- Department of Biochemistry, Faculty of Sciences, University of Douala, Douala, Cameroon
Oxidative stress is a state in which oxidants are produced in excess in the body’s tissues and cells, resulting in a biological imbalance amid the generation of reactive oxygen and nitrogen species (RONS) from redox reactions. In case of insufficient antioxidants to balance, the immune system triggers signaling cascades to mount inflammatory responses. Oxidative stress can have deleterious effects on major macromolecules such as lipids, proteins, and nucleic acids, hence, Oxidative stress and inflammation are among the multiple factors contributing to the etiology of several disorders such as diabetes, cancers, and cardiovascular diseases. Non-coding RNAs (ncRNAs) which were once referred to as dark matter have been found to function as key regulators of gene expression through different mechanisms. They have dynamic roles in the onset and development of inflammatory and oxidative stress-related diseases, therefore, are potential targets for the control of those diseases. One way of controlling those diseases is through the use of natural products, a rich source of antioxidants that have drawn attention with several studies showing their involvement in combating chronic diseases given their enormous gains, low side effects, and toxicity. In this review, we highlighted the natural products that have been reported to target ncRNAs as mediators of their biological effects on oxidative stress and several inflammation-associated disorders. Those natural products include Baicalein, Tanshinone IIA, Geniposide, Carvacrol/Thymol, Triptolide, Oleacein, Curcumin, Resveratrol, Solarmargine, Allicin, aqueous extract or pulp of Açai, Quercetin, and Genistein. We also draw attention to some other compounds including Zanthoxylum bungeanum, Canna genus rhizome, Fuzi-ganjiang herb pair, Aronia melanocarpa, Peppermint, and Gingerol that are effective against oxidative stress and inflammation-related disorders, however, have no known effect on ncRNAs. Lastly, we touched on the many ncRNAs that were found to play a role in oxidative stress and inflammation-related disorders but have not yet been investigated as targets of a natural product. Shedding more light into these two last points of shadow will be of great interest in the valorization of natural compounds in the control and therapy of oxidative stress- and inflammation-associated disorders.
1 Introduction
Oxidative stress (OS) is a state in which excess oxidants are produced in the body’s tissues and cells, resulting in a biological inequality between the generation of (RONS) from redox reactions and the antioxidant defense system. OS comes into effect in the body when there is an imbalance between free radicals and antioxidants (Michael, 2020). Reactive oxygen species (ROS) can be generated from oxygen radicals like Superoxide radicals (O2•−), hydroxyl radicals (•OH), peroxyl (RO2.), and alkoxyl (RO-) as well as non-radical molecules which are easily oxidized like ozone (O3), hydrogen peroxide (H2O2, singlet oxygen (1O2), hypochlorous acid (HOCL), (Gabriele et al., 2017) formed during normal aerobic metabolism in the body. They are generated from sources like the mitochondria, cytosol, plasma membranes, and peroxisomes and are important for tissue and cell physiological balance targeting most especially plasma membranes and macromolecules depending on the cell type and degree of exposure (Joye et al., 2004; Aylan and Metin, 2015). The antioxidant defense is made up of enzymes like superoxide dismutase, peroxidases lipases, and proteases as well as non-enzymatic systems including glutathione, thiols, and vitamins C and E (Niki, 1997). These antioxidants can be endogenous as well as exogenous and work as preventive, repair, and radical scavenging systems for defense from oxidative stress (Joye et al., 2004). In the right concentrations, ROS are useful in tissue repairs, angiogenesis, and cell proliferation (Yasuda et al., 1999) but when they are in high concentrations, they cause apoptosis and cell death, mutagenesis, carcinogenesis, and mitochondrial dysfunction (Juan et al., 2021). On the other hand, reactive nitrogen species (RNS) are molecules, for example, nitric oxides (NO), nitrogen dioxide (NO2), and peroxynitrite (ONOO.) (Klebanoff, 1980). Nitric oxide (NO.) is synthesized from l-arginine and molecular oxygen (O2). This is catalyzed by the nitric oxide synthase enzyme. On the other hand, NO. and O2.- react spontaneously and rapidly to produce peroxynitrite (ONOO−) which can be detoxified by isomerization to nitrate (NO3−) (Lugrin et al., 2014).
Given their highly reactive nature which is ascribable to the availability of valence shell electrons existing in an unpaired form or non-static bonds, and the inability of the antioxidant repair and preventive mechanisms to balance these ROS, they are able to cause cell and tissue damage (oxidative stress). This happens when they react with macromolecules such as proteins, nucleic acids, and carbohydrates by impacting various signaling pathways like the mitogen-activated protein kinase (MAPKs), Keap-1-Nrf2-ARE, and PI3K-Akt (Nayansi et al., 2017). These reactions often take place during environmental stress from radiation, diet, lifestyle, and exposure to heat molecules (Ray et al., 2012). A consequence of such reactions is their implication in accelerated aging, and pathologies such as autoimmune diseases, cardiovascular diseases, fibrotic diseases, infectious diseases, obesity, and cancers (Brieger et al., 2012).
Due to some of these pathogenic diseases which are associated with oxidative stress, the release of inflammatory stimuli, and signals such as peroxiredoxin 2 (PRDX2) (Salzano et al., 2014), the inflammatory process is triggered as a defense mechanism. This results in the pro and anti-inflammatory cytokines emission like the Nuclear Factor-kappa B/active protein-1 (NF-κB/AP-1) and tumor necrosis factor-alpha (TNF-α) (Hussain et al., 2016). Inflammation is the body’s natural defense against injury, pathogens, and diseases from both endogenous and exogenous sources (Pahwa et al., 2022). It can be acute when short-lived or chronic when long-term and marked with inflamed cell continuous infiltration by white blood cells such as macrophages and lymphocytes. This is a consequence of the body being on constant alert giving rise to the production of inflammatory cytokines and enzymes causing cell damage. As a result, there is a development of numerous disease conditions like cardiovascular diseases, fibrosis, arthritis, and cancers which are the major causes of death worldwide (Khansari et al., 2009; Needham et al., 2019; Pahwa et al., 2022). The course of inflammation is characterized by the emancipation of cytokines, for example, TNF-α, IL-6, and RONS by NADPH oxidase found on neutrophil surfaces in a process called oxidative burst (Sharma et al., 2019) and hence depletion of antioxidants (Ryan et al., 2004; Khansari et al., 2009). Inflammation leads to the continuous production of ROS from inflammatory cells that overwhelm cellular antioxidants and can cause genomic mutations and extensive DNA damage (Coussens and Werb, 2002). To target the deleterious effects of inflammation and oxidative stress, regulation at the transcriptional and posttranscriptional levels has been targeted in recent years as they present a vast array of possibilities for gene expression control through the action of non-coding RNAs (ncRNAs).
Non-coding RNAs (ncRNAs), once referred to as dark matter, are RNA genes transcribed from DNA but not translated to proteins (Moreira et al., 2008). They are made up of housekeeping ncRNAs such as transfer RNA (tRNA) of 76–90 nucleotides (nt) in length, ribosomal RNA (rRNA) 120 to 4,500 nt, small nuclear RNA (snRNA) 100–300 nt, small nucleolar RNA (snoRNA) 60 to 400 nt, telomerase RNA (TERC), tRNA-Derived Fragments (tRF) 16–28 nt and tRNA halves (tiRNA) 16–28 nt (Zhang and Niu, 2019). These housekeeping ncRNAs are highly abundant in cells due to their functions which range from translation, pre-mRNA splicing, trans-translation and telomeric DNA synthesis (Lodish et al., 2000; Uchida and Adams, 2019). On the other hand, regulatory ncRNA production is dependent on the stage of the organism’s development and cell differentiation. In addition, production is equally dependent on external stimuli. They include microRNA (mRNA) with lengths from 19 to 23 nt, piwi-interacting RNA (piRNA) 26–31 nt, circular RNA (circRNA) 100–10,000 nt, small interfering RNA (siRNA), enhancer RNA (eRNA) 50–2,000 nt (Gusic and Prokisch., 2020). These riboregulators as they are sometimes called (Erdmann et al., 2001) function mostly in transcriptional and posttranscriptional regulation of gene expression (Szyman’ski and Barciszewski, 2002). Generally, all regulatory ncRNAs with length <200 nt are referred to as short ncRNAs with miRNA being the most abundant (Lagos-Quintana et al., 2001) while >200 nt are long ncRNAs (lncRNAs) which are the most abundant RNAs. lncRNAs have characteristics similar to mRNAs such as the presence of polyadenylated tail, methylated cap at 5′ end, and presence of introns and exons (Gusic and Prokisch, 2020), however, their level of expression is low and depends on a number of factors such as the physiological state, pathological state and tissue-dependent expression (Kornienko et al., 2016; Fazal et al., 2019). lncRNAs interact with nucleic acids to control gene expression at the level of the cytoplasm and nucleus giving them diverse functions such as enhancers, decoys, scaffolds, sponges (Charles et al., 2018) and more complexity (Esteller, 2011; Statello et al., 2021). ncRNAs regulate gene expression through histone modifications, DNA binding and recruitment of chromatin modifiers, heterochromatin formation, and gene silencing (Latronico and Silveira, 2018) and their differential expression has been observed in a variety of disorders. Owing to these outstanding features of ncRNAs, they present themselves as a propitious area that is being targeted for diagnostic and therapeutic purposes.
Natural products (NP) and their derivatives have been used for various disorders like diabetes, cancers, obesity, neurological disorders, and so on (Abdullah, 2020). They are organic compounds produced by living organisms such as insects, plants, animals, humans, marine, and microorganisms from secondary metabolism (Lippincott and Wilkins, 2020). They are classified into primary and secondary metabolites having antibacterial, antioxidant, antiviral, and anti-inflammatory properties (Dutta et al., 2019) and are used in both traditional and modern medicine for disease treatment. They are relatively low in cost, readily available, easy to apply, and of lesser side effects, (Dutta et al., 2019). Although usage of natural products is limited by extinction and/or inaccessibility of some species from which they are derived, complex and time-consuming isolation process and high complexity and instability of compounds found in NP (Enna et al., 2001; Huang and Hu, 2021), they are still advantageous in their relatively low costs, availability, easy application and of lesser side effects, (Dutta et al., 2019). Natural products have the ability to penetrate just as easily and reach their targets within or on the cell. Thanks to their diversity, NP possess new and innovative strategies that can aid in novel drug discovery (Dzobo, 2022). These have attracted researchers more towards their exploitation in disease therapy thereby bringing us to the purpose of this review which is aimed at highlighting some of the natural products that modulate the expression of ncRNAs involved in oxidative stress and inflammation-related disorders.
2 Disorders associated with oxidative stress and inflammatory imbalance
2.1 Disorders associated with oxidative stress
Oxidative stress is an abnormal state that the cells or tissues of an organism sometimes go through when they are subjected to endogenous or exogenous production of radical (or reactive) oxygen species that exceed their oxidizing capacity (Migdal and Serres, 2011).
Depending on the excessive production of oxygen radical species or a decrease in antioxidant capacities, oxidative stress can, through genomic, metabolic, and functional modifications, induce the development of different pathologies (Roy et al., 2017). It can therefore be a secondary element involved in the establishment of a disease or an element participating in the complications of immune diseases. It is not only implicated in the progression of several metabolic diseases such as type 2 diabetes (Giacco and Brownlee, 2010) and gestational diabetes mellitus (Lopez-Mejia and Fajas, 2014), but also in the development of age-related diseases, for example, atherosclerosis (Migdal and Serres, 2011), cancer (Kamal et al., 2022), cataracts, muscle degeneration, neurodegenerative diseases (such as Alzheimer), corneal dystrophy (Eddaikra and Eddaikra, 2021). Also, oxidative stress is implicated in heart failure, hypertension, infertility (Ménézo et al., 2012), and neuromuscular disorders (Mezdour et al., 2017).
In addition, it has been demonstrated that in most cases, oxidative stress is the cause of chronic inflammatory reactions, thus promoting the development of several diseases and vice versa.
2.2 Disorders associated with inflammatory imbalance
Inflammation is the body’s natural defense when in contact with harmful stimuli such as pathogens, irritants, and damaging cells. This mechanism initiates the healing process (Aghasafari et al., 2019) and is found in various metabolic diseases (Rémy et al., 2022), microbial, and genetic diseases, amongst others. The overexpression of this mechanism through the excessive production of inflammatory mediators can be at the origin or partake in the onset and progression of various diseases.
The presence of chronic inflammation contributes to the progression of certain diseases like type 2 diabetes (Donath, 2021), cancers, cardiovascular diseases, atherosclerosis (Castellon and Bogdanova, 2016), the pathogenesis of hypertension (Liang X. et al., 2020), and inflammatory bowel diseases (for example, Crohn’s disease and recto -hemorrhagic colitis) (Calle and Fernandez, 2012). In certain pathologies such as renal failure where patients are constantly on dialysis, oxidative stress is coupled with chronic inflammation, making the healing process of these dialysis patients even more complicated (Belaïch and Boujraf, 2016).
2.3 Relationship between inflammation and oxidative stress
Oxidative stress and inflammation are strongly related and associated with major diseases such as obesity, diabetes, cardiovascular and neurodegenerative diseases, so much so, that if one is the major event in a disorder, the other will come later as a consequence and accentuate the former. Oxidation of macromolecules like proteins and lipids during oxidative stress cause modifications which trigger innate immunity by acting as damage-associated molecular patterns (DAMPs) and pathogen Associated Molecular patterns (PAMPs) which bind to Pattern Recognition Receptors (PRR) like toll-like receptor (TLR) that lead to signal transduction thereby activating factors of transcription like NF-κB and trigger inflammatory signals by inducing gene expression and recruiting immune cells (Tabas and Glass, 2013; Lugrin et al., 2014).
Furthermore, TLR has also been found to cause oxidative stress through the unbalanced production of proinflammatory and anti-inflammatory cytokines (Lavieri et al., 2014). Marseglia et al. demonstrated that levels of TNF- α are deregulated in weight loss and obesity, influencing the immune system, and the inflammatory process and inducing oxidative stress. OS is achieved through the production of ROS when TNF-α binds specifically to some receptors thereby promoting the NF-κB signaling pathway (Wang and Trayhurn, 2006; Marseglia et al., 2014). The latter equally states that the accumulation of adipose tissues also favors the synthesis of cytokines like TNF-α, IL-1, and IL-6 leading to the increased assembly of RONS by monocytes and macrophages which cannot be balanced by antioxidants due to their depletion in obese people thereby promoting oxidative stress. Inflammation from NF-κB pathway activation has also been found to be triggered by ROS-induced DNA-base modifications. In this situation, oxidative stress renders the redox potential oxidized at the level of cysteine and disulfide cysteine in plasma. This further causes the linkage of monocytes to endothelial cells thereby activating NF-κB and causing inflammation (Iyer et al., 2009).
Moreover, inflammation is able to trigger oxidative stress through the release of enzymes, reactive species, and chemical mediators such as cytokines and nitric oxide at inflammatory sites (Subrata, 2016). In lung cancer, for example, increased expression of NADPH oxidase 4 (NOX4) by IL-6 which is an inflammatory cytokine induces ROS production (Li et al., 2015). Yongzhong et al. in their experiment were able to demonstrate that the production of H2O2 which was dependent on activation of both the Stat1-and NF-κB pathways by human pancreatic cancer cell lines was enhanced by the IFN-γ and LPS pro-inflammatory stimulated transcription of Duox2 and DuoxA2 (Yongzhong et al., 2013). Furthermore, the development of dysplasia has been linked to the production of ROS resulting from inflammatory infiltration. The production of ROS and inflammation have equally triggered the development of benign polyps into colorectal cancers when they impair pathways such as the Wnt/-catenin and/or base excision repair pathway (Zhao et al., 2022). Hence it is important to look at the course of oxidation in pathology with inflammation as the primary pathophysiological process and vice versa as these two turn out to be related. This offers a better chance to discover the cause of the pathology and increases the chances of better treatment or management.
3 Non-coding RNAs
3.1 Classification of non-coding RNAs
Non-coding RNAs (ncRNAs) are non-protein coding genes that can either be regulatory or housekeeping based on their functions. Regulatory ncRNAs have epigenetic functions, transcriptional, and post-transcriptional regulatory functions as well. Several types of ncRNAs exist ranging from snoRNA, snRNA, tRNA, rRNA, lncRNAs, and short ncRNAs like miRNAs, siRNAs, and piRNAs.
3.2 Non-coding RNAs involved in oxidative stress and inflammation-related disorders
Oxidative stress induces exaggerated inflammatory reactions and damage to lipids, proteins, and nucleic acids or causes damage to certain tissues that can lead to complications. Several studies suggest that non-coding RNAs may function as key modulators in the response to oxidative stress and inflammation associated with pathological states (Ghafouri-Fard et al., 2020) with a good number of them investigated with natural products as shown on Table 1.
