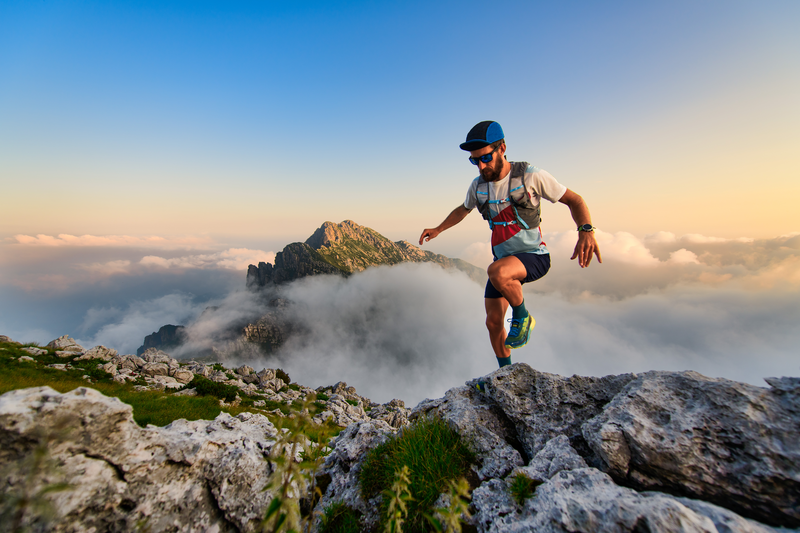
94% of researchers rate our articles as excellent or good
Learn more about the work of our research integrity team to safeguard the quality of each article we publish.
Find out more
REVIEW article
Front. Pharmacol. , 02 May 2023
Sec. Inflammation Pharmacology
Volume 14 - 2023 | https://doi.org/10.3389/fphar.2023.1045997
This article is part of the Research Topic Rising Stars in Inflammation Pharmacology: 2022 View all 5 articles
Inflammatory bowel disease (IBD) is a chronic disease that is characterized by intestinal inflammation. Epithelial damage and loss of intestinal barrier function are believed to be the hallmark pathologies of the disease. In IBD, the resident and infiltrating immune cells consume much oxygen, rendering the inflamed intestinal mucosa hypoxic. In hypoxia, the hypoxia-inducible factor (HIF) is induced to cope with the lack of oxygen and protect intestinal barrier. Protein stability of HIF is tightly controlled by prolyl hydroxylases (PHDs). Stabilization of HIF through inhibition of PHDs is appearing as a new strategy of IBD treatment. Studies have shown that PHD-targeting is beneficial to the treatment of IBD. In this Review, we summarize the current understanding of the role of HIF and PHDs in IBD and discuss the therapeutic potential of targeting PHD-HIF pathway for IBD treatment.
Inflammatory bowel disease (IBD) is a chronic inflammatory disorder of the gastrointestinal tract (Zhang and Li, 2014). The major types of IBD are Crohn’s disease (CD) and ulcerative colitis (UC). IBD is debilitating and associated with the development of a number of complications. It affects millions of people worldwide with higher incidence in developed countries (Loftus, 2004). The pathology of IBD is complex and may involve a combination of genetic, environmental factors and immunological abnormalities (Kaser et al., 2010; Ananthakrishnan et al., 2018). The core pathology of IBD is believed to be the disruption of epithelial barrier that separates the intestinal lumen from the mucosal immune system. The impairment of intestinal barrier leads to exposure of mucosal immune cells to microorganisms and antigens, which causes inflammation and ruins the integrity of the intestinal barrier, resulting in progressive and cyclical inflammation as well as a long-term damage to the intestine (Brown and Taylor, 2018). Currently, antibiotics, anti-inflammatory agents and surgery are employed in the treatment of IBD. The effectiveness of these treatments is variable and usually unsatisfactory. There is an unmet medical need for this disease.
Compared with other tissues, intestine is hypoxic and intestinal inflammation exacerbates the lack of oxygen (Taylor and Colgan, 2017). Mucosal hypoxia is an integral component of IBD. The intestine is highly dependent on the adaptive pathways activated by hypoxia. Studies have revealed that hypoxia-inducible factor (HIF) protects intestinal barrier and elicits anti-inflammatory responses. Protein stability of HIF is tightly regulated by prolyl hydroxylases (PHDs). Induction of expression of HIF through inhibition of PHDs has been shown beneficial to IBD treatment (Manresa and Taylor, 2017; Van Welden et al., 2017a). Herein, we review the current understanding of the impacts of HIF and PHDs on IBD and discuss the therapeutic potential of targeting PHD-HIF axis for the treatment of this disease.
Hypoxia is a feature of the intestinal mucosa. Under normal conditions, there is a steep oxygen gradient from the anaerobic lumen to the oxygen-rich submucosa in the gastrointestinal tract (Taylor and Colgan, 2007). The oxygen contents in the small intestinal wall and the villus tip are about 8% and 3%, respectively. The oxygen content in gut lumen is less than 2%, whereas the arteries in the submucosa have an oxygen level around 80%–100% (He et al., 1999; Fisher et al., 2013; Zeitouni et al., 2016).
Due to increased cell metabolism and decreased supply of oxygen, the inflamed regions are usually short of oxygen (Fraisl et al., 2009; Eltzschig and Carmeliet, 2011; Bartels et al., 2013; Eltzschig et al., 2014). During inflammation, the resident immune cells such as macrophages and dendritic cells are activated. These activated immune cells produce proinflammatory cytokines and chemokines, which induces differentiation of T cells and recruits inflammatory cells from blood to mucosa. The infiltrated immune cells and intestinal epithelial cells in the inflamed regions consume a large amount of oxygen (Campbell et al., 2014). In the meantime, the microthrombosis in inflamed tissues may cause decreased oxygen supply from the bloodstream (Hatoum et al., 2003). The increased oxygen consumption and decreased oxygen supply result in lack of oxygen in the inflamed mucosa. Multiple IBD models have demonstrated that the inflamed intestinal mucosa is short of oxygen and hypoxia is a common feature in the inflamed mucosa in IBD (Taylor and Colgan, 2017).
In the intestine, the adaptation of the cells to the lack of oxygen is regulated by HIF. HIF is a basic helix-loop-helix-PAS domain transcription factor that is composed of an alpha subunit (HIF-1α, -2α and -3α) and a constitutively expressed beta subunit (known as HIF-1β) (Wang et al., 1995). HIFα binds HIF-1β to form an active transcription factor. The transcription factor recruits co-factors p300 and CBP and the complex binds hypoxia responsive elements (HRE) within or near target genes to initiate transcription of the genes that are involved in cell survival, angiogenesis and metabolism (Figure 1) (Schofield and Ratcliffe, 2004; Chowdhury et al., 2008; Ortiz-Barahona et al., 2010; Biddlestone et al., 2015; Taylor and Scholz, 2022; Wicks and Semenza, 2022).
FIGURE 1. The PHD-HIF oxygen-sensing system. In well oxygenated cells (schematic on the right side), PHDs hydroxylate HIFα on specific proline residues using O2 and 2-OG as co-substrates. The E3 ubiquitin ligase pVHL recognizes the hydroxylated HIFα and mediates HIFα ubiquitination and proteasomal degradation. In hypoxic cells (left side), the PHDs’ prolyl hydroxylase activity is inhibited, leading to HIFα accumulation. HIFα then dimerizes with HIF-1β and recruits CBP and p300 co-factors. The complex binds to hypoxia response element (HRE) within or near target genes to activate transcription of these genes. Abbreviations used: HIF, hypoxia-inducible factor; PHD, prolyl hydroxylase; Ub, ubiquitin; pVHL, the von Hippel-Lindau protein; 2-OG, 2-oxoglutarate.
Stability of HIFα is controlled by PHDs. In the presence of oxygen, PHDs hydroxylate the highly conserved proline residues of HIFα. The hydroxylated HIFα is then recognized by the von Hippel-Lindau (pVHL) protein, an E3 ubiquitin ligase, and is ubiquitinated for degradation (Maxwell et al., 1999; Ivan et al., 2001) (Figure 1). PHDs are dioxygenases that use O2 and 2-oxoglutarate (2-OG) as co-substrates and Fe2+ and ascorbic acid as co-factors. The PHD family has three members: PHD1 (EglN2), PHD2 (EglN1) and PHD3 (EglN3). PHD1, PHD2 and PHD3 share conserved C-terminal regions that are responsible for the prolyl hydroxylase activity. However, these enzymes have great differences at N terminus (Epstein et al., 2001). Each protein has its tissue- and cell-specific expression pattern as well as particular cellular distribution (Metzen et al., 2003).
Oxygen is the most important factor that controls prolyl hydroxylation catalyzed by PHDs. In hypoxia, the PHDs’ enzymatic activities are inhibited, leading to accumulation of HIFα and subsequent expression of HIFα target genes. The affinity of PHDs to oxygen is relatively low and this makes PHDs sensing oxygen in a physiologically relevant concentration range (Kaelin and Ratcliffe, 2008). Recent studies indicate that the PHDs oxygen KM values are close to 100 μmol/L (Koivunen et al., 2006; Ehrismann et al., 2007), which are higher than tissue oxygen concentrations (10–30 μmol/L). The oxygen content in cells is smaller than the apparent KM for oxygen, allowing that the enzymatic activities of PHDs are strictly controlled by oxygen content over the entire physiologic range (Kaelin and Ratcliffe, 2008). This characteristic makes PHDs good oxygen sensors in tissues. The PHDs and HIFα are the core of the oxygen-sensing machinery in metazoans.
Although all PHD isoforms hydroxylate HIF-1α and HIF-2α, they have differential selectivity in relation to the hydroxylation of them (Appelhoff et al., 2004). PHD2 has a greater influence on the expression of HIF-1α than that of HIF-2α, while PHD1 and PHD3 have a greater effect on the expression of HIF-2α (Appelhoff et al., 2004). Of the PHD family members, PHD2 appears to be the primary HIFα prolyl hydroxylase and the key oxygen sensor (Berra et al., 2003; Minamishima et al., 2008; Takeda et al., 2008). The PHD2 and PHD3 genes have HRE in their promoter regions and their transcription can be modulated by oxygen contents (Metzen et al., 2005; Pescador et al., 2005). Under hypoxia, expression of PHD2 and PHD3 genes is activated and the increased PHD2 and PHD3 proteins are believed to decrease the HIF response to chronic hypoxia, which may limit HIFα protein levels under hypoxia. Although the enzymatic activities of PHD2 and PHD3 are inhibited in hypoxic conditions, their presence may cause immediately the degradation of HIFα once the oxygen levels increase, thereby forming a negative feedback regulation (Stiehl et al., 2006). Interestingly, the expression of PHD1 gene is not regulated by such a feedback mechanism (Marxsen et al., 2004).
