- Medicinal and Aromatic Plants Research Department, Pharmaceutical and Drug Industries Research Institute, National Research Centre, Giza, Egypt
A novel coronavirus strain (COVID-19) caused severe illness and mortality worldwide from 31 December 2019 to 21 March 2023. As of this writing, 761,071,826 million cases have been diagnosed worldwide, with 6,879,677 million deaths accorded by WHO organization and has spread to 228 countries. The number of deaths is closely connected to the growth of innate immune cells in the lungs, mainly macrophages, which generate inflammatory cytokines (especially IL-6 and IL-1β) that induce “cytokine storm syndrome” (CSS), multi-organ failure, and death. We focus on promising natural products and their biologically active chemical constituents as potential phytopharmaceuticals that target virus-induced pro-inflammatory cytokines. Successful therapy for this condition is currently rare, and the introduction of an effective vaccine might take months. Blocking viral entrance and replication and regulating humoral and cellular immunity in the uninfected population are the most often employed treatment approaches for viral infections. Unfortunately, no presently FDA-approved medicine can prevent or reduce SARS-CoV-2 access and reproduction. Until now, the most important element in disease severity has been the host’s immune response activation or suppression. Several medicines have been adapted for COVID-19 patients, including arbidol, favipiravir, ribavirin, lopinavir, ritonavir, hydroxychloroquine, chloroquine, dexamethasone, and anti-inflammatory pharmaceutical drugs, such as tocilizumab, glucocorticoids, anakinra (IL-1β cytokine inhibition), and siltuximab (IL-6 cytokine inhibition). However, these synthetic medications and therapies have several side effects, including heart failure, permanent retinal damage in the case of hydroxyl-chloroquine, and liver destruction in the case of remdesivir. This review summarizes four strategies for fighting cytokine storms and immunomodulatory deficiency induced by COVID-19 using natural product therapy as a potential therapeutic measure to control cytokine storms.
Highlights
⁃ The immunopathogenesis of the cytokine storms seen in severe and acute respiratory-CoV-2 infection is briefly described in this review article.
⁃ Several natural compounds as immunosuppressants that can be employed as adjuvants in the treatment of COVID-19 diseases are discussed.
1 Introduction
A virus can activate the immune system (B-cells, T-cells, macrophages, neutrophil cells, dendritic cells, and monocytes cells) and resident tissue cells, producing massive amounts of pro-inflammatory cytokines (Chugh et al., 2023). During a flu virus infection, innate immune responses are triggered by interferon (IFN)-stimulated gene regulation cascade amplification events, and IFN is mainly produced by macrophages, monocytes, and dendritic cells.
1.1 Global crisis
IAVG spearheaded the effort to achieve 70% coverage with COVID-19 vaccination rates in all nations as a global goal (Knoll and Wonodi, 2021). COVAX’s initial strategy was to attain 3% vaccination coverage, followed by 20% coverage with COVAX-secured doses by the end of 2021 (World Health Organization, 2020). In October 2021, the World Health Organization (WHO) unveiled strategies to reach global coverage for the COVID-19 vaccine by the middle of 2022. These goals were to expand globally (World Health Organization, 2020). By the end of 2021, the revised global aim was 40% total population coverage, with 70% total population coverage by mid-2022. These data, however, came from all countries’ sources of supply, not only COVAX. Conversely, COVAX would make every effort to accomplish this coverage level in a fair and equitable manner (Knoll and Wonodi, 2021).
None of these goals have been achieved. In total, 98 countries do not have 40% of their people immunized. A total of 1.4 billion people are anticipated to be eligible for vaccination (Peacocke et al., 2021), many of whom are in the highest risk groups for death and serious illness. The gaps have been especially obvious in low- and lower-middle-income countries (LICs and LMICs), with 34 of 89 advanced market commitment (AMC) members not meeting the 40% target, representing the nations most reliant on COVAX for COVID-19 vaccine access (Figure 1) (Zhang et al., 2020a).
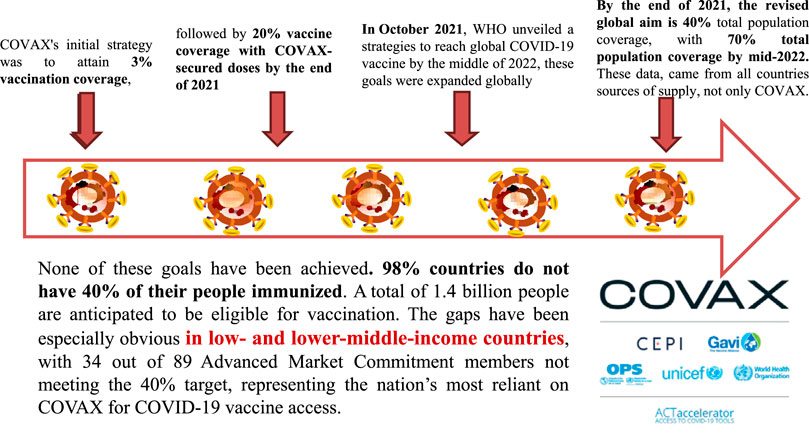
FIGURE 1. Flowcharts of “WHO unveiled strategies to reach global COVID-19 vaccine”. IAVG spearheaded the effort to achieve 70 percent coverage with COVID-19 vaccination rates in all nations as a global goals need. (Adapted from Knoll and Wonodi, 2021).
1.2 COVID-19 pathogenesis
Coronavirus is an encapsulated, non-segmented, and positive (+) RNA virus with a single-stranded sense found in animals, such as dogs, camels, bats, and cats. Because their structure resembles a crown or corona, these viruses are called coronaviruses. Coronaviruses infect humans and animals. For humans, four coronavirus strains (NL63, HKU1, OC43, and 229E) have been identified as infecting the upper respiratory tract and causing minimal symptoms (Fehr and Perlman, 2015). There are three human coronavirus strains (2019-nCoV-2, also called SARS-CoV-2, SARS-CoV, and MERS-CoV) have been identified as infecting the Lower Respiratory Tract, causing pneumonia and Death (Figure 2A). The genetic sequence of SARS-CoV-2, also known as the severe acute respiratory syndrome coronavirus, is 79% similar to that of SARS-CoV and 98% similar to the RaTG13 coronaviruses found in bats chrysanthemum (Figure 2B) (Zhou et al., 2020; Chen et al., 2021). Furthermore, SARS immunopathogenesis, caused by CoV-2, which causes airway damage, is quite similar to that of SARS-CoV. ARDS develops in extreme symptoms of SARS-CoV-2 infection, resulting in respiratory organ failure, the major cause of death. 3) Hong Kong University 1 (HKU1) isolated the Middle East respiratory syndrome coronavirus (MERS-CoV) in 2005 from a pneumonia patient in Hong Kong (Zhang et al., 2020a). Furthermore, the rise in immunological responses to viral infection causes increased quantities of inflammatory cytokines to be released by innate immune cells, resulting in the development of “cytokine storm syndrome” (CSS) (Zhang et al., 2020a). This illness causes uncontrollable inflammation, which causes numerous organ failures and eventually death. As a result of these observations, we can deduce that viral infectious diseases are not the main cause of airway damage. However, the main immune response is also important in disease progression. Additionally, older age and the presence of co-morbidities have been connected to the severity of the condition (Figure 2) (Guan et al., 2020).
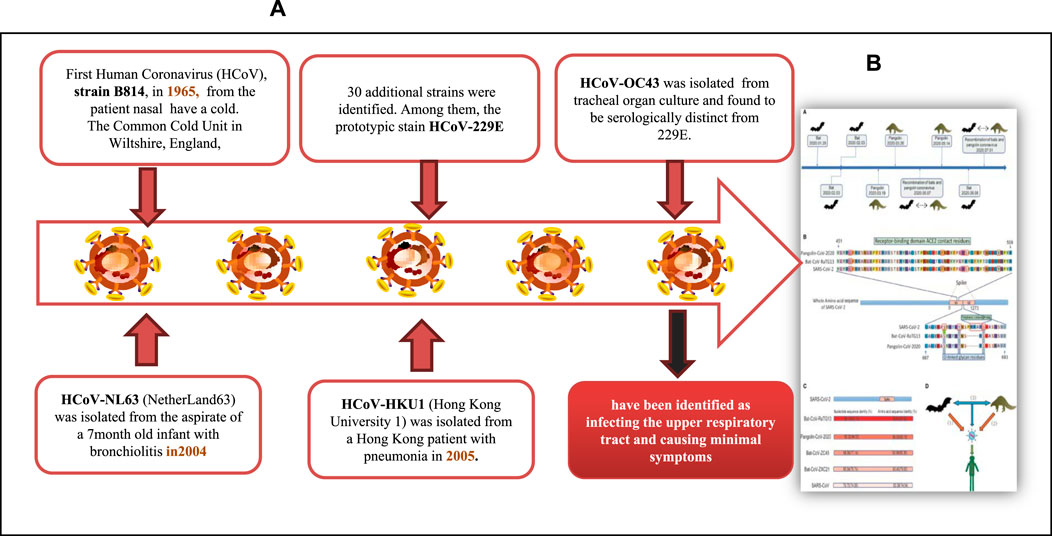
FIGURE 2. (A) COVID-19 Pathogenesis and (B) A member of a wide family of encapsulated, non-segmented, positives (+) RNA viruses’ sense single-stranded that can be found in dogs, camels, bats, and cats. (Adapted from Chen et al., 2021).
1.3 With the problem of the COVID-19 pandemic
Due to the lack of a specialized antiviral to end the infection and a proper vaccination to dissolve it, the COVID-19 epidemic has resulted in a major global health crisis and social unrest. Some people infected with severe acute respiratory CoV-2 have a well-coordinated immune response and recover. In contrast, others have a faulty immune response and do not recover, which can lead to catastrophic consequences. The secretion of enormous amounts of active mediators and inflammatory substances during a cytokine storm not only prevents the virus from spreading further in the body but also causes secondary tissue damage (Tang et al., 2020). A large cytokine and chemokine release, referred to as a “cytokine storm,” has been discovered in patients with a malfunctioning immune response.
Because the cytokine storm is the main cause of the high death rate in COVID-19 patients, it has become a key therapeutic target for reversing the disease’s progression in severely and critically ill individuals.
• Although specific causes of cytokine storms in COVID-19 have not been fully elucidated, 1) hyper-incited activated innate immune responses and 2) ACE-2 dysregulation have been proposed.
• Imagination and expression: To combat cytokine storms, immunoregulatory medications, including 1) cytokine inhibitors, 2) all corticosteroids, 3) blood purifying therapy, and 4) mesenchymal stem cell treatments, have been used (Tang et al., 2020).
Since December 2019, the pandemic, which is caused by a novel coronavirus called 2019-CoV-2 (also SARS-CoV-2) and was originally diagnosed in Wuhan, China, has prompted acute and grave global concern (Bogoch et al., 2020; Wang et al., 2020b). The WHO reportedly proclaimed COVID-19 a global epidemic on 11 March 2020, posing a serious threat to people’s health and societal stability. Severe acute respiratory infection symptoms (SARS) appeared early in the stages of COVID-19, and abrupt respiratory distress syndrome (ARDS), acute respiratory failure, and other significant consequences plagued many patients.
Much evidence indicates immunologically pathogenic changes as follows:
1) Reduced lymphocytes.
2) In COVID-19 patients, especially those severely sick, elevated cytokines are major drivers of disease progression and death.
2 Cytokine storm
The term “cytokine storm” was used to characterize the excessive immune responses that can be generated by a range of events, including virus infection, autoimmune illness, and immunotherapies. It was first used to describe RA and GVHD (Shimabukuro-Vornhagen et al., 2018).
2.1 Viral infection causes a cytokine storm
On 21 March 2023, there are 761,071,826 confirmed COVID-19 cases worldwide, including 6,879,677 deaths reported to the WHO by national authorities, and has spread to 228 countries (Chugh et al., 2023). Many studies indicate that in addition to the use of antiviral medicines to treat COVID-19, the reduction of the cytokine storm might be an effective therapeutic strategy for successfully combating the disease (Rahmati and Moosavi, 2020).
The virus can activate the immune cells system (e.g., B cells, T cells, macrophages, neutrophil cells, dendritic cells, and monocytes cells) and resident tissue cells, producing massive amounts of pro-inflammatory cytokines (Krischuns et al., 2018). During a flu virus infection, innate immune responses are triggered by IFN-stimulated gene regulation cascade amplification events, and IFN is primarily created by macrophages, monocytes, and dendritic cells (Klinkhammer et al., 2018).
In H5N1 (influenza virus infection), the serum concentration levels of IFN-induced protein-10 (IP-10), interleukin-8 (IL-8), macrophage inflammatory protein-1(MIP-1), monocyte chemoattractant protein-1 (MCP-1), monokine stimulated by IFN-γ (MIG), and CXC chemokine ligand-9 (CXCL-9) were anomalously elevated, whereas IL-17, IL-9, IL-8, IL-6, IL-15, tumor necrosis factor-alpha (TNF-α), and IL-10 were abnormally elevated in H1N1 and influenza virus infection (Thomas et al., 2017). Serum concentrations of pro-inflammatory factors [IFN-γ, IL-1β, IL-12, IL-6, IL-8, IL-18, IP-10, MCP-1, CC-chemokine ligand-2 (CCL-2), and CXCL-10] are positively connected with pulmonary fibrosis and significant lung tissue destruction in SARS-coronavirus patients, according to the latest research (Song et al., 2020; Rabaan et al., 2021) (Figure 3E). However, in MERS-coronavirus severe patients, serum levels of pro-inflammatory cytokines IL-6, IL-15, IL-17, IFN-γ, TNF-α, and chemokines IL-8, CXCL-10, and CCL-5 appeared significantly elevated (Mahallawi et al., 2018). The relevance of molecules (e.g., IL-6, IFN-γ, IL-1β, IL-8, IL-10, and TNF-α) increases in virally mediated cytokine storms (Chousterman et al., 2017). As a result, the cytokine storm has been identified as one of the leading causes of death in patients infected with SARS-CoV, MERS-CoV, or other influenza viruses (Dawood et al., 2012). Similarly, in COVID-19, cytokine storm is a typical hallmark of severe cases, and increased serum IL-6 and CRP levels are related to respiratory distress, MOF, ARDS, and poor clinical outcomes (Gómez-Pastora et al., 2020). Another research group recorded 70 reported-long COVID-19 outcomes in unvaccinated patients infected with SARS-CoV-2 matched to uninfected people, adjusted for age and sex and stratified by SARS-CoV-2 variants, and risk in patients with a breakthrough SARS-CoV-2 infection compared with unvaccinated infected controls. Risks were compared using hazard ratios and risk differences per 10,000 patients measured during the early (30–180 days) and late (180–360 days) time periods after infection. The result found that the COVID-19 infection was significantly associated with increased risks in early and late periods for anosmia and dysgeusia and a risk difference with a 95% confidence interval (Mizrahi et al., 2023).
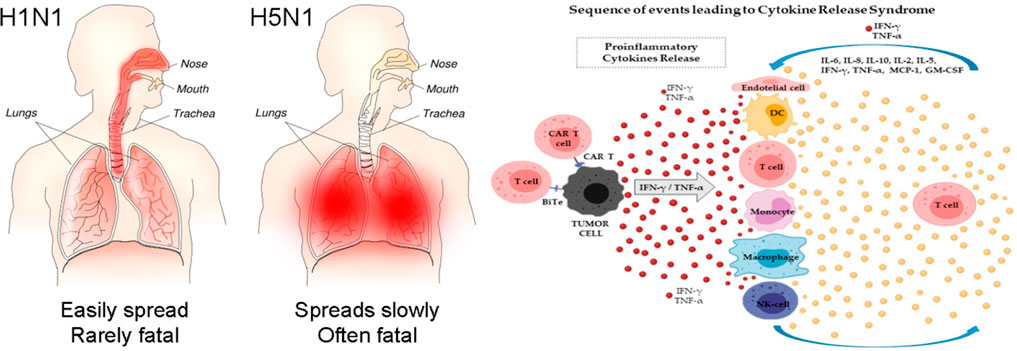
FIGURE 3. (Left) Severe viral infection, a cytokine storm develops in the lungs (Author: Tim Vickers, Source: https://upload.wikimedia.org/wikipedia/commons/b/b0/H1N1_versus_H5N1_pathology.png. (Right) The pathophysiology of cytokine storm (Adapted from Cosenza et al., 2021). (1)Viruses infect lung epithelial cells and alveolar macrophages, resulting in the production of cytokines /chemokines are produced by viruses infection (fundamentally have interferons). (2) Cytokines & chemokines that activate a macrophage and virally destroy dendritic cells initiate a cytokine storm, resulting in a more widespread immune response. (3) Chemokines released in the bloodstream attract more inflammatory cells to the irritation site, and these cells then release more inflammatory chemokines & cytokines, amplifying the “cytokine storms”.
