- 1The Affiliated Hospital of Changchun University of Chinese Medicine, Changchun, China
- 2School of Pharmacy, Changchun University of Chinese Medicine, Changchun, China
- 3Key Laboratory of Effective Components of Traditional Chinese Medicine, Changchun, China
- 4School of Clinical Medical, Changchun University of Chinese Medicine, Changchun, China
Nephropathy is a general term for kidney diseases, which refers to changes in the structure and function of the kidney caused by various factors, resulting in pathological damage to the kidney, abnormal blood or urine components, and other diseases. The main manifestations of kidney disease include hematuria, albuminuria, edema, hypertension, anemia, lower back pain, oliguria, and other symptoms. Early detection, diagnosis, and active treatment are required to prevent chronic renal failure. The concept of nephropathy encompasses a wide range of conditions, including acute renal injury, chronic kidney disease, nephritis, renal fibrosis, and diabetic nephropathy. Some of these kidney-related diseases are interrelated and may lead to serious complications without effective control. In serious cases, it can also develop into chronic renal dysfunction and eventually end-stage renal disease. As a result, it seriously affects the quality of life of patients and places a great economic burden on society and families. Ginsenoside is one of the main active components of ginseng, with anti-inflammatory, anti-tumor, antioxidant, and other pharmacological activities. A variety of monomers in ginsenosides can play protective roles in multiple organs. According to the difference of core structure, ginsenosides can be divided into protopanaxadiol-type (including Rb1, Rb3, Rg3, Rh2, Rd and CK, etc.), and protopanaxatriol (protopanaxatriol)- type (including Rg1, Rg2 and Rh1, etc.), and other types (including Rg5, Rh4, Rh3, Rk1, and Rk3, etc.). All of these ginsenosides showed significant renal function protection, which can reduce renal damage in renal injury, nephritis, renal fibrosis, and diabetic nephropathy models. This review summarizes reports on renal function protection and the mechanisms of action of these ginsenosides in various renal injury models.
1 Introduction
Nephropathy is a general term for kidney-related diseases, which refers to changes in the structure and function of the kidney caused by various factors, resulting in pathological damage to the kidney, abnormal blood or urine components, and other diseases. The main clinical manifestations of nephrosis are hematuria, albuminuria, edema, hypertension, anemia, lower back pain, oliguria, and other symptoms. Nephropathy can be divided into glomerular, renal tubular, renal interstitial, and vascular diseases according to the main components of the disease. Chronic renal diseases may eventually lead to chronic renal failure. Renal disease can be divided into acute renal injury and chronic renal failure according to the degree, reversibility, and time of occurrence of renal function damage. Nephropathy can be divided into primary and secondary nephropathy. Primary nephrosis includes immune reaction-mediated nephritis, infectious diseases of the urinary system, renal vascular diseases, renal stones, renal tumors, and congenital nephrosis. Secondary nephropathy can be induced by tumors, metabolism, autoimmune diseases, and other diseases, which are also observed with various drugs, toxins, and other damage to the kidney. Acute kidney injury (AKI) is a prevalent critical renal disease associated with a high risk of death in hospitalized patients. Data from 2015 showed that both the incidence of AKI and mortality after AKI in inpatients exceeded 20% and the prognosis of AKI did not improve significantly (Mehta, et al. 2015). Recent studies have confirmed that renal function cannot completely recover or even require long-term renal replacement therapy in some patients with AKI. AKI eventually progresses to chronic kidney disease (CKD) or end-stage renal disease (ESRD) (Bucaloiu, et al. 2012). Nephritis is one of the most common kidney-related diseases. In clinical practice, glomerulonephritis (GN) is usually referred to as nephritis, which is an immune reaction disease (Coresh, et al. 2003). Renal fibrosis (RF) is a pathophysiological change that is a progressive process of renal function, deteriorating from health to injury until loss of function occurs. RF is also involved in the terminal pathway in CKD and nephritis. The therapeutic effects of CKD and nephritis are closely related to the degree of RF (Gewin, et al. 2017). Diabetic nephropathy (DN) is another major category of kidney-related disease. DN manifests as glomerulosclerosis, caused by diabetic microangiopathy. Once DN develops, most patients develop ESRD (Reutens and Atkins, 2011). Nephropathy, especially CKD and DN, has become a global public health issue with increasing annual prevalence. These urgent problems have prompted researchers to develop more drugs and methods for the treatment of nephropathy.
In recent years, a class of chemical drugs, including selective endothelin receptor antagonists and phosphodiesterase inhibitors, have been used to improve renal function because of their effects on reducing fasting blood glucose and glycosylated hemoglobin levels, as well as anti-inflammatory and anti-fibrosis effects. However, through the summary of previous clinical experience, it was found that some drugs are very easy to produce a variety of adverse reactions in the process of use, and can induce urinary tract infections, thus reducing the efficiency of clinical treatment. After a series of research experiments medical experts found that Chinese medicine plays an important role in the treatment of the disease, and Chinese medicine can regulate blood sugar as well as lipid metabolism to minimize kidney damage, thus creating favorable conditions for promoting early recovery.
Ginsenosides are the main active components of the traditional Chinese herbal medicine Panax ginseng C. A. Mey (Song, et al. 2022). At present, nearly 200 ginsenosides have been isolated and identified from the roots, stems, leaves, flower buds, and berries of Panax ginseng C. A. Mey. (Zhao, et al. 2022a). Ginsenosides can be divided into three classes according to their aglycone structure: protopanaxadiol (PPD), protopanaxatriol (PPT), and oleanolic acid. The PPD types mainly include ginsenosides Rb1, Rd, Rg3, Rh2, CK, and F2, etc., PPT types mainly include ginsenosides Re, Rf, Rg1, Rg2, Rh1, and F1, etc., and oleanolic acid types mainly include ginsenoside Ro (Hou, et al. 2021, Liu, et al. 2022). Modern pharmacological studies have shown that ginsenosides have neuroprotective (Zarneshan, et al. 2022), anti-aging (Meng, et al. 2022), anti-oxidant (He, et al. 2022a), anti-inflammatory (Xu, et al. 2022), and anti-cancer (Zhao, et al. 2022b), effects (Figure 1). Nowadays, many renal protective drugs have been widely used, but some of them have potential adverse effects. Monomers extracted from traditional Chinese herbal medicines have attracted considerable attention as effective and safe substitutes for kidney diseases (Gao, et al. 2017). Ginsenoside has been proven to have significant renoprotective effects and can be used as an antioxidant, anti-inflammatory, and anti-apoptotic agent. This review summarizes the protective effects and molecular mechanisms of ginsenosides in various types of kidney diseases.
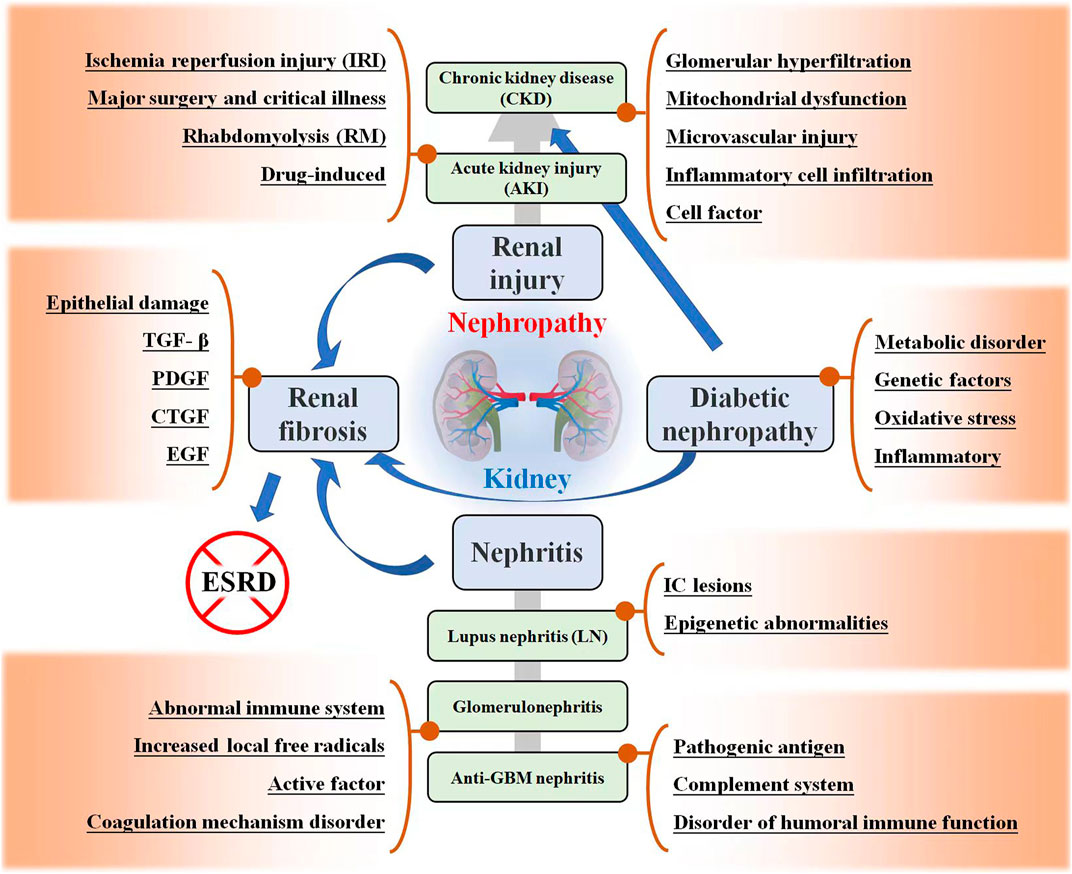
FIGURE 1. Kidney related diseases, including renal injury, renal fibrosis (RF), nephritis, and diabetic nephropathy (DN). The possible mechanisms and development trends of these diseases are listed in the figure.
2 Nephropathy
Nephropathy is a general term for common kidney diseases that seriously endanger human health, including different types of renal injury, renal failure, nephritis, renal fibrosis, and DN. The pathogenesis of kidney disease is complex and often involves multiple mechanisms, as shown in Figure 1. Different types of kidney diseases may also interact and progress. Here, the characteristics of these kidney-related diseases are introduced.
2.1 Renal injury
Acute kidney injury (AKI) is a common clinical emergency characterized by a rapid decline in renal function, which eventually leads to acute renal failure (ARF) and other organ failures. The etiology of AKI is complex and diverse and can be divided into prerenal, renal, and postrenal according to the anatomical location of the etiology. Prerenal AKI refers to a progressive decrease in blood flow perfusion in the renal parenchyma due to various causes, leading to a progressive decrease in the glomerular filtration rate (GFR). Renal AKI refers to renal parenchymal damage caused by a variety of factors, including the unrelieved renal ischemia of pre-renal AKI and damage to glomeruli, renal tubules, renal interstitium, and renal microvessels. Postrenal AKI refers to urinary tract obstruction caused by many factors and can be generally divided into intrarenal, extrarenal, and urethral obstruction (Chen and Guo, 2019). In recent years, the incidence of AKI has been increasing, and the incidence in hospitalized patients which has reached 1%–5%, has been growing rapidly. Once AKI occurs, the fatality rate of patients increases significantly, and the death rate of severe cases is >50% (Weiyun, et al. 2019).
Chronic kidney disease (CKD) is a disease of chronic renal insufficiency caused by various primary or secondary causes of renal injury and is characterized by chronic glomerular and renal tubule injury. CKD is defined as persistent urinary abnormalities, structural abnormalities, or impaired renal function, suggesting loss of functional nephrons (Romagnani et al. 2017; Diwan et al. 2018). The basic clinical manifestations of CKD include proteinuria, hematuria, hypertension, and edema. CKD pathogenesis mainly involves immune responses, cytokines, inflammatory mediators, and hemodynamic abnormalities.
