ameliorated diabetes mellitus-induced erectile dysfunction in rats by regulating fibrosis and the NOS/ERK/JNK pathway. Transl. Androl. Urol. 11, 982–995. doi:10.21037/tau-22-428
Yaribeygi, H., Farrokhi, F. R., Butler, A. E., and Sahebkar, A. (2019). Insulin resistance: Review of the underlying molecular mechanisms. J. Cell. Physiol. 234, 8152–8161. doi:10.1002/jcp.27603
Yaribeygi, H., Sathyapalan, T., Atkin, S. L., and Sahebkar, A. (2020). Molecular mechanisms linking oxidative stress and diabetes mellitus. Oxid. Med. Cell. Longev. 2020, 8609213. doi:10.1155/2020/8609213
Yuan, B., Yang, X. Q., Kou, M., Lu, C. Y., Wang, Y. Y., Peng, J., et al. (2017). Selenylation of polysaccharide from the sweet potato and evaluation of antioxidant, antitumor, and antidiabetic activities. J. Agric. Food. Chem. 65, 605–617. doi:10.1021/acs.jafc.6b04788
Zhang, C., Huang, M., Hong, R., and Chen, H. (2019a). Preparation of a Momordica charantia L. polysaccharide-chromium (III) complex and its anti-hyperglycemic activity in mice with streptozotocin-induced diabetes. Int. J. Biol. Macromol. 122, 619–627. doi:10.1016/j.ijbiomac.2018.10.200
Zhang, C., Zhang, L., Liu, H., Zhang, J., Hu, C., and Jia, L. (2018a). Antioxidation, anti-hyperglycaemia and renoprotective effects of extracellular polysaccharides from Pleurotus eryngii SI-04. Int. J. Biol. Macromol. 111, 219–228. doi:10.1016/j.ijbiomac.2018.01.009
Zhang, G., Huang, Y., Bian, Y., Wong, J. H., Ng, T. B., Wang, H. J. A. M., et al. (2006). Hypoglycemic activity of the fungi Cordyceps militaris, Cordyceps sinensis, Tricholoma mongolicum, and Omphalia lapidescens in streptozotocin-induced diabetic rats. Appl. Microbiol. Biotechno. l72, 1152–1156. doi:10.1007/s00253-006-0411-9
Zhang, J., Meng, G., Zhang, C., Lin, L., Xu, N., Liu, M., et al. (2015). The antioxidative effects of acidic-alkalic-and enzymatic-extractable mycelium zinc polysaccharides by Pleurotus djamor on liver and kidney of streptozocin-induced diabetic mice. Bmc. Complement. Altern. Med. 15, 440. doi:10.1186/s12906-015-0964-1
Zhang, J., Zhao, X., Zhao, L. Q., Zhao, J., Qi, Z., and Wang, L. A. (2017). A primary study of the antioxidant, hypoglycemic, hypolipidemic, and antitumor activities of ethanol extract of Brown slimecap mushroom, chroogomphus rutilus (agaricomycetes). Int. J. Med. Mushrooms. 19, 905–913. doi:10.1615/IntJMedMushrooms.2017024564
Zhang, K., Qi, J. X., Li, Y. Y., Gao, H. S., Shao, X. Y., Su, C. Y., et al. (2021). Antioxidative and immunological effects of Cyclocarya paliurus polysaccharides on the spleen injury of diabetic rat. J. Tradit. Chin. Med. 41, 739–746. doi:10.19852/j.cnki.jtcm.20210203.001
Zhang, L., Liu, Y., Ke, Y., Liu, Y., Luo, X., Li, C., et al. (2018b). Antidiabetic activity of polysaccharides from Suillellus luridus in streptozotocin-induced diabetic mice. Int. J. Biol. Macromol. 119, 134–140. doi:10.1016/j.ijbiomac.2018.07.109
Zhang, Q., Olatunji, O. J., Chen, H., Tola, A. J., and Oluwaniyi, O. O. (2018c). Evaluation of the anti-diabetic activity of polysaccharide from cordyceps cicadae in experimental diabetic rats. Chem. Biodivers. 15, e1800219. doi:10.1002/cbdv.201800219
Zhang, Y., Wang, H., Zhang, L., Yuan, Y., and Yu, D. (2020). Codonopsis lanceolata polysaccharide CLPS alleviates high fat/high sucrose diet-induced insulin resistance via anti-oxidative stress. Int. J. Biol. Macromol. 145, 944–949. doi:10.1016/j.ijbiomac.2019.09.185
Zhang, Z., Zhang, L., and Xu, H. (2019b). Effect of Astragalus polysaccharide in treatment of diabetes mellitus: A narrative review. J. Tradit. Chin. Med. 39, 133–138.
