- Department of Molecular Medicine, The Scripps Research Institute, La Jolla, CA, United States
Background: The Department of Health and Human Services reports that prescription pain reliever (e.g., oxycodone) misuse was initiated by 4,400 Americans each day in 2019. Amid the opioid crisis, effective strategies to prevent and treat prescription opioid use disorder (OUD) are pressing. In preclinical models, the orexin system is recruited by drugs of abuse, and blockade of orexin receptors (OX receptors) prevents drug-seeking behavior. The present study sought to determine whether repurposing suvorexant (SUV), a dual OX receptor antagonist marketed for the treatment of insomnia, can treat two features of prescription OUD: exaggerated consumption and relapse.
Methods: Male and female Wistar rats were trained to self-administer oxycodone (0.15 mg/kg, i. v., 8 h/day) in the presence of a contextual/discriminative stimulus (SD) and the ability of SUV (0–20 mg/kg, p. o.) to decrease oxycodone self-administration was tested. After self-administration testing, the rats underwent extinction training, after which we tested the ability of SUV (0 and 20 mg/kg, p. o.) to prevent reinstatement of oxycodone seeking elicited by the SD.
Results: The rats acquired oxycodone self-administration and intake was correlated with the signs of physical opioid withdrawal. Additionally, females self-administered approximately twice as much oxycodone as males. Although SUV had no overall effect on oxycodone self-administration, scrutiny of the 8-h time-course revealed that 20 mg/kg SUV decreased oxycodone self-administration during the first hour in males and females. The oxycodone SD elicited strong reinstatement of oxycodone-seeking behavior that was significantly more robust in females. Suvorexant blocked oxycodone seeking in males and reduced it in females.
Conclusions: These results support the targeting of OX receptors for the treatment for prescription OUD and repurposing SUV as pharmacotherapy for OUD.
1 Introduction
Moderate to severe pain management in the United States largely relies on prescription opioids, such as oxycodone, with over 142,000,000 total prescription opioids dispensed in 2020 (Centers for Disease Control and Prevention, 2021). Strikingly, 18.6% of drug overdose fatalities in the same year involved a prescription opioid (Centers for Disease Control and Prevention, 2022). Oxycodone is one of the most prescribed opioids (Wide-Ranging Online Data for Epidemiologic Research, 2021) and, despite similar half-lives (compared to hydrocodone, morphine) or reduced potency (compared to fentanyl, buprenorphine) compared to other prescription opioids, oxycodone ranked highly in abuse potential by patients with opioid use disorder (OUD; Zacny and Lichtor, 2008; Butler et al., 2010; Stoops et al., 2010; Remillard et al., 2019; Kibaly et al., 2021; Vearrier and Grundmann, 2021). Even the appropriate use of prescription opioids can lead to physical dependence and the escalation of drug consumption, contributing to the development of drug misuse and addiction (Volkow et al., 2019). Neuroadaptations that occur in response to repeated drug use contribute to the reinstatement of drug seeking and last well into drug abstinence (Volkow et al., 2019; Koob, 2021). Collectively, these data demonstrate the urgency to develop new therapies to reduce the motivation to seek opioids both before and after periods of abstinence.
The orexin (OX) system, also known as the hypocretin system, is involved in various physiological processes, such as sleep/wake regulation (Chemelli et al., 1999; Hoyer and Jacobson, 2013; Inutsuka and Yamanaka, 2013; Krystal et al., 2013), stress (Berridge et al., 2010; Sargin, 2019), feeding (Sakurai et al., 1998), and reward processing (Harris et al., 2005; Martin-Fardon & Weiss, 2012; Matzeu and Martin-Fardon, 2020). The OX system is strongly recruited by drug use and was shown to incur neuroadaptations with repeated drug use, including cocaine (Zhang et al., 2007; James et al., 2019; Matzeu and Martin-Fardon, 2021), alcohol (Amodeo et al., 2020), nicotine (Kane et al., 2000), and opioids (Georgescu et al., 2003; Thannickal et al., 2018; Fragale et al., 2020; Sadat-Shirazi et al., 2020). Additionally, pharmacological manipulations of OX transmission have been shown to influence drug intake and seeking of alcohol (Moorman and Aston-Jones, 2009; Shoblock et al., 2011; Martin-Fardon & Weiss, 2012), cocaine (Borgland et al., 2006; Martin-Fardon and Weiss, 2014a), and opioids (Smith and Aston-Jones, 2012; Matzeu and Martin-Fardon, 2020). Opioid exposure reportedly increases OX cell counts (Thannickal et al., 2018), and OX cells are activated in the presence of drug-paired stimuli (Harris et al., 2005; Dayas et al., 2008; Jupp et al., 2011; Martin-Fardon et al., 2018). The National Institute on Drug Abuse named OX receptor antagonists and negative allosteric modulators as pharmacotherapies with a high potential of Food and Drug Administration (FDA) approval for the treatment of some aspects of OUD (Rasmussen et al., 2018).
Suvorexant (SUV) is a dual OX receptor antagonist (DORA) approved by the FDA in 2014 and marketed by Merck as Belsomra® for the treatment of insomnia. Suvorexant is highly brain penetrant and has strong and equivalent affinity for both the OX1 receptor and OX2 receptor in both humans (OX1 receptor, Ki = 0.55 nM; OX2 receptor, Ki = 0.35 nM) and rats (OX1 receptor, Ki = 0.54 nM; OX2 receptor, Ki = 0.57 nM; Winrow et al., 2011). Acute oral (p. o.) administration of SUV (10–100 mg) in healthy men yielded maximal plasma concentrations 3 h post-administration (Sun et al., 2013). In rats, p.o. administration of 10 mg/kg SUV yielded maximal plasma concentrations 0.5 h after administration (Cox et al., 2010). Alternative DORAs (i.e., almorexant and DORA-1) have been reported to reduce alcohol intake (Anderson et al., 2014; Srinivasan et al., 2012), interfere with the expression of cocaine- and amphetamine-induced conditioned place preference (CPP; Steiner et al., 2013), reduce nicotine conditioned reinstatement (Winrow et al., 2010), and attenuate morphine-induced locomotor sensitization (Steiner et al., 2013), although these compounds have yet to obtain FDA-approval. The acute administration of SUV, however, reduced stimulant (Simmons et al., 2017; Gentile et al., 2018) and alcohol intake in rats (Flores-Ramirez et al., 2022) and reduced opioid withdrawal and craving in patients who were undergoing buprenorphine taper (Huhn et al., 2022). Therefore, SUV may indirectly reduce the risk to opioid relapse by reducing drug craving (primarily via OX1 receptor) and normalizing sleep disturbances (primarily via OX2 receptor) commonly observed in OUD patients (Peles et al., 2006; Hartwell et al., 2014).
The present study first investigated the ability of SUV to reduce excessive oxycodone intake, utilizing a model of extended oxycodone self-administration access in male and female rats. Effectively treating OUD will require strategies that not only prevent excessive opioid consumption, but also resolve a central problem in treating OUD that is chronic relapsing to opioid use, even after extended periods of abstinence (Rudd et al., 2016; Gostin et al., 2017; Volkow and Collins, 2017). Relapse is often precipitated by environmental stimuli that had repeatedly been paired with drug consumption and therefore acquired incentive-motivational value through associative learning processes. Such environmental stimuli cause relapse by eliciting the expectation of drug availability and drug craving during abstinence (Miller and Gold, 1994; O’Brien et al., 1998; Tiffany and Carter, 1998; Tiffany and Conklin, 2000; van de Laar et al., 2004). Therefore, another goal of this study was to investigate if the blockade of OX receptors with SUV prevents oxycodone-seeking behavior in a conditioned reinstatement model.
2 Materials and methods
2.1 Animals
Male (n = 16) and female (n = 16) Wistar rats (Charles River, Wilmington, MA, United States of America), 40–45 days old upon arrival, were housed two per cage in a temperature- and humidity-controlled vivarium on a reverse 12 h/12 h light/dark cycle with ad libitum access to food and water. All operant behavior procedures (self-administration, extinction, and conditioned reinstatement sessions) were performed during the rats’ active (dark) phase. These procedures were conducted in strict adherence to the National Institutes of Health’s Guide for the Care and Use of Laboratory Animals and were approved by the Institutional Animal Care and Use Committee of The Scripps Research Institute.
2.2 Drugs
Suvorexant (Belsomra®; Merck, Whitehouse Station, NJ, United States of America) pills (20 mg) were crushed and dissolved in a 20% vitamin E TPGS (D-α-tocopherol polyethylene glycol 1,000 succinate; Mazuri, Richmond, IN, United States of America) solution (Cox et al., 2010; Guo et al., 2013; Ehlers et al., 2020) which was also used for control (i.e., 0 mg/kg SUV) group injections. Once homogenized, to maximize bioavailability of the compound, SUV was administered 30 min before the test sessions at doses of 0, 10, and 20 mg/kg (5 mL/kg, p. o.; based on previously reported doses: Simmons et al., 2017; Gentile et al., 2018). Oxycodone hydrochloride (Spectrum Chemicals, St. Louis, MO, United States of America) dissolved in 0.9% sodium chloride (Hospira, United States of America) was administered intravenously (i.v., 0.15 mg/kg/0.1 mL; Wade et al., 2014; Matzeu and Martin-Fardon, 2020).
2.3 Behavioral testing and procedures
Male and female rats were surgically implanted with jugular catheters while under general anesthesia (isoflurane, 5% for induction, 1%–3% for maintenance). Intravenous catheters made of Micro-Renathane tubing (0.037-inch diameter; Braintree Scientific, Braintree, MA, United States of America) were attached to a guide cannula (Plastics One, Roanoke, VA, United States of America) and secured with dental acrylic cement to an anchoring 2 cm circular mesh. As previously described (Caine and Koob, 1993), the tubing was aseptically inserted and secured in the right jugular vein, and the attached catheter port was placed on the back, closed with a plastic cap and covered with a metal cap to keep the catheter clean and protected. After 7–10 days of recovery, oxycodone self-administration training took place in daily 8-h (extended access) sessions initiated by the extension of two retractable levers into operant chambers (29 cm × 24 cm × 19.5 cm; Med-Associates, St. Albans, VT, United States of America) and presence of the contextual/discriminative stimulus (SD; constant 70 dB white noise). Responses on the right “active lever” were reinforced on a fixed-ratio 1 (FR1) schedule by infusion of oxycodone (0.15 mg/0.1 mL/infusion, i. v.) over 4 s, followed by a 20-s timeout period (TO20) signaled by the illumination of a cue light above the lever. Responses on the left inactive lever were recorded but had no scheduled consequences.
Testing procedures were based on our previously published methods (Matzeu and Martin-Fardon, 2020) and are represented in Figure 1. Self-administration training sessions were conducted 5 days/week (Monday to Friday), interspersed by 48 h of withdrawal when the rats were kept in their home cage (Saturday and Sunday). The rats were scored for spontaneous physical withdrawal signs (i.e., jumps, paw tremors, teeth chattering, wet dog shakes, piloerection, and ptosis; Schulteis et al., 1999; Rehni et al., 2013; Matzeu and Martin-Fardon, 2020) once weekly (after sessions 1, 7, 14, and 18), 1 h before the self-administration session that corresponded to a 15-h abstinence period. The following severity scale: 0 = no signs, 1 = moderate, 2 = severe, was used to score each withdrawal measure and the sum was used as a quantitative measure of withdrawal severity. Once oxycodone self-administration was acquired and dependence was confirmed, 0, 10, or 20 mg/kg SUV (p.o.) was administered 30 min before the start of oxycodone self-administration. The SUV doses were tested in a within-subjects, Latin-square design every other day (sessions 13, 15, and 17) to control for possible order effects of SUV dosing. Self-administration sessions without prior dosing were carried out on alternate days (sessions 14, 16, and 18) to prevent carry-over effects or SUV-induced extinction of active lever pressing.
