- 1Health Science Center, Chifeng University, Chifeng, China
- 2Key Laboratory of Human Genetic Diseases in Inner Mongolia, Chifeng, China
- 3Department of Endocrinology, The Affiliated Hospital of Chifeng University, Chifeng, China
- 4Chifeng Clinical Medical College, Inner Mongolia Minzu University, Tongliao, China
- 5Department of Neurology, The Affiliated Hospital of Chifeng University, Chifeng, China
- 6Department of Cardiology, The Affiliated Hospital of Chifeng University, Chifeng, China
Cardiomyopathies are a clinically heterogeneous group of cardiac diseases characterized by heart muscle damage, resulting in myocardium disorders, diminished cardiac function, heart failure, and even sudden cardiac death. The molecular mechanisms underlying the damage to cardiomyocytes remain unclear. Emerging studies have demonstrated that ferroptosis, an iron-dependent non-apoptotic regulated form of cell death characterized by iron dyshomeostasis and lipid peroxidation, contributes to the development of ischemic cardiomyopathy, diabetic cardiomyopathy, doxorubicin-induced cardiomyopathy, and septic cardiomyopathy. Numerous compounds have exerted potential therapeutic effects on cardiomyopathies by inhibiting ferroptosis. In this review, we summarize the core mechanism by which ferroptosis leads to the development of these cardiomyopathies. We emphasize the emerging types of therapeutic compounds that can inhibit ferroptosis and delineate their beneficial effects in treating cardiomyopathies. This review suggests that inhibiting ferroptosis pharmacologically may be a potential therapeutic strategy for cardiomyopathy treatment.
Introduction
Cardiomyopathies are a clinically heterogeneous group of cardiac diseases characterized by heart muscle damage, causing cardiac muscle or myocardium disorders, diminished cardiac function, heart failure, and even sudden cardiac death (Franz et al., 2001; Schultheiss et al., 2019; Li D. et al., 2022). Cardiomyopathies are often related to electrical or mechanical dysfunction, frequently with a genetic origin or etiology (Maron et al., 2006). The 2006 American Heart Association classification categorizes and groups cardiomyopathy into primary or secondary categories (Maron et al., 2006). In primary categories (genetic, mixed, or acquired), the disease process is solely or predominantly confined to the heart. Secondary cardiomyopathies (i.e., dilated, hypertrophic, and restrictive cardiomyopathy) result from systemic conditions, i.e., cardiac involvement occurs as a part of systemic conditions (Brieler et al., 2017; Li T. et al., 2022). Researchers have divided the secondary causes of cardiomyopathy into various categories, including infectious, toxic, ischemic, metabolic, autoimmunogenic, and neuromuscular categories. The burden of ischemic cardiomyopathy (ICM), diabetic cardiomyopathy (DCM), doxorubicin-induced cardiomyopathy (DICM), and septic cardiomyopathy (SCM) is increasing in nearly all countries. The basic pathological mechanism of these cardiomyopathies (ICM, DCM, DICM and SCM) is cell death in cardiomyocytes. The pathogenesis and molecular mechanisms underlying these cardiomyopathies are poorly understood, warranting further investigation (Gilgenkrantz et al., 2021). Therefore, it is important to acquire insights into their pathogenesis to achieve the appropriate management and treatment of these disorders, thus providing support for protecting cardiac function.
In the past decades, ferroptosis, a non-apoptotic iron-dependent and peroxidation-driven regulated cell death (RCD) mechanism, has been rapidly acquiring attention in cardiomyopathies. Novel studies have explored the role of ferroptosis in DICM and ICM in murine models of cardiomyopathy (Conrad and Proneth, 2019; Fang et al., 2019), which demonstrated an association between ferroptosis and cardiac cell death induced by iron overload in vivo. Thereafter, several studies have revealed that ferroptosis plays a vital role in the pathogenesis of cardiomyopathy (Li D. et al., 2022). Meanwhile, certain compounds exert their therapeutic effects on experimental cardiomyopathy models by inhibiting ferroptosis.
In this review, we summarize the core mechanism by which ferroptosis leads to the genesis of cardiomyopathies. We focus on the emerging variety of therapeutic compounds that can inhibit ferroptosis and delineate their beneficial effects for treating cardiomyopathies. This review indicates that inhibiting ferroptosis pharmacologically may be a promising therapeutic strategy for treating cardiomyopathies.
Core molecular mechanisms underlying ferroptosis
Ferroptosis is an iron-dependent, oxidative form of non-apoptotic RCD, characterized by the iron-dependent oxidative modification of phospholipid membranes (Dixon et al., 2012). A delicate imbalance between ferroptosis inducers and inhibitors dictates its execution and induction. The inhibition of the solute carrier family 7 member 11/glutathione peroxidase 4 (SLC7A11/GPX4) antioxidant system and free iron accumulation are two key signals for inducing ferroptosis (Chen H. Y. et al., 2021). When the levels of iron-dependent ROS and lethal lipid peroxide (LPO), the two promoting factors of ferroptosis, substantially surpass the antiferroptotic capacity of ferroptosis defense systems, peroxidated phospholipid polyunsaturated fatty acids (PUFA-PL-OOH) accumulate on cellular membranes and induce its rupture, eventually resulting in ferroptosis (Lei et al., 2022). Phospholipid polyunsaturated fatty acids (PUFA-PLs) have an intrinsic susceptibility to peroxidation chemistry, which makes them the primary substrates for LPO (Hadian and Stockwell, 2020). Acyl-coenzyme A synthetase long chain family member 4 (ACSL4) catalyzes the addition of coenzyme A (CoA) to the long-chain polyunsaturated bonds of arachidonic acid (AA), causing PUFA esterification to form phospholipids. Following the activation of ACSL4, lysophosphatidylcholine acyltransferase 3 (LPCAT3) inserts acyl groups into lysophospholipids and incorporates free PUFAs into phospholipids (PL), participating in ferroptotic lipid signaling. Under the catalysis of oxidase and bioactive iron, PUFA-PLs in the membrane can be converted to phospholipid peroxides by both non-enzymatic Fenton reactions and enzymatic LPO reactions (Chen et al., 2021b; Liang et al., 2022). Iron serves as an essential cofactor for arachidonate lipoxygenases (ALOXs) and cytochrome P450 oxidoreductase (POR) to initiate the non-enzymatic Fenton reaction. ALOXs and POR promote lipid peroxidation. In enzymatic LPO, ACSL4 catalyzes the ligation of free PUFAs [such as AA and adrenic acid (AdA)] with CoA to generate PUFA-CoAs, which include AA-CoA or AdA-CoA (Dixon et al., 2015; Doll et al., 2017). Subsequently, LPCAT3 incorporates PUFA-CoAs into pLs to generate PUFA-PLs, which includes AA-phosphatidylethanolamine or AdA-phosphatidylethanolamine (Dixon et al., 2015; Kagan et al., 2017). Once the PUFA-PLs incorporated lipid bilayers, the iron-dependent enzymes (such as POR and ALOXs) and labile iron use O2 to perform a peroxidation reaction, generating peroxidated PUFA-PL or polyunsaturated-fatty-acid-containing -phospholipid hydroperoxides (PUFA-PL-OOH) (Hadian and Stockwell, 2020; Zou et al., 2020). Other membrane electron transfer proteins, particularly the NADPH oxidases, are also involved in ferroptosis by contributing to ROS production for LPO (Xie et al., 2017). LPO and its secondary products, namely, malondialdehyde (MDA) and 4-hydroxynonenal (4-HNE), cause pore formation in the lipid bilayers, eventually resulting in cell death and ferroptosis (Tang and Kroemer, 2020). Ferroptosis has acquired substantial attention in cardiomyopathy research. Further, it plays a vital role in the pathogenesis of cardiomyopathies, such as ICM, DCM, DICM, and SCM. Therapeutic strategies targeting ferroptosis may facilitate the treatment of these cardiomyopathies.
Ferroptosis in cardiomyopathy
Ferroptosis in ischemic cardiomyopathy
Ischemic cardiomyopathy (ICM) principally results from long-term ischemia/hypoxia within coronary atherosclerosis; it impairs the systolic or diastolic function of the heart. ICM represents the leading cause of heart failure (HF) worldwide (Chang et al., 2022; Del Buono et al., 2022). Further, it leads to numerous phenotypic changes, such as myocardial remodeling and HF.
Metabolic, neurohumoral, and inflammatory alterations are involved in the pathophysiological mechanisms underlying ICM, leading to hypertrophy in cardiomyocytes, fibrosis, calcium dyshomeostasis, inflammation, oxidative stress, and even cardiomyocyte death (Cabac-Pogorevici et al., 2020). Ischemic heart disease is a major contributor to the global disease burden and constitutes the leading cause of global mortality worldwide. Acute myocardial infarction (AMI) resulting from reduced oxygen supply causes initial damage to the cardiac tissues, thus making it the primary cause of disability and mortality. Myocardial reperfusion strategies and reoxygenation are effective and the preferred treatment for AMI; non-etheless, reperfusion inevitably triggers the cell death of cardiomyocytes, increases the infarct size, and worsens the condition, which is referred to as myocardial ischemia-reperfusion injury (MIRI) (Ibáñez et al., 2015). MIRI leads to oxidative stress and energy metabolism disturbances, among other issues (Li D. et al., 2021). Therefore, understanding the mechanisms of MIRI is essential for attenuating the triggers of cardiomyocyte cell death and preventing left ventricular remodeling and HF.
A novel study reported on the role of ferroptosis in ischemia/reperfusion (I/R)-induced cardiomyopathy in murine models (Fang et al., 2019), which established an in vivo correlation between ferroptosis and cardiac cell death (Conrad and Proneth, 2019). Thereafter, emerging studies delved into the pathophysiological role of ferroptosis in the development of MIRI and ICM (Figure 1). Numerous molecular mechanisms and pathways are related to the genesis of MIRI, including iron homeostasis imbalance, lipid peroxidation, and redox homeostasis imbalance. Since the introduction of ferroptosis in 2012, researchers have revisited the role of iron homeostasis imbalance, lipid peroxidation, or glutathione metabolism disorder in MIRI, thus proposing that ferroptosis participates in MIRI pathogenesis. Among all types of organ ischemia/reperfusion injury (IRI), the role of ferroptosis in the pathogenesis of MIRI has been the most extensively studied.
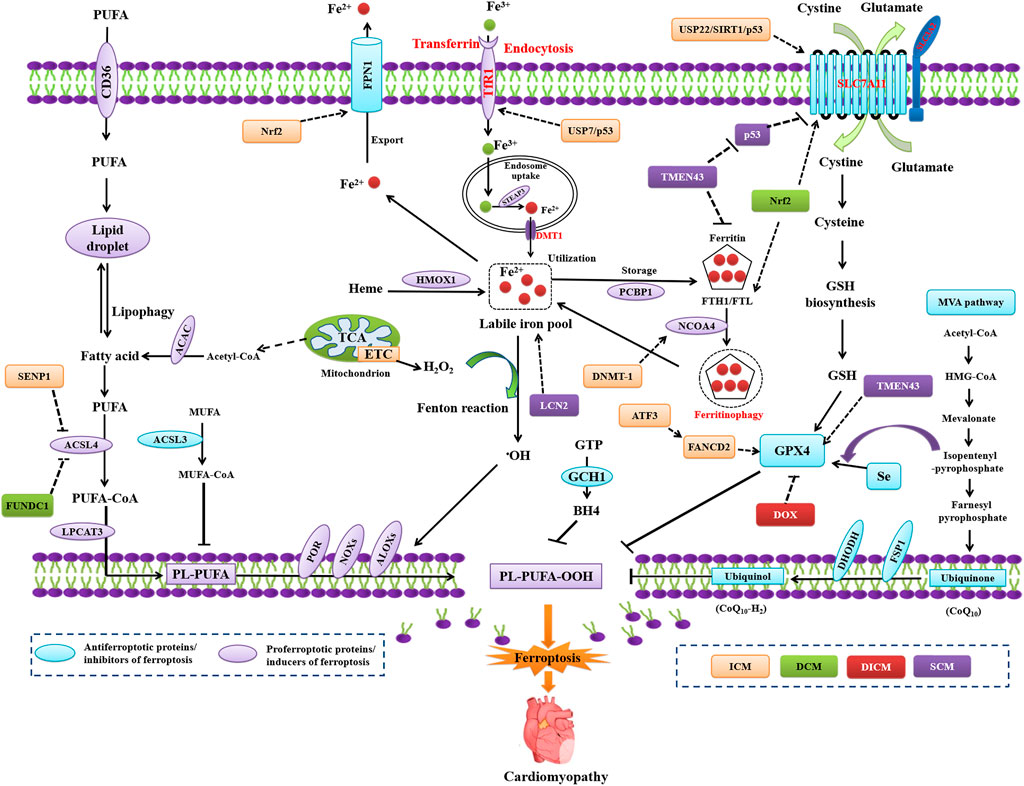
FIGURE 1. Regulation of ferroptosis in model in ICM, DCM, DICM, and SCM. ATF3, activating transcription factor 3; DNMT-1, DNA (cytosine-5) -methyltransferase 1; FUNDC1, FUN14 domain containing 1; LCN2, neutrophil-derived lipocalin-2; SENP1, sentrin-specific protease 1; TMEM43, transmembrane protein 43; USP7, ubiquitin-specific protease 7.
