- 1Medicine School, Changsha Social Work College, Changsha, Hunan, China
- 2Department of Respiratory and Critical Care Medicine, The First People’s Hospital of Huaihua, Affiliated to University of South China, Huaihua, Hunan, China
Endothelial cells, which are important metabolic and endocrine cells, play an important role in regulating vascular function. The occurrence and development of various cardiovascular and cerebrovascular diseases are associated with endothelial dysfunction. However, the underlying mechanism of vascular endothelial injury is not fully understood. It has been reported that the mechanism of endothelial injury mainly involves inflammation and oxidative stress. Moreover, endothelial progenitor cells are regarded as important contributors in repairing damaged endothelium. Multiple interventions (including chemical drugs and traditional Chinese medicines) exert endothelial protection by decreasing the release of inducing factors, suppressing inflammation and oxidative stress, and preventing endothelial cell senescence.
Introduction
Vascular endothelial cells are a single layer of flat epithelial cells located on the inner surface of the vascular lumen. They are distributed throughout large blood vessels and microvessels, and are important metabolic and endocrine organs. Vascular endothelial cells maintain circulatory stability, regulate vascular tone, and play an important role in anticoagulation and prevention of thrombosis (Bach, 2015). Vascular endothelial injury is seen in a variety of cardiovascular and cerebrovascular diseases, such as atherosclerosis, hypertension, and diabetic vascular disease, and is considered the initiating link of these diseases. Therefore, it is important to explore the factors and mechanisms of damaged endothelium, and to study the role and mechanism of changes in secreted active substances (Gimbrone and Garcia-Cardena, 2016). Endothelial cells possess the ability to proliferate and repair cell damage. Thus, endothelial cells are always in the dynamic process of being damaged and resisting damage, i.e., being repaired (Mazini et al., 2020). Considering the important role of vascular endothelium in the occurrence and development of dilemmas, the idea of interventions (drugs and biologics) to prevent and treat diseases is to facilitate the protection of endothelium and promote its repair (Miyamoto et al., 2014; Meng et al., 2018).
Endothelial injury and cardiovascular and cerebrovascular diseases
Clinical studies and animal experiments have shown that a variety of cardiovascular and cerebrovascular diseases (chronic cardiac insufficiency, diabetic vascular complications, stroke) are accompanied by endothelial cell dysfunction (Jara and Mezzano, 2008; Moon, 2021). In clinical studies, surface high-frequency ultrasound may be used to detect brachial artery blood flow–mediated endothelium-dependent dilation (FMD) and non–endothelium-dependent diastolic function (nitroglycerin-mediated dilation, NMD) to detect nitric oxide in blood (nitric oxide, NO), von Willebrand factor (vWF), asymmetric dimetylarginine (ADMA), and other indirect indicators of endothelial function (Arslan et al., 2017; Mortensen et al., 2019; Stepanova et al., 2019). The morphological changes of endothelial injury have been observed and endothelial NO levels and endothelial NO synthase (eNOS) activity were detected in different experimental animal models (hypertension, atherosclerosis, diabetes) (Kietadisorn et al., 2012).
It has been revealed that various factors, such as oxidized low-density lipoprotein (ox-LDL), hyperglycemia, homocysteine (Hcy), hypoxia, hydrogen peroxide (H2O2), and reactive aldehydes, induce endothelial injury. This has been confirmed by exogenous application of these factors in animal experiments and cultured endothelial cells to directly damage the endothelium (endothelial cells) (Li et al., 2016; Caliceti et al., 2017; Nègre-Salvayre et al., 2017; Yang et al., 2020).
Mechanism of endothelial cell injury
The mechanism of vascular endothelial cell injury is not fully understood. During the process of vascular endothelial injury, there are changes in vasodilation function, abnormal production and secretion of active substances, energy metabolism disorders, and morphological changes, and the underlying pathophysiological mechanism mainly involves inflammatory response and oxidative stress (Schinzari et al., 2017).
Inflammation and endothelial cell injury
A large number of studies have confirmed that the underlying pathophysiological mechanism of cardiovascular diseases, including atherosclerosis, involves inflammation (Zhong et al., 2019). Literature reports have demonstrated that atherosclerosis is a chronic inflammatory disease (Ross, 1999), with the characteristic of inflammatory cell infiltration and secretion of various inflammatory factors, including TNF-α, IL-6, and IL-1β (Fan et al., 2013; Jiang et al., 2019). Uncontrolled inflammation is also found in other metabolic diseases, including diabetes-induced vascular inflammation (Stepanova et al., 2019).
In addition to directly attacking vascular endothelium, oxidative stress contributes to inducing inflammation (Domingueti et al., 2016; Bai et al., 2020), which has been confirmed in a variety of cardio- and cerebrovascular diseases, such as atherosclerosis (e.g., ox-LDL), diabetes (e.g., high glucose and glycosylation end products), and hypertension (angiotensin II [Ang II]) (Pleskovič et al., 2017; Solis et al., 2021). Further research has shown that the mechanism of inflammatory response involves a variety of microRNAs (miR-126, miR-155, miR-221/222, miR-31, miR-17-3p, miR-10a, miR-663, miR-125a-5p, and miR-125b-5p) by regulating downstream target proteins (such as VCAM-1, RGS16, Ets-1, AT1R, E-selectin, ICAM-1, MAP3K7, and βTRC) (Forouzanfar and Asgharzade, 2020; Wu et al., 2020; Luan et al., 2022).
