- 1School of Intelligent Medicine, Chengdu University of Traditional Chinese Medicine, Chengdu, China
- 2School of Pharmacy, Chengdu University of Traditional Chinese Medicine, Chengdu, China
- 3Pharmaceutics Center, Institute of Medicinal Plant Development, Chinese Academy of Medical Sciences and Peking Union Medical College, Beijing, China
- 4School of Basic Medical Sciences, Chengdu University of Traditional Chinese Medicine, Chengdu, China
Background: In recent years, the incidence and mortality rates of chronic obstructive pulmonary disease (COPD) have increased significantly. Erchen Decoction combined with Xiebai Powder (ECXB) formula is mainly used to treat lung diseases in traditional Chinese medicine (TCM). However, the active ingredients of ECXB formula, COPD treatment-related molecular targets, and the mechanisms are still unclear. To reveal its underlying action of mechanism, network pharmacology, molecular docking, and molecular dynamic (MD) simulation approaches were used to predict the active ingredients and potential targets of ECXB formula in treating COPD. As a result, Herb-Symptom analysis showed that the symptoms treated by both TCM and modern medicine of ECXB formula were similar to the symptoms of COPD. Network pharmacology identified 170 active ingredients with 137 targets, and 7,002 COPD targets was obtained. 120 targets were obtained by intersection mapping, among which the core targets include MAPK8, ESR1, TP53, MAPK3, JUN, RELA, MAPK1, and AKT1. Functional enrichment analysis suggested that ECXB formula might exert its treat COPD pharmacological effects in multiple biological processes, such as cell proliferation, apoptosis, inflammatory response, and synaptic connections, and ECXB formula treated COPD of the KEGG potential pathways might be associated with the TNF signaling pathway, cAMP signaling pathway, and VEGF signaling pathway. Molecular docking showed that ECXB formula treatment COPD core active ingredients can bind well to core targets. MD simulations showed that the RELA-beta-sitosterol complex and ESR1-stigmasterol complex exhibited higher conformational stability and lower interaction energy, further confirming the role of ECXB formula in the treatment of COPD through these core components and core targets. Our study analyzed the medication rule of ECXB formula in the treatment of COPD from a new perspective and found that the symptoms treated by both TCM and modern medicine of ECXB formula were similar to the symptoms of COPD. ECXB formula could treat COPD through multi-component, multi-target, and multi-pathway synergistic effects, providing a scientific basis for further study on the mechanism of ECXB formula treatment of COPD. It also provides new ideas for drug development.
1 Introduction
Chronic obstructive pulmonary disease (COPD) is a common chronic respiratory disease mainly characterized by incompletely reversible airflow restriction. In Europe and the United States, the prevalence of COPD ranges from 3.4% to 13.4% (Blanco et al., 2018), However, in Asia, its prevalence ranges from 3.5% to 19.1% (Buist et al., 2007). Outpatient visit cost accounts for 15%–41% of the total direct cost in the United States and Europe and 4%–48% of the total direct cost in Asian countries (Rehman et al., 2020), one of the main contributors to the global burden of disease. Hence, an in-depth study on COPD is still an urgent need.
Global Strategy for the Diagnosis, Management, and Prevention of Chronic Obstructive Lung Disease: the GOLD science committee report 2019 Singh et al. (2019) proposed stable COPD treatment methods mainly include smoking cessation, bronchodilator, mucolytic agent, immunomodulator, respiratory function exercise, home oxygen therapy, and nutritional therapy. The above methods can improve the symptoms, respiratory function, and quality of life of patients with stable COPD to a certain extent, but all of them need to be maintained for a long time. Due to the high price of non-invasive ventilators, in addition, some patients believe that the comfort of wearing is not good, poor compliance, lack of knowledge, and other factors limit, little benefit, and increase the economic burden for patients.
COPD belongs to the category of “lung distension” in traditional Chinese medicine (TCM), and TCM has unique advantages in the treatment of COPD. Zhen et al. (2018) confirmed in the systematic evaluation and meta-analysis of TCM tonalizing kidney therapy (Bu shen) in the treatment of stable chronic obstructive pulmonary disease that tonalizing kidney therapy can increase lung capacity and reduce the number of CD4+ and CD8+ lymphocytes in patients with stable chronic obstructive pulmonary disease. Huang et al. (2022) found that the TCM combined with Western medicine (WM) can effectively treat the symptoms related to COPD, the treatment efficiency is significantly improved compared with traditional WM. Therefore, it is very meaningful to study the treatment of COPD with TCM.
Erchen Decoction is composed of Chenpi (Pericarpium Citri Reticulatae), Banxia (Pinellia ternata), Fulin (Poria cocos) and Gancao (Glycyrrhizae Radix et Rhizome), Xiebai Powder is composed of Sangbaipi (Mori cortex), Digupi (Lycii Cortex), Gancao (Glycyrrhizae radix et rhizome), Non-glutinous Rice, both TCM formulas have been widely used to treat respiratory diseases (Deng et al., 2020; Zhang et al., 2022). In China, two enduring and effective Chinese classical formulas derived from TCM with a long-standing history spanning centuries, namely, Erchen Decoction combined with Xiebai Powder (ECXB formula), have been extensively employed in treating COPD. Mei and Li (2012) observed the effect of ECXB formula in the treatment of acute exacerbation of COPD and found that the use of this formula can shorten the course of COPD and improve the curative effect. The empirical formula created by famous veteran doctors of TCM is derived from ECXB formula, which can control infection and the accompanied symptoms caused by infection and has good clinical effects in treating COPD (Li and Luo, 2014). So far, the main components, target, and potential synergy mechanism of ECXB formula are not yet clear.
In this study, we aimed to use the SymMap platform to analyze the relationship between the symptoms treated by ECXB formula and the symptoms of COPD. Furthermore, network pharmacology, molecular docking, and molecular dynamic (MD) simulation approaches explore potential targets and mechanisms of ECXB formula for COPD treatment. This study provided a scientific basis for future validation experiments and clinical applications. The detailed flowchart of this study is summarized in Figure 1.
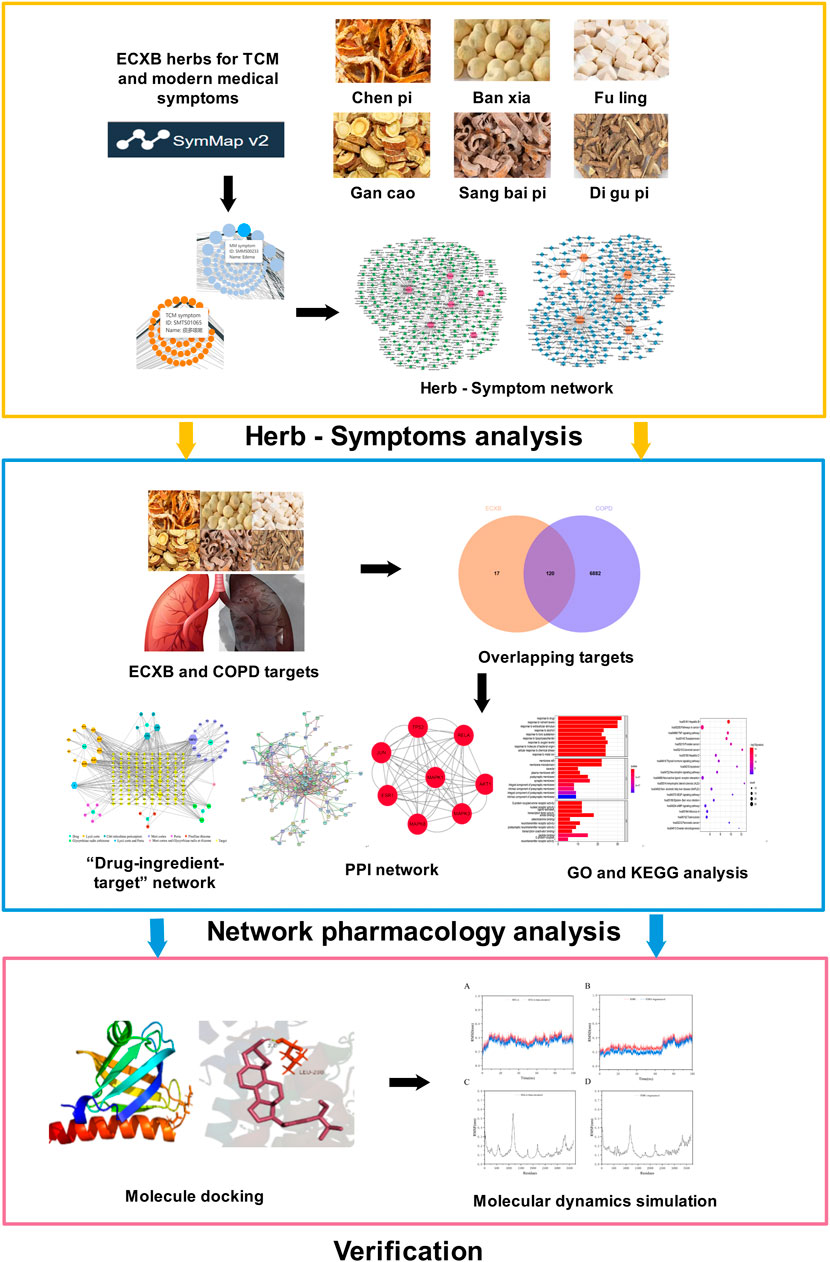
FIGURE 1. The flowchart of this study is based on Herb-Symptom analysis, network pharmacology, molecular docking, and molecular dynamics simulation for deciphering the potential mechanisms of ECXB formula treatment COPD.
2 Materials and methods
2.1 “Herb-symptom” network construction
SymMap platform (http://www.symmap.org/) (Wu et al., 2019) was utilized to collect related symptoms treated by both TCM and modern medicine of Pinellia ternata, Pericarpium Citri Reticulatae, Poria cocos, Glycyrrhizae Radix et Rhizome, Lycii cortex, and Mori cortex in the formula of ECXB. Cytoscape 3.9.0 software (Shannon et al., 2003) was used for visual analyses of the network of “Herb-TCM symptoms” and “Herb-modern medicine symptoms.” According to the International Clinical Practice Guideline of Chinese Medicine Chronic Obstructive Pulmonary Disease (Li, 2020) and Global Strategy for the Diagnosis, Management, and Prevention of Chronic Obstructive Lung Disease: the GOLD science committee report 2019 (Singh et al., 2019), related COPD symptoms were collected.