Role of dysregulation of iron metabolism in MIRI
The accumulation of iron, a core characteristic of ferroptosis, plays a pathogenic role in AMI and MIRI. Excessive iron is transported into the cardiomyocytes, thus predisposing them to undergo ferroptosis by the Fenton reaction and ROS generation after I/R (Li J. Y. et al., 2021). Ferroptosis predominantly occurs in the reperfusion phase of cardiac tissues, characterized by a gradual increase in the ACSL4, Fe2+, and MDA levels, along with decreased levels of GPX4 (Tang et al., 2021a). Cardiomyocytes are vulnerable to the dysregulation of iron homeostasis, which is central to MIRI through different pathways to increase the iron content. The heart utilizes several iron uptake transport systems, including L-type (LTCC) or T-type (TTCC) voltage-dependent Ca2+ channels, transferrin (TF) receptor (TfR1), and divalent metal transporter (DMT1) (Lillo-Moya et al., 2021).
Iron enters the cardiomyocytes principally through TfR1 as TF or through LTCC as non-TF-bound iron, TTCC, and DMT1. During MIRI, the intracellular iron-storing protein, the degraded ferritin to release iron and perform iron-mediated Fenton reaction, resulting in oxidative damage to cardiomyocytes and loss of cardiac function. Studies have demonstrated excessive iron accumulation in the myocardial scar in mice MIRI models (Baba et al., 2018; Fang et al., 2019), thereby suggesting iron overload as a primary characteristic of ferroptosis. The ferroptosis inhibitor ferostatin-1 (Fer-1) or iron chelator dexrazoxane (DXZ) inhibits cardiac remodeling and fibrosis induced by IRI (Fang et al., 2019). Increased cellular iron content exists in IRI mice, apart from decreased activities of GPX4 and ferritin heavy chain-1 (FTH1) as well as decreased glutathione (GSH) levels in the cardiac issue after MIRI (Chen et al., 2021c). Moreover, the ubiquitin-specific protease 7 (USP7)/p53 pathway activates TfR1 to exacerbate cardiomyocyte ferroptosis in subsequent I/R (Tang et al., 2021a). The pharmacological inhibition of USP7 results in increased p53 activity and decreased TfR1, thus leading to decreased ferroptosis and MIRI (Tang et al., 2021b). Therefore, the pharmacological inhibition of TfR1 activity may inhibit ferroptosis in MIRI.
Nuclear receptor coactivator 4 (NCOA4)-mediated autophagy, i.e., ferritinophagy, causes I/R-induced ferroptosis. The activation of ferritinophagy degrades ferritin and increases the availability of iron in the cells (Lin et al., 2020). FTH1 binds to NCOA4 that functions as a selective autophagy receptor; it transports intracellular ferritin to the autophagy lysosomes and releases free iron (Mancias et al., 2014). Ferritinophagy induces ferroptosis through iron homeostasis regulation and ROS production in cells (Gao et al., 2016; Hou et al., 2016; Liu H. et al., 2022). Inhibiting DNA (cytosine-5)-methyltransferase 1 attenuates ferroptosis by impeding NCOA4 -mediated ferritinophagy in diabetic MIRI (Li J. Y. et al., 2021). The iron exporter ferroportin 1 (FPN1) plays a vital role in regulating iron homeostasis. Hepcidin, an iron-regulating hormone, mediates the internalization and degradation of FPN1, which maintains cardiac iron homeostasis (Lakhal-Littleton et al., 2015). Nuclear factor erythroid 2–related factor 2 (Nrf2) transcriptionally regulates FPN1. Nrf2/FPN1 signaling activation can alleviate MIRI by inhibiting ferroptosis (Tian H. et al., 2021). FTH deletion in the myocardium upregulates heme oxygenase-1 (HO-1) among other antiferroptotic proteins, which induces SLC7A11 and finally inhibits IRI-induced ferroptosis, thereby maintaining the function of myocardium (Machado et al., 2022).
Role of LPO in MIRI
Deferoxamine therapy decreases myocardial injury by inhibiting ferroptosis in I/R-induced rat hearts. The specific redox reactions of PUFA-PLs in ischemia-induced cardiomyocytes initiate oxidative damage in the reperfusion phase. ALOX15 induction by ischemia/hypoxia initiates the oxidation of PUFA-PLs (particularly PUFA-PE) and results in cardiomyocyte ferroptosis. Further, ALOX15 ablation in mice confers resistance to PUFA-dependent ischemia-induced cardiac injury (Ma X. et al., 2022). The overexpression of activating transcription factor 3 (ATF3) inhibits the classical ferroptosis activators ras-selective lethal small molecule 3 and erastin-induced ferroptosis in cardiomyocytes. ATF3 expression increases in the early phase of reperfusion, whereas its ablation significantly aggravates IRI. The binding of ATF3 to the transcriptional start site of the FA complementation group D2 can enhance its promoter activity, thereby exerting cardioprotective effects against H/R injury through an antiferroptosis mechanism (Liu M. Z. et al., 2022). Bai and colleagues have demonstrated that SENP1 expression is upregulated by hypoxia, which protects cardiomyocytes against ferroptosis through deSUMOylating hypoxia-inducible factor-1α and ACSL4 (Bai et al., 2021).
Role of SLC7A11/GPX4 axis inhibition in MIRI
Increased levels of ACSL4, Fe2+, and MDA, along with decreased GPX4 levels, are observed in the myocardium after MIRI (Tang et al., 2021a). The inhibition of the GSH-generation pathway, either iron chelation or glutaminolysis, could alleviate IRI by blocking ferroptosis (Gao et al., 2015). A specific ferroptosis inhibitor suitable for animal models, i.e., liproxstatin 1, can protect the mouse myocardium against IRI by decreasing voltage-dependent anion-selective channel protein 1 levels and upregulating GPX4 levels (Feng et al., 2019). The expression of USP22, SIRT1, and SLC7A11 is inhibited after IRI injury, whereas p53 is highly expressed in the myocardial tissues. Conversely, the overexpression of USP22, SIRT1, or SLC7A11 reduces the degree of IRI injury by inhibiting ferroptosis and improves the viability of cardiomyocytes (Ma et al., 2020).
Ferroptosis in diabetic cardiomyopathy
DCM, a specific form of cardiomyopathy independent of hypertension and coronary artery disease (Tan et al., 2020), is caused by diabetes mellitus (DM)-associated dysregulated glucose and lipid metabolism (Tan et al., 2020). DM increases oxidative stress and activates multiple inflammatory pathways, leading to cellular injury, cardiac remodeling, and systolic and diastolic dysfunction (Tan et al., 2020; Khan et al., 2021). The eventual outcome is cardiomyocyte cell death. The clinical features and pathogenesis of DCM have been well-characterized in the past 4 decades; however, its effective therapeutic regimen is still limited, thus suggesting the need to explore novel mechanisms underlying DCM development. Ferroptosis may be associated with the pathological progression of DCM (Chen et al., 2020; Wei LY. et al., 2022; Wei Z. et al., 2022). Ferroptosis plays a role in DM (Behring et al., 2014; Bruni et al., 2018; Lutchmansingh et al., 2018; Shu et al., 2019; Krümmel et al., 2021) (Figure 1). A novel study reported on the role of ferroptosis in the heart of diabetic mice in 2022, thus demonstrating that Nrf2 activation attenuates ferroptosis by upregulating SLC7A11 and ferritin levels (Wang D. et al., 2022). GPX4 can inhibit DCM in GPX4 transgenic mouse models (Baseler et al., 2013).
The ablation of cluster of differentiation 74 (CD74; a receptor for the regulatory cytokine macrophage migration inhibitory factor) prevents DM-evoked and oxidative stress. Ferroptosis inhibitors preserve the cardiomyocyte function and inhibit LPO induced by the high glucose/high fat (HGHF) challenge in vitro. Recombinant MIF mimics HGHF-induced LPO and depletes GSH and ferroptosis. Conversely, MIF inhibitors reverse these effects mediated by recombinant MIF. Taken together, CD74 ablation rescues DCM by inhibiting ferroptosis, thus indicating CD74 as a promoter of ferroptosis (Chen H. et al., 2022). FUN14 domain-containing 1 (FUNDC1) insufficiency sensitizes DCM through ACSL4-mediated ferroptosis, thus indicating FUNDC1 as an inhibitor of ferroptosis (Pei et al., 2021). Further, long non-coding RNAs (LncRNAs) regulate ferroptosis in DCM. The lncRNA-zinc finger antisense 1 works as a competing endogenous RNA that sponges miR-150-5p and downregulates cyclin D2 (CCND2), promoting ferroptosis and DCM development (Ni J. et al., 2021). In summary, ferroptosis plays a significant role in the development of DCM. However, the molecular mechanism warrants further investigation.
Ferroptosis in DOX-induced cardiomyopathy
Anthracyclines are the most widely used anticancer chemotherapeutic agents. However, doxorubicin (DOX) causes cardiotoxicity, resulting in DICM, thereby limiting its clinical efficacy (Herrmann, 2020; Fang et al., 2023). Ferroptosis plays an essential role in the pathogenesis of DICM (Fang et al., 2023) (Figure 1). Wang et al. have demonstrated that DOX induces heart injury and increases cardiac iron levels, lipid-derived ROS, and the biomarkers of ferroptosis (Fang et al., 2019). They presented novel evidence that the contributions of ferroptosis to DICM in DOX-treated mice and its subsequent inhibition exert cardioprotection (Fang et al., 2019). Their findings were corroborated by other studies which revealed that ferroptosis is a crucial mechanism in DICM and that acyl-CoA thioesterase 1 (ACOT1) plays a critical role during the process. Thus, they demonstrated ACOT1 as a ferroptosis inhibitor and that targeting the inhibition of ferroptosis is a strategy for DICM treatment (Liu et al., 2020). Tadokoro and colleagues have revealed that DOX inhibits GPX4 and induces LPO, thus leading to mitochondria-dependent ferroptosis in a DICM mouse model (Tadokoro et al., 2020). Further, the ferroptosis inhibitor ferrostatin-1 (Fer-1) can protect cardiomyocytes against DOX-induced cell injury (Tadokoro et al., 2020). Zhang et al. (2021) have indicated that DOX upregulates high mobility group box 1 expression, which promotes ferroptosis-associated cardiotoxicity in DOX-treated rats. Fer-1 or DXZ reverse DOX-induced ferroptosis and DICM. In summary, ferroptosis inhibition is a therapeutic target for DICM.
Ferroptosis in septic cardiomyopathy
Sepsis is a life-threatening organ dysfunction resulting from dysregulated immune response to an infection. Seventy percent of patients with sepsis develop septic cardiomyopathy (SCM), which is the leading cause of sepsis-related morbidity and mortality (Nabzdyk et al., 2019; Hollenberg and Singer, 2021). Ferroptosis is involved in SCM (Figure 1). GSH depletion and the downregulation of GPX4 expression, as well as increased iron content and LPO levels, exist in cecal ligation and puncture-induced sepsis animal model, implying the involvement of ferroptosis in the pathogenesis of SCM (Wang et al., 2020). Dexmedetomidine exerts cardioprotective effects through ferroptosis inhibition by decreasing iron accumulation, downregulating the protein levels of HO-1, and inducing GPX4 (Wang et al., 2020). The ferroptosis inhibitors deferoxamine and Fer-1 can improve cardiac function and decrease mortality in septic mice by decreasing the levels of ferroptosis in cardiomyocytes (Li et al., 2020). These results support the hypothesis that ferroptosis is involved in the pathogenesis of sepsis-induced myocardial injury. Ferritinophagy-mediated ferroptosis plays a pathogenic role in sepsis-induced myocardial injury (Li et al., 2020). Li et al. (2020) have demonstrated that ferroptosis plays a crucial role in sepsis-induced cardiomyopathy in sepsis-related models, including a lipopolysaccharide (LPS)-induced model of septic cardiomyopathy (Li et al., 2020).
Specific regulators play a role in modulating ferroptosis and SCM. The transmembrane protein 43 (TMEM43), a transmembrane protein related to cardiomyopathy, protects against SCM by inhibiting ferroptosis in LPS-induced mice (Chen L. et al., 2022). The knockdown of TMEM43 in the heart aggravates LPS-induced cardiomyopathy, accompanied by an increased cardiac ferroptosis. TMEM43 overexpression decreases LPS-induced ferroptosis and cardiac injury by inhibiting LPO. TMEM43 silencing promotes ferroptosis and cell injury in LPS-induced rat H9c2 cardiomyocytes. TMEM43 downregulates the expression of P53 and ferritin but upregulates the levels of GPX4 and SLC7A11, thereby inhibiting LPS-induced ferroptosis. Fer-1 can ameliorate TMEM43 knockdown-induced deteriorating effects in LPS-induced cardiac injury. Taken together, TMEM43 protects against SCM by inhibiting ferroptosis (Chen Z. et al., 2022). The islet cell autoantigen 69, which can regulate inflammation and immune response, induces ferroptosis to cause septic cardiac dysfunction through the stimulator of interferon gene trafficking (Kong et al., 2022). The neutrophil-derived lipocalin-2 induces ferroptosis by increasing the labile iron pool in the cardiomyocytes of LPS-induced mouse SCM model (Huang Q. et al., 2022).
Pharmacological inhibition of ferroptosis for treating cardiomyopathy
Ferroptosis was first described in 2012; the studies on its role in cardiomyopathy are still in their infancy. However, existing evidence suggests a strong correlation between ferroptosis and cardiomyopathy. Thus, the inhibition of ferroptosis may be a promising target for treating cardiomyopathy. Ferroptosis reportedly plays a pathogenic role in cardiomyopathy; thus, scientists have begun identifying a targeted antiferroptosis approach for cardiomyopathy treatment. Numerous drugs have been recognized to exert a therapeutic impact on cardiomyopathy treatment by inhibiting ferroptosis. Several experimental compounds and clinical drugs inhibit ferroptosis to achieve therapeutic purposes in cardiomyopathies. The pharmacological inhibition of ferroptosis is becoming a cardioprotective strategy for cardiomyopathy prevention in vitro or in vivo. We try to sort these ferroptosis-inhibiting small molecules by mode of action. These categories maybe include activator of system Xc−, ferroptosis-inhibiting Nrf2 activators, GPX4 activator (direct or indirect), ferroptosis inhibitors through combined mechanisms, or ferroptosis inhibitors through unknown mechanisms. However, it is hard to clearly classify the ferroptosis-inhibiting small molecules into a specific categories.
