- 1Department of Anesthesiology, The Second Affiliated Hospital, Chongqing Medical University, Chongqing, China
- 2Department of Preventive Medicine, West China School of Public Health, Sichuan University, Chengdu, Sichuan, China
The role of daytime variation in the comprehensive pharmaceutical effects of commonly used opioid analgesics in clinical setting remains unclear. This study aimed to explore the differences in daytime variation among elective surgery patients who were scheduled to receive preemptive analgesia with equivalent doses of sufentanil, dezocine, and tramadol in the morning and afternoon. The analgesic effect was assessed by changes in the pressure pain threshold before and after intravenous administration of sufentanil, dezocine, and tramadol. Respiratory effects were evaluated using pulse oximetry, electrical impedance tomography, and arterial blood gas analysis. Other side effects, including nausea, sedation, and dizziness, were also recorded, and blood concentration was measured. The results showed that the analgesic effects of sufentanil, dezocine, and tramadol were significantly better in the morning than in afternoon. In the afternoon, sufentanil had a stronger sedative effect, whereas dezocine had a stronger inhibitory respiratory effect. The incidence of nausea was higher in the morning with tramadol. Additionally, significant differences in different side effects were observed among three opioids. Our results suggest that the clinical use of these three opioids necessitates the formulation of individualized treatment plans, accounting for different administration times, to achieve maximum analgesic effect with minimal side effects.
Introduction
Every year, more than 312.9 million operations are performed worldwide (Weiser et al., 2008; Weiser et al., 2016; Song and Zhu, 2021). Opioids are among the most important and commonly used intravenous analgesics for perioperative analgesia. However, opioid analgesics are often accompanied by various side effects, including respiratory depression, sedation, dizziness, and nausea (Nakatani, 2017; Baldo, 2021). Excessive drug use can lead to an increased incidence of complications, while inadequate drug use can lead to insufficient analgesic effect, which significantly affects perioperative recovery. Previous studies regarding the influence of time variation on pharmaceutical efficacy have indicated that the selection of drug treatment at different time points is important for achieving the best efficacy with minimum dose and minimal side effects (Lemmer, 1995; Warltier David et al., 2004; Saleem et al., 2019; Zaki et al., 2019). Exploring the role of time variation in the pharmaceutical effect of analgesics is expected to be beneficial for perioperative patients.
The diurnal effects of opioid efficacy and treatment sensitivity have been observed in previous studies. For example, it was found that the analgesic effect of morphine had circadian rhythm changes in mice, and that it was strongest at night (Yoshida et al., 2003; Yu et al., 2015). In addition, studies in healthy volunteers have found that oral tramadol exerts a stronger analgesic effect at night (Hummel et al., 1995). However, these studies were confined to animals or healthy volunteers, and the time points were mainly focused on morning and night hours. Additionally, the side effects of these opioids have been less explored. In clinical practice, most surgeries and therapeutic treatments are performed during the daytime (Cifarelli et al., 2021); therefore, it is more important to explore the potential differences in the clinical and side effects of analgesics between morning and afternoon hours.
Currently, in clinical practice, the most commonly used opioid analgesics are pure µ-receptor agonists, such as sufentanil and norepinephrine, 5-hydroxytryptamine reuptake inhibitors, such as tramadol, and k-receptor agonists and μ-receptor antagonists, such as dezocine. These three types of opioid analgesics have different mechanisms of action (Bravo et al., 2017; Ye et al., 2021). At present, the effects of daytime variations on the pharmaceutical effects of these three opioids remain unclear. In addition, direct clinical evidence is lacking to comprehensively evaluate the clinical effect of these three types of opioid analgesics. Based on the above information, this study aimed to comprehensively evaluate and compare the pharmaceutical effects of these analgesics by experimental pain measurement, real-time continuous pulmonary ventilation monitoring, and cardiovascular parameter monitoring.
Materials and methods
Subjects
The study was designed as a prospective matched observational study to determine the role of daytime variation in the pharmaceutical effects of three types of commonly used opioid analgesics with different mechanisms. The research protocol was in accordance with the tenets of Declaration of Helsinki and approved by the Medical Ethics Committee of the Second Affiliated Hospital of Chongqing Medical University and registered at www.chictr.org.cn [Registration number: ChiCTR2100044369 (Tramodol), ChiCTR2100050360 (Dezocine) and ChiCTR2100053467 (Sufentanil)]. All subjects signed a written informed consent form prior to inclusion in the study. All study data can be obtained by email from the corresponding author on reasonable request.
A total of 300 patients who required pre-analgesia before their operations at the Second Affiliated Hospital of Chongqing Medical University from March 2021 to May 2022 were included. In clinical practice most of the surgeries and treatments were performed in day-time (from 07:00 to 17:00), and 12:00 is commonly used as the cutoff time point between morning and afternoon, thus in this study subjects were grouped as “07:00 to 12:00” and “12:00–17:00”. Considering these three opioids are wildly used clinically, we selected tramadol, dezocine and sufentanil as investigative analgesics. Patients in this study were sequentially enrolled in the tramadol (n = 100), dezocine (n = 100), and sufentanil (n = 100) experiments. Patients in each experiment were divided into the morning (M group, 07:00 to 12:00, n = 50) and afternoon groups (A group, 12:00 to 17:00, n = 50) according to administration time.
Inclusion criteria were: 18–65 years old; 18.5 ≤ Body Mass Index (BMI) ≤ 28; American Society of Anesthesiologists grade I-II; patients undergoing elective surgery. Exclusion criteria were: long-term use of analgesics and psychotropic drugs [including opioids, nonsteroidal anti-inflammatory drugs (NSAIDs), sedatives, and antidepressants]; alcohol abuse, liver and renal dysfunction; history of opioid allergy; use of sedatives and antiemetic drugs within 24 h; use of monoamine oxidase inhibitors or antidepressants within 15 days; high risk of emergency, satiety, and reflux aspiration; history of epilepsy; history of severe chronic obstructive pulmonary disease; severe or uncontrolled bronchial asthma; pulmonary infections; severe heart disease; pregnant or lactating women; and an inability to cooperate with the study for any reason.
Study protocol
After entering the operating room, the subjects’ demographic and physiological data at baseline and the Huaxi Emotional Index were collected (Wang et al., 2017). Electrocardiography, blood pressure, arterial blood gas analysis (ABGs), SPO2 and Modified Observer’s Assessment of Alertness/Sedation (MOAA/S) were monitored before drug administration. Baseline pressure pain threshold (PPT) was measured. Electrical Impedance Tomography (EIT) monitoring was performed to record the baseline respiratory rate and change in end-expiratory lung impedance (Δ EELI). During the post-dosing test, subjects were treated with deep breathing if SPO2 was lower than 85% and oxygen inhalation via mask if SPO2 was lower than 80%.
As shown in Figure 1A, subjects in the sufentanil group were given sufentanil 0.15 μg/kg intravenously over 1 min. PPT and MOAA/S scores were tested at 3 min (onset time of analgesic effect) and 10 min (time when the peak drug concentration was reached) after administration. ABGs were performed again 10 min after administration. We recorded ΔEELI at 3 and 10 min after administration through EIT monitoring.