Zhao, C., Liao, Z., Wu, X., Liu, Y., Liu, X., Lin, Z., et al. (2014). Isolation, purification, and structural features of a polysaccharide from Phellinus linteus and its hypoglycemic effect in alloxan-induced diabetic mice. J. Food. Sci. 79, H1002–H1010. doi:10.1111/1750-3841.12464
Zhao, H., Lai, Q., Zhang, J., Huang, C., and Jia, L. (2018). Antioxidant and hypoglycemic effects of acidic-extractable polysaccharides from cordyceps militaris on type 2 diabetes mice. Oxid. Med. Cell. Longev. 2018, 9150807. doi:10.1155/2018/9150807
Zheng, Y., Bai, L., Zhou, Y., Tong, R., Zeng, M., Li, X., et al. (2019). Polysaccharides from Chinese herbal medicine for anti-diabetes recent advances. Int. J. Biol. Macromol. 121, 1240–1253. doi:10.1016/j.ijbiomac.2018.10.072
Zhou, J., Xu, G., Yan, J., Li, K., Bai, Z., Cheng, W., et al. (2015a). Rehmannia glutinosa (Gaertn) DC. polysaccharide ameliorates hyperglycemia, hyperlipemia and vascular inflammation in streptozotocin-induced diabetic mice. J. Ethnopharmacol. 164, 229–238. doi:10.1016/j.jep.2015.02.026
Zhou, J., Yan, J., Bai, Z., Li, K., and Huang, K. (2015b). Hypoglycemic activity and potential mechanism of a polysaccharide from the loach in streptozotocin-induced diabetic mice. Carbohydr. Polym. 121, 199–206. doi:10.1016/j.carbpol.2014.12.037
Zhu, J., Sun, H., Kang, X., Zhu, H., and Yan, X. (2022). Acidic polysaccharides from Buddleja officinalis inhibit angiogenesis via the Nrf2/ARE pathway to attenuate diabetic retinopathy. Food & Funct. 13, 9021–9031. doi:10.1039/d2fo01075e
Glossary
Keywords: diabetes mellitus, natural polysaccharide, oxidative stress, metabolism, mechanism
Citation: He L-Y, Li Y, Niu S-Q, Bai J, Liu S-J and Guo J-L (2023) Polysaccharides from natural resource: ameliorate type 2 diabetes mellitus via regulation of oxidative stress network. Front. Pharmacol. 14:1184572. doi: 10.3389/fphar.2023.1184572
Received: 12 March 2023; Accepted: 04 July 2023;
Published: 11 July 2023.
Edited by:
Yongmei Xie, Sichuan University, ChinaReviewed by:
Xichun Pan, Army Medical University, ChinaChongbo Zhao, Shaanxi University of Chinese Medicine, China
Mingxing Hu, Sichuan University, China
Copyright © 2023 He, Li, Niu, Bai, Liu and Guo. This is an open-access article distributed under the terms of the Creative Commons Attribution License (CC BY). The use, distribution or reproduction in other forums is permitted, provided the original author(s) and the copyright owner(s) are credited and that the original publication in this journal is cited, in accordance with accepted academic practice. No use, distribution or reproduction is permitted which does not comply with these terms.
*Correspondence: Si-Jing Liu, bGl1c2lqaW5nQGNkdXRjbS5lZHUuY24=; Jin-Lin Guo, Z3VvNTk2QGNkdXRjbS5lZHUuY24=