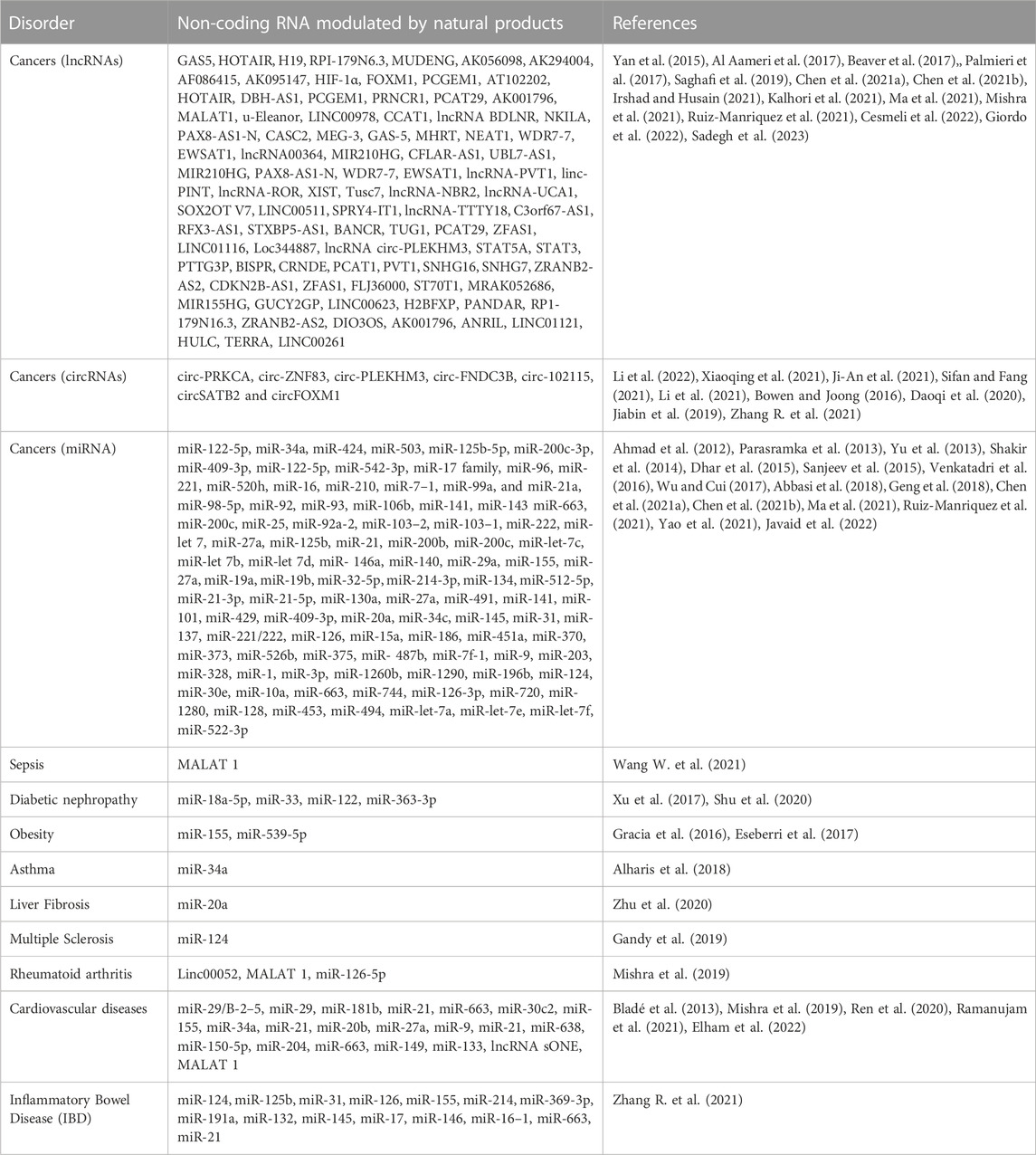
TABLE 1. Non-coding RNAs modulated by Natural Products in Oxidative Stress and Inflammation Related Disorders
3.2.1 Short non-coding RNAs
Studies have shown various circular RNAs (circRNAs) that are regulated by oxidative stress and mediate ROS production as well as promote ROS-induced cell death, cell apoptosis, and inflammation (Li Y et al., 2020; Liang J et al., 2020). Some circRNAs implicated in oxidative stress and inflammation-related disorders are circ-PRKCA, circ-ZNF83, circ-PLEKHM3, circ-FNDC3B, circ-102115 circSATB2 and circFOXM1 on which natural products modulation have been investigated in cancers and other disorders (Jiabin et al., 2019; Ji-An et al., 2021; Sifan and Fang, 2021; Xiaoqing et al., 2021; Zhang W. et al., 2022).
MicroRNAs (miRNA); with lengths between 20 and 24bp are single-stranded ncRNA with diverse functions ranging from activation or suppression of gene expression and also the control of transcription and translation. Several reviews have outlined the role of miRNAs in oxidative stress and inflammation-related disorders such as Tahamtan et al. (2018); Konovalova et al. (2019); Infante-Menéndez et al. (2023). Recent study aimed at identifying RNAs involved in the regulatory processes in various pathologies related to inflammatory disorders reveal miR-20b, miR-149, miR- 21, miR-150-5p, miR-663 involvement in cardiovascular diseases, miR-126-5p in rheumatoid arthritis, miR-146, miR-124, miR-16–1, miR-132, miR-145 in inflammatory bowel disease (Zhang S. et al., 2021). Furthermore, miR-20a is involved in the modulation of inflammation in fibrosis-related diseases (Zhou et al., 2020). In the case of cancer, numerous miRNAs have been highlighted to be involved in different types of cancers (Table 1) such as miR-122-5p, miR-200 family, miR-205, miR-145 in breast cancers, miRNA promoter (such as miR-137, miR-9, miR-let-7) hypermethylation enhances the development of colorectal cancer (Venkatadri et al., 2016).
3.2.2 Long non-coding RNAs
A lot of research has been carried out so far on long non-coding RNAs (lncRNAs) involved in oxidative stress and inflammation-related disorders with some already existing reviews such as those of Wang Y. et al. (2019); Soudeh et al. (2020); Kalhori et al. (2021); Xu and Zhang (2022); as well as Zhao et al. (2021) which outline lncRNAs with some of their mechanisms of action in oxidative stress and inflammation-related disorders. This section reviewed a few which have been investigated with natural products and expressed in disorders such as diabetes, cancers, autoimmune diseases, and neurodegenerative diseases (Parkinson’s diseases and Alzheimer’s diseases) with more equally found in Table 1.
MALAT-1 is involved in oxidative damage and complications related to diabetes such as diabetic nephropathy, vascular complications and diabetic retinopathy, and diabetic cataract. As its name suggests, it has been demonstrated that MALAT-1has an oncogenic role in various cancers, where it has been found in upregulated levels in the renal cortexes of C57BL/6 mice with streptozocin-induced type 1 diabetes (STZ) (Hu et al., 2017). The work of Hu et al. revealed that there is a mechanism of retroactive regulation between MALAT-1 and beta-Catherine related to podocyte damage caused by high amounts of glucose (Hu et al., 2017). In addition, the silencing of MALAT-1 induces a fall in the regulation of OS and inflammatory reactions in the kidney of diabetic mice. Furthermore, MALAT-1has a negative regulatory action on miR let-7f and increases the krüppel-type factor 5 (KLF5) (Chu et al., 2022). Krüppel-like factor 5 is able to bind directly to the NADPH oxidase promoter4, which induces high expression of NADPH oxidase (NOX)4 in human AC16 cardiomyocyte cells thus contributing to oxidative stress (Zhang H. et al., 2021). Therefore, MALAT1 could cause OS in diabetic kidney tissue by KLF5/NOX4 signal regulation. MALAT-1 is equally linked to diabetic retinopathy and diabetic cataract. Almost all patients with type I diabetes and 60% or more of type II diabetic patients suffer from diabetic retinopathy during their lifetime (Kyriazis et al., 2021). The role of MALAT-1 has been delineated in diabetic retinas through experimental investigation on clinical samples such as type I diabetes mice induced by streptozocin (Fong et al., 2014) and type II diabetes mice (Yan et al., 2014).
HOX antisense intergenic RNA (HOTAIR) plays a role in oxidative stress in high glucose-induced human mesangial cells (HMC) through miR-147a (Wang et al., 2022). This lncRNA could be linked to oxidative stress and inflammation associated with neurodegenerative diseases such as Alzheimer’s and Parkinson’s Diseases. It has been also reported as a key negative modulator for oxidative stress in the myocardial ischemia-reperfusion (I/R) injury (Meng et al., 2020). Rheumatoid arthritis is another inflammatory disease in which HOTAIR has been found to be upregulated leading to increased enrolment of macrophages or by acting as a sponge on miR-126-5p. In sepsis, it has been found to enhance the excretion of inflammatory cytokines IL-1β, IL-6, and TNF-α, thereby reinforcing inflammation (Zhang J. et al., 2020).
Gene 3 expressed by the mother (MEG3), also known as gene trap locus 2 (Gtl2), is located on chromosome 14q32.3 in locus Dlk1-Dio3 (Chen et al., 2021a; Chen et al., 2021b). Evidence has shown that MEG3 interacts with several microRNAs involved in the regulation of oxidative stress, such as miR181a and miR-145 (Shenouda et al., 2011; Deng et al., 2020). Its involvement has also been reviewed by Hong et al. in bone diseases and osteogenic differentiation (Hong et al., 2020).
Growth arrest-specific 5 (GAS5) enhances elevated glucose-induced kidney damage by oxidative stress reduction and can serve as a miR-221 sponge via direct and agonote2-dependent targeting (Ge et al., 2019). Its sponging abilities equally link them to miR-223-3p, to achieve their effect on inflammatory responses in the microglia cells in Parkinson’s disease (Wei et al., 2020). In addition, miR-221 is able to induce the downregulation of proteins related to proliferation and fibrosis by targeting sirtuin1 (SIRT1) which is a deacetylase protein that helps counteract oxidative stress in various diseases, including diabetes (Baldinu et al., 2004; Meng et al., 2020). In high glucose-induced human tubular cells, GAS has been proven through its heightened expression to be an inhibitor of the inflammatory and oxidative stress process by targeting miR-452-5p (Xie L. et al., 2019). The same anti-inflammatory effect on lipopolysaccharide-induced inflammation is seen in sepsis through GAS 5 targeting of miRNA-23a-3p (Zhenping and Dan, 2021).
NEAT 1 has an oncogenic role in various cancers. It is involved in the regulation of many other oxidative stress- and inflammation-related diseases (Chen et al., 2019; Cui-Cui and Fang., 2019). Its effect on inflammation is achieved through the NF- κB signaling pathway. NEAT enhances oxidative stress by inhibiting antioxidant enzymes such as SOD.
Other ncRNAs involved in oxidative stress and inflammation-related disorders are circular RNAs such as circ-PRKCA, circ-ZNF83, circ-PLEKHM3, circ-FNDC3B, circ-102115 circSATB2 and circFOXM1 on which natural products modulation have been investigated on in cancers and other chronic diseases (Zhang H. et al., 2022).
Much work has been done on ncRNA regulation by natural products in cancers, however, the effect of natural products on ncRNAs such as lncRNAs and circRNAs in other oxidative stress and inflammation-related disorders including neurodegenerative diseases, diabetes, obesity, liver and cardiovascular diseases still warrant a lot of research. It is also important to explore these aspects of research more on human subjects.
4 Natural products involved in oxidative stress and inflammation-related disorders
Natural products have different classifications, one of them being the broad classification based on the structural features and biosynthetic origin into alkaloids, polyphenols, phenylpropanoids (which are phenylalanine derived), polyketides (from malonate and acetate), and terpenoids (from isoprene, glycosides) (Osbourn and Lanzotti, 2009; Phurpa, 2018) (see Table 2 below). The four main sources from which natural products are obtained include; animals, plants, marine organisms, and microorganisms (Jabeen et al., 2014), and have a wide variety of uses in therapeutics. About 80%–90% of the world’s population is dependent on traditional medicine derived from natural products for primary healthcare and about 73% of pharmaceutical drugs or products have been derived from them (Phurpa, 2018). These products carry antioxidant, anti-inflammatory, anti-cancerous, and immunomodulatory potentials which could be exploited for the development of novel therapeutic strategies.
4.1 Natural Product’s modulation of non-coding RNAs involved in oxidative stress and inflammation-associated disorders
Natural products and their secondary metabolites have been used for decades as medicines either in combination with other product(s) or as stand-alone medicine. Secondary plant metabolites or specialized metabolites are a group of natural products or toxins produced by the lifeforms and function in the survival and fecundity of the entity. Phytochemicals possess biological characteristics such as antioxidant, anti-inflammatory and anticancer properties and are responsible for promoting health and preventing disease (Kashyap et al., 2021). Based on their chemical structures, phytochemicals are grouped into the following; phenols and polyphenols (e.g., quercetin, luteolin, genistein, resveratrol, calycosine, daidzein, and genistein), nitrogen-containing alkaloids (e.g., berberine and sanguinarine), terpenes (e.g., betulin), and sulfur compounds (e.g., sulforaphane) (Yang et al., 2019) (Table 2). Several studies especially on cancers have highlighted natural products in the modulation of oxidative stress and inflammatory disorders through their action on ncRNAs (Saghafi et al., 2019; Irshad and Husain, 2021; Kalhori et al., 2021; Piergentili et al., 2022) but more research to unveil their in-depth mechanism of action is warranted as well as their investigation in other oxidative stress and inflammatory disorders. This review has been able to outline some of these natural products and present a brief summary in Table 3.
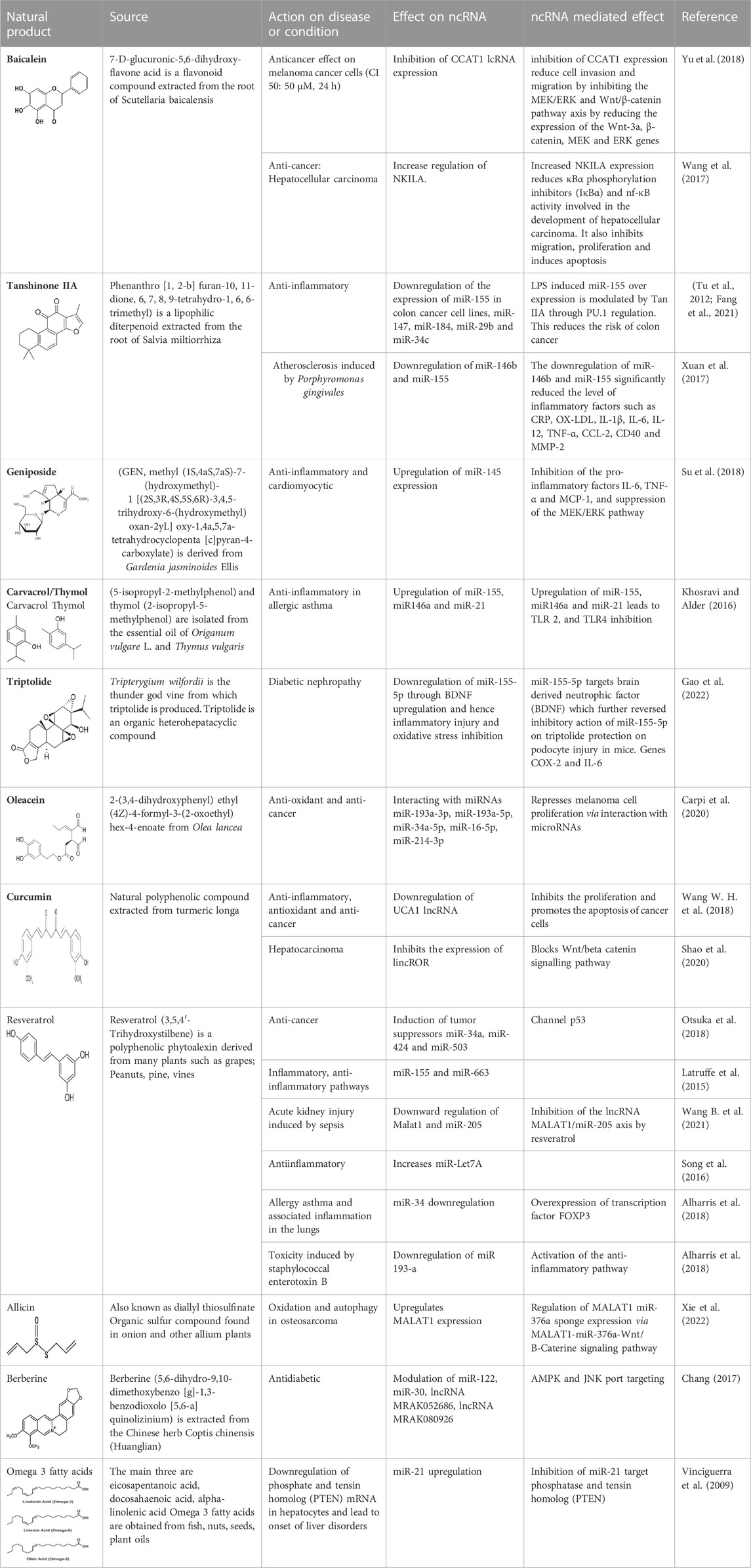
TABLE 3. A summary of Natural Products that Modulate Non-coding RNAs and its mediated effect in a given condition.
