Corrigendum: Polyphyllin I suppresses the gastric cancer growth by promoting cancer cell ferroptosis
- 1Section of Immunology, The Second Affiliated Hospital of Guangzhou University of Chinese Medicine, Guangzhou, Guangdong, China
- 2Joint Immunology Program, Guangdong Provincial Academy of Chinese Medical Sciences, Guangzhou, Guangdong, China
Background: Ferroptosis is a new form of regulated cell death characterized by the accumulation of iron-dependent lipid peroxides and membrane damages. Recent studies have identified an important role for cancer cell ferroptosis in antitumor therapy. On the other hand, polyphyllin I (PPI) has been reported to exert antitumor effects on some types of cancers. However, it remains unknown whether or not PPI regulates cancer cell ferroptosis.
Methods: Two types of human gastric cancer cells (AGS and MKN-45) were used to establish tumor xenograft models in nude mice that were treated with polyphyllin I (PPI) to observe tumor growth, while cells also were cultured for in vitro studies. Ferroptosis, based on the intracellular ROS/lipid ROS production and accumulation of ferrous ions, was detected using a fluorescence microscope and flow cytometer, while the expression of NRF2/FTH1 was measured using Western blotting assays.
Results: Here we found that PPI inhibited the gastric cancer growth in vivo and in vitro while increasing the intracellular reactive oxygen species (ROS)/lipid peroxides and ferrous ions in the gastric cancer cells. PPI also decreased the levels of nuclear factor erythroid 2-related factor 2 (NRF2) and ferritin heavy chain 1 (FTH1) in gastric cancer cells in vitro. Moreover, liproxstain-1, an inhibitor of cell ferroptosis, mostly reversed the cell ferroptosis and tumor growth arrest induced by PPI. Finally, the effects of PPI on cancer cell ferroptosis were diminished by the overexpression of NRF2.
Conclusion: For the first time, our results have demonstrated that PPI exerts its antitumor activity on the gastric cancer by, at least partially, inducing cancer cell ferroptosis via regulating NRF2/FTH1 pathway. These findings may be implicated for clinical replacement therapy of the gastric cancer.
Introduction
As a new form of regulated cell death distinct from conventional apoptosis, ferroptosis is caused by iron-dependent lipid peroxidation and subsequent cell membrane damage (Dixon et al., 2012). Increased iron accumulation, free radical production, and lipid peroxidation are critical for the induction of ferroptosis (Dixon et al., 2012; Tang et al., 2021). Although the links between ferroptosis and carcinogenesis and its roles in anticancer therapy have been extensively studied recently, the molecular mechanisms underlying ferroptosis remain poorly understood. Previous studies have revealed that ferroptosis plays a vital role in tumor occurrence and that abundant cell ferroptosis can inhibit tumor progression (Lei et al., 2022). Ferroptosis-inducing drugs may lead to cancer cell death or tumor growth arrest and affect the efficacy of chemotherapy, radiotherapy, targeted therapy, and even immunotherapy (Chen et al., 2021a; Zhang et al., 2022; Zhao et al., 2022). Therefore, agents targeting ferroptosis pathway could provide new a therapeutic strategy for treating various cancers.
Polyphyllin I (PPI), a natural ingredient extracted from the root of Paris polyphylla, has been shown to exert antitumor effects on various cancers, including the gastric cancer (Tian et al., 2020a). Polyphyllin series, including PPI, can be derived from Rhizoma paridis and are also anti-tumorous (Li et al., 2023). Mechanistically, PPI activated the AMPK or p21/CDK2/Rb pathway to inhibit the growth of non-small cell lung cancer (Wu et al., 2020; Shen et al., 2021), suppressed the tumoral angiogenesis through the Twist1/VE-cadherin pathway, and induced apoptosis in hepatocellular carcinoma (Shi et al., 2015; Xiao et al., 2018; Zeng et al., 2020). Furthermore, it induced autophagy and apoptosis in the gastric cancer and colon cancer cells by inhibiting the PDK1/Akt/mTOR signaling (He et al., 2019; Luo et al., 2022). The mechanisms underlying antitumor effects of PPI are associated with the inhibition of cell proliferation and invasion, induction of cell cycle arrest and apoptosis, promotion of autophagy, and enhancement of tumor sensitivity to the chemotherapy or other targeted therapy. The transcriptional and signal pathways initiated by PPI may include STAT3, AMPK, FOXO3 and PI3K/Akt, etc. (Han et al., 2015; Li et al., 2016; Lou et al., 2017; Liu et al., 2018; Han et al., 2020; Li et al., 2020; Lai et al., 2021). However, there has been no any report on a potential impact of PPI on cancer cell ferroptosis.
In this study, we investigated the effects of PPI on the gastric cancer using two human cancer cell lines, AGS and MKN-45. Indeed, we found that PPI inhibited the gastric cancer growth in vitro and in vivo. Importantly, for the first time, we demonstrated that PPI induced gastric cancer cell ferroptosis, as evidenced by the accumulation of ROS/lipid peroxides and enrichment of ferrous ions in the gastric cancer cells treated with PPI. Further studies revealed that NRF2/FTH1 pathways played an important role in PPI-induced cell ferroptosis in the gastric cancer. These findings suggest that PPI may serve as a novel inducer of ferroptosis for a replacement therapy of the gastric cancer.
Materials and methods
Animals, tumor models and treatment of mice
BALB/c nude mice (female, 6-8 weeks old, body weight 18-20 g) were obtained from Experimental Animal Center of Guangdong Province (Guangzhou, China). The mice were housed in a specific pathogen-free (SPF) animal facility with controlled conditions. All animal experiments complied with the National Institutes of Health guides for the care and use of laboratory animals (NIH Publications No. 8023, revised 1978), while animal protocols were approved by the Institutional Animal Care and Use Committee of the Second Affiliated Hospital of Guangzhou University of Chinese Medicine. At the end of experiments, mice were euthanized using overdoses of Pelltobarbitalum natricum.
A subcutaneous gastric tumor model was established by subcutaneously injecting 1×106 AGS cells or 2×106 MKN-45 cells near the right axilla of mice. Seven days after tumor cell inoculation, mice received daily i. p. Injection of PPI (3 mg/kg, dissolved in 1% DMSO +5% PEG300 + 5% Tween 80 + 89% deionized water), as described previously [26], or the control solution with the same solvent. Mice were weighed at day 15, while tumor volumes were measured every 3 days and calculated using a formula: length X width 2/2.
Furthermore, for the experiments with liproxstain-1 that blocks ferroptosis, 1×106 AGS cells were subcutaneously injected into BALB/c nude mice. Mice then were randomly divided into 4 groups (6 mice/group): controls, PPI (3 mg/kg), Liproxstain-1 (30 mg/kg), and both (i.p. daily). Similarly, the tumor volumes were measured every 2-3 days.
Drugs and reagents
Polyphyllin I (PPI) was purchased from Meilun Biotechnology Co., Ltd. (Lot number: MB7074, Dalian, China, purity>98%), while Liproxstatin-1 was obtained from Selleck Chemical (Lot#: S7699, Houston, United States). 3-(4,5-Dimethylthiazol-2-yl)-2,5-diphenyltetrazolium bromide (MTT) powder was a product from Sigma Aldrich (St. Louis, United States). Monoclonal antibodies specific for NRF2 and FTH1 were purchased from Cell Signaling Technology Inc. (Danvers, United States) and Abcam (China Branch, Shanghai, China), respectively.
