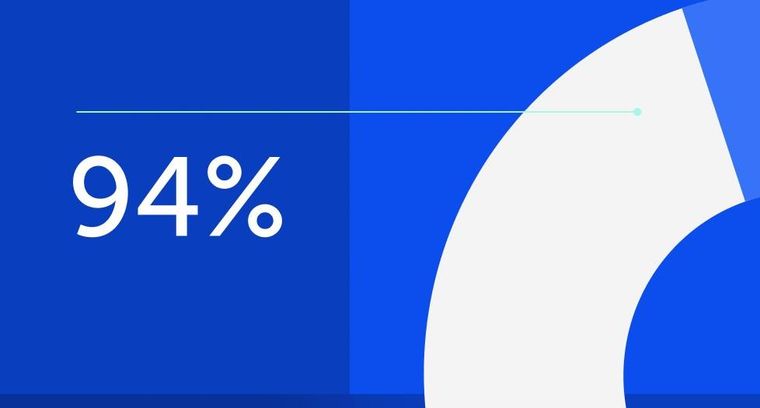
94% of researchers rate our articles as excellent or good
Learn more about the work of our research integrity team to safeguard the quality of each article we publish.
Find out more
REVIEW article
Front. Pharmacol., 26 April 2023
Sec. Ethnopharmacology
Volume 14 - 2023 | https://doi.org/10.3389/fphar.2023.1149708
This article is part of the Research TopicApplications of Herbal Medicine in Metabolic and Degenerative Diseases of AgingView all 7 articles
Ischemic retinal damage, a common condition associated with retinal vascular occlusion, glaucoma, diabetic retinopathy, and other eye diseases, threatens the vision of millions of people worldwide. It triggers excessive inflammation, oxidative stress, apoptosis, and vascular dysfunction, leading to the loss and death of retinal ganglion cells. Unfortunately, minority drugs are available for treating retinal ischemic injury diseases, and their safety are limited. Therefore, there is an urgent need to develop more effective treatments for ischemic retinal damage. Natural compounds have been reported to have antioxidant, anti-inflammatory, and antiapoptotic properties that can be used to treat ischemic retinal damage. In addition, many natural compounds have been shown to exhibit biological functions and pharmacological properties relevant to the treatment of cellular and tissue damage. This article reviews the neuroprotective mechanisms of natural compounds involve treating ischemic retinal injury. These natural compounds may serve as treatments for ischemia-induced retinal diseases.
Maintaining vision health is important in improving the quality of life of the elderly population (Song and Kim, 2021). The World Health Organization reported in the 2019 World Report on Vision that at least 2.2 billion people are either blind or visually impaired, of which at least 1 billion cases of blindness or visual impairment are preventable or have an unclear etiology (Tarik et al., 2019). In addition, the costs associated with eye diseases and visual impairments are unevenly distributed: The burden of responsibility is typically more considerable for older individuals, women, and rural residents in low- and middle-income countries. The Global Burden Study predicted that 834 million people will have some degree of visual impairment by 2050, with this trend increasing over time Study (GBaVICVLEGotGBoD, 2021). Retinal diseases have a significant impact on the preservation of vision, and the development of therapeutic interventions to delay retinal degeneration has been a focus of research in recent decades. Anti-vascular endothelial growth factor (VEGF) drugs are recognized in domestic and international guidelines as the first-line clinical treatment for retinal neovascularization caused by retinal ischemic vascular diseases (Baek et al., 2017). Whereas, long-term, repetitive vitreous cavity injection has side effects, and is inconvenient to administer for long-term treatment (Kim et al., 2019). Additionally, the costs of the treatment are expensive and it remains one of the aspects to take into consideration. Therefore, it is necessary to develop newer, safer, and more effective therapeutic strategies and agents to treat retinal diseases.
Optic nerve is a white-matter tract that contains ganglion cell axons and is also sensitive to the ischemic stimulation. Retinal ganglion cells (RGCs) have been identified as a class of neurons located in the retina and their axons travel through the retinal fiber layer into the optic nerve. RGCs are responsible for transmitting the visual information to the brain through visual pathways (Edo et al., 2020; Ziemba and Simoncelli, 2021). These fibres are essentially axons of the CNS (London et al., 2013). The retinal nerve fiber layer (RNFL) is the innermost layer of the retina and primarily consists of the axons of ganglion cell neurons, which are located below the RNFL (Jonas and Dichtl, 1996). Under ischemic conditions, an insufficient oxygen supply induces injury, apoptosis, and even loss of RGCs, eventually leading to retinal tissue damage (Khalilpour et al., 2017a; Pan et al., 2021). Studies have shown that long-term cerebral ischemic injury can cause retinal damage, affect vision (Xie et al., 2016). Therefore, ischemia-induced neuronal apoptosis has traditionally shown similarities and the presence of proper cellular communication in the blood–retina barrier (BRB) and blood–brain barrier (Madeira et al., 2015; Ramirez et al., 2018).
The clinical entities caused by retinal ischemia including retinal vein occlusion, retinal artery occlusion, diabetic retinopathy, and age-related macular degeneration result in the retinal neovascularization, which is the most common cause contributing to the exacerbation of ischemia-triggered retinal dysfunction (Osborne et al., 2004; Chang and Miller, 2005). Optic nerve diseases originated from chronic or acute ischemic stress such as glaucoma and ischemic optic neuropathy possess the similar pathophysiological disorders including the loss of retinal ganglion cells and their axons, and the damage of optic nerve. The classification of anterior or posterior ischemic optic neuropathy is based on the location of optic nerve damage, as per convention. The most common type of optic neuropathy is anterior ischemic optic neuropathy, which accounts for 90% of cases (Biousse and Newman, 2015; Tournaire-Marques, 2020). Insufficient vascular supply to the optic nerve is the most common cause contributing to the onset of ischemic optic neuropathy, which is featured by acute visual impairment in elder individuals with the age over 50. Research shows that ischemic optic neuropathy is the most common neurodegenerative disorder in older adults, with an estimated incidence of 2.3–10.2 cases per 100,000 people more than 50 years old (Hattenhauer et al., 1997; Preechawat et al., 2007; Tournaire-Marques, 2020). Retina ischemia is characterized by the reduction of retinal vascular flow, the deprivation of nutrients and oxygen as well as energy, and the accumulation of metabolic waste within the retina, which leads to severe lesion in the retina and even the optic nerve (Hattenhauer et al., 1997; Hayreh, 2011). As the extension of central nervous system, retina and optic nerve share the similar physiologic metabolisms as brain such as high oxygen-dependence and high-energy consumption, they therefore are more sensitive and venerable to the ischemic stress. Retinal ischemic injury causes energy-dependent dysfunction, tissue edema, and extensive, irreversible loss of neurons in the ganglion cell layer, which leads to retinal morphological degeneration, functional loss, and eventually, death of ganglion cells (Nagakubo et al., 2019; Lv et al., 2022). In addition, retinal cells are sensitive to ischemia, hypoxia, and energy metabolism. When ischemia persists for 60 min or more, ischemic damage often leads directly to vision loss or blindness, because RGCs are sensitive and fragile cells (Dilsiz et al., 2006; Liu et al., 2019; Qin et al., 2019). However, the extent of retinal tissue damage during ischemia depends on the severity and duration of the circulatory disturbance (Hughes, 1991; Khalilpour et al., 2017b).
Ischemia reduces blood flow, while oxygen depletion depletes substrates, such as oxygen and glucose. Energy depletion causes neuronal depolarization, which leads to the activation of glutamate receptors and increase in intracellular Ca2+ concentration (Dirnagl et al., 1999). In addition, the infiltration of proinflammatory elements leads to an increase in free radical generation and reactive oxygen species (ROS) formation, resulting in RGC death through apoptosis (Lo et al., 2003; Juybari et al., 2019).
Various herbal products derived from natural products are grown worldwide, especially in Middle Eastern, European, Asian countries with a history of exploring traditional medicine (Nagakubo et al., 2019; Lv et al., 2022). The therapeutic potential of natural herbal treatments for ischemic retinal damage has become increasingly evident in recent years. These medicines, including Scutellaria, lutein, and wolfberry, have antioxidant, anti-inflammatory, and anti-excitotoxic properties and can maintain the permeability of the retinal blood supply (Li et al., 2009; He et al., 2014; Pan et al., 2022). In addition, various natural extracts, such as resveratrol, Ginkgo biloba extract, and curcumin, have been shown to protect the CNS and exhibit neuroprotective properties (Yuan et al., 2014; Wang et al., 2017a; Luo et al., 2020). People consume flavonoids in fruits and vegetables daily and experience few side effects (Orhan et al., 2015). A growing number of researchers have found that natural extracts are effective in the prevention and treatment of ischemic diseases and their underlying mechanisms may be similar. These natural products can be classified as neuroprotective agents, that is, antioxidants, excitotoxicity inhibitors, antiapoptotic agents, neuropathic factors, and anti-inflammatory agents (Kang et al., 2010; Majid and Bin, 2011). The neuroprotective and pharmacological properties of these natural extracts provide a solid foundation for various models of ischemic injury. Given their widespread availability, low cost, and low toxicity, natural products may be effective treatments for ischemic diseases and neuropathy. This review will focus on the applications of natural compounds as neuroprotective agents in natural products. Table 1 summarizes the findings for several natural compounds discussed in this paper. (Note: some sequentially numbered references are in the table.)
