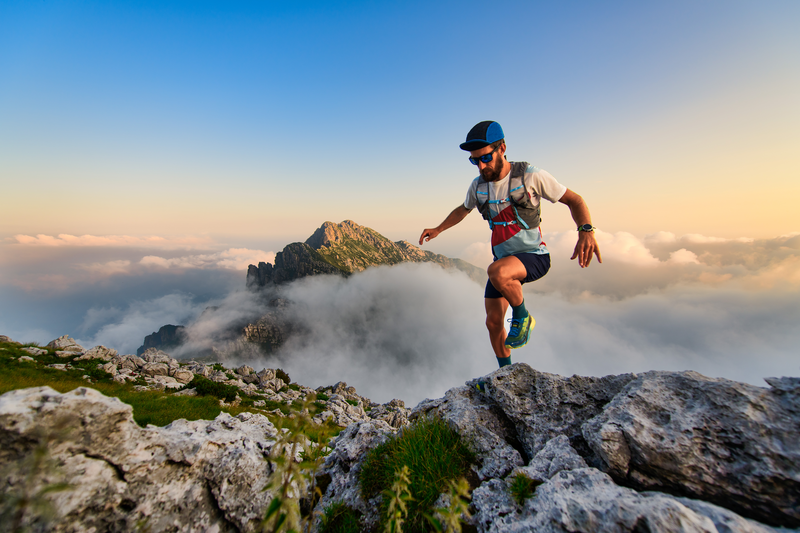
94% of researchers rate our articles as excellent or good
Learn more about the work of our research integrity team to safeguard the quality of each article we publish.
Find out more
REVIEW article
Front. Pharmacol. , 01 September 2022
Sec. Pharmacology of Anti-Cancer Drugs
Volume 13 - 2022 | https://doi.org/10.3389/fphar.2022.990445
This article is part of the Research Topic Immunity in the Development of Anti-cancer Drug Resistance View all 12 articles
Immune checkpoint inhibitors (ICIs) fight tumor progression by activating immune conditions. The inflammatory factors are playing a functional role in programmed death-1 (PD-1) or other immune checkpoints. They are involved in regulating the expression of programmed death ligand-1 (PD-L1), the only predictor recognized by the guidelines in response to ICIs. In addition, abundant components of the tumor microenvironment (TME) all interact with various immune factors contributing to the response to ICIs, including infiltration of various immune cells, extracellular matrix, and fibroblasts. Notably, the occurrence of immune-related adverse events (irAEs) in patients receiving ICIs is increasingly observed in sundry organs. IrAEs are often regarded as an inflammatory factor-mediated positive feedback loop associated with better response to ICIs. It deserves attention because inflammatory factors were observed to be different when targeting different immune checkpoints or in the presence of different irAEs. In the present review, we address the research progresses on regulating inflammatory factors for an intentional controlling anti-cancer response with immune checkpoint inhibitors.
Immunotherapy modulates the body’s immune system to fight immune evasion and immune silencing of tumors. Immune checkpoint inhibitors (ICIs) have the effect of stimulating activation and proliferation on T cells, so it is more beneficial to destroy tumor cells. Currently available immune checkpoint inhibitors are mainly programmed death-1 (PD-1) and its ligand (PD-L1), targeting cytotoxic T lymphocyte antigen-4 (CTLA-4). Further ICIs are being discovered and studied (Parry et al., 2005; Rosenberg et al., 2016), such as Lymphocyte Activation Gene-3 and T cell immunoglobulin mucin-3. Responses to ICIs have only been observed in certain solid tumors, such as gastrointestinal malignancies and unresectable or metastatic melanoma. And it is often accompanied by the possibility of resistance to ICIs (Haslam and Prasad, 2019; Puccini et al., 2020). The major reasons that account for diverse responses to ICIs are the contributions of multiple components of the tumor microenvironment (TME), including immune cells, fibroblasts, and the extracellular matrix (ECM). Thus, the interaction of inflammatory factors with components in the TME modulates responses to ICIs from different perspectives.
The number of patients being treated with ICIs is increasing, and thus cases of immune-related adverse events (irAEs) are also reported in a wide range of organs (June and Bluestone, 2017; Poto et al., 2022). Although the presence of irAEs is generally believed to be associated with better responses to ICIs, unpredictable and uncontrollable irAEs are a major obstacle preventing a complete course of ICIs therapy. The types of inflammatory factors involved in different irAEs are different, and the inflammatory factors associated with different therapeutic targets are not the same (Teulings et al., 2015; Berner et al., 2019). In addition, the discordance of inflammatory factors in TME and irAEs has also led to a new hypothesis that inflammatory factors mediate different signaling pathways involved in tumor response to ICIs and triggering of tissue-specific irAEs.
We aim to highlight the role of inflammatory factors in the TME on the response to ICIs, and also to address the involvement of inflammatory factors in the occurrence of irAEs.
Research on immune checkpoints has shown that most of them act as “brakes” in immune function, i.e., ICIs reactivate T cells to more effectively clear cancer cells (Ljunggren et al., 2018). At present, ICIs widely used in clinical applications mainly include anti-PD-1/PD-L1 and anti-CTLA-4. Additional immune checkpoint molecules are under study (Bagchi et al., 2021). The combination of PD-L1 and PD-1 will accelerate the apoptosis of immune cells, especially those with PD-1 positive. CTLA-4 also inhibits T cell activity and is up-regulated upon T cell activation (Valk et al., 2008). However, PD-L1 was a predictor for the response to ICIs rather than CTLA-4. One classification of TME is based on tumor-infiltrating lymphocytes (TILs) and PD-L1 expression, which are the main predictors of response to ICIs (Powles et al., 2014). Among them, one type with PD-L1 positive and abundant TIL infiltration had the best response to ICIs treatment (Teng et al., 2015). Furthermore, cancer ECM influences the response to immunotherapy through secreted regulators and suppressors (Umair et al., 2018; Bagaev et al., 2021). The ECM status provides important complementary information on the capture capacity of T cells (Mariathasan et al., 2018) and is considered a major common denominator of anti-ICIs (Jensen et al., 2021). Among them, cancer-associated fibroblasts (CAFs) are involved in constituting ECM fibrosis to hinder T cell infiltration and T cell activity, which produces a marked effect on immunotherapy resistance (Wang et al., 2018a; Mariathasan et al., 2018). The following summarizes the effects of inflammatory factors on the response of ICIs in TME from three aspects: PD-L1 abundance, TIL density, and ECM status (Figure 1).
FIGURE 1. Influence of Inflammatory Factors on Responses to ICIs in TME. In the TME, inflammatory factors affecting tumor response to ICIs were mainly associated with three factors, including PD-L1 abundance, TIL density, and ECM status. Many inflammatory factors affect PD-L1 expression, such as IFN-γ. Inflammatory factors and immune cells are both involved in influencing TIL infiltration with different roles. Among them, green factors or cells attract T cells to homing or promote TIL infiltration, while red ones reject T cell homing or inhibit TIL infiltration. Factors or cells that are yellow have been reported to have a dual effect. Factors affecting ECM status include biological and physical factors, and the components, immune cells and inflammatory factors, and signal paths involved are listed.
The abundance of PD-L1 in TME could predict therapeutic response to ICIs in multiple cancers, such as melanoma (Caroline et al., 2015) and cancer in lung (Borghaei et al., 2015) and bladder (Rosenberg et al., 2016). Inflammatory-related signaling was confirmed to regulate the expression of PD-L1 (Ji et al., 2015) but was detected not only on T cells (Fehrenbacher et al., 2016). PD-L1 is commonly detected by adaptive and innate immune cells, as well as epithelial cells, especially under inflammatory conditions (Sharpe et al., 2007). Many classical inflammatory factors have a hand in the regulation of PD-L1, which are discussed below respectively.
Interferons (IFNs) are secreted in large quantities by various activated immune cells, mainly by T cells and natural killer cells (NK cells). Importantly, PD-L1 of target cells can be triggered by IFN-γ (Garcia-Diaz et al., 2017). T cells secreted IFN-γ through the JAK1/JAK2-STAT1/STAT3 pathway (Karachaliou et al., 2018; Na et al., 2018; Thiem et al., 2019; Fujita, 2021). The JAK1/JAK2-STAT1 pathway has also been observed to stimulate IFN-γ secretion in NK cells (Bellucci et al., 2015). Further, IFN-γ receptor can also stimulate JAK-STAT, and STAT1 signaling is preferred (Platanias, 2005). In contrast, IFN-γ induces protein kinase D isoform 2 (PKD2), an important isoform that inhibits PD-L1 and promotes a strong antitumor immune response (Chen et al., 2012). Silencing of Male Germ Cell Associated Kinase (MAK), CRK Like Proto-Oncogene (CRKL) and PI3K signaling pathways also impair PD-L1 depending on IFN-γ (Garcia-Diaz et al., 2017). In melanoma, nuclear factor-κB(NF-κB) signal is required for IFN-γ to upregulate PD-L1 (Gowrishankar et al., 2015). Furthermore, IFN-γ receptor 1 inhibition can reduce PD-L1 expression in acute myeloid leukemia mice through the MEK-ERK and myeloid differentiation factor88 (MYD88)-tumor necrosis factor receptor-associated factor6 (TRAF6) pathways (Abiko et al., 2015). Since IFN-γ of TME is generally considered to be beneficial for immunotherapy, tumor cells may undergo survival-stressed proliferation when the IFN-γ signaling pathway is defective (Abril-Rodriguez and Ribas, 2017). This is one of the currently recognized causes of adaptive resistance during immunotherapy (Yi et al., 2018). Thus, an intact IFN-γ signaling pathway is a determinant for robust antitumor effects that persist throughout the course of ICIs treatment. It is worth noting that the close relationship between IFN-γ levels and PD-L1 was not necessarily observed in all cell types. For example, when sarcoma mice were treated with an IFN-γ blocking antibody, PD-L1 was largely abolished on tumor cells but not on tumor-associated macrophages (Noguchi et al., 2017). Therefore, more studies on the interaction of IFN-γ and PD-L1 need to be carried out.
IFN-α/β, also induces PD-L1 in cancer cells, endothelial cells and leukocytes (Schreiner et al., 2004; Eppihimer et al., 2015; Garcia-Diaz et al., 2017). IFN-α sensitizes the STAT1 signal, further increasing PD-L1 (Meng et al., 2020). However, there are limited reports on the correlation of IFN-α/β with response to ICIs, which may be due to the small effect of IFN-α/β on PD-L1 directly.
Toll-like receptors (TLRs) family, secreted by both myeloid cells and lymphocytes (Loke and Allison, 2003; Gang et al., 2013), connect innate immunity and adaptive immunity. On the one hand, PD-L1 expression is regulated by TLR4 through MyD88-dependent or MyD88-independent pathways (Lu et al., 2008). Besides, TLR4 activates the downstream NF-kB signaling pathway through transforming growth factor kinase 1 (TAK1) and activates the MAPK pathway through the transcription factor AP-1 (Qian et al., 2008). On the other hand, IFN-α production is activated by TLR4 ligands in plasmacytoid dendritic cells (PDCs) (Zhang et al., 2020). TLR3 can also cause increased PD-L1 expression in DCs (Pulko et al., 2009), endothelial cells (Cole et al., 2011) and neuroblastoma cells (Boes and Meyer-Wentrup, 2015) under conditions stimulated by polyinosinic:polycytidylic acid. However, the relevant mechanism is still unclear.
Interleukin (ILs) are a class of lymphokine that interacts between immune cells. On the one hand, a number of ILs upregulate PD-L1 on immune cells, including IL-10 (Cole et al., 2011) and IL-17 (Zhao et al., 2011) in monocytes, IL-4 (Ou et al., 2012) and IL-6 (Kim et al., 2008) in dendritic cells. IL-1β upregulates PD-L1 via the NF-κB (Kondo et al., 2010), and IL-27 via STAT1 and STAT3 signaling pathways (Karakhanova et al., 2011). In addition, IL-2, 7, 15 and 21 also increased PD-L1 in vitro (Kinter et al., 2008). On the other hand, IL in tumor cells can also cause high PD-L1, like IL-4, IL-17 in prostate and colon cancer cells (Wang et al., 2017), IL-27 in ovarian cancer cells (Carbotti et al., 2015), IL-10 in myelodysplastic syndrome blasts (Kondo et al., 2010), renal cell carcinoma (Quandt et al., 2014). IL-12-mediated regulation of PD-L1 may be more complex because IL-12 raises PD-L1 via NF-κB in macrophages while lowering PD-L1 when IFN-γ lacking (Xiong et al., 2014).
Transforming growth factor (TGF)-β production by CD8+ T cells was required for cells to consistently express PD-L1 (Baas et al., 2016). Meanwhile, TGF-β induced DCs to express PD-L1 in vitro in lung cancer (Ni et al., 2012) and hepatocellular carcinoma (Song et al., 2014), respectively. Tumor necrosis factor (TNF)-α in endothelial cells was observed to be relevant to high PD-L1 (Mazanet and Hughes, 2002). More reports are in autoimmune diseases such as Paget’s disease (Iga et al., 2019) and rheumatoid arthritis (Wasén et al., 2018). The regulation of PD-L1 expression by these promising inflammatory factors in the TME and further clarification of their roles in response to ICI therapy are warranted. In addition, CKLF (Chemokine-like factor)-like MARVEL transmembrane domain containing (CMTM)4/6 present on the cell surface binds to PD-L1 on protein level rather than transcription (Mezzadra et al., 2017).
Along with PD-L1 positivity, tumors respond well to ICIs when TILs are abundant and show drug resistance otherwise (Na et al., 2018). Thus, TIL density acts as a predictor of response to treatment with ICIs, too. It has been found that abundant TILs are associated with both adaptive upregulation of PD-L1 and the clinical benefit of immunotherapy (Xing et al., 2018). Pre-existing TILs are liberated by PD-L1/PD-L1 inhibitors and then promote tumor regression (Yagi et al., 2017; Tomioka et al., 2018). However, TILs on response to anti-CTLA-4 therapy is controversial. Baseline TIL status before treatment has been reported to predict a good therapeutic effect in melanoma (Chen et al., 2016), but no prognostic benefit has also been observed (Huang et al., 2011). Although anti-CTLA-4 is not prominent in immunotherapy as a monotherapy, it is being clinically tested in a variety of cancers as an effective means of enhancing anti-tumor response when applied with other therapies, such as chemotherapy and radiotherapy. This section focuses on those inflammatory factors and immune cells in the TME that attract or reject homing T cells and thus positively or negatively correlate with ICI responses.
