- 1Henan Stomatological Hospital, The First Affiliated Hospital of Zhengzhou University, Zhengzhou, China
- 2School of Stomatology, Wuhan University, Wuhan, China
- 3Faculty of Health Science, Campus Levanger, Nord University, Levanger, Norway
Oral squamous cell carcinoma (OSCC) is a common human malignancy with an estimated incidence of around 377,713 new cases worldwide in 2020. Despite the advance in clinical management, some of OSCC patients still miss the opportunity of completable resection of tumor, and have to accept medical therapies, e.g., chemotherapy, radiotherapy, or immunotherapy when the disease develops into the advanced stage. However, these therapies have been reported to be far from ideal due to the low efficiency of conventional delivery approaches. To obtain a better therapeutic effect, considerable attempts have been made toward to develop an effective drug delivery system (DDS). Nanoparticles (NPs) including inorganic NPs, polymer NPs, lipid NP, extracellular vesicles and cell membrane-based NPs have been evaluated as the better DDS candidates that can specifically accumulate in the tumor microenvironment along with a large amount of blood vessels. Emerging evidence suggested that NPs formulated with anticancer drugs including chemotherapeutic drugs, radiotherapy and immunotarget antibodies could remarkably improve the release and increase concentration of these drugs at the tumor site and show a better therapeutic efficacy, suggesting that NPs might serve as promising DDSs in the treatment of OSCC. Therefore, we have conducted this review to summarize recent progression and current status of diverse NPs as DDSs in this research field.
1 Introduction
Oral squamous cell carcinoma (OSCC) is the most common type of oral cancers, with an approximately 70% increase in incidence over the past 20 years (Gulland, 2016; Bugshan and Farooq, 2020). The estimated annual incidence of OSCC in 2020 was around 377,713 new cases worldwide, with Asia having the highest number of cases, followed by Europe and North America, according to statistical data obtained from 185 countries (Sung et al., 2021). The usual treatment for patients with early stage OSCC is surgery that can totally remove the tumors. The main clinical management for patients with advanced stage OCSS is diverse medical therapies i.e., radiotherapy, chemotherapy, immunotherapy, and combination therapy (Nandini et al., 2020; Deshmukh et al., 2021). Although these medical therapies have been optimized over the last several decades, however, they are still far from ideal therapeutic efficacy (Siegel et al., 2020; Surer et al., 2021) and the overall 5-year survival rate is less than 60% (Yu et al., 2019). Studies indicate that one of the main mechanisms responsible for such low therapeutic effect is the low efficacy of drug delivery system (DDS) that results in anticancer drugs being too low at the tumor site to exert their anticancer effects (Zhang et al., 2020a). Therefore, considerable attempts in the development of novel DDSs that can notably increase the concentration of drugs at the tumor site have been made (Ketabat et al., 2019). Recent studies have demonstrated that nanoparticles (NPs) as DDSs might reduce the toxicity and improve the safety and specificity of drugs, and represent as a promising tool for the treatment of OSCC (Calixto et al., 2014; Sah et al., 2018; Ketabat et al., 2019).
Theoretically, NPs usually refer to particles with a particle size between 1 and 100 nm. Extensive studies show that NPs can be specifically absorbed into the interstitial space of the tumor and are not rapidly cleared through enhanced permeability and retention (EPR) effects, that is a really stablished and important process and can induce in an enhanced accumulation of formulated anticancer drugs within the tumor microenvironment and result in a improve therapeutic effect (Nakamura et al., 2016). Currently, considerable NPs has been developed and evaluated, results showed an encouraged result in the treatment of OSCC.
CC. We have, therefore, summarized recent advances in the applications of major NPs as novel DDSs in the treatment of OSCC. These findings might help researchers and clinicians to better understand the effects of NPs in combining with anticancer medical therapies and to design novel therapies that significantly improve therapeutic response in patients with OSCC.
2 Different NPs as DDSs in enhancing the efficacy of anticancer drugs in OCSS
Modern nanotechnology is involved in various fields of clinical research and science, where such NPs-based DDSs are of major interest (Calixto et al., 2014). Several NPs have been developed as DDSs in the treatment of OSCC, which included inorganic NPs, extracellular vesicles, polymer NPs, and lipid NPs, cell membrane-based NPs and nanoenzymes (Calixto et al., 2014; Chen et al., 2023a; Mabrouk et al., 2023; Ortega et al., 2023). The improved therapeutic effect of NPs combined with anticancer agents has been demonstrated (De Felice et al., 2019). For example, Mukherjee et al. (2022) treated OSCC cell line (KB 3-1 cell) for 24 h with cetuximab (a epithelial growth factor receptor (EGFR) inhibitor) and NC in various doses. Comparing NC to cetuximab, they found a concentration-dependent cancer cell death that was remarkable. Therefore, NPs as promising DDS candidates have shown a great potential to increase cytotoxicity and overcome resistance to anticancer therapies, in the treatment of OSCC.
The following paragraphs will discuss the potential effect of NPs as effective DDSs individually.
2.1 Inorganic NPs
Inorganic NPs have been broadly used as DDSs in the treatment of tumors due to their unique physicochemical properties, e.g., facile preparation, excellent biocompatibility and wide surface conjugation chemistry (Wang et al., 2016), including various gold NPs, platinum NPs, iron NPs, manganese NPs, and silicon NPs, etc. For example, gold NPs have unique optical and surface plasmon resonance properties, making them particularly suitable for the ultra-sensitive detection and imaging-based therapeutic techniques needed to treat cancer (Singh et al., 2018). Due to the strong anticancer effect of platinum, platinum NPs also possess efficient anticancer ability despite behaving differently from platinum-containing compounds (Abed et al., 2022). Another commonly used inorganic NPs, iron oxide, is superparamagnetic at certain sizes and has been widely used as a contrast agent and drug delivery vehicle to treat cancer (Arias et al., 2018). A reduced toxicity, greater tolerance to organic solvents, and higher bioavailability than free drugs have been demonstrated in experiments (Ketabat et al., 2019). The characteristics of each inorganic nanoparticle are summarized in Table 1.