Atherosclerosis and other cardiovascular and cerebrovascular diseases show endothelial cell aging, and endothelial cells can secrete a series of inflammatory factors (such as TNF-α, IL-1β, IL-2, IL-6, IL- 8, RANTES, ICAM, VCAM), known as “aging inflammation,” which further aggravates endothelial injury (Montgolfier, 2019).
The following functional proteins have been investigated in the context of inflammatory response: ① NF-κB: It is the central link and common pathway of inflammatory response during endothelial injury. Many stimuli can activate the NF-κB signal transduction pathway and induce upregulation of the gene expression of inflammation-related cytokines. Recent studies have shown that NF-κB activity is regulated by epigenetics, such as PCB upregulation through epigenetics NF-κB subunit p65 expression induces endothelial inflammation (Zhu and Hou, 2021). ② High-mobility group protein 1 (HMGB1): It is a new type of inflammatory mediators and is associated with cardiovascular diseases (atherosclerosis, acute coronary syndrome, pulmonary hypertension) and closely related diseases (Jeong et al., 2019; Wahid et al., 2021). In the development of atherosclerosis, HMGB1 mediates the expression of proinflammatory mediators of endothelial cells during the initial stage of plaque formation, including TNF-α, IL-8, MCP-1, adhesion molecules (ICAM-1, VCAM-1), MIP-1α, and MIP-1β (Hebbel et al., 2020). In vitro experiments have shown that HMGB1 induces inflammatory responses through the TLR4 and IRF3 pathways (Florim et al., 2020). ③ Inflammasome: It is a newly discovered large molecular multiprotein complex with a molecular weight of 100 kDa, which is involved in atherosclerosis, ischemia–reperfusion injury, and type 2 diabetes. IL-1β is regarded as a pivotal inflammatory mediator, and its activation and secretion are regulated by inflammasomes (Palomo et al., 2015; Yu, 2016). In the process of inflammatory response, IL-1β induces the binding of intracellular Pro-IL-1β and inflammasome-related protein nucleotides. Increased synthesis of nucleotide-binding NLRP3 induces inflammasome assembly and activates Caspase-1, which cleaves Pro-IL-1β to generate activated IL-1β (Dinarello, 2011; Fahey and Doyle, 2019).
Oxidative stress and endothelial cell injury
It has been demonstrated that oxidative stress is an important mechanism involved in endothelial injury in atherosclerosis, diabetes, hypertension, and myocardial infarction (Ungvári et al., 2005; Husain et al., 2015). Various factors such as ox-LDL, Ang II, ADMA, hypoxia, high glucose, and reactive aldehydes can induce the generation of reactive oxygen species (ROS) (superoxide anion (O2−), H2O2, hydroxyl free (OH), hypochlorous acid (HOCl), and peroxynitrite (ONOOO-)), through direct or indirect injury to endothelial cells (Shaw et al., 2014). It is worth mentioning that the eNOS inhibitor ADMA competitively inhibits NOS and decouples it, so that it no longer catalyzes the production of NO by L-arginine, but induces O2− production to promote oxidative stress (Jiang et al., 2006; Tan et al., 2007; Yuan et al., 2015). There is accumulating evidence that the causes of ROS accumulation are associated with the following elements: ① decreased activity of ROS-scavenging enzymes, such as superoxide dismutase, catalase, and glutathione peroxidase (O'Flaherty, 2019); ② increased activity of enzymes that catalyze the generation of ROS, such as peroxidase, xanthine oxidase, monoamine oxidase, and NADPH oxidase. Among them, peroxidase is a class of heme-containing enzymes that catalyzes H2O2 (weak oxidant) into HOCl (Awad et al., 2018). Previous studies on peroxidase have focused on myeloperoxidase (MPO), which is expresses in neutrophils and monocytes. In recent years, an isoenzyme of MPO has been discovered, which is 44.5% identical to MPO. In addition to being present in the heart, liver, and pancreas, it is highly expressed in vascular endothelial cells and vascular smooth muscle cells, which is why it is also known as vascular peroxide. Changes in the activity of vascular peroxidase (VPO) are closely related to endothelial injury in atherosclerosis, diabetes, and myocardial I/R injury (Bai et al., 2011; Awad et al., 2018).
Vascular aging often occurs in atherosclerosis, diabetes, coronary heart disease, and other cardiovascular and cerebrovascular diseases (Ma et al., 2013; Ishii et al., 2020). Hypoxia, ox-LDL, high glucose, and other factors can increase the expression of aging-related proteins such as p53, thereby resulting in endothelial cell aging (Tian and Li, 2014). Recently, it has been shown in diabetic rats and endothelial cells in a high glucose–induced injury model that the expression of VPO1 is upregulated, and that endothelial cells are senescent (Liu et al., 2015). Silencing the VPO1 gene could significantly attenuate endothelial senescence. The exogenous application of HOCl could directly induce endothelial senescence, which suggests that the VPO1/HOCl pathway plays an important role in oxidative stress–induced endothelial cell aging (Yang et al., 2017). There are several lines of evidence that various factors, including oxidative stress, DNA damage, and genotoxic drugs, could induce cell senescence (Shokrzadeh et al., 2021). The mechanism involves regulating senescence-related miRNAs, which in turn regulate the expression of downstream target proteins and promote ROS generation, thereby leading to vascular aging. Overexpression of miR-146a in endothelial cells could significantly inhibit the expression of NADPH oxidase 4, and reduce the generation of ROS and endothelial senescence (Xiao et al., 2021). miR217 and miR-34a could cause downregulation on silent information regulator 1 (SIRT1) mRNA and protein, weaken antioxidant capacity, and deteriorate vascular endothelial aging (Zhang et al., 2020; Matacchione et al., 2021).