After the completion of testing the effects of SUV on oxycodone self-administration, responses on the active lever were extinguished in daily 1-h extinction sessions, during which responses had no programmed consequences (i.e., no oxycodone and no cue presentation) until rats reached an average of ≤10 active lever responses (Figure 1). To control for specificity of the SD to reinstate extinguished oxycodone seeking, a neutral stimulus (SN; i.e., the illumination of a 2.8 W house light at the top of the chamber’s front panel) was presented in place of the SD during a 1-h session during which, responding on the right active lever was followed by a TO20 signaled by a 70 dB (70 kHz) intermittent beeping tone. After the SN session, the rats were returned to their regular home cages. Two days after SN testing, 1 h prior to testing conditioned reinstatement of oxycodone-seeking behavior, the rats were scored for spontaneous physical withdrawal signs. The rats were then administered with 0 or 20 mg/kg SUV (between-subjects), and 30 min later were presented with the SD in a 1-h conditioned reinstatement session, and tested for oxycodone-seeking behavior (Figure 1).
2.4 Effect of SUV on sweetened condensed milk-reinforced behavior
To control for sedative behavioral effects of SUV (i.e., non-specific inhibitory behavioral effects), an additional group of male (n = 6) and female (n = 6) rats were trained to orally self-administer sweetened condensed milk (SCM; diluted 2:1 v/v, 0.1 mL; e.g., Martin-Fardon and Weiss, 2014a; Martin-Fardon and Weiss, 2014b; Matzeu et al., 2017; Matzeu et al., 2018) during 1-h daily (5 days/week) sessions on an FR1 TO20 (signaled by the illumination of a cue light above the right active lever) schedule for 6 sessions. The SCM group did not undergo surgery. Once SCM self-administration was acquired, 0 or 20 mg/kg SUV (p.o.) was administered 30 min before the start of SCM self-administration. To prevent carry-over effects or SUV-induced extinction of active lever pressing, a SCM self-administration session without prior dosing was carried out before testing the next dose. The order in which animals received each dose of SUV was randomized.
2.5 Statistical analyses
Statistical analyses on the development and expression of oxycodone dependence were conducted separately in males and females. However, unpaired t-test were used to confirm consistency with the literature that 1) females consume more oxycodone than males and 2) females reinstate to a higher level than males (Fulenwider et al., 2020; Kimbrough et al., 2020; Vazquez et al., 2020; Zanni et al., 2020; Towers et al., 2022). The acquisition of oxycodone and SCM self-administration was analyzed using two-way repeated-measures analysis of variance (RMANOVA), with session and lever (active vs. inactive) as factors. Oxycodone withdrawal scores were analyzed using the non-parametric Friedman test and Wilcoxon matched-pairs signed rank test. Non-parametric Spearman’s correlations determined if withdrawal scores and the number of oxycodone infusions earned in sessions 1, 7, 14, and 18 were significantly correlated. The effects of SUV on oxycodone self-administration and conditioned reinstatement were analyzed using two-way RMANOVAs with dose (self-administration) or session (extinction, SN, and SD for conditioned reinstatement) and lever as factors. Follow-up interrogation of the time-course of 8-h oxycodone self-administration following SUV administration were conducted with RMANOVAs. The effect of SUV on active and inactive responses during SCM self-administration was analyzed using two-way RMANOVAs. Significant effects in the omnibus ANOVAs were followed by Bonferroni post hoc tests. For withdrawal scores, Dunn’s post hoc tests were used. The statistical analyses were performed using GraphPad Prism 9.3.1 software. All data are expressed as the mean ± SEM. Values of p < 0.05 were considered statistically significant.
3 Results
A priori power analyses were conducted using G*Power 3 (Faul et al., 2007) to ensure enough animals were included to test the interactions between two within-subjects’ factors or two mixed factors using two-tailed tests. An estimated effect size of 0.5 was used based on data from previous studies in our laboratory. Results showed that a sample of 6–12 rats per sex were required to achieve a power of 0.80.
Three rats were lost during the acquisition of oxycodone self-administration (two because of catheter failure and one because of health complications), therefore, 15 males and 14 females were tested on the effect of SUV on oxycodone self-administration. For oxycodone conditioned reinstatement, all the males were tested (i.e., n = 8 at the 0-mg dose and n = 7 at the 20-mg/kg dose). However, due to the restricted amount of SUV available at the time of testing, 10 out the 14 females were tested for conditioned reinstatement (i.e., n = 6 at the 0-mg/kg dose and n = 4 at the 20-mg/kg dose).
Both male (n = 15) and female (n = 14) rats acquired oxycodone self-administration over the 12 sessions of training (Figures 2A, B). Responding on the active lever was significantly higher than responding on the inactive lever by session 7 in both male (p = 0.031, Bonferroni post hoc test following two-way RMANOVA, session × lever: F14,196 = 7.09, p ≤ 0.0001; Figure 2A) and female (p = 0.004, Bonferroni post hoc test following two-way RMANOVA, session × lever: F14,182 = 6.579, p ≤ 0.0001; Figure 2B) rats. At the end of the 12 training sessions, females self-administered significantly more oxycodone than males (48.55 ± 5.01 mg/kg vs. 22.49 ± 4.83 mg/kg, respectively; unpaired t-test, t27 = 3.75, p = 0.001). The number of responses on the active lever remained stable and significantly higher than responses emitted on the inactive lever in sessions 14, 16, and 18 after SUV testing (i.e., sessions 13, 15, and 18) in both males (p ≤ 0.0001, Bonferroni post hoc test following two-way RMANOVA; Figure 2A) and females (p ≤ 0.0001, Bonferroni post hoc test following two-way RMANOVA; Figure 2B). Somatic withdrawal (Figures 2C,D) was significantly more pronounced by session 14 of oxycodone self-administration vs. the first measurement in both males (p ≤ 0.0001, vs. session 1, Dunn’s test following Friedman test: 36.23, p ≤ 0.0001; Figure 2C) and females (p = 0.001, vs. session 1, Dunn’s test following Friedman test: 31.76, p ≤ 0.0001; Figure 2D). The number of active lever responses during oxycodone self-administration sessions significantly correlated with somatic signs of withdrawal that were measured at 15 h of abstinence in males (Spearman R = 0.49, p ≤ 0.0001; Figure 2C inset) and females (Spearman R = 0.29, p = 0.048; Figure 2D inset).
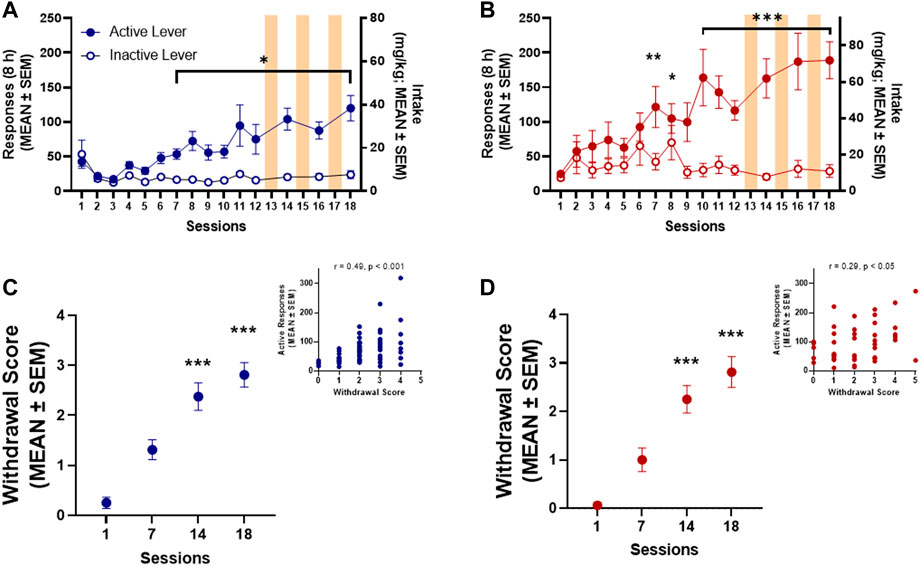
FIGURE 2. Oxycodone self-administration in males (n = 15) (A) and females (n = 14) (B). *p < 0.05, **p < 0.01, ***p < 0.001, vs. inactive lever (Dunnett’s multiple-comparison post hoc test). Somatic withdrawal in both males (C) and females (D). ***p < 0.001, vs. session 1 (Dunn’s multiple-comparison post hoc test). Insets: Correlation plot between somatic withdrawal score and number of oxycodone infusions.
The total number of responses on active and inactive levers over the 8-h self-administration session was not modified by SUV in males (two-way RMANOVA, dose: F2,28 = 0.217, p = 0.806; F1,14 = 26.07, lever: p = 0.0002; dose × lever: F2,28 = 0.777, p = 0.469; Figure 3A) or females (two-way RMANOVA, dose: F2,26 = 1.545, p = 0.232; lever: F1,13 = 74.80, p ≤ 0.0001; dose × lever: F2,26 = 0.672, Figure 3B). Because maximal plasma concentrations of SUV are reported to occur within the first 0.5 h of administration (Cox et al., 2010), the number of responses within each hour of the 8-h session was analyzed by RMANOVAs. At the highest dose tested (20 mg/kg), SUV decreased oxycodone self-administration during the first hour in males (p = 0.012, vs. 0 mg/kg, Bonferroni post hoc test following RMANOVA: F2,26 = 5.05, p = 0.014; Figure 4A) and females (p = 0.018, vs. 0 mg/kg, Bonferroni post hoc test following RMANOVA: F2,26 = 4.77, p = 0.017; Figure 5A), without any effect during the remaining hours (hours 2–8) in males (RMANOVA: p > 0.05; Figures 4B–H) or females (RMANOVA: p > 0.05; Figures 5B–H) or inactive lever responding during any of the 8-h sessions (RMANOVA: p > 0.05).
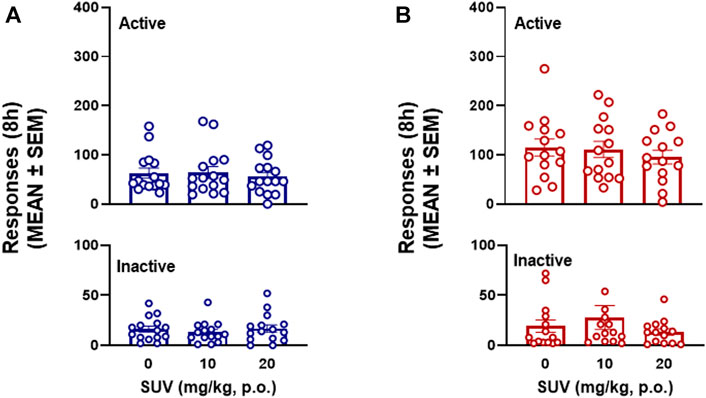
FIGURE 3. Effect of suvorexant on 8-h oxycodone self-administration in males (n = 15) (A) and females (n = 14) (B).
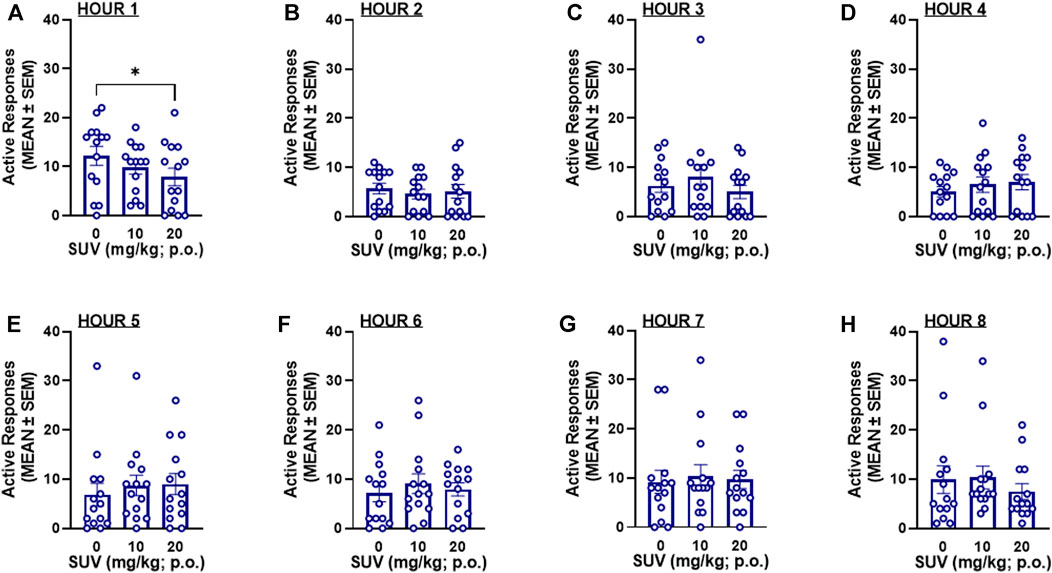
FIGURE 4. Oxycodone self-administration following suvorexant administration in males (n = 15) during the first hour of the session (A) and during each subsequent hour (B): hour 2, (C) hour 3, (D) hour 4, (E) hour 5, (F) hour 6, (G) hour 7, (H) hour 8 of the 8-h session. *p < 0.05, vs. 0 mg/kg (Bonferroni multiple-comparison post hoc test).