2.2 Cytokine release syndrome (CRS)
A severe, life-threatening illness that can emerge as a result of an infection autoimmune disease is referred to as a cytokine storm or hyper-cytokinemia. Patients infected with SARS-coronavirus and MERS-coronavirus, as well as leukemia patients receiving engineered T-cell therapies, have previously experienced a type of systemic inflammatory syndrome produced by cytokine storms (Fehr et al., 2017; Shimabukuro-Vornhagen et al., 2018). Fever, tiredness, headache, rash, arthralgia, and myalgia are symptoms of moderate cases. High temperature, headache, exhaustion, diffuse intravascular coagulation (DIC), shock, multiple organ failure (MOF), and even death are common symptoms in cases of severe symptoms (Shimabukuro-Vornhagen et al., 2018). Cytopenia, increased creatinine and liver functions, elevated concentrations of C-reactive protein (CRP), and abnormal coagulation parameters are common lab findings (Shimabukuro-Vornhagen et al., 2018). Lee and the lab team described a modified scoring system again for the severity of cytokine release syndrome (CRS), which categorized mild, moderate, severe, and life-threatening symptoms and even death, disregarding the initiating cause (Lee et al., 2014). Clinical decisions in CRS are similarly guided by this grading system. Immune suppressants should be utilized in all sick cases with grade 3 or 4 CRS and, therefore, should be started earlier in patients with substantial comorbidity or the elderly (Shimabukuro-Vornhagen et al., 2018) In Figure 3A, type II pneumocytes in airways and alveoli are first infected by SARS-CoV-2, which causes alveolar macrophages to become activated, cytokines to be produced, and further inflammatory infiltration. The SARS-CoV-2 viral lifecycle is depicted in simplified form in Figure 3B, with the creation of non-structural proteins (NSPs) that block the early IFN response in infected cells, which normally limits viral multiplication. This virulence pathway could indirectly cause an unbalanced inflammatory response. S-CSS typically affects adults and various organ systems, most notably the lymphoid, lung, heart, liver, gastrointestinal, and renal systems (Figure 3C). Multiorgan dysfunction and lung damage may feed off of each other negatively. The majority of children who develop MIS-C (Figure 3D) present with distinctive characteristics, such as pronounced cardiac and gastrointestinal involvement and less frequent or milder involvement of other systems (Cabler et al., 2020).
2.3 The pathophysiology and key of cytokine storms to determine COVID-19
Proliferating lung epithelial cells, which are the initial vectors for virus infection, can attack other cells, including pulmonary macrophages (Liu et al., 2016). When infected cells are destroyed by apoptosis or necrosis, inflammation reactions are initiated. The acute inflammatory process is the organism’s first response to damaging triggers. It is characterized by increased blood flow, which allows serum and leukocytes to reach extravascular areas of damage, the elevation of heating rate, and discomfort. The activation of pro-inflammatory cytokines or chemokines is also a sign of an acute inflammatory process response (Thomas et al., 2017). Several pro-inflammatory cytokines or chemokines can cause inflammatory cells to be recruited (Thomas et al., 2017). Overexpression of inflammation and antiviral and apoptosis genes occurs in conjunction with extensive immune cell infiltration and tissue injury, followed by the initiation of regeneration processes and damage repair. In some cases, this reparative technique can entirely recover function. More substantial pathological alterations, including multiorgan destruction, hyaline membrane development, fibrin exudates, and fibrotic healing, are evident in severe inflammation coupled with cytokine storms. When a virus infects a cell, it produces damage-associated molecular patterns (DAMPs) and pathogen-associated molecular patterns (PAMPs), which could stimulate antiviral reaction responses through neighboring cells and attract innate and adaptive immune cells such as macrophages, natural killer (NK) cells, and gamma delta T (γδT) cells. These symptoms indicate extensive capillary destruction, immunopathologic damage, and chronic organ dysfunction. Furthermore, strong inflammatory cytokines/chemokines could leak into the bloodstream and cause systemic cytokine storms, which cause multi-organ failure. Whenever the virus’s PAMP is identified by the innate immune cells’ system of pattern recognition receptors (PRRs), the inflammatory process begins. After that, the downstream signaling cascades of PRRs are initiated, and specific pro-inflammatory cytokines are produced (Figure 3F).
Patients in the intensive care unit (ICU) had significantly greater concentration levels of plasma-inflammatory cytokines such as IL-2, IL-10, IL-7, granulocyte-colony-stimulating factor (G-CSF), MCP, IFN-γ, and TNF-α than non-ICU patients (Huang et al., 2020), demonstrating a link between the cytokine storms and the severity of the disease. In COVID-19, which differed from SARS-CoV, these cytokines predicted not only Th1-cell response but also Th2-cell responses. CD4+ T-cells were stimulated and differentiated into Th1 cells following infection with SARS-CoV-2, secreting plasma-pro-inflammatory cytokines including IL-6, IFN-γ, and granulocyte–macrophage-colony-stimulating factor (GM-CSF) (Huang et al., 2020). GM-CSF may stimulate mononuclear cells, causing them to release more IL-6 and other pro-inflammatory cytokines, resulting in massive cytokine storms. As a result, the cytokine storm in SARS/COVID-19 may be a key mediated by IL-6 and GM-CSF secreted by mononuclear and T lymphocyte cells. Furthermore, the activation of monocytes could indicate that the cytokine storms in SARS/COVID-19 are linked to the breakdown of the innate-adaptive immune balance. Recent researchers also found that although the level of IL-6 in chronic COVID-19 patients was much higher than that in mild and moderate cases, the levels of CD4+ T cells, CD8+ T cells, and NK cells were lower, indicating immunosuppression (Wan et al., 2020). Although the number of CD4+ and CD8+ T cells in the peripheral blood was reduced, the number of Th17 cells was elevated, and CD8+ T cells were highly cytotoxic, suggesting that a cytokine storm may accelerate tissue destruction (Wan et al., 2020). Meanwhile, during the cytokine storms, a T lymphocyte cell becomes too activated, according to Wan et al. (2020), in Chongqing Hospital, China (Figure 4).
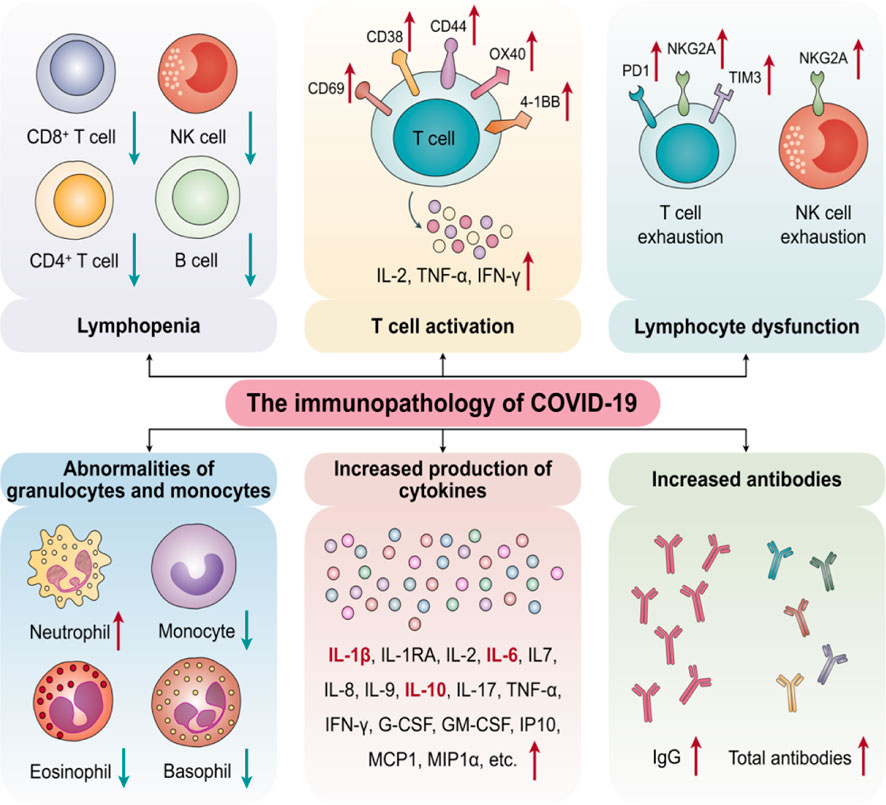
FIGURE 4. The key of Cytokine Storms to determine SARS/COVID-19 in immunopathology (Adapted from Yang et al., 2020a).
2.4 Mechanisms of cytokine storm helping in COVID-19
2.4.1 Hyper-activated innate immune responses
Adaptive and innate immune responses connect and then collaborate in close proximity to create immunological defense during the antiviral immune response process (Hosseini et al., 2020). There is a time limit for adaptive immune responses, which usually gets initiated 4–7 days after infection. Unlike adaptive immune responses, innate immune responses occur immediately after infection and are fully involved in virus clearance. However, innate immunity is relatively weak in virus clearance, and adaptive immunity is the key factor in the complete elimination of the virus (Hosseini et al., 2020). If the body does not generate effective adaptive antiviral responses in time to clear the virus, the innate immune responses will be strengthened, which cannot eliminate the virus effectively and even lead to systematic inflammation responses with the uncontrolled release of inflammatory cytokines. According to recent studies, severe and critically sick patients have a higher average age than mild cases (66 vs. 51 years), and more chronic cases are more likely to have multiple chronic disorders (72.3% vs. 37.4%). Due to the weakening of immune functioning, it takes longer for elderly patients and those with chronic diseases to establish efficient adaptive immune responses. These patients rely solely on their innate antiviral immune responses in the first stages of infection, which increases the risk of cytokine storms, severe disease onset, and mortality (Wang et al., 2020a). Although the kinetics of SARS-CoV-2 responses matches models of antiviral immune induction, it remains unclear if ongoing viral replication or immunological dysregulation causes immune hyperactivity. Pyroptosis is a massive inflammatory, caspase-1-dependent form of program cell death that happens regularly in response to internal cell pathogen infection (ICPI). It may play a role in the development of COVID-19. Rapid viral replication can increase pyroptosis, which can lead to a major release of pro-inflammatory mediators. Viral escapes to circumvent antiviral immunity and inherited or acquired weaknesses in host defense may restrict antiviral therapy, leading to abnormal immune activity and cytokine storms. As a result, the enhanced innate immune response could play a role in COVID-19/cytokine storm production (Figure 5) (Bergsbaken et al., 2009).
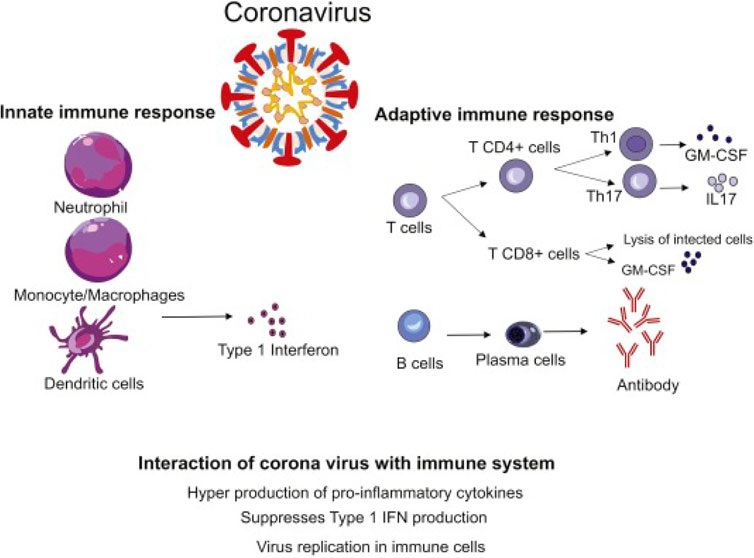
FIGURE 5. The immunological responses against COVID-9-coronavirus (CoV) disease, in both cases innate and adaptive immune responses. The secretion of numerous pro-inflammatory cytokines by neutrophils cells, monocytes cells, macrophages, and dendritic cells is referred to as a “cytokine storm.” Pulmonary immunopathology is the result of this process. Th1 cells and Th17 cells and CD+ T cells may be stimulated, exacerbating lung damage. By lysis of tumor cells, the cytotoxic T-lymphocyte (CTL) contributes to antiviral therapy. Antibodies specific to viruses are produced by B cells, and viruses are neutralized by them. (Adapted from Hosseini et al., 2020).
The secretion of numerous pro-inflammatory cytokines by neutrophil cells, monocyte cells, macrophages, and dendritic cells is referred to as a “cytokine storm.” Pulmonary immunopathology is a result of this process. Th1, Th17, and CD+ T cells may be stimulated, exacerbating lung damage. By lysis of tumor cells, the cytotoxic T lymphocytes (CTLs) contribute to antiviral therapy. Antibodies specific to viruses are produced by B cells, and they neutralize viruses (Hosseini et al., 2020).
2.4.2 ACE-2 and its downstream pathway deregulation
The SARS coronavirus is engulfed by the angiotensin-converting enzyme 2 (ACE-2) receptor, which is required for cell fusion. ACE-2 is found in almost all organs and systems and plays a significant physiological role. ACE-2 also plays a protective role in various illnesses, including acute and chronic pro-inflammatory conditions. However, ACE-2 downregulation by the overactivation of the angiotensin-II/AT1R pathway and the harmful effects of angiotensin-II (Ang-II) caused by the SARS-COVID-19 spike protein could explain the multi-organ dysfunction in ill persons. In COVID-19, the role of Ang-II in the development of macrophage activation syndrome (MAS) and the cytokine storms are discussed in greater depth in the following paragraphs. In this analysis, we investigated the most current research developments in treatment strategies that primarily aim to decrease Ang-II-induced side effects instead of virus proliferation (Banu et al., 2020). As a vital counter-regulator, ACE-2 predominantly catalyzes the breakdown of Ang-II to maintain the homeostasis of the RAAS (Datta et al., 2020). ACE-2 has been already identified as a functional hosting receptor for SARS-CoV-2. Several parameters, including age, sex, ethnicity, medication, and several co-morbidities, were associated with changed ACE-2 expression and infection severity and development (e.g., heart attack disease, metabolic syndrome, and pulmonary cancer) (Liang et al., 2020).
Recent studies have shown besides ACE-2 being a viral-binding receptor in COVID-19, it may play a role in modulating immunological responses (Catanzaro et al., 2020). In lung cancer, Chen and his coworkers discovered that the impression of many axes “mir-125b-5p-ACE-2-IL-6” in (mir-125b-5p) suppressed the impression of IL-6 by encouraging the upregulation of ACE-2 (Morassi et al., 2020). ACE-2 activity (mRNA levels and enzymatic activities) on the surfaces of host cells was significantly decreased after becoming joined by SARS-CoV-2, and IL-6, a downstream effector inside the number of fatalities receptor signaling cascade, could affect the immune response system. Cytokine storms and pneumonia may be increased as a result of the deregulation of ACE-2 induced by SARS-CoV-2, and blocking the upstream regulation mir-125b-5p could provide a novel strategy to manage COVID-19 (Figure 6) (Morassi et al., 2020).
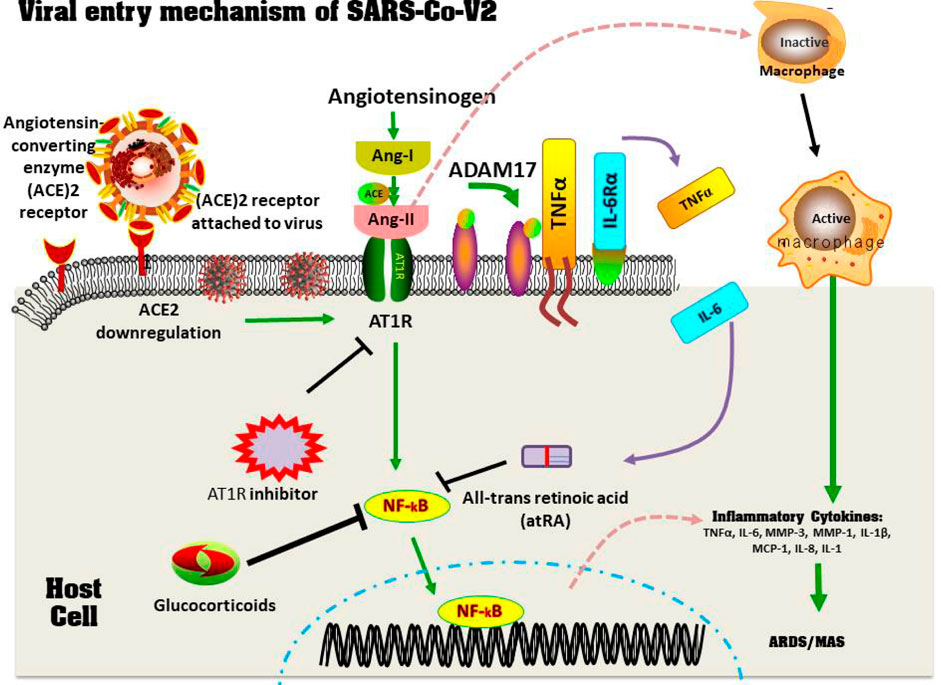
FIGURE 6. The emergence of MAS and ACE-2 Downregulation in a COVID-19 patients. Through the spike protein, the viruses accesses the host cell by connecting with the ACE-2 active site. Later, the virus suppresses ACE-2 impression, which causes Ang II to increase. Ang II is a RAS pathway product formed by the breakdown of Ang I by the enzyme ACE. Through NF-κB signaling, increased Ang-II binds with it's own receptor AT1R, modulating the gene expression of several inflammatory cytokine productions. This Ang-II/AT1R connection also affects macrophage responses, which results in the production of inflammatory cytokines productions, leading to ARDS or MAS. Furthermore, some metallo-proteases, such as ADAM17, convert these proinflammatory cytokines and ACE-2 receptors factors to a dissolved form, that aid inside the removal of surfaces ACE-2’s protective mechanism and that may contribute to SARS development. Therapy using glucocorticoids, AT1R blockers, as well as all-trans retinoic acid suppresses NF-κB regulation, which reduces cytokines storms and improves SARS-CoV2 disease severity (Based on Banu et al., 2020. Copyright. Elsevier 2020).