The intestine epithelial cells play an important role in defensing against microorganisms in the intestinal tract. In intestine epithelial cells, HIF-1α and HIF-2α are demonstrated to be critical in keeping barrier function and wound healing capacity (Karhausen et al., 2004; Ramakrishnan and Shah, 2016). The intestinal barriers are dynamic in nature. They are maintained mostly by mucus layer, intercellular tight junction (TJ) and adherens junction (AJ). Many investigations have demonstrated that HIFs have a barrier-protective function in the intestine (Furuta et al., 2001; Synnestvedt et al., 2002; Eltzschig et al., 2003). Both HIF-1α and HIF-2α are found expressed in human and mice intestine epithelial cells (Giatromanolaki et al., 2003; Glover et al., 2013; Xue et al., 2013). The involvement of HIF-1α and HIF-2α in the protection of intestinal epithelial barrier is well investigated and the results indicate that they are key regulators.
Studies of active inflammation in mouse models of IBD have shown the intestinal epithelial cell to be a primary target for hypoxia (Karhausen et al., 2004). Strong evidence has demonstrated that HIF-1α plays a crucial role in the maintenance of intestinal barrier, and it is widely regarded as a protective factor (Figure 2), making it a potential therapeutic target for IBD (Colgan and Taylor, 2010). A major line of defense to the gut microorganisms and other pathogens is the production of mucus (Hansson, 2020). The mucus is the first barrier that gut microbes and pathogens meet. The intestine goblet cells are a kind of epithelial cells that produce and secrete mucus. Several mucins such as mucin-3 and muc5ac are the major glycoproteins in mucus and expression of these mucins are regulated by HIF-1α (Louis et al., 2006; Young et al., 2007). In addition to the mucus layer, TJ forms the core mechanism regulating the integrity of intestinal barrier. Claudin-1 is a major tight junctional protein, and its expression is directly regulated by HIF-1 (Saeedi et al., 2015). Inactivation of HIF-1 resulted in a defect in the formation of tight barriers, which was redeemed by the expression of claudin-1.
FIGURE 2. Protective role of HIF in intestinal epithelial barrier. Activation of HIF-1 in the intestine epithelial cells induces a barrier-protective pathway by increasing the expression of barrier-protective proteins such as mucus, trefoil factor 3, CD39, CD73 and β-defensin-1 and TJ protein claudin-1. Activation of HIF-2 promotes the expression of CK and VEGF that promotes AJ and angiogenesis, respectively. Abbreviations: AJ, adhesion junction; CK, creatine kinase; TJ, tight junction; VEGF, vascular endothelial growth factor.
Stabilization of HIF through inhibition of PHDs promoted intestinal fibroblast-mediated collagen gel contraction, an important step in the wound-healing process (Ngo et al., 2006). It was demonstrated that pharmacological activation of HIF increased contraction of collagen gels seeded with mouse embryonic fibroblast NIH-3T3 cells (Robinson et al., 2008). Further studies using human CCD-18CO intestinal fibroblast cells were performed. When seeded in collagen gels, the cells showed a significant increase contraction when treated with PHD inhibitors, and this contraction correlated directly with increases in HIF stabilization (Keely et al., 2009). Mechanistically, HIF-1 induced expression of fibroblast integrin beta one and controlled fibroblast contraction during intestinal wound healing. HIF may also promote intestinal epithelial healing through induction of α-integrin (Goggins et al., 2021). It was shown that HIF-1α induced expression of integrins α6 and α2 to promote intestinal epithelial migration and proliferation, which played an important role in epithelial restitution. These results indicated that PHDs inhibitor stabilized HIF-1α and accelerated intestinal mucosal healing by inducing epithelial integrin expression.
In addition to direct regulation of the intestinal barrier, HIF-1α mediates several indirect mechanisms to maintain the barrier integrity. The expression of intestinal trefoil factor 3, a barrier protective protein, is regulated directly by HIF-1α (Furuta et al., 2001). Trefoil factor 3 plays an important role in repairing epithelial surfaces. Expression of CD39 and CD73, two important membrane-bound proteins, are regulated by HIF-1α. CD39 is associated with the conversion of ATP/ADP to AMP, and CD73 is involved in the degradation of AMP to adenosine (Allard et al., 2017). The CD39-and CD73-mediated degradation of ATP is critical for restoring the barrier (Synnestvedt et al., 2002). There are anti-microbial peptides in mucus layer. Beta-defensin-1, an anti-microbial peptide that is secreted by intestine epithelial cells into the mucus layer, protects against commensal overgrowth and pathogen infiltration. HIF-1α plays a critical role in the induction of production of β-defensin-1 in intestinal epithelial cells (Kelly et al., 2013). The epithelial HIF-1 may also preserve the intestinal barrier function through the induction of expression of other barrier-protective genes such as CD55 (Louis et al., 2005) and netrin-1 (Rosenberger et al., 2009), and the enhancement of extracellular adenosine signaling while inhibiting the expression of adenosine transporters and the genes related to epithelial cell death such as FADD (Hindryckx et al., 2010).
In mouse model studies, the deletion of HIF-1α in intestine epithelial cells demonstrated a major defect in the integrity of mucosal barrier. Deletion of HIF-1α in mice intestine epithelial cells showed that the absence of HIF-1α caused more severe 2,4,6-trinitrobenzene sulfonic acid (TNBS)-induced colitis, while the constitutive HIF-1α activation was protective (Karhausen et al., 2004). The mice lacking HIF-1α in intestine epithelial cells were more sensitive to bacterial toxin with a more severe colitis phenotype as compared to the control mice (Hirota et al., 2010). Together, these data suggest that epithelial HIF-1 plays a critical role in intestinal barrier protection.
HIF-2α also plays an important role in keeping homeostasis of the intestinal barrier. It regulates cell metabolism and proliferation that are required for repair of intestine epithelial injury, which is essential for highly regenerative intestinal epithelium (van der Flier and Clevers, 2009). It was reported that HIF-2α promoted the expression of creatine kinases (CKs) including CKB (brain type) and CKM (muscle type), the enzymes that are critical for rapid ATP production in intestine epithelial cells (Glover et al., 2013). The authors showed that CKs were localized to the apical intestinal epithelial cell AJ, where they were important for the AJ assembly and epithelium integrity. In a radiation-induced model of intestinal injury in mice, activation of HIF-2α was found protective by increasing crypt regeneration in vascular endothelial growth factor (VEGF)- and angiogenesis-dependent manners (Taniguchi et al., 2014). It should be noted that, in some studies, HIF-2α was found to have detrimental effects in animal models of bowel inflammation (Xue et al., 2013; Solanki et al., 2019). The role of HIF-2α in IBD remains unclear and further studies are warranted.
In hypoxia, cells shift mitochondrial respiration to glycolysis. The hypoxia-induced HIF-1α plays an important role in this metabolic switch through inducing the expression of glucose transporters and glycolytic enzymes (Seagroves et al., 2001; Kierans and Taylor, 2021), and through suppressing mitochondrial oxidative phosphorylation (Kim et al., 2006; Papandreou et al., 2006). The HIF-1α-promoted glycolysis may enhance the production and release of lactate, leading to acidification of the extracellular microenvironment (Pavlova et al., 2022), which may impact the metabolism of gut microbial communities (Taylor et al., 2022). A recent report demonstrated that the intestinal HIF-2α also positively regulated gut lactate by controlling the expression of intestinal LDHA, which shaped the gut microbiome (Wu et al., 2021). It was shown that treatment with lactate-producing Saccharomyces cerevisiae modulated gut microbiota and attenuated dextran sulfate sodium (DSS)-induced colitis in mice (Sun et al., 2021). Thus, activation of HIF through inhibition of PHDs may regulate intestinal barrier function through the induction of lactate and the modulation of gut microbiota.
Hypoxia influences immunity through induction of expression of HIFα (Dvornikova et al., 2023). HIF-1α was shown to promote neutrophil survival and enhance glycolysis (Cramer et al., 2003; Walmsley et al., 2005) (Figure 3). The macrophages rely on glycolysis to produce ATP, which is also regulated by HIF-1α (Cramer et al., 2003). The mice macrophages lacking HIF-1α cannot produce sufficient ATP, which may disserve the capability of survivability, motility, invasiveness and bacterial killing of these cells (Cramer et al., 2003). In dentric cells activation of HIF-1α promotes production of interferon, IL-22 and IL-10 and induces cell differentiation as well as cell migration (Köhler et al., 2012; Naldini et al., 2012; Wobben et al., 2013). In DSS colitis model, the mice that HIF-1α was specifically knocked out in dentric cells were more sensitive to DSS treatment as compared to the control mice (Flück et al., 2016). This was related to disrupted development of regulatory T cells (Tregs), which was caused by decreased formation of dentric cell-induced C–C chemokine receptor type 9, a marker of gut-homing T-cells, and by decreased expression of aldehyde dehydrogenase 1a2, an enzyme involved in Tregs induction. HIF-2α is also involved in the regulation of macrophages and natural killer (NK) cells. Fang et al. demonstrated that HIF-2α induced the expression of cell surface receptors and tumor-promoting cytokines in human and murine macrophages as HIF-1α did in hypoxia (Fang et al., 2009). Zhang et al. showed that HIF-2α limited NK cell cytotoxicity (Zhang et al., 2016), which indicates that HIF-2α may have an anti-inflammatory role.
FIGURE 3. Roles of HIF-1α in immune cells. HIF-1α promotes survivability and motility of macrophage, stimulates production of interferon, IL-22 and IL-10 by dentric cells, favors differentiation of Treg cells and decreases proliferation and increases death of B cells.
HIFs are also implicated in the regulation of adaptive immune cells, including T cells and B cells. It was shown that the T cell-specific HIF-1α knockout mice had more severe gut inflammation with increased amounts of TH1 and TH17 cells when treated with DSS, implying that HIF-1α favors the differentiation of Tregs (Higashiyama et al., 2012). A recent report demonstrated that stabilization of HIF-1α enhanced the production of IL-10 and IL-22 from lamina propria CD4+ T-cells with reduction of inflammatory lesions in DSS-induced mice colitis (Kim et al., 2021). The Tregs without HIF-1α could not control T cell-mediated colitis (Clambey et al., 2012). Different from HIF-1α, overactivation of HIF-2α was shown to have deleterious role in the control of Tregs function (Yamamoto et al., 2019; Ajouaou et al., 2022). The findings are different from a publication by Hsu et al. (2020) in which deletion of HIF2α, but not HIF1α, was found to affect Tregs function negatively. Of note, concomitant deletion of both HIF-1α and HIF-2α restored the suppressive activity of Tregs (Hsu et al., 2020). These studies indicate that the role of HIF-2α is ambiguous. In B cells, HIF-1α regulates the expression of alkaline pH-activated two-pore domain K+ channel K2P5.1, which is required to affect B-cell proliferation, survival, or production of cytokines (Shin et al., 2014). HIF-1α acts as a transcription factor controlling the formation of IL-10 in B cells (Meng et al., 2018). Specific deletion of pVHL in B cells stabilized HIFα, leading to decreased proliferation and increased death of B cells, and impaired formation of high-affinity IgG (Cho et al., 2016).
