- 1School of Natural Product Studies, Department of Pharmaceutical Technology, Jadavpur University, Kolkata, India
- 2Institute of Bioresources and Sustainable Development, Department of Biotechnology, Government of India, Imphal, India
- 3Department of Pharmaceutical Technology, Jadavpur University, Kolkata, India
Mollugo oppositifolia Linn. is traditionally used in neurological complications. The study aimed to investigate in-vitro neuroprotective effect of the plant extracts through testing against acetylcholinesterase (AChE), butyrylcholinesterase (BChE), and β-secretase linked to Alzheimer’s disease (AD). To understand the safety aspects, the extracts were tested for CYP450 isozymes and human hepatocellular carcinoma cell (HepG2) inhibitory potential. The heavy metal contents were estimated using atomic absorption spectroscopy (AAS). Further, the antioxidant capacities as well as total phenolic content and total flavonoid content (TFC) were measured spectrophotometrically. UPLC-QTOF-MS/MS analysis was employed to identify phytometabolites present in the extract. The interactions of the ligands with the target proteins (AChE, BChE, and BACE-1) were studied using AutoDockTools 1.5.6. The results showed that M. oppositifolia extract has more selectivity towards BChE (IC50 = 278.23 ± 1.89 μg/ml) as compared to AChE (IC50 = 322.87 ± 2.05 μg/ml). The IC50 value against β-secretase was 173.93 μg/ml. The extract showed a CC50 value of 965.45 ± 3.07 μg/ml against HepG2 cells and the AAS analysis showed traces of lead 0.02 ± 0.001 which was found to be within the WHO prescribed limits. Moreover, the IC50 values against CYP3A4 (477.03 ± 2.01 μg/ml) and CYP2D6 (249.65 ± 2.46 μg/ml) isozymes justify the safety aspects of the extract. The in silico molecular docking analysis of the target enzymes showed that the compound menthoside was found to be the most stable and showed a good docking score among all the identified metabolites. Keeping in mind the multi-targeted drug approach, the present findings suggested that M. oppositifolia extract have anti-Alzheimer’s potential.
Introduction
Alzheimer’s disease (AD) is a neurodegenerative disorder and contributes major vote to dementia-related brain complications. The cholinergic hypothesis is mostly considered an important factor and is neurochemically characterized by consistent interruptions in cholinergic neurotransmission which specifically affect the basal forebrain (Chen and Mobley, 2019; Chen et al., 2022). Cumulative evidence suggests that cholinesterase inhibitors (AChE and BChE) can improve cholinergic transmission by blocking the degradation of choline, therefore, serving as a strategy for alleviating the symptoms of AD in patients (Moreta et al., 2021). Currently, donepezil or rivastigmine (AChE inhibitors) were used to alleviate cognitive function in patients with moderate to severe AD (Marucci et al., 2021). Besides, neuropathological changes in AD brains are also manifested by Aβ (beta-amyloid) senile plaques and neurofibrillary tangles deposition where β-site amyloid precursor protein cleaving enzyme (BACE) is the rate-limiting enzyme in the Aβ peptide production (Singh et al., 2016). In the pathway of amyloidogenesis amyloid precursor protein (APP), an integral membrane protein is cleaved by β-secretase and subsequently snipped by γ-secretase. Extracellular assembly of these cleaved protein fragments with intermediate composed into oligomers and protofibrils which has a stellar role in the development of insoluble senile plaques intern disrupting the neuronal networks. Inhibition of Aβ neurotoxic function also has a modifying effect on AD (Hampel et al., 2021). Despite substantial quantities of both forms (BACE-1 and BACE-2) of the β-secretase enzyme, Ahmed et al., 2010 confirmed that the BACE-1 subtype is the major target that could cease the formation of Aβ at the very beginning of APP processing. Verubecestat and LY3314814 are the only available drug candidates that progressed to Phase 3 clinical trials as β-secretase enzyme inhibitors (Zhao et al., 2020).
The multidisciplinary based approach plays a substantial role in the discovery of new drug candidates, for example, galanthamine is one most prevalent cholinesterase inhibitors that are being used clinically in the symptomatic relief of Alzheimer’s disease (Marucci et al., 2021). Physostigmine from Physostigma venenosum Balf. is another AChE inhibitor that provided a scaffold for the development of more effective cholinesterase inhibitors like rivastigmine (Berkov et al., 2021). Besides, there are several other drug candidates under different phases of the clinical trial for example Huperzine A from Huperzia serrata (Thunb.) Trevis. resveratrol from Vitis vinifera L. nicotine from Nicotiana tabacum L. curcumin from Curcuma longa L. (Ayaz et al., 2019).
BACE and cholinesterase inhibitors both of which have serious side effects and this might help to slow down the pathophysiological alterations and be unable to reverse the disease progression. To overcome this limitation, herbal medicine could be a better approach, since they constitute a different class of secondary metabolites targeting multiple signaling pathways or proteins, and show synergistic effects with minimum side effects (Sharma et al., 2017; Bhadra et al., 2022).
Mollugo oppositifolia Linn. (Molluginaceae family) is a glabrous, dioecious annual or perennial shrub traditionally used to treat cough, fever, inflammation, pain, jaundice, abdominal pain, intestine and urinary infections, appetite condition, malaria, viral infections, helminthiasis (AsokKumar et al., 2009; Sahu et al., 2012). This edible herb is locally known as Bakkhate in Mizoram, North-East India, and owns several therapeutic reputations (Khomdram et al., 2019). In Ayurveda, it is used to cure vata, piles, kapha, and leucoderma (Hoque et al., 2011a; 2011b; Sahu et al., 2012). Different solvent extracts of the plant parts were reported to have antioxidant, antimicrobial, anti-diabetic, sedative, and anxiolytic activities (Martin-Puzon et al., 2015; Tanquilut et al., 2019; Zhang et al., 2019; Nagannawar and Jayaraj, 2020).
The plant was reported to contain oleanolic acid; lupeol; dodecanoic acid; heptanoic acid; hexadecanoic acid; stigmasterol; isobornyl acetate; 8,11,14-eicosatrienoic acid (Nagannawar and Jayaraj., 2018 and, 2020 Modi and Shah, 2015). Besides this the plant also contains benzoic acid; 4-hydroxybenzoic acid; vanillin; cinnamic acid; vitexin; spergulacin; spergulagenin-A; linoside A; spergulin A & B; spinasterol, β-sitosterol, stigmasterol; squalene (Traore et al., 2000; Ragasa et al., 2012; Ha et al., 2021).
The present study explores the inhibitory activity of the M. oppositifolia extract against cholinesterase, β-secretase and cytochromeP450 enzymes together with the assessment of antioxidant capacity, TPC, TFC, heavy metal contents, and cytotoxicity potentials. The UPLC-QTOF-MS analysis combined with molecular docking study was performed to access the interaction potential of the phytocompounds towards target enzymes.
Materials and methods
Procurement of plant material and extraction
Aerial parts of M. oppositifolia were collected from a local vegetable market, Jadavpur, Kolkata (West Bengal), and the voucher specimen (IBSD/22/M-001) was maintained at the Institute of Bioresources and Sustainable Development, Manipur, Imphal, India for future reference. The plant sample was cleaned and shade dried till constant weight was attained. Accurately weighed 100 g grounded coarsely powdered crude plant sample was macerated using 500 ml of 80% methanol for 72 h. Then the mixture was filtered through Whatman filter paper (nylon; 0.45 µm) and the filtrate was concentrated by a rotary vacuum evaporator (IKA, Japan). The lyophilized (Instrumentation India, India) extract was packed in a sealed container and stored in the freezer (4°C). The percentage yield was 10.54% w/w.
