- 1Xiyuan Hospital, China Academy of Chinese Medical Sciences, Beijing, China
- 2Beijing University of Chinese Medicine, Beijing, China
- 3Guang’anmen Hospital, China Academy of Chinese Medical Sciences, Beijing, China
Background and ethnopharmacological relevance: The morbidity and mortality of cardiovascular diseases (CVDs) are among the highest of all diseases, necessitating the search for effective drugs and the improvement of prognosis for CVD patients. Paeoniflorin (5beta-[(Benzoyloxy)methyl] tetrahydro-5-hydroxy-2-methyl-2,5-methano-1H-3,4-dioxacyclobuta [cd] pentalen-1alpha (2H)-yl-beta-D-glucopyranoside, C23H28O11) is mostly derived from the plants of the family Paeoniaceae (a single genus family) and is known to possess multiple pharmacological properties in the treatment of CVDs, making it a promising agent for the protection of the cardiovascular system.
Aim of the study: This review evaluates the pharmacological effects and potential mechanisms of paeoniflorin in the treatment of CVDs, with the aim of advancing its further development and application.
Methods: Various relevant literatures were searched in PubMed, ScienceDirect, Google Scholar and Web of Science. All eligible studies were analyzed and summarized in this review.
Results: Paeoniflorin is a natural drug with great potential for development, which can protect the cardiovascular system by regulating glucose and lipid metabolism, exerting anti-inflammatory, anti-oxidative stress, and anti-arteriosclerotic activities, improving cardiac function, and inhibiting cardiac remodeling. However, paeoniflorin was found to have low bioavailability, and its toxicology and safety must be further studied and analyzed, and clinical studies related to it must be carried out.
Conclusion: Before paeoniflorin can be used as an effective therapeutic drug for CVDs, further in-depth experimental research, clinical trials, and structural modifications or development of new preparations are required.
1 Introduction
Cardiovascular diseases (CVDs), a chronic non-communicable disease, refer to a group of disorders of the heart or blood vessels. Common CVD types include coronary heart disease, aortic disease, peripheral arterial disease, and stroke (Olvera Lopez et al., 2022). Ischemic heart disease and ischemic stroke are collectively referred to as atherosclerotic cardiovascular disease (ASCVD), which is the most prevalently encountered CVD (Arnett et al., 2019; Wong et al., 2022). Several risk factors for CVDs have been identified, such as dyslipidemia, diabetes, metabolic syndrome, hypertension, chronic kidney disease, smoking, age, and genetic history (Yusuf et al., 2020; O'Sullivan et al., 2022). At present, the mainstream therapy of CVDs is medication, including antiplatelet drugs, anticoagulants, statins, anti-thrombotic drugs, beta receptor blockers, antiarrhythmic agent and nitrates (Leong et al., 2017). Despite the availability of a wide range of drugs for clinical use, the morbidity and mortality of CVDs are the highest among all diseases, which not only pose a severe challenge to human health but also bring a huge economic burden to individuals, families, and society (Kalogeropoulos and Butler, 2022; Townsend et al., 2022). As an important problem that must be faced and solved, it is pivotal to seek effective drugs for treating CVDs, and improve the prognosis and quality of life of CVDs patients.
Paeoniflorin (C23H28O11, PubChem CID: 442534), with its chemical name as 5beta-[(Benzoyloxy)methyl] tetrahydro-5-hydroxy-2-methyl-2,5-methano-1H-3,4-dioxacyclobuta [cd] pentalen-1alpha (2H)-yl-beta-D-glucopyranoside, is a pinane monoterpene bitter glucoside distributed in the roots of Paeonia albiflora Pall, P. suffrsticosa Andr, P. delarayi Franch and other Paeoniaceae (Paeonia L.). Paeoniflorin is mostly derived from plants of Paeoniaceae (a single genus family). In 1963, paeoniflorin was first isolated from the roots of Paeonia albiflora and named by Shibata and Nakahara (1963). Further studies showed that the basic skeleton of paeoniflorin is a pinane derivative, which is chemically stable and is a water-soluble monoterpene glycoside (Xia et al., 2007; Sun B et al., 2017). As shown in Figure 1, β-d-glucopyranosyl, benzoyl, and semi-ketal-acetal structures are linked to the backbone, which formed the complete chemical structure of paeoniflorin (Zhang et al., 2022).
Studies have shown that the paeoniflorin can regulate glucose and lipid metabolism, exert anti-inflammatory, anti-oxidative stress, and anti-arteriosclerotic activities, improve cardiac function, and inhibit cardiac remodeling, thus making it a promising candidate for the treatment of CVDs and protection of the cardiovascular system (Figure 1). Whilst several previous reviews have approached the usage of paeoniflorin for neurological disorders and neurodegeneration (Jiao et al., 2021; Hong et al., 2022), analgesia (Lin et al., 2019; Ruan et al., 2021), antidepressants (Zhang et al., 2021a; Lei et al., 2022), neuroprotection (Chen et al., 2020; Guo et al., 2021), and immunomodulation (Chen et al., 2016; Yang and Wei, 2020), a review specific to paeoniflorin’s protective effect on the cardiovascular system is notably lacking. At present, no review articles about paeoniflorin protecting the cardiovascular system have been found in PubMed or other relevant databases. This article is thus dedicated to providing an overview of the pharmacological effects and possible mechanisms of paeoniflorin in the treatment of CVDs, in the hopes of further advancing its development and application (Figure 2).
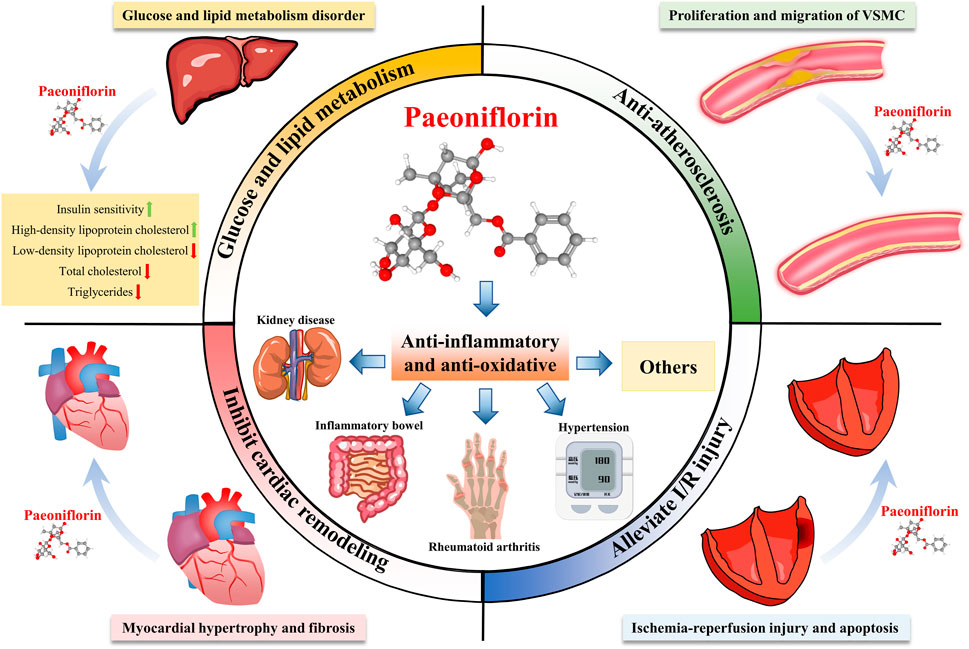
FIGURE 2. Multi-system pharmacological effects of paeoniflorin. Paeoniflorin has therapeutic effects on many diseases, and can prevent and treat kidney diseases, inflammatory bowel disease, rheumatoid arthritis, hypertension and other diseases through anti-inflammatory and anti-oxidant effects. In addition, paeoniflorin can improve glucose and lipid metabolism, resist atherosclerosis, inhibit myocardial remodeling, and improve ischemia-reperfusion injury.
2 Plant sources of paeoniflorin
Paeoniaceae is a monofamily consisting of 34 species, mainly distributed in temperate Eurasia, northwest Africa and western North Americ (Hong, 2011). In China, plants of the Paeoniaceae family are widely cultivated and used for their special medicinal benefits and ornamental value, which makes China the region with the highest concentration of Paeoniaceae in the world (Zhou et al., 2021). The most representative plant in Paeoniaceae is Paeonia lactiflora Pall (Shaoyao in Chinese). As a medicinal plant, Shaoyao was first recorded in the book Shennong’s Herbal Classic of Materia Medica and has a history of more than 2000 years (Xu et al., 2021). In Zhang Zhongjing’s book “Treatise on Febrile Diseases,” there are 113 prescriptions, 33 of which contain Shaoyao, accounting for 29% of all prescriptions. In the northern and southern dynasties, Tao Hongjing pointed out that Shaoyao can be divided into Chishao (Paeoniae Radix Rubra) and Baishao (Paeoniae Radix Alba) in Chinese, which are included in modern Chinese pharmacopoeia (Committee, 2020).
As the traditional Chinese medicines in China, Chishao and Baishao are all processed from the roots of Paeoniaceae plants. The Chinese Pharmacopoeia records that Chishao is the dried root of Paeonia lactiflora Pall or Paeonia veitchii Lynch, while Baishao is the dried root of Paeonia lactiflora Pall (Committee, 2020). Paeoniflorin is the main active ingredient of Chishao and Baishao (Zhang et al., 2022). Chishao and Baishao derive from the same species or closely related plants, which contain similar chemical components, but the paeoniflorin content in Chishao is slightly higher than that in Baishao. According to the Chinese Pharmacopoeia, the paeoniflorin content in dried Baishao should be higher than 1.6%, and in Chishao should be higher than 1.8% (Committee, 2020). In addition to the roots of Paeoniaceae, paeoniflorin is distributed throughout the plant including flowers, stems, leaves, fruits, seeds and rhizomes (Zhang et al., 2022). Since the biosynthetic pathway of paeoniflorin has not been fully elucidated, the chemical synthesis process is complicated and the production cost is high, therefore, the currently applied paeoniflorin is mainly extracted from Paeoniaceae plants (SUN L C et al., 2017). However, the low yield of this generation method does not allow for economical mass production and is increasingly difficult to meet the current increasing demand in pharmaceutical formulations. In the next stage, we should further study the specific modification stage of paeoniflorin in structure and function, further clarify the biosynthesis pathway, and increase the production of paeoniflorin on the basis of environmental protection.
3 Pharmacological effects of paeoniflorin on the cardiovascular system
3.1 Regulation of glucose and lipid metabolism
Dyslipidemia, obesity, and overweight are risk factors for hypertension, coronary heart disease, and peripheral vascular disease (Seravalle and Grassi, 2017; Pirillo et al., 2021). Paeoniflorin has been shown to reduce body weight, regulate lipid metabolism and serum glucose levels, increase insulin sensitivity in obese mice, and improve the accumulation of ectopic lipids (Zhang et al., 2015; Ma et al., 2017a). It has also been reported to significantly reduce the levels of total cholesterol, low-density lipoprotein, and triglycerides in hyperlipidemic rats (Yang et al., 2004; Li et al., 2017a). However, other studies suggest that the effect of paeoniflorin is mainly achieved by lowering cholesterol, with only a limited impact on triglyceride levels (Zhang et al., 2015). Thus, further investigation and discussion on the effects of paeoniflorin on triglyceride metabolism is warranted. The identification of lipid metabolism genes targeted by paeoniflorin has indicated it can regulate lipid synthesis and metabolism through several signaling pathways, such as the de novo, lipid oxidation, cholesterol synthesis and output pathways (Zhang et al., 2015). It has been suggested that paeoniflorin can reduce lipid synthesis by inhibiting SREBP-1c via the de novo pathway and decreasing the expression of FAS, ACC-α, and other proteins (Ma et al., 2017a). It has been found that enzyme 3-hydroxy-3-methylglutaryl coenzyme A reductase (HMG-COAR) is an important enzyme for cholesterol synthesis, and cytochrome P4507A1 (CYP7A1) is a rate-limiting enzyme in the classical pathway of bile acid synthesis. The two enzymes are the key enzymes to regulate the metabolic balance of cholesterol in the body (Osaki et al., 2015). Studies have shown that paeoniflorin can regulate cholesterol synthesis and metabolism by decreasing HMG-CoAR activity and increasing CYP7A1 expression (Zhang et al., 2015; Ma et al., 2017a). Furthermore, Paeoniflorin can attenuate the lipolysis in adipocytes and ameliorate tumor necrosis factor-α (TNF-α) -induced dysfunction of adipocytes (Kong et al., 2013). In addition, the N-acetylgalactosamine transferase 2-angiopoietin like protein 3-lipoprotein lipase (GALNT2-ANGPTL3-LPL) pathway, which is closely related to dyslipidemia and able to directly affect HDL metabolism, has been reported to be effectively regulated by paeoniflorin (Khetarpal et al., 2016; Xiao et al., 2017). A study by Xiao and colleagues showed that 6-week paeoniflorin treatment was able to significantly reduce ANGPTL3 expression, promote GALNT2 and LPL expression, increase serum HDL cholesterol levels, and regulate lipid metabolism in ApoE (−/−) mice (Xiao et al., 2018) (Table 1).
