- 1Department of Pharmacology, Faculty of Pharmaceutical Sciences, Government College University, Faisalabad, Pakistan
- 2Hamza College of Pharmaceutical and Allied Health Sciences, Lahore, Pakistan
Silibinin (SIL), a flavolignan extracted from the medicinal plant “silybum marianum (milk thistle)”, has traditionally been used to treat liver disease. This phytochemical has displayed neuroprotective properties, its activity against schizophrenia is not elucidated. The present study was designed to evaluate the antipsychotic potential of silibinin and probe its toxic potential. The acute oral toxicity study was assessed as per OECD 425 guidelines. Animals were divided into two groups of female rats (n = 6): one group served as the normal control and the other group received a 2,000 mg/kg dose of SIL. We also evaluated the antipsychotic potential of SIL. To this end, animals were divided into six groups (n = 10) of mice for both the preventive and curative protocols. Group I (CMC 1 mL/kg) served as the normal control and received CMC 1 mL/kg; group II was the diseased group treated with ketamine (10 mg/kg) i.p; group III was the standard group treated with clozapine 1 mg/kg; groups IV, V, and VI served as the treatment groups, receiving SIL 50, 100, and 200 mg/kg, respectively, orally for both protocols. Improvement in positive symptoms of the disease was evaluated by stereotypy and hyperlocomotion, while negative symptoms (behavioral despair) were determined by a forced swim test and a tail suspension test in the mice models. The results suggested that the LD50 of SIL was greater than 2,000 mg/kg. Moreover, SIL prevented and reversed ketamine-induced increase in stereotypy (p < 0.001) and behavioral despair in the forced swim and tail suspension tests (p < 0.001). Taken together, the findings suggest that silibinin is a safe drug with low toxicity which demonstrates significant antipsychotic activity against the positive and negative symptoms of schizophrenia.
1 Introduction
Phytochemicals derived from plant, animals, and marine sources have played a vital role in alleviating major human suffering. They have demonstrated therapeutic potential in reducing the risk of major health problems such as cancers, cardiovascular diseases, and different central nervous system (CNS) disorders (Kumar and Khanum, 2012). Phytochemicals have gained attention due to their therapeutic value and the fact that they have fewer adverse effects. Most people seem to believe that natural products are free of any kind of adverse properties, Paracelsus, known as the father of toxicology, stated that “all substances are poisons; it is the right dose that differentiates remedy and poison.” Hence, assessing the toxic potential of natural products is of vital importance (Newman and Cragg, 2012).
Schizophrenia is a severely debilitating disease that affects patients’ thoughts, behavior, perception, and memory (Faludi et al., 2011). The disease affects 1%–1.5% of the global population (Mcgrath et al., 2008) and is rated seventh among the most costly diseases (Ross and Margolis, 2005). Symptoms of schizophrenia cluster into three groups: positive symptoms include hallucination, thought disorder, and delusion; negative symptoms include lack of interest and pleasure and low social interaction; cognitive symptoms include memory and thinking problems (Tandon et al., 2013). The etiology of this disease is still unclear, although the dopamine hypothesis remains its mainstay. After 1980, the glutamate hypothesis was proposed as the establishing basis of schizophrenia (Yadav et al., 2019).
Pharmacotherapeutic agents for schizophrenia were introduced in 1950s and have been active for positive symptoms but ineffective against negative symptoms and cognitive deficits (Purdon et al., 2001). Typical antipsychotics have been dopamine (D2) receptor antagonists such as haloperidol and prochlorperazine. Second generation drugs (clozapine and quetiapine) were then approved for negative symptoms and cognitive impairments (Wang et al., 2019). These antipsychotic drugs are the mainstay of treatment but are unsuccessful at counteracting the symptoms and progression of the disease. Some serious adverse effects as extrapyramidal symptoms, dyslipidemia, diabetes, and hypertension are related to antipsychotic drug use (Brown, 2012; Fell et al., 2012). Therefore, there is a need to investigate new and more effective therapeutic alternatives with fewer adverse effects to increase adherence and therapeutic outcomes.
Silibinin (SIL) is a flavolignan derived from silymarin, a compound that is extracted from the seeds and fruit of the milk thistle herb (Silybum marianum). This plant belongs to the Asteraceae family and its fruit has been famous for use in medicines for centuries. Silybin A and Silybin B are two stereoisomers of silibinin (Mashhadi Akbar Boojar et al., 2020). Flavonoids are known for their antioxidant antiviral, anti-inflammatory, and neuroprotective effects (Hassan et al., 2022). According to previous findings, SIL acts as an antioxidant, anti-inflammatory, anticarcinogenic, and growth promoting agent and is used as a hepatoprotective agent—especially for liver cirrhosis (Chu et al., 2004; Shanmugam et al., 2008; Yin et al., 2011). Moreover, it is also useful for dermatological conditions and skin aging and can act as an antiviral and protective agent against retinal diseases (Singh and Agarwal, 2009; Ahmed-Belkacem et al., 2010). Furthermore, SIL is a neuroprotective agent against neural damage, ethanol-induced brain damage, Aβ-induced memory impairment, and neurotoxicity associated with lipopolysaccharide. Its antidepressant activity is also established (Mazzio et al., 1998; Yan et al., 2015), but its antipsychotic property has not been investigated. Acute toxicity study is basic and preliminary to evaluating the general safety and toxicity of a substance (Baig et al., 2022); however, despite its wide usage, toxicity data is not yet available for SIL (Kennedy et al., 1986).
Hence, the present study aims to evaluate the acute oral toxicity of SIL and determine its antipsychotic potential.
2 Materials and methods
2.1 Drug and chemicals
This study used silibinin (CAS # 22888-70-6), ketamine (CAS # 1867-66-9), clozapine (CAS # 5786-21-0), sodium hydroxide (CAS # 1310-73-2), sodium carboxymethyl cellulose, isoflurane (CAS # 26675-46-7), pyrogallol (CAS # 87-66-1), DTNB (5, 5-dithiobis-(2-nitrobenzoic acid) (CAS # 2516-96-3), Folin-Ciocalteu (CAS # F9252), potassium phosphate monobasic, and sodium phosphate monobasic. All chemicals were of analytical grade and were purchased from Sigma Aldrich (China).