TCRs (T cell receptors) are massively amplified in metastatic pancreatic cancer following anti-CTLA-4 (Hopkins et al., 2018). Within the TME, the enrichment of T cells with the same TCR is a hallmark of effective treatment with ICI therapy (Ro et al., 2017; Arakawa et al., 2019). CD8+ T cells are regarded as the key to “stem cell-like” antitumor immunity due to their unique expression of the transcription factor TCF1 (Siddiqui et al., 2019). After ipilimumab, those patients with CD45RA- had better responses, both for CD4+ T and CD8+ T cells (Subrahmanyam et al., 2018). In a study of nivolumab in non-small cell lung cancer, 18% of patients (23/126) exhibited complete or partial responses after treatment. Responders had more CD62LlowCD4+ T cells in the predose circulation than non-responders (Kagamu et al., 2020). Similar results in metastatic melanoma had shown that 9 of the 32 patients responded to ICIs. More memory CD4+ T cells were in the cancer tissues of the 9 responders, which were not only related to the expression of CD62L, but also to CCR7 and CD28 (Sade-Feldman et al., 2019). Therefore, the frequency of pre-treatment TIL populations and their dynamic changes may serve as important predictive biomarkers for distinguishing between ICI responders and non-ICI responders.
Chemotactic cytokines are a class of small cytokines that induce the orientation of responding cells into the site of infection during the immune response. Some chemokines are themselves pro-inflammatory factors, such as IL-8 (Maekawa et al., 2022), while others are regulated by inflammatory factors (Figure 2). Chemokine CC receptor (CCR) 7 binds to its selectin ligand, and chemotactic mature T cells migrate to specific targets. The phenomenon of immune desertification in the TME is a consequence of CCR7 deficiency (Berghuis et al., 2011). The expression of chemokine CC ligand (CCL) 5 is guided by IFN-α and gathers T cells in prostate cancer (Harlin et al., 2009). When triggering WNT/b-catenin, CCL4 downregulation results in failure of effector T cell recruitment and activation (Stefani et al., 2015). Both TLR4 and IL-6 levels were positively correlated with chemokine CXC receptor (CXCR) 3 expression, which may regulate the infiltration of CD+8 T cells (Muthuswamy et al., 2016). In tumor-infiltrating PDCs, CXCR4 is regulated to activate the TNF-α through NF-κB (Han et al., 2021). Inhibition of IFN-γ-induced SDF-1/MIP-1α pathway also reduces CXCR4 and CCR5 (Creery et al., 2004). The chemokine CXC ligand (CXCL) 9 and CXCL10 also contributed to the infiltration of TILs (Liu et al., 2015; Peng et al., 2015). STAT3 signaling can downregulate the CXCR3/CXCL10 axis in CD8+ T cells (Yue et al., 2015), thereby reducing their ability to penetrate the TME. CXCL9 and CXCL10 can be activated via IFN-α to promote TILs increasing in the cancer of the prostate gland (Harlin et al., 2009). CXCL11 was correlated with TLR4 levels positively (Muthuswamy et al., 2016). Regardless of tumor subtype, T cells from breast cancer patients treated with anti-PD-1 were clonally expanded, mediated CD8+ T cell homing via CXCL13, and attracted CD4+ T cells by CXCR5 (Bassez et al., 2021). In addition, CCR5, CCL2, CCL3, CCL11 and CX3CL1 were also positively associated with T cell infiltration (Peng et al., 2015; Manou et al., 2020; Bassez et al., 2021).
FIGURE 2. Chemokines regulated by inflammatory factors. In general, cancer cells in the TME, CAFs and ECM can all secrete chemokine ligands. Those T lymphocytes that express chemokine receptors on their cell surfaces are attracted to specific chemokine ligands and thus home to the TME as TILs. CXCR3-CXCL9/10/11 is a key chemokine reported to be associated with TILs infiltration, which is regulated by various signaling pathways as shown in the figure. In addition, CCR5-CCL4/5 has also been found to be associated with the infiltration of TILs through multiple pathways.
However, chemokines do not always work as expected (Bassez et al., 2021). A study of patients with metastatic urothelial carcinoma who received PD-L1 blocker found that TILs could not reach the tumor center and were trapped in the surrounding matrix (Mariathasan et al., 2018). Tumor-derived chemokines are one of the main reasons for misdirecting activated T cells to the stromal cells surrounding the tumor. For example, stromal cells surrounding pancreatic tumors produce CXCL12, which attracts effector T cells and prevents them from entering the tumor core (van der Woude et al., 2017). The CXCL9/CXCR3 axis may also have a dual role in promoting T cell migration and tumor cell metastasis (Billottet et al., 2013). High expression of CCL2, CCL22, and CXCL12 normally attracts immunosuppressive cells (Harlin et al., 2009). For non-small cell lung cancer, CCL2 blockade reduces immunosuppression and enhances cancer immunotherapy (Fridlender et al., 2010).
The TGF-β has been concerned due to its negative impact on T cells. Blocking the TGF-β signaling pathway contributes to the transition of the TME to an immune-inflammation-rich state, which has significant advantages in tumor control when combined with ICI (Mariathasan et al., 2018; Ravi et al., 2018). Moreover, stimulation of PD-1/PD-L1 by TGF-β receptor (TGF-βRII) results in a reduction in the host’s rejection of the graft, suggesting that similar processing may also be involved in the TME (Baas et al., 2016).
Several regulatory immune cells influence TIL infiltration (Gabrilovich, 2017; Mantovani et al., 2017) (Table 1), mainly including regulatory T (Treg) cells, myeloid-derived suppressor cells (MDSCs) and monocytes.
Tregs can either restrict the amount of effector T cells by depleting IL-2 (Setoguchi et al., 2005) or inhibit the function of effector T cells by producing TGF-β and IL-10 (Takahashi et al., 1998; Camisaschi et al., 2010). In turn, TGF-β and IL-10 expand Treg cells (Chen et al., 1994). In addition, Treg cells produce perforin and granzymes to kill effector T cells (Mantovani et al., 2017). Activated Treg cells express LAG-3 (Huang et al., 2004) and CTLA-4, which promote indoleamine 2,3-dioxygenase (IDO) secretion (Mellor and Munn, 2004), thereby causing T cell dysfunction (Uyttenhove et al., 2003). Small numbers of Tregs are associated with increased ICIs response and patient survival (Read et al., 2006; Friedline et al., 2009). In bladder cancer patients, however, CTLA-4 blockade resulted in an increased proportion of Tregs (Liakou et al., 2008). This suggests that the baseline status of immune cells and ICIs-related changes are of different predictive significance and that effector T cells/Treg ratio may be a more effective method for predicting response to treatment with ICIs (Sharma et al., 2021). Significantly, the effect of anti-CTLA-4 depends on antibody-dependent cytotoxic activity (ADCC) to deplete CTLA-4-expressing Treg cells in the TME, and its deletion will abolish the anti-tumor effect of anti-CTLA-4 (Bulliard et al., 2013; Selby et al., 2013). PD-1 inhibitors on Treg cells remain unclear.
Correlations between MDSCs abundance and response to ICIs have been revealed in numerous clinical trials (Meyer et al., 2014; Weber et al., 2016). MDSCs suppress the immune system by expressing PD-L1 (Noman et al., 2014), secreting IL-10 and TGFβ (Hart et al., 2011; Trikha and Carson3rd, 2014), and recruiting Tregs with CD40 (Pan et al., 2010). MDSCs impede response to ICIs not only with ipilimumab (Liakou et al., 2008) but also with anti-PD-L1 therapy (McDermott et al., 2018). MDSCs can reduce T cell reactivity (Messmer et al., 2015). In renal cell cancer, the inhibition of STAT3 aggregates MDSC, further leading to T cell suppression (Ko et al., 2009). MDSCs abolish the directional migration of CD8+ T cells when upregulated by NADPH oxidase (Nagaraj et al., 2007; Lu et al., 2011). Decreased recruitment and differentiation of MDSCs through obstruction of COX-2/PGE2 resulted in improved CTL frequency and immune responses (Obermajer et al., 2011). In addition, the immunosuppressive function of MDSCs was suppressed by downregulating arginase and iNOS, resulting in a shift in tumor dynamics towards more responsive ICIs (Orillion et al., 2017; ChristmasEntinostat et al., 2018). Targeting CXCR2 reduces the number of MDSCs in pancreatic cancer (Steele et al., 2016). Treatment against the receptors of MDSCs or their ligands CSF-1R/CSF-1 combined with immunotherapy can improve antitumor T cell activity and tumor regression (Mok et al., 2014). The combined use of CSF-1R inhibition and CXCR2 antagonism can also reduce the number of MDSCs and improve anti-PD-1 efficacy (Kumar et al., 2017a). Targeted drugs that inhibit the recruitment of tumor-infiltrating CXCR2+ MDSCs can enhance the response of ICIs (Sun et al., 2019). In addition, inhibition of MDSCs by the DNA demethylating agent 5-aza-2′-deoxycytidine also promotes antitumor immune responses (Daurkin et al., 2010).
Increased frequency of circulating monocytes at baseline predicts better ICI response (Martens et al., 2016; Krieg et al., 2018). Macrophages, granulocytes and DCs are of importance. The phagocytic capacity of tumor-associated macrophages (TAMs) is regulated by the PD-1/PD-L1 (Gordon et al., 2017). Differentiation of PD-1+ TAMs impairs effector T cell function (Li et al., 2019). Type 1 polarization (TAM-1) of TAMs is prevented by blocking leukocyte immunoglobulin-like receptor B2 (LILRB2), which improves anti-PD-L1 responses (Chen et al., 2018). CD47 blockade increases the efficiency of anti-PD-L1 in melanoma to by modulating the activity of TAMs (Sockolosky et al., 2016). Tumor-associated neutrophils (TANs) can be divided into type 1 (TAN-1) and type 2 (TAN-2), the former stimulating infiltration of effector T cells (Eruslanov et al., 2014) and the latter an immunosuppressive phenotype (Singhal et al., 2016). Increased eosinophils contribute to the polarization of macrophages in favor of TAM-1, which is further mediated by CCL5, CXCL9 and CXCL10 (Carretero et al., 2015). Conversely, basophils attract CD8+ T cells in the TME by producing CCL3 and CCL4 (Sektioglu et al., 2016). Differentiation of peripheral DCs also modulates the response to anti-PD-1 (Bolton et al., 2015).
Cancer ECM is formed through secreted regulators and repressors (Ho et al., 2020). CAFs participate in the process of tumor fibrosis and contribute to the ECM (Jensen et al., 2021). Fibrosis of the ECM through TGF-β signal (Nissen et al., 2019), is considered to be one of the key factors affecting the response to immunotherapy (Bagaev et al., 2021). A combined anti-CTLA-4 and anti-PD1 approach was linked to ECM components to enhance retention within the ECM, suggesting that ECM modulation may be an effective approach to enhance the efficacy of ICIs therapy (Ishihara et al., 2017).
The formation of ECM determines the migration and localization of immune cells to a certain extent (Hynes, 2009; Hallmann et al., 2015). Based on TME extracellular components, tumors are classified as immune-inflammatory tumors, immune-rejective tumors, and immune-desert tumors (Hegde and Chen, 2020). Immunorejecting tumors respond worse to ICIs than immunoinflammatory tumors, as T cells are blocked away from the tumor (Tumeh et al., 2014; Chen and Mellman, 2017). First, the dense ECM acts as a physical barrier to hinder the infiltration of TILs. Abundant collagen blocks homing T cells despite being activated (Evanko et al., 2012). Elevated collagen level causes patients to resist anti-PD-1/PD-L1 (Peng et al., 2020). Hyaluronic acid (HA) also prevents the move of effector cells (Jacobetz et al., 2013). Chemokine-dependent T cells were observed to infiltrate restricted due to the dense HA surrounding lung cancer tissue (Salmon et al., 2012). Second, immune cells are regulated by the composition of the ECM (Cooper et al., 2016). Collagen inhibits T cell activity by signaling through SHP-1 (Peng et al., 2020). HA promotes inflammation in the TME and increases Treg activity through TLR signaling (Nikitovic et al., 2015). Matrix metalloproteinases (MMPs) promote tolerance polarization in DCs by binding to TLR2 (Godefroy et al., 2014). Vascular endothelial growth factor (VEGF), which is abundantly present, also has inhibitory effects on DCs (Burbage and Amigorena, 2020). Chondroitin sulfate proteoglycan (VCAN) is directly associated with the reduction of TIL (Gorter et al., 2010), as it prevents T cell adhesion and migration (Peng et al., 2020). Meanwhile, it can also indirectly inhibit CTL infiltration by recruiting MDSCs (Wight et al., 2014) and regulating Batf3 DCs (Hope et al., 2017). Third, some ECM components influence inflammatory factor levels. For example, MMP-9 increases TNF-α, IL-1β, IL-6 levels (Liao et al., 2021). Low doses of collagen synergize with CXCL12 to induce the release of CD40L via p38-MAPK activation (Nakashima et al., 2020). Collagen peptides can significantly inhibit the secretion of IL-1β, TNF-α and PGE2 (Wang et al., 2018b). Thus, type III collagen propeptide is a predictor of metastatic melanoma response to ICIs (Hurkmans et al., 2020), and type IV collagen fragments can also identify melanoma patients who benefit from anti-CTLA-4 (Jensen et al., 2020).