Previously, it was believed that the kidney had a strong compensatory capacity, and the renal function of patients with AKI could recover better. However, recent studies have confirmed that the renal function of a considerable number of AKI patients that cannot be completely recovered, may even require long-term renal replacement therapy, eventually progressing to CKD or ESRD (Lo et al. 2009; Chawla et al. 2014). The important pathogenic mechanisms of AKI progression to CKD include glomerular hyperfiltration and hypertrophy, mitochondrial dysfunction (Zhan et al. 2013), cell infiltration and secretion of bioactive molecules (Venkatachalam et al. 2010), reduction in renal capillary density, and tubulointerstitial fibrosis (Chawla and Kimmel, 2012). Cytokines such as ET-1 (López-Farré, Gómez-Garre, et al. 1991), TGF- β (Gentle, et al. 2013), serum galectin-3 (Calvier, et al. 2013), and HIF (Nangaku, et al. 2013), play a role in these pathways.
Some chemotherapeutic drugs and chemical reagents for diagnosis and treatment may lead to drug-induced AKI. As a highly effective and broad-spectrum anticancer drug, the renal transport of cisplatin (CDDP) is regulated by proximal tubular transporters, which accumulate in proximal tubular epithelial cells, causing inflammation, injury, and cell death. More than a third of the patients receiving CDDP treatment suffer from nephrotoxicity, manifested as AKI, loss of serum sodium and magnesium, and dysfunction of the urine concentration (Miller, et al. 2010). AKI is the main complication of CDDP-induced nephrotoxicity.
2.2 Nephritis
In a narrow sense, nephritis refers to glomerulonephritis, which is generally referred to as nephritis in clinical practice. Broadly, nephritis includes pyelonephritis, glomerulonephritis (GN), and tubulointerstitial nephritis. Pyelonephritis is an inflammation of the renal pelvis and renal parenchyma caused by pathogenic microorganisms and is often accompanied by lower urinary tract infections. Glomerulonephritis, a disease caused by an immune reaction, is commonly referred to as nephritis and is mainly located in the glomerulus. Interstitial nephritis, also known as tubulointerstitial nephritis, is a clinical-pathological syndrome of acute and chronic renal tubulointerstitial damage caused by various factors.
Anti-glomerular basement membrane (GBM) nephritis is an autoimmune disease associated with the GBM antibody (Lahmer and Heemann, 2012). Nephritis belongs to type one rapidly progressive glomerulonephritis, the pathological classification of which is crescentic nephritis. Most patients have an acute onset, rapid progression, and poor prognosis (Fernandes, et al. 2016). Most untreated patients die of acute renal failure or pulmonary hemorrhage. The onset of the disease is characterized by rapid progressive nephritis syndrome.
Lupus nephritis (LN) is an immune injury caused by systemic lupus erythematosus (SLE) in different pathological kidney types. The clinical manifestations of LN are mostly similar to those of nephrotic syndrome or chronic glomerulonephritis, with edema, hematuria, proteinuria, hypertension, fever, rash, and other symptoms (Yung et al. 2017; Lin et al. 2019). LN is one of the main complications and lethal factors of SLE. LN pathogenesis mainly includes immune complex lesions (Anders and Fogo, 2014) and epigenetic abnormalities (Dieker, et al. 2007). In addition, abnormalities in the complement system (Hristova and Stoyanova, 2017), sexual hormone disorders (Feng, et al. 2010), and environmental effects are also related to the occurrence and development of LN.
2.3 Renal fibrosis
Renal fibrosis (RF) is a pathological result of long-term or repeated renal injury caused by single or multiple factors, the main pathological feature of which is excessive deposition of the extracellular matrix. The microscopic manifestation of RF is fibrosis of intrinsic renal cells, which is essentially the necrosis of intrinsic renal cells due to damage (Ke, et al. 2015). RF is the terminal stage of several chronic kidney diseases. It is a pathological process characterized by leukocyte infiltration, apoptosis, necrosis of renal tubular cells, the proliferation of tubulointerstitial fibroblasts, and deposition of extracellular matrix (Liu, 2010). Studies have shown that in addition to epithelial damage, the mechanism of RF is related to growth factors, such as transforming growth factor β (TGF-β) (Liu 2006), platelet-derived growth factor (Ostendorf, et al. 2014), connective tissue growth factor (Burns, et al. 2006), and epidermal growth factor (Stangou, et al. 2009). Renal fibrosis can be divided into two stages depending on the extent of damage to the intrinsic cells of the kidney and whether they can be repaired namely the reversible stage of fibrosis formation and progression, and the scar formation stage. The treatment of the first stage is of great significance for the rehabilitation of kidney disease and reversal of renal failure, which should be urgently addressed by doctors and patients. In the second stage, although it is possible to prevent the progression of renal fibrosis, it is difficult to repair scarred renal tissue (Humphreys, 2018).
2.4 Diabetic nephropathy
More than 30% of patients with diabetes suffer from DN, which is the main cause of morbidity and mortality. DN is characterized by early microalbuminuria, which gradually develops into massive albuminuria and progressive renal insufficiency, and finally forms ESRD. Unfortunately, once ESRD develops, the 5-year survival rate of patients is usually less than 20% (Natesan and Kim, 2021). The main pathological features of DN include glomerulosclerosis, tubulointerstitial fibrosis, and renal vascular disease. The pathogenic factors and pathogenesis are complex and include metabolic disorder (Xu, et al. 2018; Chen, et al. 2020), genetic factors (Wu, et al. 2021a), oxidative stress (Stehouwer, 2004; Sagoo and Gnudi, 2018), and inflammatory mechanisms (Wada and Makino, 2013), etc.
3 Application of ginsenosides in kidney related diseases
Ginsenosides are usually composed of 30 carbon atoms, with a 4-ring steroid structure and a sugar group. The history of separating ginsenosides from plants (e.g., ginseng, Panax notoginseng, American ginseng) can be traced back to 1854. More than 100 types of ginsenosides have been identified and successfully classified (Shin, et al. 2015). Each ginsenoside has at least two (C-3 and C-20) or three (C-3, C-6, and C-20) hydroxyl groups, which are free or linked to monomers, disaccharides, or trimers in most cases (Shin and Oh, 2016). The variety of ginsenosides is due to the different positions and the number of glycosyl groups connected by triterpene saponins, stereoisomerism of ginsenosides, and variable side chains at the C-20 position. Furthermore, it has created a variety of active ingredients, including anti-cancer, anti-diabetes, anti-fatigue, anti-aging, liver protection, and for kidney protection (Yenisetti et al. 2016). According to the position of the sugar group at C-3 and C-6, ginsenosides are divided into three categories: protopanaxadiol (PPD)-type saponins, protopanaxatriol (PPT)-type saponins, and oleanolic acid-type saponins (others) (Qu, et al. 2009), as shown in Figure 2. Ginsenosides with a high content of ginseng, such as Re, Rg1, Rd, and Rb1, contain many sugar residues, which makes it impossible or difficult to be directly absorbed and utilized by the human body after ingestion. These ginsenosides usually need to be metabolized and converted into smaller molecules before being absorbed by the human body, which greatly affects their biological activities. After chemical or biological transformation, the main ginsenosides can be metabolized into rare ginsenosides such as Rg3, Rk1, Rg5, CK, Rk3, and Rh4. These rare saponins show higher pharmacological activity owing to the reduction of sugar residues linked to their molecular structure, increased hydrophobicity, and enhanced cellular penetration. In addition, a variety of ginsenosides have been shown to exert anti-cancer, anti-diabetic, anti-fatigue, anti-aging, hepatoprotective and renoprotective effects (Yenisetti et al. 2016). Numerous studies have shown that ginsenosides can protect the kidneys from damage through different pathways. For example, Ginsenoside Rb1 can treat acute kidney injury by activating the Nrf2/ARE pathway (Sun et al. 2012). The mechanisms of ginsenosides to alleviate renal diseases by improving glucolipid metabolism, inhibiting oxidative stress, anti-inflammation, anti-apoptosis, regulating autophagy, and anti-fibrosis are highlighted here, as shown in Table 1.
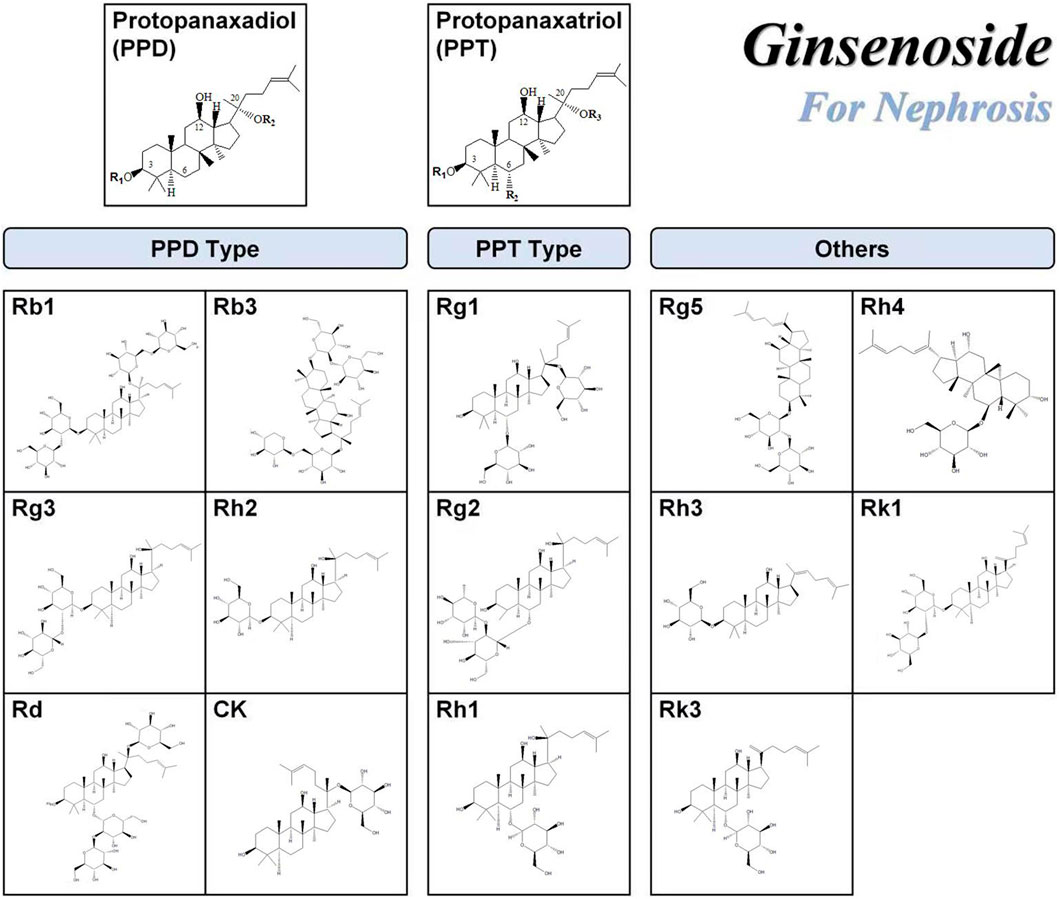
FIGURE 2. Chemical structures of ginsenosides with renal function protection. The chemical structures of the ginsenosides included in this publication were drawn using the ChemDraw program.