3.2 Anti-inflammatory effect
Inflammation is a major pathological factor contributing in the occurrence and progression of CVDs such as atherosclerosis, thrombosis, myocardial infarction, and ischemia-reperfusion injury (Kim and Conte, 2020). Paeoniflorin has prominent anti-inflammatory effects, which can act by regulating a variety of signalling pathways, such as the GPCR, MAPKs/NF-κB, PI3K/Akt/mTOR, JAK2/STAT3, and TGFβ/Smad pathways (Tu et al., 2019; Xin et al., 2019; Zhang and Wei, 2020). Paeoniflorin can achieve the regulation of anti-inflammatory effects on macrophages and endothelial cell dysfunction by regulating upstream and downstream molecules of NF-κB signaling pathway, which may indicate that paeoniflorin can treat and alleviate cardiovascular diseases from anti-inflammatory mechanism (Table 1).
TNF-α, a prototype member of the tumor necrosis factor superfamily, is predominantly secreted by macrophages and monocytes. Being a major proinflammatory cytokine, it triggers a series of inflammatory processes (Aggarwal et al., 2012). Paeoniflorin can attenuate TNF-α expression by suppressing the activation of the NF-κB signaling pathway (Fang et al., 2020). The paracrine loops of free fatty acids (FFA) and TNF-α present between adipocytes and macrophages form a vicious cycle of inflammation that increases inflammatory changes and insulin resistance in adipose tissue of obese individuals (Suganami et al., 2005). Meanwhile, paeoniflorin is capable of lowering FFA and TNF-α levels by interfering in the interaction between adipocytes and macrophages, thereby impeding the occurring of related inflammatory reactions (Jiang et al., 2012). Research has also demonstrated its capacity to inhibit TNF-α-stimulated phosphorylation at ERK, JNK, and IKK subunits, as well as reduce the expression of pro-inflammatory factors such as IL-6 and MCP-1 in adipocytes (Kong et al., 2013). Pathogen pattern recognition receptors, such as Toll-like receptors (TLRs), can regulate the cytokine response to various inflammatory stimuli (de Kleijn and Pasterkamp, 2003). TLR4 can mediate the activation of the downstream factors MyD88 and NF-κB and induce a surge in proinflammatory cytokines like Il-1β, IL-6, and TNF-α (Fitzgerald et al., 2001). Moreover, paeoniflorin has been found to effectively regulate TLR-2 and TLR-4 expression, thereby decreasing inflammation by suppression of the TLR4/MyD88/NF κB pathway (Li et al., 2013; Li et al., 2017a) (Table 1).
Macrophages are key effectors of inflammation and the innate immune response, and play an pivotal role in the pathogenesis of many CVDs (Xu et al., 2022). The study found that paeoniflorin can reduce the inflammatory response of LPS-stimulated RAW264.7 macrophages by inhibiting the NF-κB/ERK1/2/p38 MAPK signaling pathway (Bi et al., 2017; Li et al., 2022). Linked to that, macrophages show the capability to polarize to M1 and M2 phenotypes (Murray, 2017). Paeoniflorin can decrease the pro-inflammatory activities of M1 macrophages by downregulating inducible nitric oxide synthase (iNOS) expression and NO production through the NF-κB signaling pathway, while simultaneously facilitating the anti-inflammatory function of M2 macrophages by upregulating Arg-1 activity attainable by modulation of the IL-4/STAT6 signaling pathway (Zhai et al., 2016). In addition, Interleukin 33 (IL-33) is a newly identified member of the interleukin family that macrophages, such as M2 macrophages, can secrete (Furukawa et al., 2017). When tissue or cell injury occurs, the release of IL-33 increases, and further adjusts macrophage function by controlling chemokine expression and triggering macrophage polarization (Joshi et al., 2010). Paeoniflorin can regulate macrophage polarization and inhibit IL-33 production by macrophages by regulating the TLR4/NF-κB/P38 MAPK signaling pathway (Chen et al., 2020; Li et al., 2020). However, other studies have suggested that paeoniflorin cannot directly inhibit the activation of macrophages but affects macrophages by inhibiting the expression of iNOS and the production of TNF-α, IL-1β, and MCP-1 (Shao et al., 2019). Therefore, the specific action mode of paeoniflorin in regulating the function of macrophages deserves further study and discussion (Table 1).
Endothelial dysfunction is the primary pathological manifestation of several CVDs (Badimon et al., 2012). On a molecular level, inflammation associated with endoplasmic reticulum (ER) stress appears to be the primary culprit of endothelial dysfunction (ED) (Battson et al., 2017). Paeoniflorin has been reported to be able to restrain the inositol enzyme 1alpha (IRE1α)/NF-κB pathway, eventually diminishing vascular inflammation related to endoplasmic reticulum stress and subsequently reducing endothelial dysfunction (Chen et al., 2018a). Additionally, endothelial cell injury can lead to increased inter cellular adhesion molecule-1 (ICAM-1) expression, which further induces monocyte migration, adhesion, activation, and the ensuing intensify of inflammatory response locally (Most et al., 1992; Frank and Lisanti, 2008). Studies have unveiled that paeoniflorin has the capacity to reduce ICAM-1 expression and inhibit vascular damage (Chen et al., 2013) (Table 1).
By combing through the above research literature, we found that currently, there is limited evidence to directly verify the anti-inflammatory role of paeoniflorin in cardiovascular disease. However, the results of the in vitro studies do demonstrate a remarkable anti-inflammatory effect for paeoniflorin, which can regulate macrophages and endothelial cells, the two vital cell types in the cardiovascular system. Therefore, its potential anti-inflammatory role in cardiovascular disease warrants further exploration and examination. Notwithstanding, there are a few shortcomings visible in the above research. For example, the highest dose of paeoniflorin used by studies conducting cell experiments stands in great disparity, with some suggesting a maximum dose of 100 μM (Bi et al., 2017), while others pointing out that 15 μM paeoniflorin could show obvious cytotoxicity when cultured for 48 h (Li et al., 2020). Since dose concentration is the essential information in pharmacology, it may significantly hamper forward fundamental and clinical studies and, therefore, subsequent researches should focus on precisely figuring out the maximum efficacious dose of paeoniflorin in vivo or in vitro studies and provide detailed, experimental data for further studies (Table 1).
3.3 Anti-oxidative effect
In addition to hypertension, diabetes, dyslipidemia, overweight, obesity, and inflammation, increased oxidative stress i is considered to be a major contributing factor to the increased incidence of certain CVDs (Senoner and Dichtl, 2019; Shaito et al., 2022). Oxidative stress refers to the excessive generation or accumulation of free radical species, such as the oxygen reactive species (ROS) (Di Meo and Venditti, 2020). Previous studies have shown that paeoniflorin can not only downregulate ROS-producing systems, but also intensifying antioxidant enzyme systems, which helps in managing ROS levels and ameliorating the pathological damage induced by oxidative stress (Han et al., 2022; Hu and Yang, 2022; Mao et al., 2022). For instance, paeoniflorin can increase superoxide dismutase (SOD) levels, reduce malondialdehyde (MDA) concentration, and upregulate nuclear factor erythroid factor 2-related factor 2 (Nrf2) expression, thereby augmenting liver antioxidant capacity and protecting the liver from oxidative stress (Hu et al., 2017). At present, At the moment, there is only a limited number of studies regarding the antioxidant effects of paeoniflorin on CVDs. Elevated ROS levels can induce inflammation and mitochondrial dysfunction, thereby affecting endothelial cell and macrophage function and ultimately accelerating the occurrence of CVDs (Forstermann et al., 2017). Paeoniflorin can suppress the secretion of cytokines and the expression of cyclooxygenase-2 (COX-2) and iNOS in a dose-dependent manner, at the same time diminish ROS accumulation in cells without experiencing any effect on macrophage phagocytosis (Li et al., 2022). ROS can be generated in cells via the NADPH oxidase system, which consists of multiple membrane-associated and cytosolic components (Vignais, 2002). NADPH oxidase 2 (Nox2) and Nox4 are highly expressed in endothelial cells and play a role in endothelial cell-cell adhesion and motility, which represent crucial elements in the oxidative stress-induced arteriosclerosis (Van Buul et al., 2005). Paeoniflorin can reduce ROS production by inhibiting the ROS-NF-κB axis and reducing Nox2/Nox4 expression, resulting in downregulation of HIF-1alpha/VEGF levels, alleviation of mitochondrial dysfunction, and protection of human umbilical vein endothelial cells from oxidative damage induced by AOPP (Song et al., 2017). Furthermore, paeoniflorin is capable of diminishing ROS levels in cardiomyocytes by downregulating the expression of microRNA-1, thereby improving cardiomyocyte viability and restraining cardiomyocyte apoptosis induced by doxorubicin (a highly potent anthracycline antitumor antibiotic) (Li et al., 2016) (Table 1).
4 Roles of paeoniflorin in various models of cardiac diseases
4.1 Anti-atherosclerotic effect
ASCVD is defined as an unequivocally diagnosed arteriosclerosis disease that includes acute coronary syndrome, stable coronary artery disease, post-revascularization, ischemic cardiomyopathy, ischemic stroke, transient cerebral ischemia, and peripheral arteriosclerosis disease. Factors such as dyslipidemia, impaired insulin sensitivity, inflammatory state, intense oxidative stress, endothelial dysfunction and other related factors may contribute to the initiation and progression of arteriosclerosis processes (Hill et al., 2021; Pirillo et al., 2021). Most ASCVD events can be avoided by preventing the formation of risk factors and by controlling traditional cardiovascular factors (Arnett et al., 2019). In case of ASCVD, adequate drug treatment is exceptionally critical to impede the progression of the disorder, and a combination of drugs instead of augmenting the amount of a single medication can create greater efficacy and decrease risks (Kim et al., 2022). Paeoniflorin, having multiple pharmacological actions, has great utilization potentiality in managing ASCVD. The regulatory effects of paeoniflorin on glucose and lipid metabolism, inflammation, and oxidative stress suggest its remarkable anti-arteriosclerotic characteristics, as is documented in 3.1–3.4. Equally, paeoniflorin can improve the pathological morphology of the aorta in atherosclerotic rats and alleviate atherosclerosis-related inflammation by inhibiting the TLR4/MyD88/NF-κB pathway (Li et al., 2017a). The underlying pathological mechanism of arteriosclerosis involves the proliferation, migration and inflammatory response of vascular smooth muscle cells (VSMCs) (Bennett et al., 2016). It was found that paeoniflorin could activate HO-1, induce cell cycle arrest, inhibit the p38/ERK1/2/MAPK/NF-κB signaling pathway, inhibit VSMC proliferation and migration induced by ox-LDL in a dose-dependent manner, and reduce the expression of inflammatory cytokines and chemokines (Li et al., 2018a). Paeoniflorin can also promote VSMC apoptosis by upregulating caspases (Guo et al., 2017). Furthermore, paeoniflorin made distinctive anti-platelet effects, counteracting platelet aggregation and clotting and significantly deterring intra-arterial thrombosis (Koo et al., 2010; Xie et al., 2017; Ngo et al., 2019). However, there is a lack of relevant mechanistic studies, so in vivo or in vitro experiments should be designed to investigate the specific mechanism behind paeoniflorin’s anticoagulant and anti-platelet aggregation effects (Table 2).