4.1.1 Baicalein
Baicalein (5,6,7-trihydroxy flavone) is a flavonoid compound obtained from Scutellaria species. It has also been found to be present in the Indian trumpet flower. Baicalein has properties like neuroprotection, anti-inflammatory, and antioxidation. Baicalein has been shown to exert its anticancer effects in melanoma cancer cells by inhibiting the expression of CCAT1 (transcript 1 associated with colon cancer, its upregulation increases the melanoma index). Baicalein may reduce cell invasion and migration if inhibition of CCAT1 expression inhibits the MEK/ERK and Wnt/β-catenin pathway axis by reducing the expression of the Wnt-3a, β-catenin, MEK, and ERK genes, in addition to decreasing cell proliferation by increasing the percentage of apoptotic cells (Yu et al., 2018). Baicalein may reduce κBα phosphorylation inhibitors (IκBα) and nf-κ B activity by increasing the expression of NKILA interacting with nf-κ B, thereby inhibiting migration, proliferation, and inducing apoptosis. LNCRNA-NKILA is a negative regulator of NF-κ B activity and is downregulated in hepatocellular carcinoma (Wang et al., 2017). Wang L et al.’s experiment in 2017 on rat intestinal cells showed that pre-treatment with baicalein could reverse the effects of TNF-α-induced expression of miR-191a, which would improve the viability of rat small intestine epithelial cells (Pan et al., 2017).
4.1.2 Tanshinone IIA
Tanshinone IIA is a lipophilic diterpenoid medicinal herb extracted from the roots of Salvia miltiorrhiza Bunge (Danshen). It is used in the treatment of diseases like diabetes, sepsis, arthritis, and cardiovascular diseases. Some research has been done on the effects of Tan II on cancers like colorectal cancer where they are able to exert their anti-inflammatory potential by reducing Hct116 and Ht29 cell proliferation and inhibiting microRNA-155 (Tu et al., 2012).
Furthermore, Tan IIA has been shown to reduce the level of expression of some cytokines, chemokines, and acute phase proteins, for instance, TLR4, MyD88, GM-CSF, sICAM-1, CXCl-1 and MIP-1α (Cheng et al., 2018). In addition, it significantly inhibits the mRNA expression levels of IL-1β, TNF-α, and COX-2, thereby suppressing lipopolysaccharide-induced activation (LPS) of the TLR4-NF-κ B pathway (Fan et al., 2016). Tan IIA has anti-inflammatory activity by exerting a negative regulation on the expression of miR-155, miR-147, miR-184, miR-29b, and miR-34c. Moreover, Tan IIA performs a protective function in atherosclerosis induced by Porphyromonas gingivalis by exerting a negative regulatory action on the expression levels of miR-155 thereby markedly reducing inflammatory factors like CRP, OX-LDL, IL-1β, IL-6, IL-12, TNF-α, CCL-2, and MMP-2 (Xuan et al., 2017).
4.1.3 Geniposide
Geniposide is derived from Gardenia jasminoides Ellis. It is a medicinal iridoid glycoside compound with antidiabetic, anti-inflammatory and antioxidant, and cardioprotective properties. Studies performed on LPS-injured H9c2 cells have reported anti-inflammatory and cardiomyocytic protective effects of geniposide. It upregulates the expression of miR-145, inhibits the pro-inflammatory factors of IL-6, TNF-α, and MCP-1, and then suppresses the MEK/ERK pathway and at such inhibits apoptosis and promotes cell viability (Su et al., 2018). In diffuse large B-cell lymphoma, geniposide is able to knockdown lncRNA HCP5 thereby increasing miR-27b-3p (a target of HCP5) levels and so inhibiting cell proliferation and inducing apoptosis in this condition (Hu et al., 2020).
4.1.4 Carvacrol/Thymol
Carvacrol or cymophenol and thymol (2-Isopropyl-5-methylphenol) are monoterpenoid phenol isomers found in thyme oil, pepperwort oil, and wild bergamot. These isomers have antioxidant, antihypertensive, anticancer, and anti-microbial properties. In chitin-induced models, Car/Thy had the potential to suppress inflammation allergic asthma by upregulating miRNAs and factors of inflammation such as; miR-155, miR146a, and miR-21, promoter of pro-inflammatory cytokines. More so SOCS, SHIP1, and miR-155 target and negatively regulate TLR-mediated inflammation and are inhibited by chitin. Abnormal expressions of TLR2, TLR4, SOCS1, SHIP1 and miR-155, miR-146a, and miR-21 can also be reversed by the combination of Car/Thy (Khosravi and Alder, 2016).
4.1.5 Triptolide
Triptolide is derived from the medicinal herb Tripterygium wilfordii, (Yang Y. Q. et al., 2018; Yuan K et al., 2019; Tamgue et al., 2021). This triepoxyde diterpene has cytotoxic, anti-inflammatory, and anticancerous activities. Cytotoxic activities of triptolide have been highlighted in some tumors through induction of necrosis and apoptosis, and cell cycle arrest in several cell lines (Yang Y. Q. et al., 2018; Tamgue et al., 2021). The anti-inflammatory activities of triptolide are achieved through mechanisms ranging from the downregulation of NF-kb, p-TAK1, p-IκBα. It is able to reduce OS and inflammation in podocyte injury in diabetic nephropathy treatment through the downregulation of miR-155-5p which targets brain-derived neurotrophic factors. AP-1–controlled pro-inflammatory molecules such as TNF-α, IL-6, IL-12, IL-1β, and Ptgs-2 are also downregulated by triptolide in several cell types (Yang Y. Q. et al., 2018; Tamgue et al., 2021).
4.1.6 Oleacein
Oleacein is an olive secoiridoid alcohol. It is known for its anti-inflammatory and antioxidant abilities which renders them a potential treatment option for neuroinflammatory disorders. Oleacein equally has anti-cancerous effects where it represses melanoma cell proliferation by interacting with several microRNAs like miR-193a-3p to target MCL1 and K-RAS, miR-193a-5p, miR-34a-5p, miR-16-5p, miR-214-3p and their mRNA targets encoding family proteins Bcl-2 and the mTOR pathway which opposes cell survival, proliferation, and apoptosis (Carpi et al., 2020).
4.1.7 Curcumin
Curcumin is a polyphenolic compound extracted from Curcuma longa Linn. It has been demonstrated to have various properties such as anti-cancer, anti-inflammatory, antimutagenic, and antimicrobial, and is able to increase antioxidant production by the body (Mirzaei et al., 2018). The anticancerous effects of curcumin are portrayed by its ability to influence multiple signaling pathways like the NF-kB, MAPK, PTEN, and p53 which are all involved in the progression of cancers. For instance, curcumin inhibits progression and promotes cancer cell apoptosis by negatively modulating the lncRNA UCA1 (Wang R. et al., 2018), it also upregulates microRNAs such as miR-502c, miR-181b, miR-16, miR-15a, miR-146b-5p, and miR-132 while downregulating others like miR-19a, miR-19b (Piergentili et al., 2022). In hepatocarcinoma cells, it suppresses the expression of lincROR and blocks the Wnt/B-catenin signaling pathway activation (Shao et al., 2020) while another study demonstrates that it inhibits GAS5 expression in lung cancer by affecting major pathways such as NF-kB and STAT3 (De Bacco et al., 2011). Furthermore, curcumin is able through the miR-21/PTEN/Akt pathway, to reduce gastric cancer cell line proliferation by inducing apoptosis (Qiang et al., 2019). It is equally able to inhibit the expression of miR-21 and upregulate miR-34a expression in gastric cancer cells.
4.1.8 Resveratrol
Resveratrol is a polyphenolic phytoalexin derived from many plants such as grapes; groundnut, pine, and vine which revealed anti-cancer effects (Rauf et al., 2018). There have been several research demonstrating the regulation of ncRNAs by resveratrol (Giordo et al., 2022). It inhibits breast cancer cell proliferation by inducing the expression of tumor suppressor miRNAs (miR-34a, miR-424, and miR-503) through the p53 pathway (Otsuka et al., 2018) and lncRNA LINC00978 (Deng et al., 2016). The link between resveratrol and lncRNAs correlation with inflammation and oxidative stress is still being studied and its mechanism of action is yet to be clearly understood. Resveratrol appears to have a modulatory effect on miRNAs, among which are miR-155 involved in the inflammatory pathways, miR-663; an anti-inflammatory ncRNA, and oncogenic miR-21 (Latruffe et al., 2015). In addition, Malat 1 lncRNA and miR-205 were downregulated in rats with resveratrol-treated sepsis-induced acute kidney injury compared to rats not receiving treatment (Wang B. et al., 2021), the same study suggests that inhibition of lncRNA MALAT1/miR-205 by resveratrol has a protective function in sepsis-induced acute kidney injury (Wang W. et al., 2021). Also, miR-Let7A decreases inflammation by upregulation after resveratrol treatment in human THP-1 macrophages (Song et al., 2016). The negative modulatory effect of resveratrol on miR-34 attenuates asthma allergy symptoms and associated inflammation in the lungs of mice via overexpression of the transcription factor FOXP3 (Alharris et al., 2018). Low regulation of miR-193-a by resveratrol has a protective action against toxicity induced by staphylococcal enterotoxin B by activation of anti-inflammatory pathways in mice (Alharris et al., 2018).
4.1.9 Solarmargine
Solarmargine is a steroidal alkaloid glycoside derived from solanum plant species such as Solanum nigrum with anticancer activities (Kalalinia and Karimi-Sani, 2017). A study demonstrated that solarmagine regulates miR-155-induced apoptosis by repressing the expression of lncRNA HOXA1-AS and inhibits cancer cell proliferation (Meng et al., 2022).
4.1.10 Allicin
Allicin or diallythiosulfinate is an organic sulphury compound found in onion and other allium plants such as garlic. It is extracted from the allium bulb and has anti-cancer, anti-inflammatory, antibacterial, and anti-viral effects (Ba et al., 2019). Physiologically, low doses of allicin have antioxidative effects in atherosclerosis through low-density lipid protein oxidation. It functions in the treatment of so many cancers like colorectal cancers, breast cancers, and lung cancers through its proapoptotic ability. Xie et al. were able to show that allicin was able to reduce MALAT1 expression and hence inhibited osteosarcoma proliferation and progression by targeting the Wnt/β-catenin pathway thereby regulating the genes in this pathway.
4.1.11 Aqueous extract or pulp of Açai
Pulp of Acai is a source of polyphenols. Oxidative stress and inflammation make up some of the underlying conditions that lead to the advancement of non-alcoholic fatty liver disease. Acai pulp has been investigated in this condition and shown to prevent the oxidation of low-density lipoproteins (Pereira et al., 2016). Flavon velutin is an active ingredient found in acai and has been reported to act as an effective anti-inflammatory agent by blocking LPS-mediated production of TNF-α and interleukin-6 thereby inhibiting the activation of NF-κB and the MAPK pathway (Xie C et al., 2012). These two pathways are involved in the mechanism of action of certain LncRNAs such as NKILA (Liu B et al., 2015). Also, research has shown that acai can be useful against lung diseases. In this condition, it is able to exert its antioxidative effects through an inhibitory action on the NF-κB pathways and Nrf2/ARE pathway stimulation.
4.1.12 Berberine
Berberine extracted from the Chinese herb Coptis chinensis (Huanglian), is an isoquinoline alkaloid that has been known to have antidiabetic effects by targeting pathways like the AMPK and JNK pathways thereby regulating ncRNAs. Some ncRNAs regulated by berberine include miR-122, miR-30, lncRNA MRAK052686, lncRNA MRAK080926 (Chang, 2017). By so doing, berberine is able to reduce stress in the endoplasmic reticulum and overcome insulin resistance (Chang, 2017). In type 2 diabetic patients, berberine has been known to help in weight gain reduction, reduction of blood lipid level production as well as antiglycaemic. Through hepatocyte nuclear factor-4α (HNF-4α) inhibition, miR-122 expression has been reported to decrease with the treatment of berberine. This promotes a fall in expression levels of enzymes like PEPCK and G6Pase involved in hepatic gluconeogenesis.
4.1.13 Quercetin
Quercetin is a pentahydroxy flavone from the group of flavonoids of polyphenols. Plants containing this natural product include; green tea, red wine, and onions. It is commonly used but not limited to the treatment of heart conditions, diabetes, obesity, and certain cancers where they have been found to dysregulate miR-16, miR-145, MALAT-1, miR-155, miR-146a, miR-197, lncRNA SNHG7 and miR-223 (Piergentili et al., 2022). Studies have demonstrated that quercetin has a protective effect against free radicals from smoking and lowers lncRNA DBH-AS1 expression through antioxidant activities in hepatocellular carcinoma (Saghafi et al., 2019). Quercetin is also a core anti-inflammatory molecule with the ability to inhibit enzymes that are key regulators of the inflammatory process acting on its mediators through their influence on enzymes such as lipoxygenase and cyclooxygenase (Xiao et al., 2011). It also functions in lowering levels of LDL and increasing the dilation of major arteries which ease blood flow thereby preventing heart diseases.
4.1.14 Genistein
Genistein (prunetol) is a tyrosine kinase inhibitor with a 4′,5,7-trihydroxyisoflavone chemical structure. Genistein is an isoflavonoid extracted from soybeans and fava beans. Genistein has been known for various biological and therapeutic properties for so many years. In tissue culture, for example, it is used as a phytoestrogen to induce cell differentiation. Studies have documented that genistein is able to reduce the severity of histopathological changes in acute pancreatitis through the action of its antioxidant, anti-inflammatory, and anti-apoptotic properties. (Heubach et al., 2015; Prasong et al., 2022). Genistein has anti-cancerous properties as seen in its ability to suppress the progression of apoptotic cells in metastatic cancers and inhibit HOTAIR in renal and prostate cancer (Imai-Sumida et al., 2017). It also downregulates miR-34a in prostate cancer. It has also been widely researched in their modulation of ncRNAs in cancers like miR-151, miR-221, miR-222, miR-223 downregulation and miR-34a, miR-573-3p, miR-1296 upregulation in prostate cancers, miR-155 suppression in breast cancers, miR-34a, miR-200 upregulation in pancreatic cancer (Piergentili et al., 2022).
4.1.15 Omega-3 fatty acids
Omega-3 fatty acids are healthy polyunsaturated fatty acids that exist primarily in food sources, especially oily fish. To date, there are several controversial effects of omega-3 fatty acids on oxidants and antioxidants. But that does not rule out their beneficial effects in lowering triglyceride levels and rendering protection against neurodegenerative and cardiac diseases through their anti-inflammatory, anti-allergic, and cardioprotective properties. Studies conducted by a randomized clinical trial (RCT) on patients reveal that omega-3 fatty acid supplementation significantly improves decreased malondialdehyde (MDA), total antioxidant capacity, and glutathione peroxidase activity. Thus, rendering omega-3 fatty acids as factors amplifying the antioxidant defense against ROS. (Javad et al., 2019). Omega- 3 fatty acids are also involved in liver diseases by targeting miR-21 which causes PTEN degradation (Vinciguerra et al., 2009). In cancers, omega-3 unsaturated fatty acids have also been found to regulate apoptosis-related miRNAs such as miR- 17, miR- 26a, miR-200a, miR-323 (Visioli et al., 2012).
The interaction of natural products with ncRNAs could be on the basis of several natural products modulating one ncRNA; for example, miR-21 regulation by sulforaphane, icariin, and piceatannol (Irshad and Husain, 2021) or one natural product having the potential of controlling several ncRNAs. The latter is seen in an example like curcumin which has the ability to modulate several lncRNAs such as H19, LINC00623, PVT1, PANDAR, AF086415 (Mishra et al., 2019). Future research can equally exploit the combined effects of some natural products on a particular ncRNA in order to see if they react antagonistically or synergistically in the moderation of a specific ncRNA of interest.
4.2 Natural products involved in oxidative stress and inflammation-related disorders with unknown effects on ncRNAs
4.2.1 Zanthoxylum bungeanum
Zanthoxylum bungeanum Maxim (ZBM) is plant species of the Rutaceae family of traditional herbal medicine. It is widely found in Asia. The mature pericarp of ZBM is used for the treatment of diseases and as a spice Sichuan pepper used for cooking (Wang et al., 2020). It is a rich source of chemicals with about 140 chemical compounds being isolated from it including terpenoids, flavonoids, and alkaloids giving this plant a very vast array of therapeutic and medicinal functions. Antioxidant, anticancer, and hepatoprotective effects are found in Zanthoxylum alkylamides and comprises α-, β- and γ alkylamides (Ren et al., 2017). ZBM has been reported to attenuate liver injury by activating nuclear factor-κB (NF-κB) and mitogen-associated protein kinase (MAPK) signaling pathways. This is accomplished through the inhibitory action of phosphorylated p65/NF-κB, MAPK (including p38, JNK, and ERK1/2), and activation of transcription factor 3 protein expression, with further suppression of Bax, cytochrome c, caspase-9 and caspase-3 (Zhang et al., 2018). ZBM also has anti-inflammatory and anti-analgesic effects.
4.2.2 Canna genus rhizome
It is a plant used in the traditional environment for its anti-inflammatory, analgesic and antipyretic properties. It is a rich source of flavonoids, phytosterols and phenolic acids. CGE has been reported to attenuate inflammation in ulcerative colitis by down-regulating LPS/TLR4 signal pathways, cleaving caspase-1, NLRP3 decreased protein expression, and NF-ҡB expressions (Mahmoud et al., 2021).
4.2.3 Fuzi-ganjiang herb pair
Fuzi and ganjiang are widely used in China, Korea, and Japan in the treatment of ulcerative colitis (UC), vomiting, and heart failure for over 1,800 years (Huang et al., 2020). Fuzi-ganjiang contains a lot of alkaloids and has been outlined for its anti-inflammatory properties. Research carried out on DSS-induced mouse colon tissue by Huang et al. showed that 6-gingerol which is an active ingredient obtained after the combination of fuzi-ganjiang herb pair was able to inhibit the production of MPO and suppress the expression of inflammatory cytokines which in this case were IFN-γ, TNF-α, IL-1β, IL-6, IL-10, and IL-17A. the expression levels of inflammatory mediators like MPO, iNOS, and COX mRNA are also suppressed by this combination. Moreover, the MAPK, NF-κB, and STAT3 signaling pathways are also inhibited by this combination of Fuzi-gangiang in order to accomplish its anti-inflammatory activities (Huang et al., 2020).
