- 1Institutes for Systems Genetics, Frontiers Science Center for Disease-Related Molecular Network, West China Hospital, Sichuan University, Chengdu, Sichuan, China
- 2School of Pharmaceutical Sciences, Lovely Professional University, Phagwara, Punjab, India
- 3Department of Pharmacology, Chandigarh College of Pharmacy, Mohali, India
- 4National Institute of Pharmaceutical Education and Research, Hyderabad, India
- 5Chitkara College of Pharmacy, Chitkara University, Rajpura, Punjab, India
- 6Department of Pharmaceutical Sciences and Drug Research, Punjabi University, Patiala, Punjab, India
- 7Department of Rasa Shastra and Bhaishajya Kalpana, Faculty of Ayurveda, Institute of Medical Sciences, Banaras Hindu University, Varanasi, Uttar Pradesh, India
Background: Bergenin, 4-O-methyl gallic acid glucoside, is a bioactive compound found in the cortex of Mallotus japonicus (L.f.) Müll.Arg. along with many other natural resources including that from Bergenia species. The present study delineates the neuroprotective potential of bergenin through the modulation of PPAR-γ receptors.
Method: Dementia was induced in the Wistar rats by intraperitoneal (i.p.) administration of sodium azide (12.5 mg/kg for the first 5 days followed by 10 mg/kg for the next 9 days). The rats were then exposed to the Morris water maze test to assess the effect on cognitive abilities followed by a series of biochemical and histopathological evaluations.
Results: Sodium azide-treated rats exhibited a severe deterioration of memory as suggested by poor performance in the spatial learning task in addition to the enhancement of brain acetylcholinesterase potential, oxidative stress, inflammation, and amyloid-β (Aβ) accumulation. Administration of bergenin to sodium azide-treated rats significantly recovered cognition and related biochemical variations. Further, co-administration of Bisphenol A diglycidyl ether (BADGE), a PPAR-γ antagonist with bergenin challenged its neuroprotective effects.
Conclusions: The findings of our study exhibit that the cognitive restoration potential of bergenin may be attributed to its modulatory effects against cholinesterase, oxidative stress, and inflammatory markers, as well as its neuroprotective actions, thus aligning it as a possible therapy for Alzheimer’s disease-related dementia. The study also fortifies the significance of PPAR-γ receptors in dementia.
1 Introduction
Dementia is a set of symptoms caused by several neurological disorders and among which Alzheimer’s disease (AD) is the most frequent cause, accounting for 50%–70% of cases (Ping, 2015; Hampel et al., 2018). AD is an advanced neurodegenerative disorder that interferes with the person’s daily performance like difficulties in speaking, problem-solving, and other cognitive skills, along with changes in mood and behavior due to memory and neuronal loss (Moussa-Pacha et al., 2019). The pathogenesis of AD includes the accumulation of amyloid- β (Aβ) protein in the form of extracellular senile plaques and intracellular neurofibrillary tangles formed by tau protein fibrils causing synaptic loss and neurodegeneration which leads to memory impairment and other cognitive problems (Weiner et al., 2013). Aβ causes induction of calcium-dependent excitotoxicity as it is correlated with the development of reactive oxygen species (ROS) as well as reactive nitrogen species (RNS). It also impairs cellular respiration and alters the synaptic functions which are associated with cognitive deficiencies (Butterfield et al., 2014). Oxidative stress begins early during AD and is among the major molecular change in the pathogenesis of AD as demonstrated by numerous studies (Gao et al., 2017; Beg et al., 2018). The current treatments which are available for AD include acetylcholinesterase inhibitors viz. memantine clinically used for the treatment and management of patients having moderate-to-severe AD (Weller and Budson, 2018). Diagnosis of AD is a difficult process and requires the patient to go through many blood tests, physical examinations, psychiatric evaluations, and brain scans like CT, MRI, and PET. Before the early 2000s, an autopsy was done after the death of the individual to know whether a person had Alzheimer’s disease. Now with the progressive research and advances in technology, the preclinical diagnosis of AD can be done by recent non-invasive imaging techniques using Aβ- and tau-positron emission tomography (PET) tracers which allow the tracking of the evolution of AD during the patient’s lifetime (Sáez-Orellana et al., 2020).
Sodium azide is a colorless crystalline solid and is a highly toxic substance appropriate for fabricating AD-like indications in rodents and for evaluating neuroprotective agents. Sodium azide is a selective inhibitor of the cyclooxygenase (COX) enzyme that induces a reduction in the activity of complex IV of the electron transport chain (ETC) and thus causes mitochondrial dysfunction which further leads to memory deficits (Weinstock and Shoham, 2004). Peroxisome proliferator-activated receptors (PPARs) are ligand-activated transcription factors that contribute a major function in the regulation of glucose assimilation and the homeostasis of lipid metabolism. Further, they are also known to be related to repressing the expression of genes related to inflammation (Lecarpentier et al., 2017). The results of clinical and animal studies exhibit that the utilization of Peroxisome proliferator-activated receptor gamma (PPAR-γ) agonist improves both learning and memory with other AD-related pathology. Therefore, these receptor agonists represent a beneficial therapeutic target for AD (Khan et al., 2019). Bergenin act as a PPAR-γ agonist (Xiang et al., 2020) and has antioxidative (Qiao et al., 2019), anti-inflammatory activity (Lim et al., 2000), BACE1 inhibitory activity (Madaan et al., 2022), and modulation of Nrf-2/NF-κB Pathway (Shal et al., 2021). Bergenin treatment restored the actions of ETC, particularly complex I, complex II, and complex IV; curtailed the lipid peroxidation; scavenged enhanced reactive oxygen species, and up-regulated antioxidant levels leading to the amelioration of mitochondrial dysfunction (Aggarwal et al., 2016). Bisphenol A diglycidyl ether (BADGE) is a synthetic substance and has PPAR-γ antagonistic activity (Dworzanski et al., 2010).
2 Materials and methods
2.1 Laboratory animals
Wistar rats of either sex (180–220 g) 8–10 weeks of age (NIPER, Punjab, India) were employed in the present study. Standard laboratory feed, procured from Ashirwad Industries, Punjab, India, and water ad libitum were provided to the animals exposed to 12 h light and dark cycle. The experimental protocol (CCP/IAEC/Feb 2021/9) was approved by the Institutional Animal Ethics Committee (IAEC) (Reg no. 1201/PO/Re/S/CPCSEA) and as per the standard guidelines given by CPCSEA, India.
2.2 Drugs and reagents
The materials used in the experiments were of analytical quality grade and were freshly prepared before use. Sodium azide (SA) was purchased from Nice Chemicals (P) Ltd., India. Bergenin (≥98.0%, HPLC, analytical standard) and Bisphenol A diglycidyl ether (BADGE, ≥95.0%, HPLC, analytical standard) were procured from Sigma Aldrich, India.
2.3 Laboratory models
2.3.1 Sodium azide-induced dementia
Dementia was produced in Wistar rats by intraperitoneal administration of sodium azide 12.5 mg/kg for the initial 5 days followed by 10 mg/kg for the next 9 days (Virdi et al., 2020).
2.3.2 Morris water maze test
In the present study, the learning and memory of the animals were assessed using the Morris water maze test (Morris, 1984; Bhatia et al., 2021). Morris water maze (MWM) is a swimming-based exteroceptive model in which a hidden platform is positioned and the animal learns to escape onto it (Sodhi and Singh, 2014). Animals were subjected to training for four successive days and the starting position was altered with each exposure (as shown in Table 1) (Kaur and Sodhi, 2015). On day 4 of escape latency time (ELT), the time spent exploring the concealed platform in the water maze, was recorded as an index of acquisition/learning. The platform was then detached on the fifth consecutive day and the mean time disbursed in altogether four quadrants was noted as an index of the acquisition of memory (Jain and Sharma, 2016). In the search for the concealed platform, the total time spent in the target quadrant (TSTQ) by the animal on the fifth day was noted as an indicator of recovery of memory.