4.2 Improvement of cardiac function and inhibition of cardiac remodeling
Many studies have shown that paeoniflorin has pharmacological effects which improve cardiac function and inhibit cardiac remodeling. Paeoniflorin ameliorates cardiac dysfunction and regulates the levels of inflammatory cytokines (e.g., IL-1β, IL-6, IL-12, MCP-1, IFN-γ, and iNOS), by affecting the PI3K/AKT signaling pathway and reducing inflammation-related damage (Zhai and Guo, 2016). What is more, paeoniflorin has been proven to be effective in inhibiting cardiac remodeling and alleviating myocardial infarction and ischemia-reperfusion injury. It should be noted that cardiac remodeling denotes an alteration in the size, shape, and function of the heart, which is triggered by gene expression changes induced by cardiac injury or hemodynamic stress and is drastically correlated to hypertension as well as other cardiovascular abnormalities (Tomek and Bub, 2017). Of all these alterations, myocardial hypertrophy and fibrosis are the most predominant, and the severity of myocardial hypertrophy and fibrosis is closely related to the mortality of patients following heart failure (Heinzel et al., 2015; Shenasa and Shenasa, 2017). Paeoniflorin attenuates cardiac hypertrophy, fibrosis, and inflammation in spontaneously hypertensive rats by inhibiting MAPK signaling, and ameliorates pressure overload-induced cardiac remodelling (Liu et al., 2019a). Moreover, to validate the efficacy of paeoniflorin, a model of cardiac remodeling was established by aortic band (AB)-induced pressure overload in mice. The findings demonstrated that treatment with paeoniflorin decreased the heart weight to body weight ratio (HW/BW), reduced the expression of hypertrophic genes, inhibited the apoptosis of cardiomyocytes, alleviated myocardial fibrosis and improved ventricular function by suppressing the activity of the TGF-β/Smads/NF-κB pathways (Zhou et al., 2013). Strikingly, another study also demonstrated that paeoniflorin inhibited the TGF-β1/Smad signaling pathway to reduce cardiac remodeling in an isoproterenol (Iso)-induced rat cardiac remodeling model (Liu et al., 2019b) (Table 2).
Ischemic heart disease, myocardial infarction (MI), hypertension, and valvular heart disease (VHD) are common causes of heart failure (Heidenreich et al., 2022). In China, the occurrence and development of ventricular remodeling after myocardial infarction are the main causes of heart failure. To test this, a rat model of acute myocardial infarction (AMI) was established by ligation of the anterior descending coronary artery, and paeoniflorin was administered orally for 4 weeks post-surgery. Subsequent examination of doppler ultrasonography showed significantly increased left ventricular ejection fraction (LVEF), decreased left ventricular end-diastolic diameter (LVIDd), and decreased left ventricular end-systolic diameter (LVIDs). Furthermore, pathological results from myocardial samples (pericardium tissue) taken from within a 2 mm radius from the visible edge of the infarct showed that paeoniflorin treatment decreased myocardial degeneration in rats. Collectively, these results highlight the ability of paeoniflorin to enhance cardiac function and mitigate the adverse remodeling of the left ventricle post-infarction (Chen et al., 2018b). Additionally, an additional study attributed paeoniflorin’s cardioprotective effects to its ability to reduce inflammation and inhibit the iNOS signaling pathway (Chen et al., 2015) (Table 2).
4.3 Alleviation of ischemia-reperfusion injury
Early access to coronary intervention following myocardial infarction is a crucial measure for improving prognosis, yet it is paramount to not underestimate ischemia-reperfusion (I/R) injury that may occur after revascularization (Yellon and Hausenloy, 2007). To assess the effects of Paeoniflorin on I/R injury, a rat study was conducted, in which 10 mg per kilogram (mg/kg) of Paeoniflorin was intraperitoneally injected 1 h before I/R injury. The results of this study demonstrated that Paeoniflorin significantly improved hemodynamic parameters and reduced myocardial infarction size, as well as downregulated the expression of caspase-3 and Bax, while upregulating the expression of Bcl-2, thus indicating that Paeoniflorin can protect against I/R injury through its anti-apoptotic action (Misao et al., 1996). Another study also revealed the potential of Paeoniflorin to have immediate effects on I/R injury (Nizamutdinova et al., 2008). As the occurrence times of acute cardiac events cannot be predicted during clinical diagnosis and treatment, a course of traditional Chinese medicine preparations are usually taken orally for a certain period of time in order for its therapeutic properties to be adequatelyeffective. Prior to constructing the I/R rat model, Wu et al. orally administered Paeoniflorin for seven consecutive days to the experimental animals, with the last dose being 30 min prior to the induction of ischemia (Wu et al., 2020). It was shown that paeoniflorin pretreatment significantly reduced the size of myocardial infarction, the degree of myocardial injury, apoptosis, and oxidative stress. The specific mechanism of action may be related to the regulation of the MAPK signaling pathway (Wu et al., 2020) (Table 2).
4.4 Improvement of hypertension and arrhythmia
Paeoniflorin also has the pharmacological effect of improving hypertension and arrhythmia. Studies have found that, compared with its single use, the combination of Paeoniflorin-enriched extract and metoprolol can enhance the bioavailability of Paeoniflorin and contribute to a greater anti-hypertensive effect; it can concurrently reduce systolic and diastolic blood pressure in spontaneously hypertensive rats (SHR), increase NOS expression in vascular endothelium, improve the arrangement of elastic fibers and cell hypertrophy in the vascular wall, as well as limit aortic vascular damage and other organ damages (Li et al., 2017b; Li et al., 2018b). Yu et al. suggested that the anti-hypertensive mechanism of paeoniflorin may be related to the effective blocking of voltage-controlled calcium channels (VOCCs) (Lu et al., 2019). This hypothesis is further supported by research which suggests that Paeoniflorin not only blocks L-type calcium current (I(Ca-L)), inward rectifier potassium current (I(K1)) and sodium current (I(Na)) in rat cardiomyocytes, but does so without affecting the instantaneous outward potassium current (I(to1)), slow delayed rectifier current (I(Ks)) and HERG current (I(Kr)), which may partly explain its anti-arrhythmic effects with minimal pro-arrhythmic potential (Wang et al., 2011) (Table 2).
4.5 Regulation of angiogenesis
Angiogenesis has been shown to be beneficial in patients with cardiac insufficiency or myocardial infarction (Oka et al., 2014). Paeoniflorin has been demonstrated to bidirectionally regulate angiogenesis, and its angiogenic effect has been demonstrated in a zebrafish model of vascular insufficiency, as well as in human umbilical vein endothelial cells (HUVECs) (Xin et al., 2018). When Platelet-derived growth factor BB (PDGF-BB) is released in the context of vascular injury, it binds to the cell membrane receptor PDGFR-β and activates NADPH oxidase to generate large amounts of ROS. This leads to aberrant proliferation and migration of vascular smooth muscle cells (VSMCs), and consequently to arteriosclerosis and restenosis (Rivard and Andres, 2000; Lee et al., 2007; Ding et al., 2015). In such cases, it is important to limit angiogenesis. Paeoniflorin has been shown to inhibit PDGF-BB-induced VSMC proliferation by modulating ERK12 and p38 signaling pathways, suggesting the potential of paeoniflorin to act as a therapeutic for arteriosclerosis and restenosis following a percutaneous coronary intervention (Fan et al., 2018). In addition, paeoniflorin can stabilize arteriosclerosis plaques, by inhibiting both the VEGF/VEGFR2 and Jagged1/Notch1 signaling pathways (Yuan et al., 2018) (Table 2).
4.6 Improvement of insulin resistance
The contribution of insulin resistance and diabetes to the pathogenesis of CVDs has already been established (Fox et al., 2007; Hill et al., 2021). CVDs is considered to be the leading cause of death and complications in type 1 diabetes (T1D) mellitus and type 2 diabetes (T2D) mellitus (Cheng et al., 2018). The main pathological feature of T2D mellitus is chronic insulin resistance (Madsbad, 1992). The gradual decline in pancreatic β cell function is the main cause of impaired insulin sensitivity (Shoemaker et al., 2015). Recent studies have shown that paeoniflorin can improve insulin resistance and protect β cells, by inhibiting the activation of Rho kinase (ROCK) and serine phosphorylation of INSR substrate (IRS)-1, and promoting AKT and glycogen synthase kinase (GSK)-3β phosphorylation (Ma et al., 2017b). Additionally, paeoniflorin can also reduce serum insulin and glucagon levels and improve insulin sensitivity by activating the LKB1/AMPK signaling pathway (Li et al., 2018c). Furthermore, paeoniflorin also can significantly ameliorate pancreatic beta cell injury, and regulate glucose metabolism by inhibiting the p38 MAPK and JNK signaling pathways (Liu et al., 2019c) (Table 2).
5 Pharmacokinetics of paeoniflorin
Since 1985, research on paeoniflorin pharmacokinetics has gradually deepened and its effects become better understood (Hattori et al., 1985; Shen et al., 2021). Researchers have delved into studying of the processes of absorption, distribution, biochemical conversion (or metabolism), and excretion of paeoniflorin in the body, particularly its changes in blood concentration over time, which is of great help for the further development and application of paeoniflorin. The usual bioavailability of paeoniflorin absorbed by oral or intestinal perfusion is approximately at 2%–4% (Fei et al., 2016; Wang et al., 2016; Yu et al., 2019). Poor fat solubility of paeoniflorin, P-glycoprotein (P-gp)-mediated transport mechanism, and degradation by gut microbiota enzymes are causes that impede its bioavailability (Liu et al., 2006; Yu et al., 2019).
After absorption, paeoniflorin is widely distributed in various tissues, such as the heart, liver, spleen, lung, kidney, stomach, and intestines (Luo et al., 2014). Additionally, paeoniflorin can pass the blood-brain barrier by passive diffusion (Luo et al., 2014; Hu et al., 2016). Many researchers think it is the metabolite of paeoniflorin, benzoic acid, that actually reaches the barrier mentioned above (Yu et al., 2019). Most paeoniflorin is mainly eliminated in the urine via glomerular filtration of the renal system (Cheng et al., 2016). As experimental data collected from rats show, the serum concentration of paeoniflorin is dose-dependent, with a half-life (T1/2) of approximately 1.8 h (Fei et al., 2016; Chen et al., 2021). In traditional Chinese medicine, Chinese herbal preparations made by combinations of various kinds of botanical drugs can usually achieve the role of synergistic effect and attenuation of toxicity. Moreover, studies show that the compatibility of such botanical drugs or the combination of their active ingredients can play a role in altering paeoniflorin’s pharmacokinetic parameters: when combined with Angelica sinensis, its absorption rate is accelerated, peak time (T max) is shortened, T1/2 is increased, mean residence time (MRT) is prolonged, and its distribution within said tissues is widened (Luo et al., 2014). Conversely, when combined with glycyrrhizic acid, it has been noticed that the T1/2 of paeoniflorin reduces, while its drug clearance and metabolism in rats speed up (Sun et al., 2019). In addition, paeoniflorin displays distinct effects when used in combination with other pharmaceuticals such as quinidine, verapamil, sinomenine, and cyclosporine A (Chan et al., 2006; Liu et al., 2006; Gong et al., 2015; Xu et al., 2016; He et al., 2017).