Previous studies have confirmed that ROS can promote endothelial cell morphological damage and induce apoptosis (Strilic et al., 2016). Necroptosis (also known as programmed necrosis) is a newly discovered type of cell death, which is found in the pathological process of I/R injury in the heart, kidney, brain, and retina. The main mechanism involves the interaction of TNF-α with TNF receptor 1 on the cell surface, which is mediated by the RIP1/RIP3/MLKL necrosis complex. Recent studies have shown that a variety of tumor cells (human lung adenocarcinoma cell line A549, human neuroblastoma cell SH-SY5Y) co-cultured with endothelial cells can induce endothelial cell necroptosis (Lubrano and Balzan, 2014; Chen et al., 2016).
Repair and mechanism of damaged vascular endothelium
Endothelial cells possess the ability to self-proliferate and repair. Vascular endothelial injury not only affects the function of the vascular barrier and the regulatory function and secretory function of the vasodilator response, but also weakens the repair ability. Endothelial cells can slow down or even stop their own natural aging process by reducing or preventing the damage of endothelial cells and facilitating the repair of the damaged endothelial cells.
Endothelial progenitor cells and endothelial repair
Endothelial progenitor cells (EPCs) are stem cells that are homed to angiogenesis tissues. They can differentiate and proliferate into mature endothelial cells, and exert an important role in endothelial repair and angiogenesis. Several studies have demonstrated that the pathogenesis of various cardiovascular diseases (such as atherosclerosis and pulmonary hypertension) is associated with EPCs aging (Zhou et al., 2010a; Zhou et al., 2010b). After EPCs aging, their migration, adhesion function, and blood vessel formation ability are all reduced, resulting in weakened endothelial repair ability.
There are now three basic ways to treat endothelial damage with EPC: ①Transplantation of EPCs to endothelial injury sites to promote endothelial tissue regeneration and repair. It has been confirmed that the injection of EPCs into mice could significantly improve the damage of hepatic sinusoidal endothelial cells and hepatocytes while reducing the secretion of IL-6 and TNF-α, inhibiting platelet activation, and improving liver function (Qiao et al., 2015). ② The introduction of certain genes, such as calcitonin gene–related peptide (CGRP), into EPCs to enhance the protective effect of EPCs on endothelial cells. In animal experiments, EPCs transfected with CGRP in rats with pulmonary arterial hypertension can significantly improve pulmonary hypertension and reverse pulmonary vascular remodeling (Zhao et al., 2007). As shown in vitro, transfection of damaged EPCs into β2 adrenergic receptors could significantly improve the repair ability of EPCs on vascular endothelium (Ke et al., 2016). ③ Some drugs such as low-dose aspirin, resveratrol, rosiglitazone, pyrrolizone, and evodiamine can delay EPCs aging. For example, resveratrol-based derivative BTM-0512 inhibits EPCs aging in diabetic rats, and its mechanism of action involves the SIRTl-DDAH2/ADMA pathway (Yuan et al., 2015). CGRP mediates evodiamine and inhibits AngⅡ-induced EPC aging, and its mechanism is related to the upregulation of Klotho gene (Ming et al., 2016). Cisceral lipin delays ox-LDL–induced EPCs aging and upregulation of SIRT1 is involved, and the underlying mechanism involves the PI3K/Akt/ERK pathway (Xu et al., 2021).
Drugs and endothelial protection
Clinical studies and animal experiments have shown that many drugs, including chemical drugs and traditional Chinese medicines, have protective effects on endothelial cells, but the underlying mechanisms are not yet fully understood (Yang et al., 2005). This is because different studies have discussed the various entrance points of the mechanism of pharmacological protection of vascular endothelium, apart from the complexity of the pathological mechanism of vascular endothelial injury (Figure 1). There are various mechanisms by which drugs may protect the vascular endothelium: ① Reducing the generation of factors that induce endothelial cell damage: For example, lipid-lowering drugs, hypoglycemic drugs, and anti-myocardial ischemia drugs can reduce the production of blood sugar, ox-LDL, ROS, and inflammatory factors. Folic acid can inhibit Hcy production, which is an adjunct therapy for hyperhomocysteinemia-type hypertension, helps protect vascular endothelium, and reduces the incidence of stroke (Haklar et al., 1998). L-arginine can competitively prevent ADMA from inhibiting eNOS. Vascular tension invertase inhibitors can reduce the production of Ang II. ② Reducing the formation of ROS by oxidative stress inhibitors such probucol, vitamin E, and tanshinone IIA (Tang et al., 2011; Jia et al., 2012; Tsai et al., 2014). ③Inhibiting the inflammatory response: For example, aspirin, fibrate lipid-lowering drugs, and resveratrol methyl derivatives may inhibit the production of inflammatory factors (Price et al., 2012; Wang et al., 2014; Jeong et al., 2015; Pan et al., 2016; Han et al., 2020). ④ Delaying aging of endothelial cells and EPCs: For example, rosiglitazone, evodiamine, and simvastatin can inhibit endothelial aging (Fraineau et al., 2015). EPC-based transcription is regulated by epigenetic regulation, including noncoding RNA (microRNA and IncRNA), DNA methylation, histone modification (histone methylation, acetylation, and deacetylation), and some compounds (such as peptide compound inhibitor 5-azacytidine). Epigenetics increases the proliferation and migration of EPCs and enhances the ability to repair blood vessels (Fraineau et al., 2015).