In addition, as shown in Figure 1B, subjects in the dezocine group were given dezocine 0.15 mg/kg intravenously over 1 min. PPT and MOAA/S scores were tested at 5 min (onset time of analgesic effect) and 15 min (time when the peak drug concentration was reached) after administration. We performed ABGs again 15 min after administration. We recorded ΔEELI at 5 and 15 min after administration using EIT monitoring.
Subjects in the tramadol group were given tramadol 1.5 mg/kg intravenously over 1 min. PPT and MOAA/S scores were tested at 10 min (onset time of analgesic effect) and 20 min (when the peak drug concentration was reached) after administration. ABGs were performed again 20 min after administration. ΔEELI at 10 and 20 min after administration was recorded using EIT monitoring (Figure 1C).
Analgesic effect measurement
Quantitative sensory tests including PPT have been widely used to assess analgesic effect in many previous studies (Hermans et al., 2018; Schliessbach et al., 2018; Ho et al., 2020). Thus, in this study the primary outcome was a normalized value compared to the baseline PPT after analgesic administration. The PPT was measured using a hand-held electro-mechanical algorithm instrument (YISIDA-DS2; Hong Kong, China), which consisted of a pressure sensor with a probe surface area of 0.1 cm2 (Duan et al., 2014; Duan et al., 2015). The right tibialis anterior muscle, which was approximately 1–2 cm lateral to the middle tibia, was selected as the test site. Two adjacent sites at this location were marked to ensure that the investigator could repeat PPT measurements at approximately the same measurement site. The subjects lay flat on the push table in a relaxed position and were subjected to continuous pressure at approximately the same rate (0.3 kg/s) during the test. In order to avoid unnecessary tissue damage, the maximum pressure did not exceed 5 kg. When the subjects began to feel pain during the stimulation, they were asked to say “pain” and the pressure value was recorded. The average of the values at the two adjacent sites was recorded as the PPT of the subject (Duan et al., 2020).
Respiratory effect evaluation
The secondary outcome in this study was respiratory effects after analgesic use. ABGs were performed and PaO2 and PaCO2 were recorded to assess the overall effect of analgesic use on the respiratory system. EIT represented a noninvasive bedside method of monitoring the respiratory rate and impedance changes associated with different pulmonary ventilation statuses, especially those related to regional ventilation impedance change, to reflect the regional features of the lung (Zhang et al., 2020). In this study, EIT monitoring was used to record the effects of analgesics on respiration. There is a good correlation between the change in end-expiratory lung impedance (EELI) and the change in end-expiratory lung volume (Moerer et al., 2011). The change in EELI (ΔEELI) was measured relative to baseline EELI. Desaturation and bradypnea were also recorded. Desaturation was defined as SpO2 < 95% during the entire procedure after analgesic administration, and bradypnea was defined as a respiratory rate < 8.
Other outcomes
In this study, other outcomes mainly included the incidence of sedation, dizziness, nausea, and vomiting. Sedation was defined as a MOOA/S score of < 5, and all subjects were assessed twice after analgesic administration. Dizziness was evaluated by interviewing the subjects during the entire test procedure. Vomiting was recorded according to observations after analgesic administration, and nausea was assessed after the study procedure. The numeric rating scale (NRS, 0 representing none and 10 representing the worst imaginable) for nausea was recorded.
Serum detection
Blood specimens (3 ml) were collected from the radial artery using a vacuum tube with heparin after the last pressure pain measurement in all subjects. Serum was separated and stored at −80°C. Sufentanil and tramadol concentrations were detected using an enzyme-linked immunosorbent assay (ELISA) kit. The concentration of dezocine was determined using high performance liquid chromatography (HPLC).
Statistical analysis
This study was considered as an exploratory study to determine the role of daytime variation on the pharmaceutical effects of three types of commonly used opioid analgesics. Therefore, we primarily included the same sample size for different opioid analgesics. According to the primary outcome of this study, that is, normalized value compared to baseline PPT after analgesic administration, a power calculation showed that the current sample size for three types of opioid analgesics could reach a power > 0.8.
Statistical analysis was performed using SPSS 21.0, and two-sided p < 0.05 was considered statistically significant. Normally distributed quantitative data were described as mean ± standard deviation, and abnormally distributed data were presented as median (interquartile range). Qualitative data are presented as numbers (percentages). Independent sample T test, non-parametric Mann–Whitney test, or two-way repeated measures analysis of variance (ANOVA) were used to compare the morning and afternoon groups according to whether the data were normally distributed. Chi-square test or Fisher’s exact test was used to compare the incidence between the morning and afternoon groups.
Because the analgesic effect was better in the morning group, we comprehensively compared the different clinical effects of the three types of analgesics. Differences in various normally distributed quantitative parameters among the three groups were analyzed using ANOVA and post-hoc multiple comparisons with Bonferroni correction. The Kruskal–Wallis test was performed to compare the abnormally distributed data among the three groups and post-hoc multiple comparisons with Bonferroni corrections. Chi-square test or Fisher’s exact test was used for comparison of incidence between the three groups, and multiple comparisons between different analgesics were also corrected by the Bonferroni method.
Results
As shown in Figure 2, in the study 3 subjects in sufentanil experiment, seven subjects in the dezocine experiment and 5 subjects in the tramadol experiment were lost to follow-up. Thus, 96 patients receiving sufentanil (48 in each morning and afternoon group), 93 patients receiving dezocine (47 in the morning group and 46 in the afternoon group), and 95 patients receiving tramadol (48 in the morning group and 47 in the afternoon group) were included in the final analysis. The demographic and baseline characteristics of all included subjects in the morning and afternoon groups are listed in Table 1, and no significant differences were found in the three experiments.
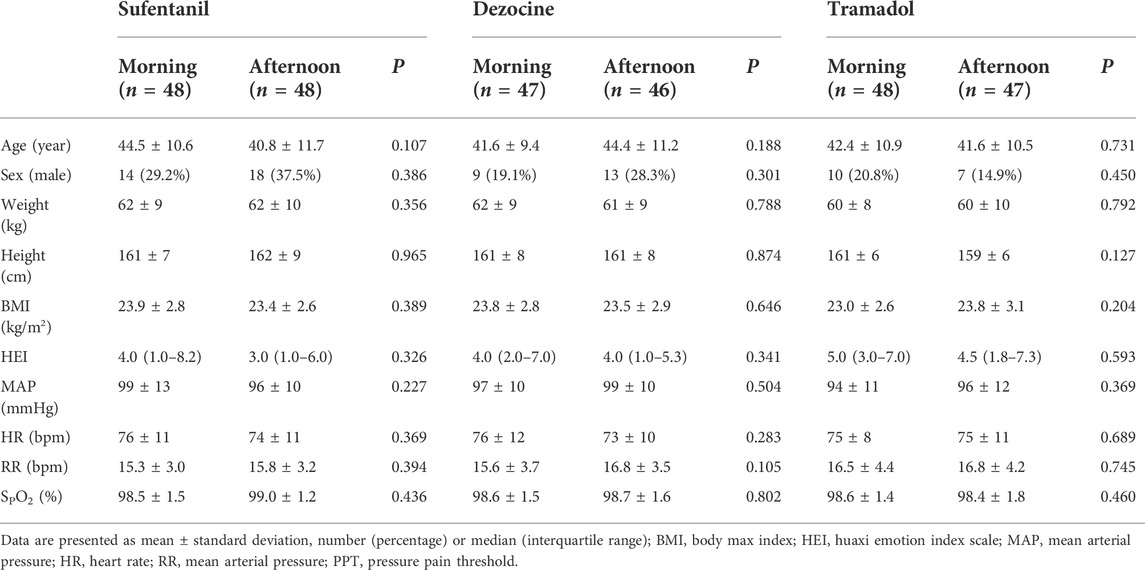
TABLE 1. Demographic and baseline characteristics for all included subjects in the morning and afternoon groups.