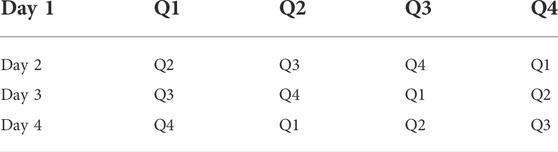
TABLE 1. Pattern of training trails on MWM test. (Q refers to quadrant). This table was adopted from our previous work (Kaur and Sodhi, 2015).
2.4 Biochemical parameters estimations
2.4.1 Collection of samples
The laboratory animals were sacrificed by cervical displacement after the completion of the planned experiment (Sodhi and Singh, 2013a). Brains were then removed, followed by homogenization with a phosphate buffer of pH 7.4. Centrifugation of the samples was then done at 3,000 rpm for 15 min (Bansal and Parle, 2010). The supernatant was then used for further experiments, related to acetylcholinesterase, total brain protein, reduced glutathione levels, nitrate/nitrite levels, thiobarbituric acid, myeloperoxidase activity, and pro-inflammatory cytokines.
2.4.2 Assessment of brain acetylcholinesterase activity
The total-brain acetylcholinesterase activity was evaluated as per the protocol given by (Ellman et al., 1961) with slight modifications as done by (Koladiya et al., 2009; Sain et al., 2011; Bhatia and Singh, 2020).
2.4.3 Assessment of brain thiobarbituric acid reactive species level
The quantitative measurement of thiobarbituric acid reactive species (TBARS) was carried out according to the method of Niehaus and Samuelsson (Niehaus and Samuelsson, 1968; Gulati and Singh, 2014). The absorbance was measured at 535 nm against a blank reagent. The values were expressed as nanomoles per mg of protein (Sharma and Singh, 2012).
2.4.4 Assessment of reduced glutathione level
Reduced glutathione (GSH) content of brain tissue was estimated using a method given by Beutler (Beutler et al., 1963; Sharma and Singh, 2012). The absorbance was measured spectrophotometrically at 412 nm. The results were expressed as micromoles of reduced glutathione per mg of protein (Sodhi and Singh, 2013a).
2.4.5 Assessment of brain myeloperoxidase level
The myeloperoxidase (MPO) activity was estimated as per the protocol given by Krawisz (Krawisz et al., 1984; Sodhi and Singh, 2013a).
2.4.6 Estimation of pro-inflammatory cytokines
The pro-inflammatory cytokines viz. TNF-α and IL-1β were measured with standard ELISA kits and the procedure adopted was that provided by the company (R & D Systems, Minneapolis, United States).
2.4.7 Assessment of brain nitrite/nitrate level
The brain nitrite/nitrate level was estimated as per the procedure given by Sastry and the team (Sastry et al., 2002) using a colorimetric assay. The results were articulated as micrograms per mg of protein.
2.4.8 Histopathological assessment
2.4.8.1 Hematoxylin and Eosin staining
Finally, brain tissue was fixed in 4% formalin to avert autolysis and putrefaction. Tissue processing was made following the standard procedures of fixation, dehydration, impregnation, embedding, sectioning, and staining with hematoxylin and eosin by the method of Bancroft (Bancroft, 2019). The micrographs of stained sections (5-μm thickness) were consequently visualized using a light microscope (×40) (Sodhi and Singh, 2013b).
2.4.8.2 Congo red staining
The brain sections (5-μm thickness) were kept in Congo red solution for 18 min (Sodhi and Singh, 2013a). Subsequently, the sections were washed with water for 20 min and then immersed in a weak base for 10 s following which the brain sections were dipped in hematoxylin for 5 min, and again cleaned with flowing water (Sodhi and Singh, 2013a; Sodhi and Singh, 2013b; Puchtler et al., 2017). The Aβ′s accumulation was studied through a light microscope (×100) (Sodhi and Singh, 2013a).
2.5 Experimental protocol
The study comprised nine separate investigational groups each consisting of 6 Wistar rats. The animals were administered sodium azide (12.5 mg/kg for the first 5 days followed by 10 mg/kg. for 9 days i.p, for 2 weeks). The drug treatment (donepezil, 0.1 mg/kg/day, i.p /bergenin, 30 mg/kg and 60 mg/kg p.o. /BADGE 30 mg/kg i.p.) was administered for 14 days starting from day 15 up to day 28. The procedure adopted was the same as performed in our previous study (Sodhi and Singh, 2013a) (Figure 1; Table 2).
2.6 Statistical evaluation
The results obtained were expressed as mean ± Standard error of the mean (S.E.M). The data from various groups were statistically examined using a one-way analysis of variance (ANOVA) followed by Bonferroni’s multiple range test. The p <0.05 was considered to be statistically significant (Kaur and Sodhi, 2015).
3 Results
3.1 Effect on cognitive parameters using Morris water maze
The results indicated that control animals exhibited a significant reduction in day 4 ELT in comparison to day 1 ELT (Kaur and Sodhi, 2015). Furthermore, during the retrieval trial on day 5 of the MWM test, it was observed that the animals spent more time in the target quadrant (Q4) in the search for the missing platform versus the total time spent in other quadrants (Q1, Q2, and Q3) suggestive of learning and memory (Kaur and Sodhi, 2015). The treatment with sodium azide significantly prevented the decline in day 4 ELT in comparison to the control group (Table 3) (Sodhi and Singh, 2013b). A marked diminution was also indicated in TSTQ (Q4) in the recovery trial on the fifth day (Figure 2). Further, change on the fourth day of ELT was not statistically significant (Table 3) and on day 5 TSTQ (Figure 2) was indicated in bergenin (30 mg/kg/day, p.o.) [BER Low-Dose (LD)] per se group in comparison to the control group (Sodhi and Singh, 2013a). In a dose-dependent way, co-administration of donepezil (0.1 mg/kg/day, i.p.) along with bergenin (30 mg/kg and 60 mg/kg p.o. [BER High-Dose (HD)] potentially decreases the fourth-day rise in ELT (Table 3) and a decline in fifth-day TSTQ in sodium azide-treated rats (Figure 2) (Sodhi and Singh, 2013a). Furthermore, co-administration of BADGE (30 mg/kg i.p.) with bergenin (30 mg/kg p.o.) antagonized the effect produced by the bergenin dose (Table 3; Figure 2) (Sodhi and Singh, 2013a). These results are aligning with our previous studies related to rifampicin and all-trans retinoic acid (Sodhi and Singh, 2013a; Kaur and Sodhi, 2015).
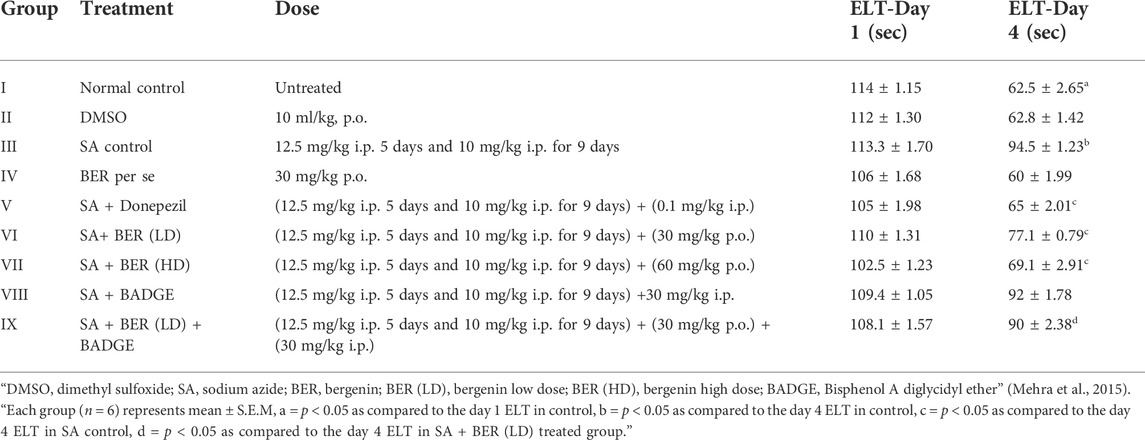
TABLE 3. “Effect of pharmacological interventions on escape latency time (ELT) on day 1 and day 4 (time in seconds) using Morris water maze (MWM) test in sodium azide-treated rats.” Performed in a way similar to our previously published work (Kaur and Sodhi, 2015).