Chemicals and reagents
AChE (C3389), BChE (C1057), ATCI (01480), BTCI (20820), DTNB (D218200), galanthamine (G1660), ketoconazole (UC280), and fluoxetine (F132) were purchased from Sigma-Aldrich (Steinheim, Germany). β-secretase Activity Assay Kit (ab65357) purchased from Abcam (Cambridge, United Kingdom). Vivid® CYP450 Screening Kit [CYP2D6 (P2972) and CYP3A4 (P2858)] were purchased from Life Technologies (United States). Methanol (≥99.8%) LC grade (Merck-Mumbai, India), 0.45 μ syringe filter (Millipore, Germany). Stock solutions for all the metals (1,000 ppm) were procured from Merck (Darmstadt, Germany). HepG2 cells were obtained from the American Type Culture Collection (ATCC HB 8065). Dulbecco’s modified Eagle’s medium (DMEM), phosphate Buffer Saline (PBS), and fetal bovine serum (FBS) were purchased from Life Technologies Ltd. (Fairlands, Johannesburg, RSA). Dimethyl sulfoxide (DMSO), trypsin, deoxyribose, thiobarbituric acid (TBA), trichloroacetic acid (TCA), MTT, EDTA, FeCl3, H2O2, NaOH, HNO3, HClO4, HCl, and H2SO4 and other reagents were analytical grade (Merck, Germany), All the working solutions were freshly prepared on the day of analysis.
The cell line was maintained in DMEM supplemented with 10% FBS, non-essential amino acids, and sodium pyruvate (1 mM). The cell line was grown at 37°C in a humidified incubator set at 5% CO2 and sub-cultured with 0.25% (w/v) trypsin and 0.53 mM EDTA for a maximum of 15 min every 2–3 days after they had 90% confluent monolayer.
Evaluation of free radical scavenging potential
DPPH radical scavenging assay
The assay was performed in a 96-well microplate as described by Das et al., 2020. The test wells contain 100 μL of the extract solution (50–300 μg/ml) and 100 μL of 0.1 mM DPPH radical solution in methanol. After 30 min of incubation in the dark, the absorbances were measured at 517 nm using a Spectramax iD3 reader (CA, United States). at 28°C. In the positive control group ascorbic acid (AA) was used as the reference standard.
The % inhibitions were calculated using the formula:
(Where A1 and A0 are the absorbances of the control and test samples respectively).
Hydroxyl radical scavenging assay
The assay was performed with slight modifications to the method described by Das et al., 2020 in a 96-well microplate A reaction mixture containing 10 μL of deoxyribose (28 mM) in phosphate buffer (50 mM, pH 7.4), 10 μL of FeCl3 (1 mM), 10 μL of EDTA (1 mM), 10 μL of H2O2 (1 mM) was prepared. To the test and positive control wells 100 μL each of the extract and ascorbic acid (reference standard) dissolved in methanol (50–300 μg/ml) were added. After 1 h of incubation at 42°C, the reaction was stopped by adding 50 μL of 50 μL of 0.5% TBA and 10% TCA in NaOH solution (50 mM) and incubated over again for 30 min at 42°C. Similarly, a control was prepared. The absorbances were recorded after 30 min of incubation at 42°C at 532 nm using a Spectramax iD3 reader (CA, United States).
The radical scavenging capacity was calculated using the formula:
(Where A1 and A0 are the absorbances of the control and test samples respectively).
Nitric oxide radical scavenging assay
The assay was performed as described by Das et al., 2020 using a 96-well microplate. To 1 ml of the extract and ascorbic acid (30–500 μg/ml) dissolved in methanol 0.5 ml of sodium nitroprusside in phosphate buffer saline (10 mM) was mixed and incubated for 180 min at 25°C. In the test and positive control wells 150 μL of the reaction mixture containing the extract or standard drug were transferred followed by the addition of 150 μL of freshly prepared Griess reagent (1% sulphanilamide in 2.5% phosphoric acid, and 0.1% naphthyl ethylene diamine dihydrochloride in 2.5% phosphoric acid in equal ratio) was transferred to 96-well microplate. Similarly, a control group was also prepared. The absorbance of the solutions was recorded at 546 nm using SpectraMax iD3 reader (CA, United States).
The % inhibitions were calculated using the following formula:
(Where A1 and A0 are the absorbances of the control and test samples respectively).
Determination of total phenolic and flavonoid contents
The total phenolic content (TPC) and total flavonoid content (TFC) present in the extract were determined spectrophotometrically as described by Pieczykolan et al., 2022. Stock solutions of the test extract and standards were prepared in methanol.
For TPC, 0.5 ml of the test extract or standard stock solutions were taken and to this 2.5 ml of 10%, Folin-Ciocalteu’s reagent and 2.5 ml of 7.5% NaHCO3 were added. In the same way, a blank was also prepared. Gallic acid (10–40 μg/ml) was used as the standard to prepare the calibration curve. The reaction mixtures were incubated for 45 min at 45°C and the absorbance was recorded at λmax 765 nm using SpectraMax iD3 reader (CA, United States). The experiment was performed in triplicate. The content of total phenolics present in the extract was expressed as gallic acid equivalent (mg of GAE/g of extract).
For TFC, 1 ml of the test extract or standard stock solutions and 1 ml of 2% AlCl3 solution in methanol were mixed. Similarly, a control was also prepared. Rutin (20–80 μg/ml) was used to prepare the calibration curve For the construction of the calibration curve. After 1 h of incubation at 28°C, absorbance was measured at λmax 415 nm. All the samples were analyzed in triplicate. The content of total flavonoids in the extract was expressed as rutin equivalent (mg of RUE/g of extract).
Evaluation of anti-Alzheimer’s potential by an in-vitro method
Cholinesterase enzyme inhibition study potential
The method for cholinesterase enzyme inhibitory activity of the extract was performed following the method described by Das et al., 2020. The wells are grouped as background, test, and positive control. Each well of the plate contained 125 μL of 3 mM DTNB and 25 μL of 15 mM ATCI or BTCI. The background and test wells contained 25 μL of the extract (50–300 μg/ml) dissolved in tris buffer (PH 8.0). To the positive control well, 25 μL of galanthamine (10–50 μg/ml) dissolved in tris buffer (PH 8.0) was transferred. After 10 min of pre-incubation at 37°C, 25 μL of 2 U/ml AChE or 1 U/mL BChE were added in each well except background wells, and the absorbance was recorded at 405 nm for every 13 s up to 78 s. The linear regression parameters were determined for each curve. The %inhibition of the enzymatic activity was calculated and the IC50 values were extrapolated. The assay was performed in triplicate (n = 3).
β-secretase enzyme inhibitory potential
The in-vitro β-secretase enzyme inhibitory activity of the plant extract was performed following the method described by Mooko et al., 2021. The wells are grouped as blank, test, positive control, and negative control. The control as well test wells contained 50 µL of the extract (7.8125–500 μg/ml) dissolved in extraction buffer. The positive control well contained 50 µL of the extraction buffer and 2 µL of active β-secretase enzyme. To the negative control well 50 µL of the extraction buffer, 2 µL of the active β-secretase enzyme, and 2 µL of β-secretase inhibitor were added. Then 50 µL of the reaction buffer was added to each well. After 10 min of incubation at 37°C, 2 µL of β-secretase substrate was added to each well except the control wells. The plate was gently shaken and incubated again at 37°C for 60 min in dark conditions. The fluorescence activity was measured using a fluorescence microplate reader (PHERAstar, BMG Labtech, United States) using an excitation wavelength of 335 nm and an emission wavelength of 460 nm. The assay was performed in triplicate and IC50 values were calculated accordingly.
In vitro cytotoxicity assessment
The toxic effect of the extracts in HepG2 cells was performed via MTT cytotoxicity assay as described by Das et al., 2022. HepG2 cells were cultured and maintained in DMEM supplemented with 10% (v/v) FBS, l-glutamine (4 mM), penicillin (100 U/mL) and streptomycin (100 μg/ml). In each well of the 96-well microtiter plate, 100 µL of the media containing cell suspension (1×104 cells/mL) was seeded and incubated at 37°C with 5% CO2 for 24 h to allow the cells to attach to the bottom of the wells. Subsequently, cells were exposed to 100 μL each of the extract solution (100–1,000 μg/ml), controls which included vehicle-treated cells exposed to 0.5% DMSO and exposed to positive inhibitor doxorubicin (0.78125–100 μg/ml). After 24 h the cells were exposed to the МТТ reagent (0.5 mg/ml) and the absorbance was measured at 570 nm using a plate reader (Multiskan Go, Thermo Fischer Scientific). Color control blanks were utilized to normalize the results and the vehicle control treated cells were considered as 100% cell viability. The results are representative of the average % inhibition of all the experiments three times repeated and given as CC50.