Inhibiting ferroptosis to treat ICM
Icariin (1) (Liu X. et al., 2021), xanthohumol (2) (Lin et al., 2022), britanin (3) (Lu et al., 2022), etomidate (4) (Lv et al., 2021),GAA(5) (Lin et al., 2021),dexmedetomidine (6) (Wang H. et al., 2022), sulforaphane (7) (Tian Y. et al., 2021), naringenin (8) (Xu et al., 2021), C3G (9) (Shan X. et al., 2021), resveratrol (10) (Xu et al., 2019; Li D. et al., 2022),5-aza-CdR (11) (Li W. et al., 2021), ferulic acid (12) (Liu XJ. et al., 2021), PDA NPs (13) (Zhang H. et al., 2021), atorvastatin (14) (Peng et al., 2022), baicalin (15) (Fan et al., 2021), and ponatinib + deferoxamine(16) (Tu et al., 2021) alleviate ICM through inhibiting ferroptosis (Table 1).
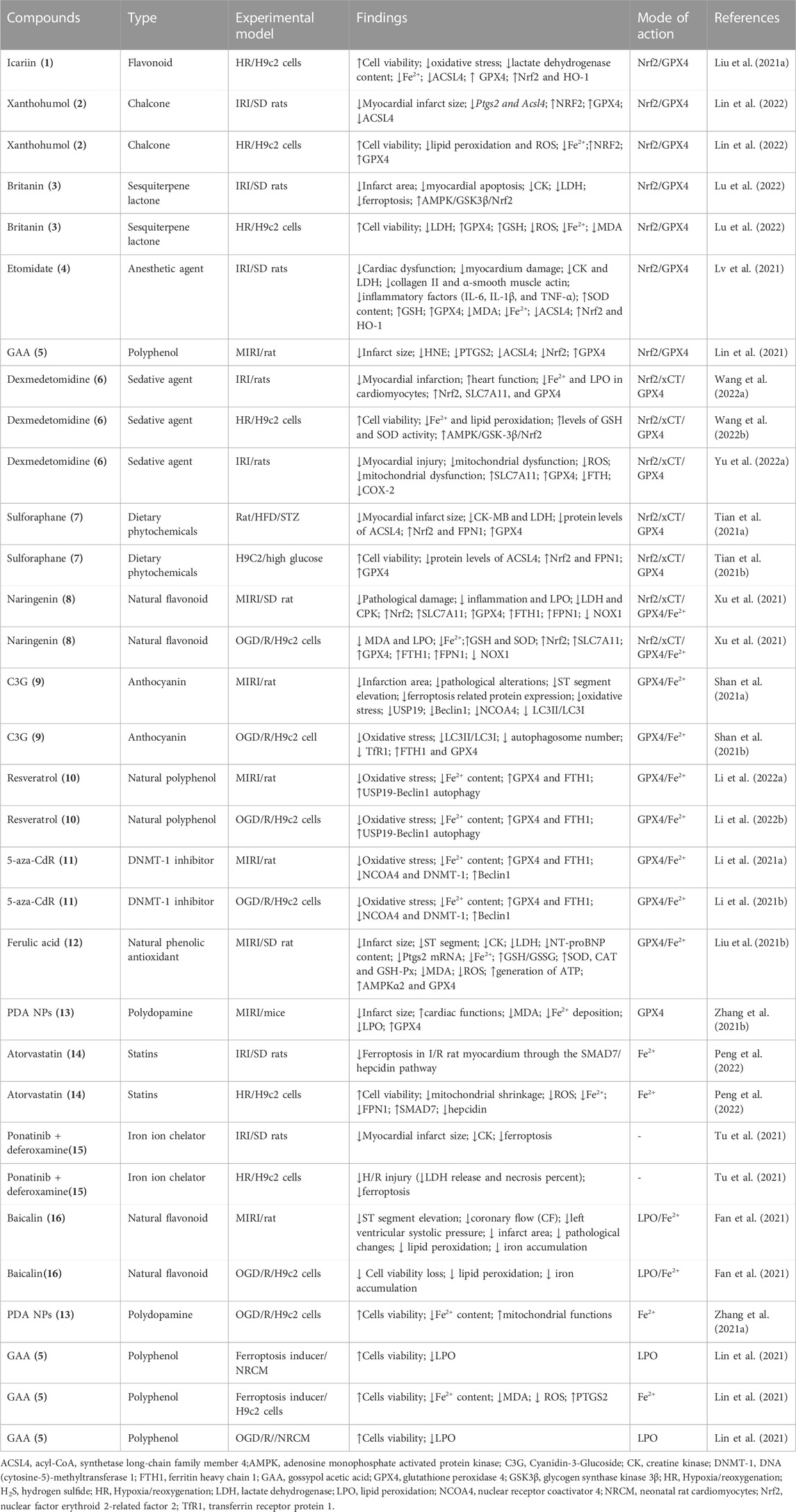
TABLE 1. Emerging compounds targeting key regulators of ferroptosis to inhibit ischemic cardiomyopathy.
Activators of System Xc-
Icariin (1), a natural flavonoid compound, is the main component of the Chinese herb Epimedium (also called YinYangHuo in Traditional Chinese Medicine) that has the functions of anti-aging, anti-inflammation, antioxidation, anti-osteoporosis, and ameliorating fibrosis (Su et al., 2023).1 is a potent inducer of Nrf2 (Moratilla-Rivera et al., 2023). 1 inhibit hypoxia/reoxygenation (H/R)-induced ferroptosis by increasing GPX4 and decreasing ACSL4 and content of Fe2+ in cardiomyocytes through activating the Nrf2/HO-1 signaling pathway (Liu X. et al., 2021). Owing to outstanding medicinal properties in preventing and curing many common health issues, 1 and its derivates, icariside II (ICS) and icaritin (ICT) have garnered great interest in drug development. 1 possesses a variety of beneficial effects in regulating cardiovascular inflammation and other biological activities. In China, YinYangHuo and its compound have been used in the treatment of numerous diseases, like AD, stroke, and depression. ICA and its metabolites, which contain flavonoids, polysaccharides, vitamin C, and other active compounds, have been proven to have cardio-cerebrovascular protective benefits (Wang et al., 2023). 1 can works as a prodrug was subjected to preclinical studies. We must realize that the oral bioavailability rate is only 12.02% for 1. Studies have shown the addition of cyclodextrins (CDs) to ICA can result in a vast increase in its water solubility, consequently achieving considerably better bioavailability (Cui et al., 2013; Jin et al., 2013). The degradation of ICA into ICS in vivo promotes ICA absorption (Cheng et al., 2015).
Xanthohumol (2) is a principal prenylated chalcone isolated from hops with its anti-inflammatory, anti-oxidative, and cancer-preventive properties (Zhou et al., 2021; Neumann et al., 2022; Vicente de Andrade Silva et al., 2023).2 protect cardiomyocytes against Fe-SP- and RSL3-induced ferroptosis by decreasing the production of lipid peroxidation and ROS, chelating iron, increasing the Nrf2 and GPX4 protein, while decreasing the mRNA levels of Ptgs2 and Acsl4, and the protein levels of ACSL4 (Lin et al., 2022). The poor solubility and stability severely limit 2 utilization (Luo et al., 2023). Britanin (3) is a potent inducer of Nrf2(Wu et al., 2017). 3 exert cardioprotective effect against IR-mediated damage through inhibiting ferroptosis by activation of the AMPK/GSK3β/Nrf2 signalling thereby upregulating GPX4 (Lu et al., 2022). Etomidate (4) is an ultrashort-acting, non-barbiturate hypnotic intravenous anesthetic agent. 4 mitigated IR-induced ICM through inhibiting ferroptosis by upregulating GPX4 expression, and decreasing the levels of MDA and iron and ACSL4. Nrf2 inhibitors ML385 eliminated the inhibition of 4 on ferroptosis induced by IR, suggesting that 4 attenuated the myocardial injury by inhibiting IR-induced ferroptosis via Nrf2(Lv et al., 2021). Gossypol Acetic Acid (GAA, 5), a natural product taken from the seeds of cotton plants, attenuates ICM through inhibiting ferroptosis by chelating iron content, and downregulating mRNA levels of Ptgs2 in RSL3, and Fe-SP-induced H9c2, inhibiting LPO in oxygen-glucose deprivation/reperfusion (OGD/R)-induced H9c2.5 attenuates IR-induced ICM through inhibiting ferroptosis by decreasing the production of LPO, increasing the Nrf2 and GPX4 protein, while decreasing the mRNA levels of Ptgs2 and Acsl4, and the protein levels of ACSL4 (Lin et al., 2021). Dexmedetomidine (6), a highly selective alpha2-adrenoceptor agonist with sedative, analgesic, sympatholytic, and hemodynamic-stabilizing properties, posess the protective effect against I/R (Xiao Z. et al., 2021; Chen Y. et al., 2021; Deng et al., 2022; Yang et al., 2022; Hu et al., 2023) and H/R (Wu W. et al., 2022; Wang L. et al., 2022) induced cardiomyocyte injury. 6 attenuates ICM through inhibiting ferroptosis by activating AMPK/GSK-3β-dependant Nrf2/SLC7A11/GPX4 (Wang et al., 2022d).
Sulforaphane (7) is a naturally occurring dietary phytochemical extracted from cruciferous vegetables (Zheng et al., 2022).7 is a potent Nrf2 activators and inhibit cardiomyopathy (Xin et al., 2018; Su et al., 2021; Wang et al., 2022e). 7 is an important member of the isothiocyanates, and is abundant in cruciferous plants with excellent anti-cancer effects (Wei LY. et al., 2022).7 attenuates ICM in diabetic rats through inhibiting ferroptosis by activation of Nrf2/FPN1 pathway (Tian H. et al., 2021). As a well known activator of Nrf2, 7 can upregulate multiple antioxidants and protect against various oxidative damages. 7 prevents rat cardiomyocytes from H/R injury in vitro via activating SIRT1 and subsequently inhibiting ER stress (Li et al., 2016). 7 protects from myocardial ischemia-reperfusion damage through activating Nrf2 (Silva-Palacios et al., 2019).7 inhibit intermittent hypoxia-induced cardiomyopathy in mice through activating Nrf2 (Zhou et al., 2018). Several clinical studies with 7 for the (supportive) treatment of non-alcoholic fatty liver disease (NCT04364360), chronic kidney disease (NCT05153174, NCT04608903) and anthracycline related cardiotoxicity in breast cancer (NCT03934905) are ongoing. A multi-center, randomized, placebo-controlled clinical trial is needed to be conducted to investigate 7 in adult patients with ICM.
The dietary natural polyphenolic flavonoid compounds are found in various citrus fruits, bergamots, tomatoes, and other fruits, naringenin (8) ameliorates myocardial injury and cardiac dysfunction (Yu et al., 2019; Ye et al., 2020; He et al., 2022; Sutanto et al., 2022). 8 alleviates ICM by regulating the Nrf2/SLC7A11/GPX4 axis to inhibit ferroptosis (Xu et al., 2021). C3G (9) (a natural anthocyanins) (Shan X. et al., 2021), resveratrol (10) (Xu et al., 2019; Li T. et al., 2022), 5-aza-CdR (11) (Li Y. et al., 2021), ferulic acid (12) (Liu XJ. et al., 2021), and polydopamine nanoparticles (PDA NPs, 13) (Zhang H. et al., 2021) alleviate ICM through inhibiting ferroptosis by upregulating GPX4.
Ferroptosis-related compounds targeting iron
13 (Zhang et al., 2021b),atorvastatin (14) (Peng et al., 2022),ponatinib/deferoxamine (15) [76], and baicalin (16) (Fan et al., 2021) are classified into this groups (Table 1).13 functions as a new type of ferroptosis inhibitor through inhibiting Fe2+ accumulation and restoring mitochondrial functions in H9c2 cells and reduced Fe2+ deposition and lipid peroxidation in a myocardial I/R injury mouse model (Zhang H. et al., 2021). Atorvastatin (14) is a potent, orally available inhibitor of hepatic 3-hydroxy-3-methylglutaryl-coenzyme A (HMG-CoA) reductase, the major rate-limiting enzyme in cholesterol synthesis.14 reversed erastin or H/R-induced cell injury in H9C2 cells through inhibiting ferroptosis by decreasing Fe2+ via upregulating expression of FPN1(Peng et al., 2022). 14 increased the expression of the SMAD7 and decreased the expression of the hepcidin in H/R-induced H9C2 cells (Peng et al., 2022).14 protects myocardium against ischemia-reperfusion injury through various mechanisms (Zuo et al., 2016; Chen et al., 2017; Yang et al., 2018; Cao et al., 2019). Two clinical studies with 14 for the treatment of dilated cardiomyopathy (www.clinicaltrials.gov,NCT01015144) and hypertrophic cardiomyopathy (www.clinicaltrials.gov,NCT00317967) were completed ongoing. However, there is no clinical trials to study the effect of 14 on ICM, remaining an open conundrum for future investigate on.15, an iron chelators can block ferroptosis. The combination of ponatinib with 15 exerted synergistic effect on reducing H/R injury, showing simultaneous suppression of ferroptosis[76].