Intestinal microenvironment acidosis also influences immune cells. Studies have shown that lactate acidosis induced by HIF-1α exerted immunomodulatory pleiotropic effects that modulate the inflammatory response (Manosalva et al., 2022). Long-term exposure to lactate results in strong anti-inflammatory effects in monocytes (Ratter et al., 2018). The anti-inflammatory effect by lactate was also observed in macrophage (Yang et al., 2020) and mast cells (Caslin et al., 2019). Lactate-driven macrophage polarization in the inflammatory microenvironment alleviates intestinal inflammation (Zhou et al., 2022). Past studies showed that lactate suppressed the innate immunity (Hoque et al., 2014) and lactate treatment protected mice against TNBS-induced colitis (Iraporda et al., 2016). Extracellular acidosis suppresses T cell-mediated immunity (Certo et al., 2021).
Studies have indicated that the three PHD family members have different roles in IBD (Watts and Walmsley, 2019; Dvornikova et al., 2023). In a DSS-induced colitis animal model, the intestinal inflammation was diminished in Phd1-deficient (Phd1_/_) but unaltered in Phd2-deficient (Phd2+/_) and Phd3-deficient (Phd3_/_) mice, suggesting that loss of Phd1, but not Phd2 or Phd3, is protective against DSS-induced colitis (Tambuwala et al., 2010; Kennel et al., 2022). We found that deletion of PHD2 in mice intestinal epithelial cells did not lead to spontaneous enteritis or colitis, nor did it confer upon mice higher susceptibility to DSS-induced colitis (Xie et al., 2018). While the mice with depletion of PHD3 in intestinal epithelial cells developed spontaneous colitis and were more sensitive to DSS treatment than the wild-type littermate controls, suggesting that PHD3 is protective against colitis (Chen et al., 2015). Interestingly, PHD3 was found to protect the intestinal epithelial barrier through stabilizing the TJ protein occludin (Chen et al., 2015) and the transcription factor ATOH1 (Xu et al., 2020), in a hydroxylase-independent manner. These results suggest that the PHD isoforms have different roles in IBD and they may function in different mechanisms.
Expression of PHD family members in inflamed intestinal tissues was determined. PHD1 levels were increased with disease severity in intestinal tissues from patients with IBD and in colonic tissues from mice with colitis (Tambuwala et al., 2010). Similarly, both mRNA and protein levels of PHD1 were found upregulated in inflamed biopsies from both UC and CD patients, while expression of PHD2 in colonic mucosa was not altered in IBD and expression of PHD3 was increased in inflamed biopsies from UC patients only at the mRNA level (Van Welden S, et al., 2013). These findings are consistent with the results that deletion of PHD1 is protective. A recent study demonstrated that PHD1 was downregulated in the mucosa in UC patients with active inflammatory disease, which might skew the hypoxic response toward enhanced protective HIF-1α stabilization in the inflamed mucosa of UC patients (Brown et al., 2020). In another study using the chemical-induced colitis mice model, expression of PHD1 and PHD2 was increased with the progression of the disease, while the expression of PHD3 remained unchanged (Bakshi et al., 2019). Examination of biopsies from UC patients indicated that PHD3 protein levels in inflamed mucosa were decreased with disease severity, which was consistent with the finding that PHD3 was protective against colitis in mice (Chen et al., 2015).
The possible roles of PHDs in other types of cell on IBD were also determined. Van Welden et al. (2017b) demonstrated that Phd1 deletion in endothelial and haematopoietic cells (Phd1f/fTie2:cre) protected mice from DSS-induced colitis, whereas the response of Phd2f/+Tie2:cre and Phd3f/fTie2:cre mice to DSS was similar to that of their littermate controls. While, in another study using a Crohn’s like ileitis mouse model, it was shown that haematopoietic Phd1-deletion did not impact experimental ileitis development (De Galan et al., 2021). It was demonstrated recently that mice lacking PHD2 expression in Tregs displayed a proinflammatory phenotype (Ajouaou et al., 2022). Deletion of PHD3 in neutrophils was found to be associated with reduced bowel inflammation in an acute mouse model of colitis (Walmsley et al., 2011). Together, these results indicate that the role of PHD isoforms may be cell-specific.
The protection of intestinal epithelial barrier by HIFα in IBD has initiated the study of PHDs-targeting as a strategy for IBD treatment. A few PHD inhibitors have been investigated in the treatment of gut inflammation in pre-clinical animal models (Table 1). In 2008, two studies demonstrated the protective effects of the PHD inhibitors dimethyloxalyl glycine (DMOG) (Cummins et al., 2008) and FG-4497 (Robinson et al., 2008) in experimental colitis of mice. Besides mice chemical colitis experiments, treatment with DMOG was also found beneficial in protection against bacterial toxin-, ischaemia and reperfusion-, and radiation-induced intestinal injury (Hirota et al., 2010; Hart et al., 2011; Taniguchi et al., 2014), and the protection was HIF-dependent. DMOG is an analogue of 2-OG and it blocks the entry of the co-substrate to the catalytic domain of PHDs, thus inhibiting PHDs’ enzymatic activity. FG-4497 blocks the active site of PHDs.
Gupta and co-workers (Gupta et al., 2014) demonstrated that, when orally administered, the PHD inhibitor TRC160334 had protective effects in TNBS and DSS mice colitis models. It was shown in Hep3B cells that TRC160334 had the ability to activate HIF-1α (Jamadarkhana et al., 2012). Jeong et al. (2015) reported that the iron chelator rosmarinic acid methyl ester inhibited PHD enzymatic activity and was able to ameliorate TNBS-induced colitis in rats, and the protection was associated with increased colonic HIF-1 activity. A recent study demonstrated that stabilization of HIF-1α by the PHD inhibitor CG-598 mitigated gut inflammation in DSS-induced colitis in mice (Kim et al., 2021).
AKB-4924 (also known as GB004), a predominant PHD inhibitor, was shown to protect against TNBS-induced mice colitis (Keely et al., 2014). This inhibitor did not have any protection of mice lacking HIF-1α in intestinal epithelial cells, indicating that epithelial HIF-1α is the target for AKB-4924-mediated protection. GB004 is an iron chelator and stabilizes HIF-1α by inhibiting PHD activity (Okumura et al., 2012). Oral administration of GB004 alleviated colonic inflammation with minor effects on protein levels of HIFα and expression of its target genes in extra intestinal organs, which limits the potential off-target effects (Marks et al., 2015). These results implicate that GB004 has preference for stabilization of HIF-1α within the gut and the intestinal epithelium is the central site of protection afforded by PHD inhibitor. HIF-1 stabilization by GB004 accelerated mice intestinal mucosal healing and reduced TNBS-induced colitis by inducing epithelial integrin expression (Goggins et al., 2021). GB004 exhibits protective effects directly on epithelial cells and drives protective effects on immune cells (Taylor et al., 2021). Administration of GB004 results in reduction in disease severity and improvements in histologic measures.
PHD inhibitors also play a role in alleviating intestinal fibrosis. It has been recently reported that PHD inhibition downregulates the expression of TGF-β1 in intestinal fibrosis (Manresa et al., 2016), indicating that the PHD inhibitors serve as anti-fibrotic agents in the treatment of IBD. Oral administration of betulinic acid hydroxamate (BAH), an inhibitor of PHDs, prevented TNBS- or DSS-induced mice colon inflammation and fibrosis (Prados et al., 2021). BAH-treated animals showed a significant reduction of fibrotic markers Tnc, Col1a2, Col3a1, Timp-1 and α-SMA and inflammatory markers F4/80+, CD3+, Il-1β and Ccl3 in colon tissue, as well as an improvement in epithelial barrier integrity and wound healing.
The aforementioned studies have demonstrated the therapeutic effects of PHD inhibitors in the treatment of animal gut inflammation. Notably, most of these studies showed the stabilization of HIF-1α and attributed the protection to the stabilization and activation of HIF-1. It should be noted that there is no conclusive demonstration that HIF-1α is the primary driver of the protective effects of these inhibitors. Inhibition of PHDs may also activate nuclear factor-kappa B (NF-κB) (Cummins et al., 2006; Welden et al., 2017). It was shown that ablation of NF-κB in epithelial cells resulted in serious chronic intestinal inflammation in mice (Nenci et al., 2007), indicating the requirement of NF-κB in the maintenance of the gut immune homeostasis. As NF-κB is a major regulator of immune and inflammatory processes (Nizet and Johnson, 2009; Capece et al., 2022), its activation induced by PHD inhibitors may also be of therapeutic benefit in IBD.
As GB004 has been shown beneficial in treatment of intestinal inflammation in animal models, it is being investigated as a potential treatment option for IBD patients. A phase IA study showed that no serious adverse events were observed when the healthy subjects received a single ascending dose of GB004, indicating a well tolerance (Levesque et al., 2019) (Table 2).
To determine the safety and pharmacokinetic profile, a multiple dose phase IA study was conducted in healthy people in Canada (Levesque et al., 2020). It is randomized, double-blinded and placebo-controlled. GB004 solution or placebo solution were orally administered at three doses once a day for 8 days. Forty-two people participated and there were no recorded serious adverse events. Following oral administration, the drug was absorbed and eliminated quickly from the systemic circulation. The influences of GB004 and placebo on levels of erythropoietin (EPO) and VEGF in plasma were similar with no dose-related effects, which highlights the predominant effect of GB004 on the intestine. The data indicated that GB004 at the doses tested did not influence the expression of EPO and VEGF in plasma. These results might be due to low accumulation of GB004 in plasma. The biopsie assay indicated that there was more GB004 in colon than in the plasma (Levesque et al., 2020). These results of the study suggest that GB004 at the doses administrated daily are safe and tolerable.
A first-in-patient, phase IB, double-blinded, placebo-controlled study was performed to evaluate the safety, tolerability and pharmacokinetics of GB004 (Danese et al., 2022). Thirty-four adult participants that had mild-to-moderate active UC were randomized to GB004 solution (120 mg) (n = 23) vs. placebo (n = 11) once daily for 28 days. After GB004 treatment, a greater proportion of the patients had reduced faecal calprotectin and mucosal healing. Formation of faecal calprotectin is induced in inflammation and its expression level correlates with disease activity and it is used as a clinical biomarker for mucosal inflammation (Jukic et al., 2021). GB004 was generally well tolerated when administered orally at 120 mg once daily for 28 days. There were no discernable difference between the treatment groups regarding systemic levels of EPO and VEGF. The concentrations of GB004 in colonic tissues on day 28 were greater than those in plasma (approximately 6 and 65 times higher than peak and average plasma concentrations). The substantially higher contents of GB004 in colon relative to those in plasma indicates a local gut effect of GB004, which may explain the absence of increased systemic levels of EPO and VEGF relative to placebo.