More intriguingly, the SARS-CoV spike protein suppresses the ACE-2 impression, resulting in an overproduction of Ang-II (the downstream of ACE-2) by the connected enzyme ACE (Imai et al., 2005). Likewise, SARS-CoV-2 would downregulate ACE-2 receptors, resulting in an overproduction of Ang-II, which could be a plausible explanation for the cytokine storms in SARS/COVID-19 (Catanzaro et al., 2020). SARS-CoV-2 occupies ACE-2 molecules on the cell surface, and Ang-II rises as a result of reduced ACE-2-mediated degradation (Catanzaro et al., 2020). The accumulating Ang-II stimulates the IL-6 amplifier through greater activation of the nuclear factor kappa B (NF-κB) system and then the IL-6/STAT3 (transcript class 3 signaling transducers and stimulators) trajectories and SARS. CoV-2 super-activates NF-κB via diagram recognition receptors (DPRs) (Hirano and Murakami, 2020). This strong positive correlation is reinforced by IL-6, which results in out-of-control cytokine release. More importantly, IL-6 is a key marker of cellular aging, and the age-related strengthening of the IL-6 amplifier could be linked to COVID-19 mortality (Figure 6) (Murakami et al., 2019; Hirano and Murakami, 2020).
Through the spike protein, the virus accesses the host cell by connecting with the ACE-2 active site. Later, the virus suppresses the ACE-2 impression, which causes Ang-II to increase. Ang-II is a RAS pathway product formed by the breakdown of Ang-I by the enzyme ACE. Through NF-κB signaling, increased Ang-II binds with its own receptor AT1R, modulating the gene expression of several inflammatory cytokine producers. This Ang II/AT1R interaction also influences the macrophage activation that in turn produces the inflammatory cytokines Thereby, inducing ARDS or MAS. Furthermore, some metalloproteases, such as ADAM17, convert these pro-inflammatory cytokines and ACE-2 receptor factors into a dissolved form that helps remove ACE-2’s surface protective mechanism and that may contribute to SARS development. Therapy using glucocorticoids, AT1R blockers, and all-trans retinoic acid suppresses NF-κB regulation, which reduces cytokine storms and improves SARS-CoV-2 disease severity (Banu et al., 2020).
3 Inhibiting cytokine storm using approved drugs are key in COVID-19
The SARS-CoV and MERS-CoV outbreaks should be used to gain significant information and insights into how to properly treat COVID-19 pneumonia patients. SARS-CoV-2 infection progresses through three stages: early infection, pulmonary phase, and hyper-inflammation phase (Siddiqi and Mehra, 2020). Targeted treatments are urgently needed to prevent cytokine storms, and the primary infection with minimal or moderate symptoms is the critical time for aggressive therapies to control subsequent degeneration (Table 1, Figure 7) (Siddiqi and Mehra, 2020).
Direct cell damage due to COVID-19 can be reduced with antiviral medications that block virus transmission and kill virus reproduction, whereas cytokine storms induced by the virus can be resisted with immunoregulatory therapies that inhibit hyper-activated inflammatory responses (Liu et al., 2020a). Several clinical trials started to look into possible treatments to manage a cytokine storm in COVID-19 cases, primarily focusing on direct cytokine inhibition and immunomodulatory medicines.
3.1 IL-6/IL-6R with different inhibitors
One meta-analysis of IL-6 concentration levels found that patients with complicated COVID-19 had 2.9-fold higher levels than those with uncomplicated disease (Coomes and Haghbayan, 2020). Another meta-analysis found a link between IL-6 levels and severity (Ulhaq and Soraya, 2020), indicating that the IL-6 level was a perfect predictor of poor prognosis in COVID-19 (Coomes and Haghbayan, 2020). In a number of ongoing COVID-19 clinical trials, IL-6/IL-6R inhibitors were employed preliminarily, and further inter-clinical trials are presently underway (Table 2; Figure 8). Tocilizumab (a type of IL-6 receptor inhibitor, IL-6R), first licensed to rheumatoid disorders, could successfully cure iatrogenic cytokine storms in patients with hematological malignancies produced by CAR-T therapy (Grupp et al., 2013).
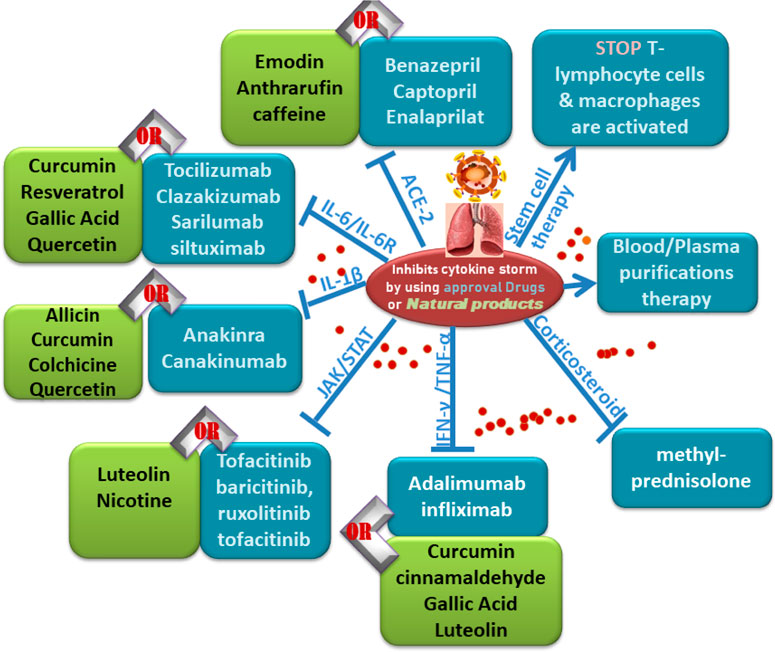
FIGURE 8. Follow charts: Different Inhibitors of cytokine storms by using approved drugs or natural products.
Tocilizumab improved fever control and respiratory functioning in 21 severe COVID-19 patients in a Chinese clinical study (ChiCTR2000029765). All individuals, including two critically ill people, started to recover and were released from the hospital (Xu et al., 2020). Roumier et al. (2020) presented that tocilizumab lowers compressive respiratory requirements (OR = 0.43) and the risk of subsequent ICU admissions (OR = 0.18) in 30 COVID-19 cases. Toniati et al. (2020) presented a single-center trial in Italy with a hundred patients with COVID-19, revealing that tocilizumab treatment was effective, long-lasting, and linked to important clinical improvement. A thorough review and meta-analysis of observational data found decreased mortality in COVID-19 patients receiving tocilizumab (Toniati et al., 2020). Furthermore, despite a delayed antiviral therapy induced by a higher initial viral load, IL-6 destruction with tocilizumab did not affect viral-specific antibody levels, showing tocilizumab’s safety for COVID-19 patients (Masiá et al., 2020).
Sarilumab is another type of IL-6R inhibitor studied in SARS-CoV-2, which could indicate a potential therapeutic advantage of early intervention with IL-6-modulatory COVID-19 treatments (Montesarchio et al., 2020). Clazakizumab is a monoclonal antibody against human IL-6. Several studies found that severely ill SARS/COVID-19 patients who took tocilizumab or sarilumab showed better results and decreased mortality rates (Gordon et al., 2021; Gupta et al., 2021). Conversely, other research on the efficacy of tocilizumab or sarilumab has produced mixed findings, with the medications failing to lower the risk of intubation or mortality in COVID-19 patients in many clinical trials (Lescure et al., 2021; Rosas et al., 2021). On 24 June 2021, tocilizumab was approved as a treatment for COVID-19 in hospitals. This decision was based on the outcomes of big clinical trials on tocilizumab (Horby et al., 2021). The EUA is only licensed for treating certain hospitalized cases already treated with corticosteroids and requiring respiratory assistance. The medicine is not approved as a general COVID-19 treatment.
Both tocilizumab and sarilumab enhanced survival in COVID-19-positive critically ill patients in the ICU (Gordon et al., 2021; Gupta et al., 2021). Furthermore, in hospitalized COVID-19 cases who required oxygen and had evidence of inflammation, tocilizumab reduced the mortality rate within 4 weeks by an average deviation of 4% compared to standard care; this finding was based on COVID-19 cases that required oxygen and had indications of inflammatory response. Tocilizumab reduced the time patients stayed in hospital care by 50% to approximately 57% (p = 0.00010) (Horby et al., 2021). The best evidence that tocilizumab treatment aids COVID-19 cases who are hospitalized was found by Hwang et al. (2022). The WHO also recommends tocilizumab and sarilumab in combination with corticosteroids to treat severe COVID-19. Tocilizumab used for the treatment of COVID-19 reduced the risk of death within 28 days by an absolute difference of 4% compared to normal care in hospitalized patients; this finding was from COVID-19 patients who required oxygen and had evidence of inflammation. Tocilizumab significantly reduced the time patients stayed in hospitals, increasing the probability of discharge within 28 days from 50% to 57% (p = 0.0001) (Horby et al., 2021). Hwang et al. (2022) offered the most conclusive evidence that tocilizumab medication helps hospitalized COVID-19 patients. In addition, tocilizumab and sarilumab, in combination with corticosteroids, are recommended by the WHO to treat severe COVID-19. According to the study (NCT04322188), patients with rapidly developing COVID-19 respiratory pneumonia who require supplemental oxygen may benefit from siltuximab (IL-6R monoclonal antibody) therapy due to lower death and chemokines hyper-inflammation in Figure 5.
3.2 Blocking of the IL-1β family
In cytokine storms, three IL-1 family cytokines are important (IL-18, IL-1β, and IL-33), with the blocking of IL-1β to prevent cytokine storms from being of particular relevance (Vardhana and Wolchok, 2020). The FDA and the European Drug Administration (EDA) have approved anakinra, an IL-1β receptor antagonist inhibiting IL-1α and IL-1β activity, for the treatment of rheumatism, young rheumatoid arthritis with the systemic onset, and familial Middle Eastern fever (Ben-Zvi et al., 2017). Anakinra could also be administered to treat infection-induced cytokine storms, which improves the survival rate of patients with severe sepsis (Shakoory et al., 2016). Anakinra has a shorter half-life than other cytokine blockers, making it safer and more appropriate for severe and critically ill patients.
According to a case series trial (NC-T04324021), large doses of injectable anakinra diminish inflammatory responses and are linked to progressive improvement in pulmonary function in severe COVID-19 sufferers (Cavalli et al., 2020). Other clinical trials testing anakinra’s usage in COVID-19 are currently ongoing (e.g., NCT-04330638, NCT-04341584, and NCT-04339712). Canakinumab, a monoclonal antibody that targets IL-1β specifically, was investigated as a COVID-19 therapy. Canakinumab was found to be safe, well-accepted, and associated with a rapid decrease in systemic inflammation and improvement in cardiac and respiratory functions (Shakoory et al., 2016). However, more randomized controlled trials are needed to increase the efficacy of anakinra and canakinumab usage in COVID-19 treatment.
3.3 JAK inhibitor
The Janus kinase/signal transducers and activators of the transcription (JAK/STAT) signaling system, which is a typical downstream effector journey for many cytokines, can inhibit many specific cytokines at the same time when JAK/STAT was inhibited (Peterson et al., 2020). Potential adverse effects, such as an increased risk of pulmonary edema (PE), elevated liver enzymes, hematological disorders, and a loss in antiviral response, must be considered (Peterson et al., 2020). Tofacitinib, baricitinib, and ruxolitinib are among the JAK inhibitors currently investigated to determine whether they can treat COVID-19 (e.g., NCT-04332042, NCT-04348695, NCT-04321993, and NCT-04348695) (Peterson et al., 2020). Baricitinib and ruxolitinib are specific inhibitors of JAK1/JAK2, a protein implicated in various cellular signals, including the pro-inflammatory IL-6, that function as immunomodulators by lowering cytotoxic T lymphocytes while increasing regulatory T cells (Modi et al., 2019; Zhang et al., 2020c).
All clinical and pulmonary function parameters improved significantly in the baricitinib-treated group compared to the baseline in a phase 2 and 3 clinical study (NCT-04358614), with no viral diseases or cardiac or hematologic side effects (Vardhana and Wolchok, 2020). Although tofacitinib is a specific JAK1/JAK3 inhibitor, it can be begun in patients who are not already using it and be continued in those using it during a pandemic (Jacobs et al., 2020).
3.4 IFN-γ inhibitor/TNF-α inhibitor
TNF-α is a cytokine that regulates IL-6 expression and is involved in various inflammatory disorders. In contrast to anti-IL-6 therapy, anti-TNF therapy was demonstrated to suppress the production of various inflammatory cytokines, including IL-6, IL-1β, and GM-CSF (Charles et al., 1999). Additionally, prior studies have found higher levels of TNF in the blood and tissues of COVID-19 patients (Del Valle et al., 2020). Anti-TNF-α medication has lately been proposed as a strategy for lowering inflammation in COVID-19 patients after IL-6 blocking showed little efficacy (Steeland et al., 2018). Anti-TNF-α drugs, such as adalimumab or infliximab, can reduce the death rate in COVID-19 sufferers, according to preliminary clinical data (Stallmach et al., 2020). Until recently, limited clinical trials have been conducted on infliximab (NCT-04344249, NCT-04425538, NCT-04593940, and NCT-04734678) and adalimumab (NCT-04705844) in COVID-19. In COVID-19, no clinical evidence or registered clinical trials have evaluated the possibility of IL-18 and IL-33 blockers. TNF-a and IFN-γ are also important inflammatory cytokines that provide good targets for cytokine storm control [69], and clinical trials to evaluate these blockers in COVID-19 (ChiCTR-2000030089 and NCT-04324021) are continuing (Blaszczak et al., 2020; Cavalli et al., 2020).
3.5 Corticosteroid hormonal therapy
Corticosteroids, a kind of steroidal hormone, inhibit histone acetyltransferase (HAT) activity and recruit histone deacetylases-2 (HDAC-2) activity to downregulate inflammatory genes by interacting with the cytoplasmic corticosteroid receptor (Darwish et al., 2011). Corticosteroids are routinely used to control cytokine storms as a result. According to data from a short trial (Yang et al., 2020b), early administration of low or medium doses of methylprednisolone had a beneficial effect on sick people with severe COVID-19. In Iran, a single-blind, randomized, controlled trial (IRCT-20200404046947N1) found that the methyl-prednisolone pulse could be an effective treatment for COVID-19 patients in the pulmonary phase who were hospitalized (Edalatifard et al., 2020). For the treatment of severe cases, clinicians must master the time and dose of corticosteroids, mainly before infection occurs. Patients who already have hypoxemia for various reasons or who take glucocorticoids on a daily basis for other chronic diseases should be treated with the utmost caution (Ji et al., 2020; Nelson et al., 2021).
3.6 Blood/plasma purification therapy
Blood/serum filtration systems, such as serum exchange, adsorption, perfusion, and blood/plasma purification, eliminate inflammatory components and thereby minimize tissue damage caused by hyper-activated inflammation mediators (Ji et al., 2020). Li’s team presented a technique that artificially purifies the blood in the liver and could quickly eliminate inflammatory responses; stop cytokine storm; favor fluid, electrolyte, and acid/base homeostasis; and improve therapeutic efficacy in critical patients (Zhang et al., 2021). In a limited experience, Ma et al. (2020) found that three cases of COVID-19 that had serum/plasma purification therapy were effective and tolerable. One case report (Wang and Hu, 2020) described the successful recovery of a severely ill patient. Based on previous experiences with SARS and MERS, Yang and his team developed a blood (serum/plasma) purification protocol for patients with COVID-19 sufferers that included four main steps: 1) deciding whether chronic COVID-19 patients need blood purification; 2) prescribing a plasma purification treatment for COVID-19 patients; 3) evaluating and adjusting blood purification measures; and 4) determining when blood purification should be stopped (Liu et al., 2020b; Yang et al., 2020c). Finally, to ensure a safe and efficient treatment, probable side effects of blood-purifying therapy (e.g., thrombocytosis, allergies, hemorrhage, and air edema) must be identified and handled as soon as feasible (Liu et al., 2020b).
3.7 Stem cell therapy
Mesenchymal MSCs also prevent T lymphocyte cells and macrophages from becoming abnormally activated and encourage their development into regulatory T cells and macrophages anti-inflammatory (Yagi et al., 2010). MSCs reduce the development of cytokine storms by inhibiting the release of pro-inflammatory cytokines (Yagi et al., 2010). Many clinical trials involving intravenous MSC delivery in COVID-19 patients were officially filed (www.clinicaltrials.gov). However, most of them are still recruiting participants. Zhao’s team recently published the outcomes of seven chronic and critically sick COVID-19 patients who had MSC transplantation therapy, demonstrating improved prognosis and successful cytokine storm avoidance with no apparent side effects (Leng et al., 2020). Other stem cell therapies, such as stem cells generated from human menstrual blood (ChiCTR-2000029606) and embryo stem cells, are also in clinical testing (ChiCTR-2000031139). Not only can stem cells (MSCs) self-renew and differentiate in multiple directions, but also they have anti-inflammatory and immune-regulatory properties (Orleans et al., 2020).
