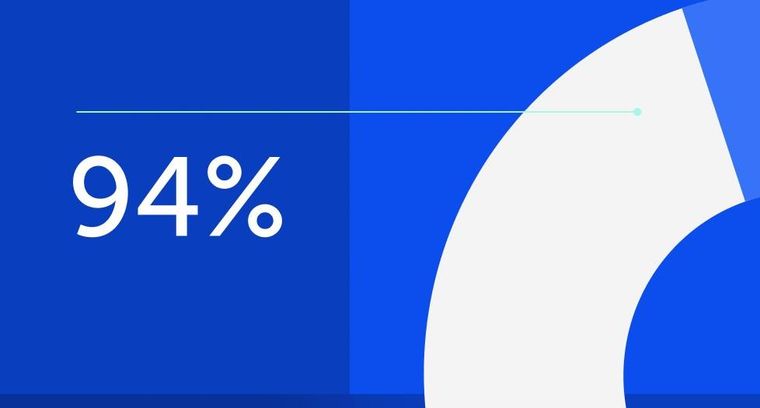
94% of researchers rate our articles as excellent or good
Learn more about the work of our research integrity team to safeguard the quality of each article we publish.
Find out more
ORIGINAL RESEARCH article
Front. Pharmacol., 03 March 2023
Sec. Ethnopharmacology
Volume 14 - 2023 | https://doi.org/10.3389/fphar.2023.1012716
This article is part of the Research TopicLaboratory-based green extraction of natural products for pharmaceutical applicationsView all 4 articles
Comfrey (Symphytum officinale L.) contains rosmarinic acid which has different pharmacological activities, such as antioxidant and anti-inflammatory activities. However, the medicinal use of comfrey is limited by the hepatotoxic effect of lycopsamine in comfrey, which overshadows the health benefits of rosmarinic acid. Natural deep eutectic solvents (NADES) have a wide range of extraction properties, that provides a new approach to the detoxification of comfrey. In the present study, betaine-based and choline chloride-based NADES were screened for selective extraction of rosmarinic acid over lycopsamine. Ultrasonication was used in conjunction with NADES extraction. The chemical profile of the NADES extracts on antioxidant, anti-inflammatory and hepatotoxic activities were investigated using some chemical reagents. Betaine-urea (1:2 molar ratio, 50% water) obtained the highest content of rosmarinic acid and a low level of lycopsamine (1.934 and 0.018 mg/g, respectively). Betaine-urea was also shown to be more effective to extract rosmarinic acid compared to methanol-UAE under the same conditions, which gave lower rosmarinic acid and higher lycopsamine levels (0.007 and 0.031 mg/g, respectively). Betaine-urea extracts showed higher antioxidant and anti-inflammatory properties as compared with other NADES extracts, however, had lower hepatotoxic profile. This study recommends the use of betaine-urea to detroxify comfrey to open wider opportunities for the development of comfrey for medicinal use.
Comfrey (Symphytum officinale L., Boraginaceae) (Wilkinson, 2003), is a perennial plant with long rough leaves and white, creamy yellow, or pale purple flowers. The leaves and roots are often consumed (Frost et al., 2013). Comfrey is a well-known traditional plant medicine with a long therapeutic history. Its preparations have been widely used for the treatment of severe muscle and joint problems, wounds and bone fractures, and inflammation (Seigner et al., 2019). Traditionally, comfrey is boiled as tea to help treat gastritis, ulcers, and lung disorders (Wilkinson, 2003). Comfrey is also used as an external preparation to heal, ulcers, sprains, and fractured bones (Smith and Jacobson, 2011). Comfrey can survive in tropical climates, thus is widespread in Indonesia (Kementerian Kesehatan Republik Indonesia, 2017), where people use to reduce inflammation, aches and pains, diarrhea, typhoid, heartburn, and bone fractures (Kinho et al., 2011).
Rosmarinic acid, which is the caffeic acid ester of 3,4-dihydroxyphenyllactic acid (Tawfeeq et al., 2018), is a common phenolic acid in comfrey (Trifan et al., 2018). Shoots and leaves of comfrey contained the most of rosmarinic acid (Dresler et al., 2017). Previous studies showed some biological activities of rosmarinic acid and its derivates such as anti-inflammatory, antioxidant, antibacterial, anti-hyperglycemic and anti-allergic activities (Tawfeeq et al., 2018; Salehi et al., 2019). The chemical structure of rosmarinic acid is shown in Figure 1.
Nevertheless, there are some restrictions for comfrey applications. Comfrey has been linked to liver damage (Frost et al., 2014), which is associated with the presence of pyrrolizidine alkaloids in the plant. Pyrrolizidine alkaloids such as lycopsamine are significantly found in of the comfrey plant (0.2%–0.4%) (Liu et al., 2009). It has been reported that lycopsamine was responsible for the hepatotoxic effects (Zsuzsanna et al., 2015; Moreira et al., 2018; Salehi et al., 2019). This is probably due to its metabolism by CYP3A4 into pyrrole derivatives, which are hepatotoxic and pulmotoxic (Janes et al., 2012). The lycopsamine-induced hepatotoxicity obscures the advantages of rosmarinic acid in comfrey. Therefore, improvement of comfrey extraction is required to maximize rosmarinic acid levels while minimize lycopsamine level in order to produce the best therapeutic effect. However, selective extraction of rosmarinic acid over lycopsamine is a challenging task due to the substantial presence of lycopsamine in comfrey. Chemical structure of lycopsamine showed in Figure 1.
Natural Deep Eutectic Solvents (NADES) have emerged as a green approach for maximizing the potential and advantages of natural metabolites (Zhang et al., 2018). NADES are made of natural components, that can be found in cells and organisms, such as organic acids, urea, glycerol, and sugars. NADES are considered safe due to non-volatility and environmentally friendly compared to organic solvents which are flammable and non-biodegradable (Cao et al., 2020). The inclusion of hydrogen bond donors (HBD) and hydrogen bond acceptors in NADES form strong hydrogen bond interactions between them, leading to low melting point mixtures. In addition, NADES have some desirable properties including ease of preparation, good extraction ability, and low cost (Cysewski and Jeliński, 2019; Liu et al., 2019). NADES can also improve the stability and shelf life of metabolites in extracts (Obluchinskaya et al., 2021). Furthermore, the solubility of metabolites dissolved in NADES has been observed to increase (Cao et al., 2020). NADES have been reported to successfully extract some natural metabolites such as phenolic metabolites in Rosmarinus officinalis L. and Mentha x piperita L. (Jeong et al., 2018; Barbieri et al., 2020) as well as alkaloid metabolites in Crinum x powelii Baker bulbs and Coffea canephora Pierre ex A.Froehner (Shawky et al., 2018; Syakfanaya et al., 2019). The present work aims to develop green approach for selective extraction of rosmarinic acid over lycopsamine from the leaves of comfrey (Symphytum officinale L). Betaine- and choline chloride-based NADES were formed with glycerol, urea, and sucrose. NADES extracts were analyzed by an HPLC-DAD. Further, the antioxidant, anti-inflammatory, and hepatotoxic chemical profiles of NADES extracts were compared with those of conventional solvent.