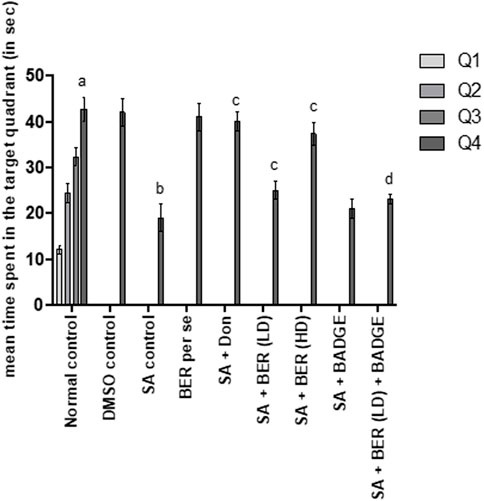
FIGURE 2. Effect of pharmacological interventions on the total time spent in the target quadrant (TSTQ) in seconds using the Morris water maze test. “DMSO, dimethyl sulfoxide; SA, sodium azide; Don, donepezil; BER, bergenin; BER (LD), bergenin low dose; BER (HD), bergenin high dose; BADGE, Bisphenol A diglycidyl ether” (Mehra et al., 2015). “Each group (n = 6) represents mean ± S.E.M, a = p ˂ 0.05 vs. time spent in quadrant one in normal control, b = p ˂ 0.05 vs. time spent in the target quadrant in normal control, c = p ˂ 0.05 vs. time spent in the target quadrant in SA control, d = p ˂ 0.05 vs. time spent in target quadrant in SA + BER (LD) treated group” (Sodhi and Singh, 2013b). One-way ANOVA followed by Bonferroni’s multiple range test.
3.2 Impact on brain acetylcholinesterase activity
Sodium azide-treated rats have suggestively boosted the brain acetylcholinesterase (AChE) activity in contrast to the rats from the control set (Figure 3) (Sodhi and Singh, 2013a). Bergenin per se (30 mg/kg p.o.) had no significant impact on brain AChE activity when compared with the control (Figure 3). Administration of donepezil and bergenin to sodium azide treated rats potentially decrease brain AChE activity while comparing with the control group (Figure 3). Co-administration of bergenin (30 mg/kg p.o.) along with BADGE (30 mg/kg i.p.) to sodium azide treated rats markedly enhanced brain AChE activity (Figure 3).
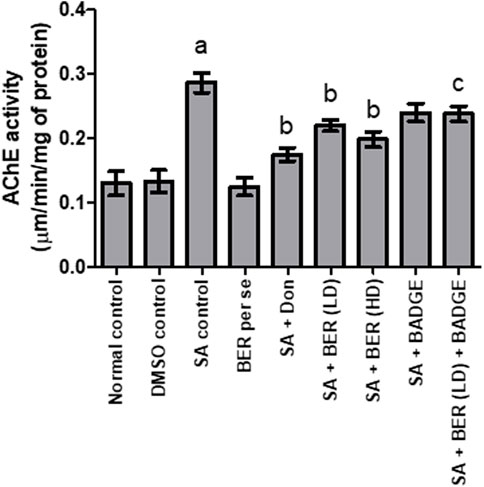
FIGURE 3. Effect of pharmacological interventions on brain AChE activity (μM of ACh hydrolyzed/min/mg protein). “DMSO, dimethyl sulfoxide; SA, sodium azide; Don, donepezil; BER, bergenin; BER (LD), bergenin low dose; BER (HD), bergenin high dose; BADGE, Bisphenol A diglycidyl ether” (Mehra et al., 2015). “Each group (n = 6) represents mean ± S.E.M, a = p ˂ 0.05 vs. normal control, b = p ˂ 0.05 vs. normal control, c = p ˂ 0.05 vs. SA control. One way ANOVA followed by Bonferroni’s multiple range test” (Gulati and Singh, 2014).
3.3 Impact on brain’s thiobarbituric acid reactive species and brain’s glutathione levels
A notable increase in the brain GSH level and a marked reduction in the brain TBARS levels was indicated in animals upon sodium azide treatment (Figures 4, 5) while comparing with the control group revealing oxidative stress induction. Bergenin (30 mg/kg, p.o.) per se has not shown any noteworthy impact on TBARS and GSH levels when compared with the control (Figures 4, 5). On the other hand, we have observed a dose-dependent relief from oxidative stress via a reduction in the TBARS level and enhancement of GSH level (Kaur et al., 2018) when co-administered donepezil and bergenin in sodium azide-treated rats (Figures 4, 5). Administration of BADGE (30 mg/kg i.p.) along with bergenin (30 mg/kg p.o.) enhanced the oxidative stress levels in sodium azide-treated rats (Figures 4, 5) (Carey et al., 2022).
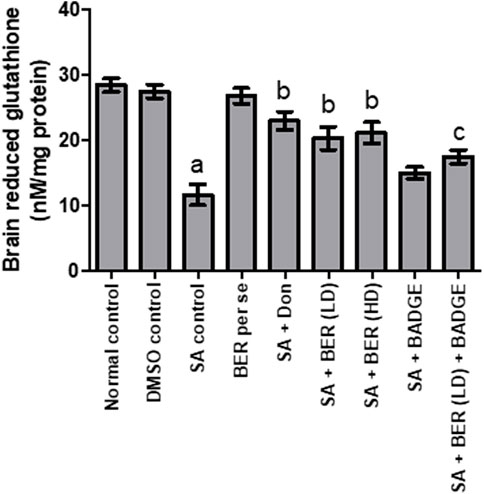
FIGURE 4. Effect of pharmacological interventions on brain GSH levels (μM/mg of protein). “DMSO, dimethyl sulfoxide; SA, sodium azide; Don, donepezil; BER, bergenin; BER (LD), bergenin low dose; BER (HD), bergenin high dose; BADGE, Bisphenol A diglycidyl ether” (Mehra et al., 2015). “Each group (n = 6) represents mean ± S.E.M, a = p ˂ 0.05 vs. normal control, b = p ˂ 0.05 vs. normal control, c = p ˂ 0.05 vs. SA control. One way ANOVA followed by Bonferroni’s multiple range test” (Gulati and Singh, 2014).
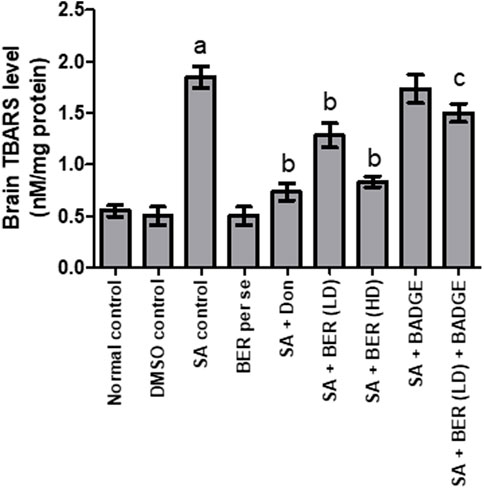
FIGURE 5. Effect of pharmacological interventions on brain TBARS level (nM/mg of protein). “DMSO, dimethyl sulfoxide; SA, sodium azide; Don, donepezil; BER, bergenin; BER (LD), bergenin low dose; BER (HD), bergenin high dose; BADGE, Bisphenol A diglycidyl ether” (Mehra et al., 2015). “Each group (n = 6) represents mean ± S.E.M, a = p ˂ 0.05 vs. normal control, b = p ˂ 0.05 vs. normal control, c = p ˂ 0.05 vs. SA control. One way ANOVA followed by Bonferroni’s multiple range test” (Sodhi and Singh, 2013b).