6 Clinical application
At present, the clinical application of paeoniflorin in traditional Chinese medicine is seldom studied by searching the relevant databases (Figure 3). Peony is the main source of paeoniflorin, especially Chishao (Zhang et al., 2022). Some Chinese patent medicines or preparations containing Peony or paeoniflorin showed good cardiovascular protection; for instance, XS0601 consists of active ingredients (Paeoniflorin and Chuangxiongol) that have been shown through animal studies to inhibit neointimal hyperplasia arteries (Xu et al., 2001). Notably, a multicenter, randomized, double-blind, placebo-controlled trial involving 335 patients has confirmed that administering of XS0601 for 6 months significantly reduces restenosis after percutaneous coronary intervention (PCI) (Chen et al., 2006). Naoxintong is composed of Chishao and 15 additional botanical drugs (Han et al., 2019). It has been proved through clinical trials to protect endothelial cells and treat coronary artery disease (Lv et al., 2016; Long-Tao, 2018). Supplementing aspirin with NXT has further been revealed to heighten the antiplatelet effect in cerebrovascular disease patients (Chen et al., 2008a). Buyang Huanwu (BYHW) decoction containing Chishao is also a traditional Chinese medicine compound preparation. Relevant clinical studies and meta-analysis have proved that its therapeutic effect on stable angina pectoris (SAP)and stroke (Zhang et al., 1995; Gao et al., 2021; Wang et al., 2022a). Recently, Wang et al. (2022b) designed a randomized, blinded, parallel controlled, multicenter clinical trial to compare the efficacy and safety of NXT and BYHW in the treatment of SAP. The results of the trial have yet to be published. Xuefu Zhuyu (XFZY) decoction is composed of Chishao and 10 additional botanical drugs (Li et al., 1998). It has great advantage in treating coronary heart disease. It can effectively relieve symptoms of angina pectoris, improve ECG, reduce the level of blood lipids, and improve endothelial function, among others (Wang and Qiu, 2019; Zhang et al., 2021b). Additionally, Shensong Yangxin (SSYX) capsule consists of Chishao and 11 additional botanical drugs. A randomized, double-blind, controlled, multicenter trial demonstrated a significant effect of SSYX capsules in reducing the number of premature ventricular contractions (PVC) and relieving symptoms associated with PVC compared with placebo or mexiletine (Zou et al., 2011). Furthermore, Tongxinluo (TXL) capsule contains radix paeoniae rubra and 11 additional botanical drugs (Hao et al., 2015). Related clinical studies have shown that TXL can anti arteriosclerosis, reduce blood lipid levels, improve angina pectoris, reduce the incidence of restenosis, and significantly reduce the incidence of no-reflow and myocardial infarction area after primary PCI (Chen et al., 2008b; Chen et al., 2011; Zhang et al., 2019). Danlou (DL) tablets are also a kind of Chinese patent medicine composed of Chishao and other botanical drugs. It has been widely used to treat coronary artery disease in China for a long time. Relevant clinical research demonstrated that DL can treat stable angina pectoris, alleviate adverse left ventricular remodeling after myocardial infarction, and reduce the peri-procedural myocardial injury among patients undergoing PCI for non-ST elevation acute coronary syndrome (Wang et al., 2015; Mao et al., 2016; Yang et al., 2020; Zhao et al., 2021).
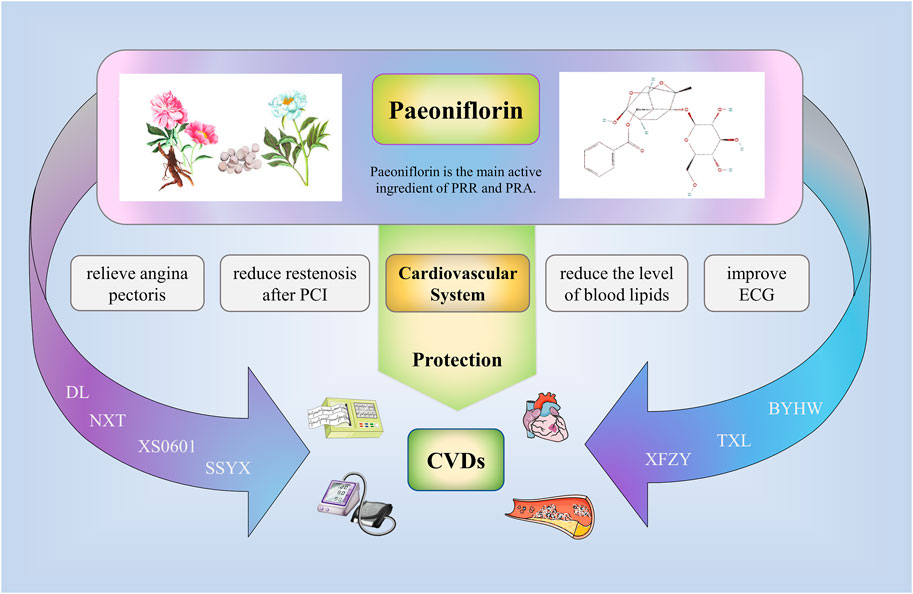
FIGURE 3. Clinical application of paeoniflorin. Some Chinese patent medicines or preparations containing Peony or paeoniflorin showed good cardiovascular protection; for example, XS0601, Naoxintong (NXT), Buyang Huanwu (BYHW), Xuefu Zhuyu (XFZY), Shensong Yangxin (SSYX), Tongxinluo (TXL), Danlou (DL). The above drugs can reduce the symptoms of angina pectoris, reduce the restenosis after PCI, reduce the level of blood lipids, improve ECG, and effectively treat the related cardiovascular diseases.
7 Conclusion and future directions
Paeoniflorin, an effective component of natural plants, protects the cardiovascular system through multiple pharmacological actions (Figure 4). It can regulate lipid synthesis and metabolism via numerous processes, such as the de novo synthesis, lipid oxidation, cholesterol synthesis, and output. Also, it can inhibit the inflammatory response induced by the NF-κB signaling pathway through multiple targets, regulate macrophage function, and inflammation-induced endothelial dysfunction. Additionally, paeoniflorin decreases oxidative stress-induced cellular dysfunction by decreasing the excessive production or accumulation of ROS. It can also stymie atherosclerosis by decreasing cholesterol deposition, and eradicating inflammatory, oxidative and platelet aggregation effects. Besides, it ameliorates cardiac dysfunction by regulating the PI3K/Akt signalling pathway, attenuating cardiac remodeling, and alleviating ischemia-reperfusion injury inspired by inhibition of the MAPK signaling pathway. Not only this, but paeoniflorin also has various pharmacological benefits, such as reduction in blood pressure, arrhythmia improvement, angiogenesis regulation, and lucubration of insulin resistance.
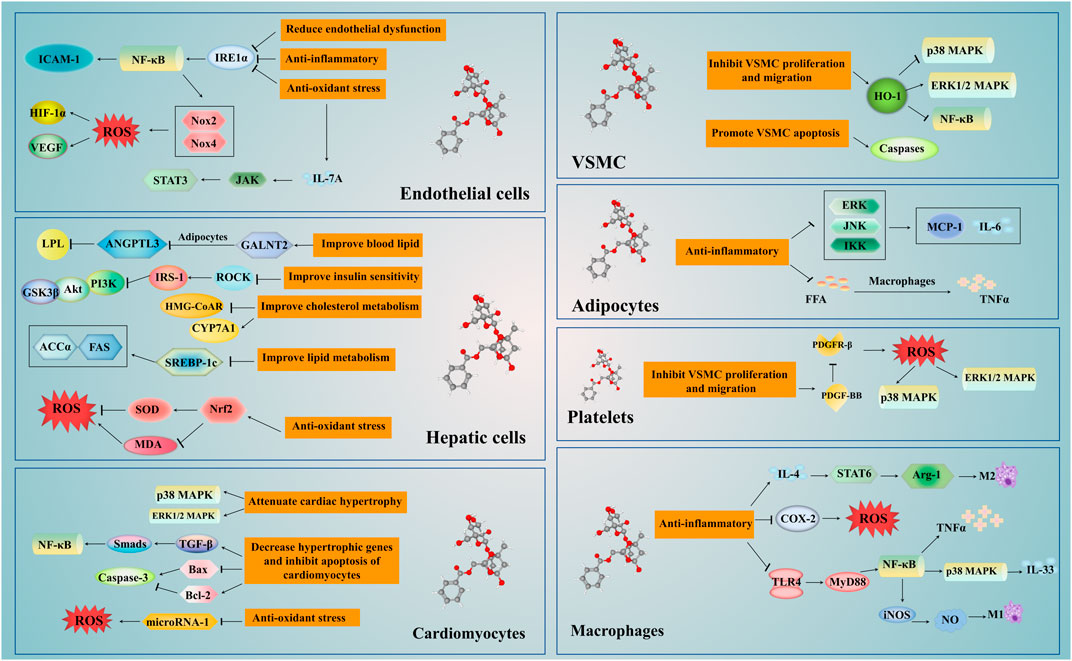
FIGURE 4. The mechanism of Paeoniflorin in the treatment of cardiovascular disease. The treatment of paeoniflorin has the advantage of multi-targets and multi-pathways. It regulates the NF-κB, p38 MAPK, ERK 1/2 MAPK and other signaling pathways in the liver, macrophages, adipocytes, vascular endothelial cells, smooth muscle cells and myocardial cells. Paeoniflorin can regulate insulin sensitivity and liver activity, and improve glucose and lipid metabolism. Moreover, paeoniflorin can improve the proliferation and migration of VSMCs, regulate the levels of blood lipids, protect the vascular endothelium and achieve the goal of anti-atherosclerosis through exerting anti-inflammatory and antioxidant stress. In addition, paeoniflorin can inhibit cardiomyocyte apoptosis and myocardial remodeling, reduce ischemia-reperfusion damage, and improve cardiac function. Abbreviations: ANGPTL3, angiopoietin like protein 3; COX-2, cyclooxygenase-2; CYP7A1, cytochrome P4507A1; FFA, free fatty acids; GALNT-2, N-acetylgalactosaminyltransferase 2; GSK3β, glycogen synthase kinase (GSK)-3β; HMG-CoAR, 3-hydroxy-3-methylglutaryl coenzyme A reductase; ICAM-1, inter cellular adhesion molecule-1; IL, interleukin; iNOS, nitric oxide synthase; IREα, inositol enzyme 1alpha; IRS, INSR substrate; LPL, lipoprotein lipase; MCP-1, monocyte chemoattractant protein-1; MDA, malondialdehyde; NF-κB, ; Nrf2, nuclear factor erythroid factor 2-related factor 2; Nox, NADPH oxidase; PDGF-BB, platelet-derived growth factor BB; ROCK, Rho kinase; ROS, reactive oxygen species; SOD, superoxide dismutase; TGF-β, transforming growth factor-β; TLR, toll like receptors; TNF-α, tumor necrosis factor-α; VSMCs, vascular smooth muscle cells.
In summary, paeoniflorin is a natural drug with high potential development. However, pharmacokinetic studies have shown that its low bioavailability, and therefore necessitating combining it with other traditional Chinese medicines to significantly improve its pharmacokinetic parameters. It has been shown that the esterified derivatives of paeoniflorin could improve the bioavailability and have beneficial pharmacodynamics. Consequently, how to further develop paeoniflorin, improve its bioavailability and extend its medicinal applications is the next focus in pharmaceutics. In regards to safety, certain basic experiments have revealed hepatoprotective effects of paeoniflorin, such as its ability to interfere with bile acid metabolism and pivotal inflammation-related targets, as well as its capacity to ameliorate cholestatic liver injury (Wei et al., 2020; Liu et al., 2022). Despite this, whether paeoniflorin could cause liver injury has not been declared yet. In addition, paeoniflorin is mainly excreted in urine through glomerular filtration, and its effect on renal function has not been reported. In attempts to promote paeoniflorin application, it is essential to analyze its possible toxicity and safety.
At present, the protective effects of paeoniflorin on CVDs are mainly based on animal or cell experimental model. Most of the corresponding clinical studies mainly address Chinese herbal compound preparations containing paeoniflorin or Chishao, with popular treatments including NXT, BYHW, XFZY, Shensong Yangxin, TXL,DL, and so on. Although most studies have proved that paeoniflorin has a wide range of effects on the prevention and treatment of CVDs, but the above studies inevitably have some objective limitations, which is not enough for the scientific research and clinical application of paeoniflorin. Therefore, the next step is to carefully design multicenter, large-scale, and randomized controlled trial studies to assess the efficacy and toxicological characteristics of paeoniflorin alone for CVDs. In addition, paeoniflorin’s basic research, including metabonomics, proteomics, genomics and network pharmacology, should be carried out to fully understand its pharmacological effects and molecular mechanisms. As a therapeutic agent with significant medical application potential, paeoniflorin is worthy of further development and utilization in the foreseeable future.
Author contributions
LL and MW contributed to the conception and design of the study. XL wrote the first draft of this manuscript. CS and XL contributed to drawing relevant diagrams and tables. JZ, LH, and ZY critically revised the article. XZ, ZW, and JC contributed to the identification of relevant references. All authors have contributed to the manuscript and approved the submitted version.