The following materials were utilized in this study. Comfrey (Symphytum officinale L.) leaves were purchased from local farmer in Karanganyar, Indonesia and authenticated in The Faculty of Biology, Universitas Gajah Mada. Choline chloride, Urea, 2,2-diphenyl-1-picrylhydrazyl (DPPH) and 2,2′-azino-bis (3-ethylbenzothiazoline-6-sulfonic acid) (ABTS) were purchased from Sigma-Aldrich (St. Louis, MO, United States). Glycerol was obtained from PT Molex Ayus (Jakarta, Indonesia). Sucrose was obtained from PT Brataco (Jakarta, Indonesia). Betaine was bought from Shandong Ruihong Biotech (Jinan city, China). Standards of lycopsamine and rosmarinic acid were bought from Phytolab (Vestenbergsgreuth, Germany). Vitamin C and sodium diclofenac were purchased from National Food and Drug Agency of Indonesia (Jakarta, Indonesia). Bovine serum albumin (BSA) was purchased from Himedia (Maharashtra, India). Solvents used were of HPLC grade.
NADES were synthesized by heating and stirring method as described elsewhere (Dai et al., 2013). A hydrogen bond donor (HBD) and a hydrogen bond acceptor (HBA) component in a specific molar ratio were added into a beaker glass. The mixture was stirred and heated at 80°C until a clear solution was generated. All compounds used in the preparation of NADES are readily accessible and low-cost, Table 1.
All NADES extractions were carried out using an ultrasonic bath (Krisbow, Jakarta, Indonesia). Comfrey leaf powder was placed in a flask and blended with NADES in a solid to liquid ratio of 1:20. Ultrasonication-assisted extraction (UAE) was carried out for 45 min at 50°C (35 W, 42 kHz). The solid residue was separated from the liquid phase by centrifugation (Hettich Zentrifugen, Germany), at 4,500 rpm for 17 min. The supernatant was filtered through a 0.45 µm Whatman micropore filter paper. The filtrate was collected and kept at 4°C until further analysis. The filtrate was collected and kept in a refrigerator until further examination (Duan et al., 2016).
To get comparative data, methanol extraction in conjunction with UAE was performed. The methanol-UAE extraction was conducted for 50 min at 50°C with a ratio of solid to liquid of 1:60. The filtrates were evaporated using a rotary vacuum evaporator (R-215, Buchi, Switzerland). The concentrated filtrates were collected and refrigerated for future use in analysis (Janes et al., 2012).
The contents of rosmarinic acid and lycopsamine in comfrey extracts were determined by an Agilent 1200 HPLC system (Analytical Instruments, CA, United States) with a UV-Vis spectroscopy detector. A Zorbax Eclipse XDB-C18 column (4,6 × 150 mm, 5 μm; Agilent Technologies Co., Ltd, Santa Clara, United States) was used for chromatographic separation. The investigated substances were separated using isocratic elution for 15 min with 30% of acetonitrile as phase B and 0.1% of formic acid as phase A. The injection volume was 20 µL. Column separation was carried out at room temperature with a mobile phase at flow rate of 0.5 mL/min. The HPLC chromatogram of rosmarinic acid and lycopsamine was identified at 210 and 330 nm, respectively (Syarifah et al., 2022). Calibration curves were generated for rosmarinic acid (4–20 μg/mL) and lycopsamine (10–100 ng/mL) at five concentration points.
DPPH radical scavenging profiling was conducted based on a reported method (Vongsak et al., 2018). In a 96 well plate was mixed 100 µL of comfrey extract at different concentrations in methanol (4–20 μg/mL) and 100 µL of 253.6 µM of DPPH reagents in methanol. The plate was incubated for 20 min at 37°C, thereafter the absorbance was measured at 560 nm on a microplate reader (Glomax Promega, United States). Methanol in place of sample was used as a blank. Rosmarinic acid, lycopsamine, and vitamin C were used as positive control. All measurements were conducted in triplicate. The DPPH chemical profile was determined using Equation 1:
Where Ablank was absorbance of the blank solution and Asample was the absorbance of sample solution. The IC50 values were calculated by a linier regression generated from the inhibition percentage plotted against concentration.
ABTS•+ cation radical reagents were generated by mixing equal volumes of 7 mM ABTS reagent and 2.45 mM potassium persulfate solutions in the dark at room temperature for 16–20 h. ABTS•+ solution was then adjusted to an absorbance of 0.70 ± 0.02 at 600 nm by adding methanol. Into a 96-well plate was added 100 µL of comfrey extracts at different concentrations and 100 µL of ABTS•+ solution. The reduction in absorbance was observed after 6 min (Trifan et al., 2018). Experiments were conducted in triplicate. The ABTS•+ radical scavenging profiling was calculated using Eq. 1.
Anti-inflammatory profile of comfrey extracts was evaluated using reagent based on their inhibition on protein denaturation, according to a method described elsewhere (Heendeniya et al., 2018). Reaction mixture was prepared containing 100 µL of samples at different concentrations (4–20 μg/mL), 140 µL of freshly prepared phosphate buffer saline (10 mM, pH 6.3), and 20 µL of egg albumin extracted from hen egg or 45 µL of bovine serum albumin solution (0.5%, w/v). The reaction mixture was incubated at 37°C for 15 min. The mixture was further heated at 70°C for 5 min. After cooling down at ambient temperature, the turbidity of the reaction mixture was measured at 600 nm using a microplate reader (Glomax Promega, United States) against phosphate buffer saline as a blank. Sodium diclofenac was used as a positive control. The experiments were performed in triplicate. The inhibitory profile was determined based on Eq. 1.