3.4 Effect on brain myeloperoxidase levels
Treatment with sodium azide significantly enhanced the brain MPO activity in comparison to the control group (Figure 6) (Sodhi and Singh, 2013a). Bergenin per se produced no significant change in brain MPO levels (Sodhi and Singh, 2014) in contrast to the control group (Figure 6). Administration of donepezil and bergenin to sodium azide-treated rats significantly reduced the brain MPO activity indicative of anti-inflammatory effect (Figure 6). Co-administration of BADGE (30 mg/kg i.p.) with bergenin (30 mg/kg p.o.) to sodium azide treated rats markedly enhanced brain MPO activity (Figure 6).
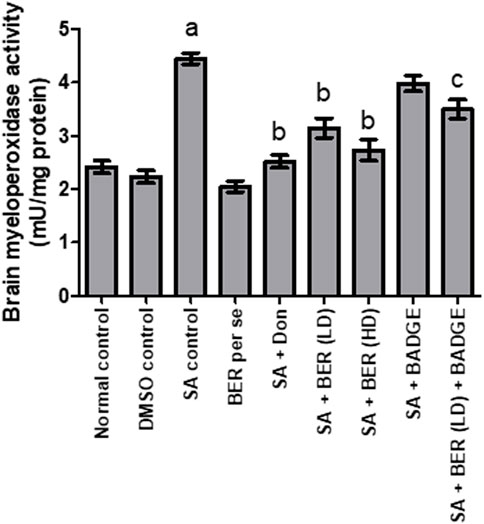
FIGURE 6. Effect of pharmacological interventions on brain MPO level (U/mg of protein). “DMSO, dimethyl sulfoxide; SA, sodium azide; Don, donepezil; BER, bergenin; BER (LD), bergenin low dose; BER (HD), bergenin high dose; BADGE, Bisphenol A diglycidyl ether” (Mehra et al., 2015). “Each group (n = 6) represents mean ± S.E.M, a = p ˂ 0.05 vs. normal control, b = p ˂ 0.05 vs. normal control, c = p ˂ 0.05 vs. SA control. One way ANOVA followed by Bonferroni’s multiple range test” (Sodhi and Singh, 2013b).
3.5 Impact on pro-inflammatory cytokines
Treatment with sodium azide significantly enhanced the level of proinflammatory cytokines like IL-1 β and TNF-α when compared with the control group rats (Figure 7). Bergenin per se did not express any significant impact on of IL-1 β and TNF-α levels. Administration of donepezil and bergenin to sodium azide-treated rats significantly attenuated the IL-1 β and TNF-α levels. Administration of BADGE (30 mg/kg i.p.) along with bergenin (30 mg/kg p.o.) to sodium azide-treated rats markedly enhanced the expression of IL-1 β and TNF-α (Carey et al., 2022).
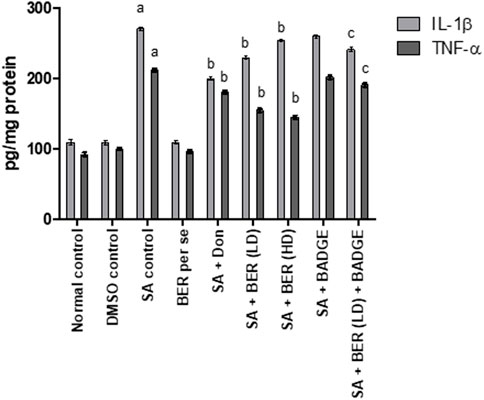
FIGURE 7. Impact of bergenin on brain cytokine levels (TNF-α and IL-1β) (pg/mg of protein). “DMSO, dimethyl sulfoxide; SA, sodium azide; Don, donepezil; BER, bergenin; BER (LD), bergenin low dose; BER (HD), bergenin high dose; BADGE, Bisphenol A diglycidyl ether” (Mehra et al., 2015). “Each group (n = 6) represents mean ± S.E.M, a = p ˂ 0.05 vs. normal control, b = p ˂ 0.05 vs. normal control, c = p ˂ 0.05 vs. SA control. One-way ANOVA followed by Bonferroni’s multiple range test”.
3.6 Impact on brain nitrite/nitrate level
Treatment with sodium azide significantly enhanced the brain nitrate/nitrite levels in comparison with the control rats (Figure 8). No significant alteration was detected in the brain nitrite/nitrate levels in the bergenin per se group while the comparison is made with the control rats’ group (Figure 8). Co-administration of donepezil (0.1 mg/kg/day, i.p.) and bergenin (30 and 60 mg/kg p.o.) to sodium azide rats markedly reduced the brain nitrate/nitrite levels when compared to the normal group (Figure 8). Concomitant usage of BADGE (30 mg/kg i.p.) with bergenin (30 mg/kg p.o.) to sodium azide-treated rats markedly enhanced brain nitrate/nitrite levels (Figure 8). These experiments were designed in a similar way to our previous experiments and other researcher’s results (Kaur and Sodhi, 2015; Ashwlayan, 2017).
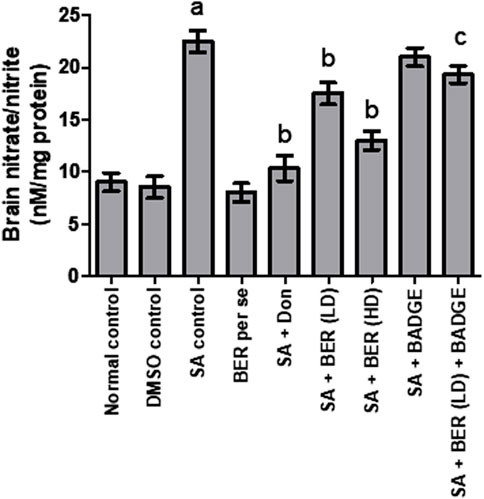
FIGURE 8. Effect of pharmacological interventions on brain nitrite/nitrate levels. “DMSO, dimethyl sulfoxide; SA, sodium azide; Don, donepezil; BER, bergenin; BER (LD), bergenin low dose; BER (HD), bergenin high dose; BADGE, Bisphenol A diglycidyl ether” (Mehra et al., 2015). “Each group (n = 6) represents mean ± S.E.M, a = p ˂ 0.05 vs. normal control, b = p ˂ 0.05 vs. normal control, c = p ˂ 0.05 vs. SA control. One-way ANOVA followed by Bonferroni’s multiple range test.”
3.7 Histopathological alterations in the brain
Brain sections stained with hematoxylin and eosin exhibited severe neutrophil infiltration in sodium azide-treated rats indicative of neuroinflammation. Administration of donepezil and bergenin to sodium azide-treated rats produced a significant reduction in neutrophilic infiltration. Furthermore, sodium azide-treated rats demonstrated noticeable congo red staining observed as orange-red coloration signifying Aβ deposition (Sodhi and Singh, 2013b). Reduced Congo red deposition was observed in the donepezil and bergenin-administered sodium azide-treated rats (Figure 9) (Sodhi and Singh, 2013b).