2.1.1 Gold NPs (AuNPs or GNPs)
Gold NPs, as DDS, have been extensively studied, and promising results were observed. For example, Abdel Hamid et al. (2021) used AuNS as a drug delivery vectors to evaluate the combination effect of cytotoxic chemo-drugs 5- fluorouracil (5-Fu), camptothecin (CPT), and small molecule inhibitor fibroblast growth factor receptor1-inhibitor (FGFR1i) both in vitro and in vivo in the oral cancer, survival analysis data showed that Syrian golden male hamsters treated with conjugating AuNSs with FGFR1i, 5Fu, and CPT could remarkedly enhance survival time to more than 27 days, which was much longer than animals treated with free FGFR1i (survival time 13.5 days). In addition, animals treated with FGFR1i-AuNSs induced the highest tumor volume reduction with a 2-fold decrease (−63.09%) compared with those injected by CPT-AuNSs (−32.1%) or 5Fu-AuNSs (−43.4%) (Abdel Hamid et al., 2021). When Gamal-Eldeen et al. (2021) examined the effect of gum arabic encapsulated gold NPsNPs (GA-AuNPs) on the hypoxia regulators in tongue squamous cell carcinoma (CAL-27 cells) in vitro, they found that GA-AuNPs can remarkably reduce cell viability with IC50 of 392.3 and 247.3 μg/mL after 24 and 48 h, respectively, and increase CAL-27 cell death rate via enhanced apoptosis. In addition, GA-AuNPs significantly inhibited hypoxia production in a dose-dependent manner, which could be via the decreased expression of hypoxia-regulating miRNAs (miR-210 and miR-21), hypoxia inducible factor-1 α (HIF-1α) and c-Myc (factors influence cell survival and angiogenesis) by GA-AuNPs (30% IC50, for 48 h) (Gamal-Eldeen et al., 2021). Park et al. (2021) investigated the effectiveness of a novel combination therapy by using gold nanoparticles (GNP) conjugated to anti programmed cell death protein ligand 1 (PD-L1) antibodies and nonthermal plasma (NTP) in PD-L1 expressing SCC-25 cells. They found that immunotarget anti- PD-L1 antibody and NTP-conjugated gold NPs could specifically bind to SCC-25 cells and induced an increase in the selective uptake of anti-PD-L1 antibody + GNP on SCC-25 cells, but significantly reduced tumor cell viability. In addition, the expression of apoptosis-related proteins and the number of dead cells were remarkably increased after treatment with anti-PD-L1 antibody + GNP + NTP. Thus, their results suggested a synergistic therapeutic effect than monotherapy.
In addition, some gold NPs can also increase the respond to environmental conditions such as light and heat. These features allowed gold NPs conjugated with radiotherapy, phototherapy, or photothermal therapy to potent their therapeutic effect. Indeed, Inanc Surer et al. (2021) used nanodrug complex containing cetuximab (CTX) and cisplatin (CDDP) conjugated with gold NPs to evaluate its therapeutic effects in both radioresistant oral cancer cell line (UPCI-SCC-131) and fibroblast cell line (NIH-3T3) in vitro. The results showed that the combination of nanodrugs GNP-CTX or CDDP with radiotherapy significantly result in 2-fold and 9-fold greater decrease in the colony number of radioresistant oral cancer cells than radiotherapy alone or free CTX combined with radiotherapy respectively (Surer et al., 2021). Furthermore, PEG-stabilized, PDPN antibody and doxorubicin (DOX)-conjugated gold NPs and Ph-stabilized DOX-AuNPs (DOX-NN-AuNPs) in combining with laser treatment could significantly increase apoptosis and decrease the growth of tumor cells (Liu et al., 2020; Essawy et al., 2021). The study by Chen et al. (2021) showed that gold nano-sesame beads (GNSbs) in combining with 2 Gy irradiation significantly increased the cytotoxic activity and decreased the proliferation activity of CAL-27 cells in a concentration-dependent manner, and the formation of reactive oxygen species (ROS) as compared with irradiation alone (Chen et al., 2021). In mice with CAL-27 orthotopically injected into the oral wall model, radiotherapy combining with GNSbs (delivered every 3 days for a total dose of 10 Gy (irradiation × 5 times) and 54 mg kg−1 GNSbs) treatment resulted in a significantly decrease in average tumor size from day 0 to day 21 (Chen et al., 2021). These results suggest that GNSbs is a promising radioenhancer that enhances the therapeutic effect of radiotherapy in OSCC.
2.1.2 Mesoporous silica NPs (MSNs)
Due to their distinguishing characteristics, such as pore volume, large specific surface area, controllable particle size, and great biocompatibility, MSNs as DDSs have attracted considerable attention and been intensively studied (Wang et al., 2015). Shi et al. (2019) reported that delivery of MTH1 inhibitor (TH287) and MDR1 siRNA via hyaluronic acid-based MSN in CAL-27 OSCC cells could induce more effective anticancer effects in vitro. In male Balb/c mice with subcutaneously injecting 2 × 106 CAL27 cells model, both SiTMSN and HA-siTMSN showed an antitumor effect. However, HA-siTMSN induced 4-fold decrease in the tumor volume compared to that of control and 2-fold compared to that of the siTMSN. indicating its stronger anti-oral cancer efficacy. By using the urokinase plasminogen activator receptor (uPAR)-targeting ligand AE105 decorated dendritic mesoporous silica nanoparticles (DMSN) encapsulating photonic active ultrasmall Cu2−xS NPs and sonosensitizer Rose Bengal (RB) (Cu2−xS-RB@DMSN-AE105, abbreviated as CRDA), Zuo et al. (2020) showed an increase of CRAD at tumor site and induced higher cytotoxicity, inhibit cell proliferation, reduce tumor cells by increasing apoptosis rate in OSCC cells. Furthermore, they further evaluated synergetic therapeutics of CRDAs against OSCC xenografts in BALB/c mice, their results showed that CRAD combined with photonic hyperthermal therapy (PTT) or sonodynamic therapy (SDT) in mice elicited enhanced therapeutic efficacy with an inhibition rate of 103.4%. The eradication of the xenografts in the CRDAs + US + laser group were enhanced, the survival rate was increased and living time of mice was prolonged (Zuo et al., 2020).
2.1.3 Other inorganic NPs
There are other inorganic NPs have been assessed. For example, both platinum nanocomposite (PtNCP) beads and superparamagnetic iron oxide NPs (SPION) were found to be cytotoxic to OSCC cells (Tanaka et al., 2019; Afrasiabi et al., 2021), Tanaka et al. (2019) reported that PtNCP could significantly inhibit tumor growth and caused more pathological necrosis area in subcutaneously xenografting mice with human squamous cell carcinoma cells, HSC-3-M3. They found that the mean tumor volumes in HSC-3-M3 cells xenografts in mice receiving PtNCP treatment for 14 days were significantly reduced than that in untreated group (PtNCP treated group vs. untreated group: 91.38 vs. 206.18 mm3). The inhibition rate on tumor volume in PtNCP treated group was 44.32% compared to untreated group (Tanaka et al., 2019). Histologically, necrotic areas in tumors were frequently observed in the PtNCP beads-treated group compared to the untreated group (Tanaka et al., 2019). Their findings suggest an inhibitory effect of PtNCP on OSCC.
The use of hollow mesoporous MnO2 (H-MnO2) nanoshells formulated with docetaxel and cisplatin and highly dispersed calcined hydroxyapatite NPs (nano-SHAP) loaded with zoledronic acid (ZA) were both shown to inhibit OSCC cell proliferation (Murata et al., 2018; Zhou et al., 2021). Murata et al. (Zhou et al., 2021) evaluated the effect of hydroxyapatite anoparticle as a new DDS on OSCC cell line, their results showed that Nano-SHAP with ZA suppressed remarkably OSCC tumor cell growth, damage tumor cells and upregulate apoptosis in OSCC in vivo.
By using dual-modal optical imaging rare earth nanoparticle (RENP) probes with peptide functionalization (RENP@C@Au (UCA)), Lin et al. (2021) demonstrated that it could effectively target Cal-27 tongue squamous cell carcinoma (TSCC) cells, enhance cell uptake of drug, inhibit invasion and metastasis, cause concentration-dependent apoptosis, and has an excellent in vivo clearance rate without causing significant organ damage.
