- 1Department of Pharmacology, College of Medicine, University of Arizona, Tucson, AZ, United States
- 2Comprehensive Pain and Addiction Center, University of Arizona, Tucson, AZ, United States
Alzheimer’s disease (AD) is a complex neurological disorder characterized by accumulation of amyloid plaques and neurofibrillary tangles. Long term investigation of AD pathogenesis suggests that β-site amyloid precursor protein [APP] cleaving enzyme 1 (BACE1) and γ-secretase enzymes promote the amyloidogenic pathway and produce toxic Aβ peptides that are predisposed to aggregate in the brain. Hence, the targeted inhibition of BACE1/γ-secretase expression and function is a promising approach for AD therapy. Several reports have suggested that the opioid family of G-protein coupled receptors modulate the etiology of AD progression. It has also been found that changes in the signaling pathways of opioid receptors increased the expression of BACE1 and γ-secretase, and is strongly correlated with abnormal production of Aβ and pathogenesis of AD. Thus, the opioid receptor family is a promising candidate for targeted drug development to treat AD. In this review, we outline the involvement and mechanisms of opioid receptor signaling modulation in Alzheimer’s Disease progression.
Introduction
Alzheimer’s Disease (AD) is a neurodegenerative disorder that is responsible for nearly 60 to 80 percent of cases of dementia worldwide. Although the cause is still undetermined, it likely includes a combination of genetic, environmental, and lifestyle factors. Dementia has been listed as the fifth largest cause of death across the globe by the World Health Organization. A current total of 50 million people with dementia globally was estimated by AD International and is projected to increase to 152 million by 2050. The number of AD patients in the USA, which is presently 5 million, is estimated to increase to 16 million by 2050, further increasing the personal and socioeconomic burden of this disease. Every 3 seconds a patient develops dementia and the current estimate of treatment costs of dementia is currently $1 trillion USD, which is projected to double by the year 2030. In the majority of sporadic cases of AD, age plays a major role, and with an aging population worldwide, cases of AD are set to rise dramatically over the coming decades (Alzheimer’s Association, 2013; Reynolds, 2019).
AD is a multifactorial progressive neurodegenerative disease characterized by memory and neuronal loss, difficulties in speaking, problem solving, and other cognitive skills, along with changes in mood and behavior, which interfere with the person’s daily performance (Ballard et al., 2011). The neuropathological hallmarks of AD include extracellular accumulation of amyloid β (Aβ) protein and intracellular accumulation of neurofibrillary tangles induced by hyper-phosphorylated Tau protein. Aβ proteins are 37–43 amino acid containing peptides that are produced from amyloid precursor protein (APP) through sequential cleavage by β-site amyloid precursor protein [APP]-cleaving enzyme 1 (BACE1) and γ-secretase. Of the two cleavage products, Aβ40 and Aβ42, AD pathogenesis correlates with the production and accumulation of Aβ42, which aggregates to form β-amyloid plaques (Figure 1) (Reynolds, 2019). Aβ42 peptides are thought to be prone to aggregation and toxicity due to their longer length (Canter et al., 2016). No disease-modifying therapies have been approved for the treatment of AD and available treatments are for symptom management with limited disease-modifying efficacy. There are many putative susceptibility risk genes for AD that have been reported, such as Apolipoprotein E (ApoE) (Corder et al., 1993), Glycogen Synthase Kinase 3-β (GSK3β) (Kwok et al., 2008; Hernández et al., 2009), Dual-Specificity Tyrosine-Regulated Kinase 1A (DYRK1A) (Kimura et al., 2007), Tau (Myers et al., 2005; Caffrey and Wade-Martins, 2007), Translocase of Outer Mitochondrial Membrane 40 Homolog (TOMM40) (Chiba-Falek et al., 2018), and Phosphatidylinositol Binding Clathrin Assembly Protein (PICALM) (Mercorio et al., 2018). Apart from these listed genetics risk factors, the APOE*ε4 allele has been shown to be responsible for Aβ accumulation and is considered the strongest confirmed genetic risk factor for early and late onset AD, while the ε2 allele is considered protective. (Corder et al., 1993; Strittmatter et al., 1993; Morris et al., 2011).
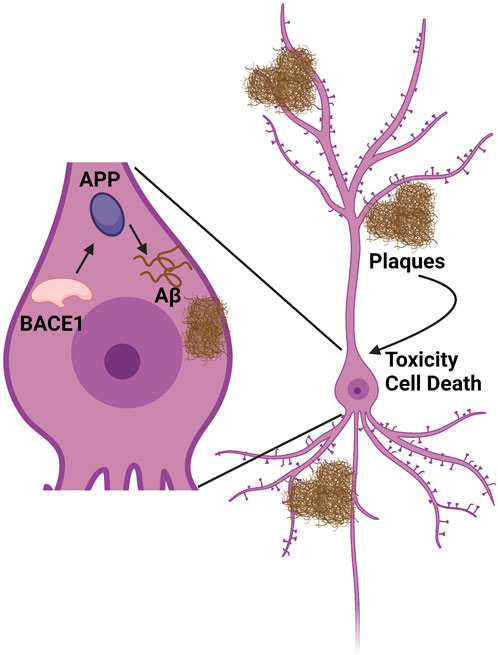
FIGURE 1. Amyloid synthesis cascade pathway. BACE1 cleaves APP to produce Aβ peptide. This peptide accumulates both intracellularly and extracellularly into tangles/plaques, which in turn cause inflammation, toxicity, and cell death. Figure created using www.biorender.com.
ApoE a lipid binding protein, is synthesized by astrocytes in the central nervous system (CNS) and synthesized cholesterol is transported to neurons through ApoE receptors. ApoE is polymorphic with three major alleles, namely, APOE*ε2, APOE*ε3, and APOE*ε4. People with two APOE*ε4 alleles are at a 15 times higher risk of AD as compared to APOE*ε3 carriers, and APOE*ε2 allele carriers have protection against AD. Notably, the APOE*ε3 is considered to be the most common allele and is believed to play a neutral role in the disease - neither decreasing nor increasing risk.