4 Natural immunosuppressants as adjuvants in managing COVID-19 to target the cytokine storm
With no specific antiviral drugs to cure the virus and no vaccination to stop it, the COVID-19 outbreak generated a worldwide health crisis with massive side effects. Although some people infected with SARS-CoV-2 had a well-coordinated immune response and recovered, others had a defective immune response that led to significant consequences such as ARDS, sepsis, and MOF, all linked to increased death and disability.
According to previous studies, a huge cytokine/chemokine syndrome release dubbed “cytokine storms” was discovered in patients with a malfunctioning immune response. As a result, these patients had greater levels of pro-inflammatory/modulatory cytokines (e.g., IL-β1, IL-2, IL-4, IL-6, IL-7, IL-9, IL-10, IL-12, IL-13, IL-17, TNF-α, INF-ℽ, G-CSF, GM-CSF, M-CSF, and HGF) and chemokines (CXCL-8, MCP-1, IP10, MIP-1, and MIP).
Immunomodulatory activities have long existed in natural products, crude extracts, and metabolites. Additionally, the ability of several plant-derived bioactive constituents to reduce the cytokine storms associated with inflammatory disease has been investigated. The CSS seen in COVID-19 sufferers is briefly discussed in this review, with an emphasis on several natural-immunosuppressant drugs obtained from plant extracts that can help reduce the cytokine storms seen in severe COVID-19 sufferers (Figure 9; Table 3).
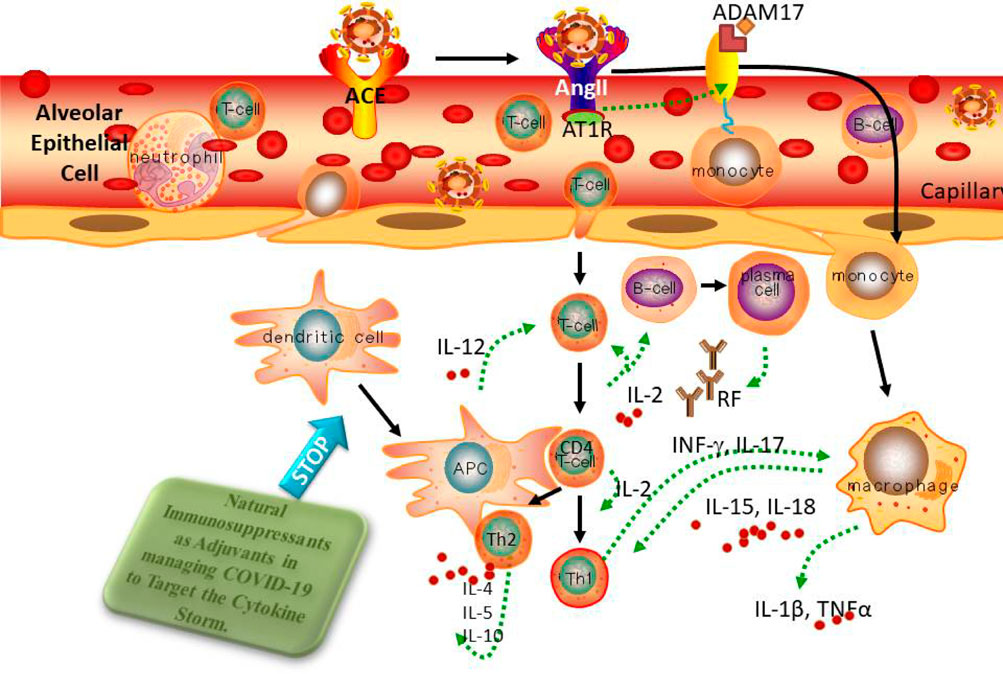
FIGURE 9. Blocking the cytokine storm is a unique, potential natural treatment technique for reducing the excessive influx of cytokines seen at the infected site.
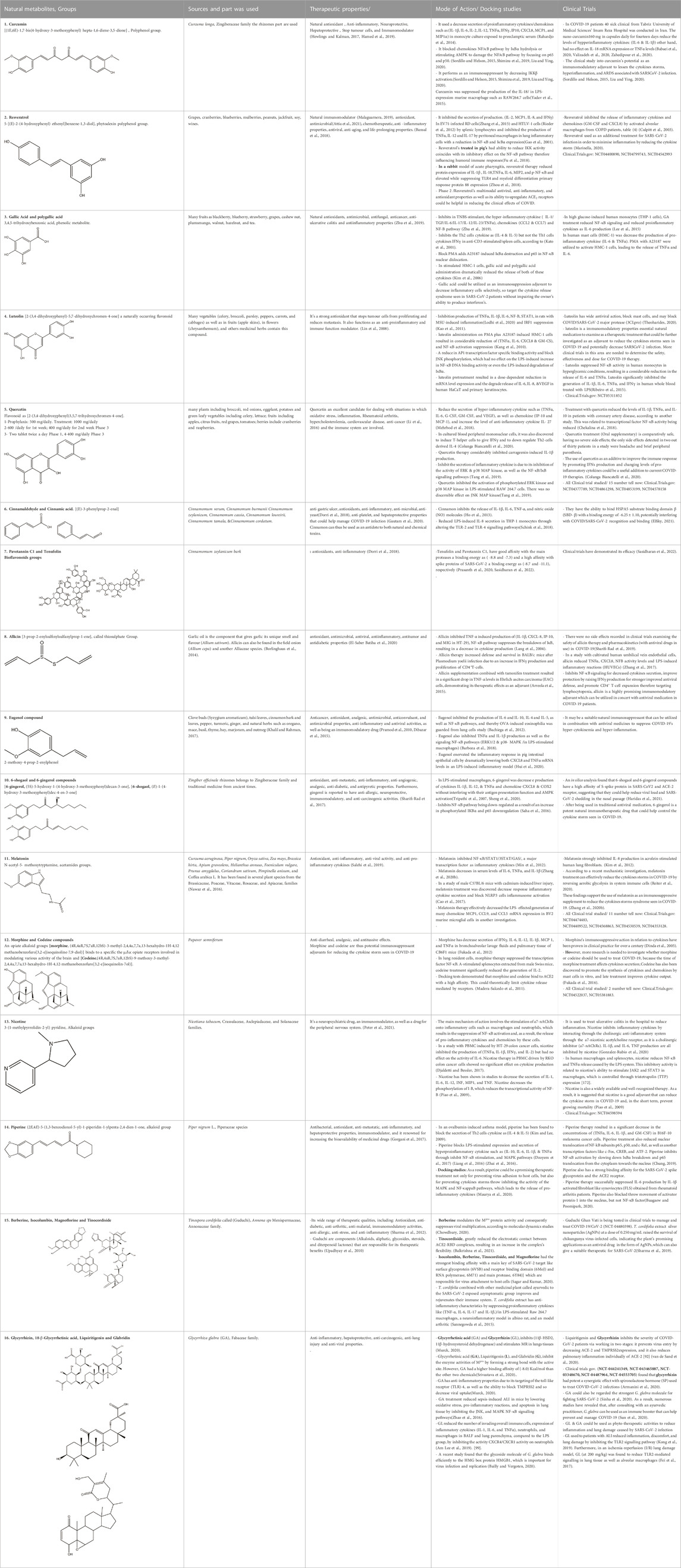
Table 3. Chemical structure of Natural compound list as Immunosuppressants as Adjuvants in managing COVID-19 to Target the Cytokine Storm. The remainder of this table can be found in the Supplementary Materisl.
Curcumin, piperine, luteolin, phenylnaphthalene, withanolides, anonaine, resveratrol, emodin, anthrarufin, and caffeine are natural immunomodulatory compounds obtained from plant sources known to limit the generation and secretion of inflammatory cytokines/chemokines (Hamed et al., 2019; El-Gengaihi et al., 2020a; Rolta et al., 2021a; Rolta et al., 2021b; Mohammed et al., 2022b; Mohammed et al., 2022c; Rolta et al., 2022). This inhibitory impact is mediated through analysis and planning involved in the generation and release of cytokines and chemokines (e.g., NF-κB, JAK/STAT, and MAPK/ERK). In the combined effect of antiviral medications and other therapeutic drugs now in use, the use of these natural immunosuppressive drugs as adjuvants to reduce the cytokine storms presents a novel, synergistic strategy for the therapies and effective recovery of COVID-19 patients. The effects of luteolin on TNF-induced phosphorylation, nuclear translocation, and DNA binding of NF-κB were significantly decreased. The TNF-induced expression level of the NFKB1 and RELA genes, which encode the two NF-κB subunits (p50 and p65, respectively), was also reduced by luteolin treatment, suggesting that luteolin suppresses pro-inflammatory cytokine production through decreasing NF-κB induction (Kaur et al., 2015).
Although the benefits of these synthetic therapeutic immunomodulatory drugs are undeniable, they are typically coupled with negative side effects. With chloroquine and hydroxychloroquine treatment, cardiomyopathy, neurological, and gastrointestinal side effects have been observed, and because COVID-19 patients are more sensitive due to co-morbidities, there are worries about safety (Bull-Otterson et al., 2020; Colafrancesco et al., 2020; Gevers et al., 2020). Therapies for COVID-19 patients using IL-6 receptor antagonists, such as sarilumab and tocilizumab drugs, which have been linked to endocrinological, hematological, gastrointestinal, and cardiovascular side effects, raise safety concerns (Atal and Fatima, 2020). The side effects of IL-2 inhibitors of cyclosporine and tacrolimus are well-known and can be quite dangerous (Cure et al., 2020). IL-1 blockers, such as anakinra and canakinumab, have a relatively favorable safety profile. However, they can cause elevated liver enzyme levels, myopathy, and moderate leukopenia (Table 2) (Colafrancesco et al., 2020). Anti-TNF therapy side effects have been described infrequently (Caso et al., 2020). Ruxolitinib, a JAK/STAT inhibitor, was delivered to participants with COVID-19 and was shown to cause severe medication responses, causing the study’s early termination (Esposito et al., 2011). With the administration of another oral JAK/STAT inhibitor, baricitinib, there is a risk of severe infections.
Statin treatment in patients with ARDS and sepsis has yielded unsatisfactory results, leading to skepticism about using them as COVID-19 therapy adjuncts. Hypernatremia and dysphagia were observed as side effects in a study of rhACE2 medication for patients with ARDS (Barone et al., 2020). There is a risk of lethal unfavorable thrombotic complications and reactions if MSCs are administered incorrectly, demanding a complete comprehension of the option and application routes of this therapy (Moll et al., 2020).
5 Conclusion
COVID/SARS-CoV-2 has sparked widespread alarm and, in a short time, has become a pandemic. Scientists from all over the world are working around the clock to develop efficient medications to combat this unusual viral disease. We provided an overview of the pharmacological targets found in COVID-CoV-2 in this brief analysis. We summarized the single-target and multi-target techniques in depth in addition to the target present. It is conceivable to deduce from the findings of many studies that the multi-target method is preferable to the single-target approach. Although very few studies are available, the multi-target strategy is steadily gaining traction in the field of drug discovery. We also discussed the medications currently in clinical trials and their inhibitory mechanisms near the end of this review. Numerous vaccine studies are receiving greater attention in clinical studies than drug therapy trials. The extensive study offered here may aid in understanding the many pharmacological targets and the multi-target strategy against SARS-CoV-2. The multi-target technique, while promising, has a different risk-benefit profile. Inter-medication has a substantial risk of hazard because unwanted targeting and targeting of host homolog proteins can have negative consequences. Compounds that are big and do not conform to Lipinski’s rule are harmful to the host and have higher drug accumulation and less bioavailability.
As a result, the identification of safe and effective COVID-19 treatments with minimal side effects is critical. Several in silico analyses have revealed that natural chemicals derived from plants could be efficient antiviral agents against SARS-CoV-2. Plants produce a staggering array of natural chemicals, many of which have enormous medicinal promise. However, a lack of understanding of natural compound mechanisms of action is a flaw that effectively hinders medical practitioners from accepting plants as phytotherapy. For the prevention and management of COVID-19, the Indian government’s Ministries of AYUSH recently proposed various immunity-boosting methods based on Ayurveda (the oldest healing science, dating back to 5000 years). The WHO recently recommended traditional ancient herbs in the therapies for COVID-19-related health problems that can be used. We have discussed some of the most potent phytotherapeutics present in traditional natural medicines that doctors can use as therapeutic agents to decrease viral infection and toxicity associated with SARS-CoV-2 in this review. We also provided guidance on how to better understand the value of natural plants and how to employ them to strengthen the host’s antiviral defenses.
Author contributions
The author confirms being the sole contributor to this work and has approved it for publication.
Acknowledgments
The author would like to express her great thanks and appreciation to the National Research Centre, Dokki, Egypt, for providing all facilities for this work.
Conflict of interest
The author declares that the research was conducted in the absence of any commercial or financial relationships that could be construed as a potential conflict of interest.
Publisher’s note
All claims expressed in this article are solely those of the authors and do not necessarily represent those of their affiliated organizations or those of the publisher, the editors, and the reviewers. Any product that may be evaluated in this article, or claim that may be made by its manufacturer, is not guaranteed or endorsed by the publisher.
Supplementary material
The Supplementary Material for this article can be found online at: https://www.frontiersin.org/articles/10.3389/fphar.2023.1111329/full#supplementary-material
References
Ahmad, J., Khan, I., Blundell, R., Azzopardi, J., and Mahomoodally, M. F. (2020). Stevia rebaudiana Bertoni.: An updated review of its health benefits, industrial applications and safety. Trends Food Sci. Technol. 100, 177–189. doi:10.1016/j.tifs.2020.04.030
al-Kuraishy, , al-Fakhrany, , Elekhnawy, E., al-Gareeb, A. I., Alorabi, M., de Waard, M., et al. (2022). Traditional herbs against COVID-19: Back to old weapons to combat the new pandemic. Eur. J. Med. Res. 27, 186. doi:10.1186/s40001-022-00818-5
Am Lee, S., Lee, S. H., Kim, J. Y., and Lee, W. S. (2019). Effects of glycyrrhizin on lipopolysaccharide-induced acute lung injury in a mouse model. J. Thorac. Dis. 11, 1287–1302. doi:10.21037/jtd.2019.04.14
Armanini, D., Fiore, C., Bielenberg, J., Sabbadin, C., and Bordin, L. (2020). Coronavirus-19: Possible therapeutic implications of spironolactone and dry extract of Glycyrrhiza glabra L.(Licorice). Front. Pharmacol. 11, 558418. doi:10.3389/fphar.2020.558418
Arreola, R., Quintero-FabiáN, S., LóPEZ-Roa, R. I., Flores-Gutierrez, E. O., Reyes-Grajeda, J. P., Carrera-Quintanar, L., et al. (2015). Immunomodulation and anti-inflammatory effects of garlic compounds. J. Immunol. Res., 2015, 401630, doi:10.1155/2015/401630
Atal, S., and Fatima, Z. (2020). IL-6 inhibitors in the treatment of serious COVID-19: A promising therapy? Pharm. Med. 34, 223–231. doi:10.1007/s40290-020-00342-z
Attia, G. H., Marrez, D. A., Mohammed, M. A., Albarqi, H. A., Ibrahim, A. M., and Raey, M. A. E. (2021). Synergistic effect of Mandarin peels and hesperidin with sodium nitrite against some food pathogen microbes. Molecules 26, 3186. doi:10.3390/molecules26113186
Babaei, F., Nassiri-Asl, M., and Hosseinzadeh, H. (2020). Curcumin (a constituent of turmeric): New treatment option against COVID-19. Food Sci. Nutr. 8, 5215–5227. doi:10.1002/fsn3.1858
Bachiega, T. F., de Sousa, J. P. B., Bastos, J. K., and Sforcin, J. M. (2012). Clove and eugenol in noncytotoxic concentrations exert immunomodulatory/anti-inflammatory action on cytokine production by murine macrophages. J. Pharm. Pharmacol. 64, 610–616. doi:10.1111/j.2042-7158.2011.01440.x
Bailly, C., and Vergoten, G. (2020). Glycyrrhizin: An alternative drug for the treatment of COVID-19 infection and the associated respiratory syndrome? Pharmacol. Ther. 214, 107618. doi:10.1016/j.pharmthera.2020.107618
Balkrishna, A., Pokhrel, S., Singh, J., and Varshney, A. 2020. Withanone from Withania somnifera may inhibit novel coronavirus (COVID-19) entry by disrupting interactions between viral S-protein receptor binding domain and host ACE2 receptor.
Balkrishna, A., Pokhrel, S., and Varshney, A. (2021). Tinocordiside from Tinospora cordifolia (Giloy) may curb SARS-CoV-2 contagion by disrupting the electrostatic interactions between host ACE2 and viral S-protein receptor binding domain. Comb. Chem. High Throughput Screen. 24, 1795–1802. doi:10.2174/1386207323666201110152615
Banerjee, A., Czinn, S. J., Reiter, R. J., and Blanchard, T. G. (2020). Crosstalk between endoplasmic reticulum stress and anti-viral activities: A novel therapeutic target for COVID-19. Life Sci. 255, 117842. doi:10.1016/j.lfs.2020.117842
Bansal, M., Singh, N., Pal, S., Dev, I., and Ansari, K. M. (2018). Chemopreventive role of dietary phytochemicals in colorectal cancer. Adv. Mol. Toxicol. 12, 69–121.