Human hepatocytes (HepaRG) were obtained from Sigma-Aldrich (United States). HepaRG were cultured in Roswell Park Memorial Institute (RPMI) 1,640 medium, supplemented with 10% (v/v) fetal bovine serum (FBS), 1% antibiotics, and 1% fungizone. The cells were maintained at 37°C with 5% CO2. Trypsin (0.25%) was used to harvest cells at 90%–100% confluency. Trypan blue was used to color the cells for death cell detection. Comfrey stock solution (1,000 g/mL) was prepared in DMSO, which was further diluted with basal media to obtain reagen solutions of different concentrations (You et al., 2018).
The cytotoxic effect of comfrey on HepaRG cells was assessed using the MTS 3-(4,5-dimethylthiazol-2-yl)-5-(3-carboxymethoxyphenyl)-2-(4-sulfophenyl)-2H-tetrazolium) reagent. The cells were seeded in a 96-well plate at a density of 20,000 cells/well with culture medium (FBS, 10%, v/v). The next day, the cells were treated with various concentrations of comfrey extracts 0, 25, 50 and 100 g/mL. After incubation for 24 h, 10 µL of MTS solution was added. The mixtures were further incubated for 1–4 h at 37°C. The absorbance was measured with an ELISA reader at 490 nm. DMSO solution (0.1%) was used as a negative control. The LC50 values were calculated using the probit model (You et al., 2018).
The LC50 values were calculated by a linier regression generated from the chemical profile of cell death percentage plotted against concentration.
Sample in FTIR assay was 10 mg of rosmarinic acid standard, 10 mL of NADES betain-urea liquid and 10 mL of 1% mixture of rosmarinic acid standard in NADES betain-urea. The FTIR test was carried out using the Thermo Scientific - Nicolet iS50 FTIR + NIR Spectrometer provided by the ILRC laboratory of the University of Indonesia. The FTIR spectra was observed structural changes of rosmarinic acid due to solvation in NADES.
Sample in 1H-NMR assay was 10 mg of rosmarinic acid standard, 10 mL of NADES betain-urea liquid and 10 mL of 10% mixture of rosmarinic acid standard in NADES betain-urea. 1H-NMR assay was carried out using the JEOL-ECZ 500R with CD3OD as solvent at LIPI Serpong. The 1H-NMR assay spectra were evaluated the hydrogen bond of sample.
Morphological observation was carried out by a Jeol JSM-IT200 equipment. Dried plant materials (leaf powder, methanol, and NADES extracts) each 50 g were aerated to dryness. Samples were further dehydrated under high vacuum, then coated with thin layer of gold. An accelerated voltage of 20 kV was used. Samples were observed under SEM analyser for the shape and the stomatas.
In this work, six different combinations of NADES were screened for selective extraction of rosmarinic acid over lycopsamine from comfrey leaves (Table 1). The studied NADES were composed of choline chloride or betaine as hydrogen bond acceptor (HBA), whereas urea, sucrose, or glycerol were used as hydrogen bond donor (HBD), in a 1:2 molar ratio of HBA:HBD. Each NADES was added with 50% (v/v) water to reduce the viscosity.
Ultrasonication was used to assist extraction. The same basic extraction conditions were followed (50°C, 1:20 solid to liquid ratio, and 50 min extraction time). The residue of NADES extracts was re-extracted using methanol to evaluate the effectiveness of the extraction process. Methanol extraction using the same extraction procedures was used for comparison purpose. Figure 2 shows the metabolite of rosmarinic acid and lycopsamine in each NADES following extraction of comfrey leaves.
FIGURE 2. Comparison of rosmarinic acid and lycopsamine contents obtained by extraction of comfrey leaves in different NADES. The residue was further re-extracted using methanol to assess the contents of the bioactive metabolites.
HPLC method was used for analyses of rosmarinic acid and lycopsamine contents in the extract. The chromatogram of standard metabolites is shown in Figure 3. Analyses results in Figure 2 shows that each NADES gave different levels of rosmarinic acid and lycopsamine. However, all NADES showed better extraction performance with regard to rosmarinic acid and lycopsamine contents, when compared with methanol extraction. Of these NADES, betaine-urea extracted the highest level of rosmarinic acid (1.934 mg/g), while successfully maintain the lowest content of lycopsamine (0.018 mg/g). Significant amount of lycopsamine and low amount of rosmarinic acid was quantified from the residue which was re-extracted using methanol (0.102 and 0.240 mg/g, respectively). It was noted that, the least amount of rosmarinic acid was quantified from methanol extract (0.007 mg/g).
FIGURE 3. HPLC chromatograms of the standard metabolites (A) rosmarinic acid (B) lycopsamine, and (C) extracts obtained by ultrasound assisted extraction of comfrey leaves.
The chemical antioxidant profile of comfrey extracted by different NADES are shown in Figure 4. For comparison, the antioxidant profile of methanol extract of comfrey and standards were also determined. In general, all NADES extracts showed significantly higher antioxidant profile by DPPH and ABTS assays when compared with methanol extract. It was also worth noting that the antioxidant profile of NADES extracts were comparable to those of standards rosmarinic acid, lycopsamine, vitamin C in both assays. Comfrey extracted by betaine-urea showed the highest ABTS scavenging chemical profile (IC50 0.33 μg/mL), whereas its chemical profile by DPPH reagent was the second strongest (IC50 7.36 μg/mL) after extract derived from choline chloride-glycerol.
FIGURE 4. Comparison of antioxidant activities of comfrey leaf extracts by different NADES and methanol, and those of standard metabolites.
The ability of NADES extracts to profile inflammation was evaluated chemically by protein denaturation inhibition assay, using BSA and egg protein. Results are shown in Figure 5. Generally, all NADES extracts exhibited stronger anti-inflammatory chemical profile compared to methanol extract. Some of NADES extracts showed even stronger chemical profile when compared to standards rosmarinic acid, lycopsamine, and sodium diclofenac. Compared to other NADES extracts, betaine-urea extract was the most active to inflammation profile using BSA, as shown by the lowest IC50 value. Strong profile was also shown when using egg protein, although other NADES extracts showed stronger profiles, such as choline chloride-urea, betaine-glycerol, and betaine-sucrose extracts.
FIGURE 5. Comparison of anti-inflammatory activities of comfrey leaf extracts by different NADES and methanol, and those of standard metabolites.
The LC50 values of hepatotoxic profile of extracts are shown in Table 2. NADES betain-urea extract showed the highest LC50 of cell death using chemical reagent. In contrast, the residue obtained from betaine-urea extract exhibited the lowest LC50. The methanol extract showed significant hepatotoxicity profile as indicated by a low LC50.