Cell lines and cultures
Human gastric cancer cell line, MKN-45, was provided by Chinese Academy of Sciences Cell Bank of Type Culture Collection (Shanghai, China), while AGS human cell line was purchased from American Type Culture Collection (ATCC, Manassas, United States). These cells were cultured in RPMI-1640 medium (Gibco, United States), supplemented with 10% (v/v) fetal bovine serum (Hyclone, United States), 100 μg/mL streptomycin and 100 U/mL penicillin (Gibco), in a humidified incubator containing 5% CO2 at 37°C. Cells with passage numbers of 15–20 were used for all experiments.
MTT assays evaluating cell growth or expansion in vitro
Cell growth or expansion was tested using the 3-(4, 5-dimethylthiazol-2-yl)-2, 5- diphenyltetrazolium bromide (MTT) dye reduction methods. After the culture with PPI, the cells were incubated with the medium containing 10% MTT solution (5 mg/mL in PBS) at 37°C for 4 h. The absorption was measured at 570 nm using a microplate reader (Perkin Elmer, Victor X5, United States). The cell viability (%) was calculated based on a formula: (absorbance of test sample/absorbance of control) ×100%.
Determination of intracellular levels of ROS and lipid peroxidation
To measure ROS production, we labeled AGS and MKN-45 cells with a DCFH-DA fluorescent probe (MCE, United States). Briefly, cells were washed after treatment with PPI and stained with 1 mL of DCFH-DA (10 μM, in HBSS) at 37°C for 30 min. Cells were then washed and re-suspended in HBSS. The fluorescence intensity (MFI) of DCFH-DA was measured using a flow cytometer (ACEA, Novo Quanteon, United States).
To measure lipid-ROS levels, a C11-BODIPY fluorescent probe (ThermoFisher, United States) was used to determine lipid peroxidation. After the treatment, cells were incubated in HBSS containing 5 μM C11-BODIPY at 37°C for 30 min and harvested for flow cytometric analyses. Mean fluorescence intensity (MFI) was used to represent lipid-ROS levels.
Detection of intracellular Fe2+ ions
To measure the levels of intracellular Fe2+ ions, we used fluorescent probes to label Fe2+ in cells. Briefly, AGS and MKN-45 cells were seeded in 48-well and 6-well plates, respectively, at a density of 1 × 105 cells/mL and incubated overnight. After different treatments, cells were then washed and incubated in HBSS solution containing FerroOrange (Dojindo, Japan) with a concentration of 1 μM at 37°C in a 5% CO2 incubator for 30 min. Cells were then observed under an inverted fluorescence microscope, with fluorescence images acquired immediately. Additionally, cells were harvested and resuspended in HBSS for flow cytometric analyses to further quantify intracellular Fe2+ ion levels.
Western blotting
We determined the expression of FTH1 and NRF2 proteins via Western blot analyses. Briefly, protein lysates were prepared from cultured cells or mouse tumor tissue using an ice-cold cell lysis buffer (ThermoFisher, United States) supplemented with a protease inhibitor cocktail (Roche, Switzerland). Equal amounts of proteins were separated by 12% SDS polyacrylamide gels using a vertical electrophoresis system (Bio-Rad, United States), and then proteins were transferred to PVDF membranes (Millipore, United States) using semi-dry transfer (Bio-Rad). After blocking with 5% BSA, the membranes were incubated with primary antibodies, including FTH1 (CST, 1:1000), NRF2 (Abcam, 1:1000) or GAPDH (Abcam, 1:10000), at 4°C overnight, followed by incubation with secondary antibodies for 1 hour at room temperature. Protein levels were detected using ChemiDoc XRS + Imagine System (Bio-Rad, United States), while data were analyzed using the Image Lab software.
Statistical analysis
Statistical analyses were carried out using GraphPad Prism 8.0 software (La Jolla, CA, United States). All data were analyzed using the unpaired t-test or One-way ANOVA. Asterisks shown in the figures indicate significant differences in comparisons of experimental groups with the corresponding controls. The statistical significance was assumed at a value of p < 0.05.
Results
PPI inhibits the gastric cancer growth
Here, we tested the efficacy of PPI on the gastric cancer using two xenograft tumor models. AGS and MKN-45 cancer cells were subcutaneously injected into the nude mice. Tumor-bearing mice were treated with PPI. We then assessed the tumor volumes and weights of the mice (Figure 1). We found that the AGS tumor weight of PPI-treated mice was significantly reduced compared to that of the control group 15 days after inoculation (Figures 1A, B). Mice treated with PPI also exhibited smaller AGS tumor volumes than did the control group on days 12 and 15, respectively (Figure 1C). Similar findings were observed in MKN-45 tumors (Figures 1D–F). These findings confirmed that PPI indeed inhibits the gastric cancer growth.
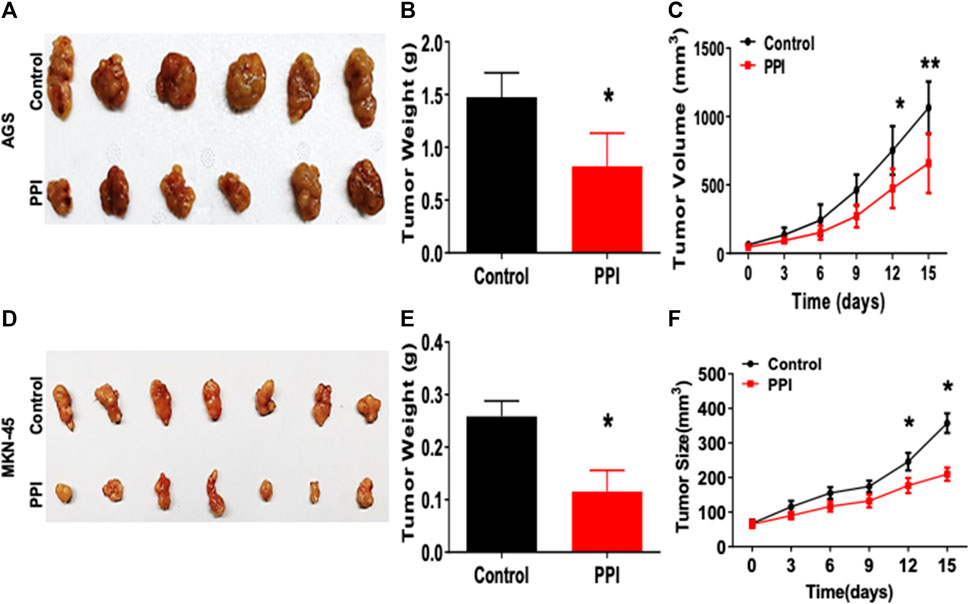
FIGURE 1. PPI inhibits the tumor growth of AGS and MKN-45 cells in BALB/c nude mice. BALB/c nude mice were injected subcutaneously with 1 × 106 AGS or 2 × 106 MKN-45 human tumor cells. One week after inoculation, mice were administrated with the solvent (Control) or PPI (3 mg/kg/day) for up to 15 days. (A) Tumor xenografts (AGS) were harvested at day 15. Shown are photos of the tumors derived from control vehicle- and PPI-treated mice. (B) Then, the tumor weights were also measured at day 15. (C) The tumor volumes were determined at various time points. (D–F) Similarly, Tumors (MKN-45) also were harvested for taking tumor photos (D) and measuring tumor weights (E) as well as tumor volumes (F). Data are presented as mean ± SD (n = 6 mice/group) and analyzed using the unpaired-t test (*p < 0.05).