2.2 Collection of active ingredients and prediction on targets of ECXB formula
The active ingredients of ECXB formula were searched by the TCM Systems Pharmacology Database and Analysis Platform (TCMSP, https://old.tcmsp-e.com/tcmsp.php) (Ru et al., 2014). Oral bioavailability (OB) (Xu et al., 2012) represents the percentage of an orally administered dose of an unchanged drug that reaches the systemic circulation, which reveals the convergence of the ADME process. High oral bioavailability is often a key indicator to determine the drug-like property of bioactive molecules as therapeutic agents. Drug-likeness (DL) (Tao et al., 2013) is a qualitative concept used in drug design to estimate how “drug-like” a prospective compound is, which helps to optimize pharmacokinetic and pharmaceutical properties, such as solubility and chemical stability. The “drug-like” level of the compounds is 0.18, which is used as a selection criterion for the “drug-like” compounds in traditional Chinese herbs. Therefore, the active ingredients were screened according to the following criteria: OB ≥ 30% and DL ≥ 0.18. Using the PubChem database (https://pubchem.ncbi.nlm.nih.gov/) (Kim et al., 2021), the SMILE number corresponding to the active ingredients screened from the TCMSP database was downloaded. The SMILE structure of active ingredients was uploaded to the Swiss Target Prediction database (http://www.swisstargetprediction.ch/) (Daina et al., 2019) for target prediction. The predicted targets of active ingredients were obtained and integrated. UniProt database (https://www.uniprot.org/) (Bateman et al., 2021) was used for standardized and unified processing of target names, and the species was limited to “HUMAN.”
2.3 Collection of disease target and prediction of intersection target
By taking “Chronic obstructive pulmonary diseases” as the keyword, the related targets were searched in the GeneCards® database (https://www.genecards.org) (Safran et al., 2021) and OMIM ® database (https://omim.org/) (Amberger et al., 2015). The predicted targets of ECXB formula were mapped to the related targets of COPD, and the common targets of ECXB formula in the treatment of COPD were obtained and further visualized using a Venn diagram.
2.4 “Drug-ingredient-target” network and core active ingredients screening
The common targets of ECXB formula in the treatment of COPD have imported into Cytoscape 3.9.0 software, the network diagram of “Drug-ingredient-target” was constructed, and the core active ingredients were screened according to the degree value ranking.
2.5 PPI network construction and screening of core protein targets
The intersection target of ECXB formula in the treatment of COPD was imported into the STRING online website (https://cn.string-db.org/) (Szklarczyk et al., 2019), and the protein type was set as “Homo sapiens”, the highest confidence parameter score was greater than 0.7, and other parameters were set as default values (network type: full STRING network, meaning of network edges: evidence). The core network of ECXB formula for the treatment of COPD protein interaction was constructed, and the TSV file was exported. Node1, node2, and the combined score in the TSV file were imported into Cytoscape 3.9.0 software, mapping interaction networks, and screening core protein targets.
2.6 Enrichment analysis of GO and KEGG
Gene Ontology (GO) analysis and Kyoto Encyclopedia of Gene and Genome (KEGG) pathway analysis for ECXB formula in the treatment of COPD targets were conducted by using the DAVID database (https://david.ncifcrf.gov/) (Huang et al., 2009b; Sherman et al., 2022). The results of the two analysis methods were mapped in the form of histograms and bubbles by using Bioinformatics online platform (http://www.bioinformatics.com.cn/) (Huang et al., 2009a).
2.7 Molecular docking verification
In the PDB database (https://www.rcsb.org/) (Berman, 2000), the crystal structure of the core target protein was selected and its “PDB” format file was downloaded. The core active ingredients in the “2D SDF” structure were downloaded from the PubChem database and imported into Chem3D 18.0 for their optimization. The PDB files of ligand molecules were imported into AutoDock Tools for processing, and saved as a PDBQT format file for later use. AutoDock 4.2 was used to dock the processed active ingredients with the core target protein (Morris et al., 2009). Using the minimum binding energy as the docking result of the target protein and ligand, the Pymol 2.5.2 software (https://pymol.org/2/) was used for observation and mapping.
2.8 Molecular dynamics simulation
GROMACS (2020.3) (Van Der Spoel et al., 2005) was used to analyze the MD simulation to check the stability of the protein-ligand complexes. The docking models of the complexes on the top two molecular docking results were used as the initial conformation for MD simulations. The root mean square deviation (RMSD) of each complex was analyzed to measure the stability of the complex system according to the degree of the molecular structure change. The root mean square fluctuation (RMSF) of the identified complexes was analyzed to understand the relative fluctuation of proteins. The receptor-ligand binding free energy was calculated using the Molecular Mechanics Poisson−Boltzmann Surface Area (MMPBSA) method in the 25 ns MD simulation trajectory.
3 Results
3.1 Analysis of “herb-symptom” network
According to the information related to symptoms treated by both TCM and modern medicine of diseases treated by herbs in the formula of ECXB collected on the SymMap platform, a total of 258 TCM symptoms were obtained, including 93 symptoms treated by Pinelliae rhizome, 54 by Citri reticulatae pericarpium, 84 by Poria, 116 by Glycyrrhizae radix et rhizome, 16 by Lycii cortex, and 16 by Mori cortex, as shown in Figure 2 (Supplementary Table S1). A total of 207 symptoms were treated by modern medicine, including 104 by Pinelliae rhizome, 37 by Citri reticulatae pericarpium, 81 by Poria, 33 by Glycyrrhizae radix et rhizome, 16 by Lycii cortex, and 21 by Mori cortex, as shown in Figure 3 (Supplementary Table S2). Symptoms targeted by TCM and modern medicine as well as COPD symptoms are shown in Table 1. It was found that the symptoms treated by both TCM and modern medicine of ECXB formula were the same as those of COPD patients with dyspnea, coughing up phlegm, chronic cough, and chest breathing, indicated that ECXB formula treatment for COPD is consistent with symptomatic treatment of TCM.
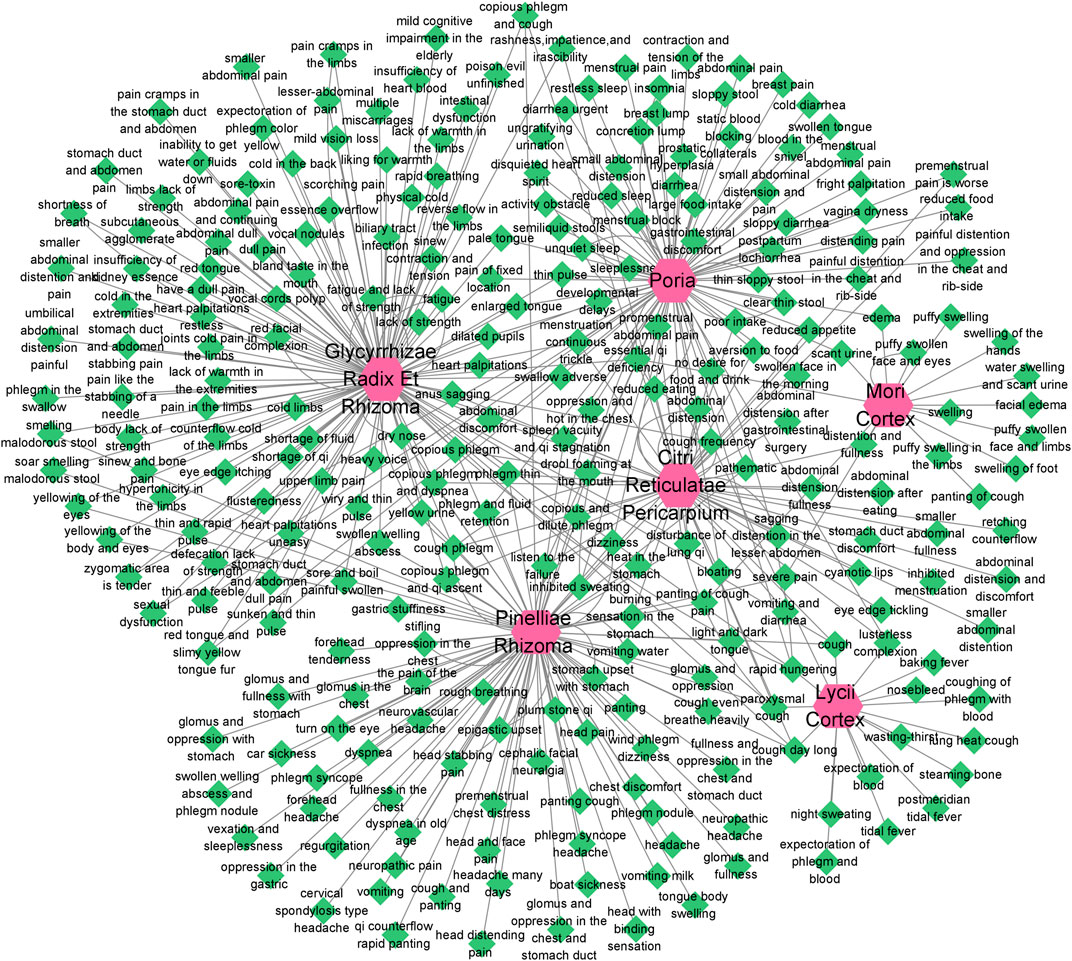
FIGURE 2. “Herb-TCM Symptom” network diagram. The pink node represents herbs, while green nodes show the TCM Symptoms.