In addition, photothermal Therapy has been shown to be a potential therapeutic approach in treating OSCC (Ran et al., 2023). Based on the properties of near-infrared (NIR) excitable upconversion NPs (UCN), photosensitizers such as photodynamic therapy (PDT) agents (Lucky et al., 2016), sulfur-doped carbon dots (S-CD) (Li et al., 2020a), nitrogen-rich mesoporous carbon Nanosphere void spaces trapping ultra-small nitrogen-doped carbon quantum dots (NCQDs) (Das et al., 2019) have been reported to show an enhanced therapeutic effect. Graphene quantum dots (GQDs) as the photosensitizer and GQD-polyethylene glycol (PEG) obtained by combining with PEG (Zhang et al., 2020b) have also been found to enhance the antitumor effect in the combination with different degrees of light in subcutaneously xenografting mice with SCC VII cells. They found that SCC VII tumor-bearing C3H mice treated with GQD-PEG plus irradiation induced tumor size reduction exceeding 70%, compared with control groups, they concluded that such antitumor effect might be attributable to the efficient tumor accumulations mediated by the EPR effect and the high 1O2 toxicity induced from photoactivity (Zhang et al., 2020b).
For the convenience of readers, the characteristics of these NPs are summarized in Table 1.
2.2 Extracellular vesicles (EVs)
EVs are natural nano-sized lipid bilayer vesicles released by all cell types and can be found in biological fluids such as blood, saliva, breast milk, cerebrospinal fluids and malignant ascites (Elsharkasy et al., 2020). Multiple studies have shown that EVs have a similar specific cellular tropism, which function as target vesicles for specific tissues and/or organs. Exosomes (EXO), nanoscale vesicles secreted by various cells, have the ability to cross biological barriers, including the most impenetrable blood-brain barrier (Batrakova and Kim, 2015; Wiklander et al., 2015) and attract a high attention (Wiklander et al., 2015).
Qiu et al. (2020) found that the loading and release of CTX from mesenchymal stromal cell (MSC)-derived EXO (MSC-EXO) significantly inhibited tumor growth by the activation of PI3K, Akt and mTOR and the induction of apoptosis in SCC-25 cells in a dose-dependent manner. They further showed that such inhibitory degree was in an effective synergistic and pharmacological effect. By developing a pH/light sensitive drug system based on milk-EXO for OSCC therapy, Zhang et al. (2020c found that a bovine milk EXO-based EXO-doxorubicin (DOX)-anthracene endoperoxide derivative (Exo@Dox-EPT1) could remarkably increase cellular uptake, release of DOX under an acidic microenvironment and generate ROS in combination with 808 nm NIR laser stimulation [31]. This new milk-EXO-based DDS exhibited an significant control effect on drug-release, biocompatibility and OSCC cell growth.
Apart from its inhibitory effect on OSCC cells, bitter melon-derived extracellular vesicles (BMEV) have been found to suppress the expression of NLRP3 and IL-1β and reduce the tumor resistance to 5-FU via an increase in apoptosis rate in OSCC cells (Yang et al., 2021). By using γδ T cell-derived extracellular vesicles (γδ TDE) loaded with miR-138, Li et al. (2019) showed that γδ TDE could directly inhibit OSCC tumor progression by up-regulating anti-tumor immunity through enhanced CD8 + T cells in pre-immunized immunocompetent C3H mice.
Regarding the role of EVs as DDSs in treating OSCC, we have summarized it in Table 2.
2.3 Polymer NPs
Polymer NPs, including natural polymers and semi-synthetic polymer NPs, possess the promoting potential to cellular permeability (Charbe et al., 2020; Xia et al., 2021). Among them, some polymer NPs with good biocompatibility and controlled drug release as targeted DDSs have been evaluated (Rizvi and Saleh, 2018).
Kurakula and Naveen (2020) loaded quercetin-doped chitosan-coated simvastatin (SIM) NPs in an in situ gel (ISG) (SIM-QRC NP-loaded ISGs) and demonstrated an delay of drug release, which resulted in a markedly increase in caspase-3-mediated apoptosis and tumor suppressor protein expression in tongue SSC cells. Enășescu et al. (2021) found that lutein and poly (d,l-lactide-co-glycolide) (PLGA) NPs potentially downregulated matrix metallopeptidase 9 level in human OSCC cells, which has been recognized as a protecting factor that could against local invasion in tumors (Chakraborty et al., 2023). Furthermore, both DOX-loaded catechol (Cat)-modified chitosan/hyaluronic acid (HA) NPs (Cat-NPs) and phloretin-loaded chitosan NPs (PhCsNPs) could increase the release of DOX, which significantly inhibited cancer cell growth and increased apoptosis rate in human oral cancer cells (Mariadoss et al., 2019; Pornpitchanarong et al., 2020). Endo et al. (2013) reported that commonly used chemotherapy drug cisplatin-carrying polymer micelles (NC-6004) showed an equivalent antitumor effect as free cisplatin in vivo, although the inhibitory effect on the growth of oral cancer cells was less than that of free cisplatin in vitro. The authors also found that the toxicity of NC-6004 to kidney and the incidence of lymphatic metastasis were lower than free cisplatin (Endo et al., 2013). Li et al. (2020b) found that polylactic acid (PLA) combined with CDDP-chloroquine (CQ) NPs (CDDP/CQ-PLA NPs) could induce a stronger activation of caspase-3 pathway and the induction of ROS than PLA combined with CDDP NPs (CDDP-PLA NPs), which leaded to an increased rate of caspase-dependent apoptosis and but lower rate of autophagy in OSCC (Li et al., 2020b).
Polymer NPs with targeting function have broad prospects as DDS. All-trans retinoic acid (ATRA) can be loaded onto PLGA-PEG and then modified with PD-L1 antibody to prepare ATRA- Targeted α-t- FU-PLGA NPs (Srivastava et al., 2019), glutathione (GSH)-sensitive and folic acid (FA)-targeted paclitaxel-loaded NPs (FA-PEG-SS-PCL@PTX, FA-NPs) (Fan et al., 2020a), cRGD targeted polycarbonate (PCA) copolymer-based NPs (NanoPCA) loaded with DOX (NanoPCA-cRGD) (Yunxia et al., 2018) have been proved to have a good targeting effect, which can precisely release and enhance antitumor efficacy of diverse anti-tumor drugs. To evaluate the potential effect of polymer NPs on immune checkpoint inhibitor in oral dysplasia and squamous carcinoma cells, Chen et al. (2020) examined CD8+ T cells surrounding PD-L1-positive cells in the tumor microenvironment. Both in vitro and in vivo results showed that CD8+ T cells were more activated after ATRA-PLGA-PEG-PD-L1 treatment (Chen et al., 2020).
Finally, polymer NPs combined with radiotherapy also exhibited an improved therapeutic effect on OSSC. Lang et al. (2020) reported that radiation combined with capivasertib-encapsulated cathepsin B (CTSB)-reactive NPs could significantly reduce tumor cell viability and increase the rate of apoptosis than radiation alone, or free capivasertib in OSCC cells, providing a novel strategy to improve therapeutic strategy for patients with radiation resistant.