4.2.4 Aronia melanocarpa
Generally used in the production of juices and jams. Another name for it is black chokeberry and has been found to have ethnopharmacological properties due to the richness in the biologically active molecules of the natural product with pharmaceutical and physiological effects (Li et al., 2022). In obesity-induced inflammation in adipose tissue, Aronia extract has been shown in vivo and in vitro, to inhibit phosphorylation of the NF-kB pathway and P65. Extracts from this plant have been found to possess hypolipidemic and antioxidative properties. For example, anthocyanin-rich fraction from this fruit plant was able to reduce the levels of ROS caused by high glucose through its scavenging action in pancreatic β cells. The fruit juice of black chokeberry has equally been shown to have hypoglycaemic functions (Valcheva-Kuzmanova et al., 2007).
4.2.5 Peppermint
Peppermint (Mentha piperita L.) is extracted from the peppermint plant and contains important compounds such as menthol, iso-menthone, and neomenthol. It is used worldwide for its anti-inflammatory, antioxidant, antiviral, antimicrobial, and antibacterial activities among others (Sun et al., 2014). In inflammatory bowel disease, one of its key components menthols is able to decrease the production of inflammatory cytokines such as IL-1, IL-6, IL-23, and TNF- α and free radicals in colonic tissues. PEO is also able to enhance the upregulation of anti-inflammatory cytokines in irritable bowel syndrome (Zhao et al., 2022). However, indebt knowledge of the mechanisms of action in their various pharmacological effects is still to be uncovered. This is of importance due to the diverse use of these products universally giving reason to believe that it holds an affluent potential if investigated in its ability to target ncRNAs in oxidative stress and inflammation-related disorders.
4.2.6 Gingerol
It is an amphiphilic phenolic lipid obtained from the ginger rhizome. Its anti-inflammatory activities have been documented in HDM-induced asthma in rats where 6-gingerol is able to cause a decline in neutrophilic inflammation by reducing levels of •NO, IL-6, TNF- α and myeloperoxidase (Babajide et al., 2022). It also has a protective role by decreasing proinflammatory cytokines and oxidative stress in liver diseases (Alsahli et al., 2021). Cote et al. (2022) in their review of the role of some phytochemicals in inflammation-associated disorders outlined gingerol activities in cancers where it was able to reduce inflammation thereby decreasing tumors, in cardiovascular diseases, and equally in fasting blood glucose, inflammation, and oxidative stress reduction in diabetes.
5 Non-coding RNAs involved in oxidative stress and inflammation-related disorders on which the effect of natural products has not been investigated
Several authors have documented the implication of natural product-modulated ncRNAs; especially miRNAs and lncRNAs in various types of cancers, still leaving much to be done with regard to other oxidative and inflammation-related disorders like neurodegenerative disorders (for example, Parkinson’s diseases and Alzheimer’s disease), atherosclerosis or rheumatic diseases with a good number of regulatory ncRNAs on which natural products have not been investigated (Gámez-Valero et al., 2020; Zhang W. et al., 2022; Infante-Menéndez et al., 2023). We, therefore, highlight in this section and in Table 4, some of these ncRNAs that have not yet been investigated with natural substances in the context of diseases related to oxidative stress and inflammation.
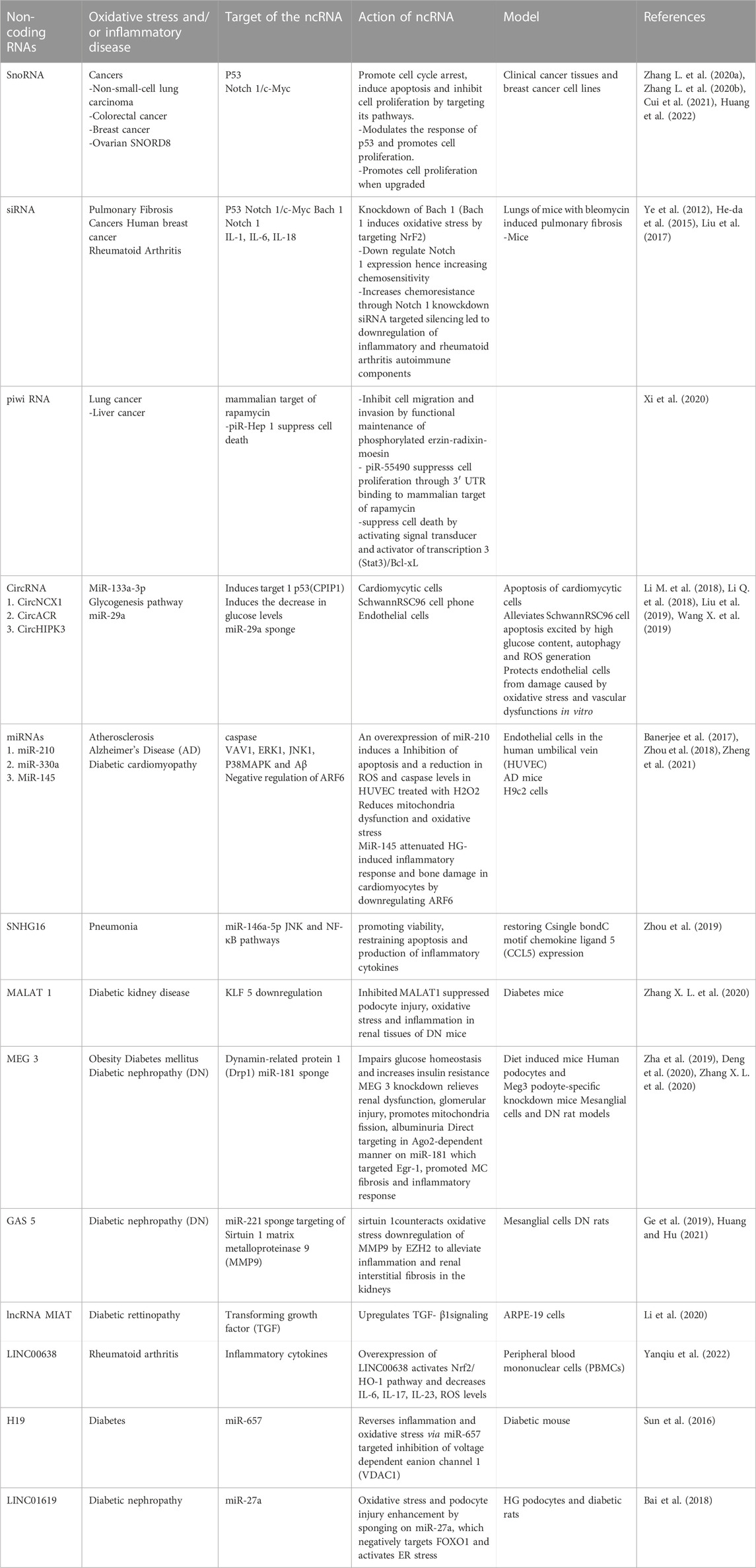
TABLE 4. Non-coding RNAs involved in Oxidative stress and Inflammation related Disorders on which the Effect of Natural Products has not been Investigated.
As part of the regulation of oxidative stress, several lncRNAs act by increasing apoptosis like lncRNA BDNF-AS, FOXD3-AS1, Linc 00,963, and lncRNA ODRU (Wang X. et al., 2019) but antisense lncRNA natural BDNF-AS also regulates oxidative stress by reducing cell viability but its expression is inversely proportional to the synthesis of superoxide dismutase and catalase; Linc 00,963 also modulates the expression of the lncRNA FoxO3 in order to be able to attenuate renal fibrosis and oxidative stress during chronic renal failure; the lncRNA ODRU downregulates the level of Bcl2 expression through its interaction with the AKT and JNK signaling pathways as well as the PI4K alpha proteins. In diabetes, lncRNA SNHG16 increases the production of ROS and apoptosis via the increase in the level of expression of the KLF9 factor and that of miR-106a, MALAT 1, GAS5, CASC2, HOTAIR, MEG 3 are also implicated in this disorder (Chu et al., 2022). LncRNA MIAT is overexpressed following oxidation in the context of hypoxic pulmonary hypertension and its silencing by knockdown leads to the suppression of oxidative stress (Li, et al., 2020).
Furthermore, lncRNA LUCAT1 thanks to the increase in its level of expression, post-transcriptionally regulates the splicing and stability of NR4A2, leading to the activation of the production of inflammatory cytokines and interferons during inflammatory bowel and lung disease (Vierbuchen et al., 2022). This lncRNA is also upregulated in asthma and chronic obstructive pulmonary disease. lncRNA FAO regulates inflammation in macrophage cells followed by restoration of homeostasis by activating fatty acid oxidation via its interaction with the HADHB subunit of the mitochondrial trifunctional protein for FAO activation (Nakayama et al., 2020). Zhao et al., in 2022 showed that lncRNA Carl is an apoptosis-related lncRNA; during intestinal inflammation, the expression or overexpression of the lncRNA Carl leads to the expression of IL-1 beta and of the ptgs2 gene via its interaction with NF-kB P65; lncRNA CHRF leads to an increase in cytokine and oxidant levels following its overexpression, it also triggers the inflammation signaling pathway by activating MyD88 through miR-489; lncRNA FIRRE acts at the post-transcriptional level to regulate proinflammatory genes by interacting with hnRNPU proteins and by binding to the p56 subunit of NFkB; lncRNA PTPRE-AS1 acts via the MAPK/ERK1/2 signaling pathway to regulate inflammation via increasing and decreasing levels of TNF-alpha and IL-10 respectively; Long intergenic RNA not encoding proteins 638 (Linc00638) is implicated in several autoimmune diseases (Yanqiu et al., 2022). It regulates oxidative stress and inflammation especially by targeting inflammatory cytokines like IL-6, IL-17, IL-23 and regulating the Nrf2HO-1 pathway in rheumatoid arthritis (Sun et al., 2021). In human breast cancer, Small interfering RNA (siRNA) has been found to downregulate Notch-1 expression thereby causing an increase in chemosensitivity (Yang et al., 2010) while in prostate cancer, Notch silencing by siRNA increased chemoresistance by promoting cell growth inhibition (Ye et al., 2012). Piwi-interacting RNAs (piRNAs) have been reported to play a role in hepatocellular carcinoma, renal cell cancer, multiple myeloma (Yan et al., 2014), cardiovascular diseases (Yang J. et al., 2018), Alzheimer’s disease, and Parkinson’s disease (Qiu et al., 2017; Schulze et al., 2018).
We also observed that some lncRNAs can regulate oxidative stress and inflammation at the same time. The lncRNA Linc00638 acts by activating the Nrf2/HO-1 signaling pathways following an increase in its level of expression to inhibit oxidative stress and inflammation in rheumatoid arthritis (Sun et al., 2021); lncRNA OIP5-AS1 promotes inflammation and oxidative stress via up modulation of miR-128-3P expression to inhibit cyclin-dependent kinase 2A (Li et al., 2019).
6 Conclusion
We reviewed natural products that display biological effects on oxidative stress and inflammation-associated disorders via mechanisms involving the targeting of ncRNAs. Since this research axis is still emerging, there is a pressing need to shed more light on the mechanisms of these ncRNA-mediated biological activities which will advance the valorization of these natural compounds in the control and treatment of oxidative stress- and inflammation-associated disorders. Furthermore, an investigation is also warranted in the areas of the unknown ncRNAs-mediated mechanisms underlying natural products’ bioactivities and effects, ncRNAs involved in oxidative stress and inflammation-related disorders on which natural products have not been investigated as well as more natural products which may be implicated in common therapeutic remedies but whose effect has not been evaluated in the domain of oxidative stress and inflammation-related disorders.
Author contributions
OT, JN, and FT designed the writing plan. JN, MT, CK worked on the introduction, the relationship between oxidative stress and inflammation, and disorders associated with oxidative stress and inflammatory imbalance. FT, JN, SN, and CM did the literature search on ncRNAs involved in oxidative stress and inflammatory disorders as well as the regulation of ncRNAs by natural products in oxidative stress and inflammatory disorders. JN, CM, MT, US, NN, and FT did the literature search on ncRNAs, oxidative stress, and natural products involved in the modulation of oxidative stress and inflammatory disorders. JN wrote the conclusion. JN, FT, US, MT, SN, MT, CK, NN, and CM wrote the draft. DF, AM, AN, and OT reviewed, edited, and proofread the manuscript. All authors contributed to the article and approved the submitted version.
Conflict of interest
The authors declare that the research was conducted in the absence of any commercial or financial relationships that could be construed as a potential conflict of interest.
Publisher’s note
All claims expressed in this article are solely those of the authors and do not necessarily represent those of their affiliated organizations, or those of the publisher, the editors and the reviewers. Any product that may be evaluated in this article, or claim that may be made by its manufacturer, is not guaranteed or endorsed by the publisher.
References
Abbasi, B., Iqbal, J., Mahmood, T., Khalil, A. T., Ali, B., Kanwal, S., et al. (2018). Role of dietary phytochemicals in modulation of miRNA expression: Natural swords combating breast cancer. Asian Pac J. Trop. Med. 11, 501–509. doi:10.4103/1995-7645.242314
Abdullah, M. A. (2020). Targeting micro-RNAs by natural products: A novel future therapeutic strategy to combat cancer. Am. J. Transl. Res. 12 (7), 3531–3556. Published online 2020 Jul 15, PMCID: PMC7407688. PMID: 32774718.
Aghasafari, P., George, U., and Pidaparti, R. (2019). A review of inflammatory mechanism in airway diseases. Inflamm. Res. 68, 59–74. doi:10.1007/s00011-018-1191-2
Ahmad, A., Sarkar, S. H., Bitar, B., Ali, S., Aboukameel, A., Sethi, S., et al. (2012). Garcinol regulates EMT and Wnt signaling pathways in vitro and in vivo, leading to anticancer activity against breast cancer cells. Mol. Cancer Ther. 11 (10), 2193–2201. PMID: 2282PMCID: PMC3836047. doi:10.1158/1535-7163.MCT-12-0232-T
Ahmed, M. A. E-B. (2014). “Sequence analysis of industrially important genes from trichoderma,” in Biotechnology and Biology of Trichoderma. Editors V. K. Gupta, M. Schmoll, A. Herrera-Estrellaet al. (Elsevier), 377–392.
Al Aameri, R. F. H., Sheth, S., Alanisi, E. M. A., Borse, V., Mukherjea, D., Rybak, L. P., et al. (2017). Tonic suppression of PCAT29 by the IL-6 signaling pathway in prostate cancer: Reversal by resveratrol. PLoS One 12, e0177198. doi:10.1371/journal.pone.0177198
Alharris, E., Alghetaa, H., Seth, R., Chatterjee, S., Singh, N. P., Nagarkatti, M., et al. (2018). Resveratrol attenuates allergic asthma and associated inflammation in the lungs through regulation of miRNA-34a that targets FoxP3 in mice. Front. Immunol. 9, 2992. doi:10.3389/fimmu.2018.02992
Alsahli, M. A., Almatroodi, S. A., Almatroudi, A., Khan, A. A., Anwar, S., Almutary, A. G., et al. (2021). 6-Gingerol, a major ingredient of ginger attenuates diethylnitrosamine-induced liver injury in rats through the modulation of oxidative stress and anti-inflammatory activity. Mediat. Inflamm. 2021, 6661937. doi:10.1155/2021/6661937
Aylan, O., and Metin, O. (2015). Biochemistry of reactive oxygen and nitrogenspecies. London, UK: IntechOpen. doi:10.5772/61193
Ba, L., Gao, J., Chen, Y., Qi, H., Dong, C., Pan, H., et al. (2019). Allicin attenuates pathological cardiac hypertrophy by inhibiting autophagy via activation of PI3K/Akt/mTOR and MAPK/ERK/mTOR signaling pathways. Phytomedicine 58, 152765. doi:10.1016/j.phymed.2018.11.025
Babajide, O. A., Olajide, T. A., and Olayinka, E. T. (2022). 6-gingerol attenuates pulmonary inflammation and oxidative stress in mice model of house dust mite-induced asthma. Adv. Redox Res. 5, 100036. doi:10.1016/j.arres.2022.100036
Bai, X., Geng, J., Li, X., Wan, J., Liu, J., Zhou, Z. M., et al. (2018). Long noncoding RNA LINC01619 regulates MicroRNA-27a/forkhead box protein O1 and endoplasmic reticulum stress-mediated podocyte injury in diabetic nephropathy. Antioxidants Redox Signal. 29, 355–376. doi:10.1089/ars.2017.7278
Baldinu, P., Cossu, A., Manca, A., Satta, M. P., Sini, M. C., Rozzo, C., et al. (2004). Identification of a novel candidate gene, CASC2, in a region of common allelic loss at chromosome 10q26 in human endometrial cancer. Buzz. Mutat. 23, 318–326. doi:10.1002/humu.20015
Banerjee, J., Khanna, S., and Bhattacharya, A. (2017). MicroRNA regulation of oxidative stress. Oxid. Med. Cell. Longev. 2017, 28721156. doi:10.1155/2017/2872156
Beaver, L. M., Kuintzle, R., Buchanan, A., Wiley, M. W., Glasser, S. T., Wong, C. P., et al. (2017). Long noncoding RNAs and sulforaphane: A target for chemoprevention and suppression of prostate cancer. J. Nutr. Biochem. 42, 72–83. doi:10.1016/j.jnutbio.2017.01.001
Belaïch, R., and Boujraf, S. (2016). Facteurs inflammatoires et stress oxydant chez les hémodialysés: Effets et stratégies thérapeutiques. Médecine Des. Mal. Métaboliques 10, 38–42. doi:10.1016/s1957-2557(16)30009-8
Bladé, C., Baselga-Escudero, L., Salvadó, M. J., and Arola-Arnal, A. (2013). miRNAs, polyphenols, and chronic disease. Mol. Nutr. Food Res. 57 (1), 58–70. doi:10.1002/mnfr.201200454
Bowen, D., and Joong, S. S. (2016). Targeting epithelial–mesenchymal transition (EMT) to overcome drug resistance in cancer.
