- 1Department of Anaesthesiology and Perioperative Medicine, The Key Laboratory of Anesthesiology and Perioperative Medicine of Anhui Higher Education Institutes, The Second Hospital of Anhui Medical University, Hefei, China
- 2Department of Urology, The Second Hospital of Anhui Medical University, Hefei, China
Background: For localized disease, partial nephrectomy of small tumors continues to be the gold-standard treatment. However, temporary clamping is routinely performed during this process to control renal blood flow, which can cause renal ischemic/reperfusion injury. We evaluated whether dexmedetomidine postconditioning (DPOC) can reduce renal ischemic/reperfusion injury for patients receiving laparoscopic partial nephrectomy (LPN).
Methods: This randomized double-blind controlled trial included 77 patients who were scheduled for LPN at our hospital. Patients were randomly allocated to the DPOC or control group. DPOC was performed via intravenous administration of dexmedetomidine at 0.6 μg kg−1 for 10 min immediately after unclamping the renal artery. In the control group, saline was administered in place of dexmedetomidine under the same protocol. All participants underwent a 6-month follow-up. The primary outcome were the values of 99mTc-DTPA-GFR in the affected kidney at one and 6 months post-LPN.
Result: The GFR values in the DPOC group (35.65 ± 4.89 ml min−1.1.73 m−2) were significantly higher than those the control group (33.10 ± 5.41 ml min−1.1.73 m−2; p = 0.022) at 1 month after LPN. There was no statistically significant difference in GFR value between the two groups at 6 months after LPN.
Conclusion: DPOC provides therapeutic benefits to LPN patients, at least on a short-term basis, by alleviating renal ischemic/reperfusion injury.
Clinical Trial Registration: Chinese Clinical Trial Registry, identifier [ChiCTR-TRC-14004766].
Introduction
Laparoscopic partial nephrectomy (LPN) has become the standard treatment for small renal tumors (Huang et al., 2006). However, LPN typically requires temporary clamping of renal blood flow, which can prolong the warm ischemia time (Lane et al., 2008; Thompson et al., 2010). This procedure can also induce renal ischemic/reperfusion injury (IRI) and result in acute kidney injury (AKI). The kidney is highly sensitive to IRI due to its high energy demands and intricate microvascular network. Gill et al. (2007) reported that postoperative severe acute kidney injury, as defined by a glomerular filtration rate (GFR) < 15ml−1 min−1 or postoperative dialysis for 30 days, occurs in 3.6% of LPN patients. Lane et al. (2008) further indicate that a warm ischemia time of 20 min during LPN leads to substantially impaired renal function. It is therefore imperative to develop methods that reduce kidney injury in patients undergoing LPN surgery.
Ischemia preconditioning (IPRC) and ischemia postconditioning (IPOC) are two classic protective strategies commonly used in the treatment of IRI (Zhao et al., 2003; Xue et al., 2017). However, because the timing and onset of ischemia are generally unpredictable, it can be difficult to determine whether or not IPRC should be performed. IPOC, defined as rapid intermittent interruptions of blood flow in the early phase of reperfusion, has been proven to effectively protect kidneys from IRI in animal studies (Liu et al., 2007; Jiang et al., 2010; Tan et al., 2015). Although IPOC would seem to be the appropriate treatment, the temporary clamping of the renal artery in humans remains ethically controversial.
An alternative approach, known as pharmacological postconditioning (PPOC), mimics the protective effect of IPOC but uses pharmacological agents rather than clamping. Because alpha-2 adrenoceptors are widely found in the renal proximal and distal tubules, along with the peri-tubular vasculature, the alpha-2 adrenoceptor agonist dexmedetomidine is a logical candidate for use in IPOC. In addition to selectively activating alpha-2 adrenergic agonists, dexmedetomidine is known to have sedative, anxiolytic, hemodynamic stabilizing, and diuretic effects (Kim et al., 2019). PPOC using dexmedetomidine has been found to significantly reduce renal tubule damage and renal IRI in rat kidneys (Kocoglu et al., 2009; Gu et al., 2011; Balci et al., 2017; Kim et al., 2018). By inhibiting ischemia-induced excess noradrenalin secretion, dexmedetomidine may prevent the potential destructive effects of free-oxygen radical production (Kiliç et al., 2012). However, these promising results are based on in vitro and animal studies; it has not yet been determined whether dexmedetomidine postconditioning (DPOC) during LPN in human patients will reduce renal IRI. The goal of this study is accordingly to assess the effectiveness of DPOC in alleviating IRI in patients receiving LPN.