Funding
This study was financially supported by the National Natural Science Foundation of China (Grant No. 81973689), Beijing Natural Science Foundation (7202176), Capital Health Development Scientific Research Program (2022-2-4172), and State Administration of Traditional Chinese Medicine young scholars of Qi Huang (No. 7 [2020]).
Conflict of interest
The authors declare that the research was conducted in the absence of any commercial or financial relationships that could be construed as a potential conflict of interest.
Publisher’s note
All claims expressed in this article are solely those of the authors and do not necessarily represent those of their affiliated organizations, or those of the publisher, the editors and the reviewers. Any product that may be evaluated in this article, or claim that may be made by its manufacturer, is not guaranteed or endorsed by the publisher.
References
Aggarwal, B. B., Gupta, S. C., and Kim, J. H. (2012). Historical perspectives on tumor necrosis factor and its superfamily: 25 years later, a golden journey. Blood 119 (3), 651–665. doi:10.1182/blood-2011-04-325225
Arnett, D. K., Blumenthal, R. S., Albert, M. A., Buroker, A. B., Goldberger, Z. D., Hahn, E. J., et al. (2019). 2019 ACC/AHA guideline on the primary prevention of cardiovascular disease: Executive summary: A report of the American college of cardiology/American heart association task force on clinical practice guidelines. Circulation 140 (11), e563–e595. doi:10.1161/CIR.0000000000000677
Badimon, L., Romero, J. C., Cubedo, J., and Borrell-Pagès, M. (2012). Circulating biomarkers. Thromb. Res. 130 (1), S12–S15. doi:10.1016/j.thromres.2012.08.262
Battson, M. L., Lee, D. M., and Gentile, C. L. (2017). Endoplasmic reticulum stress and the development of endothelial dysfunction. Am. J. Physiol. Heart Circ. Physiol. 312 (3), H355–H367. doi:10.1152/ajpheart.00437.2016
Bennett, M. R., Sinha, S., and Owens, G. K. (2016). Vascular smooth muscle cells in atherosclerosis. Circ. Res. 118 (4), 692–702. doi:10.1161/CIRCRESAHA.115.306361
Bi, X., Han, L., Qu, T., Mu, Y., Guan, P., Qu, X., et al. (2017). Anti-inflammatory effects, SAR, and action mechanism of monoterpenoids from radix paeoniae Alba on LPS-stimulated RAW 264.7 cells. Molecules 22 (5), 715. doi:10.3390/molecules22050715
Chan, K., Liu, Z. Q., Jiang, Z. H., Zhou, H., Wong, Y. F., Xu, H. X., et al. (2006). The effects of sinomenine on intestinal absorption of paeoniflorin by the everted rat gut sac model. J. Ethnopharmacol. 103 (3), 425–432. doi:10.1016/j.jep.2005.08.020
Chen, C., Du, P., and Wang, J. (2015). Paeoniflorin ameliorates acute myocardial infarction of rats by inhibiting inflammation and inducible nitric oxide synthase signaling pathways. Mol. Med. Rep. 12 (3), 3937–3943. doi:10.3892/mmr.2015.3870
Chen, D. K., Zhang, H. Q., and Zhang, J. H. (2008). Intervening effect of naoxintong on anti-platelet treatment with aspirin. Zhongguo Zhong Xi Yi Jie He Za Zhi 28 (9), 843–846.
Chen, D., Li, Y., Wang, X., Li, K., Jing, Y., He, J., et al. (2016). Generation of regulatory dendritic cells after treatment with paeoniflorin. Immunol. Res. 64 (4), 988–1000. doi:10.1007/s12026-015-8773-7
Chen, H., Dong, Y., He, X., Li, J., and Wang, J. (2018). Paeoniflorin improves cardiac function and decreases adverse postinfarction left ventricular remodeling in a rat model of acute myocardial infarction. Drug Des. Devel Ther. 12, 823–836. doi:10.2147/DDDT.S163405
Chen, J., Zhang, M., Zhu, M., Gu, J., Song, J., Cui, L., et al. (2018). Paeoniflorin prevents endoplasmic reticulum stress-associated inflammation in lipopolysaccharide-stimulated human umbilical vein endothelial cells via the IRE1α/NF-κB signaling pathway. Food Funct. 9 (4), 2386–2397. doi:10.1039/c7fo01406f
Chen, K. J., Shi, D. z., Xu, H., Lü, S. z., Li, T. c., Ke, Y. n., et al. (2006). XS0601 reduces the incidence of restenosis: A prospective study of 335 patients undergoing percutaneous coronary intervention in China. Chin. Med. J. Engl. 119 (1), 6–13. doi:10.1097/00029330-200601010-00002
Chen, Q., Liu, Y., Zhang, Y., Jiang, X., Zhang, Y., and Asakawa, T. (2020). An in vitro verification of the effects of paeoniflorin on lipopolysaccharide-exposed microglia. Evid. Based Complement. Altern. Med. 2020, 5801453. doi:10.1155/2020/5801453
Chen, Q., Yin, C., Li, Y., Yang, Z., and Tian, Z. (2021). Pharmacokinetic interaction between peimine and paeoniflorin in rats and its potential mechanism. Pharm. Biol. 59 (1), 129–133. doi:10.1080/13880209.2021.1875013
Chen, T., Guo, Z. P., Wang, L., Qin, S., Cao, N., Li, M. M., et al. (2013). Paeoniflorin suppresses vascular damage and the expression of E-selectin and ICAM-1 in a mouse model of cutaneous Arthus reaction. Exp. Dermatol 22 (7), 453–457. doi:10.1111/exd.12174
Chen, W., Sun, X., Wang, W. j., Fu, X. d., Fu, D. y., Zhou, J. m., et al. (2008). Effects of tongxinluo capsule on cardiac ventricle remodeling after myocardial infarction: A multicentre clinical research. Zhonghua Yi Xue Za Zhi 88 (32), 2271–2273.
Chen, Z. Q., Hong, L., and Wang, H. (2011). Effect of tongxinluo capsule on platelet activities and vascular endothelial functions as well as prognosis in patients with acute coronary syndrome undergoing percutaneous coronary intervention. Zhongguo Zhong Xi Yi Jie He Za Zhi 31 (4), 487–491.
Cheng, C., Lin, J. z., Li, L., Yang, J. l., Jia, W. w., Huang, Y. h., et al. (2016). Pharmacokinetics and disposition of monoterpene glycosides derived from Paeonia lactiflora roots (Chishao) after intravenous dosing of antiseptic XueBiJing injection in human subjects and rats. Acta Pharmacol. Sin. 37 (4), 530–544. doi:10.1038/aps.2015.103
Cheng, Y. J., Imperatore, G., Geiss, L. S., Saydah, S. H., Albright, A. L., Ali, M. K., et al. (2018). Trends and disparities in cardiovascular mortality among U.S. Adults with and without secf-reported diabetes, 1988-2015. Diabetes Care 41 (11), 2306–2315. doi:10.2337/dc18-0831
de Kleijn, D., and Pasterkamp, G. (2003). Toll-like receptors in cardiovascular diseases. Cardiovasc Res. 60 (1), 58–67. doi:10.1016/s0008-6363(03)00348-1
Di Meo, S., and Venditti, P. (2020). Evolution of the knowledge of free radicals and other oxidants. Oxid. Med. Cell Longev. 2020, 9829176. doi:10.1155/2020/9829176
Ding, Z., Liu, S., Wang, X., Deng, X., Fan, Y., Sun, C., et al. (2015). Hemodynamic shear stress via ROS modulates PCSK9 expression in human vascular endothelial and smooth muscle cells and along the mouse aorta. Antioxid. Redox Signal 22 (9), 760–771. doi:10.1089/ars.2014.6054
Fan, X., Wu, J., Yang, H., Yan, L., and Wang, S. (2018). Paeoniflorin blocks the proliferation of vascular smooth muscle cells induced by plateletderived growth factorBB through ROS mediated ERK1/2 and p38 signaling pathways. Mol. Med. Rep. 17 (1), 1676–1682. doi:10.3892/mmr.2017.8093
Fang, Y., Wu, L. C., Ma, K., Pan, G., Yang, S., Zheng, Y., et al. (2020). Paeoniflorin alleviates lipopolysaccharide-induced disseminated intravascular coagulation by inhibiting inflammation and coagulation activation. Drug Dev. Res. 81 (4), 517–525. doi:10.1002/ddr.21647
Fei, F., Yang, H., Peng, Y., Wang, P., Wang, S., Zhao, Y., et al. (2016). Sensitive analysis and pharmacokinetic study of the isomers paeoniflorin and albiflorin after oral administration of Total Glucosides of White Paeony Capsule in rats. J. Chromatogr. B Anal. Technol. Biomed. Life Sci. 1022, 30–37. doi:10.1016/j.jchromb.2016.04.005
Fitzgerald, K. A., Palsson-McDermott, E. M., Bowie, A. G., Jefferies, C. A., Mansell, A. S., Brady, G., et al. (2001). Mal (MyD88-adapter-like) is required for Toll-like receptor-4 signal transduction. Nature 413 (6851), 78–83. doi:10.1038/35092578
Forstermann, U., Xia, N., and Li, H. (2017). Roles of vascular oxidative stress and nitric oxide in the pathogenesis of atherosclerosis. Circ. Res. 120 (4), 713–735. doi:10.1161/CIRCRESAHA.116.309326
Fox, C. S., Coady, S., Sorlie, P. D., D'Agostino, R. B., Pencina, M. J., Vasan, R. S., et al. (2007). Increasing cardiovascular disease burden due to diabetes mellitus: The framingham heart study. Circulation 115 (12), 1544–1550. doi:10.1161/CIRCULATIONAHA.106.658948
Frank, P. G., and Lisanti, M. P. (2008). ICAM-1: Role in inflammation and in the regulation of vascular permeability. Am. J. Physiol. Heart Circ. Physiol. 295 (3), H926–H927. doi:10.1152/ajpheart.00779.2008
Furukawa, S., Moriyama, M., Miyake, K., Nakashima, H., Tanaka, A., Maehara, T., et al. (2017). Interleukin-33 produced by M2 macrophages and other immune cells contributes to Th2 immune reaction of IgG4-related disease. Sci. Rep. 7, 42413. doi:10.1038/srep42413
Gao, L., Xiao, Z., Jia, C., and Wang, W. (2021). Effect of buyang huanwu decoction for the rehabilitation of ischemic stroke patients: A meta-analysis of randomized controlled trials. Health Qual. Life Outcomes 19 (1), 79. doi:10.1186/s12955-021-01728-6
Gong, C., Yang, H., Wei, H., Qi, C., and Wang, C. H. (2015). Pharmacokinetic comparisons by UPLC-MS/MS of isomer paeoniflorin and albiflorin after oral administration decoctions of single-herb Radix Paeoniae Alba and Zengmian Yiliu prescription to rats. Biomed. Chromatogr. 29 (3), 416–424. doi:10.1002/bmc.3292
Guo, K., Zhang, Y., Li, L., Zhang, J., Rong, H., Liu, D., et al. (2021). Neuroprotective effect of paeoniflorin in the mouse model of Parkinson's disease through α-synuclein/protein kinase C δ subtype signaling pathway. Neuroreport 32 (17), 1379–1387. doi:10.1097/WNR.0000000000001739
Guo, Y., Zhao, Y., Li, L., Wei, X., Gao, P., Zhou, Y., et al. (2017). Concentrationdependent effects of paeoniflorin on proliferation and apoptosis of vascular smooth muscle cells. Mol. Med. Rep. 16 (6), 9567–9572. doi:10.3892/mmr.2017.7776
Han, J., Tan, H., Duan, Y., Chen, Y., Zhu, Y., Zhao, B., et al. (2019). The cardioprotective properties and the involved mechanisms of NaoXinTong Capsule. Pharmacol. Res. 141, 409–417. doi:10.1016/j.phrs.2019.01.024
Han, X., Hu, S., Yang, Q., Sang, X., Tang, D., and Cao, G. (2022). Paeoniflorin ameliorates airway inflammation and immune response in ovalbumin induced asthmatic mice: From oxidative stress to autophagy. Phytomedicine 96, 153835. doi:10.1016/j.phymed.2021.153835
Hao, P. P., Jiang, F., Chen, Y. G., Yang, J., Zhang, K., Zhang, M. X., et al. (2015). Traditional Chinese medication for cardiovascular disease. Nat. Rev. Cardiol. 12 (2), 115–122. doi:10.1038/nrcardio.2014.177
Hattori, M., Shu, Y. Z., Shimizu, M., Hayashi, T., Morita, N., Kobashi, K., et al. (1985). Metabolism of paeoniflorin and related compounds by human intestinal bacteria. Chem. Pharm. Bull. (Tokyo) 33 (9), 3838–3846. doi:10.1248/cpb.33.3838
He, R., Xu, Y., Peng, J., Ma, T., Li, J., and Gong, M. (2017). The effects of 18β-glycyrrhetinic acid and glycyrrhizin on intestinal absorption of paeoniflorin using the everted rat gut sac model. J. Nat. Med. 71 (1), 198–207. doi:10.1007/s11418-016-1049-2