CytochromeP450 enzymes inhibition assay
A fluorogenic assay was performed in black 96-well microplates following the method described by Das et al., 2022. The test extract, 10 μM ketoconazole (CYP3A4), and 10 μM fluoxetine (CYP2D6) were dissolved in 100 mM Vivid CYP450 reaction buffer and diluted in 2-fold serial dilution. To each well 40 μL of the test extract or standard inhibitors and 50 μL of prepared master pre-mix (containing reaction buffer, P450 Baculosomes Plus Reagent, and Regeneration System) were added and pre-incubated for 20 min at 37°C. Inhibition potential was tested against CYP3A4 (5 nM), and CYP2D6 (10 nM) isozymes. The reaction was started by the addition of 10 μL of NADP+ and the appropriate substrate (10 μM concentrations of fluorogenic substrates namely, 7-benzyloxymethyloxy-3-cyanocoumarin and 7-ethoxymethoxy-3-cyanocoumarin were used for CYP3A4 and CYP2D6 respectively) mixture in reaction buffer and again incubated for 10 min at 37°C. The fluorescence activity was measured using a fluorescence microplate reader (PHERAstar, BMG Labtech, United States) with an excitation wavelength of 415 nm and an emission wavelength of 460 nm. Accordingly, percentage inhibition and IC50 value were determined.
Estimation of heavy metals content
Heavy metals content estimation was performed using atomic absorption spectroscopy (Thermo Fisher AA 303; Thermo Fisher, Nasik, Maharashtra, India). Accurately weighed 1.0 g of the dried extract was treated with 3 ml of conc. HNO3 for 4–5 h. To this 3 ml of the acid mixture containing HNO3:HClO4 (2:1) was added and heated for 5–6 h at 120°C–130°C until the fumes stop and the solution became clear. At that point, 10 ml of milli-Q water was added and again boiled for 10–15 min till the volume was reduced to half. The resulting solution was cooled to room temperature and filtered using Whatman filter paper. The filtrate volume was adjusted to 50 ml with Milli-Q water. Similarly, a blank was also prepared (Nema et al., 2014). The experiment was repeated three times. For the analysis of the heavy metals, the instrumental condition is maintained as mentioned in Supplementary Table S1.
Chemical profiling by UPLC-QTOF-MS
Tentative identifications of the compounds were performed using Waters UPLC hyphenated with a Waters Synapt G2 QTOF instrument. Analysis was performed using an Acquity UPLC BEH C18 1.7 daysµm (2.1 × 100 mm column) column operating at a 0.300 ml/min flow rate. For analysis accurately weighted 1 mg of the extract was dissolved in 50% methanol (LC grade) and filtered through a 0.22 µm syringe filter. The mobile phase used was: A, 0.1% HCO2H in LC grade water and B, MeOH + 0.1% HCO2H. MS source ESI operated in both positive and negative ionization modes, capillary voltage and endplate voltage were set at 2600V and 2000 V respectively. Nitrogen was used as nebulizing gas at 10 L/h and m/z range was set from 50 to 1,200 amu. Gradient elution started with 3% B which remain linear until 14 min. From 14 to 16 min elution was kept constant with 100% B. A linear gradient of 3% B was then used to reach completion until 20 min. The mass data were processed by integrating with MassLynx v 4.1 (Waters Corporation, Milford, MA, United States), TagetLynx (Waters Corporation, Milford, MA, United States) and Openchrom (Eclipse Foundation Inc. Ottawa, Canada) software, which provided a list of possible elemental formulae.
Molecular modeling studies
Protein preparation
The Protein Data Bank (https://www.rcsb.org) was used to obtain the 3D crystal structures of AChE (PDB ID: 4M0E), BChE (PDB ID: 5K5E), and BACE 1 (PDB ID: 6OD6) with resolutions of 2.0, 2.8, and 2.0 Å respectively. Discovery Studio Visualizer v21.1.020298 was used to eliminate the complexes, inhibitors, non-essential water molecules, and all heteroatoms. On AutoDockTools 1.5.6, the macromolecule preparation procedure for docking studies was followed. Gasteiger charges were added after non-polar hydrogens were merged. The eliminated complex structures were then applied to undergo docking assessments (Zilbeyaz et al., 2021).
Ligand preparation
PubChem (https://pubchem.ncbi.nlm.nih.gov) was used to retrieve the three-dimensional structure of six small compounds discovered using LC-MS data in. sdf format. The mmff94 technique was used to achieve energy minimization for those molecules. This force field technique optimized the ligand conformer, resulting in a low-energy conformer. The ligand molecules were also added with hydrogen atoms, and their bond orders were fixed. PyRx was used to carry out a molecular docking study based on the conformation of the ligands with the lowest energy (Latha and Saddala, 2017; Lakshmanan et al., 2019).
Molecular docking
The interactions of the ligands with the target proteins were studied using molecular docking simulations. The docking was done with the PyRx 0.8 docking tool, utilizing a grid-based approach (−17.1948771822; −42.3786708589; 25.5801503824 for AChE; −3.3503745278; 9.52083690694; 14.4095583015 for BChE and −36.9335726532; −45.3980852787; 19.5144221109 for BACE-1) and the rigid dock strategy with the AutoDock Vina inbuilt algorithm were used to calculate the binding energy (Kcal/mol) of all protein-ligand complexes. Discovery Studio Visualizer was used to investigate the characteristics of the protein-ligand complex and their interactions. The hydrogen bond interactions with amino acids, π-Alkyl, π-π, π-σ interactions, and distance were estimated after the binding site was seen (Abdel-Hamid and McCluskey, 2014; Dallakyan and Olson, 2015).
Statistical analysis
The experimental data were expressed as mean ± SEM of triplicate. The significance of the difference between the groups was assessed by an unpaired t-test. The statistical significance was calculated using GraphPad Prism 5.0, a p < 0.05 was considered to be statistically significant.
Results
Evaluation of free radical scavenging potential
The antioxidant potential of M. oppositifolia was performed spectrophotometrically. The respective IC50 value was determined from the calibration curve after plotting varying concentrations against %inhibition. The IC50 values were found to be 93.21 ± 2.16 and 138.24 ± 1.41 μg/ml for ascorbic acid (AA) and M. oppositifolia extract, respectively by the DPPH method. The IC50 values for hydroxyl radical scavenging activity were 98.73 ± 1.96 and 189.43 ± 1.69 μg/ml for ascorbic acid (AA) and M. oppositifolia extract. For the nitric oxide radical scavenging assay, the IC50 values were 78.9 ± 0.63 and 264.32 ± 1.02 μg/ml for ascorbic acid (AA) and M. oppositifolia extract, respectively.
Determination of TPC and TFC
The TPC and TFC present in M. oppositifolia extract were expressed in terms of gallic acid equivalent (y = 0.0084x + 0.0055; r2 = 0.92) and rutin equivalent (y = 0.0029x–0.0051; r2 = 0.98). The concentration of total phenols and flavonoids were found to be 11.23 ± 0.56 mg of GAE/g of extract and 21.51 ± 0.64 mg of RUE/g of extract respectively.
Assessment of anti-Alzheimer’s potential by in-vitro methods
The cholinesterase enzyme inhibitors are a significant therapeutic target and are considered first-line pharmacotherapeutics for AD. The in-vitro cholinesterase enzyme inhibition activity of the test material and the standard were expressed as IC50 values. The IC50 values for the AChE inhibitory activity of M. oppositifolia extract and galanthamine (Standard inhibitor) were found to be 322.87 ± 2.05 and 32.71 ± 0.57 μg/ml. The IC50 values for BChE inhibition were 278.23 ± 1.89 and 20.15 ± 0.16 μg/ml respectively (Figure 1).