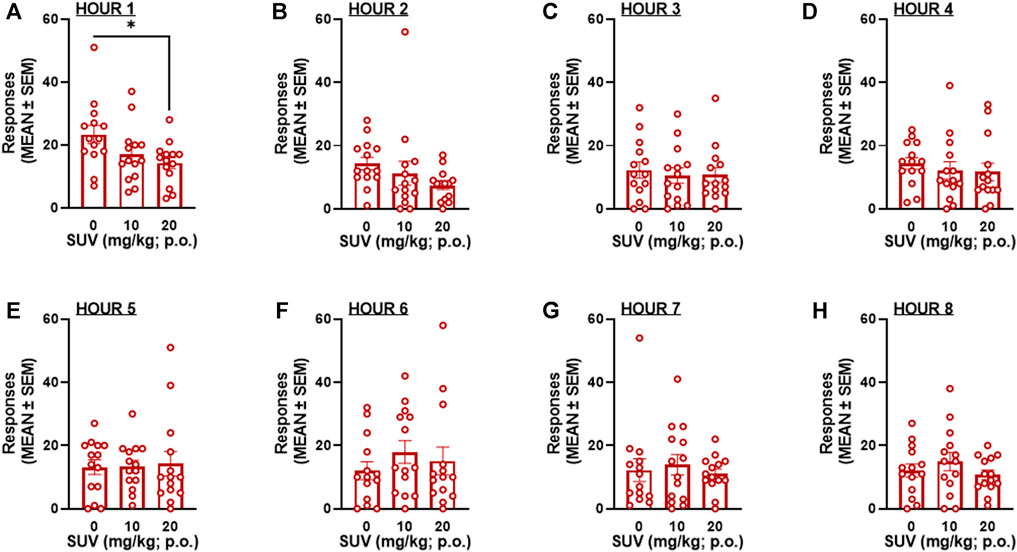
FIGURE 5. Oxycodone self-administration following suvorexant administration in females (n = 14) during the first hour of the session (A) and during each subsequent hour (B): hour 2, (C) hour 3, (D) hour 4, (E) hour 5, (F) hour 6, (G) hour 7, (H) hour 8 of the 8-h session. *p < 0.05, vs. 0 mg/kg (Bonferroni multiple-comparison post hoc test).
At the initiation of extinction training (i.e., session 1), males emitted an average of 22.87 ± 6.08 responses on the active lever vs. 56.93 ± 10.19 responses for females (unpaired t-test, t27 = 2.92, p = 0.007). Males took an average of 4.53 ± 0.83 sessions to reach extinction (i.e., ≤10 responses at the active lever). Females took significantly more sessions (9.57 ± 1.34) to reach extinction than males (unpaired t-test, t27 = 3.24, p = 0.003).
Following extinction training, males (Wilcoxon matched-pairs signed rank test: 105, p ≤ 0.0001) and females (Wilcoxon matched-pairs signed rank test: 88, p = 0.0007) exhibited a significant decrease in physical withdrawal signs compared to that displayed on self-administration session 18. Presentation of the SD (but not the SN) under vehicle conditions (i.e., 0 mg/kg SUV) elicited the reinstatement of oxycodone seeking in males (p = 0.0003, 0 mg/kg vs. EXT, Bonferroni post hoc test following two-way mixed-effects analysis: session × lever: F3,35 = 6.72, p = 0.0003; Figure 6A) and females (p ≤ 0.0001, 0 mg/kg vs. EXT, Bonferroni post hoc test following two-way mixed-effects analysis: session × lever: F3,22 = 15.01, p ≤ 0.0001; Figure 6B). Females exhibited stronger reinstatement than males (unpaired t-test: t12 = 4.27, p = 0.001; Figures 6A,B for comparison). The 20 mg/kg dose of SUV blocked conditioned reinstatement in males (p = 0.01, vs. 0 mg/kg, Bonferroni post hoc test; Figure 6A), to extinction levels (p ≥ 0.999, vs. EXT, Bonferroni post hoc test; Figure 6A). In females, although 20 mg/kg SUV significantly reduced conditioned reinstatement (p = 0.019, 0 mg/kg vs. 20 mg/kg, Bonferroni post hoc test; Figure 6B), substantially significant reinstatement remained (p = 0.038, vs. EXT, Bonferroni post hoc test; Figure 6B). Inactive responses were not significantly affected by any of the tests (p > 0.05, Bonferroni post hoc tests).
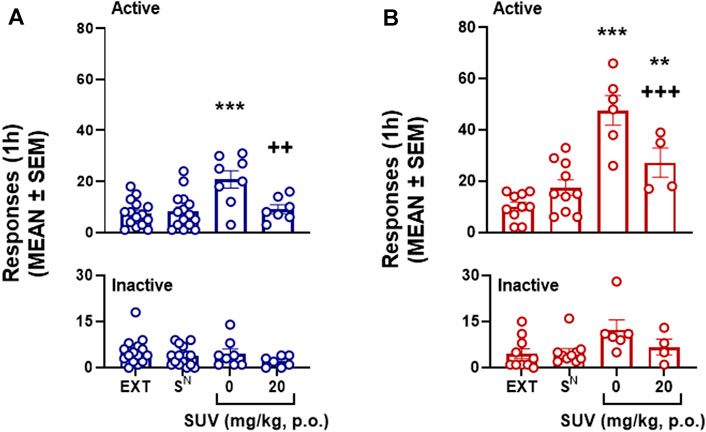
FIGURE 6. Effect of suvorexant on conditioned reinstatement of oxycodone-seeking behavior in males (n = 15, n = 7-8 per dose) (A) and females (n = 10, n = 4-6 per dose) (B). **p < 0.01, ***p < 0.001, vs. respective EXT; ++p < 0.01, +++p < 0.001, vs. respective 0 mg/kg (Bonferroni multiple-comparison post hoc test). EXT, extinction.
Over the 6 training sessions, both male (n = 6) and female (n = 6) rats acquired SCM self-administration (Figures 7A, B). Responding on the active lever was significantly higher that responses on the inactive lever by session 4 in males (p = 0.028, Bonferroni post hoc test following two-way RMANOVA, session × lever: F6,30 = 4.36, p = 0.0008; Figure 7A) and session 2 in females (p ≤ 0.0001, Bonferroni post hoc test following two-way RMANOVA, session × lever: F6,30 = 11.09, p = 0.0004; Figure 7B). At the end of the 6 training sessions, females self-administered significantly more SCM than males (61.67 ± 5.72 mg/kg vs. 29.31 ± 8.35 mg/kg, respectively; unpaired t-test, t10 = 3.20, p = 0.009). The number of responses on the active lever remained significantly higher than responses on the inactive lever during the baseline session between SUV testing (i.e., session 10) in both males (p ≤ 0.0001, Bonferroni post hoc test following two-way RMANOVA; Figure 7A) and females (p ≤ 0.0001, Bonferroni post hoc test following two-way RMANOVA; Figure 7B). The total number of responses on active and inactive levers over the 1-h SCM self-administration session was not modified by SUV in males (two-way RMANOVA: dose: F1,5 = 1.520, p = 0.272; lever: F1,5 = 8.76, p = 0.031; dose × lever: F1,5 = 1.203, p = 0.322, Figure 7C) or females (two-way RMANOVA: dose: F1,5 = 0.119, p = 0.743; lever: F1,5 = 43.66, p = 0.001; dose × lever: F1,5 = 0.139, p = 0.724; Figure 7D).
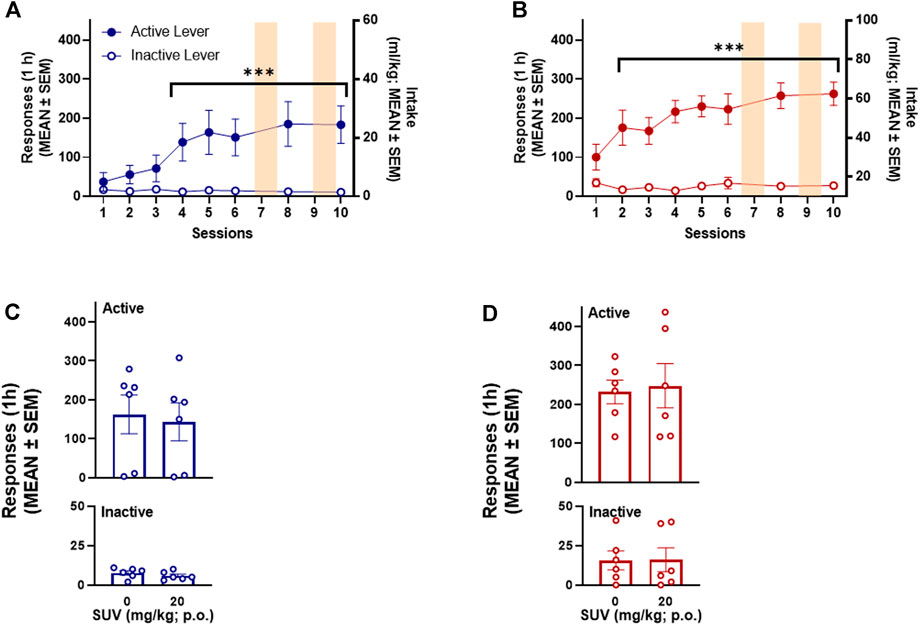
FIGURE 7. Effect of suvorexant on SCM-reinforced behavior. SCM self-administration in males (n = 6) (A) and females (n = 6) (B). ***p < 0.001, vs. inactive lever (Dunnett’s multiple-comparison post hoc test). Lack of effect of SUV on active and inactive responses during 1-h SCM self-administration in males (n = 6) (C) and females (n = 6) (D).
4 Discussion
In the present study, both male and female rats readily acquired oxycodone self-administration with 8-h access, confirming earlier findings (e.g., Wade et al., 2014; Mavrikaki et al., 2017; Nguyen et al., 2018; 2019; Blackwood et al., 2019; Matzeu and Martin-Fardon, 2020; Kimbrough et al., 2020). Acquisition of oxycodone self-administration was characterized by significant increases in oxycodone intake and was correlated with exhibited physical signs of oxycodone withdrawal, verifying dependence. The present study also found that the administration of SUV, an FDA-approved DORA for the treatment of insomnia, treated two critical aspects of OUD by 1) decreasing oxycodone intake and 2) reducing the reinstatement of oxycodone seeking induced by oxycodone contextual stimuli. Altogether, the results endorse targeting the OX system for the treatment of substance use disorders and highlight the potential significance of repurposing SUV for the treatment of prescription OUD.