Inhibiting ferroptosis to treat DCM
6- Gingerol (17) (Wu X. et al., 2022), curcumin (18) (Wei J. et al., 2022), canagliflozin (19) (Du et al., 2022), L6H21 (20) (Sumneang et al., 2022), sulforaphane (7) (Wang Z. et al., 2022), and SR9009 (21) (Huang Q. et al., 2022) attenuate DCM through inhibiting ferroptosis (Table 2). The major active components of ginger 17 have protect cardioprotective effect (Zhang et al., 2019; Ma et al., 2021; Han et al., 2022a; Han et al., 2022b). 17 alleviates DCM through inhibiting ferroptosis by decreaseing the expression of FACL4 and the content of iron and enhancing the expression of Nrf2/GPX4 (Wu X. et al., 2022). 18, a natural polyphenol phytochemical derived from turmeric with antioxidant, anti-inflammatory, and anticancer properties, activates Nrf2/HO-1 signaling to relieve diabetic cardiomyopathy injury (Ren et al., 2020; Wu et al., 2020; Wei et al., 2021; Wu S. et al., 2022).18 alleviates DCM through inhibiting ferroptosis by activating Nrf2, leading to upregulating GPX4, highlighting a potentially new therapeutic route for investigation for the treatment DCM(Wei J. et al., 2022). 19 is an anti-diabetes drug belongs to sodium-glucose cotransporter 2 inhibitor with extra cardiovascular benefits (Zhang et al., 2023). 19 alleviates DCM through inhibiting ferroptosis by activating system Xc−/GSH/GPX4 axis and regulating iron homeostasis (Du et al., 2022). Myeloid differentiation factor 2 (MD2) inhibitor L6H21 (20) alleviates DCM through inhibiting ferroptosis in prediabetic rats (Sumneang et al., 2022). As a Rev-erbs agonist SR9009 (21) alleviates DCM through inhibiting ferroptosis through by down-regulating ferritinophagy (Huang Y. et al., 2022). Sulforaphane (7) also works as an activators of System Xc− to alleviates DCM(Wang D. et al., 2022).
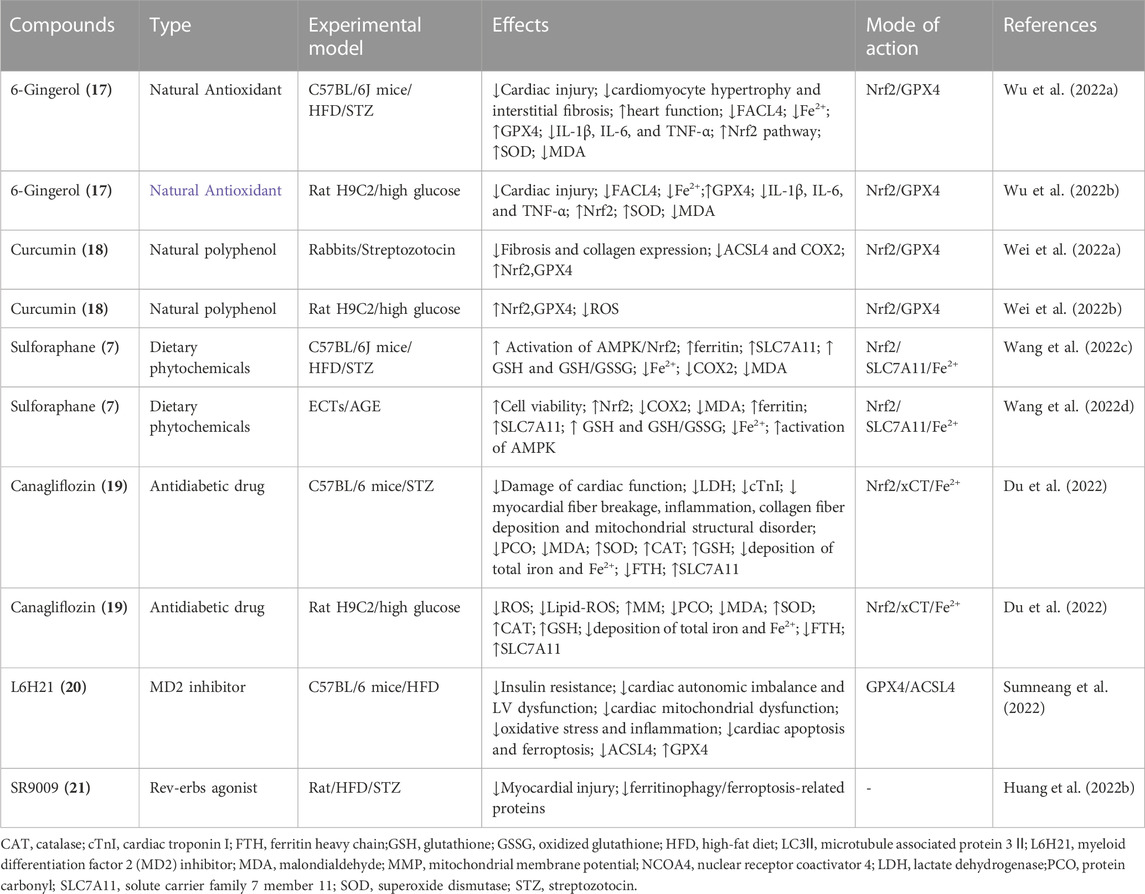
TABLE 2. Emerging compounds targeting key regulators of ferroptosis to inhibit diabetic cardiomyopathy.
Inhibiting ferroptosis to treat DICM
Compounds that alleviate DICM through inhibiting ferroptosis include huaier polysaccharide (22) (Ma X. et al., 2022), LCZ696 (23) (Liu X. et al., 2022), fisetin (24) (Li D. et al., 2021), salidroside (25) (Chen H. et al., 2022), resveratrol (10) (Yu P. et al., 2022), dexazoxane (26) (Zhang H. et al., 2021), melatonin(27) (Sun et al., 2022), EGCG(28) (He et al., 2021), 16d and 16e (29) (Xu et al., 2022), ethoxyquin (30) (Tadokoro et al., 2022), and 7j (31) (You et al., 2022) inhibit DICM through inhibiting ferroptosis (Table 3). 22 is a naturally occurring bioactive macromolecule, found in Huaier fungus and has been shown to exert antitumor and antimetastasis activity (Tian Y. et al., 2021; Gou et al., 2022).22 attenuates DICM through inhibiting ferroptosis by upregulating GPX4, suggesting its a direct activators of GPX4 (Ma X. H. et al., 2022). 23 is a first-in-class angiotensin receptor neprilysin inhibitor that can attenuate DICM through inhibiting ferroptosis (Liu H. et al., 2022). 23 significantly attenuated DICM by decreasing lipid ROS and MDA and increasing GPX4 and GSH. 23 activate AKT leading to increase SIRT3 expression and deacetylated SOD2. SIRT3 knockdown and AKT inhibition reversed the protective effect of 23 in H9c2 cells, suggesting that 23 prevents DICM by inhibiting ferroptosis via AKT/SIRT3/SOD2 signaling pathway (Liu M. Z. et al., 2022). Fisetin (24), a naturally occurring polyphenol that is frequently present in a variety of fruits and vegetables, exert cardioprotective effect against cardiomyopathy (Althunibat et al., 2019; ALTamimi et al., 2021).24 attenuates DICM through inhibiting ferroptosis in vivo and in vitro by activating SIRT1/Nrf2 signaling pathway (Li J. Y. et al., 2021). 24 increases GPX4 and GSH, thereby reducing the MDA and lipid ROS levels. Moreover, 24 exerted its protective effect by increasing the SIRT1 expression and the Nrf2 mRNA and protein levels and its nuclear translocation, which resulted in the activation of its downstream genes such as HO-1 and FTH1. Selective inhibition of SIRT1 reversed the protective effects of 24 in the H9c2 cells, suggesting 24 exerts its therapeutic effects againstDICM by inhibiting ferroptosis via SIRT1/Nrf2 signaling pathway activation (Li W. et al., 2021). Salidroside (25), a glucoside of the phenylpropanoid tyrosol isolated from Rhodiola rosea L, is a natural bioactive compound with anti-oxidative, anti-inflammatory, and neuroprotective properties (Zhao et al., 2021; Jin et al., 2022). 25 has cardiovascular benefits against cardiomyopathy (Ni T. et al., 2021; Li Y. et al., 2021). 25 attenuates DICM through inhibiting ferroptosis in vivo and in vitro by limiting iron accumulation, restoring GPX4, and preventing LPO (Chen L. et al., 2022). AMPK inhibitor compound C reversed protective function of 25 against DICM, suggesting that 25 markedly downregulated ferroptosis by activating AMPK-dependent signaling pathways (Chen Z. et al., 2022). Dexazoxane(26) (Zhang Y. et al., 2021), melatonin(27) (Sun et al., 2022), and EGCG(28) (He et al., 2021) also works as a GPX4 activators to attenuates DICM through inhibiting ferroptosis.
While compounds 16d and 16e (29) (Xu et al., 2022), ethoxyquin (30) (Tadokoro et al., 2022), and resveratrol (10) (Yu P. et al., 2022) functions as inhibitors of LPO to attenuates DICM through inhibiting ferroptosis. 29 is two derivatives of steviol, an ent-kaurene diterpenoid, possesses broad-spectrum bioactivity. 29 attenuates DICM in zebrafish cardiomyopathy model through inhibiting ferroptosis via suppressing the GSH depletion, iron accumulation, and LPO, decreasing ROS overproduction, and restoring the mitochondrial membrane potential (Xu et al., 2022). Ethoxyquin (6-ethoxy-1,2-dihydro-2,2,4-trimethylquinoline, 30 is a competent radical-trapping antioxidant. 30 effectively prevented GPX4-deficient ferroptosis in cultured cardiomyocytes, accompanied by the suppression of MDA and mitochondrial lipid peroxides (Tadokoro et al., 2022). 30 ameliorated DICM in vivo through decreasing the levels of lipid peroxides such as MDA and acrolein (Tadokoro et al., 2022). 10 works as a potent p62 activator has potential as a therapeutic target in preventing DICM via inhibiting ferroptosis (Yu W. et al., 2022). The 2-vinyl-10H-phenothiazine derivatives 7j (31) is a new class of ferroptosis inhibitors, maintaining high ferroptosis inhibitory activity (EC50 = 0.001 µM on the erastin-induced HT1080 cell ferroptosis model) (You et al., 2022). 31 acted as a ROS scavenger displayed favorable pharmacokinetic properties and exhibited no obvious toxicity in vivo and vitro and could relieve DICM, providing a promising lead compound for drug discovery targeting ferroptosis to treat DICM(You et al., 2022).
Inhibiting ferroptosis to treat SCM
Compounds that alleviate SCM through inhibiting ferroptosis include vitamin B6 (32) (Shan M. et al., 2021), ferrostatin-1 (33) (Xiao Y. et al., 2021), puerarin (34) (Zhou et al., 2022), H2S (NaHS) (35) (Cao et al., 2022), dexmedetomidine (6) (Wang et al., 2020), resveratrol (10) (Wang H. et al., 2022; Zeng et al., 2023), and attenuate SCM through inhibiting ferroptosis (Table 4).10 alleviate SCM through inhibiting ferroptosis via decreasing LPO, and increasing Sirt1/Nrf2 expression. EX527, a selective Sirt1 inhibitor reversed the protective effect of 10 against ferroptosis (Zeng et al., 2023). This observation was corroborated by other study, which reported 10 upregulated miR-149 and downregulated HMGB1 to inhibit ferroptosis and improve SCM(Wang L. et al., 2022). Vitamin B6 (32) can suppress LPS-induced oxidative stress and LPO that lead to ferroptosis in vivo and in vitro through activating Nrf2. 32 can regulate the expression of iron regulatory proteins, maintaining intracellular iron homeostasis (Shan M. et al., 2021). Ferrostatin-1 (33), a ferroptosis inhibitor, improves SCM through inhibiting ferroptosis (Xiao Y. et al., 2021).33 alleviate SCM through inhibiting ferroptosis via decreasing LPO, PTGS2, ferritin light chain (FTL) and ferritin heavy chain (FTH1), while upregulating GPX4 and ferroportin (FPN, SLC40A1) (Xiao Z. et al., 2021). Compounds puerarin (34) is an isoflavone compound derived from Pueraria lobata in traditional Chinese medicine with cardiovascular benefits against cardiomyopathy (Qin et al., 2016; Li et al., 2017; Yin et al., 2019; Wang et al., 2022d). 34 inhibite SCM induced by LPS through inhibiting ferroptosis via upregulating GPX4 and ferritin and downregulating ACSL4, TfR, and iron content (Zhou et al., 2022).
Conclusions and perspectives
The pathophysiology of cardiomyopathies is complex and still undergoing extensive investigation. In this review, we appraised articles that emphasized research progress in the pathological roles of ferroptosis in ICM, DCM, DICM, and SCM and ferroptosis inhibitors to mitigate cardiomyopathies. Meanwhile, researchers have identified novel targeted treatments for these cardiomyopathies through the pharmacological inhibition of ferroptosis. The pharmacological inhibition of ferroptosis is a potential therapeutic target for these cardiomyopathies, with potential novel drug targets and strategies for these diseases. However, the current research on the role of ferroptosis in cardiomyopathies is still in the infancy, and is still poorly understood. And more studies are required to clarify its role and functional mechanisms. Furthermore, most data reported in the literature are derived from experimental studies that do not directly report clinical applications and implications. Although a phase III clinical trial is underway to determine if resveratrol exert the potential heart benefits of resveratrol in patients with non-ischemic cardiomyopathy (phase III, n = 40, NCT01914081). In addition, a multi-center, randomized, placebo-controlled phase II clinical trial is also being conducted to investigate the LCZ696 in adult patients with non-obstructive hypertrophic cardiomyopathy (nHCM) (phase II, n = 45, NCT04164732). However, there is still lacking the study directly targeting ferroptosis to treat ICM, DCM, DICM, and SCM using bioactive compounds. Therefore, more clinical studies need to be conducted to inform practical treatment and management strategies. Despite these considerations, the current evidence strongly indicates that inhibiting ferroptosis marks a significant new direction for treating cardiomyopathies.