The effects of polymer NPs as DDSs in the oral cancer were summarized in Tables 3, 4.
2.4 Lipid NPs
Lipid NPs are delivered into tumor tissue through the passively targeted EPR effects. To obtain an selective targeting efficacy, ligands can also be attached to the surface of lipid NPs. Lipid NPs are ideal carriers for drugs with low water solubility because of their ability to penetrate cancer cells and the high stability, allowing the controlled release of loaded drugs, and protecting drugs from chemical degradation (Coelho et al., 2010).
In the context of OSCC, Bharadwaj et al. (2019) used solid lipid NPs (SLN) loaded with paclitaxel (PTX), 5-FU and ascorbic acid individually to treat OSCC cells. They reported that the combination of SLN loaded with PTX and ascorbic acid respectively exhibited a better therapeutic efficacy in the treatment of OSCC in 4-NQO induced OSCC mouse model. After 2 weeks treatment, pathological analysis showed that dysplastic degree of tumor was decreased. Their results provided valuable insights for the design of novel combinational therapeutic strategies for the treatment of OSCC. Gusti-Ngurah-Putu et al. (2019) evaluated the therapeutic efficacy of PDT + lipid-platinum-chloride nanoparticles (LPC NPs) on a xenograft model of OSCC. Mice treated with PDT + LPC exhibited an significantly reduced tumor volume by up to ∼112% as compared with the control mice, histological analysis confirmed that proliferation index was decreased, but apoptosis rate increased in OSCC cells. In addition, side effects on renal damage was reduced (Gusti-Ngurah-Putu et al., 2019). Their results indicated that combined PDT with LPC NPs could significantly enhance the medicinal outcome in human OSCC.
3 Cell membrane-based NPs
Recently, studies revealed that cell membrane-based NPs are an emerging technique that uses cell membranes to directly coat the outermost layer of NPs (Pereira-Silva et al., 2020) and can maximize drug targeting by exploiting the inherited bio-functionalities of cell membranes (CM) extracted from source cells and might potentially enhance the effect of anticancer drugs (Pereira-Silva et al., 2020). Thus, CM-based NPs have received widespread attention in the field of cancer (Sushnitha et al., 2020).
Using this approach, Shi et al. (2020) prepared poly (β-amino ester)/PLGA nanoparticles co-loaded with indocyanine green and Nrf2-siRNA and then encapsulated them in vesicles derived from OSCC CM to form M@PPI-siRNA. They reported that photosensitizer indocyanine green (ICG) and Nrf2-siRNA encapsulated within the vesicles of cancer cell CM showed an synergistic anticancer effect of PTT and amplified PDT in oral tongue squamous cell carcinoma cells through an inhibitory effect on the proliferation and stimulatory effect on the apoptosis of oral tongue squamous cell carcinoma cells. Dai et al. (2022) developed the biomimetic nanomaterial PCN-CQ@CCM that could homologously adhere to cancer cells, enhancing the retention and uptake of nanomaterials in the tumor microenvironment. Their results showed that such biomimetic nanomaterial system could synergically potent the effect of PDT by inhibiting macrophage phagocytosis in oral cancer (Dai et al., 2022). Chen et al. (2023b) further synthetized an biomimetic nanomaterial system named cobalt-ferrocene metal–organic framework (Co-Fc) and loaded with the classical autophagy inhibitor hydroxychloroquine (HCQ) (Co-Fc@HCQ) nanoparticles and then constructed with CMM extracted from CAL-27 OSCC cells, which reduced immune escape and macrophage phagocytosis in OSCC cells. These findings indicate that CM may be used as an efficient synergist of PDT for OSCC treatment.
Taken together above reviewing data, we have summarized current findings in Figure 1.
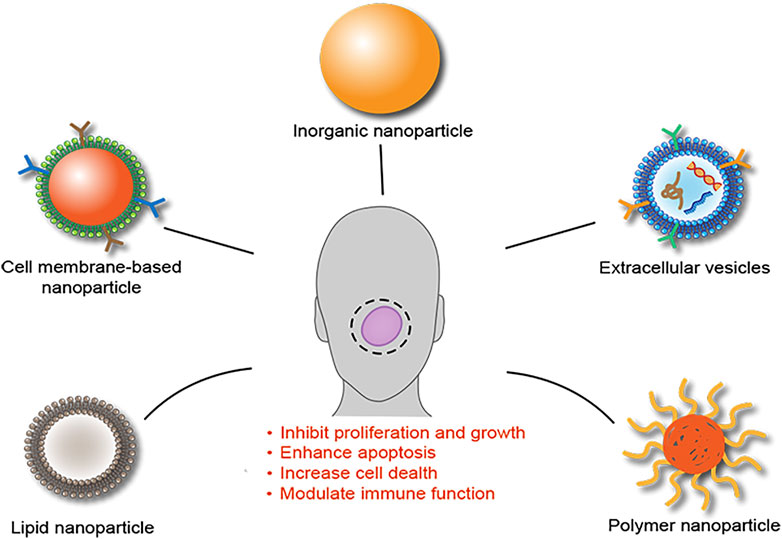
FIGURE 1. Schematic summary of NPs as DDSs for the treatment of OSCC. Analysis of current studies suggested that several NPs, including inorganic NPs, extracellular vesicles, polymer NPs, lipid NPs, and cell membrane-based NPs, have been developed as DDSs for the treatment of OSCC and showed an enhanced therapeutic efficacy through multiple mechanisms.
4 Current challenges of the strategies of NPs as DDSs in OSCC
Despite encouraging findings demonstrating their exciting potential of NPs as DDSs in the treatment of OSCC, however a number of challenges remain. For example, experimental models used in vivo and in vitro studies were different to human OSCC in the oral environment. Most of in vitro models used OSCC cell lines that derived from human OSCC and cultured in experimental media and treated with NPs combined with anticancer drugs, which is much simple than physiological oral condition. Even in vivo animal models, whether subcutaneous xenotransplanted mice or in situ oral tumor mice, face problems such as lack of normal-precancerous-cancerous process, which is different to the process of human OSCC (Montenegro et al., 2016). Thus, how to develop an experimental model that well mimics the human oral environment and can be used for the verification of in vivo animal and in vitro cellular findings become necessary. Various novel NPs-based DDSs have been extensively studied. However, in vivo bioavailability data was still waiting to be investigated (Sachdeva et al., 2022). Furthermore, studies on long-term toxicity and side effects of NPs in human body are needed to be conducted. Therefore, more detailed studies are required to evaluate biosafety of all types of NPs. Particularly, there is a lack of ongoing and completed clinical trials in patients with OSCC so far (De Felice et al., 2019), which is a necessary step for the definition of the potential oncology applications of NPs as DDSs in daily clinical practice in the treatment of OSCC in the future. Therefore, how to develop NPs as DDSs in a cheaper, easier, and faster way still remains a technical hurdle. Until these issues are resolved, clinical trials can be considered.