Traditional Chinese medicine uses agent with antioxidant, anti-stimulant, anti-neuroinflammatory, antiapoptotic, anti-spasmodic, and anti-aging proportion. Modern pharmacological studies have shown that the active ingredients of natural extracts have preventive effects on neuropathy, inhibitory effects on apoptosis caused by ischemia and hypoxia, beneficial effects on cardiovascular health, and therapeutic effects on neurons in patients with ischemic optic neuropathy. Multiple ophthalmological studies show that conventional drugs can inhibit oxidative stress and inflammatory responses, with prevent apoptosis and RGC loss in retinal ischemia injury (Li et al., 2018a; Huang et al., 2020b; Rivera-Pérez et al., 2020). Natural products (Figure 1) are ideal candidates for treating retinal ischemia. Figure 1 summarizes the molecular structures of natural compounds, such as resveratrol, astaxanthin, icariin, and Lycium barbarum polysaccharides (LBPs). These compounds are ranked by the number of studies in which they have been investigated (Figure 2). Taken together, the literature demonstrates the feasibility of developing natural medicines for clinical use.
FIGURE 1. A wide range of natural products from plants and the structural formulae of natural chemical compounds.
FIGURE 2. The current number of research articles for each natural compound. Resveratrol: 6 studies; astaxanthin: 4 studies.
The inflammatory response is a regulatory process that prevents excessive damage to the body and represents a pathological provocative state resulting from the dysfunction of body-controlled mechanisms. Inflammation or ischemia can alter the levels of angiogenic factors released by damaged cells (Montezano et al., 2010). The acute inflammatory process begins at the microvascular site near the damaged tissue and induces the infiltration of leukocytes into the surrounding tissue (Woda and Hof, 2011). Research links inflammation to the development of neurodegenerative diseases, such as ischemic optic neuropathy (Guan et al., 2020).
Recent studies have shown that retinal ischemia–reperfusion (I/R) injury leads to an inflammatory response (Li et al., 2018b). Puerarin and sulforaphane have been reported to improve RGC injury caused by retinal I/R by inhibiting the activation of the toll-like receptor 4/nucleotide-binding oligomerization domains, leucine-rich repeats, and pyrin domain-containing 3 (NLRP3) inflammasome (Gong et al., 2019; Guan et al., 2020). The inhibitory activity of theissenolactone-C achieves retinal protection through extracellular regulated protein kinase (ERK)- and nuclear factor-kappa B (NF-κB)-dependent pathways. In addition, it has a strong inhibitory effect on matrix metalloproteinase 9 (MMP-9) activity after I/R injury induced by intraocular pressure (Lin et al., 2019). In another study, the protective mechanism of icariin was characterized by atypical activation of NF-κB induced by the CEBP-β/granulocyte colony-stimulating factor axis, providing long-term neuroprotective effects in patients with optic nerve ischemia through anti-inflammatory effects (Desai et al., 2022). Huang demonstrated that Epimedium exerts anti-inflammatory effects in a rodent anterior ischemic optic neuropathy (rAION) model, inhibits nuclear factor-kappa B kinase to phosphorylate phosphatase and alleviate tensin homolog and activate threonine protein kinase (Akt) in response to ischemic stress (Huang et al., 2020a). Further research found that butylens benzonitrile can effectively prevent the apoptosis induced by rAION and that the inhibition of NF-κB signaling can reduce the inflammatory response of blood-derived macrophages infiltrating the optic nerve, thereby alleviating neuroinflammation and facilitating the improvement of RGCs (Chou et al., 2022). The administration of Houttuynia cordata after retinal I/R injury has been found to inhibit the upregulation of TNF-α, inducible nitric oxide synthase (iNOS), and IL-1β in the treatment of ischemic events by activating microglia to promote the survival of RGCs (Ren and Zhang, 2020).
Scutellaria baicalensis is the main component of many traditional Chinese medicines, and its safety and efficacy in clinical applications have been demonstrated. Baicalin exerts potent anti-inflammatory effects by reducing the induction of proinflammatory cytokines and inhibiting PI3K/NF-κB phosphorylation (Pan et al., 2022). Oroxylin A is a flavonoid that regulates nuclear factor E2-related factor 2 (Nrf2) and its antioxidant enzymes NAD(P)H quinone oxidoreductase 1 and home oxidoreductase 1 (HO-1). Activating these enzymes reduces optic nerve swelling and inflammatory cell infiltration while modulating microglial polarization. Oroxylin A can significantly improve demyelination and optic nerve edema, reduce the number of ED1-positive cells, and effectively relieve ischemia (Huang et al., 2018; Chien et al., 2021), while 5α-Androst-3β-5α-6β-triol can negatively regulate Kelch-like ECH (enoyl-CoA hydratase)-related protein 1 and its downstream blood oxygenase 1 (Sheng et al., 2019). The primary mechanism of Prunus cerasus seed extract depends on HO-1 signaling, whereas echinacoside prevents I/R-induced retinal damage by activating antioxidant enzymes and inhibiting inflammation (Varga et al., 2017; Li et al., 2018a). Algae oil inhibits ERK activation to reduce iNOS, IL-1β, TNF-α, and Cl-caspase-3 levels and increase ciliary neurotrophic factor levels in rAION models (Huang et al., 2020b). The expression of caspase-3, caspase-8, superoxide dismutase (SOD) 2, and inflammation-related proteins and the phosphorylation of p38 have been reported to significantly increase in rats with ischemic injury, and green tea extract has been shown to increase the number of surviving RGCs (Yang et al., 2019). Moreover, capsaicin has NF-κB-mediated effects on ischemic injury by inhibiting the anti-inflammatory and retinal protective effects of CXCL10/CXCR3 (Wang et al., 2017b).
ROS-molecular oxygen (O2) reduction metabolites with high biological activities and excessive ROS levels are toxic to cells, eventually causing cell death (Sutherland et al., 2005). Echinacoside administration has been reported to improve retinal morphology, alleviate optic neuritis, and apoptosis, significantly reducing I/R-induced retinal oxidative stress after 7 days (Li et al., 2018a). Crocin is a pharmacologically active ingredient in turmeric that has been reported to significantly increase the level of glutathione (GSH) and SOD and decrease the formation of ROS and the activity of malondialdehyde (MDA) after ischemic retinal injury (Chen et al., 2015). Pomegranate acts as an antioxidant to attenuate retinal structural and functional I/R damage by activating Nrf2 (Hashem et al., 2017). Studies have shown that caffeic caffeic acid phenethyl ester attenuates I/R-induced apoptosis, significantly reduces the level of MDA in rat retinas, and increases the expression of SOD, GSH peroxidase, and catalase in the inner nuclear layer (INL) and RGC layer in rats (Shi et al., 2010).
According to the literature, mangiferin may have neuroprotective properties that could help prevent RGC loss due to oxidative stress. This prevention is achieved by decreasing the levels of hypoxia-inducible factor-1 alpha (HIF-1α) and glial fibrillary acidic protein and increasing the levels of silent mating information regulation 2 homolog 1 (SIRT1) in the retinas of ischemic mice (Kim et al., 2016). In contrast, the anti-RGC oxidation activity of baicalein appears to play a role in the downregulation of MMP-9 (Chao et al., 2013). In in vitro and in vivo studies, astaxanthin has prevented cell death via concentration-dependent inhibition of ROS, illustrating that astaxanthin inhibits retinal cell death through its antioxidant effects (Otsuka et al., 2016). Endoplasmic reticulum stress plays a crucial role in retinal vascular degeneration by upregulating eukaryotic initiation factor 2 alpha (eIF2α) homologous protein-CHOP and inositol requiring-enzyme 1α-X-box-binding protein 1 pathway, which leads to oxidative stress (Li et al., 2012). Studies have shown that lutein treatment can help reduce cell death and improve outcomes in the eyes subjected to I/R injury because of the ability of lutein to scavenge ROS, reduce inflammation, and protect against cell death signaling pathways. The neuroprotective effects of lutein are associated with reduced oxidative stress (Li et al., 2009).
The protective effects of LBPs and epigallocatechin-3-gallate against I/R injury also involve the activation of the Nrf2/HO-1 retinal antioxidant pathway (He et al., 2014; Rivera-Pérez et al., 2020). Betulinic acid derived from birch can increase the mRNA expression of antioxidant enzymes SOD3 and HO-1 after I/R and reduce the level of ROS (Musayeva et al., 2021). These findings demonstrate the vital role of natural compounds in combating oxidative stress.
The apoptotic process is triggered when defective mitochondria cannot be repaired or are removed by the quality control process and ROS reduction mechanisms of cells (Nagata, 2018). This apoptotic process may lead to irreversible loss of function when the retina is damaged (Remé et al., 2000). Many researchers are investigating therapeutic approaches to halting the process of irreversible vision loss by targeting and reducing the apoptotic activity caused by ischemic retinal lesions.
