- 1School of Food and Bioengineering, Food Microbiology Key Laboratory of Sichuan Province, Xihua University, Chengdu, China
- 2College of Pharmaceutical Science, Yunnan University of Chinese Medicine, Kunming, China
Acori Tatarinowii Rhizoma (ATR, Shi Chang Pu in Chinese), a natural product with multiple targets in various diseases. This review provides the comprehensive summary of the chemical composition, pharmacological effects, pharmacokinetics parameters and toxicity of ATR. The results indicated that ATR possesses a wide spectrum of chemical composition, including volatile oil, terpenoids, organic acids, flavonoids, amino acids, lignin, carbohydrates and so on. Accumulating evidence from various studies has shown that ATR exerts a wide range of pharmacological properties, including protecting nerve cells, alleviating learning and memory impairment, anti-ischemic, anti-myocardial ischemia, anti-arrhythmic, anti-tumor, anti-bacterial, and anti-oxidant activities. Currently, ATR is widely used in the central nervous system, cardiovascular system, gastrointestinal digestive system, respiratory system in China, and for the treatment of epilepsy, depression, amnesia, consciousness, anxiety, insomnia, aphasia, tinnitus, cancers, dementia, stroke, skin diseases, and other complex diseases. Pharmacokinetic studies indicated that β-asarone, α-asarone, cis-methylisoeugenol, and asarylaldehyde, the active components of ATR, were absorbed slowly after oral administration of ATR. Moreover, toxicity studies have suggested that ATR has no carcinogenic, teratogenic and mutagenic toxicity. Nevertheless, long term or high-dose toxicity testing in animals to explore the acute and chronic toxicity of acori Tatarinowii Rhizoma is still lacking. In view of good pharmacological activities, ATR is expected to be a potential drug candidate for the treatment of Alzheimer’s disease, depression, or ulcerative colitis. However, further studies are needed to elucidate its chemical composition, pharmacological effects, molecular mechanisms and targets, improve its oral bioavailability, and clarify its potential toxicity.
Introduction
Acori Tatarinowii Rhizoma (ATR, Shi Chang Pu in Chinese) is the dried rhizome of Acorus tatarinowii Schott., a perennial herb of the Araceae Juss (Yan et al., 2020b). It is first recorded in the classic works of traditional Chinese medicine “Shen Nong’s Materia Medica,” and is listed as a top grade. The effects of ATR are mainly to resuscitate, calm the mind, resolve shi (dampness) and harmonize the wei (stomach) (Lam et al., 2016b). Clinically, ATR is widely used for neurological disorders, cardiovascular system, gastrointestinal digestive system, respiratory system in China (Lam et al., 2016b; Li et al., 2018a), and for the treatment of epilepsy, depression, amnesia, consciousness, anxiety, insomnia, aphasia, tinnitus, cancers, dementia, stroke, skin diseases, and other complex diseases (Lee et al., 2004; Liu et al., 2013; Lam et al., 2019; Li J. et al., 2021). In recent years, its pharmacological research has shown that ATR has a variety of pharmacological effects, including anti-epileptic, sedative, hypnotic, anti-convulsant, anti-tussive, anti-asthmatic, anti-oxidant, anti-tumor and so on (Wu et al., 2015; Lam et al., 2017a; Fu et al., 2020; Shi et al., 2020; Zhang W. et al., 2022). Previous studies indicated that ATR is promising as a potential drug candidate for the treatment of Alzheimer’s disease (AD), depression, or ulcerative colitis. In view of the exact clinical efficacy of ATR and the continuous discovery of new pharmacological activities and active ingredients, it has been widely concerned worldwide in recent years and has become one of the hot researched Chinese medicine varieties in the medical field.
The chemical composition and pharmacological effects of ATR have been extensively reported over the past few decades, and its pharmacokinetics and toxicity have also been studied in varying degrees. However, most of the previous reports are scattered, lacking systematic summary and induction of ATR. Therefore, this review aims to provide a comprehensive summary and discussion of its chemical composition, pharmacology, pharmacokinetics and toxicity characteristics, thereby contributing to the further clinical practice and application of ATR.
Chemical composition of ATR
The chemical composition of ATR are mainly volatile components and non-volatile components. The ATR essential oil (ATEO) is considered to be the active component of ATR, and the content of ATEO is the only indicator for the determination of ATR content. At present, there are various researches on volatile parts and relatively less research on non-volatile parts. The volatile components are relatively complex, and the main structural types are phenylpropanoids (simple phenylpropanoids, lignans and coumarins) and terpenoids (monoterpenes, sesquiterpenes, diterpenoids and triterpenes). Non-volatile components are mainly alkaloids, aldehydes and acids, quinones and ketones, sterols, amino acids, and carbohydrates. The results of the ATR chemical composition study will contribute to the development of its quality research.
Volatile composition
Researchers used analytical testing techniques such as chromatography and GC-MS to analyze the chemical components of ATR from different origins, different batches, different extraction methods and different parts. Previous studies indicated that the main chemical constituents in ATR were volatile oils, which are the important indicator for quality evaluation of ATR. α-Asarone and β-asarone accounted for 95% of ATR volatile oils and were identified as characteristic components (Figure 1) (Lam et al., 2016a). The “Pharmacopoeia of The People’s Republic of China” (2020 Edition) records that the volatile oil content of ATR should not be less than 1.0% (mL/g). Currently, multiple kinds of volatile oil components were found in ATR (Table 1).
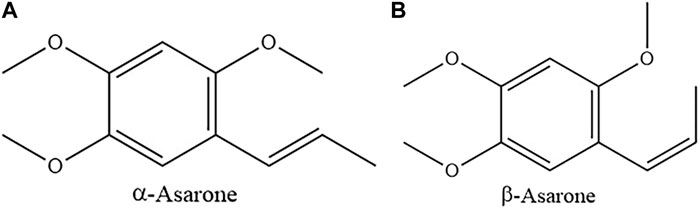
FIGURE 1. The chemical structure of α-asarone (PubChem CID: 636822) (A), and β-asarone (PubChem CID: 5281758) (B).
Non-volatile components
Most of the non-volatile components of ATR are in its aqueous extraction and organic solvent extraction parts, and the n-butanol fraction of the ethanol extract can be separated and purified to obtain alkaloids, phenylpropanoid derivatives, and furan compounds, pyrone compounds, organic acid compounds and diterpene glycoside compounds (Dong et al., 2008; Yang et al., 2021). This study summarizes the terpenoids, organic acids, and flavonoids components in ATR. Among them, the terpenoids in ATR include shyobunone, acoronene, cycloartenol, lupeol, and daucosterol (Table 2). The organic acids in ATR include fumaric acid, benzoic acid, nicotinic acid, 4-hydroxybenzoic acid, protocatechuic acid, vanillic acid, suberic acid, caffeic acid. (Table 3). The flavonoids in ATR include astragalin, rhodionin, rhoifolin, kaempferol-3-rutinoside (Table 4).
In addition to the above components, ATR also contains amino acids, lignin, carbohydrates and other chemical components. Among the amino acids, both human essential amino acids, and human semi-essential amino acids are contained. In addition, ATR also contains glucose and trace elements, alkaloids, etc. (Hu et al., 2019). The lignan components include bergapten, marmesin, eudesmin and so on. Its carbohydrate components are mainly glucose, maltose, fructose and mannose (Shi et al., 2021). Zhang et al., focused on large molecular components such as polysaccharides in ATR, speculating that the immune activity of ATR may be related to active polysaccharide components. In their study, DEAE-52 cellulose and Sephadex G-100 column chromatography were used to separate and purify polysaccharide from water chestnut base extracted polysaccharide. The eluent with absorbance greater than 0.3 in polysaccharide is collected and freeze-dried, and is named RATAPW. The average molecular weight of RATAPW was 2.51 × 104 Da, and the total carbohydrate content of RATAPW was 98.23% ± 0.29%. Monosaccharide composition, methylation and nuclear magnetic resonance (NMR) analysis showed that the polysaccharide was α-1,4-glucan with short α-1,6 branches (Zhang Y. et al., 2022).
Pharmacological effects of ATR
Effects on the central nervous system
The regulation of ATR on the central nervous system is particularly significant and has a bidirectional regulatory effect. Modern research shows that ATR and its active components α-asarone and β-asarone have effects on calming the nerves, anti-epileptic, anti-depression, anti-AD, anti-convulsant, and anti-dementia (Yang et al., 2021). Moreover, ATR and its active components also have a good protective effect on brain tissue and nerve cells, and can improve the permeability of the blood-brain barrier (BBB), promote the entry of other substances into the brain tissue through the BBB, and improve the blood concentration and bioavailability of drugs in the brain tissue (Jiang et al., 2018). The high safety and obvious curative effect of ATR in the treatment of central nervous system disease make it have a good development and application prospect. However, studies also showed that α-asarone and β-asarone could shrink the endothelial cells and loosen the tight connection, thus increasing the permeability of the BBB and assisting the drug to enter the brain tissue, which is in contradiction with the above research mechanism (Huang et al., 2016). Thus, whether asarone has protective effect on BBB needs further research to confirm.