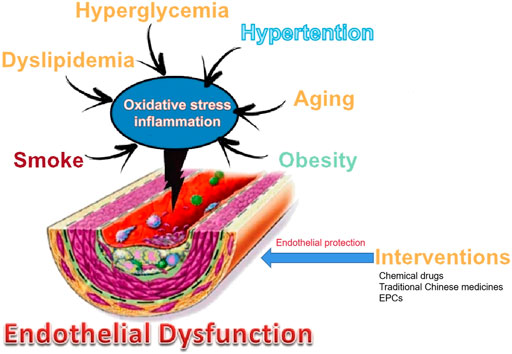
FIGURE 1. Main vascular risk factors and their involvement in endothelial dysfunction and intervention.
Conclusion
Vascular endothelial injury is the initiating link of various cardiovascular and cerebrovascular diseases. In addition to the changes in its own morphology and function, endothelial injury causes endothelial cells to secrete endogenous active substances and affect vascular smooth muscle, which affects vasodilation. It has also been demonstrated that a number of variables, including hypoxia and others, can cause endothelial interstitial change and promote vascular remodeling (Tian and Li, 2014). The exact mechanism of endothelial cell injury is not fully understood. Inflammation and oxidative stress are known as important pathophysiological mechanisms of endothelial injury. It is known that there is an interaction between inflammation and oxidative stress, but the network relationship of their interaction and its key molecules are yet to be elucidated. The aging and regulation mechanisms of endothelial cells and EPCs also need to be further explored. With the deepening of research on endothelial injury and repair, new targets for protecting vascular endothelium may be discovered, which will provide new ideas to find drugs to protect damaged endothelium. It has been proven that a variety of traditional Chinese medicines have protective effects on vascular endothelium, and the separation and purification of active ingredients and monomers in traditional Chinese medicine will be an important way to develop drugs for vascular endothelial protection.
Author contributions
YH analyzed, interpreted, and presented results for group discussions. YH and CS provided rationale, background, framework, and feedback. All authors have approved the manuscript. All authors have agreed with both to be personally accountable for the author’s contributions and to ensure that questions related to the accuracy or integrity of any part of the work.
Funding
This work was supported by Hunan Natural Science Foundation (grant no. 2020JJ7090).
Acknowledgments
We thank LetPub (www.letpub.com) for its linguistic assistance during the preparation of this manuscript.
Conflict of interest
The authors declare that the research was conducted in the absence of any commercial or financial relationships that could be construed as a potential conflict of interest.
Publisher’s note
All claims expressed in this article are solely those of the authors and do not necessarily represent those of their affiliated organizations, or those of the publisher, the editors and the reviewers. Any product that may be evaluated in this article, or claim that may be made by its manufacturer, is not guaranteed or endorsed by the publisher.
Supplementary material
The Supplementary Material for this article can be found online at: https://www.frontiersin.org/articles/10.3389/fphar.2022.997272/full#supplementary-material
References
Arslan, M., Yilmaz, G., Mentese, A., Yilmaz, H., Karahan, S. C., and Koksal, I. (2017). Importance of endothelial dysfunction biomarkers in patients with Crimean-Congo hemorrhagic fever. J. Med. Virol. 89 (12), 2084–2091. doi:10.1002/jmv.24881
Awad, M. A., Aldosari, S. R., and Abid, M. R. (2018). Genetic alterations in oxidant and anti-oxidant enzymes in the vascular system. Front. Cardiovasc. Med. 5, 107. doi:10.3389/fcvm.2018.00107
Bach, L. A. (2015). Endothelial cells and the IGF system. J. Mol. Endocrinol. 54 (1), R1–R13. doi:10.1530/JME-14-0215
Bai, B., Yang, Y., Wang, Q., Li, M., Tian, C., Liu, Y., et al. (2020). NLRP3 inflammasome in endothelial dysfunction. Cell Death Dis. 11 (9), 776. doi:10.1038/s41419-020-02985-x
Bai, Y. P., Hu, C. P., Yuan, Q., Peng, J., Shi, R. Z., Yang, T. L., et al. (2011). Role of VPO1, a newly identified heme-containing peroxidase, in ox-LDL induced endothelial cell apoptosis. Free Radic. Biol. Med. 51(8) (1), 1492–1500. doi:10.1016/j.freeradbiomed.2011.07.004