3.1 PPD type ginsenosides
The PPD type ginsenoside uses PPD as an aglycone. Generally, ginsenosides are divided into two configurations: 20 S)—PPD and 20 R)—PPD, according to the substitution of C-3 or C-20 hydroxyl groups of aglycones by different sugar groups. The structural formulas of common PPD-type ginsenosides are shown in Figure 1. The PPD ginsenoside group has been shown to have significant pharmacological activities, including ginsenosides Rb1, Rb2, Rb3, RC, Rg3, Rh2, Rd, and CK (Table 2). PPD has good biological activities such as antioxidant and anti-inflammatory (Yang et al. 2019). Several studies have reported the nephroprotective effects of PPD and related mechanisms.
3.1.1 Ginsenoside Rb1
Ginsenoside Rb1 is one of the main active monomers of ginseng and has the ability to scavenge oxygen free radicals and thus has antioxidant activity. Ginsenoside Rb1 has been shown to inhibit renal ischemia-reperfusion injury and interstitial fibrosis and reduce renal cell apoptosis and oxidative damage. For instance, ginsenoside Rb1 upregulates Nrf2 and heme oxygenase-1 (HO-1) by activating the nuclear factor-related factor 2 (Nrf2)/ARE pathway, which in turn attenuates acute kidney injury caused by intestinal ischemia-reperfusion (Sun et al. 2012). In addition, the possible protective effects and mechanisms of ginsenoside Rb1 on oxidative damage and renal interstitial fibrosis in rats with unilateral ureteral obstruction (UUO) have been widely studied. Fan et al. found that ginsenoside Rb1 significantly inhibited renal interstitial fibrosis in UUO rats by down-regulating TGF-β1 (Xie et al. 2009) expression or ihibiting Bip/eIF2α/CHOP signaling-mediated EMT (Ni et al. 2022). There are reports that ginsenoside Rb1 treats acute kidney injury by activating the Nrf2/ARE pathway which acts against oxidative stress (Sun et al. 2012). Ginsenoside Rb1 can further prevent autophagy by inhibiting the Wnt/β-catenin pathway (Xu, et al. 2017) or by regulating Akt-independent (cell proliferation and survival) and AMPK-dependent mTOR signaling-involved in cell survival under energy stress (Zhou et al. 2019), thereby reducing oxidative stress and inflammation in patients with CKD (Zhang, et al. 2022). In detail, ginsenoside Rg1 treatment significantly reduced ROS production and inhibited NOX4 and NLRP3 inflammatory vesicle activation, which in turn ameliorated LPS-induced chronic kidney injury and renal fibrosis (Zhang et al. 2022). Rb1 can also inhibit the Wnt/β-linked protein pathway by activating peroxisome proliferator-activated receptor γ (PPAR-γ) to exert anti-calcium properties and thus improve the symptoms of CKD. In recent years, studies have found that ginsenoside Rb1 can regulate Type two diabetic nephropathy by regulating the expression of miR-3550 and further combining with Wnt/β-catenin signaling (Shao et al. 2019) or inhibiting aldose reductase activity (He et al. 2022b).
3.1.2 Ginsenoside Rb3
Ginsenoside Rb3, which is one of the main pharmacologically active ingredients, mainly exists in the roots, flower buds, stems, and leaves of Panax ginseng; the roots, stems, and leaves of Panax quinquefolium; and the stems and leaves of Panax notoginseng. According to reports, ginsenoside Rb3 can regulate cisplatin-induced AKI by regulating AMPK/mTOR-mediated autophagy and inhibiting apoptosis in vitro and in vivo (Xing et al. 2019). Among them, Li et al. demonstrated for the first time the protective effect and potential mechanism of ginsenoside Rb3 on cisplatin-induced renal failure, restoring the antioxidant system by regulating the AMPK/mTOR signaling pathway, and inhibiting proximal tubular damage by inhibiting ROS-mediated apoptosis and autophagy. Some evidence suggests that the TGF-β pathway may lead to cisplatin-induced nephrotoxicity, and ginsenoside Rb3 can have a protective effect on nephrotoxicity in the treatment of oral cancer with CPT through TGF-β pathway-mediated mitochondrial apoptosis (Wu et al. 2021b).
3.1.3 Ginsenoside Rd
Ginsenoside Rd is a rare type of saponin. The content of Rd in ginseng is very low, while that in Panax notoginseng is approximately 0.36%–1.47%, which is higher than that in ginseng. Intestinal enzymes can metabolize Rb1 with high content into Rd; therefore, Rd is one of the important forms of saponins that are absorbed and utilized by the intestine after metabolism. Recent studies have found that ginsenoside Rd has strong biological activity, especially protective effects on the kidneys. For example, ginsenosides-Rd eliminate the damaging effects of oxidative stress on the kidneys by inhibiting free radical lipid peroxidation (Yokozawa and Liu 2000). Moreover, ginsenoside Rd can regulate cisplatin-induced AKI by inhibiting free radical-mediated lipid peroxidation while inhibiting apoptosis (Yokozawa and Dong 2001). In the research of exploring new anti-kidney cancer drugs, ginsenoside Rd has played an important role. Studies have said that ginsenoside Rd can regulate kidney cancer by inhibiting TRPM7 channel activity (Kim 2013).
3.1.4 Ginsenoside Rg3
Ginsenoside Rg3 is one of the main active substances in ginseng and has extensive pharmacological effects. With the new foci of the research, Rg3 was found to have anti-tumor effects (Sin et al. 2012; Ding et al. 2015), reducing the cardiotoxicity and nephrotoxicity of chemotherapy drugs (Han et al. 2016), and anti-cicatricial (Tang et al. 2018). Through literature reading, we find that there is a growing number of studies focusing on ginsenoside Rg3 to improve acute kidney injury. For example, Rg3 can regulate D-galactose-induced AKI by inhibiting the renal oxidative stress caused by d-galactose and simultaneously activating the PI3K/AKT signaling pathway to attenuate the apoptosis of liver and kidney cells (Sun, et al. 2013). Rg3 can regulate LPS-induced AKI by reducing the expression of NF-kB and iNOS proteins and reducing the expression of COX-2 and HO-1 proteins (Kang, et al. 2007). Numerous studies have also shown that ginsenoside Rg3 can improve cisplatin-induced AKI by modulating multiple pathways. In details, ginsenosides Rg3 can regulate cisplatin-induced AKI by regulating PI3K/AKT and NF-κB-mediated apoptosis and inflammatory pathways (Zhang et al. 2021). Ginsenosides Rg3 also reduces cisplatin-induced AKI by inhibiting apoptosis and autophagy to suppress NLRP3 (Zhai et al. 2021) and blocking the JNK-p53-cysteine asparticase-3 signaling cascade (Han et al. 2016). In addition, Rg3 can also regulate type 2 diabetic nephropathy mainly focusing on pathways that regulate MAPK/NF-κB signaling pathway (Li et al. 2021), inhibit inflammation and oxidative stress response (Zhou et al. 2020) and late glycosylation end product formation (Kang et al. 2010b). There have also been recent studies that Rg3 can regulate kidney cancer by blockading of TRPM7 channel activity (Kim et al. 2011).
3.1.5 Ginsenoside Rh2
Ginsenoside Rh2 is a rare saponin found in Panax ginseng. Rh2 was first found in red ginseng and was later isolated from American ginseng, Panax notoginseng, and other plants. Rh2 can regulate the immune, central nervous, endocrine, and cardiovascular systems, etc., and has anti-tumor, anti-allergy, anti-depression, anti-aging, and improved myocardial ischemic effects. Ginsenoside Rh2 regulates cisplatin-induced AKI by acting on a caspase-mediated pathway (Qi, et al. 2019). Rh2 regulates type two diabetic nephropathy by downregulating discoid domain receptor 1 (Shen, et al. 2021).
3.1.6 Ginsenoside CK(M1)
Ginsenoside CK does not exist in natural ginseng but is a metabolite produced by diol-type ginsenosides (such as Rb1, Rb2, and Rc) under the action of intestinal flora after oral administration (Chen, et al. 2015). CK is one of the main components of ginsenosides that play a role in the body with high biological activity, including inhibition of T Cells (Kang, et al. 2010a), promotion of tumor cell apoptosis (Cho, et al. 2009), anti-inflammation (Joh et al. 2011) and protection of the myocardium (Tsutsumi, et al. 2011). Ginsenoside CK can modulate primary glomerulonephritis by inhibiting NLRP3 inflammasome activation in renal tissue, macrophages, and bone marrow-derived dendritic cells, increasing SIRT1 expression, and triggering autophagy-mediated inhibition of NLRP3 inflammable bodies (Wu, et al. 2020). Ginsenoside CK can regulate tubulointerstitial nephritis by inhibiting NLRP3 inflammasome initiation and mitochondria-related activation signaling in tubulointerstitial lesions (Hsu, et al. 2020). Ginsenoside CK can regulate type two DN by inhibiting NLRP3 inflammasome activation and the NF-κB/p38 signaling pathway in DN in high-fat diet/streptozotocin-induced diabetic mice (Song, et al. 2018) enhancing antioxidant capacity, and reducing the damage of TGF-β1 in renal tissue (Shao, et al. 2015). Ginsenoside CK regulates kidney cancer by regulating reactive oxygen species (ROS) and Testis-associated highly conserved oncogenic long-stranded non-coding RNA (LNRNA THOR) (Chen, et al. 2021). Ginsenoside M1 can regulate acute severe lupus nephritis by inhibiting NLRP3 inflammasome activation and differentially regulating T-cell function (Lin, et al. 2019).
3.2 PPT type ginsenosides
PPT-type ginsenosides take protopanaxatriol as an aglycone. Generally, ginsenosides are divided into two configurations: 20 S)—PPT and 20 R)—PPT, according to the substitution of C-6 or C-20 hydroxyl groups of aglycones by different sugar groups. The structural formulae of common PPT-type ginsenosides are shown in Figure 1. The PPT ginsenoside group has been shown to have significant pharmacological activities, mainly Re, Rg1, Rg2, and Rh1, as shown in Table 3.
3.2.1 Ginsenoside Rg1
Among all the PPT saponins, ginsenoside Rg1 ranks second only to ginsenoside Re. In addition, studies have shown that the ginsenoside Rg1 content in Panax notoginseng is high (Rg1 accounts for 20% of the total saponins of Panax notoginseng, and Re is only 2.5%), and thus has great development value (Yang, et al. 2015). Rg1 has many functions including anti-aging, anti-oxidation, and improved immunity and memory (monakhov, Baisong et al. 2018). Ginsenoside Rg1 can regulate D-galactose-induced AKI by preventing DNA damage by attenuating oxidative stress (Fan, et al. 2016). Rg1 can regulate CKD by inhibiting NOX4-NLRP3 inflammazone signaling pathways in mice (Liu, et al. 2010). Rg1 can regulate anti-GBM GN, a rare autoimmune disease, by increasing renal blood flow (Hattori, et al. 1991) and activating NRF2 signaling (Guo, et al. 2019). Ginsenoside Rg1 can inhibit the development of renal fibrosis by modulating various pathways, such as the downregulation of protein TGF-β1 expression (Xie, et al. 2008a), blocking TEMT by inhibiting the expression of TSP-1 (Xie et al. 2008b), thereby inhibiting the activation of TGF-β1 (Li, et al. 2015), inhibiting endoplasmic reticulum stress-induced apoptosis in rats after unilateral ureteral obstruction (Xie et al. 2008c), inhibiting TGF-β1-induced transdifferentiation of rat renal tubular epithelial cells (Shen, et al. 2020), inhibiting NOX4 and NLRP3 inflammasome activation in SAMP8 mice, and regulating the Klotho/TGF-β1/Smad signaling pathway (Li et al. 2018). Ginsenoside Rg1 can inhibit the development of type 2 DN by regulating the PI3K/AKT/FOXO3 pathway (Liu, et al. 2021), reducing the expression of TGF-β1 and the already mentioned inflammatory response factors in renal tissue (Ma et al. 2010), and reducing the expression of TNF-α and MCP-1 (Zhang, et al. 2009). The combination of Rg1 and Astragalus IV can reduce oxidative stress and inhibit the TGF-β1/Smad signaling cascade in renal fibrosis in rats with DN (Du, et al. 2018).