Astaxanthin, a natural product of synthesis of lutein carotenoids biosynthesized by various halogens, can inhibit the apoptosis of RGCs caused by I/R through the Nrf2/HO-1 pathway and relieve the symptoms of severe retinal diseases (Li et al., 2020). The antioxidant effects of vitexin are mediated by the activation of the Nrf2-related signaling pathway in retinal cells and the prevention of RGC apoptosis in RIR rats (Li et al., 2021b). As part of the same retinal I/R model, G. biloba extract has been shown to reduce RGC apoptosis (Wang and Ai, 2005; Yuan et al., 2014). Crocin has been observed to prevent rat retinal I/R-induced RGC apoptosis by activating the PI3K/Akt signaling pathway (Qi et al., 2013). A significant reduction in the number of apoptotic cells has been observed in the RGC layer of rat retina after oral administration of astaxanthin. A possible mechanism of the neuroprotective effect is to activate the Akt/mTOR signaling pathway to combat apoptosis and protect against free radical damage (Lin et al., 2018; Lin et al., 2020). Resveratrol acts as a potential neuroprotective agent. Mechanistically, resveratrol treatment significantly downregulates caspase-8 and caspase-3 expression, reduces SOD activity, and inhibits RGC apoptosis. In the mitochondria, resveratrol regulates the ratio of L-Opa1 to S-Opa1, and its protective effect may be mediated through the SIRT1-c-Jun N-terminal kinase (JNK)/SIRT1-Akt pathway (Seong et al., 2017; Wu et al., 2020a; Luo et al., 2020; Pang et al., 2020). Vincamine might protect rats from rAION by affecting the PI3K/Akt/eNOS signaling pathway (Li et al., 2021a). In another study, curcumin inhibited phosphorylated JNK activity in SHR after retinal I/R injury (Wang et al., 2017a). Baicalein prevents retinal ischemia by exerting antiapoptotic effects, upregulating HO-1, and downregulating HIF-1α and VEGF (Chao et al., 2013). Research has shown that leaf extract KIOM-2015E prevents RGC degeneration in posterior rats following MCAO-induced (I/R) models (Kim et al., 2020). Quercetin has been found to exhibit protective effects against I/R injury in the retina by inhibiting the apoptosis of cells in the INL, suggesting that other natural flavonoids, such as hesperetin and naringenin, may have similar protective effects (Kara et al., 2014; Arikan et al., 2015). Primary cultured RGCs treated with tetrandrine maintain the mitochondrial membrane potential and inhibit caspase-3 and Bcl-2 expression after I/R damage (Li et al., 2014). In retinal precursor cells (R28), gastrodin induces the PI3K, Akt, and Nrf2 signaling pathways protectively against oxygen and glucose deprivation/reoxygenation-induced injury (Li et al., 2022).
When administered orally, one dose of epigallocatechin gallate decreases retinal neuronal death and the apoptotic response to light in vitro. Puerarin and epigallocatechin gallate similarly protect against hypoxia-induced apoptosis in human retinal pigment epithelial cells (ARPE19) by activating the PI3K/Akt pathway (Zhang et al., 2008; Nguyen et al., 2019). These findings provide support for further investigation of natural compounds as novel protective agents against retinal ischemia.
During ischemic injury, the pathophysiological process of neuronal damage involves two excitatory amino acid transmitters: glutamate and aspartate. In excess, these neurotransmitters cause excitotoxicity, resulting in neuronal degeneration and a marked increase in extracellular concentrations of glutamate (Rothman and Olney, 1986; Choi and Rothman, 1990). The neuroprotective effect of the Chinese herbal medicine Honghua extract involves the inhibition of excitotoxicity (Romano et al., 1993). As another critical discovery, vinpocetine protects inner retinal neurons with functional NMDA glutamate receptors against retinal ischemia (Nivison-Smith et al., 2018).
Previous research has demonstrated that the administration of sulforaphane dramatically reduces the loss of retinal function caused by ischemia and induces pronounced thickening of the inner retinal layer (Ambrecht et al., 2014). Daily administration of LBP has been shown to effectively alleviate ischemia-induced retinal dysfunction and reduce associated neuronal death and glial cell activation (Yang et al., 2017). In mice injured by optic nerve crush, treatment with ethanolic extract of Rhus coriaria and linoleic acid yields anti-inflammatory effects (Khalilpour et al., 2018). Furthermore, research has shown that vinpocetine reduces intracellular cation channel permeability and apoptosis (Nivison-Smith et al., 2014; Nivison-Smith et al., 2017). In addition, emodin may protect neurons from ischemic retinal injury by downregulating β-catenin/VEGF protein expression during ischemia, while Allium cepa pretreatment protects I/R mice from retinal neuronal damage by regulating neurotrophic factors (Chao et al., 2020; Kumar et al., 2020). Interestingly, escin has been observed to have a synergistic protective effect against BRB disruption (Zhang et al., 2013). Finally, Dendrobium nobile Lindley can prevent retinal ischemic/hypoxic changes by downregulating the level of placental growth factor and upregulating the level of Norrie disease protein (Chao et al., 2018).
The significance of herbal medicine cannot be overstated in the betterment of patients afflicted with diverse illnesses. With supplementation of diet with herbal substances containing phytochemicals, the efficacy of conventional medication has been observed to increase. In the clinical treatment of retinal diseases, the primary means of assessing treatment outcomes include the evaluation of the best-corrected visual acuity (BCVA), central macular thickness (CMT), blood flow, macular pigment optical density (MPOD), and contrast sensitivity; optical coherence tomography; angiography; and electroretinogram (ERG).
Recently, phytochemicals have been shown to have the potential for beneficial effects on retinal diseases (Wimpissinger et al., 2007; Li et al., 2021c). A placebo-controlled randomized clinical trial evaluated the BCVA and CMT of patients before and after the intervention and every month thereafter for 3 months. It demonstrated a noteworthy distinction between log MAR changes in the 15-mg-crocin and placebo groups before and after the trial. Daily consumption of 15 mg of crocin proved effective in treating diabetic macular edema (DME), significantly decreasing the macular thickness and improving the BCVA (Yorgun, 2019). In another research, the oral administration of saffron significantly increased the amplitude of the fERGs of patients when compared with both baseline and placebo supplementation. In addition, the fERG thresholds were observed to decrease after saffron supplementation but not after placebo supplementation in comparison with the baseline values. These data indicate that consuming saffron supplements may lead to rapid and significant improvements in retinal function in individuals with early AMD (Falsini et al., 2010).
A study of patients undergoing curcumin therapy for 3 months showed that 84% of patients experienced an improvement in their visual acuity, and 92% exhibited a decrease in macular edema. These findings suggest that curcumin–phospholipid could be a beneficial treatment option for diabetic retinopathy (Mazzolani et al., 2018). Several clinical trials have shown that daily consumption of L. barbarum L. as a dietary supplement can help preserve the visual acuity and macular structure and increase the MPOD (Bucheli et al., 2011; Chan et al., 2019; Li et al., 2021c).
Clinical trials have revealed that several natural herbal compounds can dilate the blood vessels and augment blood flow. A randomized, double-blind, placebo-controlled study showed a significant increase in the macular square blur rate and choroidal blood flow velocity after 4 weeks of AXT ingestion compared with pre-ingestion values (Saito et al., 2012). Puerarin exhibits properties that can lower blood viscosity, enhance microcirculation, and effectively treat diabetic retinopathy (Ren et al., 2000). Oral consumption of antioxidants (i.e., G. biloba) for a month has been shown to increase ocular blood flow in the retinal and retrobulbar vascular beds in individuals with glaucoma (Wimpissinger et al., 2007; Harris et al., 2018).
Currently, the clinical management of ischemic retinal injury-related diseases involves the utilization of G. biloba leaves and compound lauryl injections. A clinical phase 3 trial (NCT02388984) among 480 participants is currently underway to assess the effectiveness and safety of Danshen dripping pills in treating diabetic retinopathy. The trial is double-blind and randomized. Meanwhile, another clinical trial (NCT04117022) is recruiting participants to evaluate the capacity of ERG to identify changes in global retinal function after treatment with carotenoid vitamin supplements in patients with diabetic retinopathy.
The primary limitation of clinical trials is the restricted sample size. This is attributed to various factors, such as differences in the treatment response and age of patients, execution criteria, and feedback authenticity. Additionally, discrepancies may arise in the judgment of investigators, inadequate follow-up time, and lack of placebo control. Furthermore, uncontrollable factors such as environmental and spatial factors may also affect the study outcomes. Therefore, it is crucial to consider all aspects of study design thoughtfully.
Although natural products are widely used for disease prevention and treatment, limited information is available regarding their mechanism of action and potential toxicity. The most frequently observed toxic side effect of natural products is hepatorenal toxicity, which can result in metabolic disturbances, electrolyte imbalances, acute kidney injury, chronic kidney disease, and even mortality.
Most present-day mechanistic exploratory investigations have been conducted to assess the toxicology, safety, and efficacy of the active components of natural products. Mechanistic studies or clinical trials of potent pharmacological active ingredients are frequently conducted within the safe dosage limits. Natural products generally exhibit a milder toxicity profile than do synthetic drugs. Clinical trials have revealed minimal adverse reactions, although one such trial has reported increased appetite, swollen feet, and stomach pain among patients who were administered saffron without any other notable adverse effects (Yorgun, 2019).
Most neurodegenerative diseases are accompanied by apoptosis, stress, and inflammatory response; ocular involvement is common and often leads to decreased vision or vision loss (McKee et al., 2011; Cordeiro et al., 2017). Many proteins are involved in cellular injury following ischemia and hypoxia, including HIF-1α, SIRT1, Nrf2, NF-κB, ERK, p38, PKC, and NLRP3 (Sun et al., 2001; Mishra et al., 2018; Hong et al., 2020; Parker et al., 2020; Song et al., 2020). The literature demonstrates that neuroprotective therapy is crucial from a strategic perspective.