Hypoxia promotes glycolysis and thus excess lactate production, leading to acidification of the extracellular microenvironment, which may influence the therapeutic efficacy of drugs (Singh et al., 2021). This phase IB trial study showed the therapeutic benefits of GB004 in the treatment of UC, suggesting that this compound works in lactate acidosis.
Currently, a larger phase 2 SHIFT-UC study (NCT03860896) about GB004 on active UC is ongoing.
Several PHD inhibitors have been developed and their potential in treating IBD and other diseases such as anemia associated with chronic kidney disease (CKD) are under investigation (Welden et al., 2017; Semenza, 2019). Enhanced angiogenesis and increased expression of EPO were observed in conditional knockout of PHD2 (Takeda et al., 2006; Takeda et al., 2007; Katschinski, 2009). These findings and previous results that HIF-induced EPO production and concomitantly enhanced erythropoiesis (Semenza and Wang, 1992) imply that HIF activation by inhibiting PHDs is favorable to people with anemia and ischemia-related diseases. Pharmacological manipulation of PHD-HIF axis has been quested for treating disorders related to local and systemic hypoxia. PHD inhibitors were developed for the treatment of chronic kidney disease (CKD)-related anemia (Welden et al., 2017; Semenza, 2019; Wish et al., 2021; Macdougall, 2022). At least six PHD inhibitors roxadustat (FG-4592), daprodustat (GSK1278863), vadadustat (AKB-6548), molidustat (BAY 85-3934), enarodustat (JTZ-951) and desidustat have been developed and the phase 3 clinical trials showed that their effects were non-inferior to current EPO-stimulating agents (Sugahara et al., 2022). Roxadustat was first approved in China for treatment of CKD-related anemia patients receiving hemodialysis or peritoneal dialysis in 2018 (Dhillon, 2019) and for the treatment of CKD-related anemia patients not receiving dialysis in 2019 (Li et al., 2020). Roxadustat was then launched in other countries such as Japan, Chile, South Korea, the European Union, and the United Kingdom (Sugahara et al., 2022). On 01 Feb 2023, FDA approved daprodustat as the first oral treatment for anemia caused by CKD for adults who have been receiving dialysis for at least 4 months (https://www.fda.gov/).
Though no adverse effects were observed in studies of GB004 in IBD patients, there are not any long-term clinical data with GB004. The worry about the long-term use of PHD inhibitors are raised (Welden et al., 2017). It is well known that HIFα is highly expressed in cancer cells and its expression is positively linked with cancer aggressiveness and mortality (Schito and Semenza, 2016). Thus, the risk of HIF-activating therapies to promote tumor should be assessed. Activation of HIFα promotes EPO expression and subsequent erythrocyte formation. The agents that stimulate EPO expression are associated with an increased risk of thromboembolic diseases (Vittori et al., 2021; Semenza, 2022). The risk of fibrosis is another concern when using inhibitors of PHD. Intestinal fibrosis is a common complication of IBD (D'Alessio et al., 2022). More than 30% of IBD patients have intestinal fibrosis (Ramakrishnan and Shah, 2016). It was demonstrated that activation of HIF1α in epithelial cells promoted fibrogenesis in vivo. Higgins et al. (2007) provided clinical and genetic evidence that HIF-1 activation in renal epithelial cells might promote fibrogenesis through the induction of extracellular matrix-modifying factors and lysyl oxidase. The disturbance of biochemical processes by inhibition of PHDs is also a concern. Currently, some of the tested PHD inhibitors are analogues of 2-OG. In humans, there are many 2-OG-dependent dioxygenases and these enzymes hydroxylate proteins involved in many biological processes such as collagen and hormone synthesis and fatty acid metabolism (McDonough et al., 2010; Markolovic et al., 2015; Losman et al., 2020). The use of 2-OG analogues might potentially influence these reactions and result in side effects. Some PHD inhibitors are Fe2+ chelators and may inhibit other enzymes requiring Fe2+, which could also lead to unwanted adverse effects.
IBD is a chronic inflammatory disorder of intestine and is characterized by disrupted intestinal barrier and dysregulated immune. HIFα plays a critical role in protecting intestinal epithelial barrier and maintaining the healthy mucosal function. Many studies have demonstrated that stabilization of HIFα through inhibition of PHDs is protective in experimental colitis. Targeting PHD-HIF system to repair the disrupted intestinal barrier is becoming a novel strategy for therapy of IBD. A few PHD inhibitors have proven to be beneficial in several models of IBD and clinical trials are ongoing. As there is potential side effect for persistent activation of HIFs, systemic exposure to PHD inhibitors may cause adverse effect. Thus, a long-term follow-up is required to confirm the safety of the treatment with PHD inhibitors. The potential side effects of PHD inhibitors should be assessed before clinical use. Developing PHD inhibitors that are intestine preferential localization might be an approach to reduce the adverse effect. In summary, development of the inhibitors targeting PHDs may meet the unmet needs for IBD treatment and will have deep impact on medicine.
JL, HZ, JG, MY, and JF wrote the manuscript. JL and HZ prepared the figures and table. JG revised critically the manuscript. MY and JF obtained the funding.
This work was supported by the grants from Ministry of Science and Technology of China (2020YFA0803301), National Natural Science Foundation of China (82073061, 32000434), and Shandong Natural Science Foundation (ZR2020MH206, ZR2020QH193).
The authors apologize that, due to limited space, many excellent papers could not be cited.
The authors declare that the research was conducted in the absence of any commercial or financial relationships that could be construed as a potential conflict of interest.
All claims expressed in this article are solely those of the authors and do not necessarily represent those of their affiliated organizations, or those of the publisher, the editors and the reviewers. Any product that may be evaluated in this article, or claim that may be made by its manufacturer, is not guaranteed or endorsed by the publisher.
Ajouaou, Y., Azouz, A., Taquin, A., Denanglaire, S., Hussein, H., Krayem, M., et al. (2022). The oxygen sensor prolyl hydroxylase domain 2 regulates the in vivo suppressive capacity of regulatory T cells. Elife 11, e70555. doi:10.7554/eLife.70555
Allard, B., Longhi, M. S., Robson, S. C., and Stagg, J. (2017). The ectonucleotidases CD39 and CD73: Novel checkpoint inhibitor targets. Immunol. Rev. 276 (1), 121–144. doi:10.1111/imr.12528
Ananthakrishnan, A. N., Bernstein, C. N., Iliopoulos, D., Macpherson, A., Neurath, M. F., Ali, R. A. R., et al. (2018). Environmental triggers in IBD: A review of progress and evidence. Nat. Rev. Gastroenterol. Hepatol. 15 (1), 39–49. doi:10.1038/nrgastro.2017.136
Appelhoff, R. J., Tian, Y. M., Raval, R. R., Turley, H., Harris, A. L., Pugh, C. W., et al. (2004). Differential function of the prolyl hydroxylases PHD1, PHD2, and PHD3 in the regulation of hypoxia-inducible factor. J. Biol. Chem. 279 (37), 38458–38465. doi:10.1074/jbc.M406026200
Bakshi, H. A., Mishra, V., Satija, S., Mehta, M., Hakkim, F. L., Kesharwani, P., et al. (2019). Dynamics of prolyl hydroxylases levels during disease progression in experimental colitis. Inflammation 42 (6), 2032–2036. doi:10.1007/s10753-019-01065-3
Bartels, K., Grenz, A., and Eltzschig, H. K. (2013). Hypoxia and inflammation are two sides of the same coin. Proc. Natl. Acad. Sci. U. S. A. 110 (46), 18351–18352. doi:10.1073/pnas.1318345110
Berra, E., Benizri, E., Ginouves, A., Ginouvès, A., Volmat, V., Roux, D., et al. (2003). HIF prolyl-hydroxylase 2 is the key oxygen sensor setting low steady-state levels of HIF-1alpha in normoxia. EMBO J. 22 (16), 4082–4090. doi:10.1093/emboj/cdg392
Biddlestone, J., Bandarra, D., and Rocha, S. (2015). The role of hypoxia in inflammatory disease (review). Int. J. Mol. Med. 35 (4), 859–869. doi:10.3892/ijmm.2015.2079
Brown, E., Rowan, C., Strowitzki, M. J., Fagundes, R. R., Faber, K. N., Güntsch, A., et al. (2020). Mucosal inflammation downregulates PHD1 expression promoting a barrier-protective HIF-1α response in ulcerative colitis patients. FASEB J. 34 (3), 3732–3742. doi:10.1096/fj.201902103R
Brown, E., and Taylor, C. T. (2018). Hypoxia-sensitive pathways in intestinal inflammation. J. Physiol. 596 (15), 2985–2989. doi:10.1113/jp274350
Campbell, E. L., Bruyninckx, W. J., Kelly, C. J., Glover, L. E., McNamee, E. N., Bowers, B. E., et al. (2014). Transmigrating neutrophils shape the mucosal microenvironment through localized oxygen depletion to influence resolution of inflammation. Immunity 40 (1), 66–77. doi:10.1016/j.immuni.2013.11.020