Brieger, K., Schiavone, S., Miller, F. J., and Krause, K. H. (2012). Reactive oxygen species: From health to disease. Swiss Med. Wkly. 142, 13659. doi:10.4414/smw.2012.13659
Calle, M. C., and Fernandez, M. L. (2012). Inflammation and type 2 diabetes. Diabetes Metab. 38 (3), 183–191. doi:10.1016/j.diabet.2011.11.006
Carpi, S., Polini, B., Manera, C., Maria, D., Salsano, J. E., Macchia, M., et al. (2020). MiRNA modulation and antitumor activity by the extra-virgin olive oil polyphénol Oleacein in human melanoma cells. Front. Pharmacol. 11, 574317. doi:10.3389/fphar.2020.574317
Castellon, X., and Bogdanova, V. (2016). Chronic inflammatory diseases and endothelial dysfunction. Aging Dis. 7 (1), 81–89. PMID: 26815098. doi:10.14336/AD.2015.0803
Cesmeli, S., Goker Bagca, B., Caglar, H. O., Ozates, N. P., Gunduz, C., and Biray Avci, C. (2022). Combination of resveratrol and BIBR1532 inhibits proliferation of colon cancer cells by repressing expression of LncRNAs. Med. Oncol. 39, 12–10. doi:10.1007/s12032-021-01611-w
Chang, W. (2017). Non-coding RNAs and berberine: A new mechanism of its anti-diabetic activities. Eur. J. Pharmacol. 795, 8–12. doi:10.1016/j.ejphar.2016.11.055
Charles, R., Lalith, J., and Eichhorn, P. J. A. (2018). Platforms for investigating LncRNA functions. Oak Brook, Illinois, United States: SLAS TECHNOLOGY: Translating Life Sciences Innovation, 247263031878063. doi:10.1177/2472630318780639
Chaves, S. K., Feitosa, C. M., and da S Araújo, L. (2016). Alkaloids pharmacological activities - prospects for the development of phytopharmaceuticals for neurodegenerative diseases. Curr. Pharm. Biotechnol. 17 (7), 629–635. doi:10.2174/138920101707160503201541
Chen, D., Daniel, K. G., Kuhn, D. J., Kazi, A., Bhuiyan, M., Li, L., et al. (2004). Green tea and tea polyphenols in cancer prevention. Front. Biosci. 9, 2618–2631. doi:10.2741/1421
Chen, X., Tan, X. R., Li, S. J., and Zhang, X. X. (2019). LncRNA NEAT1 promotes hepatic lipid accumulation via regulating miR-146a-5p/ROCK1 in nonalcoholic fatty liver disease. Life Sci. 235, 116829. doi:10.1016/j.lfs.2019.116829
Chen, Y., Huang, C., Zhu, S. Y., Zou, H. C., Xu, C. Y., and Chen, Y. X. (2021a). Overexpression of HOTAIR attenuates Pi-induced vascular calcification by inhibiting Wnt/β-catenin through regulating miR-126/Klotho/SIRT1 axis. Mol. Cell. Biochem. 476, 3551–3561. doi:10.1007/s11010-021-04164-8
Chen, Y., Xu, H., Liu, C., Gu, M., Zhan, M., Chen, Q., et al. (2021b). LncRNA DIO3OS regulated by TGF-β1 and resveratrol enhances epithelial mesenchymal transition of benign prostatic hyperplasia epithelial cells and proliferation of prostate stromal cells. Transl. Androl. Urol. 10, 643–653. doi:10.21037/tau-20-1169
Cheng, J., Chen, T., Li, P., Wen, J., Pang, N., Zhang, L., et al. (2018). Sodium tanshinone IIA sulfonate prevents lipopolysaccharide-induced inflammation via suppressing nuclear factor-κB signaling pathway in human umbilical vein endothelial cells. Can. J. Physiol. Pharmacol. 96, 26–31. doi:10.1139/cjpp-2017-0023
Chu, P. M., Yu, C. C., Tsai, K. L., and Hsieh, P. L. (2022). Regulation of oxidative stress by long non-coding RNAs in vascular complications of diabetes. Life (Basel) 12 (2), 274. doi:10.3390/life12020274
Cote, B., Elbarbry, F., Bui, F., Su, J. W., Seo, K., Nguyen, A., et al. (2022). Mechanistic basis for the role of phytochemicals in inflammation-associated chronic diseases. Molecules 27 (3), 781. doi:10.3390/molecules27030781
Coussens, L. M., and Werb, Z. (2002). Inflammation and cancer. Nature 420, 860–867. doi:10.1038/nature01322
Cui, C., Liu, Y., Gerloff, D., Rohde, C., Pauli, C., Köhn, M., et al. (2021). NOP10 predicts lung cancer prognosis and its associated small nucleolar RNAs drive proliferation and migration. Oncogene 40, 909–921. doi:10.1038/s41388-020-01570-y
Cui-Cui, Z., and Fang, N. (2019). LncRNA NEAT1 promotes inflammatory response in sepsis-induced liver injury via the Let-7a/TLR4 axis. Int. Immunopharmacol. 75, 1567–5769.
Daoqi, Z., Shao, M., Yang, J., Fang, M., Liu, S., Lou, D., et al. (2020). Curcumin enhances radiosensitization of nasopharyngeal carcinoma via mediating regulation of tumor stem-like cells by a CircRNA network. J. Cancer. 11 (8), 2360–2370. doi:10.7150/jca.39511
De Bacco, F., Luraghi, P., Medico, E., Reato, G., Girolami, F., Perera, T., et al. (2011). Induction of MET by ionizing radiation and its role in radioresistance and invasive growth of cancer. Jnci J. Natl. Cancer Inst. 103, 645–661. doi:10.1093/jnci/djr093
Deg, L. L., Chi, Y. Y., Liu, L., Huang, N. S., Wang, L., and Wu, J. (2016). LINC00978 predicts poor prognosis in breast cancer patients. Sci. Rep. 6, 37936. doi:10.1038/srep37936
Deng, Q., Wen, R., Liu, S., Chen, X., Song, S., Li, X., et al. (2020). Increased long noncoding RNA maternally expressed gene 3 contributes to podocyte injury induced by high glucose through regulation of mitochondrial fission. Cell. death Dis. 11, 814. doi:10.1038/s41419-020-03022-7
Dhar, S., Kumar, A., Rimando, A. M., Zhang, X., and Levenson, A. S. (2015). Resveratrol and pterostilbene epigenetically restore PTEN expression by targeting oncomiRs of the miR-17 family in prostate cancer. Oncotarget 6, 27214–27226. doi:10.18632/oncotarget.4877
Domitrović, R., and Potočnjak, I. (2016). A comprehensive overview of hepatoprotective natural compounds: Mechanism of action and clinical perspectives. Arch. Toxicol. 90 (1), 39–79. PMID: 26377694. doi:10.1007/s00204-015-1580-z
Donath, M. Y. (2021). Glucose or insulin, which is the culprit in patients with COVID-19 and diabetes? Cell. Metab. 33 (1), 2–4. PMID: 3324PMCID: PMC7685630. doi:10.1016/j.cmet.2020.11.015
Dutta, S., Mahalanobish, S., Saha, S., Ghosh, S., and Sil, P. C. (2019). Natural products: An upcoming therapeutic approach to cancer. Food Chem. Toxicol. 128, 240–255. doi:10.1016/j.fct.2019.04.012
Dzobo, K. (2022). The role of natural products as sources of therapeutic agents for innovative drug discovery. Compr. Pharmacol. 2022, 408–422. doi:10.1016/B978-0-12-820472-6.00041-4
Eddaikra, A., and Eddaikra, N. (2021). ‘Endogenous enzymatic antioxidant defense and pathologies’. Antioxidants - benefits, sources, mechanisms of action. London, UK: IntechOpen. doi:10.5772/intechopen.95504
Elham, S. T., Chamasemani, A., Firouzabadi, N., and Mousaei, M. (2022). ncRNAs and polyphenols: new therapeutic strategies for hypertension. RNA Biol. 19 (1), 575–587. doi:10.1080/15476286.2022.2066335
Enna, S. J., Williams, M., Barret, J. F., Ferkany, J. W., Kenakin, T., and Porsolt, R. D. (2001). Current protocols in Pharmacology || natural products as a foundation for drug discovery. doi:10.1002/0471141755.ph0911s46
Erdmann, V. A., Barciszewska, M. Z., Szymanski, M., Hochberg, A., de Groot, N., and Barciszewski, J. (2001). The non-coding RNAs as riboregulators. Nucleic Acids Res. 29, 189–193. doi:10.1093/nar/29.1.189
Eseberri, I., Lasa, A., Miranda, J., Gracia, A., and Portillo, M. P. (2017). Potential miRNA involvement in the anti-adipogenic effect of resveratrol and its metabolites. PLoS One 12, e0184875. doi:10.1371/journal.pone.0184875
Esteller, M. (2011). Non-coding RNAs in human disease. Nat. Rev. Genet. 12, 861–874. doi:10.1038/nrg3074
Fan, G., Jiang, X., Wu, X., Fordjour, P. A., Miao, L., Zhang, H., et al. (2016). Anti-inflammatory activity of tanshinone IIA in LPS-stimulated RAW264.7 macrophages via miRNAs and TLR4-NF-κ B pathway. Inflammation 39, 375–384. doi:10.1007/s10753-015-0259-1
Fang, Z. Y., Zhang, M., Liu, J. N., Zhao, X., Zhang, Y. Q., and Fang, L. (2021). Tanshinone IIA: A review of its anticancer effects. Front. Pharmacol. 11, 611087. doi:10.3389/fphar.2020.611087
Fazal, F. M., Han, S., Parker, K. R., Kaewsapsak, P., Xu, J., Boettiger, A. N., et al. (2019). Atlas of Subcellular RNA localization revealed by APEX-Seq. Cell. 178 (2), 473–490. doi:10.1016/j.cell.2019.05.027
Fong, N., Kim, H., Zhou, Y., Ji, X., Qiu, J., Saldi, T., et al. (2014). Pre-mRNA splicing is facilitated by an optimal RNA polymerase II elongation rate. Genes Dev. 28 (23), 2663–2676. doi:10.1101/gad.252106.114
Gabriele, P., Irrera, N., Cucinotta, M., Pallio, G., Mannino, F., Arcoraci, V., et al. (2017). Oxidative stress: Harms and benefits for human health. doi:10.1155/2017/8416763
Gámez-Valero, A., Guisado-Corcoll, A., Herrero-Lorenzo, M., Solaguren-Beascoa, M., and Martí, E. (2020). Non-coding RNAs as sensors of oxidative stress in neurodegenerative diseases. Antioxidants (Basel) 9 (11), 1095. doi:10.3390/antiox9111095
Gandy, K. A. O., Zhang, J., Nagarkatti, P., and Nagarkatti, M. (2019). Resveratrol (3, 5, 4'-Trihydroxy-trans-Stilbene) attenuates a mouse model of multiple sclerosis by altering the miR-124/sphingosine kinase 1 Axis in encephalitogenic T cells in the brain. J. Neuroimmune Pharmacol. 14 (3), 462–477. PMID: 30941623. doi:10.1007/s11481-019-09842-5
Gao, J., Liang, Z., Zhao, F., Liu, X., and Ma, N. (2022). Triptolide inhibits oxidative stress and inflammation via the microRNA-155-5p/brain-derived neurotrophic factor to reduce podocyte injury in mice with diabetic nephropathy. Bioengineered 13 (5), 12275–12288. doi:10.1080/21655979.2022.2067293
Ge, X., Xu, B., Xu, W., Xia, L., Xu, Z., Shen, L., et al. (2019). Long non-coding RNA GAS5 inhibits cell proliferation and fibrosis in diabetic nephropathy by sponging miR-221 and modulating SIRT1 expression. Aging 11, 8745–8759. doi:10.18632/aging.102249
Geng, W., Guo, X., Zhang, L., Ma, Y., Wang, L., Liu, Z., et al. (2018). Resveratrol inhibits proliferation, migration and invasion of multiple myeloma cells via NEAT1-mediated wnt/β-catenin signaling pathway. Biomed. Pharmacother. 107, 484–494. doi:10.1016/j.biopha.2018.08.003
Ghafouri-Fard, S., Shoorei, H., and Taheri, M. (2020). Non-coding RNAs are involved in the response to oxidative stress. Biomed. Pharmacother. 127, 110228. doi:10.1016/j.biopha.2020.110228
Giacco, F., and Brownlee, M. (2010). Oxidative stress and diabetic complications. Circ. Res. 107 (9), 1058–1070. doi:10.1161/CIRCRESAHA.110.223545
Giordo, R., Wehbe, Z., Posadino, A. M., Erre, G. L., Eid, A. H., Mangoni, A. A., et al. (2022). Disease-associated regulation of non-coding RNAs by resveratrol: Molecular insights and therapeutic applications. Front. Cell. Dev. Biol. 10, 894305. doi:10.3389/fcell.2022.894305
Gracia, A., Miranda, J., Fernández-Quintela, A., Eseberri, I., Garcia-Lacarte, M., Milagro, F. I., et al. (2016). Involvement of miR-539-5p in the inhibition of de novo lipogenesis induced by resveratrol in white adipose tissue. Food Funct. 7, 1680–1688. doi:10.1039/c5fo01090j
Gusic, M., and Prokisch, H. (2020). ncRNAs: New players in mitochondrial health and disease? Front. Genet. 11, 95. doi:10.3389/fgene.2020.00095
He-da, Z., Sun, D., Mao, L., Zhang, J., Jiang, L., Li, J., et al. (2015). MiR-139-5p inhibits the biological function of breast cancer cells by targeting Notch1 and mediates chemosensitivity to docetaxel. Biochem. Biophys. Res. Commun. 465 (4), 702–713. doi:10.1016/j.bbrc.2015.08.053
Heubach, J., Monsior, J., Deenen, R., Niegisch, G., Szarvas, T., Niedworok, C., et al. (2015). The long noncoding RNA HOTAIR has tissue and cell type-dependent effects on HOX gene expression and phenotype of urothelial cancer cells. Mol. Cancer. 14, 108. doi:10.1186/s12943-015-0371-8
Hong, S., Youk, T., Lee, S. J., Kim, K. M., and Vajdic, C. M. (2020). Bone metastasis and skeletal-related events in patients with solid cancer: A Korean nationwide health insurance database study. PLoS ONE 15 (7), e0234927. doi:10.1371/journal.pone.0234927
Hu, L., Zhao, J., Liu, Y., Liu, X., Lu, Q., Zeng, Z., et al. (2020). Geniposide inhibits proliferation and induces apoptosis of diffuse large B-cell lymphoma cells by inactivating the HCP5/miR-27b-3p/MET axis. Int. J. Med. Sci. 17 (17), 2735–2743. doi:10.7150/ijms.51329
Hu, M., Wang, R., Li, X., Fan, M., Lin, J., Zhen, J., et al. (2017). LncRNA MALAT1 is dysregulated in diabetic nephropathy and involved in high glucose-induced podocyte injury via its interplay with β-catenin. J. Cell. Mol. Med. 21, 2732–2747. doi:10.1111/jcmm.13189
Huang, C., Dong, J., Jin, X., Zhang, D., and Wang, F., (2020). Intestinal anti-inflammatory effects of fuzi-ganjiang herb pair against DSS-induced ulcerative colitis in mice. J. Ethnopharmacol. 261, 112951. PMID: 32574670. doi:10.1016/j.jep.2020.112951
Huang, L., and Hu, X. (2021). Molecular mechanisms and functions of lncRNAs in the inflammatory reaction of diabetes mellitus. Int. J. Endocrinol. 2021, 2550399. doi:10.1155/2021/2550399
Huang, Z. H., Du, Yp., Wen, Jt., Lu, B. F., and Zhao, Y. (2022). snoRNAs: functions and mechanism in biological processes, and roles in tumor pathophysiology. Cell. Death Discov. 8 (1), 259. doi:10.1038/s41420-022-01056-8
Hussain, T., Tan, B., Yin, Y., Blachier, F., Tossou, M. C., and Rahu, N. (2016). Oxidative stress and inflammation: What polyphenols can do for us? Oxid. Med. Cell. Longev. 2016, 7432797. doi:10.1155/2016/7432797
Imai-Sumida, M., Majid, S., Dasgupta, P., Kulkarni, P., Saini, S., Bhagirath, D., et al. (2017). Abstract 3449: Genistein inhibits renal cancer progression through long non-coding RNA HOTAIR suppression. Cancer Res. 77, 3449. doi:10.1158/1538-7445.am2017-3449
Infante-Menéndez, J., González-López, P., Huertas-Lárez, R., Gómez-Hernández, A., and Escribano, Ó. (2023). Oxidative stress modulation by ncRNAs and their emerging role as therapeutic targets in atherosclerosis and non-alcoholic fatty liver disease. Antioxidants 12, 262. doi:10.3390/antiox12020262
Irshad, R., and Husain, M. (2021). Natural products in the reprogramming of cancer epigenetics. Toxicol. Appl. Pharmacol. 417, 115467. PMID: 33631231. doi:10.1016/j.taap.2021.115467
Iyer, S. S., Pulskens, W. P., Sadler, J. J., Butter, L. M., Teske, G. J., Ulland, T. K., et al. (2009). Necrotic cells trigger a sterile inflammatory response through the Nlrp3 inflammasome. Proc. Natl. Acad. Sci. U. S. A. 106 (48), 20388–20393. doi:10.1073/pnas.0908698106
Jabeen, S., Hanifa, M. S., Khan, M. M., and Qadri, R. W. K. (2014). Natural products sources and their active compounds on disease prevention: A review. IJCBS 6, 76–83.