Oxycodone self-administration under extended access conditions was described as a reliable model for studying OUD, which was supported herein. Both male and female rats increased their intake of oxycodone over the course of self-administration training and exhibited withdrawal signs that significantly correlated with the amount of oxycodone consumed, verifying dependence. Interestingly, at the end of self-administration training (session 12), females took approximately twice the amount of oxycodone as males (48.55 ± 5.01 mg/kg vs. 22.49 ± 4.83 mg/kg, respectively), consistent with another study that used virtually the same conditions described herein (Kimbrough et al., 2020). Suvorexant significantly decreased oxycodone intake at the highest dose tested (20 mg/kg) in both males and females, an effect that occurred in the first hour of the 8-h session. This effect was not the result of a non-specific locomotion effect of SUV (sedation) because it did not affect SCM-reinforced behavior. This suggests that SUV could be beneficial in reducing excessive intake that is seen during initiation of the self-administration session (i.e., loading phase) but not during later parts of the self-administration session (i.e., maintenance phase). The results are consistent with previous reports that the OX1 receptor antagonist SB334867 reduced oxycodone (Matzeu and Martin-Fardon, 2020) and heroin (Smith and Aston-Jones, 2012) self-administration and the motivation for fentanyl (Fragale et al., 2019; Fragale et al., 2020) and remifentanil (Porter-Stransky et al., 2017; Mohammadkhani et al., 2020) on behavioral economics tasks. OX2 receptor antagonists have been reported to reduce heroin (Schmeichel et al., 2014) but not oxycodone (Matzeu and Martin-Fardon, 2020) self-administration, suggesting different roles for OX1 and OX2 receptors in the expression of opioid intake. Likewise, nicotine self-administration is reduced by OX1 receptor (Hollander et al., 2008) but not OX2 receptor (Uslaner et al., 2014) antagonism. Alcohol self-administration is reduced by both OX1 receptor (Lawrence et al., 2006; Richards et al., 2008; Anderson et al., 2014) and OX2 receptor (Shoblock et al., 2011; Brown et al., 2013) antagonists. Cocaine self-administration is also reduced by OX1 receptor antagonists (Hutcheson et al., 2011; Hollander et al., 2012; Brodnik et al., 2015; Foltin and Evans, 2018), but the salience or level of effort that is required to obtain drug may influence the ability of OX1 receptor antagonists to reduce cocaine-seeking behavior (Borgland et al., 2009; España et al., 2010). Still to be tested, however, is whether SUV may also reduce the intake of other prescription opioids with similar or shorter duration of action vs oxycodone (e.g., fentanyl, hydrocodone, morphine, etc.), as SUV effects occurred within the first hour of the self-administration session. Likewise, SUV may be less effective at reducing intake of prescription opioids with longer half-lives (e.g., buprenorphine, methadone, etc.). Nevertheless, the current results add to the existing preclinical literature that SUV can reduce oxycodone intake in addition to stimulants (Simmons et al., 2017; Gentile et al., 2018) and alcohol intake (Flores-Ramirez et al., 2022), supporting that SUV may be an effective treatment strategy for reducing intake of various drugs of abuse (i.e., opioids, stimulants, and alcohol). However, despite preclinical reports indicating that 10 and 30 mg/kg SUV can reduce stimulant intake in rats (Simmons et al., 2017; Gentile et al., 2018), a recent report indicates that 10 and 20 mg/kg SUV increased cocaine intake in a clinical setting (Stoops et al., 2022). It is possible that maintaining SUV levels through chronic (minimum of 3 days) administration (Stoops et al., 2022) elicits different effects on drug intake and seeking compared to that of acute SUV administration utilized in preclinical studies (e.g., current study; Simmons et al., 2017; Gentile et al., 2018; Flores-Ramirez et al., 2022). As such, clinical dose-response studies on the efficacy of SUV to reduce oxycodone intake and seeking under acute and chronic treatment regimens should be fully evaluated.
A secondary objective of the present study was to test whether SUV prevents oxycodone conditioned reinstatement following extinction training. Despite the disappearance of the physical signs of withdrawal at the time of the oxycodone conditioned reinstatement tests, presentation of the oxycodone SD elicited the reinstatement of oxycodone seeking in males and females paralleling what is observed in the clinic where high vulnerability to relapse is still an issue even during post-detoxification period (e.g., Volkow et al., 2016; Koob, 2021). The administration of SUV (20 mg/kg) significantly reduced the conditioned reinstatement of oxycodone-seeking behavior in males and females, with a stronger efficacy in males. These findings provide preclinical evidence of the efficacy of OX receptor blockade in reducing oxycodone-seeking behavior. Consistent with the literature reporting the actions of OX receptor antagonists on drug intake, previous studies showed that the blockade of OX1 receptors with SB334867 can prevent conditioned reinstatement of various drugs of abuse (i.e., opioids: Smith and Aston-Jones, 2012; Porter-Stransky et al., 2017; Fragale et al., 2019; Matzeu and Martin-Fardon, 2020; alcohol; Lawrence et al., 2006; Jupp et al., 2011; Martin-Fardon and Weiss, 2014b; nicotine; Plaza- Zabala et al., 2013; and cocaine; Smith et al., 2009; Smith et al., 2010; Hutcheson et al., 2011; Martin-Fardon and Weiss, 2014a; Bentzley and Aston-Jones, 2015). While another DORA, TCS1102, was reportedly unable to significantly decrease the conditioned reinstatement of alcohol- (Brown et al., 2013) or nicotine- (Plaza-Zabala et al., 2013; Khoo et al., 2017) seeking behavior, recent experiments from our group revealed that SUV (5 mg/kg) effectively reduced stress-induced reinstatement of alcohol seeking in alcohol-dependent rats (Flores-Ramirez et al., 2022). It is currently unclear if the differences in TCS1102 and SUV efficacy to reduce alcohol seeking are due to differences in the procedures utilized (i.e., conditioned vs stress-induced reinstatement) or in the potency to inhibit OX2 receptors (TCS1102: Ki = 3.0; Bergman et al., 2008) (SUV Ki = 0.35 nM; Winrow et al., 2011). More so, while the efficacy of each OX receptor antagonist should be compared directly, SUV holds a significant advantage over SB334867 and TCS1102 as it has already been approved by the FDA as exhibiting greater medical utility than risk. Likewise, while other DORAs have also been FDA-approved for the treatment of insomnia (lemborexant and daridorexant), the ability of these drugs to reduce drug intake and seeking have yet to be tested.
Although the influence of sex on the development and expression of oxycodone dependence was not directly assessed in the current study, the results are in agreement with the existing literature indicating that female rats exhibit a higher motivation to obtain oxycodone compared to male rats (Fulenwider et al., 2020; Kimbrough et al., 2020; Zanni et al., 2020). In clinical settings, women reportedly experience greater spontaneous (Back et al., 2011) and cue-induced (Yu et al., 2007; Moran et al., 2018) opioid craving and report more severe complications with drug use (Hernandez-Avila et al., 2004; Huhn et al., 2019). Likewise, female rats exhibit higher extinction responding and enhanced conditioned reinstatement of heroin (Vazquez et al., 2020) and fentanyl (Towers et al., 2022). Furthermore, the current results show that the ability for the oxycodone-paired SD to reinstate oxycodone-seeking behavior was significantly stronger in females under vehicle conditions (50.67 ± 8.19 responses vs. 20.75 ± 3.40 responses in males). Therefore, females may exhibit greater activation of neural circuits that are activated in the presence of opioids and opioid-conditioned cues when compared with males, which could explain the stronger reinstatement of oxycodone-seeking behavior in females. The precise mechanisms responsible for the sex differences described here and in the literature are yet to be elucidated. However, sex-specific changes in gene expression (George et al., 2021) and/or dopaminergic reactivity (Mayberry et al., 2022) with opioid use have recently been reported. Sex hormones may also influence the effects of opioids as opioid receptor availability (Smith et al., 2006) and methadone doses needed for women in OUD treatment (Chiang et al., 2017) have been shown to vary with estrogen and estradiol levels, respectively. Evidence that women are more likely to be prescribed opioids and misuse prescription opioids than men (Hirschtritt et al., 2018) highlights the urgency to elucidate the specific mechanisms that underlie sex differences in the sensitivity to opioid use and dependence.
Even though SUV is approved for the treatment of insomnia, therefore reducing wakefulness, it is unlikely that reductions of oxycodone self-administration and conditioned reinstatement described here were the result of heavy sedation. Reductions in the number of responses were not observed during 1-h SCM self-administration sessions and were specific to the behavior directed at the active lever during oxycodone self-administration and conditioned reinstatement testing. Notably, our laboratory has also reported that alcohol-reinforced behavior in non-dependent animals was not significantly reduced by the same 20 mg/kg dose of SUV as that used in the current studies (Flores-Ramirez et al., 2022). These results are consistent with earlier findings reporting that the effects of pharmacological manipulation of the OX system are more likely to be observed when the OX system is hyperactivated (Mahler et al., 2014; Pich and Melotto, 2014) such as, for example, when studying behaviors directed towards highly salient rewards (Borgland et al., 2009; Cason and Aston-Jones, 2013; Zink et al., 2018). These findings are also consistent with other studies that showed that blockade of OX1 receptor or both OX1 and OX2 receptors selectively effect drug-over food-seeking behavior in rats with a history of drug exposure (Winrow et al., 2010; Hollander et al., 2012; Martin-Fardon and Weiss, 2014a; Martin-Fardon et al., 2018; also see Cason et al., 2010). As such, the results of the current studies and previous reports support that the ability for SUV to reduce oxycodone intake and seeking are not likely to be solely due to sedative effects of SUV.
OX cell bodies are found in the hypothalamus only, but OX neurons project throughout the brain and to regions that are pivotal in the regulation of motivation and responses to drug-related stimuli (Peyron et al., 1998; Fadel and Deutch, 2002; Baldo et al., 2003; Sakurai and Mieda, 2011). Suvorexant may reduce drug intake and drug seeking by acutely attenuating the effects of drug-induced neuroadaptations of the OX system (see Georgescu et al., 2003; Thannickal et al., 2018; Fragale et al., 2020; Sadat-Shirazi et al., 2020) and/or downstream circuits. For example, chronic SUV treatment with higher doses than those utilized in the current studies were shown to block morphine-induced enhancement of whole brain CREB and p-ERK protein expression (Esmaili-Shahzade-Ali-Akbari et al., 2021). Although approaches utilizing systemic administration of OX ligands to understand how the OX system influences motivational processes that underlie compulsive opioid taking and seeking have been successful, it is also important to uncover the unique contributions of discreet brain regions and circuits by correlating neural activities with changes in drug-seeking behavior. Such pharmacological manipulations of specific OX circuits have yet to be conducted in combination with opioid self-administration and conditioned reinstatement models but are outside the scope of the current study. Nevertheless, the existing literature suggests that the actions of OX within numerous brain regions contribute to the expression of OUD. For example, OX receptor activation in the ventral tegmental area enhances drug-induced dopaminergic transmission (see Harris et al., 2005; Baimel and Borgland, 2012) and, as such, the administration of OX receptor antagonists in this region attenuated the acquisition and expression of morphine-induced CPP (Narita et al., 2006; Aston-Jones et al., 2010; Farahimanesh et al., 2017). Likewise, OX receptor signaling in the nucleus accumbens also contributes to the acquisition and extinction of morphine-induced CPP (Sadeghi et al., 2016; Alizamini et al., 2017; Farahimanesh et al., 2018). In the locus coeruleus, OX increases tyrosine hydroxylase expression (McGregor et al., 2022) and stimulation of OX receptors in the locus coeruleus modulates glutamate (Mousavi et al., 2014; Hooshmandi et al., 2017b; Ghaemi-Jandabi et al., 2017) and GABA transmission (Davoudi et al., 2016) shown to contribute to the expression of morphine withdrawal. OX1 receptor signaling in the lateral paragigantocellularis (Rezaei et al., 2020) and dorsal hippocampus (Hooshmandi et al., 2017a) has also been associated with the expression of opioid withdrawal. OX receptor signaling in the dorsal hippocampus has also been shown to be involved in the acquisition, expression, and reinstatement of morphine-induced CPP (Guo et al., 2015; Sadeghi et al., 2016; Alizamini et al., 2018; Farahimanesh et al., 2018; also see Zarrabian et al., 2020; Vaseghi et al., 2022). These findings suggest that OX system activities throughout a wide system of brain regions contribute to the expression of behaviors related to opioid taking and seeking. Further testing will be required to delineate the exact anatomical and network bases of the behavioral effects of SUV.