ApoE proteins influence with varying ability Aβ clearance from the brain, with ApoE2 being the most effective and ApoE4 the least effective (Liu et al., 2013). The APOE*ε4 allele is consistently linked to abnormal Aβ aggregation and predicts longitudinal Aβ accumulation in plaque-free elderly individuals without dementia. Conversely, APOE*ε2 carriers are protected against longitudinal Aβ accumulation (Lim et al., 2017). Unfortunately, it is evident that women are more prone to develop AD and other dementias than men. The female population with dementia is higher than males, and of the total 5.6 million AD patients, nearly 3.5 million are women and 2.1 million are men. In support of this, several studies reported that the APOE*ε4 genotype is more strongly associated with dementia in women as compared to men (Farrer et al., 1997). Together, these studies highlight the central role of the secretases and ApoE systems in regulating AD pathogenesis, and suggest that new approaches to therapeutically target these systems could be effective in modifying disease pathogenesis of AD.
Introduction to the opioid receptor family
In the early 1990s, three important opioid receptor family members were identified and cloned after decades of investigation, namely, the μ (mu-opioid receptor-MOR), κ (kappa-opioid receptor-KOR), and δ (delta-opioid receptor-DOR) opioid receptors. In 1994, another opioid receptor was discovered, the nociceptin/orphanin FQ receptor (NOP), also called the opioid receptor-like orphan receptor (ORL) (Xia, 2015; Vaidya et al., 2018). The opioid receptor family modulates important physiological processes, including analgesia, stress response, immune response, and neuroendocrine function. The roles, locations, and functions of the opioid receptor family members are summarized in Table 1. The expression of opioid receptors and peptides are found in regions vulnerable to AD pathology in the CNS, including regions of the brain like the hippocampus and cortex, which are important for cognition and are affected heavily by AD. The opioid receptors play important roles in synaptic activation, learning and memory. Administration of opioid antagonists has been found to significantly improve the memory performance of rats (Gallagher, 1985). These antagonists and similar drugs discussed here are summarized in Table 2. However, later studies have failed to support the efficacy of the non-selective antagonists naloxone or naltrexone in improving AD symptoms (Sunderland et al., 1986). Despite this, there is a strong overlap in the distribution of opioid receptors and amyloid plaque location in AD patients, leading the field to postulate a role of these three opioid receptors in the pathology of AD. Opioid receptor knockout mouse studies further demonstrated a potential regulatory role in AD due to the distinct and opposing roles of opioid receptors in modulating different animal behaviors important in AD, such as locomotion, anxiety, depressive behavior, or alcohol intake (Kieffer and Gavériaux-Ruff, 2002).
Opioid receptors are members of a superfamily of 7 transmembrane spanning (7TM) G protein-coupled receptors (GPCRs). So far, almost 370 non-olfactory GPCRs have been identified, out of which nearly 90% are present in the brain, playing crucial roles in mood, cognition, pain, appetite, and synaptic transmission (Vassilatis et al., 2003). Opioid receptors are also found in the nervous system, lungs, heart, liver, and the gastrointestinal and reproductive tracts (Jutkiewicz, 2018). GPCRs are involved in multiple neurotransmitter systems that are associated with AD; glutamatergic, serotonergic, adrenergic and peptidergic pathways in particular are dysregulated in this neurodegenerative disorder and are impacted by opioid receptor activity (Thathiah and De Strooper, 2011). Targeting these systems might protect against disease progression by modulating the formation of Aβ plaques or aberrant signaling following plaque formation (Thathiah and De Strooper, 2011). Activation of opioid receptors occurs by endogenous peptides as well as opioid drugs such as morphine, which are administered exogenously. These are not only effective analgesics, but also cause side effects such as addiction and are categorized as drugs of abuse, providing a note of caution in exploiting this receptor family (Satoh et al., 2000a).
There are many notable similarities in the primary structures of MOR, DOR and KOR along with their function and mechanisms of intracellular signaling (SKARPHEDINSSON and THOREN, 1988). Adding further complexity, both homomeric and heteromeric complex formation between opioid receptors and non-opioid receptors can lead to modification of the response to a particular opioid ligand (Satoh et al., 2000; Wei and Loh, 2002; Pasternak, 2004; Ananthan, 2008). A pharmacological response generated by action of opioid ligands might be due to interaction with different opioid receptor complexes. On the other hand, synthetic opioid peptides and alkaloids are very selective for MOR, DOR, and KOR, and have been explored largely for defining pharmacological properties of isolated opioid receptors. This means that potential unexplored complexities of opioid receptor function could impact their activity in AD.
GPCRs serve as versatile targets from the perspective of drug discovery. Various drugs which target GPCRs can act either as agonists or as antagonists for the signaling of G protein. A conformational change is promoted after binding of agonist to a GPCR resulting in the activation of receptor-associated heterotrimeric G proteins and further downstream signaling pathways. However, a small family of multifunctional GPCR regulatory or adaptor proteins known as the β-arrestins, which have an almost universal role in facilitating traditional GPCR desensitization, are also capable of initiating distinct, independent signaling events (DeWire et al., 2007). In AD pathogenesis, GPCRs are found to be associated with various stages of APP proteolysis, which includes modulation of APP processing by the α-, β- and γ-secretases and regulating degradation of Aβ and Aβ-mediated toxicity (Thathiah and De Strooper, 2011; Wisely et al., 2014). Studies have shown that GPCRs can bind to β-secretase and γ-secretase, which are key enzymes in the hydrolytic processing of APP (Liu X. et al., 2013; Nelson and Sheng, 2013; Thathiah et al., 2013). Unfortunately, the signaling mechanisms, which mediate these effects have not been completely explored and the regulation of the γ-secretase complex by GPCRs as hypothesized remains unexplained.
The opioid receptors are involved in learning and memory and are dysregulated in specific regions of the AD brain (Mathieu-Kia et al., 2001). Recent studies suggest that these GPCRs and one of their ligands, enkephalin, are involved in modulation of β-secretase and subsequent Aβ generation (Thathiah and De Strooper, 2011).
The role of opioid receptors in modulating Alzheimer’s disease
Opioid receptors have a modulatory effect on the regulation of neurotransmitters such as acetylcholine, norepinephrine, GABA, glutamate, and serotonin, which have been implicated in the pathogenesis of AD. A close relationship exists between Aβ generation and the opioid system, as dysfunction of the opioid receptors causes retardation of endocytosis and degradation of the BACE1 enzyme; this enzyme is required for generating monomeric forms of Aβ, including Aβ42, which further aggregates into bioactive conformational species and is responsible for initiation of toxicity in AD (Hampel et al., 2020). This directly suggests that dysfunctional opioid receptors can contribute to AD pathology. It has also been reported that alteration in the signaling pathway of opioid receptors is strongly correlated with abnormal production of Aβ and pathogenesis of AD (Sunderland et al., 1986).