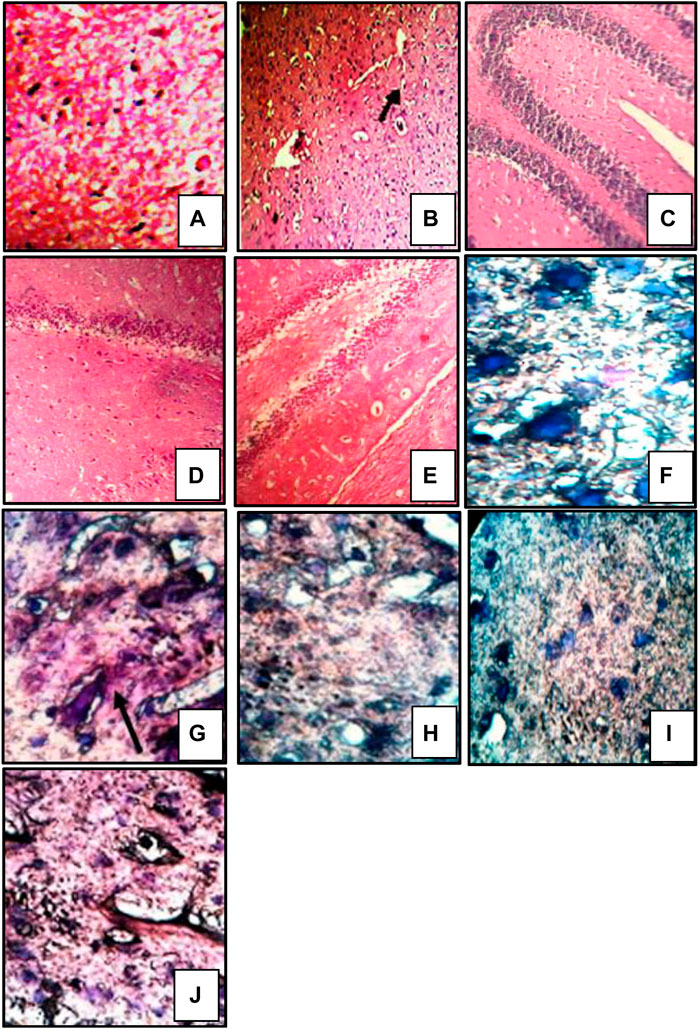
FIGURE 9. Histological sections of the brain were stained with HE staining, magnification 400 × (A–E), and congo red staining, magnification 1,000 x; oil immersion (I,J) (Sodhi and Singh, 2014). “Control (A) shows normal histological features. SA-treated rat (B) showed focal as well as diffuse severe neutrophilic infiltration, congestion of blood vessels, and pericellular edema. Bergenin administered (30 and 60 mg/kg) SA-treated rats (C,D) respectively featuring mild neutrophilic infiltration and pericellular edema. BADGE administration (30 mg/kg) along with bergenin (30 mg/kg) to SA-treated rats (E) showed focal as well as diffuse severe neutrophilic infiltration. Control (F) congo red deposition was not detected. SA-treated rat (G) showing orange-red deposits of congo red. Bergenin administered (30 and 60 mg/kg) SA-treated rats (H,I) respectively showed significant congo red deposits. BADGE administration (30 mg/kg) along with bergenin (30 mg/kg) to SA-treated rats (J) showing orange-red deposits of congo red.”
4 Discussion
Alzheimer’s disease (AD) is a progressive multifarious neurodegenerative disorder characterized by senile plaques containing extracellular amyloidal protein deposits and intracellular neurofibrillary tangles (Kumar et al., 2015). Our study was undertaken to determine the defensive effect of bergenin on dementia induced by sodium azide in Wistar rats. We observed a significant development in cognitive parameters and biochemical alterations with the administration of bergenin indicative of its neuroprotective potential in certain markers of dementia (Neha et al., 2014). Further to gain evidence regarding the neuroprotective effect of bergenin we evaluated a series of parameters related to cognition, biochemistry, and histopathology. MWM test was conducted in the current study since it is amongst the commonly used well-established exteroceptive models to evaluate learning and memory in rodents including rats (Sharma and Singh, 2012; Sodhi and Singh, 2013b; Rani et al., 2015). Normal acquisition of memory in control untreated rats is denoted by a marked decline in escape latency time during acquisition trials and retrieval of memory is indicated by an enhancement of mean TSTQ in pursuit of the missing platform (Saraf et al., 2003; Kaur and Sodhi, 2015).
A sodium azide-induced experimental dementia model was used in our study to produce dementia of AD type. Chronic administration of sodium azide in rats produces pathological processes similar to that of AD, like reduction of mitochondrial complex IV activity (Nöldner, 2017). Further, the synthesis of the Aβ peptide through the distorted breakdown of the Aβ -protein precursor (AβPP) is pivotal in the pathology of AD. Disturbed processing of AβPP is observed during sodium azide treatment (Henriques et al., 2005). Sodium azide causes a diminution in mitochondrial cytochrome oxidase activity, an enhancement in the expression of amyloidogenic AβPP and Aβ 1–42 level, beta-site amyloid precursor protein cleaving enzyme 1 (BACE1), and attenuation in the neurotrophin’s expression in the region of hippocampus when administered to rats (Zhang et al., 2018). It has been demonstrated that there exists an association between “mitochondrial dysfunction and autophagy” in the course of AD’s pathology. Owing to inadequate digestion of oxidatively damaged macromolecules and organelles by autophagy, neurons progressively accumulate lipofuscin which could aggravate neuronal dysfunction (Moreira et al., 2010).
Results of our study indicate that administration of sodium azide (12.5 mg/kg for 5 days and 10 mg/kg for the next 9 days) resulted in the rigorous decline of spatial memory as signified by MWM test performance evaluated employing ELT and mean TSTQ (Morris, 1984). Moreover, a significant rise in acetylcholinesterase activity, nitrate/nitrite levels, and oxidative stress (shown by a significant increase in TBARS level and a decrease in GSH level) had also been observed (Kaur and Sodhi, 2015). Additionally, MPO activity was increased which is indicative of neuroinflammation. Donepezil acted as the positive control in the current investigation since donepezil is well accepted by the “U.S. Food and Drug Administration (USFDA)” to be efficacious for the management of moderate to severe levels of AD (Zhang and Gordon, 2018) and has been verified in our previous studies for experimental dementia (Sodhi and Singh, 2013a).
Bergenin is a colorless crystalline compound chemically occurring as a C-glucoside of 4-O-methyl gallic acid. It exhibits antiviral, antifungal, anti-inflammatory, antitumor, antitussive, antidiabetic, antiplasmodial, antihepatotoxic, antiulcerogenic, antiarrhythmic, and wound healing properties (Bajracharya, 2015). It has been reported that bergenin stimulates PPAR-γ, inhibits TGF-β, promotes autophagy, and reduces liver fibrosis by preventing hepatocyte necrosis and extracellular matrix development (Xia et al., 2020; Xiang et al., 2020). Several studies have proposed the anti-inflammatory and anti-oxidative potential of bergenin in different pathological states (Oliveira et al., 2019; Villarreal et al., 2020). Bergenin has been identified as a PPAR-γ agonist to promote nuclear translocation and transcriptional activity of PPAR-γ. It also augments the expression of mRNA related to CD36, LPL, and ap2 (Dave et al., 2012). In addition, it has also been depicted that bergenin enhances the expression of Sirtuin 1, inhibits the NF-κB-p65 acetylation, and increases NF-κB-p65 and IκBα association (Wang et al., 2018). Another study indicated that bergenin and its analogs have an inhibitory effect on BACE1 and hence may be useful in Alzheimer’s disease (Kashima and Miyazawa, 2013). It has been documented that the PPAR response element is located in the BACE-1’s promoter region, and the binding of PPAR to the response element suppresses the expression of BACE-1 and hence restrains Aβ synthesis (Heneka et al., 2005).
In this present study, we observed that the administration of PPAR-γ agonist, bergenin (30 mg/kg and 60 mg/kg; p.o.) for 2 weeks to sodium azide-treated rats prominently improved the cognitive deficiencies evoked by sodium azide. Bergenin treatment led to a noteworthy decrease in fourth-day ELT and an increase in the fifth-day TSTQ in the MWM test in contrast to the sodium azide-treated group (Kumar and Singh, 2017). Administration of bergenin caused a noteworthy decrease in brain AChE activity, brain TBARS, brain nitrite/nitrate level, and brain MPO activity which were increased due to sodium azide administration, and an increase in GSH level was also observed (Gulati and Singh, 2014). Furthermore, bergenin administration reduced the histopathological alterations, exemplified by low neutrophilic accumulation, and pericellular edema, which recommends a noticeable protective and anti-inflammatory effect against the toxic effects of sodium azide (Sodhi and Singh, 2014).