Protective effect on nerve cells
α-Asarone and β-asarone are considered to be the main active components of ATR as a Chinese herbal medicine, and both can be potential candidates for drug development in neurodegenerative diseases. Lam et al., found that when performed to cultured rat astrocytes, ATR volatile oil, α-asarone and β-asarone could dose-dependently stimulate the expression and secretion of neurotrophic factor, namely, nerve growth factor (NGF), brain-derived neurotrophic factor (BDNF) and glial-derived neurotrophic factor (GDNF) in cultured PC12 cells (Lam et al., 2016a; Lam et al., 2017a; Lam et al., 2019). In addition, β-asarone could protect PC12 cells from amyloid beta (Aβ)-induced damage and regulates the expression of autophagy factors. Simultaneously, the cytoprotective effects of ATR oil, α-asarone and β-asarone on tert-butyl hydroperoxide (tBHP)-induced astrocyte injury were also revealed, which reduced tBHP-induced accumulation of reactive oxygen species (ROS) in astrocytes. Moreover, the activity of the transfected anti-oxidant response element (ARE) promoter construct (pARE-Luc) and the mRNAs encoding anti-oxidant enzymes were regulated by ATR oil and asarones, whose gene expression may be mediated by Akt phosphorylation (Lam et al., 2017a; Lam et al., 2017b). Moreover, ATR volatile oil, α-asarone, or β-asarone induces the transcriptional activation of neurofilament promoters and potentiates NGF-induced neurite outgrowth and neurofilament expression. Mechanism research found ATR volatile oil, α-asarone or β-asarone, induces phosphorylation of cAMP-response element binding protein (CREB) and cAMP-mediated transcriptional activity. Above all, α-asarone and β-asarone synergistically increase transcriptional activation of neurofilament promoters (Lam et al., 2016a). α-Asarone and β-asarone, as the main active ingredient in volatile oil, play a wide and important pharmacological role protecting nerve cells. Asarones or ATR volatile oil promoting axons might help to find potential agents for treating various neurodegenerative diseases.
β-Asarone could improve the degree of cerebral edema in rats with ischemia-reperfusion injury, and it have effects on reducing oxidative stress injury, inhibiting brain cell apoptosis, regulating amino acid levels and excitatory amino acid toxicity (Huang et al., 2020). Furthermore, it could increase the expression levels of Glutamic acid, Aspartic acid, and gamma-aminobutyric acid, and improve cerebral ischemia tolerance (Ke and Fang, 2003), thereby reducing the damage to neurons caused by the above substances during cerebral ischemia-reperfusion, thus playing a role in brain protection. Li et al., established a PC12 cell line with APPswe-overexpressing as a cellular model of Aβ-induced injury and assessed autophagic flux-related proteins as well as the number and morphology of autophagosomes and autolysosomes (Li Z. et al., 2021). The results indicated that β-asarone could reduce the expression levels of Beclin-1, p62, LC3-II, and Aβ1-42. β-Asarone decreased the number of autophagosomes and increased the number of autolysosomes. Studies have shown that β-asarone can protect PC12 cells from Aβ-induced damage by promoting autophagic flux, which can be achieved by enhancing autophagosome-lysosome fusion and/or lysosomal function. In addition, the essential oil is also considered as the active fraction of ATR. To investigate the anti-oxidative stress effects of ATEO, a H2O2-stressed neuronal cell model was established by Yan et al.,. ATEO treatment increased the viability of cells affected by H2O2-mediated injury, inhibited the accumulation of ROS, and induced the expression of several anti-oxidant proteins (SOD, GPx, and UCPs). It was suggested that ATEO may effectively prevent H2O2-induced cell damage by activating CREB/peroxisome proliferator-activated receptor gamma coactivator 1-alpha (PGC-1α) signaling in PC12 cells and exerting an anti-oxidative stress effect (Yan et al., 2020b). In addition, the ATR polysaccharides could protect PC12 cells from H2O2-induced injury, which could substantially stimulate nitric oxide (NO) production and phagocytic activity in RAW264.7, and promoted splenocyte proliferation (Zhang W. et al., 2015). The results showed that ATR polysaccharide could serve as a novel natural source of anti-oxidant and immune enhancer (Table 5; Figure 2).
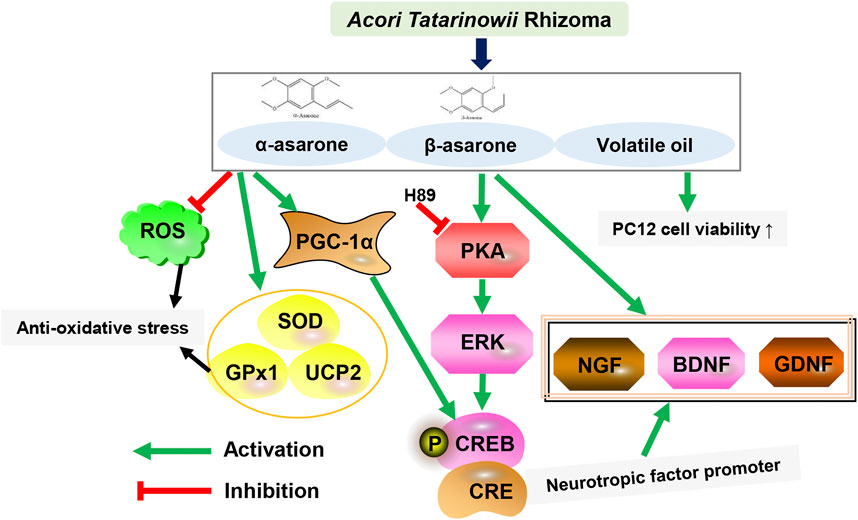
FIGURE 2. The main biological activities and potential mechanism of ATR on the central nervous system.
Effects on learning and memory impairment
ATR extract and its active ingredient, asarones could promote aberrant neural progenitor cell (NPC) proliferation. Oral administration of ATR enhanced NPC proliferation and neurogenesis in the hippocampus of adult and aged mice as well as in transgenic AD model mice. ATR and its components also enhanced the proliferation of cultured NPCs in vitro. Mechanistic studies have shown that ATR and asarones could activate the key kinase cascades in neurogenesis, extracellular signal-regulated kinase (ERK), but not Akt. Studies have shown that oral administration of ATR and asarones could be used as preventive and regenerative therapeutics to promote neurogenesis against age-related neurodegeneration and neurodegenerative disorders (Mao et al., 2015). Ning et al. (2021) explored the protective effect of Polygoni Multiflori Radix Praeparata (PMRP) combined with ATR (PA) on scopolamine-induced cognitive impairment in mice and its potential mechanism. PA was found to increase the concentration of neurotransmitters in the hippocampus, activate the BDNF/ERK/CREB signaling pathway, and increase the p90 ribosome expression of S6 kinase (p90RSK) and postsynaptic density (PSD) 95 proteins. Therefore, PA alleviates cognitive deficits by enhancing synapse-associated proteins, suggesting its therapeutic potential for the treatment of aging-related diseases such as AD (Table 5; Figure 2).
System biology research could predict the active components of ATR, as well as its corresponding targets and potential mechanism, and provide new ideas and directions for further research on the mechanism of ATR. Studies have (Song et al., 2018; Liu et al., 2020; Zhang et al., 2020) revealed a multicomponent synergistic mechanism and molecular targets of ATR in AD using a system pharmacology strategy. The results showed that the active components of ATR were involved in cancer, inflammation, cell metabolism, metabolic pathways and other related biological processes (Zhang et al., 2020), and were associated with a variety of predicted targets, such as amyloid precursor protein (APP), estrogen receptor 1 (ESR1), peroxisome proliferator activated receptor gamma (PPARG), androgen receptor (AR), muscarinic acetylcholine receptor M1 (CHRM1), caspase 3 (CASP3), janus kinase 2 (JAK2), mitogen-activated protein kinase 14 (MAPK14), prostaglandin G/H synthase 1 (PTGS1), protein kinase cAMP-activated catalytic subunit alpha (PRKACA). Moreover, most compounds in ATR have anti-fibrillar amyloid plaque, anti-inflammatory and anti-tau phosphorylation effects (Song et al., 2018). The potential mechanisms were found to be mainly involved in phosphoinositide 3-kinase (PI3K)-Akt, JAK2, MAPK, protein tyrosine phosphatase non-receptor type 1 (PTPN1) signaling pathway, neuroactive ligand-receptor interaction, as well as fluid shear stress and atherosclerosis (Liu et al., 2020; Zhang et al., 2020). ATR mainly acts on the kinase domain receptor (KDR) gene. Organ distribution showed that the targets of active ingredients were mainly located in AD-related whole blood, heart, liver, brain and muscle (Song et al., 2018; Liu et al., 2020). The network pharmacology technology has been systematically applied to the study of the target and potential mechanism corresponding to the active components of ATR, providing a new idea and direction for further study of the mechanism of ATR in AD. These findings suggested that ESR1, JAK2, PRKACA, and PTPN1 were considered as therapeutic targets for further research on ATR treatment of AD. However, more molecular biological methods are needed to demonstrate these targets.