2.2 Animals
Adult Wister female rats weighing 200–300 g were used to examine acute toxicity. Male and female Swiss albino mice (approximately 30 g) were purchased from Government College University Faisalabad, Pakistan, and were used for behavioral studies of schizophrenia. The animals were kept in the department’s animal house at 22 ± 2ᵒC with a relative humidity of 44%–56% and a 12 h light–dark cycle. They were provided with a standard rodent pellet diet and water. The animals were acclimatized to handling and the room and apparatus before experimentation commenced. Ethical approval for the animal studies was obtained from the Animal Ethics Committee of Government College University Faisalabad, reference number GCUF/ERC/2015.
2.3 Acute oral toxicity studies
The OECD’s (Organization for Economic Co-operation and Development) 425 Guidelines were followed for performing an acute oral toxicity study. In this study, two groups of healthy adult Wister rats were used (n = 6). The animals were fasted overnight but were allowed access to water. Group I received 0.5% carboxy methyl cellulose (CMC) (1 mL/100 g) and was designated as normal control. Group II, the treatment group, received 2000 mg/kg of SIL orally. Initially, only one animal received SIL (2000 mg/kg) by a single oral dose and observations were made for 24 h. If the animal survived, four further animals were administered single oral doses of SIL (2,000 mg/kg). The animals were observed for mortality, changes in general behavior, weight change, and any kind of allergies for 14 days (Choi et al., 2021).
2.4 Hematological and biochemical analysis
After 14 days, animals were anesthetized with 3%–5% isoflurane diluted with oxygen. Blood samples were collected after anesthesia by cardiac puncture, and hematological and biochemical parameters were evaluated. Hematological parameters included WBC, RBC, Hb, platelet count, hematocrit (HCT) value, mean corpuscular hemoglobin concentration (MCHC), mean corpuscular volume (MCV), and mean corpuscular hemoglobin (MCH) using a hematology analyzer (Norma, USA). For biochemical tests, plasma and serum were separated and biochemical parameters were estimated. The lipid profiling of cholesterol and triglycerides was measured using their specific kits (CC1132, and CC1302 by MTD Diagnostic, Italy, respectively). Liver function tests for alanine transaminase (ALT) and aspartate transaminase (AST) were performed using their appropriate kits (CC1223, CC1213 by MTD Diagnostic, Italy, respectively) (Steinberg et al., 2019).
2.5 Determination of oxidative stress markers
2.5.1 Preparation of organ tissue homogenates
After euthanasia with 3%–5% isoflurane anesthesia diluted with oxygen, the mice brains were collected from all animals in each group with scarification; the brains were washed in a cold solution of normal saline. Brain tissue homogenates were prepared by adding a 0.1 M phosphate buffer of pH 7.4 in a 1:10 ratio. Homogenates were then centrifuged at 6,000 rpm at 4ᵒC for a period of 10 min. Supernatants were then separated for performing different biochemical tests. Organs such as the heart, kidney, lung, liver, and spleen were also collected and weighed separately. Tissue homogenates were prepared similarly to brain homogenate.
2.5.2 Estimation of glutathione level
In 1 mL of tissue homogenate of each of the above organs (heart, brain, kidney, liver, and spleen), 10% trichloroacetic acid (1 mL) was added for precipitation of protein. In 4 mL of phosphate solution, supernatant and 5,5-dithiobis-2-nitrobenzoic acid (DTNB) reagent (0.5 mL) were added and absorbance was measured at 412 nm. The level of GSH is expressed in μg or level of glutathione per mg of protein. GSH level was then measured using the formula (Bhangale and Acharya, 2016):
where Y is the absorbance at 412 nm, Bt is homogenate of brain tissue, DF is dilution factor, and Vu is the aliquot volume (1 mL).
2.5.3 Estimation of the catalase level
Mixed into 0.05 mL of supernatant (tissue homogenate of each organ—heart, brain, kidney, liver, and spleen) was 1.95 mL of phosphate buffer (50mM, pH 7.0). The above solution was mixed with 1 mL of 30 mM hydrogen peroxide (H2O2) and absorbance was recorded at 240 nm. Values were expressed in micromoles of H2O2 oxidized/min/mg of protein. The catalase level was determined by formula (Anwar et al., 2021).
where O.D is the change in absorbance/minute while E is the extinction coefficient (0.071 mmol4/cm) of H2O2.
2.5.4 Estimation of superoxide dismutase level
Tissue homogenates (0.1 mL) of heart, brain, kidney, liver, and spleen were added to 2.8 mL of 0.1 M potassium phosphate buffer (pH 7.4) and 0.1 mL pyrogallol solution. The absorbance of the mixture was measured on a UV spectrophotometer at 325 nm (X. Li, 2012).
By using a regression line equation, SOD level was thus determined:
2.5.5 Determination of malnodialdehyde level
The supernatant of 1 mL of each organ (heart, brain, kidney, liver, and spleen) and 1 mL of thiobarbituric acid (4.0 mM) were mixed in 100 mL of glacial acetic acid. A mixture of 3 mL of sample was shaken and set aside for 15 min and kept for cooling. Centrifugation was done at 35,000 rpm for 10 min. Absorbance was taken at 532 nm. Malnodialdehyde was quantified as micromole per mg of protein. The following formula was used for MDA quantification:
where Abs 532 is absorbance, Vt is mixture volume (4 mL), 1.56 × 105 is the molar extinction coefficient, wt is the weight of brain, and Vu is the volume of aliquot (1 mL) (Bhangale and Acharya, 2016).
2.5.6 Estimation of nitrite level
Griess reagent was used to determine nitrite level by spectrophotometer. A mixture was made by adding equal quantities of Griess reagent and tissue homogenate of each organ (heart, brain, kidney, liver, and spleen). Incubation was done for 10 min and then absorbance was taken at 546 nm. Nitrite level was calculated by the following regression line equation for sodium nitrite (Anwar et al., 2021):
2.5.7 Estimation of protein content
Three solutions were prepared. A) 1% NaK tartrate in H2O; B) 0.5% CuSO4.5H2O; C) 2% Na2CO3 dissolved in 0.1 N NaOH in water. Reagent 1 was formed by mixing Solutions A (48 mL), B (1 mL), and C (1 mL). Reagent 2 was prepared in 2:1 part (one part folin-phenol [2 N]: one part H2O). For protein content determination, we added 0.2 mL tissue homogenate of each organ (heart, brain, kidney, liver, and spleen) to 4.5 mL of reagent and then incubated it for 10 min. Reagent 2 (0.5 mL) was then added to the mixture and incubated again for 30 min. The absorbance of the mixture was noted at 660 nm. The protein contents were determined by the following regression line equation of BSA and expressed in μg/mL (Lowry et al., 1951):
2.6 Evaluation of antipsychotic potential
2.6.1 Study protocol
The effect of SIL on ketamine-induced schizophrenia-like behavioral alterations was evaluated by a procedure following Ben-Azu et al. (2018) with modifications for both the preventive and treatment protocols. Animals were grouped in two phases, with six groups for the preventive study and six groups for the curative tests (n = 10).