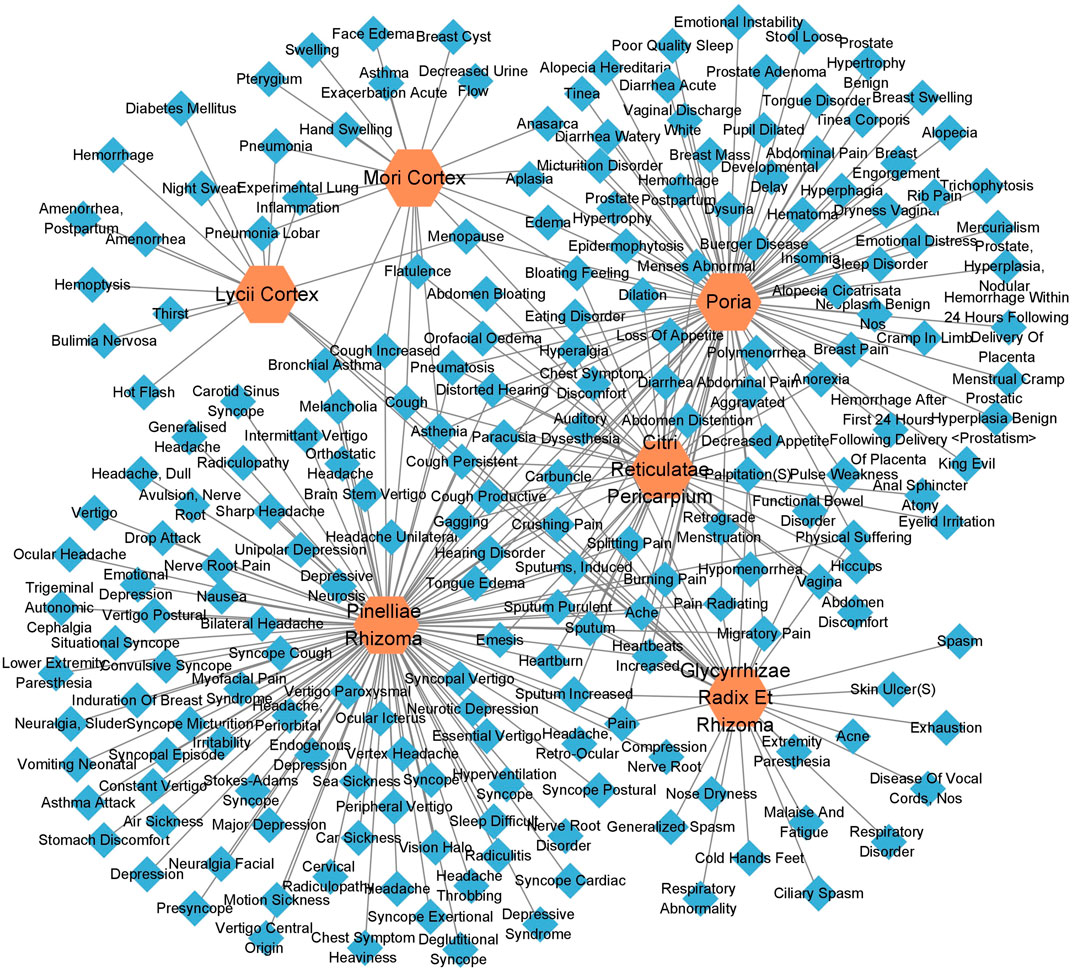
FIGURE 3. “Herb-modern medicine Symptom” network diagram. The orange node represents herbs, while the blue nodes show modern medicine Symptoms.
3.2 Acquisition of active ingredients and predicted targets
According to the screening procedure, a total of 1,314 active ingredients of ECXB formula were obtained from TCMSP, including 386 in Pinelliae rhizome, 147 in Citri reticulatae pericarpium, 69 in Poria, 75 in Glycyrrhizae radix et rhizome, 239 in Lycii cortex, and 398 in Mori cortex. Guiding by the ADME and DL standard (OB threshold ≥30% and DL threshold ≥0.18), 170 active ingredients were obtained after removing duplicated compounds. Among them, 13 were extracted from Pinelliae rhizome, 5 in Citri reticulatae pericarpium, 15 in Poria, 92 in Glycyrrhizae radix et rhizome, 13 in Lycii cortex, and 32 in Mori cortex (Supplementary Table S3). Partial results are listed in Table 2. 137 prediction targets of ECXB formula were obtained from the Swiss Target Prediction platform.
3.3 Acquisition of targets for COPD and prediction of intersection target
A total of 7002 COPD-related targets were collected from GeneCards® and OMIM ® databases (Supplementary Table S4). The predicted targets of ECXB formula were intersected with COPD-related targets, then 120 potential targets of ECXB formula for the treatment of COPD were obtained, and Venn diagrams were drawn in Figure 4 (Supplementary Table S5).
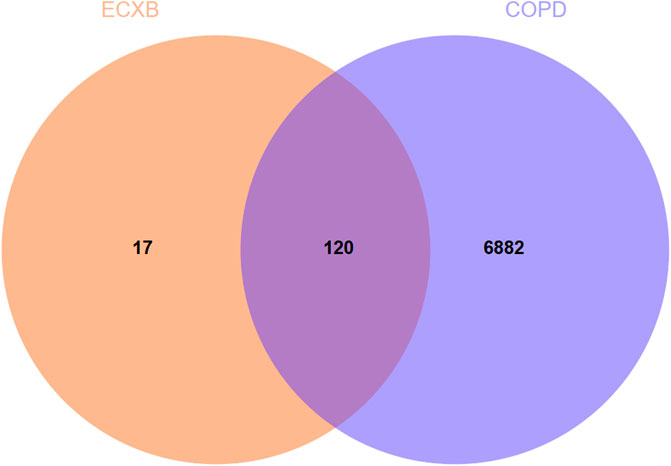
FIGURE 4. Venn diagram of ECXB formula combined with COPD. The Venn map showed that 120 targets were shared between ECXB formula and COPD.
3.4 Analysis of the “drug-ingredient-target” network and screening of core active ingredients
The 120 overlapping targets of ECXB formula and COPD as well as these targets corresponding ingredients were imported into Cytoscape software to construct a “Drug-ingredient-target” network. As shown in Figure 5, nodes in the network diagram represented drugs, active ingredients, and targets. The edges indicated that the nodes can interact with each other. The top 10 active ingredients of degree value were selected as the core ingredients, including kaempferol, hederagenin, naringenin, nobiletin, beta-sitosterol, stigmasterol, acacetin, OIN, atropine, and sugiolas (Table 3).
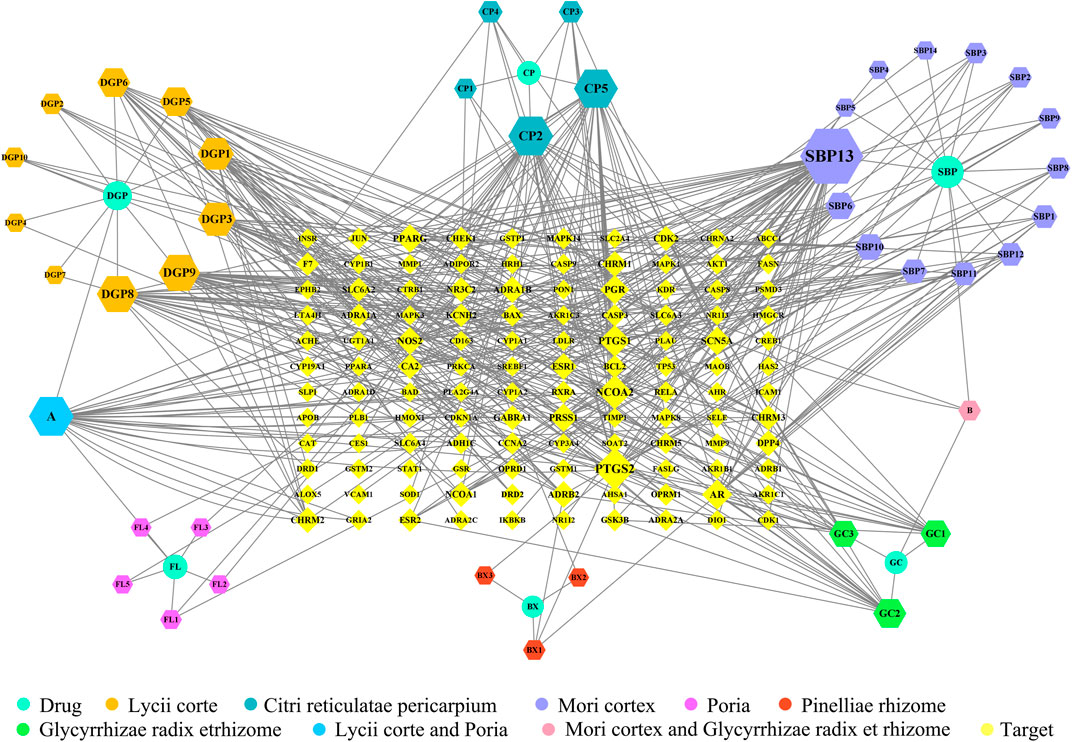
FIGURE 5. “Drug-ingredient-target” network diagram of ECXB formula in the treatment of COPD. The node size and node label size are determined by the node degree value. The higher the degree value, the larger will be the node and label.
3.5 Analysis of PPI network and screening of core targets
The 120 intersecting targets were imported into the STRING database, and the obtained information was imported into Cytoscape software to construct a PPI network between ECXB formula and the treatment of COPD, as shown in Figure 6. Using Cytoscape CytoNCA, under the condition that the values of DC, BC, CC, EC, LAC, and NC were all greater than their corresponding median values (Supplementary Tables S6–S8). Among them, 8 core targets were screened, including MAPK8, ESR1, TP53, MAPK3, JUN, RELA, MAPK1, and AKT1, as shown in Figure 7.
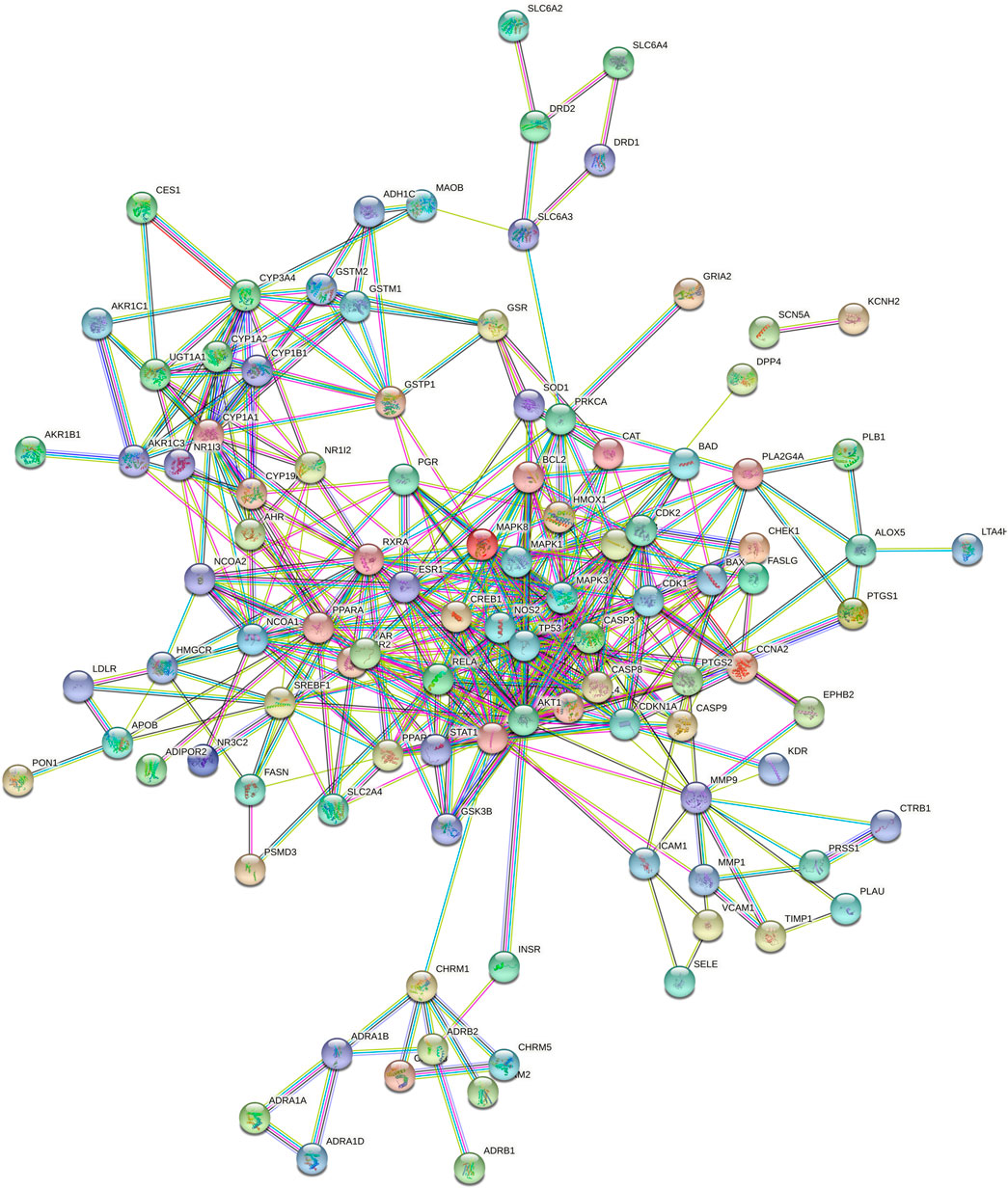
FIGURE 6. Protein interaction network diagram. 120-node and 424-edge PPI network of potential targets of the ECXB formula working on COPD was acquired at the String database.