Effects on the cardiovascular system
In recent years, the in-depth development and application of ATR in the cardiovascular field has become a research hotspot. ATR is widely used in the prevention and treatment of cardiovascular diseases such as coronary heart disease, hypertension and hyperlipidemia due to its multi-channel, multi-target and comprehensive regulation characteristics in the treatment of diseases. A series of in vitro studies have shown that ATR and its chemical components can effectively protect vascular endothelium and cardiomyocytes, and play an important role in anti-platelet aggregation, improving blood rheology, regulating blood lipids, resisting arrhythmia, lowering blood pressure, resisting myocardial hypertrophy and atherosclerosis. The current research is mainly manifested in the following aspects.
Anti-myocardial ischemia effect
Myocardial ischemia-reperfusion injury refers to the interruption of myocardial blood supply in a short period of time, and the restoration of blood supply within a certain period of time. This is an unavoidable anomaly that is clearly contrary to the purpose of treatment (Tsao et al., 2022). The volatile oil of ATR and β-asarone have anti-myocardial ischemia effects, which can reduce the levels of endothelin (ET), calcitonin gene-related peptide (CGRP), and norepinephrine (NE) in rats with myocardial ischemia, and increase NO level. In addition, it could increase serum superoxide dismutase (SOD) levels, decreased malondialdehyde (MDA) and creatine kinase (CK) levels (Zhong et al., 2019). Furthermore, Wang et al., used sodium dithionite (Na2S2O4) to induce myocardial ischemia/reperfusion injury (MI/RI) in cardiomyocytes, and explored the protective effect of the active ingredient β-asarone of ATR on myocardial cells from ischemia-reperfusion injury. It was found that β-asarone could significantly improve cell viability, reduce the content of lactate dehydrogenase (LDH) and CK in culture medium, stabilize mitochondrial membrane potential (MMP), and effectively inhibit mitochondrial damage in cardiomyocytes. The results showed that β-asarone had a significant protective effect on MI/RI cardiomyocytes (Wang et al., 2008a; Wang et al., 2008b).
Anti-arrhythmic effect
ATR has a certain anti-arrhythmic effect. Studies have found that aconitine could cause atrial, ventricular premature beats or the formation atrial tachycardia, short paroxysmal ventricular tachycardia, and ventricular tachycardia in rats. Epinephrine could cause single or multi-source premature ventricular contractions, paroxysmal ventricular tachycardia in rabbits. Barium chloride can induce bidirectional ventricular tachycardia-based arrhythmia in rabbits. The production of these arrhythmias is related to changes in autonomic nerves, mediators and myocardial excitability and automaticity. Shen et al., found that the volatile oil components of ATR may antagonize aconitine-induced arrhythmia in rats. Simultaneously, it is resistant to arrhythmias induced by epinephrine or barium chloride in rabbits (Shen et al., 1993). A certain concentration of ATR volatile oil could reduce the beating frequency of myocardial cells and improve the vitality of myocardial cells, so as to achieve the purpose of anti-arrhythmia. Specifically, 100–160 mg/L volatile oil from ATR can improve the survival rate of normal cardiomyocytes. The concentration of volatile oil from ATR is 140 mg/L, the survival rate of cardiac myocytes is at the top of the parabola. When the concentration is lower than 160 mg/L, there is no abnormal change in the morphology of cardiomyocytes. However, there are phenomena such as cell synapse thinning, retraction, and cytoplasmic shrinkage while the concentration is higher than 180 mg/L (Wu et al., 2009). Therefore, the volatile oil of ATR could reduce the beating frequency and enhance the viability of cardiomyocytes in a certain concentration, but it might have a certain adverse effect on normal cardiac myocytes when the concentration is too high.
Anti-tumor effects
The main component volatile oil of ATR, β-asarone inhibited the growth of colon cancer cells and the proliferation of gastric cancer cells (Zou et al., 2012; Wu et al., 2015). Studies have shown that 30–60 μM asarone inhibited the cell viability, proliferation, colony-forming ability, migration, invasion, and adhesion of human glioma U251 cells. It regulated the levels of key proteins involved in the death receptor pathway and mitochondrial apoptosis pathway. Simultaneously, β-asarone regulated cell cycle-related proteins and inhibited tumor growth and induces apoptosis. It inhibits epithelial-mesenchymal transition (EMT) by up-regulating E-cadherin and down-regulating vimentin, and reduces the expression of the oncogenic protein hnRNPA2/B1 in a concentration- and time-dependent manner, which may be the effect of β-asarone on glioma cell invasion and EMT (Li et al., 2018a; Li et al., 2018b). In addition, β-asarone had a significant dose-dependent inhibitory effect on the cell proliferation and induction on cell apoptosis of human gastric cancer cell lines (SGC-7901, BGC-823, and MKN-28), as well as inhibiting effect on the invasion, migration and adhesion of BGC-823 cells. Furthermore, β-asarone inhibited the gastric cancer cell growth by upregulating the expression of caspase-3, caspase-8, caspase-9, Bcl2-associated X (Bax), Bcl-2 homologous antagonist/killer (Bak), reversion-inducing cysteine-rich protein with kazal motifs (RECK), E-cadherin and downregulating MMP-2, MMP-9, MMP-14, Bcl-2, Bcl-xL, and N-cadherin (Wu et al., 2015). Tao et al. (2020) investigated the exact mechanism of β-asarone in gastric cancer. The current study showed that β-asarone had a dose-dependent inhibitory effect on three gastric cancer cell lines (MGC803, SGC7901, and MKN74) at different differentiation stages. Meanwhile, under both normoxia and CoCl2-induced hypoxia, β-asarone could induce apoptosis of gastric cancer cells and block gastric cancer cells in G2/M phase of cell cycle. Besides, it decreased LDH activity in gastric cancer cells. Mechanistically, β-asarone reduces the expression of pyruvate dehydrogenase kinase (PDK) 1, phospho(p)-PDK1, PDK4, hypoxia-inducible factor 1-α (HIF-1α), c-myc, STAT5, and p-STAT5 to influence tumor glycolysis. Ultimately, β-asarone increased chemosensitization and inhibited tumor glycolysis (Table 6). In general, there are few studies on the pharmacology and clinical application of ATR in anti-tumor, and more studies are needed to investigate the effective components, pharmacological effects and molecular biological mechanisms of ATR in anti-tumor.
Effects on the digestive system
The free-volatile oil decoction of ATR, total volatile oil, β-asarone and α-asarone could inhibit the spontaneous contraction of isolated rabbit intestine, antagonize the intestinal spasm caused by acetylcholine (Ach), histamine phosphate (Hist) and BaCl2, and enhance intestinal peristalsis in rats and intestinal propulsion in mice. In addition, these components could also promote bile secretion in rats and promote the advancement of intestinal contents in mice. The above-mentioned effect is the strongest with total volatile oil, followed by α-asarone, β-asarone, and the free-volatile oil decoction of ATR was the weakest (Hu et al., 1999). The α-asarone and β-asarone contained in the volatile oil of ATR could improve the intestinal absorption of 3,4,5-trimethoxycinnamic acid (TMCA), the active ingredient of Polygalae Radix (PR), by inhibiting the function of p-glycoprotein (P-gp) in the intestinal segment (Meng et al., 2019). At the same time, the volatile oil of calamus could inhibit the intestinal absorption of saikosaponin a and promote the intestinal absorption of ginsenosides. The mechanism of promoting absorption may be related to the inhibition of P-gp (Yang et al., 2018; Wang et al., 2019). ATR could promote digestion and regulate gastrointestinal movement. It is mainly used for stomachache and abdominal pain in clinic.
Effects on the respiratory system
Modern research shows that ATR indeed have a therapeutic effect on respiratory diseases. Li et al., investigated the anti-asthmatic effect of β-asarone on guinea pig asthma induced by ovalbumin aerosol inhalation sensitization (Li et al., 2006). The results showed that β-asarone could prolong the asthma attack latency and fall latency of model guinea pigs by spraying and gavage. However, the effect of β-asarone in spray administration was better than that in gavage administration. Xu studied the cough-relieving, phlegm-relieving and asthmatic effects of β-asarone, the active ingredient of calamus volatile oil, through experiments such as phlegm-relieving experiments in mice, cough-relieving experiments in mice, and effects on immune organs in mice (Xu, 2007). The results showed that β-asarone can increase the excretion of phenol red, which could reduce the incubation period and the number of cough attacks in cough-induced mice. Moreover, it could increase the immune organ indices of mice.