Capece, D., Verzella, D., Flati, I., Arboretto, P., Cornice, J., and Franzoso, G. (2022). NF-κB: Blending metabolism, immunity, and inflammation. Trends Immunol. 43 (9), 757–775. doi:10.1016/j.it.2022.07.004
Caslin, H. L., Abebayehu, D., AbdulQayum, A., Haque, T. T., Taruselli, M. T., Paez, P. A., et al. (2019). Lactic acid inhibits lipopolysaccharide-induced mast cell function by limiting glycolysis and ATP availability. J. Immunol. 203, 453–464. doi:10.4049/jimmunol.1801005
Certo, M., Tsai, C. H., Pucino, V., Ho, P. C., and Mauro, C. (2021). Lactate modulation of immune responses in inflammatory versus tumour microenvironments. Nat. Rev. Immunol. 21 (3), 151–161. doi:10.1038/s41577-020-0406-2
Chen, Y., Zhang, H. S., Fong, G. H., Xi, Q. L., Wu, G. H., Bai, C. G., et al. (2015). PHD3 stabilizes the tight junction protein occludin and protects intestinal epithelial barrier function. J. Biol. Chem. 290 (33), 20580–20589. doi:10.1074/jbc.M115.653584
Cho, S. H., Raybuck, A. L., Stengel, K., Wei, M., Beck, T. C., Volanakis, E., et al. (2016). Germinal centre hypoxia and regulation of antibody qualities by a hypoxia response system. Nature 537 (7619), 234–238. doi:10.1038/nature19334
Chowdhury, R., Hardy, A., and Schofield, C. J. (2008). The human oxygen sensing machinery and its manipulation. Chem. Soc. Rev. 37 (7), 1308–1319. doi:10.1039/b701676j
Clambey, E. T., McNamee, E. N., Westrich, J. A., Glover, L. E., Campbell, E. L., Jedlicka, P., et al. (2012). Hypoxia-inducible factor-1 alpha-dependent induction of FoxP3 drives regulatory T-cell abundance and function during inflammatory hypoxia of the mucosa. Proc. Natl. Acad. Sci. U. S. A. 109 (41), E2784–E2793. doi:10.1073/pnas.1202366109
Colgan, S. P., and Taylor, C. T. (2010). Hypoxia: An alarm signal during intestinal inflammation. Nat. Rev. Gastroenterol. Hepatol. 7 (5), 281–287. doi:10.1038/nrgastro.2010.39
Cramer, T., Yamanishi, Y., Clausen, B. E., Förster, I., Pawlinski, R., Mackman, N., et al. (2003). HIF-1alpha is essential for myeloid cell-mediated inflammation. Cell 112 (5), 645–657. doi:10.1016/s0092-8674(03)00154-5
Cummins, E. P., Berra, E., Comerford, K. M., Ginouves, A., Fitzgerald, K. T., Seeballuck, F., et al. (2006). Prolyl hydroxylase-1 negatively regulates IkappaB kinase-beta, giving insight into hypoxia-induced NF-kappaB activity. Proc. Natl. Acad. Sci. U. S. A. 103 (48), 18154–18159. doi:10.1073/pnas.0602235103
Cummins, E. P., Seeballuck, F., Keely, S. J., Mangan, N. E., Callanan, J. J., Fallon, P. G., et al. (2008). The hydroxylase inhibitor dimethyloxalylglycine is protective in a murine model of colitis. Gastroenterology 134 (1), 156–165. doi:10.1053/j.gastro.2007.10.012
D'Alessio, S., Ungaro, F., Noviello, D., Lovisa, S., Peyrin-Biroulet, L., and Danese, S. (2022). Revisiting fibrosis in inflammatory bowel disease: The gut thickens. Nat. Rev. Gastroenterol. Hepatol. 19 (3), 169–184. doi:10.1038/s41575-021-00543-0
Danese, S., Levesque, B. G., Feagan, B. G., Jucov, A., Bhandari, B. R., Pai, R. K., et al. (2022). Randomised clinical trial: A phase 1b study of GB004, an oral HIF-1α stabiliser, for treatment of ulcerative colitis. Aliment. Pharmacol. Ther. 55 (4), 401–411. doi:10.1111/apt.16753
De Galan, C., De Vos, M., Hindryckx, P., Laukens, D., and Van Welden, S. (2021). Long-term environmental hypoxia exposure and haematopoietic prolyl hydroxylase-1 deletion do not impact experimental Crohn's like ileitis. Biol. (Basel) 10 (9), 887. doi:10.3390/biology10090887
Dhillon, S. (2019). Roxadustat: First global approval. Drugs 79 (5), 563–572. doi:10.1007/s40265-019-01077-1
Dvornikova, K. A., Platonova, O. N., and Bystrova, E. Y. (2023). Hypoxia and intestinal inflammation: Common molecular mechanisms and signaling pathways. Int. J. Mol. Sci. 24 (3), 2425. doi:10.3390/ijms24032425
Ehrismann, D., Flashman, E., Genn, D. N., Mathioudakis, N., Hewitson, K. S., Ratcliffe, P. J., et al. (2007). Studies on the activity of the hypoxia-inducible-factor hydroxylases using an oxygen consumption assay. Biochem. J. 401 (1), 227–234. doi:10.1042/bj20061151
Eltzschig, H. K., Bratton, D. L., and Colgan, S. P. (2014). Targeting hypoxia signalling for the treatment of ischaemic and inflammatory diseases. Nat. Rev. Drug Discov. 13 (11), 852–869. doi:10.1038/nrd4422
Eltzschig, H. K., and Carmeliet, P. (2011). Hypoxia and inflammation. N. Engl. J. Med. 364 (7), 656–665. doi:10.1056/NEJMra0910283
Eltzschig, H. K., Ibla, J. C., Furuta, G. T., Leonard, M. O., Jacobson, K. A., Enjyoji, K., et al. (2003). Coordinated adenine nucleotide phosphohydrolysis and nucleoside signaling in posthypoxic endothelium: Role of ectonucleotidases and adenosine A2B receptors. J. Exp. Med. 198 (5), 783–796. doi:10.1084/jem.20030891
Epstein, A. C., Gleadle, J. M., McNeill, L. A., Hewitson, K. S., O'Rourke, J., Mole, D. R., et al. (2001). C. elegans EGL-9 and mammalian homologs define a family of dioxygenases that regulate HIF by prolyl hydroxylation. Cell 107 (1), 43–54. doi:10.1016/s0092-8674(01)00507-4
Fang, H. Y., Hughes, R., Murdoch, C., Coffelt, S. B., Biswas, S. K., Harris, A. L., et al. (2009). Hypoxia-inducible factors 1 and 2 are important transcriptional effectors in primary macrophages experiencing hypoxia. Blood 114:844–859. doi:10.1182/blood-2008-12-195941
Fisher, E. M., Khan, M., Salisbury, R., and Kuppusamy, P. (2013). Noninvasive monitoring of small intestinal oxygen in a rat model of chronic mesenteric ischemia. Cell Biochem. Biophys. 67 (2), 451–459. doi:10.1007/s12013-013-9611-y
Flück, K., Breves, G., Fandrey, J., and Winning, S. (2016). Hypoxia-inducible factor 1 in dendritic cells is crucial for the activation of protective regulatory T cells in murine colitis. Mucosal Immunol. 9 (2), 379–390. doi:10.1038/mi.2015.67
Fraisl, P., Aragonés, J., and Carmeliet, P. (2009). Inhibition of oxygen sensors as a therapeutic strategy for ischaemic and inflammatory disease. Nat. Rev. Drug Discov. 8 (2), 139–152. doi:10.1038/nrd2761
Furuta, G. T., Turner, J. R., Taylor, C. T., Hershberg, R. M., Comerford, K., Narravula, S., et al. (2001). Hypoxia-inducible factor 1-dependent induction of intestinal trefoil factor protects barrier function during hypoxia. J. Exp. Med. 193 (9), 1027–1034. doi:10.1084/jem.193.9.1027
Giatromanolaki, A., Sivridis, E., Maltezos, E., Papazoglou, D., Simopoulos, C., Gatter, K. C., et al. (2003). Hypoxia inducible factor 1alpha and 2alpha overexpression in inflammatory bowel disease. J. Clin. Pathol. 56 (3), 209–213. doi:10.1136/jcp.56.3.209
Glover, L. E., Bowers, B. E., Saeedi, B., Ehrentraut, S. F., Campbell, E. L., Bayless, A. J., et al. (2013). Control of creatine metabolism by HIF is an endogenous mechanism of barrier regulation in colitis. Proc. Natl. Acad. Sci. U. S. A. 110 (49), 19820–19825. doi:10.1073/pnas.1302840110
Goggins, B. J., Minahan, K., Sherwin, S., Soh, W. S., Pryor, J., Bruce, J., et al. (2021). Pharmacological HIF-1 stabilization promotes intestinal epithelial healing through regulation of α-integrin expression and function. Am. J. Physiol. Gastrointest. Liver Physiol. 320 (4), G420–G438. doi:10.1152/ajpgi.00192.2020
Gupta, R., Chaudhary, A. R., Shah, B. N., Jadhav, A. V., Zambad, S. P., Gupta, R. C., et al. (2014). Therapeutic treatment with a novel hypoxia-inducible factor hydroxylase inhibitor (TRC160334) ameliorates murine colitis. Clin. Exp. Gastroenterol. 7, 13–23. doi:10.2147/ceg.S51923
Hansson, G. C. (2020). Mucins and the microbiome. Annu. Rev. Biochem. 89, 769–793. doi:10.1146/annurev-biochem-011520-105053
Hart, M. L., Grenz, A., Gorzolla, I. C., Schittenhelm, J., Dalton, J. H., and Eltzschig, H. K. (2011). Hypoxia-inducible factor-1α-dependent protection from intestinal ischemia/reperfusion injury involves ecto-5'-nucleotidase (CD73) and the A2B adenosine receptor. J. Immunol. 186 (7), 4367–4374. doi:10.4049/jimmunol.0903617
Hatoum, O. A., Miura, H., and Binion, D. G. (2003). The vascular contribution in the pathogenesis of inflammatory bowel disease. Am. J. Physiol. Heart Circ. Physiol. 285 (5), H1791–H1796. doi:10.1152/ajpheart.00552.2003
He, G., Shankar, R. A., Chzhan, M., Samouilov, A., Kuppusamy, P., and Zweier, J. L. (1999). Noninvasive measurement of anatomic structure and intraluminal oxygenation in the gastrointestinal tract of living mice with spatial and spectral EPR imaging. Proc. Natl. Acad. Sci. U. S. A. 96 (8), 4586–4591. doi:10.1073/pnas.96.8.4586
Higashiyama, M., Hokari, R., Hozumi, H., Kurihara, C., Ueda, T., Watanabe, C., et al. (2012). HIF-1 in T cells ameliorated dextran sodium sulfate-induced murine colitis. J. Leukoc. Biol. 91 (6), 901–909. doi:10.1189/jlb.1011518
Higgins, D. F., Kimura, K., Bernhardt, W. M., Shrimanker, N., Akai, Y., Hohenstein, B., et al. (2007). Hypoxia promotes fibrogenesis in vivo via HIF-1 stimulation of epithelial-to-mesenchymal transition. J. Clin. Invest. 117 (12), 3810–3820. doi:10.1172/jci30487