PPI inhibits the growth of gastric cancer cells in vitro and induces their ferroptosis
Previous reports showed that PPI inhibited the tumor growth via several mechanisms (Dong et al., 2018; Zhang et al., 2018; Han et al., 2020). In this study, we first confirmed that PPI indeed suppressed both AGS and MKN-45 cancer cell growth in vitro in a dose-dependent manner based on MTT assays (Figure 2A, B). We then determined the effects of PPI on the levels of intracellular reactive oxygen species (ROS), lipid peroxidation and ferrous ions in the gastric cancer cells 24 h after cell culture. Our data showed that the intracellular levels of both ROS and Lipid-ROS in the gastric cancer cells treated with PPI were significantly increased compared to the control group (Figures 2C, D). In addition, using a flow cytometer, we revealed that PPI increased the levels of ferrous ions in both AGS and MKN-45 cells (Figure 2E). These findings suggest that PPI promotes the gastric cancer cell ferroptosis.
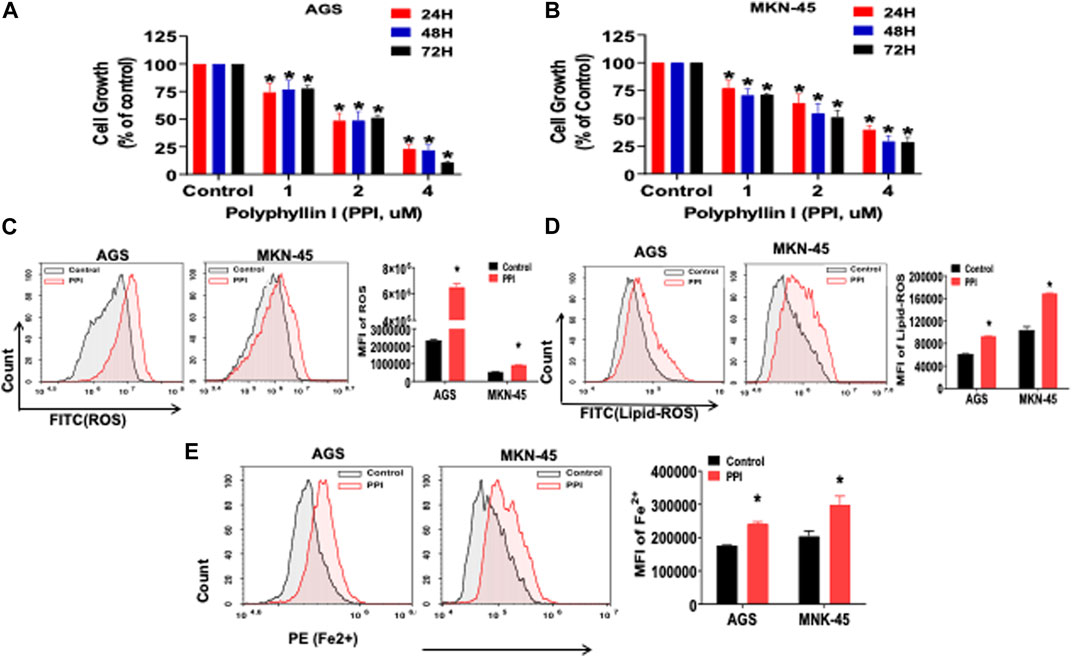
FIGURE 2. PPI suppresses cell growth and induces ferroptosis in the gastric cancer cells. (A, B) The cell growth (expansion) of AGS and MKN-45 cells after treatment with PPI (0, 1, 2, 3, 4 μM, for 24, 48 and 72 h, respectively) was examined using MTT assays. (C, D) The levels of cellular ROS and lipid peroxides (lipid-ROS) after the treatment with PPI (3 μM) for 24 h in AGS and MKN-45 cells were analyzed using a flow cytometer. (E) The levels of intracellular ferrous ions (Fe2+) in AGS and MKN-45 cells after PPI treatment for 24 h were quantified using a flow cytometer. Data are shown as mean ± SD while p values were determined using one-way ANOVA (*p < 0.05).
A ferroptosis inhibitor largely diminishes the effects of PPI on gastric cancer cell growth and ferroptosis in vitro
Ferroptosis is mainly characterized by the accumulation of iron-dependent lipid peroxides, which can be reversed by ferroptosis inhibitors (Li et al., 2021). A specific ferroptosis inhibitor, Liproxstain-1, was used to confirm whether the anticancer effects of PPI were mediated partially by its induction of cell ferroptosis. We found that Liproxstain-1 largely abolished the effects of PPI on cell growth in both AGS and MKN-45 cells (Figure 3A). Furthermore, PPI increased the intracellular ROS and Lipid-ROS levels in the cancer cells whereas these effects of PPI were largely blocked by Liproxstain-1 (Figure 3B, C). In addition, Liproxstain-1 also reduced the PPI-induced accumulation of ferrous ions based on fluorescence staining (Figure 4A) and flow analysis (Figure 4B). These results indicate that PPI inhibits the gastric cancer cell growth by, at least partially, inducing cell ferroptosis.
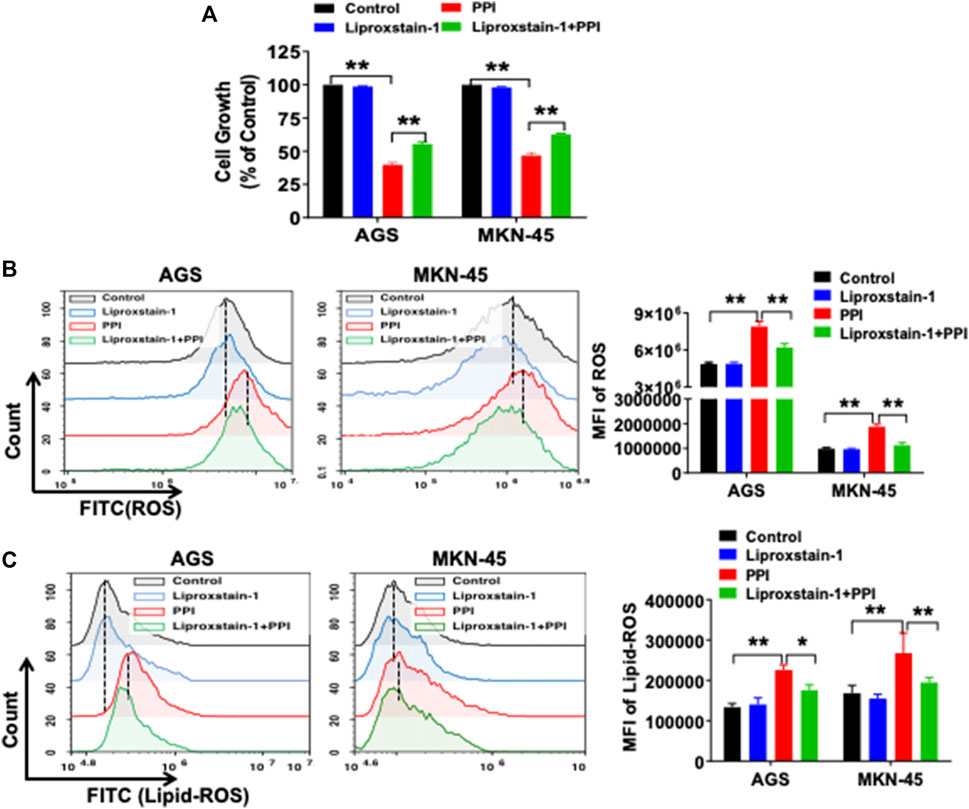
FIGURE 3. A ferroptosis inhibitor reverses PPI-induced ferroptosis and suppression of gastric cancer cell growth in vitro. (A) AGS and MKN-45 cells were pretreated with an inhibitor of ferroptosis, liproxstain-1 (200 nM), for 2 h, and then PPI was added for an additional 24 h. Cell growth/expansion was measured via MTT assays. (B and C) Intracellular ROS and lipid peroxides (lipid-ROS) levels were measured using flow cytometry. Data are shown as mean ± SD. p values were determined using one-way ANOVA (*p < 0.05, **p < 0.01).