Caliceti, C., Rizzo, P., Ferrari, R., Fortini, F., Aquila, G., Leoncini, E., et al. (2017). Novel role of the nutraceutical bioactive compound berberine in lectin-like OxLDL receptor 1-mediated endothelial dysfunction in comparison to lovastatin. Nutr. Metab. Cardiovasc. Dis. 27 (6), 552–563. doi:10.1016/j.numecd.2017.04.002
Chen, D., Yu, J., and Zhang, L. (2016). Necroptosis: An alternative cell death program defending against cancer. Biochim. Biophys. Acta 1865 (2), 228–236. doi:10.1016/j.bbcan.2016.03.003
Dinarello, C. A. (2011). Interleukin-1 in the pathogenesis and treatment of inflammatory diseases. Blood 117 (14), 3720–3732. doi:10.1182/blood-2010-07-273417
Domingueti, C. P., Dusse, L. M., Md, C., de Sousa, L. P., Gomes, K. B., and Fernandes, A. P. (2016). Diabetes mellitus: The linkage between oxidative stress, inflammation, hypercoagulability and vascular complications. J. Diabetes Complicat. 30 (4), 738–745. doi:10.1016/j.jdiacomp.2015.12.018
Fahey, E., and Doyle, S. L. (2019). IL-1 family cytokine regulation of vascular permeability and angiogenesis. Front. Immunol. 10, 1426. doi:10.3389/fimmu.2019.01426
Fan, W., Cheng, K., Qin, X., Narsinh, K. H., Wang, S., Hu, S., et al. (2013). mTORC1 and mTORC2 play different roles in the functional survival of transplanted adipose-derived stromal cells in hind limb ischemic mice via regulating inflammation in vivo. Stem Cells 31 (1), 203–214. doi:10.1002/stem.1265
Florim, G. M. S., Caldas, H. C., Gonçalves, N. N., Bueno, G. O. B. E., Baptista, M. A. S. F., Fernandes-Charpiot, I. M. M., et al. (2020). Activation of HMGB1-TLR4 pathway and inflammasome contribute to enhanced inflammatory response in extended criteria and kidneys with KDPI ≥85. Transplantation 104 (4), 724–730. doi:10.1097/TP.0000000000003048
Forouzanfar, F., and Asgharzade, S. (2020). MicroRNAs in noise-induced hearing loss and their regulation by oxidative stress and inflammation. Curr. Drug Targets 21 (12), 1216–1224. doi:10.2174/1389450121666200615145552
Fraineau, S., Palii, C. G., Allan, D. S., and Brand, M. (2015). Epigenetic regulation of endothelial-cell-mediated vascular repair. FEBS J. 282 (9), 1605–1629. doi:10.1111/febs.131831
Gimbrone, M. J., and Garcia-Cardena, G. (2016). Endothelial cell dysfunction and the pathobiology of atherosclerosis. Circ. Res. 118 (4), 620–636. doi:10.1161/CIRCRESAHA.115.306301
Haklar, G., Sirikci, O., Ozer, N. K., and Yalcin, A. S. (1998). Measurement of reactive oxygen species by chemiluminescence in diet-induced atherosclerosis: Protective roles of vitamin E and probucol on different radical species. Int. J. Clin. Lab. Res. 28 (2), 122–126. doi:10.1007/s005990050031
Han, X., Jiang, G., and Shi, Q. (2020). Effects of antihyperglycemics on endothelial progenitor cells. Zhejiang Da Xue Xue Bao Yi Xue Ban. 49 (5), 629–636. doi:10.3785/j.issn.1008-9292.2020.10.13
Hebbel, R. P., Wei, P., Milbauer, L., Corban, M. T., Solovey, A., Kiley, J., et al. (2020). Abnormal endothelial gene expression associated with early coronary atherosclerosis. J. Am. Heart Assoc. 9 (14), e016134. doi:10.1161/JAHA.120.016134
Husain, K., Hernandez, W., Ansari, R. A., and Ferder, L. (2015). Inflammation, oxidative stress and renin angiotensin system in atherosclerosis. World J. Biol. Chem. 6 (3), 209–217. doi:10.4331/wjbc.v6.i3.209
Ishii, M., Kaikita, K., Sakamoto, K., Seki, T., Kawakami, K., Nakai, M., et al. (2020). Characteristics and in-hospital mortality of patients with myocardial infarction in the absence of obstructive coronary artery disease in super-aging society. Int. J. Cardiol. 301, 108–113. doi:10.1016/j.ijcard.2019.09.037
Jara, A., and Mezzano, S. (2008). Vascular damage in chronic kidney disease]. Rev. Med. Chil. 136 (11), 1476–1484. doi:10.4067/s0034-98872008001100016
Jeong, J., Lee, J., Lim, J., Cho, S., An, S., Lee, M., et al. (2019). Soluble RAGE attenuates AngII-induced endothelial hyperpermeability by disrupting HMGB1-mediated crosstalk between AT1R and RAGE. Exp. Mol. Med. 51 (9), 1–15. doi:10.1038/s12276-019-0312-5
Jeong, S. O., Son, Y., Lee, J. H., Cheong, Y. K., Park, S. H., Chung, H. T., et al. (2015). Resveratrol analog piceatannol restores the palmitic acid-induced impairment of insulin signaling and production of endothelial nitric oxide via activation of anti-inflammatory and antioxidative heme oxygenase-1 in human endothelial cells. Mol. Med. Rep. 12 (1), 937–944. doi:10.3892/mmr.2015.3553