Materials and methods
Patients
This study was approved by the Ethics Committee of the Second Hospital of Anhui Medical University (LW201405). All protocols were registered at www.chictr.org.cn (Reference No: ChiCTR-TRC-14004766, 06/06/2014) and implemented in accordance with the principles of the Helsinki Declarations. The clinical trial was performed in compliance with CONSORT guidelines. All patients enrolled in the trial gave written informed consent. Patients enlisted in this randomized controlled trial were those diagnosed with kidney tumors and waiting for LPN surgery at our hospital between June 2014 and December 2015. The inclusion criteria for this trial were as follows: American Society of Anesthesiologists’ physical status of either 1 or 2, a maximum tumor diameter of 4–6 cm, and normal contralateral renal function (based on a differential renal function measurement of >40% obtained via radionuclide scintigraphy). We excluded patients older than 75 years and those with a history of one or more of the following diseases: heart, pulmonary, or hepatic disease, diabetes mellitus, or renal transplantation. We also excluded patients with acute renal disease, serum creatinine >1.5 mg dL−1, BUN >20 mg dL−1, or significant inflammation (chest radiographs suggest large-scale high-density shadows, white blood cells >1.0 × 109 and patient temperature >38.5°C).
Study design and randomization
Before surgery, the enrolled patients were assigned to either the DPOC group or control group in equal proportions. The randomization was performed by a computer program and the group assignments were sealed in sequentially ordered envelopes to obscure the random allocations. Nurses with knowledge of the group to which each patient was allocated prepared either dexmedetomidine or saline placebo in 50 ml syringes for the anesthesiologists, who administered the drugs and were blinded throughout the study. Accordingly, patients, surgeons, investigators and statisticians were all blinded to group allocation until the trial was complete.
In the DPOC group, a bolus dose of dexmedetomidine (0.6 μg kg−1) was infused for 10 min immediately after the renal artery was unclamped. The time point and length of administration, as well as the dose of dexmedetomidine, were selected according to previous studies (Tufanogullari et al., 2008; Kocoglu et al., 2009; Dahmani et al., 2010; Li et al., 2016; Balci et al., 2017; Cheng et al., 2018; Kim et al., 2018; Bunte et al., 2020), In the control group, saline was given in the same dose and according to the same protocol as the DPOC group.
Anesthesia and surgery procedure
All surgeries were completed by one surgical team (D.X.Y. and T.Z.) according to established techniques described in the literature (Haber and Gill, 2006). After the patient arrived at the operating room, routine monitoring was established, which included electrocardiogram, pulse oximetry, end-tidal carbon dioxide (PetCO2), and invasive arterial pressure. General anesthesia was induced in patients using a target-controlled infusion of propofol (AstraZeneca S.P.A., Macclesfield, United Kingdom), aiming for a plasma concentration of 3.0–3.5 μg mL−1. The following additional routine anesthetics and drugs were also given: midazolam at 0.03 mg kg−1 (Yichang Humanwell Pharmaceutical Co., Ltd., Yichang, China), sufentanil at 0.5 μg kg−1 (Yichang Humanwell Pharmaceutical Co., Ltd., Yichang, China), and rocuronium at 0.6 mg kg−1 (N.V. Organon, Oss, the Netherlands). Following tracheal intubation, patients were ventilated with an oxygen and air mixture (FiO2 = 0.4) and a PetCO2 of 35–45 mmHg. Anesthesia was maintained with intravenous remifentanil (0.2–0.3 μg kg−1 h−1) and target-controlled infusions of propofol and cisatracurium besylate (0.1 mg kg−1 min−1). During the operation, bispectral index values in all groups were maintained at 45 ± 5 by regulating the propofol infusion rate. Following closure of the peritoneum, cisatracurium besylate infusion was stopped. Propofol and remifentanil were discontinued in inpatients after wound closure. Lactated Ringer’s solution was used in both groups to maintain a stable fluid balance. When the heart rate (HR) was less than 50 beats/min, patients were treated with 0.5 mg IV atropine. When the HR was higher than 120 beats/min, patients were treated with 20 mg IV esmolol. When the mean arterial pressure (MAP) was lower than 60 mmHg, phenylephrine 40 μg was intravenously injected; when the MAP was higher than 90 mmHg, nicardipine 0.25 mg mg was intravenously delivered. Following completion of the surgery, the patients were sent to the post-anesthesia care unit for recovery. A patient-controlled intravenous analgesia device was used to alleviate postoperative pain for all patients. Sufentanil (0.04–0.06 μg kg−1 h−1) was continuously administered for 48 h. For patients who still felt pain, a bolus dose of 0.15 μg Sufenanil was self-administered as needed (no more than once every 15 min).