Javad, H., Morvaridzadeh, M., Maroufizadeh, S., Akbari, A., Yavari, M., Amirinejad, A., et al. (2019). Omega-3 fatty acids supplementation and oxidative stress parameters: A systematic review and meta-analysis of clinical trials. Pharmacol. Res. 149, 104462. PMID: 31563611. doi:10.1016/j.phrs.2019.104462
Javaid, A., Zahra, D., Rashid, F., Mashraqi, M., Alzamami, A., Khurshid, M., et al. (2022). Regulation of micro-RNA, epigenetic factor by natural products for the treatment of cancers: Mechanistic insight and translational association. Saudi J. Biol. Sci. 29 (6), 103255. doi:10.1016/j.sjbs.2022.03.005
Jiabin, Y., Zhu, D., Liu, S., Shao, M., Liu, Y., Li, A., et al. (2019). Curcumin enhances radiosensitization of nasopharyngeal carcinoma by regulating circRNA network.
Ji-An, Z., Nie, W., Dong, L., Liu, W., and Wei, W. (2021). A curcumin analog GL63 inhibits the malignant behaviors of hepatocellular carcinoma by inactivating the JAK2/STAT3 signaling pathway via the circular RNA zinc finger protein 83/microRNA-324-5p/cyclin-dependent kinase 16 axis.
Joye, K. W., Ash, S. L., and Catignani, G. L. (2004). Antioxidants and prevention of chronic disease. Crit. Rev. Food Sci. Nutr. 44 (4), 275–295. doi:10.1080/10408690490468489
Juan, C. A., Pérez de la Lastra, J. M., Plou, F. J., and Pérez-Lebeña, E. (2021). The chemistry of reactive oxygen species (ROS) revisited: Outlining their role in biological macromolecules (DNA, lipids and proteins) and induced pathologies. Int. J. Mol. Sci. 22 (9), 4642. doi:10.3390/ijms22094642
Kalalinia, F., and Karimi-Sani, I. (2017). Anticancer properties of solamargine: A systematic review. Phytother. Res. 31 (6), 858–870. PMID: 28383149. doi:10.1002/ptr.5809
Kalhori, M. R., Khodayari, H., Khodayari, S., Vesovic, M., Jackson, G., Farzaei, M. H., et al. (2021). Regulation of long non-coding RNAs by plant secondary metabolites: A novel anticancer therapeutic approach.
Kamal, F., Khan, M. A., Lee-Smith, W., Sharma, S., Imam, Z., Jowhar, D., et al. (2022). Outcomes of endoscopic submucosal dissection for treatment of superficial pharyngeal cancers: Systematic review and meta-analysis. Dig. Dis. Sci. 67, 3518–3528. doi:10.1007/s10620-021-07225-6
Kashyap, D., Tuli, H. S., Yerer, M. B., Sharma, A., Sak, K., Srivastava, S., et al. (2021). Natural product-based nanoformulations for cancer therapy: Opportunities and challenges. Semin. Cancer Biol. 69, 5–23. doi:10.1016/j.semcancer.2019.08.014
Khansari, N., Shakiba, Y., and Mahmoudi, M. (2009). Chronic inflammation and oxidative stress as a major cause of age- related diseases and cancer. Recent Pat. Inflamm. Allergy Drug Discov. 3 (1), 73–80. doi:10.2174/187221309787158371
Khatkar, A., and Sharma, K. K. (2019). Phenylpropanoids and its derivatives: Biological activities and its role in food, pharmaceutical and cosmetic industries. Crit. Rev. Food Sci. Nutr. 60, 1–21. doi:10.1080/10408398.2019.1653822
Khosravi, A. R., and Alder, D. J. (2016). Chitin-induced airway epithelial cell innate immune responses are inhibited by carvacrol/thymol. PLoS ONE 11, e0159459. doi:10.1371/journal.pone.0159459
Klebanoff, S. J. (1980). Oxygen metabolism and the toxic properties of phagocytes. Ann. Intern. Med. 93, 480–489. doi:10.7326/0003-4819-93-3-480
Konovalova, J., Gerasymchuk, D., Parkkinen, I., Chmielarz, P., and Domanskyi, A. (2019). Interaction between microRNAs and oxidative stress in neurodegenerative diseases. doi:10.3390/ijms20236055
Kornienko, A. E., Dotter, C. P., Guenzl, P. M., Gisslinger, H., Gisslinger, B., Cleary, C., et al. (2016). Long non-coding RNAs display higher natural expression variation than protein-coding genes in healthy humans. Genome Biol. 17, 14. doi:10.1186/s13059-016-0873-8
Kurek, J. (2019). Alkaloids—their importance in nature and human life. London, UK: IntechOpen. Introductory Chapter: Alkaloids—Their Importance in Nature and for Human Life.
Kyriazis, I. D., Hoffman, M., Gaignebet, L., Lucchese, A. M., Markopoulou, E., Palioura, D., et al. (2021). KLF5 is induced by FOXO1 and causes oxidative stress and diabetic cardiomyopathy. Circ. Res. 128, 335–357. doi:10.1161/CIRCRESAHA.120.316738
Lagos-Quintana, M., Rauhut, R., Lendeckel, W., and Tuschl, T. (2001). Identification of novel genes coding for small expressed RNAs. Science 294, 853–858. doi:10.1126/science.1064921
Latronico, A. C., and Silveira, L. (2018). Genetic and epigenetic control of puberty. doi:10.1016/B978-0-12-801238-3.65226-1
Latruffe, N., Lançon, A., Frazzi, R., Aires, V., Delmas, D., Michaille, J.-J., et al. (2015). Exploring new ways of regulation by resveratrol involving miRNAs, with emphasis on inflammation. Ann. N.Y. Acad. Sci. 1348, 97–106. doi:10.1111/nyas.12819
Lavieri, R., Piccioli, P., Carta, S., Delfino, L., Castellani, P., and Rubartelli, A. (2014). TLR costimulation causes oxidative stress with unbalance of proinflammatory and anti-inflammatory cytokine production. J. Immunol. 192 (11), 5373–5381. doi:10.4049/jimmunol.1303480
Li, H., Chen, X., Liu, J., Chen, M., Huang, M., Huang, G., et al. (2021). Ethanol extract of Centella asiatica alleviated dextran sulfate sodium-induced colitis: Restoration on mucosa barrier and gut microbiota homeostasis. J. Ethnopharmacol. 2021, 113445. doi:10.1016/j.jep.2020.113445
Li, M., Ding, W., Tariq, M. A., Chang, W., Zhang, X., Xu, W., et al. (2018). A circular transcript of ncx1 gene mediates ischemic myocardial injury by targeting miR-133a-3p. Theranostic 8, 5855–5869. doi:10.7150/thno.27285
Li, Q., Pang, L., Yang, W., Liu, X., Su, G., and Dong, Y. (2018). Long non-coding RNA of myocardial infarction associated transcript (LncRNA-MIAT) promotes diabetic retinopathy by upregulating transforming growth factor-β1 (TGF-β1) signaling. Med. Sci. Monit. 24, 9497–9503. PMID: 3059PMCID: PMC6328291. doi:10.12659/MSM.911787
Li, X., Cao, Q., Wang, Y., and Wang, Y. (2019). Retracted article: LncRNA OIP5-AS1 contributes to ox-LDL-induced inflammation and oxidative stress through regulating the miR-128-3p/cdkn2a axis in macrophages.
Li, Y., Gao, X., Wang, Z., Liu, W., Xu, F., Hu, Y., et al. (2020). Circular RNA 4099 aggravates hydrogen peroxide-induced injury by down-regulating microRNA-706 in L02 cells. Life Sci. 241, 116826. doi:10.1016/j.lfs.2019.116826
Li, Y., Wu, X., Gao, H., Jin, J. M., Li, A. X., Kim, Y. S., et al. (2015). Piwi-interacting RNAs (piRNAs) are dysregulated in renal cell carcinoma and associated with tumor metastasis and Cancer-specific survival. Mol. Med. 21, 381–388. doi:10.2119/molmed.2014.00203
Li, Y., Zhang, X., Yang, P., Zhang, Z., and Wu, H. (2022). Preparation methods, structural characteristics, and biological activity of polysaccharides from Salvia miltiorrhiza: A review. J. Ethnopharmacol. 305, 116090. doi:10.1016/j.jep.2022.116090
Liang, X., Xiao, L., Luo, Y., and Xu, J. (2020). Prevalence and risk factors of childhood hypertension in urban-rural areas of China: A cross-sectional study. Int. J. Hypertens. 2020, 2374231. doi:10.1155/2020/2374231
Liang., J., Shen, Y. C., Zhang, X. Y., Chen, C., Zhao, H., and Hu, J. (2020). Circular RNA HIPK3 downregulation mediates hydrogen peroxide-induced cytotoxicity in human osteoblasts. Aging 12, 1159–1170. doi:10.18632/aging.102674
Lippincott, W., and Wilkins, (2016). Natural products: Naturally occurring compounds that are end products of secondary metabolism; often, they are unique compounds for particular organisms or classes of organisms.
Liu, B., Sun, L., Liu, Q., Gong, C., Yao, Y., Lv, X., et al. (2015). A cytoplasmic NF-κB interacting long noncoding RNA blocks IκB phosphorylation and suppresses breast cancer metastasis. Cancer Cell. 27 (3), 370–381. PMID: 25759022. doi:10.1016/j.ccell.2015.02.004
Liu, T., De Los Santos, F, G., and Phan, S. H. (2017). The bleomycin model of pulmonary fibrosis. Methods Mol. Biol. 1627, 27–42. doi:10.1007/978-1-4939-7113-8_2
Liu, Y., Chen, X., Yao, J., and Kang, J. (2019). Circular RNA ACR relieves high glucose-aroused RSC96 cell apoptosis and autophagy via declining microRNA-145-3p. J. Cell. Biochem. 122, 1252. doi:10.1002/jcb.29568
Lodish, H., Berk, A., and Zipursky, S. L. (2000). Molecular cell biology. 4th edition. New York: W. H. Freeman. Section 4.4, The Three Roles of RNA in Protein Synthesis.
Lopez-Mejia, I. C., and Fajas, L. (2014). Cell cycle regulation of mitochondrial function. Curr. Opin. Cell. Biol. 33, 19–25. PMID: 25463842. doi:10.1016/j.ceb.2014.10.006
Lugrin, J., Velin, R., Roumen, N. P., and Liaudet, L. (2014). The role of oxidative stress during inflammatory processes. Biol. Chem. 395 (2), 203–230. doi:10.1515/hsz-2013-0241
Ma, D., Wei, J., Chen, S., Wang, H., Ning, L., Luo, S. H., et al. (2021). Fucoidan inhibits the progression of hepatocellular carcinoma via causing lncRNA LINC00261 overexpression. Front. Oncol. 11, 653902. doi:10.3389/fonc.2021.653902
Mahmoud, T. N., El-Maadawy, W. H., Kandil, Z. A., Khalil, H., El-Fiky, N. M., and El Alfy, T. S. M. A. (2021). Canna x generalis L.H. Bailey rhizome extract ameliorates dextran sulfate sodium-induced colitis via modulating intestinal mucosal dysfunction, oxidative stress, inflammation, and TLR4/NF-ҡB and NLRP3 inflammasome pathways. J. Ethnopharmacol. 269, 113670. PMID: 33301917. doi:10.1016/j.jep.2020.113670
Marseglia, L., Manti, S., D’Angelo, G., Nicotera, A., Parisi, E., Di Rosa, G., et al. (2014). Oxidative stress in obesity: A critical component in human diseases. Int. J. Mol. Sci. 16 (1), 378–400. doi:10.3390/ijms16010378
Ménézo, Y., Entezami, F., Lichtblau, I., Cohen, M., Belloc, S., and Brack, M. (2012). Oxidative stress and fertility: False evidence and bad recipes. Gynecol. Obstet. Fertil. 40 (12), 787–796. PMID: 23177978. doi:10.1016/j.gyobfe.2012.09.032
Meng, T., Qin, W., and Liu, B. (2020). SIRT1 Antagonizes oxidative stress in diabetic vascular complication. front Endocrinol. 11, 568861. doi:10.3389/fendo.2020.568861
Meng, Y., Jin, M., Yuan, D., Zhao, Y., Kong, X., Guo, X., et al. (2022). Solamargine inhibits the development of hypopharyngeal squamous cell carcinoma by decreasing LncRNA HOXA11-as expression. Front. Pharmacol. 13, 887387. doi:10.3389/fphar.2022.887387
Mezdour, H., Hanfer, M., Menad, A., and Ameddah, S. (2017). Oxidative stress and its relationship with the emergence of various stomach damages. Batna J. Med. Sci. 4 (2), 145–148. doi:10.48087/BJMSra.2017.4204
Migdal, C., and Serres, M. (2011). Reactive oxygen species and oxidative stress. Med. Sci. Paris. 27, 405–412. doi:10.1051/medsci/2011274017
Miranda, J. L., Patricia, J. E., Michele, A. M., Moroney, , Hoult, J. R. S., and Halliwell, B. (1991). Inhibition of mammalian 5-lipoxygenase and cyclo-oxygenase by flavonoids and phenolic dietary additives: Relationship to antioxidant activity and to iron ion-reducing ability. Biochem. Pharmacol. 42, 1673–1681. ISSN 0006-2952. doi:10.1016/0006-2952(91)90501-u
Mirzaei, H., Masoudifar, A., Sahebkar, A., Zare, N., Sadri Nahand, J., Rashidi, B., et al. (2018). MicroRNA: A novel target of curcumin in cancer therapy. J. Cell. Physiol. 233 (4), 3004–3015. PMID: 28617957. doi:10.1002/jcp.26055
Mishra, S., Verma, S. S., Rai, V., Awasthee, N., Chava, S., Hui, K. M., et al. (2019). Long non-coding RNAs are emerging targets of phytochemicals for cancer and other chronic diseases. Cell. Mol. Life Sci. 76 (10), 1947–1966. PMID: 3087PMCID: PMC7775409. doi:10.1007/s00018-019-03053-0
Moreira, P. I., Nunomura, A., Nakamura, M., Takeda, A., Shenk, J. C., Aliev, G., et al. (2008). Nucleic acid oxidation in Alzheimer disease. Free Radic. Biol. Med. 44, 1493–1505. doi:10.1016/j.freeradbiomed.2008.01.002
Nakayama, Y., Fujiu, K., Yuki, R., Oishi, Y., Morioka, M. S., Isagawa, T., et al. (2020). A long noncoding RNA regulates inflammation resolution by mouse macrophages through fatty acid oxidation activation. Proc. Natl. Acad. Sci. U. S. A. 117 (25), 14365–14375. doi:10.1073/pnas.2005924117
Nayansi, J., Ryu, J. J., Choi, E., and Kaushik, N. K. (2017). Generation and Role of reactive oxygen and Nitrogen species induced by Plasma, lasers, chemical, Agents and other systems in dentistry. Oxidative Med. Cell. Longev. 2017, 7542540. doi:10.1155/2017/7542540
Needham, E. J., Helmy, A., Zanier, E. R., Jones, J. L., Coles, A. J., and Menon, D. K. (2019). The immunological response to traumatic brain injury. J. Neuroimmunol. 332, 112–125. doi:10.1016/j.jneuroim.2019.04.005
Niki, E. (1997). “Free radicals in chemistry and biochemistry,” in Food and free radicals (New York: Hiramatsu Plenum Press).
Osbourn, A. E., and Lanzotti, V. (2009). Plant-derived natural products introduction to the different classes of natural products. doi:10.1007/978-0-387-85498-4_1
Otsuka, K., Yamamoto, Y., and Ochiya, T. (2018). Regulatory role of resveratrol, a microRNA-controlling compound, in HNRNPA1 expression, which is associated with poor prognosis in breast cancer. Oncotarget 9 (37), 24718–24730. PMID: 2987PMCID: PMC5973863. doi:10.18632/oncotarget.25339
Pahwa, R., Goyal, A., and Jialal, I. (2022). Chronic inflammation. Treasure Island (FL): StatPearls Publishing. Available from: https://www.ncbi.nlm.nih.gov/books/NBK493173.