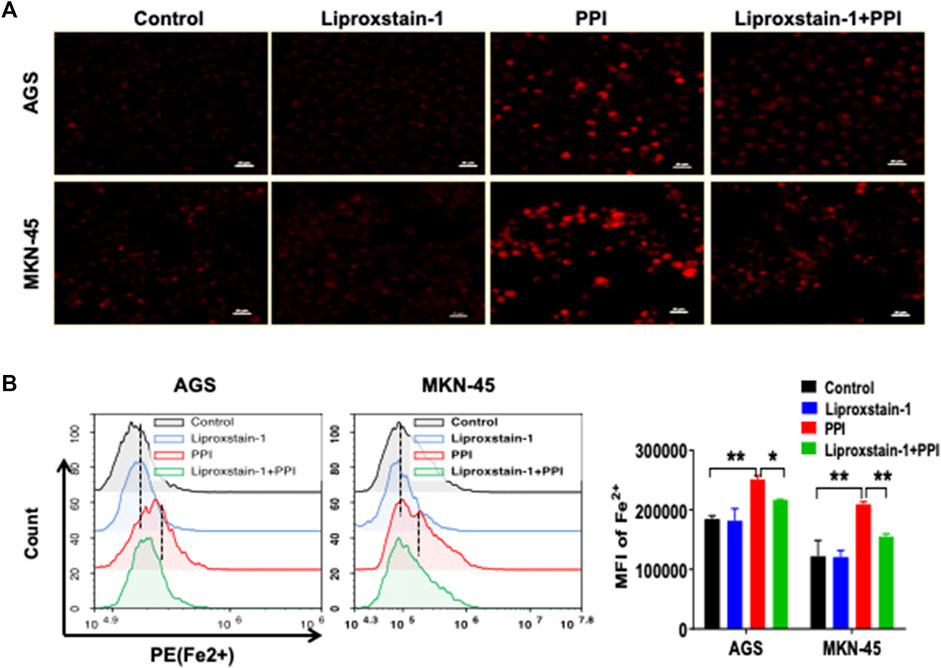
FIGURE 4. A ferroptosis inhibitor is also confirmed to block PPI-induced accumulation of Fe2+ in gastric cancer cells. AGS and MKN-45 cells were treated with liproxstain-1 and/or PPI as described in Figure 3. They were then labeled with FerroOrange fluorescent probes to detect intracellular ferrous (Fe2+) concentrations through fluorescence microscopy (A) and flow cytometry (B). Scale bars = 50 µm. Data are shown as mean ± SD while p values were determined using one-way ANOVA (*p < 0.05, **p < 0.01).
Inhibition of ferroptosis by Liproxstain-1 also partially reverses the inhibitory effects of PPI on tumor growth in vivo
To further determine if the effects of PPI on the gastric cancer growth in vivo are also dependent on its induction of ferroptosis, we administrated Liproxstain-1 and/or PPI in tumor-bearing mice. As expected, PPI indeed reduced the tumor weights (Figure 5A, B). However, we found that Liproxstain-1 treatment reversed, at least in part, the tumor weight loss induced by PPI treatment (Figure 5A, B). Similarly, treatment with Liproxstain-1 also blocked the inhibitory effects of PPI on the tumor growth based on the tumor volumes at various time points (Figure 5C). These results further suggest that PPI inhibits the gastric cancer growth in vivo by, at least partially, inducing cancer cell ferroptosis.
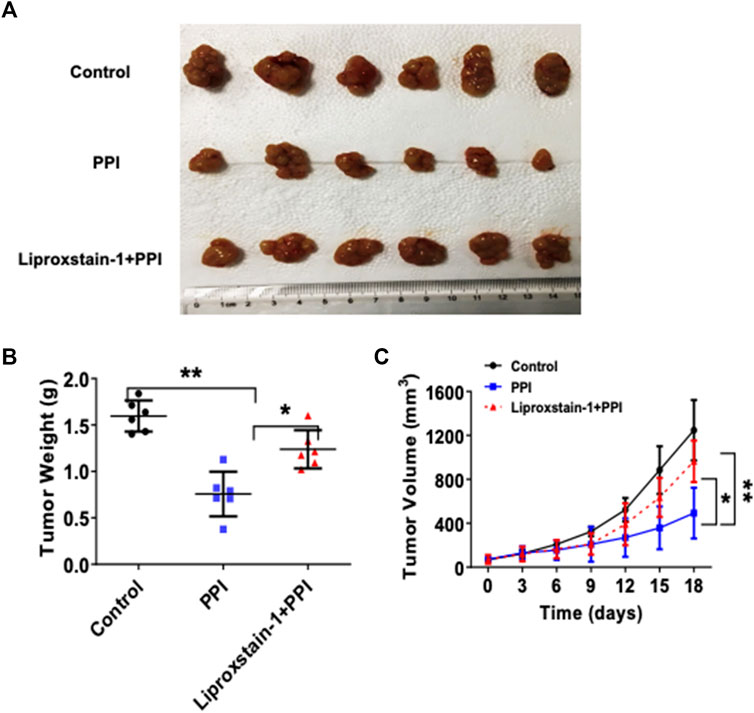
FIGURE 5. Liproxstain-1 reverses the inhibitory effects of PPI on tumor growth in vivo. BALB/c nude mice were subcutaneously injected with 1×106 AGS cells. One week later, the mice were administered with PPI (3 mg/kg/day) alone or in combination with liproxstain-1. (A) The tumor xenografts were harvested at day 15, and photographs of tumor xenografts were taken (n = 6 mice/group). (B) The weights of tumors derived from the nude mice were also measured at day 15. (C) Finally, tumor volumes were determined at various time points for up to 18 days. Data are presented as mean ± SD (n = 6 mice/group, *p < 0.05; and **p < 0.01).