Remodeling of the ECM is thought to be a key factor in immune cell trafficking and activation of the immune cycle (Huse, 2017). In bladder cancer patients, PD-L1 expression is discordant at metastatic sites and the primary tumor, suggesting the dynamic nature of the ECM (Mukherji et al., 2016). TGF-β has been reported to increase the synthesis and deposition of type I collagen and downregulate MMP-2 (Türlü et al., 2021). Activation of the RhoA/ROCK signaling pathway and transcription of SNAIL1 and TWIST1 genes are both triggered by TGF-β (Gaggioli et al., 2007; García-Palmero et al., 2016; Chung et al., 2021). At the same time, TGF-βR is also necessary for the deposition of collagen and fibronectin (Shuang and Chakrabarty, 2010; Robert et al., 2018). TNF-α and fibroblast growth factor (FGF)-2 are also important adjuvants in the process of promoting collagen synthesis (Katsumata et al., 2019; Muchová et al., 2021). In contrast, IL-1α/β promotes IL-6 and IL-8 and inhibits ECM, by regulating the expression of Versioncan (Chang et al., 2017; Osei et al., 2020). Interferon regulatory factor 9 regulates VCAN transcription independently of JAK-STAT signaling (Brunn et al., 2021). Immune cells are also involved in engineering ECM components (Bhattacharjee et al., 2019). For example, macrophages produce Hyaluronic Acid Synthase 1 via TLR2 and Syndecan-4 via TLR2, TLR3 and TLR4 (Chang et al., 2017). Also, the M1 macrophages to produce MMP-10 contribute to vascular remodeling through STAT1 signaling (Chi et al., 2022). TGF-β secreted by CD4+ T cells is also involved in vascular reorganization (Li et al., 2020).
Activated CAFs often build a matrix-dense barrier in the ECM to protect tumor cells and trap T cells, which affects the efficacy of ICIs (Godefroy et al., 2014). In metastatic urothelial carcinoma patients treated with PD-L1 monoclonal antibody, activated TGF-β signaling pathway in CAFs may hinder T cells from infiltrating tumors (Mariathasan et al., 2018). In co-culture with triple-negative breast cancer, TNF-α and IL-1β induced CXCL8/CCL5 secretion to suppress CD8+ T cells (Liubomirski et al., 2019). The resistance of breast cancer bone metastases to ICB is thought to be the release and resorption of TGF-β after osteoclast induction of osteogenesis, reducing the number of Th1 (Jiao et al., 2019). In addition, p-STAT-3, which is associated with metastatic brain tumors, reduces CD8+ T cell activity and increases the abundance of CD74+ macrophages, potentially playing a role in ICIs (Priego et al., 2018). CAFs have been reported to increase the expression of Fas and PD-1 to control the binding with PD-L2 for the former and Fas ligand for the latter (Lakins et al., 2018). Loss of Chitinase-3-like 1 in CAFs also increases the TILs (Cohen et al., 2017). Meanwhile, CAF inhibits NK protein (NKp) 44, 30, DNAX accessory molecule-1 (DNAM-1), and poliovirus receptors (Calon et al., 2014; TomokoInoueKatsuyukiTaguchi et al., 2016), which are the activating receptors of NK cells. High levels of IL-6 secreted by CAFs summon MDSCs and amplify PD-L1 in hepatocellular cancer (Liu et al., 2017). CSF-1 in the TME contributes to the reduction of chemotactic factors in CAFs. CSF-1 receptor and CXCR2 are key items for MDSCs gathering (Kumar et al., 2017b). CTLA-4 antibodies were more effective when combined with the GM-CSF vaccine (van Elsas et al., 1999). CAFs can also secrete other negative factors, such as CXCL12, CCL2, and VEGF (Grum-Schwensen et al., 2005; Chen et al., 2017). CXCL12/CXCL12R helps sensitize to anti-PD-L1 therapy, resulting in T cell aggregation and cancer regression (Feig et al., 2013). In gastric and colon cancers, fibroblast activation protein (FAP) in CAFs leads to more CCL2 and less IFN-γ, eventually damaging the outcome of ICIs therapy (Chen et al., 2017), while FAP Inhibition reverses this resistance (Wen et al., 2017). DNA vaccines against FAP induce activation of CD8+ and CD4+ T cells to enhance responses to ICIs (Xia et al., 2016). A pan-cancer analysis showed that TGF-β signaling in CAFs activated “ECM-up” signaling, independent of CAFs or TGF-β activation, a predictor associated with anti-PD-1 resistance, even better than T Cellular inflammatory signaling and mutational burden (Chakravarthy et al., 2018).
Given the complexity and dynamics of the tumor microenvironment, a single biomarker may not be sufficient, and a combination of multiple markers is supposed to be paneled (Binnewies et al., 2018; Kitano et al., 2018). Initial tumor biopsy assessment of PD-L1 abundance, TIL infiltration, and ECM status, combined with longitudinal assessment of changes in each factor during treatment, may have a unique potential to predict tumor phenotypes that respond to ICIs.
Treatment with ICIs induces systemic and local inflammatory responses (Tsukamoto et al., 2018), starting from the interaction between inflammatory factors and their recruitment to immune cells. Inflammatory factors mediate each other. For example, the combination of anti-PD-L1 and anti-IL-6 antibodies increases IFN-γ and Th1 in melanoma mice (Tsukamoto et al., 2018), turning off IL-6/STAT3 pathway, which further activates IFN-γ/STAT1 for causing local inflammation (Xiang et al., 2020). In addition, inflammatory factors contribute to the accumulation of immune cells. TNF-α contributes to Th17 differentiation in colon cancer treated with anti-CTLA-4 (Beck et al., 2006), possibly due to IL-23/Th17 axis activation (Liu et al., 2021). In addition, eosinophils in the skin (Voskens et al., 2013) and macrophages in acute diabetes (Hu et al., 2020) are also the result of the application of ICIs by IFN-α or γ.
Further, the expansion of the inflammatory factor cascade leads to immune damage to normal tissues, which is the main cause of side effects. IrAEs can occur in various organs (Figure 3) and are associated with good responses to ICIs (Saleh et al., 2019). However, an overresponse can hinder the course of ICIs treatment (Saleh et al., 2019), and the presence of severe irAEs leads to discontinuation of ICIs therapy temporarily or permanently according to guidelines. Induction of increased immune-promoting cells, such as a marked expansion of Th17 cells, is an independent risk factor for severe colitis in patients receiving ipilimumab (Tarhini et al., 2015). In contrast, immunosuppressive cells, such as Treg cells, tend to be depleted after treatment with ICIs (Simpson et al., 2013). The influx of myeloid cells also stimulates organ damage (Nam et al., 2019). In addition, different irAEs may have their specific cytokine profiles (Table 2). Circulating baseline IL-17 levels may help predict patients who are likely to develop severe diarrhea and colitis after treatment with ICIs (Young et al., 2018). However, IL-22 was not elevated (Luoma et al., 2020). The inflammatory response in rheumatoid arthritis is often accompanied by abundant TNF-α and IL-6 (Mcinnes et al., 2016). PD-1 blockade results in increases in IFN-γ, TNF-α, IL-2, 6, 17, and 23 (Dulos et al., 2012; Mcinnes et al., 2016). These changes may trigger psoriasis when treated with PD-1 inhibitors (Murata et al., 2017). However, IL-8 was not associated with dermatitis (Tanaka et al., 2017). In addition, upregulation of CXCL9, CXCL10, and CXCL11 has also been observed to correlate with PD-1 drug-related skin toxicity (Goldinger et al., 2016; Vivar et al., 2017). Prior to treatment, higher concentrations of IL-1β, 2, and GM-CSF were exposed in thyroid irAE (Kurimoto et al., 2020). Anti-TNF monoclonal antibodies prevent colitis (Perez-Ruiz et al., 2019), but at the same time may exacerbate cardiotoxicity associated with ICIs (Kwon et al., 2003) and may exacerbate interstitial lung disease (Akiyama et al., 2016). Pneumonitis, serum sickness, encephalitis, and systemic inflammatory response syndrome were all improved when anti-IL-6 antibodies were used to treat severe IRAE (Stroud et al., 2019). However, it increases the risk of inflammatory bowel disease (Korzenik et al., 2019). Arthritis induced by ICIs can also be ameliorated by inhibition of IL-6 and TNF-α (Sang et al., 2017; Cappelli et al., 2018). Other cytokines, such as IL-12p70, 1α, 1RA and 13, IFN-α2, CSF, CX3CL1, and FGF-2, were also differentially expressed in the plasma of severe irAEs patients before and during ICIs treatment (Tarhini et al., 2015), but they The association with specific classes of IRAEs and their associated mechanisms are unclear.
FIGURE 3. Organs susceptible to inflammatory toxicity induced by ICIs. Almost all organs may develop irAEs after receiving ICIs (Poto et al., 2022). Among them, the most common side effects occurred in skin, with an incidence rate of 40%. Gastrointestinal (GI) system with the occurrence of 30%. Followed by lungs and thyroid. The incidence of joints, blood components, and kidneys are all around 5%, while ocular, myocardial, and nerve involvement have been reported rare with a rate less than 1%.
Less than half of the cases respond to ICIs (Young et al., 2018), and there is a lack of effective predictors. A personalized panel composed of various inflammatory factors is a proposed predictive tool, the main factors including IFN, TLR, IL, TNF-α and TGF-β. They were selected for affecting PD-L1 expression and immune cell infiltration. They not only activate signaling pathways or regulate chemokines but also participate in ECM remodeling or balance pro- and anti-inflammatory effects.
IrAEs occurred more frequently when ICIs treatment was shifted from monotherapy to combination therapy (Johnson et al., 2020). Balancing anti-inflammatory and pro-inflammatory is expected to resolve the dilemma between anticancer and anti-irAEs of ICIs therapy, so some combination drugs have been tried other than glucocorticoids. The first is inflammatory pathway inhibitors. PI3K blockade fights cancer and reduces side effects by silencing inflammatory pathways (Eschweiler et al., 2022). The second is inflammatory factor inhibitors, which have a bidirectional effect. Anti- and pro-inflammatory are regulated by TNF through binding to different receptors (TNFR1 and TNFR2) (Chen et al., 2021), and by IL-6 through activation of different signals (JAK-STAT3 or PI3K-Akt) (Nguyen et al., 2014). The third is small molecule inhibitors of immune checkpoints and nanoparticles (Zhang et al., 2019). Small-molecule inhibitors can reduce affinity to reduce IRAEs, while nanoparticles facilitate targeting to improve anticancer efficacy.
The influence of inflammatory factors is not only applicable to ICIs treatment, but also to other immunotherapies. Immune-related side effects are triggered consistently across various immunotherapies, all resulting from cytokine cascades (Weber et al., 2015). For example, in adaptive immune cell therapy, IL-6, IL-1 are the main triggers of cytokine release syndrome. Likewise, up-regulated IFN and IL-2 caused gastrointestinal reactions and thyroid damage during cytokine therapy. Due to the universality of the effects and side effects of inflammatory factors in various immunotherapies, the understanding of inflammatory factors in ICIs may be extended to immunotherapy in pan-cancer and benefit more patients.
YW did the literature research and wrote the article. SY and HQ revised the manuscripts. All authors read and approved the final manuscript.
The authors declare that the research was conducted in the absence of any commercial or financial relationships that could be construed as a potential conflict of interest.
All claims expressed in this article are solely those of the authors and do not necessarily represent those of their affiliated organizations, or those of the publisher, the editors and the reviewers. Any product that may be evaluated in this article, or claim that may be made by its manufacturer, is not guaranteed or endorsed by the publisher.