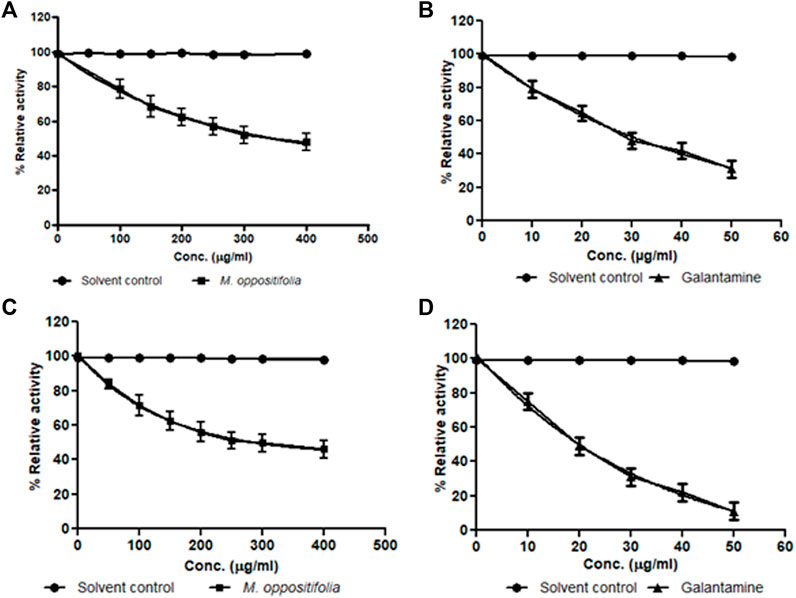
FIGURE 1. Dose-response curve for Solvent control (•); M. oppositifolia (■) and galanthamine (▲) for (A,B) acetylcholinesterase and (C,D) butyrylcholinesterase enzyme inhibition.
The current treatment in AD is aimed at lowering β-amyloid plaque accumulation in the cerebellum. The effect of the extracts on the β-secretase enzyme was determined through a β-secretase assay kit using the fluorescence method. The hydro-alcoholic extract of M. oppositifolia inhibited the β-secretase enzyme with an IC50 value of 173.93 μg/ml and the standard inhibitor showed a value of 41.09 μg/ml (Figure 2). The results suggested the possibility of M. oppositifolia extract in reducing or preventing the formation of the β-amyloid peptides.
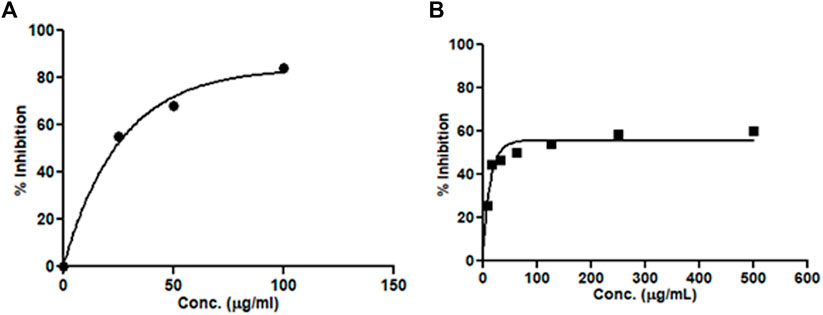
FIGURE 2. Concentration-dependent β-secretase enzyme inhibitory activity by (A) standard inhibitor and (B) M. oppositifolia extract.
Safety assessment
Cytotoxicity assessment in HepG2 cell line
The results from the in-vitro cytotoxicity assay showed that M. oppositifolia extract has a CC50 value of 965.45 ± 3.07 μg/ml whereas the standard cytotoxic agent doxorubicin showed much more toxic against HepG2 cells with CC50 value of 5.25 ± 0.24 μg/ml at 24 h. The results indicate that the test material is the least toxic in the HepG2 cell line.
CYP450 isoenzymes inhibition potential
To understand the interaction potential of the extract with drug metabolizing enzymes M. oppositifolia extract was tested with different cytochromeP450 isozymes. Respective positive controls (ketoconazole for CYP3A4 & fluoxetine for CYP2D6) for each isozyme were used to confirm the assay precision.
The IC50 values of the M. oppositifolia extract and standards against CYP3A4 and CYP2D6 isozymes are represented in Figure 3. Against CYP3A4 and CYP2D6 the IC50 values were found to be 477.03 ± 2.01 and 249.65 ± 2.46 μg/ml of the extract and for the standard IC50 values were 6.66 ± 1.87 (ketoconazole) and 7.93 ± 1.56 μg/ml (fluoxetine) respectively.
Quantitative estimation of heavy metals content by atomic absorption spectroscopy
In the present study, the concentration of heavy metals was determined using the standard calibration curve of the standard heavy metals solution having optimal detectable concentration ranges. The toxic heavy metals concentration was documented in terms of parts per million (ppm) as shown in Table 1.
Chemical profiling by UPLC-QTOF-MS
Tentative identification of compounds present in the hydro-alcohol extracts of M. oppositifolia was carried out using Waters Synapt G2 QTOF in both negative and positive ionization modes generating the accurate mass or quasimolecular ion and formula. The total ion chromatograms and full MS reports of the extracts are provided in Figure 4; Table 2, respectively.
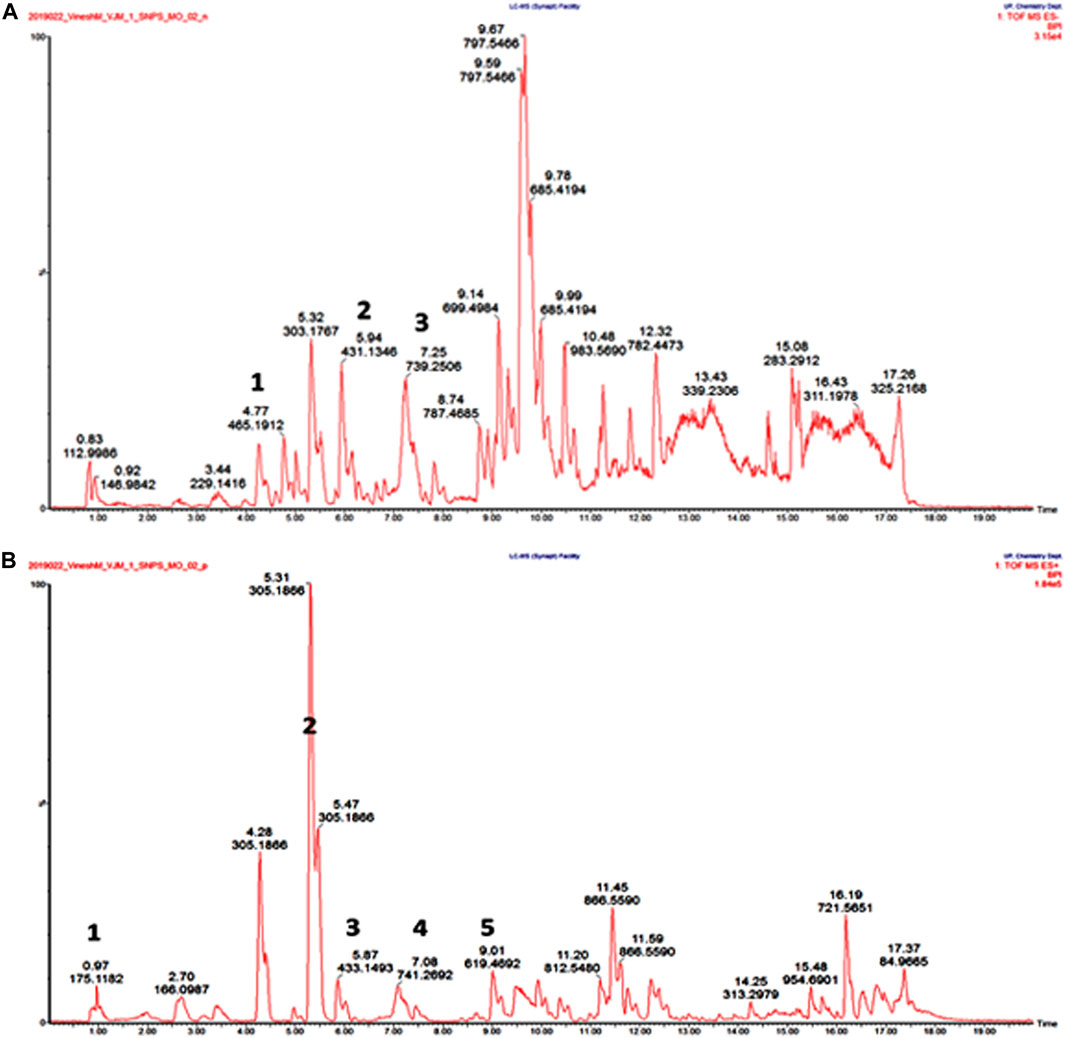
FIGURE 4. UPLC-QTOF-MS/MS total ion chromatogram for M. oppositifolia extract (A) and (B) represent positive and negative ionization modes, respectively.