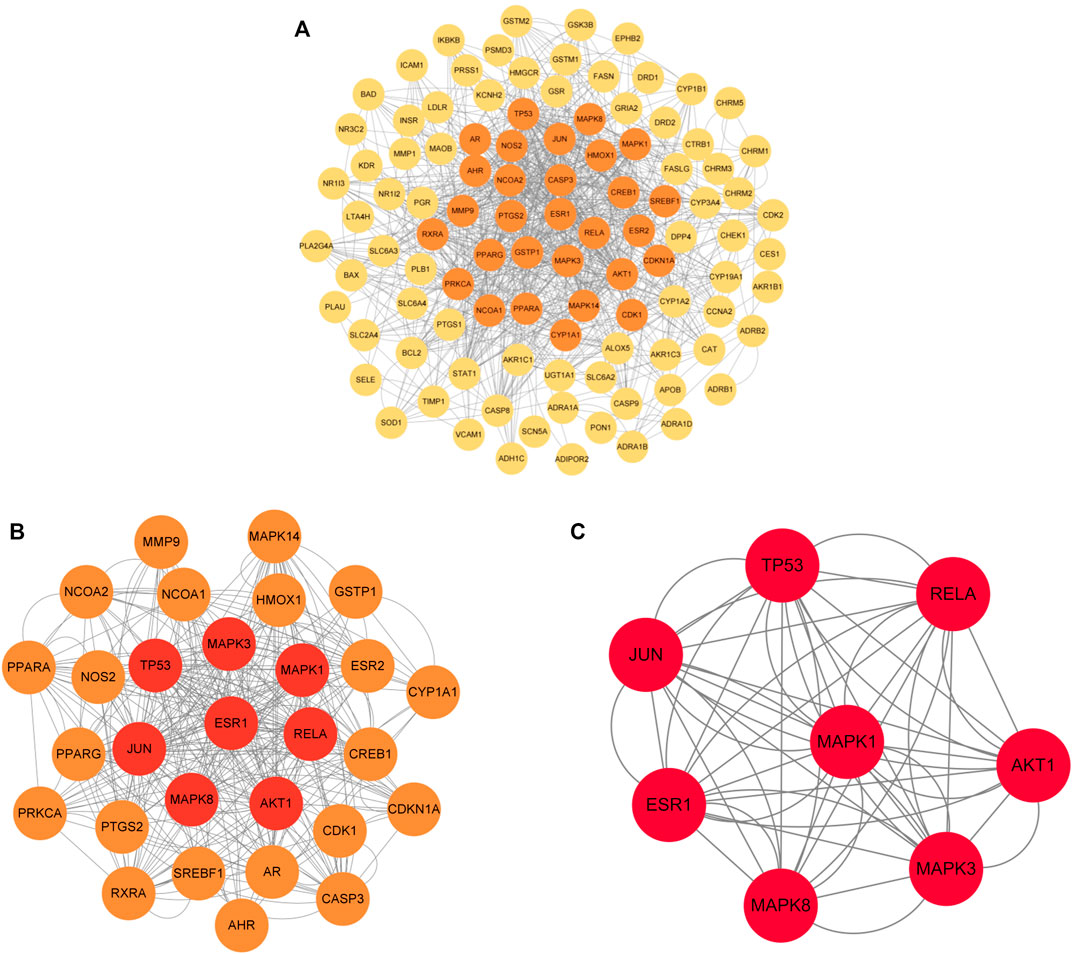
FIGURE 7. Core protein interaction network. (A) Interactive PPI network of ECXB formula-related targets and COPD-related targets. (B) PPI network of significant proteins extracted from (A). (C) PPI network of candidate COPD targets for ECXB formula treatment extracted from (B).
3.6 Analysis of GO and KEGG pathway
The intersecting targets of ECXB formula for the treatment of COPD were imported into the DAVID database to screen GO entries and KEGG signaling pathways of p < 0.01. GO functional enrichment analysis was performed under the conditions of Count ≥ 4, p ≤ 0.01, FDR (False Discovery Rate) ≤0.01, and a total of 563 results were obtained. There were 403 Biological Processes (BPs), 109 Molecular Functions (MFs), and 51 Cellular Components (CCs) (Supplementary Table S9). The top 10 results of count values were selected to draw a histogram, as shown in Figure 8.
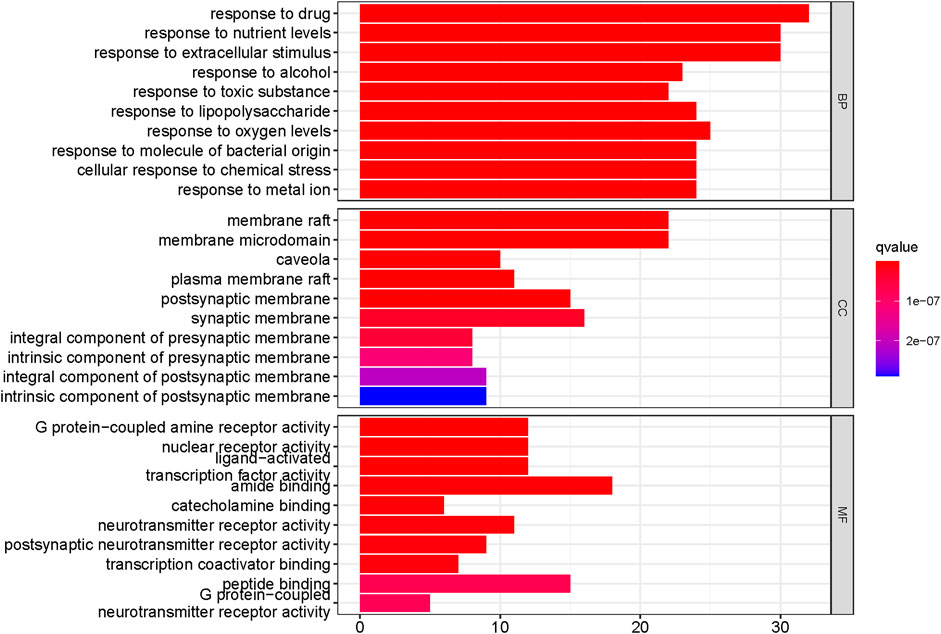
FIGURE 8. Functional enrichment analysis of the top 10 in GO. The Y-axis shows the enriched GO items of target genes. The X-axis shows the gene counts for the items. The color indicates the q value.
KEGG pathway analysis showed that the intersecting targets mapped 87 KEGG signaling pathways (Supplementary Table S10), and the top 20 pathways were selected to draw a bubble map, as shown in Figure 9. Detailed data are shown in Table 4. The targets and 20 pathways were imported into Cytoscape to construct a “target-pathway” network (Figure 10). Therefore, the network analysis suggested that ECXB formula may play a therapeutic role in COPD treatment by regulating signaling pathways, including the TNF signaling pathway, cAMP signaling pathway, VEGF signaling pathway, Hepatitis B Pathways, and Hepatitis C Pathways, suggesting that these pathways may mediate the treatment of COPD. Among them, the TNF signaling pathway was the KEGG pathway with a large number of gene enrichment and obvious significance. The visualization analysis of the TNF signaling pathway is shown in Figure 11.
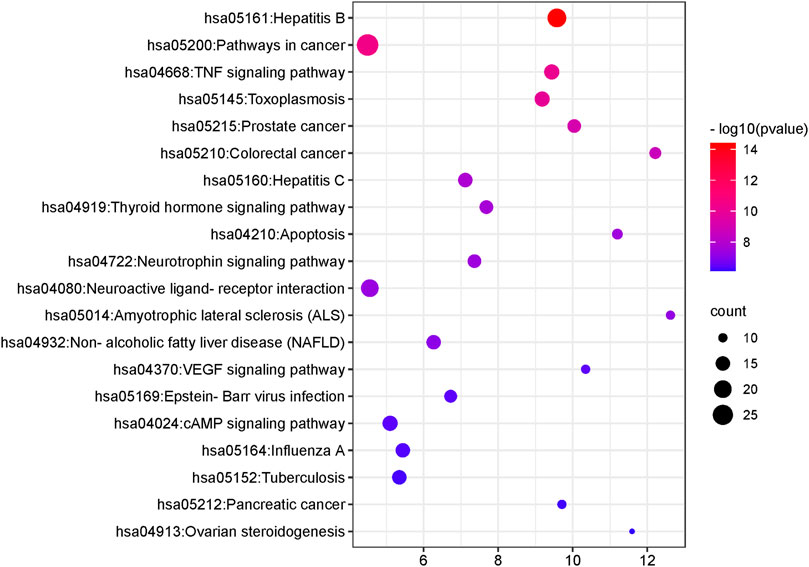
FIGURE 9. The first 20 KEGG pathways. The Y-axis shows the enriched KEGG pathways for target genes. The X-axis shows the gene counts for the items. The color indicates the p-value.