The present study compared betaine based- and choline chloride-based- NADES to achieve high rosmarinic acid and low lycopsamine extraction. Rosmarinic acid (a phenolic metabolites) was commonly found in the Lamiaceae and Boraginaceae families (Öztürk et al., 2011), including in comfrey. Together with rosmarinic acid, lycopsamine was also present in significance in comfrey. Lycopsamin was the most abundant PA found in the leaves and in this study the leaves of the komfrey plant were only used. Lycopsamine as one of the most widely used lycopsamines from leaf parts is expected to represent a significant reduction in PA from extracting leaf parts of comfrey plants (Salehi et al., 2019). The medicinal use of comfrey is limited by the hepatoxic effect of lycopsamine (Cao et al., 2008). Selective extraction was achieved by using betaine-urea, which was able to extract rosmarinic acid more than eight times higher than choline chloride-urea. Betaine-urea was also able to selectively target rosmarinic acid over lycopsamine compared to other studied NADES (Figure 2). The re-extraction of the residue identified decreased level of rosmarinic acid, whereas observed high content of lycopsamine. As seen in Figure 2 lycopsamine was shown to be better extracted using methanol. These results demonstrated successful selectivity of betaine-urea extraction towards rosmarinic acid, indicating potential function to detroxify comfrey. As previously mentioned, despite the different health benefits of comfrey, its medicinal use is limited by the hepatoxic effect of lycopsamine (Cao et al., 2008), which was found in significance in comfrey.
NADES constituents (HBA and HBD) and their molar ratio determine the physicochemical properties of NADES, which influence the affinity between target metabolites and the extraction solvents (Table 1). The NADES component forms hydrogen bond interactions and Van der Walls interactions by pairing a hydrogen bond acceptor (HBA) and a hydrogen bond donor (HBD) at suitable molar ratios (Smith et al., 2014; Kalhor and Ghandi, 2019). However, the formation of hydrogen bond networks in NADES leads to highly viscous solvents. Thus, in the present study, each NADES was added with water (50%, v/v) which greatly reduced the viscosity. Excessive water addition (more than 50%) is avoided as this addition destroys hydrogen bond interactions between HBA and HBD (Dai et al., 2013). The incorporation of water in NADES may also modulate the polarity of NADES. These effects subsequently increased mass transfer and enhanced extraction efficiency.
Comfrey leaves showed the morphology of the dried comfrey leaf cells before extraction which was still in a large state after extraction in methanol showed the morphology of comfrey leaves after extraction with methanol and sonication, the damage to the leaf cells became smaller but the stomata part of the sample was still intact. Meanwhile, samples of NADES extract experienced greater stomata damage and this was in line with high levels because the more damaged the stomata, the higher the metabolites that came out into the solvent.
In the present work, ultrasonication was employed as the energy source for extraction. The effect of NADES combined with ultrasonication can be observed on the morphological changes of the comfrey leaves as seen in the SEM images (Figure 6). The cavitation bubbles implode on the cell surface and disrupt the cell wall, allowing the particle size to decrease and the mass transfer process from the cell into the solvent to rise. Figure 6B shows that ultrasonication in methanol extraction broke down and destroyed the morphological structure of comfrey (Figure 6B). This helps release various metabolites into the solvent during the extraction process. The UAE-NADES extraction was seen to change the leaf surface to become large and formed a fibrous network (Figure 6C). This may be due to the implosion cavitation bubbles near the leaf cell surface that may erode the leaf structure and help release metabolites into the solvent. The ultrasound-assisted extraction (UAE) method yielded superior secondary metabolite extraction results than the NADES solvent with the maceration technique (Mansinhos et al., 2021). Therefore, UAE extraction procedures were performed for both methanol and NADES solvents (Kanedi et al., 2017; Ruesgas-Ramón et al., 2017; Vinatoru et al., 2017).
FIGURE 6. Scanning electron micrographs of comfrey leaves before extraction (A), after extraction in methanol (B), and NADES (C).
The FTIR absorption spectrum was used to observed structural changes of rosmarinic acid due to solvation in NADES (Figure 7). For this purpose, The FTIR spectra of rosmarinic acid, NADES (betaine-urea), and rosmarinic acid in NADES were compared. FTIR spectra of standard rosmarinic acid in NADES and NADES (betaine-urea) showed characteristic hydroxyl peaks in the 3,000–3,500 cm−1 region. In addition, the solubility of rosmarinic acid in NADES solution was seen at a low intensity peaks in the 2,500–3,000 cm−1 region. The peaks in the 1,000 cm−1 region indicated the hydrogen bonds formed between the hydroxyl and carbonyl groups. This shows that hydrogen bonding plays an important role in the high dissolution of phytochemical metabolites in NADES. The displacement of OH group signals in 1H NMR proton spectra confirmed the establishment of hydrogen bonds, as shown in Figure 8. In the 1H–1H NOESY spectra (Figure 9), it was observed the presence of the spatial heteromolecular interaction between water molecules and the OH groups of the NADES components, supporting the establishment of hydrogen bonds between them.
FIGURE 7. FTIR spectra of (A) standard rosmarinic acid (B) rosmarinic acid in NADES (betaine-urea), and (C) NADES (betaine-urea).
Previously, choline chloride and betaine-based NADES have been used for the extraction of phenolic metabolites and alkaloids from plant materials. For example, phenolic metabolites extracted from Rosmarinus officinale L. using choline chloride-1,2 propanediol (1:2 molar ratio, 10% of water content) gave the best results in rosmarinic acid extraction (13,563.0 μg/g) (Barbieri et al., 2020). Extraction of caffeine components (alkaloid derivatives) in Camellia sinensis L. Kuntze gave the best results using NADES-UAE choline chloride-lactic acid (1:1 molar ratio, 31% water content) (Cai et al., 2019). Betaine-urea NADES was used to extract chlorogenic acid (a phenolic metabolites) and caffeine (an alkaloid) from Coffea canephora Pierre ex A.Froehner, yielding higher chlorogenic acid (28.62 mg/g) and lower caffeine (7.89 mg/g) (Syakfanaya et al., 2019). In the present study, betaine-urea was proved to be selective toward rosmarinic acid but not lycopsamine. These results are indicative of prospective use of betaine-urea to detoxify comfrey due to the lower content lycopsamine in the betaine-urea extract. This finding may lead to the more usability of comfrey for its antioxidant and anti-inflammatory chemical profiles, whereas at the same time less toxic to liver.