Hindryckx, P., De Vos, M., Jacques, P., Ferdinande, L., Peeters, H., Olievier, K., et al. (2010). Hydroxylase inhibition abrogates TNF-alpha-induced intestinal epithelial damage by hypoxia-inducible factor-1-dependent repression of FADD. J. Immunol. 185 (10), 6306–6316. doi:10.4049/jimmunol.1002541
Hirota, S. A., Fines, K., Ng, J., Traboulsi, D., Lee, J., Ihara, E., et al. (2010). Hypoxia-inducible factor signaling provides protection in Clostridium difficile-induced intestinal injury. Gastroenterology 139 (1), 259–269. doi:10.1053/j.gastro.2010.03.045
Hoque, R., Farooq, A., Ghani, A., Gorelick, F., and Mehal, W. Z. (2014). Lactate reduces liver and pancreatic injury in Toll-like receptor- and inflammasome-mediated inflammation via GPR81-mediated suppression of innate immunity. Gastroenterology 146 (7), 1763–1774. doi:10.1053/j.gastro.2014.03.014
Hsu, T. S., Lin, Y. L., Wang, Y. A., Mo, S. T., Chi, P. Y., Lai, A. C. Y., et al. (2020). HIF-2 is indispensable for regulatory T cell function. Nat. Commun. 11, 5005–5016. doi:10.1038/s41467-020-18731-y
Iraporda, C., Romanin, D. E., Bengoa, A. A., Errea, A. J., Cayet, D., Foligne, B., et al. (2016). Local treatment with lactate prevents intestinal inflammation in the TNBS-induced colitis model. Front. Immunol. 7, 651. doi:10.3389/fimmu.2016.00651
Ivan, M., Kondo, K., Yang, H., Kim, W., Valiando, J., Ohh, M., et al. (2001). HIFalpha targeted for VHL-mediated destruction by proline hydroxylation: Implications for O2 sensing. Science 292 (5516), 464–468. doi:10.1126/science.1059817
Jamadarkhana, P., Chaudhary, A., Chhipa, L., Dubey, A., Mohanan, A., Gupta, R., et al. (2012). Treatment with a novel hypoxia-inducible factor hydroxylase inhibitor (TRC160334) ameliorates ischemic acute kidney injury. Am. J. Nephrol. 36 (3), 208–218. doi:10.1159/000341870
Jeong, S., Park, H., Hong, S., Yum, S., Kim, W., and Jung, Y. (2015). Lipophilic modification enhances anti-colitic properties of rosmarinic acid by potentiating its HIF-prolyl hydroxylases inhibitory activity. Eur. J. Pharmacol. 747, 114–122. doi:10.1016/j.ejphar.2014.11.030
Jukic, A., Bakiri, L., Wagner, E. F., Tilg, H., and Adolph, T. E. (2021). Calprotectin: From biomarker to biological function. Gut 70 (10), 1978–1988. doi:10.1136/gutjnl-2021-324855
Kaelin, W. G., and Ratcliffe, P. J. (2008). Oxygen sensing by metazoans: The central role of the HIF hydroxylase pathway. Mol. Cell 30 (4), 393–402. doi:10.1016/j.molcel.2008.04.009
Karhausen, J., Furuta, G. T., Tomaszewski, J. E., Johnson, R. S., Colgan, S. P., and Haase, V. H. (2004). Epithelial hypoxia-inducible factor-1 is protective in murine experimental colitis. J. Clin. Invest. 114 (8), 1098–1106. doi:10.1172/jci21086
Kaser, A., Zeissig, S., and Blumberg, R. S. (2010). Inflammatory bowel disease. Annu. Rev. Immunol. 28, 573–621. doi:10.1146/annurev-immunol-030409-101225
Katschinski, D. M. (2009). In vivo functions of the prolyl-4-hydroxylase domain oxygen sensors: Direct route to the treatment of anaemia and the protection of ischaemic tissues. Acta Physiol. (Oxf) 195 (4), 407–414. doi:10.1111/j.1748-1716.2008.01952.x
Keely, S., Campbell, E. L., Baird, A. W., Hansbro, P. M., Shalwitz, R. A., Kotsakis, A., et al. (2014). Contribution of epithelial innate immunity to systemic protection afforded by prolyl hydroxylase inhibition in murine colitis. Mucosal Immunol. 7 (1), 114–123. doi:10.1038/mi.2013.29
Keely, S., Glover, L. E., MacManus, C. F., Campbell, E. L., Scully, M. M., Furuta, G. T., et al. (2009). Selective induction of integrin beta1 by hypoxia-inducible factor: Implications for wound healing. FASEB J. 23 (5), 1338–1346. doi:10.1096/fj.08-125344
Kelly, C. J., Glover, L. E., Campbell, E. L., Kominsky, D. J., Ehrentraut, S. F., Bowers, B. E., et al. (2013). Fundamental role for HIF-1α in constitutive expression of human β defensin-1. Mucosal Immunol. 6 (6), 1110–1118. doi:10.1038/mi.2013.6
Kennel, K. B., Burmeister, J., Radhakrishnan, P., Giese, N. A., Giese, T., Salfenmoser, M., et al. (2022). The HIF-prolyl hydroxylases have distinct and nonredundant roles in colitis-associated cancer. JCI Insight 7 (22), e153337. doi:10.1172/jci.insight.153337
Kierans, S. J., and Taylor, C. T. (2021). Regulation of glycolysis by the hypoxia-inducible factor (HIF): Implications for cellular physiology. J. Physiol. 599 (1), 23–37. doi:10.1113/JP280572
Kim, J. W., Tchernyshyov, I., Semenza, G. L., and Dang, C. V. (2006). HIF-1-mediated expression of pyruvate dehydrogenase kinase: A metabolic switch required for cellular adaptation to hypoxia. Cell Metab. 3 (3), 177–185. doi:10.1016/j.cmet.2006.02.002
Kim, Y. I., Yi, E. J., Kim, Y. D., Lee, A. R., Chung, J., Ha, H. C., et al. (2021). Local stabilization of hypoxia-inducible factor-1α controls intestinal inflammation via enhanced gut barrier function and immune regulation. Front. Immunol. 11, 609689. doi:10.3389/fimmu.2020.609689
Köhler, T., Reizis, B., Johnson, R. S., Weighardt, H., and Förster, I. (2012). Influence of hypoxia-inducible factor 1α on dendritic cell differentiation and migration. Eur. J. Immunol. 42 (5), 1226–1236. doi:10.1002/eji.201142053
Koivunen, P., Hirsilä, M., Kivirikko, K. I., and Myllyharju, J. (2006). The length of peptide substrates has a marked effect on hydroxylation by the hypoxia-inducible factor prolyl 4-hydroxylases. J. Biol. Chem. 281 (39), 28712–28720. doi:10.1074/jbc.M604628200
Levesque, B., Flynn, M., Peters, K., and Buch, A. J. (2019). P051 phase 1a safety and pharmacokinetic effects of GB004, a novel prolyl hydroxylase inhibitor and potential therapy for inflammatory bowel disease. Am. J. Gastroenterol. 114, S13. doi:10.14309/01.ajg.0000613172.41188.d4
Levesque, B., Meadows, K. T., Buch, A., Flynn, M., Opiteck, G. J. I. B. D., Olson, A., et al. (2020). GB004, a novel gut-targeted prolyl hydroxylase inhibitor for inflammatory bowel disease: First in-human multiple dose study in healthy subjects with gut biopsies. Inflamm. Bowel Dis. 26, S9–S10. doi:10.1093/ibd/zaa010.023
Li, Z., Tu, Y., and Liu, B. (2020). Treatment of renal anemia with roxadustat: Advantages and achievement. Kidney Dis. 6 (2), 65–73. doi:10.1159/000504850
Loftus, E. V. (2004). Clinical epidemiology of inflammatory bowel disease: Incidence, prevalence, and environmental influences. Gastroenterology 126 (6), 1504–1517. doi:10.1053/j.gastro.2004.01.063
Losman, J. A., Koivunen, P., and Kaelin, W. G. (2020). 2-Oxoglutarate-dependent dioxygenases in cancer. Nat. Rev. Cancer 20 (12), 710–726. doi:10.1038/s41568-020-00303-3
Louis, N. A., Hamilton, K. E., Canny, G., Shekels, L. L., Ho, S. B., and Colgan, S. P. (2006). Selective induction of mucin-3 by hypoxia in intestinal epithelia. J. Cell Biochem. 99 (6), 1616–1627. doi:10.1002/jcb.20947
Louis, N. A., Hamilton, K. E., Kong, T., and Colgan, S. P. (2005). HIF-dependent induction of apical CD55 coordinates epithelial clearance of neutrophils. FASEB J. 19 (8), 950–959. doi:10.1096/fj.04-3251com
Macdougall, I. C. (2022). Hypoxia-inducible factor prolyl hydroxylase enzyme inhibitors: Ready for primetime? Curr. Opin. Nephrol. Hypertens. 31 (5), 399–405. doi:10.1097/mnh.0000000000000813
Manosalva, C., Quiroga, J., Hidalgo, A. I., Alarcón, P., Anseoleaga, N., Angélica Hidalgo, M., et al. (2022). Role of lactate in inflammatory processes: Friend or foe. Front. Immunol. 12, 808799. doi:10.3389/fimmu.2021.808799
Manresa, M. C., Tambuwala, M. M., Radhakrishnan, P., Harnoss, J. M., Brown, E., Cavadas, M. A., et al. (2016). Hydroxylase inhibition regulates inflammation-induced intestinal fibrosis through the suppression of ERK-mediated TGF-β1 signaling. [corrected]. Am. J. Physiol. Gastrointest. Liver Physiol. 311, G1076–G1090. doi:10.1152/ajpgi.00229.2016
Manresa, M. C., and Taylor, C. T. (2017). Hypoxia inducible factor (HIF) hydroxylases as regulators of intestinal epithelial barrier function. Cell Mol. Gastroenterol. Hepatol. 3 (3), 303–315. doi:10.1016/j.jcmgh.2017.02.004
Markolovic, S., Wilkins, S. E., and Schofield, C. J. (2015). Protein hydroxylation catalyzed by 2-Oxoglutarate-dependent oxygenases. J. Biol. Chem. 290 (34), 20712–20722. doi:10.1074/jbc.R115.662627
Marks, E., Goggins, B. J., Cardona, J., Cole, S., Minahan, K., Mateer, S., et al. (2015). Oral delivery of prolyl hydroxylase inhibitor: AKB-4924 promotes localized mucosal healing in a mouse model of colitis. Inflamm. Bowel Dis. 21 (2), 267–275. doi:10.1097/mib.0000000000000277
Marxsen, J. H., Stengel, P., Doege, K., Heikkinen, P., Jokilehto, T., Wagner, T., et al. (2004). Hypoxia-inducible factor-1 (HIF-1) promotes its degradation by induction of HIF-alpha-prolyl-4-hydroxylases. Biochem. J. 381 (3), 761–767. doi:10.1042/BJ20040620
Maxwell, P. H., Wiesener, M. S., Chang, G. W., Clifford, S. C., Vaux, E. C., Cockman, M. E., et al. (1999). The tumour suppressor protein VHL targets hypoxia-inducible factors for oxygen-dependent proteolysis. Nature 399 (6733), 271–275. doi:10.1038/20459