Banu, N., Panikar, S. S., Leal, L. R., and Leal, A. R. (2020). Protective role of ACE2 and its downregulation in SARS-CoV-2 infection leading to macrophage activation syndrome: Therapeutic implications. Life Sci. 256, 117905. doi:10.1016/j.lfs.2020.117905
Barboza, J. N., Da Silva Maia Bezerra Filho, C., Silva, R. O., Medeiros, J. V. R., and de Sousa, D. P. (2018). An overview on the anti-inflammatory potential and antioxidant profile of eugenol. Oxidative Med. Cell. Longev.
Barone, M., Ucciferri, C., Cipollone, G., and Mucilli, F. (2020). Recombinant human angiotensin-converting enzyme 2 and COVID-19 acute respiratory distress syndrome: A theoretical or a real resource. Ejmo 4, 139–140. doi:10.14744/ejmo.2020.47992
Ben-Zvi, I., Kukuy, O., Giat, E., Pras, E., Feld, O., Kivity, S., et al. (2017). Anakinra for colchicine-resistant familial mediterranean fever: A randomized, double-blind, placebo-controlled trial. Arthritis & Rheumatology 69, 854–862. doi:10.1002/art.39995
Bergsbaken, T., Fink, S. L., and Cookson, B. T. (2009). Pyroptosis: Host cell death and inflammation. Nat. Rev. Microbiol. 7, 99–109. doi:10.1038/nrmicro2070
Blaszczak, A., Trinidad, J. C., and Cartron, A. M. (2020). Adalimumab for treatment of hidradenitis suppurativa during the COVID-19 pandemic: Safety considerations. J. Am. Acad. Dermatology 83, e31. doi:10.1016/j.jaad.2020.04.030
Bogoch, I. I., Watts, A., Thomas-Bachli, A., Huber, C., Kraemer, M. U., and Khan, K. (2020). Potential for global spread of a novel coronavirus from China. J. travel Med. 27, taaa011. taaa011. doi:10.1093/jtm/taaa011
Borlinghaus, J., Albrecht, F., Gruhlke, M. C., Nwachukwu, I. D., and Slusarenko, A. J. (2014). Allicin: Chemistry and biological properties. Molecules 19, 12591–12618. doi:10.3390/molecules190812591
Buagaew, A., and Poomipark, N. (2020). Protective effect of piperine from Piper chaba fruits on LPS-induced inflammation in human intestinal cell line. J. Med. Plants Res. 14, 438–444. doi:10.5897/jmpr2020.6996
Bull-Otterson, L., Gray, E. B., Budnitz, D. S., Strosnider, H. M., Schieber, L. Z., Courtney, J., et al. (2020). Hydroxychloroquine and chloroquine prescribing patterns by provider specialty following initial reports of potential benefit for COVID-19 treatment—United States, january–june 2020. Morb. Mortal. Wkly. Rep. 69, 1210–1215. doi:10.15585/mmwr.mm6935a4
Cabler, S. S., French, A. R., and Orvedahl, A. (2020). A cytokine circus with a viral ringleader: SARS-CoV-2-associated cytokine storm syndromes. Trends Mol. Med. 26, 1078–1085. doi:10.1016/j.molmed.2020.09.012
Cao, Z., Fang, Y., Lu, Y., Tan, D., du, C., Li, Y., et al. (2017). Melatonin alleviates cadmium-induced liver injury by inhibiting the TXNIP-NLRP3 inflammasome. J. Pineal Res. 62, e12389. doi:10.1111/jpi.12389
Caso, F., Costa, L., Ruscitti, P., Navarini, L., Del Puente, A., Giacomelli, R., et al. (2020). Could Sars-coronavirus-2 trigger autoimmune and/or autoinflammatory mechanisms in genetically predisposed subjects? Autoimmun. Rev. 19, 102524. doi:10.1016/j.autrev.2020.102524
Catanzaro, M., Fagiani, F., Racchi, M., Corsini, E., Govoni, S., and Lanni, C. (2020). Immune response in COVID-19: Addressing a pharmacological challenge by targeting pathways triggered by SARS-CoV-2. Signal Transduct. Target. Ther. 5, 84–10. doi:10.1038/s41392-020-0191-1
Cavalli, G., de Luca, G., Campochiaro, C., della-Torre, E., Ripa, M., Canetti, D., et al. (2020). Interleukin-1 blockade with high-dose anakinra in patients with COVID-19, acute respiratory distress syndrome, and hyperinflammation: A retrospective cohort study. Lancet Rheumatology 2, e325–e331. doi:10.1016/S2665-9913(20)30127-2
Charles, P., Elliott, M. J., Davis, D., Potter, A., Kalden, J. R., Antoni, C., et al. (1999). Regulation of cytokines, cytokine inhibitors, and acute-phase proteins following anti-TNF-α therapy in rheumatoid arthritis. J. Immunol. 163, 1521–1528. doi:10.4049/jimmunol.163.3.1521
Chekalina, N., Burmak, Y., Petrov, Y., Borisova, Z., Manusha, Y., Kazakov, Y., et al. (2018). Quercetin reduces the transcriptional activity of NF-kB in stable coronary artery disease. Indian Heart J. 70, 593–597. doi:10.1016/j.ihj.2018.04.006
Chen, L.-X., He, H., and Qiu, F. (2011). Natural withanolides: An overview. Nat. Product. Rep. 28, 705–740. doi:10.1039/c0np00045k
Chen, X., Kang, Y., Luo, J., Pang, K., Xu, X., Wu, J., et al. (2021). Next-generation sequencing reveals the progression of COVID-19. Front. Cell. Infect. Microbiol. 11, 632490. doi:10.3389/fcimb.2021.632490
Chourasia, M., Koppula, P. R., Battu, A., Ouseph, M. M., and Singh, A. K. (2021). EGCG, a green tea catechin, as a potential therapeutic agent for symptomatic and asymptomatic SARS-CoV-2 infection. Molecules 26, 1200. doi:10.3390/molecules26051200
Chousterman, B. G., Swirski, F. K., and Weber, G. F. (2017). Cytokine storm and sepsis disease pathogenesis. In Seminars in immunopathology. Springer, 517–528.
Chowdhury, P. (2020). In silico investigation of phytoconstituents from Indian medicinal herb ‘Tinospora cordifolia (giloy)’against SARS-CoV-2 (COVID-19) by molecular dynamics approach. J. Biomol. Struct. Dyn. 39, 6792–6809. doi:10.1080/07391102.2020.1803968
Chugh, A., Sehgal, I., Khurana, N., Verma, K., Rolta, R., Vats, P., et al. (2023). Comparative docking studies of drugs and phytocompounds for emerging variants of SARS-CoV-2. 3 Biotech. 13, 36. doi:10.1007/s13205-022-03450-6
Chung, H. Y. (2019). Anti-inflammatory and antioxidant activities of piperine on t. BHP-induced Ac2F cells.
Ciampi, E., Uribe-San-Martin, R., CáRCAMO, C., Cruz, J. P., Reyes, A., Reyes, D., et al. (2020). Efficacy of andrographolide in not active progressive multiple sclerosis: A prospective exploratory double-blind, parallel-group, randomized, placebo-controlled trial. BMC Neurol. 20, 1–10. doi:10.1186/s12883-020-01745-w
Colafrancesco, S., Scrivo, R., Barbati, C., Conti, F., and Priori, R. (2020). Targeting the immune system for pulmonary inflammation and cardiovascular complications in COVID-19 patients. Front. Immunol. 11, 1439. doi:10.3389/fimmu.2020.01439
Colunga Biancatelli, R. M. L., Berrill, M., Catravas, J. D., and Marik, P. E. (2020). Quercetin and vitamin C: An experimental, synergistic therapy for the prevention and treatment of SARS-CoV-2 related disease (COVID-19). Front. Immunol. 11, 1451. doi:10.3389/fimmu.2020.01451
Coomes, E. A., and Haghbayan, H. (2020). Interleukin-6 in COVID-19: A systematic review and meta-analysis. Rev. Med. virology 30, 1–9. doi:10.1002/rmv.2141
Cosenza, M., Sacchi, S., and Pozzi, S. (2021). Cytokine release syndrome associated with T-cell-based therapies for hematological malignancies: Pathophysiology, clinical presentation, and treatment. Int. J. Mol. Sci. 22, 7652. doi:10.3390/ijms22147652
Culpitt, S., Rogers, D., Fenwick, P., Shah, P., de Matos, C., Russell, R., et al. (2003). Inhibition by red wine extract, resveratrol, of cytokine release by alveolar macrophages in COPD. Thorax 58, 942–946. doi:10.1136/thorax.58.11.942
Cure, E., Kucuk, A., and Cure, M. C. (2020). Cyclosporine therapy in cytokine storm due to coronavirus disease 2019 (COVID-19). Rheumatol. Int. 1, 1177–1179. doi:10.1007/s00296-020-04603-7
Darwish, I., Mubareka, S., and Liles, W. C. (2011). Immunomodulatory therapy for severe influenza. Expert Rev. anti-infective Ther. 9, 807–822. doi:10.1586/eri.11.56
Datta, P. K., Liu, F., Fischer, T., Rappaport, J., and Qin, X. (2020). SARS-CoV-2 pandemic and research gaps: Understanding SARS-CoV-2 interaction with the ACE2 receptor and implications for therapy. Theranostics 10, 7448–7464. doi:10.7150/thno.48076
Dawood, F. S., Iuliano, A. D., Reed, C., Meltzer, M. I., Shay, D. K., Cheng, P.-Y., et al. (2012). Estimated global mortality associated with the first 12 months of 2009 pandemic influenza A H1N1 virus circulation: A modelling study. Lancet Infect. Dis. 12, 687–695. doi:10.1016/S1473-3099(12)70121-4
Deftereos, S. G., Giannopoulos, G., Vrachatis, D. A., Siasos, G. D., Giotaki, S. G., Gargalianos, P., et al. (2020). Effect of colchicine vs standard care on cardiac and inflammatory biomarkers and clinical outcomes in patients hospitalized with coronavirus disease 2019: The GRECCO-19 randomized clinical trial. JAMA Netw. open 3, e2013136. doi:10.1001/jamanetworkopen.2020.13136
Del Valle, D. M., Kim-Schulze, S., Huang, H.-H., Beckmann, N. D., Nirenberg, S., Wang, B., et al. (2020). An inflammatory cytokine signature predicts COVID-19 severity and survival. Nat. Med. 26, 1636–1643. doi:10.1038/s41591-020-1051-9
Dibazar, S. P., Fateh, S., and Daneshmandi, S. (2015). Immunomodulatory effects of clove (syzygium aromaticum) constituents on macrophages: In vitro evaluations of aqueous and ethanolic components. J. Immunotoxicol. 12, 124–131. doi:10.3109/1547691X.2014.912698
Dinda, A., Gitman, M., and Singhal, P. C. (2005). Immunomodulatory effect of morphine: Therapeutic implications. Expert Opin. drug Saf. 4, 669–675. doi:10.1517/14740338.4.4.669
Djaldetti, M., and Bessler, H. (2017). Nicotine modifies cytokine production by human mononuclears stimulated by colon cancer cells. Colorectal Canc 3.
Dorri, M., Hashemitabar, S., and Hosseinzadeh, H. (2018). Cinnamon (cinnamomum zeylanicum) as an antidote or a protective agent against natural or chemical toxicities: A review. Drug Chem. Toxicol. 41, 338–351. doi:10.1080/01480545.2017.1417995
Dzoyem, J., Mcgaw, L., Kuete, V., and Bakowsky, U. (2017). Anti-inflammatory and anti-nociceptive activities of African medicinal spices and vegetables. Medicinal spices and vegetables from Africa. Elsevier.
Edalatifard, M., Akhtari, M., Salehi, M., Naderi, Z., Jamshidi, A., Mostafaei, S., et al. (2020). Intravenous methylprednisolone pulse as a treatment for hospitalised severe COVID-19 patients: Results from a randomised controlled clinical trial. Eur. Respir. J. 56, 2002808. doi:10.1183/13993003.02808-2020
el-Gengaihi, S. E., Aboul-Enein, A. M., and Mohammed, M. A. (2020a). Antiproliferative effect and chemical constituents of annona species. Plant Arch. 20, 2650–2657.
el-Gengaihi, S. E., Hamed, M. A., Khalaf-Allah, A. E.-R. M., and Mohammed, M. A. (2013). Golden berry juice attenuates the severity of hepatorenal injury. J. Diet. Suppl. 10, 357–369. doi:10.3109/19390211.2013.830675
el-Gengaihi, S. E., Mohammed, M. A., Aboubaker, D., Shoaib, R. M., Asker, M., Abdelhamid, S., et al. (2020b). Chemical, biological, and molecular studies on different citrus species wastes. Plant Arch. 20, 2773–2782.
el-Saber Batiha, G., Magdy Beshbishy, A., G Wasef, L., Elewa, Y. H., A al-Sagan, A., el-Hack, A., et al. (2020). Chemical constituents and pharmacological activities of garlic (allium sativum L.): A review. Nutrients 12, 872. doi:10.3390/nu12030872
Elfiky, A. A. (2021). Natural products may interfere with SARS-CoV-2 attachment to the host cell. J. Biomol. Struct. Dyn. 39, 3194–3203. doi:10.1080/07391102.2020.1761881
Enmozhi, S. K., Raja, K., Sebastine, I., and Joseph, J. (2021). Andrographolide as a potential inhibitor of SARS-CoV-2 main protease: An in silico approach. J. Biomol. Struct. Dyn. 39, 3092–3098. doi:10.1080/07391102.2020.1760136
Esposito, S., Tagliabue, C., Bosis, S., and Principi, N. (2011). Levofloxacin for the treatment of Mycoplasma pneumoniae-associated meningoencephalitis in childhood. Int. J. Antimicrob. agents 37, 472–475. doi:10.1016/j.ijantimicag.2011.01.008
Fehr, A. R., Channappanavar, R., and Perlman, S. (2017). Middle East respiratory syndrome: Emergence of a pathogenic human coronavirus. Annu. Rev. Med. 68, 387–399. doi:10.1146/annurev-med-051215-031152
Fehr, A. R., and Perlman, S. (2015). Coronaviruses: An overview of their replication and pathogenesis. Coronaviruses 1282, 1–23. doi:10.1007/978-1-4939-2438-7_1
Fei, L., Jifeng, F., Tiantian, W., Yi, H., and Linghui, P. (2017). Glycyrrhizin ameliorate ischemia reperfusion lung injury through downregulate TLR2 signaling cascade in alveolar macrophages. Front. Pharmacol. 8, 389. doi:10.3389/fphar.2017.00389
Fu, Q., Cui, Q., Yang, Y., Zhao, X., Song, X., Wang, G., et al. (2018). Effect of resveratrol dry suspension on immune function of piglets. Evidence-Based Complementary Altern. Med. 2018, 1, 10. doi:10.1155/2018/5952707
Fukada, T., Kato, H., Ozaki, M., and Yagi, J. (2016). Impact of the timing of morphine administration on lipopolysaccharide-mediated lethal endotoxic shock in mice. Shock Inj. Inflamm. Sepsis Laboratory Clin. Approaches 45, 564–569. doi:10.1097/SHK.0000000000000541
Fukada, T., Nakayama, R., Iwakiri, H., Kato, H., Yagi, J., and Ozaki, M. (2012). Opioids inhibit lipopolysaccharide-mediated lethal shock in mice.