Jia, L. Q., Yang, G. L., Ren, L., Chen, W. N., Feng, J. Y., Cao, Y., et al. (2012). Tanshinone IIA reduces apoptosis induced by hydrogen peroxide in the human endothelium-derived EA.hy926 cells. J. Ethnopharmacol. 143 (1), 100–108. doi:10.1016/j.jep.2012.06.007
Jiang, D. J., Jia, S. J., Yan, J., Zhou, Z., Yuan, Q., and Li, Y. J. (2006). Involvement of DDAH/ADMA/NOS pathway in nicotine-induced endothelial dysfunction. Biochem. Biophys. Res. Commun. 349 (2), 683–693. doi:10.1016/j.bbrc.2006.08.115
Jiang, S. J., Tsai, P. I., Peng, S. Y., Chang, C. C., Chung, Y., Tsao, H. H., et al. (2019). A potential peptide derived from cytokine receptors can bind proinflammatory cytokines as a therapeutic strategy for anti-inflammation. Sci. Rep. 9 (1), 2317. doi:10.1038/s41598-018-36492-z
Ke, X., Shu, X. R., Wu, F., Hu, Q. S., Deng, B. Q., Wang, J. F., et al. (2016). Overexpression of the beta 2AR gene improves function and re-endothelialization capacity of EPCs after arterial injury in nude mice. Stem Cell Res. Ther. 7 (1), 73. doi:10.1186/s13287-016-0335-y
Kietadisorn, R., Juni, R. P., and Moens, A. L. (2012). Tackling endothelial dysfunction by modulating NOS uncoupling: New insights into its pathogenesis and therapeutic possibilities. Am. J. Physiol. Endocrinol. Metab. 302 (5), E481–E495. doi:10.1152/ajpendo.00540.2011
Li, P., Yin, Y. L., Guo, T., Sun, X. Y., Ma, H., Zhu, M. L., et al. (2016). Inhibition of aberrant MicroRNA-133a expression in endothelial cells by statin prevents endothelial dysfunction by targeting GTP cyclohydrolase 1 in vivo. Circulation 134 (22), 1752–1765. doi:10.1161/CIRCULATIONAHA.116.017949
Liu, S. Y., Yuan, Q., Li, X. H., Hu, C. P., Hu, R., Zhang, G. G., et al. (2015). Role of vascular peroxidase 1 in senescence of endothelial cells in diabetes rats. Int. J. Cardiol. 197, 182–191. doi:10.1016/j.ijcard.2015.06.098
Luan, X., Zhou, X., Fallah, P., Pandya, M., Lyu, H., Foyle, D., et al. (2022). MicroRNAs: Harbingers and shapers of periodontal inflammation. Semin. Cell Dev. Biol. 124, 85–98. doi:10.1016/j.semcdb.2021.05.030
Lubrano, V., and Balzan, S. (2014). LOX-1 and ROS, inseparable factors in the process of endothelial damage. Free Radic. Res. 48 (8), 841–848. doi:10.3109/10715762.2014.929122
Ma, Q. L., Zhang, G. G., and Peng, J. (2013). Vascular peroxidase 1: A novel enzyme in promoting oxidative stress in cardiovascular system. Trends cardiovasc. Med. 23 (5), 179–183. doi:10.1016/j.tcm.2012.11.002
Matacchione, G., Gurău, F., Silvestrini, A., Tiboni, M., Mancini, L., Valli, D., et al. (2021). Anti-SASP and anti-inflammatory activity of resveratrol, curcumin and β-caryophyllene association on human endothelial and monocytic cells. Biogerontology 22 (3), 297–313. doi:10.1007/s10522-021-09915-0
Mazini, L., Rochette, L., Admou, B., Amal, S., and Malka, G. (2020). Hopes and limits of adipose-derived stem cells (ADSCs) and mesenchymal stem cells (MSCs) in wound healing. Int. J. Mol. Sci. 21 (4), 1306. doi:10.3390/ijms21041306
Meng, L. B., Chen, K., Zhang, Y. M., and Gong, T. (2018). Common injuries and repair mechanisms in the endothelial lining. Chin. Med. J. 131 (19), 2338–2345. doi:10.4103/0366-6999.241805
Ming, G. F., Tang, Y. J., Hu, K., Chen, Y., Huang, W. H., and Xiao, J. (2016). Visfatin attenuates the ox-LDL-induced senescence of endothelial progenitor cells by upregulating SIRT1 expression through the PI3K/Akt/ERK pathway. Int. J. Mol. Med. 38 (2), 643–649. doi:10.3892/ijmm.2016.2633
Miyamoto, N., Pham, L. D., Seo, J. H., Kim, K. W., Lo, E. H., and Arai, K. (2014). Crosstalk between cerebral endothelium and oligodendrocyte. Cell. Mol. Life Sci. 71 (6), 1055–1066. doi:10.1007/s00018-013-1488-9
Montgolfier, O. D. (2019). Philippe Pouliot, Marc-Antoine Gillis, Guylaine Ferland, Frédéric Lesage, Nathalie Thorin-Trescases, Éric Thorin. Systolic hypertension-induced neurovascular unit disruption magnifies vascular cognitive impairment in middle-age atherosclerotic LDLr-/-:hApoB+/+mice. Geroscience 41 (5), 511–532.