Anti-bacterial and anti-oxidant effects
To date, several publications have reported the anti-oxidant capacity of α-asarone, β-asarone (Mukherjee et al., 2008), and isoshyobunone, calacorene, and isocalamendiol are essential oils with anti-oxidant activity (Lubsandorzhieva et al., 2013). γ-asarone shows fungitoxicity against Aspergillus flavus (Varma et al., 2002). δ-Cadinene in Psidium cattleianum Sabine has anti-microbial and anti-oxidant activities (Scur et al., 2016). The volatile oil of ATR has a good inhibitory effect on staphylococcus epidermidis, group A streptococcus and shigella flexneri (Zheng et al., 2015). Qiu et al., found that the microwave water extract of ATR has effective anti-bacterial components (Liu and Qiu, 2012). The microwave water extract of ATR has obvious anti-bacterial effect on staphylococcus aureus and pseudomonas aeruginosa. Secondly, it has a certain inhibitory effect on salmonella paratyphi B, shigella sonnei, staphylococcus epidermidis, salmonella typhi, acinetobacter, shigella flexneri and escherichia coli. In addition, α-asarone could regulate the activity of matrix metalloproteinases and anti-oxidant activities (Park and Kim, 2018).
Other pharmacological effects
The RATAPW isolated from ATR by Zhang et al., can promote the production of tumor necrosis factor alpha (TNF-α) in RAW264.7 macrophages through the nuclear factor kappa B (NF-kB) molecular signaling pathway (Zhang Y. et al., 2022). Treatment with 200 μg/mL RATAPW increased the proliferation rate of spleen lymphocytes by 38.77%. RATAPW also enhanced ConA-induced T cell and lipopolysaccharide (LPS)-induced B cell proliferation in a dose-dependent manner. The research lays the foundation for the discovery of natural polysaccharide immunomodulators or functional foods from ATR.
Clinical application of compound prescription containing ATR
The composition of traditional Chinese medicine is relatively complex. In traditional Chinese medicine, which advocates syndrome differentiation and treatment, reasonable compatibility will make it play the advantage of multi-target simultaneous action. The compound formula with ATR as the main component will produce the effect of “1 plus one is greater than two” to a certain extent. Some compounds preparations, including Kaixin San (KXS), Xian-He-Cao-Chang-Yan formula (XHCYF), Longshengzhi capsule (LSZ), Smart Soup etc. contain ATR, which are also widely used in clinical treatment for various diseases.
Kaixin San (KXS)
KXS is a traditional Chinese herbal preparation with memory-enhancing properties that has been used for thousands of years in the medical care of depression, senile dementia, forgetfulness, and dizziness (Zhu et al., 2013). It consists of two functional pairs of herbs: Ginseng Radix (GR), PR, ATR, and Poria cum Radix Pini (PRP) (Zhu et al., 2016a; Zhu et al., 2016b; Yan et al., 2017; Cao et al., 2018; Dong et al., 2020; Qu et al., 2021). Qu et al., found that KXS exerts anti-depressant effects in chronic unpredictable mild stress-induced depression-like mice (Qu et al., 2021). It inhibits the activation of microglia and reduces the expression of pro-inflammatory cytokines in the mouse hippocampus. Kaixin powder extract decreased lipopolysaccharide-induced expression of inflammatory factors in BV2 cells by inhibiting toll-like receptor 4/inhibitor of kappa B kinase/nuclear factor kappa-B (TLR4/IKK/NF-κB) pathway in mice BV2 microglia cell lines. (Dong et al. (2021) also investigated the anti-depressant mechanism of KXS in a rat model of chronic mild stress induced by different stress methods. The results identified 33 differentially expressed proteins: seven upregulated and 26 downregulated. Functional analysis revealed that these differentially expressed proteins are involved in synaptic plasticity, neurodevelopment, and neurogenesis. In chronic mild stress (CMS)-induced depression in rats and in H2O2-stressed astrocytes model, KXS treatment could significantly alleviate CMS-induced depression symptoms, restore neurotransmitter quality, and increase the expressions of neurotrophic factors and their corresponding receptors, promote neurogenesis (Zhu et al., 2012). Moreover, KXS had the highest tendency to increase NGF, GDNF, and BDNF expression by activating cAMP-dependent signaling pathways as well as stimulating enzymes responsible for neurotrophic factor synthesis (Zhu et al., 2013; Zhu et al., 2016b; Cao et al., 2018). These therapeutic effects might be related to the modification of Erk1/2 and CREB phosphorylation (Yan et al., 2016). The anti-depressant-like effects of KXS might be mediated by increased neurotrophic factor expression in astrocytes and weren’t dependent on estrogen receptor or protein kinase-mediated signaling (Zhu et al., 2013). KXS could significantly enhance the expression levels of synaptotagmin and PSD95 by stimulating the cAMP-dependent pathway in chronic unpredictable mild stress (CUMS)-induced depressive rats, which is beneficial to synaptogenesis by inducing synaptic expression, possibly accounting for its anti-depressant effect in vivo and in vitro (Zhu et al., 2016a). In PC12 cultures, a single application of KXS had no effect on the neuronal differentiation, but showed robust effects in enhancing NGF-induced neurite outgrowth and neurofilament expression. Enhancement by KXS is mediated through the NGF receptor, tropomyosin receptor kinase (Trk) A (Yan et al., 2017). KXS might exert anti-depressant-like effects that induce neuronal differentiation (Zhu et al., 2016b; Yan et al., 2017; Dong et al., 2020; Qu et al., 2021), which supports the clinical use of this decoction.
Xian-He-Cao-Chang-Yan formula
Li et ai., investigated the bioactive ingredients and therapeutic mechanisms of Xian-He-Cao-Chang-Yan formula (XHCF) (composition: Agrimoniae Herba, Coptidis Rhizoma, Aucklandiae Radix, Cicadae Periostracum, ATR, and Platycodonis Radix) on dextran sulfate sodium (DSS)-induced ulcerative colitis (UC) and LPS-stimulated RAW 264.7 cells (Li J. et al., 2021). The results indicated that XHCF could effectively improve DSS-induced acute colitis. It regulates macrophage polarization and inhibits glycolysis, downregulating HK2 expression in LPS-challenged macrophages. Furthermore, XHCF enhanced the phosphorylation of adenosine 5′-monophosphate (AMP)-activated protein kinase (AMPK) both in vivo and in vitro, suggesting that AMPK is involved in XHCF function.
Longshengzhi capsule (LSZ)
LSZ has been approved by the China Food and Drug Administration for treatment of patients with cardiovascular/cerebrovascular disease. Yin et al., determined the effect of LSZ on AD processes using double transgenic mice expressing the amyloid-β precursor protein and mutant human presenilin 1 (APP/PS1) to mimic AD (Yin et al., 2020). Studies have demonstrated anti-oxidative stress, anti-inflammatory and neuroprotective effects of LSZ in AD-like pathology, and its potential mechanism was to enhance neuronal survival in HT-22 cells by partially modulating the FAS/Bcl-2/p53 pathway.
Smart Soup
Smart Soup is a traditional Chinese medicine formula composed of ATR, PRP, and PR, which is a typical anti-memory disorder prescription. Hou et al. (2014), evaluated the efficacy of SS on AD. Oral administration of SS ameliorates cognitive impairment in AD transgenic mice, reduces Aβ levels, delays Aβ amyloidosis, and reduces Aβ-induced brain gliosis and neuronal loss in AD mice. Consistently, SS treatment reduced amyloid-related motor dysfunction and premature death in AD transgenic flies. Mechanistic studies show that ATR exerts neuroprotective effects on anti-bodies and plays a role in the treatment of AD (Table 7).
Pharmacokinetics of ATR
Literature studies have found that there are relatively few pharmacokinetic studies on ATR and its active components. Ning et al. investigated the pharmacokinetic parameters of active components such as cis-methyl isoeugenol, β-asarone, α-asarone, and asarylaldehyde in ATR (2.16 g/kg, p. o.) in rats. The results indicated that β-asarone, α-asarone, cis-methylisoeugenol, and asarylaldehyde were absorbed slowly after oral administration of ATR (Tmax = 4.78, 3.54, 3.51, and 2.21 h, respectively) (Ning et al., 2021) (Table 8). Due to the limited references reporting on the pharmacokinetic parameters of ATR, more studies need to be designed to further clarify the pharmacokinetic parameters of the absorption, distribution, metabolism and excretion processes of ATR as well as its active components.
Toxicity of ATR
Currently, the toxicological studies on ATR focus on its active components. The median lethal dose (LD50) of ATR volatile oil to mice was 0.22 ± 0.055 mL/kg. At the therapeutic dose, ATR volatile oil could significantly slow down the heart rate and prolong the P-R interval (Wu et al., 2009). Preliminary experiments found that the beating frequency of cardiomyocytes cultured in vitro was slowed down. Therefore, it is speculated that ATR volatile oil acts by inhibiting myocardial excitability and automaticity (Shen et al., 1993). It is worth noting that the existing research has proved that α-asarone and β-asarone may have carcinogenicity, teratogenicity and mutagenicity toxicity (Chellian et al., 2017). Therefore, it is necessary to pay attention to the contraindications and dosage of drugs. Simultaneously, the balance between curative effect and toxic and side effects should be grasped in clinical use. According to the literature reports, ATR has anti-cancer activity (Wu et al., 2004). Nevertheless, long term or high-dose toxicity testing in animals to explore the acute and chronic toxicity of ATR is still lacking. Thus, more meaningful studies need to be further designed to explore the toxicology of ATR.