Ketamine was given to all groups except group I1; group III received clozapine; groups IV, V, and VI received SIL with a 30 min interval after ketamine. In the preventive protocol, SIL was administered 30 min before ketamine in groups IV, V, and VI while, in the curative protocol, SIL was given to these groups after 30 min of ketamine administration.
2.7 Behavioral tests
2.7.1 Ketamine-induced stereotypy
The effect of SIL on stereotypy induced by ketamine was assessed by any repetitive, functionless activity of mice in a transparent observation cage (23 × 20 × 20 cm). Stereotypical behavior was counted as head movement, intermittent sniffing, licking, and chewing for 2 min after 5, 10, 15, 30, and 60-min intervals. After each observation, the chamber was cleaned with 70% ethanol to remove any odor.
Initially, animals were individually placed in cylindrical metal cages for 15 min prior to the test to acclimatize to the environment. After ketamine administration, the mice were immediately placed in a cage at the base. Scoring for stereotypy was set as:
0 = no stereotypy,
1 = head movements,
2 = intermittent sniffing,
3 = chewing,
4 = licking intensely (Eneni et al., 2020).
2.7.2 Ketamine-induced hyperlocomotion
Locomotor activity was measured by an activity meter. An acclimatization time of 10 min was given to each animal before testing. After acclimatization, animals were individually placed in an actophotometer, and total locomotor activity was counted for 5 min. Before and after ketamine administration, animals were placed in the actophotometer. Observations were made in a square closed arena of 30 cm that was equipped with infrared-light-sensitive photocells. The total number of crossings was automatically calculated for 5 min. After each test session, the chamber was cleaned with 70% ethanol (Bishnoi et al., 2008).
2.7.3 Ketamine-induced immobility
2.7.3.1 Immobility in forced swim test
The mice were acclimatized in a glass cylinder of 33 cm height and 20.5 cm diameter that contained water 20 cm deep at 25ᵒC. They were removed after 15 min and returned to the cage. This was referred to as “training day”. The next day, the mice were again placed in a cylinder for 6 min. Their swimming activity was recorded after the initial first 2-min period. Their duration of immobility was determined where “immobility” refers to a condition when no other activity was present despite that needed to keep the animal`s head above the water. The animals were then dried with a towel after the test was completed (Chen et al., 2021).
2.7.3.2 Immobility in tail suspension test
To determine immobility, a tail suspension test was performed. The mice were suspended from a tail hanger with adhesive tape that was wrapped around their tails from about 2 to 3 cm from the tip, and about 30 cm above the floor in a box (25 × 25×30 cm). The animals were observed for 6 min and the duration of their immobility was measured manually. Immobility was determined when the animal hung passively and remained motionless (Arruda et al., 2008).
2.8 Statistical analysis
All results were presented as mean ± SEM. Statistical significance difference was analyzed by one-way ANOVA followed by Dunnett`s t-test and two-way ANOVA followed by Bonferroin`s multiple comparison test. *p < 0.05, **p < 0.01, and ***p < 0.001 denoted mild, moderate, and high levels of significance.
3 Results
3.1 Acute toxicity studies
3.1.1 Effect of treatments on body and organ weight
The body weights of the treatment groups were measured daily until the 14th day. Significant increases in body weight were seen in group (SIL 2,000 mg/kg) treated animals compared with Day 1 (Table 1). There was no significant difference in the weights of the selected organs compared with the normal control group (Table 2).
3.1.2 Treatment effect on hematology parameters
On the 14th day, blood was collected from each group through cardiac puncture. Table 3 showed that, except for the platelet and WBCs count, all parameters show a non-significant difference compared to the control group. The platelet count significantly decreased and the WBCs count was significantly increased compared to the control group.
3.1.3 Effect of treatments on biochemical markers in the acute oral toxicity study
The SIL (2,000 mg/kg) treated group showed a significant increase in all biochemical markers (renal, hepatic, and lipid profile) compared with the normal control group. Uric acid and protein levels had parallel values as compared to control (Table 4).
3.1.4 Oxidative stress markers in acute toxicity studies
Oxidative stress biomarkers were estimated in selected organs (heart, brain, kidney, liver, and spleen) to identify signs of toxicity and cellular destruction. A significant reduction in endogenous antioxidants (SOD, GSH, and CAT) was observed in the kidney while MDA levels in the kidney had risen when compared to the control group. The spleen and liver displayed a significant rise in SOD and CAT versus control. MDA and nitrite levels were significantly reduced in the brain and heart compared to normal control groups (Figure 1).
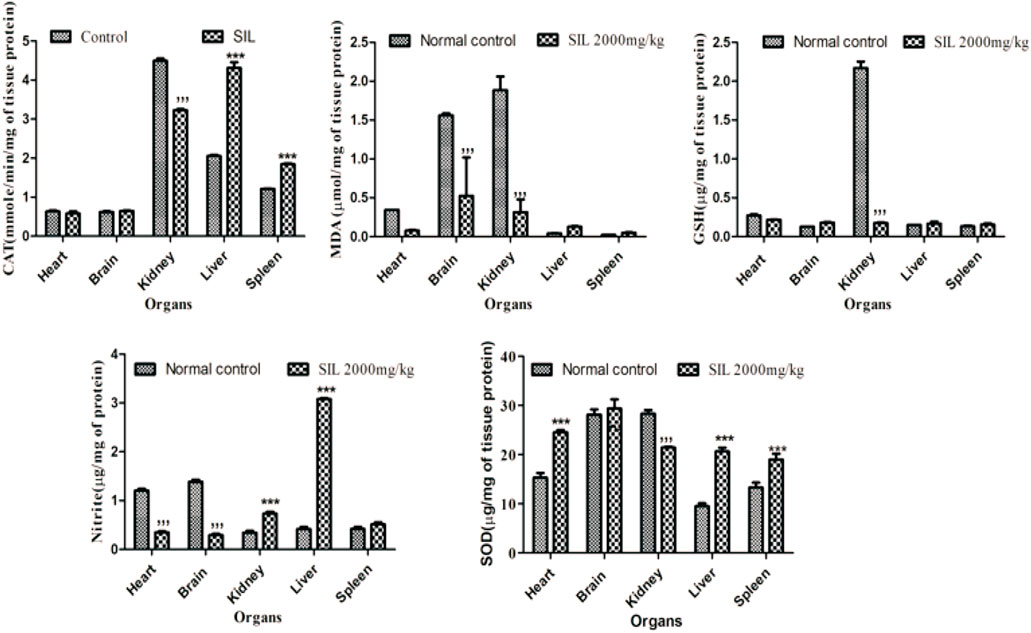
FIGURE 1. Effect of normal control and SIL treatment on oxidative stress markers. Data presented as mean ± SEM. n = 6. Here, ***p < 0.001 high significant increase vs. the control group, and ***p < 0.001 significant decrease compared with the normal control group.