Finally, it should be noted that the doses utilized in the present study (10 and 20 mg/kg) are higher than the doses found to be required to achieve at least 90% OX receptor occupancy (Cox et al., 2010) and to reduce active wake time in drug-naive rats with an OX receptor occupancy ≥65% (Gotter et al., 2013). As such, one may argue that off-target effects (on non-OX receptors) of 20 mg/kg SUV could have contributed to reductions in oxycodone intake and seeking. To note, however, that SUV is reportedly highly selective for OX1/OX2 receptors antagonism, having ∼6000-fold intrinsic selectivity over 170 known receptors and enzymes (Cox et al., 2010; Winrow et al., 2011). This suggests that, although selectivity has yet to be tested with the 10 and 20 mg/kg SUV dose, it is unlikely that, in the present study, off-target effects are solely responsible for the SUV effects. Moreover, it was reported that in healthy men, acute oral administration of SUV (10–100 mg) produced maximal plasma concentrations within 3 h (Sun et al., 2013) and in rats, a 10-mg oral dose of SUV yielded maximal plasma concentrations 30 min after administration (Cox et al., 2010). Additionally, administration of 30 mg/kg SUV reduced active wake and increased sleep within the first 2 h post-administration in drug-naive rats (Cox et al., 2010; Winrow et al., 2011) suggesting that, even though a history of oxycodone exposure may alter SUV ability to elicit sedative effects, the effects of SUV measured in the present study matched closely to the SUV pharmacokinetics described in earlier preclinical and clinical characterization of SUV. Further, although SUV affinity to bind to OX1 and OX2 receptors are similar between humans and rats (Cox et al., 2010; Winrow et al., 2011), the time to reach maximal SUV plasma concentrations is longer in humans compared to rats (Cox et al., 2010; Sun et al., 2013). Suvorexant may therefore effectively reduce drug intake and drug seeking in humans for a longer period than what was observed in rats. This will need to be tested carefully in a clinical setting before any decision should be made on safely repurposing SUV for the treatment of prescription OUD.
In conclusion, the results of the present study support continued research on the efficacy of DORAs for the treatment of substance use disorders and indicate that SUV, a drug that is already FDA-approved for the treatment of insomnia, reduces oxycodone self-administration and conditioned reinstatement in both male and female rats. Although the specific OX circuits through which SUV reduces oxycodone taking and seeking are still to be determined, the present findings underscore the significance of targeting the OX system for the treatment of substance use disorders. Studies to determine the efficacy of SUV in clinical populations relative to existing treatments for OUD are warranted. Importantly, the present study suggests that repurposing SUV could be a good alternative for the treatment of prescription OUD to prevent excessive oxycodone taking, craving, and relapse.
Data availability statement
The raw data supporting the conclusion of this article will be made available by the authors, without undue reservation.
Ethics statement
The animal study was reviewed and approved by Institutional Animal Care and Use Committee of the Scripps Research Institute.
Author contributions
Data analysis and interpretation and drafting of the manuscript was conducted by JI. FF-R and BM contributed to intellectual content of the manuscript. Data acquisition was conducted by AM. RM-F contributed through the conceptualization of experiments, data interpretation, and revising intellectual content of the manuscript.
Funding
This work was supported by the National Institute on Alcohol Abuse and Alcoholism (Grant No. AA026999 and AA028549 to RM-F, AA006420 to RM-F and BM, and T32 AA007456 to JI and FF-R) and the National Institute on Drug Abuse (Grant No. DA053443 to RM-F).
Acknowledgments
This is publication number 30212 from The Scripps Research Institute. The authors thank Michael Arends for proofreading the manuscript.
Conflict of interest
The authors declare that the research was conducted in the absence of any commercial or financial relationships that could be construed as a potential conflict of interest.
Publisher’s note
All claims expressed in this article are solely those of the authors and do not necessarily represent those of their affiliated organizations, or those of the publisher, the editors and the reviewers. Any product that may be evaluated in this article, or claim that may be made by its manufacturer, is not guaranteed or endorsed by the publisher.
Abbreviations
CPP, conditioned place preferences; DORA, dual orexin receptor antagonist; i.v., intravenous; OX, orexin; OX1 receptor, orexin-1 receptor; OX2 receptor, orexin-2 receptor; OX receptor, orexin receptor; OUD, opioid use disorder; p.o., oral; RMANOVA, repeated-measures analysis of variance; SD, contextual/discriminative stimulus; SN, neutral stimulus.
References
Alizamini, M. M., Farzinpour, Z., Ezzatpanah, S., and Haghparast, A. (2017). Role of intra-accumbal orexin receptors in the acquisition of morphine-induced conditioned place preference in the rats. Neurosci. Lett. 660, 1–5. doi:10.1016/j.neulet.2017.09.007
Alizamini, M. M., Kavianpour, M., Karimi-Haghighi, S., Fatahi, Z., and Haghparast, A. (2018). Intra-hippocampal administration of orexin receptor antagonists dose-dependently attenuates reinstatement of morphine seeking behavior in extinguished rats. Peptides 110, 40–46. doi:10.1016/j.peptides.2018.10.011
Amodeo, L. R., Liu, W., Wills, D. N., Vetreno, R. P., Crews, F. T., and Ehlers, C. L. (2020). Adolescent alcohol exposure increases orexin-A/hypocretin-1 in the anterior hypothalamus. Alcohol 88, 65–72. doi:10.1016/j.alcohol.2020.06.003
Anderson, R. I., Becker, H. C., Adams, B. L., Jesudason, C. D., and Rorick-Kehn, L. M. (2014). Orexin-1 and orexin-2 receptor antagonists reduce ethanol self-administration in high-drinking rodent models. Front. Neurosci. 8, 33. doi:10.3389/fnins.2014.00033
Aston-Jones, G., Smith, R. J., Sartor, G. C., Moorman, D. E., Massi, L., Tahsili-Fahadan, P., et al. (2010). Lateral hypothalamic orexin/hypocretin neurons: A role in reward-seeking and addiction. Brain Res. 1314, 74–90. doi:10.1016/j.brainres,2009.09.106
Back, S. E., Payne, R. L., Wahlquist, A. H., Carter, R. E., Stroud, Z., Haynes, L., et al. (2011). Comparative profiles of men and women with opioid dependence: Results from a national multisite effectiveness trial. Am. J. Drug Alcohol Abuse 37 (5), 313–323. doi:10.3109/00952990.2011.596982
Baimel, C., and Borgland, S. L. (2012). Hypocretin modulation of drug-induced synaptic plasticity. Prog. Brain Res. 198, 123–131. doi:10.1016/B978-0-444-59489-1.00008-2
Baldo, B. A., Daniel, R. A., Berridge, C. W., and Kelley, A. E. (2003). Overlapping distributions of orexin/hypocretin- and dopamine-β-hydroxylase immunoreactive fibers in rat brain regions mediating arousal, motivation, and stress. J. Comp. Neurol. 464 (2), 220–237. doi:10.1002/cne.10783
Bentzley, B. S., and Aston-Jones, G. (2015). Orexin-1 receptor signaling increases motivation for cocaine-associated cues. Eur. J. Neurosci. 41 (9), 1149–1156. doi:10.1111/ejn.12866
Bergman, J. M., Roecker, A. J., Mercer, S. P., Bednar, R. A., Reiss, D. R., Ransom, R. W., et al. (2008). Proline bis-amides as potent dual orexin receptor antagonists. Bioorg Med. Chem. Lett. 18 (4), 1425–1430. doi:10.1016/j.bmcl.2008.01.001
Berridge, C. W., España, R. A., and Vittoz, N. M. (2010). Hypocretin/orexin in arousal and stress. Brain Res. 1314, 91–102. doi:10.1016/j.brainres.2009.09.019
Blackwood, C. A., Hoerle, R., Leary, M., Schroeder, J., Job, M. O., McCoy, M. T., et al. (2019). Molecular adaptations in the rat dorsal striatum and hippocampus following abstinence-induced incubation of drug seeking after escalated oxycodone-self-administration. Mol. Neurobiol. 56 (5), 3603–3615. doi:10.1007/s12035-018-1318-z
Borgland, S. L., Chang, S., Bowers, M. S., Thompson, J. L., Vittoz, N., Floresco, S. B., et al. (2009). Orexin A/Hypocretin-1 selectively promotes motivation for positive reinforcers. J. Neurosci. 29 (36), 11215–11225. doi:10.1523/JNEUROSCI.6096-08.2009
Borgland, S. L., Taha, S. A., Sarti, F., Fields, H. L., and Bonci, A. (2006). Orexin A in the VTA is critical for the induction of synaptic plasticity and behavioral sensitization to cocaine. Neuron 49, 589–601. doi:10.1016/j.neuron.2006.01.016
Brodnik, Z. D., Bernstein, D. L., Prince, C. D., and España, R. A. (2015). Hypocretin receptor 1 blockade preferentially reduces high effort responding for cocaine without promoting sleep. Behav. Brain Res. 291, 377–384. doi:10.1016/j.bbr.2015.05.051
Brown, R. M., Khoo, S. Y. S., and Lawrence, A. J. (2013). Central orexin (hypocretin) 2 receptor antagonism reduces ethanol self-administration, but not cue-conditioned ethanol-seeking, in ethanol-preferring rats. Int. J. Neuropsychopharmacol. 16 (9), 2067–2079. doi:10.1017/S1461145713000333
Butler, S. F., Fernandez, K. C., Chang, A., Benoit, C., Morey, L. C., Black, R., et al. (2010). Measuring attractiveness for abuse of prescription opioids. Pain Med. 11, 67–80. doi:10.1111/j.1526-4637.2009.00736.x
Caine, S. B., and Koob, G. F. (1993). Modulation of cocaine self-administration in the rat through D-3 dopamine receptors. Science 260, 1814–1816. doi:10.1126/science.8099761
Cason, A. M., and Aston-Jones, G. (2013). Role of orexin/hypocretin in conditioned sucrose-seeking in rats. Psychopharm 226 (1), 155–165. doi:10.1007/s00213-012-2902-y
Cason, A. M., Smith, R. J., Tahsili-Fahadan, P., Moorman, D. E., Sartor, G. C., and Aston-Jones, G. (2010). Role of orexin/hypocretin in reward-seeking and addiction: Implications for obesity. Physiol. Behav. 100 (SI5), 419–428. doi:10.1016/j.physbeh.2010.03.009
Centers for Disease Control and Prevention (2022). State unintentional drug overdose reporting system (SUDORS). Atlanta, GA: US Department of Health and Human Services, CDC; 2022, Nov 3. Access at: https://www.cdc.gov/drugoverdose/fatal/dashboard.
Centers for Disease Control and Prevention (2021). U.S. Opioid dispensing rate maps. Atlanta, GA: US Department of Health and Human Services, CDC; 2022, Nov 3. Access at: https://www.cdc.gov/drugoverdose/rxrate-maps/index.html.