Conclusion and perspective
Summary of evidence
As a traditional Chinese medicine in China, ATR has a long history of medicinal use and a wide range of pharmacological effects. ATR treatment of diseases has the characteristics of multi-component, multi-target, and multi-pathway synergy, among which there are many active monomer components, and the potential mechanism is complex and diverse. The volatile drugs with aromatic odor are administered through nasal inhalation and smell, which has become an effective way to prevent and treat diseases of the central nervous system (Zhang et al., 2021). The volatile oil of ATR is the main pharmacological component, and it has shown definite curative effect and good application prospect in the prevention and treatment of central nervous system diseases. Modern pharmacological studies have shown that ATR has an extremely wide range of pharmacological effects, and has a good preventive effect on a variety of diseases, especially central nervous system diseases, such as anti-depression, anti-AD, anti-Parkinson’s disease (PD), anti-epileptic, anti-cerebral ischemia-reperfusion injury and other effects, which has gradually become one of the hot studies in the field of medicine (Chellian et al., 2017).
At present, the central nervous system effect of ATR is mainly related to its regulation of cholinergic system, regulation of synaptic plasticity, antioxidation and protection of neurons. ATR is often used in combination with PR and GR in the treatment of central nervous system diseases. It is composed of KXS, PR powder and other well-known prescription for intelligence. It is the representative and basic prescription for future generations of prescription for intelligence. It has a good development and application prospect because of its high safety and obvious curative effect. However, since the pathogenesis of central nervous system diseases and the chemical components of ATR are relatively complex, more comprehensive and systematic research on the pathogenesis and pharmacological effects of central nervous system diseases should be strengthened in the future to provide more sufficient theoretical basis for ATR to treat central nervous system diseases.
α-Asarone and β-asarone are isomers of each other. Because of their volatile oil properties, α-asarone and β-asarone can quickly pass through the BBB to exert pharmacological effects on the central nervous system (Lam et al., 2019), and showing a very promising application prospect. Based on the aromatic odor of α-asarone and β-asarone and their easy penetration through the BBB, nasal inhalation and olfactory administration has opened up a new way for the volatile oil of ATR to prevent and treat central nervous system diseases, especially mental diseases. This is an efficient, low-toxic, safe and simple route of administration, which is worthy of in-depth research and exploration.
Correlation between the chemical constituents and pharmacological mechanism of ATR
From this study, ATR can be used to prevent and treat central nervous system diseases, cardiovascular system diseases, gastrointestinal digestive system diseases, respiratory system diseases, etc. Therefore, this study summarizes the correlation between its chemical constituents and its pharmacological mechanism of action. A large number of experiments have shown that the volatile oil of ATR has a two-way regulation of excitation and inhibition on the central nervous system. It has antiarrhythmic, antithrombotic and protective effects on cardiac cells and blood vessels. Besides, ATR has anti-spasmodic and anti-asthmatic effects on the respiratory system and can promote digestion and regulate gastrointestinal movement for the digestive system. In terms of anti-epilepsy, α-asarone can reduce the number of discharges; linalool reduces transmitter release; methyl eugenol acts on the nervous system; polysaccharides inhibit serotonin reuptake. In terms of anti-depressant effects, β-asarone can improve behavioral disorders and increase neuronal energy supply. β-pinene can interact with monoamine systems. Linalool and artemisinin can reduce depression. Volatile oils and their aqueous extracts, methanol extracts, β-asarone, eugenol, caffeic acid, etc. can play an anti-dementia role by inhibiting β-amyloid aggregation and fiber formation, inhibiting acetylcholine esterase (AChE) activity, relieving autophagy, improving memory, and inhibiting acetylcholine activity, etc. In terms of cardiovascular activity, volatile oil, β-asarone, camphor, eugenol, elemene and caffeic acid have effect on reducing atherosclerotic blood lipids cholesterol (CHOL) and low-density lipoprotein cholesterol (LDL-C), enhancing permeability, exciting myocardium, dilating blood vessels, protecting cardiomyocytes. ATR water extract, total volatile oil, eugenol, cinnamic aldehyde, β-asarone, α-asarone block the receptors, relieve smooth muscle spasm, antagonize intestinal spasm, protect gastric function, inhibit intestinal contraction, etc. Gastrointestinal muscles and play a role in stomach. For the respiratory system, ATR can inhibit the generation of ROS, increase tracheal secretion, dilute sputum, inhibit bronchoconstriction, relax tracheal smooth muscle, block MAPK and NF-κB to exert anti-tussive and asthmatic effects. Other chemical basis and pharmacological mechanism of action are shown in Figure 3.
Research limitations and problems
Currently, the research on ATR mainly focuses on the volatile components. The traditional usage is to boil it into a water decoction, and its volatile components will be lost (Yang et al., 2021). Whether and to what extent the loss of volatile components of ATR and related preparations has an impact on the quality of the drug is unknown. Some studies have reported that its volatile components are present in both oil and water media. Whether these two media are the same and what are the similarities and differences when they exert their medicinal effects is worthy of further study. There are various studies on the volatile components of ATR, such as α-asarone and β-asarone, but less research on its non-volatile components. Thus, there are still insufficient studies on the systemic activity and pharmacological mechanism, active ingredients, pharmacokinetic characteristics of volatile and non-volatile components of ATR. Besides, the qualitative and quantitative analysis of various chemical components in ATR still needs to be detected and analyzed by ultrahigh performance liquid chromatography quadrupole time of flight mass spectrometry (UHPLC/Q-TOF-MS). In addition, there are few reports on pharmacokinetics and toxicology of ATR and its active components in the current study. Therefore, a more comprehensive and in-depth excavation of the effect of ATR will promote its new clinical use, thereby providing useful guidance for improving its medicinal value. The further study can combine ATR with modern pharmaceutical technology to explore whether it can be used as a drug carrier to assist chemical medicine to achieve the leap of BBB, reduce the dosage of chemical medicine, reduce adverse reactions, and make ATR more widely applicable. It is expected to provide scientific basis for the promotion and application of ATR in the prevention and treatment of various diseases, and can become an antiepileptic drug with significant clinical efficacy, low toxicity and more safety.
As a common traditional Chinese medicine, ATR has a long history of application in China, and is widely used in the treatment of diseases of the central nervous system, cardiovascular system, digestive system and respiratory system. However, the chemical components, pharmacological activity, toxicological effect and molecular mechanism of ATR need to be further explored. The future study should focus on clarifying the chronic toxicity and acute toxicity of ATR to further clarify its safety, so as to guide the clinical rational use of drugs and the development of new drugs. In addition, nanotechnology and chemical modification can improve the oral bioavailability of ATR and expand the scope of drug clinical treatment.
Author contributions
JW wrote and amended the manuscript. YY conceived and designed the study. JH checked crucial information of this manuscript. All data were generated in-house, and no paper mill was used. All authors agree to be accountable for all aspects of work ensuring integrity and accuracy.
Funding
This study was supported by the Xihua University Talent Introduction Project (Z211060), the introduction of talents research start-up and supporting funds of Yunnan University of Chinese Medicine, and Yunnan Provincial Science and Technology Department-Applied Basic Research Joint Special Funds of Chinese Medicine (202101AZ070001-028).
Conflict of interest
The authors declare that the research was conducted in the absence of any commercial or financial relationships that could be construed as a potential conflict of interest.
Publisher’s note
All claims expressed in this article are solely those of the authors and do not necessarily represent those of their affiliated organizations, or those of the publisher, the editors and the reviewers. Any product that may be evaluated in this article, or claim that may be made by its manufacturer, is not guaranteed or endorsed by the publisher.
References
Cao, C., Xiao, J., Liu, M., Ge, Z., Huang, R., Qi, M., et al. (2018). Active components, derived from kai-xin-san, A herbal formula, increase the expressions of neurotrophic factor NGF and BDNF on mouse astrocyte primary cultures via cAMP-dependent signaling pathway. J. Ethnopharmacol. 224, 554–562. doi:10.1016/j.jep.2018.06.007
Chellian, R., Pandy, V., and Mohamed, Z. (2017). Pharmacology and toxicology of α- and β-asarone: A review of preclinical evidence. Phytomedicine 32, 41–58. doi:10.1016/j.phymed.2017.04.003
Dong, X. Z., Wang, D. X., Zhang, T. Y., Liu, X., Liu, P., and Hu, Y. (2020). Identification of protein targets for the antidepressant effects of Kai-Xin-San in Chinese medicine using isobaric tags for relative and absolute quantitation. Neural Regen. Res. 15, 302–310. doi:10.4103/1673-5374.265555
Dong, Y., Shi, R., and Liu, B. (2007). Study on chemical compositions of acorus tatarinowii schott (Ⅰ). J. Beijing Univ. Traditional Chin. Med. 30, 61–63.