Collected data
Urological surgeons evaluated the tumor complexity with reference to the Preoperative Aspects and Dimensions Used for an Anatomical classification (PADUA) scoring system (Ficarra et al., 2009). To evaluate acute renal impairment, serum concentrations of neutrophil gelatinase-associated lipocalin (NGAL) and urinary concentrations of retinol-binding protein (RBP) were collected. Samples for the NGAL assay were collected intravenously at two and 6 h after surgery. These samples were then quantitatively monitored shortly after collection using the Triage NGAL assay (Alere, Inc., San Diego, CA). Urinary RBP was collected via catheterization before surgery, 24 h post-surgery, and 48 h post-surgery. The RBP was measured according to the micro-ELISA method using an automated instrument, TekTIME (BioMerieux, France). To evaluate long-term renal function, serum creatinine levels and the technetium 99mTc-diethylene triamine pentacetic acid (DTPA) GFR were measured preoperatively, 1 month post-surgery, and 6 months post-surgery. An estimated GFR (eGFR) was calculated based on the updated CKD-EPI equation: eGFR (mLmin−1.1.73 m−2) = 175× (serum creatinine) −1.154 × (age) −0.203 × 0.742 (if female) × 1.212 (if black) (Levey et al., 2009). GFR values were estimated using the renal dynamic imaging method, with intravenous injection of 99mTc-DTPA (purity >95%) produced by Jiang Yuan Pharmaceutical Factory (Jiangsu province, China), which was followed by measurement of the renal uptake ratio over time according to the Gates method. The GFRs were normalized by body surface area (1.73 m2). All surgeries were completed by one surgical team (D.X.Y. and T.Z.) in accordance with established techniques described in the literature, Perioperative adverse events were recorded.
The primary outcomes of this study were the values of 99mTc-DTPA-GFR in the affected kidney at 1 and 6 months post-LPN. The secondary outcomes were as follows: serum NGAL concentration (measured preoperatively, 2 h post-surgery, and 6 h post-surgery); urinary RBP levels (measured preoperatively, 24 h after surgery, and 48 h after surgery); and serum creatinine levels and eGFRs (measured preoperatively, 1 month after LPN, and 6 months after LPN).
Statistical analysis
Sample size calculations were based on a pilot study and historical data (Huang et al., 2013). To detect a clinically important difference between groups, 3.2 ml min−1.1.73 m−2 99mTc-DTPA GFR is sufficient, assuming an SD of 4.25 at 1 month after surgery in the affected kidney. The total sample size required is 76 when the level of significance (α) is 0.05 and the power is 0.9. Considering a dropout rate of 15% for various reasons, we estimated that 45 patients were needed in each group.
Variables were expressed as mean (SD), median with interquartile range (IQR), and percentages (%). Continuous variables were analyzed via ANOVA (for repeated measurements), student’s t-test, or Mann-Whitney U-test; moreover, categorical variables were analyzed using the Chi-square test (Prism9; GraphPad Software, San Diego, CA). p values <0.05 were considered statistically significant.
Results
A total of 90 patients were initially enrolled in this trial. Of these, six patients refused to participate, four patients experienced repeated occlusion during the surgery, and three patients were lost during the follow-up. After all patients listed above were excluded, a total of 77 patients completed this study: 38 in the control group and 39 in the DPOC group, as detailed in the CONSORT diagram in Figure 1. Adverse events included 4 cases of postoperative severe hematuria (2 patients in each group). 3 cases of postoperative peripheral hematoma (2 patients in the control group, 1 patient in the DPOC group). All the complications were cured by corresponding treatment.