Two-way repeated ANOVA analysis showed that the interactive effects of time and group for pressure pain threshold at different time points for sufentanil (p < 0.001) and tramadol (p = 0.001) were statistically significant, but not for dezocine (p = 0.062, Figure 3). In the sufentanil experiment, both of the normalized values compared to baseline PPT at 3 min (1.31 ± 0.37 vs. 1.11 ± 0.29, p = 0.003) and 10 min (1.32 ± 0.39 vs. 1.10 ± 0.29, p = 0.002) in the morning group are higher than those in the afternoon group (Figure 2A). In the dezocine experiment, as shown in Figure 3B the normalized values compared to baseline PPT at 5 min (1.31 ± 0.34 vs. 1.17 ± 0.31, p = 0.046) in the morning group is higher than that in the afternoon group, and no difference was found at 15 min (1.39 ± 0.41 vs. 1.31 ± 0.46, p = 0.363). And in the tramadol experiment, both of the normalized values compared to baseline PPT at 10 min (1.19 ± 0.27 vs. 1.07 ± 0.27, p = 0.028) and 20 min (1.25 ± 0.33 vs. 1.04 ± 0.34, p = 0.003) in the morning group are higher than those in the afternoon group (Figure 3C).
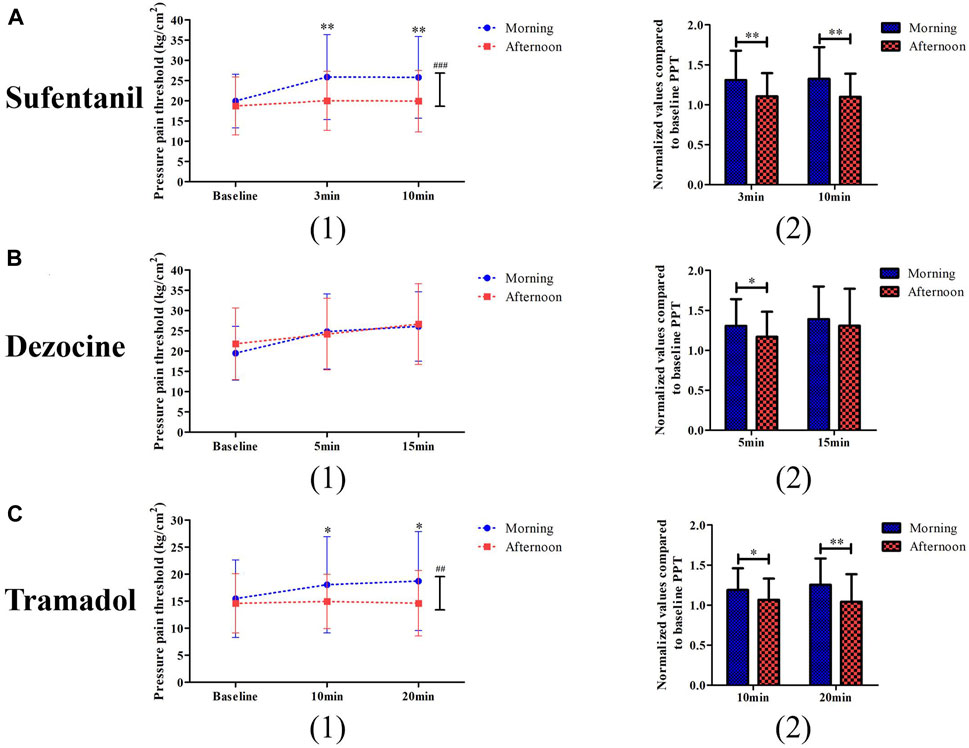
FIGURE 3. Comparisons of pressure pain threshold (1) and its changed levels (2) between the morning group and afternoon group for patients receiving sufentanil treatment (A), dezocine treatment (B) and tramadol treatment (C). (*p < 0.05 and **p < 0.01 compared with the afternoon group; ##p < 0.01 and ###p < 0.001 represents the time and group effects for two way repeat ANOVA analysis).
No significant difference was found in blood pressure or heart rate during the study procedure for the different analgesic experiments (Supplementary Figure S1). In the two-way repeated analysis of PaO2 (p < 0.001) and PaCO2 (p = 0.014), the interactive effects of time and group for dezocine were statistically significant, and no significant difference was found in the sufentanil and tramadol experiments (Figure 4). In the dezocine experiment, both normalized values compared to baseline PaO2 (1.31 ± 0.37 vs. 1.11 ± 0.29, p = 0.003) and PaCO2 (1.32 ± 0.39 vs. 1.10 ± 0.29, p = 0.002) were higher in the morning group than in the afternoon group. The other outcomes of the morning and afternoon groups are listed in Table 2. In the sufentanil experiment, the incidence of sedation (52.1% vs. 22.9%, p = 0.003) 10 min after analgesic use in the afternoon group was significantly higher than in the morning group. In addition, the incidence of nausea (50.0% vs. 29.8%, p = 0.043) during the study procedure was significantly higher in the morning group than in the afternoon group of the tramadol experiment.