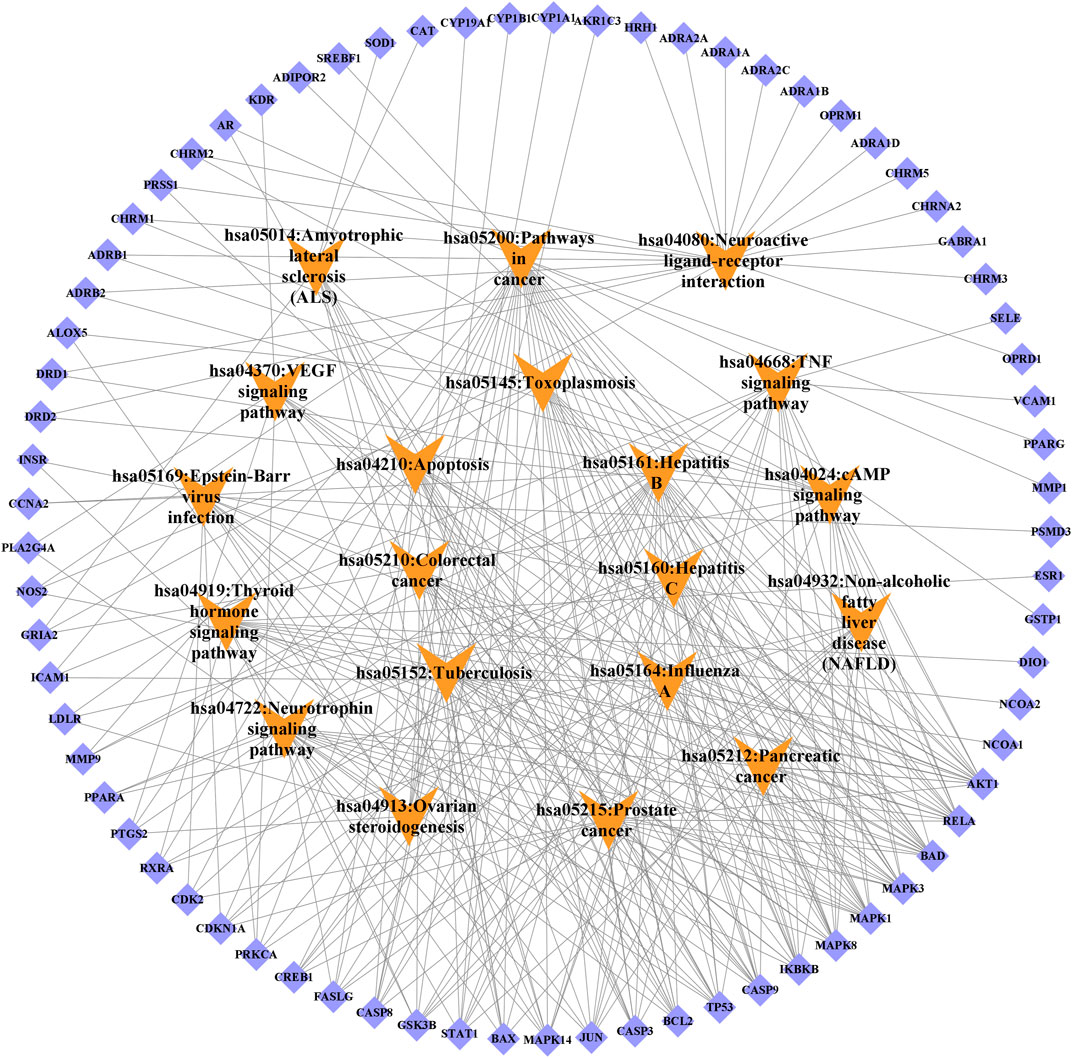
FIGURE 10. Target-signal pathway network diagram. The orange boxes indicate the signal pathways. The blue boxes indicate the gene names.
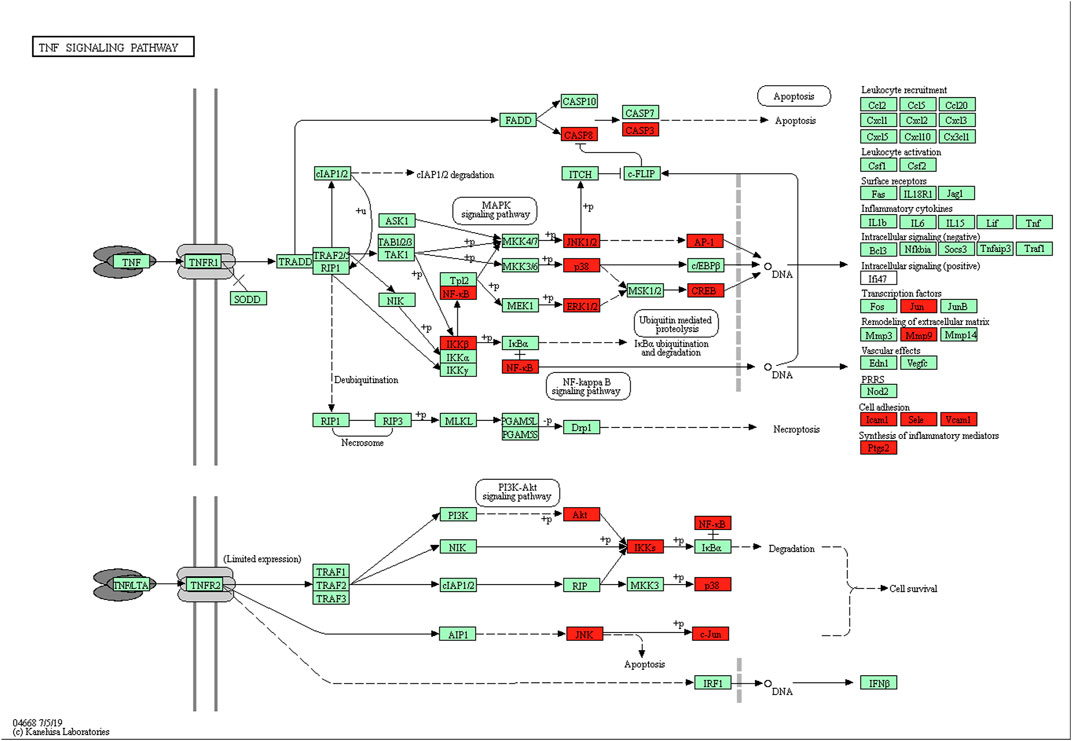
FIGURE 11. The TNF-α signaling pathway of potential target genes of ECXB formula in COPD. Arrows indicate upstream and downstream relationships between genes. The red blocks are ECXB formula target genes in the network.
3.7 Analysis of molecular docking
The selected top ten active ingredients included hederagenin (MOL000296), beta-sitosterol (MOL000358), kaempferol (MOL000422), Stigmasterol (MOL000449), OIN (MOL001552), acacetin (MOL001689), Atropine (MOL002219), surgical (MOL002222), naringenin (MOL004328), and nobiletin (MOL005828). The protein structures of core targets were acquired online from RCSB PDB, including MAPK8 (PDB ID: 2XRW), TP53 (PDB ID: 6GGC), MAPK3 (PDB ID: 4QTB), MAPK1 (PDB ID: 4ZZN), AKT1 (PDB ID: 1unq), ESR1 (PDB ID: 7baa), JUN (PDB ID: 6y3v), and RELA (PDB ID: 6nv2). The molecular docking for the top ten active ingredients and the protein structures of core targets were conducted by using AutoDock 4.2. The results of molecular docking indicate that the lower the binding energy, the more stable the active ingredients and targets are (Supplementary Table S11). Processed by PyMOL software, the optimal docking image of the active ingredients and the targets was displayed. It can be seen from Figure 12 that the best affinity modes were RELA-beta-sitosterol and ESR1-stigmasterol. Table 5.
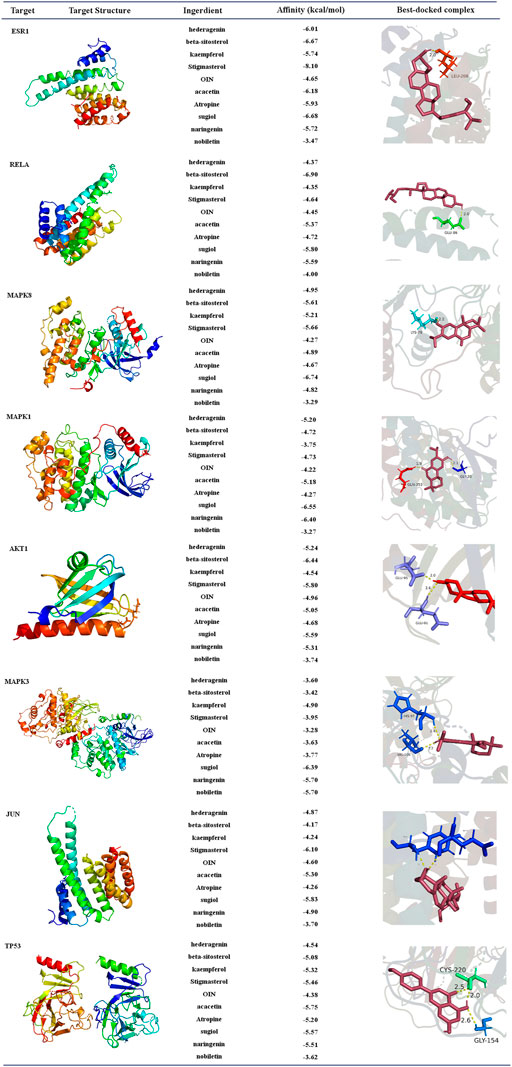
FIGURE 12. Diagram of molecular docking results. Proteins are shown to interact with molecules. The docking scores are shown in the middle. The binding amino acidic sites and other details are shown on the right.
3.8 Results of molecular dynamics simulation
We selected the top two ingredient-target dockings (RELA-beta-sitosterol and ESR1-stigmasterol) to conduct molecular dynamics simulations. After 100 ns of MD simulations, the dynamic evolutions of the RELA-beta-sitosterol and ESR1-stigmasterol complexes could be analyzed. The RMSD curve represents positional deviations in the protein. As can be seen from Figures 13A, B, the RELA-beta-sitosterol complex was in an equilibrium state and the average RMSD value was 0.34448675 during 80–100 ns. Meanwhile, the ESR1-stigmasterol complex had a rise within 90 ns and tended to balance out the last 10 ns with the average RMSD value was 0.35742059. The low fluctuation of the two complexes indicated that their stability was stronger. The RMSF curve represents the fluctuation of the protein amino acid residues. The fluctuations of the two complexes in the 1,108–1,203 regions were relatively high. Inversely, most of the residues fluctuated at lower values in other regions, suggesting that the binding residues of RELA-beta-sitosterol and ESR1-stigmasterol were stable (Figures 13C, D). In addition, the binding energy of RELA-beta-sitosterol was −129.870 kcal/mol, and the ESR1-stigmasterol complex was −121.686 kcal/mol (Table 4).