In the current study, antioxidant profile of NADES extracts were carried out to investigate the efficiency of extraction. The antioxidant profiles were evaluated based on their scavenging ability on the radical DPPH and ABTS reagent molecules. Both DPPH and ABTS radicals have sufficient capacity to accept electrons and hydrogen atoms from samples containing antioxidant metabolites (Francenia Santos-Sánchez et al., 2019).
Previously, comfrey roots extracted by aqueous ethanol were demonstrated to have high antioxidant activities as measured by DPPH radical reagent and ABTS cation radical reagent scavenging abilities, reducing power, and 15-lipoxygenase inhibition assay, which were associated with the phenolic metabolites in comfrey root extract (Trifan et al., 2018). Similarly, Neagu et al. (2010) found correlation between the antioxidant capacity profiled using DPPH and ABTS reagents and polyphenol content of the root ethanolic extracts (Neagu et al., 2010). It was well known that extraction capacity of NADES towards phenolic metabolites were closely correlated with the antioxidant profile of the extracts (Fu et al., 2021). Rosmarinic acid which was the target metabolites in this study belongs to the category of phenolic metabolites. Similar findings were observed in the present study. Betaine–urea extract, which was demonstrated to obtain the highest content of rosmarinic acid (Figure 2), gave the highest antioxidant profile, when compared with other NADES and methanol extracts (Figure 3). These results indicate that rosmarinic acid may be responsible for the antioxidant chemical profile observed in the NADES extracts. Rosmarinic acid contains two phenolic rings, both of which have ortho-hydroxy groups which may be crucial in its antioxidant activity. The selective extraction towards rosmarinic acid was further confirmed by antioxidant profile of residues of NADES extracts. It was expected that betaine-urea extract showed the weakest antioxidant profile. This is due to the lowest content of rosmarinic acid left in the comfrey residue. Rosmarinic acid was reported to have therapeutic effects due to its antioxidant activity (Jurić et al., 2021), (Benedec et al., 2015). In addition, rosmarinic acid has been reported to have different biological activities such as HIV1, antitumor, antihepatitic suppression, liver protection, blood clot suppression, and anti-inflammatory (Aldoghachi et al., 2021).
Endogenous variables that promote the generation of reactive oxygen species can initiate the inflammatory process. If free radicals are present in significant concentrations in cells, they will cause tissue damage (Francenia Santos-Sánchez et al., 2019). The presence of protein denaturation events is associated to the inflammatory response. Protein denaturation in cells or intercellular molecules linked to tissue injury As a result, a metabolites’s capacity to suppress protein denaturation indicates possible anti-inflammatory effect (Osman et al., 2016). In the present study, comfrey leaf extracts were observed to inhibit protein denaturation (Figure 5). The comfrey plant has long been used as a topical analgesic and anti-inflammatory. Comfrey plants can be used to alleviate muscular discomfort, mend fractures and wounds, and function as an anti-inflammatory (Seigner et al., 2019). Previous research has indicated that comfrey plants have analgesic and anti-inflammatory properties due to the presence of phenolic substances such as rosmarinate acid. Rosmarinic acid inhibits the production and release of pro-inflammatory mediators (Oliviu et al., 2017). Several studies have also indicated that polyphenols have an anti-inflammatory impact (Boşca et al., 2015).
Rosmarinic acid has been shown to inhibit complement activation both in vivo and in vitro. Rosmarinic acid inhibits complement activation by covalently reacting with the activated compliment component C3b, at the site of inflammation where complement activation is taking place, without the side effects of other drugs, such as glucocorticoids and anti-inflammatory drugs (Colica et al., 2018). Rosmarinic acid may be a potent inhibitor of the expression of the pro-inflammatory gene cyclooxygenase 2 (COX2). It is considered a risk factor for both HT29 cells of colon cancer and the non-malignant breast epithelial cell line MCF10A (Scheckel et al., 2008).
Pyrrolizidine alkaloids (PAs) are common secondary plant metabolites that are widely distributed around the world, and about half of them have been reported to be hepatotoxic. PA is toxic in the liver through cytochrome P450 enzyme-catalyzed metabolic activation. Exposure to PA can cause liver damage such as liver sinusoidal obstruction syndrome (HSOS), cirrhosis, and cancer (Yokoyama et al., 2018; You et al., 2018). Lycopsamine is one of the pyrrolizidine alkaloid metabolites reported in the comfrey (Salehi et al., 2019). In this study, rosmarinic acid level influence the hepatotoxic level of comfrey beside the low level of lycopsamine (Table 2). Rosmarinic acid as phenolic metabolites in comfrey play a role for hepatoprotective profile (Touiss et al., 2021).
Rosmarinic acid is a diphenol metabolites found in many botanical drugs and spices and is considered a potential pharmaceutical natural product. Numerous reports have shown the hepatoprotective effect of rosmarinic acid through various mechanisms. These mechanisms include elimination or reduction of superoxide or peroxynitrite activity, reduction of indicators of liver toxicity such as aspartate aminotransferase, alanine aminotransferase, glutathione oxide, lipid peroxidation, and catalase, glutathione peroxidase, and super. Includes enzymatic activity associated with antioxidants such as oxidative dismutase. Rosmarinic acid also inhibited hepatic proliferation, TGFβ1, CTGF and αSMA expression in cultured HSCs. It reduced the degree of fibrosis and improved biochemical indicators and histopathological morphology. It also had a significant impact on inflammation and some inflammatory mediators (Elufioye and Habtemariam, 2019).
The betaine-urea-based NADES extraction was the better option for extracting rosmarinic acid and lycopsamine from comfrey (Symphytum officinale L.), with levels of 1.934 mg/g and 0.018 mg/g, respectively. The antioxidant, anti-inflammatory and hepatotoxic profile of betaine-urea NADES extraction was found to be considerable. Rosmarinic acid was shown to have antioxidant and anti-inflammatory chemical profile but also less hepatotoxic. Selective extraction towards rosmarinic acid over lycopsamine allows detoxification comfrey leaves, to further the investigation into the utilization of comfrey leaves as anti-inflammatory and antioxidant agent.
The original contributions presented in the study are included in the article/supplementary material, further inquiries can be directed to the corresponding author.
Conceptualization, AM, HS and ANS; methodology, AM and ANS; software, ANS; validation, HS, HH and AM; formal analysis, ANS, AM and HS; investigation, ANS; resources, ANS and AM; data curation, ANS; writing—original draft preparation, ANS; writing—literature review, manuscript review and editing, AS, AM and HS; visualization, ANS and AS; supervision, HS and AM; project administration, AM; funding acquisition, AM All authors have read and agreed to the published version of the manuscript.