Dong, Y., Shi, R., and Liu, B. (2008). Study on chemical constituents of non-volatile parts of acorus calamus. China Pharm. 17, 18–20. doi:10.3969/j.issn.1006-4931.2008.20.015
Fu, Y., Yang, Y., Shi, J., Bishayee, K., Lin, L., Lin, Y., et al. (2020). Acori tatarinowii rhizoma extract ameliorates Alzheimer's pathological syndromes by repairing myelin injury and lowering Tau phosphorylation in mice. Pharmazie 75, 395–400. doi:10.1691/ph.2020.0492
Hou, Y., Wang, Y., Zhao, J., Li, X., Cui, J., Ding, J., et al. (2014). Smart Soup, a traditional Chinese medicine formula, ameliorates amyloid pathology and related cognitive deficits. PLoS One 9, e111215. doi:10.1371/journal.pone.0111215
Hu, J., Gu, J., and Wang, Z. (1999). Effects of Acori Tatarinowii Rhizoma and its active ingredients on the digestive system. Pharmacol. Clin. Chin. Materia Medica 15, 16–18. doi:10.1016/B978-008043005-8/50012-3
Hu, X., Tang, Y., and Yuan, J. (2019). Isolation and identification the compositions of alkaloids in Acorus tatarinowii. China Pharm. 30, 642–645.
Huang, L., Deng, M., He, Y., Lu, S., Ma, R., and Fang, Y. (2016). β-asarone and levodopa co-administration increase striatal dopamine level in 6-hydroxydopamine induced rats by modulating P-glycoprotein and tight junction proteins at the blood-brain barrier and promoting levodopa into the brain. Clin. Exp. Pharmacol. Physiol. 43, 634–643. doi:10.1111/1440-1681.12570
Huang, L., Zhu, C., Lin, L., Wang, J., and Li, L. (2020). Effects of different resuscitation-inducing herbs on excitation-sedation condition of nervous system. Acta Chin. Med. 35, 1501–1504. doi:10.16368/j.issn.1674-8999.2020.07.335
Jiang, C., Liu, X., Gong, J., and Cai, G. (2018). The research progress of calamus to regulate the permeability of blood-brain barrier and the mechanism. Ginseng Res. 30, 44–45. doi:10.19403/j.cnki.1671-1521.2018.01.013
Ke, X., and Fang, Y. (2003). Effects of volatile oil of acorus tatarinowii schott on amino acids in cerebral ischemia-reperfusion. Chin. J. Gerontology 23, 302–303.
Lam, K. Y., Chen, J., Lam, C. T., Wu, Q., Yao, P., Dong, T. T., et al. (2016a). Asarone from acori tatarinowii rhizoma potentiates the nerve growth factor-induced neuronal differentiation in cultured PC12 cells: A signaling mediated by protein kinase A. PLoS One 11, e0163337. doi:10.1371/journal.pone.0163337
Lam, K. Y. C., Huang, Y., Yao, P., Wang, H., Dong, T. T. X., Zhou, Z., et al. (2017a). Comparative study of different acorus species in potentiating neuronal differentiation in cultured PC12 cells. Phytother. Res. 31, 1757–1764. doi:10.1002/ptr.5904
Lam, K. Y. C., Wu, Q. Y., Hu, W. H., Yao, P., Wang, H. Y., Dong, T. T. X., et al. (2019). Asarones from Acori Tatarinowii Rhizoma stimulate expression and secretion of neurotrophic factors in cultured astrocytes. Neurosci. Lett. 707, 134308. doi:10.1016/j.neulet.2019.134308
Lam, K. Y. C., Yao, P., Wang, H., Duan, R., Dong, T. T. X., and Tsim, K. W. K. (2017b). Asarone from acori tatarinowii rhizome prevents oxidative stress-induced cell injury in cultured astrocytes: A signaling triggered by Akt activation. PLoS One 12, e0179077. doi:10.1371/journal.pone.0179077
Lam, K. Y., Ku, C. F., Wang, H. Y., Chan, G. K., Yao, P., Lin, H. Q., et al. (2016b). Authentication of Acori Tatarinowii Rhizoma (Shi Chang Pu) and its adulterants by morphological distinction, chemical composition and ITS sequencing. Chin. Med. 11, 41. doi:10.1186/s13020-016-0113-x
Lee, J. Y., Lee, J. Y., Yun, B. S., and Hwang, B. K. (2004). Antifungal activity of beta-asarone from rhizomes of Acorus gramineus. J. Agric. Food Chem. 52, 776–780. doi:10.1021/jf035204o
Li, G., Chen, F., Shen, L., Shen, S., Zhang, J., and Si, J. (2013). Study on chemical constituents from roots and rhizomes of Acorus tatarinowii. Chin. Traditional Herb. Drugs 44, 808–811. doi:10.7501/j.issn.0253-2670
Li, J., Li, M., Ye, K., Jiang, Q., Wang, M., Wen, X., et al. (2021). Chemical profile of Xian-He-Cao-Chang-Yan formula and its effects on ulcerative colitis. J. Ethnopharmacol. 267, 113517. doi:10.1016/j.jep.2020.113517
Li, L., Wu, M., Wang, C., Yu, Z., Wang, H., Qi, H., et al. (2018a). β-Asarone inhibits invasion and EMT in human glioma U251 cells by suppressing splicing factor HnRNP A2/B1. Molecules 23, 671. doi:10.3390/molecules23030671
Li, L., Yang, Y., Wu, M., Yu, Z., Wang, C., Dou, G., et al. (2018b). Beta-asarone induces LoVo colon cancer cell apoptosis by up-regulation of caspases through a mitochondrial pathway in vitro and in vivo. Molecules 23, 5291–5298. doi:10.7314/apjcp.2012.13.10.5291
Li, L., Zou, Y., Shi, C., Wei, G., Lin, S., Jiang, H., et al. (2006). Research on the asthma-reducing effect of asthma model in Guinea pigs treated with β-asarone through stomach-perfusion and spray administration. Chin. Archives Traditional Chin. Med. 24, 2244–2245. doi:10.13193/j.archtcm.2006.12.86.lil.040
Li, Z., Ma, J., Kuang, Z., and Jiang, Y. (2021). β-Asarone attenuates aβ-induced neuronal damage in PC12 cells overexpressing APPswe by restoring autophagic flux. Front. Pharmacol. 12, 701635. doi:10.3389/fphar.2021.701635
Liu, C., Liu, X., and Yang, H. (2006). GC-MS analysis of essential oils from Acorus Tatarinowii Schott. Chin. Archives Traditional Chin. Med. 24, 1280–1281. doi:10.13193/j.archtcm.2006.07.98.liuchh.043
Liu, L., Wang, J., Shi, L., Zhang, W., Du, X., Wang, Z., et al. (2013). β-Asarone induces senescence in colorectal cancer cells by inducing lamin B1 expression. Phytomedicine 20, 512–520. doi:10.1016/j.phymed.2012.12.008
Liu, S., He, C., Liao, Y., Liu, H., Mao, W., and Shen, Z. (2020). Enhancing and complementary mechanisms of synergistic action of acori tatarinowii rhizoma and codonopsis radix for Alzheimer's disease based on systems pharmacology. Evid. Based Complement. Altern. Med. 2020, 6317230. doi:10.1155/2020/6317230
Liu, W., Zhang, B., Xin, Z., Ren, D., and Yi, L. (2017). GC-MS fingerprinting combined with chemometric methods reveals key bioactive components in acori tatarinowii rhizoma. Int. J. Mol. Sci. 18, 1342. doi:10.3390/ijms18071342
Liu, Y., and Qiu, T. (2012). Study on anti-inflammatory and in vitro antibacterial effects of microwave water extract of Acori Tatarinowii Rhizoma. Strait Pharm. J. 24 (6), 2. doi:10.3969/j.issn.1006-3765.2012.06.008
Lubsandorzhieva, P. B., Boldanova, N. B., and Dashinamzhilov, Z. B. (2013). Chemical composition and in vitro antioxidant activity of essential oil from a hepatoprotective herbal mix. Pharm. Chem. J. 47, 58–61. doi:10.1007/s11094-013-0897-2
Mao, J., Huang, S., Liu, S., Feng, X. L., Yu, M., Liu, J., et al. (2015). A herbal medicine for Alzheimer's disease and its active constituents promote neural progenitor proliferation. Aging Cell. 14, 784–796. doi:10.1111/acel.12356
Meng, X., Jia, P., Zhang, Y., Wang, S., Zheng, X., Chen, Z., et al. (2019). Effects of Acori Tatarinowii Rhizome on intestinal absorption characteristics of Polygalae Radix based on the regulation of p-glycoprotein. Res. Pract. Chin. Med. 33, 19–23. doi:10.13728/j.1673-6427.2019.05.006
Mukherjee, P. K., Kumar, V., Mal, M., and Houghton, P. J. (2008). Acorus calamus.: Scientific validation of ayurvedic tradition from natural resources. Pharm. Biol. 45, 651–666. doi:10.1080/13880200701538724
Ni, G., and Yu, D. (2013). Chemical constituents from rhizomes of Acorus tatarinowii. China J. Chin. Materia Medica 38, 569–573. doi:10.4268/cjcmm20130420
Ning, F., Chen, L., Liu, X., Zhu, Y., Hu, J., Xie, G., et al. (2021). Combination of Polygoni Multiflori radix Praeparata and acori tatarinowii rhizoma alleviates learning and memory impairment in scopolamine-treated mice by regulating synaptic-related proteins. Front. Pharmacol. 12, 679573. doi:10.3389/fphar.2021.679573
Park, H. J., and Kim, M. M. (2018). α-asarone modulates activity of matrix metalloproteinase as well as antioxidant activity. J. Life Sci. 25, 1000–1006. doi:10.5352/jls.2015.25.9.1000
Qu, S., Liu, M., Cao, C., Wei, C., Meng, X. E., Lou, Q., et al. (2021). Chinese medicine formula kai-xin-san ameliorates neuronal inflammation of CUMS-induced depression-like mice and reduces the expressions of inflammatory factors via inhibiting TLR4/IKK/NF-κB pathways on BV2 cells. Front. Pharmacol. 12, 626949. doi:10.3389/fphar.2021.626949
Scur, M. C., Pinto, F. G., Pandini, J. A., Costa, W. F., Leite, C. W., and Temponi, L. G. (2016). Antimicrobial and antioxidant activity of essential oil and different plant extracts of Psidium cattleianum Sabine. Braz J. Biol. 76, 101–108. doi:10.1590/1519-6984.13714
Shen, J., Xiao, L., and Zhang, D. (1993). Experimental study on antiarrhythmic effect of volatile oil from Acori Tatarinowii Rhizoma. Guangzhou Med. J. 24, 44–45.