3.2.2 Ginsenoside Rg2 and Rh1
Rg2 is an intermediate product of ginsenoside Re metabolism in vivo. Rg2 has many biological activities, such as affecting the sensitivity of the opposite nerve cell process receptors, promoting intercellular communication, and reducing the neural activity caused by electricity in the rat hippocampus (Sala, et al. 2002). Ginsenoside Rg2 and Rh1 can regulate AKI by blocking LPS-TLR4 signaling, which reduces p38-STAT1 activation and NF-κB translocation, which in turn suppresses the transcription of inflammatory cytokines and mediators, such as IFN-β, TNF-α, IL-1β, and iNOS (Huynh, et al. 2020).
3.2.3 Ginsenoside Rh1
Ginsenoside Rh1 is a rare saponin found in red ginseng, Panax notoginseng, and American ginseng in trace (Jeon, et al. 2020). Because of its remarkable immunoregulatory activity, Rh1 has high medicinal value in the treatment of many senile diseases (Tam, et al. 2018). In addition, Rh1 can inhibit inflammatory reaction (Vinh et al. 2017), regulate abnormal immune responses in hypersensitive disease (Han and Kim, 2020), and inhibit tumor cell proliferation (Yi, 2019). Ginsenoside Rh1 can regulate type two DN by regulating oxidative stress, angiotensin II (Ang-II), and inflammatory processes, as well as AMPK/PI3K/Akt-mediated inflammatory and apoptosis signaling pathways (Su, et al. 2021).
3.3 Other ginsenosides
Other types of ginsenosides include dammarane-type tetracyclic triterpenoids. Some of these saponins are isolated from ginseng with very little content, whereas others are obtained after chemical treatment. Compared with the original PPD- and PPT-type ginsenosides, only the skeleton of aglycone or the side chain of the parent nucleus was partially changed.
3.3.1 Ginsenoside Rg5
Ginsenoside Rg5 is a derivative of ginsenoside, one of the main components of red ginseng (Kim, et al. 1996). Rg5 is a secondary saponin obtained from PPD-type saponins (Rb1, Rb2, Rb3, Rc, and Rd) via regioselective hydrolysis and stereoselective dehydration (Lee, et al. 2009). In animal and human clinical trials, Rg5 not only has significant effects in improving lung inflammation (Kim et al. 2012) and improving memory (Yao et al. 2014), anti-cancer (Liang et al. 2015), but also reduces cisplatin induced nephrotoxicity (Li et al. 2016). Ginsenoside Rh3 can regulate cisplatin-induced AKI by inhibiting inflammation, oxidative stress, and apoptosis (Li et al. 2016). Ginsenoside Rh3 can inhibit the development of type two diabetic nephropathy by inhibiting NLRP3 inflammasome activation and the MAPK signaling pathway in high-fat diet/streptozotocin-induced diabetic mice (Zhu, et al. 2020).
3.3.2 Ginsenoside Rh3
Ginsenoside Rh3 is a metabolite of ginsenoside Rg5 in the human body (Kim et al. 2013), but it has better effects on various pharmacological activities than Rg5 (Shin et al. 2006). Ginsenoside Rh3 can regulate cisplatin-induced AKI by inhibiting JNK and ERK mitogen-activated protein kinase signaling cascades (Lee and Kang, 2017).
3.3.3 Ginsenoside Rg3, Rg5 and Rk1
Black ginseng, a new processed product of ginseng, has a unique processing method (Gao, et al. 2017). Black ginseng contains rare saponins that are different from ginseng and red ginseng, and its representative active components are Rg3, Rg5, and Rk1 (Gao, et al. 2017). As a tetracyclic triterpene discovered in recent years, Rk1 has attracted much attention because of its biological activities, such as antitumor, blood glucose regulation, and nervous system protection (Gao, et al. 2017). Ginsenoside Rk1 regulates cisplatin-induced AKI by regulating inflammation and apoptosis (Park, et al. 2015).
3.3.4 Ginsenoside Rh4 and Rk3
Ginsenoside Rk3/Rh4 comprises a pair of isomers, which are obtained by removing a sugar group from ginsenoside Rg1 and converting it to Rh1, and then removing a water molecule at C20 position from Rh1. Ginsenoside Rh4 and Rk3 can regulate cisplatin-induced AKI by inhibiting oxidation (Baek, et al. 2006).
4 Prospect and conclusion
Nowadays, kidney-related diseases have become common worldwide, such as diabetic nephropathy, chronic kidney disease, acute kidney disease, and hypertensive nephropathy. The causes of kidney diseases are complex, and since the pathogenesis of most kidney diseases is not fully understood, the treatment of kidney diseases is mostly empirical and lacks etiologic treatment tools (Liu and Xiao 1992). At the same time, many drugs that protect the kidney have been widely used, but some of them have strong adverse effects. For this reason, monomeric components derived from traditional Chinese herbal medicines have received much attention as effective and safe alternative drugs for kidney diseases. Currently, ginsenosides as natural drugs have been widely approved to exert therapeutic effects on kidney-related drugs in vivo and in vitro. In the existing studies, we found that ginsenosides for the treatment of nephropathy mainly focus on mechanisms through the inhibition of inflammatory responses and oxidative stress. Emerging reports suggest that ginsenosides can have some binding activity to the glucocorticoid receptor and can promote its nuclear translocation, while some ginsenosides can exert anti-inflammatory effects by inhibiting the activity of NF-κB. Recent findings have confirmed that NF-κB is closely associated with foot cell injury. This shows that NF-κB pathway is a hot pathway for kidney disease research. Current research mainly focuses on ginsenosides, with little research on other components such as ginsenosides polysaccharides, which should be more widely explored in depth. The efficacy of ginsenosides in the treatment of kidney disease has become clear, and in the future, in-depth research on ginsenosides in the treatment of kidney disease can be conducted from multiple angles, levels and directions to provide better treatment for patients.
In conclusion, the pathogenesis of several major kidney-related diseases is discussed in this review. The mechanism of ginsenosides in various types of nephropathy has been summarized, including PPD and PPT types. Although ginsenosides have been studied in nephropathy, their mechanism of action has not been fully elucidated. The animal model of nephropathy was used as the basis for further research and discussion of the pathogenesis and mechanism of drug action. The establishment of animal models of nephropathy should be consistent with the pathology and course of kidney disease, which is a goal of researchers. Therefore, it is necessary to further study other related effects of ginsenosides on kidney-related diseases through appropriate animal models, which are expected to develop new drugs for the clinical treatment of drug-induced nephrotoxic diseases, diabetic nephropathy, renal fibrosis, and other kidney diseases.
Author contributions
MF and XL contributed equally to this work; WG and DZ designed the review; MF, XL, QW, MS, XF, YZ, DW, and HL contributed to the writing of the manuscript.
Funding
This work was supported by the National Natural Science Foundation of China (Grant Number: 81973468), Jilin province science and technology development plan item (Grant Number: 20200708081YY), Jilin Provincial Development and Reform Commission (Grant Number: 2021C035-5).
Conflict of interest
The authors declare that the research was conducted in the absence of any commercial or financial relationships that could be construed as a potential conflict of interest.
The handling editor declared a past collaboration with the author MF.
Publisher’s note
All claims expressed in this article are solely those of the authors and do not necessarily represent those of their affiliated organizations, or those of the publisher, the editors and the reviewers. Any product that may be evaluated in this article, or claim that may be made by its manufacturer, is not guaranteed or endorsed by the publisher.
References
Anders, H. J., and Fogo, A. B. (2014). Immunopathology of lupus nephritis. Semin Immunopathol 36 (4), 443–459. doi:10.1007/s00281-013-0413-5
Bae, E. A., Han, M. J., Kim, E. J., and Kim, D. H. (2004). Transformation of ginseng saponins to ginsenoside Rh2 by acids and human intestinal bacteria and biological activities of their transformants. Arch Pharm Res 27 (1), 61–67. doi:10.1007/BF02980048
Baek, S. H., Piao, X. L., Lee, U. J., Kim, H. Y., and Park, J. H. (2006). Reduction of Cisplatin-induced nephrotoxicity by ginsenosides isolated from processed ginseng in cultured renal tubular cells. Biol Pharm Bull 29 (10), 2051–2055. doi:10.1248/bpb.29.2051
Baisong, Z., [j], K. m., Yameng, L., and li, P.-y. (2018). Progress in bioactivity of Ginsenoside Rg1 %J. Chinese Journal of Traditional Chinese Medicine 33 (04), 1463–1465.
Berek, L., Szabó, D., Petri, I. B., Shoyama, Y., Lin, Y. H., and Molnár, J. (2001). Effects of naturally occurring glucosides, solasodine glucosides, ginsenosides and parishin derivatives on multidrug resistance of lymphoma cells and leukocyte functions. Vivo 15 (2), 151–156.
Bucaloiu, I. D., Kirchner, H. L., Norfolk, E. R., Hartle, J. E., and Perkins, R. M. (2012). Increased risk of death and de novo chronic kidney disease following reversible acute kidney injury. Kidney Int 81 (5), 477–485. doi:10.1038/ki.2011.405
Burns, W. C., Twigg, S. M., Forbes, J. M., Pete, J., Tikellis, C., Thallas-Bonke, V., Thomas, M. C., Cooper, M. E., and Kantharidis, P. (2006). Connective tissue growth factor plays an important role in advanced glycation end product-induced tubular epithelial-to-mesenchymal transition: implications for diabetic renal disease. J Am Soc Nephrol 17 (9), 2484–2494. doi:10.1681/ASN.2006050525
Cai, J., Huang, K., Han, S., Chen, R., Li, Z., Chen, Y., Chen, B., Li, S., Xinhua, L., and Yao, H. (2022). A comprehensive system review of pharmacological effects and relative mechanisms of Ginsenoside Re: Recent advances and future perspectives. Phytomedicine 102, 154119. doi:10.1016/j.phymed.2022.154119
Calvier, L., Miana, M., Reboul, P., Cachofeiro, V., Martinez-Martinez, E., de Boer, R. A., Poirier, F., Lacolley, P., Zannad, F., Rossignol, P., and López-Andrés, N. (2013). Galectin-3 mediates aldosterone-induced vascular fibrosis. Arterioscler Thromb Vasc Biol 33 (1), 67–75. doi:10.1161/ATVBAHA.112.300569
Chan, R. Y., Chen, W. F., Dong, A., Guo, D., and Wong, M. S. (2002). Estrogen-like activity of ginsenoside Rg1 derived from Panax notoginseng. J Clin Endocrinol Metab 87 (8), 3691–3695. doi:10.1210/jcem.87.8.8717
Chawla, L. S., and Kimmel, P. L. (2012). Acute kidney injury and chronic kidney disease: an integrated clinical syndrome. Kidney Int 82 (5), 516–524. doi:10.1038/ki.2012.208
Chawla, L. S., Eggers, P. W., Star, R. A., and Kimmel, P. L. (2014). Acute kidney injury and chronic kidney disease as interconnected syndromes. N Engl J Med 371 (1), 58–66. doi:10.1056/NEJMra1214243
Chen, ling, and guo, J. p. (2019). Research progress of Radix sophora nigricans. %J Ginseng study 31 (01), 42–46.