Palmieri, G., Paliogiannis, P., Sini, M. C., Manca, A., Palomba, G., Doneddu, V., et al. (2017). Long non-coding RNA CASC2 in human cancer. Writ. Rev. Oncol. Hematol. 111, 31–38. doi:10.1016/j.critrevonc.2017.01.003
Pan, Y., Qian, J. X., Lu, S. Q., Chen, J. W., Zhao, X. D., Jiang, Y., et al. (2017). Protective effects of tanshinone IIA sodium sulfonate on ischemia-reperfusion-induced myocardial injury in rats. Iran. J. Basic. Med. Sci. 20, 308–315. doi:10.22038/ijbms.2017.8361
Parasramka, M. A., Ali, S., Banerjee, S., Deryavoush, T., Sarkar, F. H., and Gupta, S. (2013). Garcinol sensitizes human pancreatic adenocarcinoma cells to gemcitabine in association with microRNA signatures. Mol. Nutr. Food Res. 57 (2), 235–248. PMID: 23293055. doi:10.1002/mnfr.201200297
Pereira, R. R., Guerra, J. F. D. C., Lage, N. N., Lopes, J. M. M., and Silva, M. (2016). Açai (euterpe oleracea mart.) upregulates paraoxonase 1 gene expression and activity with concomitant reduction of hepatic steatosis in high-fat diet-fed rats. Oxid. Med. Cell. Longev. 2016, 8379105. doi:10.1155/2016/8379105
Phurpa, W. (2018). Therapeutic applications of natural products in herbal medicines, biodiscovery programs, and biomedicine. J. Biol. Act. Prod. Nat. 8 (1), 1–20. doi:10.1080/22311866.2018.1426495
Piergentili, R., Basile, G., Nocella, C., Carnevale, R., Marinelli, E., Patrone, R., et al. (2022). Using ncRNAs as tools in cancer diagnosis and treatment-the way towards personalized medicine to improve patients' health. Int. J. Mol. Sci. 23 (16), 9353. doi:10.3390/ijms23169353
Prasong, S., Sriko, J., Somanawat, K., Chayanupatkul, M., Klaikeaw, N., and Werawatganon, D. (2022). Genistein mitigated oxidative stress, inflammation and apoptosis in acute L-arginine-induced pancreatitis in mice 2022.
Qiang, Z., Meng, L., Yi, C., Yu, L., Chen, W., and Sha, W. (2019). Curcumin regulates the miR-21/PTEN/Akt pathway and acts in synergy with PD98059 to induce apoptosis of human gastric cancer MGC-803 cells. J. Int. Med. Res. 47, 1288–1297. doi:10.1177/0300060518822213
Qiu, W., Guo, X., Lin, X., Yang, Q., Zhang, W., Zhang, Y., et al. (2017). Transcriptome-wide piRNA profiling in human brains of Alzheimer's disease. Neurobiol. Aging 57, 170–177. doi:10.1016/j.neurobiolaging.2017.05.020
Ramanujam, D., Schön, A. P., Beck, C., Vaccarello, P., Felician, G., Dueck, A., et al. (2021). MicroRNA-21–dependent macrophage-to-fibroblast signaling determines the cardiac response to pressure overload. Circulation 143 (15), 1513–1525. doi:10.1161/CIRCULATIONAHA.120.050682
Rauf, A., Imran, M., Butt, M. S., Nadeem, M., Peters, D. G., and Mubarak, M. S. (2018). Resveratrol as an anti-cancer agent: A review. Crit. Rev. Food Sci. Nutr. 58 (9), 1428–1447. doi:10.1080/10408398.2016.1263597
Ray, P. D., Huang, B. W., and Tsuji, Y. (2012). Reactive oxygen species (ROS) homeostasis and redox regulation in cellular signaling. Cell. Signal 24 (5), 981–990. doi:10.1016/j.cellsig.2012.01.008
Rémy, B., Minty, M., Thomas, C., and Blasco, V. (2022). Implication des bactéries orales et intestinales dans le décours des maladies cardio-métaboliques et du diabète de type 2. Médecine Des. Mal. Métaboliques 16, 121–133. doi:10.1016/j.mmm.2022.01.003
Ren, D., Li, F., Gao, A., Cao, Q., Liu, Y., and Zhang, J. (2020). Hypoxia-induced apoptosis of cardiomyocytes is restricted by ginkgolide B-downregulated microRNA-29. Cell. Cycle 19 (10), 1067–1076. doi:10.1080/15384101.2020.1731651
Ren, T., Zhu, Y., Xia, X., Ding, Y., Guo, J., and Kan, J. (2017). Zanthoxylum alkylamides ameliorate protein metabolism disorder in STZ-induced diabetic rats. J. Mol. Endocrinol. 58 (3), 113–125. doi:10.1530/JME-16-0218
Roy, J., Galano, J. M., Durand, T., Jean-Yves, L. G., and Lee, C. Y. (2017). Physiological role of reactive oxygen species as promoters of natural defenses. FASEB J. 31, 3729–3745. doi:10.1096/fj.201700170R
Ruiz-Manriquez, L. M., Estrada-Meza, C., Benavides-Aguilar, J., Ledesma-Pacheco, S., Torres-Copado, A., Serrano-Cano, F., et al. (2021). Phytochemicals mediated modulation of microRNAs and long non-coding RNAs in cancer prevention and therapy. Phytotherapy Res. 36, 705–729. doi:10.1002/ptr.7338
Ryan, K. A., Smith, M. F., Sanders, M. K., and Ernst, P. B. (2004). Reactive oxygen and nitrogen species differentially regulate Toll-like receptor 4-mediated activation of NF-kappa B and interleukin-8 expression. Infect. Immun. 72, 2123–2130. doi:10.1128/iai.72.4.2123-2130.2004
Sadegh, R., Rajani, H. F., Mohammadkhani, N., Ramirez-Coronel, A. A., and Maleki, M. (2023). Long non-coding RNAs as novel targets for phytochemicals to cease cancer metastasis. Molecular 28, 987. doi:10.3390/molecules28030987
Saghafi, T., Taheri, R. A., Parkkila, S., and Zolfaghari Emameh, R. (2019). Phytochemicals as modulators of long non-coding RNAs and inhibitors of cancer-related carbonic anhydrases. Int. J. Mol. Sci. 20 (12), 2939. doi:10.3390/ijms20122939
Salzano, S., Checconi, P., Hanschmann, E. M., Lillig, C. H., Bowler, L. D., Chan, P., et al. (2014). Linkage of inflammation and oxidative stress via release of glutathionylated peroxiredoxin-2. which acts as a danger signal. Proc. Natl. Acad. Sci. U. S. A. 111 (33), 12157–12162. doi:10.1073/pnas.1401712111
Sanjeev, K. S., Arora, S., Averett, C., Singh, S., and Singh, A. P. (2015). Modulation of MicroRNAs by phytochemicals in cancer: Underlying mechanisms and translational significance. BioMed Res. Int. 2015, 848710. Article ID 848710. doi:10.1155/2015/848710
Schulze, M., Sommer, A., Plötz, S., Farrell, M., Winner, B., Grosch, J., et al. (2018). Sporadic Parkinson's disease derived neuronal cells show disease-specific mRNA and small RNA signatures with abundant deregulation of piRNAs. Acta Neuropathol. Commun. 6 (1), 58. doi:10.1186/s40478-018-0561-x
Shakir, M. S., Li, W., Morris, N. L., Matter, M. S., Colburn, N. H., Kim, Y. S., et al. (2014). Resveratrol prevents tumorigenesis in mouse model of Kras activated sporadic colorectal cancer by suppressing oncogenic Kras expression. Carcinogenesis 35, 2778–2786. doi:10.1093/carcin/bgu209
Shao, J., Shi, C. J., Li, Y., Zhang, F. W., Pan, F. F., Fu, W. M., et al. (2020). LincROR mediates the suppressive effects of curcumin on hepatocellular carcinoma through inactivating wnt/β-catenin signaling. Front. Pharmacol. 11, 847. doi:10.3389/fphar.2020.00847
Sharma, A., Shahzad, B., Rehman, A., Bhardwaj, R., Landi, M., and Zheng, B. (2019). Response of phenylpropanoid pathway and the role of polyphenols in plants under abiotic stress. Molecules 24 (13), 2452. doi:10.3390/molecules24132452
Sharma, U. K., Sharma, A. K., and Pandey, A. K. (2016). Medicinal attributes of major phenylpropanoids present in cinnamon. BMC Complement. Altern. Med. 16, 156. doi:10.1186/s12906-016-1147-4
Shenouda, S. M., Widlansky, M. E., Chen, K., Xu, G., Holbrook, M., Tabit, C. E., et al. (2011). Altered mitochondrial dynamics contributes to endothelial dysfunction in diabetes mellitus. Broadcast 124, 444–453. doi:10.1161/CIRCULATIONAHA.110.014506
Shu, L., Zhao, H., Huang, W., Hou, G., Song, G., and Ma, H. (2020). Resveratrol upregulates mmu-miR-363-3p via the PI3K-akt pathway to improve insulin resistance induced by a high-fat diet in mice. Dmso 13, 391–403. doi:10.2147/dmso.s240956
Sifan, S., and Fang, H. (2021). Curcumin inhibits ovarian cancer progression by regulating circ-PLEKHM3/miR-320a/SMG1 axis.
Singla, R. K., Dubey, A. K., Garg, A., Sharma, R. K., Fiorino, M., Ameen, S. M., et al. (2019). Natural polyphenols: Chemical classification, definition of classes, subcategories, and structures. J. AOAC Int. 102 (5), 1397–1400. PMID: 31200785. doi:10.5740/jaoacint.19-0133
Smith, S., and Tsai, S. C. (2007). The type I fatty acid and polyketide synthases: A tale of two megasynthases. Nat. Product. Rep. 24 (5), 1041–1072. doi:10.1039/b603600g
Song, J., Jun, M., Ahn, M.-R., and Kim, O. Y. (2016). Involvement of miR-Let7A in inflammatory response and cell survival/apoptosis regulated by resveratrol in THP-1 macrophage. Nutr. Res. Pract. 10, 377–384. doi:10.4162/nrp.2016.10.4,377
Soto-Blanco, B. (2022). “Chapter 12 - herbal glycosides in healthcare,” in Herbal biomolecules in healthcare applications. Editors S. C. Mandal, A. K. Nayak, and A. K. Dhara (Cambridge, Massachusetts: Academic Press), 239–282.
Soudeh, G. F., Shoorei, H., and Taheri, M. (2020). Non-coding RNAs are involved in the response to oxidative stress. Biomed. Pharmacother. 127, 110228. doi:10.1016/j.biopha.2020.110228
Statello, L., Guo, C.-J., Chen, L.-L., and Huarte, M. (2021). Gene regulation by long non-coding RNAs and its biological functions. Nat. Rev. Mol. Cell. Biol. 22 (22), 96–118. doi:10.1038/s41580-020-00315-9
Su, Q., Yao, J., and Sheng, C. (2018). Geniposide attenuates LPS-induced injury via up-regulation of miR-145 in H9c2 cells. Inflammation 41, 1229–1237. doi:10.1007/S10753-018-0769-8
Subrata, K. B. (2016). Does the interdependence between oxidative stress and inflammation explain the antioxidant paradox? Oxidative Med. Cell. Longev. 2016, 9. Article ID 5698931. doi:10.1155/2016/5698931
Sun, H. J., Hou, B., Wang, X., Zhu, X. X., Li, K. X., and Qiu, L. Y. (2016). Endothelial dysfunction and cardiometabolic diseases: Role of long non-coding RNAs. Life Sci. 167, 6–11. doi:10.1016/j.lfs.2016.11.005
Sun, Y., Liu, J., Xin, L., Zhou, Q., Chen, X., Ding, X., et al. (2021). The low expression of long non-coding RNA Linc00638 contributes to the progression of rheumatoid arthritis by regulating inflammation and oxidative stress. J. South Med. Univ. 41 (7), 965–971doi. doi:10.12122/j.issn.1673-4254.2021.07.01
Sun, Z., Wang, H., Wang, J., Zhou, L., and Yang, P. (2014). Chemical composition and anti-inflammatory, cytotoxic and antioxidant activities of essential oil from leaves of mentha piperita grown in China. PLoS ONE 9 (12), e114767. doi:10.1371/journal.pone.0114767
Szyman’ski, M., and Barciszewski, J. (2002). Beyond the proteome: Non-coding regulatory RNAs. Genome Biol. 3, reviews0005. doi:10.1186/gb-2002-3-5-reviews0005
Tabas, I., and Glass, C. K. (2013). Anti-inflammatory therapy in chronic disease: Challenges and opportunities. Science 339 (6116), 166–172. doi:10.1126/science.1230720
Tahamtan, A., Teymoori-Rad, M., Nakstad, B., and Salimi, V. (2018). Anti-inflammatory MicroRNAs and their potential for inflammatory diseases treatment. Front. Immunol. 9, 1377. doi:10.3389/fimmu.2018.01377
Tamgue, O., Chia, J. E., and Brombacher, F. (2021). Triptolide modulates the expression of inflammation-associated lncRNA-PACER and lincRNA-p21 in Mycobacterium tuberculosis-infected monocyte-derived macrophages. Front. Pharmacol. 12, 618462. doi:10.3389/fphar.2021.618462
Tu, J., Xing, Y., Guo, Y., Tang, F., Guo, L., and Xi, T. (2012). TanshinoneIIA ameliorates inflammatory microenvironment of colon cancer cells via repression of microRNA-155. Int. Immunopharmacol. 14 (4), 353–361. doi:10.1016/j.intimp.2012.08.015
Uchida, S., and Adams, J. C. (2019). Roles of non-coding RNAs in human diseases [introductory editorial for the theme: Roles of non-coding RNAs in human diseases]. Am. J. Physiology-Cell Physiology. ajpcell. 317, C1–C2. doi:10.1152/ajpcell.00114.2019
Valcheva-Kuzmanova, S., Kuzmanov, K., Tancheva, S., and Belcheva, A. (2007). Hypoglycemic and hypolipidemic effects of Aronia melanocarpa fruit juice in streptozotocin-induced diabetic rats. Methods Find. Exp. Clin. Pharmacol. 29 (2), 101–105. doi:10.1358/mf.2007.29.2.1075349
Venkatadri, R., Muni, T., Iyer, A. K. V., Yakisich, J. S., and Azad, N. (2016). Role of apoptosis-related miRNAs in resveratrol-induced breast cancer cell death. Cell. Death Dis. 7, e2104. doi:10.1038/cddis.2016.6
Veronique, H., and Christine, M. (2012). Evidence for a protective effect of polyphenols-containing foods on cardiovascular health: An update for clinicians. Bethesda, Maryland, United States: NCBI.