McDonough, M. A., Loenarz, C., Chowdhury, R., Clifton, I. J., and Schofield, C. J. (2010). Structural studies on human 2-oxoglutarate dependent oxygenases. Curr. Opin. Struct. Biol. 20 (6), 659–672. doi:10.1016/j.sbi.2010.08.006
Meng, X., Grötsch, B., Luo, Y., Knaup, K. X., Wiesener, M. S., Chen, X. X., et al. (2018). Hypoxia inducible factor-1 is a critical transcription factor for IL-10-producing B cells in autoimmune disease. Nat. Commun. 9, 251. doi:10.1038/s41467-017-02683-x
Metzen, E., Berchner-Pfannschmidt, U., Stengel, P., Marxsen, J. H., Stolze, I., Klinger, M., et al. (2003). Intracellular localisation of human HIF-1 alpha hydroxylases: Implications for oxygen sensing. J. Cell Sci. 116 (7), 1319–1326. doi:10.1242/jcs.00318
Metzen, E., Stiehl, D. P., Doege, K., Marxsen, J. H., Hellwig-Bürgel, T., and Jelkmann, W. (2005). Regulation of the prolyl hydroxylase domain protein 2 (phd2/egln-1) gene: Identification of a functional hypoxia-responsive element. Biochem. J. 387 (3), 711–717. doi:10.1042/bj20041736
Minamishima, Y. A., Moslehi, J., Bardeesy, N., Cullen, D., Bronson, R. T., and Kaelin, W. G. (2008). Somatic inactivation of the PHD2 prolyl hydroxylase causes polycythemia and congestive heart failure. Blood 111 (6), 3236–3244. doi:10.1182/blood-2007-10-117812
Naldini, A., Morena, E., Pucci, A., Miglietta, D., Riboldi, E., Sozzani, S., et al. (2012). Hypoxia affects dendritic cell survival: Role of the hypoxia-inducible factor-1α and lipopolysaccharide. J. Cell Physiol. 227 (2), 587–595. doi:10.1002/jcp.22761
Nenci, A., Becker, C., Wullaert, A., Gareus, R., van Loo, G., Danese, S., et al. (2007). Epithelial NEMO links innate immunity to chronic intestinal inflammation. Nature 446 (7135), 557–561. doi:10.1038/nature05698
Ngo, O., Ramalingam, P., Phillips, J. A., and Furuta, G. T. (2006). Collagen gel contraction assay. Methods Mol. Biol. 341, 103–109. doi:10.1385/1-59745-113-4:103
Nizet, V., and Johnson, R. S. (2009). Interdependence of hypoxic and innate immune responses. Nat. Rev. Immunol. 9 (9), 609–617. doi:10.1038/nri2607
Okumura, C. Y., Hollands, A., Tran, D. N., Olson, J., Dahesh, S., von Köckritz-Blickwede, M., et al. (2012). A new pharmacological agent (AKB-4924) stabilizes hypoxia inducible factor-1 (HIF-1) and increases skin innate defenses against bacterial infection. J. Mol. Med. Berl. 90 (9), 1079–1089. doi:10.1007/s00109-012-0882-3
Ortiz-Barahona, A., Villar, D., Pescador, N., Amigo, J., and del Peso, L. (2010). Genome-wide identification of hypoxia-inducible factor binding sites and target genes by a probabilistic model integrating transcription-profiling data and in silico binding site prediction. Nucleic Acids Res. 38 (7), 2332–2345. doi:10.1093/nar/gkp1205
Papandreou, I., Cairns, R. A., Fontana, L., Lim, A. L., and Denko, N. C. (2006). HIF-1 mediates adaptation to hypoxia by actively downregulating mitochondrial oxygen consumption. Cell Metab. 3 (3), 187–197. doi:10.1016/j.cmet.2006.01.012
Pavlova, N. N., Zhu, J., and Thompson, C. B. (2022). The hallmarks of cancer metabolism: Still emerging. Cell Metab. 34 (3), 355–377. doi:10.1016/j.cmet.2022.01.007
Pescador, N., Cuevas, Y., Naranjo, S., Alcaide, M., Villar, D., Landázuri, M. O., et al. (2005). Identification of a functional hypoxia-responsive element that regulates the expression of the egl nine homologue 3 (egln3/phd3) gene. Biochem. J. 390 (1), 189–197. doi:10.1042/bj20042121
Prados, M. E., García-Martín, A., Unciti-Broceta, J. D., Palomares, B., Collado, J. A., Minassi, A., et al. (2021). Betulinic acid hydroxamate prevents colonic inflammation and fibrosis in murine models of inflammatory bowel disease. Acta Pharmacol. Sin. 42 (7), 1124–1138. doi:10.1038/s41401-020-0497-0
Ramakrishnan, S. K., and Shah, Y. M. (2016). Role of intestinal HIF-2α in Health and disease. Annu. Rev. Physiol. 78, 301–325. doi:10.1146/annurev-physiol-021115-105202
Ratter, J. M., Rooijackers, H. M. M., Hooiveld, G. J., Hijmans, A. G. M., de Galan, B. E., Tack, C. J., et al. (2018). In vitro and in vivo effects of lactate on metabolism and cytokine production of human primary PBMCs and monocytes. Front. Immunol. 9, 2564. doi:10.3389/fimmu.2018.02564
Robinson, A., Keely, S., Karhausen, J., Gerich, M. E., Furuta, G. T., and Colgan, S. P. (2008). Mucosal protection by hypoxia-inducible factor prolyl hydroxylase inhibition. Gastroenterology 134 (1), 145–155. doi:10.1053/j.gastro.2007.09.033
Rosenberger, P., Schwab, J. M., Mirakaj, V., Masekowsky, E., Mager, A., Morote-Garcia, J. C., et al. (2009). Hypoxia-inducible factor-dependent induction of netrin-1 dampens inflammation caused by hypoxia. Nat. Immunol. 10 (2), 195–202. doi:10.1038/ni.1683
Saeedi, B. J., Kao, D. J., Kitzenberg, D. A., Dobrinskikh, E., Schwisow, K. D., Masterson, J. C., et al. (2015). HIF-dependent regulation of claudin-1 is central to intestinal epithelial tight junction integrity. Mol. Biol. Cell 26 (12), 2252–2262. doi:10.1091/mbc.E14-07-1194
Schito, L., and Semenza, G. L. (2016). Hypoxia-inducible factors: Master regulators of cancer progression. Trends Cancer 2 (12), 758–770. doi:10.1016/j.trecan.2016.10.016
Schofield, C. J., and Ratcliffe, P. J. (2004). Oxygen sensing by HIF hydroxylases. Nat. Rev. Mol. Cell Biol. 5 (5), 343–354. doi:10.1038/nrm1366
Seagroves, T. N., Ryan, H. E., Lu, H., Wouters, B. G., Knapp, M., Thibault, P., et al. (2001). Transcription factor HIF-1 is a necessary mediator of the pasteur effect in mammalian cells. Mol. Cell Biol. 21 (10), 3436–3444. doi:10.1128/MCB.21.10.3436-3444.2001
Semenza, G. L. (2019). Pharmacologic targeting of hypoxia-inducible factors. Annu. Rev. Pharmacol. Toxicol. 59, 379–403. doi:10.1146/annurev-pharmtox-010818-021637
Semenza, G. L. (2022). Regulation of erythropoiesis by the hypoxia-inducible factor pathway: Effects of genetic and pharmacological perturbations. Annu. Rev. Med. 74, 307–319. doi:10.1146/annurev-med-042921-102602
Semenza, G. L., and Wang, G. L. (1992). A nuclear factor induced by hypoxia via de novo protein synthesis binds to the human erythropoietin gene enhancer at a site required for transcriptional activation. Mol. Cell Biol. 12 (12), 5447–5454. doi:10.1128/mcb.12.12.5447
Shin, D. H., Lin, H., Zheng, H., Kim, K. S., Kim, J. Y., Chun, Y. S., et al. (2014). HIF-1α-mediated upregulation of TASK-2 K⁺ channels augments Ca²⁺ signaling in mouse B cells under hypoxia. J. Immunol. 193, 4924–4933. doi:10.4049/jimmunol.1301829
Singh, S. V., Chaube, B., Mayengbam, S,S., Singh, A., Malvi, P., Mohammad, N., et al. (2021). Metformin induced lactic acidosis impaired response of cancer cells towards paclitaxel and doxorubicin: Role of monocarboxylate transporter. Biochim. Biophys. Acta Mol. Basis Dis. 1867 (3), 166011. doi:10.1016/j.bbadis.2020.166011
Solanki, S., Devenport, S. N., Ramakrishnan, S. K., and Shah, Y. M. (2019). Temporal induction of intestinal epithelial hypoxia-inducible factor-2α is sufficient to drive colitis. Am. J. Physiol. Gastrointest. Liver Physiol. 317 (2), G98–G107. doi:10.1152/ajpgi.00081.2019
Stiehl, D. P., Wirthner, R., Köditz, J., Spielmann, P., Camenisch, G., and Wenger, R. H. (2006). Increased prolyl 4-hydroxylase domain proteins compensate for decreased oxygen levels. Evidence for an autoregulatory oxygen-sensing system. J. Biol. Chem. 281 (33), 23482–23491. doi:10.1074/jbc.M601719200
Sugahara, M., Tanaka, T., and Nangaku, M. (2022). Future perspectives of anemia management in chronic kidney disease using hypoxia-inducible factor-prolyl hydroxylase inhibitors. Pharmacol. Ther. 239, 108272. doi:10.1016/j.pharmthera.2022.108272
Sun, S., Xu, X., Liang, L., Wang, X., Bai, X., Zhu, L., et al. (2021). Lactic acid-producing probiotic Saccharomyces cerevisiae attenuates ulcerative colitis via suppressing macrophage pyroptosis and modulating gut microbiota. Front. Immunol. 12, 777665. doi:10.3389/fimmu.2021.777665
Synnestvedt, K., Furuta, G. T., Comerford, K. M., Louis, N., Karhausen, J., Eltzschig, H. K., et al. (2002). Ecto-5'-nucleotidase (CD73) regulation by hypoxia-inducible factor-1 mediates permeability changes in intestinal epithelia. J. Clin. Invest. 110 (7), 993–1002. doi:10.1172/jci15337
Takeda, K., Aguila, H. L., Parikh, N. S., Li, X., Lamothe, K., Du, L. J., et al. (2008). Regulation of adult erythropoiesis by prolyl hydroxylase domain proteins. Blood 111 (6), 3229–3235. doi:10.1182/blood-2007-09-114561
Takeda, K., Cowan, A., and Fong, G. H. (2007). Essential role for prolyl hydroxylase domain protein 2 in oxygen homeostasis of the adult vascular system. Circulation 116 (7), 774–781. doi:10.1161/circulationaha.107.701516
Takeda, K., Ho, V. C., Takeda, H., Duan, L. J., Nagy, A., and Fong, G. H. (2006). Placental but not heart defects are associated with elevated hypoxia-inducible factor alpha levels in mice lacking prolyl hydroxylase domain protein 2. Mol. Cell Biol. 26 (22), 8336–8346. doi:10.1128/mcb.00425-06