Unlike other human cancers, such as gastric, colorectal and lung cancers, OSCC is not rich in angiogenesis, which means that the level of chemotherapeutic drugs (such as paclitaxel and platinum-based drugs) that normally enter the circulation and reach the tumor site, by oral or intravenous administration, is limited (Bhat et al., 2021; Kitamura et al., 2021). In addition, the use of these free drugs is faced with poor therapeutic efficacy and severe side effects. Therefore, NPs-based therapies become particularly suitable for enhancing the bioavailability of chemotherapeutic drugs and target delivery to the tumor site, which can significantly improve the therapeutic efficacy and reduce side effects (De Felice et al., 2019). In addition, unlike tumors located deeply in the human body, such as colon cancer and liver cancer, OSCC is located at surface such as the buccal mucosa and tongue, which makes that OSCC is suitable for combinational treatment with NPs-mediated/enhanced PDT/PTT (Fan et al., 2020b). Also, hydrogels that can be applied to the surface of the skin or placed inside the surgical wound are options for the treatment of OSCC (Sepantafar et al., 2017).
5 Conclusion marks and perspectives
OSCC patients with advanced stage are often unresectable and metastatic, therapeutic approaches are commonly chemotherapy, radiotherapy, and biotherapy, which have various limitations and shortcomings and result in a decreased therapeutic response in clinical practice. For example, these conventional therapeutic approaches lack optimal anticancer effects, however, they may induce strong site effects e.g., non-specific cell toxicity that can significantly damage health cells during the treatment period and some patients have to cease therapies. Therefore, the improvement of these therapeutic efficacy become critically important. It is becoming evident that NPs can be used as DDSs and combined with different therapies to achieve an better therapeutic effect in patients with OSCC due to their ultra-small size, functional modification, and responsiveness to heat, light and other conditions as compared with conventional drug delivery approaches. Further work that verifies and confirms the improvement of clinical efficacy of different NPs as DDSs combining with anticancer drugs in treating patients with OSCC is necessary and important.
Author contributions
GC conceived the outline of the manuscript. SC and HL performed the electronic search for literatures and selection, data extraction and analysis. All authors contributed to the article and approved the submitted version.
Conflict of interest
The authors declare that the research was conducted in the absence of any commercial or financial relationships that could be construed as a potential conflict of interest.
Publisher’s note
All claims expressed in this article are solely those of the authors and do not necessarily represent those of their affiliated organizations, or those of the publisher, the editors and the reviewers. Any product that may be evaluated in this article, or claim that may be made by its manufacturer, is not guaranteed or endorsed by the publisher.
References
Abdel Hamid, H. M., Darwish, Z. E., Elsheikh, S. M., Mourad, G. M., Donia, H. M., and Afifi, M. M. (2021). Following cytotoxic nanoconjugates from injection to halting the cell cycle machinery and its therapeutic implications in oral cancer. BMC Cancer 21 (1), 170. doi:10.1186/s12885-021-07849-x
Abed, A., Derakhshan, M., Karimi, M., Shirazinia, M., Mahjoubin-Tehran, M., Homayonfal, M., et al. (2022). Platinum nanoparticles in biomedicine: Preparation, anti-cancer activity, and drug delivery vehicles. Front. Pharmacol. 13, 797804. doi:10.3389/fphar.2022.797804
Afrasiabi, M., Seydi, E., Rahimi, S., Tahmasebi, G., Jahanbani, J., and Pourahmad, J. (2021). The selective toxicity of superparamagnetic iron oxide nanoparticles (SPIONs) on oral squamous cell carcinoma (OSCC) by targeting their mitochondria. J. Biochem. Mol. Toxicol. 35 (6), 1–8. doi:10.1002/jbt.22769
Arias, L. S., Pessan, J. P., Vieira, A. P. M., Lima, T. M. T., Delbem, A. C. B., and Monteiro, D. R. (2018). Iron oxide nanoparticles for biomedical applications: A perspective on synthesis, drugs, antimicrobial activity, and toxicity. Antibiotics 7 (2), 46. doi:10.3390/antibiotics7020046
Batrakova, E. V., and Kim, M. S. (2015). Using exosomes, naturally-equipped nanocarriers, for drug delivery. J. Control Release 219, 396–405. doi:10.1016/j.jconrel.2015.07.030
Bharadwaj, R., Sahu, B. P., Haloi, J., Laloo, D., Barooah, P., Keppen, C., et al. (2019). Combinatorial therapeutic approach for treatment of oral squamous cell carcinoma. Artif. Cells Nanomed Biotechnol. 47 (1), 572–585. doi:10.1080/21691401.2019.1573176
Bhat, A. A., Yousuf, P., Wani, N. A., Rizwan, A., Chauhan, S. S., Siddiqi, M. A., et al. (2021). Tumor microenvironment: An evil nexus promoting aggressive head and neck squamous cell carcinoma and avenue for targeted therapy. Signal Transduct. Target. Ther. 6 (1), 12. doi:10.1038/s41392-020-00419-w
Bugshan, A., and Farooq, I. (2020). Oral squamous cell carcinoma: Metastasis, potentially associated malignant disorders, etiology and recent advancements in diagnosis. F1000Res. 9, 229. doi:10.12688/f1000research.22941.1
Calixto, G., Bernegossi, J., Fonseca-Santos, B., and Chorilli, M. (2014). Nanotechnology-based drug delivery systems for treatment of oral cancer: A review. Int. J. Nanomedicine 9, 3719–3735. doi:10.2147/IJN.S61670
Chakraborty, S., Suresh, T. N. R., and Mohiyuddin, A. S. (2023). Role of matrix metalloproteinase 9 in predicting lymph node metastases in oral squamous cell carcinoma. Cureus 15 (1), e33495. doi:10.7759/cureus.33495
Charbe, N. B., Amnerkar, N. D., Ramesh, B., Tambuwala, M. M., Bakshi, H. A., Aljabali, A. A. A., et al. (2020). Small interfering RNA for cancer treatment: Overcoming hurdles in delivery. Acta Pharm. Sin. B 10 (11), 2075–2109. doi:10.1016/j.apsb.2020.10.005
Chen, J., Zhu, Z., Pan, Q., Bai, Y., Yu, M., and Zhou, Y. (2023). Targeted therapy of oral squamous cell carcinoma with cancer cell membrane coated Co-fc nanoparticles via autophagy inhibition. Adv. Funct. Mater. 2023, 2300235. doi:10.1002/adfm.202300235
Chen, L., Kong, Q., Tian, M., Zhang, Q., Xia, C., and Deng, C. (2023). Zn(0.4)Mg(0.6)Fe(2)O(4) nanoenzyme: A novel chemo-sensitizer for the chemotherapy treatment of oral squamous cell carcinoma. Nanoscale Adv. 5 (3), 851–860. doi:10.1039/d2na00750a
Chen, M. H., Chen, M. H., Li, C. Y., Tung, F. I., Chen, S. Y., and Liu, T. Y. (2021). Using gold-nanorod-filled mesoporous silica nanobeads for enhanced radiotherapy of oral squamous carcinoma. Nanomaterials 11 (9), 2235. doi:10.3390/nano11092235
Chen, X. J., Zhang, X. Q., Tang, M. X., Liu, Q., and Zhou, G. (2020). Anti-PD-L1-modified and ATRA-loaded nanoparticles for immuno-treatment of oral dysplasia and oral squamous cell carcinoma. Nanomedicine (Lond) 15 (10), 951–968. doi:10.2217/nnm-2019-0397
Coelho, J. F., Ferreira, P. C., Alves, P., Cordeiro, R., Fonseca, A. C., Gois, J. R., et al. (2010). Drug delivery systems: Advanced technologies potentially applicable in personalized treatments. EPMA J. 1 (1), 164–209. doi:10.1007/s13167-010-0001-x
Dai, H., Yan, H., Dong, F., Zhang, L., Du, N., Sun, L., et al. (2022). Tumor-targeted biomimetic nanoplatform precisely integrates photodynamic therapy and autophagy inhibition for collaborative treatment of oral cancer. Biomater. Sci. 10 (6), 1456–1469. doi:10.1039/d1bm01780b
Das, R. K., Panda, S., Bhol, C. S., Bhutia, S. K., and Mohapatra, S. (2019). N-doped carbon quantum dot (NCQD)-Deposited carbon capsules for synergistic fluorescence imaging and photothermal therapy of oral cancer. Langmuir 35 (47), 15320–15329. doi:10.1021/acs.langmuir.9b03001
De Felice, F., Cavallini, C., Barlattani, A., Tombolini, M., Brugnoletti, O., Tombolini, V., et al. (2019). Nanotechnology in oral cavity carcinoma: Recent trends and treatment opportunities. Nanomater. (Basel) 9 (11), 1546. doi:10.3390/nano9111546
Deshmukh, V., and Shekar, K. (2021). “Oral squamous cell carcinoma: Diagnosis and treatment planning,” in Oral and maxillofacial surgery for the clinician. Editors K. Bonanthaya, E. Panneerselvam, S. Manuel, V. V. Kumar, and A. Rai (Singapore: Springer Nature Singapore), 1853–1867.