PPAR—γ did contribute an important and vital role in modulating gene expression associated with multiple diseases which include obesity, diabetes, and cancer (Janani and Ranjitha Kumari, 2015). Study indicates that PPAR- γ stimulation mitigates the inflammation associated with chronic and acute neurological insults with the major mechanism involving PPAR- γ (Kapadia, 2008). PPAR-γ-induced neuroprotection may be an outcome of avoidance of microglial activation and inflammatory cytokine and chemokine expression. PPAR-γ impedes NF-κB action by hindering NF-κB nuclear translocation and challenging NF-κB for co-activators, therefore, holding potential for the treatment of neuroinflammatory disorders (Chen et al., 2012). Wang and the team conducted another study on APP/PS1 mice treated with a natural PPAR-γ agonist, astragaloside IV, which increased the activity of PPAR-γ and inhibited BACE1 thereby decreasing Aβ levels and neuritic plaque formation significantly (Wang et al., 2016).
In the present investigation, BADGE, a PPAR-γ antagonist was administered to the rats to verify whether it reverses the neuroprotective effects produced by bergenin. Administration of BADGE to bergenin-treated sodium azide rats significantly reversed the protective biochemical and histopathological alterations produced by bergenin. A marked deterioration of memory was also indicated. Hence it may be worth suggesting that bergenin may elicit neuroprotective effects in AD-type dementia owing to its memory restorative, antioxidative, anti-inflammatory, and anticholinesterase activity. It may also be suggested that neuroprotective effects produced by bergenin may involve the activation of PPAR-γ receptors.
5 Conclusion
Our study provides evidence that bergenin improves cognition and memory in sodium azide-induced experimental dementia by virtue of its neuroprotective, memory restorative, anti-cholinesterase, anti-oxidative and anti-inflammatory activity accompanied by a decrease in brain neutrophil infiltration (Sodhi and Singh, 2013a). It may also be concluded that bergenin produced its beneficial action through the activation of PPAR-γ receptors. Nevertheless, further studies are needed to confirm the neuroprotective activity of bergenin through PPAR-γ activation.
Data availability statement
The original contributions presented in the study are included in the article/Supplementary Material, further inquiries can be directed to the corresponding authors.
Ethics statement
The animal study was reviewed and approved by The experimental protocol (CCP/IAEC/Feb2021/9) and “the Institutional Animal Ethics Committee (IAEC)” (Reg no. 1201/PO/Re/S/CPCSEA) and as per the standard guidelines given by CPCSEA, India.
Author contributions
All authors listed have made a substantial, direct, and intellectual contribution to the work and approved it for publication.
Funding
This work was supported by the National Natural Science Foundation of China (32070671), the COVID-19 research projects of West China Hospital Sichuan University (Grant no. HX-2019-nCoV-057) as well as the regional innovation cooperation between Sichuan and Guangxi Provinces (2020YFQ0019).
Acknowledgments
The authors would like to thank the National Natural Science Foundation of China, West China Hospital Sichuan University as well as the regional innovation cooperation between Sichuan and Guangxi Provinces for providing the necessary funding.
Conflict of interest
All authors declare that the research was conducted in the absence of any commercial or financial relationships that could be construed as a potential conflict of interest.
Publisher’s note
All claims expressed in this article are solely those of the authors and do not necessarily represent those of their affiliated organizations, or those of the publisher, the editors and the reviewers. Any product that may be evaluated in this article, or claim that may be made by its manufacturer, is not guaranteed or endorsed by the publisher.
References
Aggarwal, D., Gautam, D., Sharma, M., and Singla, S. K. (2016). Bergenin attenuates renal injury by reversing mitochondrial dysfunction in ethylene glycol induced hyperoxaluric rat model. Eur. J. Pharmacol. 791, 611-621. doi:10.1016/j.ejphar.2016.10.002
Ashwlayan, V. D. (2017). Antidementic activity of Brassica oleracea L. Var. Italica (brassicaceae) flower on memory deficit in young male rats. Pharm. Pharmacol. Int. J. 5 (3). doi:10.15406/ppij.2017.05.00124
Bajracharya, G. B. (2015). Diversity, pharmacology and synthesis of bergenin and its derivatives: Potential materials for therapeutic usages. Fitoterapia 101, 133-152. doi:10.1016/j.fitote.2015.01.001
Bansal, N., and Parle, M. (2010). Beneficial effect of chyawanprash on cognitive function in aged mice. Pharm. Biol. 49 (1), 2-8. doi:10.3109/13880209.2010.489904
Beg, T., Jyoti, S., Naz, F., RahulAli, F., Ali, S. K., et al. (2018). Protective effect of kaempferol on the transgenic Drosophila model of Alzheimer’s disease. CNS Neurol. Disord. Drug Targets 17 (6), 421-429. doi:10.2174/1871527317666180508123050
Beutler, E., Duron, O., and Kelly, B. M. (1963). Improved method for the determination of blood glutathione. J. Lab. Clin. Med. 61, 882-888.
Bhatia, P., Kaur, G., and Singh, N. (2021). Ozagrel a thromboxane A2 synthase inhibitor extenuates endothelial dysfunction, oxidative stress and neuroinflammation in rat model of bilateral common carotid artery occlusion induced vascular dementia. Vascul. Pharmacol. 137. 106827, doi:10.1016/j.vph.2020.106827
Bhatia, P., and Singh, N. (2020). Ameliorative effect of ozagrel, a thromboxane A2 synthase inhibitor, in hyperhomocysteinemia‐induced experimental vascular cognitive impairment and dementia. Fundam. Clin. Pharmacol. 35 (4), 650-666. doi:10.1111/fcp.12610
Butterfield, D. A., Di Domenico, F., and Barone, E. (2014). Elevated risk of type 2 diabetes for development of alzheimer disease: A key role for oxidative stress in brain. Biochim. Biophys. Acta 1842 (9), 1693-1706. doi:10.1016/j.bbadis.2014.06.010
Carey, L. M., Xu, Z., Rajic, G., Makriyannis, A., Romero, J., Hillard, C., et al. (2022). Peripheral sensory neuron CB2 cannabinoid receptors are necessary for both CB2-mediated antinociceptive efficacy and sparing of morphine tolerance in a mouse model of neuropathic pain. doi: doi:10.1101/2022.05.16.492135
Chen, Y.-C., Wu, J.-S., Tsai, H.-D., Huang, C.-Y., Chen, J.-J., Sun, G. Y., et al. (2012). Peroxisome proliferator-activated receptor gamma (PPAR-γ) and neurodegenerative disorders. Mol. Neurobiol. 46 (1), 114-124. doi:10.1007/s12035-012-8259-8
Dave, S., Kaur, N. J., Nanduri, R., Dkhar, H. K., Kumar, A., and Gupta, P. (2012). Inhibition of adipogenesis and induction of apoptosis and lipolysis by stem bromelain in 3T3-L1 adipocytes. PLoS One 7 (1), e30831. doi:10.1371/journal.pone.0030831
Dworzanski, T., Celinski, K., Korolczuk, A., Slomka, M., Radej, S., Czechowska, G., et al. (2010). Influence of the peroxisome proliferator-activated receptor gamma (PPAR-gamma) agonist, rosiglitazone and antagonist, biphenol-A-diglicydyl ether (BADGE) on the course of inflammation in the experimental model of colitis in rats. J. Physiol. Pharmacol. 61 (6), 683-693.