Abiko, K., Matsumura, N., Hamanishi, J., HorikawaN. , , MuRakami, R., Yamaguchi, K., et al. (2015). IFN-γ from lymphocytes induces PD-L1 expression and promotes progression of ovarian cancer. Br. J. Cancer 112 (9), 1501–1509. doi:10.1038/bjc.2015.101
Abril-Rodriguez, G., and Ribas, A. (2017). SnapShot: Immune checkpoint inhibitors. Cancer Cell 31 (6), 848. doi:10.1016/j.ccell.2017.05.010
Akiyama, M., Kaneko, Y., Yamaoka, K., Kondo, H., and Takeuchi, T. (2016). Association of disease activity with acute exacerbation of interstitial lung disease during tocilizumab treatment in patients with rheumatoid arthritis: A retrospective, case-control study. Rheumatol. Int. 36, 881–889. doi:10.1007/s00296-016-3478-3
Arakawa, A., Vollmer, S., Tietze, J., Galinski, A., Heppt, M. V., Burdek, M., et al. (2019). Clonality of CD4+ blood T cells predicts longer survival with CTLA4 or PD-1 checkpoint inhibition in advanced melanoma. Front. Immunol. 10, 1336. doi:10.3389/fimmu.2019.01336
Baas, M., Besançon, A., Goncalves, T., Valette, F., Yagita, H., Sawitzki, B., et al. (2016). TGFβ-dependent expression of PD-1 and PD-L1 controls CD8+ T cell anergy in transplant tolerance[J]. Elife 5, e08133. doi:10.7554/eLife.08133
Bagaev, A., Kotlov, N., Nomie, K., Svekolkin, V., Gafurov, A., Isaeva, O., et al. (2021). Conserved pan-cancer microenvironment subtypes predict response to immunotherapy. Cancer Cell 39 (6), 845–865.e7. e7. doi:10.1016/j.ccell.2021.04.014
Bagchi, S., Yuan, R., and Engleman, E. G. (2021). Immune checkpoint inhibitors for the treatment of cancer: Clinical impact and mechanisms of response and resistance. Annu. Rev. Pathol. 16 (1), 223–249. doi:10.1146/annurev-pathol-042020-042741
Bassez, A., Vos, H., Van Dyck, L., Floris, G., Arijs, I., Desmedt, C., et al. (2021). A single-cell map of intratumoral changes during anti-PD1 treatment of patients with breast cancer. Nat. Med. 27 (5), 820–832. doi:10.1038/s41591-021-01323-8
Beck, K. E., Blansfield, J. A., Tran, K. Q., Feldman, A. L., Hughes, M. S., Royal, R. E., et al. (2006). Enterocolitis in patients with cancer after antibody blockade of cytotoxic T-lymphocyte-associated antigen 4. J. Clin. Oncol. 24 (15), 2283–2289. doi:10.1200/JCO.2005.04.5716
Bellucci, R., Martin, A., Bommarito, D., Wang, K., Hansen, S. H., Freeman, G. J., et al. (2015). Interferon-γ-induced activation of JAK1 and JAK2 suppresses tumor cell susceptibility to NK cells through upregulation of PD-L1 expression. Oncoimmunology 4 (6), e1008824. doi:10.1080/2162402X.2015.1008824
Berghuis, D., Santos, S. J., Baelde, H. J., Taminiau, A. H., Egeler, R. M., Schilham, M. W., et al. (2011). Pro-inflammatory chemokine-chemokine receptor interactions within the Ewing sarcoma microenvironment determine CD8(+) T-lymphocyte infiltration and affect tumour progression. J. Pathol. 223, 347–357. doi:10.1002/path.2819
Berner, F., Bomze, D., Diem, S., Ali, O. H., Fassler, M., Ring, S., et al. (2019). Association of checkpoint inhibitor-induced toxic effects with shared cancer and tissue antigens in non-small cell lung cancer. JAMA Oncol. 5 (7), 1043–1047. doi:10.1001/jamaoncol.2019.0402
Bhattacharjee, O., Ayyangar, U., Kurbet, A. S., Ashok, D., and Raghavan, S. (2019). Unraveling the ECM-immune cell crosstalk in skin diseases. Front. Cell Dev. Biol. 7, 68. doi:10.3389/fcell.2019.00068
Billottet, C., Quemener, C., and Bikfalvi, A. (2013). CXCR3, a double-edged sword in tumor progression and angiogenesis. Biochim. Biophys. Acta 1836 (2), 287–295. doi:10.1016/j.bbcan.2013.08.002
Binnewies, M., Roberts, E. W., Kersten, K., Chan, V., Fearon, D. F., Merad, M., et al. (2018). Understanding the tumor immune microenvironment (TIME) for effective therapy. Nat. Med. 24 (5), 541–550. doi:10.1038/s41591-018-0014-x
Boes, M., and Meyer-Wentrup, F. (2015). TLR3 triggering regulates PD-L1 (CD274) expression in human neuroblastoma cells. Cancer Lett. 361 (1), 49–56. doi:10.1016/j.canlet.2015.02.027
Bolton, H. A., Zhu, E., Terry, A. M., Guy, T. V., Koh, W. P., Tan, S. Y., et al. (2015). Selective Treg reconstitution during lymphopenia normalizes DC costimulation and prevents graft-versus-host disease. J. Clin. Invest 125, 3627–3641. doi:10.1172/JCI76031
Borghaei, H., Paz-Ares, L., Horn, L., Spigel, D. R., Steins, M., Ready, N. E., et al. (2015). Nivolumab versus docetaxel in advanced nonsquamous non-small-cell lung cancer. N. Engl. J. Med. Overseas. Ed. 373, 1627–1639. doi:10.1056/nejmoa1507643
Brunn, D., Turkowski, K., Günther, S., Weigert, A., Muley, T., Kriegsmann, M., et al. (2021). Interferon regulatory factor 9 promotes lung cancer progression via regulation of versican. Cancers (Basel) 13 (2), 208. doi:10.3390/cancers13020208
Bulliard, Y., Jolicoeur, R., Windman, M., Rue, S. M., Ettenberg, S., Knee, D. A., et al. (2013). Activating Fc γ receptors contribute to the antitumor activities of immunoregulatory receptor-targeting antibodies. J. Exp. Med. 210, 1685–1693. doi:10.1084/jem.20130573
Burbage, M., and Amigorena, S. (2020). A dendritic cell multitasks to tackle cancer. Nature 584 (7822), 533–534. doi:10.1038/d41586-020-02339-9
Calon, A., Tauriello, D., and Batlle, E. (2014). TGF-beta in CAF-mediated tumor growth and metastasis. Semin. Cancer Biol. 25, 15–22. doi:10.1016/j.semcancer.2013.12.008
Camisaschi, C., Casati, C., Rini, F., Perego, M., De Filippo, A., Triebel, F., et al. (2010). LAG-3 expression defines a subset of CD4(+)CD25(high)Foxp3(+) regulatory T cells that are expanded at tumor sites. J. Immunol. 184, 6545–6551. doi:10.4049/jimmunol.0903879
Cappelli, L. C., Brahmer, J. R., Forde, P. M., Le, D. T., Lipson, E. J., Naidoo, J., et al. (2018). Clinical presentation of immune checkpoint inhibitor-induced inflammatory arthritis differs by immunotherapy regimen[J]. Seminars Arthritis Rheumatism 48 (3), 553–557. doi:10.1016/j.semarthrit.2018.02.011
Carbotti, G., Barisione, G., Airoldi, I., Mezzanzanica, D., Bagnoli, M., Ferrero, S., et al. (2015). IL-27 induces the expression of Ido and PD-L1 in human cancer cells. Oncotarget 6 (41), 43267–43280. doi:10.18632/oncotarget.6530
Caroline, R., Long, G. V., Brady, B., Dutriaux, C., Maio, M., Mortier, L., et al. (2015). Nivolumab in previously untreated melanoma without BRAF mutation[J]. N. Engl. J. Med. 372 (4), 320–330.
Carretero, R., Sektioglu, I. M., Garbi, N., Salgado, O. C., Beckhove, P., and Hammerling, G. J. (2015). Eosinophils orchestrate cancer rejection by normalizing tumor vessels and enhancing infiltration of CD8(+) T cells. Nat. Immunol. 16 (6), 609–617. doi:10.1038/ni.3159
Chakravarthy, A., Khan, L., Bensler, N. P., Bose, P., and De Carvalho, D. D. (2018). TGF-β-associated extracellular matrix genes link cancer-associated fibroblasts to immune evasion and immunotherapy failure. Nat. Commun. 9 (1), 4692. doi:10.1038/s41467-018-06654-8
Chang, M. Y., Kang, I., Gale, M., Manicone, A. M., Kinsella, M. G., Braun, K. R., et al. (2017). Versican is produced by Trif- and type I interferon-dependent signaling in macrophages and contributes to fine control of innate immunity in lungs. Am. J. Physiol. Lung Cell. Mol. Physiol. 313 (6), L1069–L1086. doi:10.1152/ajplung.00353.2017
Chen, A. Y., Wolchok, J. D., and Bass, A. R. (2021). TNF in the era of immune checkpoint inhibitors: Friend or foe?[J]. Nat. Rev. Rheumatol. 17 (4), 213–223. doi:10.1038/s41584-021-00584-4
Chen, D. S., and Mellman, I. (2017). Elements of cancer immunity and the cancer-immune set point. Nature 541 (7637), 321–330. doi:10.1038/nature21349
Chen, H. M., Touw, W., Wang, Y. S., Kang, K., Mai, S., Zhang, J., et al. (2018). Blocking immunoinhibitory receptor LILRB2 reprograms tumor-associated myeloid cells and promotes antitumor immunity. J. Clin. Invest. 128 (12), 5647–5662. doi:10.1172/JCI97570
Chen, J., Feng, Y., Lu, L., Wang, H., Dai, L., Li, Y., et al. (2012). Interferon-γ-induced PD-L1 surface expression on human oral squamous carcinoma via PKD2 signal pathway. Immunobiology 217 (4), 385–393. doi:10.1016/j.imbio.2011.10.016
Chen, L., Qiu, X., Wang, X., and He, J. (2017). FAP positive fibroblasts induce immune checkpoint blockade resistance in colorectal cancer via promoting immunosuppression. Biochem. Biophys. Res. Commun. 487 (1), 8–14. doi:10.1016/j.bbrc.2017.03.039
Chen, P. L., Roh, W., Reuben, A., Cooper, Z. A., Spencer, C. N., Prieto, P. A., et al. (2016). Analysis of immune signatures in longitudinal tumor samples yields insight into biomarkers of response and mechanisms of resistance to immune checkpoint blockade. Cancer Discov. 6 (8), 827–837. doi:10.1158/2159-8290.CD-15-1545
Chen, Y., Kuchroo, V., Inobe, J., Hafler, D. A., and Weiner, H. L. (1994). Regulatory T cell clones induced by oral tolerance: Suppression of autoimmune encephalomyelitis. Science 265 (5176), 1237–1240. doi:10.1126/science.7520605
Chi, P. L., Cheng, C. C., Hung, C. C., Wang, M. T., Liu, H. Y., Ke, M. W., et al. (2022). MMP-10 from M1 macrophages promotes pulmonary vascular remodeling and pulmonary arterial hypertension. Int. J. Biol. Sci. 18 (1), 331–348. doi:10.7150/ijbs.66472
ChristmasEntinostat, B. J., Rafie, C. I., Hopkins, A. C., Scott, B. A., Ma, H. S., Cruz, K. A., et al. (2018). Entinostat converts immune-resistant breast and pancreatic cancers into checkpoint-responsive tumors by reprogramming tumor-infiltrating MDSCs. Cancer Immunol. Res. 6, 1561–1577. doi:10.1158/2326-6066.CIR-18-0070
Chung, J. Y. F., Chan, M. K. K., Li, J. S. F., Chan, A. S. W., Tang, P. C. T., Leung, K. T., et al. (2021). TGF-Β signaling: From tissue fibrosis to tumor microenvironment. Int. J. Mol. Sci. 22 (14), 7575. doi:10.3390/ijms22147575
Cohen, N., Shani, O., Raz, Y., Sharon, Y., Hoffman, D., Abramovitz, L., et al. (2017). Fibroblasts drive an immunosuppressive and growth-promoting microenvironment in breast cancer via secretion of Chitinase 3-like 1. Oncogene 36, 4457–4468. doi:10.1038/onc.2017.65
Cole, J. E., Navin, T. J., Cross, A. J., Goddard, M. E., Alexopoulou, L., Mitra, A. T., et al. (2011). Unexpected protective role for Toll-like receptor 3 in the arterial wall. Proc. Natl. Acad. Sci. U. S. A. 108 (6), 2372–2377. doi:10.1073/pnas.1018515108
Cooper, Z. A., Reuben, A., Spencer, C. N., Prieto, P. A., Austin-Breneman, J. L., Jiang, H., et al. (2016). Distinct clinical patterns and immune infiltrates are observed at time of progression on targeted therapy versus immune checkpoint blockade for melanoma. Oncoimmunology 5 (3), e1136044. doi:10.1080/2162402X.2015.1136044
Creery, D., Weiss, W., Lim, W. T., AZiZ, Z., Angel, J. B., and KumAr, A. (2004). Down-regulation of CXCR-4 and CCR-5 expression by interferon-gamma is associated with inhibition of chemotaxis and human immunodeficiency virus (HIV) replication but not HIV entry into human monocytes. Clin. Exp. Immunol. 137 (1), 156–165. doi:10.1111/j.1365-2249.2004.02495.x
Daurkin, I., Eruslanov, E., Vieweg, J., and Kusmartsev, S. (2010). Generation of antigen-presenting cells from tumor-infiltrated CD11b myeloid cells with DNA demethylating agent 5-aza-2'-deoxycytidine. Cancer Immunol. Immunother. 59, 697–706. doi:10.1007/s00262-009-0786-4
Dulos, J., Carven, G. J., Boxtel, S. V., Evers, S., Driessen-Engels, L. J. A., Hobo, W., et al. (2012). PD-1 blockade augments Th1 and Th17 and suppresses Th2 responses in peripheral blood from patients with prostate and advanced melanoma cancer. J. Immunother. 35 (2), 169–178. doi:10.1097/CJI.0b013e318247a4e7
Eppihimer, M. J., Gunn, J., Freeman, G. J., Greenfield, E. A., Chernova, T., Erickson, J., et al. (2015). Expression and regulation of the PD-L1 immunoinhibitory molecule on microvascular endothelial cells. Microcirculation 9 (2), 133–145. doi:10.1038/sj/mn/7800123
Eruslanov, E. B., Bhojnagarwala, P. S., Quatromoni, J. G., Stephen, T. L., Ranganathan, A., Deshpande, C., et al. (2014). Tumor-associated neutrophils stimulate T cell responses in early-stage human lung cancer. J. Clin. Invest. 124 (12), 5466–5480. doi:10.1172/JCI77053
Eschweiler, S., Ramírez-Suástegui, C., Li, Y., King, E., Chudley, L., Thomas, J., et al. (2022). Intermittent PI3Kδ inhibition sustains anti-tumour immunity and curbs irAEs. Nature 605 (7911), 741–746. doi:10.1038/s41586-022-04685-2
Evanko, S. P., Potter-Perigo, S., Bollyky, P. L., Nepom, G. T., and Wight, T. N. (2012). Hyaluronan and versican in the control of human T-lymphocyte adhesion and migration. Matrix Biol. 31 (2), 90–100. doi:10.1016/j.matbio.2011.10.004
Fehrenbacher, L., Spira, A., Ballinger, M., Kowanetz, M., Vansteenkiste, J., Mazieres, J., et al. (2016). Atezolizumab versus docetaxel for patients with previously treated non-small-cell lung cancer (POPLAR): A multicentre, open-label, phase 2 randomised controlled trial. Lancet 387 (10030), 1837–1846. doi:10.1016/S0140-6736(16)00587-0
Feig, C., Jones, J. O., Kraman, M., Wells, R. J. B., Deonarine, A., Chan, D. S., et al. (2013). Targeting CXCL12 from FAP-expressing carcinoma-associated fibroblasts synergizes with anti-PD-L1 immunotherapy in pancreatic cancer. Proc. Natl. Acad. Sci. U. S. A. 110 (50), 20212–20217. doi:10.1073/pnas.1320318110
Fridlender, Z. G., Buchlis, G., Kapoor, V., Cheng, G., Sun, J., Singhal, S., et al. (2010). CCL2 blockade augments cancer immunotherapy. Cancer Res. 70 (1), 109–118. doi:10.1158/0008-5472.CAN-09-2326
Friedline, R. H., Brown, D. S., Nguyen, H., Kornfeld, H., Lee, J., Zhang, Y., et al. (2009). CD4+ regulatory T cells require CTLA-4 for the maintenance of systemic tolerance. J. Exp. Med. 206 (2), 421–434. doi:10.1084/jem.20081811
Fujita, M. (2021). EGCG inhibits tumor growth in melanoma by targeting JAK-STAT signaling and its downstream PD-L1/PD-L2-PD1 Axis in tumors and enhancing cytotoxic T-cell responses[J]. Pharmaceuticals 14 (11), 1081.