Elsharkasy, O. M., Nordin, J. Z., Hagey, D. W., de Jong, O. G., Schiffelers, R. M., Andaloussi, S. E., et al. (2020). Extracellular vesicles as drug delivery systems: Why and how?. Adv. Drug Deliv. Rev. 159, 332–343. doi:10.1016/j.addr.2020.04.004
Enășescu, D. A., Moisescu, M. G., Imre, M., Greabu, M., Ripszky Totan, A., Stanescu-Spinu, I., et al. (2021). Lutein treatment effects on the redox status and metalloproteinase-9 (MMP-9) in oral cancer squamous cells—are there therapeutical hopes?. Materials 14 (11), 2968. doi:10.3390/ma14112968
Endo, K., Ueno, T., Kondo, S., Wakisaka, N., Murono, S., Ito, M., et al. (2013). Tumor-targeted chemotherapy with the nanopolymer-based drug NC-6004 for oral squamous cell carcinoma. Cancer Sci. 104 (3), 369–374. doi:10.1111/cas.12079
Essawy, M. M., El-Sheikh, S. M., Raslan, H. S., Ramadan, H. S., Kang, B., Talaat, I. M., et al. (2021). Function of gold nanoparticles in oral cancer beyond drug delivery: Implications in cell apoptosis. Oral Dis. 27 (2), 251–265. doi:10.1111/odi.13551
Fan, H. Y., Zhu, Z. L., Zhang, W. L., Yin, Y. J., Tang, Y. L., Liang, X. H., et al. (2020). Light stimulus responsive nanomedicine in the treatment of oral squamous cell carcinoma. Eur. J. Med. Chem. 199, 112394. doi:10.1016/j.ejmech.2020.112394
Fan, L., Wang, J., Xia, C., Zhang, Q., Pu, Y., Chen, L., et al. (2020). Glutathione-sensitive and folate-targeted nanoparticles loaded with paclitaxel to enhance oral squamous cell carcinoma therapy. J. Mater Chem. B 8 (15), 3113–3122. doi:10.1039/c9tb02818h
Gamal-Eldeen, A. M., Baghdadi, H. M., Afifi, N. S., Ismail, E. M., Alsanie, W. F., Althobaiti, F., et al. (2021). Gum Arabic-encapsulated gold nanoparticles modulate hypoxamiRs expression in tongue squamous cell carcinoma. Mol. Cell. Toxicol. 17 (2), 111–121. doi:10.1007/s13273-021-00117-w
Gusti-Ngurah-Putu, E. P., Huang, L., and Hsu, Y. C. (2019). Effective combined photodynamic therapy with lipid platinum chloride nanoparticles therapies of oral squamous carcinoma tumor inhibition. J. Clin. Med. 8 (12), 2112. doi:10.3390/jcm8122112
Ketabat, F., Pundir, M., Mohabatpour, F., Lobanova, L., Koutsopoulos, S., Hadjiiski, L., et al. (2019). Controlled drug delivery systems for oral cancer treatment-current status and future perspectives. Pharmaceutics 11 (7), 302. doi:10.3390/pharmaceutics11070302
Kitamura, N., Sento, S., Yoshizawa, Y., Sasabe, E., Kudo, Y., and Yamamoto, T. (2021). Current trends and future prospects of molecular targeted therapy in head and neck squamous cell carcinoma. Int. J. Mol. Sci. 22 (1), 240. doi:10.3390/ijms22010240
Kurakula, M., and Naveen, N. R. (2020). In situ gel loaded with chitosan-coated simvastatin nanoparticles: Promising delivery for effective anti-proliferative activity against tongue carcinoma. Mar. Drugs 18 (4), 201. doi:10.3390/md18040201
Lang, L., Lam, T., Chen, A., Jensen, C., Duncan, L., Kong, F. C., et al. (2020). Circumventing AKT-associated radioresistance in oral cancer by novel nanoparticle-encapsulated capivasertib. Cells 9 (3), 533. doi:10.3390/cells9030533
Li, L., Lu, S., Liang, X., Cao, B., Wang, S., Jiang, J., et al. (2019). γδTDEs: An efficient delivery system for miR-138 with anti-tumoral and immunostimulatory roles on oral squamous cell carcinoma. Mol. Ther. Nucleic Acids 14, 101–113. doi:10.1016/j.omtn.2018.11.009
Li, Q., Liu, X., Yan, W., and Chen, Y. (2020). Antitumor effect of poly lactic acid nanoparticles loaded with cisplatin and chloroquine on the oral squamous cell carcinoma. Aging (Albany NY) 13 (2), 2593–2603. doi:10.18632/aging.202297
Li, Q., Zhou, R., Xie, Y., Li, Y., Chen, Y., and Cai, X. (2020). Sulphur-doped carbon dots as a highly efficient nano-photodynamic agent against oral squamous cell carcinoma. Cell Prolif. 53 (4), e12786. doi:10.1111/cpr.12786
Lin, B., Wu, J., Wang, Y., Sun, S., Yuan, Y., Tao, X., et al. (2021). Peptide functionalized upconversion/NIR II luminescent nanoparticles for targeted imaging and therapy of oral squamous cell carcinoma. Biomater. Sci. 9 (3), 1000–1007. doi:10.1039/d0bm01737j
Liu, Z., Shi, J., Zhu, B., and Xu, Q. (2020). Development of a multifunctional gold nanoplatform for combined chemo-photothermal therapy against oral cancer. Nanomedicine (Lond) 15 (7), 661–676. doi:10.2217/nnm-2019-0415
Lucky, S. S., Idris, N. M., Huang, K., Kim, J., Li, Z., Thong, P. S., et al. (2016). In vivo biocompatibility, biodistribution and therapeutic efficiency of titania coated upconversion nanoparticles for photodynamic therapy of solid oral cancers. Theranostics 6 (11), 1844–1865. doi:10.7150/thno.15088
Mabrouk, A. A., El-Mezayen, N. S., Tadros, M. I., El-Gazayerly, O. N., and El-Refaie, W. M. (2023). Novel mucoadhesive celecoxib-loaded cubosomal sponges: Anticancer potential and regulation of myeloid-derived suppressor cells in oral squamous cell carcinoma. Eur. J. Pharm. Biopharm. 182, 62–80. doi:10.1016/j.ejpb.2022.12.003
Mariadoss, A. V. A., Vinayagam, R., Senthilkumar, V., Paulpandi, M., Murugan, K., Xu, B., et al. (2019). Phloretin loaded chitosan nanoparticles augments the pH-dependent mitochondrial-mediated intrinsic apoptosis in human oral cancer cells. Int. J. Biol. Macromol. 130, 997–1008. doi:10.1016/j.ijbiomac.2019.03.031