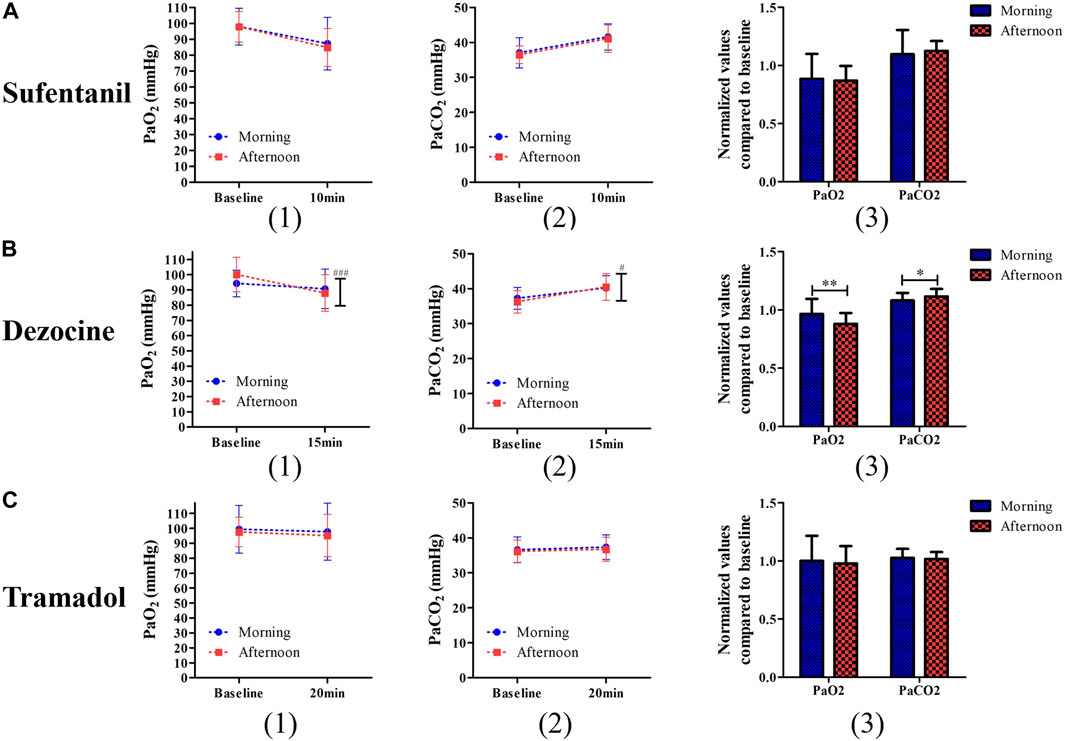
FIGURE 4. Comparisons of PaO2 (1) and PaCO2 (2) and their changed levels (3) after test procedure between the morning group and afternoon group for patients receiving sufentanil treatment (A), dezocine treatment (B) and tramadol treatment (C). (*p < 0.05 and **p < 0.01 compared with the afternoon group; #p < 0.05 and ###p < 0.001 represents the time and group effects for two way repeat analysis of variance).
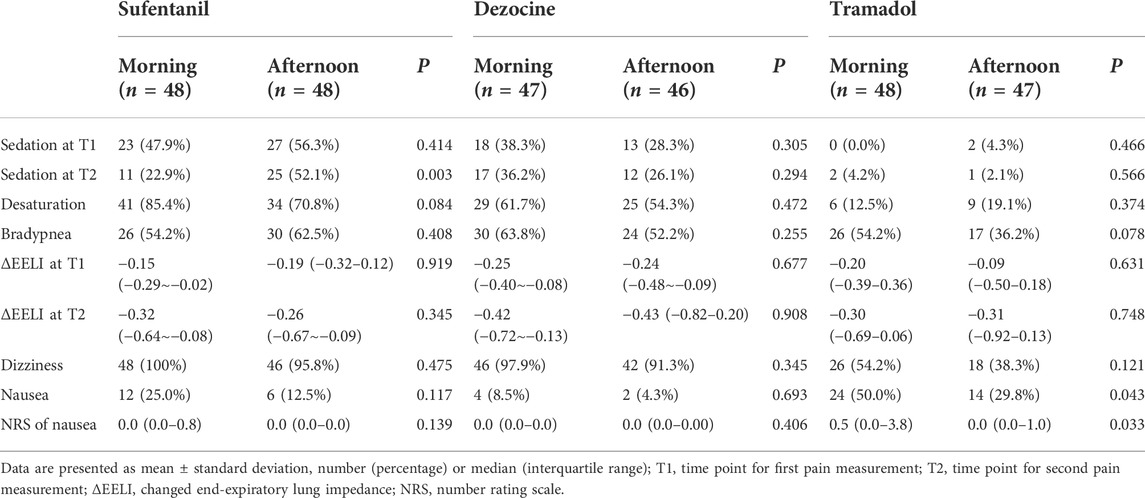
TABLE 2. Comparisons of side effects between the morning and afternoon groups after different analgesic using.
The blood detection results between the morning and afternoon groups after different analgesic uses are listed in Table 3. In the sufentanil experiment, no significant difference was found in sufentanil concentration between the morning and afternoon groups. In the tramadol experiment, tramadol concentration (658 ± 241 vs. 0.915 ± 352 ng/ml, p < 0.001) in the morning group was lower than that in the afternoon group. Finally, no significant differences were observed in the dezocine experiment.
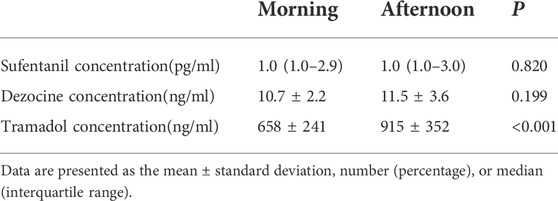
TABLE 3. Comparisons of blood detection results between the morning and afternoon groups after different analgesic using.
Comparisons of analgesic effects and other side effects in the morning group among different analgesics are shown in Figure 5, and no significant differences existed in demographic and baseline characteristics among the sufentanil, dezocine, and tramadol groups (Supplementary Table S1). No significant difference was found in normalized values compared with baseline PPT among the different analgesics. The incidences of sedation, dizziness, and desaturation in the tramadol group were lower than those in the other groups. The normalized value compared to the baseline PaO2 in the tramadol group was higher than that of the sufentanil group, and the normalized value compared to the baseline PaCO2 was significantly lower in the tramadol group. However, the incidence of nausea was significantly higher in the tramadol group than in the sufentanil and dezocine groups.
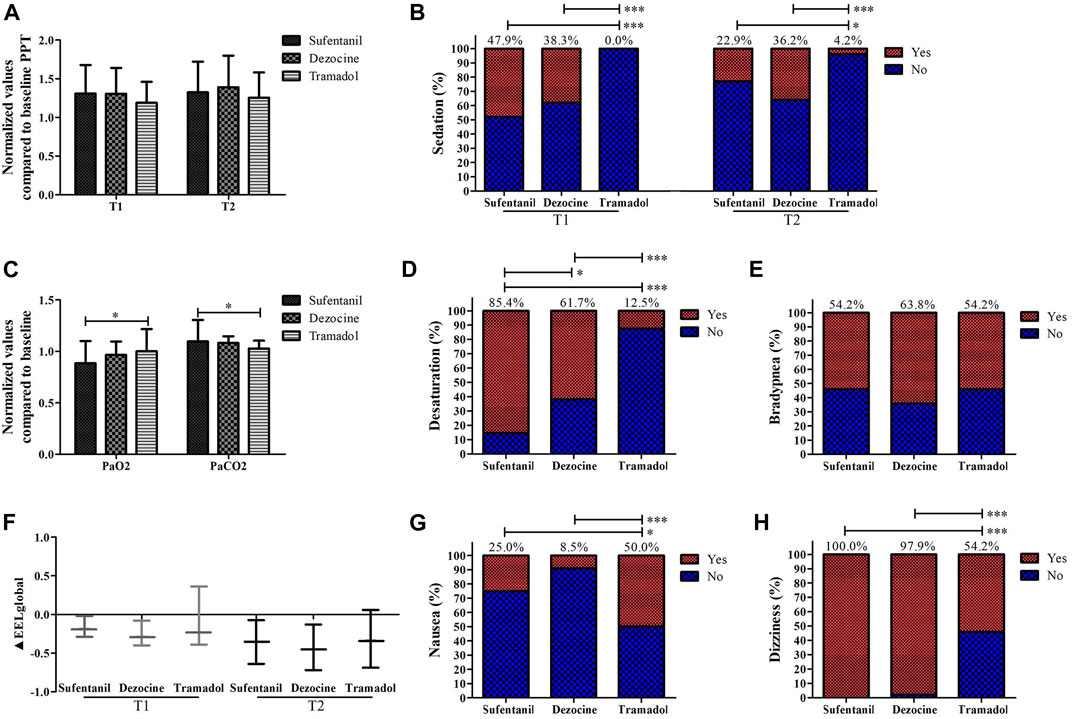
FIGURE 5. Comparisons of the values of analgesic effects (A), sedation (B), PaO2 and PaCO2 (C), the incidences of desaturation (D), bradypnea (E), ΔEELI (F); nausea (G) and dizziness (H) in the morning group for patients receiving sufentanil, dezocine and tramadol. (T1, onset time of analgesic effect; T2, time of peak concentration; *p < 0.05, **p < 0.01 and ***p < 0.001 compared with the afternoon group).