Chen, J., Wu, H., Wang, Q., Chang, Y., Liu, K., and Wei, W. (2015). Ginsenoside metabolite compound K suppresses T-cell priming via modulation of dendritic cell trafficking and costimulatory signals, resulting in alleviation of collagen-induced arthritis. J Pharmacol Exp Ther 353 (1), 71–79. doi:10.1124/jpet.114.220665
Chen, Y., Chen, J., Jiang, M., Fu, Y., Zhu, Y., Jiao, N., Liu, L., Du, Q., Wu, H., Xu, H., and Sun, J. (2020). Loganin and catalpol exert cooperative ameliorating effects on podocyte apoptosis upon diabetic nephropathy by targeting AGEs-RAGE signaling. Life Sci 252, 117653. doi:10.1016/j.lfs.2020.117653
Chen, S., Ye, H., Gong, F., Mao, S., Li, C., Xu, B., Ren, Y., and Yu, R. (2021). Ginsenoside compound K exerts antitumour effects in renal cell carcinoma via regulation of ROS and lncRNA THOR. Oncol Rep 45 (4), 38. doi:10.3892/or.2021.7989
Cho, S. H., Chung, K. S., Choi, J. H., Kim, D. H., and Lee, K. T. (2009). Compound K, a metabolite of ginseng saponin, induces apoptosis via caspase-8-dependent pathway in HL-60 human leukemia cells. BMC Cancer 9, 449. doi:10.1186/1471-2407-9-449
Coresh, J., Astor, B. C., Greene, T., Eknoyan, G., and Levey, A. S. (2003). Prevalence of chronic kidney disease and decreased kidney function in the adult US population: Third National Health and Nutrition Examination Survey. Am J Kidney Dis 41 (1), 1–12. doi:10.1053/ajkd.2003.50007
Dieker, J. W., Fransen, J. H., van Bavel, C. C., Briand, J. P., Jacobs, C. W., Muller, S., Berden, J. H., and van der Vlag, J. (2007). Apoptosis-induced acetylation of histones is pathogenic in systemic lupus erythematosus. Arthritis Rheum 56 (6), 1921–1933. doi:10.1002/art.22646
Ding, S., Li, C., Cheng, N., Cui, X., Xu, X., and Zhou, G. (2015). Redox Regulation in Cancer Stem Cells. Oxid Med Cell Longev 2015, 750798. doi:10.1155/2015/750798
Diwan, V., Brown, L., and Gobe, G. C. (2018). Adenine-induced chronic kidney disease in rats. Nephrology (Carlton) 23 (1), 5–11. doi:10.1111/nep.13180
Du, N., Xu, Z., Gao, M., Liu, P., Sun, B., and Cao, X. (2018). Combination of Ginsenoside Rg1 and Astragaloside IV reduces oxidative stress and inhibits TGF-β1/Smads signaling cascade on renal fibrosis in rats with diabetic nephropathy. Drug Des Devel Ther 12, 3517–3524. doi:10.2147/DDDT.S171286
Fan, Y., Xia, J., Jia, D., Zhang, M., Zhang, Y., Huang, G., and Wang, Y. (2016). Mechanism of ginsenoside Rg1 renal protection in a mouse model of d-galactose-induced subacute damage. Pharm Biol 54 (9), 1815–1821. doi:10.3109/13880209.2015.1129543
Feng, F., Nyland, J., Banyai, M., Tatum, A., Silverstone, A. E., and Gavalchin, J. (2010). The induction of the lupus phenotype by estrogen is via an estrogen receptor-alpha-dependent pathway. Clin Immunol 134 (2), 226–236. doi:10.1016/j.clim.2009.10.004
Fernandes, R., Freitas, S., Cunha, P., Alves, G., and Cotter, J. (2016). Goodpasture's syndrome with absence of circulating anti-glomerular basement membrane antibodies: a case report. J Med Case Rep 10, 205. doi:10.1186/s13256-016-0984-6
Fujimoto, J., Sakaguchi, H., Aoki, I., Toyoki, H., Khatun, S., and Tamaya, T. (2001). Inhibitory effect of ginsenoside-Rb2 on invasiveness of uterine endometrial cancer cells to the basement membrane. Eur J Gynaecol Oncol 22 (5), 339–341.
Gao, Y., Chu, S., Zhang, Z., and Chen, N. (2017). Hepataprotective effects of ginsenoside Rg1 - A review. J Ethnopharmacol 206, 178–183. doi:10.1016/j.jep.2017.04.012
Gentle, M. E., Shi, S., Daehn, I., Zhang, T., Qi, H., Yu, L., D'Agati, V. D., Schlondorff, D. O., and Bottinger, E. P. (2013). Epithelial cell TGFβ signaling induces acute tubular injury and interstitial inflammation. J Am Soc Nephrol 24 (5), 787–799. doi:10.1681/ASN.2012101024
Gewin, L., Zent, R., and Pozzi, A. (2017). Progression of chronic kidney disease: too much cellular talk causes damage. Kidney Int 91 (3), 552–560. doi:10.1016/j.kint.2016.08.025
Guo, X., Zhang, J., Liu, M., and Zhao, G. C. (2019). Protective effect of ginsenoside Rg1 on attenuating anti-GBM glomerular nephritis by activating NRF2 signalling. Artif Cells Nanomed Biotechnol 47 (1), 2972–2979. doi:10.1080/21691401.2019.1640712
Guo, M., Shao, S., Wang, D., Zhao, D., and Wang, M. (2021). Recent progress in polysaccharides from Panax ginseng C A. Meyer. Food Funct 12 (2), 494–518. doi:10.1039/d0fo01896a
Guo, J., Wang, R., and Min, F. (2022). Ginsenoside Rg1 ameliorates sepsis-induced acute kidney injury by inhibiting ferroptosis in renal tubular epithelial cells. J Leukoc Biol 112, 1065–1077. doi:10.1002/JLB.1A0422-211R
Han, M. J., and Kim, D. H. (2020). Effects of Red and Fermented Ginseng and Ginsenosides on Allergic Disorders. Biomolecules 10 (4), 634. doi:10.3390/biom10040634
Han, M. S., Han, I. H., Lee, D., An, J. M., Kim, S. N., Shin, M. S., Yamabe, N., Hwang, G. S., Yoo, H. H., Choi, S. J., Kang, K. S., and Jang, H. J. (2016). Beneficial effects of fermented black ginseng and its ginsenoside 20(S)-Rg3 against cisplatin-induced nephrotoxicity in LLC-PK1 cells. J Ginseng Res 40 (2), 135–140. doi:10.1016/j.jgr.2015.06.006
Hattori, T., Ito, M., and Suzuki, Y. (1991). Studies on antinephritic effects of plant components in rats (2): Effects of ginsenosides on original-type anti-GBM nephritis in rats and its mechanisms. Nihon Yakurigaku Zasshi 97 (2), 127–134. doi:10.1254/fpj.97.2_127
He, B., Chen, D., Zhang, X., Yang, R., Yang, Y., Chen, P., and Shen, Z. (2022a). Oxidative Stress and Ginsenosides: An Update on the Molecular Mechanisms. Oxid Med Cell Longev 2022, 9299574. doi:10.1155/2022/9299574
He, J. Y., Hong, Q., Chen, B. X., Cui, S. Y., Liu, R., Cai, G. Y., Guo, J., and Chen, X. M. (2022b). Ginsenoside Rb1 alleviates diabetic kidney podocyte injury by inhibiting aldose reductase activity. Acta Pharmacol Sin 43 (2), 342–353. doi:10.1038/s41401-021-00788-0
Hristova, M. H., and Stoyanova, V. S. (2017). Autoantibodies against complement components in systemic lupus erythematosus - role in the pathogenesis and clinical manifestations. Lupus 26 (14), 1550–1555. doi:10.1177/0961203317709347
Hsu, W. H., Hua, K. F., Tuan, L. H., Tsai, Y. L., Chu, L. J., Lee, Y. C., Wong, W. T., Lee, S. L., Lai, J. H., Chu, C. L., Ho, L. J., Chiu, H. W., Hsu, Y. J., Chen, C. H., Ka, S. M., and Chen, A. (2020). Compound K inhibits priming and mitochondria-associated activating signals of NLRP3 inflammasome in renal tubulointerstitial lesions. Nephrol Dial Transplant 35 (1), 74–85. doi:10.1093/ndt/gfz073
Humphreys, B. D. (2018). Mechanisms of Renal Fibrosis. Annu Rev Physiol 80, 309–326. doi:10.1146/annurev-physiol-022516-034227
Huynh, D. T. N., Baek, N., Sim, S., Myung, C. S., and Heo, K. S. (2020). Minor Ginsenoside Rg2 and Rh1 Attenuates LPS-Induced Acute Liver and Kidney Damages via Downregulating Activation of TLR4-STAT1 and Inflammatory Cytokine Production in Macrophages. Int J Mol Sci 21 (18), 6656. doi:10.3390/ijms21186656
Jeon, J. H., Lee, S., Lee, W., Jin, S., Kwon, M., Shin, C. H., Choi, M. K., and Song, I. S. (2020). Herb-Drug Interaction of Red Ginseng Extract and Ginsenoside Rc with Valsartan in Rats. Molecules 25 (3), 622. doi:10.3390/molecules25030622
Joh, E. H., Lee, I. A., Jung, I. H., and Kim, D. H. (2011). Ginsenoside Rb1 and its metabolite compound K inhibit IRAK-1 activation--the key step of inflammation. Biochem Pharmacol 82 (3), 278–286. doi:10.1016/j.bcp.2011.05.003
Kang, K. S., Kim, H. Y., Yamabe, N., Park, J. H., and Yokozawa, T. (2007). Preventive effect of 20(S)-ginsenoside Rg3 against lipopolysaccharide-induced hepatic and renal injury in rats. Free Radic Res 41 (10), 1181–1188. doi:10.1080/10715760701581740
Kang, K. S., Yamabe, N., Kim, H. Y., Park, J. H., and Yokozawa, T. (2010a). Effects of heat-processed ginseng and its active component ginsenoside 20(S)-Rg3 on the progression of renal damage and dysfunction in type 2 diabetic Otsuka Long-Evans Tokushima Fatty rats. Biol Pharm Bull 33 (6), 1077–1081. doi:10.1248/bpb.33.1077
Kang, X., Chen, J., Qin, Q., Wang, F., Wang, Y., Lan, T., Xu, S., Wang, F., Xia, J., Ekberg, H., Qi, Z., and Liu, Z. (2010b). Isatis tinctoria L. combined with co-stimulatory molecules blockade prolongs survival of cardiac allografts in alloantigen-primed mice. Transpl Immunol 23 (1-2), 34–39. doi:10.1016/j.trim.2010.03.006