Montenegro, M. F., Sundqvist, M. L., Nihlen, C., Hezel, M., Carlstrom, M., Weitzberg, E., et al. (2016). Profound differences between humans and rodents in the ability to concentrate salivary nitrate: Implications for translational research. Redox Biol. 10, 206–210. doi:10.1016/j.redox.2016.10.011
Mukherjee, D., Dash, P., Ramadass, B., and Mangaraj, M. (2022). Nanocurcumin in oral squamous cancer cells and its efficacy as a chemo-adjuvant. Cureus 14 (5), e24678. doi:10.7759/cureus.24678
Murata, T., Kutsuna, T., Kurohara, K., Shimizu, K., Tomeoku, A., and Arai, N. (2018). Evaluation of a new hydroxyapatite nanoparticle as a drug delivery system to oral squamous cell carcinoma cells. Anticancer Res. 38 (12), 6715–6720. doi:10.21873/anticanres.13040
Nakamura, Y., Mochida, A., Choyke, P. L., and Kobayashi, H. (2016). Nanodrug delivery: Is the enhanced permeability and retention effect sufficient for curing cancer?. Bioconjug Chem. 27 (10), 2225–2238. doi:10.1021/acs.bioconjchem.6b00437
Nandini, D. B., Rao, R. S., Hosmani, J., Khan, S., Patil, S., and Awan, K. H. (2020). Novel therapies in the management of oral cancer: An update. Dis. Mon. 66 (12), 101036. doi:10.1016/j.disamonth.2020.101036
Ortega, A., da Silva, A. B., da Costa, L. M., Zatta, K. C., Onzi, G. R., da Fonseca, F. N., et al. (2023). Thermosensitive and mucoadhesive hydrogel containing curcumin-loaded lipid-core nanocapsules coated with chitosan for the treatment of oral squamous cell carcinoma. Drug Deliv. Transl. Res. 13 (2), 642–657. doi:10.1007/s13346-022-01227-1
Park, J., Jang, Y.-S., Choi, J.-H., Ryu, M., Kim, G.-C., Byun, J. H., et al. (2021). Anticancer efficacy of nonthermal plasma therapy combined with PD-L1 antibody conjugated gold nanoparticles on oral squamous cell carcinoma. Appl. Sci. 11 (10), 4559. doi:10.3390/app11104559
Pereira-Silva, M., Santos, A. C., Conde, J., Hoskins, C., Concheiro, A., Alvarez-Lorenzo, C., et al. (2020). Biomimetic cancer cell membrane-coated nanosystems as next-generation cancer therapies. Expert Opin. Drug Deliv. 17 (11), 1515–1518. doi:10.1080/17425247.2020.1813109
Pornpitchanarong, C., Rojanarata, T., Opanasopit, P., Ngawhirunpat, T., and Patrojanasophon, P. (2020). Catechol-modified chitosan/hyaluronic acid nanoparticles as a new avenue for local delivery of doxorubicin to oral cancer cells. Colloids Surf. B Biointerfaces 196, 111279. doi:10.1016/j.colsurfb.2020.111279
Qiu, Y., Sun, J., Qiu, J., Chen, G., Wang, X., Mu, Y., et al. (2020). Antitumor activity of cabazitaxel and MSC-TRAIL derived extracellular vesicles in drug-resistant oral squamous cell carcinoma. Cancer Manag. Res. 12, 10809–10820. doi:10.2147/CMAR.S277324
Ran, J., Liu, T., Song, C., Wei, Z., Tang, C., Cao, Z., et al. (2023). Rhythm mild-temperature photothermal therapy enhancing immunogenic cell death response in oral squamous cell carcinoma. Adv. Healthc. Mater 12 (6), e2202360. doi:10.1002/adhm.202202360
Rizvi, S. A. A., and Saleh, A. M. (2018). Applications of nanoparticle systems in drug delivery technology. Saudi Pharm. J. 26 (1), 64–70. doi:10.1016/j.jsps.2017.10.012
Sachdeva, A., Dhawan, D., Jain, G. K., Yerer, M. B., Collignon, T. E., Tewari, D., et al. (2022). Novel strategies for the bioavailability augmentation and efficacy improvement of natural products in oral cancer. Cancers (Basel) 15 (1), 268. doi:10.3390/cancers15010268
Sah, A. K., Vyas, A., Suresh, P. K., and Gidwani, B. (2018). Application of nanocarrier-based drug delivery system in treatment of oral cancer. Artif. Cells Nanomed Biotechnol. 46 (4), 650–657. doi:10.1080/21691401.2017.1373284
Sepantafar, M., Maheronnaghsh, R., Mohammadi, H., Radmanesh, F., Hasani-sadrabadi, M. M., Ebrahimi, M., et al. (2017). Engineered hydrogels in cancer therapy and diagnosis. Trends Biotechnol. 35 (11), 1074–1087. doi:10.1016/j.tibtech.2017.06.015
Shi, S., Wang, Y., Wang, B., Chen, Q., Wan, G., Yang, X., et al. (2020). Homologous-targeting biomimetic nanoparticles for photothermal therapy and Nrf2-siRNA amplified photodynamic therapy against oral tongue squamous cell carcinoma. Chem. Eng. J. 388, 124268. doi:10.1016/j.cej.2020.124268
Shi, X. L., Li, Y., Zhao, L. M., Su, L. W., and Ding, G. (2019). Delivery of MTH1 inhibitor (TH287) and MDR1 siRNA via hyaluronic acid-based mesoporous silica nanoparticles for oral cancers treatment. Colloids Surf. B Biointerfaces 173, 599–606. doi:10.1016/j.colsurfb.2018.09.076
Siegel, R. L., Miller, K. D., and Jemal, A. (2020). Cancer statistics, 2020. CA Cancer J. Clin. 70 (1), 7–30. doi:10.3322/caac.21590
Singh, P., Pandit, S., Mokkapati, V. R. S. S., Garg, A., Ravikumar, V., and Mijakovic, I. (2018). Gold nanoparticles in diagnostics and therapeutics for human cancer. Int. J. Mol. Sci. 19 (7), 1979. doi:10.3390/ijms19071979
Srivastava, S., Gupta, S., Mohammad, S., and Ahmad, I. (2019). Development of alpha-tocopherol surface-modified targeted delivery of 5-fluorouracil-loaded poly-D, L-lactic-co-glycolic acid nanoparticles against oral squamous cell carcinoma. J. Cancer Res. Ther. 15 (3), 480–490. doi:10.4103/jcrt.JCRT_263_18