Discussion
In contrast to previous studies on animals or small numbers of volunteers, which focused on the differences between day and night administration of analgesics, this study included patients undergoing elective surgery under general anesthesia and observed the difference in the analgesic effect of intravenous equivalent doses of tramadol, dezocine, and sufentanil in the morning and afternoon. Our study also investigated differences in cardiovascular reactions, respiratory depression, nausea, and vomiting at different time points. Therefore, this study has direct guiding significance in clinical practice. In this study, we found that the intravenous administration of sufentanil, dezocine, and tramadol in the morning produced a better analgesic effect than in the afternoon. In terms of side effects, tramadol is more prone to nausea and vomiting in the morning, dezocine is more prone to hypoxemia and hypercapnia in the morning, and sufentanil is more prone to sedation in the afternoon. In addition, when opioid analgesics with different mechanisms are used in the morning, there are significant differences in the occurrence of adverse reactions under similar analgesic effects.
Previous studies have explored the chronopharmacology of μ-receptor-activated analgesics in different ways. Some studies have found that the analgesic effect in the dark phase is greater than in the light phase after morphine injection in mice, and this difference is related to the degree of μ-receptor expression in the brain (Yoshida et al., 2003; Yu et al., 2015). In this study, we determined that intravenous sufentanil administration in the morning produces a better analgesic effect. Pharmacology and pharmacokinetics may affect the time-related pharmacodynamics (Dallmann et al., 2014). In this study, we found that there was no difference in the blood concentration of sufentanil in the morning and afternoon when the blood concentration of sufentanil reached its peak, which indicated that the time difference in the analgesic effect of sufentanil might be due to pharmacological rather than pharmacokinetic differences. It is known that sufentanil mainly activates the μ-receptor, hyperpolarizes the postsynaptic membrane through the G-protein coupling mechanism, and prevents the conduction of pain impulses, thus exerting an analgesic effect (Pasternak, 1993; Zöllner and Stein, 2007; Zöllner and Schäfer, 2008). A previous study found that the expression of μ-receptors in the periaqueductal gray at 14:00 p.m. was significantly higher than that at 8:00 a.m. in mouse models (Takada et al., 2013). In contrast, we speculate that in humans, the level of μ-receptors in the periaqueductal gray is higher in the morning than in the afternoon. Therefore, the difference in sufentanil efficacy between the morning and afternoon may be due to the circadian rhythm of μ-receptor expression. In addition, we found that the incidence of the sedative effect of sufentanil after 10 min of administration in the afternoon was higher than that in the morning. Sufentanil can combine with the μ-receptor of the central nervous system to exert analgesic and sedative effects, and can also activate presynaptic receptors on GABA neurons to reduce central excitability (Zöllner and Stein, 2007; Trescot et al., 2008; Rocha et al., 2011; Huang et al., 2020). Animal studies have shown that the GABA neuronal response has a significant rhythm, and compared with ZT2, GABA neuronal reactivity at ZT6 is significantly lower (Wagner et al., 1997; Schellinger et al., 2022). In contrast, it can be speculated that GABA neuronal reactivity is stronger in the afternoon in humans. Therefore, stronger sedation with opioid application in the afternoon may be related to the time difference in GABA neuronal reactivity.
Dezocine is a κ-receptor agonist and μ-receptor agonist, which is an analgesic widely used in the perioperative period (Liu et al., 2014; Wang et al., 2018; Ye et al., 2021); however at present, there is no research to explore the chronopharmacology of this kind of analgesic. In this study, we found that the analgesic effect of dezocine in the morning was better than that in the afternoon; however, the time difference was not as obvious as that of sufentanil, which may be related to its different analgesic mechanisms. The blood test results showed that there was no significant difference in the blood concentration of dezocine at different times. Therefore, similar to sufentanil, we speculate that the reason for the time difference in the analgesic effect is related to the time difference in pharmacology. However, in the side effects we found that PaO2 decreased and PaCO2 increased significantly after dezocine administration in the afternoon compared to that in the morning. However, although the respiratory rate and pulmonary volume detected by EIT both decreased in the morning and afternoon, there was no difference in respiratory rate and EIT results between the different time durations. Thus, we speculated that dezocine administration in the afternoon might have a more significant inhibitory effect on the reactivity of the respiratory center. Activation of the μ-receptor can inhibit excitatory synaptic transmission in the respiratory center (Varga et al., 2020; Baertsch et al., 2021; Liu et al., 2021), while the expression of the μ-receptor may be lower in the afternoon than in the morning. Thus, the effect of antagonism of the μ-receptor for dezocine may also be weaker in the afternoon, so the application of dezocine in the afternoon can more strongly inhibit the respiratory network of the brain stem. However, this requires further validation in the future. Nevertheless, it is necessary consider this time difference in the clinical application of dezocine, and special attention should be paid to respiratory monitoring when using dezocine in the afternoon.
Tramadol is an inhibitor of serotonin and norepinephrine reuptake and has a μ-receptor excitatory effect (Grond and Sablotzki, 2004; Ogbemudia et al., 2022). One study had performed painful stimulation of the nasal mucosa of 18 healthy volunteers and found that the analgesic effect produced by oral tramadol at night was stronger (Hummel et al., 1995); in contrast to this study, we explored the role of daytime variation in the analgesic effect of intravenous tramadol, and the results showed that it was stronger in the morning, than in the afternoon. The degree and incidence of nausea and vomiting after tramadol administration in the morning were also higher than those in the afternoon. Blood sample tests show that the serum concentration of tramadol in the afternoon is significantly higher than that in the morning, CYP2D6 is the key metabolic enzyme of tramadol, and CYP2D6 converts tramadol into the active metabolite O-desmethyltramadol (M1) and plays a pharmacological role (Dean and Kane, 2012; Lassen et al., 2015; Miotto et al., 2017). However, previous studies have shown that CYP2D6 is less expressed in the afternoon than in the morning (Matsunaga et al., 2012); therefore it can be speculated that tramadol in the morning can be converted into an active metabolite by the CYP2D6 enzyme. This phenomenon contributes to the lower blood concentration of tramadol in the morning than in the afternoon, thus inducing stronger pharmacological effects in the morning.