Chemelli, R. M., Willie, J. T., Sinton, C. M., Elmquist, J. K., Scammell, T., Lee, C., et al. (1999). Narcolepsy in orexin knockout mice: Molecular genetics of sleep regulation. Cell. 98 (4), 437–451. doi:10.1016/s0092-8674(00)81973-x
Chiang, Y. C., Wang, R. Y., Huang, C. L., Chen, S. H., Ho, W. J., Lane, H. Y., et al. (2017). Reduced dosing and liability in methadone maintenance treatment by targeting oestrogen signal for morphine addiction. J. Cell. Mol. Med. 21 (12), 3552–3564. doi:10.1111/jcmm.13266
Cox, C. D., Breslin, M. J., Whitman, D. B., Schreier, J. D., McGaughey, G. B., Bogusky, M. J., et al. (2010). Discovery of the dual orexin receptor antagonist [(7R)-4-(5-chloro-1,3-benzoxazol-2-yl)-7-methyl-1,4-diazepan-1-yl] [5-methyl-2-(2H-1,2,3-triazol-2-yl)phenyl]methanone (MK-4305) for the treatment of insomnia. J. Med. Chem. 53 (14), 5320–5332. doi:10.1021/jm100541c
Davoudi, M., Azizi, H., Mirnajafi-Zadeh, J., and Semnanian, S. (2016). The blockade of GABAA receptors attenuates the inhibitory effect of orexin type 1 receptors antagonist on morphine withdrawal syndrome in rats. Neurosci. Lett. 617, 201–206. doi:10.1016/j.neulet.2016.02.022
Dayas, C. V., McGranahan, T. M., Martin-Fardon, R., and Weiss, F. (2008). Stimuli linked to ethanol availability activate hypothalamic CART and orexin neurons in a reinstatement model of relapse. Biol. Psychiatry 63, 152–157. doi:10.1016/j.biopsych.2007.02.002
Ehlers, C. L., Benedict, J., Wills, D., and Sanchez-Alavez, M. (2020). PSPH-D-18-00526: Effect of a dual orexin receptor antagonist (DORA-12) on sleep and event-related oscillations in rats exposed to ethanol vapor during adolescence. Psychopharmacol. Berl. 237 (10), 2917–2927. doi:10.1007/s00213-019-05371-4
Esmaili-Shahzade-Ali-Akbari, P., Hosseinzadeh, H., and Mehri, S. (2021). Effect of suvorexant on morphine tolerance and dependence in mice: Role of NMDA, AMPA, ERK and CREB proteins. Neurotoxicol 84, 64–72. doi:10.1016/j.neuro.2021.02.005
España, R. A., Oleson, E. B., Locke, J. L., Brookshire, B. R., Roberts, D. C. S., and Jones, S. R. (2010). The hypocretin-orexin system regulates cocaine self-administration via actions on the mesolimbic dopamine system. Eur. J. Neurosci. 31 (2), 336–348. doi:10.1111/j.1460-9568.2009.07065.x
Fadel, J., and Deutch, A. Y. (2002). Anatomical substrates of orexin-dopamine interactions: Lateral hypothalamic projections to the ventral tegmental area. Neuroscience 111 (2), 379–387. doi:10.1016/s0306-4522(02)00017-9
Farahimanesh, S., Karimi, S., and Haghparast, A. (2018). Role of orexin-1 receptors in the dorsal hippocampus (CA1 region) in expression and extinction of the morphine-induced conditioned place preference in the rats. Peptides 101, 25–31. doi:10.1016/j.peptides.2017.12.017
Farahimanesh, S., Zarrabian, S., and Haghparast, A. (2017). Role of orexin receptors in the ventral tegmental area on acquisition and expression of morphine-induced conditioned place preference in the rats. Neuropeptides 66, 45–51. doi:10.1016/j.npep.2017.08.003
Faul, F., Erdfelder, E., Lang, A.-G., and Buchner, A. (2007). G*Power 3: A flexible statistical power analysis program for the social, behavioral, and biomedical sciences. Behav. Res. Methods 39, 175–191. doi:10.3758/BF03193146
Flores-Ramirez, F. J., Illenberger, J. M., Pascasio, G. E., Matzeu, A., Mason, B. J., and Martin-Fardon, R. (2022). Alternative use of suvorexant (Belsomra®) for the prevention of alcohol drinking and seeking in rats with a history of alcohol dependence. Front Behav Neurosci 16, 1085882. doi:10.3389/fnbeh.2022.1085882
Foltin, R. W., and Evans, S. M. (2018). Hypocretin/orexin antagonists decrease cocaine self-administration by female rhesus monkeys. Drug Alcohol Depend. 188, 318–327. doi:10.1016/j.drugalcdep.2018.04.018
Fragale, J. E., Pantazis, C. B., James, M. H., and Aston-Jones, G. (2019). The role of orexin-1 receptor signaling in demand for the opioid fentanyl. Neuropsychopharmacology 44 (10), 1690–1697. doi:10.1038/s41386-019-0420-x
Fragale, J. F., James, M. H., and Aston-Jones, G. (2020). Intermittent self-administration of fentanyl induces a multifaceted addiction state associated with persistent changes in the orexin system. Addict. Biol. 26 (3), e12946. doi:10.1111/adb.12946
Fulenwider, H. D., Nennig, S. E., Hafeez, H., Price, M. E., Baruffaldi, F., Pravetoni, M., et al. (2020). Sex differences in oral oxycodone self-administration and stress-primed reinstatement in rats. Addict. Biol. 25 (6), e12822. doi:10.1111/adb.12822
Gentile, T. A., Simmons, S. J., Barker, D. J., Shaw, J. K., España, R. A., and Muschamp, J. W. (2018). Suvorexant, an orexin/hypocretin receptor antagonist, attenuates motivational and hedonic properties of cocaine. Addict. Biol. 23 (1), 247–255. doi:10.1111/adb.12507
George, B. E., Barth, S. H., Kuiper, L. B., Holleran, K. M., Lacy, R. T., Raab-Graham, K. F., et al. (2021). Enhanced heroin self-administration and distinct dopamine adaptations in female rats. Neuropsychopharmacology 46, 1724–1733. doi:10.1038/s41386-021-01035-0
Georgescu, D., Zachariou, V., Barrot, M., Mieda, M., Willie, J. T., Eisch, A. J., et al. (2003). Involvement of the lateral hypothalamic peptide orexin in morphine dependence and withdrawal. J. Neurosci. 23 (8), 3106–3111. doi:10.1523/JNEUROSCI.23-08-03106.2003
Ghaemi-Jandabi, M., Azizi, H., Ahmadi-Soleimani, S. M., and Semnanian, S. (2017). Intracoerulear microinjection of orexin-A induces morphine withdrawal-like signs in rats. Brain Res. Bull. 130, 107–111. doi:10.1016/j.brainresbull.2017.01.010
Gostin, L. O., Hodge, J. G., and Noe, S. A. (2017). Reframing the opioid epidemic as a national emergency. JAMA 18 (16), 1539–1540. doi:10.1001/jama.2017.13358
Gotter, A. L., Winrow, C. J., Brunner, J., Garson, S. L., Fox, S. V., Binns, J., et al. (2013). The duration of sleep promoting efficacy by dual orexin receptor antagonists is dependent upon receptor occupancy threshold. BMC Neurosci. 14, 90. doi:10.1186/1471-2202-14-90
Guo, S. J., Cui, Y., Huang, Z. Z., Liu, H., Zhang, X. Q., Jiang, J. X., et al. (2015). Orexin A-mediated AKT signaling in the dentate gyrus contributes to the acquisition, expression, and reinstatement of morphine-induced conditioned place preference. Addict. Biol. 21 (3), 547–559. doi:10.1111/adb.12236
Guo, Y., Luo, J., Tan, S., Otieno, B. O., and Zhang, Z. (2013). The applications of Vitamin E TPGS in drug delivery. Eur. J. Pharm. Sci. 49 (2), 175–186. doi:10.1016/j.ejps.2013.02.006
Harris, G. C., Wimmer, M., and Jones, G. A. (2005). A role for lateral hypothalamic orexin neurons in reward seeking. Nature 437, 556–559. doi:10.1038/nature04071
Hartwell, E. E., Pfeifer, J. G., McCauley, J. L., Moran-Santa Maria, M., and Back, S. E. (2014). Sleep disturbances and pain among individuals with prescription opioid dependence. Addict. Behav. 39 (10), 1537–1542. doi:10.1016/j.addbeh.2014.05.025
Hernandez-Avila, C. A., Rounsaville, B. J., and Kranzler, H. R. (2004). Opioid-cannabis- and alcohol-dependent women show more rapid progression to substance abuse treatment. Drug Alcohol Depend. 74 (3), 265–272. doi:10.1016/j.drugalcdep.2004.02.001
Hirschtritt, M. E., Delucchi, K. L., and Olfson, M. (2018). Outpatient, combined use of opioid and benzodiazepine medications in the United States, 1993-2014. Prev. Med. Rep. 9, 49–54. doi:10.1016/j.pmedr.2017.12.010
Hollander, J. A., Lu, Q., Cameron, M. D., and Kenny, P. J. (2008). Insular hypocretin transmission regulates nicotine reward. PNAS 105 (49), 19480–19485. doi:10.1073/pnas.0808023105
Hollander, J. A., Pham, D., Fowler, C. D., and Kenny, P. J. (2012). Hypocretin-1 receptors regulate the reinforcing and reward-enhancing effects of cocaine: Pharmacological and behavioral genetics evidence. Front. Behav. Neurosci. 6, 47. doi:10.3389/fnbeh.2012.00047
Hooshmandi, B., Azizi, H., Javan, M., and Semnanian, S. (2017b). Intra-LC microinjection of orexin type-1 receptor antagonist SB-334867 attenuates the expression of glutamate-induced opiate withdrawal like signs during the active phase in rats. Neurosci. Lett. 636, 276–281. doi:10.1016/j.neulet.2016.10.051
Hooshmandi, B., Hosseinmardi, N., Janahmandi, M., Khakpai, F., Rohampour, K., and Doostmohammadi, J. (2017a). Antagonism of orexin type-1 receptors (OX1Rs) attenuates naloxone-precipitated morphine withdrawal syndrome in rat dorsal hippocampus. Pharmacol. Biochem. Beh 158, 39–48. doi:10.1016/j.pbb.2017.06.001
Hoyer, D., and Jacobson, L. H. (2013). Orexin in sleep, addiction, and more: Is the perfect insomnia drug at hand? Neuropetides 47, 477–488. doi:10.1016/j.npep.2013.10.009
Huhn, A. S., Finan, P. H., Gamaldo, C. E., Hammond, A. S., Umbricht, A., Bergeria, C. L., et al. (2022). Suvorexant ameliorated sleep disturbance, opioid withdrawal, and craving during a buprenorphine taper. Sci. Transl. Med. 14 (60), eabn8238–12. doi:10.1126/scitranslmed.abn8238
Huhn, A. S., Tompkins, D. A., Campbell, C. M., and Dunn, K. E. (2019). Individuals with chronic pain who misuse prescription opioids report sex-based differences in pain and opioid withdrawal. Pain Med. 20 (10), 1942–1947. doi:10.1093/pm/pny295
Hutcheson, D. M., Quarta, D., Halbout, B., Rigal, A., Valerio, E., and Heidbreder, C. (2011). Orexin-1 receptor antagonist SB-334867 reduces the acquisition and expression of cocaine-conditioned reinforcement and the expression of amphetamine-conditioned reward. Behav. Pharmacol. 22 (2), 173–181. doi:10.1097/FBP.0b013e328343d761
Inutsuka, A., and Yamanaka, A. (2013). The physiological role of orexin/hypocretin neurons in the regulation of sleep/wakefulness and neuroendocrine functions. Front. Endocrinol. 4 (18), 18. doi:10.3389/fendo.2013.00018
James, M. H., Stopper, C. M., Zimmer, B. A., Kroll, N. E., Bowrey, H. E., and Aston-Jones, G. (2019). Increased number and activity of a lateral subpopulation of hypothalamic orexin/hypocretin neurons underlies the expression of an addicted state in rats. Biol. Psychiatry 85, 925–935. doi:10.1016/j.biopsych.2018.07.022
Jupp, B., Krstew, E., Dezsi, G., and Lawrence, A. J. (2011). Discrete cue-conditioned alcohol-seeking after protracted abstinence: Pattern of neural activation and involvement of orexin receptors. Br. J. Pharmacol. 162, 880–889. doi:10.1111/j.1476-5381.2010.01088.x