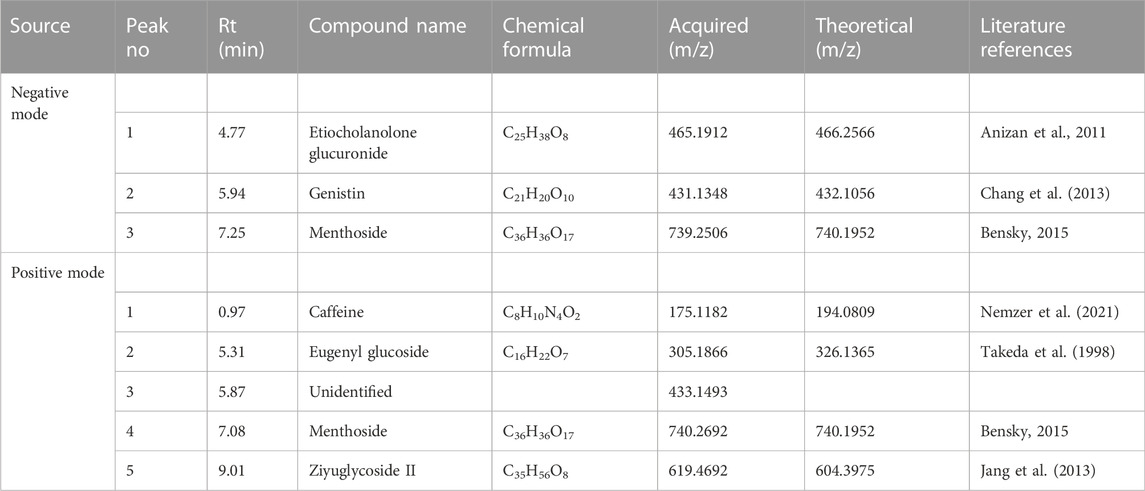
TABLE 2. UPLC-QTOF-MS profiles of M. oppositifolia hydro-alcohol extract (analysis in both negative and positive mode).
A total of 07 compounds from 07 different chemical classes like steroids, isoflavones, flavonoids, methylxanthines, triterpenoid saponin, anthraquinone, and phenolic glycosides were characterized (Figure 4). A steroid glucosiduronic acid namely etiocholanolone glucuronide was detected at Rt 4.6 min showing molecular ion [M-H]- at m/z values at 465.1912 (Anizan et al., 2001). Genstin, a flavonoid derivative at 5.94 min produces molecular ion [M-H]- at m/z 431.1348 (Chang et al., 2013). Another flavonoid class of compound at Rt 7.25and 7.08 showed molecular ion [M-H]- and [M + H]+ at m/z 739.2506 and 740.2692 identified to be Menthoside (Bensky et al., 2015). Caffeine, a methylxanthine class of compound was identified at Rt. 0.97 showing [M + H]+ at m/z 175.1182 (Nemzer et al., 2021). A phenolic glycoside, Eugenyl glucoside was detected at Rt. 5.31 showing [M + H]+ at m/z 305.1866 (Takeda et al., 1998). A triterpenoid saponin namely Ziguglycoside II was detected at Rt. 9.01 showing [M + H]+ at m/z 619.4692 (Jang et al., 2013).
Molecular docking study against AChE, BChE, and BACE-1 enzymes
The ligand–enzyme complexes with the compounds were placed in the same pocket of the AChE, BChE, and BACE-1 enzymes by PyRx 0.8.
The docking study of the compounds against AChE (PDB ID: 4M0E) possessed good docking scores ranging from −6.5 to −11.1 (Supplementary Figure S1; Supplementary Table S1). The most stable was menthoside with a docking score of -11.1 followed by genistin, while the least stable among the six compounds was caffeine. The reference standard compound galanthamine showed a docking score of −7.6. Other than Caffeine, all the compounds were found to interact via multiple hydrogen bonds with different amino acids in the active site of the macromolecule. Furthermore, other than conventional hydrogen bonds, the ligands also possessed different π interactions, including π-π stacked, π-σ, and π-alkyl interactions. The crucial amino acids within the active site that are mostly found to interact with the ligand molecules are TRP286, TYR124, and TYR341 residues (Supplementary Figure S2). The compound menthoside possessed conventional hydrogen bonds with VAL340, TRP286, SER293, and π -interactions with TYR72, HIS287, and TRP286 residues in the active site. The standard reference compound galanthamine was found to interact with SER293 via conventional hydrogen bonds by accepting the oxygen atoms, and also showed π -interactions with PHE297, PHE338, TRP286, TYR341 residues in the active site.
The docking study of the compounds against BChE (PDB ID: 5K5E) showed good docking scores ranging from -6.6 to -11.3 (Supplementary Figure S3; Supplementary Table S3). The most stable was menthoside with a docking score of −11.3 followed by ziyuglycoside II, while the least stable among the six compounds was caffeine. The reference standard compound galanthamine showed a docking score of −9.4. Other than Caffeine, all the compounds were found to interact via multiple hydrogen bonds with different amino acids in the active site of the macromolecule. Furthermore, other than conventional hydrogen bonds, the ligands also possessed different π interactions, including π-π stacked, π-σ, π-cation, and π-alkyl interactions. The crucial amino acids within the active site that are mostly found to interact with the ligand molecules are HIS438, GLY116, and TRP82 residues (Supplementary Figure S4). On the other hand, Menthoside possessed conventional hydrogen bonds with GLY115, GLY283, TYR282, ASN289, and π-interactions with HIS438, TRP82, and TYR332 residues. The compound galanthamine shared conventional hydrogen bonds with SER198, GLY115, GLY116, GLY117, and π -interactions with TRP82 residues in the active site.
The docking study of the compounds against BACE-1 (PDB ID: 6OD6) also possessed good docking scores ranging from −5.1 to −9.8 (Supplementary Figure S5; Supplementary Table S4). The most stable was menthoside showed a docking score of -9.8 followed by ziyuglycoside II, while the least stable among the six compounds was caffeine. All the compounds were found to interact via multiple hydrogen bonds with different amino acids in the active site of the macromolecule. Furthermore, other than conventional hydrogen bonds, the ligands also possessed different π interactions, including π-π stacked, π-σ, amide-π stacked, and π-alkyl interactions. The crucial amino acids within the active site that are mostly found to interact with the ligand molecules are PHE108, GLN73, ILE118, GLY34, TYR71, and TYR198 residues (Supplementary Figure S6). On the other hand, Menthoside possessed conventional hydrogen bonds with ARG128, SER36, PHE108, ASP228, and π-interactions with VAL69, ARG128, and TYR71 residues.