Ischemic retinal injury is caused by several factors, including diabetic retinopathy, DME, glaucoma, AMD, ischemic optic neuropathy, and retinal I/R injury. Neurons undergo apoptosis when they do not receive adequate signals (Claes et al., 2019). Inflammatory cytokines, including TNFα and FasL, which is membrane-bound, can directly trigger apoptosis (Gregory et al., 2011; Roh et al., 2012). Low-grade inflammation leads to a sequence of cellular abnormalities and injury to the retina tissue because of the increased presence and significant involvement of proinflammatory mediators, adhesion molecules, chemokines, and growth factors in the development of ischemic retinal injury (Tang et al., 2023). When injury or stress occurs in the retina, inflammatory cells are activated, and harmful stimuli trigger endothelial cells and pericytes to secrete proinflammatory factors. In response to proinflammatory cytokine stimulation, endothelial cells produce intercellular adhesion molecules, attracting leukocyte adhesion to capillaries. Once attached, these leukocytes cause capillary blockage and disrupt the tight junctions between endothelial cells (Lutty, 2013). In vitro findings suggest that inflammatory cytokines may be the primary factor responsible for apoptosis (Doganay et al., 2002).
Oxidative stress has been studied as part of the pathogenesis of complex retinal diseases. During apoptosis, mitochondrial ROS cause oxidative stress, which has a role in apoptosis. Oxidative stress-inducing ROS are naturally produced through aerobic metabolism and can also be generated by external factors, such as ultraviolet light, smoke, and heavy metals (Fletcher, 2010). Fortunately, eukaryotic cells have established protective strategies to combat ROS by enhancing endogenous antioxidant production (Lo et al., 2006). Hypoxia, free radicals, and toxins can produce ROS and damage the cell membrane, resulting in DNA damage and apoptosis (Auguy et al., 2015). The primary source of ROS is mitochondrial electron transport chain complex I, which produces superoxide anion (O2−) (Sinha et al., 2015), which can be further oxidized by NOS, generating peroxynitrite (ONOO−). The lipid peroxidation products formed by peroxynitrite are 4-hydroxynonenal and MDA, which have been shown to induce neuronal apoptosis (Aoyama et al., 2012). Notably, researchers have discovered that flavonoids can prevent vision loss caused by ischemic retinal damage through apoptosis (Dua et al., 2015). This process involves multiple cell types, including neurons and glial cells. During this process, activated microglia produce several inflammatory mediators that stimulate inflammation, such as cytokines and chemokines. Many factors can trigger microglial activation, including physical injury, neurodegeneration, infection, or neuroinflammatory diseases, such as ischemic optic neuropathy (Airaksinen et al., 2008; Al Mamun et al., 2020). Microglia release inflammatory mediators associated with neurodegeneration, and the CNS expresses proinflammatory mediators. The IL-1β level is significantly increased in the optic nerve head tissue in patients with ischemic optic neuropathy (La Morgia et al., 2016). This increase in the IL-1β level may be related to the loss of RGCs or axons due to degenerative changes. Natural extracts have been shown to ameliorate inflammation-induced necrosis of RGCs, preventing the worsening of optic nerve degeneration (Wang et al., 2017a). In addition, glutamate, a neuroexcitotoxic substance, plays a significant role in the death of RGCs, resulting in reduced or impaired vision (Khalilpour et al., 2017b). Furthermore, studies have shown that natural products minimize the damage caused by excitotoxicity through their neuroprotective properties (Yang et al., 2019).
Ischemic retinal injury is the fundamental pathological mechanism for various ophthalmic ailments, including ischemic optic neuropathy, AMD, and I/R injury. Notably, although the findings of some clinical trials conducted with herbs have promising, they offer strategies for the clinical treatment of ischemic retinal diseases, with broad implications for reversing the visual function. Therefore, each strategy strives toward achieving a deeper comprehension of the etiology of retinal diseases and the evolution of potential treatments in their respective fields. This will help clinicians better understand the efficacy and full potential of herbs.
This review summarizes the mechanisms of ischemic retinal injury and the potential role of neuroprotective agents (Figure 3). Neuroprotection involves the slowing or blocking of processes leading to nerve cell death in neurodegenerative diseases. Natural compounds have the advantages of being widely available and inexpensive and having few side effects. In summary, considering the significant potential of natural compounds in treating retinal ischemic injury diseases, it is necessary to further develop and conduct in-depth research.
FIGURE 3. Mechanisms of the neuroprotective effects of natural compounds involved in retinal ischemic injury. Model: 1) intraocular hypertension, 2) laser induction with rose bengal, 3) vascular occlusion, and 4) optic nerve crush injury.
QH: writing–original draft, data charting, data collation. LX: data collation. YS: data collation. WL: data collation. XX: revising–original draft. All authors have read and agreed to the published version of the manuscript.
This work was supported by the National Natural Science Foundation of China, Grant/Award Number: No. 81460086. Sichuan Science and Technology Program (2021YJ0230); Thousand Talents Plan of Sichuan Province, Sichuan Provincial People’s Hospital, University of Electronic Science and Technology of China; Guoluo Zhi-Hui Engineering Project.
The authors declare that the research was conducted in the absence of any commercial or financial relationships that could be construed as a potential conflict of interest.
All claims expressed in this article are solely those of the authors and do not necessarily represent those of their affiliated organizations, or those of the publisher, the editors and the reviewers. Any product that may be evaluated in this article, or claim that may be made by its manufacturer, is not guaranteed or endorsed by the publisher.
Airaksinen, P. J., Doro, S., and Veijola, J. (2008). Conformal geometry of the retinal nerve fiber layer. Proc. Natl. Acad. Sci. U. S. A. 105 (50), 19690–19695. doi:10.1073/pnas.0801621105
Al Mamun, A., Chauhan, A., Qi, S., Ngwa, C., Xu, Y., Sharmeen, R., et al. (2020). Microglial IRF5-IRF4 regulatory axis regulates neuroinflammation after cerebral ischemia and impacts stroke outcomes. Proc. Natl. Acad. Sci. U. S. A. 117 (3), 1742–1752. doi:10.1073/pnas.1914742117
Ambrecht, L., McDonnell, J. F., Perlman, J. I., and Bu, P. (2014). Protected retinal function by sulforaphane on retinal ischemic injury. Investigative Ophthalmol. Vis. Sci. 55 (13), 1891. doi:10.1016/j.exer.2015.06.030
Aoyama, T., Paik, Y. H., Watanabe, S., Laleu, B., Gaggini, F., Fioraso-Cartier, L., et al. (2012). Nicotinamide adenine dinucleotide phosphate oxidase in experimental liver fibrosis: GKT137831 as a novel potential therapeutic agent. Hepatology 56 (6), 2316–2327. doi:10.1002/hep.25938
Arikan, S., Ersan, I., Karaca, T., Kara, S., Gencer, B., Karaboga, I., et al. (2015). Quercetin protects the retina by reducing apoptosis due to ischemia-reperfusion injury in a rat model. Arq. Bras. Oftalmol. 78 (2), 100–104. doi:10.5935/0004-2749.20150026
Auguy, F., Fahr, M., Moulin, P., El Mzibri, M., Smouni, A., Filali-Maltouf, A., et al. (2015). Transcriptome changes in Hirschfeldia incana in response to lead exposure. Front. Plant Sci. 6, 1231. doi:10.3389/fpls.2015.01231
Baek, A., Yoon, S., Kim, J., Baek, Y. M., Park, H., Lim, D., et al. (2017). Autophagy and KRT8/keratin 8 protect degeneration of retinal pigment epithelium under oxidative stress. Autophagy 13 (2), 248–263. doi:10.1080/15548627.2016.1256932
Biousse, V., and Newman, N. J. (2015). Ischemic optic neuropathies. N. Engl. J. Med. 372 (25), 2428–2436. doi:10.1056/NEJMra1413352
Bucheli, P., Vidal, K., Shen, L., Gu, Z., Zhang, C., Miller, L. E., et al. (2011). Goji berry effects on macular characteristics and plasma antioxidant levels. Optom. Vis. Sci. 88 (2), 257–262. doi:10.1097/OPX.0b013e318205a18f
Chan, H. H., Lam, H. I., Choi, K. Y., Li, S. Z., Lakshmanan, Y., Yu, W. Y., et al. (2019). Delay of cone degeneration in retinitis pigmentosa using a 12-month treatment with Lycium barbarum supplement. J. Ethnopharmacol. 236, 336–344. doi:10.1016/j.jep.2019.03.023
Chang, S. H., and Miller, N. R. (2005). The incidence of vision loss due to perioperative ischemic optic neuropathy associated with spine surgery: The johns hopkins hospital experience. Spine (Phila Pa 1976) 30, 1299–1302. doi:10.1097/01.brs.0000163884.11476.25
Chao, H. M., Chuang, M. J., Liu, J. H., Liu, X. Q., Ho, L. K., Pan, W. H., et al. (2013). Baicalein protects against retinal ischemia by antioxidation, antiapoptosis, downregulation of HIF-1α, VEGF, and MMP-9 and upregulation of HO-1. J. Ocul. Pharmacol. Ther. 29 (6), 539–549. doi:10.1089/jop.2012.0179
Chao, W. H., Lai, M. Y., Pan, H. T., Shiu, H. W., Chen, M. M., and Chao, H. M. (2018). Dendrobium nobile Lindley and its bibenzyl component moscatilin are able to protect retinal cells from ischemia/hypoxia by dowregulating placental growth factor and upregulating Norrie disease protein. BMC Complement. Altern. Med. 18 (1), 193. doi:10.1186/s12906-018-2256-z
Chao, H. W., Chen, Y. K., Liu, J. H., Pan, H. T., Lin, H. M., and Chao, H. M. (2020). Emodin protected against retinal ischemia insulted neurons through the downregulation of protein overexpression of β-catenin and vascular endothelium factor. BMC Complement. Med. Ther. 20 (1), 338. doi:10.1186/s12906-020-03136-7
Chen, L., Qi, Y., and Yang, X. (2015). Neuroprotective effects of crocin against oxidative stress induced by ischemia/reperfusion injury in rat retina. Ophthalmic Res. 54 (3), 157–168. doi:10.1159/000439026
Chien, J. Y., Lin, S. F., Chou, Y. Y., Huang, C. F., and Huang, S. P. (2021). Protective effects of oroxylin A on retinal ganglion cells in experimental model of anterior ischemic optic neuropathy. Antioxidants (Basel) 10 (6), 902. doi:10.3390/antiox10060902
Choi, D. W., and Rothman, S. M. (1990). The role of glutamate neurotoxicity in hypoxic-ischemic neuronal death. Annu. Rev. Neurosci. 13, 171–182. doi:10.1146/annurev.ne.13.030190.001131
Chou, Y. Y., Chien, J. Y., Ciou, J. W., and Huang, S. P. (2022). The protective effects of n-butylidenephthalide on retinal ganglion cells during ischemic injury. Int. J. Mol. Sci. 23 (4), 2095. doi:10.3390/ijms23042095
Claes, M., De Groef, L., and Moons, L. (2019). Target-derived neurotrophic factor deprivation puts retinal ganglion cells on death row: Cold hard evidence and caveats. Int. J. Mol. Sci. 20 (17), 4314. doi:10.3390/ijms20174314
Cordeiro, M. F., Normando, E. M., Cardoso, M. J., Miodragovic, S., Jeylani, S., Davis, B. M., et al. (2017). Real-time imaging of single neuronal cell apoptosis in patients with glaucoma. Brain 140 (6), 1757–1767. doi:10.1093/brain/awx088
Davey, P. G., Wang, Y., Gierhart, D. L., and Baudry, M. (2020). Neuroprotective effects of zeaxanthin in a mouse model of retinal ischemia/reperfusion injury. Investigative Ophthalmol. Vis. Sci. 61 (7), 655.