3.1.5 Histopathological analysis
Histopathological analysis showed normal cellular architecture, and no significant change was observed in selected organs after treatment compared to the normal control group (Figure 2).
3.2 Behavioral observations
3.2.1 Effect of SIL on ketamine-induced stereotypy in preventive and treatment protocols
Stereotypy (in minutes) was counted in all treatment groups in both the preventive and treatment protocols. Animals treated with ketamine showed significant stereotypic behaviors compared to the control group. Observations were made between 10 and 30 min in both the treatment and preventive protocols. SIL at all dose levels reduced the stereotypy counts compared to the ketamine group in the treatment protocol, and hence, there was a highly significant (p < 0.001) and dose-dependent response to decreasing stereotypy (Figure 3B). In the preventive protocol, SIL at 100 mg/kg showed a significant (p < 0.01) reduction in stereotypy while SIL (200 mg/kg) and clozapine showed highly significant (p < 0.001) decreases in the stereotypy score (Figure 3A).
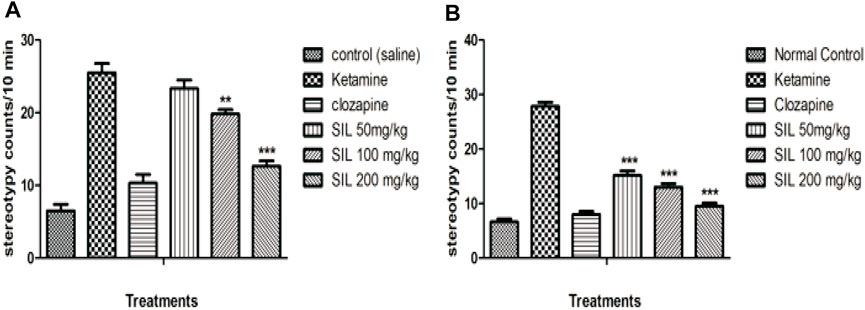
FIGURE 3. Effect of SIL on ketamine-induced stereotypy in preventive (A) and curative (B) protocols. Values expressed here as mean ± SEM; n = 10, where **p < 0.01, ***p < 0.001 compared to the ketamine group.
3.2.2 Effect of SIL on ketamine-induced hyperlocomotion in preventive and treatment protocols
In the open field test, locomotor activity was significantly increased in the ketamine group when compared with control in both the preventive and curative treatment protocols. While the results showed SIL at all doses and clozapine significantly (p < 0.001) reduced the hyperlocomotion induced by ketamine in both protocols. Moreover, in the preventive protocol, SIL at 100 mg/kg showed a significant reduction (p < 0.01) in the number of crossings. Hence SIL represented a dose-dependent decrease in hyperlocomotion in comparison to the ketamine group (Figures 4A, B).
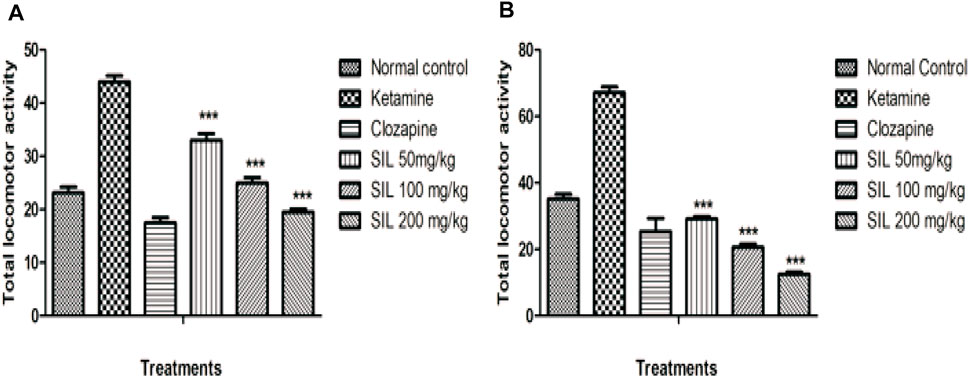
FIGURE 4. Effect of SIL on ketamine-induced hyperlocomotion in preventive (A) and curative (B) protocols. Values are expressed as mean ± SEM; n = 10, ***p < 0.001 as compared to the ketamine group.
3.2.3 Effect of SIL on ketamine-induced immobility by forced swim test in preventive and treatment protocols
Behavioral despair was evaluated by immobility time in the forced swim test in all treatment groups in the preventive as well as treatment protocols. Ketamine injection produced a marked increase in immobility time that connotes behavioral despair when compared with the saline group. SIL showed a significant (p < 0.001) and dose-dependent reduction in immobility in all treatment groups in the treatment protocol. In the preventive protocol, only the highest dose group of 200 mg/kg exhibited a significant reduction in immobility time (Figures 5A, B).