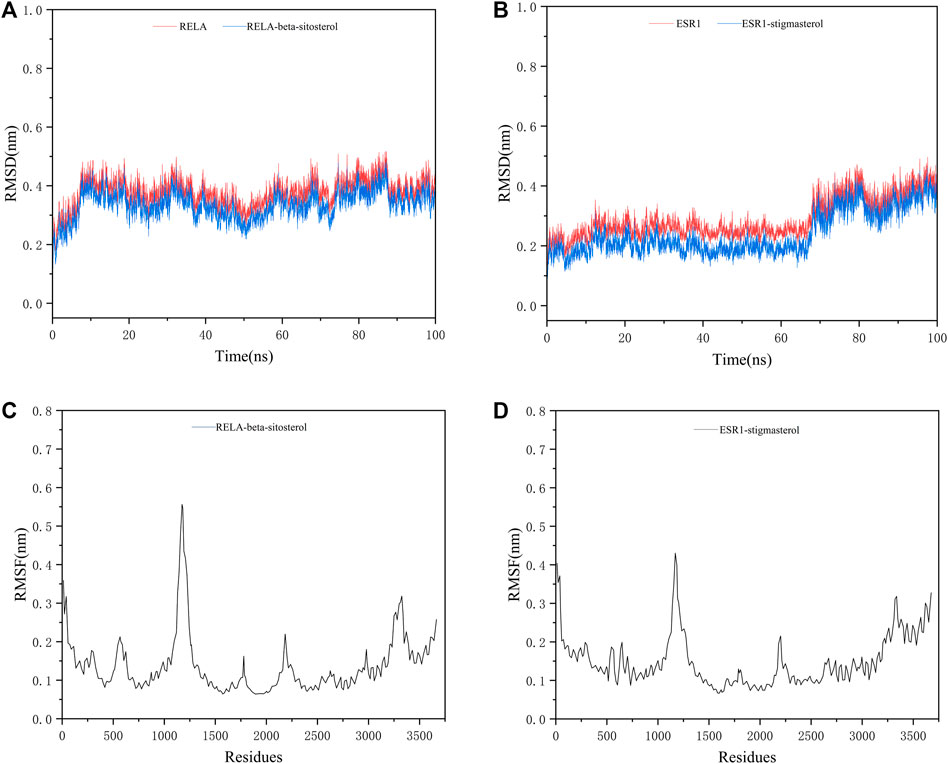
FIGURE 13. The MD simulation results of two complexes. (A) The RMSD analysis for the RELA-beta-sitosterol complex. (B) The RMSD analysis for the ESR1-stigmasterol complex. (C) The RMSF analysis for the RELA-beta-sitosterol complex. (D) The RMSF analysis for the ESR1-stigmasterol complex.
4 Discussion
COPD is a chronic inflammatory airway disease that can be prevented and treated. In recent 10 years, the prevalence of COPD in Chinese over 40 years old has increased from 8.2% to 13.7%, and the risk of hospitalization and mortality has also increased (Zhang et al., 2019; Zhang et al., 2019). Notably, TCM treatment can relieve the symptoms of COPD patients and improve their survival rate. As classical formulas of TCM in COPD treatment, ECXB formula has respectively obtained some achievements in clinical efficacy and action of mechanism. However, the study of the combination of the two formulas for COPD is still in the initial stage. In this study, we used the SymMap platform to collect related symptoms treated by both TCM and modern medicine of herbs in the formula of ECXB to conduct Herb-Symptom comparative analysis, and applied the network pharmacology, molecular docking, and MD simulation to explore the potential mechanisms of ECXB formula on COPD in order to provide theoretical basis for the following validation studies.
Firstly, we comparative analysis Herb-Symptom network symptoms targeted by TCM and modern medicine as well as COPD symptoms, and found that the symptoms treated by both TCM and modern medicine of herbs in the formula of ECXB were similar to the symptoms of COPD patients, indicating that ECXB formula treatment of COPD conforms to the symptomatic treatment principle of TCM. Then we analyzed the “Drug-ingredient-target” network, hederagenin and naringenin (the main ingredients of Citri reticulatae pericarpium and Poria in Erchen Decoction), and kaempferol and hederagenin (the main ingredients of Mori cortex and Lycii cortex in Xiebai Powder), are the core active ingredients of ECXB formula for COPD treatment. Hederagenin is a pentacyclic triterpenoid compound isolated from plants, which has been shown to have strong antibacterial, anti-inflammatory, and anti-tumor biological activities (Ndjateu et al., 2014; Fang et al., 2018). Notably, it can reduce the level of proinflammatory cytokines in serum and lung tissue and reduce the damage of oxidative stress response to the lung (Wang and Zhao, 2022). Naringenin has anti-inflammatory, scavenging free radicals, inhibiting peroxidation, lowering blood lipids, and inhibiting tumor growth (Annadurai et al., 2013; Alam et al., 2014), and it is thought to be beneficial in COPD due to its anti-inflammatory properties (Chin et al., 2020). Liu et al. (2018) study indicated that naringenin attenuates inflammation in COPD in cigarette smoke induced mouse model and involves suppression of NF-κB, and they concluded that it can be a potential therapeutic agent for the treatment of COPD-related inflammation. Kaempferol has antioxidant stress activity and anti-inflammatory activity, which significantly inhibits the expression of the MAPK pathway, reduces the production of various inflammatory factors, and effectively inhibits inflammation (Park et al., 2006; Alam et al., 2014). Tsou et al. (2014) study found that kaempferol was found to be IKK2 inhibitor and helped prevent COPD occurrence and worsening. In summary, it can be inferred that the core ingredients of ECXB formula play a therapeutic role by affecting inflammatory factors related to COPD.
PPI network analysis results suggest that the core targets of ECXB formula for the treatment of COPD mainly include MAPK8, ESR1, TP53, MAPK3, JUN, RELA, MAPK1, and AKT1. Among them, MAPK8, MAPK3, and MAPK1 are intracellular serine/threonine protein kinases, which can change gene expression by phosphorylation of transcription factors, promote the production of inflammatory factors TNF-α, IL-1, IL-6, and induce a series of inflammatory and immune responses, thus playing a role in the pathogenesis of COPD (Kang et al., 2013; Duan et al., 2020). TP53 is a tumor suppressor that plays a variety of roles in controlling cell cycle checkpoints, apoptosis, and DNA repair. In COPD smokers, downregulation of TP53 - and p53-related signal transduction may lead to lung tumors (Zhou et al., 2021). ESR1 gene can code estrogen receptors, and studies have found that estrogen signaling may play a key role in lung diseases. Overexpression of ESR1 m RNA is closely related to the prognosis of non-small cell lung cancer (Suga et al., 2008), while ESR1 is expressed in lung tissues of patients with COPD, but its specific mechanism remains unclear. JUN is the most widely used protein in activating protein 1 complex, (Bin et al. (2020) discovered that c-Jun is a potential novel therapeutic target for COPD, JNK inhibition by erythromycin restores corticosteroid sensitivity via the inhibition of c-Jun expression. AKT1 is an important factor in the PI3K-AKT signaling pathway, which plays a key role in many physiological processes such as cell growth and survival and is associated with airway inflammation and lung function changes in asthma (gianni, 2019). In conclusion, the core targets may influence the onset and progression of COPD through inflammatory immunity, reducing inflammatory factors and oxidation-reduction reaction, and so on.
We also performed GO function and KEGG pathway enrichment analyses. Response to drug, response to nutrient levels, and response to extracellular stimulation may be the key biological processes in the ECXB formula treatment of COPD (Bidan et al., 2015; Beijers et al., 2022). Membrane raft and amide binding were the most significant cellular component and molecular functions, respectively. It has been reported that preponderance of saturated fatty acids in plasma membrane of erythrocytes of COPD patients which may decrease the membrane fluidity and possibly impair the functions of the plasma membrane in the disease (Gangopadhyay et al., 2012). According to the KEGG analysis, the TNF signaling pathway, cAMP signaling pathway, and VEGF signaling pathway were considered key pathways in the treatment of COPD by ECXB formula. The TNF pathway is critical in inflammatory response by regulating both apoptosis and proliferation. Lee et al. (2018) display that fisetin is a good therapeutic drug for the treatment of inflammatory lung diseases, such as COPD, by inhibiting the TNF-alpha/NF-kappa B signaling pathway. Arora et al. (2022b) discovered that a significant reduction in TNF-α levels and concomitant suppression of asthma symptoms after oral administration of solasodine in ovalbumin-sensitized rats are indicative of the possible therapeutic role of phytocompound in chronic asthma irresponsive to ICS. Liu et al. (2015) study results suggested that IL-15 inhibited protein degradation in skeletal muscle in COPD rats, which may be mediated by the TNF-alpha and UPP pathway. Arora et al. (2021) and Arora et al. (2022a) studies demonstrate that biomarkers of the inflammatory response, including cell count, immunoglobulin E, cytokines such as interleukin (IL-4), −5, −1β, tumor necrosis factor (TNF)-α, etc. The findings that Mesua ferrea L. and Clerodendrum serratum in allergic asthma may be related to the ability of the plant to attenuate inflammatory cell responses and thus to the production of inflammatory and pro-inflammatory cytokines in the airways. The cAMP pathway plays a major role in COPD signaling. For instance, Dunne et al. (2019) study showed that roflumilast inhibits neutrophil chemotaxis directly via a cAMP-mediated mechanism requiring activation of Epac1 and that Epac1 activators could reduce COPD neutrophilic inflammation. Nabissi et al. (2018) work demonstrated that thyme extract is effective in stimulating CBF by inducing an increase of cAMP and Ca2+ levels, thus supporting its therapeutical use in the treatment of COPD. The VEGF signaling pathway has been related to the pathogenesis of COPD. Zhang et al. (2022) the efficacy of electroacupuncture (EA) with respect to the regulation of microvascular remodeling induced by VEGF/PI3K/Akt was evaluated in a rat model of COPD, showed that reduced pulmonary vascular remodeling via mechanisms possibly related to the VEGF/PI3K/Akt pathway. Willems-Widyastuti et al. (2011) study suggested that TGF-beta (1) could play a key role in bronchial angiogenesis and vascular remodeling via VEGF pathway in asthma. The active ingredients of ECXB formula may act on these signaling pathways in the treatment of COPD.
The network pharmacology results were verified for ECXB formula in the treatment of COPD by the molecular docking of the 8 core targets and the top ten selected active ingredients. The results of the molecular docking indicated that the core active ingredients of ECXB formula in the treatment of COPD could bind well with their core targets. Among them, RELA-beta-sitosterol and ESR1-stigmasterol had the lowest binding scores. MD simulation was employed to further verify the results obtained. The results of molecular dynamics simulation suggested that RELA-beta-sitosterol and ESR1-stigmasterol could bind tightly, and the binding energies of the complexes were −129.870 kcal/mol, and −121.686 kcal/mol, respectively.