Kane, J. K., Parker, S. L., Matta, S. G., Fu, Y., Sharp, B. M., and Li, M. D. (2000). Nicotine up-regulates expression of orexin and its receptors in rat brain. Endocrinology 141, 3623–3629. doi:10.1210/endo.141.10.7707
Khoo, S. Y. S., McNally, G. P., and Clemens, K. J. (2017). The dual orexin receptor antagonist TCS1102 does not affect reinstatement of nicotine-seeking. PLoS ONE 12 (3), e0173967. doi:10.1371/journal.pone.0173967
Kibaly, C., Alderete, J. A., Liu, S. H., Nasef, H. S., Law, P. Y., Evans, C. J., et al. (2021). Oxycodone in the opioid epidemic: High ‘liking’, ‘wanting’, and abuse liability. Cell. Mol. Neurobiol. 41 (5), 899–926. doi:10.1007/s10571-020-01013-y
Kimbrough, A., Kononoff, J., Simpson, S., Kallupi, M., Sedighim, S., Palomino, K., et al. (2020). Oxycodone self-administration and withdrawal behaviors in male and female Wistar rats. Psychopharmacology 237, 1545–1555. doi:10.1007/s00213-020-05479-y
Koob, G. F. (2021). Neurobiology of opioid addiction: Opponent process, hyperkatifeia, and negative reinforcement. Biol. Psychiatry 87 (1), 44–53. doi:10.1016/j.biopsych.2019.05.023
Krystal, A. D., Benca, R. M., and Kilduff, T. S. (2013). Understanding the sleep-wake cycle: Sleep, insomnia, and the orexin system. J. Clin. Psychiatry 74, 3–20. doi:10.4088/JCP.13011su1c
Lawrence, A. J., Cowen, M. S., Yang, H., Chen, F., and Oldfield, B. (2006). The orexin system regulates alcohol-seeking in rats. Br. J. Pharmacol. 148 (6), 752–759. doi:10.1038/sj.bjp.0706789
Mahler, S. V., Moorman, D. E., Smith, R. J., James, M. H., and Aston-Jones, G. (2014). Motivational activation: A unifying hypothesis of orexin/hypocretin function. Nat. Neurosci. 17 (10), 1298–1303. doi:10.1038/nn.3810
Martin-Fardon, R., and Weiss, F. (2012). N-(2-methyl-6-benzoxazolyl)-N’-1,5-naphthyridin-4-yl urea (SB334867), a hypocretin receptor-1 antagonist, preferentially prevents ethanol seeking: comparison with natural reward seeking. Addict. Biol. 19, 233–236. doi:10.1111/j.1369-1600.2012.00480.x
Martin-Fardon, R., Cauvi, G., Kerr, T. M., and Weiss, F. (2018). Differential role of hypothalamic orexin/hypocretin neurons in reward seeking motivated by cocaine versus palatable food. Addict. Biol. 23, 6–15. doi:10.1111/adb.12441
Martin-Fardon, R., and Weiss, F. (2014a). Blockade of hypocretin receptor-1 preferentially prevents cocaine seeking: Comparison with natural reward seeking. NeuroReport 25, 485–488. doi:10.1097/WNR.0000000000000120
Martin-Fardon, R., and Weiss, F. (2014b). N-(2-methyl-6-benzoxazolyl)-N’-1,5-naphthyridin-4-yl urea (SB334867), a hypocretin receptor-1 antagonist, preferentially prevents ethanol seeking: Comparison with natural reward seeking. Addict. Biol. 19, 233–236. doi:10.1111/j.1369-1600.2012.00480.x
Matzeu, A., Cauvi, G., Kerr, T. M., Weiss, F., and Martin-Fardon, R. (2017). The paraventricular nucleus of the thalamus is differentially recruited by stimuli conditioned to the availability of cocaine versus palatable food. Addict. Biol. 22 (1), 70–77. doi:10.1111/adb.12280
Matzeu, A., Kallupi, M., George, O., Schweitzer, P., and Martin-Fardon, R. (2018). Dynorphin counteracts orexin in the paraventricular nucleus of the thalamus: Cellular and behavioral evidence. Neuropsychopharmacology 43 (5), 1010–1020. doi:10.1038/npp.2017.250
Matzeu, A., and Martin-Fardon, R. (2021). Cocaine-seeking behavior induced by orexin A administration in the posterior paraventricular nucleus of the thalamus is not long-lasting: Neuroadaptation of the orexin system during cocaine abstinence. Front. Behav. Neurosci. 15, 620868. doi:10.3389/fnbeh.2021.620868
Matzeu, A., and Martin-Fardon, R. (2020). Targeting the orexin system for prescription opioid use disorder: orexin-1 receptor blockade prevents oxycodone taking and seeking in rats. Neuropsychopharmacology 164, 107906. doi:10.1016/j.neuropharm.2019.107906
Mavrikaki, M., Praventoni, M., Page, S., Potter, D., and Chartoff, E. (2017). Oxycodone self-administration in male and female rats. Psychopharmacology 234 (6), 977–987. doi:10.1007/s00213-017-4536-6
Mayberry, H. L., Bavley, C. C., Karbalaei, R., Peterson, D. R., Bongiovanni, A. R., Ellis, A. S., et al. (2022). Transcriptomics in the nucleus accumbens shell reveal sex- and reinforcer-specific signatures associated with morphine and sucrose craving. Neuropsychopharmacology 47, 1764–1775. doi:10.1038/s41386-022-01289-2
McGregor, R., Wu, M. F., Holmes, B., Lam, H. A., Maidment, N. T., Gera, J., et al. (2022). Hypocretin/Orexin interactions with norepinephrine contribute to the opiate withdrawal syndrome. J. Neurosci. 42 (2), 255–263. doi:10.1523/JNEUROSCI.1557-21.2021
Miller, N. S., and Gold, M. S. (1994). Dissociation of “conscious desire” (craving) from and relapse in alcohol and cocaine dependence. Ann. Clin. Psychiatry 6 (2), 99–106. doi:10.3109/10401239409148988
Mohammadkhani, A., James, M. H., Pantazis, C. B., and Aston-Jones, G. (2020). Persistent effects of the orexin-1 receptor antagonist SB-334867 on motivation for the fast acting opioid remifentanil. Brain Res. 1731, 146461. doi:10.1016/j.brainres.2019.146461
Moorman, D. E., and Aston-Jones, G. (2009). Orexin-1 receptor antagonism decreases ethanol consumption and preference selectively in high-ethanol-preferring Sprague-Dawley rats. Alcohol 43 (5), 379–386. doi:10.1016/j.alcohol.2009.07.002
Moran, L. M., Kowalczyk, W. J., Phillips, K. A., Vahabzadeh, M., Lin, J. L., Mezghanni, M., et al. (2018). Sex differences in daily life stress and craving in opioid-dependent patients. AJDAA 44 (5), 512–523. doi:10.1080/00952990.2018.1454934
Mousavi, Y., Azizi, H., Mirnajafi-Zadeh, J., Javan, M., and Semnanian, S. (2014). Blockade of orexin type-1 receptors in locus coeruleus nucleus attenuates the development of morphine dependency in rats. Neurosci. Lett. 578, 90–94. doi:10.1016/j.neulet.2014.06.038
Narita, M., Nagumo, Y., Hashimoto, S., Nartia, M., Khotib, J., Miyatake, M., et al. (2006). Direct involvement of orexinergic systems in the activation of the mesolimbic dopamine pathway and related behaviors induced by morphine. J. Neurosci. 26 (2), 398–405. doi:10.1523/JNEUROSCI.2761-05.2006
Nguyen, J. D., Grant, Y., Creehan, K. M., Hwang, C. S., Vandewater, S. A., Janda, K. D., et al. (2019). Δ9-tetrahydrocannabinol attenuates oxycodone self-administration under extended access conditions. Neuropsychopharmacology 151, 127–135. doi:10.1016/j.neuropharm.2019.04.010
Nguyen, J. D., Hwang, C. S., Grant, Y., Janda, K. D., and Taffe, M. A. (2018). Prophylactic vaccination protects against the development of oxycodone self-administration. Neuropharmacology 138, 292–303. doi:10.1016/j.neuropharm.2018.06.026
O’Brien, C. P., Childress, A. R., Ehrman, R., and Robbin, S. J. (1998). Conditioning factors in drug abuse: Can they explain compulsion? J. Psychopharmacol. 12 (1), 15–22. doi:10.1177/026988119801200103
Peles, E., Schreiber, S., and Adelson, M. (2006). Variables associated with perceived sleep disorders in methadone maintenance treatment (MMT) patients. Drug Alcohol Depend. 82 (2), 103–110. doi:10.1016/j.drugalcdep.2005.08.011
Peyron, C., Tighe, D. K., van den Pol, A. N., de Lecea, L., Heller, C., Sutcliffe, G., et al. (1998). Neurons containing hypocretin (orexin) project to multiple neuronal systems. J. Neurosci. 18 (23), 9996–10015. doi:10.1523/JNEUROSCI.18-23-09996.1998
Pich, E. M., and Melotto, S. (2014). Orexin 1 receptor antagonists in compulsive behavior and anxiety: Possible therapeutic use. Front. Neurosci. 8, 26. doi:10.3389/fnins.2014.00026
Plaza-Zabala, A., Flores, A., Martin-Garcia, E., Saravia, R., Maldonado, R., and Berrendero, F. (2013). A role for hypocretin/orexin receptor-1 in cue-induced reinstatement of nicotine-seeking behavior. Neuropsychopharmacology 38, 1724–1736. doi:10.1038/npp.2013.72
Porter-Stransky, K. A., Bentzley, B. S., and Aston-Jones, G. (2017). Individual differences in orexin-I receptor modulation of motivation for the opioid remifentanil. Addict. Biol. 22 (2), 303–317. doi:10.1111/adb.12323
Rasmussen, K., White, D. A., and Acri, J. B. (2018). NIDA’s medication development priorities in response to the opioid crisis: Ten most wanted. Neuropsychopharmacology 44, 657–659. doi:10.1038/s41386-018-0292-5
Rehni, A. K., Jaggi, A. S., and Singh, N. (2013). Opioid withdrawal syndrome: Emerging concepts and novel therapeutic targets. CNS Neurol. Disord. Drug Targets 12, 112–125. doi:10.2174/1871527311312010017
Remillard, D., Kaye, A. D., and McAnally, H. (2019). Oxycodone’s unparalleled addictive potential: Is it time for a moratorium? Curr. Pain Headache Rep. 23 (15), 15. doi:10.1007/s11916-019-0751-7
Rezaei, Z., Kourosh-Arami, M., Azizi, H., and Semnanian, S. (2020). Orexin type-1 receptor inhibition in the rat lateral paragigantocellularis nucleus attenuates development of morphine dependence. Neurosci. Lett. 724, 134875. doi:10.1016/j.neulet.2020.134875
Richards, J. K., Simms, J. A., Steensland, P., Taha, S. A., Borgland, S. L., Bonci, A., et al. (2008). Inhibition of orexin-1/hypocretin-1 receptors inhibits yohimbine-induced reinstatement of ethanol and sucrose seeking in Long-Evans rats. Psychopharmacology 199, 109–117. doi:10.1007/s00213-008-1136-5
Rudd, R. A., Seth, P., David, F., and Scholl, L. (2016). Increases in drug and opioid-involved overdose deaths- United States, 2010-2015. MMWR Morb. Mortal. Wkly. Rep. 65 (50-51), 1445–1452. doi:10.15585/mmwr.mm655051e1
Sadat-Shirazi, M., Soltani, H., Nikpour, N., Haghshenas, M., Khalifeh, S., Mokri, A., et al. (2020). Alteration of orexin-A and PKCα in the postmortem brain of pure-opioid and multi-drug abusers. Neuropeptides 83, 102074. doi:10.1016/j.npep.2020.102074
Sadeghi, B., Ezzatpanah, S., and Haghparast, A. (2016). Effects of dorsal hippocampal orexin-2 receptor antagonism on the acquisition, expression, and extinction of morphine-induced place preference in rats. Psychopharmacology 233, 2329–2341. doi:10.1007/s00213-016-4280-3
Sakurai, T., Amemiya, A., Ishii, M., Matsuzaki, I., Chemelli, R. M., Kirokazu, T., et al. (1998). Orexins and orexin receptors: A family of hypothalamic neuropeptides and G-protein coupled receptors that regulate feeding behavior. Cell. 92 (4), 573–585. doi:10.1016/s0092-8674(00)80949-6