Ke, B., Zhang, A., Wu, X., and Fang, X. (2015). The Role of Krüppel-like Factor 4 in Renal Fibrosis. Front Physiol 6, 327. doi:10.3389/fphys.2015.00327
Keum, Y. S., Han, S. S., Chun, K. S., Park, K. K., Park, J. H., Lee, S. K., and Surh, Y. J. (2003). Inhibitory effects of the ginsenoside Rg3 on phorbol ester-induced cyclooxygenase-2 expression, NF-kappaB activation and tumor promotion. Mutat Res 523-524, 75–85. doi:10.1016/s0027-5107(02)00323-8
Kim, Y. S., and Jin, S. H. (2004). Ginsenoside Rh2 induces apoptosis via activation of caspase-1 and -3 and up-regulation of Bax in human neuroblastoma. Arch Pharm Res 27 (8), 834–839. doi:10.1007/BF02980175
Kim, S. I., Park, J. H., Ryu, J.-H., Park, J. D., Lee, Y. H., Park, J.-H., Kim, T.-H., Kim, J. M., and Baek, N.-I. (1996). Ginsenoside Rg5, a genuine dammarane glycoside from Korean red ginseng. Archives of Pharmacal Research 19 (6), 551–553. doi:10.1007/bf02986026
Kim, B. J., Nah, S. Y., Jeon, J. H., So, I., and Kim, S. J. (2011). Transient receptor potential melastatin 7 channels are involved in ginsenoside Rg3-induced apoptosis in gastric cancer cells. Basic Clin Pharmacol Toxicol 109 (4), 233–239. doi:10.1111/j.1742-7843.2011.00706.x
Kim, T. W., Joh, E. H., Kim, B., and Kim, D. H. (2012). Ginsenoside Rg5 ameliorates lung inflammation in mice by inhibiting the binding of LPS to toll-like receptor-4 on macrophages. Int Immunopharmacol 12 (1), 110–116. doi:10.1016/j.intimp.2011.10.023
Kim, E. J., Jung, I. H., Van Le, T. K., Jeong, J. J., Kim, N. J., and Kim, D. H. (2013). Ginsenosides Rg5 and Rh3 protect scopolamine-induced memory deficits in mice. J Ethnopharmacol 146 (1), 294–299. doi:10.1016/j.jep.2012.12.047
Kim, B. J. (2013). Involvement of melastatin type transient receptor potential 7 channels in ginsenoside Rd-induced apoptosis in gastric and breast cancer cells. J Ginseng Res 37 (2), 201–209. doi:10.5142/jgr.2013.37.201
Lahmer, T., and Heemann, U. (2012). Anti-glomerular basement membrane antibody disease: a rare autoimmune disorder affecting the kidney and the lung. Autoimmun Rev 12 (2), 169–173. doi:10.1016/j.autrev.2012.04.002
Lee, H. L., and Kang, K. S. (2017). Protective effect of ginsenoside Rh3 against anticancer drug-induced apoptosis in LLC-PK1 kidney cells. J Ginseng Res 41 (2), 227–231. doi:10.1016/j.jgr.2017.01.011
Lee, B. H., Lee, S. J., Hur, J. H., Lee, S., Sung, J. H., Huh, J. D., Moon, C. K., et al. (1998). In vitro antigenotoxic activity of novel ginseng saponin metabolites formed by intestinal bacteria. Planta Med 64 (6), 500–503. doi:10.1055/s-2006-957501
Lee, Y. J., Jin, Y. R., Lim, W. C., Park, W. K., Cho, J. Y., Jang, S., and Lee, S. K. (2003a). Ginsenoside-Rb1 acts as a weak phytoestrogen in MCF-7 human breast cancer cells. Arch Pharm Res 26 (1), 58–63. doi:10.1007/BF03179933
Lee, Y., Jin, Y., Lim, W., Ji, S., Choi, S., Jang, S., and Lee, S. (2003b). A ginsenoside-Rh1, a component of ginseng saponin, activates estrogen receptor in human breast carcinoma MCF-7 cells. J Steroid Biochem Mol Biol 84 (4), 463–468. doi:10.1016/s0960-0760(03)00067-0
Lee, J. Y., Shin, J. W., Chun, K. S., Park, K. K., Chung, W. Y., Bang, Y. J., Sung, J. H., and Surh, Y. J. (2005). Antitumor promotional effects of a novel intestinal bacterial metabolite (IH-901) derived from the protopanaxadiol-type ginsenosides in mouse skin. Carcinogenesis 26 (2), 359–367. doi:10.1093/carcin/bgh313
Lee, S. M., Shon, H. J., Choi, C. S., Hung, T. M., Min, B. S., and Bae, K. (2009). Ginsenosides from heat processed ginseng. Chem Pharm Bull (Tokyo) 57 (1), 92–94. doi:10.1248/cpb.57.92
Li, S. S., Ye, J. M., Deng, Z. Y., Yu, L. X., Gu, X. X., and Liu, Q. F. (2015). Ginsenoside-Rg1 inhibits endoplasmic reticulum stress-induced apoptosis after unilateral ureteral obstruction in rats. Ren Fail 37 (5), 890–895. doi:10.3109/0886022X.2015.1015427
Li, W., Yan, M. H., Liu, Y., Liu, Z., Wang, Z., Chen, C., Zhang, J., and Sun, Y. S. (2016). Ginsenoside Rg5 Ameliorates Cisplatin-Induced Nephrotoxicity in Mice through Inhibition of Inflammation, Oxidative Stress, and Apoptosis. Nutrients 8 (9), 566. doi:10.3390/nu8090566
Li, S. S., He, A. L., Deng, Z. Y., and Liu, Q. F. (2018). Ginsenoside-Rg1 Protects against Renal Fibrosis by Regulating the Klotho/TGF-β1/Smad Signaling Pathway in Rats with Obstructive Nephropathy. Biol Pharm Bull 41 (4), 585–591. doi:10.1248/bpb.b17-00934
Li, Y., Hou, J. G., Liu, Z., Gong, X. J., Hu, J. N., Wang, Y. P., Liu, W. C., Lin, X. H., Wang, Z., and Li, W. (2021). Alleviative effects of 20(R)-Rg3 on HFD/STZ-induced diabetic nephropathy via MAPK/NF-κB signaling pathways in C57BL/6 mice. J Ethnopharmacol 267, 113500. doi:10.1016/j.jep.2020.113500
Liang, L. D., He, T., Du, T. W., Fan, Y. G., Chen, D. S., and Wang, Y. (2015). Ginsenoside-Rg5 induces apoptosis and DNA damage in human cervical cancer cells. Mol Med Rep 11 (2), 940–946. doi:10.3892/mmr.2014.2821
Lin, T. J., Wu, C. Y., Tsai, P. Y., Hsu, W. H., Hua, K. F., Chu, C. L., Lee, Y. C., Chen, A., Lee, S. L., Lin, Y. J., Hsieh, C. Y., Yang, S. R., Liu, F. C., and Ka, S. M. (2019). Accelerated and Severe Lupus Nephritis Benefits From M1, an Active Metabolite of Ginsenoside, by Regulating NLRP3 Inflammasome and T Cell Functions in Mice. Front Immunol 10, 1951. doi:10.3389/fimmu.2019.01951
Liu, C. X., and Xiao, P. G. (1992). Recent advances on ginseng research in China. J Ethnopharmacol 36 (1), 27–38. doi:10.1016/0378-8741(92)90057-x
Liu, Z. Q., Luo, X. Y., Sun, Y. X., Chen, Y. P., and Wang, Z. C. (2002). Can ginsenosides protect human erythrocytes against free-radical-induced hemolysis? Biochim Biophys Acta 1572 (1), 58–66. doi:10.1016/s0304-4165(02)00281-7
Liu, Z. Q., Luo, X. Y., Liu, G. Z., Chen, Y. P., Wang, Z. C., and Sun, Y. X. (2003). In vitro study of the relationship between the structure of ginsenoside and its antioxidative or prooxidative activity in free radical induced hemolysis of human erythrocytes. J Agric Food Chem 51 (9), 2555–2558. doi:10.1021/jf026228i
Liu, W. J., Tang, H. T., Jia, Y. T., Ma, B., Fu, J. F., Wang, Y., Lv, K. Y., and Xia, Z. F. (2010). Notoginsenoside R1 attenuates renal ischemia-reperfusion injury in rats. Shock 34 (3), 314–320. doi:10.1097/SHK.0b013e3181ceede4
Liu, Z., Li, C., Zhang, Q., and Tao, M. (2012). Effect of Renshen polysaccharides on oxidative injury in kidney IR rabbits. Carbohydr Polym 90 (2), 773–777. doi:10.1016/j.carbpol.2012.05.040
Liu, H., Lu, X., Hu, Y., and Fan, X. (2020). Chemical constituents of Panax ginseng and Panax notoginseng explain why they differ in therapeutic efficacy. Pharmacol Res 161, 105263. doi:10.1016/j.phrs.2020.105263
Liu, H., Chen, W., Lu, P., Ma, Y., Liang, X., and Liu, Y. (2021). Ginsenoside Rg1 attenuates the inflammation and oxidative stress induced by diabetic nephropathy through regulating the PI3K/AKT/FOXO3 pathway. Ann Transl Med 9 (24), 1789. doi:10.21037/atm-21-6234
Liu, T., Zhu, L., and Wang, L. (2022). A narrative review of the pharmacology of ginsenoside compound K. Ann Transl Med 10 (4), 234. doi:10.21037/atm-22-501
Liu, Y. (2006). Renal fibrosis: new insights into the pathogenesis and therapeutics. Kidney Int 69 (2), 213–217. doi:10.1038/sj.ki.5000054
Liu, Y. (2010). New insights into epithelial-mesenchymal transition in kidney fibrosis. J Am Soc Nephrol 21 (2), 212–222. doi:10.1681/ASN.2008121226
Lo, L. J., Go, A. S., Chertow, G. M., McCulloch, C. E., Fan, D., Ordoñez, J. D., and Hsu, C. Y. (2009). Dialysis-requiring acute renal failure increases the risk of progressive chronic kidney disease. Kidney Int 76 (8), 893–899. doi:10.1038/ki.2009.289
López-Farré, A., Gómez-Garre, D., Bernabeu, F., López-Novoa, J. M., and Lopez-FArre, A. (1991). A role for endothelin in the maintenance of post-ischaemic renal failure in the rat. J Physiol 444, 513–522. doi:10.1113/jphysiol.1991.sp018891
Ma, X., Xie, X., Zuo, C., and Fan, J. (2010). Effects of ginsenoside Rg1 on streptozocin-induced diabetic nephropathy in rats. Sheng Wu Yi Xue Gong Cheng Xue Za Zhi 27 (2), 342–347.