Vierbuchen, T., Agarwal, S., Johnson, J. L., Galia, L., Lei, X., Stein, K., et al. (2022). The lncRNA LUCAT1 is elevated in inflammatory disease and restrains inflammation by regulating the splicing and stability of NR4A2. Proc. Natl. Acad. Sci. U. S. A. 120 (1), e2213715120. doi:10.1073/pnas.2213715120
Vinciguerra, M., Sgroi, A., Veyrat-Durebex, C., Rubbia-Brandt, L., Buhler, L. H., and Foti, M. (2009). Unsaturated fatty acids inhibit the expression of tumor suppressor phosphatase and tensin homolog (PTEN) via microRNA-21 up-regulation in hepatocytes. Hepatology 49 (4), 1176–1184. doi:10.1002/hep.22737
Visioli, F., Giordano, E., Nicod, N. M., and Dávalos, A. (2012). Molecular targets of omega 3 and conjugated linoleic Fatty acids - "micromanaging" cellular response. Front. Physiol. 3, 42. doi:10.3389/fphys.2012.00042
Wang, B., and Trayhurn, P. (2006). Acute and prolonged effects of TNF-alpha on the expression and secretion of inflammation-related adipokines by human adipocytes differentiated in culture. Pflüg. Arch. 452, 418–427. doi:10.1007/s00424-006-0055-8
Wang, B., Wang, Y., Xu, K., Zeng, Z., Xu, Z., Yue, D., et al. (2021). Resveratrol alleviates sepsis-induced acute kidney injury by deactivating the lncRNA MALAT1/MiR-205 axis. cejoi 46, 295–304. doi:10.5114/ceji.2021.109195
Wang, L., Zhang, R., Chen, J., Wu, Q., and Kuang, Z. (2017). Baicalin protects against TNF-α-induced injury by down-regulating miR-191a that targets the tight junction protein ZO-1 in IEC-6 cells. Biol. Pharm. Bull. 40 (4), 435–443. PMID: 28111380. doi:10.1248/bpb.b16-00789
Wang, R., Ma, Z., Feng, L., Yang, Y., Tan, C., Shi, Q., et al. (2018). LncRNA MIR31HG targets HIF1A and P21 to facilitate head and neck cancer cell proliferation and tumorigenesis by promoting cell-cycle progression. Mol. Cancer 17 (1), 162. doi:10.1186/s12943-018-0916-8
Wang, W., Yang, N., Yang, Y. H., Wen, R., and Liu, C. F. (2021). Non-coding RNAs: Master regulators of inflammasomes in inflammatory diseases. J. Inflamm. Res. 14, 5023–5050. doi:10.2147/JIR.S332840
Wang, W. H., Chen, J., Zhang, B. R., Lu, S. J., Wang, F., Peng, L., et al. (2018). Curcumin inhibits proliferation and enhances apoptosis in A549 cells by downregulating lncRNA UCA1. Pharmazie 73 (7), 402–407. doi:10.1691/ph.2018.8402
Wang, X., Shen, C., Zhu, J., Shen, G., Li, Z., and Dong, J. (2019). Long noncoding RNAs in the regulation of oxidative stress. Oxid. Med. Cell. Longev. 2019, 1318795. doi:10.1155/2019/1318795
Wang, X., Wang, W., HuangFu, W., Liu, Z., and Zhao, F. (2022). LncRNA HOTAIR facilitates high glucose-induced mesangial cell proliferation, fibrosis and oxidative stress in diabetic nephropathy via regulating miR-147a/WNT2B axis. Diabetol. Metab. Syndr. 14 (33). doi:10.1186/s13098-022-00802-3
Wang, Y., Yang, S. H., Zhong, K., Jiang, T., Zhang, M., Kwan, H. Y., et al. (2020). Network pharmacology-based strategy for the investigation of the anti-obesity effects of an ethanolic extract of Zanthoxylum bungeanumMaxim. Front. Pharmacol. 11, 572387. doi:10.3389/fphar.2020.572387
Wang, Y., Zhao, R., Liu, W., Wang, Z., Rong, J., Long, X., et al. (2019). Exosomal circHIPK3 released from hypoxia-pretreated cardiomyocytes regulates oxidative damage in cardiac microvascular endothelial cells via the miR-29a/IGF-1 pathway. Oxidative Med. Cell. Longev. 2019, 7954657. doi:10.1155/2019/7954657
Wei, X., Zhang, L., Geng, Y., Liu, Y., and Zhang, N. (2020). Long noncoding RNA GAS5 promotes microglial inflammatory response in Parkinson's disease by regulating NLRP3 pathway through sponging miR-223-3p. Int. Immunopharmacol. 85, 106614. ISSN 1567-5769. doi:10.1016/j.intimp.2020.106614
Wu, F., and Cui, L. (2017). Resveratrol suppresses melanoma by inhibiting NF-κB/miR-221 and inducing TFG expression. Arch. Dermatol Res. 309, 823–831. doi:10.1007/s00403-017-1784-6
Xi, W., Pan, Y., Yuan, F., Zhang, J., Xie, Me, Yang, F., et al. (2020). The biogenesis and functions of piRNAs in human diseases. Mol. Ther. - Nucleic Acids 21, 108–120. ISSN 2162-2531. doi:10.1016/j.omtn.2020.05.023
Xiao, X., Shi, D., Liu, L., Wang, J., Xie, X., Kang, T., et al. (2011). Quercetin suppresses cyclooxygenase-2 expression and angiogenesis through inactivation of P300 signaling. PLoS One 6 (8), e22934. doi:10.1371/journal.pone.0022934
Xiaoqing, X., Zhang, X., Zhang, Y., and Wang, Z. (2021). Curcumin suppresses the malignancy of non-small cell lung cancer by modulating the circ-PRKCA/miR-384/ITGB1 pathway.
Xie, C., Kang, J., Li, Z., Schauss, A. G., Badger, T. M., Nagarajan, S., et al. (2012). The açaí flavonoid velutin is a potent anti-inflammatory agent: Blockade of LPS-mediated TNF-α and IL-6 production through inhibiting NF-κB activation and MAPK pathway. J. Nutr. Biochem. 23, 1184–1191. PMID: 22137267. doi:10.1016/j.jnutbio.2011.06.013
Xie, C., Wu, W., Tang, A., Luo, N., and Tan, Y. (2019). lncRNA GAS5/miR-452-5p reduces oxidative stress and pyroptosis of high-glucose-stimulated renal tubular cells. Diabetes, Metabolic Syndrome Obes. Targets Ther. 12, 2609–2617. doi:10.2147/DMSO.S228654
Xie, L., Tang, H., Song, J., Long, J., Zhang, L., and Li, X. (2019). Chrysophanol: A review of its pharmacology, toxicity and pharmacokinetics. J. Pharm. Pharmacol. 71, 1475–1487. doi:10.1111/jphp.13143
Xie, W., Chang, W., Wang, X., Liu, F., Wang, X., Yuan, D., et al. (2022). Allicin inhibits osteosarcoma growth by promoting oxidative stress and autophagy via the inactivation of the lncRNA MALAT1-miR-376a-wnt/β-catenin signaling pathway. Oxid. Med. Cell. Longev. 2022, 4857814. doi:10.1155/2022/4857814
Xu, X., and Zhang, Y. (2022). Regulation of oxidative stress by long non-coding RNAs in central nervous system disorders. Front. Mol. Neurosci. 15, 931704. doi:10.3389/fnmol.2022.931704
Xu, X. H., Ding, D. F., Yong, H. J., Dong, C. L., You, N., Ye, X. L., et al. (2017). Resveratrol transcriptionally regulates miRNA-18a-5p expression ameliorating diabetic nephropathy via increasing autophagy. Eur. Rev. Med. Pharmacol. Sci. 21, 4952–4965.
Xuan, Y., Gao, Y., Huang, H., Wang, X., Cai, Y., and Luan, Q. X. (2017). Tanshinone IIA attenuates atherosclerosis in apolipoprotein E knockout mice infected with porphyromonas gingivalis. Ignition 40, 1631–1642. doi:10.1007/s10753-017-0603-8
Yan, H., Wu, Q. L., Sun, C. Y., Ai, L. S., Deng, J., Zhang, L., et al. (2014). piRNA-823 contributes to tumorigenesis by regulating de novo DNA methylation and angiogenesis in multiple myeloma. Leukemia 29 (1), 196–206. PMID: 24732595. doi:10.1038/leu.2014.135
Yan, X., Hu, Z., Feng, Y., Hu, X., Yuan, J., Zhao, S. D., et al. (2015). Comprehensive genomic characterization of long non-coding RNAs across human cancers. Cancer Cell. 28 (4), 529–540. doi:10.1016/j.ccell.2015.09.006
Yang, J., Xue, F. T., Li, Y. Y., Liu, W., and Zhang, S. (2018). Exosomal piRNA sequencing reveals differences between heart failure and healthy patients. Eur. Rev. Med. Pharmacol. Sci. 22 (22), 7952–7961. doi:10.26355/eurrev_201811_16423
Yang, X., Jiang, J., Zhang, C., and Li, Y. (2019). Baicalein restrains proliferation, migration, and invasion of human malignant melanoma cells by down-regulating colon cancer associated transcript-1. Braz. J. Med. Biol. Res. 52, 8934. doi:10.1590/1414-431X20198934
Yang, Y. Q., Yan, X. T., Wang, K., Tian, R. M., Lu, Z. Y., Wu, L. L., et al. (2018). Triptriolide alleviates lipopolysaccharide-induced liver injury by Nrf2 and NF-κB signaling pathways. Front. Pharmacol. 9, 999. doi:10.3389/fphar.2018.00999
Yang, Z., Li, H., Sun, J., Gao, J., Liu, W., Li, B., et al. (2010). DC-chol/DOPE cationic liposomes: A comparative study of the influence factors on plasmid pDNA and siRNA gene delivery. Int. J. Pharm. 390, 198–207. ISSN 0378-5173. doi:10.1016/j.ijpharm.2010.01.035
Yanqiu, S., Jian, L., Jianting, W., Dan, H., Qin, Z., Xianheng, Z., et al. (2022). Overexpression of the long non-coding RNA LINC00638 inhibits inflammation and oxidative stress in rheumatoid arthritis fibroblast-like synoviocytes by regulating the Nrf2-HO1 pathway. Immun. Inflamm. Dis. 10, e663. doi:10.1002/iid3.663
Yao, S., Gao, M., Wang, Z., Wang, W., Zhan, L., and Wei, B. (2021). Upregulation of MicroRNA-34a sensitizes ovarian cancer cells to resveratrol by targeting bcl-2. Yonsei Med. J. 62, 691–701. doi:10.3349/ymj.2021.62.8.691
Yasuda, M., Ohzeki, Y., Shimizu, S., Naito, S., Ohtsuru, A., Yamamoto, T., et al. (1999). Stimulation of in vitro angiogenesis by hydrogen peroxide and the relation with ETS-1 in endothelial cells. Life Sci. 64, 249–258. doi:10.1016/s0024-3205(98)00560-8
Ye, Q. F., Zhang, Y. C., Peng, X. Q., Long, Z., Ming, Y. Z., and He, L. Y. (2012). siRNA-mediated silencing of Notch-1 enhances docetaxel induced mitotic arrest and apoptosis in prostate cancer cells. Asian Pac J. Cancer Prev. 13 (6), 2485–2489. PMID: 22938409. doi:10.7314/apjcp.2012.13.6.2485
Yongzhong, W., Lu, J., Antony, S., Juhasz, A., Han, L., Jiang, G., et al. (2013). Activation of TLR4 is required for the synergistic induction of dual oxidase 2 and dual oxidase A2 by IFN-γ and lipopolysaccharide in human pancreatic cancer cell lines. J. Immunol. 190 (4), 1859–1872. doi:10.4049/jimmunol.1201725
Yu, X., Tang, W., Yang, Y., Tang, L., Dai, R., Pu, B., et al. (2018). Long noncoding RNA NKILA enhances the anti-cancer effects of baicalein in hepatocellular carcinoma via the regulation of NF-κB signaling. Chem. Interact. 285, 48–58. doi:10.1016/j.cbi.2018.02.027
Yu, Y., Sarkar, F. H., and Majumdar, A. P. N. (2013). Down-regulation of miR-21 induces differentiation of chemoresistant colon cancer cells and enhances susceptibility to therapeutic regimens. Transl. Oncol. 6 (2), 180–186. doi:10.1593/tlo.12397
Yuan, K., Li, X., Lu, Q., Zhu, Q., Jiang, H., Wang, T., et al. (2019). Application and mechanisms of triptolide in the treatment of inflammatory diseases-A review. Front. Pharmacol. 10, 1469. PMID: 31866868. doi:10.3389/fphar.2019.01469
Zha, F., Qu, X., Tang, B., Li, J., Wang, Y., Zheng, P., et al. (2019). Long non-coding RNA MEG3 promotes fibrosis and inflammatory response in diabetic nephropathy via miR-181a/Egr-1/TLR4 axis. Aging 11, 3716–3730. doi:10.18632/aging.102011
Zhang, C. C., and Niu, F. (2019). LncRNA NEAT1 promotes inflammatory response in sepsis-induced liver injury via the Let-7a/TLR4 axis. Int. Immunopharmacol. 75, 105731. doi:10.1016/j.intimp.2019.105731
Zhang, H., Liu, X., Liu, Y., Liu, J., Gong, X., Li, G., et al. (2022). Crosstalk between regulatory non-coding RNAs and oxidative stress in Parkinson's disease. Front. Aging Neurosci. 14, 975248. doi:10.3389/fnagi.2022.975248
Zhang, H., Yan, Y., Hu, Q., and Zhang, X. (2021). LncRNA MALAT1/microRNA let-7f/KLF5 axis regulates podocyte lesions in diabetic nephropathy. Sci. Life 266, 118794. doi:10.1016/j.lfs.2020.118794
Zhang, J., Chen, M., Zhai, Y., and Fu, Y. (2020). Retracted: HOTAIR regulates lipopolysaccharide-induced inflammatory response in hepatocytes. J. Cell. Physiol. 235 (5), 4247–4255. doi:10.1002/jcp.29301
Zhang, L., Ma, R., Gao, M., Zhao, Y., Lv, X., Zhu, W., et al. (2020a). SNORA72 activates the Notch1/c-Myc pathway to promote stemness transformation of ovarian cancer cells. Front. Cell. Dev. Biol. 8, 583087. doi:10.3389/fcell.2020.583087
Zhang, L., Zhao, S., and Zhu, Y. (2020b). Long noncoding RNA growth arrest-specific transcript 5 alleviates renal fibrosis in diabetic nephropathy by downregulating matrix metalloproteinase 9 through recruitment of enhancer of zeste homolog 2. FASEB J. 34, 2703–2714. doi:10.1096/fj.201901380RR
Zhang, R., Ma, X., Jiang, L., Xia, W., Li, H., Zhao, N., et al. (2021). Decreased lncRNA SNHG16 accelerates oxidative stress induced pathological angiogenesis in human retinal microvascular endothelial cells by regulating miR-195/mfn2 Axis. Curr. Pharm. Des. 27, 3047–3060. doi:10.2174/1381612827666210202141541
Zhang, S., Long, F., Lin, H., Wang, X., Jiang, G., and Wang, T. (2021). Regulatory roles of phytochemicals on circular RNAs in cancer and other chronic diseases. Pharmacol. Res. 174, 105936. doi:10.1016/j.phrs.2021.105936
Zhang, W., Lu, C., Cai, S., Feng, Y., Shan, J., and Di, L. (2022). Aconiti lateralis radix praeparata as potential anticancer herb: Bioactive compounds and molecular mechanisms. Front. Pharmacol. 13, 870282. PMID: 35662730. doi:10.3389/fphar.2022.870282
Zhang, X.-L., Zhu, H.-Q., Zhang, Y., Zhang, N. Y., Jiao, J.-S., and Xing, X.-Y. (2020). LncRNA CASC2 regulates high glucose-induced proliferation, extracellular matrix accumulation and oxidative stress of human mesangial cells via miR-133b/FOXP1 axis. Eur. Rev. Med. Pharmacol. Sci. 24, 802–812. doi:10.26355/eurrev_202001_20063
Zhang, Y., Wang, M., Dong, H., Yu, X., and Zhang, J. (2018). Anti-hypoglycemic and hepatocyte-protective effects of hyperoside from Zanthoxylum bungeanum leaves in mice with high-carbohydrate/high-fat diet and alloxan-induced diabetes. Int. J. Mol. Med. 41 (1), 77–86. doi:10.3892/ijmm.2017.3211
Zhao, H., Ming, T., Tang, S., Ren, S., Yang, H., Liu, M., et al. (2022). Wnt signaling in colorectal cancer: Pathogenic role and therapeutic target. Mol. Cancer 21 (1), 144. doi:10.1186/s12943-022-01616-7
Zhao, Q., Pang, G., Yang, L., Chen, S., Xu, R., and Shao, W. (2021). Long noncoding RNAs regulate the inflammatory responses of macrophages. Cells 11 (1), 5. doi:10.3390/cells11010005
Zheng, W., Li, T., Wei, J., Zhang, Y., Zuo, Q., and Lin, Y. (2021). Identification of miR-145 as a regulator of the cardiomyocyte inflammatory response and oxidative stress under hyperglycemia. Exp. Ther. Med. 21, 467. doi:10.3892/etm.2021.9898
Zhenping, G., and Dan, H. (2021). lncRNA GAS 5-mediated miRNA-23a-3p promotes inflammation and cell apoptosis by targeting TLR4 in a cell model of sepsis. doi:10.3892/mmr.2021.12149
Zhou, J., Zhao, Y., Li, Z., Zhu, M., Wang, Z., Li, Y., et al. (2020). miR-103a-3p regulates mitophagy in Parkinson's disease through Parkin/Ambra1 signaling. Pharmacol. Res. 160, 105197. PMID: 32942015. doi:10.1016/j.phrs.2020.105197
Zhou, Y., Wang, F., Li, W., Hong, H., Chen, J., Tian, Y., et al. (2018). Protective effects of microRNA-330 on namyloid B-protein production, oxidative stress, and mitochondrial dysfunction in Alzheimer’s disease by targeting VAV1 via the MAPK signaling pathway.
Zhou, Z., Zhu, Y., Gao, G., and Zhang, Y. (2019). Long noncoding RNA SNHG16 targets miR-146a-5p/CCL5 to regulate LPS-induced WI-38 cell apoptosis and inflammation in acute pneumonia. Life Sci. 228, 189–197. doi:10.1016/j.lfs.2019.05.008
Keywords: oxidative stress, inflammation, disorders, non-coding RNAs, natural products
Citation: Ngum JA, Tatang FJ, Toumeni MH, Nguengo SN, Simo USF, Mezajou CF, Kameni C, Ngongang NN, Tchinda MF, Dongho Dongmo FF, Akami M, Ngane Ngono AR and Tamgue O (2023) An overview of natural products that modulate the expression of non-coding RNAs involved in oxidative stress and inflammation-associated disorders. Front. Pharmacol. 14:1144836. doi: 10.3389/fphar.2023.1144836
Received: 15 January 2023; Accepted: 24 March 2023;
Published: 24 April 2023.
Edited by:
Enrico Sangiovanni, University of Milan, ItalyReviewed by:
Bhargab Kalita, Amrita Vishwa Vidyapeetham (Kochi Campus), IndiaHaibo Xu, Chengdu University of Traditional Chinese Medicine, China
Copyright © 2023 Ngum, Tatang, Toumeni, Nguengo, Simo, Mezajou, Kameni, Ngongang, Tchinda, Dongho Dongmo, Akami, Ngane Ngono and Tamgue. This is an open-access article distributed under the terms of the Creative Commons Attribution License (CC BY). The use, distribution or reproduction in other forums is permitted, provided the original author(s) and the copyright owner(s) are credited and that the original publication in this journal is cited, in accordance with accepted academic practice. No use, distribution or reproduction is permitted which does not comply with these terms.
*Correspondence: Ousman Tamgue, b3VzdGhlbzIwMTRAZ21haWwuY29t
†These authors have contributed equally to this work