Heidenreich, P. A., Bozkurt, B., Aguilar, D., Allen, L. A., Byun, J. J., Colvin, M. M., et al. (2022). 2022 AHA/ACC/HFSA guideline for the management of heart failure: Executive summary: A report of the American college of cardiology/American heart association joint committee on clinical practice guidelines. Circulation 145 (18), e876–e894. doi:10.1161/CIR.0000000000001062
Heinzel, F. R., Hohendanner, F., Jin, G., Sedej, S., and Edelmann, F. (2015). Myocardial hypertrophy and its role in heart failure with preserved ejection fraction. J. Appl. Physiol. 119 (10), 1233–1242. doi:10.1152/japplphysiol.00374.2015
Hill, M. A., Yang, Y., Zhang, L., Sun, Z., Jia, G., Parrish, A. R., et al. (2021). Insulin resistance, cardiovascular stiffening and cardiovascular disease. Metabolism 119, 154766. doi:10.1016/j.metabol.2021.154766
Hong, H., Lu, X., Wu, C., Chen, J., Chen, C., Zhang, J., et al. (2022). A review for the pharmacological effects of paeoniflorin in the nervous system. Front. Pharmacol. 13, 898955. doi:10.3389/fphar.2022.898955
Hu, H., Zhu, Q., Su, J., Wu, Y., Zhu, Y., Wang, Y., et al. (2017). Effects of an enriched extract of paeoniflorin, a monoterpene glycoside used in Chinese herbal medicine, on cholesterol metabolism in a hyperlipidemic rat model. Med. Sci. Monit. 23, 3412–3427. doi:10.12659/msm.905544
Hu, P. Y., Liu, D., Zheng, Q., Wu, Q., Tang, Y., and Yang, M. (2016). Elucidation of transport mechanism of paeoniflorin and the influence of ligustilide, senkyunolide I and senkyunolide A on paeoniflorin transport through mdck-mdr1 cells as blood-brain barrier in vitro model. Molecules 21 (3), 300. doi:10.3390/molecules21030300
Hu, Y., and Yang, W. (2022). Paeoniflorin can improve acute lung injury caused by severe acute pancreatitis through Nrf2/ARE pathway. Comput. Math. Methods Med. 2022, 5712219. doi:10.1155/2022/5712219
Jiang, B., Qiao, J., Yang, Y., and Lu, Y. (2012). Inhibitory effect of paeoniflorin on the inflammatory vicious cycle between adipocytes and macrophages. J. Cell Biochem. 113 (8), 2560–2566. doi:10.1002/jcb.22173
Jiao, F., Varghese, K., Wang, S., Liu, Y., Yu, H., Booz, G. W., et al. (2021). Recent insights into the protective mechanisms of paeoniflorin in neurological, cardiovascular, and renal diseases. J. Cardiovasc Pharmacol. 77 (6), 728–734. doi:10.1097/FJC.0000000000001021
Joshi, A. D., Oak, S. R., Hartigan, A. J., Finn, W. G., Kunkel, S. L., Duffy, K. E., et al. (2010). Interleukin-33 contributes to both M1 and M2 chemokine marker expression in human macrophages. BMC Immunol. 11, 52. doi:10.1186/1471-2172-11-52
Kalogeropoulos, A. P., and Butler, J. (2022). Worsening cardiovascular disease epidemiology in the United States: The time for preparation is now. J. Am. Coll. Cardiol. 80 (6), 579–583. doi:10.1016/j.jacc.2022.05.035
Khetarpal, S. A., Schjoldager, K. T., Christoffersen, C., Raghavan, A., Edmondson, A. C., Reutter, H. M., et al. (2016). Loss of function of GALNT2 lowers high-density lipoproteins in humans, nonhuman primates, and rodents. Cell Metab. 24 (2), 234–245. doi:10.1016/j.cmet.2016.07.012
Kim, A. S., and Conte, M. S. (2020). Specialized pro-resolving lipid mediators in cardiovascular disease, diagnosis, and therapy. Adv. Drug Deliv. Rev. 159, 170–179. doi:10.1016/j.addr.2020.07.011
Kim, B. K., Hong, S. J., Lee, Y. J., Hong, S. J., Yun, K. H., Hong, B. K., et al. (2022). Long-term efficacy and safety of moderate-intensity statin with ezetimibe combination therapy versus high-intensity statin monotherapy in patients with atherosclerotic cardiovascular disease (RACING): A randomised, open-label, non-inferiority trial. Lancet 400 (10349), 380–390. doi:10.1016/S0140-6736(22)00916-3
Kong, P., Chi, R., Zhang, L., Wang, N., and Lu, Y. (2013). Effects of paeoniflorin on tumor necrosis factor-alpha-induced insulin resistance and changes of adipokines in 3T3-L1 adipocytes. Fitoterapia 91, 44–50. doi:10.1016/j.fitote.2013.08.010
Koo, Y. K., Kim, J. M., Koo, J. Y., Kang, S. S., Bae, K., Kim, Y. S., et al. (2010). Platelet anti-aggregatory and blood anti-coagulant effects of compounds isolated from Paeonia lactiflora and Paeonia suffruticosa. Pharmazie 65 (8), 624–628.
Lee, C. K., Lee, H. M., Kim, H. J., Park, H. J., Won, K. J., Roh, H. Y., et al. (2007). Syk contributes to PDGF-BB-mediated migration of rat aortic smooth muscle cells via MAPK pathways. Cardiovasc Res. 74 (1), 159–168. doi:10.1016/j.cardiores.2007.01.012
Lei, C., Chen, Z., Fan, L., Xue, Z., Chen, J., Wang, X., et al. (2022). Integrating metabolomics and network analysis for exploring the mechanism underlying the antidepressant activity of paeoniflorin in rats with CUMS-induced depression. Front. Pharmacol. 13, 904190. doi:10.3389/fphar.2022.904190
Leong, D. P., Joseph, P. G., McKee, M., Anand, S. S., Teo, K. K., Schwalm, J. D., et al. (2017). Reducing the global burden of cardiovascular disease, Part 2: Prevention and treatment of cardiovascular disease. Circ. Res. 121 (6), 695–710. doi:10.1161/CIRCRESAHA.117.311849
Li, B., Yang, Z. B., Lei, S. S., Su, J., Jin, Z. W., Chen, S. H., et al. (2018). Combined antihypertensive effect of paeoniflorin enriched extract and metoprolol in spontaneously hypertensive rats. Pharmacogn. Mag. 14 (53), 44–52. doi:10.4103/pm.pm_483_16
Li, B., Yang, Z. B., Lei, S. S., Su, J., Pang, M. X., Yin, C., et al. (2017). Beneficial effects of paeoniflorin enriched extract on blood pressure variability and target organ damage in spontaneously hypertensive rats. Evid. Based Complement. Altern. Med. 2017, 5816960. doi:10.1155/2017/5816960
Li, H., Jiao, Y., and Xie, M. (2017). Paeoniflorin ameliorates atherosclerosis by suppressing TLR4-mediated NF-κB activation. Inflammation 40 (6), 2042–2051. doi:10.1007/s10753-017-0644-z
Li, J. Z., Tang, X. N., Li, T. T., Liu, L. J., Yu, S. Y., Zhou, G. Y., et al. (2016). Paeoniflorin inhibits doxorubicin-induced cardiomyocyte apoptosis by downregulating microRNA-1 expression. Exp. Ther. Med. 11 (6), 2407–2412. doi:10.3892/etm.2016.3182
Li, J. Z., Wu, J. H., Yu, S. Y., Shao, Q. R., and Dong, X. M. (2013). Inhibitory effects of paeoniflorin on lysophosphatidylcholine-induced inflammatory factor production in human umbilical vein endothelial cells. Int. J. Mol. Med. 31 (2), 493–497. doi:10.3892/ijmm.2012.1211
Li, W., Tao, W., Chen, J., Zhai, Y., Yin, N., and Wang, Z. (2020). Paeoniflorin suppresses IL-33 production by macrophages. Immunopharmacol. Immunotoxicol. 42 (3), 286–293. doi:10.1080/08923973.2020.1750628
Li, W., Zhi, W., Liu, F., Zhao, J., Yao, Q., and Niu, X. (2018). Paeoniflorin inhibits VSMCs proliferation and migration by arresting cell cycle and activating HO-1 through MAPKs and NF-κB pathway. Int. Immunopharmacol. 54, 103–111. doi:10.1016/j.intimp.2017.10.017
Li, Y., Chen, K., and Shi, Z. (1998). Effect of xuefu zhuyu pill on blood stasis syndrome and risk factor of atherosclerosis. Zhongguo Zhong Xi Yi Jie He Za Zhi 18 (2), 71–73.
Li, Y. C., Qiao, J. Y., Wang, B. Y., Bai, M., Shen, J. D., and Cheng, Y. X. (2018). Paeoniflorin ameliorates fructose-induced insulin resistance and hepatic steatosis by activating LKB1/AMPK and AKT pathways. Nutrients 10 (8), 1024. doi:10.3390/nu10081024
Li, Y., Yin, S., Chen, X., Shi, F., Wang, J., and Yang, H. (2022). The inhibitory effect of paeoniflorin on reactive oxygen species alleviates the activation of NF-κB and MAPK signalling pathways in macrophages. Microbiol. Read. 168 (8). doi:10.1099/mic.0.001210
Lin, Y. T., Huang, W. S., Tsai, H. Y., Lee, M. M., and Chen, Y. F. (2019). In vivo microdialysis and in vitro HPLC analysis of the impact of paeoniflorin on the monoamine levels and their metabolites in the rodent brain. Biomed. (Taipei) 9 (2), 11. doi:10.1051/bmdcn/2019090211
Liu, M., Ai, J., Feng, J., Zheng, J., Tang, K., Shuai, Z., et al. (2019). Effect of paeoniflorin on cardiac remodeling in chronic heart failure rats through the transforming growth factor β1/Smad signaling pathway. Cardiovasc Diagn Ther. 9 (3), 272–280. doi:10.21037/cdt.2019.06.01
Liu, T., Zhang, N., Kong, L., Chu, S., Zhang, T., Yan, G., et al. (2022). Paeoniflorin alleviates liver injury in hypercholesterolemic rats through the ROCK/AMPK pathway. Front. Pharmacol. 13, 968717. doi:10.3389/fphar.2022.968717
Liu, X., Chen, K., Zhuang, Y., Huang, Y., Sui, Y., Zhang, Y., et al. (2019). Paeoniflorin improves pressure overload-induced cardiac remodeling by modulating the MAPK signaling pathway in spontaneously hypertensive rats. Biomed. Pharmacother. 111, 695–704. doi:10.1016/j.biopha.2018.12.090
Liu, Y., Han, J., Zhou, Z., and Li, D. (2019). Paeoniflorin protects pancreatic beta cells from STZ-induced damage through inhibition of the p38 MAPK and JNK signaling pathways. Eur. J. Pharmacol. 853, 18–24. doi:10.1016/j.ejphar.2019.03.025
Liu, Z. Q., Jiang, Z. H., Liu, L., and Hu, M. (2006). Mechanisms responsible for poor oral bioavailability of paeoniflorin: Role of intestinal disposition and interactions with sinomenine. Pharm. Res. 23 (12), 2768–2780. doi:10.1007/s11095-006-9100-8
Long-Tao, L. (2018). Chinese experts consensus on clinical application of naoxintong capsule. Chin. J. Integr. Med. 24 (3), 232–236. doi:10.1007/s11655-018-2981-6
Lu, Y., Deng, Y., Liu, W., Jiang, M., and Bai, G. (2019). Searching for calcium antagonists for hypertension disease therapy from Moutan Cortex, using bioactivity integrated UHPLC-QTOF-MS. Phytochem. Anal. 30 (4), 456–463. doi:10.1002/pca.2828
Luo, N., Li, Z., Qian, D., Qian, Y., Guo, J., Duan, J. A., et al. (2014). Simultaneous determination of bioactive components of Radix Angelicae Sinensis-Radix Paeoniae Alba herb couple in rat plasma and tissues by UPLC-MS/MS and its application to pharmacokinetics and tissue distribution. J. Chromatogr. B Anal. Technol. Biomed. Life Sci. 963, 29–39. doi:10.1016/j.jchromb.2014.05.036
Lv, P., Peng, Q., Liu, Y., Jin, H., Liu, R., et al. (2016). Treatment with the herbal medicine, naoxintong improves the protective effect of high-density lipoproteins on endothelial function in patients with type 2 diabetes. Mol. Med. Rep. 13 (3), 2007–2016. doi:10.3892/mmr.2016.4792
Ma, Z., Chu, L., Liu, H., Wang, W., Li, J., Yao, W., et al. (2017). Beneficial effects of paeoniflorin on non-alcoholic fatty liver disease induced by high-fat diet in rats. Sci. Rep. 7, 44819. doi:10.1038/srep44819
Ma, Z., Liu, H., Wang, W., Guan, S., Yi, J., and Chu, L. (2017). Paeoniflorin suppresses lipid accumulation and alleviates insulin resistance by regulating the Rho kinase/IRS-1 pathway in palmitate-induced HepG2Cells. Biomed. Pharmacother. 90, 361–367. doi:10.1016/j.biopha.2017.03.087
Madsbad, S. (1992). Hypoglycemia in diabetes mellitus. Symptoms, epidemiology, physiopathology, causes and treatment. Ugeskr. Laeger 154 (5), 246–250.