However, it should be noted that there were certain limitations of the network pharmacology. The study based on network pharmacology is a static network analysis while the occurrence and development of disease and the action of drugs are both dynamic processes. Therefore, the following experiments in vivo or in vitro can be carried out on this basis to explore the deeper mechanism of ECXB formula in the treatment of COPD. Despite the limitations of this study, the results revealed that it had certain clinical value and research significance.
5 Conclusion
Altogether, we analyzed the role of ECXB formula in treating COPD from the Herb-Symptom network relationship and utilized network pharmacology, molecular docking, and MD simulation to explore the potential mechanisms of ECXB formula in treating COPD. The results indicated that hederagenin, naringenin, kaempferol, and other effective ingredients of ECXB formula showed therapeutic effects against COPD via multiple targets and multiple pathways. In addition, the good activities of the core active ingredients and the core targets were verified by molecular docking and MD simulation. In summary, the results of this study provided a reference for clinical application and guidance for further experimental verification of ECXB formula for the treatment of COPD.
Data availability statement
The original contributions presented in the study are included in the article/Supplementary Material, further inquiries can be directed to the corresponding authors.
Author contributions
HY: conception and design; designed the research methodology; writing-review and editing. BH: data analysis and interpretation; writing-original draft. YZ: validation; writing-review and editing. ZY: validation; writing-review and editing. YF: data analysis and interpretation collected and sorted data. CW: administrative support. CX: data analysis and editing. QF: administrative support. All author listed have made a substantial, direct, and intellectual contribution to the work and approved it for publication.
Funding
This research was supported by the National Key R&D Program of China (2018YFC1704104); the National Natural Science Foundation of China (82105054); the Natural Science Foundation of Sichuan Province (2023NSFSC1811); the “Xinglin Scholar” Scientific Research Promotion Plan of Chengdu University of TCM (CCZD2021002); the TCM Scientific Research Special project of Sichuan TCM Administration (2021MS559).
Acknowledgments
We sincerely appreciate the databases of SymMap, TCMSP, PubChem, SwissTargetPrediction, Uniport, GeneCards®, OMIM®, STRING, DAVID, Bioinformatics, PDB and Cytoscape, Chem3D, AutoDock 4, PyMol, GROMACS software for provided the data and making the charts. The contents of the manuscript preprint of the first (20 May 2022) and second (03 June 2022) editions are available online (https://www.researchsquare.com/article/rs-1646303/v1).
Conflict of interest
The authors declare that the research was conducted in the absence of any commercial or financial relationships that could be construed as a potential conflict of interest.
Publisher’s note
All claims expressed in this article are solely those of the authors and do not necessarily represent those of their affiliated organizations, or those of the publisher, the editors and the reviewers. Any product that may be evaluated in this article, or claim that may be made by its manufacturer, is not guaranteed or endorsed by the publisher.
Supplementary material
The Supplementary Material for this article can be found online at: https://www.frontiersin.org/articles/10.3389/fphar.2023.1117238/full#supplementary-material
References
Alam, M. A., Subhan, N., Rahman, M. M., Uddin, S. J., Reza, H. M., and Sarker, S. D. (2014). Effect of citrus flavonoids, naringin and naringenin, on metabolic syndrome and their mechanisms of action. Adv. Nutr. 5, 404–417. doi:10.3945/an.113.005603
Amberger, J. S., Bocchini, C. A., Schiettecatte, F., Scott, A. F., and Hamosh, A. (2015). OMIM.org: Online Mendelian Inheritance in Man (OMIM®), an online catalog of human genes and genetic disorders. Nucleic Acids Res. 43, D789–D798. doi:10.1093/nar/gku1205
Annadurai, T., Thomas, P. A., and Geraldine, P. (2013). Ameliorative effect of naringenin on hyperglycemia-mediated inflammation in hepatic and pancreatic tissues of Wistar rats with streptozotocin-nicotinamide-induced experimental diabetes mellitus. Free Radic. Res. 47, 793–803. doi:10.3109/10715762.2013.823643
Arora, P., Ansari, S. H., and Nainwal, L. M. (2022a). Clerodendrum serratum extract attenuates production of inflammatory mediators in ovalbumin-induced asthma in rats. Turk. J. Chem. 46, 330–341. doi:10.55730/1300-0527.3310
Arora, P., Ansari, S. H., and Nainwal, L. M. (2021). Mesua ferrea L. (Calophyllaceae) exerts therapeutic effects in allergic asthma by modulating cytokines production in asthmatic rats. Turk. J. Bot. 45, 820–832. doi:10.3906/bot-2111-22
Arora, P., Nainwal, L. M., Gupta, G., Singh, S. K., Chellappan, D. K., Oliver, B. G., et al. (2022b). Orally administered solasodine, a steroidal glycoalkaloid, suppresses ovalbumin-induced exaggerated Th2-immune response in rat model of bronchial asthma. Chem. Biol. Interact. 366, 110138. doi:10.1016/j.cbi.2022.110138
Bateman, A., Martin, M. J., Orchard, S., Magrane, M., Agivetova, R., Ahmad, S., et al. (2021). UniProt: The universal protein knowledgebase in 2021. Nucleic Acids Res. 49, D480–D489. doi:10.1093/nar/gkaa1100
Beijers, R. J., Iersel, L. E. J. van, Schuurman, L. T., Hageman, R. J. J., Simons, S. O., Helvoort, A. V., et al. (2022). Effect of targeted nutrient supplementation on physical activity and health-related quality of life in COPD: Study protocol for the randomised controlled NUTRECOVER trial. BMJ Open 12, e059252. doi:10.1136/bmjopen-2021-059252
Berman, H. M., Westbrook, J., Feng, Z., Gilliland, G., Bhat, T. N., Weissig, H., et al. (2000). The protein data bank. Nucleic Acids Res. 28, 235–242. doi:10.1093/nar/28.1.235
Bidan, C. M., Veldsink, A. C., Meurs, H., and Gosens, R. (2015). Airway and extracellular matrix Mechanics in COPD. Front. Physiol. 6, 346. doi:10.3389/fphys.2015.00346
Bin, Y. F., Ma, N., Lu, Y. X., Sun, X. J., Liang, Y., Bai, J., et al. (2020). Erythromycin reverses cigarette smoke extract-induced corticosteroid insensitivity by inhibition of the JNK/c-Jun pathway. Free Radic. Biol. Med. 152, 494–503. doi:10.1016/j.freeradbiomed.2019.11.020
Blanco, I., Diego, I., Bueno, P., Fernandez, E., Casas-Maldonado, F., Esquinas, C., et al. (2018). Geographical distribution of COPD prevalence in Europe, estimated by an inverse distance weighting interpolation technique. Int. J. Chronic Obstr. Pulm. Dis. 13, 57–67. doi:10.2147/COPD.S150853
Buist, A. S., McBurnie, M. A., Vollmer, W. M., Gillespie, S., Burney, P., Mannino, D. M., et al. (2007). International variation in the prevalence of COPD (the BOLD study): A population-based prevalence study. Lancet 370, 741–750. doi:10.1016/S0140-6736(07)61377-4
Chin, L. H., Hon, C. M., Chellappan, D. K., Chellian, J., Madheswaran, T., Zeeshan, F., et al. (2020). Molecular mechanisms of action of naringenin in chronic airway diseases. Eur. J. Pharmacol. 879, 173139. doi:10.1016/j.ejphar.2020.173139
Daina, A., Michielin, O., and Zoete, V. (2019). Swiss target prediction: Updated data and new features for efficient prediction of protein targets of small molecules. Nucleic Acids Res. 47, W357–W364. doi:10.1093/nar/gkz382
Deng, L., Zhang, X., Dong, Y., Wang, L., Chen, K., Zheng, M., et al. (2020). Erchen decoction combined with Sanziyangqin decoction for chronic obstructive pulmonary disease A protocol for systematic review and meta-analysis. Med. Baltim. 99, e22315. doi:10.1097/MD.0000000000022315
Duan, R., Niu, H., Yu, T., Cui, H., Yang, T., Hao, K., et al. (2020). Identification and bioinformatic analysis of circular RNA expression in peripheral blood mononuclear cells from patients with chronic obstructive pulmonary disease. Int. J. Chronic Obstr. Pulm. Dis. 15, 1391–1401. doi:10.2147/COPD.S252896
Dunne, A. E., Kawamatawong, T., Fenwick, P. S., Davies, C. M., Tullett, H., Barnes, P. J., et al. (2019). Direct inhibitory effect of the PDE4 inhibitor roflumilast on neutrophil migration in chronic obstructive pulmonary disease. Am. J. Respir. Cell. Mol. Biol. 60, 445–453. doi:10.1165/rcmb.2018-0065OC
Fang, K., Zhang, X. H., Han, Y. T., Wu, G. R., Cai, D. S., Xue, N. N., et al. (2018). Design, synthesis, and cytotoxic analysis of novel hederagenin-pyrazine derivatives based on partial least squares discriminant analysis. Int. J. Mol. Sci. 19, 2994. doi:10.3390/ijms19102994
Gangopadhyay, S., Vijayan, V. K., and Bansal, S. K. (2012). Lipids of erythrocyte membranes of COPD patients: A quantitative and qualitative study. COPD J. Chronic Obstr. Pulm. Dis. 9, 322–331. doi:10.3109/15412555.2012.668581
Huang, D. W., Sherman, B. T., and Lempicki, R. A. (2009a). Bioinformatics enrichment tools: Paths toward the comprehensive functional analysis of large gene lists. Nucleic Acids Res. 37, 1–13. doi:10.1093/nar/gkn923
Huang, D. W., Sherman, B. T., and Lempicki, R. A. (2009b). Systematic and integrative analysis of large gene lists using DAVID bioinformatics resources. Nat. Protoc. 4, 44–57. doi:10.1038/nprot.2008.211
Huang, P., Lin, X., Liu, Y., and Hou, Z. (2022). The efficacy and safety of combined traditional Chinese and Western medicine in the treatment of chronic obstructive pulmonary disease complicated with respiratory failure: A systematic review and meta-analysis study. Ann. Pallliat. Med. 11, 1102–1111. doi:10.21037/apm-22-272
Kang, Y., Wang, F., Lu, Z., Ying, H., Zhang, H., Ding, W., et al. (2013). MAPK kinase 3 potentiates Chlamydia HSP60-induced inflammatory response through distinct activation of NF-kappa B. J. Immunol. 191, 386–394. doi:10.4049/jimmunol.1300481
Kim, S., Chen, J., Cheng, T., Gindulyte, A., He, J., He, S., et al. (2021). PubChem in 2021: New data content and improved web interfaces. Nucleic Acids Res. 49, D1388–D1395. doi:10.1093/nar/gkaa971
Lee, S., Ro, H., Jin, H., Choi, J. H., Kim, M. O., Lee, J., et al. (2018). Fisetin inhibits TNF-α/NF-κB-induced IL-8 expression by targeting PKCδ in human airway epithelial cells. Cytokine 108, 247–254. doi:10.1016/j.cyto.2018.01.004
Li, J. (2020). International clinical Practice guideline of Chinese medicine chronic obstructive pulmonary disease. World Chin. Med. 15, 39–1092. doi:10.4103/wjtcm.wjtcm_9_20
Li, X., and Luo, X. (2014). Clinical application of qingfei huatan decoction from doctor WANG's. China. J. TCM. 29, 3469–3471.