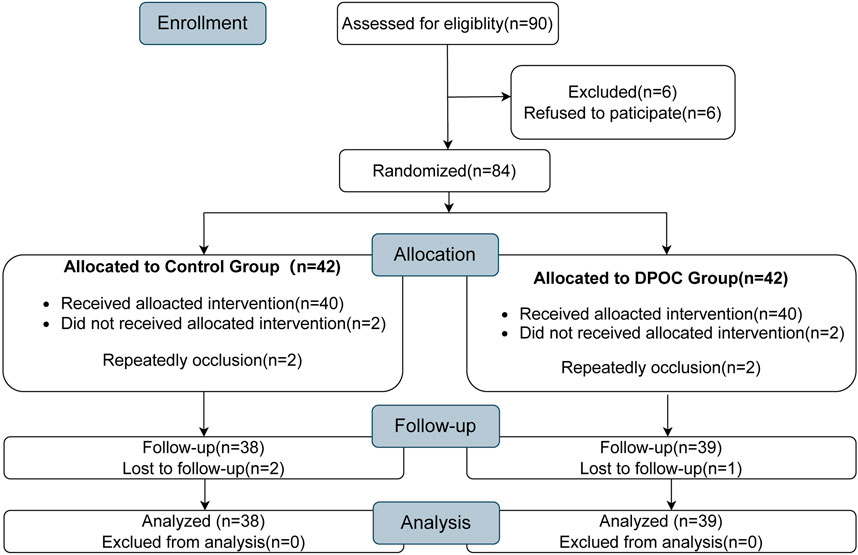
FIGURE 1. CONSORT diagram showing the flow of participants throughout each stage of the randomized trial.
The basic patient demographics and tumor characteristics of the two groups are listed in Table 1, while operative and postoperative characteristics are presented in Table 2. There were no differences in perioperative data between two groups (all p > 0.05).
There were no statistical differences between the control and DPOC groups in terms of preoperative serum NGAL concentrations. At two and 6 h after LPN, the level of serum NGAL [mean (SD)] in the control group was significantly higher than that in the DPOC group (control vs. DPOC: 228.2 ± 63.18 μg L−1 vs.160.4 ± 48.88 μg L−1 at 2 h after LPN; 247.0 ± 61.49 μg L−1 vs. 181.7 ± 55.96 μg L−1 at 6 h after LPN; p < 0.0001) (Figure 2A). LPN also triggered a notable rise in urinary RBP levels [mean (SD)] (from 0.11 ± 0.02 mg L−1 to 0.67 ± 0.15 mg L−1) at 24 h in the control group. However, this rise was less dramatic following DPOC treatment (from 0.10 ± 0.03 mg L−1 to 0.44 ± 0.17 mg L−1) at 24 h in the DPOC group; p < 0.0001 (Figure 2B). The levels of serum NGAL and urinary RBP were significantly higher after surgery in the two groups compared to the baseline (Figure 2).
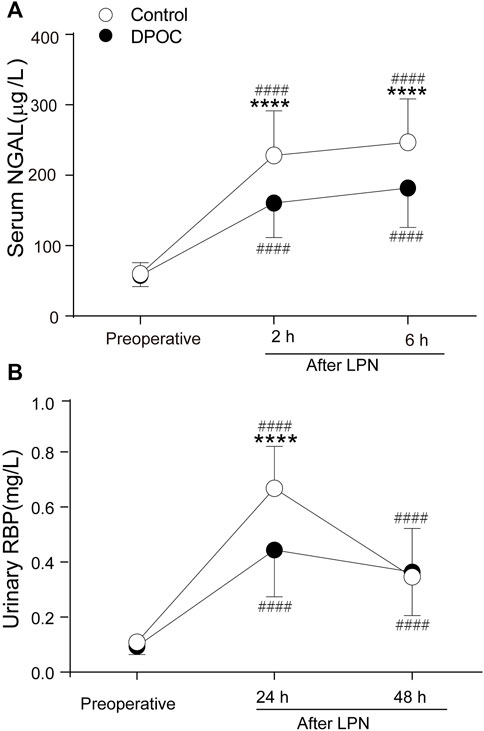
FIGURE 2. Levels of serum NGAL (A) and urinary RBP (B) before and after surgery between DPOC or control group. ****p < 0.0001 vs. control group. ####p < 0.0001 vs. Baseline. NGAL = neutrophil gelatinase-associated lipocalin; RBP = Urinary retinol-binding protein; Control = Control group; DPOC = dexmedetomidine postconditioning group.