No approved disease-modifying therapies are available for AD and the few agents available for symptomatic treatment are less effective. Various efforts have taken place for prevention or elimination of beta-amyloid plaques, including targeting the BACE1 and γ-secretase enzymes, but unfortunately none of these strategies have been clinically successful. This suggests that new approaches to inhibiting the production of Aβ in the first place may be needed (Scheltens et al., 2016).
Studies have reported that indirectly modulating the function of enzymes via modulating GPCRs could serve as a novel strategy for reducing the production of Aβ peptide with less side effects. Among GPCRs that influence amyloidogenesis, the DOR has been shown to play an important role in the trafficking and function of BACE1, γ-secretase, and the production of Aβ peptide. Studies have reported that DOR levels in certain specific regions of AD brains were elevated compared with non-AD brains. These notable areas included the frontal cortex, caudate, and hippocampus (Hiller et al., 1987; Mathieu-Kia et al., 2001). It has been suggested that the DOR along with the β2 adrenergic receptor (β2AR) leads to promotion of cleavage of the APP C-terminal fragment mediated by γ-secretase, once it is generated by β-secretase (Ni et al., 2006a). The DOR has shown a strong modulatory role in AD by showing its effect on secretase activity after stimulation with its agonist for 30 min, which enhanced BACE1 and γ-secretase activities to 143% and 156% respectively. This was reported using a fluorogenic substrate assay using a HEK293T cell line overexpressed with DOR, while the activity of α-secretase was unaltered (Teng et al., 2010). Research on postmortem brains of AD patients has shown that MOR, DOR, and KOR are differentially altered in distinct brain areas (Mathieu-Kia et al., 2001c). DOR binding is decreased in the amygdala and ventral putamen, and MOR binding is decreased in the hippocampus and subiculum (Mathieu-Kia et al., 2001) of postmortem brain samples from patients with AD. The levels of leu-enkephalin and dynorphin A (the endogenous opioid peptides for DOR and KOR, respectively) were increased in the frontal cortex of patients with AD in this same study. Elevation of the opioid precursors pre-proenkephalin and met-enkephalin was found to contribute to the cognitive and behavioral decline in a transgenic mouse model of AD, suggesting the involvement of DOR in AD pathology (Meilandt et al., 2008a). Early studies on possible treatment of AD using the non-selective opioid antagonists naloxone and naltrexone did not find them to be efficacious. However, more recent studies focusing on specific subtypes of opioid receptor have revealed that the DOR in particular plays a significant role in AD pathology (Teng et al., 2010; Liu et al., 2013).
Studies have reported that agonist-induced activation of DOR has been shown to increase BACE1 and γ-secretase activity in vitro in cells and in vivo in an AD mouse model leading to increased production of Aβ peptide (Figure 2) (Ni et al., 2006; Teng et al., 2010). Mechanistic studies revealed that DOR forms a complex with β- and γ-secretases and that the DOR mediates co-endocytic sorting of this complex into late endosomal/lysosomal (LEL) compartments, in which the generation of Aβ takes place. DOR antagonism using naltrindole substantially reversed AD pathology caused by APP/Presenilin overexpression in mice, including memory deficits, reactive glia formation, Aβ production, and BACE1/γ-secretase activity in the brain (Teng et al., 2010). Similarly, it has been observed in vivo in an AD mouse model that DOR knockdown resulted in reduced accumulation of Aβ40 in the hippocampus. However, there was no effect on the more hydrophobic (and more toxic) Aβ42 (Teng et al., 2010). No effect was observed with administration of MOR antagonist on the generation of Aβ or amyloid plaque formation, and MOR antagonist was not able to reverse the learning and memory deficiency of the AD mouse model (Teng et al., 2010), although another group reported improved spatial memory retention in this transgenic AD mouse model with MOR antagonist treatment (Meilandt et al., 2008). Importantly, neither activation of DOR in vitro nor blockage of DOR in vivo affected the processing of Notch, N-cadherin, or APLP-1 by either BACE1 or γ-secretase, thus providing direct evidence that antagonism of DOR specifically blocks the amyloidogenic pathway and efficaciously prevents AD progression in mice (Teng et al., 2010). However, DOR antagonists have not yet been explored as therapeutic agents for AD in humans.
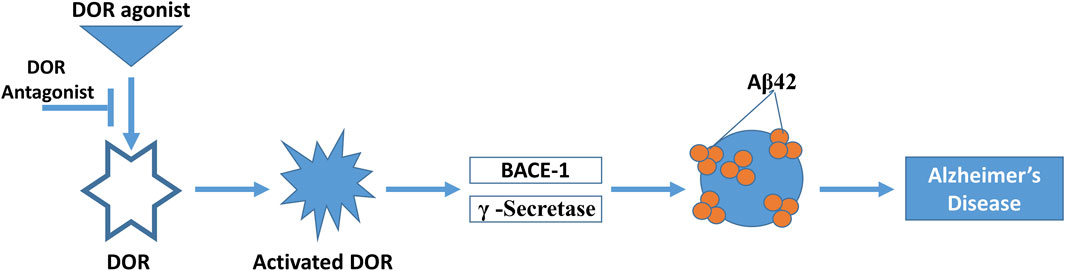
FIGURE 2. Interaction of the DOR with Alzheimer’s pathology. DOR is activated by DOR agonist, which in turn activates BACE1 and γ-secretase activity. This produces Aβ42 protein aggregates, producing at least in part Alzheimer’s disease pathology. This process can be reversed by DOR antagonist treatment.
In contrast to the above work, a recent study reported very different roles of DOR and MOR in the regulation and activity of BACE1 expression, highlighting the possible neuroprotective role of DOR against AD injury. Activation of DOR with its specific agonist UFP-512 substantially decreased BACE1 expression and its activity in a highly differentiated rat PC-12 cell line with imitated AD injury, whereas DOR antagonism with naltrindole reversed the UFP-512 effects while causing an enhancement of BACE1 expression and activity along with Aβ42 production under physiological conditions (Antonino et al., 2022). Knocking-down DOR in vivo increased BACE1 protein expression and its activity for APP processing, leading to a significant increase in Aβ42 production (Antonino et al., 2022). A different study found an association between opioid abuse and combined neuroinflammation and hyperphosphorylated tau in patients, which may confirm a pro-AD role for MOR activation (Anthony et al., 2010). Considering the opposite roles of MOR and DOR in activating BACE1 expression, there is a possibility to come up with a novel strategy against AD by differentially targeting DOR and MOR (Xu et al., 2020). Overall, these contrasting results of the studies reported above highlight the complexity of opioid activity in AD and highlights the need for further study to work out these differences.