The equivalent dose ratios of sufentanil, dezocine, and tramadol to morphine were 1:10000, 1:1 and 10:1 respectively (Pandit et al., 1985; Grond and Sablotzki, 2004; Fentanyl, 2012); thus, the doses of the three drugs in this study were equivalent. Based on the time comparison, we found that the analgesic effect was better in the morning. Therefore, we chose the morning to compare the comprehensive clinical effects of the three drugs. The results showed that there was no difference in the analgesic effect among the three drugs, which was consistent with the clinical equivalent dose. We found that PaO2 decreased and PaCO2 increased most significantly in the sufentanil group, and the incidence of hypoxic saturation was also significantly higher than in the other two groups, followed by dezocine; however, there was no significant difference in respiratory rate and EIT. This may be directly related to the pharmacological mechanisms of the three types of opioids. Respiratory depression is one of the most harmful side effects of opioids, and this finding might be helpful for choosing type and doses of analgesics when treatment is administered in the morning.
Regarding other side effects, we found that the incidence of nausea and vomiting caused by intravenous tramadol was the highest among the three drugs. The causes of nausea and vomiting caused by tramadol may be various, and their occurrence may be related to the activation of μ-receptors and 5-HT receptors in the chemical receptor trigger region (CTZ) of vomiting center in the fourth ventricle of the brain (Mallick-Searle and Fillman, 2017). Therefore, the incidence of adverse reactions of nausea and vomiting caused by intravenous tramadol was significantly higher than that caused by the other two opioids. When tramadol is administered in the morning, it is necessary to consider the occurrence of nausea and vomiting. In addition, compared to tramadol, sufentanil and dezocine produced significantly more sedative effects and dizziness. Therefore, when clinicians select drugs to make pain treatment plans, they should fully consider the differences in adverse reactions to opioids via different mechanisms.
Although the current research has yielded several interesting findings, it is necessary to consider the limitations of this research. First, while we observed the time-efficacy rhythms of opioid analgesics with different mechanisms at different time points, we only studied drugs commonly used in clinics at these times in the morning and afternoon, and further studies are needed to explore their complete circadian rhythm in other periods. Second, this study observed three representative opioids with different mechanisms; other opioids such as oxycodone, hydromorphone, and naborphine were not included. Because of the differences in drug structures, the specific time-pharmacodynamics of these analgesics in clinical practice remains unclear. Thirdly, this study didn’t record the subjects’ sleep time, which may be one confounding factor for difference of opioids’ pharmacological effects between the morning and afternoon, and its potential effects needs further exploration. In addition, the patients included in this study were selected according to relatively strict inclusion and exclusion criteria; thus, the role of daytime variation in these opioids’ clinical pharmaceutical effects for patients with various complications needs to be further explored. For example, some differences induced by daytime variations may be amplified or reduced by certain complications.
In this study, the clinical effects of these three commonly used opioid analgesics were comprehensively evaluated and compared using an experimental pain measurement method combined with real-time continuous monitoring technology and evaluated at different time points. Sufentanil, dezocine, and tramadol, which were intravenously administered in the morning had better analgesic effects, and the side effects of the three drugs also differed at different times. In addition, the side effects of the three drugs used during the same period were significant. Therefore, when clinicians use these three kinds of opioids in their daily clinical practice, it is necessary to formulate individualized treatment plans according to different times and patient conditions to obtain maximum analgesic effect and minimum side effects.
Data availability statement
The raw data supporting the conclusion of this article will be made available by the authors, without undue reservation.
Ethics statement
The studies involving human participants were reviewed and approved by Medical Ethics Committee of the Second Affiliated Hospital of Chongqing Medical University. The patients/participants provided their written informed consent to participate in this study.
Author contributions
GD, LD, and HH contributed to study design; WG, XY, JC, AY, GC, SL, and YZ contributed to data collection; WG and HL contributed to data analysis; GD, WG, and HH contributed to writing the manuscript.
Funding
This work was supported by the National Natural Science Foundation of China (No. 82171210) and Kuanren Talents’ Project of The Second Affiliated Hospital of Chongqing Medical University.
Acknowledgments
We thank all the participants who contribute the selfless help to us.
Conflict of interest
The authors declare that the research was conducted in the absence of any commercial or financial relationships that could be construed as a potential conflict of interest.
Publisher’s note
All claims expressed in this article are solely those of the authors and do not necessarily represent those of their affiliated organizations, or those of the publisher, the editors and the reviewers. Any product that may be evaluated in this article, or claim that may be made by its manufacturer, is not guaranteed or endorsed by the publisher.
Supplementary material
The Supplementary Material for this article can be found online at: https://www.frontiersin.org/articles/10.3389/fphar.2022.993506/full#supplementary-material
SUPPLEMENTARY FIGURE S1 | Comparisons of the mean arterial pressure (1) and heart rate (2) during test procedure between the morning and afternoon groups for patients receiving sufentanil (A), dezocine (B) and tramadol (C).
Abbreviations
ABGs, arterial blood gas analysis; EIT, electrical Impedance Tomography; EELI, end-expiratory lung impedance; ELISA, enzyme-linked immunosorbent assay; HPLC, high-performance liquid chromatography; MOAA/S, Modified Observer’s Assessment of Alertness/Sedation; NRS, numeric rating scale; PPT, pressure pain threshold.
References
Baertsch, N. A., Bush, N. E., Burgraff, N. J., and Ramirez, J. M. (2021). Dual mechanisms of opioid-induced respiratory depression in the inspiratory rhythm-generating network. Elife 17, e67523. Epub 2021/08/18. doi:10.7554/eLife.67523 |
Baldo, B. A. (2021). Toxicities of opioid analgesics: Respiratory depression, histamine release, hemodynamic changes, hypersensitivity, serotonin toxicity. Arch. Toxicol. 95, 2627–2642. Epub 2021/05/12. doi:10.1007/s00204-021-03068-2 | |
Bravo, L., Mico, J. A., and Berrocoso, E. (2017). Discovery and development of tramadol for the treatment of pain. Expert Opin. Drug Discov. 12, 1281–1291. Epub 2017/09/19. doi:10.1080/17460441.2017.1377697 | |
Cifarelli, C. P., McMichael, J. P., Forman, A. G., Mihm, P. A., Cifarelli, D. T., Lee, M. R., et al. (2021). Surgical start time impact on hospital length of stay for elective inpatient procedures. Cureus 13, e16259. Epub 2021/07/20. doi:10.7759/cureus.16259 | |
Dallmann, R., Brown, S. A., and Gachon, F. (2014). Chronopharmacology: New insights and therapeutic implications. Annu. Rev. Pharmacol. Toxicol. 54, 339–361. Epub 2013/10/29. doi:10.1146/annurev-pharmtox-011613-135923 | |
Dean, L., and Kane, M. (2012). “Tramadol therapy and CYP2D6 genotype,” in Medical genetics summaries (Bethesda (MD): National Center for Biotechnology Information US).