Moon, Y. (2021). Predictive and preventive mucosal communications in particulate matter exposure-linked renal distress. J. Pers. Med. 11 (2), 118. doi:10.3390/jpm11020118
Mortensen, L. A., Bistrup, C., Stubbe, J., Carlström, M., Checa, A., Wheelock, C. E., et al. (2019). Effect of spironolactone for 1 yr on endothelial function and vascular inflammation biomarkers in renal transplant recipients. Am. J. Physiol. Ren. Physiol. 317 (3), F529–F539. doi:10.1152/ajprenal.00025.2019
Nègre-Salvayre, A., Garoby-Salom, S., Swiader, A., Rouahi, M., and Pucelle, M. (2017). Proatherogenic effects of 4-hydroxynonenal. Free Radic. Biol. Med. 111, 127–139. doi:10.1016/j.freeradbiomed.2016.12.038
O'Flaherty, C. (2019). Orchestrating the antioxidant defenses in the epididymis. Andrology 7 (5), 662–668. doi:10.1111/andr.12630
Palomo, J., Dietrich, D., Martin, P., Palmer, G., and Gabay, C. (2015). The interleukin (IL)-1 cytokine family–Balance between agonists and antagonists in inflammatory diseases. Cytokine 76 (1), 25–37. doi:10.1016/j.cyto.2015.06.017
Pan, W., Yu, H., Huang, S., and Zhu, P. (2016). Resveratrol protects against TNF-α-induced injury in human umbilical endothelial cells through promoting sirtuin-1-induced repression of NF-KB and p38 MAPK. PLoS One 11 (1), e0147034. doi:10.1371/journal.pone.0147034e147 034
Pleskovič, A., Mš, L., Vujkovac, A. C., Nikolajević Starčević, J., Gazdikova, K., Caprnda, M., et al. (2017). C-reactive protein as a marker of progression of carotid atherosclerosis in subjects with type 2 diabetes mellitus. Vasa. 46 (3), 187–192. doi:10.1024/0301-1526/a000614
Price, E. T., Welder, G. J., and Zineh, I. (2012). Modulatory effect of fenofibrate on endothelial production of neutrophil chemokines IL-8 and ENA-78. Cardiovasc. Drugs Ther. 26 (2), 95–99. doi:10.1007/s10557-011-6368-7
Qiao, J., Qi, K., Chu, P., Mi, H., Yang, N., Yao, H., et al. (2015). Infusion of endothelial progenitor cells ameliorates liver injury in mice after haematopoietic stem cell transplantation. Liver Int. 35 (12), 2611–2620. doi:10.1111/liv.12849
Ross, R. (1999). Atherosclerosis–an inflammatory disease. N. Engl. J. Med. 340 (2), 115–126. doi:10.1056/NEJM199901143400207
Schinzari, F., Tesauro, M., and Cardillo, C. (2017). Endothelial and perivascular adipose tissue abnormalities in obesity-related vascular dysfunction: Novel targets for treatment. J. Cardiovasc. Pharmacol. 69 (6), 360–368. doi:10.1097/FJC.0000000000000469
Shaw, A., Doherty, M. K., Mutch, N. J., MacRury, S. M., and Megson, I. L. (2014). Endothelial cell oxidative stress in diabetes: A key driver of cardiovascular complications? Biochem. Soc. Trans. 42 (4), 928–933. doi:10.1042/BST20140113
Shokrzadeh, M., Bagheri, A., Ghassemi-Barghi, N., Rahmanian, N., and Eskandani, M. (2021). Doxorubicin and doxorubicin-loaded nanoliposome induce senescence by enhancing oxidative stress, hepatotoxicity, and in vivo genotoxicity in male Wistar rats. Naunyn. Schmiedeb. Arch. Pharmacol. 394 (8), 1803–1813. doi:10.1007/s00210-021-02119-w
Solis, J. G., Enciso Lopez, E. S., Bautista Santos, A., Anda Garay, J. C., Calixto Rodriguez, J. L., Moreno Alcantar, R., et al. (2021). Effect of antiviral agents on atherosclerosis in patients with chronic hepatitis C. Arch. Med. Res. 52 (7), 764–771. doi:10.1016/j.arcmed.2021.04.009
Stepanova, T. V., Ivanov, A. N., Tereshkina, N. E., Popyhova, E. B., and Lagutina, D. D. (2019). Markers of endothelial dysfunction: Pathogenetic role and diagnostic significance. Klin. Lab. Diagn. 64 (1), 34–41. doi:10.18821/0869-2084-2018-63-34-41
Strilic, B., Yang, L., Albarran-Juarez, J., Wachsmuth, L., Han, K., Muller, U. C., et al. (2016). Tumour-cell-induced endothelial cell necroptosis via death receptor 6 promotes metastasis. Nature 536 (7615), 215–218. doi:10.1038/nature19076
Tan, B., Jiang, D. J., Huang, H., Jia, S-J., Jiang, J-L., Hu, C-P., et al. (2007). Taurine protects against low-density lipoprotein-induced endothelial dysfunction by the DDAH/ADMA pathway. Vasc. Pharmacol. 46 (5), 338–345.