Gao, X., Xu, Y. X., Janakiraman, N., Chapman, R. A., and Gautam, S. C. (2001). Immunomodulatory activity of resveratrol: Suppression of lymphocyte proliferation, development of cell-mediated cytotoxicity, and cytokine production. Biochem. Pharmacol. 62, 1299–1308. doi:10.1016/s0006-2952(01)00775-4
Gautam, S., Gautam, A., Chhetri, S., and Bhattarai, U. (2020). Immunity against COVID-19: Potential role of ayush kwath. J. Ayurveda Integr. Med. 13, 100350. doi:10.1016/j.jaim.2020.08.003
Gevers, S., Kwa, M., Wijnans, E., and van Nieuwkoop, C. (2020). Safety considerations for chloroquine and hydroxychloroquine in the treatment of COVID-19. Clin. Microbiol. Infect. 26, 1276–1277. doi:10.1016/j.cmi.2020.05.006
GóMEZ-Pastora, J., Weigand, M., Kim, J., Wu, X., Strayer, J., Palmer, A. F., et al. (2020). Hyperferritinemia in critically ill COVID-19 patients–is ferritin the product of inflammation or a pathogenic mediator? Clin. Chimica Acta; Int. J. Clin. Chem. 509, 249–251. doi:10.1016/j.cca.2020.06.033
Gonzalez-Rubio, J., Navarro-Lopez, C., Lopez-Najera, E., Lopez-Najera, A., Jimenez-Diaz, L., Navarro-Lopez, J. D., et al. (2020). Cytokine release syndrome (CRS) and nicotine in COVID-19 patients: Trying to calm the storm. Front. Immunol. 11, 1359. doi:10.3389/fimmu.2020.01359
Gordon, A. C., Mouncey, P. R., al-Beidh, F., Rowan, K. M., Nichol, A. D., Arabi, Y. M., et al. (2021). Interleukin-6 receptor antagonists in critically ill patients with Covid-19. N. Engl. J. Med. 384, 1491–1502. doi:10.1056/NEJMoa2100433
Gorgani, L., Mohammadi, M., Najafpour, G. D., and Nikzad, M. (2017). Piperine—the bioactive compound of black pepper: From isolation to medicinal formulations. Compr. Rev. Food Sci. Food Saf. 16, 124–140. doi:10.1111/1541-4337.12246
Grupp, S. A., Kalos, M., Barrett, D., Aplenc, R., Porter, D. L., Rheingold, S. R., et al. (2013). Chimeric antigen receptor–modified T cells for acute lymphoid leukemia. N. Engl. J. Med. 368, 1509–1518. doi:10.1056/NEJMoa1215134
Guan, W.-J., Liang, W.-H., Zhao, Y., Liang, H.-R., Chen, Z.-S., Li, Y.-M., et al. (2020). Comorbidity and its impact on 1590 patients with COVID-19 in China: A nationwide analysis. Eur. Respir. J. 55, 2001227. doi:10.1183/13993003.01227-2020
Gupta, M., and Kaur, G. (2019). Withania somnifera (L.) Dunal ameliorates neurodegeneration and cognitive impairments associated with systemic inflammation. BMC complementary Altern. Med. 19, 217–218. doi:10.1186/s12906-019-2635-0
Gupta, S., Wang, W., Hayek, S. S., Chan, L., Mathews, K. S., Melamed, M. L., et al. (2021). Association between early treatment with tocilizumab and mortality among critically ill patients with COVID-19. JAMA Intern. Med. 181, 41–51. doi:10.1001/jamainternmed.2020.6252
Hamed, M. A., Mohammed, M. A., Aboul Naser, A. F., Matloub, A. A., Fayed, D. B., Ali, S. A., et al. (2019). Optimization of curcuminoids extraction for evaluation against Parkinson’s disease in rats. J. Biol. Act. Prod. Nat. 9, 335–351. doi:10.1080/22311866.2019.1698317
Haridas, M., Sasidhar, V., Nath, P., Abhithaj, J., Sabu, A., and Rammanohar, P. (2021). Compounds of citrus medica and zingiber officinale for COVID-19 inhibition: In silico evidence for cues from Ayurveda. Future J. Pharm. Sci. 7, 13–19. doi:10.1186/s43094-020-00171-6
Hellou, E., Mohsin, J., Elemy, A., Hakim, F., Mustafa-Hellou, M., and Hamoud, S. (2022). Effect of ArtemiC in patients with COVID-19: A phase II prospective study. J. Cell. Mol. Med. 26, 3281–3289. doi:10.1111/jcmm.17337
Hewlings, S. J., and Kalman, D. S. (2017). Curcumin: A review of its effects on human health. Foods 6, 92. doi:10.3390/foods6100092
Hirano, T., and Murakami, M. (2020). COVID-19: A new virus, but a familiar receptor and cytokine release syndrome. Immunity 52, 731–733. doi:10.1016/j.immuni.2020.04.003
Ho, S.-C., Chang, K.-S., and Chang, P.-W. (2013). Inhibition of neuroinflammation by cinnamon and its main components. Food Chem. 138, 2275–2282. doi:10.1016/j.foodchem.2012.12.020
Horby, P. W., Pessoa-Amorim, G., Peto, L., Brightling, C. E., Sarkar, R., Thomas, K., et al. (2021). Tocilizumab in patients admitted to hospital with COVID-19 (RECOVERY): Preliminary results of a randomised, controlled, open-label, platform trial. Medrxiv. doi:10.1101/2021.02.11.21249258
Hosseini, A., Hashemi, V., Shomali, N., Asghari, F., Gharibi, T., Akbari, M., et al. (2020). Innate and adaptive immune responses against coronavirus. Biomedicine & Pharmacotherapy 132, 110859. doi:10.1016/j.biopha.2020.110859
Huang, C., Wang, Y., Li, X., Ren, L., Zhao, J., Hu, Y., et al. (2020). Clinical features of patients infected with 2019 novel coronavirus in Wuhan, China. lancet 395, 497–506. doi:10.1016/S0140-6736(20)30183-5
Hui, Q., Ammeter, E., Liu, S., Yang, R., Lu, P., Lahaye, L., et al. (2020). Eugenol attenuates inflammatory response and enhances barrier function during lipopolysaccharide-induced inflammation in the porcine intestinal epithelial cells. J. Animal Sci. 98, skaa245. doi:10.1093/jas/skaa245
Hwang, Y.-C., Lu, R.-M., Su, S.-C., Chiang, P.-Y., Ko, S.-H., Ke, F.-Y., et al. (2022). Monoclonal antibodies for COVID-19 therapy and SARS-CoV-2 detection. J. Biomed. Sci. 29, 1–50. doi:10.1186/s12929-021-00784-w
Imai, Y., Kuba, K., Rao, S., Huan, Y., Guo, F., Guan, B., et al. (2005). Angiotensin-converting enzyme 2 protects from severe acute lung failure. Nature 436, 112–116. doi:10.1038/nature03712
Jacobs, J., Clark-Snustad, K., and Lee, S. (2020). Case report of a SARS-CoV-2 infection in a patient with ulcerative colitis on tofacitinib. Inflamm. bowel Dis. 26, e64. doi:10.1093/ibd/izaa093
Jayakumar, T., Hsieh, C. Y., Lee, J. J., and Sheu, J. R. (2013). Experimental and clinical pharmacology of Andrographis paniculata and its major bioactive phytoconstituent andrographolide. Evidence-Based Complementary and Alternative Medicine, 2013. doi:10.1155/2013/846740
Ji, J., Zhang, J., Shao, Z., Xie, Q., Zhong, L., and Liu, Z. (2020). Glucocorticoid therapy does not delay viral clearance in COVID-19 patients. Crit. Care 24 (565), 1–4. doi:10.1186/s13054-020-03287-6
Kang, O.-H., Choi, J.-G., Lee, J.-H., and Kwon, D.-Y. (2010). Luteolin isolated from the flowers of Lonicera japonica suppresses inflammatory mediator release by blocking NF-kappaB and MAPKs activation pathways in HMC-1 cells. Molecules 15, 385–398. doi:10.3390/molecules15010385
Kao, T.-K., Ou, Y.-C., Lin, S.-Y., Pan, H.-C., Song, P.-J., Raung, S.-L., et al. (2011). Luteolin inhibits cytokine expression in endotoxin/cytokine-stimulated microglia. J. Nutr. Biochem. 22, 612–624. doi:10.1016/j.jnutbio.2010.01.011
Kashyap, V. K., Dhasmana, A., Yallapu, M. M., Chauhan, S. C., and Jaggi, M. (2020). Withania somnifera as a potential future drug molecule for COVID-19. Future Sci.
Kato, K., Yamashita, S., Kitanaka, S., and Toyoshima, S. (2001). Effect of gallic acid derivatives on secretion of Th1 cytokines and Th2 cytokines from anti CD3-stimulated spleen cells. Yakugaku zasshi J. Pharm. Soc. Jpn. 121, 451–457. doi:10.1248/yakushi.121.451
Kaur, G., Singh, N., Samuel, S. S., Bora, H. K., Sharma, S., Pachauri, S. D., et al. (2015). Withania somnifera shows a protective effect in monocrotaline-induced pulmonary hypertension. Pharm. Biol. 53, 147–157. doi:10.3109/13880209.2014.912240
Khalaf-Allah, A. E.-R. M., el-Gengaihi, S. E., Hamed, M. A., Zahran, H. G., and Mohammed, M. A. (2016). Chemical composition of golden berry leaves against hepato-renal fibrosis. J. Diet. Suppl. 13, 378–392. doi:10.3109/19390211.2015.1099584
Khalil, A. A., Rahman, U., Khan, M. R., Sahar, A., Mehmood, T., and Khan, M. (2017). Essential oil eugenol: Sources, extraction techniques and nutraceutical perspectives. RSC Adv. 7, 32669–32681. doi:10.1039/c7ra04803c
Khan, F. R., Kazmi, S. M. R., Iqbal, N. T., Iqbal, J., Ali, S. T., and Abbas, S. A. (2020). A quadruple blind, randomised controlled trial of gargling agents in reducing intraoral viral load among hospitalised COVID-19 patients: A structured summary of a study protocol for a randomised controlled trial. Trials 21, 1–4. doi:10.1186/s13063-020-04634-2
Kim, G. D., Lee, S. E., Kim, T. H., Jin, Y. H., Park, Y. S., and Park, C. S. (2012). Melatonin suppresses acrolein-induced IL-8 production in human pulmonary fibroblasts. J. pineal Res. 52, 356–364. doi:10.1111/j.1600-079X.2011.00950.x
Kim, S.-H., Jun, C.-D., Suk, K., Choi, B.-J., Lim, H., Park, S., et al. (2006). Gallic acid inhibits histamine release and pro-inflammatory cytokine production in mast cells. Toxicol. Sci. 91, 123–131. doi:10.1093/toxsci/kfj063
Kim, S. H., and Lee, Y. C. (2009). Piperine inhibits eosinophil infiltration and airway hyperresponsiveness by suppressing T cell activity and Th2 cytokine production in the ovalbumin-induced asthma model. J. Pharm. Pharmacol. 61, 353–359. doi:10.1211/jpp/61.03.0010
Klinkhammer, J., Schnepf, D., Ye, L., Schwaderlapp, M., Gad, H. H., Hartmann, R., et al. (2018). IFN-λ prevents influenza virus spread from the upper airways to the lungs and limits virus transmission. elife 7, e33354. doi:10.7554/eLife.33354
Knoll, M. D., and Wonodi, C. (2021). Oxford–AstraZeneca COVID-19 vaccine efficacy. Lancet 397, 72–74. doi:10.1016/S0140-6736(20)32623-4
Kong, D., Wang, Z., Tian, J., Liu, T., and Zhou, H. (2019). Glycyrrhizin inactivates toll-like receptor (TLR) signaling pathway to reduce lipopolysaccharide-induced acute lung injury by inhibiting TLR2. J. Cell. physiology 234, 4597–4607. doi:10.1002/jcp.27242
Koshak, A. E., Koshak, E. A., Mobeireek, A. F., Badawi, M. A., Wali, S. O., Malibary, H. M., et al. (2021). Nigella sativa for the treatment of COVID-19: An open-label randomized controlled clinical trial. Complementary Ther. Med. 61, 102769. doi:10.1016/j.ctim.2021.102769
Krischuns, T., Gunl, F., Henschel, L., Binder, M., Willemsen, J., Schloer, S., et al. (2018). Phosphorylation of TRIM28 enhances the expression of IFN-β and proinflammatory cytokines during HPAIV infection of human lung epithelial cells. Front. Immunol. 9, 2229. doi:10.3389/fimmu.2018.02229
Kumar, A., Prasad, G., Srivastav, S., Gautam, V. K., and Sharma, N. (2020). Efficacy and safety of guduchi ghan vati in the management of asymptomatic COVID-19 infection: An open label feasibility study. MedRxiv, 2020–09.
Kurose, K., and Yatagai, M. (2005). Components of the essential oils of Azadirachta indica A. Juss, Azadirachta siamensis Velton, and Azadirachta excelsa (Jack) Jacobs and their comparison. J. Wood Sci. 51, 185–188. doi:10.1007/s10086-004-0640-4
Lang, A., Lahav, M., Sakhnini, E., Barshack, I., Fidder, H. H., Avidan, B., et al. (2004). Allicin inhibits spontaneous and TNF-α induced secretion of proinflammatory cytokines and chemokines from intestinal epithelial cells. Clin. Nutr. 23, 1199–1208. doi:10.1016/j.clnu.2004.03.011
Lee, D. W., Gardner, R., Porter, D. L., Louis, C. U., Ahmed, N., Jensen, M., et al. (2014). Current concepts in the diagnosis and management of cytokine release syndrome. Blood 124 (2), 188–195.
Lee, W., Lee, S. Y., Son, Y.-J., and Yun, J.-M. (2015). Gallic acid decreases inflammatory cytokine secretion through histone acetyltransferase/histone deacetylase regulation in high glucose-induced human monocytes. J. Med. food 18, 793–801. doi:10.1089/jmf.2014.3342
Leng, Z., Zhu, R., Hou, W., Feng, Y., Yang, Y., Han, Q., et al. (2020). Transplantation of ACE2-mesenchymal stem cells improves the outcome of patients with COVID-19 pneumonia. Aging Dis. 11, 216–228. doi:10.14336/AD.2020.0228
Lescure, F.-X., Honda, H., Fowler, R. A., Lazar, J. S., Shi, G., Wung, P., et al. (2021). Sarilumab in patients admitted to hospital with severe or critical COVID-19: A randomised, double-blind, placebo-controlled, phase 3 trial. Lancet Respir. Med. 9, 522–532. doi:10.1016/S2213-2600(21)00099-0
Li, Y., He, S., Tang, J., Ding, N., Chu, X., Cheng, L., et al. (2017). Andrographolide inhibits inflammatory cytokines secretion in LPS-stimulated RAW264. 7 cells through suppression of NF-κB/MAPK signaling pathway. Evidence-based Complementary Altern. Med., 2017, 1, 9. doi:10.1155/2017/8248142
Li, Y., Yao, J., Han, C., Yang, J., Chaudhry, M., Wang, S., et al. (2016). Quercetin, inflammation and immunity. Nutrients 8, 167. doi:10.3390/nu8030167
Liang, W., Guan, W., Chen, R., Wang, W., Li, J., Xu, K., et al. (2020). Cancer patients in SARS-CoV-2 infection: A nationwide analysis in China. lancet Oncol. 21, 335–337. doi:10.1016/S1470-2045(20)30096-6
Liang, Y.-D., Bai, W.-J., Li, C.-G., Xu, L.-H., Wei, H.-X., Pan, H., et al. (2016). Piperine suppresses pyroptosis and interleukin-1β release upon ATP triggering and bacterial infection. Front. Pharmacol. 7, 390. doi:10.3389/fphar.2016.00390
Lin, Y., Shi, R., Wang, X., and Shen, H.-M. (2008). Luteolin, a flavonoid with potential for cancer prevention and therapy. Curr. cancer drug targets 8, 634–646. doi:10.2174/156800908786241050
Liu, J., Bodnar, B. H., Meng, F., Khan, A. I., Wang, X., Saribas, S., et al. (2021). Epigallocatechin gallate from green tea effectively blocks infection of SARS-CoV-2 and new variants by inhibiting spike binding to ACE2 receptor. Cell. & Biosci. 11 (1), 1–15. doi:10.1186/s13578-021-00680-8
Liu, J., Zheng, X., Tong, Q., Li, W., Wang, B., Sutter, K., et al. (2020a). Overlapping and discrete aspects of the pathology and pathogenesis of the emerging human pathogenic coronaviruses SARS-CoV, MERS-CoV, and 2019-nCoV. J. Med. virology 92, 491–494. doi:10.1002/jmv.25709
Liu, M.-Y., Zheng, B., Zhang, Y., and Li, J.-P. (2020b). Role and mechanism of angiotensin-converting enzyme 2 in acute lung injury in coronavirus disease 2019. Chronic Dis. Transl. Med. 6, 98–105. doi:10.1016/j.cdtm.2020.05.003
Liu, Q., Zhou, Y.-H., and Yang, Z.-Q. (2016). The cytokine storm of severe influenza and development of immunomodulatory therapy. Cell. Mol. Immunol. 13, 3–10. doi:10.1038/cmi.2015.74
Liu, Z., and Ying, Y. (2020). The inhibitory effect of curcumin on virus-induced cytokine storm and its potential use in the associated severe pneumonia. Front. Cell. Dev. Biol. 8, 479. doi:10.3389/fcell.2020.00479
Lodhi, S., Vadnere, G. P., Patil, K. D., and Patil, T. P. (2020). Protective effects of luteolin on injury induced inflammation through reduction of tissue uric acid and pro-inflammatory cytokines in rats. J. traditional complementary Med. 10, 60–69. doi:10.1016/j.jtcme.2019.02.004
Lu, J., Ma, Y., Wu, J., Huang, H., Wang, X., Chen, Z., et al. (2019). A review for the neuroprotective effects of andrographolide in the central nervous system. Biomed. Pharmacother. 117, 109078. doi:10.1016/j.biopha.2019.109078
Ma, J., Xia, P., Zhou, Y., Liu, Z., Zhou, X., Wang, J., et al. (2020). Potential effect of blood purification therapy in reducing cytokine storm as a late complication of critically ill COVID-19, 214. Orlando, Fla: Clinical Immunology, 108408.