Author contributions
Conception and design: HS and XH; administrative support: All authors; collection and assembly of data: All authors; data analysis and interpretation: All authors; manuscript writing: HS; final approval of manuscript: All authors.
Funding
This work was supported in part by the Natural Science Foundation of Inner Mongolia Autonomous Region (IMAR) (2021MS08131, 2021LHMS08024, 2022MS08046), Science Foundation of Universities of IMAR (NJZY23038), and Key Laboratory of Research on Human Genetic Diseases at Universities of Inner Mongolia Autonomous Region (YC202305, YC202304).
Conflict of interest
The authors declare that the research was conducted in the absence of any commercial or financial relationships that could be construed as a potential conflict of interest.
Publisher’s note
All claims expressed in this article are solely those of the authors and do not necessarily represent those of their affiliated organizations, or those of the publisher, the editors and the reviewers. Any product that may be evaluated in this article, or claim that may be made by its manufacturer, is not guaranteed or endorsed by the publisher.
References
Altamimi, J. Z., BinMowyna, M. N., AlFaris, N. A., Alagal, R. I., El-Kott, A. F., and Al-Farga, A. M. (2021). Fisetin protects against streptozotocin-induced diabetic cardiomyopathy in rats by suppressing fatty acid oxidation and inhibiting protein kinase R. Saudi Pharm. J. 29 (1), 27–42. doi:10.1016/j.jsps.2020.12.003
Althunibat, O. Y., Al Hroob, A. M., Abukhalil, M. H., Germoush, M. O., Bin-Jumah, M., and Mahmoud, A. M. (2019). Fisetin ameliorates oxidative stress, inflammation and apoptosis in diabetic cardiomyopathy. Life Sci. 221, 83–92. doi:10.1016/j.lfs.2019.02.017
Baba, Y., Higa, J. K., Shimada, B. K., Horiuchi, K. M., Suhara, T., Kobayashi, M., et al. (2018). Protective effects of the mechanistic target of rapamycin against excess iron and ferroptosis in cardiomyocytes. Am. J. Physiol. Heart Circ. Physiol. 314, H659–H668. doi:10.1152/ajpheart.00452.2017
Bai, Y. T., Xiao, F. J., Wang, H., Ge, R. L., and Wang, L. S. (2021). Hypoxia protects H9c2 cells against Ferroptosis through SENP1-mediated protein DeSUMOylation. Int. J. Med. Sci. 18, 1618–1627. doi:10.7150/ijms.50804
Baseler, W. A., Dabkowski, E. R., Jagannathan, R., Thapa, D., Nichols, C. E., Shepherd, D. L., et al. (2013). Reversal of mitochondrial proteomic loss in Type 1 diabetic heart with overexpression of phospholipid hydroperoxide glutathione peroxidase. Am. J. Physiol. Regul. Integr. Comp. Physiol. 304, R553–R565. doi:10.1152/ajpregu.00249.2012
Behring, J. B., Kumar, V., Whelan, S. A., Chauhan, P., Siwik, D. A., Costello, C. E., et al. (2014). Does reversible cysteine oxidation link the Western diet to cardiac dysfunction. FASEB J. 28, 1975–1987. doi:10.1096/fj.13-233445
Brieler, J., Breeden, M. A., and Tucker, J. (2017). Cardiomyopathy: An overview. Am. Fam. Physician 96, 640–646.
Bruni, A., Pepper, A. R., Pawlick, R. L., Gala-Lopez, B., Gamble, A. F., Kin, T., et al. (2018). Ferroptosis-inducing agents compromise in vitro human islet viability and function. Cell Death Dis. 9, 595. doi:10.1038/s41419-018-0506-0
Cabac-Pogorevici, I., Muk, B., Rustamova, Y., Kalogeropoulos, A., Tzeis, S., and Vardas, P. (2020). Ischaemic cardiomyopathy. Pathophysiological insights, diagnostic management and the roles of revascularisation and device treatment. Gaps and dilemmas in the era of advanced technology. Eur. J. Heart Fail. 22, 789–799. doi:10.1002/ejhf.1747
Cao, B., Bi, G., and Wang, Y. (2019). Pharmacology of atorvastatin on myocardial ischemia-reperfusion in rats and drug effect analysis. Pak J. Pharm. Sci. 32, 2443–2447.
Cao, G., Zeng, Y., Zhao, Y., Lin, L., Luo, X., Guo, L., et al. (2022). H2S regulation of ferroptosis attenuates sepsis-induced cardiomyopathy. Mol. Med. Rep. 26 (5), 335. doi:10.3892/mmr.2022.12851
Chang, X., Toan, S., Li, R., and Zhou, H. (2022). Therapeutic strategies in ischemic cardiomyopathy: Focus on mitochondrial quality surveillance. EBioMedicine 84, 104260. doi:10.1016/j.ebiom.2022.104260
Chen, H., Zhu, J., Le, Y., Pan, J., Liu, Y., Liu, Z., et al. (2022a). Salidroside inhibits doxorubicin-induced cardiomyopathy by modulating a ferroptosis-dependent pathway. Phytomedicine 99, 153964. doi:10.1016/j.phymed.2022.153964
Chen, H. Y., Xiao, Z. Z., Ling, X., Xu, R. N., Zhu, P., and Zheng, S. Y. (2021a). ELAVL1 is transcriptionally activated by FOXC1 and promotes ferroptosis in myocardial ischemia/reperfusion injury by regulating autophagy. Mol. Med. Camb. Mass 27, 14. doi:10.1186/s10020-021-00271-w
Chen, L., Cai, P., Cheng, Z., Zhang, Z., and Fang, J. (2017). Pharmacological postconditioning with atorvastatin calcium attenuates myocardial ischemia/reperfusion injury in diabetic rats by phosphorylating GSK3β. Exp. Ther. Med. 14, 25–34. doi:10.3892/etm.2017.4457
Chen, L., Yin, Z., Qin, X., Zhu, X., Chen, X., Ding, G., et al. (2022b). CD74 ablation rescues type 2 diabetes mellitus-induced cardiac remodeling and contractile dysfunction through pyroptosis-evoked regulation of ferroptosis. Pharmacol. Res. 176, 106086. doi:10.1016/j.phrs.2022.106086
Chen, X., Kang, R., Kroemer, G., and Tang, D. (2021b). Ferroptosis in infection, inflammation, and immunity. J. Exp. Med. 218, e20210518. doi:10.1084/jem.20210518
Chen, X., Li, J., Kang, R., Klionsky, D. J., and Tang, D. (2021c). Ferroptosis: Machinery and regulation. Autophagy 17, 2054–2081. doi:10.1080/15548627.2020.1810918
Chen, Y., Cao, S., Chen, H., Yin, C., Xu, X., and Yang, Z. (2021d). Dexmedetomidine preconditioning reduces myocardial ischemia-reperfusion injury in rats by inhibiting the PERK pathway. Arq. Bras. Cardiol. 117 (6), 1134–1144. doi:10.36660/abc.20200672
Chen, Y., Hua, Y., Li, X., Arslan, I. M., Zhang, W., and Meng, G. (2020). Distinct types of cell death and the implication in diabetic cardiomyopathy. Front. Pharmacol. 11, 42. doi:10.3389/fphar.2020.00042
Chen, Z., Cao, Z., Gui, F., Zhang, M., Wu, X., Peng, H., et al. (2022c). TMEM43 protects against sepsis-induced cardiac injury via inhibiting ferroptosis in mice. Cells 11. doi:10.3390/cells11192992
Cheng, T., Zhang, Y., Zhang, T., Lu, L., Ding, Y., and Zhao, Y. (2015). Comparative pharmacokinetics study of icariin and icariside II in rats. Molecules 20, 21274–21286. doi:10.3390/molecules201219763
Conrad, M., and Proneth, B. (2019). Broken hearts: Iron overload, ferroptosis and cardiomyopathy. Cell Res. 29, 263–264. doi:10.1038/s41422-019-0150-y
Cui, L., Zhang, Z., Sun, E., Jia, X., and Qian, Q. (2013). Effect of β-cyclodextrin complexation on solubility and enzymatic hydrolysis rate of icariin. J. Nat. Sci. Biol. Med. 4, 201–206. doi:10.4103/0976-9668.107291
Del Buono, M. G., Moroni, F., Montone, R. A., Azzalini, L., Sanna, T., and Abbate, A. (2022). Ischemic cardiomyopathy and heart failure after acute myocardial infarction. Curr. Cardiol. Rep. 24, 1505–1515. doi:10.1007/s11886-022-01766-6
Deng, X., Ye, F., Zeng, L., Luo, W., Tu, S., Wang, X., et al. (2022). Dexmedetomidine mitigates myocardial ischemia/reperfusion-induced mitochondrial apoptosis through targeting lncRNA HCP5. Am. J. Chin. Med. 50 (6), 1529–1551. doi:10.1142/S0192415X22500641
Dixon, S. J., Lemberg, K. M., Lamprecht, M. R., Skouta, R., Zaitsev, E. M., Gleason, C. E., et al. (2012). Ferroptosis: An iron-dependent form of nonapoptotic cell death. Cell 149, 1060–1072. doi:10.1016/j.cell.2012.03.042
Dixon, S. J., Winter, G. E., Musavi, L. S., Lee, E. D., Snijder, B., Rebsamen, M., et al. (2015). Human haploid cell genetics reveals roles for lipid metabolism genes in nonapoptotic cell death. ACS Chem. Biol. 10, 1604–1609. doi:10.1021/acschembio.5b00245
Doll, S., Proneth, B., Tyurina, Y. Y., Panzilius, E., Kobayashi, S., Ingold, I., et al. (2017). ACSL4 dictates ferroptosis sensitivity by shaping cellular lipid composition. Nat. Chem. Biol. 13, 91–98. doi:10.1038/nchembio.2239
Du, S., Shi, H., Xiong, L., Wang, P., and Shi, Y. (2022). Canagliflozin mitigates ferroptosis and improves myocardial oxidative stress in mice with diabetic cardiomyopathy. Front. Endocrinol. (Lausanne) 13, 1011669. doi:10.3389/fendo.2022.1011669
Fan, Z., Cai, L., Wang, S., Wang, J., and Chen, B. (2021). Baicalin prevents myocardial ischemia/reperfusion injury through inhibiting ACSL4 mediated ferroptosis. Front. Pharmacol. 12, 628988. doi:10.3389/fphar.2021.628988
Fang, X., Ardehali, H., Min, J., and Wang, F. (2023). The molecular and metabolic landscape of iron and ferroptosis in cardiovascular disease. Nat. Rev. Cardiol. 20, 7–23. doi:10.1038/s41569-022-00735-4
Fang, X., Wang, H., Han, D., Xie, E., Yang, X., Wei, J., et al. (2019). Ferroptosis as a target for protection against cardiomyopathy. Proc. Natl. Acad. Sci. U.S.A. 116, 2672–2680. doi:10.1073/pnas.1821022116
Feng, Y., Madungwe, N. B., Imam Aliagan, A. D., Tombo, N., and Bopassa, J. C. (2019). Liproxstatin-1 protects the mouse myocardium against ischemia/reperfusion injury by decreasing VDAC1 levels and restoring GPX4 levels. Biochem. Biophys. Res. Commun. 520, 606–611. doi:10.1016/j.bbrc.2019.10.006
Franz, W. M., Müller, O. J., and Katus, H. A. (2001). Cardiomyopathies: From genetics to the prospect of treatment. Lancet 358, 1627–1637. doi:10.1016/S0140-6736(01)06657-0
Gao, M., Monian, P., Pan, Q., Zhang, W., Xiang, J., and Jiang, X. (2016). Ferroptosis is an autophagic cell death process. Cell Res. 26, 1021–1032. doi:10.1038/cr.2016.95
Gao, M., Monian, P., Quadri, N., Ramasamy, R., and Jiang, X. (2015). Glutaminolysis and transferrin regulate ferroptosis. Mol. Cell 59, 298–308. doi:10.1016/j.molcel.2015.06.011
Gilgenkrantz, H., Mallat, A., Moreau, R., and Lotersztajn, S. (2021). Targeting cell-intrinsic metabolism for antifibrotic therapy. J. Hepatol. 74, 1442–1454. doi:10.1016/j.jhep.2021.02.012
Gou, Y., Zheng, X., Li, W., Deng, H., and Qin, S. (2022). Polysaccharides produced by the mushroom trametes robiniophila murr boosts the sensitivity of hepatoma cells to oxaliplatin via the miR-224-5p/ABCB1/P-gp Axis. Integr. Cancer Ther. 21, 15347354221090221. doi:10.1177/15347354221090221
Hadian, K., and Stockwell, B. R. (2020). SnapShot: Ferroptosis. Cell 181 (5), 1188–1188.e1. doi:10.1016/j.cell.2020.04.039
Han, X., Liu, P., Zheng, B., Zhang, M., Zhang, Y., Xue, Y., et al. (2022a). 6-Gingerol exerts a protective effect against hypoxic injury through the p38/Nrf2/HO-1 and p38/NF-κB pathway in H9c2 cells. J. Nutr. Biochem. 104, 108975. doi:10.1016/j.jnutbio.2022.108975