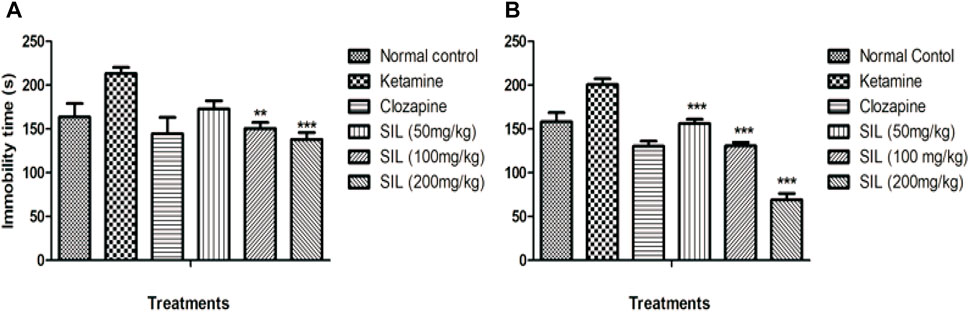
FIGURE 5. Effect of SIL on ketamine-induced immobility in forced swim test in preventive (A) and treatment (B) protocols. Values are represented here as mean ± SEM; n = 10, where **p < 0.01, ***p < 0.001 compared to the ketamine group.
3.2.4 Effect of SIL on ketamine-induced behavioral despair by tail suspension test in preventive and treatment protocols
Behavioral despair was also evaluated by the tail suspension test in treatment and preventive protocols (Figures 6A, B). Ketamine (10 mg/kg) significantly induced immobility and behavioral despair compared with the control group. In the curative protocol, SIL significantly (p < 0.001) and dose dependently reduced immobility time in mice. Only clozapine and SIL 200 mg/kg showed a highly significant (p < 0.001) reduction in ketamine-induced immobility (Figures 6A, B).
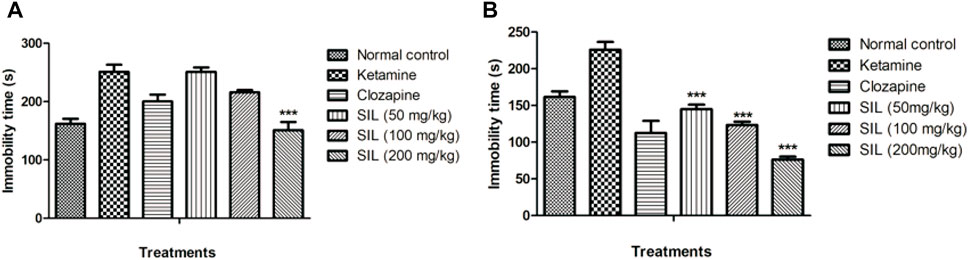
FIGURE 6. Effect of SIL on ketamine-induced immobility by tail suspension test in preventive (A) and treatment (B) protocols. Values expressed as mean ± SEM; n=10, ***p<0.001 compared to the ketamine group.
4 Discussion
Phytochemicals are derived from natural origins and provide cost-effective, valuable, and relatively safe alternatives for allopathic medicines (Saleem et al., 2022; Datta et al., 2021). The WHO and FDA have emphasized the validation of safe and effective uses of natural chemicals by conducting scientific studies (Saleem et al., 2017) and preliminary toxicity studies are important for the safe use of phytochemicals. Silibinin is known for its various pharmacological properties, like treating diabetes, hypertension, dyslipidemia; it has been utilized in China, the USA, and Europe for thousands of years for its folkloric properties against liver diseases and its hepatoprotective properties (Mazzio et al., 1998; Takke and Shende, 2019). However, comprehensive knowledge about its safety and toxicity is still lacking. Hence, this study was designed to evaluate the LD50 of SIL by following OECD guidelines in an animal model which will help to determine the safe dose range for managing its adverse effects in clinical practice (Anwar et al., 2021). The main purpose of this study was to observe animals after a single high-dose administration for any life-threatening toxicities or adverse effects. The results of this study provide evidence that SIL, according to the globally harmonized classification system, can be placed in the fifth group where LD50 > 2000 mg/kg in a lower toxicity class (Saleem et al., 2017). Undeniably, hematological and biochemical estimations remain the hallmarks of the health status of the body, as these are sensitive to changes produced by chemical toxins. Platelets are known to play an important role in coagulation (Li et al., 2008); results have revealed a decrease in the level of platelet counts, which might be linked to the hemostatic property of SIL. Moreover, the elevated WBC count is suggestive of the immunopotentiation property of SIL by endogenous defense system to the adverse effects of silibinin. As the liver and kidney are primary organs for the metabolism and elimination of chemicals, alterations in the liver’s function parameters and renal function tests are known to be connected to the toxicity of said organs (Moses et al., 2018). Moreover, an increase in cholesterol and triglyceride showed the hyperlipidemic effect of SIL, while elevation in ALT and AST might show some hepatic injury. Liver damage may cause increased permeability of cell membranes that could result in a release of aminotransferases into the blood (Ogunlana et al., 2013). These findings also demonstrate that elevated renal function parameters may also be related to mild renal injury. Moreover, histopathological findings appear quite normal, and no significant change appeared in the selected organs studied.
Continuous exposure to reactive oxygen species in the living system causes oxidative stress damage, adversely affecting proteins, lipids, nucleic acid, and whole-body systems. Necrosis, apoptosis, and irreversible cellular damage are linked with oxidative stress (Jones and Libert, 2006; Nair et al., 2015). The current studies estimated the endogenous levels of oxidants and antioxidants in the major organs of animals’ bodies after treatment with SIL. There was a significant reduction in antioxidants (GSH, CAT, and SOD) in the kidney and an increase in the oxidant MDA. SIL as a lipophilic compound might be excreted through the kidney, indicative of a decrease in its antioxidant parameters. Moreover, an increase in the level of antioxidants (SOD and CAT) in the liver and spleen and a reduction in MDA and nitrite in the brain and heart shows the increased antioxidant property of SIL in these respective organs.
Another aim of the present study is to demonstrate the efficacy of SIL in protecting and reversing ketamine-induced psychosis-like behavioral manifestations. In recent research on schizophrenia, the use of animal models that can present different symptoms of schizophrenia (positive, negative, and cognitive) has become more popular (Chatterjee et al., 2012b). Schizophrenia, being a complex neuropsychiatric disease, involves different neurochemical alterations, including the effect of dopamine, gamma amino butyric acid (GABA), and glutamate on motor neuron function (Vasconcelos et al., 2015). Our results support previous findings that ketamine (an NMDA blocker) can induce multifarious behavioral alterations (i.e., stereotypy, hyperlocomotion, and behavioral despair) that are relevant to the positive and the negative symptoms of the disease. Ketamine-induced stereotypy and hyperlocomotion have remained important measures of positive symptoms (Ben-Azu et al., 2016). These symptoms have been previously linked with the NMDA receptor blockade that is present in inhibitory GABA neurons in the mesolimbic region of the brain. This inhibition causes behavioral alterations called stereotypy that are characterized by aimless and repetitive motor activity (Chatterjee et al., 2012b). Furthermore, ketamine may act as an indirect dopamine agonist, which might also explain its valuable role in behavioral stimulations (Irifune et al., 1991). Clozapine is a widely used drug for schizophrenia patients in clinical settings as well as a reference drug for researchers on schizophrenia (Vasconcelos et al., 2015). Thus, our study demonstrated the effect of SIL to prevent and reverse ketamine-induced stereotypy (as evidenced through decreased stereotypic head movements, intermittent sniffing, intense licking, and chewing in mice) and hyperlocomotions (number of crossings), revealing its antipsychotic potential against positive symptoms (Ben-Azu et al., 2018).