Gabrilovich, D. I. (2017). Myeloid-derived suppressor cells. Cancer Immunol. Res. 5 (1), 3–8. doi:10.1158/2326-6066.CIR-16-0297
Gaggioli, C., Hooper, S., Hidalgo-Carcedo, C., Grosse, R., Marshall, J. F., Harrington, K., et al. (2007). Fibroblast-led collective invasion of carcinoma cells with differing roles for RhoGTPases in leading and following cells. Nat. Cell Biol. 9 (12), 1392–1400. doi:10.1038/ncb1658
Gang, H., Qianjun, W., Yongliang, Z., Qiangguo, G., Yun, B., et al. (2013). NF-κB plays a key role in inducing CD274 expression in human monocytes after lipopolysaccharide treatment[J]. Plos One 8 (4), e61602.
Garcia-Diaz, A., Shin, D. S., Moreno, B. H., Saco, J., Escuin-Ordinas, H., Rodriguez, G. A., et al. (2017). Interferon receptor signaling pathways regulating PD-L1 and PD-L2 expression. Cell Rep. 19 (6), 1189–1201. doi:10.1016/j.celrep.2017.04.031
García-Palmero, I., Torres, S., Bartolomé, R. A., Pelaez-GArciA, A., Larriba, M. J., Lopez-LucendoM., , et al. (2016). Twist1-induced activation of human fibroblasts promotes matrix stiffness by upregulating palladin and collagen α1(VI). Oncogene 35 (40), 5224–5236. doi:10.1038/onc.2016.57
Godefroy, E., Gallois, A., Idoyaga, J., Merad, M., Tung, N., Monu, N., et al. (2014). Activation of toll-like receptor-2 by endogenous matrix metalloproteinase-2 modulates dendritic-cell-mediated inflammatory responses. Cell Rep. 9 (5), 1856–1870. doi:10.1016/j.celrep.2014.10.067
Goldinger, S. M., Stieger, P., Meier, B., Micaletto, S., Contassot, E., French, L. E., et al. (2016). Cytotoxic cutaneous adverse drug reactions during anti-PD-1 therapy. Clin. Cancer Res. 22 (16), 4023–4029. doi:10.1158/1078-0432.CCR-15-2872
Gordon, S. R., Maute, R. L., Dulken, B. W., Hutter, G., George, B. M., McCracken, M. N., et al. (2017). PD-1 expression by tumour-associated macrophages inhibits phagocytosis and tumour immunity. Nature 545 (7655), 495–499. doi:10.1038/nature22396
Gorter, A., Zijlmans, H. J., van Gent, H., Trimbos, J. B., Fleuren, G. J., and Jordanova, E. S. (2010). Versican expression is associated with tumor-infiltrating CD8-positive T cells and infiltration depth in cervical cancer. Mod. Pathol. 23 (12), 1605–1615. doi:10.1038/modpathol.2010.154
Gowrishankar, K., Gunatilake, D., Gallagher, S. J., Tiffen, J., Rizos, H., and Hersey, P. (2015). Inducible but not constitutive expression of PD-L1 in human melanoma cells is dependent on activation of NF-κB[J]. Plos One 10 (4), e0123410. doi:10.1371/journal.pone.0123410
Grum-Schwensen, B., Klingelhofer, J., Berg, C. H., El-Naaman, C., Grigorian, M., Lukanidin, E., et al. (2005). Suppression of tumor development and metastasis formation in mice lacking the S100A4(mts1) gene. Cancer Res. 65 (9), 3772–3780. doi:10.1158/0008-5472.CAN-04-4510
Hallmann, R., Zhang, X., Di Russo, J., Li, L., Song, J., Hannocks, M. J., et al. (2015). The regulation of immune cell trafficking by the extracellular matrix. Curr. Opin. Cell Biol. 36, 54–61. doi:10.1016/j.ceb.2015.06.006
Han, N., Li, X., Wang, Y., Wang, L., Zhang, C., Zhang, Z., et al. (2021). Increased tumor-infiltrating plasmacytoid dendritic cells promote cancer cell proliferation and invasion via TNF-α/NF-κB/CXCR-4 pathway in oral squamous cell carcinoma. J. Cancer 12 (10), 3045–3056. doi:10.7150/jca.55580
Harlin, H., Meng, Y., Peterson, A. C., Zha, Y., Tretiakova, M., Slingluff, C., et al. (2009). Chemokine expression in melanoma metastases associated with CD8+ T-cell recruitment. Cancer Res. 69 (7), 3077–3085. doi:10.1158/0008-5472.CAN-08-2281
Hart, K. M., Byrne, K. T., Molloy, M. J., Usherwood, E. M., and Berwin, B. (2011). IL-10 immunomodulation of myeloid cells regulates a murine model of ovarian cancer. Front. Immunol. 2, 29. doi:10.3389/fimmu.2011.00029
Haslam, A., and Prasad, V. (2019). Estimation of the percentage of US patients with cancer who are eligible for and respond to checkpoint inhibitor immunotherapy drugs. JAMA Netw. Open 2 (5), e192535. doi:10.1001/jamanetworkopen.2019.2535
Hegde, P. S., and Chen, D. S. (2020). Top 10 challenges in cancer immunotherapy. Immunity 52, 17–35. doi:10.1016/j.immuni.2019.12.011
Ho, Y. J., Li, J. P., Fan, C. H., Liu, H. L., and Yeh, C. K. (2020). Ultrasound in tumor immunotherapy: Current status and future developments. J. Control. Release 323, 12–23. doi:10.1016/j.jconrel.2020.04.023
Hope, C., Emmerich, P. B., Papadas, A., Pagenkopf, A., Matkowskyj, K. A., Van De Hey, D. R., et al. (2017). Versican-derived matrikines regulate batf3-dendritic cell differentiation and promote T cell infiltration in colorectal cancer. J. Immunol. 199 (5), 1933–1941. doi:10.4049/jimmunol.1700529
Hopkins, A. C., Yarchoan, M., Durham, J. N., Yusko, E. C., Rytlewski, J. A., Robins, H. S., et al. (2018). T cell receptor repertoire features associated with survival in immunotherapy-treated pancreatic ductal adenocarcinoma. JCI Insight 3 (13), 122092. doi:10.1172/jci.insight.122092
Hu, H., Zakharov, P. N., Peterson, O. J., and Unanue, E. R. (2020). Cytocidal macrophages in symbiosis with CD4 and CD8 T cells cause acute diabetes following checkpoint blockade of PD-1 in NOD mice. Proc. Natl. Acad. Sci. U. S. A. 117 (49), 31319–31330. doi:10.1073/pnas.2019743117
Huang, C. T., Workman, C. J., Flies, D., Pan, X., Marson, A. L., Zhou, G., et al. (2004). Role of LAG-3 in regulatory T cells. Immunity 21 (4), 503–513. doi:10.1016/j.immuni.2004.08.010
Huang, R. R., Jalil, J., Economou, J. S., Chmielowski, B., Koya, R. C., Mok, S., et al. (2011). CTLA4 blockade induces frequent tumor infiltration by activated lymphocytes regardless of clinical responses in humans. Clin. Cancer Res. 17 (12), 4101–4109. doi:10.1158/1078-0432.CCR-11-0407
Hurkmans, D. P., Jensen, C., Koolen, S. L. W., Aerts, J., Karsdal, M. A., Mathijssen, R. H. J., et al. (2020). Blood-based extracellular matrix biomarkers are correlated with clinical outcome after PD-1 inhibition in patients with metastatic melanoma. J. Immunother. Cancer 8 (2), e001193. doi:10.1136/jitc-2020-001193
Huse, M. (2017). Mechanical forces in the immune system. Nat. Rev. Immunol. 17, 679–690. doi:10.1038/nri.2017.74
Hynes, R. O. (2009). The extracellular matrix: Not just pretty fibrils. Science 326 (5957), 1216–1219. doi:10.1126/science.1176009
Iga, N., Otsuka, A., Yamamoto, Y., Nakashima, C., Honda, T., Kitoh, A., et al. (2019). Accumulation of exhausted CD8+ T cells in extramammary Paget's disease. PLoS ONE 14 (1), e0211135. doi:10.1371/journal.pone.0211135
Ishihara, J., Fukunaga, K., Ishihara, A., Larsson, H. M., Potin, L., Hosseinchi, P., et al. (2017). Matrix-binding checkpoint immunotherapies enhance antitumor efficacy and reduce adverse events. Sci. Transl. Med. 9 (415), eaan0401. doi:10.1126/scitranslmed.aan0401
Jacobetz, M. A., Chan, D. S., Neesse, A., Bapiro, T. E., Cook, N., Frese, K. K., et al. (2013). Hyaluronan impairs vascular function and drug delivery in a mouse model of pancreatic cancer. Gut 62 (1), 112–120. doi:10.1136/gutjnl-2012-302529
Jensen, C., Nissen, N. I., Arenstorff, C., Karsdal, M. A., and Willumsen, N. (2021). Serological assessment of collagen fragments and tumor fibrosis may guide immune checkpoint inhibitor therapy. J. Exp. Clin. Cancer Res. 40 (1), 326. doi:10.1186/s13046-021-02133-z
Jensen, C., Sinkeviciute, D., Madsen, D. H., Onnerfjord, P., Hansen, M., Schmidt, H., et al. (2020). Granzyme B degraded type IV collagen products in serum identify melanoma patients responding to immune checkpoint blockade. Cancers (Basel) 12 (10), 2786. doi:10.3390/cancers12102786
Ji, M., Liu, Y., Li, Q., Li, X. D., Zhao, W. Q., Zhang, H., et al. (2015). PD-1/PD-L1 pathway in non-small-cell lung cancer and its relation with EGFR mutation. J. Transl. Med. 13 (1), 5. doi:10.1186/s12967-014-0373-0
Jiao, S., Subudhi, S. K., Aparicio, A., Ge, Z., Guan, B., Miura, Y., et al. (2019). Differences in tumor microenvironment dictate T helper lineage polarization and response to immune checkpoint therapy. Cell 179 (5), 1177–1190. e13. doi:10.1016/j.cell.2019.10.029
Johnson, D. B., Reynolds, K. L., Sullivan, R. J., Balko, J. M., Patrinely, J. R., Cappelli, L. C., et al. (2020). Immune checkpoint inhibitor toxicities: Systems-based approaches to improve patient care and research[J]. Lancet Oncol. 21 (8), e398–e404. doi:10.1016/S1470-2045(20)30107-8
June, C. H., and Bluestone, J. A. (2017). Is autoimmunity the achilles' heel of cancer immunotherapy? [j]. Nat. Med. 23 (8), 540–547. doi:10.1038/nm.4321
Kagamu, H., Kitano, S., Ou, Y., Yoshimura, K., Horimoto, K., Kitazawa, M., et al. (2020). CD4+ T-cell immunity in the peripheral blood correlates with response to anti-PD-1 therapy. Cancer Immunol. Res. 8 (3), 334–344. doi:10.1158/2326-6066.CIR-19-0574
Karachaliou, N., Gonzalez-Cao, M., Crespo, G., Drozdowskyj, A., Aldeguer, E., Gimenez-Capitan, A., et al. (2018). Interferon gamma, an important marker of response to immune checkpoint blockade in non-small cell lung cancer and melanoma patients. Ther. Adv. Med. Oncol. 10, 1758834017749748. doi:10.1177/1758834017749748
Karakhanova, S., Bedke, T., Enk, A. H., and Mahnke, K. (2011). IL-27 renders DC immunosuppressive by induction of B7-H1. J. Leukoc. Biol. 89 (6), 837–845. doi:10.1189/jlb.1209788
Katsumata, K., Ishihara, J., Mansurov, A., Ishihara, A., Raczy, M. M., Yuba, E., et al. (2019). Targeting inflammatory sites through collagen affinity enhances the therapeutic efficacy of anti-inflammatory antibodies. Sci. Adv. 5 (11), eaay1971. doi:10.1126/sciadv.aay1971
Kim, S. H., Oh, J., Choi, J. Y., Jang, J. Y., Kang, M. W., and Lee, C. E. (2008). Identification of human thioredoxin as a novel IFN-gamma-induced factor: Mechanism of induction and its role in cytokine production. BMC Immunol. 9, 64. doi:10.1186/1471-2172-9-64
Kinter, A. L., Godbout, E. J., Mcnally, J. P., Sereti, I., Roby, G. A., O'Shea, M. A., et al. (2008). The common gamma-chain cytokines IL-2, IL-7, IL-15, and IL-21 induce the expression of programmed death-1 and its ligands. J. Immunol. 181 (10), 6738–6746. doi:10.4049/jimmunol.181.10.6738
Kitano, S., Nakayama, T., and Yamashita, M. (2018). Biomarkers for immune checkpoint inhibitors in melanoma. Front. Oncol. 8 (7), 270. doi:10.3389/fonc.2018.00270