Discussion
The brain is a much metabolically active body part and the polyunsaturated fatty acids contents are highly susceptible to oxidative damage. The detrimental role of ROS in AD initiation and progression results from higher Aβ production and accumulation of plaque (Cobley et al., 2018; Singh et al., 2019; Sharma and Kim, 2021). Thus antioxidants may be more effective to prevent and reverse AD progression (Gezici et al., 2020; Veurink et al., 2020). Phenols and flavonoids are considered potential natural antioxidants having the ability to scavenge free radicals and reactive oxygen species because of their conjugated ring structures and OH group’s substitution (Lopa et al., 2021). A study by Wang et al., 2014 suggested that treatment of the polyphenols combination resulted in improved protection against cognitive impairments in mice. Similarly, the presence of a substantial amount of phenolics and flavonoids in the hydro-alcoholic extract of M. oppositifolia suggests that it could considerably improve the care of patients with AD.
Currently, the US-FDA and Health Canada-approved acetylcholinesterase and NMDA antagonists alleviate cognitive functions in AD. Drugs like donepezil, rivastigmine, and galanthamine were the available drug candidate as cholinesterase inhibitors which might help to slow down the pathophysiological alterations of clinical symptoms, but not be able to reverse the disease progression (Grossberg et al., 2019).
The current treatment in AD is also aimed at lowering β-amyloid plaque accumulation in the cerebellum. A study by Murata et al., 2015 suggested that herbs like Curcuma longa and Piper nigrum as promising candidates for inhibiting the β-secretase enzyme. Besides, some phytomolecules like neferine (Nelumbo nucifera), ginsenoside Rg1 (Panax notoginseng), hispidin (Phellinus linteus), 2,2′,4′-trihydroxychalcone (Glycyrrhiza glabra), etc. showed promising outcomes as β-secretase inhibitors (Zhang and Tanzi, 2012). Because of its multifactorial nature, AD can adequately be managed through the “One-compound multiple-targets paradigm” treatment strategy. To overcome this limitation, herbal medicine could be a better approach, since they constitute the different classes of secondary metabolites targeting multiple signaling pathways or proteins, and show synergistic effects. The cholinesterase and β-secretase enzyme inhibitory potential of M. oppositifolia extract may serve as a good lead for the development of AD drug candidates.
Polypharmacy increases the risk of drug-related interactions leading to significant clinical complications. A study by Sonali et al., 2013 suggested that the co-administration of CYP3A4 and CYP2D6 interacting drugs not significantly might partially be involved in the plasma concentration of anti-AD drugs like donepezil to a clinically significant extent (Perry and Howes, 2011; Auxtero et al., 2021). So, it is important to collect consistent information on the safety of herbal products for their safe and effective use. The present finding indicated that the extract showed the least interaction potential against the CYP-isozymes compared to standard inhibitors and implies their safety aspects. Additionally, the quantified toxic heavy metals concentration in the plant extract was found to be well within the prescribed limits (Cd < 1 ppm, Pb < 1 ppm, Hg < 5 ppm) (Mukhopadhyay, 2018).
The UPLC-QTOF-MS analysis tentatively identified a total of 07 compounds from 07 different chemical classes. Genistein is an isoflavone distributed widely in the leguminous and fabaceae family. Isoflavone is well documented for its antioxidant, anti-inflammatory, and anti-cancer activities (Sharifi-Rad et al., 2021). Mentha piperita was reported to contain menthoside as one of the flavonoids reported for its antioxidant, and anti-inflammatory properties (Biswas et al., 2014). Ziguglycoside II from the root part of Sanguisorba officinalis was reported to have antioxidant properties (Byun et al., 2021).
The in silico molecular docking analysis showed menthoside as the most stable compound with a good docking score toward AChE, BChE and BACE-1 enzymes among all the identified metabolites. From binding site prediction the amino acids SER293 and TRP286 for AChE, GLY115 and TRP82 for BChE ARG128, SER36, PHE108, ASP228, VAL69, ARG128, and TYR71 for BACE-1 were found to be essential for the establishment of strong interaction.
The combination therapy concept has been explored as a novel approach in preventing and treating neurodegenerative conditions. The present findings suggested that M. oppositifolia could be effective in the development of lead against cholinesterase and β-Secretase enzymes useful in AD.
Conclusion
Since global developments in the field of medication, no ultimate cure for Alzheimer’s disease has been recognized till date. Research from the past decades has come up with a few cholinergic drugs and NMDA receptor antagonists that do not affect the main pathological hallmarks of Alzheimer’s disease but rather somewhat delay the inevitable symptomatic disease progression. Being a multifactorial disease, there is a huge medical necessity for newer and more effective remedies that are free from deleterious side effects. It is noteworthy to study the ethnobotanical claim with high-throughput screening will cultivate novel therapeutic leads in drug development, thus contributing to a newer sight in the treatment of neurodegenerative disorders like Alzheimer’s disease. The findings concluded that M. oppositifolia could be a better optional pot in search of active moieties for multi-targeted drug approach due to its anti-cholinesterase and β-secretase enzyme inhibitory potential. The least cytotoxic effect on the HepG2 cell line and the cytochromeP450 isozyme inhibitory assay confirms its safety upon co-administration with CYP450 3A4 and 2D6 substrates drug candidates. The molecular docking analysis showed affinity and selectivity of the phytometabolites toward target enzymes. It can be said that herbs with rich polyphenols content might be preferable choices for the prevention of AD because of their multi-functions. Further detailed investigation is very much prerequisite to know the mechanistic approach toward the development of drugs against Alzheimer’s disease.
Data availability statement
The original contributions presented in the study are included in the article/Supplementary Material, further inquiries can be directed to the corresponding author.
Author contributions
BD carried out the experiment, methodology, data analysis, and drafted the manuscript. AS helped with docking and network analysis. PM conceptualized, supervised the study, data analysis, reviewed and edited the manuscript. PH assisted in data analysis, reviewed and edited the manuscript. NS reviewed and edited the manuscript. PB analyzed the data, reviewed and edited the manuscript.
Funding
The authors are thankful to the Department of Biotechnology (DBT), Government of India, for funding grant [BT/PR45281/NER/95/1934/2022].
Conflict of interest
The authors declare that the research was conducted in the absence of any commercial or financial relationships that could be construed as a potential conflict of interest.
Publisher’s note
All claims expressed in this article are solely those of the authors and do not necessarily represent those of their affiliated organizations, or those of the publisher, the editors and the reviewers. Any product that may be evaluated in this article, or claim that may be made by its manufacturer, is not guaranteed or endorsed by the publisher.
Supplementary material
The Supplementary Material for this article can be found online at: https://www.frontiersin.org/articles/10.3389/fphar.2022.990926/full#supplementary-material
References
Abdel-Hamid, M. K., and McCluskey, A. (2014). In silico docking, molecular dynamics and binding energy insights into the bolinaquinone-clathrin terminal domain binding site. Molecules 19 (5), 6609–6622. doi:10.3390/molecules19056609
Ahmed, R. R., Holler, C. J., Webb, R. L., Li, F., Beckett, T. L., and Murphy, M. P. (2010). BACE1 and BACE2 enzymatic activities in Alzheimer's disease. J. Neurochem 112 (4), 1045–1053. doi:10.1111/j.1471-4159.2009.06528.x
Anizan, S., Bichon, E., Di Nardo, D., Monteau, F., Cesbron, N., and Antignac, J. P., (2001). Screening of 4-androstenedione misuse in cattle by LC-MS/MS profiling of glucuronide and sulfate steroids in urine. Talanta 86, 186–194. doi:10.1016/j.talanta.2011.08.058
AsokKumar, K., UmaMaheswari, M., Sivashanmugam, A. T., SubhadraDevi, V., Subhashini, N., and Ravi, T. K. (2009). Free radical scavenging and antioxidant activities of Glinus oppositifolius (carpet weed) using different in vitro assay systems. Pharm. Biol 47, 474–482. doi:10.1080/13880200902817901
Auxtero, M. D., Chalante, S., Abade, M. R., Jorge, R., and Fernandes, A. I. (2021). Potential herb–drug interactions in the management of age-related cognitive dysfunction. Pharmaceutics 13 (1), 124. doi:10.3390/pharmaceutics13010124
Ayaz, M., Ullah, F., Sadiq, A., Kim, M. O., and Ali, T. (2019). Editorial: Natural products-based drugs: Potential therapeutics against alzheimer's disease and other neurological disorders. Front. Pharmacol 10, 1417. doi:10.3389/fphar.2019.01417
Bensky, D., Clavey, S., Stoger, E., and Gamble, A. (2015). Chinese herbal medicine: Materia medica. Portable 3rd ed. Washington: Eastland Press.