Madera-Salcedo, I. K., Cruz, S. L., and Gonzalez-Espinosa, C. (2011). Morphine decreases early peritoneal innate immunity responses in Swiss–Webster and C57BL6/J mice through the inhibition of mast cell TNF-α release. J. Neuroimmunol. 232, 101–107. doi:10.1016/j.jneuroim.2010.10.017
Mahallawi, W. H., Khabour, O. F., Zhang, Q., Makhdoum, H. M., and Suliman, B. A. (2018). MERS-CoV infection in humans is associated with a pro-inflammatory Th1 and Th17 cytokine profile. Cytokine 104, 8–13. doi:10.1016/j.cyto.2018.01.025
Malaguarnera, L. (2019). Influence of resveratrol on the immune response. Nutrients 11, 946. doi:10.3390/nu11050946
Mandlik, D. S., and Namdeo, A. G. (2021). Pharmacological evaluation of Ashwagandha highlighting its healthcare claims, safety, and toxicity aspects. J. Diet. Suppl. 18, 183–226. doi:10.1080/19390211.2020.1741484
Marinella, M. A. (2020). Indomethacin and resveratrol as potential treatment adjuncts for SARS-CoV-2/COVID-19. Int. J. Clin. Pract. 74, e13535. doi:10.1111/ijcp.13535
Masiá, M., FernáNDEZ-GonzáLEZ, M., Padilla, S., Ortega, P., GarcíA, J. A., Agulló, V., et al. (2020). Impact of interleukin-6 blockade with tocilizumab on SARS-CoV-2 viral kinetics and antibody responses in patients with COVID-19: A prospective cohort study. EBioMedicine 60, 102999. doi:10.1016/j.ebiom.2020.102999
Maurya, S. P., Das, B. K., Singh, R., and Tyagi, S. (2019). Effect of Withania somnifer on CD38 expression on CD8+ T lymphocytes among patients of HIV infection. Clin. Immunol. 203, 122–124. doi:10.1016/j.clim.2019.04.003
Maurya, V. K., Kumar, S., Prasad, A. K., Bhatt, M. L., and Saxena, S. K. (2020). Structure-based drug designing for potential antiviral activity of selected natural products from Ayurveda against SARS-CoV-2 spike glycoprotein and its cellular receptor. Virusdisease 31, 179–193. doi:10.1007/s13337-020-00598-8
Mehrbod, P., Abdalla, M. A., Fotouhi, F., Heidarzadeh, M., Aro, A. O., Eloff, J. N., et al. (2018). Immunomodulatory properties of quercetin-3-O-α-L-rhamnopyranoside from Rapanea melanophloeos against influenza a virus. BMC complementary Altern. Med. 18 (1), 1–10. doi:10.1186/s12906-018-2246-1
Meng, W., Xiaoliang, R., Xiumei, G., Vincieri, F. F., and Bilia, A. R. (2009). Stability of active ingredients of traditional Chinese medicine (TCM). Nat. Product. Commun. 4, 1934578X0900401–1776. doi:10.1177/1934578x0900401229
Min, K. J., Jang, J. H., and Kwon, T. K. (2012). Inhibitory effects of melatonin on the lipopolysaccharide-induced CC chemokine expression in BV2 murine microglial cells are mediated by suppression of Akt-induced NF-κB and STAT/GAS activity. J. pineal Res. 52, 296–304. doi:10.1111/j.1600-079X.2011.00943.x
Mizrahi, B., Sudry, T., Flaks-Manov, N., Yehezkelli, Y., Kalkstein, N., Akiva, P., et al. (2023). Long covid outcomes at one year after mild SARS-CoV-2 infection: Nationwide cohort study. bmj, 380.
Modi, B., Hernandez-Henderson, M., Yang, D., Klein, J., Dadwal, S., Kopp, E., et al. (2019). Ruxolitinib as salvage therapy for chronic graft-versus-host disease. Biol. Blood Marrow Transplant. 25, 265–269. doi:10.1016/j.bbmt.2018.09.003
Mohammed, M. A., Elgammal, E. W., Gaara, A. H., and el Raey, M. A. (2022a). Synergistic effect of Silver and ZnO nanoparticles green synthesized by Vitis vinifera stem extract with Ampicillin against some pathogenic microbes. Egypt. J. Chem. 65, 1–2.
Mohammed, M. A., Hamed, M. A., el-Gengaihi, S. E., Enein, A. M. A., Kachlicki, P., and Hassan, E. M. (2022b). Profiling of secondary metabolites and DNA typing of three different Annona cultivars grown in Egypt. Metabolomics 18, 49–21. doi:10.1007/s11306-022-01911-w
Mohammed, M. A., Ibrahim, B. M., Abdel-Latif, Y., Hassan, A. H., el Raey, M. A., Hassan, E. M., et al. (2022c). Pharmacological and metabolomic profiles of Musa acuminata wastes as a new potential source of anti-ulcerative colitis agents. Sci. Rep. 12 (1), 10595. doi:10.1038/s41598-022-14599-8
Moll, G., Drzeniek, N., Kamhieh-Milz, J., Geissler, S., Volk, H.-D., and Reinke, P. (2020). MSC therapies for COVID-19: Importance of patient coagulopathy, thromboprophylaxis, cell product quality and mode of delivery for treatment safety and efficacy. Front. Immunol. 11, 1091. doi:10.3389/fimmu.2020.01091
Montesarchio, V., Parrella, R., Iommelli, C., Bianco, A., Manzillo, E., Fraganza, F., et al. (2020). Outcomes and biomarker analyses among patients with COVID-19 treated with interleukin 6 (IL-6) receptor antagonist sarilumab at a single institution in Italy. J. Immunother. cancer 8, e001089. doi:10.1136/jitc-2020-001089
Morassi, M., Bagatto, D., Cobelli, M., D’Agostini, S., Gigli, G. L., Bna, C., et al. (2020). Stroke in patients with SARS-CoV-2 infection: Case series. J. neurology 267, 2185–2192. doi:10.1007/s00415-020-09885-2
Murakami, M., Kamimura, D., and Hirano, T. (2019). Pleiotropy and specificity: Insights from the interleukin 6 family of cytokines. Immunity 50, 812–831. doi:10.1016/j.immuni.2019.03.027
Murck, H. (2020). Symptomatic protective action of glycyrrhizin (licorice) in COVID-19 infection? Front. Immunol. 11, 1239. doi:10.3389/fimmu.2020.01239
National Academies Of Sciences, E. (2017). The health effects of cannabis and cannabinoids: The current state of evidence and recommendations for research.
Nawaz, M. A., Huang, Y., Bie, Z., Ahmed, W., Reiter, R. J., Niu, M., et al. (2016). Corrigendum: Melatonin: Current status and future perspectives in plant science. Front. plant Sci. 7, 714. doi:10.3389/fpls.2016.00714
Nelson, B. C., Laracy, J., Shoucri, S., Dietz, D., Zucker, J., Patel, N., et al. (2021). Clinical outcomes associated with methylprednisolone in mechanically ventilated patients with COVID-19. Clin. Infect. Dis. 72, e367–e372. doi:10.1093/cid/ciaa1163
Nie, X., Chen, S.-R., Wang, K., Peng, Y., Wang, Y. T., Wang, D., et al. (2017). Attenuation of innate immunity by andrographolide derivatives through NF-κB signaling pathway. Sci. Rep. 7 (1), 4738. doi:10.1038/s41598-017-04673-x
Orleans, L., Is Vice, H., and Manchikanti, L. (2020). Expanded umbilical cord mesenchymal stem cells (UC-MSCs) as a therapeutic strategy in managing critically ill COVID-19 patients: The case for compassionate use. Pain physician 23, E71–E83. doi:10.36076/ppj.2020/23/e71
Ortega-PeñA, M., and GonzáLEZ-Cuevas, R. (2021). Familiar dermatologic drugs as therapies for COVID-19. Actas Dermo-Sifiliográficas Engl. Ed. 112, 118–126. doi:10.1016/j.ad.2020.09.004
Pandit, M., and Latha, N. (2020). In silico studies reveal potential antiviral activity of phytochemicals from medicinal plants for the treatment of COVID-19 infection.
Peacocke, E. F., Heupink, L. F., FrøNSDAL, K., Dahl, E. H., and Chola, L. (2021). Global access to COVID-19 vaccines: A scoping review of factors that may influence equitable access for low and middle-income countries. BMJ open 11, e049505. doi:10.1136/bmjopen-2021-049505
Peter, A. E., Sandeep, B., Rao, B. G., and Kalpana, V. L. (2021). Calming the storm: Natural immunosuppressants as adjuvants to target the cytokine storm in COVID-19. Front. Pharmacol. 11, 583777. doi:10.3389/fphar.2020.583777
Peterson, D., Damsky, W., and King, B. (2020). The use of Janus kinase inhibitors in the time of severe acute respiratory syndrome coronavirus 2 (SARS-CoV-2). J. Am. Acad. Dermatology 82, e223–e226. doi:10.1016/j.jaad.2020.03.099
Piantoni, S., Patroni, A., Toniati, P., Furloni, R., Franceschini, F., Andreoli, L., et al. (2020). Why not to use colchicine in COVID-19? An oldanti-inflammatory drug for a novel auto-inflammatory disease. Rheumatology 59, 1769–1770. doi:10.1093/rheumatology/keaa217
Piao, W.-H., Campagnolo, D., Dayao, C., Lukas, R. J., Wu, J., and Shi, F.-D. (2009). Nicotine and inflammatory neurological disorders. Acta Pharmacol. Sin. 30, 715–722. doi:10.1038/aps.2009.67
Plat, J., van Brakel, L., and Mensink, R. P. (2022). Plant stanol esters might optimise the immune response and improve the SARS-CoV-2/COVID-19 vaccine efficacy in overweight and obese subjects. Br. J. Nutr. 127, 1117–1118. doi:10.1017/S0007114521001781
Pramod, K., Ansari, S. H., and Ali, J. (2010). Eugenol: A natural compound with versatile pharmacological actions. Nat. Product. Commun. 5, 1934578X1000501–2006. doi:10.1177/1934578x1000501236
Prasanth, D., Murahari, M., Chandramohan, V., Panda, S. P., Atmakuri, L. R., and Guntupalli, C. (2020). In silico identification of potential inhibitors from Cinnamon against main protease and spike glycoprotein of SARS CoV-2. J. Biomol. Struct. Dyn. 39, 4618–4632. doi:10.1080/07391102.2020.1779129
Rabaan, A. A., al-Ahmed, S. H., Muhammad, J., Khan, A., Sule, A. A., Tirupathi, R., et al. (2021). Role of inflammatory cytokines in COVID-19 patients: A review on molecular mechanisms, immune functions, immunopathology and immunomodulatory drugs to counter cytokine storm. Vaccines 9, 436. doi:10.3390/vaccines9050436
Rahardjo, B., Widjajanto, E., Sujuti, H., and Keman, K. (2014). Curcumin decreased level of proinflammatory cytokines in monocyte cultures exposed to preeclamptic plasma by affecting the transcription factors NF-κB and PPAR-γ. Biomarkers Genomic Med. 6, 105–115. doi:10.1016/j.bgm.2014.06.002
Rahmati, M., and Moosavi, M. A. (2020). Cytokine-targeted therapy in severely ill COVID-19 patients: Options and cautions. mortality, 105954.
Reiter, R. J., Sharma, R., Ma, Q., Dominquez-Rodriguez, A., Marik, P. E., and Abreu-Gonzalez, P. (2020). Melatonin inhibits COVID-19-induced cytokine storm by reversing aerobic glycolysis in immune cells: A mechanistic analysis. Med. drug Discov. 6, 100044. doi:10.1016/j.medidd.2020.100044
Rhimi, W., Mohammed, M. A., Zarea, A. A. K., Greco, G., Tempesta, M., Otranto, D., et al. (2022). Antifungal, antioxidant and antibiofilm activities of essential oils of Cymbopogon spp. Antibiotics 11, 829. doi:10.3390/antibiotics11060829
Ribeiro, D., Freitas, M., Tome, S. M., Silva, A. M., Laufer, S., Lima, J. L., et al. (2015). Flavonoids inhibit COX-1 and COX-2 enzymes and cytokine/chemokine production in human whole blood. Inflammation 38, 858–870. doi:10.1007/s10753-014-9995-x
Rieder, S. A., Nagarkatti, P., and Nagarkatti, M. (2012). Multiple anti-inflammatory pathways triggered by resveratrol lead to amelioration of staphylococcal enterotoxin B-induced lung injury. Br. J. Pharmacol. 167, 1244–1258. doi:10.1111/j.1476-5381.2012.02063.x
Rolta, R., Salaria, D., Sharma, B., Awofisayo, O., Fadare, O. A., Sharma, S., et al. (2022). Methylxanthines as potential inhibitor of SARS-CoV-2: An in silico approach. Curr. Pharmacol. Rep. 8, 149–170. doi:10.1007/s40495-021-00276-3
Rolta, R., Salaria, D., Sharma, P., Sharma, B., Kumar, V., Rathi, B., et al. (2021a). Phytocompounds of rheum emodi, thymus serpyllum, and artemisia annua inhibit spike protein of SARS-CoV-2 binding to ACE2 receptor: In silico approach. Curr. Pharmacol. Rep. 7, 135–149. doi:10.1007/s40495-021-00259-4
Rolta, R., Yadav, R., Salaria, D., Trivedi, S., Imran, M., Sourirajan, A., et al. (2021b). In silico screening of hundred phytocompounds of ten medicinal plants as potential inhibitors of nucleocapsid phosphoprotein of COVID-19: An approach to prevent virus assembly. J. Biomol. Struct. Dyn. 39, 7017–7034. doi:10.1080/07391102.2020.1804457
Rosas, I. O., BräU, N., Waters, M., Go, R. C., Hunter, B. D., Bhagani, S., et al. (2021). Tocilizumab in hospitalized patients with severe Covid-19 pneumonia. N. Engl. J. Med. 384, 1503–1516. doi:10.1056/NEJMoa2028700
Roumier, M., Paule, R., Matthieu, G., Vallee, A., and Ackermann, F. (2020). Interleukin-6 blockade for severe COVID-19. medrxiv.
Sagar, V., and Kumar, A. H. (2020). Efficacy of natural compounds from Tinospora cordifolia against SARS-CoV-2 protease, surface glycoprotein and RNA polymerase. Virology, 1–10.
Saha, P., Katarkar, A., Das, B., Bhattacharyya, A., and Chaudhuri, K. (2016). 6-gingerol inhibits Vibrio cholerae-induced proinflammatory cytokines in intestinal epithelial cells via modulation of NF-κB. Pharm. Biol. 54, 1606–1615. doi:10.3109/13880209.2015.1110598
Sajjadi, S., Mehregan, I., and Taheri, M. (2015). Essential oil composition of Hypericum triquetrifolium Turra growing wild in Iran. Res. Pharm. Sci. 10, 90–94.
Salehi, B., Sharopov, F., Fokou, P. V. T., Kobylinska, A., Jonge, L. D., Tadio, K., et al. (2019). Melatonin in medicinal and food plants: Occurrence, bioavailability, and health potential for humans. Cells 8, 681. doi:10.3390/cells8070681
Sannegowda, K., Venkatesha, S., and Moudgil, K. (2015). Tinospora cordifolia inhibits autoimmune arthritis by regulating key immune mediators of inflammation and bone damage. Int. J. Immunopathol. Pharmacol. 28, 521–531. doi:10.1177/0394632015608248
Sasidharan, S., Sarkar, N., and Saudagar, P. (2022). Discovery of compounds inhibiting SARS-COV-2 multi-targets. J. Biomol. Struct. Dyn., 1–16. doi:10.1080/07391102.2021.2025149
Scarsi, M., Piantoni, S., Colombo, E., Airó, P., Richini, D., Miclini, M., et al. (2020). Association between treatment with colchicine and improved survival in a single-centre cohort of adult hospitalised patients with COVID-19 pneumonia and acute respiratory distress syndrome. Ann. rheumatic Dis. 79, 1286–1289. doi:10.1136/annrheumdis-2020-217712
Schink, A., Naumoska, K., Kitanovski, Z., Kampf, C. J., FröHLICH-Nowoisky, J., Thines, E., et al. (2018). Anti-inflammatory effects of cinnamon extract and identification of active compounds influencing the TLR2 and TLR4 signaling pathways. Food & Funct. 9, 5950–5964. doi:10.1039/c8fo01286e
Seigneuric, C., Camara, B., Delmont, J., Busato, F., Payen, J., Armengaud, M., et al. (2008). Quinquina and man. Med. Trop. Rev. Corps Sante Colon. 68, 459–462.