Mehta, R. L., Cerdá, J., Burdmann, E. A., Tonelli, M., García-García, G., Jha, V., Susantitaphong, P., Rocco, M., Vanholder, R., Sever, M. S., Cruz, D., Jaber, B., Lameire, N. H., Lombardi, R., Lewington, A., Feehally, J., Finkelstein, F., Levin, N., Pannu, N., Thomas, B., Aronoff-Spencer, E., and Remuzzi, G. (2015). International Society of Nephrology's 0by25 initiative for acute kidney injury (zero preventable deaths by 2025): a human rights case for nephrology. Lancet 385 (9987), 2616–2643. doi:10.1016/S0140-6736(15)60126-X
Meng, H., Liu, X. K., Li, J. R., Bao, T. Y., and Yi, F. (2022). Bibliometric analysis of the effects of ginseng on skin. J Cosmet Dermatol 21 (1), 99–107. doi:10.1111/jocd.14450
Miller, R. P., Tadagavadi, R. K., Ramesh, G., and Reeves, W. B. (2010). Mechanisms of Cisplatin nephrotoxicity. Toxins (Basel) 2 (11), 2490–2518. doi:10.3390/toxins2112490
Nangaku, M., Rosenberger, C., Heyman, S. N., and Eckardt, K. U. (2013). Regulation of hypoxia-inducible factor in kidney disease. Clin Exp Pharmacol Physiol 40 (2), 148–157. doi:10.1111/1440-1681.12005
Natesan, V., and Kim, S. J. (2021). Diabetic Nephropathy - a Review of Risk Factors, Progression, Mechanism, and Dietary Management. Biomol Ther (Seoul) 29 (4), 365–372. doi:10.4062/biomolther.2020.204
Ni, Y. H., Deng, H. F., Zhou, L., Huang, C. S., Wang, N. N., Yue, L. X., Li, G. F., Yu, H. J., Zhou, W., and Gao, Y. (2022). Ginsenoside Rb1 Ameliorated Bavachin-Induced Renal Fibrosis via Suppressing Bip/eIF2α/CHOP Signaling-Mediated EMT. Front Pharmacol 13, 872474. doi:10.3389/fphar.2022.872474
Ostendorf, T., Boor, P., van Roeyen, C. R., and Floege, J. (2014). Platelet-derived growth factors (PDGFs) in glomerular and tubulointerstitial fibrosis. Kidney Int Suppl 4 (1), 65–69. doi:10.1038/kisup.2014.12
Park, E. K., Shin, Y. W., Lee, H. U., Kim, S. S., Lee, Y. C., Lee, B. Y., and Kim, D. H. (2005). Inhibitory effect of ginsenoside Rb1 and compound K on NO and prostaglandin E2 biosyntheses of RAW264.7 cells induced by lipopolysaccharide. Biol Pharm Bull 28 (4), 652–656. doi:10.1248/bpb.28.652
Park, J. Y., Choi, P., Kim, T., Ko, H., Kim, H. K., Kang, K. S., and Ham, J. (2015). Protective Effects of Processed Ginseng and Its Active Ginsenosides on Cisplatin-Induced Nephrotoxicity: In Vitro and in Vivo Studies. J Agric Food Chem 63 (25), 5964–5969. doi:10.1021/acs.jafc.5b00782
Popovich, D. G., and Kitts, D. D. (2002). Structure-function relationship exists for ginsenosides in reducing cell proliferation and inducing apoptosis in the human leukemia (THP-1) cell line. Arch Biochem Biophys 406 (1), 1–8. doi:10.1016/s0003-9861(02)00398-3
Qi, Z., Li, W., Tan, J., Wang, C., Lin, H., Zhou, B., Liu, J., and Li, P. (2019). Effect of ginsenoside Rh(2) on renal apoptosis in cisplatin-induced nephrotoxicity in vivo. Phytomedicine 61, 152862. doi:10.1016/j.phymed.2019.152862
Qu, C., Bai, Y., Jin, X., Wang, Y., Zhang, K., You, J., and Zhang, H. (2009). Study on ginsenosides in different parts and ages of Panax quinquefolius L. Food Chemistry 115 (1), 340–346. doi:10.1016/j.foodchem.2008.11.079
Reutens, A. T., and Atkins, R. C. (2011). Epidemiology of diabetic nephropathy. Contrib Nephrol 170, 1–7. doi:10.1159/000324934
Romagnani, P., Remuzzi, G., Glassock, R., Levin, A., Jager, K. J., Tonelli, M., Massy, Z., Wanner, C., and Anders, H. J. (2017). Chronic kidney disease. Nat Rev Dis Primers 3, 17088. doi:10.1038/nrdp.2017.88
Sagoo, M. K., and Gnudi, L. (2018). Diabetic nephropathy: Is there a role for oxidative stress? Free Radic Biol Med 116, 50–63. doi:10.1016/j.freeradbiomed.2017.12.040
Sala, F., Mulet, J., Choi, S., Jung, S. Y., Nah, S. Y., Rhim, H., Valor, L. M., Criado, M., and Sala, S. (2002). Effects of ginsenoside Rg2 on human neuronal nicotinic acetylcholine receptors. J Pharmacol Exp Ther 301 (3), 1052–1059. doi:10.1124/jpet.301.3.1052
Shao, X., Li, N., Zhan, J., Sun, H., An, L., and Du, P. (2015). Protective effect of compound K on diabetic rats. Nat Prod Commun 10 (2), 1934578X1501000–245. doi:10.1177/1934578x1501000206
Shao, X., Chen, C., Miao, C., Yu, X., Li, X., Geng, J., Fan, D., Lin, X., Chen, Z., and Shi, Y. (2019). Expression analysis of microRNAs and their target genes during experimental diabetic renal lesions in rats administered with ginsenoside Rb1 and trigonelline. Pharmazie 74 (8), 492–498. doi:10.1691/ph.2019.8903
Shen, X., Dong, X., Han, Y., Li, Y., Ding, S., Zhang, H., Sun, Z., Yin, Y., Li, W., and Li, W. (2020). Ginsenoside Rg1 ameliorates glomerular fibrosis during kidney aging by inhibiting NOX4 and NLRP3 inflammasome activation in SAMP8 mice. Int Immunopharmacol 82, 106339. doi:10.1016/j.intimp.2020.106339
Shen, B., Wang, F., Zhou, Y., Li, T., He, C., and Zhao, W. (2021). Ginsenoside Rh2 inhibits renal fibrosis and renal cell apoptosis in rats with diabetic nephropathy by downregulating discoid domain receptor 1. Nan Fang Yi Ke Da Xue Xue Bao 41 (7), 1107–1113. doi:10.12122/j.issn.1673-4254.2021.07.21
Shin, K. C., and Oh, D. K. (2016). Classification of glycosidases that hydrolyze the specific positions and types of sugar moieties in ginsenosides. Crit Rev Biotechnol 36 (6), 1036–1049. doi:10.3109/07388551.2015.1083942
Shin, Y. W., Bae, E. A., and Kim, D. H. (2006). Inhibitory effect of ginsenoside Rg5 and its metabolite ginsenoside Rh3 in an oxazolone-induced mouse chronic dermatitis model. Arch Pharm Res 29 (8), 685–690. doi:10.1007/BF02968253
Shin, B. K., Kwon, S. W., and Park, J. H. (2015). Chemical diversity of ginseng saponins from Panax ginseng. J Ginseng Res 39 (4), 287–298. doi:10.1016/j.jgr.2014.12.005
Sin, S., Kim, S. Y., and Kim, S. S. (2012). Chronic treatment with ginsenoside Rg3 induces Akt-dependent senescence in human glioma cells. Int J Oncol 41 (5), 1669–1674. doi:10.3892/ijo.2012.1604
Song, W., Wei, L., Du, Y., Wang, Y., and Jiang, S. (2018). Protective effect of ginsenoside metabolite compound K against diabetic nephropathy by inhibiting NLRP3 inflammasome activation and NF-κB/p38 signaling pathway in high-fat diet/streptozotocin-induced diabetic mice. Int Immunopharmacol 63, 227–238. doi:10.1016/j.intimp.2018.07.027
Song, X., Wang, L., and Fan, D. (2022). Insights into Recent Studies on Biotransformation and Pharmacological Activities of Ginsenoside Rd. Biomolecules 12 (4), 512. doi:10.3390/biom12040512
Stangou, M., Alexopoulos, E., Papagianni, A., Pantzaki, A., Bantis, C., Dovas, S., Economidou, D., Leontsini, M., and Memmos, D. (2009). Urinary levels of epidermal growth factor, interleukin-6 and monocyte chemoattractant protein-1 may act as predictor markers of renal function outcome in immunoglobulin A nephropathy. Nephrology (Carlton) 14 (6), 613–620. doi:10.1111/j.1440-1797.2008.01051.x
Stehouwer, C. D. (2004). Endothelial dysfunction in diabetic nephropathy: state of the art and potential significance for non-diabetic renal disease. Nephrol Dial Transplant 19 (4), 778–781. doi:10.1093/ndt/gfh015
Su, W. Y., Li, Y., Chen, X., Li, X., Wei, H., Liu, Z., Shen, Q., Chen, C., Wang, Y. P., and Li, W. (2021). Ginsenoside Rh1 Improves Type 2 Diabetic Nephropathy through AMPK/PI3K/Akt-Mediated Inflammation and Apoptosis Signaling Pathway. Am J Chin Med 49 (5), 1215–1233. doi:10.1142/S0192415X21500580
Sun, Q., Meng, Q. T., Jiang, Y., and Xia, Z. Y. (2012). Ginsenoside Rb1 attenuates intestinal ischemia reperfusion induced renal injury by activating Nrf2/ARE pathway. Molecules 17 (6), 7195–7205. doi:10.3390/molecules17067195
Sun, Q., Meng, Q. T., Jiang, Y., Liu, H. M., Lei, S. Q., Su, W. T., Duan, W. N., Wu, Y., Xia, Z. Y., and Xia, Z. Y. (2013). Protective effect of ginsenoside Rb1 against intestinal ischemia-reperfusion induced acute renal injury in mice. PLoS One 8 (12), e80859. doi:10.1371/journal.pone.0080859
Surh, Y. J., Lee, J. Y., Choi, K. J., and Ko, S. R. (2002). Effects of selected ginsenosides on phorbol ester-induced expression of cyclooxygenase-2 and activation of NF-kappaB and ERK1/2 in mouse skin. Ann N Y Acad Sci 973, 396–401. doi:10.1111/j.1749-6632.2002.tb04672.x
Tam, D. N. H., Truong, D. H., Nguyen, T. T. H., Quynh, L. N., Tran, L., Nguyen, H. D., Shamandy, B. E., Le, T. M. H., Tran, D. K., Sayed, D., Vu, V. V., Mizukami, S., Hirayama, K., and Huy, N. T. (2018). Ginsenoside Rh1: A Systematic Review of Its Pharmacological Properties. Planta Med 84 (3), 139–152. doi:10.1055/s-0043-124087
Tang, M., Wang, W., Cheng, L., Jin, R., Zhang, L., Bian, W., and Zhang, Y. (2018). The inhibitory effects of 20(R)-ginsenoside Rg3 on the proliferation, angiogenesis, and collagen synthesis of hypertrophic scar derived fibroblasts in vitro. Iran J Basic Med Sci 21 (3), 309–317. doi:10.22038/ijbms.2018.19451.5153
Tsutsumi, Y. M., Tsutsumi, R., Mawatari, K., Nakaya, Y., Kinoshita, M., Tanaka, K., and Oshita, S. (2011). Compound K, a metabolite of ginsenosides, induces cardiac protection mediated nitric oxide via Akt/PI3K pathway. Life Sci 88 (15-16), 725–729. doi:10.1016/j.lfs.2011.02.011
Venkatachalam, M. A., Griffin, K. A., Lan, R., Geng, H., Saikumar, P., and Bidani, A. K. (2010). Acute kidney injury: a springboard for progression in chronic kidney disease. Am J Physiol Renal Physiol 298 (5), F1078–1094. doi:10.1152/ajprenal.00017.2010
Vinh, L. B., Lee, Y., Han, Y. K., Kang, J. S., Park, J. U., Kim, Y. R., Yang, S. Y., and Kim, Y. H. (2017). Two new dammarane-type triterpene saponins from Korean red ginseng and their anti-inflammatory effects. Bioorg Med Chem Lett 27 (23), 5149–5153. doi:10.1016/j.bmcl.2017.10.058
Wada, J., and Makino, H. (2013). Inflammation and the pathogenesis of diabetic nephropathy. Clin Sci (Lond) 124 (3), 139–152. doi:10.1042/CS20120198
Weiyun, Z., liu, F.-g., and male, H. t. (2019). Changes of contents of three rare ginsenosides in the process of black production %J. Shi Zhen National medicine and national medicine 30 (02), 325–327.