Ko, J. S., Zea, A. H., Rini, B. I., Ireland, J. L., Elson, P., Cohen, P., et al. (2009). Sunitinib mediates reversal of myeloid-derived suppressor cell accumulation in renal cell carcinoma patients. Clin. Cancer Res. 15, 2148–2157. doi:10.1158/1078-0432.CCR-08-1332
Kondo, A., Yamashita, T., Tamura, H., Zhao, W., Tsuji, T., Shimizu, M., et al. (2010). Interferon-gamma and tumor necrosis factor-alpha induce an immunoinhibitory molecule, B7-H1, via nuclear factor-kappaB activation in blasts in myelodysplastic syndromes. Blood 116 (7), 1124–1131. doi:10.1182/blood-2009-12-255125
Korzenik, J., Larsen, M. D., Nielsen, J., Kjeldsen, J., and Norgard, B. M. (2019). Increased risk of developing Crohn's disease or ulcerative colitis in 17 018 patients while under treatment with anti-TNFα agents, particularly etanercept, for autoimmune diseases other than inflammatory bowel disease. Aliment. Pharmacol. Ther. 50, 289–294. doi:10.1111/apt.15370
Krieg, C., Nowicka, M., Guglietta, S., Schindler, S., Hartmann, F. J., Weber, L. M., et al. (2018). High-dimensional single-cell analysis predicts response to anti-PD-1 immunotherapy. Nat. Med. 24 (2), 144–153. doi:10.1038/nm.4466
Kumar, V., Donthireddy, L., Marvel, D., Condamine, T., Wang, F., Lavilla-Alonso, S., et al. (2017). Cancer-associated fibroblasts neutralize the anti-tumor effect of CSF1 receptor blockade by inducing PMN-MDSC infiltration of tumors[J]. Cancer Cell 32 (5), 654–668. e5. doi:10.1016/j.ccell.2017.10.005
Kumar, V., Donthireddy, L., Marvel, D., Condamine, T., Wang, F., Lavilla-Alonso, S., et al. (2017). Cancer-associated fibroblasts neutralize the anti-tumor effect of CSF1 receptor blockade by inducing PMN-MDSC infiltration of tumors. Cancer Cell 32, 654–668. doi:10.1016/j.ccell.2017.10.005
Kurimoto, C., Inaba, H., Ariyasu, H., Iwakura, H., Ueda, Y., Uraki, S., et al. (2020). Predictive and sensitive biomarkers for thyroid dysfunctions during treatment with immune-checkpoint inhibitors. Cancer Sci. 111, 1468–1477. doi:10.1111/cas.14363
Kwon, H. J., Cote, T. R., Cuffe, M. S., Kramer, J. M., and Braun, M. M. (2003). Case reports of heart failure after therapy with a tumor necrosis factor antagonist. Ann. Intern. Med. 138 (10), 807–811. doi:10.7326/0003-4819-138-10-200305200-00008
Lakins, M. A., Ghorani, E., Munir, H., Martins, C. P., and Shields, J. D. (2018). Cancer-associated fibroblasts induce antigen-specific deletion of CD8 + T Cells to protect tumour cells. Nat. Commun. 9 (1), 948. doi:10.1038/s41467-018-03347-0
Li, B., Song, T. N., Wang, F. R., Yin, C., Li, Z., Lin, J. P., et al. (2019). Tumor-derived exosomal HMGB1 promotes esophageal squamous cell carcinoma progression through inducing PD1+ TAM expansion. Oncogenesis 8 (3), 17. doi:10.1038/s41389-019-0126-2
Li, S., Liu, M., Do, M. H., Chou, C., Stamatiades, E. G., Nixon, B. G., et al. (2020). Cancer immunotherapy via targeted TGF-β signalling blockade in TH cells. Nature 587 (7832), 121–125. doi:10.1038/s41586-020-2850-3
Liakou, C. I., Kamat, A., Tang, D. N., Chen, H., Sun, J., Troncoso, P., et al. (2008). CTLA-4 blockade increases IFNgamma-producing CD4+ICOShi cells to shift the ratio of effector to regulatory T cells in cancer patients. Proc. Natl. Acad. Sci. U. S. A. 105 (39), 14987–14992. doi:10.1073/pnas.0806075105
Liao, Y., Zhu, E., and Zhou, W. (2021). Ox-LDL aggravates the oxidative stress and inflammatory responses of THP-1 macrophages by reducing the inhibition effect of miR-491-5p on MMP-9. Front. Cardiovasc. Med. 8, 697236. doi:10.3389/fcvm.2021.697236
Liu, H., Shen, J., and Lu, K. (2017). IL-6 and PD-L1 blockade combination inhibits hepatocellular carcinoma cancer development in mouse model. Biochem. Biophys. Res. Commun. 486 (2), 239–244. doi:10.1016/j.bbrc.2017.02.128
Liu, J., Feng, L., Yu, P., Wang, L., Chen, X., Wang, D., et al. (2015). Local production of the chemokines CCL5 and CXCL10 attracts CD8+ T lymphocytes into esophageal squamous cell carcinoma. Oncotarget 6 (28), 24978–24989. doi:10.18632/oncotarget.4617
Liu, J. M., Jin, Q. X., Fujimoto, M., Li, F. F., Jin, L. B., Yu, R., et al. (2021). Dihydroartemisinin alleviates imiquimod-induced psoriasis-like skin lesion in mice involving modulation of IL-23/Th17 Axis. Front. Pharmacol. 12, 704481. doi:10.3389/fphar.2021.704481
Liubomirski, Y., Lerrer, S., Meshel, T., Morein, D., Rubinstein-Achiasaf, L., Sprinzak, D., et al. (2019). Notch-mediated tumor-stroma-inflammation networks promote invasive properties and CXCL8 expression in triple-negative breast cancer. Front. Immunol. 10, 804. doi:10.3389/fimmu.2019.00804
Ljunggren, H. G., Jonsson, R., and Höglund, P. (2018). Seminal immunologic discoveries with direct clinical implications: The 2018 Nobel Prize in Physiology or Medicine honours discoveries in cancer immunotherapy. Scand. J. Immunol. 88 (6), e12731. doi:10.1111/sji.12731
Loke, P., and Allison, J. P. (2003). PD-L1 and PD-L2 are differentially regulated by Th1 and Th2 cells. Proc. Natl. Acad. Sci. U. S. A. 100 (9), 5336–5341. doi:10.1073/pnas.0931259100
Lu, T., Ramakrishnan, R., Altiok, S., Youn, J. I., Cheng, P., Celis, E., et al. (2011). Tumor-infiltrating myeloid cells induce tumor cell resistance to cytotoxic T cells in mice. J. Clin. Invest. 121, 4015–4029. doi:10.1172/JCI45862
Lu, Y. C., Yeh, W. C., and Ohashi, P. S. (2008). LPS/TLR4 signal transduction pathway. Cytokine 42 (2), 145–151. doi:10.1016/j.cyto.2008.01.006
Luoma, A. M., Suo, S., Williams, H. L., Sharova, T., Sullivan, K., Manos, M., et al. (2020). Molecular pathways of colon inflammation induced by cancer immunotherapy. Cell 182 (3), 655–671. e622. doi:10.1016/j.cell.2020.06.001
Maekawa, N., Konnai, S., Asano, Y., Sajiki, Y., Deguchi, T., Okagawa, T., et al. (2022). Exploration of serum biomarkers in dogs with malignant melanoma receiving anti-PD-L1 therapy and potential of COX-2 inhibition for combination therapy. Sci. Rep. 12 (1), 9265. doi:10.1038/s41598-022-13484-8
Manou, D., Bouris, P., Kletsas, D., Gotte, M., Greve, B., Moustakas, A., et al. (2020). Serglycin activates pro-tumorigenic signaling and controls glioblastoma cell stemness, differentiation and invasive potential. Matrix Biol. Plus 6-7, 100033. doi:10.1016/j.mbplus.2020.100033
Mantovani, A., Marchesi, F., Malesci, A., Laghi, L., and Allavena, P. (2017). Tumour-associated macrophages as treatment targets in oncology. Nat. Rev. Clin. Oncol. 14, 399–416. doi:10.1038/nrclinonc.2016.217
Mariathasan, S., Turley, S. J., Nickles, D., Castiglioni, A., Yuen, K., Wang, Y., et al. (2018). TGFβ attenuates tumour response to PD-L1 blockade by contributing to exclusion of T cells. Nature 554 (7693), 544–548. doi:10.1038/nature25501
Martens, A., Wistuba-Hamprecht, K., Yuan, J., Postow, M. A., Wong, P., Capone, M., et al. (2016). Increases in absolute lymphocytes and circulating CD4+ and CD8+ T cells are associated with positive clinical outcome of melanoma patients treated with ipilimumab. Clin. Cancer Res. 22 (19), 4848–4858. doi:10.1158/1078-0432.CCR-16-0249
Mazanet, M. M., and Hughes, C. (2002). B7-H1 is expressed by human endothelial cells and suppresses T cell cytokine synthesis. J. Immunol. 169 (7), 3581–3588. doi:10.4049/jimmunol.169.7.3581
McDermott, D. F., Huseni, M. A., Atkins, M. B., Motzer, R. J., Rini, B. I., Escudier, B., et al. (2018). Clinical activity and molecular correlates of response to atezolizumab alone or in combination with bevacizumab versus sunitinib in renal cell carcinoma. Nat. Med. 24 (6), 749–757. doi:10.1038/s41591-018-0053-3
Mcinnes, I. B., Buckley, C. D., and Isaacs, J. D. (2016). Cytokines in rheumatoid arthritis - shaping the immunological landscape. Nat. Rev. Rheumatol. 12, 63–68. doi:10.1038/nrrheum.2015.171
Mellor, A. L., and Munn, D. H. (2004). Ido expression by dendritic cells: Tolerance and tryptophan catabolism. Nat. Rev. Immunol. 4, 762–774. doi:10.1038/nri1457
Meng, S., Liu, L. L., Hou, J., Xu, Y., Qin, L., Liu, W., et al. (2020). PD-L1 upregulation by IFN-α/γ-mediated Stat1 suppresses anti-HBV T cell response[J]. PLoS One 15 (7), e0228302.
Messmer, M. N., Netherby, C. S., Banik, D., and Abrams, S. I. (2015). Tumor-induced myeloid dysfunction and its implications for cancer immunotherapy. Cancer Immunol. Immunother. 64, 1–13. doi:10.1007/s00262-014-1639-3
Meyer, C., Cagnon, L., Costa-Nunes, C. M., Baumgaertner, P., Montandon, N., Leyvraz, L., et al. (2014). Frequencies of circulating MDSC correlate with clinical outcome of melanoma patients treated with ipilimumab. Cancer Immunol. Immunother. 63, 247–257. doi:10.1007/s00262-013-1508-5
Mezzadra, R., Chong, S., Jae, L. T., Gomez-Eerland, R., de Vries, E., Wu, W., et al. (2017). Identification of CMTM6 and CMTM4 as PD-L1 protein regulators. Nature 549 (7670), 106–110. doi:10.1038/nature23669
Mok, S., Koya, R. C., Tsui, C., Xu, J., Robert, L., Wu, L., et al. (2014). Inhibition of CSF-1 receptor improves the antitumor efficacy of adoptive cell transfer immunotherapy. Cancer Res. 74, 153–161. doi:10.1158/0008-5472.CAN-13-1816
Muchová, J., Hearnden, V., Michlovská, L., Vistejnova, L., Zavadakova, A., Smerkova, K., et al. (2021). Mutual influence of selenium nanoparticles and FGF2-stab® on biocompatible properties of collagen/chitosan 3D scaffolds: In vitro and ex ovo evaluation. J. Nanobiotechnology 19 (1), 103. doi:10.1186/s12951-021-00849-w
Mukherji, D., Jabbour, M. N., Saroufim, M., Temraz, S., Nasr, R., Charafeddine, M., et al. (2016). Programmed death-ligand 1 expression in muscle-invasive bladder cancer cystectomy specimens and lymph node metastasis: A reliable treatment selection biomarker? Clin. Genitourin. Cancer 14 (2), 183–187. doi:10.1016/j.clgc.2015.12.002
Murata, S., Kaneko, S., Harada, Y., Aoi, N., and Morita, E. (2017). Case of de novo psoriasis possibly triggered by nivolumab. J. Dermatol. 44, 99–100. doi:10.1111/1346-8138.13450
Muthuswamy, R., Corman, J. M., Dahl, K., Chatta, G. S., and Kalinski, P. (2016). Functional reprogramming of human prostate cancer to promote local attraction of effector CD8(+) T cells. Prostate 76 (12), 1095–1105. doi:10.1002/pros.23194
Na, L., Formisano, L., Gonzalez-Ericsson, P. I., Sanchez, V., Dean, P. T., Opalenik, S. R., et al. (2018). Melanoma response to anti-PD-L1 immunotherapy requires JAK1 signaling, but not JAK2[J]. OncoImmunology 7 (3), e1438106.