In a genetic meta-analysis of AD patient cohorts, Sarajarvi and others found that a heterozygous DOR-Phe27Cys mutation increased the risk of AD, especially in late-stage AD patient postmortem brain samples in which there was a significant upregulation of BACE1 and γ-secretase activities (Sarajarvi et al., 2015). This is an important finding since approximately 25% of AD patients are heterozygous for the DOR-Phe27Cys variation and could potentially be used in patient selection and stratification for clinical studies using DOR antagonists as novel AD therapeutics. These observations clearly suggest that antagonism of DOR could be a novel therapeutic approach for the treatment of AD (Sarajärvi et al., 2015). In contrast to antagonism of DOR, agonist activation of MOR has also been reported to have salutary activity against AD through several mechanisms (Cui et al., 2011; Wang et al., 2015a; Dhull and Kumar, 2018). These studies suggest that selective antagonists of DOR and mixed DOR-antagonist/MOR-agonists could potentially emerge as a new therapeutic strategy against AD with fewer side effects (Figure 2).
Several biochemical and pharmacological studies using MOR and DOR ligands gave an early indication of physical and functional interactions between the two receptors (Starke et al., 1990). The MOR and DOR are present in pain modulating regions of the CNS on overlapping populations of neurons. It has also been shown that both MOR and DOR are present within the same functionally distinct heterodimeric or hetero-oligomeric complexes (George et al., 2000; Gomes et al., 2000; Levac et al., 2002; Fan et al., 2005). The physiological and pharmacological significance of MOR/DOR interactions have been substantiated by recent studies using opioid receptor gene knockout animals (Kieffer, 1999). Both MOR and DOR have an intermodulatory effect suggesting that ligands having a mixed interaction profile at both the receptors or at specific dimer pairs could serve as a novel therapeutic approach for the treatment of AD.
The KOR plays an important role in cognitive and learning functions and may also be involved in modulating AD pathology. The KOR (k1 and k2 variants) is among the most abundant brain opioid receptors. In the human brain, KOR has a wide and distinct distribution in the neocortex, striatum, thalamus, amygdala, and hippocampus (Simonin et al., 1995; Hiller and Fan, 1996) and is implicated in the pathophysiology of depression, anxiety, and alcoholism (McLaughlin et al., 2003; Tejeda et al., 2012). KOR activation was associated with stress-related dysmnesia, and these receptors could regulate glutamate neurotransmission and affect synaptic plasticity underlying memory formation. An early study showed evidence of increased kappa binding sites of AD brains at autopsy, indicating that KOR may also be involved in AD and other disorders, such as epilepsy and Tourette’s syndrome (Loacker et al., 2007; Cai and Ratka, 2012).
AD is a complex disease affected by both environmental and genetic factors (Xu et al., 2013). Epigenetics has emerged as an intermediate between environmental and genetic factors which could impact AD (Cai and Ratka, 2012). As an important component of epigenetics, genes with significantly changed DNA methylation have been found in AD patients (Van den Hove et al., 2014). Targeting epigenetic DNA methylation could be a therapeutic target for neurodegenerative disease such as Huntington’s disease and AD (Chang et al., 2014). Studies conducted on methylation of opioid receptor genes found that promoter DNA methylation may be dysregulated and thus may play a role in disease pathology (Sun et al., 2015). Several studies found that increased methylation levels of the four opioid receptor genes OPRM1, OPRL1, OPRK1 and OPRD1 indicate the epigenetic involvement of the opioid system in AD patients compared with healthy subjects (Xu et al., 2018). The above findings also suggest that opioid receptor genes could be used as potential methylation biomarkers for the diagnosis of AD (Ji et al., 2015a; Sun et al., 2015). A higher level of DNA methylation in the KOR (OPRK1) promoter CpG site was observed in patients with AD as compared to controls and this high methylation of OPRK1 could contribute to the risk of AD through its downregulation of gene expression (Ji et al., 2015).
Lastly, mechanistic/mammalian target of rapamycin (mTOR) has a very important role in neuronal plasticity, learning and memory and has a close association with many neurodevelopmental and neuropsychiatric disorders (Costa-Mattioli and Monteggia, 2013). Studies have reported that MOR activation attenuated Aβ oligomer-induced neurotoxicity through mTOR signaling. It may provide new insight into the pathological process and useful strategy for therapeutic interventions against Aβ neurotoxicity by targeting this mTOR/MOR relationship (Wang et al., 2015).
Future perspective
The studies above emphasize the importance of opioid receptors in AD modulation; however, the area needs to be explored further for better understanding. AD is a complex neurological disorder and currently there are no approved disease modifying therapies available. Efforts are underway to eliminate β-amyloid plaques through inhibiting two notable enzymes such as BACE1 and the γ-secretase enzyme. Nevertheless, these approaches have not yet achieved clinical success. Studies have recommended that indirect modulation of the function of these enzymes via opioid receptors be pursued to achieve reduction in Aβ pathology and identify lead compounds for further development as candidate AD therapeutics.
Author contributions
PT conceived the initial idea for the manuscript and wrote the first draft. JS contributed to editing and refining the manuscript to the final form, and also secured funding to support this work.
Funding
This work was funded by R01DA038635-S1 and institutional funds from the University of Arizona.
Conflict of interest
JS has an equity stake in Teleport Pharmaceuticals, LLC and Botanical Results, LLC.
The remaining author declares that the research was conducted in the absence of any commercial or financial relationships that could be construed as a potential conflict of interest.
Publisher’s note
All claims expressed in this article are solely those of the authors and do not necessarily represent those of their affiliated organizations, or those of the publisher, the editors and the reviewers. Any product that may be evaluated in this article, or claim that may be made by its manufacturer, is not guaranteed or endorsed by the publisher.