Data regarding renal function (99mTc-DTPA GFRs), serum creatinine levels, and eGFRs-are provided in Figure 3. The 99mTc-DTPA GFR of the affected kidney in the DPOC group (35.65 ± 4.89 ml min−1 1.73 m−2) was significantly higher than that in the control group (33.10 ± 5.41 ml min−1.1.73 m−2) at 1 month after LPN, p = 0.022 (Figure 3A). The trend shows that the 99mTc-DTPA GFR of the affected kidney in the DPOC group was higher than that in the control group at 6 months after surgery, but there was no statistical difference between the two groups (p > 0.05). However, while the 99mTc-DTPA GFR value in the affected kidney at 6 months after surgery (37.85 ± 4.01 ml min−1 1.73 m−2) essentially returned to baseline (39.66 ± 4.30 ml min−1 1.73 m−2) in the DPOC group (p > 0.05), the same value at the same timepoint (36.09 ± 5.10 ml min−1 1.73 m−2) was still lower than the baseline (40.36 ± 5.28 ml min−1 1.73 m−2) in the control group (p < 0.001). No significant differences were observed in the 99mTc-DTPA GFR of the contralateral kidney and total kidney at one and 6 months after LPN (Figures 3B,C; p > 0.05). Furthermore, the serum creatinine levels and eGFRs in the control and DPOC groups did not significantly differ at various time points (Figures 3D,E; p > 0.05). To further confirm our findings, we calculated the GFR ratio for the affected kidney to total kidney (Figure 3F). This ratio was significantly higher in the DPOC group compared with the control group one and 6 months after LPN (46.27 ± 4.60% vs. 43.85 ± 5.11% at 1 month, p = 0.032; 48.59 ± 4.61% vs. 46.25 ± 5.47% at 6 months, p = 0.046). This ratio returned to baseline 6 months after LPN in the DPOC group compared to the value before operation (48.59 ± 4.61% vs. 50.87 ± 5.36% in the DPOC group, p > 0.05); however, the ratio in the control group at this timepoint was still significantly lower than pre-operation levels (46.25 ± 5.47% vs. 50.78 ± 4.89% in the control group, p < 0.001) (Figure 3F).
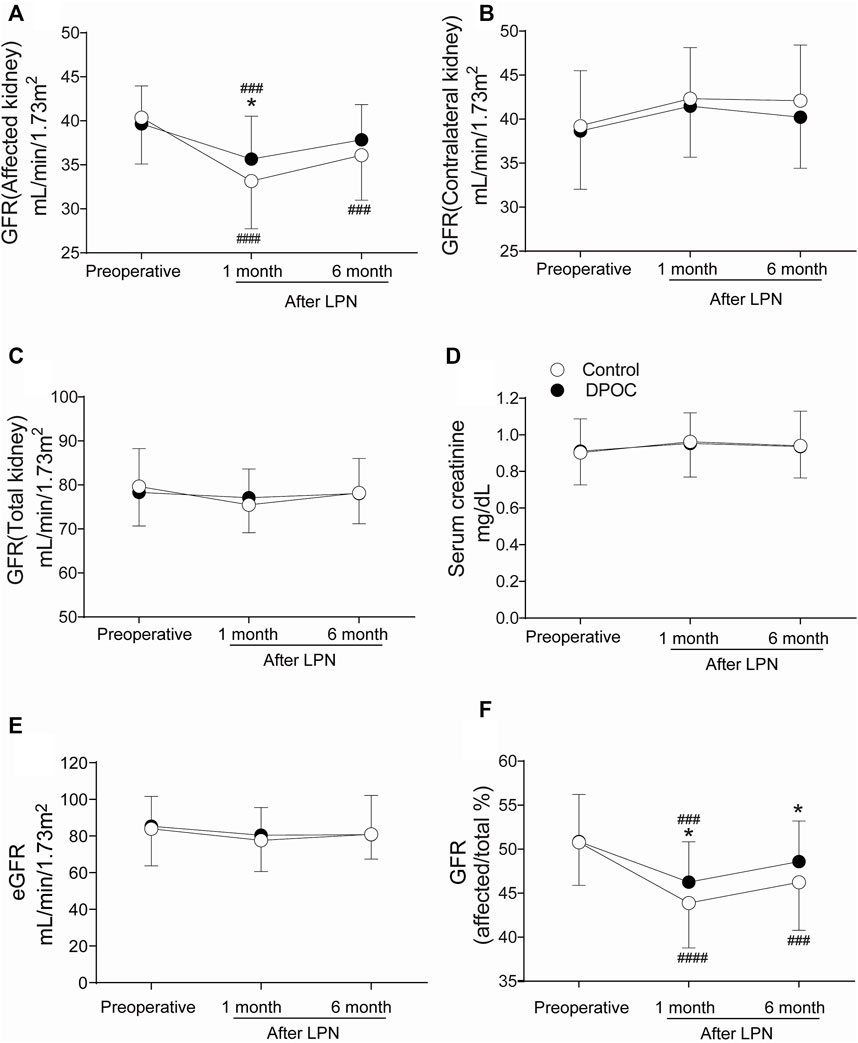
FIGURE 3. Renal function at one and 6 months after LPN between DPOC or control group. (A) GFR of the affected kidney; (B) GFR of the contralateral kidney; (C) GFR of the total kidney; (D) Serum creatinine; (E) Estimated glomerular filtration rate (eGFR); (F) Contribution change of the affected kidney to total renal function. All GFR values were evaluated by 99mTc-DTPA renal scintigraphy. *p < 0.05 vs. control group. ###p < 0.001 vs. Baseline; ####p < 0.0001 vs. Baseline. GFR = Glomerular filtration rate.