Senthil Kumar, K., Gokila Vani, M., Wang, C.-S., Chen, C.-C., Chen, Y.-C., Lu, L.-P., et al. (2020). Geranium and lemon essential oils and their active compounds downregulate angiotensin-converting enzyme 2 (ACE2), a SARS-CoV-2 spike receptor-binding domain, in epithelial cells. Plants 9, 770. doi:10.3390/plants9060770
Shakoory, B., Carcillo, J. A., Chatham, W. W., Amdur, R. L., Zhao, H., Dinarello, C. A., et al. (2016). Interleukin-1 receptor blockade is associated with reduced mortality in sepsis patients with features of macrophage activation syndrome: Reanalysis of a prior phase III trial. Crit. care Med. 44, 275–281. doi:10.1097/CCM.0000000000001402
Sharifi-Rad, J., Cristina Cirone Silva, N., Jantwal, A., D Bhatt, I., Sharopov, F., C Cho, W., et al. (2019). Therapeutic potential of allicin-rich garlic preparations: Emphasis on clinical evidence toward upcoming drugs formulation. Appl. Sci. 9, 5555. doi:10.3390/app9245555
Sharifi-Rad, M., Varoni, E. M., Salehi, B., Sharifi-Rad, J., Matthews, K. R., Ayatollahi, S. A., et al. (2017). Plants of the genus Zingiber as a source of bioactive phytochemicals: From tradition to pharmacy. Molecules 22, 2145. doi:10.3390/molecules22122145
Sharma, U., Bala, M., Kumar, N., Singh, B., Munshi, R. K., and Bhalerao, S. (2012). Immunomodulatory active compounds from Tinospora cordifolia. J. Ethnopharmacol. 141, 918–926. doi:10.1016/j.jep.2012.03.027
Sharma, V., Kaushik, S., Pandit, P., Dhull, D., Yadav, J. P., and Kaushik, S. (2019). Green synthesis of silver nanoparticles from medicinal plants and evaluation of their antiviral potential against chikungunya virus. Appl. Microbiol. Biotechnol. 103, 881–891. doi:10.1007/s00253-018-9488-1
Sheng, Y., Wu, T., Dai, Y., Ji, K., Zhong, Y., and Xue, Y. (2020). The effect of 6-gingerol on inflammatory response and Th17/Treg balance in DSS-induced ulcerative colitis mice. Ann. Transl. Med. 8, 442. doi:10.21037/atm.2020.03.141
Shimabukuro-Vornhagen, A., GöDEL, P., Subklewe, M., Stemmler, H. J., SchlößER, H. A., Schlaak, M., et al. (2018). Cytokine release syndrome. J. Immunother. cancer 6, 56–14. doi:10.1186/s40425-018-0343-9
Shimizu, K., Funamoto, M., Sunagawa, Y., Shimizu, S., Katanasaka, Y., Miyazaki, Y., et al. (2019). Anti-inflammatory action of curcumin and its use in the treatment of lifestyle-related diseases. Eur. Cardiol. Rev. 14, 117–122. doi:10.15420/ecr.2019.17.2
Shree, P., Mishra, P., Selvaraj, C., Singh, S. K., Chaube, R., Garg, N., et al. (2020). Targeting COVID-19 (SARS-CoV-2) main protease through active phytochemicals of ayurvedic medicinal plants–Withania somnifera (Ashwagandha), Tinospora cordifolia (Giloy) and Ocimum sanctum (Tulsi)–a molecular docking study. J. Biomol. Struct. Dyn. 40, 190–203. doi:10.1080/07391102.2020.1810778
Siddiqi, H. K., and Mehra, M. R. (2020). COVID-19 illness in native and immunosuppressed states: A clinical–therapeutic staging proposal. J. heart lung Transplant. 39, 405–407. doi:10.1016/j.healun.2020.03.012
Sinha, S. K., Prasad, S. K., Islam, M. A., Gurav, S. S., Patil, R. B., Alfaris, N. A., et al. (2020). Identification of bioactive compounds from Glycyrrhiza glabra as possible inhibitor of SARS-CoV-2 spike glycoprotein and non-structural protein-15: A pharmacoinformatics study. J. Biomol. Struct. Dyn. 39, 4686–4700. doi:10.1080/07391102.2020.1779132
Song, P., Li, W., Xie, J., Hou, Y., and You, C. (2020). Cytokine storm induced by SARS-CoV-2. Clin. Chim. acta 509, 280–287. doi:10.1016/j.cca.2020.06.017
Sordillo, P. P., and Helson, L. (2015). Curcumin suppression of cytokine release and cytokine storm. A potential therapy for patients with Ebola and other severe viral infections. vivo 29, 1–4.
Srivastava, V., Yadav, A., and Sarkar, P. (2020). Molecular docking and ADMET study of bioactive compounds of Glycyrrhiza glabra against main protease of SARS-CoV2. Mater. Today Proc. 49, 2999–3007. doi:10.1016/j.matpr.2020.10.055
Stallmach, A., Kortgen, A., Gonnert, F., Coldewey, S. M., Reuken, P., and Bauer, M. (2020). Infliximab against severe COVID-19-induced cytokine storm syndrome with organ failure—A cautionary case series. Crit. Care 24 (1), 1–3. doi:10.1186/s13054-020-03158-0
Steeland, S., Libert, C., and Vandenbroucke, R. E. (2018). A new venue of TNF targeting. Int. J. Mol. Sci. 19, 1442. doi:10.3390/ijms19051442
Sun, Y., Jiang, M., Park, P.-H., and Song, K. (2020). Transcriptional suppression of androgen receptor by 18β-glycyrrhetinic acid in LNCaP human prostate cancer cells. Archives pharmacal Res. 43, 433–448. doi:10.1007/s12272-020-01228-z
Tang, J., Diao, P., Shu, X., Li, L., and Xiong, L. (2019). Quercetin and quercitrin attenuates the inflammatory response and oxidative stress in LPS-induced RAW264. 7 cells: In vitro assessment and a theoretical model. BioMed Res. Int., 2019, 7039802. doi:10.1155/2019/7039802
Tang, L., Yin, Z., Hu, Y., and Mei, H. (2020). Controlling cytokine storm is vital in COVID-19. Front. Immunol. 11, 570993. doi:10.3389/fimmu.2020.570993
Thakar, A., Panara, K., Shah, H., Kalsariya, B., Ruparel, S., Jain, N., et al. (2022). Guduchi ghanavati (Ayurveda medication) improves the perceived immunity in individuals at risk of SARS-CoV-2: A multicentred, controlled, before-and-after study. Eur. J. Integr. Med. 53, 102131. doi:10.1016/j.eujim.2022.102131
Theoharides, T. C. (2020). COVID-19, pulmonary mast cells, cytokine storms, and beneficial actions of luteolin. Oxford, England: Biofactors.
Thomas, M., Mani, R. S., Philip, M., Adhikary, R., Joshi, S., Revadi, S. S., et al. (2017). Proinflammatory chemokines are major mediators of exuberant immune response associated with Influenza A (H1N1) pdm09 virus infection. J. Med. virology 89, 1373–1381. doi:10.1002/jmv.24781
Toniati, P., Piva, S., Cattalini, M., Garrafa, E., Regola, F., Castelli, F., et al. (2020). Tocilizumab for the treatment of severe COVID-19 pneumonia with hyperinflammatory syndrome and acute respiratory failure: A single center study of 100 patients in brescia, Italy. Autoimmun. Rev. 19, 102568. doi:10.1016/j.autrev.2020.102568
Tripathi, M. K., Singh, P., Sharma, S., Singh, T. P., Ethayathulla, A., and Kaur, P. (2021). Identification of bioactive molecule from Withania somnifera (Ashwagandha) as SARS-CoV-2 main protease inhibitor. J. Biomol. Struct. Dyn. 39, 5668–5681. doi:10.1080/07391102.2020.1790425
Tripathi, S., Maier, K. G., Bruch, D., and Kittur, D. S. (2007). Effect of 6-gingerol on pro-inflammatory cytokine production and costimulatory molecule expression in murine peritoneal macrophages. J. Surg. Res. 138, 209–213. doi:10.1016/j.jss.2006.07.051
Ulhaq, Z. S., and Soraya, G. V. (2020). Interleukin-6 as a potential biomarker of COVID-19 progression. Med. maladies Infect. 50, 382–383. doi:10.1016/j.medmal.2020.04.002
Upadhyay, A. K., Kumar, K., Kumar, A., and Mishra, H. S. (2010). Tinospora cordifolia (Willd.) Hook. f. and Thoms.(Guduchi)–validation of the Ayurvedic pharmacology through experimental and clinical studies. Int. J. Ayurveda Res. 1, 112–121. doi:10.4103/0974-7788.64405
Valizadeh, H., Abdolmohammadi-Vahid, S., Danshina, S., Gencer, M. Z., Ammari, A., Sadeghi, A., et al. (2020). Nano-curcumin therapy, a promising method in modulating inflammatory cytokines in COVID-19 patients. Int. Immunopharmacol. 89, 107088. doi:10.1016/j.intimp.2020.107088
van de Sand, L., Bormann, M., Alt, M., Schipper, L., Heilingloh, C. S., Todt, D., et al. (2021). Glycyrrhizin Effectively Inhibits SARS-CoV-2 Replication by Inhibiting the Viral Main Protease. Viruses 13 (4), 609. doi:10.3390/v13040609
Vardhana, S. A., and Wolchok, J. D. (2020). The many faces of the anti-COVID immune response. J. Exp. Med. 217, e20200678. doi:10.1084/jem.20200678
Varma, A., Padh, H., and Shrivastava, N. (2011). Andrographolide: A new plant-derived antineoplastic entity on horizon. Evidence-Based Complementary Altern. Med., 2011, 1, 9. doi:10.1093/ecam/nep135
Wan, S., Yi, Q., Fan, S., Lv, J., Zhang, X., Guo, L., et al. (2020). Characteristics of lymphocyte subsets and cytokines in peripheral blood of 123 hospitalized patients with 2019 novel coronavirus pneumonia (NCP). MedRxiv.
Wang, D., Hu, B., Hu, C., Zhu, F., Liu, X., Zhang, J., et al. (2020a). Clinical characteristics of 138 hospitalized patients with 2019 novel coronavirus–infected pneumonia in Wuhan, China. Jama 323, 1061–1069. doi:10.1001/jama.2020.1585
Wang, L., Wang, Y., Ye, D., and Liu, Q. (2020b). Review of the 2019 novel coronavirus (SARS-CoV-2) based on current evidence. Int. J. Antimicrob. agents 55, 105948. doi:10.1016/j.ijantimicag.2020.105948
Wang, Q., and Hu, Z. (2020). Successful recovery of severe COVID-19 with cytokine storm treating with extracorporeal blood purification. Int. J. Infect. Dis. 96, 618–620. doi:10.1016/j.ijid.2020.05.065
Wang, W., Wang, J., Dong, S.-F., Liu, C.-H., Italiani, P., Sun, S.-H., et al. (2010). Immunomodulatory activity of andrographolide on macrophage activation and specific antibody response. Acta Pharmacol. Sin. 31, 191–201. doi:10.1038/aps.2009.205
Wong, S. Y., Tan, M. G., Banks, W. A., Wong, W. F., Wong, P. T.-H., and Lai, M. K. (2016). Andrographolide attenuates LPS-stimulated up-regulation of CC and CXC motif chemokines in rodent cortex and primary astrocytes. J. neuroinflammation 13, 34–11. doi:10.1186/s12974-016-0498-6
World Health Organization (2020). Modes of transmission of virus causing COVID-19: Implications for IPC precaution recommendations: Scientific brief, 29 March 2020. China: World Health Organization.
Xu, X., Han, M., Li, T., Sun, W., Wang, D., Fu, B., et al. (2020). Effective treatment of severe COVID-19 patients with tocilizumab. Proc. Natl. Acad. Sci. 117, 10970–10975. doi:10.1073/pnas.2005615117
Yadav, R., Jee, B., and Awasthi, S. K. (2015). Curcumin suppresses the production of pro-inflammatory cytokine interleukin-18 in lipopolysaccharide stimulated murine macrophage-like cells. Indian J. Clin. Biochem. 30, 109–112. doi:10.1007/s12291-014-0452-2
Yagi, H., Soto-Gutierrez, A., Parekkadan, B., Kitagawa, Y., Tompkins, R. G., Kobayashi, N., et al. (2010). Mesenchymal stem cells: Mechanisms of immunomodulation and homing. Cell. Transplant. 19, 667–679. doi:10.3727/096368910X508762
Yang, L., Liu, S., Liu, J., Zhang, Z., Wan, X., Huang, B., et al. (2020a). COVID-19: Immunopathogenesis and immunotherapeutics. Signal Transduct. Target. Ther. 5, 128–8. doi:10.1038/s41392-020-00243-2
Yang, R., Xiong, Y., Ke, H., Chen, T., and Gao, S. (2020b). The role of methylprednisolone on preventing disease progression for hospitalized patients with severe COVID-19. Eur. J. Clin. investigation 50, e13412. doi:10.1111/eci.13412
Yang, X. H., Sun, R. H., Zhao, M. Y., Chen, E. Z., Liu, J., Wang, H. L., et al. (2020c). Expert recommendations on blood purification treatment protocol for patients with severe COVID-19. Chronic Dis. Transl. Med. 6 (02), 106–104.
Zahedipour, F., Hosseini, S. A., Sathyapalan, T., Majeed, M., Jamialahmadi, T., al-Rasadi, K., et al. (2020). Potential effects of curcumin in the treatment of COVID-19 infection. Phytotherapy Res. 34, 2911–2920. doi:10.1002/ptr.6738
Zhai, W.-J., Zhang, Z.-B., Xu, N.-N., Guo, Y.-F., Qiu, C., Li, C.-Y., et al. (2016). Piperine plays an anti-inflammatory role in Staphylococcus aureus endometritis by inhibiting activation of NF-κB and MAPK pathways in mice. Evidence-Based Complementary Altern. Med. 2016, 1–10. doi:10.1155/2016/8597208
Zhang, B., Zhou, X., Qiu, Y., Song, Y., Feng, F., Feng, J., et al. (2020a). Clinical characteristics of 82 cases of death from COVID-19. PloS one 15, e0235458. doi:10.1371/journal.pone.0235458
Zhang, L., Li, Y., Gu, Z., Wang, Y., Shi, M., Ji, Y., et al. (2015). Resveratrol inhibits enterovirus 71 replication and pro-inflammatory cytokine secretion in rhabdosarcoma cells through blocking IKKs/NF-κB signaling pathway. PloS one 10, e0116879. doi:10.1371/journal.pone.0116879
Zhang, M., Pan, H., Xu, Y., Wang, X., Qiu, Z., and Jiang, L. (2017). Allicin decreases lipopolysaccharide-induced oxidative stress and inflammation in human umbilical vein endothelial cells through suppression of mitochondrial dysfunction and activation of Nrf2. Cell. Physiology Biochem. 41, 2255–2267. doi:10.1159/000475640
Zhang, R., Wang, X., Ni, L., di, X., Ma, B., Niu, S., et al. (2020b). COVID-19: Melatonin as a potential adjuvant treatment. Life Sci. 250, 117583. doi:10.1016/j.lfs.2020.117583
Zhang, X., Zhang, Y., Qiao, W., Zhang, J., and Qi, Z. (2020c). Baricitinib, a drug with potential effect to prevent SARS-COV-2 from entering target cells and control cytokine storm induced by COVID-19. International immunopharmacology 86, 106749.
Zhang, Y., Yu, L., Tang, L., Zhu, M., Jin, Y., Wang, Z., et al. (2021). A promising anti-cytokine-storm targeted therapy for COVID-19: The artificial-liver blood-purification system. Eng. (Beijing, China) 7, 11–13. doi:10.1016/j.eng.2020.03.006
Zhao, H., Zhao, M., Wang, Y., Li, F., and Zhang, Z. (2016). Glycyrrhizic acid prevents sepsis-induced acute lung injury and mortality in rats. J. Histochem. Cytochem. 64, 125–137. doi:10.1369/0022155415610168
Zhou, P., Yang, X.-L., Wang, X.-G., Hu, B., Zhang, L., Zhang, W., et al. (2020). A pneumonia outbreak associated with a new coronavirus of probable bat origin. nature 579, 270–273. doi:10.1038/s41586-020-2012-7
Zhou, Z. X., Mou, S. F., Chen, X. Q., Gong, L. L., and Ge, W. S. (2018). Anti-inflammatory activity of resveratrol prevents inflammation by inhibiting NF-κB in animal models of acute pharyngitis. Mol. Med. Rep. 17, 1269–1274. doi:10.3892/mmr.2017.7933
Zhu, L., Gu, P., and Shen, H. (2019). Gallic acid improved inflammation via NF-κB pathway in TNBS-induced ulcerative colitis. Int. Immunopharmacol. 67, 129–137. doi:10.1016/j.intimp.2018.11.049
Glossary
SARS severe acute respiratory syndrome
MERS Middle East respiratory syndrome
CRS cytokine release syndrome
DIC diffuse intravascular coagulation
MOF multiple organ failure
ARDS abrupt respiratory distress syndrome
Sepsis early recognition and optimized treatment
DAMPs damage-associated patterns
PAMPs pathogen-associated patterns
Tfh cell T follicular helper cell
IFN- ℽ interferon-gamma
MCP-1 monocyte chemoattractant protein-1
MIP-1 macrophage inflammatory protein-1
IL-6 interleukin-6
IP-10 interferon-induced protein-10
TNF-α tumor necrosis factor alfa
MAS macrophage activation syndrome
ACE-2 angiotensin-converting enzyme-2
TAK Janus kinase
COPD chronic obstructive pulmonary disease
ATRA all trans-retinoic acid
MIS-C multisystem inflammatory syndrome in children
S-CSS associated cytokine storm syndrome
NLRP-3 NLR-family pyrin domain containing 3
RBD receptor binding domain
IAVG Independent Allocation of Vaccines Group
RAAS renin–angiotensin–aldosterone system
G-CSF granulocyte colony-stimulating factor
GM-CSF granulocyte–macrophage colony-stimulating factor
M-CSF macrophage colony-stimulating factor
HGF hepatocyte growth factor
CXCL-8 C-X-C motif chemokine ligand 8
ICU intensive care unit
RA rheumatoid arthritis
GVHD graft-versus-host disease
ICPI intracerebral pathogenicity index
Keywords: cytokine storm, immunomodulatory, natural products, SARS-CoV-2, clinical trials drugs
Citation: Mohammed MA (2023) Fighting cytokine storm and immunomodulatory deficiency: By using natural products therapy up to now. Front. Pharmacol. 14:1111329. doi: 10.3389/fphar.2023.1111329
Received: 29 November 2022; Accepted: 14 March 2023;
Published: 12 April 2023.
Edited by:
Mainul Haque, National Defence University of Malaysia, MalaysiaReviewed by:
Joyce Ogidigo, National Biotechnology Development Agency, NigeriaRajan Rolta, Shoolini University, India
Engy Elekhnawy, Tanta University, Egypt
Copyright © 2023 Mohammed. This is an open-access article distributed under the terms of the Creative Commons Attribution License (CC BY). The use, distribution or reproduction in other forums is permitted, provided the original author(s) and the copyright owner(s) are credited and that the original publication in this journal is cited, in accordance with accepted academic practice. No use, distribution or reproduction is permitted which does not comply with these terms.
*Correspondence: Mona A. Mohammed, monaarafamohammed@yahoo.com, on.ibrahim@nrc.sci.eg