Sung, H., Ferlay, J., Siegel, R. L., Laversanne, M., Soerjomataram, I., Jemal, A., et al. (2021). Global cancer statistics 2020: GLOBOCAN estimates of incidence and mortality worldwide for 36 cancers in 185 countries. CA Cancer J. Clin. 71 (3), 209–249. doi:10.3322/caac.21660
Surer, S. I., Elcitepe, T. B., Akcay, D., Daskin, E., Calibasi Kocal, G., Arican Alicikus, Z., et al. (2021). A promising, novel radiosensitizer nanodrug complex for oral cavity cancer: Cetuximab and cisplatin-conjugated gold nanoparticles. Balk. Med. J. 38 (5), 278–286. doi:10.5152/balkanmedj.2021.21013
Sushnitha, M., Evangelopoulos, M., Tasciotti, E., and Taraballi, F. (2020). Cell membrane-based biomimetic nanoparticles and the immune system: Immunomodulatory interactions to therapeutic applications. Front. Bioeng. Biotechnol. 8, 627. doi:10.3389/fbioe.2020.00627
Tanaka, M., Okinaga, T., Iwanaga, K., Matsuo, K., Toyono, T., Sasaguri, M., et al. (2019). Anticancer effect of novel platinum nanocomposite beads on oral squamous cell carcinoma cells. J. Biomed. Mater Res. B Appl. Biomater. 107 (7), 2281–2287. doi:10.1002/jbm.b.34320
Wang, F., Li, C., Cheng, J., and Yuan, Z. (2016). Recent advances on inorganic nanoparticle-based cancer therapeutic agents. Int. J. Environ. Res. Public Health 13 (12), 1182. doi:10.3390/ijerph13121182
Wang, Y., Zhao, Q., Han, N., Bai, L., Li, J., Liu, J., et al. (2015). Mesoporous silica nanoparticles in drug delivery and biomedical applications. Nanomedicine 11 (2), 313–327. doi:10.1016/j.nano.2014.09.014
Wiklander, O. P., Nordin, J. Z., O'Loughlin, A., Gustafsson, Y., Corso, G., Mager, I., et al. (2015). Extracellular vesicle in vivo biodistribution is determined by cell source, route of administration and targeting. J. Extracell. Vesicles 4, 26316. doi:10.3402/jev.v4.26316
Xia, W., Tao, Z., Zhu, B., Zhang, W., Liu, C., Chen, S., et al. (2021). Targeted delivery of drugs and genes using polymer nanocarriers for cancer therapy. Int. J. Mol. Sci. 22 (17), 9118. doi:10.3390/ijms22179118
Yang, M., Luo, Q., Chen, X., and Chen, F. (2021). Bitter melon derived extracellular vesicles enhance the therapeutic effects and reduce the drug resistance of 5-fluorouracil on oral squamous cell carcinoma. J. Nanobiotechnology 19 (1), 259. doi:10.1186/s12951-021-00995-1
Yu, A. J., Choi, J. S., Swanson, M. S., Kokot, N. C., Brown, T. N., Yan, G., et al. (2019). Association of race/ethnicity, stage, and survival in oral cavity squamous cell carcinoma: A seer study. OTO Open 3 (4), 2473974X19891126. doi:10.1177/2473974X19891126
Yunxia, L., Wenjuan, D., Zhiguang, F., Huanhuan, W., Jiexin, W., Yuan, L., et al. (2018). pH-Responsive polycarbonate copolymer-based nanoparticles for targeted anticancer drug delivery. Chem. Res. Chin. Univ. 34, 1041–1050. doi:10.1007/s40242-018-8147-5
Zhang, M., Liang, J., Yang, Y., Liang, H., Jia, H., and Li, D. (2020). Current trends of targeted drug delivery for oral cancer therapy. Front. Bioeng. Biotechnol. 8, 618931. doi:10.3389/fbioe.2020.618931
Zhang, Q., Xiao, Q., Yin, H., Xia, X., Pu, Y., He, Z., et al. (2020). Milk-exosome based pH/light sensitive drug system to enhance anticancer activity against oral squamous cell carcinoma. RSC Adv. 1 (47), 28314–28323. doi:10.1039/d0ra05630h
Zhang, X., Li, H., Yi, C., Chen, G., Li, Y., Zhou, Y., et al. (2020). Host immune response triggered by graphene quantum-dot-mediated photodynamic therapy for oral squamous cell carcinoma. Int. J. Nanomedicine 15, 9627–9638. doi:10.2147/IJN.S276153
Zhou, Z.-H., Liang, S.-Y., Zhao, T.-C., Chen, X.-Z., Cao, X.-K., Qi, M., et al. (2021). Overcoming chemotherapy resistance using pH-sensitive hollow MnO2 nanoshells that target the hypoxic tumor microenvironment of metastasized oral squamous cell carcinoma. J. Nanobiotechnology 19 (1), 157. doi:10.1186/s12951-021-00901-9
Zuo, J., Huo, M., Wang, L., Li, J., Chen, Y., and Xiong, P. (2020). Photonic hyperthermal and sonodynamic nanotherapy targeting oral squamous cell carcinoma. J. Mater Chem. B 8, 9084–9093. doi:10.1039/d0tb01089h
Glossary
Keywords: nanoparticles, drug delivery system, oral squamous cell carcinoma, anticancer drug, treatment
Citation: Cui S, Liu H and Cui G (2023) Nanoparticles as drug delivery systems in the treatment of oral squamous cell carcinoma: current status and recent progression. Front. Pharmacol. 14:1176422. doi: 10.3389/fphar.2023.1176422
Received: 28 February 2023; Accepted: 15 May 2023;
Published: 24 May 2023.
Edited by:
Rovshan Khalilov, Baku State University, AzerbaijanReviewed by:
Miguel Pereira-Silva, University of Coimbra, PortugalMarina Santiago Franco, Technical University of Munich, Germany
Copyright © 2023 Cui, Liu and Cui. This is an open-access article distributed under the terms of the Creative Commons Attribution License (CC BY). The use, distribution or reproduction in other forums is permitted, provided the original author(s) and the copyright owner(s) are credited and that the original publication in this journal is cited, in accordance with accepted academic practice. No use, distribution or reproduction is permitted which does not comply with these terms.
*Correspondence: Guanglin Cui, Guanglin.cui@nord.no