Ellman, G. L., Courtney, K. D., Andres, V., and Featherstone, R. M. (1961). A new and rapid colorimetric determination of acetylcholinesterase activity. Biochem. Pharmacol. 7 (2), 88-95. doi:10.1016/0006-2952(61)90145-9
Gao, C., Chang, P., Yang, L., Wang, Y., Zhu, S., Shan, H., et al. (2017). Neuroprotective effects of hydrogen sulfide on sodium azide-induced oxidative stress in PC12 cells. Int. J. Mol. Med. 41, 242, 250. doi:10.3892/ijmm.2017.3227
Gulati, P., and Singh, N. (2014). Neuroprotective effect of tadalafil, a PDE-5 inhibitor, and its modulation by L-NAME in mouse model of ischemia-reperfusion injury. J. Surg. Res. 186 (1), 475-483. doi:10.1016/j.jss.2013.08.005
Hampel, H., Toschi, N., Babiloni, C., Baldacci, F., Black, K. L., Bokde, A. L. W., et al. (2018). Revolution of Alzheimer Precision Neurology. Passageway of Systems Biology and Neurophysiology. J. Alzheimer's Dis. 64(s1), S47-S105–S105. doi: doi:10.3233/jad-179932
Heneka, M. T., Sastre, M., Dumitrescu-Ozimek, L., Hanke, A., Dewachter, I., Kuiperi, C., et al. (2005). Acute treatment with the PPARgamma agonist pioglitazone and ibuprofen reduces glial inflammation and Abeta1-42 levels in APPV717I transgenic mice. Brain 128 (6), 1442-1453. doi:10.1093/brain/awh452
Henriques, A. G., Domingues, S. C. T. S., Fardilha, M., da Cruz e Silva, E. F., and da Cruz e Silva, O. A. B. (2005). Sodium azide and 2-deoxy-D-glucose-induced cellular stress affects phosphorylation-dependent AbetaPP processing. J. Alzheimer's Dis. 7 (3), 201-212. doi:10.3233/jad-2005-7302
Jain, S., and Sharma, B. (2016). Effect of ruthenium red, a ryanodine receptor antagonist in experimental diabetes induced vascular endothelial dysfunction and associated dementia in rats. Physiol. Behav. 164, 140-150. doi:10.1016/j.physbeh.2016.05.052
Janani, C., and Ranjitha Kumari, B. D. (2015). PPAR gamma gene – a review. Diabetes Metab. Syndr. 9 (1), 46-50. doi:10.1016/j.dsx.2014.09.015
Kapadia, R., Yi, J. H., and Vemuganti, R. (2008). Mechanisms of anti-inflammatory and neuroprotective actions of PPAR-gamma agonists. Front. Biosci. 13 (13), 1813-1826. doi:10.2741/2802
Kashima, Y., and Miyazawa, M. (2013). Structure-activity relationships for bergenin analogues as β-secretase (BACE1) inhibitors. J. Oleo Sci. 62 (6), 391-401. doi:10.5650/jos.62.391
Kaur, J., Sodhi, R. K., Madan, J., Chahal, S. K., and Kumar, R. (2018). Forskolin convalesces memory in high fat diet-induced dementia in wistar rats—plausible role of pregnane x receptors. Pharmacol. Rep. 70 (1), 161-171. doi:10.1016/j.pharep.2017.07.009
Kaur, P., and Sodhi, R. K. (2015). Memory recuperative potential of rifampicin in aluminum chloride-induced dementia: Role of pregnane X receptors. Neuroscience 288, 24-36. doi:10.1016/j.neuroscience.2014.12.033
Khan, M. A., Alam, Q., Haque, A., Ashafaq, M., Khan, M. J., Ashraf, G. M., et al. (2019). Current progress on peroxisome proliferator-activated receptor gamma agonist as an emerging therapeutic approach for the treatment of alzheimer's disease: An update. Curr. Neuropharmacol. 17 (3), 232-246. doi:10.2174/1570159x16666180828100002
Koladiya, R. U., Jaggi, A. S., Singh, N., and Sharma, B. K. (2009). Beneficial effects of donepezil on vascular endothelial dysfunction-associated dementia induced by L-methionine in rats. J. Health Sci. (El. Monte). 55 (2), 215-225. doi:10.1248/jhs.55.215
Krawisz, J. E., Sharon, P., and Stenson, W. F. (1984). Quantitative assay for acute intestinal inflammation based on myeloperoxidase activity. Gastroenterology 87 (6), 1344-1350. doi:10.1016/0016-5085(84)90202-6
Kumar, A., Singh, A., and Ekavali, (2015). A review on alzheimer's disease pathophysiology and its management: An update. Pharmacol. Rep. 67 (2), 195-203. doi:10.1016/j.pharep.2014.09.004
Kumar, A., and Singh, N. (2017). Pharmacological activation of protein kinase A improves memory loss and neuropathological changes in a mouse model of dementia of Alzheimer’s type. Behav. Pharmacol. 28 (23), 187-198. doi:10.1097/fbp.0000000000000294
Lecarpentier, Y., Claes, V., Vallée, A., and Hébert, J.-L. (2017). Interactions between PPAR gamma and the canonical wnt/beta-catenin pathway in type 2 diabetes and colon cancer. PPAR Res. 2017, 5879090–9. doi:10.1155/2017/5879090
Lim, H.-K., Kim, H.-S., Choi, H.-S., Oh, S., and Choi, J. (2000). Hepatoprotective effects of bergenin, a major constituent of Mallotus japonicus, on carbon tetrachloride-intoxicated rats. J. Ethnopharmacol. 72 (3), 469-474. doi:10.1016/s0378-8741(00)00260-9
Madaan, R., Singla, R. K., Kumar, S., Dubey, A. K., Kumar, D., Sharma, P., et al. (2022). Bergenin - a biologically active scaffold: Nanotechnological perspectives. Curr. Top. Med. Chem. 22 (2), 132-149. doi:10.2174/1568026621666211015092654
Mehra, R., Sodhi, R. K., and Aggarwal, N. (2015). Memory restorative ability of clioquinol in copper–cholesterol-induced experimental dementia in mice. Pharm. Biol. 53 (9), 1250-1259. doi:10.3109/13880209.2014.974061
Moreira, P. I., Carvalho, C., Zhu, X., Smith, M. A., and Perry, G. (2010). Mitochondrial dysfunction is a trigger of Alzheimer's disease pathophysiology. Biochim. Biophys. Acta 1802 (1), 2-10. doi:10.1016/j.bbadis.2009.10.006
Morris, R. (1984). Developments of a water-maze procedure for studying spatial learning in the rat. J. Neurosci. Methods 11 (1), 47-60. doi:10.1016/0165-0270(84)90007-4
Moussa‐Pacha, N. M., Abdin, S. M., Omar, H. A., Alniss, H., and Al‐Tel, T. H. (2019). BACE1 inhibitors: Current status and future directions in treating Alzheimer's disease. Med. Res. Rev. 40 (1), 339-384. doi:10.1002/med.21622
NehaKumar, A., Jaggi, A. S., Sodhi, R. K., and Singh, N. (2014). Silymarin ameliorates memory deficits and neuropathological changes in mouse model of high-fat-diet-induced experimental dementia. Naunyn. Schmiedebergs. Arch. Pharmacol. 387 (8), 777-787. doi:10.1007/s00210-014-0990-4
Niehaus, W. G., and Samuelsson, B. (1968). Formation of malonaldehyde from phospholipid arachidonate during microsomal lipid peroxidation. Eur. J. Biochem. 6 (1), 126-130. doi:10.1111/j.1432-1033.1968.tb00428.x
Nöldner, M. (2017). "Oral treatment with the Ginkgo biloba extract EGb 761® prevents sodium azide-induced memory deficits in rats", in: 65th international congress and annual meeting of the society for medicinal plant and natural product research (GA 2017).).
Oliveira, G. A. L., Araujo, A. K. S., Pacheco, G., Oliveira, A. P., Carvalho, J. L., Chaves, L. S., et al. (2019). Anti-inflammatory properties of bergenin in mice. J. Appl. Pharm. Sci. 9 (07), 069–077.
Ping, S. (2015). Study of the role of SSAO/VAP-1 in OGD conditions using SSAO/VAP-1-expressing HUVEC and human brain endothelial cells (hCMEC/D3) as experimental models of ischemic stroke, and its possible nexus with Alzheimer's disease. Catalonia, Spain: PhD, Universitat Autonoma de Barcelona.