Wu, C. Y., Hua, K. F., Hsu, W. H., Suzuki, Y., Chu, L. J., Lee, Y. C., Takahata, A., Lee, S. L., Wu, C. C., Nikolic-Paterson, D. J., Ka, S. M., and Chen, A. (2020). IgA Nephropathy Benefits from Compound K Treatment by Inhibiting NF-κB/NLRP3 Inflammasome and Enhancing Autophagy and SIRT1. J Immunol 205 (1), 202–212. doi:10.4049/jimmunol.1900284
Wu, J., Jiang, C., Hua, Y., Liu, X., and You, C. (2021a). Association between polymorphisms of cytokine genes and diabetic nephropathy: A comprehensive systematic review and meta-analysis. Int J Clin Pract 75 (11), e14634. doi:10.1111/ijcp.14634
Wu, W. J., Tang, Y. F., Dong, S., and Zhang, J. (2021b). Ginsenoside Rb3 Alleviates the Toxic Effect of Cisplatin on the Kidney during Its Treatment to Oral Cancer via TGF-β-Mediated Mitochondrial Apoptosis. Evid Based Complement Alternat Med 2021, 6640714. doi:10.1155/2021/6640714
Xie, X. S., Liu, H. C., Zuo, C., Deng, Y., and Fan, J. M. (2008a). The effect of ginsenoside Rg1 on the renal interstitial fibrosis of UUO rat. Sichuan Da Xue Xue Bao Yi Xue Ban 39 (2), 218–222.
Xie, X. S., Yang, M., Liu, H. C., Zuo, C., Li, Z., Deng, Y., and Fan, J. M. (2008b). Influence of ginsenoside Rg1, a panaxatriol saponin from Panax notoginseng, on renal fibrosis in rats with unilateral ureteral obstruction. J Zhejiang Univ Sci B 9 (11), 885–894. doi:10.1631/jzus.B0820024
Xie, X. S., Liu, H. C., Li, H. J., and Fan, J. M. (2008c). Ginsenoside R(g1) inhibit transdifferentiation in rat renal tubular epethelial cells induced by TGF-beta1. Zhongguo Zhong Yao Za Zhi 33 (17), 2136–2141.
Xie, X. S., Liu, H. C., Yang, M., Zuo, C., Deng, Y., and Fan, J. M. (2009). Ginsenoside Rb1, a panoxadiol saponin against oxidative damage and renal interstitial fibrosis in rats with unilateral ureteral obstruction. Chin J Integr Med 15 (2), 133–140. doi:10.1007/s11655-009-0133-9
Xing, J. J., Hou, J. G., Ma, Z. N., Wang, Z., Ren, S., Wang, Y. P., Liu, W. C., Chen, C., and Li, W. (2019). Ginsenoside Rb3 provides protective effects against cisplatin-induced nephrotoxicity via regulation of AMPK-/mTOR-mediated autophagy and inhibition of apoptosis in vitro and in vivo. Cell Prolif 52 (4), e12627. doi:10.1111/cpr.12627
Xu, X., Lu, Q., Wu, J., Li, Y., and Sun, J. (2017). Impact of extended ginsenoside Rb1 on early chronic kidney disease: a randomized, placebo-controlled study. Inflammopharmacology 25 (1), 33–40. doi:10.1007/s10787-016-0296-x
Xu, J. L., Gan, X. X., Ni, J., Shao, D. C., Shen, Y., Miao, N. J., Xu, D., Zhou, L., Zhang, W., and Lu, L. M. (2018). SND p102 promotes extracellular matrix accumulation and cell proliferation in rat glomerular mesangial cells via the AT1R/ERK/Smad3 pathway. Acta Pharmacol Sin 39 (9), 1513–1521. doi:10.1038/aps.2017.184
Xu, L., Xiao, S., Lee, J. J., Li, X., and Zhao, Y. (2022). Gender-Related Differences in Tissue Distribution, Excretion, and Metabolism Studies of Panaxadiol in Rats and Anti-inflammatory Study. J Agric Food Chem 70 (28), 8672–8679. doi:10.1021/acs.jafc.2c02618
Yang, L., Zhang, X. Y., Li, K., Li, A. P., Yang, W. D., Yang, R., Wang, P., Zhao, Z. H., Cui, F., Qin, Y., Yang, J. H., Tao, H. L., Sun, T., Chen, S., Yu, P. H., Liu, H. J., and Yang, C. (2019). Protopanaxadiol inhibits epithelial-mesenchymal transition of hepatocellular carcinoma by targeting STAT3 pathway. Cell Death Dis 10 (9), 630. doi:10.1038/s41419-019-1733-8
Yao, H., Jin, Y., Yang, J., Li, L., Sun, T., Shi, X., and Li, X.-w. (2014). Conversion Rule of Rare Ginsenosides Produced from Major Ginsenosides by Confined Microiwave Promoted Degradation Method. Chemical Journal of Chinese Universities-chinese 35, 2317.
Yenisetti, S. C., Manjunath, M. J., and Muralidhara, C. (2016). Neuropharmacological Properties of Withania somnifera - Indian Ginseng: An Overview on Experimental Evidence with Emphasis on Clinical Trials and Patents. Recent Pat CNS Drug Discov 10 (2), 204–215. doi:10.2174/1574889810666160615014106
Yi, Y. S. (2019). Ameliorative effects of ginseng and ginsenosides on rheumatic diseases. J Ginseng Res 43 (3), 335–341. doi:10.1016/j.jgr.2018.04.004
Yokozawa, T., and Dong, E. (2001). Role of ginsenoside-Rd in cisplatin-induced renal injury: special reference to DNA fragmentation. Nephron 89 (4), 433–438. doi:10.1159/000046116
Yokozawa, T., and Liu, Z. W. (2000). The role of ginsenoside-Rd in cisplatin-induced acute renal failure. Ren Fail 22 (2), 115–127. doi:10.1081/jdi-100100858
Yung, S., Yap, D. Y., and Chan, T. M. (2017). Recent advances in the understanding of renal inflammation and fibrosis in lupus nephritis. F1000Res. 6, 874. doi:10.12688/f1000research.10445.1
Zarneshan, S. N., Fakhri, S., and Khan, H. (2022). Targeting Akt/CREB/BDNF signaling pathway by ginsenosides in neurodegenerative diseases: A mechanistic approach. Pharmacol Res 177, 106099. doi:10.1016/j.phrs.2022.106099
Zhai, J., Gao, H., Wang, S., Zhang, S., Qu, X., Zhang, Y., Tao, L., Sun, J., Song, Y., and Fu, L. (2021). Ginsenoside Rg3 attenuates cisplatin-induced kidney injury through inhibition of apoptosis and autophagy-inhibited NLRP3. J Biochem Mol Toxicol 35 (11), e22896. doi:10.1002/jbt.22896
Zhan, M., Brooks, C., Liu, F., Sun, L., and Dong, Z. (2013). Mitochondrial dynamics: regulatory mechanisms and emerging role in renal pathophysiology. Kidney Int 83 (4), 568–581. doi:10.1038/ki.2012.441
Zhang, L. N., Xie, X. S., Zuo, C., and Fan, J. M. (2009). Effect of ginsenoside Rgl on the expression of TNF-alpha and MCP-1 in rats with diabetic nephropathy. Sichuan Da Xue Xue Bao Yi Xue Ban 40 (3), 466–471.
Zhang, J. J., Zhou, Y. D., Liu, Y. B., Wang, J. Q., Li, K. K., Gong, X. J., Lin, X. H., Wang, Y. P., Wang, Z., and Li, W. (2021). Protective Effect of 20(R)-Ginsenoside Rg3 Against Cisplatin-Induced Renal Toxicity via PI3K/AKT and NF-[Formula: see text]B Signaling Pathways Based on the Premise of Ensuring Anticancer Effect. Am J Chin Med 49 (7), 1739–1756. doi:10.1142/S0192415X21500828
Zhang, D., Ji, P., Sun, R., Zhou, H., Huang, L., Kong, L., Li, W., and Li, W. (2022). Ginsenoside Rg1 attenuates LPS-induced chronic renal injury by inhibiting NOX4-NLRP3 signaling in mice. Biomed Pharmacother 150, 112936. doi:10.1016/j.biopha.2022.112936
Zhao, A., Liu, N., Yao, M., Zhang, Y., Yao, Z., Feng, Y., Liu, J., and Zhou, G. (2022a). A Review of Neuroprotective Effects and Mechanisms of Ginsenosides From Panax Ginseng in Treating Ischemic Stroke. Front Pharmacol 13, 946752. doi:10.3389/fphar.2022.946752
Zhao, L., Zhang, Y., Li, Y., Li, C., Shi, K., Zhang, K., and Liu, N. (2022b). Therapeutic effects of ginseng and ginsenosides on colorectal cancer. Food Funct 13 (12), 6450–6466. doi:10.1039/d2fo00899h
Zhou, P., Zhan, X., g, , Guo, M., Guo, R., Wang, L., Zhang, Z., Lin, Z., Dong, M., Dai, H., Ji, X., and Lu, H. (2019). Ginsenoside Rb1 ameliorates CKD-associated vascular calcification by inhibiting the Wnt/β-catenin pathway. J Cell Mol Med 23 (10), 8724747088–7098. doi:10.1111/jcmm.14611
Zhou, T., Sun, L., Yang, S., Lv, Y., Cao, Y., Gang, X., and Wang, G. (2020). 20(S)-Ginsenoside Rg3 Protects Kidney from Diabetic Kidney Disease via Renal Inflammation Depression in Diabetic Rats. J Diabetes Res 2020, 7152176. doi:10.1155/2020/7152176
Zhou, S., He, Y., Zhang, W., Xiong, Y., Jiang, L., Wang, J., Cui, X., Qu, Y., and Ge, F. (2021a). Ophiocordyceps lanpingensis polysaccharides alleviate chronic kidney disease through MAPK/NF-κB pathway. J Ethnopharmacol 276, 114189. doi:10.1016/j.jep.2021.114189
Zhou, S., Zhou, Y., Yu, J., Jiang, L., Xiang, Y., Wang, J., Du, Y., Cui, X., and Ge, F. (2021b). A neutral polysaccharide from Ophiocordyceps lanpingensis restrains cisplatin-induced nephrotoxicity. Food Sci Nutr 9 (7), 3602–3616. doi:10.1002/fsn3.2317
Zhu, Y., Zhu, C., Yang, H., Deng, J., and Fan, D. (2020). Protective effect of ginsenoside Rg5 against kidney injury via inhibition of NLRP3 inflammasome activation and the MAPK signaling pathway in high-fat diet/streptozotocin-induced diabetic mice. Pharmacol Res 155, 104746. doi:10.1016/j.phrs.2020.104746
Keywords: ginsenoside, kidney, nephropathy, animal model, mechanism
Citation: Fan M, Lan X, Wang Q, Shan M, Fang X, Zhang Y, Wu D, Luo H, Gao W and Zhu D (2023) Renal function protection and the mechanism of ginsenosides: Current progress and future perspectives. Front. Pharmacol. 14:1070738. doi: 10.3389/fphar.2023.1070738
Received: 15 October 2022; Accepted: 24 January 2023;
Published: 06 February 2023.
Edited by:
Wei Li, Jilin Agricultural University, ChinaCopyright © 2023 Fan, Lan, Wang, Shan, Fang, Zhang, Wu, Luo, Gao and Zhu. This is an open-access article distributed under the terms of the Creative Commons Attribution License (CC BY). The use, distribution or reproduction in other forums is permitted, provided the original author(s) and the copyright owner(s) are credited and that the original publication in this journal is cited, in accordance with accepted academic practice. No use, distribution or reproduction is permitted which does not comply with these terms.
*Correspondence: Wenyi Gao, Z2Fvd3lAY2N1Y20uZWR1LmNu; Difu Zhu, emh1ZGZAY2N1Y20uZWR1LmNu
†These authors have contributed equally to this work