Duan, G., Guo, S., Zhan, H., Qi, D., Zhang, Y., and Zhang, X. (2015). A new real-time method for detecting the effect of fentanyl using the preoperative pressure pain threshold and narcotrend index: A randomized study in female surgery patients. Med. Baltim. 94, e316. Epub 2015/01/09. doi:10.1097/MD.0000000000000316 | |
Duan, G., Wu, Z., Duan, Z., Yang, G., Fang, L., Chen, F., et al. (2020). Effects of spicy stimulation and spicy-food consumption on human pain sensitivity: A healthy volunteer study. J. Pain 21, 848–857. doi:10.1016/j.jpain.2019.11.011 | |
Duan, G., Xiang, G., Zhang, X., Guo, S., and Zhang, Y. (2014). An improvement of mechanical pain sensitivity measurement method: The smaller sized probes may detect heterogeneous sensory threshold in healthy male subjects. Pain Med. 15, 272–280. doi:10.1111/pme.12245 | |
Fentanyl (2012). LiverTox: Clinical and research information on drug-induced liver injury (Bethesda (MD): National Institute of Diabetes and Digestive and Kidney Diseases).
Grond, S., and Sablotzki, A. (2004). Clinical pharmacology of tramadol. Clin. Pharmacokinet. 43, 879–923. Epub 2004/10/29. doi:10.2165/00003088-200443130-00004 | |
Hermans, L., Nijs, J., Calders, P., De Clerck, L., Moorkens, G., Hans, G., et al. (2018). Influence of morphine and naloxone on pain modulation in rheumatoid arthritis, chronic fatigue syndrome/fibromyalgia, and controls: A double-blind, randomized, placebo-controlled, cross-over study. Pain Pract. official J. World Inst. Pain. 18:418–430. doi:10.1111/papr.12613 |
Ho, K. W. D., Wallace, M. R., Staud, R., and Fillingim, R. B. (2020). OPRM1, OPRK1, and COMT genetic polymorphisms associated with opioid effects on experimental pain: A randomized, double-blind, placebo-controlled study. Pharmacogenomics J. 20, 471–481. doi:10.1038/s41397-019-0131-z | |
Huang, Y. H., Wu, Y. W., Chuang, J. Y., Chang, Y. C., Chang, H. F., Tao, P. L., et al. (2020). Morphine produces potent antinociception, sedation, and hypothermia in humanized mice expressing human mu-opioid receptor splice variants. Pain 161, 1177–1190. Epub 2020/02/11. doi:10.1097/j.pain.0000000000001823Jun | |
Hummel, T., Kraetsch, H. G., Lotsch, J., Hepper, M., Liefhold, J., and Kobal, G. (1995). Analgesic effects of dihydrocodeine and tramadol when administered either in the morning or evening. Chronobiol. Int. 12, 62–72. Epub 1995/02/01. doi:10.3109/07420529509064501 | |
Lassen, D., Damkier, P., and Brøsen, K. (2015). The pharmacogenetics of tramadol. Clin. Pharmacokinet. 54, 825–836. Epub 2015/04/26. doi:10.1007/s40262-015-0268-0 | |
Lemmer, B. (1995). Clinical chronopharmacology: The importance of time in drug treatment. Ciba Found. Symp. 183, 235–247. ; discussion 247-253. Epub 1995/01/01. doi:10.1002/9780470514597.ch13 | |
Liu, R., Huang, X. P., Yeliseev, A., Xi, J., and Roth, B. L. (2014). Novel molecular targets of dezocine and their clinical implications. Anesthesiology 120, 714–723. Epub 2013/11/23. doi:10.1097/ALN.0000000000000076 | |
Liu, S., Kim, D. I., Oh, T. G., Pao, G. M., Kim, J. H., Palmiter, R. D., et al. (2021). Neural basis of opioid-induced respiratory depression and its rescue. Proc. Natl. Acad. Sci. U. S. A., 118, e2022134118. Epub 2021/06/03. doi:10.1073/pnas.2022134118 | |
Mallick-Searle, T., and Fillman, M. (2017). The pathophysiology, incidence, impact, and treatment of opioid-induced nausea and vomiting. J. Am. Assoc. Nurse Pract. 29, 704–710. Epub 2017/11/14. doi:10.1002/2327-6924.12532 | |
Matsunaga, N., Inoue, M., Kusunose, N., Kakimoto, K., Hamamura, K., Hanada, Y., et al. (2012). Time-dependent interaction between differentiated embryo chondrocyte-2 and CCAAT/enhancer-binding protein α underlies the circadian expression of CYP2D6 in serum-shocked HepG2 cells. Mol. Pharmacol. 81, 739–747. Epub 2012/02/23. doi:10.1124/mol.111.076406 | |
Miotto, K., Cho, A. K., Khalil, M. A., Blanco, K., Sasaki, J. D., and Rawson, R. (2017). Trends in tramadol: Pharmacology, metabolism, and misuse. Anesth. Analg. 124, 44–51. Epub 2016/11/20. doi:10.1213/ANE.0000000000001683 | |
Moerer, O., Hahn, G., and Quintel, M. (2011). Lung impedance measurements to monitor alveolar ventilation. Curr. Opin. Crit. Care 17, 260–267. Epub 2011/04/12. doi:10.1097/MCC.0b013e3283463c9c | |
Nakatani, T. (2017). Opioid therapy and management of side effects associated with opioids. Gan Kagaku Ryoho. 44, 294–297. Epub 2017/04/22.