Furthermore, SIL was also evaluated for its effect on negative symptoms of schizophrenia by ketamine-induced immobility duration by a forced swim test and tail suspension test. Both models represented behavioral despair and a lack of motivational behavior suggestive of the negative symptoms of disease (Chatterjee et al., 2012a). Our findings are in line with previous research as intraperitoneal injection of a sub-anesthetic dose of ketamine demonstrates behavioral despair related to negative symptoms of schizophrenia represented by increased immobility in the forced swim and tail suspension tests (Eneni et al., 2020). The administration of SIL reduced the immobility period in these tests comparable with clozapine. This and other antipsychotic drugs act to manage the negative symptoms of schizophrenia, generally acting as antagonists on 5HT2 receptors (Glatt et al., 1995; George et al., 2020). Accordingly, our findings imply that SIL can act as antipsychotic drug and may attenuate the positive and negative symptoms of schizophrenia, warranting further investigation of its mechanism of action.
Conclusion
From the findings of this acute toxicity study, it can be concluded that the LD50 of SIL is more than 2000 mg/kg, and there was no sign of morbidity and mortality seen in animals, except for some alterations in renal function test parameters and lipid profile. More importantly, this study revealed that SIL can attenuate positive and negative symptoms of schizophrenia and can act like an atypical antipsychotic. However, the results suggest that it must be evaluated in future chronic and repeated administration of silibinin to completely ensure its safety. Antipsychotic potential can be determined in more detail by long-term studies. Furthermore, a more scientific approach can further identify the mechanistic study of silibinin as an antipsychotic drug.
Data availability statement
The raw data supporting the conclusions of this article will be made available by the authors, without undue reservation.
Ethics statement
Ethical approval for the animal studies was obtained from the Animal Ethics Committee of Government College University Faisalabad with reference number GCUF/ERC/2015.
Author contributions
US and BA supervised the project; QA and IK performed all the research work and drafted the manuscript.
Acknowledgments
The authors are thankful to Government College University Faisalabad for providing research facilities to carry out the research.
Conflict of interest
The authors declare that the research was conducted in the absence of any commercial or financial relationships that could be construed as a potential conflict of interest.
Publisher’s note
All claims expressed in this article are solely those of the authors and do not necessarily represent those of their affiliated organizations, or those of the publisher, the editors, and the reviewers. Any product that may be evaluated in this article, or claim that may be made by its manufacturer, is not guaranteed or endorsed by the publisher.
References
Ahmed-Belkacem, A., Ahnou, N., Barbotte, L., Wychowski, C., Pallier, C., Brillet, R., et al. (2010). Silibinin and related compounds are direct inhibitors of hepatitis C virus RNA-dependent RNA polymerase. Gastroenterology 138 (3), 1112–1122. doi:10.1053/j.gastro.2009.11.053
Anwar, F., Saleem, U., Rehman, A., Ahmad, B., Froeyen, M., Mirza, M. U., et al. (2021). Toxicity evaluation of the naphthalen-2-yl 3,5-dinitrobenzoate: A drug candidate for alzheimer disease. Front. Pharmacol. 12, 607026. doi:10.3389/fphar.2021.607026
Arruda, M., Soures, P., Honorio, J., Lima, R., Chaves, E., Lobato, R., et al. (2008). Activities of the antipsychotic drugs haloperidol and risperidone on behavioural effects induced by ketamine in mice. Sci. Pharm. 76, 673–687. doi:10.3797/scipharm.0810-11
Baig, M. W., Majid, M., Nasir, B., Hassan, S. S. U., Bungau, S., and Haq, I. U. (2022). Toxicity evaluation induced by single and 28-days repeated exposure of withametelin and daturaolone in Sprague Dawley rats. Front. Pharmacol. 13, 999078. doi:10.3389/fphar.2022.999078
Ben-Azu, B., Aderibigbe, A. O., Ajayi, A. M., Eneni, A. E. O., Umukoro, S., and Iwalewa, E. O. (2018). Involvement of GABAergic, BDNF and Nox-2 mechanisms in the prevention and reversal of ketamine-induced schizophrenia-like behavior by morin in mice. Brain Res. Bull. 139, 292–306. doi:10.1016/j.brainresbull.2018.03.006
Ben-Azu, B., Aderibigbe, A. O., Ajayi, A. M., and Iwalewa, E. O. (2016). Neuroprotective effects of the ethanol stem bark extracts of Terminalia ivorensis in ketamine-induced schizophrenia-like behaviors and oxidative damage in mice. Pharm. Biol. 54 (12), 2871–2879. doi:10.1080/13880209.2016.1190382
Bhangale, J. O., and Acharya, S. R. (2016). Anti-Parkinson activity of petroleum ether extract of Ficus religiosa (L.) leaves. Adv. Pharmacol. Sci. 2016, 9436106. doi:10.1155/2016/9436106
Bishnoi, M., Chopra, K., and Kulkarni, S. K. (2008). Protective effect of Curcumin, the active principle of turmeric (Curcuma longa) in haloperidol-induced orofacial dyskinesia and associated behavioural, biochemical and neurochemical changes in rat brain. Pharmacol. Biochem. Behav. 88 (4), 511–522. doi:10.1016/j.pbb.2007.10.009