Mao, L., Chen, J., Cheng, K., Dou, Z., Leavenworth, J. D., Yang, H., et al. (2022). Nrf2-Dependent protective effect of paeoniflorin on α-naphthalene isothiocyanate-induced hepatic injury. Am. J. Chin. Med. 50 (5), 1331–1348. doi:10.1142/S0192415X22500562
Mao, S., Wang, L., Ouyang, W., Zhou, Y., Qi, J., Guo, L., et al. (2016). Traditional Chinese medicine, Danlou tablets alleviate adverse left ventricular remodeling after myocardial infarction: Results of a double-blind, randomized, placebo-controlled, pilot study. BMC Complement. Altern. Med. 16 (1), 447. doi:10.1186/s12906-016-1406-4
Misao, J., Hayakawa, Y., Ohno, M., Kato, S., Fujiwara, T., and Fujiwara, H. (1996). Expression of bcl-2 protein, an inhibitor of apoptosis, and Bax, an accelerator of apoptosis, in ventricular myocytes of human hearts with myocardial infarction. Circulation 94 (7), 1506–1512. doi:10.1161/01.cir.94.7.1506
Most, J., Schwaeble, W., and Dierich, M. P. (1992). Expression of intercellular adhesion molecule-1 (ICAM-1) on human monocytes. Immunobiology 185 (2-4), 327–336. doi:10.1016/S0171-2985(11)80650-9
Murray, P. J. (2017). Macrophage polarization. Annu. Rev. Physiol. 79, 541–566. doi:10.1146/annurev-physiol-022516-034339
Ngo, T., Kim, K., Bian, Y., Noh, H., Lim, K. M., Chung, J. H., et al. (2019). Antithrombotic effects of paeoniflorin from Paeonia suffruticosa by selective inhibition on shear stress-induced platelet aggregation. Int. J. Mol. Sci. 20 (20), 5040. doi:10.3390/ijms20205040
Nizamutdinova, I. T., Jin, Y. C., Kim, J. S., Yean, M. H., Kang, S. S., Kim, Y. S., et al. (2008). Paeonol and paeoniflorin, the main active principles of Paeonia albiflora, protect the heart from myocardial ischemia/reperfusion injury in rats. Planta Med. 74 (1), 14–18. doi:10.1055/s-2007-993775
O'Sullivan, J. W., Raghavan, S., Marquez-Luna, C., Luzum, J. A., Damrauer, S. M., Ashley, E. A., et al. (2022). Polygenic risk scores for cardiovascular disease: A scientific statement from the American heart association. Circulation 146 (8), e93–e118. doi:10.1161/CIR.0000000000001077
Oka, T., Akazawa, H., Naito, A. T., and Komuro, I. (2014). Angiogenesis and cardiac hypertrophy: Maintenance of cardiac function and causative roles in heart failure. Circ. Res. 114 (3), 565–571. doi:10.1161/CIRCRESAHA.114.300507
Olvera Lopez, E., Ballard, B. D., and Jan, A. (2022). Cardiovascular disease. Treasure Island (FL: StatPearls.
Osaki, Y., Nakagawa, Y., Miyahara, S., Iwasaki, H., Ishii, A., Matsuzaka, T., et al. (2015). Skeletal muscle-specific HMG-CoA reductase knockout mice exhibit rhabdomyolysis: A model for statin-induced myopathy. Biochem. Biophys. Res. Commun. 466 (3), 536–540. doi:10.1016/j.bbrc.2015.09.065
Pirillo, A., Casula, M., Olmastroni, E., Norata, G. D., and Catapano, A. L. (2021). Global epidemiology of dyslipidaemias. Nat. Rev. Cardiol. 18 (10), 689–700. doi:10.1038/s41569-021-00541-4
Rivard, A., and Andres, V. (2000). Vascular smooth muscle cell proliferation in the pathogenesis of atherosclerotic cardiovascular diseases. Histol. Histopathol. 15 (2), 557–571. doi:10.14670/HH-15.557
Ruan, Y., Ling, J., Ye, F., Cheng, N., Wu, F., Tang, Z., et al. (2021). Paeoniflorin alleviates CFA-induced inflammatory pain by inhibiting TRPV1 and succinate/SUCNR1-HIF-1α/NLPR3 pathway. Int. Immunopharmacol. 101, 108364. doi:10.1016/j.intimp.2021.108364
Senoner, T., and Dichtl, W. (2019). Oxidative stress in cardiovascular diseases: Still a therapeutic target? Nutrients 11 (9), 2090. doi:10.3390/nu11092090
Seravalle, G., and Grassi, G. (2017). Obesity and hypertension. Pharmacol. Res. 122, 1–7. doi:10.1016/j.phrs.2017.05.013
Shaito, A., Aramouni, K., Assaf, R., Parenti, A., Orekhov, A., Yazbi, A. E., et al. (2022). Oxidative stress-induced endothelial dysfunction in cardiovascular diseases. Front. Biosci. (Landmark Ed. 27 (3), 105. doi:10.31083/j.fbl2703105
Shao, Y. X., Gong, Q., Qi, X. M., Wang, K., and Wu, Y. G. (2019). Paeoniflorin ameliorates macrophage infiltration and activation by inhibiting the TLR4 signaling pathway in diabetic nephropathy. Front. Pharmacol. 10, 566. doi:10.3389/fphar.2019.00566
Shen, C., Shen, B., Zhu, J., Wang, J., Yuan, H., and Li, X. (2021). Glycyrrhizic acid-based self-assembled micelles for improving oral bioavailability of paeoniflorin. Drug Dev. industrial Pharm. 47 (2), 207–214. doi:10.1080/03639045.2020.1862178
Shenasa, M., and Shenasa, H. (2017). Hypertension, left ventricular hypertrophy, and sudden cardiac death. Int. J. Cardiol. 237, 60–63. doi:10.1016/j.ijcard.2017.03.002
Shibata, S., and Nakahara, M. (1963). Studies on the constituents of Japanese and Chinese crude drugs. VIII. Paeoniflorin, A glucoside of Chinese paeony root.(1). Chem. Pharm. Bull. 11 (3), 372–378. doi:10.1248/cpb.11.372
Shoemaker, R., Yiannikouris, F., Thatcher, S., and Cassis, L. (2015). ACE2 deficiency reduces beta-cell mass and impairs beta-cell proliferation in obese C57BL/6 mice. Am. J. Physiol. Endocrinol. Metab. 309 (7), E621–E631. doi:10.1152/ajpendo.00054.2015
Song, S., Xiao, X., Guo, D., Mo, L., Bu, C., Ye, W., et al. (2017). Protective effects of Paeoniflorin against AOPP-induced oxidative injury in HUVECs by blocking the ROS-HIF-1α/VEGF pathway. Phytomedicine 34, 115–126. doi:10.1016/j.phymed.2017.08.010
Suganami, T., Nishida, J., and Ogawa, Y. (2005). A paracrine loop between adipocytes and macrophages aggravates inflammatory changes: Role of free fatty acids and tumor necrosis factor alpha. Arterioscler. Thromb. Vasc. Biol. 25 (10), 2062–2068. doi:10.1161/01.ATV.0000183883.72263.13
Sun, B., Dai, G., Bai, Y., Zhang, W., Zhu, L., Chu, J., et al. (2017). Determination of paeoniflorin in rat plasma by ultra-high performance liquid chromatography-tandem mass spectrometry and its application to a pharmacokinetic study. J. Chromatogr. Sci. 55 (10), 1006–1012. doi:10.1093/chromsci/bmx066
Sun, H., Wang, J., and Lv, J. (2019). Effects of glycyrrhizin on the pharmacokinetics of paeoniflorin in rats and its potential mechanism. Pharm. Biol. 57 (1), 550–554. doi:10.1080/13880209.2019.1651876
Sun, L.-C., Li, S., Wang, F., and Xin, F. (2017). Research progresses in the synthetic biology of terpenoids. Biotechnol. Bull. 33 (1), 64.
Tomek, J., and Bub, G. (2017). Hypertension-induced remodelling: On the interactions of cardiac risk factors. J. Physiol. 595 (12), 4027–4036. doi:10.1113/JP273043
Townsend, N., Kazakiewicz, D., Lucy Wright, F., Timmis, A., Huculeci, R., Torbica, A., et al. (2022). Epidemiology of cardiovascular disease in Europe. Nat. Rev. Cardiol. 19 (2), 133–143. doi:10.1038/s41569-021-00607-3
Tu, J., Guo, Y., Hong, W., Fang, Y., Han, D., Zhang, P., et al. (2019). The regulatory effects of paeoniflorin and its derivative paeoniflorin-6'-O-benzene sulfonate CP-25 on inflammation and immune diseases. Front. Pharmacol. 10, 57. doi:10.3389/fphar.2019.00057
Van Buul, J. D., Fernandez-Borja, M., Anthony, E. C., and Hordijk, P. L. (2005). Expression and localization of NOX2 and NOX4 in primary human endothelial cells. Antioxid. Redox Signal 7 (3-4), 308–317. doi:10.1089/ars.2005.7.308
Vignais, P. V. (2002). The superoxide-generating NADPH oxidase: Structural aspects and activation mechanism. Cell Mol. Life Sci. 59 (9), 1428–1459. doi:10.1007/s00018-002-8520-9
Wang, C., Yuan, J., Zhang, L. L., and Wei, W. (2016). Pharmacokinetic comparisons of Paeoniflorin and Paeoniflorin-6'O-benzene sulfonate in rats via different routes of administration. Xenobiotica 46 (12), 1142–1150. doi:10.3109/00498254.2016.1149633
Wang, L., Mao, S., Qi, J. y., Ren, Y., Guo, X. f., Chen, K. j., et al. (2015). Effect of Danlou tablet on peri-procedural myocardial injury among patients undergoing percutaneous coronary intervention for non-ST elevation acute coronary syndrome: A study protocol of a multicenter, randomized, controlled trial. Chin. J. Integr. Med. 21 (9), 662–666. doi:10.1007/s11655-015-2284-1
Wang, R., Ren, J., Li, S., Bai, X., Guo, W., Yang, S., et al. (2022). Efficacy evaluation of buyang huanwu decoction in the treatment of ischemic stroke in the recovery period: A systematic review of randomized controlled trials. Front. Pharmacol. 13, 975816. doi:10.3389/fphar.2022.975816
Wang, R. R., Zhang, Y. H., Ran, Y. Q., and Pu, J. L. (2011). The effects of paeoniflorin monomer of a Chinese herb on cardiac ion channels. Chin. Med. J. Engl. 124 (19), 3105–3111.