Ogbemudia, B., Qu, G., Henson, C., Esfandiary, L., Przkora, R., and Victor, S. (2022). Tramadol use in perioperative care and current controversies. Curr. Pain Headache Rep. 26, 241–246. Epub 2022/02/19. doi:10.1007/s11916-022-01021-1 | |
Pandit, S. K., Kothary, S. P., Pandit, U. A., and Kunz, N. R. (1985). Double-blind placebo-controlled comparison of dezocine and morphine for post-operative pain relief. Can. Anaesth. Soc. J. 32, 583–591. Epub 1985/11/01. doi:10.1007/BF03011403 | |
Pasternak, G. W. (1993). Pharmacological mechanisms of opioid analgesics. Clin. Neuropharmacol. 16, 1–18. Epub 1993/02/01. doi:10.1097/00002826-199302000-00001 | |
Rocha, A. P., Barros, G. A., Nascimento Jdos, S., Santos, K. P., Vasconcelos, L. M., and Castro, P. A. (2011). Sedation with sufentanil and clonidine in patients undergoing heart catheterization. Arq. Bras. Cardiol. 96, 219–226. Epub 2011/01/29. doi:10.1590/s0066-782x2011005000012 | |
Saleem, U., Iman, S., Akhtar, M. F., Saleem, A., Anwar, F., and Ahmad, B. (2019). Chronopharmacology: Appraising the influence of biorhythms on the efficacy and safety of antihypertensive drugs. Crit. Rev. Eukaryot. Gene Expr. 29, 499–509. Epub 2019/01/01. doi:10.1615/CritRevEukaryotGeneExpr.2019028841 | |
Schellinger, J. N., Sun, Q., Pleinis, J. M., An, S. W., Hu, J., Mercenne, G., et al. (2022). Chloride oscillation in pacemaker neurons regulates circadian rhythms through a chloride-sensing WNK kinase signaling cascade. Curr. Biol. 28 (32), 1429–1438.e6. doi:10.1016/j.cub.2022.03.017 |
Schliessbach, J., Siegenthaler, A., Butikofer, L., Vuilleumier, P., Juni, P., Stamer, U., et al. (2018). Predicting drug efficacy in chronic low back pain by quantitative sensory tests. Eur. J. Pain 22, 973–988. doi:10.1002/ejp.1183 | |
Song, B., and Zhu, J. (2021). A novel application of ketamine for improving perioperative sleep disturbances. Nat. Sci. Sleep. 13, 2251–2266. Epub 2022/01/08. doi:10.2147/NSS.S341161 | |
Takada, T., Yamashita, A., Date, A., Yanase, M., Suhara, Y., Hamada, A., et al. (2013). Changes in the circadian rhythm of mRNA expression for µ-opioid receptors in the periaqueductal gray under a neuropathic pain-like state. Synapse 67, 216–223. doi:10.1002/syn.21633 | |
Trescot, A. M., Datta, S., Lee, M., and Hansen, H. (2008). Opioid pharmacology. Pain Physician 11, S133–S153. Epub 2008/06/17. doi:10.36076/ppj.2008/11/s133 | |
Varga, A. G., Reid, B. T., Kieffer, B. L., and Levitt, E. S. (2020). Differential impact of two critical respiratory centres in opioid-induced respiratory depression in awake mice. J. Physiol. 598, 189–205. Epub 2019/10/08. doi:10.1113/JP278612 | |
Wagner, S., Castel, M., Gainer, H., and Yarom, Y. (1997). GABA in the mammalian suprachiasmatic nucleus and its role in diurnal rhythmicity. Nature 387, 598–603. doi:10.1038/42468 | |
Wang, J., Guo, W. J., Zhang, L., Deng, W., Wang, H. Y., Yu, J. Y., et al. (2017). The development and validation of Huaxi emotional-distress index (HEI): A Chinese questionnaire for screening depression and anxiety in non-psychiatric clinical settings. Compr. Psychiatry 76, 87–97. Epub 2017/04/27. doi:10.1016/j.comppsych.2017.04.001 | |
Wang, Y. H., Chai, J. R., Xu, X. J., Ye, R. F., Zan, G. Y., Liu, G. Y., et al. (2018). Pharmacological characterization of dezocine, a potent analgesic acting as a κ partial agonist and μ partial agonist. Sci. Rep. 8, 14087. Epub 2018/09/22. doi:10.1038/s41598-018-32568-y | |
Warltier David, C., Chassard, D., and Bruguerolle, B. (2004). Chronobiology and anesthesia. Anesthesiology 100, 413–427. doi:10.1097/00000542-200402000-00034 | |
Weiser, T. G., Haynes, A. B., Molina, G., Lipsitz, S. R., Esquivel, M. M., Uribe-Leitz, T., et al. (2016). Size and distribution of the global volume of surgery in 2012. Bull. World Health Organ. 94, 201–209f. Epub 2016/03/12. doi:10.2471/BLT.15.159293 | |
Weiser, T. G., Regenbogen, S. E., Thompson, K. D., Haynes, A. B., Lipsitz, S. R., Berry, W. R., et al. (2008). An estimation of the global volume of surgery: A modelling strategy based on available data. Lancet 372, 139–144. Epub 2008/06/28. doi:10.1016/S0140-6736(08)60878-8 | |
Ye, R. R., Jiang, S., Xu, X., Lu, Y., Wang, Y. J., and Liu, J. G. (2021). Dezocine as a potent analgesic: Overview of its pharmacological characterization. Acta Pharmacol. Sin. 4, 1646–1657. Epub 2021/11/06. doi:10.1038/s41401-021-00790-6 |
Yoshida, M., Ohdo, S., Takane, H., Tomiyoshi, Y., Matsuo, A., Yukawa, E., et al. (2003). Chronopharmacology of analgesic effect and its tolerance induced by morphine in mice. J. Pharmacol. Exp. Ther. 305, 1200–1205. Epub 2003/03/11. doi:10.1124/jpet.103.049031 | |
Yu, Z. Q., Zhang, C. L., Xu, Y. J., Chang, M. J., Jin, J. J., Luo, L., et al. (2015). Chronopharmacology of analgesic effect and tolerance induced by six narcotic analgesics in mice. Drug Res. 65, 141–146. Epub 2014/05/02. doi:10.1055/s-0034-1374617 | |
Zaki, N. F. W., Yousif, M., BaHammam, A. S., Spence, D. W., Bharti, V. K., Subramanian, P., et al. (2019). Chronotherapeutics: Recognizing the importance of timing factors in the treatment of disease and sleep disorders. Clin. Neuropharmacol. 42, 80–87. Epub 2019/05/15. doi:10.1097/WNF.0000000000000341 | |
Zhang, R., He, H., Yun, L., Zhou, X., Wang, X., Chi, Y., et al. (2020). Effect of postextubation high-flow nasal cannula therapy on lung recruitment and overdistension in high-risk patient. Crit. Care 24, 82. Epub 2020/03/08. doi:10.1186/s13054-020-2809-7 | |
Zöllner, C., and Schäfer, M. (2008). Opioids in anesthesia. Anaesthesist 57, 729–740. ; quiz 741-722. Epub 2008/08/08. doi:10.1007/s00101-008-1408-9 | |
Keywords: daytime variation, pharmaceutical effect, tramadol, sufentanil, dezocine
Citation: Gan W, Yang X, Chen J, Lyu H, Yan A, Chen G, Li S, Zhang Y, Dan L, Huang H and Duan G (2022) Role of daytime variation in pharmaceutical effects of sufentanil, dezocine, and tramadol: A matched observational study. Front. Pharmacol. 13:993506. doi: 10.3389/fphar.2022.993506
Received: 13 July 2022; Accepted: 30 August 2022;
Published: 16 September 2022.
Edited by:
Anick Bérard, Université de Montréal, CanadaReviewed by:
Zongbin Song, Xiangya Hospital, Central South University, ChinaHong-Bing Xiang, Huazhong University of Science and Technology, China
Copyright © 2022 Gan, Yang, Chen, Lyu, Yan, Chen, Li, Zhang, Dan, Huang and Duan. This is an open-access article distributed under the terms of the Creative Commons Attribution License (CC BY). The use, distribution or reproduction in other forums is permitted, provided the original author(s) and the copyright owner(s) are credited and that the original publication in this journal is cited, in accordance with accepted academic practice. No use, distribution or reproduction is permitted which does not comply with these terms.
*Correspondence: He Huang, aHVhbmdoZUBjcW11LmVkdS5jbg==; Guangyou Duan, ZHVhbmd5QGhvc3BpdGFsLmNxbXUuZWR1LmNu
†These authors have contributed equally to this work