Han, X., Yang, Y., Zhang, M., Chu, X., Zheng, B., Liu, C., et al. (2022b). Protective effects of 6-gingerol on cardiotoxicity induced by arsenic trioxide through AMPK/SIRT1/PGC-1α signaling pathway. Front. Pharmacol. 13, 868393. doi:10.3389/fphar.2022.868393
He, H., Wang, L., Qiao, Y., Yang, B., Yin, D., and He, M. (2021). Epigallocatechin-3-gallate pretreatment alleviates doxorubicin-induced ferroptosis and cardiotoxicity by upregulating AMPKα2 and activating adaptive autophagy. Redox Biol. 48, 102185. doi:10.1016/j.redox.2021.102185
He, Y., Wang, S., Sun, H., Li, Y., and Feng, J. (2022). Naringenin ameliorates myocardial injury in STZ-induced diabetic mice by reducing oxidative stress, inflammation and apoptosis via regulating the Nrf2 and NF-κB signaling pathways. Front. Cardiovasc Med. 9, 946766. doi:10.3389/fcvm.2022.946766
Herrmann, J. (2020). Adverse cardiac effects of cancer therapies: Cardiotoxicity and arrhythmia. Nat. Rev. Cardiol. 17, 474–502. doi:10.1038/s41569-020-0348-1
Hollenberg, S. M., and Singer, M. (2021). Pathophysiology of sepsis-induced cardiomyopathy. Nat. Rev. Cardiol. 18, 424–434. doi:10.1038/s41569-020-00492-2
Hou, W., Xie, Y., Song, X., Sun, X., Lotze, M. T., Zeh, H. J., et al. (2016). Autophagy promotes ferroptosis by degradation of ferritin. Autophagy 12, 1425–1428. doi:10.1080/15548627.2016.1187366
Hu, B., Tian, T., Li, X. T., Hao, P. P., Liu, W. C., Chen, Y. G., et al. (2023). Dexmedetomidine postconditioning attenuates myocardial ischemia/reperfusion injury by activating the Nrf2/Sirt3/SOD2 signaling pathway in the rats. Redox Rep. 28 (1), 2158526. doi:10.1080/13510002.2022.2158526
Huang, Q., Tian, L., Zhao, X., Lei, S., Zhao, B., Qiu, Z., et al. (2022a). Rev-erbs agonist SR9009 alleviates ischemia-reperfusion injury by heightening endogenous cardioprotection at onset of type-2 diabetes in rats: Down-regulating ferritinophagy/ferroptosis signaling. Biomed. Pharmacother. 154, 113595. doi:10.1016/j.biopha.2022.113595
Huang, Y., Zhang, N., Xie, C., You, Y., Guo, L., Ye, F., et al. (2022b). Lipocalin-2 in neutrophils induces ferroptosis in septic cardiac dysfunction via increasing labile iron pool of cardiomyocytes. Front. Cardiovasc Med. 9, 922534. doi:10.3389/fcvm.2022.922534
Ibáñez, B., Heusch, G., Ovize, M., and Van de Werf, F. (2015). Evolving therapies for myocardial ischemia/reperfusion injury. J. Am. Coll. Cardiol. 65, 1454–1471. doi:10.1016/j.jacc.2015.02.032
Jin, M., Wang, C., Xu, Y., Zhang, Z., Wu, X., Ye, R., et al. (2022). Pharmacological effects of salidroside on central nervous system diseases. Biomed. Pharmacother. 156, 113746. doi:10.1016/j.biopha.2022.113746
Jin, X., Zhang, Z. H., Sun, E., and Jia, X. B. (2013). β-cyclodextrin assistant flavonoid glycosides enzymatic hydrolysis. Pharmacogn. Mag. 9, S11–S18. doi:10.4103/0973-1296.117851
Kagan, V. E., Mao, G., Qu, F., Angeli, J. P., Doll, S., Croix, C. S., et al. (2017). Oxidized arachidonic and adrenic PEs navigate cells to ferroptosis. Nat. Chem. Biol. 13, 81–90. doi:10.1038/nchembio.2238
Khan, S., Ahmad, S. S., and Kamal, M. A. (2021). Diabetic cardiomyopathy: From mechanism to management in a nutshell. Endocr. Metab. Immune Disord. Drug Targets 21, 268–281. doi:10.2174/1871530320666200731174724
Kong, C., Ni, X., Wang, Y., Zhang, A., Zhang, Y., Lin, F., et al. (2022). ICA69 aggravates ferroptosis causing septic cardiac dysfunction via STING trafficking. Cell Death Discov. 8, 187. doi:10.1038/s41420-022-00957-y
Krümmel, B., Plötz, T., Jörns, A., Lenzen, S., and Mehmeti, I. (2021). The central role of glutathione peroxidase 4 in the regulation of ferroptosis and its implications for pro-inflammatory cytokine-mediated beta-cell death. Biochim. Biophys. Acta Mol. Basis Dis. 1867, 166114. doi:10.1016/j.bbadis.2021.166114
Lakhal-Littleton, S., Wolna, M., Carr, C. A., Miller, J. J., Christian, H. C., Ball, V., et al. (2015). Cardiac ferroportin regulates cellular iron homeostasis and is important for cardiac function. Proc. Natl. Acad. Sci. U.S.A. 112, 3164–3169. doi:10.1073/pnas.1422373112
Lei, G., Zhuang, L., and Gan, B. (2022). Targeting ferroptosis as a vulnerability in cancer. Nat. Rev. Cancer 22, 381–396. doi:10.1038/s41568-022-00459-0
Li, D., Liu, X., Pi, W., Zhang, Y., Yu, L., Xu, C., et al. (2021a). Fisetin attenuates doxorubicin-induced cardiomyopathy in vivo and in vitro by inhibiting ferroptosis through SIRT1/nrf2 signaling pathway activation. Front. Pharmacol. 12, 808480. doi:10.3389/fphar.2021.808480
Li, D., Pi, W., Sun, Z., Liu, X., and Jiang, J. (2022a). Ferroptosis and its role in cardiomyopathy. Biomed. Pharmacother. = Biomedecine Pharmacother. 153, 113279. doi:10.1016/j.biopha.2022.113279
Li, J. Y., Liu, S. Q., Yao, R. Q., Tian, Y. P., and Yao, Y. M. (2021b). A novel insight into the fate of cardiomyocytes in ischemia-reperfusion injury: From iron metabolism to ferroptosis. Front. Cell Dev. Biol. 9, 799499. doi:10.3389/fcell.2021.799499
Li, N., Wang, W., Zhou, H., Wu, Q., Duan, M., Liu, C., et al. (2020). Ferritinophagy-mediated ferroptosis is involved in sepsis-induced cardiac injury. Free Radic. Biol. Med. 160, 303–318. doi:10.1016/j.freeradbiomed.2020.08.009
Li, T., Tan, Y., Ouyang, S., He, J., and Liu, L. (2022b). Resveratrol protects against myocardial ischemia-reperfusion injury via attenuating ferroptosis. Gene 808, 145968. doi:10.1016/j.gene.2021.145968
Li, W., Lu, M., Zhang, Y., Xia, D., Chen, Z., Wang, L., et al. (2017). Puerarin attenuates the daunorubicin-induced apoptosis of H9c2 cells by activating the PI3K/Akt signaling pathway via the inhibition of Ca2+ influx. Int. J. Mol. Med. 40 (6), 1889–1894. doi:10.3892/ijmm.2017.3186
Li, W., Li, W., Wang, Y., Leng, Y., and Xia, Z. (2021c). Inhibition of DNMT-1 alleviates ferroptosis through NCOA4 mediated ferritinophagy during diabetes myocardial ischemia/reperfusion injury. Cell Death Discov. 7, 267. doi:10.1038/s41420-021-00656-0
Li, Y., Wei, X., Liu, S. L., Zhao, Y., Jin, S., and Yang, X. Y. (2021d). Salidroside protects cardiac function in mice with diabetic cardiomyopathy via activation of mitochondrial biogenesis and SIRT3. Phytother. Res. 35 (8), 4579–4591. doi:10.1002/ptr.7175
Li, Y. P., Wang, S. L., Liu, B., Tang, L., Kuang, R. R., Wang, X. B., et al. (2016). Sulforaphane prevents rat cardiomyocytes from hypoxia/reoxygenation injury in vitro via activating SIRT1 and subsequently inhibiting ER stress. Acta Pharmacol. Sin. 37, 344–353. doi:10.1038/aps.2015.130
Liang, D., Minikes, A. M., and Jiang, X. (2022). Ferroptosis at the intersection of lipid metabolism and cellular signaling. Mol. Cell 82, 2215–2227. doi:10.1016/j.molcel.2022.03.022
Lillo-Moya, J., Rojas-Solé, C., Muñoz-Salamanca, D., Panieri, E., Saso, L., and Rodrigo, R. (2021). Targeting Ferroptosis against Ischemia/Reperfusion Cardiac Injury, 10. doi:10.3390/antiox10050667Antioxidants (Basel, Switz.
Lin, J. H., Yang, K. T., Lee, W. S., Ting, P. C., Luo, Y. P., Lin, D. J., et al. (2022). Xanthohumol protects the rat myocardium against ischemia/reperfusion injury-induced ferroptosis. Oxid. Med. Cell Longev. 2022, 9523491. doi:10.1155/2022/9523491
Lin, J. H., Yang, K. T., Ting, P. C., Luo, Y. P., Lin, D. J., Wang, Y. S., et al. (2021). Gossypol acetic acid attenuates cardiac ischemia/reperfusion injury in rats via an antiferroptotic mechanism. Biomolecules 11 (11), 1667. doi:10.3390/biom11111667
Lin, Q., Hou, S., Dai, Y., and JiangLin, N. Y. (2020). Monascin exhibits neuroprotective effects in rotenone model of Parkinson's disease via antioxidation and anti-neuroinflammation. Neuroreport 31, 637–643. doi:10.1097/WNR.0000000000001467
Liu, H., Mo, H., Yang, C., Mei, X., Song, X., Lu, W., et al. (2022a). A novel function of ATF3 in suppression of ferroptosis in mouse heart suffered ischemia/reperfusion. Free Radic. Biol. Med. 189, 122–135. doi:10.1016/j.freeradbiomed.2022.07.006
Liu, M. Z., Kong, N., Zhang, G. Y., Xu, Q., Xu, Y., Ke, P., et al. (2022b). The critical role of ferritinophagy in human disease. Front. Pharmacol. 13, 933732. doi:10.3389/fphar.2022.933732
Liu, X., Li, D., Pi, W., Wang, B., Xu, S., Yu, L., et al. (2022c). LCZ696 protects against doxorubicin-induced cardiotoxicity by inhibiting ferroptosis via AKT/SIRT3/SOD2 signaling pathway activation. Int. Immunopharmacol. 113 (1), 109379. doi:10.1016/j.intimp.2022.109379
Liu, X., Qi, K., Gong, Y., Long, X., Zhu, S., Lu, F., et al. (2021a). Ferulic acid alleviates myocardial ischemia reperfusion injury via upregulating AMPKα2 expression-mediated ferroptosis depression. J. Cardiovasc Pharmacol. 79, 489–500. doi:10.1097/FJC.0000000000001199
Liu, X. J., Lv, Y. F., Cui, W. Z., Li, Y., Liu, Y., Xue, Y. T., et al. (2021b). Icariin inhibits hypoxia/reoxygenation-induced ferroptosis of cardiomyocytes via regulation of the Nrf2/HO-1 signaling pathway. FEBS Open Bio 11 (11), 2966–2976. doi:10.1002/2211-5463.13276
Liu, Y., Zeng, L., Yang, Y., Chen, C., Wang, D., and Wang, H. (2020). Acyl-CoA thioesterase 1 prevents cardiomyocytes from Doxorubicin-induced ferroptosis via shaping the lipid composition. Cell Death Dis. 11, 756. doi:10.1038/s41419-020-02948-2
Lu, H., Xiao, H., Dai, M., Xue, Y., and Zhao, R. (2022). Temporal stability of parent-reported behavior problems in late talkers over 2 years: A prospective case-control study from toddlerhood to preschool age. Pharm. Biol. 60 (1), 38–45. doi:10.1186/s11689-022-09445-x
Luo, J., Yang, B., Yang, X., Ji, S., Guo, Z., Liu, Y., et al. (2023). Sophorolipid-based microemulsion delivery system: Multifaceted enhancement of physicochemical properties of xanthohumol. Food Chem. 413, 135631. doi:10.1016/j.foodchem.2023.135631
Lutchmansingh, F. K., Hsu, J. W., Bennett, F. I., Badaloo, A. V., McFarlane-Anderson, N., Gordon-Strachan, G. M., et al. (2018). Glutathione metabolism in type 2 diabetes and its relationship with microvascular complications and glycemia. PLoS ONE 13, e0198626. doi:10.1371/journal.pone.0198626
Lv, Z., Wang, F., Zhang, X., Zhang, X., Zhang, J., and Liu, R. (2021). Etomidate attenuates the ferroptosis in myocardial ischemia/reperfusion rat model via Nrf2/HO-1 pathway. Shock 56 (3), 440–449. doi:10.1097/SHK.0000000000001751
Ma, S. Q., Guo, Z., Liu, F. Y., Hasan, S. G., Yang, D., Tang, N., et al. (2021). 6-Gingerol protects against cardiac remodeling by inhibiting the p38 mitogen-activated protein kinase pathway. Acta Pharmacol. Sin. 42 (10), 1575–1586. doi:10.1038/s41401-020-00587-z
Ma, S., Sun, L., Wu, W., Wu, J., Sun, Z., and Ren, J. (2020). USP22 protects against myocardial ischemia-reperfusion injury via the SIRT1-p53/slc7a11-dependent inhibition of ferroptosis-induced cardiomyocyte death. Front. Physiol. 11, 551318. doi:10.3389/fphys.2020.551318