PPI downregulates NRF2 and FTH1 in the gastric cancer cells
Nuclear factor erythroid 2-related factor 2 (NRF2) is a specific transcription factor that plays a role in mitigating lipid peroxidation and ferroptosis (Dodson et al., 2019). On the other hand, ferritin heavy chain 1 (FTH1), a heavy subunit of ferritin, is also a key regulator of ferroptosis (Tian et al., 2020a; Fang et al., 2021). Using Western blot assays to detect ferroptosis-related proteins in the cancer cells after PPI treatment in vitro, we found that PPI downregulated the expression of both NRF2 and FTH1 (Figure 6A) in both AGS and MKN-45 cancer cells, whereas Liproxstain-1 largely reversed this effect of PPI (Figure 6B), indicating that PPI-induced ferroptosis in the gastric cancer cells is associated with its regulation of NRF2/FTH1 pathway.
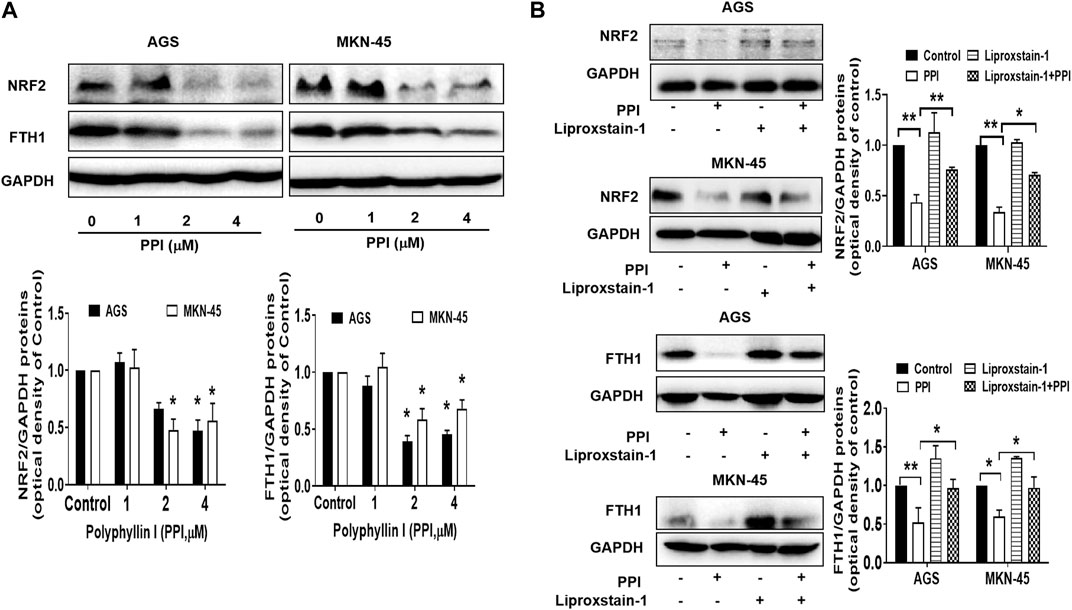
FIGURE 6. Effects of PPI on the expression of NRF2 and FTH1 in gastric cancer cells. AGS and MKN-45 cells were cultured and treated with PPI at various concentrations for 24 h, and then the protein levels of NRF2 and FTH1, without (A) or with (B) Liproxstain-1 treatment, were detected using Western blot assays. Data are presented as mean ± SD, while p values were determined using one-way ANOVA (*p < 0.05, and **p < 0.01, as indicated).
PPI induces ferroptosis of the gastric cancer cells by downregulating NRF2
Previous studies have found that NRF2 controls key signaling components of ferroptosis at a transcriptional level (Dong et al., 2021; Fu et al., 2021; Guan et al., 2022). We also found that PPI inhibited NRF2 expression in the gastric cancer cells (Figure 6AB). Here we further demonstrated that overexpression of NRF2 partially reversed the inhibitory effects of PPI on gastric cancer cell growth (Figure 7A) and mostly blocked the production of lipid-ROS and accumulation of Fe2+ induced by PPI (Figure 7B, C). On the other hand, exogenously increased expression of NRF2 also upregulated FTH1 expression, whereas PPI treatment failed to reduce FTH1 expression in the face of NRF2 overexpression (Figure 7D). Taken together, our findings indicate that NRF2 controls ferroptosis through FTH1, while PPI induces cell ferroptosis and tumor repression via suppressing NRF2/FTH1 pathway.
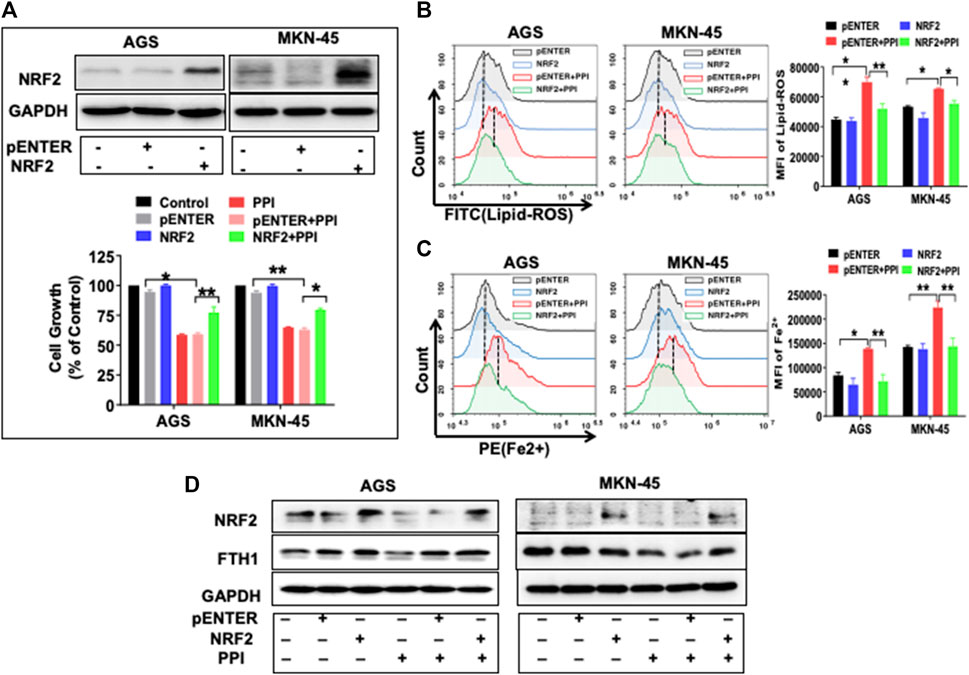
FIGURE 7. NRF2 overexpression reverses the effects of PPI on cell growth and ferroptosis in the gastric cancer cells. AGS and MKN-45 cells were transfected with NRF2 plasmid or the pENTER control vector for 24 h before they were exposed to PPI (3 μM) for an additional 24 h (A) NRF2 expression was confirmed after the transfection, while cell growth was assessed using MTT assays. (B and C) The levels of intracellular lipid peroxides (lipid-ROS) and ferrous ions (Fe2+) were detected using flow cytometry after the transfections and/or PPI treatment. Data are shown as mean ± SD while p values were determined through one-way ANOVA (*p < 0.05, **p < 0.01, compared with pENTER-vector control). (D) The expression of NRF2/FTH1 was analyzed using Western blot. Shown is one set of two or three separate experiments.