Tang, C., Xue, H. L., Bai, C. L., and Fu, R. (2011). Regulation of adhesion molecules expression in TNF-α-stimulated brain microvascular endothelial cells by tanshinone IIA: Involvement of NF-kappa B and ROS generation. Phytother. Res. 25 (3), 376–380. doi:10.1002/ptr.3278
Tian, X. L., and Li, Y. (2014). Endothelial cell senescence and age-related vascular diseases. J. Genet. Genomics 41 (9), 485–495. doi:10.1016/j.jgg.2014.08.001
Tsai, K. L., Huang, P. H., Kao, C. L., Leu, H. B., Cheng, Y. H., Liao, Y. W., et al. (2014). Aspirin attenuates vinorelbine-induced endothelial inflammation via modulating SIRT1/AMPK axis. Biochem. Pharmacol. 88 (2), 189–200. doi:10.1016/j.bcp.2013.12.005
Ungvári, Z., Gupte, S. A., Recchia, F. A., Bátkai, S., and Pacher, P. (2005). Role of oxidative-nitrosative stress and downstream pathways in various forms of cardiomyopathy and heart failure. Curr. Vasc. Pharmacol. 3 (3), 221–229. doi:10.2174/1570161054368607
Wahid, A., Chen, W., Wang, X., and Tang, X. (2021). High-mobility group box 1 serves as an inflammation driver of cardiovascular disease. Biomed. Pharmacother. 139, 111555. doi:10.1016/j.biopha.2021.111555
Wang, W., Bai, L., Qiao, H., Lu, Y., Yang, L., Zhang, J., et al. (2014). The protective effect of fenofibrate against TNF-α-induced CD40 expression through SIRT1-mediated deacetylation of NF-kappa B in endothelial cells. Inflammation 37 (1), 177–185. doi:10.1007/s10753-013-9728-6
Wu, Y. L., Li, H. F., Chen, H. H., and Lin, H. (2020). MicroRNAs as biomarkers and therapeutic targets in inflammation- and ischemia-reperfusion-related acute renal injury. Int. J. Mol. Sci. 21 (18), 6738. doi:10.3390/ijms21186738
Xiao, L., Gu, Y., Ren, G., Chen, L., Liu, L., Wang, X., et al. (2021). miRNA-146a mimic inhibits NOX4/P38 signalling to ameliorate mouse myocardial ischaemia reperfusion (I/R) injury. Oxid. Med. Cell. Longev. 2021, 6366254. doi:10.1155/2021/6366254
Xu, S., Ilyas, I., Little, P. J., Li, H., Kamato, D., Zheng, X., et al. (2021). Endothelial dysfunction in atherosclerotic cardiovascular diseases and beyond: From mechanism to pharmacotherapies. Pharmacol. Rev. 73 (3), 924–967. doi:10.1124/pharmrev.120.000096
Yang, C., Zhao, Y., Ren, D., and Yang, X. (2020). Protective effect of saponins-enriched fraction of gynostemma pentaphyllum against high choline-induced vascular endothelial dysfunction and hepatic damage in mice. Biol. Pharm. Bull. 43 (3), 463–473. doi:10.1248/bpb.b19-00805
Yang, F., Tan, H. M., and Wang, H. (2005). Hyperhomocysteinemia and atherosclerosis. Sheng Li Xue Bao 57 (2), 103–114.
Yang, W., Liu, Z., Xu, Q., Peng, H., Chen, L., Huang, X., et al. (2017). Involvement of vascular peroxidase 1 in angiotensin II-induced hypertrophy of H9c2 cells. J. Am. Soc. Hypertens. 11 (8), 519–529. doi:10.1016/j.jash.2016.08.002
Yu, Je-Wook (2016). Mitochondria and the NLRP3 inflammasome: Physiological and pathological relevance. Arch. Pharm. Res. 39 (11), 1503–1518. doi:10.1007/s12272-016-0827-4
Yuan, Q., Hu, C. P., Gong, Z. C., Bai, Y. P., Liu, S. Y., Li, Y. J., et al. (2015). Accelerated onset of senescence of endothelial progenitor cells in patients with type 2 diabetes mellitus: Role of dimethylarginine dimethylaminohydrolase 2 and asymmetric dimethylarginine. Biochem. Biophys. Res. Commun. 458 (4), 869–876. doi:10.1016/j.bbrc.2015.02.050
Zhang, C., Wang, H., and Yang, B. (2020). miR-146a regulates inflammation and development in patients with abdominal aortic aneurysms by targeting CARD10. Int. Angiol. 39 (4), 314–322. doi:10.23736/S0392-9590.20.04283-2
Zhao, Q., Liu, Z., Wang, Z., Yang, C., Liu, J., and Lu, J. (2007). Effect of prepro-calcitonin gene-related peptide-expressing endothelial progenitor cells on pulmonary hypertension. Ann. Thorac. Surg. 84 (2), 544–552. doi:10.1016/j.athoracsur.2007.03.067
Zhong, S., Li, L., Shen, X., Li, Q., Xu, W., Wang, X., et al. (2019). An update on lipid oxidation and inflammation in cardiovascular diseases. Free Radic. Biol. Med. 144, 266–278. doi:10.1016/j.freeradbiomed.2019.03.036
Zhou, Z., Peng, J., Wang, C. J., Li, D., Li, T. T., Hu, C. P., et al. (2010). Accelerated senescence of endothelial progenitor cells in hypertension is related to the reduction of calcitonin gene-related peptide. J. Hypertens. 28 (5), 931–939. doi:10.1097/HJH.0b013e3283399326
Zhou, Zhi, Hu, C. P., Wang, C. J., Li, T. T., Peng, J., and Li, Y. J. (2010). Calcitonin gene-related peptide inhibits angiotensin II-induced endothelial progenitor cells senescence through up-regulation of klotho expression. Atherosclerosis 213 (1), 92–101. doi:10.1016/j.atherosclerosis.2010.08.050
Keywords: endothelial cells, endothelial injury, endothelial repair, inflammatory response, oxidative stress, endothelial progenitor cells
Citation: Huang Y, Song C, He J and Li M (2022) Research progress in endothelial cell injury and repair. Front. Pharmacol. 13:997272. doi: 10.3389/fphar.2022.997272
Received: 18 July 2022; Accepted: 24 August 2022;
Published: 13 September 2022.
Edited by:
Nektarios Barabutis, University of Louisiana at Monroe, United StatesReviewed by:
Eugenia Piragine, University of Pisa, ItalyMohammad Afaz Uddin, University of Pittsburgh, United States
Copyright © 2022 Huang, Song, He and Li. This is an open-access article distributed under the terms of the Creative Commons Attribution License (CC BY). The use, distribution or reproduction in other forums is permitted, provided the original author(s) and the copyright owner(s) are credited and that the original publication in this journal is cited, in accordance with accepted academic practice. No use, distribution or reproduction is permitted which does not comply with these terms.
*Correspondence: Jianbin He, aGpiMDkxOWhoQDE2My5jb20=; Min Li, MjMzMDY5MjU1N0BxcS5jb20=