Brown, A. S. (2012). Epidemiologic studies of exposure to prenatal infection and risk of schizophrenia and autism. Dev. Neurobiol. 72 (10), 1272–1276. doi:10.1002/dneu.22024
Chatterjee, M., Jaiswal, M., and Palit, G. (2012a). Comparative evaluation of forced swim test and tail suspension test as models of negative symptom of schizophrenia in rodents. ISRN Psychiatry 2012, 595141. doi:10.5402/2012/595141
Chatterjee, M., Verma, R., Ganguly, S., and Palit, G. (2012b). Neurochemical and molecular characterization of ketamine-induced experimental psychosis model in mice. Neuropharmacology 63 (6), 1161–1171. doi:10.1016/j.neuropharm.2012.05.041
Chen, S. T., Hsieh, C. P., Lee, M. Y., Chen, L. C., Huang, C. M., Chen, H. H., et al. (2021). Betaine prevents and reverses the behavioral deficits and synaptic dysfunction induced by repeated ketamine exposure in mice. Biomed. Pharmacother. 144, 112369. doi:10.1016/j.biopha.2021.112369
Choi, H. J., Yun, J. W., Kim, Y. H., Kwon, E., Hyon, M. K., Kim, J. Y., et al. (2021). Evaluation of acute and subacute toxicity of sodium taurodeoxycholate in rats. Drug Chem. Toxicol. 44 (3), 268–276. doi:10.1080/01480545.2019.1609493
Chu, S. C., Chiou, H. L., Chen, P. H., Yang, S. F., and Hsieh, Y. S. (2004). Silibinin inhibits the invasion of human lung cancer cells via decreased productions of urokinase-plasminogen activator and matrix metalloproteinase-2. Mol. Carcinog. 40 (3), 143–149. doi:10.1002/mc.20018
Datta, S., Ramamurthy, P. C., Anand, U., Singh, S., Singh, A., Dhanjal, D. S., et al. (2021). Wonder or evil?: Multifaceted health hazards and health benefits of Cannabis sativa and its phytochemicals. Saudi J. Biol. Sci. 28 (12), 7290–7313. doi:10.1016/j.sjbs.2021.08.036
Eneni, A. O., Ben-azu, B., Ajayi, A. M., and Aderibigbe, A. O. (2020). Diosmin attenuates schizophrenia-like behavior, oxidative stress, and acetylcholinesterase activity in mice. Drug metabolism pesonalised Ther. 35 (4). doi:10.1515/dmpt-2020-0119
Faludi, G., Dome, P., and Lazary, J. (2011). Origins and perspectives of schizophrenia research. Neuropsychopharmacol. Hung 13 (4), 185–192. doi:10.5706/nph201112001
Fell, M. J., McKinzie, D. L., Monn, J. A., and Svensson, K. A. (2012). Group II metabotropic glutamate receptor agonists and positive allosteric modulators as novel treatments for schizophrenia. Neuropharmacology 62 (3), 1473–1483. doi:10.1016/j.neuropharm.2011.06.007
George, M. Y., Menze, E. T., Esmat, A., Tadros, M. G., and El-Demerdash, E. (2020). Potential therapeutic antipsychotic effects of Naringin against ketamine-induced deficits in rats: Involvement of Akt/GSK-3β and Wnt/β-catenin signaling pathways. Life Sci. 249, 117535. doi:10.1016/j.lfs.2020.117535
Glatt, C. E., Snowman, A. M., Sibley, D. R., and Snyder, S. H. (1995). Clozapine: Selective labeling of sites resembling 5HT6 serotonin receptors may reflect psychoactive profile. Mol. Med. Camb. Mass 1 (4), 398–406. doi:10.1007/bf03401577
Hassan, S. S. U., Samanta, S., Dash, R., Karpinski, T. M., Habibi, E., Sadiq, A., et al. (2022). The neuroprotective effects of fisetin, a natural flavonoid in neurodegenerative diseases: Focus on the role of oxidative stress. Front. Pharmacol. 1313 (10), 1015835. doi:10.3389/fphar.2022.1015835
Irifune, M., Shimizu, T., and Nomoto, M. (1991). Ketamine-induced hyperlocomotion associated with alteration of presynaptic components of dopamine neurons in the nucleus accumbens of mice. Pharmacol. Biochem. Behav. 40 (2), 399–407. doi:10.1016/0091-3057(91)90571-I
Jones, D., and Libert, M. (2006). Antioxidants and Redox signaling. Redefining oxidative stress 8 (9), 1865–1879.
Kennedy, G. L., Ferenz, R. L., and Burgess, B. A. (1986). Estimation of acute oral toxicity in rats by determination of the approximate lethal dose rather than the LD50. J. Appl. Toxicol. 6 (3), 145–148. doi:10.1002/jat.2550060302
Kumar, G. P., and Khanum, F. (2012). Neuroprotective potential of phytochemicals. Pharmacogn. Rev. 6 (12), 81–90. doi:10.4103/0973-7847.99898
Li, M., Jia, Z., Hu, Z., Zhang, R., and Shen, T. (2008). Experimental study on the hemostatic activity of the Tibetan medicinal herb lamiophlomis rotata. Phytother. Res. 765, 759–765. doi:10.1002/ptr.2359
Li, X. (2012). Improved pyrogallol autoxidation method: A reliable and cheap superoxide-scavenging assay suitable for all antioxidants. J. Agric. Food Chem. 60 (25), 6418–6424. doi:10.1021/jf204970r
Lowry, O. H., Rosebrough, N. J., Farr, A. L., and Randall, R. J. (1951). Protein measurement with the Folin phenol reagent. J. Biol. Chem. 193 (1), 265–275. doi:10.1016/s0021-9258(19)52451-6
Mashhadi Akbar Boojar, M., Mashhadi Akbar Boojar, M., and Golmohammad, S. (2020). Overview of Silibinin anti-tumor effects. J. Herb. Med. 23, 100375. doi:10.1016/j.hermed.2020.100375
Mazzio, E. A., Harris, N., and Soliman, K. F. A. (1998). Food constituents attenuate monoamine oxidase activity and peroxide levels in C6 astrocyte cells. Planta Medica 64 (7), 603–606. doi:10.1055/s-2006-957530
Mcgrath, J., Saha, S., Chant, D., and Welham, J. (2008). Schizophrenia: A concise overview of incidence, prevalence, and mortality. Epidemiol. Rev. 30, 67–76. doi:10.1093/epirev/mxn001
Moses, W., Oboma, I., and Raymond, I. (2018). Toxicological studies on the aqueous leaf extract of Pavetta crassipes (K. Schum) in rodents.