Puchtler, H., Sweat, F., and Levine, M. (2017). On the binding of Congo red by amyloid. J. Histochem. Cytochem. 10 (3), 355-364. doi:10.1177/10.3.355
Qiao, S., Liu, R., Lv, C., Miao, Y., Yue, M., Tao, Y., et al. (2019). Bergenin impedes the generation of extracellular matrix in glomerular mesangial cells and ameliorates diabetic nephropathy in mice by inhibiting oxidative stress via the mTOR/β-TrcP/Nrf2 pathway. Free Radic. Biol. Med. 145, 118-135. doi:10.1016/j.freeradbiomed.2019.09.003
Rani, A., NehaSodhi, R. K., and Kaur, A. (2015). Protective effect of a calcium channel blocker “diltiazem” on aluminum chloride-induced dementia in mice. Naunyn. Schmiedebergs. Arch. Pharmacol. 388 (11), 1151-1161. doi:10.1007/s00210-015-1148-8
Sáez-Orellana, F., Octave, J.-N., and Pierrot, N. (2020). Alzheimer’s disease, a lipid story: Involvement of peroxisome proliferator-activated receptor α. Cells 9 E1215(5). doi:10.3390/cells9051215
Sain, H., Sharma, B., Jaggi, A. S., and Singh, N. (2011). Pharmacological investigations on potential of peroxisome proliferator-activated receptor-gamma agonists in hyperhomocysteinemia-induced vascular dementia in rats. Neuroscience 192, 322-333. doi:10.1016/j.neuroscience.2011.07.002
Saraf, M. K., Kishore, K., Thomas, K. M., Sharma, A., and Singh, M. (2003). Role of platelet activating factor in triazolobenzodiazepines-induced retrograde amnesia. Behav. Brain Res. 142 (1-2), 31-40. doi:10.1016/s0166-4328(02)00365-0
Sastry, K. V. H., Moudgal, R. P., Mohan, J., Tyagi, J. S., and Rao, G. S. (2002). Spectrophotometric determination of serum nitrite and nitrate by copper–cadmium alloy. Anal. Biochem. 306 (1), 79-82. doi:10.1006/abio.2002.5676
Shal, B., Khan, A., Khan, A. U., Ullah, R., Ali, G., Islam, S. U., et al. (2021). Alleviation of memory deficit by bergenin via the regulation of reelin and nrf-2/NF-κB pathway in transgenic mouse model. Int. J. Mol. Sci. 22 (12), 6603. doi:10.3390/ijms22126603
Sharma, B., and Singh, N. (2012). Salutary effect of NFκB inhibitor and folacin in hyperhomocysteinemia-hyperlipidemia induced vascular dementia. Prog. Neuropsychopharmacol. Biol. Psychiatry 38 (2), 207-215. doi:10.1016/j.pnpbp.2012.03.013
Sodhi, R. K., and Singh, N. (2013a). All-trans retinoic acid rescues memory deficits and neuropathological changes in mouse model of streptozotocin-induced dementia of Alzheimer's type. Prog. Neuropsychopharmacol. Biol. Psychiatry 40, 38-46. doi:10.1016/j.pnpbp.2012.09.012
Sodhi, R. K., and Singh, N. (2013b). Defensive effect of lansoprazole in dementia of AD type in mice exposed to streptozotocin and cholesterol enriched diet. PLoS ONE 8 e70487(7). doi:10.1371/journal.pone.0070487
Sodhi, R. K., and Singh, N. (2014). Liver X receptor agonist T0901317 reduces neuropathological changes and improves memory in mouse models of experimental dementia. Eur. J. Pharmacol. 732, 50-59. doi:10.1016/j.ejphar.2014.03.025
Villarreal, C. F., Santos, D. S., Lauria, P. S. S., Gama, K. B., Espírito-Santo, R. F., Juiz, P. J. L., et al. (2020). Bergenin reduces experimental painful diabetic neuropathy by restoring redox and immune homeostasis in the nervous system. Int. J. Mol. Sci. 21 (14), 4850. doi:10.3390/ijms21144850
Virdi, J. K., Bhanot, A., Jaggi, A. S., and Agarwal, N. (2020). Investigation on beneficial role of l-carnosine in neuroprotective mechanism of ischemic postconditioning in mice: Possible role of histidine histamine pathway. Int. J. Neurosci. 130 (10), 983-998. doi:10.1080/00207454.2020.1715393
Wang, K., Li, Y.-f., Lv, Q., Li, X.-m., Dai, Y., and Wei, Z.-f. (2018). Bergenin, acting as an agonist of PPARγ, ameliorates experimental colitis in mice through improving expression of SIRT1, and therefore inhibiting NF-κB-Mediated macrophage activation. Front. Pharmacol. 8. 981, doi:10.3389/fphar.2017.00981
Wang, X., Wang, Y., Hu, J.-P., Yu, S., Li, B.-K., Cui, Y., et al. (2016). Astragaloside IV, a natural PPARγ agonist, reduces Aβ production in Alzheimer’s disease through inhibition of BACE1. Mol. Neurobiol. 54 (4), 2939-2949. doi:10.1007/s12035-016-9874-6
Weiner, M. W., Veitch, D. P., Aisen, P. S., Beckett, L. A., Cairns, N. J., Green, R. C., et al. (2013). The alzheimer's disease neuroimaging initiative: A review of papers published since its inception. Alzheimers Dement. 9, (5) e111-e194. doi:10.1016/j.jalz.2013.05.1769
Weinstock, M., and Shoham, S. (2004). Rat models of dementia based on reductions in regional glucose metabolism, cerebral blood flow and cytochrome oxidase activity. J. Neural Transm. 111 (3), 347-366. doi:10.1007/s00702-003-0058-y
Weller, J., and Budson, A. (2018). Current understanding of Alzheimer’s disease diagnosis and treatment. F1000Res. 7. F1000 Faculty Rev-1161, doi:10.12688/f1000research.14506.1
Xia, Y., Li, J., Chen, K., Feng, J., Guo, C., and Kaundal, R. K. (2020). Bergenin attenuates hepatic fibrosis by regulating autophagy mediated by the PPAR-γ/TGF-β pathway. PPAR Res. 2020, 6694214–13. doi:10.1155/2020/6694214
Xiang, S., Chen, K., Xu, L., Wang, T., and Guo, C. (2020). Bergenin Exerts Hepatoprotective Effects by Inhibiting the Release of Inflammatory Factors, Apoptosis and Autophagy via the PPAR-γ Pathway. Drug Des. Devel. Ther. 14, 129-143. doi:10.2147/dddt.S229063
Zhang, N., and Gordon, M. L. (2018). Clinical efficacy and safety of donepezil in the treatment of Alzheimer’s disease in Chinese patients. Clin. Interv. Aging 13, 1963-1970. doi:10.2147/cia.S159920
Keywords: dementia, Alzheimer’s disease, bergenin, PPAR-γ, BADGE, memory, oxidative stress, neuroinflammation
Citation: Singla RK, Dhonchak K, Sodhi RK, Arockia Babu M, Madan J, Madaan R, Kumar S, Sharma R and Shen B (2022) Bergenin ameliorates cognitive deficits and neuropathological alterations in sodium azide-induced experimental dementia. Front. Pharmacol. 13:994018. doi: 10.3389/fphar.2022.994018
Received: 14 July 2022; Accepted: 24 August 2022;
Published: 29 September 2022.
Edited by:
Gokhan Zengin, Selcuk University, TurkeyReviewed by:
Stanislav Kotlyarov, Ryazan State Medical University named after academician I.P. Pavlov, RussiaPuja Gulati, Desh Bhagat University, India
Copyright © 2022 Singla, Dhonchak, Sodhi, Arockia Babu, Madan, Madaan, Kumar, Sharma and Shen. This is an open-access article distributed under the terms of the Creative Commons Attribution License (CC BY). The use, distribution or reproduction in other forums is permitted, provided the original author(s) and the copyright owner(s) are credited and that the original publication in this journal is cited, in accordance with accepted academic practice. No use, distribution or reproduction is permitted which does not comply with these terms.
*Correspondence: Rupinder K. Sodhi, Y2NwLnJ1cGluZGVyQGNnYy5lZHUuaW4=; Bairong Shen, YmFpcm9uZy5zaGVuQHNjdS5lZHUuY24=
†These authors have contributed equally to this work and share first authorship