Berkov, S., Atanasova, M., Georgiev, B., Bastida, J., and Doytchinova, I. A. (2021). The amaryllidaceae alkaloids: An untapped source of acetylcholinesterase inhibitors. Phytochem. Rev 21, 1415–1443. doi:10.1007/s11101-021-09790-0
Bhadra, S., Pundir, C., Das, J., Kar, A., Chanda, J., and Das, B., (2022). “Evaluation of bioactive compounds as AChE inhibitors from medicinal plants,” in Evidence-based validation of herbal medicine. Editor P. K. Mukherjee (Netherlands, USA): Elsevier Science, 349–388. doi:10.1016/B978-0-323-85542-6.00002-0
Biswas, N. N., Saha, S., and Ali, M. K. (2014). Antioxidant, antimicrobial, cytotoxic and analgesic activities of ethanolic extract of Mentha arvensis L. Asian pac. J. Trop. Biomed 4 (10), 792–797. doi:10.12980/APJTB.4.2014C1298
Byun, N.-Y., Cho, J.-H., and Yim, S.-H. (2021). Correlation between antioxidant activity and anti-wrinkle effect of ethanol extracts of Sanguisorba Officinalis L. Food Sci. Technol 41, 791–798. doi:10.1590/fst.10921
Chang, L., Zhang, X. X., Ren, Y. P., Cao, L., Zhi, X. R., and Zhang, L. T. (2013). Simultaneous quantification of six major flavonoids from fructus sophorae by LC-ESI-MS/MS and statistical analysis. Indian J. Pharm. Sci 75 (3), 330–338. doi:10.4103/0250-474X.117437
Chen, X-Q., and Mobley, W. C. (2019). Exploring the pathogenesis of alzheimer disease in basal forebrain cholinergic neurons: Converging insights from alternative hypotheses. Front. Neurosci 13, 446. doi:10.3389/fnins.2019.00446
Chen, Z.-R., Huang, J.-B., Yang, S.-L., and Hong, F.-F. (2022). Role of cholinergic signaling in Alzheimer’s disease. Molecules 27, 1816. doi:10.3390/molecules27061816
Cobley, J. N., Fiorello, M. L., and Bailey, D. M. (2018). 13 reasons why the brain is susceptible to oxidative stress. Redox Biol 15, 490–503. doi:10.1016/j.redox.2018.01.008
Dallakyan, S., and Olson, A. J. (2015). Small-molecule library screening by docking with PyRx. Methods Mol. Biol 1263, 243–250. doi:10.1007/978-1-4939-2269-7_19
Das, B., Kar, A., Bhowmik, R., Karmakar, S., Tripathy, S., and Matsabisa, M. G., (2022). Quality related safety evaluation of a South African traditional formulation (PHELA®) as novel anti-biofilm candidate. Molecules 27 (4), 1219. doi:10.3390/molecules27041219
Das, B., Kar, A., Matsabisa, M., and Mukherjee, P. (2020). Anti-cholinesterase potential of standardized extract of PHELA a traditional South African medicine formulation. J. Herb. Med 22, 100348. doi:10.1016/j.hermed.2020.100348
Gezici, S., Koçum, D., Yayla, F., Sekeroglu, N., and Khan, A. A. (2020). Screening for in vitro antioxidant activities, polyphenolic contents and neuroprotective potentials of Clinopodium serpyllifolium subsp. serpyllifolium endemic to Turkey. Ann. Phytomed 9 (1), 181–186. doi:10.21276/ap.2020.9.1.23
Grossberg, G. T., Tong, G., Burke, A. D., and Tariot, P. N. (2019). Present algorithms and future treatments for alzheimer's disease. J. Alzheimers Dis 67 (4), 1157–1171. doi:10.3233/JAD-180903
Ha, D. T., Thu, N. T., Ha, T. T., Thuy, V. H., Van, N. H., and Luc, T. Q., (2021). Traphanoside GO1, a new triterpenoid saponin from the aerial parts of Glinus oppositifolius with the inhibitory effect on PGE2 production in LPS-induced HepG2 cells. Nat. Prod. Res 35 (23), 5125–5131. doi:10.1080/14786419.2020.1782405
Hampel, H., Hardy, J., Blennow, K., Chen, C., Perry, G., and Kim, S. H., (2021). The amyloid-β pathway in alzheimer's disease. Mol. Psychiatry 26 (10), 5481–5503. doi:10.1038/s41380-021-01249-0
Hoque, N., Habib, R., Imam, M. Z., Ahmed, J., and Rana, S. (2011a). Analgesic and antiinflammatory potential of methanol extract of Glinus oppositifolius L. Aust. J. Basic Appl. Sci 5, 729–733.
Hoque, N., Imam, M. Z., Akter, S., Ahmed, J., Mazumder, E. H., and Hasan, S. M., (2011b). Antioxidant and antihyperglycemic activities of methanolic extract of Glinus oppositifolius leaves. J. Appl. Pharm. Sci 1, 50–53.
Jang, Y. A., Kim, Y. H., Shin, J. C., Han, S. I., and Lee, J. T. (2013). The study on pharmacologic activation as cosmetic material of Sanguisorbae radix. Korea J. Herbology 28 (5), 79–85. doi:10.6116/kjh.2013.28.5.79
Khomdram, S. D., Fanai, L., and Yumkham, S. D. (2019). Local knowledge of edible flowers used in Mizoram. Indian J. Tradit. Knowl 18, 714–723.
Lakshmanan, D. K., Prathiviraj, R., Murugesan, S., Ravichandran, G., Abbirami, E., and Sivasudha, T. (2019). HRLC-ESI-MS based identification of active small molecules from Cissus quadrangularis and likelihood of their action towards the primary targets of osteoarthritis. J. Mol. Struct 1199, 127048. doi:10.1016/j.molstruc.2019.127048
Latha, M. S., and Saddala, M. S. (2017). Molecular docking based screening of a simulated HIF-1 protein model for potential inhibitors. Bioinformation 13 (11), 388–393. doi:10.6026/97320630013388
Lopa, S. S., Al-Amin, M. Y., Hasan, M. K., Ahammed, M. S., Islam, K. M., and Alam, A. H. M. K., (2021). Phytochemical analysis and cholinesterase inhibitory and antioxidant activities of enhydra fluctuans relevant in the management of alzheimer's disease. Int. J. Food Sci 2021, 8862025. doi:10.1155/2021/8862025
Martin-Puzon, J. J. R., Valle, D. L., and Rivera, W. L. (2015). TLC profiles and antibacterial activity of Glinus oppositifolius L. Aug. DC. (Molluginaceae) leaf and stem extracts against bacterial pathogens. Asian pac. J. Trop. Dis 5 (7), 569–574. doi:10.1016/s2222-1808(15)60838-6
Marucci, G., Buccioni, M., Ben, D. D., Lambertucci, C., Volpini, R., and Amenta, F. (2021). Efficacy of acetylcholinesterase inhibitors in Alzheimer's disease. Neuropharmacology 190, 108352. doi:10.1016/j.neuropharm.2020.108352
Modi, K., and Shah, M. (2015). Pharmacognostic specifications and quantification of oleanolic acid and lupeol in Mollugo oppositifolia Linn. Phcog. J 7 (2), 83–88. doi:10.5530/pj.2015.2.1
Mooko, T., Bala, A., Tripathy, S., Kumar, C. S., Mahadevappa, C. P., and Chaudhary, S. K., (2021). Cannabis sativa L. Flower and bud extracts inhibited in vitro cholinesterases and β-secretase enzymes activities: Possible mechanisms of cannabis use in alzheimer disease. Endocr. Metab. Immune Disord. Drug Targets 22, 297–309. doi:10.2174/1871530321666210222124349
Moreta, M. P.-G., Burgos-Alonso, N., Torrecilla, M., Marco-Contelles, J., and Bruzos-Cidón, C. (2021). Efficacy of acetylcholinesterase inhibitors on cognitive function in Alzheimer’s disease. Rev. Rev. Biomed 9, 1689. doi:10.3390/biomedicines9111689
Mukhopadhyay, G. (2018). Atomic spectroscopy analysis of heavy metals in plants. Int. J. Pharm. Biol. Sci. Arch 9 (3), 175–179.