Desai, T. D., Wen, Y. T., Daddam, J. R., Cheng, F., Chen, C. C., Pan, C. L., et al. (2022). Long term therapeutic effects of icariin-loaded PLGA microspheres in an experimental model of optic nerve ischemia via modulation of CEBP-β/G-CSF/noncanonical NF-κB axis. Bioeng. Transl. Med. 7 (2), e10289. doi:10.1002/btm2.10289
Dilsiz, N., Sahaboglu, A., Yıldız, M. Z., and Reichenbach, A. (2006). Protective effects of various antioxidants during ischemia-reperfusion in the rat retina. Graefe's Archive Clin. Exp. Ophthalmol. 244 (5), 627–633. doi:10.1007/s00417-005-0084-6
Dirnagl, U., Iadecola, C., and Moskowitz, M. A. (1999). Pathobiology of ischaemic stroke: An integrated view. Trends Neurosci. 22 (9), 391–397. doi:10.1016/s0166-2236(99)01401-0
Doganay, S., Evereklioglu, C., Er, H., Türköz, Y., Sevinç, A., Mehmet, N., et al. (2002). Comparison of serum NO, TNF-alpha, IL-1beta, sIL-2R, IL-6 and IL-8 levels with grades of retinopathy in patients with diabetes mellitus. Eye (Lond) 16 (2), 163–170. doi:10.1038/sj.eye.6700095
Dua, T. K., Dewanjee, S., Gangopadhyay, M., Khanra, R., Zia-Ul-Haq, M., and De Feo, V. (2015). Ameliorative effect of water spinach, Ipomea aquatica (Convolvulaceae), against experimentally induced arsenic toxicity. J. Transl. Med. 13, 81. doi:10.1186/s12967-015-0430-3
Edo, A., Sugita, S., Futatsugi, Y., Sho, J., Onishi, A., Kiuchi, Y., et al. (2020). Capacity of retinal ganglion cells derived from human induced pluripotent stem cells to suppress T-Cells. Int. J. Mol. Sci. 21 (21), 7831.
Falsini, B., Piccardi, M., Minnella, A., Savastano, C., Capoluongo, E., Fadda, A., et al. (2010). Influence of saffron supplementation on retinal flicker sensitivity in early age-related macular degeneration. Invest. Ophthalmol. Vis. Sci. 51 (12), 6118–6124. doi:10.1167/iovs.09-4995
Fletcher, A. E. (2010). Free radicals, antioxidants and eye diseases: Evidence from epidemiological studies on cataract and age-related macular degeneration. Ophthalmic Res. 44 (3), 191–198. doi:10.1159/000316476
GBaVICVLEGotGBoD (2021). Trends in prevalence of blindness and distance and near vision impairment over 30 years: An analysis for the global burden of disease study. Lancet Glob. Health. 9 (2), e130–e143.
Gong, Y., Cao, X., Gong, L., and Li, W. (2019). Sulforaphane alleviates retinal ganglion cell death and inflammation by suppressing NLRP3 inflammasome activation in a rat model of retinal ischemia/reperfusion injury. Int. J. Immunopathol. Pharmacol. 33, 2058738419861777. doi:10.1177/2058738419861777
Gregory, M. S., Hackett, C. G., Abernathy, E. F., Lee, K. S., Saff, R. R., Hohlbaum, A. M., et al. (2011). Opposing roles for membrane bound and soluble Fas ligand in glaucoma-associated retinal ganglion cell death. PLoS One 6 (3), e17659. doi:10.1371/journal.pone.0017659
Guan, L., Li, C., Zhang, Y., Gong, J., Wang, G., Tian, P., et al. (2020). Puerarin ameliorates retinal ganglion cell damage induced by retinal ischemia/reperfusion through inhibiting the activation of TLR4/NLRP3 inflammasome. Life Sci. 256, 117935. doi:10.1016/j.lfs.2020.117935
Harris, A., Gross, J., Moore, N., Do, T., Huang, A., Gama, W., et al. (2018). The effects of antioxidants on ocular blood flow in patients with glaucoma. Acta Ophthalmol. 96 (2), e237–e241. doi:10.1111/aos.13530
Hashem, H. E., Abd El-Haleem, M. R., Amer, M. G., and Bor'i, A. (2017). Pomegranate protective effect on experimental ischemia/reperfusion retinal injury in rats (histological and biochemical study). Ultrastruct. Pathol. 41 (5), 346–357. doi:10.1080/01913123.2017.1346737
Hattenhauer, M. G., Leavitt, J. A., Hodge, D. O., Grill, R., and Gray, D. T. (1997). Incidence of nonarteritic anterior ischemic optic neuropathy. Am. J. Ophthalmol. 123 (1), 103–107. doi:10.1016/s0002-9394(14)70999-7
Hayreh, S. S. (2011). Management of ischemic optic neuropathies. Indian J. Ophthalmol. 59 (2), 123–136. doi:10.4103/0301-4738.77024
He, M., Pan, H., Chang, R. C., So, K. F., Brecha, N. C., and Pu, M. (2014). Activation of the Nrf2/HO-1 antioxidant pathway contributes to the protective effects of Lycium barbarum polysaccharides in the rodent retina after ischemia-reperfusion-induced damage. PLoS One 9 (1), e84800. doi:10.1371/journal.pone.0084800
Hong, J., Min, Y., Wuest, T., and Lin, P. C. (2020). Vav1 is essential for HIF-1α activation via a lysosomal VEGFR1-mediated degradation mechanism in endothelial cells. Cancers (Basel) 12 (6), 1374. doi:10.3390/cancers12061374
Huang, S-P., Chien, J-Y., Kapupara, K., Chou, Y-Y., and Tsai, R-K. (2018). Neuroprotective effects of oroxylin A in a rodent model of nonarteritic anterior ischemic optic neuropathy. Investigative Ophthalmol. Vis. Sci. 59 (9), 2499.
Huang, C-T., Desai, T., Cheng, F., Wen, Y-T., and Tsai, R-K. (2019). Neuroprotective effect of icariin in a rat model of anterior ischemic optic neuropathy. Investigative Ophthalmol. Vis. Sci. 60 (9), 4857.
Huang, C-T., Desai, T., Wen, Y-T., and Tsai, R-K. (2020a). The neuroprotective effects of icariin–loaded polylactic acid–glycolic acid copolymer (PLGA-icariin) in a rat model of anterior ischemic optic neuropathy (rAION). Investigative Ophthalmol. Vis. Sci. 61 (7), 2481.
Huang, T. L., Wen, Y. T., Ho, Y. C., Wang, J. K., Lin, K. H., and Tsai, R. K. (2020b). Algae oil treatment protects retinal ganglion cells (RGCs) via ERK signaling pathway in experimental optic nerve ischemia. Mar. Drugs 18 (2), 83. doi:10.3390/md18020083
Hughes, W. F. (1991). Quantitation of ischemic damage in the rat retina. Exp. Eye Res. 53 (5), 573–582. doi:10.1016/0014-4835(91)90215-z
Juybari, K. B., Hosseinzadeh, A., Ghaznavi, H., Kamali, M., Sedaghat, A., Mehrzadi, S., et al. (2019). Melatonin as a modulator of degenerative and regenerative signaling pathways in injured retinal ganglion cells. Curr. Pharm. Des. 25 (28), 3057–3073. doi:10.2174/1381612825666190829151314
Jonas, J. B., and Dichtl, A. (1996). Evaluation of the retinal nerve fiber layer. Surv. Ophthalmol. 40 (5), 369–378.