Shi, B., Liu, J., Zhang, Q., Wang, S., Jia, P., Bian, L., et al. (2020). Effect of co-administration of Acori Tatarinowii Rhizoma volatile oil on pharmacokinetic fate of xanthotoxol, oxypeucedanin hydrate, and byakangelicin from Angelicae Dahuricae Radix in rat. J. Sep. Sci. 43, 2349–2362. doi:10.1002/jssc.201901250
Shi, J., Ji, L., Luo, Q., Yu, H., Huang, S., and Li, C. (2021). Research progress on the prediction and analysis of chemical constituents, pharmacological effects and quality markers of Acori Tatarinowii Rhizoma. Chin. Tradit. Pat. Med. 43, 1286–1290. doi:10.3969/j.issn.1001-1528.2021.05.033
Song, Z., Yin, F., Xiang, B., Lan, B., and Cheng, S. (2018). Systems pharmacological approach to investigate the mechanism of acori tatarinowii rhizoma for Alzheimer's disease. Evid. Based Complement. Altern. Med. 2018, 5194016. doi:10.1155/2018/5194016
Tang, Y., Ren, G., Huang, Q., Yang, G., Liu, J., Yuan, J., et al. (2014). GC-MS analysis of the chemical constituents of Acori Tatarinowii Rhizoma volatile oil. Jiangxi J. Traditional Chin. Med. 45, 60–62.
Tao, H., Ding, X., Wu, J., Liu, S., Sun, W., Nie, M., et al. (2020). β-Asarone increases chemosensitivity by inhibiting tumor glycolysis in gastric cancer. Evid. Based Complement. Altern. Med. 2020, 6981520. doi:10.1155/2020/6981520
Tong, X., and Cheng, Y. (2011). Chemical constituents from Acorus tatarinowii. Nat. Prod. Res. Dev. 23, 404–409. doi:10.16333/j.1001-6880.2011.03.004
Tsao, C. W., Aday, A. W., Almarzooq, Z. I., Alonso, A., Beaton, A. Z., Bittencourt, M. S., et al. (2022). Heart disease and stroke statistics-2022 update: A report from the American heart association. Circulation 145, e153–e639. doi:10.1161/CIR.0000000000001052
Varma, J., Tripathi, M., Ram, V. J., Pandey, V. B., and Dubey, N. K. (2002). γ-Asarone-the fungitoxic principle of the essential oil of Caesulia axillaris. World J. Microbiol. Biotechnol. 18, 277–279. doi:10.1023/A:1014905111973
Wang, B., Pei, K., Wang, X., Chen, L., Qin, K., Wang, Y., et al. (2015). Determination of 26 volatile components in Acori Tatarinowii Rhizoma by gas chromatography-mass spectrometry. Lishizhen Med. Materia Medica Res. 26, 2627–2630.
Wang, D., Liu, X., Ji, J., Peng, D., and Zhou, A. (2019). Effect of acori tatarinowii rhizoma on intestinal absorption of ginsenosides in dingzhi xiaowan. Chin. J. Exp. Traditional Med. Formulae 25, 7–13. doi:10.13422/j.cnki.syfjx.20190552
Wang, Q., Wu, Q., and Chen, Y. (2008a). Effects of β-asarone mitochondrial membrane potential of cardiac myocytes with ischemia reperfusion injury. Traditional Chin. Drug Res. Clin. Pharmacol. 19, 451–454. doi:10.19378/j.issn.1003-9783.2008.06.012
Wang, Q., Wu, Q., and Chen, Y. (2008b). Protective effects of β-asarone against myocardial ischemia/reperfusion injury in cultured cardiac myocytes. Chin. J. Inf. TCM 15, 44–46.
Wu, H., Fang, Y., and Li, R. (2004). Pharmacological action and toxicology of acori tatarinowii rhizoma on CNS. Chin. Archives Traditional Chin. Med. 22, 127–128+132. doi:10.13193/j.archtcm.2004.01.126.wuhb.059
Wu, J., Zhang, X. X., Sun, Q. M., Chen, M., Liu, S. L., Zhang, X., et al. (2015). β-Asarone inhibits gastric cancer cell proliferation. Oncol. Rep. 34, 3043–3050. doi:10.3892/or.2015.4316
Wu, Q., Wang, S., Yuan, D., and Wu, X. (2013). Quality study of volatile oils from rhizoma acori tatarinowii. J. Guangzhou Univ. Traditional Chin. Med. 30, 72–77. doi:10.13359/j.cnki.gzxbtcm.2013.01.028
Wu, Q., Yuan, D., Wang, Q., and Wu, X. (2009). Effects of volatile oil of Rhizoma Acori Tatarinowii on morphology and cell viability in cultured cardiac myocytes. J. Chin. Med. Mater. 32, 242–245. doi:10.13863/j.issn1001-4454.2009.02.037
Wu, X., Liang, H., Wu, X., Huang, Y., Chen, J., and Liu, C. (2017). The chemical constituents of Acorus tatarinowii. J. Ningxia Med. Univ. 39, 53–55. doi:10.16050/j.cnki.issn1674-6309.2017.01.014
Xu, J. (2007). Experimental study on the therapeutic effect of Acori Tatarinowii Rhizoma on bronchial asthma. Hubei. J. Traditional Chin. Med. 29, 7–8. doi:10.3969/j.issn.1000-0704.2007.09.003
Yan, L., Hu, Q., Mak, M. S., Lou, J., Xu, S. L., Bi, C. W., et al. (2016). A Chinese herbal decoction, reformulated from Kai-Xin-San, relieves the depression-like symptoms in stressed rats and induces neurogenesis in cultured neurons. Sci. Rep. 6, 30014. doi:10.1038/srep30014
Yan, L., Liu, Z., Xu, L., Qian, Y., Song, P., and Wei, M. (2020). Identification of volatile active components in Acori Tatarinowii Rhizome essential oil from different regions in China by C6 glioma cells. BMC Complement. Med. Ther. 20, 255. doi:10.1186/s12906-020-03020-4
Yan, L., Mahady, G., Qian, Y., Song, P., Jian, T., Ding, X., et al. (2020). The essential oil from acori tatarinowii rhizome (the dried rhizome of acorus tatarinowii schott) prevents hydrogen peroxide-induced cell injury in PC12 cells: A signaling triggered by CREB/PGC-1 α activation. Evid. Based Complement. Altern. Med. 2020, 4845028. doi:10.1155/2020/4845028
Yan, L., Wei, M., Gong, A. G., Song, P., Lou, J., Bi, C. W., et al. (2017). A modified Chinese herbal decoction (Kai-Xin-San) promotes NGF-induced neuronal differentiation in PC12 cells via up-regulating trk A signaling. Front. Cell. Dev. Biol. 5, 118. doi:10.3389/fcell.2017.00118
Yang, F., Wang, Z., Li, C., and Liang, J. (2018). The effect of volatile oil of Acori Tatarinowii Rhizoma on the absorption and transport of saikosaponin in Caco-2 cell model. Mod. Med. Health Res. 2, 152–154.