References
Al-Hasani, R., and Bruchas, M. R. (2011). Molecular mechanisms of opioid receptor-dependent signaling and behavior. Anesthesiology 115, 1363–1381. doi:10.1097/ALN.0b013e318238bba6
Alzheimer ’s Association (2013). Alzheimer ’s disease facts and figures. Alzheimers Dement. 9, 208. doi:10.1016/j.jalz.2013.02.003
Ananthan, S. (2008). Opioid ligands with mixed μ/δ opioid receptor interactions: An emerging approach to novel analgesics. Drug Addict. Basic Res. Ther. 8, 367–380. doi:10.1007/978-0-387-76678-2_23
Anthony, I. C., Norrby, K. E., Dingwall, T., Carnie, F. W., Millar, T., Arango, J. C., et al. (2010). Predisposition to accelerated Alzheimer-related changes in the brains of human immunodeficiency virus negative opiate abusers. Brain 133, 3685–3698. doi:10.1093/brain/awq263
Antonino, M., Marmo, P., Freites, C. L., Quassollo, G. E., Sánchez, M. F., Lorenzo, A., et al. (2022). Aβ assemblies promote amyloidogenic processing of APP and intracellular accumulation of Aβ42 through go/gβγ signaling. Front. Cell Dev. Biol. 10, 852738. doi:10.3389/fcell.2022.852738
Ballard, C., Gauthier, S., Corbett, A., Brayne, C., Aarsland, D., and Jones, E. (2011). Alzheimer’s disease. Lancet 377, 1019–1031. doi:10.1016/S0140-6736(10)61349-9
Barkin, R. L., Barkin, S. J., and Barkin, D. S. (2006). Propoxyphene (dextropropoxyphene): A critical review of a weak opioid analgesic that should remain in antiquity. Am. J. Ther. 13 (6), 534–542. PMID: 17122535. (n.d.). doi:10.1097/01.mjt.0000253850.86480.fb
Caffrey, T. M., and Wade-Martins, R. (2007). Functional MAPT haplotypes: Bridging the gap between genotype and neuropathology. Neurobiol. Dis. 27, 1–10. doi:10.1016/j.nbd.2007.04.006
Cai, Z., and Ratka, A. (2012). Opioid system and Alzheimer’s disease. Neuromolecular Med. 14, 91–111. doi:10.1007/s12017-012-8180-3
Canter, R. G., Penney, J., and Tsai, L. H. (2016). The road to restoring neural circuits for the treatment of Alzheimer’s disease. Nature 539, 187–196. doi:10.1038/nature20412
Chang, L., Wang, Y., Ji, H., Dai, D., Xu, X., Jiang, D., et al. (2014). Elevation of peripheral BDNF promoter methylation links to the risk of Alzheimer’s disease. PLoS One 9, e110773. doi:10.1371/journal.pone.0110773
Chen, S. L., Huang, E. Y. K., Chow, L. H., and Tao, P. L. (2005). Dextromethorphan differentially affects opioid antinociception in rats. Br. J. Pharmacol. 144, 400–404. doi:10.1038/sj.bjp.0706086
Chiba-Falek, O., Gottschalk, W. K., and Lutz, M. W. (2018). The effects of the TOMM40 poly-T alleles on Alzheimer’s disease phenotypes. Alzheimer’s Dementia 14, 692–698. doi:10.1016/j.jalz.2018.01.015
Comer, S. D., Sullivan, M. A., Yu, E., Rothenberg, J. L., Kleber, H. D., Kampman, K., et al. (2006). Injectable, sustained-release naltrexone for the treatment of opioid dependence: A randomized, placebo-controlled trial. Arch. Gen. Psychiatry 63 (2), 210–218. PMID: 16461865; PMCID: PMC4200530. doi:10.1001/archpsyc.63.2.210
Corder, E. H., Saunders, A. M., Strittmatter, W. J., Schmechel, D. E., Gaskell, P. C., Small, G. W., et al. (1993). Gene dose of apolipoprotein E type 4 allele and the risk of Alzheimer’s disease in late onset families. Sci. (1979) 261, 921–923. doi:10.1126/science.8346443
Costa-Mattioli, M., and Monteggia, L. M. (2013). mTOR complexes in neurodevelopmental and neuropsychiatric disorders. Nat. Neurosci. 16, 1537–1543. doi:10.1038/nn.3546
Cui, J., Wang, Y., Dong, Q., Wu, S., Xiao, X., Hu, J., et al. (2011). Morphine protects against intracellular amyloid toxicity by inducing estradiol release and upregulation of Hsp70. J. Neurosci. 31, 16227–16240. doi:10.1523/JNEUROSCI.3915-11.2011
DeWire, S. M., Ahn, S., Lefkowitz, R. J., and Shenoy, S. K. (2007). Beta-arrestins and cell signaling. Annu. Rev. Physiol. 69, 483–510. doi:10.1146/annurev.physiol.69.022405.154749
Dhull, D. K., and Kumar, A. (2018). Tramadol ameliorates behavioural, biochemical, mitochondrial and histological alterations in ICV-STZ-induced sporadic dementia of Alzheimer’s type in rats. Inflammopharmacology 26, 925–938. doi:10.1007/s10787-017-0431-3
Fan, T., Varghese, G., Nguyen, T., Tse, R., O’Dowd, B. F., and George, S. R. (2005). A role for the distal carboxyl tails in generating the novel pharmacology and G protein activation profile of μ and δ opioid receptor hetero-oligomers. J. Biol. Chem. 280, 38478–38488. doi:10.1074/jbc.M505644200
Farrer, L. A., Cupples, L. A., Haines, J. L., Hyman, B., Kukull, W. A., Mayeux, R., et al. (1997). Effects of age, sex, and ethnicity on the association between apolipoprotein E genotype and alzheimer disease: A meta-analysis. J. Am. Med. Assoc. 278, 1349–1356. doi:10.1001/jama.1997.03550160069041
Förster, K., Kuno, A., Solenkova, N., Felix, S. B., and Krieg, T. (2007). The δ-opioid receptor agonist DADLE at reperfusion protects the heart through activation of pro-survival kinases via EGF receptor transactivation. Am. J. Physiol. Heart Circ. Physiol. 293. H1604. doi:10.1152/ajpheart.00418.2007
Gallagher, M. (1985). Effect of β-funaltrexamine on retention of passive-avoidance conditioning. Behav. Neural Biol. 44, 499–502. doi:10.1016/S0163-1047(85)90976-8
George, S. R., Fan, T., Xie, Z., Tse, R., Tam, V., Varghese, G., et al. (2000). Oligomerization of μ- and δ-opioid receptors: Generation of novel functional properties. J. Biol. Chem. 275, 26128–26135. doi:10.1074/jbc.M000345200