Kang, K. D., Majid, A. S., Kim, K. A., Kang, K., Ahn, H. R., Nho, C. W., et al. (2010). Sulbutiamine counteracts trophic factor deprivation induced apoptotic cell death in transformed retinal ganglion cells. Neurochem. Res. 35 (11), 1828–1839. doi:10.1007/s11064-010-0249-5
Kara, S., Gencer, B., Karaca, T., Tufan, H. A., Arikan, S., Ersan, I., et al. (2014). Protective effect of hesperetin and naringenin against apoptosis in ischemia/reperfusion-induced retinal injury in rats. ScientificWorldJournal 2014, 797824. doi:10.1155/2014/797824
Khalilpour, S., Latifi, S., Behnammanesh, G., Majid, A. M. S. A., Majid, A. S. A., and Tamayol, A. (2017). Ischemic optic neuropathy as a model of neurodegenerative disorder: A review of pathogenic mechanism of axonal degeneration and the role of neuroprotection. J. Neurological Sci. 375, 430–441. doi:10.1016/j.jns.2016.12.044
Khalilpour, S., Latifi, S., Behnammanesh, G., Majid, A., Majid, A. S. A., and Tamayol, A. (2017). Ischemic optic neuropathy as a model of neurodegenerative disorder: A review of pathogenic mechanism of axonal degeneration and the role of neuroprotection. J. Neurol. Sci. 375, 430–441. doi:10.1016/j.jns.2016.12.044
Khalilpour, S., Behnammanesh, G., Suede, F., Ezzat, M. O., Muniandy, J., Tabana, Y., et al. (2018). Neuroprotective and anti-inflammatory effects of Rhus coriaria extract in a mouse model of ischemic optic neuropathy. Biomedicines 6 (2), 48. doi:10.3390/biomedicines6020048
Kim, S. J., Sung, M. S., Heo, H., Lee, J. H., and Park, S. W. (2016). Mangiferin protects retinal ganglion cells in ischemic mouse retina via SIRT1. Curr. Eye Res. 41 (6), 844–855. doi:10.3109/02713683.2015.1050736
Kim, J., Park, J. R., Choi, J., Park, I., Hwang, Y., Bae, H., et al. (2019). Tie2 activation promotes choriocapillary regeneration for alleviating neovascular age-related macular degeneration. Sci. Adv. 5 (2), eaau6732. doi:10.1126/sciadv.aau6732
Kim, Y. H., Oh, T. W., Park, E., Yim, N. H., Cho, W. K., and Ma, J. Y. (2020). Neuroprotective effects of Acer palmatum thumb. leaf extract (KIOM-2015E) against ischemia/reperfusion-induced injury in the rat retina. Mol. Vis. 26, 691–704.
Kumar, S., Modgil, S., Bammidi, S., Minhas, G., Shri, R., Kaushik, S., et al. (2020). Allium cepa exerts neuroprotective effect on retinal ganglion cells of pterygopalatine artery (PPA) ligated mice. J. Ayurveda Integr. Med. 11 (4), 489–494. doi:10.1016/j.jaim.2019.08.002
La Morgia, C., Ross-Cisneros, F. N., Koronyo, Y., Hannibal, J., Gallassi, R., Cantalupo, G., et al. (2016). Melanopsin retinal ganglion cell loss in Alzheimer disease. Ann. Neurol. 79 (1), 90–109. doi:10.1002/ana.24548
Li, S. Y., Fu, Z. J., Ma, H., Jang, W. C., So, K. F., Wong, D., et al. (2009). Effect of lutein on retinal neurons and oxidative stress in a model of acute retinal ischemia/reperfusion. Invest. Ophthalmol. Vis. Sci. 50 (2), 836–843. doi:10.1167/iovs.08-2310
Li, C., Wang, L., Huang, K., and Zheng, L. (2012). Endoplasmic reticulum stress in retinal vascular degeneration: Protective role of resveratrol. Investigative Ophthalmol. Vis. Sci. 53 (6), 3241–3249. doi:10.1167/iovs.11-8406
Li, W., Yang, C., Lu, J., Huang, P., Barnstable, C. J., Zhang, C., et al. (2014). Tetrandrine protects mouse retinal ganglion cells from ischemic injury. Drug Des. Devel Ther. 8, 327–339. doi:10.2147/DDDT.S55407
Li, L., Wang, Y., Qin, X., Zhang, J., and Zhang, Z. (2018a). Echinacoside protects retinal ganglion cells from ischemia/reperfusion-induced injury in the rat retina. Mol. Vis. 24, 746–758.
Li, L., Xu, L., Chen, W., Li, X., Xia, Q., Zheng, L., et al. (2018b). Reduced annexin A1 secretion by ABCA1 causes retinal inflammation and ganglion cell apoptosis in a murine glaucoma model. Front. Cell. Neurosci. 12, 347. doi:10.3389/fncel.2018.00347
Li, Y., Wang, Q., Chu, C., and Liu, S. (2020). Astaxanthin protects retinal ganglion cells from acute glaucoma via the Nrf2/HO-1 pathway. J. Chem. Neuroanat. 110, 101876. doi:10.1016/j.jchemneu.2020.101876
Li, L., Su, Y., Liu, J., and Chen, C. (2021a). Efficacy of Vincamine treatment in a rat model of anterior ischemic optic neuropathy. Eur. J. Ophthalmol. 31 (6), 3442–3449. doi:10.1177/1120672120974283
Li, M. L., Fan, K., and Cui, H. P. (2021b). Protective effect of vitexin on retinal ganglion cells in rat retinal ischemia-reperfusion injury model. Chin. J. Exp. Ophthalmol. 39 (3), 7. doi:10.3760/cma.j.cn115989-20200509-00319
Li, X., Holt, R. R., Keen, C. L., Morse, L. S., Yiu, G., and Hackman, R. M. (2021). Goji berry intake increases macular pigment optical density in healthy adults: A randomized pilot trial. Nutrients 13 (12), 4409. doi:10.3390/nu13124409
Li, S., Yang, Q., Zhou, Z., Yang, X., Liu, Y., Hao, K., et al. (2022). Gastrodin protects retinal ganglion cells from ischemic injury by activating phosphatidylinositol 3-kinase/protein kinase B/nuclear factor erythroid 2-related factor 2 (PI3K/AKT/Nrf2) signaling pathway. Bioengineered 13 (5), 12625–12636. doi:10.1080/21655979.2022.2076499
Lin, W-N., Wen, Y-T., and Tsai, R-K. (2018). Neuroprotective effect of astaxanthin in a rat model of anterior ischemic optic neuropathy. Investigative Ophthalmol. Vis. Sci. 59 (9), 2498.
Lin, F-L., Cheng, Y-W., Yu, M., Ho, J-D., Kuo, Y-C., Chiou, G. C. Y., et al. (2019). The fungus-derived retinoprotectant theissenolactone C improves glaucoma-like injury mediated by MMP-9 inhibition. Phytomedicine 56, 207–214. doi:10.1016/j.phymed.2018.11.002
Lin, W. N., Kapupara, K., Wen, Y. T., Chen, Y. H., Pan, I. H., and Tsai, R. K. (2020). Haematococcus pluvialis-derived astaxanthin is a potential neuroprotective agent against optic nerve ischemia. Mar. Drugs 18 (2), 85. doi:10.3390/md18020085
Liu, J. L., Wang, C. X., Ning, Y., Sun, Q., and Zhang, J. S. (2013). Effects of emodin on the retinal ganglion cells after ischemia reperfusion injury in rats. J. China Med. Univ. 42 (1), 4–7.
Liu, W., Xia, F., Ha, Y., Zhu, S., Li, Y., Folorunso, O., et al. (2019). Neuroprotective effects of HSF1 in retinal ischemia-reperfusion injury. Invest. Ophthalmol. Vis. Sci. 60 (4), 965–977. doi:10.1167/iovs.18-26216
Lo, E. H., Dalkara, T., and Moskowitz, M. A. (2003). Mechanisms, challenges and opportunities in stroke. Nat. Rev. Neurosci. 4 (5), 399–415. doi:10.1038/nrn1106
Lo, S. C., Li, X., Henzl, M. T., Beamer, L. J., and Hannink, M. (2006). Structure of the Keap1:Nrf2 interface provides mechanistic insight into Nrf2 signaling. EMBO J. 25 (15), 3605–3617. doi:10.1038/sj.emboj.7601243
London, A., Benhar, I., and Schwartz, M. (2013). The retina as a window to the brain-from eye research to CNS disorders. Nat. Rev. Neurol. 9 (1), 44–53. doi:10.1038/nrneurol.2012.227
Luo, J., He, T., Yang, J., Yang, N., Li, Z., and Xing, Y. (2020). SIRT1 is required for the neuroprotection of resveratrol on retinal ganglion cells after retinal ischemia-reperfusion injury in mice. Graefes Arch. Clin. Exp. Ophthalmol. 258 (2), 335–344. doi:10.1007/s00417-019-04580-z
Lutty, G. A. (2013). Effects of diabetes on the eye. Invest. Ophthalmol. Vis. Sci. 54 (14), ORSF81–87. doi:10.1167/iovs.13-12979
Lv, D., Xu, J., Qi, M., Wang, D., Xu, W., Qiu, L., et al. (2022). A strategy of screening and binding analysis of bioactive components from traditional Chinese medicine based on surface plasmon resonance biosensor. J. Pharm. Anal. 12 (3), 500–508.
Madeira, M. H., Ambrósio, A. F., and Santiago, A. R. (2015). Glia-mediated retinal neuroinflammation as a biomarker in alzheimer's disease. Ophthalmic Res. 54 (4), 204–211. doi:10.1159/000440887
Majid, A., and Bin, A. S. (2011). The influence of selected sulphur containing compounds on retinal cell death: Neuroprotective effects of hydrogen sulphide in a glaucoma model. Oxford: University of Oxford.