Discussion
There are currently few effective treatments available for the gastric cancer, especially advanced one. Chemotherapy is still one of the standard treatments for the gastric cancer. Unfortunately, chemotherapy resistance remains to be a major obstacle to the cancer treatment (Santero et al., 2022). More recently, targeted therapy and immunotherapy have provided new promising approaches to the treatment of the gastric cancer. However, their clinical application has been limited due to the relevant side effects, resulting in unsatisfactory therapeutic outcomes (Attia and Smyth, 2021; Basile et al., 2021). Therefore, it’s compelling to seek new therapeutic drugs for efficiently treating the gastric cancer with few side effects. In current study, we found that PPI suppressed the gastric cancer growth in vivo and in vitro. PPI also induced the gastric cancer cell ferroptosis, whereas suppression of ferroptosis mostly reversed the effects of PPI on the cancer growth. Finally, induction of cancer cell ferroptosis by PPI was largely dependent on its regulation of the NRF2/FTH1 pathway.
Ferroptosis, a novel form of the regulated cell death, is characterized by iron-dependent lipid peroxidation induced by excessive ROS and may be important for overcoming the resistance of tumor cells to chemotherapy (Pu et al., 2022). The accumulation of Fe2+ with lipid ROS is one of the hallmarks of ferroptosis and is critical for cell fate (Chen et al., 2021b). On the other hand, P53, a tumor suppressor, can regulate the ferroptosis of tumor cells as well as their autophagy and apoptosis (Ji et al., 2022). It may either negatively or positively regulate cancer cell ferroptosis, depending on its specific roles in reprogramming a metabolic network. Recent studies have also shown that ferroptosis plays an important role in cancer therapy since induction of ferroptosis has emerged as a novel strategy for anticancer treatment (Hassannia et al., 2019; Mou et al., 2019; Li and Li, 2020). Therefore, some studies have been looking for new anticancer drugs simply based on their capacity of inducing cancer cell ferroptosis. Others have developed a novel cancer therapy by combining iron oxide nanoparticles with cancer-selective knockdown of seven key ferroptosis-resistant genes, which exhibited antitumor effects on a variety of cancer cells (Luo et al., 2022). However, agents based on metal irons, especially nano-system, have been in the early stages of development. More extensive studies and even clinical trials are needed to confirm their efficacy (Hu et al., 2022).
As an important source for the development of new anticancer compounds, many natural ingredients extracted from traditional herbs or plants have been shown to enhance ferroptosis in cancer cells (Kong et al., 2021; Wen et al., 2021; Zhang et al., 2022; Shao et al., 2022). Polyphyllin I (PPI), a natural product isolated from the roots of Paris polyphylla, exerts its anticancer effects on different types of cancers (Tian et al., 2020b). However, it remains unknown whether and how PPI induces cancer cell ferroptosis, a new anticancer target. In current study, we found that PPI inhibited the tumor growth of both AGS and MKN-45 cells while increasing their intracellular levels of lipid peroxides and ferrous ions. Subsequently, a ferroptosis inhibitor, liproxstain-1, partially reversed the inhibitory effects of PPI on the gastric cancer cell growth in vitro and in vivo. Therefore, our findings suggest that PPI can suppress the gastric cancer growth by, at least in part, inducing gastric cancer cell ferroptosis.
FTH1, a heavy subunit of the ferritin, is a key regulator of cell ferroptosis, because its levels regulate susceptibility to ferroptosis (Tian et al., 2020b; Fang et al., 2021). On the other hand, NRF2 and its upstream/downstream signaling pathways also play an important role in the regulation of cell ferroptosis (Dodson et al., 2019). However, the exact regulatory mechanisms underlying their roles in ferroptosis remain unclear. In this study, we found that PPI promoted the gastric cancer cell ferroptosis by regulating the NRF2/FTH1 axis given that the overexpression of NRF2 largely reversed the gastric cancer cell ferroptosis induced by PPI. It remains unknown how PPI alters NRF2/FTH1 axis. It could do so by regulating cell death-associated microRNAs or simply interacting with the antioxidant pathway.
In conclusion, we have demonstrated that PPI inhibits the gastric cancer growth by inducing the cancer cell ferroptosis via regulating the NRF2/FTH1 pathway. Our data have provided novel insights into the molecular mechanisms underlying the effects of PPI on the gastric cancer cell growth and ferroptosis. Understanding of mechanisms responsible for cancer cell ferroptosis may help design new drugs for targeted cancer therapy.
Data availability statement
The original contributions presented in the study are included in the article/supplementary material, further inquiries can be directed to the corresponding authors.
Ethics statement
The animal study was reviewed and approved by Institutional Animal Care and Use Committee of the Second Affiliated Hospital of Guangzhou University of Chinese Medicine.
Author contributions
FZ performed experiments and wrote the original manuscript; YW and QZ performed some experiments and analyzed data; QC and CLL performed some experiments; FQ, HL and YC analyzed data; HH maintained mouse colonies and performed animal-related experimental procedures; WL provided vital reagents and general ideas; and ZD oversaw the project, designed experiments and edited the manuscript. All authors read and approved this manuscript.
Funding
This study was supported by grants from National Natural Science Foundation of China (82104605), Natural Science Foundation of Guangdong Province (2019A1515110741), and Guangzhou Municipal Science and Technology Bureau (202201020347, 202102010287 and 202102021001).
Conflict of interest
The authors declare that the research was conducted in the absence of any commercial or financial relationships that could be construed as a potential conflict of interest.
Publisher’s note
All claims expressed in this article are solely those of the authors and do not necessarily represent those of their affiliated organizations, or those of the publisher, the editors and the reviewers. Any product that may be evaluated in this article, or claim that may be made by its manufacturer, is not guaranteed or endorsed by the publisher.
Abbreviations
FTH1, Ferritin heavy chain 1; NRF2, Nuclear factor erythroid 2-related factor 2; PPI, Polyphyllin I; ROS, Reactive oxygen species.