Gomes, I., Jordan, B. A., Gupta, A., Trapaidze, N., Nagy, V., and Devi, L. A. (2000). Heterodimerization of mu and delta opioid receptors: A role in opiate synergy. J. Neurosci. 20, RC110–5. doi:10.1523/JNEUROSCI.20-22-j0007.2000
Granier, S., Manglik, A., Kruse, A. C., Kobilka, T. S., Thian, F. S., Weis, W. I., et al. (2012). Structure of the δ-opioid receptor bound to naltrindole. Nature 485, 400–404. doi:10.1038/nature11111
Hampel, H., Vassar, R., De Strooper, B., Hardy, J., Willem, M., Singh, N., et al. (2020). The β-secretase BACE1 in alzheimer’s disease. Biol. Psychiatry, 89, 745–756. doi:10.1016/j.biopsych.2020.02.001
Hernández, F., Barreda, E. G. de, Fuster-Matanzo, A., Goñi-Oliver, P., Lucas, J. J., and Avila, J. (2009). The role of GSK3 in Alzheimer disease. Brain Res. Bull. 80, 248–250. doi:10.1016/j.brainresbull.2009.05.017
Hiller, J. M., and Fan, L. Q. (1996). Laminar distribution of the multiple opioid receptors in the human cerebral cortex. Neurochem. Res. 21, 1333–1345. doi:10.1007/BF02532374
Hiller, J. M., Itzhak, Y., and Simon, E. J. (1987). Selective changes in mu, delta and kappa opioid receptor binding in certain limbic regions of the brain in Alzheimer's disease patients. Brain Res. 406, 17–23. doi:10.1016/0006-8993(87)90764-5
Ji, H., Wang, Y., Liu, G., Xu, X., Dai, D., Chen, Z., et al. (2015a). OPRK1 promoter hypermethylation increases the risk of Alzheimer’s disease. Neurosci. Lett. 606, 24–29. doi:10.1016/j.neulet.2015.08.027
Jutkiewicz, E. M. (2018). Correction to: Delta opioid receptor pharmacology and therapeutic applications. Handb. Exp. Pharmacol. 247, C1–C2. PMID: 31309359. doi:10.1007/978-3-319-95133-1_1001
Kieffer, B. L., and Gavériaux-Ruff, C. (2002). Exploring the opioid system by gene knockout. Prog. Neurobiol. 66, 285–306. doi:10.1016/S0301-0082(02)00008-4
Kieffer, B. L. (1999). Opioids: First lessons from knockout mice. Trends Pharmacol. Sci. 20, 19–26. doi:10.1016/S0165-6147(98)01279-6
Kimura, R., Kamino, K., Yamamoto, M., Nuripa, A., Kida, T., Kazui, H., et al. (2007). The DYRK1A gene, encoded in chromosome 21 Down syndrome critical region, bridges between β-amyloid production and tau phosphorylation in Alzheimer disease. Hum. Mol. Genet. 16, 15–23. doi:10.1093/hmg/ddl437
Krieter, P., Gyaw, S., Crystal, R., and Skolnick, P. (2019). Fighting fire with fire: Development of intranasal nalmefene to treat synthetic opioid overdose. J. Pharmacol. Exp. Ther. 371, 409–415. doi:10.1124/jpet.118.256115
Kwok, J. B. J., Loy, C. T., Hamilton, G., Lau, E., Hallupp, M., Williams, J., et al. (2008). Glycogen synthase kinase-3beta and tau genes interact in Alzheimer's disease. Ann. Neurol. 64, 446–454. doi:10.1002/ana.21476
Levac, B. A. R., O’Dowd, B. F., and George, S. R. (2002). Oligomerization of opioid receptors: Generation of novel signaling units. Curr. Opin. Pharmacol. 2, 76–81. doi:10.1016/S1471-4892(02)00124-8
Lim, T. Y., Stringfellow, E. J., Stafford, C. A., DiGennaro, C., Homer, J. B., Wakeland, W., et al. (2022). Modeling the evolution of the US opioid crisis for national policy development. Proc. Natl. Acad. Sci. U. S. A. 119 (23), e2115714119. Epub 2022 May 31. PMID: 35639699; PMCID: PMC9191351. doi:10.1073/pnas.2115714119
Lim, Y. Y., and Mormino, E. C.Alzheimer's Disease Neuroimaging Initiative (2017). APOE genotype and early β-amyloid accumulation in older adults without dementia. Neurology 89, 1028–1034. doi:10.1212/WNL.0000000000004336
Listos, J., Łupina, M., Talarek, S., Mazur, A., Orzelska-Górka, J., and Kotlińska, J. (2019). The mechanisms involved in morphine addiction: An overview. Int. J. Mol. Sci. 20. 4302, doi:10.3390/ijms20174302
Liu, C. C., Kanekiyo, T., Xu, H., and Bu, G. (2013). Apolipoprotein e and Alzheimer disease: Risk, mechanisms and therapy. Nat. Rev. Neurol. 9, 106–118. doi:10.1038/nrneurol.2012.263
Liu, X., Zhao, X., Zeng, X., Bossers, K., Swaab, D. F., Zhao, J., et al. (2013b). β-Arrestin1 regulates γ-secretase complex assembly and modulates amyloid-β pathology. Cell Res. 23, 351–365. doi:10.1038/cr.2012.167
Loacker, S., Sayyah, M., Wittmann, W., Herzog, H., and Schwarzer, C. (2007). Endogenous dynorphin in epileptogenesis and epilepsy: Anticonvulsant net effect via kappa opioid receptors. Brain 130, 1017–1028. doi:10.1093/brain/awl384
Mafi, A., Kim, S. K., and Goddard, W. A. (2021). The G protein-first activation mechanism of opioid receptors by Gi protein and agonists. QRB Discov. 2, e9. doi:10.1017/qrd.2021.7
Mathieu-Kia, A. M., Fan, L. Q., Kreek, M. J., Simon, E. J., and Hiller, J. M. (2001c). Mu-delta- and kappa-opioid receptor populations are differentially altered in distinct areas of postmortem brains of Alzheimer's disease patients. Brain Res. 893, 121–134. doi:10.1016/S0006-8993(00)03302-3
McLaughlin, J. P., Marton-Popovici, M., and Chavkin, C. (2003). Kappa opioid receptor antagonism and prodynorphin gene disruption block stress-induced behavioral responses. J. Neurosci. 23, 5674–5683. doi:10.1523/JNEUROSCI.23-13-05674.2003
Meilandt, W. J., Yu, G. Q., Chin, J., Roberson, E. D., Palop, J. J., Wu, T., et al. (2008a). Enkephalin elevations contribute to neuronal and behavioral impairments in a transgenic mouse model of Alzheimer’s disease. J. Neurosci. 28, 5007–5017. doi:10.1523/JNEUROSCI.0590-08.2008