Mazzolani, F., Togni, S., Giacomelli, L., Eggenhoffner, R., and Franceschi, F. (2018). Oral administration of a curcumin-phospholipid formulation (Meriva®) for treatment of chronic diabetic macular edema: A pilot study. Eur. Rev. Med. Pharmacol. Sci. 22 (11), 3617–3625. doi:10.26355/eurrev_201806_15189
McKee, C. T., Wood, J. A., Shah, N. M., Fischer, M. E., Reilly, C. M., Murphy, C. J., et al. (2011). The effect of biophysical attributes of the ocular trabecular meshwork associated with glaucoma on the cell response to therapeutic agents. Biomaterials 32 (9), 2417–2423. doi:10.1016/j.biomaterials.2010.11.071
Mishra, M., Duraisamy, A. J., and Kowluru, R. A. (2018). Sirt1: A guardian of the development of diabetic retinopathy. Diabetes 67 (4), 745–754. doi:10.2337/db17-0996
Montezano, A. C., Burger, D., Paravicini, T. M., Chignalia, A. Z., Yusuf, H., Almasri, M., et al. (2010). Nicotinamide adenine dinucleotide phosphate reduced oxidase 5 (Nox5) regulation by angiotensin II and endothelin-1 is mediated via calcium/calmodulin-dependent, rac-1-independent pathways in human endothelial cells. Circ. Res. 106 (8), 1363–1373. doi:10.1161/CIRCRESAHA.109.216036
Musayeva, A., Unkrig, J. C., Zhutdieva, M. B., Manicam, C., Ruan, Y., Laspas, P., et al. (2021). Betulinic acid protects from ischemia-reperfusion injury in the mouse retina. Cells 10 (9), 2440. doi:10.3390/cells10092440
Nagakubo, T., Kumano, T., Ohta, T., Hashimoto, Y., and Kobayashi, M. (2019). Copper amine oxidases catalyze the oxidative deamination and hydrolysis of cyclic imines. Nat. Commun. 10 (1), 413.
Nagata, S. (2018). Apoptosis and clearance of apoptotic cells. Annu. Rev. Immunol. 36, 489–517. doi:10.1146/annurev-immunol-042617-053010
Nguyen, N., Le, M-A., Wen, Y-T., and Tsai, R-K. (2019). Puerarin protects retinal pigment epithelium (ARPE19) against hypoxia-induced apoptosis through activation of the PI3/Akt pathway. Investigative Ophthalmol. Vis. Sci. 60 (9), 5706.
Nivison-Smith, L., Acosta, M. L., Misra, S., O’Brien, B. J., and Kalloniatis, M. (2014). Vinpocetine regulates cation channel permeability of inner retinal neurons in the ischaemic retina. Neurochem. Int. 66, 1–14. doi:10.1016/j.neuint.2014.01.003
Nivison-Smith, L., Khoo, P., Acosta, M. L., and Kalloniatis, M. (2017). Pre-treatment with vinpocetine protects against retinal ischemia. Exp. Eye Res. 154, 126–138. doi:10.1016/j.exer.2016.11.018
Nivison-Smith, L., Khoo, P., Acosta, M. L., and Kalloniatis, M. (2018). Vinpocetine protects inner retinal neurons with functional NMDA glutamate receptors against retinal ischemia. Exp. Eye Res. 167, 1–13. doi:10.1016/j.exer.2017.10.008
Orhan, I. E., Daglia, M., Nabavi, S. F., Loizzo, M. R., Sobarzo-Sánchez, E., and Nabavi, S. M. (2015). Flavonoids and dementia: An update. Curr. Med. Chem. 22 (8), 1004–1015. doi:10.2174/0929867322666141212122352
Osborne, N. N., Casson, R. J., Wood, J. P. M., Chidlow, G., Graham, M., and Melena, J. (2004). Retinal ischemia: Mechanisms of damage and potential therapeutic strategies. Prog. Retin. Eye Res. 23 (1), 91–147. doi:10.1016/j.preteyeres.2003.12.001
Otsuka, T., Shimazawa, M., Inoue, Y., Nakano, Y., Ojino, K., Izawa, H., et al. (2016). Astaxanthin protects against retinal damage: Evidence from in vivo and in vitro retinal ischemia and reperfusion models. Curr. Eye Res. 41 (11), 1465–1472. doi:10.3109/02713683.2015.1127392
Pan, H., He, M., Liu, R., Brecha, N. C., Yu, A. C., and Pu, M. (2014). Sulforaphane protects rodent retinas against ischemia-reperfusion injury through the activation of the Nrf2/HO-1 antioxidant pathway. PLoS One 9 (12), e114186. doi:10.1371/journal.pone.0114186
Pan, L., Cho, K-S., Yi, I., To, C-H., Chen, D. F., and Do, C-W. (2021). Baicalein, baicalin, and wogonin: Protective effects against ischemia-induced neurodegeneration in the brain and retina. Oxidative Med. Cell. Longev. 2021, 8377362. doi:10.1155/2021/8377362
Pan, L., Sze, Y. H., Yang, M., Tang, J., Zhao, S., Yi, I., et al. (2022). Baicalein-A potent pro-homeostatic regulator of microglia in retinal ischemic injury. Front. Immunol. 13, 837497. doi:10.3389/fimmu.2022.837497
Pang, Y., Qin, M., Hu, P., Ji, K., Xiao, R., Sun, N., et al. (2020). Resveratrol protects retinal ganglion cells against ischemia induced damage by increasing Opa1 expression. Int. J. Mol. Med. 46 (5), 1707–1720. doi:10.3892/ijmm.2020.4711
Parker, S. J., Amendola, C. R., Hollinshead, K. E. R., Yu, Q., Yamamoto, K., Encarnación-Rosado, J., et al. (2020). Selective alanine transporter utilization creates a targetable metabolic niche in pancreatic cancer. Cancer Discov. 10 (7), 1018–1037. doi:10.1158/2159-8290.CD-19-0959
Preechawat, P., Bruce, B. B., Newman, N. J., and Biousse, V. (2007). Anterior ischemic optic neuropathy in patients younger than 50 years. Am. J. Ophthalmol. 144 (6), 953–960. doi:10.1016/j.ajo.2007.07.031
Qi, Y., Chen, L., Zhang, L., Liu, W-B., Chen, X-Y., and Yang, X-G. (2013). Crocin prevents retinal ischaemia/reperfusion injury-induced apoptosis in retinal ganglion cells through the PI3K/AKT signalling pathway. Exp. Eye Res. 107, 44–51. doi:10.1016/j.exer.2012.11.011
Qin, X., Li, N., Zhang, M., Lin, S., Zhu, J., Xiao, D., et al. (2019). Tetrahedral framework nucleic acids prevent retina ischemia-reperfusion injury from oxidative stress via activating the Akt/Nrf2 pathway. Nanoscale 11 (43), 20667–20675. doi:10.1039/c9nr07171g
Ramirez, J. M. R., Garcia, E. S., Hoz, R. D., Pancorbo, R. Y., Ines, L-C., and Gregorio, P. G. (2018). Evaluación de los cambios precoces de la retina en la enfermedad de Alzheimer y contribución de la papila en la progresión de la enfermedad - ScienceDirect. Rev. Española Geriatría Gerontol. 53, 3. doi:10.1016/j.regg.2018.04.007
Remé, C. E., Grimm, C., Hafezi, F., Wenzel, A., and Williams, T. P. (2000). Apoptosis in the retina: The silent death of vision. News Physiol. Sci. 15, 120–124. doi:10.1152/physiologyonline.2000.15.3.120
Ren, L. M., and Zhang, Y. H. (2020). Houttuynia cordata Thunb rescues retinal ganglion cells through inhibiting microglia activation in a rat model of retinal ischemia-reperfusion. Int. J. Ophthalmol. 13 (12), 1880–1886. doi:10.18240/ijo.2020.12.06
Ren, P., Hu, H., and Zhang, R. (2000). [Observation on efficacy of puerarin in treating diabetic retinopathy]. Zhongguo Zhong Xi Yi Jie He Za Zhi 20 (8), 574–576.
Rivera-Pérez, J., Martínez-Rosas, M., Conde-Castañón, C. A., Toscano-Garibay, J. D., Ruiz-Pérez, N. J., Flores, P. L., et al. (2020). Epigallocatechin 3-gallate has a neuroprotective effect in retinas of rabbits with ischemia/reperfusion through the activation of Nrf2/HO-1. Int. J. Mol. Sci. 21 (10), 3716. doi:10.3390/ijms21103716
Roh, M., Zhang, Y., Murakami, Y., Thanos, A., Lee, S. C., Vavvas, D. G., et al. (2012). Etanercept, a widely used inhibitor of tumor necrosis factor-α (TNF-α), prevents retinal ganglion cell loss in a rat model of glaucoma. PLoS One 7 (7), e40065. doi:10.1371/journal.pone.0040065
Romano, C., Price, M., Bai, H. Y., and Olney, J. W. (1993). Neuroprotectants in Honghua: Glucose attenuates retinal ischemic damage. Invest. Ophthalmol. Vis. Sci. 34 (1), 72–80.