Wang, S., and Qiu, X. J. (2019). The efficacy of xue fu zhu Yu prescription for hyperlipidemia: A meta-analysis of randomized controlled trials. Complement. Ther. Med. 43, 218–226. doi:10.1016/j.ctim.2019.02.008
Wang, Y., Xu, Y., Zhang, L., Huang, S., Dou, L., Yang, J., et al. (2022). Comparison of buyang huanwu granules and naoxintong capsules in the treatment of stable angina pectoris: Rationale and design of a randomized, blinded, multicentre clinical trial. Trials 23 (1), 65. doi:10.1186/s13063-021-05914-1
Wei, S., Ma, X., Niu, M., Wang, R., Yang, T., Wang, D., et al. (2020). Mechanism of paeoniflorin in the treatment of bile duct ligation-induced cholestatic liver injury using integrated metabolomics and network pharmacology. Front. Pharmacol. 11, 586806. doi:10.3389/fphar.2020.586806
Wong, N. D., Budoff, M. J., Ferdinand, K., Graham, I. M., Michos, E. D., Reddy, T., et al. (2022). Atherosclerotic cardiovascular disease risk assessment: An American Society for Preventive Cardiology clinical practice statement. Am. J. Prev. Cardiol. 10, 100335. doi:10.1016/j.ajpc.2022.100335
Wu, F., Ye, B., Wu, X., Lin, X., Li, Y., Wu, Y., et al. (2020). Paeoniflorin on rat myocardial ischemia reperfusion injury of protection and mechanism research. Pharmacology 105 (5-6), 281–288. doi:10.1159/000503583
Xia, S. M., Shen, R., Sun, X. Y., Shen, L. L., Yang, Y. M., Ke, Y., et al. (2007). Development and validation of a sensitive liquid chromatography-tandem mass spectrometry method for the determination of paeoniflorin in rat brain and its application to pharmacokinetic study. J. Chromatogr. B Anal. Technol. Biomed. Life Sci. 857 (1), 32–39. doi:10.1016/j.jchromb.2007.06.022
Xiao, H. B., Liang, L., Luo, Z. F., and Sun, Z. L. (2018). Paeoniflorin regulates GALNT2-ANGPTL3-LPL pathway to attenuate dyslipidemia in mice. Eur. J. Pharmacol. 836, 122–128. doi:10.1016/j.ejphar.2018.08.006
Xiao, H. B., Wang, J. Y., and Sun, Z. L. (2017). ANGPTL3 is part of the machinery causing dyslipidemia majorily via LPL inhibition in mastitis mice. Exp. Mol. Pathol. 103 (3), 242–248. doi:10.1016/j.yexmp.2017.11.003
Xie, P., Cui, L., Shan, Y., and Kang, W. Y. (2017). Antithrombotic effect and mechanism of radix paeoniae rubra. Biomed. Res. Int. 2017, 9475074. doi:10.1155/2017/9475074
Xin, Q. Q., Yang, B. R., Zhou, H. F., Wang, Y., Yi, B. W., Cong, W. H., et al. (2018). Paeoniflorin promotes angiogenesis in A vascular insufficiency model of zebrafish in vivo and in human umbilical vein endothelial cells in vitro. Chin. J. Integr. Med. 24 (7), 494–501. doi:10.1007/s11655-016-2262-2
Xin, Q., Yuan, R., Shi, W., Zhu, Z., Wang, Y., and Cong, W. (2019). A review for the anti-inflammatory effects of paeoniflorin in inflammatory disorders. Life Sci. 237, 116925. doi:10.1016/j.lfs.2019.116925
Xu, H., Shi, D. Z., and Chen, K. J. (2001). Effect of xiongshao capsule on vascular remodeling in porcine coronary balloon injury model. Zhongguo Zhong Xi Yi Jie He Za Zhi 21 (8), 591–594.
Xu, J. X., Cao, Y., Zhu, Y. J., Li, X. Y., Ge, D. Z., et al. (2021). Modern research progress of traditional Chinese medicine Paeoniae Radix Alba and prediction of its Q-markers. Zhongguo Zhong Yao Za Zhi 46 (21), 5486–5495. doi:10.19540/j.cnki.cjcmm.20210818.201
Xu, W., Zhao, Y., Qin, Y., Ge, B., Gong, W., Wu, Y., et al. (2016). Enhancement of exposure and reduction of elimination for paeoniflorin or albiflorin via Co-administration with total Peony glucosides and hypoxic pharmacokinetics comparison. Molecules 21 (7), 874. doi:10.3390/molecules21070874
Xu, X., Wang, Y., Li, Y., Zhang, B., and Song, Q. (2022). The future landscape of macrophage research in cardiovascular disease: A bibliometric analysis. Curr. Probl. Cardiol. 47 (10), 101311. doi:10.1016/j.cpcardiol.2022.101311
Yang, G., He, H., Li, H., Shen, Z., Zhou, S., Lu, B., et al. (2020). Effects of Danlou tablet for the treatment of stable angina pectoris: A study protocol of a randomized, double-blind, and placebo-controlled clinical trial. Med. Baltim. 99 (49), e23416. doi:10.1097/MD.0000000000023416
Yang, H. O., Ko, W. K., Kim, J. Y., and Ro, H. S. (2004). Paeoniflorin: An antihyperlipidemic agent from Paeonia lactiflora. Fitoterapia 75 (1), 45–49. doi:10.1016/j.fitote.2003.08.016
Yang, X. Z., and Wei, W. (2020). CP-25, a compound derived from paeoniflorin: Research advance on its pharmacological actions and mechanisms in the treatment of inflammation and immune diseases. Acta Pharmacol. Sin. 41 (11), 1387–1394. doi:10.1038/s41401-020-00510-6
Yellon, D. M., and Hausenloy, D. J. (2007). Myocardial reperfusion injury. N. Engl. J. Med. 357 (11), 1121–1135. doi:10.1056/NEJMra071667
Yu, J. B., Zhao, Z. X., Peng, R., Pan, L. B., Fu, J., Ma, S. R., et al. (2019). Gut microbiota-based pharmacokinetics and the antidepressant mechanism of paeoniflorin. Front. Pharmacol. 10, 268. doi:10.3389/fphar.2019.00268
Yuan, R., Shi, W., Xin, Q., Yang, B., Hoi, M. P., Lee, S. M., et al. (2018). Tetramethylpyrazine and paeoniflorin inhibit oxidized LDL-induced angiogenesis in human umbilical vein endothelial cells via VEGF and notch pathways. Evid. Based Complement. Altern. Med. 2018, 3082507. doi:10.1155/2018/3082507
Yusuf, S., Joseph, P., Rangarajan, S., Islam, S., Mente, A., Hystad, P., et al. (2020). Modifiable risk factors, cardiovascular disease, and mortality in 155 722 individuals from 21 high-income, middle-income, and low-income countries (PURE): A prospective cohort study. Lancet 395 (10226), 795–808. doi:10.1016/S0140-6736(19)32008-2
Zhai, J., and Guo, Y. (2016). Paeoniflorin attenuates cardiac dysfunction in endotoxemic mice via the inhibition of nuclear factor-κB. Biomed. Pharmacother. 80, 200–206. doi:10.1016/j.biopha.2016.03.032
Zhai, T., Sun, Y., Li, H., Zhang, J., Huo, R., Li, H., et al. (2016). Unique immunomodulatory effect of paeoniflorin on type I and II macrophages activities. J. Pharmacol. Sci. 130 (3), 143–150. doi:10.1016/j.jphs.2015.12.007
Zhang, H., Liang, M. J., and Ma, Z. X. (1995). Clinical study on effects of buyang huanwu decoction on coronary heart disease. Zhongguo Zhong Xi Yi Jie He Za Zhi 15 (4), 213–215.
Zhang, L., and Wei, W. (2020). Anti-inflammatory and immunoregulatory effects of paeoniflorin and total glucosides of paeony. Pharmacol. Ther. 207, 107452. doi:10.1016/j.pharmthera.2019.107452
Zhang, L., Yang, B., and Yu, B. (2015). Paeoniflorin protects against nonalcoholic fatty liver disease induced by a high-fat diet in mice. Biol. Pharm. Bull. 38 (7), 1005–1011. doi:10.1248/bpb.b14-00892
Zhang, M., Liu, Y., Xu, M., Zhang, L., Liu, Y., Liu, X., et al. (2019). Carotid artery plaque intervention with tongxinluo capsule (capital): A multicenter randomized double-blind parallel-group placebo-controlled study. Sci. Rep. 9 (1), 4545. doi:10.1038/s41598-019-41118-z
Zhang, S., Chen, Z. L., Tang, Y. P., Duan, J. L., and Yao, K. W. (2021). Efficacy and safety of xue-fu-zhu-yu decoction for patients with coronary heart disease: A systematic review and meta-analysis. Evid. Based Complement. Altern. Med. 2021, 9931826. doi:10.1155/2021/9931826
Zhang, S., Jiang, M., Yan, S., Liang, M., Wang, W., Yuan, B., et al. (2021). Network pharmacology-based and experimental identification of the effects of paeoniflorin on major depressive disorder. Front. Pharmacol. 12, 793012. doi:10.3389/fphar.2021.793012
Zhang, X. X., Zuo, J. Q., Wang, Y. T., Duan, H. Y., Yuan, J. H., and Hu, Y. H. (2022). Paeoniflorin in Paeoniaceae: Distribution, influencing factors, and biosynthesis. Front. Plant Sci. 13, 980854. doi:10.3389/fpls.2022.980854
Zhao, X., Chen, Y., Li, L., Zhai, J., Yu, B., Wang, H., et al. (2021). Effect of DLT-SML on chronic stable angina through ameliorating inflammation, correcting dyslipidemia, and regulating gut microbiota. J. Cardiovasc Pharmacol. 77 (4), 458–469. doi:10.1097/FJC.0000000000000970
Zhou, H., Yang, H. X., Yuan, Y., Deng, W., Zhang, J. Y., Bian, Z. Y., et al. (2013). Paeoniflorin attenuates pressure overload-induced cardiac remodeling via inhibition of TGFβ/Smads and NF-κB pathways. J. Mol. Histol. 44 (3), 357–367. doi:10.1007/s10735-013-9491-x
Zhou, S.-L., Xu, C., Liu, J., Yu, Y., Wu, P., Cheng, T., et al. (2021). Out of the pan-himalaya: Evolutionary history of the Paeoniaceae revealed by phylogenomics. J. Syst. Evol. 59 (6), 1170–1182. doi:10.1111/jse.12688
Keywords: paeoniflorin, cardiovascular diseases, mechanism, pharmacology, traditional Chinese medicine
Citation: Li X, Sun C, Zhang J, Hu L, Yu Z, Zhang X, Wang Z, Chen J, Wu M and Liu L (2023) Protective effects of paeoniflorin on cardiovascular diseases: A pharmacological and mechanistic overview. Front. Pharmacol. 14:1122969. doi: 10.3389/fphar.2023.1122969
Received: 13 December 2022; Accepted: 10 April 2023;
Published: 30 May 2023.
Edited by:
Hai-Dong Guo, Shanghai University of Traditional Chinese Medicine, ChinaReviewed by:
Peng Zhou, Anhui University of Chinese Medicine, ChinaWei Zhou, China Pharmaceutical University, China
Copyright © 2023 Li, Sun, Zhang, Hu, Yu, Zhang, Wang, Chen, Wu and Liu. This is an open-access article distributed under the terms of the Creative Commons Attribution License (CC BY). The use, distribution or reproduction in other forums is permitted, provided the original author(s) and the copyright owner(s) are credited and that the original publication in this journal is cited, in accordance with accepted academic practice. No use, distribution or reproduction is permitted which does not comply with these terms.
*Correspondence: Min Wu, d3VtaW4xOTc2MjAwMEAxMjYuY29t; Longtao Liu, bGl1bG9uZ3RhbzE5NzZAMTI2LmNvbQ==