Mercorio, R., Pergoli, L., Galimberti, D., Favero, C., Carugno, M., Dalla Valle, E., et al. (2018). PICALM gene methylation in blood of Alzheimer’s disease patients is associated with cognitive decline. J. Alzheimer’s Dis. 65, 283–292. doi:10.3233/JAD-180242
Metcalf, M. D., Yekkirala, A. S., Powers, M. D., Kitto, K. F., Fairbanks, C. A., Wilcox, G. L., et al. (2012). The δ opioid receptor agonist SNC80 selectively activates heteromeric μ-δ Opioid receptors. ACS Chem. Neurosci. 3, 505–509. doi:10.1021/cn3000394
Morris, J. C., Roe, C. M., Xiong, C., Fagan, A. M., Goate, A. M., Holtzman, D. M., et al. (2011). APOE predicts Aβ but not tau alzheimer’s pathology in cognitively normal aging. Ann. Neurol. 67, 122–131. doi:10.1002/ana.21843.APOE
Moussawi, K., Ortiz, M. M., Gantz, S. C., Tunstall, B. J., Marchette, R. C. N., Bonci, A., et al. (2020). Fentanyl vapor self-administration model in mice to study opioid addiction. Sci. Adv. 6 (32), eabc0413. PMID: 32821843; PMCID: PMC7406365. doi:10.1126/sciadv.abc0413
Myers, A. J., Kaleem, M., Marlowe, L., Pittman, A. M., Lees, A. J., Fung, H. C., et al. (2005). The H1c haplotype at the MAPT locus is associated with Alzheimer’s disease. Hum. Mol. Genet. 14, 2399–2404. doi:10.1093/hmg/ddi241
Nam, M. H., Won, W., Han, K. S., and Lee, C. J. (2021). Signaling mechanisms of μ-opioid receptor (MOR) in the hippocampus: Disinhibition versus astrocytic glutamate regulation. Cell. Mol. Life Sci. 78, 415–426. doi:10.1007/s00018-020-03595-8
Nelson, C. D., and Sheng, M. (2013). GPR3 stimulates Aβ production via interactions with APP and β-arrestin2. PLoS One 8, e74680. doi:10.1371/journal.pone.0074680
Ni, Y., Zhao, X., Bao, G., Zou, L., Teng, L., Wang, Z., et al. (2006a). Activation of beta2-adrenergic receptor stimulates gamma-secretase activity and accelerates amyloid plaque formation. Nat. Med. 12, 1390–1396. doi:10.1038/nm1485
O’Connor, A., Schug, S. A., Cardwell, H., and O'Connor, A. (2000). A comparison of the efficacy and safety of morphine and pethidine as analgesia for suspected renal colic in the emergency setting. J. Accid. Emerg. Med. 17, 261–264. doi:10.1136/emj.17.4.261
Pasternak, G. W. (2004). Multiple opiate receptors: Déjà vu all over again. Neuropharmacology 47, 312–323. doi:10.1016/j.neuropharm.2004.07.004
Pathan, H., and Williams, J. (2012). Basic opioid pharmacology: An update. Br. J. Pain 6, 11–16. doi:10.1177/2049463712438493
Reynolds, D. S. (2019). A short perspective on the long road to effective treatments for Alzheimer’s disease. Br. J. Pharmacol. 176, 3636–3648. doi:10.1111/bph.14581
Sakamoto, K., Yamada, D., Yamanaka, N., Nishida, M., Iio, K., Nagase, H., et al. (2021). A selective delta opioid receptor agonist SNC80, but not KNT-127, induced tremor-like behaviors via hippocampal glutamatergic system in mice. Brain Res. 1757, 147297. doi:10.1016/j.brainres.2021.147297
Sarajärvi, T., Marttinen, M., Natunen, T., Kauppinen, T., Mäkinen, P., Helisalmi, S., et al. (2015). Genetic variation in δ-opioid receptor associates with increased β- and γ-secretase activity in the late stages of alzheimer’s disease. J. Alzheimer’s Dis. 48, 507–516. doi:10.3233/JAD-150221
Satoh, M., Seki, T., and Minami, M. (2000a). Opioid receptors. Tanpakushitsu Kakusan Koso 45, 953–990. doi:10.1146/annurev.biochem.73.011303.073940
Scheltens, P., Blennow, K., Breteler, M. M. B., de Strooper, B., Frisoni, G. B., Salloway, S., et al. (2016). Alzheimer’s disease. Lancet 388, 505–517. doi:10.1016/S0140-6736(15)01124-1
Simonin, F., Gavériaux-Ruff, C., Befort, K., Matthes, H., Lannes, B., Micheletti, G., et al. (1995). kappa-Opioid receptor in humans: cDNA and genomic cloning, chromosomal assignment, functional expression, pharmacology, and expression pattern in the central nervous system. Proc. Natl. Acad. Sci. U. S. A. 92, 7006–7010. doi:10.1073/pnas.92.15.7006
Skarphedinsson, J. O., and Thoren, P. (1988). Endorphin mechanisms are responsible for the beneficial effects of opioid antagonists on cerebral function during relative cerebral ischaemia in rats. Acta Physiol. Scand. 132, 281–288. doi:10.1111/j.1748-1716.1988.tb08331.x
Starke, K., Board, E., Eichelbaum, M., Ganten, D., Hofmann, F., Kobilka, B., et al. (1990). Handb. Exp. Pharmacol. 167. doi:10.1016/0165-6147(90)90130-Z
Stefanucci, A., della Valle, A., Scioli, G., Marinaccio, L., Pieretti, S., Minosi, P., et al. (2022). Discovery of κ opioid receptor (KOR)-Selective d -tetrapeptides with improved in vivo antinociceptive effect after peripheral administration. ACS Med. Chem. Lett. 13, 1707–1714. doi:10.1021/acsmedchemlett.2c00237
Stefanucci, A., Minosi, P., Pieretti, S., Tanguturi, P., Molnar, G., Scioli, G., et al. (2023). Design of analgesic trivalent peptides with low withdrawal symptoms: Probing the antinociceptive profile of novel linear and cyclic peptides as opioid Pan ligands. ACS Chem. Neurosci. 14, 506, 515. doi:10.1021/acschemneuro.3c00005
Stefanucci, A., Novellino, E., Mirzaie, S., Macedonio, G., Pieretti, S., Minosi, P., et al. (2017). Opioid receptor activity and analgesic potency of DPDPE peptide analogues containing a xylene bridge. ACS Med. Chem. Lett. 8, 449–454. doi:10.1021/acsmedchemlett.7b00044