Tambuwala, M. M., Cummins, E. P., Lenihan, C. R., Kiss, J., Stauch, M., Scholz, C. C., et al. (2010). Loss of prolyl hydroxylase-1 protects against colitis through reduced epithelial cell apoptosis and increased barrier function. Gastroenterology 139 (6), 2093–2101. doi:10.1053/j.gastro.2010.06.068
Taniguchi, C. M., Miao, Y. R., Diep, A. N., Wu, C., Rankin, E. B., Atwood, T. F., et al. (2014). PHD inhibition mitigates and protects against radiation-induced gastrointestinal toxicity via HIF2. Sci. Transl. Med. 6 (236), 236ra64. doi:10.1126/scitranslmed.3008523
Taylor, C. T., and Colgan, S. P. (2007). Hypoxia and gastrointestinal disease. J. Mol. Med. Berl. 85 (12), 1295–1300. doi:10.1007/s00109-007-0277-z
Taylor, C. T., and Colgan, S. P. (2017). Regulation of immunity and inflammation by hypoxia in immunological niches. Nat. Rev. Immunol. 17 (12), 774–785. doi:10.1038/nri.2017.103
Taylor, C. T., and Scholz, C. C. (2022). The effect of HIF on metabolism and immunity. Nat. Rev. Nephrol. 18 (9), 573–587. doi:10.1038/s41581-022-00587-8
Taylor, M. K., Murphy, S., Levesque, B. G., Carter, L. L., and Salter-Cid, L. J. J. (2021). GB004 drives protective effects on immune cells and epithelial cells using human ex vivo monolayer and co-culture systems. J. Crohns Colitis 15, S165–S166. doi:10.1093/ecco-jcc/jjab076.188
Taylor, S. J., Winter, M. G., Gillis, C. C., Silva, L. A. D., Dobbins, A. L., Muramatsu, M. K., et al. (2022). Colonocyte-derived lactate promotes E. coli fitness in the context of inflammation-associated gut microbiota dysbiosis. Microbiome 10 (1), 200. doi:10.1186/s40168-022-01389-7
van der Flier, L. G., and Clevers, H. (2009). Stem cells, self-renewal, and differentiation in the intestinal epithelium. Annu. Rev. Physiol. 71, 241–260. doi:10.1146/annurev.physiol.010908.163145
Van Welden, S., Selfridge, A. C., and Hindryckx, P. (2017a). Intestinal hypoxia and hypoxia-induced signalling as therapeutic targets for IBD. Nat. Rev. Gastroenterol. Hepatol. 14 (10), 596–611. doi:10.1038/nrgastro.2017.101
Van Welden, S., De Vos, M., Wielockx, B., Tavernier, S. J., Dullaers, M., Neyt, S., et al. (2017b). Haematopoietic prolyl hydroxylase-1 deficiency promotes M2 macrophage polarization and is both necessary and sufficient to protect against experimental colitis. J. Pathol. 241 (4), 547–558. doi:10.1002/path.4861
Van Welden, S., Laukens, D., Ferdinande, L., De Vos, M., and Hindryckx, P. (2013). Differential expression of prolyl hydroxylase 1 in patients with ulcerative colitis versus patients with Crohn's disease/infectious colitis and healthy controls. J. Inflamm. (Lond) 10 (1), 36. doi:10.1186/1476-9255-10-36
Vittori, D. C., Chamorro, M. E., Hernández, Y. V., Maltaneri, R. E., and Nesse, A. B. (2021). Erythropoietin and derivatives: Potential beneficial effects on the brain. J. Neurochem. 158 (5), 1032–1057. doi:10.1111/jnc.15475
Walmsley, S. R., Chilvers, E. R., Thompson, A. A., Vaughan, K., Marriott, H. M., Parker, L. C., et al. (2011). Prolyl hydroxylase 3 (PHD3) is essential for hypoxic regulation of neutrophilic inflammation in humans and mice. J. Clin. Invest. 121 (3), 1053–1063. doi:10.1172/JCI43273
Walmsley, S. R., Print, C., Farahi, N., Peyssonnaux, C., Johnson, R. S., Cramer, T., et al. (2005). Hypoxia-induced neutrophil survival is mediated by HIF-1alpha-dependent NF-kappaB activity. J. Exp. Med. 201 (1), 105–115. doi:10.1084/jem.20040624
Wang, G. L., Jiang, B. H., Rue, E. A., and Semenza, G. L. (1995). Hypoxia-inducible factor 1 is a basic-helix-loop-helix-PAS heterodimer regulated by cellular O2 tension. Proc. Natl. Acad. Sci. U. S. A. 92 (12), 5510–5514. doi:10.1073/pnas.92.12.5510
Watts, E. R., and Walmsley, S. R. (2019). Inflammation and hypoxia: HIF and PHD isoform selectivity. Trends Mol. Med. 25 (1), 33–46. doi:10.1016/j.molmed.2018.10.006
Welden, S. V., Selfridge, A. C., and Hindryckx, P. (2017). Intestinal hypoxia and hypoxia-induced signalling as therapeutic targets for IBD. Nat. Rev. Gastroenterol. Hepatol. 14, 596–611. doi:10.1038/nrgastro.2017.101
Wicks, E. E., and Semenza, G. L. (2022). Hypoxia-inducible factors: Cancer progression and clinical translation. J. Clin. Invest. 132 (11), e159839. doi:10.1172/JCI159839
Wish, J. B., Eckardt, K. U., Kovesdy, C. P., Fishbane, S., Spinowitz, B. S., and Berns, J. S. (2021). Hypoxia-inducible factor stabilization as an emerging therapy for CKD-related anemia: Report from a scientific workshop sponsored by the national kidney foundation. Am. J. Kidney Dis. 78 (5), 709–718. doi:10.1053/j.ajkd.2021.06.019
Wobben, R., Hüsecken, Y., Lodewick, C., Gibbert, K., Fandrey, J., and Winning, S. (2013). Role of hypoxia inducible factor-1α for interferon synthesis in mouse dendritic cells. Biol. Chem. 394 (4), 495–505. doi:10.1515/hsz-2012-0320
Wu, Q., Liang, X., Wang, K., Lin, J., Wang, X., Wang, P., et al. (2021). Intestinal hypoxia-inducible factor 2α regulates lactate levels to shape the gut microbiome and alter thermogenesis. Cell Metab. 33 (10), 1988–2003.e7. doi:10.1016/j.cmet.2021.07.007
Xie, Y., Yuan, T., Qin, Y., Weng, Z., and Fang, J. (2018). Prolyl hydroxylase 2 is dispensable for homeostasis of intestinal epithelium in mice. Acta Biochim. Biophys. Sin. (Shanghai) 50 (6), 540–546. doi:10.1093/abbs/gmy037
Xu, Y. M., Gao, Q., Zhang, J. Z., Lu, Y. T., Xing, D. M., Qin, Y. Q., et al. (2020). Prolyl hydroxylase 3 controls the intestine goblet cell generation through stabilizing ATOH1. Cell Death Differ. 27 (7), 2131–2142. doi:10.1038/s41418-020-0490-7
Xue, X., Ramakrishnan, S., Anderson, E., Taylor, M., Zimmermann, E. M., Spence, J. R., et al. (2013). Endothelial PAS domain protein 1 activates the inflammatory response in the intestinal epithelium to promote colitis in mice. Gastroenterology 145 (4), 831–841. doi:10.1053/j.gastro.2013.07.010
Yamamoto, A., Hester, J., Macklin, P. S., Kawai, K., Uchiyama, M., Biggs, D., et al. (2019). Systemic silencing of PHD2 causes reversible immune regulatory dysfunction. J. Clin. Inves 129 (9), 3640–3656. doi:10.1172/JCI124099
Yang, K., Xu, J., Fan, M., Tu, F., Wang, X., Ha, T., et al. (2020). Lactate suppresses macrophage pro-inflammatory response to LPS stimulation by inhibition of YAP and NF-κB activation via GPR81-mediated signaling. Front. Immunol. 11, 587913. doi:10.3389/fimmu.2020.587913
Young, H. W., Williams, O. W., Chandra, D., Bellinghausen, L. K., Pérez, G., Suárez, A., et al. (2007). Central role of Muc5ac expression in mucous metaplasia and its regulation by conserved 5' elements. Am. J. Respir. Cell Mol. Biol. 37 (3), 273–290. doi:10.1165/rcmb.2005-0460OC
Zeitouni, N. E., Chotikatum, S., von Köckritz-Blickwede, M., and Naim, H. Y. (2016). The impact of hypoxia on intestinal epithelial cell functions: Consequences for invasion by bacterial pathogens. Mol. Cell Pediatr. 3 (1), 14. doi:10.1186/s40348-016-0041-y
Zhang, J., Han, C., Dai, H., Hou, J., Dong, Y., Cui, X., et al. (2016). Hypoxia-inducible factor-2 limits natural killer T cell cytotoxicity in renal ischemia/reperfusion injury. J. Am. Soc. Nephrol. 27, 92–106. doi:10.1681/ASN.2014121248
Zhang, Y. Z., and Li, Y. Y. (2014). Inflammatory bowel disease: Pathogenesis. World J. Gastroenterol. 20 (1), 91–99. doi:10.3748/wjg.v20.i1.91
Keywords: prolyl hydroxylases, hypoxia inducible factor, inflammatory bowel disease, intestine epithelial, barrier function, inhibitor, therapeutics
Citation: Lun J, Zhang H, Guo J, Yu M and Fang J (2023) Hypoxia inducible factor prolyl hydroxylases in inflammatory bowel disease. Front. Pharmacol. 14:1045997. doi: 10.3389/fphar.2023.1045997
Received: 16 September 2022; Accepted: 18 April 2023;
Published: 02 May 2023.
Edited by:
Deping Kong, Shanghai Jiao Tong University, ChinaReviewed by:
Lakhveer Singh, DIT University, IndiaCopyright © 2023 Lun, Zhang, Guo, Yu and Fang. This is an open-access article distributed under the terms of the Creative Commons Attribution License (CC BY). The use, distribution or reproduction in other forums is permitted, provided the original author(s) and the copyright owner(s) are credited and that the original publication in this journal is cited, in accordance with accepted academic practice. No use, distribution or reproduction is permitted which does not comply with these terms.
*Correspondence: Jing Fang, amZhbmdAcWR1LmVkdS5jbg==
†These authors have contributed equally to this work
Disclaimer: All claims expressed in this article are solely those of the authors and do not necessarily represent those of their affiliated organizations, or those of the publisher, the editors and the reviewers. Any product that may be evaluated in this article or claim that may be made by its manufacturer is not guaranteed or endorsed by the publisher.
Research integrity at Frontiers
Learn more about the work of our research integrity team to safeguard the quality of each article we publish.