Rothman, S. M., and Olney, J. W. (1986). Glutamate and the pathophysiology of hypoxic--ischemic brain damage. Ann. Neurol. 19 (2), 105–111. doi:10.1002/ana.410190202
Saito, M., Yoshida, K., Saito, W., Fujiya, A., Ohgami, K., Kitaichi, N., et al. (2012). Astaxanthin increases choroidal blood flow velocity. Graefes Arch. Clin. Exp. Ophthalmol. 250 (2), 239–245. doi:10.1007/s00417-011-1843-1
Seong, H., Ryu, J., Yoo, W. S., Kim, S. J., Han, Y. S., Park, J. M., et al. (2017). Resveratrol ameliorates retinal ischemia/reperfusion injury in C57bl/6J mice via downregulation of caspase-3. Curr. Eye Res. 42 (12), 1650–1658. doi:10.1080/02713683.2017.1344713
Sheng, L., Lu, B., Chen, H., Du, Y., Chen, C., Cai, W., et al. (2019). Marine-steroid derivative 5α-androst-3β, 5α, 6β-triol protects retinal ganglion cells from Ischemia⁻Reperfusion injury by activating Nrf2 pathway. Mar. Drugs 17 (5), 267. doi:10.3390/md17050267
Shi, Y., Wu, X., Gong, Y., Qiu, Y., Zhang, H., Huang, Z., et al. (2010). Protective effects of caffeic acid phenethyl ester on retinal ischemia/reperfusion injury in rats. Curr. Eye Res. 35 (10), 930–937. doi:10.3109/02713683.2010.494820
Sinha, R. A., Singh, B. K., Zhou, J., Wu, Y., Farah, B. L., Ohba, K., et al. (2015). Thyroid hormone induction of mitochondrial activity is coupled to mitophagy via ROS-AMPK-ULK1 signaling. Autophagy 11 (8), 1341–1357. doi:10.1080/15548627.2015.1061849
Song, J., and Kim, Y. K. (2021). Targeting non-coding RNAs for the treatment of retinal diseases. Mol. Ther. Nucleic Acids 24, 2162–2531. (Print)). doi:10.1016/j.omtn.2021.02.031
Song, H., Zhao, C., Yu, Z., Li, Q., Yan, R., Qin, Y., et al. (2020). UAF1 deubiquitinase complexes facilitate NLRP3 inflammasome activation by promoting NLRP3 expression. Nat. Commun. 11 (1), 6042. doi:10.1038/s41467-020-19939-8
Sun, Y., Jin, K., Mao, X. O., Zhu, Y., and Greenberg, D. A. (2001). Neuroglobin is up-regulated by and protects neurons from hypoxic-ischemic injury. Proc. Natl. Acad. Sci. U. S. A. 98 (26), 15306–15311. doi:10.1073/pnas.251466698
Sutherland, B. A., Shaw, O. M., Clarkson, A. N., Jackson, D. N., Sammut, I. A., and Appleton, I. (2005). Neuroprotective effects of (-)-epigallocatechin gallate following hypoxia-ischemia-induced brain damage: Novel mechanisms of action. FASEB J. 19 (2), 258–260. doi:10.1096/fj.04-2806fje
Tang, L., Xu, G. T., and Zhang, J. F. (2023). Inflammation in diabetic retinopathy: Possible roles in pathogenesis and potential implications for therapy. Neural Regen. Res. 18 (5), 976–982. doi:10.4103/1673-5374.355743
Tarik, J., Paul, G., and Fadela, C. (2019). WHO launches first World report on vision. Geneva: News release. Available at: https://www.who.int/news/item/08-10-2019-who-launches-first-world-report-on-vision.
Tournaire-Marques, E. (2020). Ischemic optic neuropathies. J. Fr. Ophtalmol. 43 (6), 552–558. doi:10.1016/j.jfo.2019.10.020
Varga, B., Priksz, D., Lampé, N., Bombicz, M., Kurucz, A., Szabó, A. M., et al. (2017). Protective effect of Prunus cerasus (sour cherry) seed extract on the recovery of ischemia/reperfusion-induced retinal damage in zucker diabetic fatty rat. Molecules 22 (10), 1782. doi:10.3390/molecules22101782
Wang, H. X., and Ai, Y. D. (2005). Protective function of Ginkgo biloba extract 761 on rabbits' retinal ischemic injury. Int. J. Ophthalmol. 5 (6), 1168–1171.
Wang, S., Ye, Q., Tu, J., Zhang, M., and Ji, B. (2017). Curcumin protects against hypertension aggravated retinal ischemia/reperfusion in a rat stroke model. Clin. Exp. Hypertens. 39 (8), 711–717. doi:10.1080/10641963.2017.1313854
Wang, J., Tian, W., Wang, S., Wei, W., Wu, D., Wang, H., et al. (2017). Anti-inflammatory and retinal protective effects of capsaicin on ischaemia-induced injuries through the release of endogenous somatostatin. Clin. Exp. Pharmacol. Physiol. 44 (7), 803–814. doi:10.1111/1440-1681.12769
Wimpissinger, B., Berisha, F., Garhoefer, G., Polak, K., and Schmetterer, L. (2007). Influence of Ginkgo biloba on ocular blood flow. Acta Ophthalmol. Scand. 85 (4), 445–449. doi:10.1111/j.1600-0420.2007.00887.x
Woda, J. M., and Hof, W. V. (2011). Use of stem cells to reduce leukocyte extravasation. Europe PMC [abstract].
Wu, Y., Pang, Y., Wei, W., Shao, A., Deng, C., Li, X., et al. (2020). Resveratrol protects retinal ganglion cell axons through regulation of the SIRT1-JNK pathway. Exp. Eye Res. 200, 108249. doi:10.1016/j.exer.2020.108249
Wu, I. H., Chan, S. M., and Lin, C. T. (2020). The neuroprotective effect of submicron and blended Lycium barbarum for experiment retinal ischemia and reperfusion injury in rats. J. Vet. Med. Sci. 82 (11), 1719–1728. doi:10.1292/jvms.19-0646
Xie, C., Ginet, V., Sun, Y., Koike, M., Zhou, K., Li, T., et al. (2016). Neuroprotection by selective neuronal deletion of Atg7 in neonatal brain injury. Autophagy 12 (2), 410–423. doi:10.1080/15548627.2015.1132134
Yang, D., So, K. F., and Lo, A. C. (2017). Lycium barbarum polysaccharide extracts preserve retinal function and attenuate inner retinal neuronal damage in a mouse model of transient retinal ischaemia. Clin. Exp. Ophthalmol. 45 (7), 717–729. doi:10.1111/ceo.12950
Yang, Y., Xu, C., Chen, Y., Liang, J. J., Xu, Y., Chen, S. L., et al. (2019). Green tea extract ameliorates ischemia-induced retinal ganglion cell degeneration in rats. Oxid. Med. Cell. Longev. 2019, 8407206. doi:10.1155/2019/8407206
Yorgun, M. A. (2019). Effects of crocin on diabetic maculopathy: A placebo-controlled randomized clinical trial. Am. J. Ophthalmol. 204, 141–142. doi:10.1016/j.ajo.2019.02.040
Yuan, H. H., Zhou, W., Wu, G. Z., and Bao, H. Y. (2014). Relationship between protective effects of ginkgo biloba extract on retinal ischemia-reperfusion injury and autophagy. J. Shanghai Jiaot. Univ. 34 (5), 635–639. doi:10.3969/j.issn.1674-8115.2014.05.013
Zhang, B., Rusciano, D., and Osborne, N. N. (2008). Orally administered epigallocatechin gallate attenuates retinal neuronal death in vivo and light-induced apoptosis in vitro. Brain Res. 1198, 141–152. doi:10.1016/j.brainres.2007.12.015
Zhang, F., Li, Y., Zhang, L., and Mu, G. (2013). Synergistic protective effects of escin and low-dose glucocorticoids on blood-retinal barrier breakdown in a rat model of retinal ischemia. Mol. Med. Rep. 7 (5), 1511–1515. doi:10.3892/mmr.2013.1374
Zhu, M., Choy, B. N., and Lai, J. S. (2018). Neuro-protective effect of resveratrol on ischemia/reperfusion-Induced Retinal Injury in mice. Investigative Ophthalmol. Vis. Sci. 59 (9), 6116.
Keywords: ischemia, neuroprotective, retinal ischemia, natural product, treatment
Citation: He Q, Xiao L, Shi Y, Li W and Xin X (2023) Natural products: protective effects against ischemia-induced retinal injury. Front. Pharmacol. 14:1149708. doi: 10.3389/fphar.2023.1149708
Received: 22 January 2023; Accepted: 05 April 2023;
Published: 26 April 2023.
Edited by:
Xiaming Liu, Huazhong University of Science and Technology, ChinaReviewed by:
Prabhat Upadhyay, Massachusetts General Hospital and Harvard Medical School, United StatesCopyright © 2023 He, Xiao, Shi, Li and Xin. This is an open-access article distributed under the terms of the Creative Commons Attribution License (CC BY). The use, distribution or reproduction in other forums is permitted, provided the original author(s) and the copyright owner(s) are credited and that the original publication in this journal is cited, in accordance with accepted academic practice. No use, distribution or reproduction is permitted which does not comply with these terms.
*Correspondence: Xiaorong Xin, eHJnYzE5QGhvdG1haWwuY29t
Disclaimer: All claims expressed in this article are solely those of the authors and do not necessarily represent those of their affiliated organizations, or those of the publisher, the editors and the reviewers. Any product that may be evaluated in this article or claim that may be made by its manufacturer is not guaranteed or endorsed by the publisher.
Research integrity at Frontiers
Learn more about the work of our research integrity team to safeguard the quality of each article we publish.