Nagaraj, S., Gupta, K., Pisarev, V., Kinarsky, L., Sherman, S., Kang, L., et al. (2007). Altered recognition of antigen is a mechanism of CD8+ T cell tolerance in cancer. Nat. Med. 13, 828–835. doi:10.1038/nm1609
Nakashima, D., Onuma, T., Tanabe, K., Kito, Y., Uematsu, K., Mizutani, D., et al. (2020). Synergistic effect of collagen and CXCL12 in the low doses on human platelet activation. PLoS One 15 (10), e0241139. doi:10.1371/journal.pone.0241139
Nam, S., Lee, A., Lim, J., and Lim, J. S. (2019). Analysis of the expression and regulation of PD-1 protein on the surface of myeloid-derived suppressor cells (MDSCs). Biomol. Ther. 27 (1), 63–70. doi:10.4062/biomolther.2018.201
Nguyen, D. P., Li, J., and Tewari, A. K. (2014). Inflammation and prostate cancer: The role of interleukin 6 (IL-6). BJU Int. 113 (6), 986–992. doi:10.1111/bju.12452
Ni, X. Y., Sui, H. X., Liu, Y., Ke, S. Z., Wang, Y. N., and Gao, F. G. (2012). TGF-beta of lung cancer microenvironment upregulates B7H1 and GITRL expression in dendritic cells and is associated with regulatory T cell generation[D]. Oncol. Rep. 28, 615–621. doi:10.3892/or.2012.1822
Nikitovic, D., Tzardi, M., Berdiaki, A., Tsatsakis, A., and Tzanakakis, G. N. (2015). Cancer microenvironment and inflammation: Role of hyaluronan. Front. Immunol. 6, 169. doi:10.3389/fimmu.2015.00169
Nissen, N. I., Karsdal, M., and Willumsen, N. (2019). Collagens and Cancer associated fibroblasts in the reactive stroma and its relation to Cancer biology. J. Exp. Clin. Cancer Res. 38 (1), 115. doi:10.1186/s13046-019-1110-6
Noguchi, T., Ward, J. P., Gubin, M. M., Arthur, C. D., Lee, S. H., Hundal, J., et al. (2017). Temporally distinct PD-L1 expression by tumor and host cells contributes to immune escape. Cancer Immunol. Res. 5 (2), 106–117. doi:10.1158/2326-6066.CIR-16-0391
Noman, M. Z., Desantis, G., Janji, B., Hasmim, M., Karray, S., Dessen, P., et al. (2014). PD-L1 is a novel direct target of HIF-1α, and its blockade under hypoxia enhanced MDSC-mediated T cell activation. J. Exp. Med. 211, 781–790. doi:10.1084/jem.20131916
Obermajer, N., Muthuswamy, R., Lesnock, J., Edwards, R. P., and Kalinski, P. (2011). Positive feedback between PGE2 and COX2 redirects the differentiation of human dendritic cells toward stable myeloid-derived suppressor cells. Blood 118, 5498–5505. doi:10.1182/blood-2011-07-365825
Orillion, A., Hashimoto, A., Damayanti, N., Shen, L., Adelaiye-Ogala, R., Arisa, S., et al. (2017). Entinostat neutralizes myeloid-derived suppressor cells and enhances the antitumor effect of PD-1 inhibition in murine models of lung and renal cell carcinoma. Clin. Cancer Res. 23, 5187–5201. doi:10.1158/1078-0432.CCR-17-0741
Osei, E. T., Mostaço-Guidolin, L., Hsieh, A., Warner, S. M., Al-Fouadi, M., Wang, M., et al. (2020). Epithelial-interleukin-1 inhibits collagen formation by airway fibroblasts: Implications for asthma. Sci. Rep. 10 (1), 8721. doi:10.1038/s41598-020-65567-z
Ou, J. N., Wiedeman, A. E., and Stevens, A. M. (2012). TNF-α and TGF-β counter-regulate PD-L1 expression on monocytes in systemic lupus erythematosus. Sci. Rep. 2, 295. doi:10.1038/srep00295
Pan, P. Y., Ma, G., Weber, K. J., Ozao-Choy, J., Wang, G., Yin, B., et al. (2010). Immune stimulatory receptor CD40 is required for T-cell suppression and T regulatory cell activation mediated by myeloid-derived suppressor cells in cancer. Cancer Res. 70, 99–108. doi:10.1158/0008-5472.CAN-09-1882
Parry, R. V., Chemnitz, J. M., Frauwirth, K. A., Lanfranco, A. R., Braunstein, I., Kobayashi, S. V., et al. (2005). CTLA-4 and PD-1 receptors inhibit T-cell activation by distinct mechanisms. Mol. Cell. Biol. 25 (21), 9543–9553. doi:10.1128/MCB.25.21.9543-9553.2005
Peng, D., Kryczek, I., Nagarsheth, N., Zhao, L., Wei, S., Wang, W., et al. (2015). Epigenetic silencing of TH1-type chemokines shapes tumour immunity and immunotherapy[J]. Nature 527 (7577), 249–253. doi:10.1038/nature15520
Peng, D. H., Rodriguez, B. L., Diao, L., Chen, L., Wang, J., Byers, L. A., et al. (2020). Collagen promotes anti-PD-1/PD-L1 resistance in cancer through LAIR1-dependent CD8+ T cell exhaustion. Nat. Commun. 11 (1), 4520. doi:10.1038/s41467-020-18298-8
Perez-Ruiz, E., Minute, L., Otano, I., Alvarez, M., Ochoa, M. C., Belsue, V., et al. (2019). Prophylactic TNF blockade uncouples efficacy and toxicity in dual CTLA-4 and PD-1 immunotherapy[J]. Nature 569 (7756), 1–7. doi:10.1038/s41586-019-1162-y
Platanias, L. C. (2005). Mechanisms of type-I- and type-II-interferon-mediated signalling. Nat. Rev. Immunol. 5 (5), 375–386. doi:10.1038/nri1604
Poto, R., Troiani, T., Criscuolo, G., Marone, G., Ciardiello, F., Tocchetti, C. G., et al. (2022). Holistic approach to immune checkpoint inhibitor-related adverse events. Front. Immunol. 30 (13), 804597. doi:10.3389/fimmu.2022.804597
Powles, T., Eder, J. P., Fine, G. D., Braiteh, F. S., Loriot, Y., Cruz, C., et al. (2014). MPDL3280A (anti-PD-L1) treatment leads to clinical activity in metastatic bladder cancer. Nature 194 (7528), 558–562. doi:10.1038/nature13904
Priego, N., Zhu, L., Monteiro, C., Mulders, M., Wasilewski, D., Bindeman, W., et al. (2018). STAT3 labels a subpopulation of reactive astrocytes required for brain metastasis[J]. Nat. Med. 24, 1024–1035. doi:10.1038/s41591-018-0044-4
Puccini, A., Battaglin, F., Iaia, M. L., Lenz, H. J., and Salem, M. E. (2020). Overcoming resistance to anti-PD1 and anti-PD-L1 treatment in gastrointestinal malignancies. J. Immunother. Cancer 8 (1), e000404. doi:10.1136/jitc-2019-000404
Pulko, V., Liu, X., Krco, C. J., Harris, K. J., Frigola, X., Kwon, E. D., et al. (2009). TLR3-stimulated dendritic cells up-regulate B7-H1 expression and influence the magnitude of CD8 T cell responses to tumor vaccination. J. Immunol. 183 (6), 3634–3641. doi:10.4049/jimmunol.0900974
Qian, Y., Deng, J., Geng, L., Xie, H., Jiang, G., Zhou, L., et al. (2008). TLR4 signaling induces B7-H1 expression through MAPK pathways in bladder cancer cells. Cancer Invest. 26 (8), 816–821. doi:10.1080/07357900801941852
Quandt, D., Jasinski-Bergner, S., Müller, U., Schulze, B., and Seliger, B. (2014). Synergistic effects of IL-4 and TNFα on the induction of B7-H1 in renal cell carcinoma cells inhibiting allogeneic T cell proliferation. J. Transl. Med. 12, 151. doi:10.1186/1479-5876-12-151
Ravi, R., Noonan, K. A., Pham, V., Bedi, R., Zhavoronkov, A., Ozerov, I. V., et al. (2018). Bifunctional immune checkpoint-targeted antibody-ligand traps that simultaneously disable TGFβ enhance the efficacy of cancer immunotherapy. Nat. Commun. 9 (1), 741. doi:10.1038/s41467-017-02696-6
Read, S., Greenwald, R., Izcue, A., Robinson, N., Mandelbrot, D., Francisco, L., et al. (2006). Blockade of CTLA-4 on CD4+CD25+ regulatory T cells abrogates their function in vivo. J. Immunol. 177 (7), 4376–4383. doi:10.4049/jimmunol.177.7.4376
Ro, W., Che, P. L., Reube, A., Spencer, C. N., Prieto, P. A., Miller, J. P., et al. (2017). Integrated molecular analysis of tumor biopsies on sequential CTLA-4 and PD-1 blockade reveals markers of response and resistance[J]. Sci. Transl. Med. 9 (379), eaah3560. doi:10.1126/scitranslmed.aah3560
Robert, F., Douglas, N., Christine, B., Popratiloff, A., and Uittenbogaart, C. H. (2018). TGF-Β sustains tumor progression through biochemical and mechanical signal transduction[J]. Cancers 10 (6), 199. doi:10.3390/cancers10060199
Rosenberg, J. E., Hoffman-Censits, J., Powles, T., van der Heijden, M. S., Balar, A. V., Necchi, A., et al. (2016). Atezolizumab in patients with locally advanced and metastatic urothelial carcinoma who have progressed following treatment with platinum-based chemotherapy: A single-arm, multicentre, phase 2 trial. Lancet 387, 1909–1920. doi:10.1016/S0140-6736(16)00561-4
Sade-Feldman, M., Yizhak, K., Bjorgaard, S. L., Ray, J. P., de Boer, C. G., Jenkins, R. W., et al. (2019). Defining T cell states associated with response to checkpoint immunotherapy in melanoma. Cell 175 (4), 998–1013. doi:10.1016/j.cell.2018.10.038
Saleh, K., Khalife-Saleh, N., and Kourie, H. R. (2019). Do immune-related adverse events correlate with response to immune checkpoint inhibitors?[J]. Immunotherapy 11, 257–259. doi:10.2217/imt-2018-0201
Salmon, H., Franciszkiewicz, K., Damotte, D., Dieu-Nosjean, M. C., Validire, P., Trautmann, A., et al. (2012). Matrix architecture defines the preferential localization and migration of T cells into the stroma of human lung tumors. J. Clin. Invest. 122 (3), 899–910. doi:10.1172/JCI45817
Sang, T., Tayar, J., Trinh, V. A., Suarez-Almazor, M., Garcia, S., Hwu, P., et al. (2017). Successful treatment of arthritis induced by checkpoint inhibitors with tocilizumab: A case series. Ann. Rheum. Dis. 76, 2061–2064. doi:10.1136/annrheumdis-2017-211560
Schreiner, B., Mitsdoerffer, M., Kieseier, B. C., Chen, L., Hartung, H. P., Weller, M., et al. (2004). Interferon-beta enhances monocyte and dendritic cell expression of B7-H1 (PD-L1), a strong inhibitor of autologous T-cell activation: Relevance for the immune modulatory effect in multiple sclerosis. J. Neuroimmunol. 155 (1-2), 172–182. doi:10.1016/j.jneuroim.2004.06.013
Sektioglu, I. M., Carretero, R., Bulbuc, N., Bald, T., Tuting, T., Rudensky, A. Y., et al. (2016). Basophils promote tumor rejection via chemotaxis and infiltration of CD8+ T cells. Cancer Res. 77, 291–302. doi:10.1158/0008-5472.CAN-16-0993
Selby, M. J., Engelhardt, J. J., Quigley, M., Henning, K. A., Chen, T., Srinivasan, M., et al. (2013). Anti-CTLA-4 antibodies of IgG2a isotype enhance antitumor activity through reduction of intratumoral regulatory T cells. Cancer Immunol. Res. 1, 32–42. doi:10.1158/2326-6066.CIR-13-0013
Setoguchi, R., Hori, S., Takahashi, T., and Sakaguchi, S. (2005). Homeostatic maintenance of natural Foxp3(+) CD25(+) CD4(+) regulatory T cells by interleukin (IL)-2 and induction of autoimmune disease by IL-2 neutralization. J. Exp. Med. 201 (5), 723–735. doi:10.1084/jem.20041982
Sharma, N., Atolagbe, O. T., Ge, Z., and Allison, J. P. (2021). LILRB4 suppresses immunity in solid tumors and is a potential target for immunotherapy[J]. J. Exp. Med. 218 (7), e20201811. doi:10.1084/jem.20201811
Sharpe, A. H., Wherry, E. J., Ahmed, R., and Freeman, G. J. (2007). The function of programmed cell death 1 and its ligands in regulating autoimmunity and infection. Nat. Immunol. 8 (3), 239–245. doi:10.1038/ni1443
Shuang, H., and Chakrabarty, S. (2010). Regulation of fibronectin and laminin receptor expression, fibronectin and laminin secretion in human colon cancer cells by transforming growth factor-beta 1. Int. J. Cancer 57 (5), 742–746. doi:10.1002/ijc.2910570522
Siddiqui, I., Schaeuble, K., Chennupati, V., Fuertes Marraco, S. A., Calderon-Copete, S., Pais Ferreira, D., et al. (2019). Intratumoral Tcf1+PD-1+cd8+ T cells with stem-like properties promote tumor control in response to vaccination and checkpoint blockade immunotherapy. Immunity 50 (1), 195–211. doi:10.1016/j.immuni.2018.12.021
Simpson, T. R., Li, F., Montalvo-Ortiz, W., Sepulveda, M. A., Bergerhoff, K., Arce, F., et al. (2013). Fc-dependent depletion of tumor-infiltrating regulatory T cells co-defines the efficacy of anti-CTLA-4 therapy against melanoma. J. Exp. Med. 210, 1695–1710. doi:10.1084/jem.20130579
Singhal, S., Bhojnagarwala, P. S., O'Brien, S., Moon, E. K., Garfall, A. L., Rao, A. S., et al. (2016). Origin and role of a subset of tumor-associated neutrophils with antigen-presenting cell features in early-stage human lung cancer[J]. Cancer Cell 30, 120–135. doi:10.1016/j.ccell.2016.06.001
Sockolosky, J. T., Dougan, M., Ingram, J. R., Ho, C. C. M., Kauke, M. J., Almo, S. C., et al. (2016). Durable antitumor responses to CD47 blockade require adaptive immune stimulation. Proc. Natl. Acad. Sci. U. S. A. 113 (19), E2646–E2654. doi:10.1073/pnas.1604268113
Song, S., Yuan, P., Wu, H., Chen, J., Fu, J., Li, P., et al. (2014). Dendritic cells with an increased PD-L1 by TGF-β induce T cell anergy for the cytotoxicity of hepatocellular carcinoma cells. Int. Immunopharmacol. 20 (1), 117–123. doi:10.1016/j.intimp.2014.02.027
Steele, C. W., Karim, S. A., Leach, J. D. G., Bailey, P., Upstill-Goddard, R., Rishi, L., et al. (2016). CXCR2 inhibition profoundly suppresses metastases and augments immunotherapy in pancreatic ductal adenocarcinoma. Cancer Cell 29, 832–845. doi:10.1016/j.ccell.2016.04.014
Stefani, S., Riyue, B., and Gajewski, T. F. (2015). Melanoma-intrinsic β-catenin signalling prevents anti-tumour immunity[J]. Nature 523 (7559), 231–235.