Yang, H., Wu, S., Li, H., and Li, G. (2021). Research progress of calamus and prediction analysis of quality markers. Chin. J. New Drugs 30, 1213–1219. doi:10.3969/j.issn.1003-3734.2021.13.010
Yin, Z., Wang, X., Zheng, S., Cao, P., Chen, Y., Yu, M., et al. (2020). LongShengZhi capsule attenuates alzheimer-like pathology in APP/PS1 double transgenic mice by reducing neuronal oxidative stress and inflammation. Front. Aging Neurosci. 12, 582455. doi:10.3389/fnagi.2020.582455
Zhang, W., He, J., Hu, Y., Lu, J., Zhao, J., and Li, P. (2022). Chemical structure and immune activation of a glucan from rhizoma acori tatarinowii. Front. Nutr. 9, 942241. doi:10.3389/fnut.2022.942241
Zhang, W., Song, D., Xu, D., Wang, T., Chen, L., and Duan, J. (2015). Characterization of polysaccharides with antioxidant and immunological activities from Rhizoma Acori Tatarinowii. Carbohydr. Polym. 133, 154–162. doi:10.1016/j.carbpol.2015.07.018
Zhang, X., Yi, L., Deng, B., Chen, L., Shi, S., Zhuang, Y., et al. (2015). Discrimination of Acori Tatarinowii Rhizoma and Acori Calami Rhizoma based on quantitative gas chromatographic fingerprints and chemometric methods. J. Sep. Sci. 38, 4078–4085. doi:10.1002/jssc.201500730
Zhang, Y., Long, Y., Yu, S., Li, D., Yang, M., Guan, Y., et al. (2021). Natural volatile oils derived from herbal medicines: A promising therapy way for treating depressive disorder. Pharmacol. Res. 164, 105376. doi:10.1016/j.phrs.2020.105376
Zhang, Y., Tong, L., Chen, G., Deng, J., Zhang, L., Li, H., et al. (2022). Analysis of medication rule of primary epilepsy based on xiaocheng yan's clinical experience collection of epilepsy. Evid. Based Complement. Altern. Med. 2022, 9539944. doi:10.1155/2022/9539944
Zhang, Y., Wu, Y., Fu, Y., Lin, L., Lin, Y., Ji, L., et al. (2020). Anti-Alzheimer's disease molecular mechanism of acori tatarinowii rhizoma based on network pharmacology. Med. Sci. Monit. Basic Res. 26, e924203. doi:10.12659/MSMBR.924203
Zheng, Y., Yu, A., Xu, S., and Qiu, T. (2015). In vitro antibacterial activity and anti-inflammatory effect of volatile oil of Acori Tatarinowii Rhizoma. Strait Pharm. J. 27, 260–263.
Zhong, R., Wang, X., Wan, L., Shen, C., Shen, B., Wang, J., et al. (2019). Study on preparation of volatile oil from Acorus tatarinowii self-nanoemulsion dropping pills and its protective effect on acute myocardial ischemia injury. China J. Chin. Materia Medica 44, 1357–1362. doi:10.19540/j.cnki.cjcmm.20181220.006
Zhu, K. Y., Fu, Q., Xie, H. Q., Xu, S. L., Cheung, A. W., Zheng, K. Y., et al. (2010). Quality assessment of a formulated Chinese herbal decoction, kaixinsan, by using rapid resolution liquid chromatography coupled with mass spectrometry: A chemical evaluation of different historical formulae. J. Sep. Sci. 33 (23-24), 3666–3674. doi:10.1002/jssc.201000498
Zhu, K. Y., Mao, Q. Q., Ip, S. P., Choi, R. C., Dong, T. T., Lau, D. T., et al. (2012). A standardized Chinese herbal decoction, kai-xin-san, restores decreased levels of neurotransmitters and neurotrophic factors in the brain of chronic stress-induced depressive rats. Evid. Based Complement. Altern. Med. 2012, 149256. doi:10.1155/2012/149256
Zhu, K. Y., Xu, S. L., Choi, R. C., Yan, A. L., Dong, T. T., and Tsim, K. W. (2013). Kai-xin-san, a Chinese herbal decoction containing ginseng radix et rhizoma, polygalae radix, acori tatarinowii rhizoma, and poria, stimulates the expression and secretion of neurotrophic factors in cultured astrocytes. Evid. Based Complement. Altern. Med. 2013, 731385. doi:10.1155/2013/731385
Zhu, Y., Duan, X., Cheng, X., Cheng, X., Li, X., Zhang, L., et al. (2016a). Kai-Xin-San, a standardized traditional Chinese medicine formula, up-regulates the expressions of synaptic proteins on hippocampus of chronic mild stress induced depressive rats and primary cultured rat hippocampal neuron. J. Ethnopharmacol. 193, 423–432. doi:10.1016/j.jep.2016.09.037
Zhu, Y., Duan, X., Huang, F., Cheng, X., Zhang, L., Liu, P., et al. (2016b). Kai-Xin-San, a traditional Chinese medicine formula, induces neuronal differentiation of cultured PC12 cells: Modulating neurotransmitter regulation enzymes and potentiating NGF inducing neurite outgrowth. J. Ethnopharmacol. 193, 272–282. doi:10.1016/j.jep.2016.08.013
Zou, X., Liu, S. L., Zhou, J. Y., Wu, J., Ling, B. F., and Wang, R. P. (2012). Beta-asarone induces LoVo colon cancer cell apoptosis by up-regulation of caspases through a mitochondrial pathway in vitro and in vivo. Asian Pac J. Cancer Prev. 13, 5291–5298. doi:10.7314/apjcp.2012.13.10.5291
Glossary
ATR Acori Tatarinowii Rhizoma
CNKI China National Knowledge Infrastructure
VMIS VIP medicine information system
CBM Chinese Biomedical Database
ATEO The essential oil of ATR
NMR Nuclear magnetic resonance
AD Anti-Alzheimer’s disease
BBB Blood-brain barrier
NGF Nerve growth factor
BDNF Brain-derived neurotrophic factor
GDNF Glial-derived neurotrophic factor
Aβ Amyloid beta
tBHP Tert-butyl hydroperoxide
ROS Reactive oxygen species
ARE Anti-oxidant response element
CREB cAMP-response element binding protein
SOD Superoxide dismutase
NO Nitric oxide
NPC Neural progenitor cell
ERK Extracellular signal-regulated kinase
APP Amyloid precursor protein
ESR1 Estrogen receptor 1
PPARG Peroxisome proliferator activated receptor gamma
AR Androgen receptor
CHRM1 Muscarinic acetylcholine receptor M1
CASP3 Caspase 3
JAK2 Janus kinase 2
MAPK14 Mitogen-activated protein kinase 14
PTGS1 Prostaglandin G/H synthase 1
PTPN1 Protein tyrosine phosphatase non-receptor type 1
KDR Kinase domain receptor
ET Endothelin
CGRP Calcitonin gene-related peptide
NE Norepinephrine
MDA Malondialdehyde
CK Creatine kinase
MI/RI Myocardial ischemia/reperfusion injury
LDH Lactate dehydrogenase
MMP Mitochondrial membrane potential
EMT Epithelial-mesenchymal transition
Bax Bcl2-associated X
Bak Bcl-2 homologous antagonist/killer
PDK Pyruvate dehydrogenase kinase
Ach Acetylcholine
Hist Histamine phosphate
TMCA 3,4,5-trimethoxycinnamic acid
PR Polygalae Radix
P-gp P-glycoprotein
TNF-α Tumor necrosis factor alpha
NF-kB Nuclear factor kappa B
LPS Lipopolysaccharide
KXS Kaixin San
XHCYF Xian-He-Cao-Chang-Yan formula5
LSZ Longshengzhi capsule
GR Ginseng Radix
PRP Poria cum Radix Pini
TLR4/IKK/NF-κB Toll-like receptor 4/inhibitor of kappa B kinase/nuclear factor kappa-B
CMS Chronic mild stress
CUMS Chronic unpredictable mild stress
NGF Nerve growth factor
Trk Tropomyosin receptor kinase
XHCF Xian-He-Cao-Chang-Yan formula
DSS Dextran sulfate sodium
UC Ulcerative colitis
AMP Adenosine 5‘-monophosphate
AMPK Activated protein kinase
APP Amyloid-β precursor protein
PS1 Presenilin 1
PD Anti-Parkinson’s disease
AChE Acetylcholine esterase
CHOL Cholesterol
LDL-C Low-density lipoprotein cholesterol.
Keywords: Acori tatarinowii rhizoma, chemical composition, pharmacology, pharmacokinetics, toxicity, review
Citation: Wen J, Yang Y and Hao J (2023) Acori Tatarinowii Rhizoma: A comprehensive review of its chemical composition, pharmacology, pharmacokinetics and toxicity. Front. Pharmacol. 14:1090526. doi: 10.3389/fphar.2023.1090526
Received: 05 November 2022; Accepted: 06 March 2023;
Published: 16 March 2023.
Edited by:
Uraiwan Panich, Mahidol University, ThailandReviewed by:
Thomas Heinbockel, Howard University, United StatesShuang Zhang, Hefei University of Technology, China
Copyright © 2023 Wen, Yang and Hao. This is an open-access article distributed under the terms of the Creative Commons Attribution License (CC BY). The use, distribution or reproduction in other forums is permitted, provided the original author(s) and the copyright owner(s) are credited and that the original publication in this journal is cited, in accordance with accepted academic practice. No use, distribution or reproduction is permitted which does not comply with these terms.
*Correspondence: Jianxia Wen, d2VuangzMkAxNjMuY29t; Junjie Hao, MjAwNTEwMjIzOEAxNjMuY29t