Nair, A. R., Lee, W. K., Smeets, K., Swennen, Q., Sanchez, A., Thévenod, F., et al. (2015). Glutathione and mitochondria determine acute defense responses and adaptive processes in cadmium-induced oxidative stress and toxicity of the kidney. Archives Toxicol. 89 (12), 2273–2289. doi:10.1007/s00204-014-1401-9
Newman, D. J., and Cragg, G. M. (2012). Natural products as sources of new drugs over the 30 years from 1981 to 2010. J. Nat. Prod. 75 (3), 311–335. doi:10.1021/np200906s
Ogunlana, O. O., Ogunlana, O. E., Adeneye, A. A., Udo-Chijioke, O., Dare-Olipede, T., Olagunju, J. A., et al. (2013). Evaluation of the toxicological profile of the leaves and young twigs of Caesalpinia bonduc (Linn) roxb. Afr. J. Traditional, Complementary, Altern. Med. AJTCAM/Afr. Netw. Ethnomedicines 10 (6), 504–512. doi:10.4314/ajtcam.v10i6.20
Purdon, S. E., Malla, A., Labelle, A., and Lit, W. (2001). Neuropsychological change in patients with schizophrenia after treatment with quetiapine or haloperidol. J. Psychiatry Neurosci. 26 (2), 137–149.
Ross, C. A., and Margolis, R. L. (2005). Neurogenetics: Insights into degenerative diseases and approaches to schizophrenia. Clin. Neurosci. Res. 5, 3–14. doi:10.1016/j.cnr.2005.07.001
Saleem, A., ul-Ain, Q., and Akhtar, M. F. (2022). Alternative therapy of psychosis: Potential phytochemicals and drug targets in the management of schizophrenia. Front. Pharmacol. 13, 895668. doi:10.3389/fphar.2022.895668
Saleem, U., Amin, S., Ahmad, B., Azeem, H., Anwar, F., and Mary, S. (2017). Acute oral toxicity evaluation of aqueous ethanolic extract of Saccharum munja Roxb. roots in albino mice as per OECD 425 TG. Toxicol. Rep. 4, 580–585. doi:10.1016/j.toxrep.2017.10.005
Shanmugam, K., Holmquist, L., Steele, M., Stuchbury, G., Berbaum, K., Schulz, O., et al. (2008). Plant-derived polyphenols attenuate lipopolysaccharide-induced nitric oxide and tumour necrosis factor production in murine microglia and macrophages. Mol. Nutr. Food Res. 52 (4), 427–438. doi:10.1002/mnfr.200700180
Singh, R. P., and Agarwal, R. (2009). Cosmeceuticals and silibinin. Clin. Dermatology 27 (5), 479–484. doi:10.1016/j.clindermatol.2009.05.012
Steinberg, P., van der Voet, H., Goedhart, P. W., Kleter, G., Kok, E. J., Pla, M., et al. (2019). Lack of adverse effects in subchronic and chronic toxicity/carcinogenicity studies on the glyphosate-resistant genetically modified maize NK603 in Wistar Han RCC rats. Archives Toxicol. 93, 1095–1139. doi:10.1007/s00204-019-02400-1
Takke, A., and Shende, P. (2019). Nanotherapeutic silibinin: An insight of phytomedicine in healthcare reformation. Nanomedicine Nanotechnol. Biol. Med. 21, 102057. doi:10.1016/j.nano.2019.102057
Tandon, R., Gaebel, W., Barch, D. M., Bustillo, J., Gur, R. E., Heckers, S., et al. (2013). De fi nition and description of schizophrenia in the DSM-5. Schizophrenia Res. 150, 3–10. doi:10.1016/j.schres.2013.05.028
Vasconcelos, G. S., Ximenes, N. C., de Sousa, C. N. S., Oliveira, T. de Q., Lima, L. L. L., de Lucena, D. F., et al. (2015). Alpha-lipoic acid alone and combined with clozapine reverses schizophrenia-like symptoms induced by ketamine in mice: Participation of antioxidant, nitrergic and neurotrophic mechanisms. Schizophrenia Res. 165 (2–3), 163–170. doi:10.1016/j.schres.2015.04.017
Wang, L., Zhang, Y., Wang, C., Zhang, X., Wang, Z., Liang, X., et al. (2019). A natural product with high affinity to Sigma and 5-HT7 receptors as novel therapeutic drug for negative and cognitive symptoms of schizophrenia. Neurochem. Res. 44 (11), 2536–2545. doi:10.1007/s11064-019-02873-7
Yadav, M., Jindal, D. K., Parle, M., Kumar, A., and Dhingra, S. (2019). Targeting oxidative stress, acetylcholinesterase, proinflammatory cytokine, dopamine and GABA by eucalyptus oil (Eucalyptus globulus) to alleviate ketamine-induced psychosis in rats. Inflammopharmacology 27 (2), 301–311. doi:10.1007/s10787-018-0455-3
Yan, W. J., Tan, Y. C., Xu, J. C., Tang, X. P., Zhang, C., Zhang, P. B., et al. (2015). Protective effects of silibinin and its possible mechanism of action in mice exposed to chronic unpredictable mild stress. Biomol. Ther. 23 (3), 245–250. doi:10.4062/biomolther.2014.138
Keywords: silibinin, acute oral toxicity, schizophrenia, ketamine, oxidative stress
Citation: Ain QU, Saleem U, Ahmad B and Khalid I (2023) Pharmacological screening of silibinin for antischizophrenic activity along with its acute toxicity evaluation in experimental animals. Front. Pharmacol. 14:1111915. doi: 10.3389/fphar.2023.1111915
Received: 30 November 2022; Accepted: 11 January 2023;
Published: 02 February 2023.
Edited by:
Arshad Mahmood, Al Ain University, United Arab EmiratesReviewed by:
Syed Shams Ul Hassan, Shanghai Jiao Tong University, ChinaAamir Mushtaq, Government College University Lahore, Pakistan
Copyright © 2023 Ain, Saleem, Ahmad and Khalid. This is an open-access article distributed under the terms of the Creative Commons Attribution License (CC BY). The use, distribution or reproduction in other forums is permitted, provided the original author(s) and the copyright owner(s) are credited and that the original publication in this journal is cited, in accordance with accepted academic practice. No use, distribution or reproduction is permitted which does not comply with these terms.
*Correspondence: Qurat Ul Ain, quratkhawaja2@gmail.com; Uzma Saleem, uzma95@gmail.com