Ma, X., Gao, H., Yang, B., Zhao, H., and Zhu, Z. (2022a). Huaier polysaccharide attenuates doxorubicin-induced acute cardiotoxicity by regulating ferroptosis. Bull. Exp. Biol. Med. 174 (1), 37–42. doi:10.1007/s10517-022-05644-7
Ma, X. H., Liu, J. H., Liu, C. Y., Sun, W. Y., Duan, W. J., Wang, G., et al. (2022b). ALOX15-launched PUFA-phospholipids peroxidation increases the susceptibility of ferroptosis in ischemia-induced myocardial damage. Signal Transduct. Target Ther. 7, 288. doi:10.1038/s41392-022-01090-z
Machado, S. E., Spangler, D., Stacks, D. A., Darley-Usmar, V., Benavides, G. A., Xie, M., et al. (2022). Counteraction of myocardial ferritin heavy chain deficiency by heme oxygenase-1. Int. J. Mol. Sci. 23, 8300. doi:10.3390/ijms23158300
Mancias, J. D., Wang, X., Gygi, S. P., Harper, J. W., and Kimmelman, A. C. (2014). Quantitative proteomics identifies NCOA4 as the cargo receptor mediating ferritinophagy. Nature 509, 105–109. doi:10.1038/nature13148
Maron, B. J., Towbin, J. A., Thiene, G., Antzelevitch, C., Corrado, D., Arnett, D., et al. (2006). Contemporary definitions and classification of the cardiomyopathies: An American heart association scientific statement from the council on clinical cardiology, heart failure and transplantation committee; quality of care and outcomes research and functional genomics and translational biology interdisciplinary working groups; and council on epidemiology and prevention. Circulation 113, 1807–1816. doi:10.1161/CIRCULATIONAHA.106.174287
Moratilla-Rivera, I., Sánchez, M., Valdés-González, J. A., and Gómez-Serranillos, M. P. (2023). Natural products as modulators of Nrf2 signaling pathway in neuroprotection. Int. J. Mol. Sci. 24 (4), 3748. doi:10.3390/ijms24043748
Nabzdyk, C. S., Couture, E. J., Shelton, K., Cudemus, G., and Bittner, E. A. (2019). Sepsis induced cardiomyopathy: Pathophysiology and use of mechanical circulatory support for refractory shock. J. Crit. Care 54, 228–234. doi:10.1016/j.jcrc.2019.09.001
Neumann, H. F., Frank, J., Venturelli, S., and Egert, S. (2022). Bioavailability and cardiometabolic effects of xanthohumol: Evidence from animal and human studies. Mol. Nutr. Food Res. 66 (6), e2100831. doi:10.1002/mnfr.202100831
Ni, J., Li, Y., Xu, Y., and Guo, R. (2021a). Salidroside protects against cardiomyocyte apoptosis and ventricular remodeling by AKT/HO-1 signaling pathways in a diabetic cardiomyopathy mouse model. Phytomedicine 82, 153406. doi:10.1016/j.phymed.2020.153406
Ni, T., Huang, X., Pan, S., and Lu, Z. (2021b). Inhibition of the long non-coding RNA ZFAS1 attenuates ferroptosis by sponging miR-150-5p and activates CCND2 against diabetic cardiomyopathy. J. Cell. Mol. Med. 25, 9995–10007. doi:10.1111/jcmm.16890
Pei, Z., Liu, Y., Liu, S., Jin, W., Luo, Y., Sun, M., et al. (2021). FUNDC1 insufficiency sensitizes high fat diet intake-induced cardiac remodeling and contractile anomaly through ACSL4-mediated ferroptosis. Metab. Clin. Exp. 122, 154840. doi:10.1016/j.metabol.2021.154840
Peng, Y., Liao, B., Zhou, Y., Zeng, W., and Zeng, Z. Y. (2022). Atorvastatin inhibits ferroptosis of H9C2 cells by regulatingSMAD7/hepcidin expression to improve ischemia-reperfusion injury. Cardiol. Res. Pract. 2022, 3972829. doi:10.1155/2022/3972829
Qin, H., Zhang, Y., Wang, R., Du, X., Li, L., and Du, H. (2016). Puerarin suppresses Na+-K+-ATPase-Mediated systemic inflammation and CD36 expression, and alleviates cardiac lipotoxicity in vitro and in vivo. J. Cardiovasc Pharmacol. 68 (6), 465–472. doi:10.1097/FJC.0000000000000431
Ren, B. C., Zhang, Y. F., Liu, S. S., Cheng, X. J., Yang, X., Cui, X. G., et al. (2020). Curcumin alleviates oxidative stress and inhibits apoptosis in diabetic cardiomyopathy via Sirt1-Foxo1 and PI3K-Akt signalling pathways. J. Cell Mol. Med. 24 (21), 12355–12367. doi:10.1111/jcmm.15725
Schultheiss, H. P., Fairweather, D., Caforio, A., Escher, F., Hershberger, R. E., Lipshultz, S. E., et al. (2019). Dilated cardiomyopathy. Nat. Rev. Dis. Prim. 5, 32. doi:10.1038/s41572-019-0084-1
Shan, M., Yu, X., Li, Y., Fu, C., and Zhang, C. (2021a). Vitamin B6 alleviates lipopolysaccharide-induced myocardial injury by ferroptosis and apoptosis regulation. Front. Pharmacol. 12, 766820. doi:10.3389/fphar.2021.766820
Shan, X., Lv, Z. Y., Yin, M. J., Chen, J., Wang, J., and Wu, Q. N. (2021b). The protective effect of cyanidin-3-glucoside on myocardial ischemia-reperfusion injury through ferroptosis. Oxid. Med. Cell Longev. 2021, 8880141. doi:10.1155/2021/8880141
Shu, T., Lv, Z., Xie, Y., Tang, J., and Mao, X. (2019). Hepcidin as a key iron regulator mediates glucotoxicity-induced pancreatic β-cell dysfunction. Endocr. Connect. 8, 150–161. doi:10.1530/EC-18-0516
Silva-Palacios, A., Ostolga-Chavarría, M., Sánchez-Garibay, C., Rojas-Morales, P., Galván-Arzate, S., Buelna-Chontal, M., et al. (2019). Sulforaphane protects from myocardial ischemia-reperfusion damage through the balanced activation of Nrf2/AhR. Free Radic. Biol. Med. 143, 331–340. doi:10.1016/j.freeradbiomed.2019.08.012
Su, J., Wu, T., Cao, S., Pei, J., and Zhao, L. (2023). Screening and characterization of a β-xylosidase from Bifidobacterium breve K-110 and its application in the biotransformation of the total flavonoids of epimedium to icariin with α-l-rhamnosidase. Bioorg Chem. 132, 106364. doi:10.1016/j.bioorg.2023.106364
Su, X., Wang, S., Zhang, H., Yang, G., Bai, Y., Liu, P., et al. (2021). Sulforaphane prevents angiotensin II-induced cardiomyopathy by activation of Nrf2 through epigenetic modification. J. Cell Mol. Med. 25 (9), 4408–4419. doi:10.1111/jcmm.16504
Sumneang, N., Oo, T. T., Singhanat, K., Maneechote, C., Arunsak, B., Nawara, W., et al. (2022). Inhibition of myeloid differentiation factor 2 attenuates cardiometabolic impairments via reducing cardiac mitochondrial dysfunction, inflammation, apoptosis and ferroptosis in prediabetic rats. Biochim. Biophys. Acta Mol. Basis Dis. 1868, 166301. doi:10.1016/j.bbadis.2021.166301
Sun, X., Sun, P., Zhen, D., Xu, X., Yang, L., Fu, D., et al. (2022). Melatonin alleviates doxorubicin-induced mitochondrial oxidative damage and ferroptosis in cardiomyocytes by regulating YAP expression. Toxicol. Appl. Pharmacol. 437, 115902. doi:10.1016/j.taap.2022.115902
Sutanto, H., Hertanto, D. M., Susilo, H., and Wungu, C. (2022). Grapefruit flavonoid naringenin sex-dependently modulates action potential in an in Silico human ventricular cardiomyocyte model. Antioxidants (Basel). 11 (9), 1672. doi:10.3390/antiox11091672
Tadokoro, T., Ikeda, M., Abe, K., Ide, T., Miyamoto, H. D., Furusawa, S., et al. (2022). Ethoxyquin is a competent radical-trapping antioxidant for preventing ferroptosis in doxorubicin cardiotoxicity. J. Cardiovasc Pharmacol. 80 (5), 690–699. doi:10.1097/FJC.0000000000001328
Tadokoro, T., Ikeda, M., Ide, T., Deguchi, H., Ikeda, S., Okabe, K., et al. (2020). Mitochondria-dependent ferroptosis plays a pivotal role in doxorubicin cardiotoxicity. JCI Insight 5, e132747. doi:10.1172/jci.insight.132747
Tan, Y., Zhang, Z., Zheng, C., Wintergerst, K. A., Keller, B. B., and Cai, L. (2020). Mechanisms of diabetic cardiomyopathy and potential therapeutic strategies: Preclinical and clinical evidence. Nat. Rev. Cardiol. 17, 585–607. doi:10.1038/s41569-020-0339-2
Tang, D., and Kroemer, G. (2020). Ferroptosis. Curr. Biol. 30, R1292–R1297. doi:10.1016/j.cub.2020.09.068
Tang, L. J., Luo, X. J., Tu, H., Chen, H., Xiong, X. M., Li, N. S., et al. (2021a). Ferroptosis occurs in phase of reperfusion but not ischemia in rat heart following ischemia or ischemia/reperfusion. Naunyn Schmiedeb. Arch. Pharmacol. 394, 401–410. doi:10.1007/s00210-020-01932-z
Tang, L. J., Zhou, Y. J., Xiong, X. M., Li, N. S., Zhang, J. J., Luo, X. J., et al. (2021b). Ubiquitin-specific protease 7 promotes ferroptosis via activation of the p53/TfR1 pathway in the rat hearts after ischemia/reperfusion. Free Radic. Biol. Med. 162, 339–352. doi:10.1016/j.freeradbiomed.2020.10.307
Tian, H., Xiong, Y., Zhang, Y., Leng, Y., Tao, J., Li, L., et al. (2021a). Activation of NRF2/FPN1 pathway attenuates myocardial ischemia-reperfusion injury in diabetic rats by regulating iron homeostasis and ferroptosis. Cell Stress Chaperones 27, 149–164. doi:10.1007/s12192-022-01257-1
Tian, Y., Wu, J., Zeng, L., Zhou, L., Hu, Y., Pan, Q., et al. (2021b). Huaier polysaccharides suppress triple-negative breast cancer metastasis and epithelial-mesenchymal transition by inducing autophagic degradation of Snail. Cell Biosci. 11 (1), 170. doi:10.1186/s13578-021-00682-6
Tu, H., Zhou, Y. J., Tang, L. J., Xiong, X. M., Zhang, X. J., Ali Sheikh, M. S., et al. (2021). Combination of ponatinib with deferoxamine synergistically mitigates ischemic heart injury via simultaneous prevention of necroptosis and ferroptosis. Eur. J. Pharmacol. 898, 173999. doi:10.1016/j.ejphar.2021.173999
Vicente de Andrade Silva, G., Demaman Arend, G., Antonio Ferreira Zielinski, A., Di Luccio, M., and Ambrosi, A. (2023). Xanthohumol properties and strategies for extraction from hops and brewery residues: A review. Food Chem. 404 (2), 134629. doi:10.1016/j.foodchem.2022.134629
Wang, C., Yuan, W., Hu, A., Lin, J., Xia, Z., Yang, C. F., et al. (2020). Dexmedetomidine alleviated sepsis-induced myocardial ferroptosis and septic heart injury. Mol. Med. Rep. 22, 175–184. doi:10.3892/mmr.2020.11114
Wang, D., Bu, T., Li, Y., He, Y., Yang, F., and Zou, L. (2022a). Pharmacological activity, pharmacokinetics, and clinical research progress of puerarin. Antioxidants (Basel) 11 (11), 2121. doi:10.3390/antiox11112121
Wang, H., Yang, G., Tian, Y., Li, J., Meng, L., Jiang, X., et al. (2022b). Sulforaphane inhibits angiotensin II-induced cardiomyocyte apoptosis by acetylation modification of Nrf2. Aging (Albany NY) 14 (16), 6740–6755. doi:10.18632/aging.204247
Wang, L., Wang, S., Jia, T., Sun, X., Xing, Z., Liu, H., et al. (2022c). Dexmedetomidine prevents cardiomyocytes from hypoxia/reoxygenation injury via modulating tetmethylcytosine dioxygenase 1-mediated DNA demethylation of Sirtuin1. Bioengineered 13 (4), 9369–9386. doi:10.1080/21655979.2022.2054762
Wang, X., Chen, X., Zhou, W., Men, H., Bao, T., Sun, Y., et al. (2022d). Ferroptosis is essential for diabetic cardiomyopathy and is prevented by sulforaphane via AMPK/NRF2 pathways. Acta Pharm. Sin. B 12, 708–722. doi:10.1016/j.apsb.2021.10.005
Wang, X., Simayi, A., Fu, J., Zhao, X., and Xu, G. (2022e). Resveratrol mediates the miR-149/HMGB1 axis and regulates the ferroptosis pathway to protect myocardium in endotoxemia mice. Am. J. Physiol. Endocrinol. Metab. 323, E21–E32. doi:10.1152/ajpendo.00227.2021