Sakurai, T., and Mieda, M. (2011). Connectomics of orexin-producing neurons: Interface of systems of emotion, energy, homeostasis, and arousal. Trends Pharmacol. Sci. 32 (8), 451–462. doi:10.1016/j.tips.2011.03.007
Sargin, D. (2019). The role of the orexin system in stress response. Neuropharmacology 154, 68–78. doi:10.1016/j.neuropharm.2018.09.034
Schmeichel, B. E., Barbier, E., Misra, K. K., Content, C., Schlosburg, J. E., Grigoriadis, D., et al. (2014). Hypocretin receptor 2 antagonism dose-dependently reduces escalated heroin self-administration in rats. Neuropsychopharmacology 40, 1123–1129. doi:10.1038/npp.2014.293
Schulteis, G., Heyser, C. J., and Koob, G. F. (1999). Differential expression of response-disruptive and somatic indices of opiate withdrawal during the initiation and development of opiate dependence. Behav. Pharmacol. 10 (3), 235–242. doi:10.1097/00008877-199905000-00001
Shoblock, J. R., Welty, N., Aluisio, L., Fraser, I., Motley, S. T., Morton, K., et al. (2011). Selective Blockade of the orexin-2 receptor attenuates ethanol self-administration, place preference and reinstatement. Psychopharmacology 215, 191–203. doi:10.1007/s00213-010-2127-x
Simmons, S. J., Martotana, R., Philogene-Khalid, H., Tran, F. H., Gentile, T. A., Xu, X. Y., et al. (2017). Role of hypocretin/orexin receptor blockade on drug-taking and ultrasonic vocalizations (USVs) associated with low-effort self-administration of cathinone-derived 3,4-methylenedioxypyrovalerone (MDPV) in rats. Psychopharmacology 234 (21), 3207–3215. doi:10.1007/s00213-017-4709-3
Smith, R. J., and Aston-Jones, G. (2012). Orexin/hypocretin 1 receptor antagonist reduces heroin self-administration and cue-induced heroin seeking. Eur. J. Neurosci. 35 (5), 798–804. doi:10.1111/j.1460-9568.2012.08013.x
Smith, R. J., See, R. E., and Aston-Jones, G. (2009). Orexin/hypocretin signaling at the OX1 receptor regulates cue-elicited cocaine-seeking. Eur. J. Neurosci. 30 (3), 493–503. doi:10.1111/j.1460-9568.2009.06844.x
Smith, R. J., Tashsili-Fahadan, P., and Aston-Jones, G. (2010). Orexin/hypocretin is necessary for context-driven cocaine-seeking. Neuropharmacology 58 (1), 179–184. doi:10.1016/j.neuropharm.2009.06.042
Smith, Y. R., Stohler, C. S., Nichols, T. E., Bueller, J. A., Koeppe, R. A., and Zubieta, J. K. (2006). Pronociceptive and antinociceptive effects of estradiol through endogenous opioid neurotransmission in women. J. Neurosci. 26 (21), 5777–5785. doi:10.1523/JNEUROSCI.5223-05.2006
Srinivasan, S., Simms, J. A., Nielsen, C. K., Lieske, S. P., Bito-Onon, J. J., Yi, H., et al. (2012). The dual orexin/hypocretin receptor antagonist, almorexant, in the ventral tegmental area attenuates ethanol self-administration. PLoS ONE 7 (9), e44726. doi:10.1371/journal.pone.0044726
Steiner, M. A., Lecourt, H., and Jenck, F. (2013). The dual orexin receptor antagonist, almorexant, alone and in combination with morphine, cocaine and amphetamine, on conditioned place preference and locomotor sensitization in the rat. Int. J. Neuropsychopharmacol. 16, 417–432. doi:10.1017/S1461145712000193
Stoops, W. W., Hatton, K. W., Lofwall, M. R., Nuzzo, P. A., and Walsh, S. L. (2010). Intravenous oxycodone, hydrocodone, and morphine in recreational opioid users: Abuse potential and relative potencies. Psychopharmacol. Berl. 212 (2), 193–203. doi:10.1007/s00213-010-1942-4
Stoops, W. W., Strickland, J. C., Hatton, K. W., Hays, L. R., Rayapati, A. O., Lile, J. A., et al. (2022). Suvorexant maintenance enhances the reinforcing but not subjective and physiological effects of intravenous cocaine in humans. Pharmacol. Biochem. Behav. 220, 173466. doi:10.1016/j.pbb.2022.173466
Sun, H., Kennedy, W. P., Wilbraham, D., Lewis, N., Calder, N., Li, X., et al. (2013). Effects of Suvorexant, an Orexin Receptor Antagonist, on sleep parameters as measured by polysomnography in healthy men. Sleep 36 (2), 259–267. doi:10.5665/sleep.2386
Thannickal, T. C., John, J., Shan, L., Swaab, D. F., Wu, M., Ramanathan, L., et al. (2018). Opiates increase the number of hypocretin-producing cells in human and mouse brain and reverse cataplexy in a mouse model of narcolepsy. Sci. Transl. Med. 10, eaao4953. doi:10.1126/scitranslmed.aao4953
Tiffany, S. T., and Carter, B. L. (1998). Is craving the source of compulsive drug use? J. Psychopharmacol. 12 (1), 23–30. doi:10.1177/026988119801200104
Tiffany, S. T., and Conklin, C. A. (2000). A cognitive processing model of alcohol craving and compulsive alcohol use. Addiction 95 (8), 145–153. doi:10.1080/09652140050111717
Towers, E. B., Setaro, B., and Lynch, W. J. (2022). Sex- and dose-dependent differences in the development of an addiction-like phenotype following extended-access fentanyl self-administration. Front. Pharmacol. 13, 841873. doi:10.3389/fphar.2022.841873
Uslaner, J. M., Winrow, C. J., Gotter, A. L., Roecker, A. J., Coleman, P. J., Hutson, P. H., et al. (2014). Selective orexin 2 receptor antagonism blocks cue-induced reinstatement, but not nicotine self-administration or nicotine-induced reinstatement. Behav. Brain Res. 269, 61–65. doi:10.1016/j.bbr.2014.04.012
van de Laar, M. C., Licht, R., Franken, I. H. A., and Hendriks, V. M. (2004). Event-related potentials indicate motivational relevance of cocaine cues in abstinent cocaine addicts. Psychopharmacol. Berl. 177 (1-2), 121–129. doi:10.1007/s00213-004-1928-1
Vaseghi, S., Zarrabian, S., and Haghparast, A. (2022). Reviewing the role of the orexinergic system and stressors in modulating mood and reward-related behaviors. Neurosci. Biobehav Rev. 133, 104516. doi:10.1016/j.neubiorev.2021.104516
Vazquez, M., Frazier, J. H., Reichel, C. M., and Peters, J. (2020). Acute ovarian hormone treatment in freely cycling female rats regulates distinct aspects of heroin seeking. Learn Mem. 27, 6–11. doi:10.1101/lm.050187.119
Vearrier, D., and Grundmann, O. (2021). Clinical pharmacology, toxicity, and abuse potential of opioids. J. Clin. Pharmacol. 61 (S2), S70–S88. doi:10.1002/jcph.1923
Volkow, N. D., and Collins, F. S. (2017). The role of science in addressing the opioid crisis. N. Engl. J. Med. 337 (4), 391–394. doi:10.1056/NEJMsr1706626
Volkow, N. D., Jones, E. B., Einstein, E. B., and Wargo, E. M. (2019). Prevention and treatment of opioid misuse and addiction: A review. JAMA Psychiatry 76 (2), 208–216. doi:10.1001/jamapsychiatry.2018.3126
Volkow, N. D., Koob, G. F., and McLellan, A. T. (2016). Neurobiologic advances from the brain disease model of addiction. N. Engl. J. Med. 374 (4), 363–371. doi:10.1056/NEJMra1511480
Wade, C. L., Vendruscolo, L. F., Schlosburg, J. E., Hernandez, D. O., and Koob, G. F. (2014). Compulsive-Like responding for opioid analgesics in rats with extended access. Neuropsychopharmacology 40 (2), 421–428. doi:10.1038/npp.2014.188
Wide-Ranging Online Data for Epidemiologic Research (2021). Wide-ranging online data for epidemiologic research (WONDER). Atlanta, GA: CDC, National Center for Health Statistics. Available at: httt://wonder.cdc.gov.
Winrow, C. J., Gotter, A. L., Cox, C. D., Doran, S. M., Tannenbaum, P. L., Breslin, M. J., et al. (2011). Promotion of sleep by suvorexant-a novel dual orexin receptor antagonist. J. Neurogenet. 25 (1-2), 52–61. doi:10.3109/01677063.2011.566953
Winrow, C. J., Tanis, K. Q., Reiss, D. R., Rigby, A. M., Uslander, J. M., Uebele, V. N., et al. (2010). Orexin receptor antagonism prevents transcriptional and behavioral plasticity resulting from stimulant exposure. Neuropsychopharmacology 58, 185–194. doi:10.1016/j.neuropharm.2009.07.008
Yu, J., Zhang, S., Epstein, D. H., Fang, Y., Shi, J., Qin, H., et al. (2007). Gender and stimulus difference in cue-induced responses in abstinent heroin users. Pharmacol. Biochem. Behav. 86 (3), 485–492. doi:10.1016/j.pbb.2007.01.008
Zacny, J. P., and Lichtor, S. A. (2008). Within-subject comparison of the psychopharmacological profiles of oral oxycodone and oral morphine in non-drug-abusing volunteers. Psychopharmacol. Berl. 196 (1), 105–116. doi:10.1007/s00213-007-0937-2
Zanni, G., DeSalle, M. J., Deutsch, H. M., Barr, G. A., and Eisch, A. J. (2020). Female and male rats readily consume and prefer oxycodone to water in a chronic, continuous access, two-bottle oral voluntary paradigm. Neuropharmacology 167, 107978. doi:10.1016/j.neuropharm.2020.107978
Zarrabian, S., Riahi, E., Karimi, S., Razavi, Y., and Haghparast, A. (2020). The potential role of the orexin reward system in future treatments for opioid drug abuse. Brain Res. 1731, 146028. doi:10.1016/j.brainres.2018.11.023
Zhang, Y., Mao, L. M., Liu, X. Y., and Wang, J. Q. (2007). Long-lasting up-regulation of orexin receptor type 2 protein levels in the rat nucleus accumbens after chronic cocaine administration. J. Neurochem. 103, 400–407. doi:10.1111/j.1471-4159.2007.04748.x
Keywords: prescription opioid use disorder, reinstatement, dual orexin receptor antagonists, oxycodone, suvorexant
Citation: Illenberger JM, Flores-Ramirez FJ, Matzeu A, Mason BJ and Martin-Fardon R (2023) Suvorexant, an FDA-approved dual orexin receptor antagonist, reduces oxycodone self-administration and conditioned reinstatement in male and female rats. Front. Pharmacol. 14:1127735. doi: 10.3389/fphar.2023.1127735
Received: 19 December 2022; Accepted: 17 April 2023;
Published: 27 April 2023.
Edited by:
Kabirullah Lutfy, Western University of Health Sciences, United StatesReviewed by:
Esa R. Korpi, University of Helsinki, FinlandJames K. Rowlett, University of Mississippi Medical Center, United States
Copyright © 2023 Illenberger, Flores-Ramirez, Matzeu, Mason and Martin-Fardon. This is an open-access article distributed under the terms of the Creative Commons Attribution License (CC BY). The use, distribution or reproduction in other forums is permitted, provided the original author(s) and the copyright owner(s) are credited and that the original publication in this journal is cited, in accordance with accepted academic practice. No use, distribution or reproduction is permitted which does not comply with these terms.
*Correspondence: Jessica M. Illenberger, jillenberger@scripps.edu