References
Attia, H., and Smyth, E. (2021). Evolving therapies in advanced oesophago-gastric cancers and the increasing role of immunotherapy. Expert Rev. Anticanc 21, 535–546. doi:10.1080/14737140.2021.1866548
Basile, D., Simionato, F., Calvetti, L., Cappetta, A., Pesavento, A., Mongillo, M., et al. (2021). Comparing immunotherapies to other frequently used treatments of gastric cancer. Expert Rev. Clin. Phar 14, 1221–1232. doi:10.1080/17512433.2021.1938546
Chen, X., Kang, R., Kroemer, G., and Tang, D. L. (2021a). Broadening horizons: The role of ferroptosis in cancer. Nat. Rev. Clin. Oncol. 18, 280–296. doi:10.1038/s41571-020-00462-0
Chen, X., Li, J. B., Kang, R., Klionsky, D. J., and Tang, D. L. (2021b). Ferroptosis: Machinery and regulation. Autophagy 17, 2054–2081. doi:10.1080/15548627.2020.1810918
Dixon, S. J., Lemberg, K. M., Lamprecht, M. R., Skouta, R., Zaitsev, E. M., Gleason, C. E., et al. (2012). Ferroptosis: An iron-dependent form of nonapoptotic cell death. Cell. 149, 1060–1072. doi:10.1016/j.cell.2012.03.042
Dodson, M., Castro-Portuguez, R., and Zhang, D. D. (2019). NRF2 plays a critical role in mitigating lipid peroxidation and ferroptosis. Redox Biol. 23, 101107. doi:10.1016/j.redox.2019.101107
Dong, H., Xia, Y., Jin, S., Xue, C., Wang, Y., Hu, R., et al. (2021). Nrf2 attenuates ferroptosis-mediated IIR-ALI by modulating TERT and SLC7A11. Cell. Death Dis. 12, 1027. doi:10.1038/s41419-021-04307-1
Dong, R. Z., Guo, J. M., Zhang, Z. W., Zhou, Y. M., and Hua, Y. H. (2018). Polyphyllin I inhibits gastric cancer cell proliferation by downregulating the expression of fibroblast activation protein alpha (FAP) and hepatocyte growth factor (HGF) in cancer-associated fibroblasts. Biochem. Bioph Res. Co. 497, 1129–1134. doi:10.1016/j.bbrc.2018.02.193
Fang, Y. Y., Chen, X. C., Tan, Q. Y., Zhou, H. H., Xu, J., and Gu, Q. (2021). Inhibiting ferroptosis through disrupting the NCOA4-FTH1 interaction: A new mechanism of action. Acs Cent. Sci. 7, 980–989. doi:10.1021/acscentsci.0c01592
Fu, D., Wang, C., Yu, L., and Yu, R. (2021). Induction of ferroptosis by ATF3 elevation alleviates cisplatin resistance in gastric cancer by restraining Nrf2/Keap1/xCT signaling. Cell. Mol. Biol. Lett. 26, 26. doi:10.1186/s11658-021-00271-y
Guan, D., Zhou, W., Wei, H., Wang, T., Zheng, K., Yang, C., et al. (2022). Ferritinophagy-mediated ferroptosis and activation of keap1/nrf2/HO-1 pathway were conducive to EMT inhibition of gastric cancer cells in action of 2,2'-Di-pyridineketone hydrazone dithiocarbamate butyric acid ester. Oxid. Med. Cell. Longev. 2022, 3920664. doi:10.1155/2022/3920664
Han, J., Kan, J., Wang, X. G., Yuan, Q. L., Song, X. Y., Huang, M., et al. (2020). Polyphyllin I suppresses proliferation and promotes apoptosis of gastric cancer cell by inhibiting stat3 phosphorylation. Transl. Cancer Res. 9, 4715–4725. doi:10.21037/tcr-20-66
Han, W. H., Hou, G. X., and Liu, L. (2015). Polyphyllin I (PPI) increased the sensitivity of hepatocellular carcinoma HepG2 cells to chemotherapy. Int. J. Clin. Exp. Med. 8, 20664–20669.
Hassannia, B., Vandenabeele, P., and Vanden Berghe, T. (2019). Targeting ferroptosis to iron out cancer. Cancer Cell. 35, 830–849. doi:10.1016/j.ccell.2019.04.002
He, J. L., Yu, S., Guo, C. J., Tan, L., Song, X. M. T., Wang, M., et al. (2019). Polyphyllin I induces autophagy and cell cycle arrest via inhibiting PDK1/Akt/mTOR signal and downregulating cyclin B1 in human gastric carcinoma HGC-27 cells. Biomed. Pharmacother. 117, 109189. doi:10.1016/j.biopha.2019.109189
Hu, H., Xu, Q., Mo, Z., Hu, X., He, Q., Zhang, Z., et al. (2022). New anti-cancer explorations based on metal ions. J. Nanobiotechnology 20, 457. doi:10.1186/s12951-022-01661-w
Ji, H., Wang, W., Li, X., Han, X., Zhang, X., Wang, J., et al. (2022). p53: A double-edged sword in tumor ferroptosis. Pharmacol. Res. 177, 106013. doi:10.1016/j.phrs.2021.106013
Kong, N., Chen, X., Feng, J., Duan, T., Liu, S., Sun, X., et al. (2021). Baicalin induces ferroptosis in bladder cancer cells by downregulating FTH1. Acta Pharm. Sin. B 11, 4045–4054. doi:10.1016/j.apsb.2021.03.036
Lai, L., Shen, Q. P., Wang, Y. J., Chen, L. T., Lai, J. J., Wu, Z. B., et al. (2021). Polyphyllin I reverses the resistance of osimertinib in non-small cell lung cancer cell through regulation of PI3K/Akt signaling. Toxicol. Appl. Pharm. 419, 115518. doi:10.1016/j.taap.2021.115518
Lei, G., Zhuang, L., and Gan, B. (2022). Targeting ferroptosis as a vulnerability in cancer. Nat. Rev. Cancer 22, 381–396. doi:10.1038/s41568-022-00459-0
Li, D., and Li, Y. (2020). The interaction between ferroptosis and lipid metabolism in cancer. Signal Transduct. Target Ther. 5, 108. doi:10.1038/s41392-020-00216-5
Li, J., Jia, J., Zhu, W., Chen, J., Zheng, Q., and Li, D. (2023). Therapeutic effects on cancer of the active ingredients in rhizoma paridis. Front. Pharmacol. 14, 1095786. doi:10.3389/fphar.2023.1095786
Li, J., Ma, W., Cheng, X., Zhang, X., Xie, Y., Ji, Z., et al. (2020). Activation of FOXO3 pathway is involved in polyphyllin I-induced apoptosis and cell cycle arrest in human bladder cancer cells. Arch. Biochem. Biophys. 687, 108363. doi:10.1016/j.abb.2020.108363
Li, L., Wu, J., Zheng, F., Tang, Q., Wu, W., and Hann, S. S. (2016). Inhibition of EZH2 via activation of SAPK/JNK and reduction of p65 and DNMT1 as a novel mechanism in inhibition of human lung cancer cells by polyphyllin I. J. Exp. Clin. Cancer Res. 35, 112. doi:10.1186/s13046-016-0388-x
Li, Y. J., Zeng, X. L., Lu, D. H., Yin, M. N., Shan, M. R., and Gao, Y. (2021). Erastin induces ferroptosis via ferroportin-mediated iron accumulation in endometriosis. Hum. Reprod. 36, 951–964. doi:10.1093/humrep/deaa363
Liu, X., Sun, Z., Deng, J., Liu, J., Ma, K., Si, Y., et al. (2018). Polyphyllin I inhibits invasion and epithelial-mesenchymal transition via CIP2A/PP2A/ERK signaling in prostate cancer. Int. J. Oncol. 53, 1279–1288. doi:10.3892/ijo.2018.4464