This study was supported by the research grant from the Republic of Indonesia’s Ministry of Research, Technology, and Higher Education (grant number NKB-374/UN2.RST/HKP.05.00/2021).
The authors would like to acknowledge the support from The Indonesian Ministry of Research, Technology, and Higher Education through World Class Research scheme, grant number NKB-374/UN2.RST/HKP.05.00/2021 and Badan Riset dan Inovasi Nasional (BRIN) through the National Metabolomics Collaborative Research Center, grant number 151/PKS/WRIII-DISTP/UI/2022. Authors would like to acknowledge scientific and technical support from Advanced Characterization Laboratories Bandung, National Research and Innovation Agency ELayanan Sains.
The authors declare that the research was conducted in the absence of any commercial or financial relationships that could be construed as a potential conflict of interest.
All claims expressed in this article are solely those of the authors and do not necessarily represent those of their affiliated organizations, or those of the publisher, the editors and the reviewers. Any product that may be evaluated in this article, or claim that may be made by its manufacturer, is not guaranteed or endorsed by the publisher.
Aldoghachi, F. E. H., Noor Al-Mousawi, U. M., and Shari, F. H. (2021). Antioxidant activity of rosmarinic acid extracted and purified from Mentha piperita. Arch. Razi Inst. 76 (5), 1279–1287. doi:10.22092/ari.2021.356072.1770
Barbieri, J. B., Goltz, C., Batistão Cavalheiro, F., Theodoro Toci, A., Igarashi-Mafra, L., and Mafra, M. R. (2020). Deep eutectic solvents applied in the extraction and stabilization of rosemary (Rosmarinus officinalis L.) phenolic compounds. Ind. Crops Prod. 144, 112049. doi:10.1016/j.indcrop.2019.112049
Benedec, D., Hanganu, D., Oniga, I., Tiperciuc, B., Olah, N-K., Raita, O., et al. (2015). Assessment of rosmarinic acid content in six Lamiaceae species extracts and their antioxidant and antimicrobial potential. Pak J. Pharm. Sci. 28 (6), 2297–2303. Available at: http://www.ncbi.nlm.nih.gov/pubmed/26687747.
Boşca, A. B., Dinte, E., Colosi, H., Ilea, A., Câmpian, R. S., Uifălean, A., et al. (2015). Curcumin effect on nitro-oxidative stress in ligature-induced rat periodontitis. Rom. Biotechnol. Lett. 20 (4), 10708–10717.
Cai, C., Li, F., Liu, L., and Tan, Z. (2019). Deep eutectic solvents used as the green media for the efficient extraction of caffeine from Chinese dark tea. Sep. Purif. Technol. 227, 115723. doi:10.1016/j.seppur.2019.115723
Cao, J., Cao, J., Wang, H., Chen, L., Cao, F., and Su, E. (2020). Solubility improvement of phytochemicals using (natural) deep eutectic solvents and their bioactivity evaluation. J. Mol. Liq. 318, 113997. doi:10.1016/j.molliq.2020.113997
Cao, Y., Colegate, S. M., and Edgar, J. A. (2008). Safety assessment of food and herbal products containing hepatotoxic pyrrolizidine alkaloids: Interlaboratory consistency and the importance of N -oxide determination. Phytochem. Anal. 19 (6), 526–533. doi:10.1002/pca.1079
Colica, C., Di Renzo, L., Aiello, V., De Lorenzo, A., and Abenavoli, L. (2018). Rosmarinic acid as potential anti-inflammatory agent. Rev. Recent Clin. Trials 13 (4), 240–242. doi:10.2174/157488711304180911095818
Cysewski, P., and Jeliński, T. (2019). Optimization, thermodynamic characteristics and solubility predictions of natural deep eutectic solvents used for sulfonamide dissolution. Int. J. Pharm. 570, 118682. doi:10.1016/j.ijpharm.2019.118682
Dai, Y., van Spronsen, J., Witkamp, G., Verpoorte, R., and Choi, Y. H. (2013). Natural deep eutectic solvents as new potential media for green technology. Anal. Chim. Acta 766, 61–68. doi:10.1016/j.aca.2012.12.019
Dresler, S., Szymczak, G., and Wójcik, M. (2017). Comparison of some secondary metabolite content in the seventeen species of the boraginaceae family. Pharm. Biol. 55 (1), 691–695. doi:10.1080/13880209.2016.1265986
Duan, L., Dou, L-L., Guo, L., Li, P., and Liu, E-H. (2016). Comprehensive evaluation of deep eutectic solvents in extraction of bioactive natural products. ACS Sustain Chem. Eng. 4 (4), 2405–2411. doi:10.1021/acssuschemeng.6b00091
Elufioye, T. O., and Habtemariam, S. (2019). Hepatoprotective effects of rosmarinic acid: Insight into its mechanisms of action. Biomed. Pharmacother. 112, 108600. doi:10.1016/j.biopha.2019.108600
Francenia Santos-Sánchez, N., Salas-Coronado, R., Villanueva-Cañongo, C., and Hernández-Carlos, B. (2019).”Antioxidant compounds and their antioxidant mechanism” in Antioxidants (London: IntechOpen), 1–28. Available at: https://www.intechopen.com/books/antioxidants/antioxidant-compounds-and-their-antioxidant-mechanism.
Frost, R., MacPherson, H., and O’Meara, S. (2013). A critical scoping review of external uses of comfrey (Symphytum spp.). Complement. Ther. Med. 21 (6), 724–745. doi:10.1016/j.ctim.2013.09.009
Frost, R., O’Meara, S., and MacPherson, H. (2014). The external use of comfrey: A practitioner survey. Complement. Ther. Clin. Pract. [Internet] 20 (4), 347–355. doi:10.1016/j.ctcp.2014.07.003
Fu, X., Wang, D., Belwal, T., Xu, Y., Li, L., and Luo, Z. (2021). Sonication-synergistic natural deep eutectic solvent as a green and efficient approach for extraction of phenolic compounds from peels of Carya cathayensis Sarg. Food Chem. 355, 129577. doi:10.1016/j.foodchem.2021.129577
Heendeniya, S. N., Ratnasooriya, W. D., and Pathirana, R. N. (2018). In vitro investigation of anti-inflammatory activity and evaluation of phytochemical profile of Syzygium caryophyllatum. J. Pharmacogn. Phytochem. 7 (1), 1759–1763.