Stroud, C. R., Hegde, A., Cherry, C., Naqash, A. R., Sharma, N., Addepalli, S., et al. (2019). Tocilizumab for the management of immune mediated adverse events secondary to PD-1 blockade. J. Oncol. Pharm. Pract. 25 (3), 551–557. doi:10.1177/1078155217745144
Subrahmanyam, P. B., Dong, Z., Gusenleitner, D., Giobbie-Hurder, A., Severgnini, M., Zhou, J., et al. (2018). Distinct predictive biomarker candidates for response to anti-CTLA-4 and anti-PD-1 immunotherapy in melanoma patients. J. Immunother. Cancer 6 (1), 18. doi:10.1186/s40425-018-0328-8
Sun, L., Clavijo, P. E., Robbins, Y., Patel, P., Friedman, J., Greene, S., et al. (2019). Inhibiting myeloid-derived suppressor cell trafficking enhances T cell immunotherapy. JCI Insight 4 (7), e126853. doi:10.1172/jci.insight.126853
Takahashi, T., Kuniyasu, Y., Toda, M., SakaguchiN., , ItohM., , IwataM., , et al. (1998). Immunologic self-tolerance maintained by CD25+CD4+ naturally anergic and suppressive T cells: Induction of autoimmune disease by breaking their anergic/suppressive state. Int. Immunol. 10 (12), 1969–1980. doi:10.1093/intimm/10.12.1969
Tanaka, R., Okiyama, N., Okune, M., Ishitsuka, Y., Watanabe, R., Furuta, J., et al. (2017). Serum level of interleukin-6 is increased in nivolumab-associated psoriasiform dermatitis and tumor necrosis factor-alpha is a biomarker of nivolumab recativity[J]. J. Dermatological Sci. 86, 71–73. doi:10.1016/j.jdermsci.2016.12.019
Tarhini, A. A., Zahoor, H., Lin, Y., Malhotra, U., Sander, C., Butterfield, L. H., et al. (2015). Baseline circulating IL-17 predicts toxicity while TGF-β1 and IL-10 are prognostic of relapse in ipilimumab neoadjuvant therapy of melanoma. J. Immunother. Cancer 3 (1), 39. doi:10.1186/s40425-015-0081-1
Teng, M., Ngiow, S. F., Ribas, A., and Smyth, M. J. (2015). Classifying cancers based on T-cell infiltration and PD-L1. Cancer Res. 75 (11), 2139–2145. doi:10.1158/0008-5472.CAN-15-0255
Teulings, H. E., Limpens, J., Jansen, S. N., Zwinderman, A. H., Reitsma, J. B., Spuls, P. I., et al. (2015). Vitiligo-like depigmentation in patients with stage III-IV melanoma receiving immunotherapy and its association with survival: A systematic review and meta-analysis. J. Clin. Oncol. 33 (7), 773–781. doi:10.1200/JCO.2014.57.4756
Thiem, A., Hesbacher, S., Kneitz, H., di Primio, T., Heppt, M. V., Hermanns, H. M., et al. (2019). IFN-gamma-induced PD-L1 expression in melanoma depends on p53 expression. J. Exp. Clin. Cancer Res. 38 (1), 397. doi:10.1186/s13046-019-1403-9
Tomioka, N., Azuma, M., Ikarashi, M., Yamamoto, M., Sato, M., Watanabe, K. I., et al. (2018). The therapeutic candidate for immune checkpoint inhibitors elucidated by the status of tumor-infiltrating lymphocytes (TILs) and programmed death ligand 1 (PD-L1) expression in triple negative breast cancer (TNBC). Breast Cancer 25, 34–42. doi:10.1007/s12282-017-0781-0
Tomoko, I., Katsuyuki, A., Kawana, K., Taguchi, A., Nagamatsu, T., Fujimoto, A., et al. (2016). Cancer-associated fibroblast suppresses killing activity of natural killer cells through downregulation of poliovirus receptor (PVR/CD155), a ligand of activating NK receptor. Int. J. Oncol. 49, 1297–1304. doi:10.3892/ijo.2016.3631
Trikha, P., and Carson3rd, W. E. (2014). Signaling pathways involved in MDSC regulation. Biochim. Biophys. Acta 1846, 55–65. doi:10.1016/j.bbcan.2014.04.003
Tsukamoto, H., Fujieda, K., Miyashita, A., Fukushima, S., Ikeda, T., and Kubo, Y. (2018). Combined blockade of IL6 and PD-1/PD-L1 signaling abrogates mutual regulation of their immunosuppressive effects in the tumor microenvironment[J]. Cancer Res. 78, 5011–5022. doi:10.1158/0008-5472.CAN-18-0118
Tumeh, P. C., Harview, C. L., Yearley, J. H., Shintaku, I. P., Taylor, E. J. M., Robert, L., et al. (2014). PD-1 blockade induces responses by inhibiting adaptive immune resistance. Nature 515 (7528), 568–571. doi:10.1038/nature13954
Türlü, C., Willumsen, N., Marando, D., Schjerling, P., Biskup, E., Hannibal, J., et al. (2021). A human cellular model for colorectal anastomotic repair: The effect of localization and transforming growth factor-β1 treatment on collagen deposition and biomarkers. Int. J. Mol. Sci. 22 (4), 1616. doi:10.3390/ijms22041616
Umair, M. M., Athanasios, P., Adam, P., Evan, F., Zachary, M., Sibgha, G., et al. (2018). Tumor matrix remodeling and novel immunotherapies: The promise of matrix-derived immune biomarkers[J]. J. Immunother. Cancer 6 (1), 65.
Uyttenhove, C., Pilotte, L., Theate, I., Stroobant, V., Colau, D., Parmentier, N., et al. (2003). Evidence for a tumoral immune resistance mechanism based on tryptophan degradation by indoleamine 2, 3-dioxygenase. Nat. Med. 9, 1269–1274. doi:10.1038/nm934
Valk, E., Rudd, C. E., and Schneider, H. (2008). CTLA-4 trafficking and surface expression. Trends Immunol. 29 (6), 272–279. doi:10.1016/j.it.2008.02.011
van der Woude, L. L., Gorris, M. A. J., Halilovic, A., Figdor, C. G., and de Vries, I. J. M. (2017). Migrating into the tumor: A roadmap for T cells[J]. Trends Cancer 3 (11), 797. doi:10.1016/j.trecan.2017.09.006
van Elsas, A., Hurwitz, A. A., and Allison, J. P. (1999). Combination immunotherapy of B16 melanoma using anti-cytotoxic T lymphocyte-associated antigen 4 (CTLA-4) and granulocyte/macrophage colony-stimulating factor (GM-CSF)-producing vaccines induces rejection of subcutaneous and metastatic tumors accompanied by autoimmune depigmentation. J. Exp. Med. 190 (3), 355–366. doi:10.1084/jem.190.3.355
Vivar, K. L., Deschaine, M., Messina, J., Divine, J. M., Rabionet, A., Patel, N., et al. (2017). Epidermal programmed cell death-ligand 1 expression in TEN associated with nivolumab therapy. J. Cutan. Pathol. 44, 381–384. doi:10.1111/cup.12876
Voskens, C. J., Goldinger, S. M., Loquai, C., Robert, C., Kaehler, K. C., Berking, C., et al. (2013). The price of tumor control: An analysis of rare side effects of anti-CTLA-4 therapy in metastatic melanoma from the ipilimumab network. PLoS One 8, e53745. doi:10.1371/journal.pone.0053745
Wang, J., Luo, D., Liang, M., Zhang, T., Yin, X., Zhang, Y., et al. (2018). Spectrum-effect relationships between high-performance liquid chromatography (HPLC) fingerprints and the antioxidant and anti-inflammatory activities of collagen peptides. Molecules 23 (12), 3257. doi:10.3390/molecules23123257
Wang, L., Abdel, S., Szabo, P. M., Chasalow, S. D., Castillo-Martin, M., Domingo-Domenech, J., et al. (2018). EMT- and stroma-related gene expression and resistance to PD-1 blockade in urothelial cancer. Nat. Commun. 9 (1), 3503. doi:10.1038/s41467-018-05992-x
Wang, X., Yang, L., Huang, F., Zhang, Q., Liu, S., Ma, L., et al. (2017). Inflammatory cytokines IL-17 and TNF-α up-regulate PD-L1 expression in human prostate and colon cancer cells. Immunol. Lett. 184, 7–14. doi:10.1016/j.imlet.2017.02.006
Wasén, C., Erlandsson, M., Bossios, A., Ekerljung, L., Malmhall, C., Toyra Silfversward, S., et al. (2018). Smoking is associated with low levels of soluble PD-L1 in rheumatoid arthritis. Front. Immunol. 9, 1677. doi:10.3389/fimmu.2018.01677
Weber, J., Gibney, G., Kudchadkar, R., Yu, B., Cheng, P., Martinez, A. J., et al. (2016). Phase I/II study of metastatic melanoma patients treated with nivolumab who had progressed after ipilimumab. Cancer Immunol. Res. 4, 345–353. doi:10.1158/2326-6066.CIR-15-0193
Weber, J. S., Yang, J. C., Atkins, M. B., and Disis, M. L. (2015). Toxicities of immunotherapy for the practitioner. J. Clin. Oncol. 33 (18), 2092–2099. doi:10.1200/JCO.2014.60.0379
Wen, X., He, X., Jiao, F., Wang, C., Sun, Y., Ren, X., et al. (2017). Fibroblast activation protein-α-positive fibroblasts promote gastric cancer progression and resistance to immune checkpoint blockade. Oncol. Res. 25 (4), 629–640. doi:10.3727/096504016X14768383625385
Wight, T. N., Kinsella, M. G., Evanko, S. P., Potter-Perigo, S., and Merrilees, M. J. (2014). Versican and the regulation of cell phenotype in disease. Biochim. Biophys. Acta 1840 (8), 2441–2451. doi:10.1016/j.bbagen.2013.12.028
Xia, Q., Zhang, F. F., Geng, F., Liu, C. L., Xu, P., Lu, Z. Z., et al. (2016). Anti-tumor effects of DNA vaccine targeting human fibroblast activation protein alpha by producing specific immune responses and altering tumor microenvironment in the 4T1 murine breast cancer model[J]. Cancer Immunol. Immunother. 65 (5), 613–624. doi:10.1007/s00262-016-1827-4
Xiang, X., Feng, D., Hwang, S., Ren, T., Wang, X., Trojnar, E., et al. (2020). Interleukin-22 ameliorates acute-on-chronic liver failure by reprogramming impaired regeneration pathways in mice. J. Hepatol. 72 (4), 736–745. doi:10.1016/j.jhep.2019.11.013
Xing, X., Guo, J., Wen, X., Li, B., Dong, B., Feng, Q., et al. (2018). Analysis of PD1, PDL1, PDL2 expression and T cells infiltration in 1014 gastric cancer patients. Oncoimmunology 7, e1356144. doi:10.1080/2162402X.2017.1356144
Xiong, H. Y., Ma, T. T., Wu, B. T., Lin, Y., and Tu, Z. G. (2014). IL-12 regulates B7-H1 expression in ovarian cancer-associated macrophages by effects on NF-κB signalling. Asian pac. J. Cancer Prev. 15 (14), 5767–5772. doi:10.7314/apjcp.2014.15.14.5767
Yagi, T., Baba, Y., Ishimoto, T., Iwatsuki, M., Miyamoto, Y., Yoshida, N., et al. (2017). PD-L1 expression, tumor-infiltrating lymphocytes, and clinical outcome in patients with surgically resected esophageal cancer[J]. Ann. Surg. 269 (3), 1. doi:10.1097/SLA.0000000000002616
Yi, G., Yang, J., Cai, Y., Fu, S., Zhang, N., Fu, X., et al. (2018). IFN-γ-mediated inhibition of lung cancer correlates with PD-L1 expression and is regulated by PI3K-akt signaling: IFN-γ-mediated signaling and function in lung adenocarcinoma[J]. Int. J. Cancer 143 (4), 931–943. doi:10.1002/ijc.31357
Young, A., Quandt, Z., and Bluestone, J. A. (2018). The balancing act between cancer immunity and autoimmunity in response to immunotherapy. Cancer Immunol. Res. 6 (12), 1445–1452. doi:10.1158/2326-6066.CIR-18-0487
Yue, C., Shen, S., Deng, J., Priceman, S. J., Li, W., Huang, A., et al. (2015). STAT3 in CD8+ T cells inhibits their tumor accumulation by downregulating CXCR3/CXCL10 Axis. Cancer Immunol. Res. 3 (8), 864–870. doi:10.1158/2326-6066.CIR-15-0014
Zhang, R., Zhu, Z., Lv, H., Li, F., Sun, S., Li, J., et al. (2019). Immune checkpoint blockade mediated by a small-molecule nanoinhibitor targeting the PD-1/PD-L1 pathway synergizes with photodynamic therapy to elicit antitumor immunity and antimetastatic effects on breast cancer. Small 15 (49), e1903881. doi:10.1002/smll.201903881
Zhang, W., Lim, S. M., Hwang, J., Ramalingam, S., Kim, M., and Jin, J. O. (2020). Monophosphoryl lipid A-induced activation of plasmacytoid dendritic cells enhances the anti-cancer effects of anti-PD-L1 antibodies[J]. Cancer Immunol. Immunother. 70, 1–12. doi:10.1007/s00262-020-02715-4
Keywords: immune checkpoint inhibitors, inflammatory factors, programmed death-1, immune-related adverse events, tumor microenvironment
Citation: Wu Y, Yu S and Qiao H (2022) Understanding the functional inflammatory factors involved in therapeutic response to immune checkpoint inhibitors for pan-cancer. Front. Pharmacol. 13:990445. doi: 10.3389/fphar.2022.990445
Received: 10 July 2022; Accepted: 10 August 2022;
Published: 01 September 2022.
Edited by:
Heng Sun, University of Macau, ChinaCopyright © 2022 Wu, Yu and Qiao. This is an open-access article distributed under the terms of the Creative Commons Attribution License (CC BY). The use, distribution or reproduction in other forums is permitted, provided the original author(s) and the copyright owner(s) are credited and that the original publication in this journal is cited, in accordance with accepted academic practice. No use, distribution or reproduction is permitted which does not comply with these terms.
*Correspondence: Shan Yu, eXVzaGFuQGhyYm11LmVkdS5jbg==; Hong Qiao, cWhvbmcwODIzQDE2My5jb20=
Disclaimer: All claims expressed in this article are solely those of the authors and do not necessarily represent those of their affiliated organizations, or those of the publisher, the editors and the reviewers. Any product that may be evaluated in this article or claim that may be made by its manufacturer is not guaranteed or endorsed by the publisher.
Research integrity at Frontiers
Learn more about the work of our research integrity team to safeguard the quality of each article we publish.