Murata, K., Matsumura, S., Yoshioka, Y., Ueno, Y., and Matsuda, H. (2015). Screening of β-secretase and acetylcholinesterase inhibitors from plant resources. J. Nat. Med 69, 123–129. doi:10.1007/s11418-014-0859-3
Nagannawar, A. G., and Jayaraj, M. E. (2018). Pharmacognosy and preliminary phytochemical investigation on Mollugo oppositifolia L., A traditional medicinal herb. J. Pharmacogn. Phytochem 7, 509–512.
Nagannawar, A. G., and Jayaraj, M. (2020). GC-MS analysis of bioactive compounds from ethanolic extract of whole plant of mollugo oppositifolia l. and their pharmacological activities. Int. J. Pharm. Sci. Res 11 (5), 2504–2509.
Nema, N. K., Maity, N., Sarkar, B. K., and Mukherjee, P. K. (2014). Determination of trace and heavy metals in some commonly used medicinal herbs in Ayurveda. Toxicol. Ind. Health 30 (10), 964–968. doi:10.1177/0748233712468015
Nemzer, B., Abshiru, N., and Al-Taher, F. (2021). Identification of phytochemical compounds in coffea arabica whole coffee cherries and their extracts by LC-MS/MS. J. Agric. Food Chem 69 (11), 3430–3438. doi:10.1021/acs.jafc.0c05937
Perry, E., and Howes, M. J. R. (2011). Medicinal plants and dementia therapy: Herbal hopes for brain aging? CNS Neurosci. Ther 17, 683–698. doi:10.1111/j.1755-5949.2010.00202.x
Pieczykolan, A., Pietrzak, W., Dos Santos Szewczyk, K., Gawlik-Dziki, U., and Nowak, R. (2022). LC-ESI-MS/MS polyphenolic profile and in vitro study of cosmetic potential of aerva lanata (L.) juss. Herb extracts. Molecules 27, 1259. doi:10.3390/molecules27041259
Ragasa, C., Espineli, D., Mandia, E., Don, M., and Shen, C. (2012). A new triterpene from Glinus oppositifolius. Chin. J. Nat. Med 10 (4), 284–286. doi:10.1016/s1875-5364(12)60057-0
Sahu, S. K., Das, D., Tripathy, N. K., Dinda, S. C., and Sundeep Kumar, H. K. (2012). Evaluation of hypoglycemic activity of Mollugo pentaphylla and Glinus oppositifolius (L). Rasayan. J. Chem 5 (1), 57–62.
Sharifi-Rad, J., Quispe, C., Imran, M., Rauf, A., Nadeem, M., and Gondal, T. A., (2021). Genistein: An integrative overview of its mode of action, pharmacological properties, and Health benefits. Oxid. Med. Cell. Longev 19, 3268136. doi:10.1155/2021/3268136
Sharma, C., and Kim, S. R. (2021). Linking oxidative stress and proteinopathy in Alzheimer’s disease. Antioxidants (Basel) 10, 1231. doi:10.3390/antiox10081231
Sharma, S., Nehru, B., and Saini, A. (2017). Inhibition of Alzheimer's amyloid-beta aggregation in vitro by carbenoxolone: Insight into mechanism of action. Neurochem. Int 108, 481–493. doi:10.1016/j.neuint.2017.06.011
Singh, A., Kukreti, R., Saso, L., and Kukreti, S. (2019). Oxidative stress: A key modulator in neurodegenerative diseases. Molecules 24 (8), 1583. doi:10.3390/molecules24081583
Singh, S. K., Srivastav, S., Yadav, A. K., Srikrishna, S., and Perry, G. (2016). Overview of Alzheimer's disease and some therapeutic approaches targeting aβ by using several synthetic and herbal compounds. Oxid. Med. Cell. Longev 7361613. doi:10.1155/2016/7361613
Sonali, N., Tripathi, M., Sagar, R., Velpandian, T., and Subbiah, V. (2013). Clinical effectiveness of rivastigmine monotherapy and combination therapy in Alzheimer's patients. CNS Neurosci. Ther 19 (2), 91–97. doi:10.1111/cns.12036
Takeda, Y., Ooiso, Y., Masuda, T., Honda, G., Otsuka, H., and Sezik, E., (1998). Iridoid and eugenol glycosides from Nepeta cadmea. Phytochemistry 49 (3), 787–791. doi:10.1016/S0031-9422(98)00125-3
Tanquilut, N. C., Alonzo, N. Q., James, A. E., Rowlands, D. K., Sanchez, G. C., and Jose, R. D. S., (2019). Hypoglycemic activity of Antidesma bunius (L.) Spreng and Mollugo oppositifolia L. fresh and alcoholic extracts in the db/db diabetic mouse model. J. Med. Plants Res 13 (17), 396–400. doi:10.5897/JMPR2019.6812
Traore, F., Faure, R., Ollivier, E., Gasquet, M., Azas, N., and Debrauwer, L., (2000). Structure and antiprotozoal activity of triterpenoid saponins from Glinus oppositifolius. Planta Med 66 (4), 368–371. doi:10.1055/s-2000-8551
Veurink, G., Perry, G., and Singh, S. K. (2020). Role of antioxidants and a nutrient rich diet in Alzheimer's disease. Open Biol 10 (6), 200084. doi:10.1098/rsob.200084
Wang, J., Bi, W., Cheng, A., Freire, D., Vempati, P., and Zhao, W., (2014). Targeting multiple pathogenic mechanisms with polyphenols for the treatment of Alzheimer's disease-experimental approach and therapeutic implications. Front. Aging Neurosci 6, 42. doi:10.3389/fnagi.2014.00042
Zhang, C., and Tanzi, R. E. (2012). Natural modulators of amyloid-beta precursor protein processing. Curr. Alzheimer Res 1-15.
Zhang, L., Huang, Y., Wu, C., Du, Y., Li, P., and Wang, M., (2019). Network Pharmacology based research on the combination mechanism between escin and low Dose glucocorticoids in anti-rheumatoid arthritis. Front. Pharmacol 10, 280. doi:10.3389/fphar.2019.00280
Zhao, J., Liu, X., Xia, W., Zhang, Y., and Wang, C. (2020). Targeting amyloidogenic processing of APP in Alzheimer’s disease. Front. Mol. Neurosci 13, 137. doi:10.3389/fnmol.2020.00137
Keywords: molecular docking, Mollugo oppositifolia Linn., Alzheimer’s disease, herb-drug interaction, safety evaluation, metabolite profiling and identification
Citation: Das B, Bhardwaj PK, Sharma N, Sarkar A, Haldar PK and Mukherjee PK (2023) Evaluation of Mollugo oppositifolia Linn. as cholinesterase and β-secretase enzymes inhibitor. Front. Pharmacol. 13:990926. doi: 10.3389/fphar.2022.990926
Received: 11 July 2022; Accepted: 05 December 2022;
Published: 04 January 2023.
Edited by:
Sevgi Gezici, University of Gaziantep, TurkeyReviewed by:
Amany Abdel-Rahman Mohamed, Zagazig University, EgyptMuhammad Shahid, University of the Punjab, Pakistan
Copyright © 2023 Das, Bhardwaj, Sharma, Sarkar, Haldar and Mukherjee. This is an open-access article distributed under the terms of the Creative Commons Attribution License (CC BY). The use, distribution or reproduction in other forums is permitted, provided the original author(s) and the copyright owner(s) are credited and that the original publication in this journal is cited, in accordance with accepted academic practice. No use, distribution or reproduction is permitted which does not comply with these terms.
*Correspondence: Pardeep K. Bhardwaj, pardeep2128@gmail.com