Janes, D., Kalamar, B., and Kreft, S. (2012). Improved method for isolation of lycopsamine from roots of comfrey (Symphytum officinale). Nat. Prod. Commun. 7 (7), 1934578X1200700–2. doi:10.1177/1934578X1200700713
Jeong, K. M., Jin, Y., Yoo, D. E., Han, S. Y., Kim, E. M., and Lee, J. (2018). One-step sample preparation for convenient examination of volatile monoterpenes and phenolic compounds in peppermint leaves using deep eutectic solvents. Food Chem. 251, 69–76. doi:10.1016/j.foodchem.2018.01.079
Jurić, T., Mićić, N., Potkonjak, A., Milanov, D., Dodić, J., Trivunović, Z., et al. (2021). The evaluation of phenolic content, in vitro antioxidant and antibacterial activity of Mentha piperita extracts obtained by natural deep eutectic solvents. Food Chem. 362, 130226. doi:10.1016/j.foodchem.2021.130226
Kalhor, P., and Ghandi, K. (2019). Deep eutectic solvents for pretreatment, extraction, and catalysis of biomass and food waste. Molecules 24 (22), 4012. doi:10.3390/molecules24224012
Kanedi, M., Sutyarso, , Busman, H., Nurcahyani, N., and Nurkhasanah, W. (2017). Root extract of purwoceng (pimpinella pruatjan) enhances aggressiveness, but not libido, in male mice. Annu. Res. Rev. Biol. 20 (2), 1–6. doi:10.9734/arrb/2017/36975
Kementerian Kesehatan Republik Indonesia (2017). Farmakope herbal Indonesia. II. Jakarta: Majalah farmasi nasional, 530–531.
Kinho, J., Arini, D. I. D., Tabba, S., Kama, H., Kafiar, Y., Shabri, S., et al. (2011). Tumbuhan obat tradisional di Sulawesi Utara jilid 1. (Traditional medicinal plants in North Sulawesi) [Internet]. Available at: https://www.forda-mof.org/files/Tumbuhan-Obat-Tradisional-di-Sulut-Jilid-I-pdf.
Liu, F., Wan, S. Y., Jiang, Z., Li, S. F. Y., Ong, E. S., and Osorio, J. C. C. (2009). Determination of pyrrolizidine alkaloids in comfrey by liquid chromatography–electrospray ionization mass spectrometry. Talanta 80 (2), 916–923. doi:10.1016/j.talanta.2009.08.020
Liu, Y., Li, J., Fu, R., Zhang, L., Wang, D., and Wang, S. (2019). Enhanced extraction of natural pigments from Curcuma longa L. using natural deep eutectic solvents. Ind. Crops Prod. 140, 111620. doi:10.1016/j.indcrop.2019.111620
Mansinhos, I., Gonçalves, S., Rodríguez-Solana, R., Ordóñez-Díaz, J. L., Moreno-Rojas, J. M., and Romano, A. (2021). Ultrasonic-assisted extraction and natural deep eutectic solvents combination: A green strategy to improve the recovery of phenolic compounds from lavandula pedunculata subsp. lusitanica (chaytor) franco. Antioxidants (Basel). 10 (4), 582. doi:10.3390/antiox10040582
Moreira, R., Pereira, D., Valentão, P., and Andrade, P. (2018). Pyrrolizidine alkaloids: Chemistry, Pharmacology, toxicology and food safety. Int. J. Mol. Sci. 19 (6), 1668. doi:10.3390/ijms19061668
Neagu, E., Roman, G. P., and Radu, G. L. (2010). Antioxidant capacity of some Symphytum officinalis extracts processed by ultrafiltration. Rom. Biotechnol. Lett. 15 (4), 5505–5511.
Obluchinskaya, E. D., Pozharitskaya, O. N., Zakharova, L. V., Daurtseva, A. V., Flisyuk, E. V., and Shikov, A. N. (2021). Efficacy of natural deep eutectic solvents for extraction of hydrophilic and lipophilic compounds from fucus vesiculosus. Molecules 26 (14), 4198. doi:10.3390/molecules26144198
Oliviu, V., Simona, C., Cristina, M., Claudia Crina, T., Borza;, C. C., and Laurian, V. (2017). Anti-inflammatory and antinociceptive effect of Symphytum officinale root. Rom. Biotechnol. Lett.
Osman, N., Sidik, N., Awal, A., Adam, N., and Rezali, N. (2016). In vitro xanthine oxidase and albumin denaturation inhibition assay of Barringtonia racemosa L. and total phenolic content analysis for potential anti-inflammatory use in gouty arthritis. J. Intercult. Ethnopharmacol. 5 (4), 343–349. doi:10.5455/jice.20160731025522
Öztürk, N., Tunçel, M., Uysal, U. D., Oncu-Kaya, E. M., and Koyuncu, O. (2011). Determination of rosmarinic acid by high-performance liquid chromatography and its application to certain salvia species and rosemary. Food Anal. Methods 4 (3), 300–306. doi:10.1007/s12161-010-9164-2
Ruesgas-Ramón, M., Figueroa-Espinoza, M. C., and Durand, E. (2017). Application of deep eutectic solvents (DES) for phenolic compounds extraction: Overview, challenges, and opportunities. J. Agric. Food Chem. 65 (18), 3591–3601. doi:10.1021/acs.jafc.7b01054
Salehi, B., Sharopov, F., Boyunegmez Tumer, T., Ozleyen, A., Rodríguez-Pérez, C., Ezzat, S. M., et al. (2019). Symphytum species: A comprehensive review on chemical composition, food applications and phytopharmacology. Molecules 24 (12), 2272. doi:10.3390/molecules24122272
Scheckel, K. A., Degner, S. C., and Romagnolo, D. F. (2008). Rosmarinic acid antagonizes activator protein-1-dependent activation of cyclooxygenase-2 expression in human cancer and nonmalignant cell lines. J. Nutr. 138 (11), 2098–2105. doi:10.3945/jn.108.090431
Seigner, J., Junker-Samek, M., Plaza, A., D‘Urso, G., Masullo, M., Piacente, S., et al. (2019). A Symphytum officinale root extract exerts anti-inflammatory properties by affecting two distinct steps of NF-κB signaling. Front. Pharmacol. 10, 289. doi:10.3389/fphar.2019.00289
Shawky, E., Takla, S. S., Hammoda, H. M., and Darwish, F. A. (2018). Evaluation of the influence of green extraction solvents on the cytotoxic activities of Crinum (Amaryllidaeae) alkaloid extracts using in-vitro-in-silico approach. J. Ethnopharmacol 227, 139–149. doi:10.1016/j.jep.2018.08.040
Smith, D. B., and Jacobson, B. H. (2011). Effect of a blend of comfrey root extract (Symphytum officinale L.) and tannic acid creams in the treatment of osteoarthritis of the knee: Randomized, placebo-controlled, double-blind, multiclinical trials. J. Chiropr. Med. [Internet] 10 (3), 147–156. doi:10.1016/j.jcm.2011.01.003
Smith, E. L., Abbott, A. P., and Ryder, K. S. (2014). Deep eutectic solvents (DESs) and their applications. Chem. Rev. 114 (21), 11060–11082. doi:10.1021/cr300162p
Syakfanaya, A. M., Saputri, F. C., and Munim, A. (2019). Simultaneously extraction of caffeine and chlorogenic acid from Coffea canephora bean using natural deep eutectic solvent-based ultrasonic assisted extraction. Pharmacogn. J. 11 (2), 267–271. doi:10.5530/pj.2019.11.41
Syarifah, A. N., Suryadi, H., and Mun’im, A. (2022). Validation of rosmarinic acid quantification using high- performance liquid chromatography in various plants. Pharmacogn. J. 14 (1), 165–171. Available at: https://phcogj.com/article/1750.