Liu, J., Yao, J., and Zhang, J. (2018). Naringenin attenuates inflammation in chronic obstructive pulmonary disease in cigarette smoke induced mouse model and involves suppression of NF-κB. J. Microbiol. Biotechnol. doi:10.4014/jmb.1810.10061
Liu, Z., Fan, W., Chen, J., Liang, Z., and Guan, L. (2015). The role of Interleukin 15 in protein degradation in skeletal muscles in rats of chronic obstructive pulmonary disease. Int. J. Clin. Exp. Med. 8, 1976–1984.
Mei, W., and Li, X. (2012). Observation on the curative effect of Xeibai Power combined with Erchen Decoction on acute exacerbation of chronic obstructive pulmonary disease. Guide. China Med. 10, 277–278. doi:10.15912/j.cnki.gocm.2012.30.272
Morris, G. M., Huey, R., Lindstrom, W., Sanner, M. F., Belew, R. K., Goodsell, D. S., et al. (2009). AutoDock4 and AutoDockTools4: Automated docking with selective receptor flexibility. J. Comput. Chem. 30, 2785–2791. doi:10.1002/jcc.21256
Nabissi, M., Marinelli, O., Morelli, M. B., Nicotra, G., Iannarelli, R., Amantini, C., et al. (2018). Thyme extract increases mucociliary-beating frequency in primary cell lines from chronic obstructive pulmonary disease patients. Biomed. Pharmacother. 105, 1248–1253. doi:10.1016/j.biopha.2018.06.004
Ndjateu, F. S. T., Tsafack, R. B. N., Nganou, B. K., Awouafack, M. D., Wabo, H. K., Tene, M., et al. (2014). Antimicrobial and antioxidant activities of extracts and ten compounds from three Cameroonian medicinal plants: Dissotis perkinsiae (Melastomaceae), Adenocarpus mannii (Fabaceae) and Barteria fistulosa (Passifloraceae). S. Afr. J. Bot. 91, 37–42. doi:10.1016/j.sajb.2013.11.009
Park, J. S., Rho, H. S., Kim, D. H., Chang, I. S., et al. (2006). Enzymatic preparation of kaempferol from green tea seed and its antioxidant activity. J. Agric. Food Chem. 54, 2951–2956. doi:10.1021/jf052900a
Rehman, A. U., Hassali, M. A. A., Muhammad, S. A., Shah, S., Abbas, S., Ali, I. A. B. H., et al. (2020). The economic burden of chronic obstructive pulmonary disease (COPD) in the USA, Europe, and Asia: Results from a systematic review of the literature. Expert Rev. pharmacoecon. Outcomes Res. 20, 661–672. doi:10.1080/14737167.2020.1678385
Ru, J., Li, P., Wang, J., Zhou, W., Li, B., Huang, C., et al. (2014). Tcmsp: A database of systems pharmacology for drug discovery from herbal medicines. J. Cheminformatics 6, 13. doi:10.1186/1758-2946-6-13
Safran, M., Rosen, N., Twik, M., BarShir, R., Stein, T. I., Dahary, D., et al. (2021). “The GeneCards suite,” in Practical guide to life science databases. Editors I. Abugessaisa, and T. Kasukawa (Singapore: Springer), 27–56. doi:10.1007/978-981-16-5812-9_2
Shannon, P., Markiel, A., Ozier, O., Baliga, N. S., Wang, J. T., Ramage, D., et al. (2003). Cytoscape: A software environment for integrated models of biomolecular interaction networks. Genome Res. 13, 2498–2504. doi:10.1101/gr.1239303
Sherman, B. T., Hao, M., Qiu, J., Jiao, X., Baseler, M. W., Lane, H. C., et al. (2022). David: A web server for functional enrichment analysis and functional annotation of gene lists (2021 update). Nucleic Acids Res. 50, W216–W221. doi:10.1093/nar/gkac194
Singh, D., Agusti, A., Anzueto, A., Barnes, P. J., Bourbeau, J., Celli, B. R., et al. (2019). Global Strategy for the Diagnosis, management, and prevention of chronic obstructive lung disease: The GOLD science committee report 2019. Eur. Resp. J. 53, 1900164. doi:10.1183/13993003.00164-2019
Suga, Y., Miyajima, K., Oikawa, T., Maeda, J., Usuda, J., Kajiwara, N., et al. (2008). Quantitative p16 and ESR1 methylation in the peripheral blood of patients with non-small cell lung cancer. Oncol. Rep. 20, 1137–1142.
Szklarczyk, D., Gable, A. L., Lyon, D., Junge, A., Wyder, S., Huerta-Cepas, J., et al. (2019). STRING v11: Protein-protein association networks with increased coverage, supporting functional discovery in genome-wide experimental datasets. Nucleic Acids Res. 47, D607–D613. doi:10.1093/nar/gky1131
Tao, W., Xu, X., Wang, X., Li, B., Wang, Y., Li, Y., et al. (2013). Network pharmacology-based prediction of the active ingredients and potential targets of Chinese herbal Radix Curcumae formula for application to cardiovascular disease. J. Ethnopharmacol. 145, 1–10. doi:10.1016/j.jep.2012.09.051
Tsou, Y. A., Huang, H. J., Lin, W. W. Y., and Chen, C. Y. C. (2014). Lead screening for chronic obstructive pulmonary disease of IKK2 inhibited by traditional Chinese medicine. Evid. based Complement. Altern. Med. 2014, 465025. doi:10.1155/2014/465025
Van Der Spoel, D., Lindahl, E., Hess, B., Groenhof, G., Mark, A. E., and Berendsen, H. J. C. (2005). Gromacs: Fast, flexible, and free. J. Comput. Chem. 26, 1701–1718. doi:10.1002/jcc.20291
Wang, L., and Zhao, M. (2022). Suppression of NOD-like receptor protein 3 inflammasome activation and macrophage M1 polarization by hederagenin contributes to attenuation of sepsis-induced acute lung injury in rats. Bioengineered 13, 7262–7276. doi:10.1080/21655979.2022.2047406
Willems-Widyastuti, A., Alagappan, V. K. T., Arulmani, U., Vanaudenaerde, B. M., de Boer, W. I., Mooi, W. J., et al. (2011). Transforming growth factor-beta 1 induces angiogenesis in vitro via VEGF production in human airway smooth muscle cells. Indian J. biochem. Biophys. 48, 262–269.
Wu, Y., Zhang, F., Yang, K., Fang, S., Bu, D., Li, H., et al. (2019). SymMap: An integrative database of traditional Chinese medicine enhanced by symptom mapping. Nucleic Acids Res. 47, D1110–D1117. doi:10.1093/nar/gky1021
Xu, X., Zhang, W., Huang, C., Li, Y., Yu, H., Wang, Y., et al. (2012). A novel chemometric method for the prediction of human oral bioavailability. Int. J. Mol. Sci. 13, 6964–6982. doi:10.3390/ijms13066964
Zhang, L., Tian, Y., Zhao, P., Jin, F., Miao, Y., Liu, Y., et al. (2022a). Electroacupuncture attenuates pulmonary vascular remodeling in a rat model of chronic obstructive pulmonary disease via the VEGF/PI3K/Akt pathway. Acupunct. Med. 40, 389–400. doi:10.1177/09645284221078873
Zhang, Q., Zong, Y., Yang, L., Wang, K., and Wang, Y. (2022b). Clinical evaluation and exploration of mechanisms for modified xiebai powder or modified xiebai powder combined with western medicine in the treatment of pneumonia. Comput. Math. Method Med. 2022, 2287470. doi:10.1155/2022/2287470
Zhang, X. W., Liu, W., Jiang, H. L., and Mao, B. (2019b). Dissection of pharmacological mechanism of Chinese herbal medicine yihuo huatan formula on chronic obstructive pulmonary disease: A systems pharmacology-based study. Sci. Rep. 9, 13431. doi:10.1038/s41598-019-50064-9
Zhang, Y. Z., Wu, Q. J., Xing, X. X., Chen, Y. Y., and Wang, H. (2019a). Effects of SIRT1/Akt pathway on chronic inflammatory response and lung function in patients with asthma. Eur. Rev. Med. Pharmacol. Sci. 23, 4948–4953. doi:10.26355/eurrev_201906_18085
Zhen, G., Yingying, L., and Jingcheng, D. (2018). Traditional Chinese medicine tonifying kidney therapy (Bu shen) for stable chronic obstructive pulmonary disease Protocol for a systematic review and meta-analysis. Med. Baltim. 97, e13701. doi:10.1097/MD.0000000000013701
Keywords: chronic obstructive pulmonary disease, Erchen decoction, Xiebai powder, synergistic mechanism, traditional Chinese medicine, herb-symptom analysis
Citation: Ye H, He B, Zhang Y, Yu Z, Feng Y, Wen C, Xi C and Feng Q (2023) Herb-symptom analysis of Erchen decoction combined with Xiebai powder formula and its mechanism in the treatment of chronic obstructive pulmonary disease. Front. Pharmacol. 14:1117238. doi: 10.3389/fphar.2023.1117238
Received: 06 December 2022; Accepted: 10 May 2023;
Published: 19 May 2023.
Edited by:
Poonam Arora, Shree Guru Gobind Singh Tricentenary University, IndiaReviewed by:
Abhitinder Kumar, GD Goenka University, IndiaYunsen Zhang, University of Macau, China
Yahong Chen, Peking University Third Hospital, China
Lalit Mohan Nainwal, GD Goenka University, India
Copyright © 2023 Ye, He, Zhang, Yu, Feng, Wen, Xi and Feng. This is an open-access article distributed under the terms of the Creative Commons Attribution License (CC BY). The use, distribution or reproduction in other forums is permitted, provided the original author(s) and the copyright owner(s) are credited and that the original publication in this journal is cited, in accordance with accepted academic practice. No use, distribution or reproduction is permitted which does not comply with these terms.
*Correspondence: Chuanbiao Wen, wenchuanbiao@cdutcm.edu.cn; Chongcheng Xi, xichongcheng@bucm.edu.cn; Quansheng Feng, fengqs118@163.com