Wang, Y., Shang, C., Zhang, Y., Xin, L., Jiao, L., Xiang, M., et al. (2023). Regulatory mechanism of icariin in cardiovascular and neurological diseases. Biomed. Pharmacother. = Biomedecine Pharmacother. 158, 114156. doi:10.1016/j.biopha.2022.114156
Wang, Z., Yao, M., Jiang, L., Wang, L., Yang, Y., Wang, Q., et al. (2022f). Dexmedetomidine attenuates myocardial ischemia/reperfusion-induced ferroptosis via AMPK/GSK-3β/Nrf2 axis. Biomed. Pharmacother. 154, 113572. doi:10.1016/j.biopha.2022.113572
Wei, D. Z., Li, D., Zheng, D. M., An, Z. N., Xing, X. J., Jiang, D. W., et al. (2021). Curcumin conjugated gold nanoclusters as perspective therapeutics for diabetic cardiomyopathy. Front. Chem. 9, 763892. doi:10.3389/fchem.2021.763892
Wei, J., Zhao, Y., Liang, H., Du, W., and Wang, L. (2022a). Preliminary evidence for the presence of multiple forms of cell death in diabetes cardiomyopathy. Acta Pharm. Sin. B 12, 1–17. doi:10.1016/j.apsb.2021.08.026
Wei, L. Y., Zhang, J. K., Zheng, L., and Chen, Y. (2022b). The functional role of sulforaphane in intestinal inflammation: A review. Food Funct. 13 (2), 514–529. doi:10.1039/d1fo03398k
Wei, Z., Shaohuan, Q., Pinfang, K., and Chao, S. (2022c). Curcumin attenuates ferroptosis-induced myocardial injury in diabetic cardiomyopathy through the Nrf2 pathway. Cardiovasc Ther. 2022, 3159717. doi:10.1155/2022/3159717
Wu, G., Zhu, L., Yuan, X., Chen, H., Xiong, R., Zhang, S., et al. (2017). Britanin ameliorates cerebral ischemia-reperfusion injury by inducing the Nrf2 protective pathway. Antioxid. Redox Signal 27 (11), 754–768. doi:10.1089/ars.2016.6885
Wu, S., Zhu, J., Wu, G., Hu, Z., Ying, P., Bao, Z., et al. (2022c). 6-Gingerol alleviates ferroptosis and inflammation of diabetic cardiomyopathy via the Nrf2/HO-1 pathway. Oxid. Med. Cell Longev. 2022, 3027514. doi:10.1155/2022/3027514
Wu, W., Du, Z., and Wu, L. (2022a). Dexmedetomidine attenuates hypoxia-induced cardiomyocyte injury by promoting telomere/telomerase activity: Possible involvement of ERK1/2-Nrf2 signaling pathway. Cell Biol. Int. 46 (7), 1036–1046. doi:10.1002/cbin.11799
Wu, X., Huang, L., Zhou, X., and Liu, J. (2020). Curcumin protects cardiomyopathy damage through inhibiting the production of reactive oxygen species in type 2 diabetic mice. Biochem. Biophys. Res. Commun. 530 (1), 15–21. doi:10.1016/j.bbrc.2020.05.053
Wu, X., Zhou, X., Lai, S., Liu, J., and Qi, J. (2022b). Curcumin activates Nrf2/HO-1 signaling to relieve diabetic cardiomyopathy injury by reducing ROS in vitro and in vivo. FASEB J. 36 (9), e22505. doi:10.1096/fj.202200543RRR
Xiao, Y., Li, J., Qiu, L., Jiang, C., Huang, Y., Liu, J., et al. (2021a). Dexmedetomidine protects human cardiomyocytes against ischemia-reperfusion injury through α2-adrenergic receptor/AMPK-dependent autophagy. Front. Pharmacol. 12, 615424. doi:10.3389/fphar.2021.615424
Xiao, Z., Kong, B., Fang, J., Qin, T., Dai, C., Shuai, W., et al. (2021b). Ferrostatin-1 alleviates lipopolysaccharide-induced cardiac dysfunction. Bioengineered 12, 9367–9376. doi:10.1080/21655979.2021.2001913
Xie, Y., Zhu, S., Song, X., Sun, X., Fan, Y., Liu, J., et al. (2017). The tumor suppressor p53 limits ferroptosis by blocking DPP4 activity. Cell Rep. 20, 1692–1704. doi:10.1016/j.celrep.2017.07.055
Xin, Y., Bai, Y., Jiang, X., Zhou, S., Wang, Y., Wintergerst, K. A., et al. (2018). Sulforaphane prevents angiotensin II-induced cardiomyopathy by activation of Nrf2 via stimulating the Akt/GSK-3ß/Fyn pathway. Redox Biol. 15, 405–417. doi:10.1016/j.redox.2017.12.016
Xu, C., Ou, E., Li, Z., Chen, Z., Jia, Q., Xu, X., et al. (2022). Synthesis and in vivo evaluation of new steviol derivatives that protect against cardiomyopathy by inhibiting ferroptosis. Bioorg Chem. 129, 106142. doi:10.1016/j.bioorg.2022.106142
Xu, G., Zhao, X., Fu, J., and Wang, X. (2019). Resveratrol increase myocardial Nrf2 expression in type 2 diabetic rats and alleviate myocardial ischemia/reperfusion injury (MIRI). Ann. Palliat. Med. 8 (5), 565–575. doi:10.21037/apm.2019.11.25
Xu, S., Wu, B., Zhong, B., Lin, L., Ding, Y., Jin, X., et al. (2021). Naringenin alleviates myocardial ischemia/reperfusion injury by regulating the nuclear factor-erythroid factor 2-related factor 2 (Nrf2)/System xc-/glutathione peroxidase 4 (GPX4) axis to inhibit ferroptosis. Bioengineered 12 (2), 10924–10934. doi:10.1080/21655979.2021.1995994
Yang, C., Zhao, D., Liu, G., Zheng, H., Yang, H., Yang, S., et al. (2018). Atorvastatin attenuates metabolic remodeling in ischemic myocardium through the downregulation of UCP2 expression. Int. J. Med. Sci. 15, 517–527. doi:10.7150/ijms.22454
Yang, F. Y., Zhang, L., Zheng, Y., and Dong, H. (2022). Dexmedetomidine attenuates ischemia and reperfusion-induced cardiomyocyte injury through p53 and forkhead box O3a (FOXO3a)/p53-upregulated modulator of apoptosis (PUMA) signaling signaling. Bioengineered 13 (1), 1377–1387. doi:10.1080/21655979.2021.2017611
Ye, G., Wang, M., Liu, D., Cheng, L., Yin, X., Zhang, Q., et al. (2020). Mechanism of naringenin blocking the protection of LTB4/BLT1 receptor against septic cardiac dysfunction. Ann. Clin. Lab. Sci. 50 (6), 769–774.
Yin, M. S., Zhang, Y. C., Xu, S. H., Liu, J. J., Sun, X. H., Liang, C., et al. (2019). Puerarin prevents diabetic cardiomyopathy in vivo and in vitro by inhibition of inflammation. J. Asian Nat. Prod. Res. 21 (5), 476–493. doi:10.1080/10286020.2017.1405941
You, J., Yang, W., Ma, R., Xia, A., Zhang, G., Fang, Z., et al. (2022). Discovery of 2-vinyl-10H-phenothiazine derivatives as a class of ferroptosis inhibitors with minimal human Ether-a-go-go related gene (hERG) activity for the treatment of DOX-induced cardiomyopathy. Bioorg Med. Chem. Lett. 74, 128911. doi:10.1016/j.bmcl.2022.128911
Yu, L. M., Dong, X., Xue, X. D., Zhang, J., Li, Z., Wu, H. J., et al. (2019). Naringenin improves mitochondrial function and reduces cardiac damage following ischemia-reperfusion injury: The role of the AMPK-SIRT3 signaling pathway. Food Funct. 10 (5), 2752–2765. doi:10.1039/c9fo00001a
Yu, P., Zhang, J., Ding, Y., Chen, D., Sun, H., Yuan, F., et al. (2022a). Dexmedetomidine post-conditioning alleviates myocardial ischemia-reperfusion injury in rats by ferroptosis inhibition via SLC7A11/GPX4 axis activation. Hum. Cell 35 (3), 836–848. doi:10.1007/s13577-022-00682-9
Yu, W., Chen, C., Xu, C., Xie, D., Wang, Q., Liu, W., et al. (2022b). Activation of p62-NRF2 Axis protects against doxorubicin-induced ferroptosis in cardiomyocytes: A novel role and molecular mechanism of resveratrol. Am. J. Chin. Med. 50 (8), 2103–2123. doi:10.1142/S0192415X22500902
Zeng, Y., Cao, G., Lin, L., Zhang, Y., Luo, X., Ma, X., et al. (2023). Resveratrol attenuates sepsis-induced cardiomyopathy in rats through anti-ferroptosis via the sirt1/nrf2 pathway. J. Invest Surg. 36, 2157521. doi:10.1080/08941939.2022.2157521
Zhang, H., Wang, Z., Liu, Z., Du, K., and Lu, X. (2021a). Protective effects of dexazoxane on rat ferroptosis in doxorubicin-induced cardiomyopathy through regulating HMGB1. Front. Cardiovasc Med. 8, 685434. doi:10.3389/fcvm.2021.685434
Zhang, W., Liu, X., Jiang, Y., Wang, N., Li, F., and Xin, H. (2019). 6-Gingerol attenuates ischemia-reperfusion-induced cell apoptosis in human AC16 cardiomyocytes through HMGB2-JNK1/2-NF-κb pathway. Evid. Based Complement. Altern. Med. 2019, 8798653. doi:10.1155/2019/8798653
Zhang, W., Lu, J., Wang, Y., Sun, P., Gao, T., Xu, N., et al. (2023). Canagliflozin attenuates lipotoxicity in cardiomyocytes by inhibiting inflammation and ferroptosis through activating AMPK pathway. Int. J. Mol. Sci. 24 (1), 858. doi:10.3390/ijms24010858
Zhang, Y., Ren, X., Wang, Y., Chen, D., Jiang, L., Li, X., et al. (2021b). Targeting ferroptosis by polydopamine nanoparticles protects heart against ischemia/reperfusion injury. ACS Appl. Mater Interfaces 13 (45), 53671–53682. doi:10.1021/acsami.1c18061
Zhao, C. C., Wu, X. Y., Yi, H., Chen, R., and Fan, G. (2021). The therapeutic effects and mechanisms of salidroside on cardiovascular and metabolic diseases: An updated review. Chem. Biodivers. 18 (7), e2100033. doi:10.1002/cbdv.202100033
Zheng, W., Li, X., Zhang, T., and Wang, J. (2022). Biological mechanisms and clinical efficacy of sulforaphane for mental disorders. Gen. Psychiatr. 35, e100700. doi:10.1136/gpsych-2021-100700
Zhou, B., Zhang, J., Chen, Y., Liu, Y., Tang, X., Xia, P., et al. (2022). Puerarin protects against sepsis-induced myocardial injury through AMPK-mediated ferroptosis signaling. Aging (Albany NY) 14, 3617–3632. doi:10.18632/aging.204033
Zhou, K., Yang, S., and Li, S. M. (2021). Naturally occurring prenylated chalcones from plants: Structural diversity, distribution, activities and biosynthesis. Nat. Prod. Rep. 38 (12), 2236–2260. doi:10.1039/d0np00083c
Zhou, S., Wang, J., Yin, X., Xin, Y., Zhang, Z., Cui, T., et al. (2018). Nrf2 expression and function, but not MT expression, is indispensable for sulforaphane-mediated protection against intermittent hypoxia-induced cardiomyopathy in mice. Redox Biol. 19, 11–21. doi:10.1016/j.redox.2018.07.014
Zou, Y., Li, H., Graham, E. T., Deik, A. A., Eaton, J. K., Wang, W., et al. (2020). Cytochrome P450 oxidoreductase contributes to phospholipid peroxidation in ferroptosis. Nat. Chem. Biol. 16, 302–309. doi:10.1038/s41589-020-0472-6
Keywords: cardiomyopathies, ferroptosis, ferroptosis inhibitor, treatment, bioactive compounds
Citation: Sun H, Chen D, Xin W, Ren L, LI Q and Han X (2023) Targeting ferroptosis as a promising therapeutic strategy to treat cardiomyopathy. Front. Pharmacol. 14:1146651. doi: 10.3389/fphar.2023.1146651
Received: 17 January 2023; Accepted: 05 April 2023;
Published: 13 April 2023.
Edited by:
Shusheng Wang, Tulane University, United StatesReviewed by:
Yao Tong, Tulane University, United StatesYafeng Li, The Fifth Hospital of Shanxi Medical University, China
Copyright © 2023 Sun, Chen, Xin, Ren, LI and Han. This is an open-access article distributed under the terms of the Creative Commons Attribution License (CC BY). The use, distribution or reproduction in other forums is permitted, provided the original author(s) and the copyright owner(s) are credited and that the original publication in this journal is cited, in accordance with accepted academic practice. No use, distribution or reproduction is permitted which does not comply with these terms.
*Correspondence: Qiang LI, liqiang1980_1981@126.com; Xuchen Han, hanxuchen2004@163.com
†These authors have contributed equally to this work