Lou, W., Chen, Y., Zhu, K. Y., Deng, H. Z., Wu, T. H., and Wang, J. (2017). Polyphyllin I overcomes EMT-associated resistance to erlotinib in lung cancer cells via IL-6/STAT3 pathway inhibition. Biol. Pharm. Bull. 40, 1306–1313. doi:10.1248/bpb.b17-00271
Luo, Q., Jia, L., Huang, C., Qi, Q., Jahangir, A., Xia, Y., et al. (2022a). Polyphyllin I promotes autophagic cell death and apoptosis of colon cancer cells via the ROS-inhibited AKT/mTOR pathway. Int. J. Mol. Sci. 23, 9368. doi:10.3390/ijms23169368
Luo, T., Wang, Y., and Wang, J. (2022b). Ferroptosis assassinates tumor. J. Nanobiotechnology 20, 467. doi:10.1186/s12951-022-01663-8
Mou, Y., Wang, J., Wu, J., He, D., Zhang, C., Duan, C., et al. (2019). Ferroptosis, a new form of cell death: Opportunities and challenges in cancer. J. Hematol. Oncol. 12, 34. doi:10.1186/s13045-019-0720-y
Pu, F., Chen, F., Zhang, Z., Shi, D., Zhong, B., Lv, X., et al. (2022). Ferroptosis as a novel form of regulated cell death: Implications in the pathogenesis, oncometabolism and treatment of human cancer. Genes. Dis. 9, 347–357. doi:10.1016/j.gendis.2020.11.019
Santero, M., Meade, A. G., Acosta-Dighero, R., Gonzalez, L., Melendi, S., Sola, I., et al. (2022). European clinical practice guidelines on the use of chemotherapy for advanced oesophageal and gastric cancers: A critical review using the AGREE II and the AGREE-REX instruments. Clin. Transl. Oncol. 24, 1588–1604. doi:10.1007/s12094-022-02807-w
Shao, M., Jiang, Q., Shen, C., Liu, Z., and Qiu, L. (2022). Sinapine induced ferroptosis in non-small cell lung cancer cells by upregulating transferrin/transferrin receptor and downregulating SLC7A11. Gene 827, 146460. doi:10.1016/j.gene.2022.146460
Shen, Z. C., Wang, J., Ke, K. B., Chen, R., Zuo, A. X., Zhang, R. P., et al. (2021). Polyphyllin I, a lethal partner of Palbociclib, suppresses non-small cell lung cancer through activation of p21/CDK2/Rb pathway in vitro and in vivo. Cell. Cycle 20, 2494–2506. doi:10.1080/15384101.2021.1991121
Shi, Y. M., Yang, L., Geng, Y. D., Zhang, C., and Kong, L. Y. (2015). Polyphyllin I induced-apoptosis is enhanced by inhibition of autophagy in human hepatocellular carcinoma cells. Phytomedicine 22, 1139–1149. doi:10.1016/j.phymed.2015.08.014
Tang, D. L., Chen, X., Kang, R., and Kroemer, G. (2021). Ferroptosis: Molecular mechanisms and health implications. Cell. Res. 31, 107–125. doi:10.1038/s41422-020-00441-1
Tian, Y., Gong, G. Y., Ma, L. L., Wang, Z. Q., Song, D., and Fang, M. Y. (2020a). Anti-cancer effects of Polyphyllin I: An update in 5 years. Chem. Biol. Interact. 316, 108936. doi:10.1016/j.cbi.2019.108936
Tian, Y., Lu, J., Hao, X. Q., Li, H., Zhang, G. Y., Liu, X. L., et al. (2020b). FTH1 inhibits ferroptosis through ferritinophagy in the 6-OHDA model of Parkinson's disease. Neurotherapeutics 17, 1796–1812. doi:10.1007/s13311-020-00929-z
Wen, Y., Chen, H., Zhang, L., Wu, M., Zhang, F., Yang, D., et al. (2021). Glycyrrhetinic acid induces oxidative/nitrative stress and drives ferroptosis through activating NADPH oxidases and iNOS, and depriving glutathione in triple-negative breast cancer cells. Free Radic. Biol. Med. 173, 41–51. doi:10.1016/j.freeradbiomed.2021.07.019
Wu, Y. Z., Si, Y., Xiang, Y. C., Zhou, T., Liu, X. W., Wu, M. W., et al. (2020). Polyphyllin I activates AMPK to suppress the growth of non-small-cell lung cancer via induction of autophagy. Arch. Biochem. Biophys. 687, 108285. doi:10.1016/j.abb.2020.108285
Xiao, T., Zhong, W. L., Zhao, J. M., Qian, B. X., Liu, H. J., Chen, S., et al. (2018). Polyphyllin I suppresses the formation of vasculogenic mimicry via Twist1/VE-cadherin pathway. Cell. Death Dis. 9, 906. doi:10.1038/s41419-018-0902-5
Zeng, Y. W., Zhang, Z. Q., Wang, W. P., You, L. T., Dong, X. X., Yin, X. B., et al. (2020). Underlying mechanisms of apoptosis in HepG2 cells induced by polyphyllin I through Fas death and mitochondrial pathways. Toxicol. Mech. Method 30, 397–406. doi:10.1080/15376516.2020.1747125
Zhang, C., Liu, X., Jin, S., Chen, Y., and Guo, R. (2022a). Ferroptosis in cancer therapy: A novel approach to reversing drug resistance. Mol. Cancer 21, 47. doi:10.1186/s12943-022-01530-y
Zhang, R., Pan, T., Xiang, Y., Zhang, M., Xie, H., Liang, Z., et al. (2022b). Curcumenol triggered ferroptosis in lung cancer cells via lncRNA H19/miR-19b-3p/FTH1 axis. Bioact. Mater 13, 23–36. doi:10.1016/j.bioactmat.2021.11.013
Zhang, Y. F., Huang, P., Liu, X. W., Xiang, Y. C., Zhang, T., Wu, Y. Z., et al. (2018). Polyphyllin I inhibits growth and invasion of cisplatin-resistant gastric cancer cells by partially inhibiting CIP2A/PP2A/Akt signaling axis. J. Pharmacol. Sci. 137, 305–312. doi:10.1016/j.jphs.2018.07.008
Keywords: ferroptosis, gastric cancer, NRF2, polyphyllin I, anticancer drug
Citation: Zheng F, Wang Y, Zhang Q, Chen Q, Liang C-L, Liu H, Qiu F, Chen Y, Huang H, Lu W and Dai Z (2023) Polyphyllin I suppresses the gastric cancer growth by promoting cancer cell ferroptosis. Front. Pharmacol. 14:1145407. doi: 10.3389/fphar.2023.1145407
Received: 16 January 2023; Accepted: 27 March 2023;
Published: 04 April 2023.
Edited by:
Zhijie Xu, Central South University, ChinaReviewed by:
Xu-Sheng Liu, Hubei University of Medicine, ChinaFanhua Kang, Xiangya Changde Hospital, China
Copyright © 2023 Zheng, Wang, Zhang, Chen, Liang, Liu, Qiu, Chen, Huang, Lu and Dai. This is an open-access article distributed under the terms of the Creative Commons Attribution License (CC BY). The use, distribution or reproduction in other forums is permitted, provided the original author(s) and the copyright owner(s) are credited and that the original publication in this journal is cited, in accordance with accepted academic practice. No use, distribution or reproduction is permitted which does not comply with these terms.
*Correspondence: Weihui Lu, d2VpaHVpLmx1QGd6dWNtLmVkdS5jbg==; Zhenhua Dai, emRhaTIwMDlAb3V0bG9vay5jb20=