Strain, E. C., Bigelow, G. E., Liebson, I. A., and Stitzer, M. L. (1999). Moderate-vs high-dose methadone in the treatment of opioid dependence: A randomized trial. JAMA 281 (11), 1000–1005. doi:10.1001/jama.281.11.1000
Strittmatter, W. J., Saunders, A. M., Schmechel, D., Pericak-Vance, M., Enghild, J., Salvesen, G. S., et al. (1993). Apolipoprotein E: High-avidity binding to β-amyloid and increased frequency of type 4 allele in late-onset familial Alzheimer disease. Proc. Natl. Acad. Sci. U. S. A. 90, 1977–1981. doi:10.1073/pnas.90.5.1977
Sun, Y., Sahbaie, P., Liang, D. Y., Li, W., Shi, X., Kingery, P., et al. (2015). DNA methylation modulates nociceptive sensitization after incision. PLoS One 10, e0142046. doi:10.1371/journal.pone.0142046
Sunderland, T., Tariot, P. N., Herbert, W., Murphy, D. L., Newhouse, P. A., Mueller, E. A., et al. (1986). Pharmacologic modelling of Alzheimer’s disease, Prog. Neuro-Psychopharmacology Biol. Psychiatry 10, 599–610. doi:10.1016/0278-5846(86)90030-8
Tanguturi, P., Pathak, V., Zhang, S., Moukha-Chafiq, O., Augelli-Szafran, C. E., and Streicher, J. M. (2022). Correction: Tanguturi et al. Discovery of Novel Delta Opioid Receptor (DOR) Inverse Agonist and Irreversible (Non-Competitive) Antagonists. Molecules 26, 1969. doi:10.3390/molecules27061969
Tejeda, H. A., Shippenberg, T. S., and Henriksson, R. (2012). The dynorphin/κ-opioid receptor system and its role in psychiatric disorders. Cell. Mol. Life Sci. 69, 857–896. doi:10.1007/s00018-011-0844-x
Teng, L., Zhao, J., Wang, F., Ma, L., and Pei, G. (2010). A GPCR/secretase complex regulates beta- and gamma-secretase specificity for Abeta production and contributes to AD pathogenesis. Cell Res. 20, 138–153. doi:10.1038/cr.2010.3
Thathiah, A., and De Strooper, B. (2011). The role of G protein-coupled receptors in the pathology of Alzheimer’s disease. Nat. Rev. Neurosci. 12, 73–87. doi:10.1038/nrn2977
Thathiah, A., Horré, K., Snellinx, A., Vandewyer, E., Huang, Y., Ciesielska, M., et al. (2013). β-Arrestin 2 regulates Aβ generation and γ-secretase activity in Alzheimer’s disease. Nat. Med. 19, 43–49. doi:10.1038/nm.3023
Vaidya, B., Sifat, A. E., Karamyan, V. T., and Abbruscato, T. J. (2018). The neuroprotective role of the brain opioid system in stroke injury. Drug Discov. Today 23, 1385–1395. doi:10.1016/j.drudis.2018.02.011
Van den Hove, D. L., Kompotis, K., Lardenoije, R., Kenis, G., Mill, J., Steinbusch, H. W., et al. (2014). Epigenetically regulated microRNAs in Alzheimer’s disease. Neurobiol. Aging 35, 731–745. doi:10.1016/j.neurobiolaging.2013.10.082
Vassilatis, D. K., Hohmann, J. G., Zeng, H., Li, F., Ranchalis, J. E., Mortrud, M. T., et al. (2003). The G protein-coupled receptor repertoires of human and mouse. Proc. Natl. Acad. Sci. U. S. A. 100, 4903–4908. doi:10.1073/pnas.0230374100
Wang, Y., Wang, Y. X., Liu, T., Law, P. Y., Loh, H. H., Qiu, Y., et al. (2015a). μ-Opioid receptor attenuates Aβ oligomers-induced neurotoxicity through mTOR signaling. CNS Neurosci. Ther. 21, 8–14. doi:10.1111/cns.12316
Wei, L. N., and Loh, H. H. (2002). Regulation of opioid receptor expression. Curr. Opin. Pharmacol. 2, 69–75. doi:10.1016/S1471-4892(01)00123-0
Wisely, E. V., Xiang, Y. K., and Oddo, S. (2014). Genetic suppression of β2-adrenergic receptors ameliorates tau pathology in a mouse model of tauopathies. Hum. Mol. Genet. 23, 4024–4034. doi:10.1093/hmg/ddu116
Xia, Y. (2015). Neural functions of the delta- opioid receptor. Berlin/Heidelberg, Germany: Springer.
Xu, C., Liu, G., Ji, H., Chen, W., Dai, D., Chen, Z., et al. (2018). Elevated methylation of OPRM1 and OPRL1 genes in Alzheimer’s disease. Mol. Med. Rep. 18, 4297–4302. doi:10.3892/mmr.2018.9424
Xu, X., Wang, Y., Wang, L., Liao, Q., Chang, L., Xu, L., et al. (2013). Meta-analyses of 8 polymorphisms associated with the risk of the alzheimer’s disease. PLoS One 8, e73129. doi:10.1371/journal.pone.0073129
Xu, Y., Zhi, F., Balboni, G., Yang, Y., and Xia, Y. (2020). Opposite roles of δ- and μ-opioid receptors in BACE1 regulation and alzheimer’s injury. Front. Cell Neurosci. 14, 88–13. doi:10.3389/fncel.2020.00088
Keywords: neurodegenerative disorders, Alzheimer’s disease, opioid receptors, β-secretase-1, amyloid-β
Citation: Tanguturi P and Streicher JM (2023) The role of opioid receptors in modulating Alzheimer’s Disease. Front. Pharmacol. 14:1056402. doi: 10.3389/fphar.2023.1056402
Received: 28 September 2022; Accepted: 20 February 2023;
Published: 01 March 2023.
Edited by:
Budheswar Dehury, Regional Medical Research Center (ICMR), IndiaReviewed by:
Shamseddin Ahmadi, University of Kurdistan, IranSunita Panda, Regional Medical Research Center (ICMR), India
Copyright © 2023 Tanguturi and Streicher. This is an open-access article distributed under the terms of the Creative Commons Attribution License (CC BY). The use, distribution or reproduction in other forums is permitted, provided the original author(s) and the copyright owner(s) are credited and that the original publication in this journal is cited, in accordance with accepted academic practice. No use, distribution or reproduction is permitted which does not comply with these terms.
*Correspondence: John M. Streicher, anN0cmVpY2hlckBhcml6b25hLmVkdQ==