Tawfeeq, A. A., Mahdi, M. F., Abaas, I. S., and Alwan, A. H. (2018). Isolation, quantification, and identification of rosmarinic acid, gas chromatography-mass spectrometry analysis of essential oil, cytotoxic effect, and antimicrobial investigation of Rosmarinus officinalis leaves. Asian J. Pharm. Clin. Res. 11 (6), 126. doi:10.22159/ajpcr.2018.v11i6.24134
Touiss, I., Ouahhoud, S., Harnafi, M., Khatib, S., Bekkouch, O., Amrani, S., et al. (2021). Toxicological evaluation and hepatoprotective efficacy of rosmarinic acid-rich extract from ocimum basilicum L. Evidence-Based Complement. Altern. Med. 2021, 6676998. doi:10.1155/2021/6676998
Trifan, A., Opitz, S. E. W., Josuran, R., Grubelnik, A., Esslinger, N., Peter, S., et al. (2018). Is comfrey root more than toxic pyrrolizidine alkaloids? Salvianolic acids among antioxidant polyphenols in comfrey (Symphytum officinale L.) roots. Food Chem. Toxicol. 112, 178–187. doi:10.1016/j.fct.2017.12.051
Vinatoru, M., Mason, T. J., and Calinescu, I. (2017). Ultrasonically assisted extraction (UAE) and microwave assisted extraction (MAE) of functional compounds from plant materials. Trac. - Trends Anal. Chem. 97, 159–178. doi:10.1016/j.trac.2017.09.002
Vongsak, B., Kongkiatpaiboon, S., Jaisamut, S., and Konsap, K. (2018). Comparison of active constituents, antioxidant capacity, and α-glucosidase inhibition in Pluchea indica leaf extracts at different maturity stages. Food Biosci. 25, 68–73. doi:10.1016/j.fbio.2018.08.006
Wilkinson, J. (2003). A laboratory evaluation of comfrey (Symphytum officinale L.) as a forage crop for ensilage. Anim. Feed Sci. Technol. 104 (1–4), 227–233. doi:10.1016/s0377-8401(02)00293-6
Yokoyama, Y., Sasaki, Y., Terasaki, N., Kawataki, T., Takekawa, K., Iwase, Y., et al. (2018). Comparison of drug metabolism and its related hepatotoxic effects in HepaRG, cryopreserved human hepatocytes, and HepG2 cell cultures. Biol. Pharm. Bull. 41 (5), 722–732. doi:10.1248/bpb.b17-00913
You, L., Dong, X., Yin, X., Yang, C., Leng, X., Wang, W., et al. (2018). Rhein induces cell death in HepaRG cells through cell cycle arrest and apoptotic pathway. Int. J. Mol. Sci. 19 (4), 1060. doi:10.3390/ijms19041060
Zhang, Q. W., Lin, L. G., and Ye, W. C. (2018). Techniques for extraction and isolation of natural products: A comprehensive review. Chin. Med. (United Kingdom) 13 (1), 20–26. doi:10.1186/s13020-018-0177-x
Zsuzsanna, R., Assessor, B-S., and Csupor, D. (2015). Assessment report on Symphytum officinale L., radix. Eur. Med. Agency 44, 1–27. Available at: http://www.ema.europa.eu/docs/en_GB/document_library/Herbal_-_HMPC_assessment_report/2015/06/WC500187600.pdf.
Keywords: Symphytum officinale, comfrey, rosmarinic acid, lycopsamine, NADES, HPLC-DAD, detoxification, bioactivities
Citation: Syarifah AN, Suryadi H, Hayun H, Simamora A and Mun’im A (2023) Detoxification of comfrey (Symphytum officinale L.) extract using natural deep eutectic solvent (NADES) and evaluation of its anti-inflammatory, antioxidant, and hepatoprotective properties. Front. Pharmacol. 14:1012716. doi: 10.3389/fphar.2023.1012716
Received: 05 August 2022; Accepted: 10 February 2023;
Published: 03 March 2023.
Edited by:
Cecilia Faraloni, National Research Council (CNR), ItalyReviewed by:
Latifa Bouissane, Université Sultan Moulay Slimane, MoroccoCopyright © 2023 Syarifah, Suryadi, Hayun, Simamora and Mun’im. This is an open-access article distributed under the terms of the Creative Commons Attribution License (CC BY). The use, distribution or reproduction in other forums is permitted, provided the original author(s) and the copyright owner(s) are credited and that the original publication in this journal is cited, in accordance with accepted academic practice. No use, distribution or reproduction is permitted which does not comply with these terms.
*Correspondence: Abdul Mun’im, bXVuaW1AZmFybWFzaS51aS5hYy5pZA==
Disclaimer: All claims expressed in this article are solely those of the authors and do not necessarily represent those of their affiliated organizations, or those of the publisher, the editors and the reviewers. Any product that may be evaluated in this article or claim that may be made by its manufacturer is not guaranteed or endorsed by the publisher.
Research integrity at Frontiers
Learn more about the work of our research integrity team to safeguard the quality of each article we publish.