Discussion
Since Zhao et al. (2003) first reported that IPOC can ameliorate myocardial ischemia/reperfusion, IPOC has been widely deployed to protect many organs from IRI (Jiang et al., 2010; Esposito et al., 2015; Ricca et al., 2015). Jiang et al. (2010) showed that IPOC can provide renal protection against IRI. Additionally, numerous studies focused on the IPOC mechanism have identified a range of endogenous and exogenous agonists that can produce similar therapeutic effects. For instance, dexmedetomidine can mimic IPOC’s renal protective effect by activating the PI3K-Akt survival cascade to induce cytoprotectant (Gu et al., 2011). Dexmedetomidine has been widely used in recent years as an anesthetic adjuvant during surgery due to its favorable perioperative hemodynamic stability and its intraoperative anesthesia-sparing effect (Ibrahim et al., 2019). Lempiainen, et al. (2014) suggested that dexmedetomidine preconditioning (DPRC), but not DPOC, restrain renal IRI. Nevertheless, several investigations have established the ability of DPOC to ameliorate heart, brain and kidney IRI (Kocoglu et al., 2009; Dahmani et al., 2010; Gu et al., 2011; Balci et al., 2017; Cheng et al., 2018; Bunte et al., 2020; Zhang et al., 2020). Our study is the first to conduct a randomized controlled trial on the renoprotection of DPOC in patients undergoing LPN. The data from this study suggest that DPOC can slow the decline of the GFR score and decrease the serum NGAL and urinary RBP values in the affected kidney. This indicates that DPOC can reduce acute renal impairment and improve at least short-term LPN outcomes.
Plasma NGAL provides a good diagnostic biomarker for AKI development within 48 h after surgery (area under ROC 0.78, 95%; CI 0.65–0.90) (Cruz et al., 2010). Our study shows that DPOC partially suppressed the increase in NGAL at 2 h and 6 h after LPN, while NGAL was substantially increased in the control group. Urinary RBP is considered a sensitive renal proximal tubular injury diagnosis index (Adiyanti and Loho, 2012; Obermüller et al., 2014). The normal urinary RBP content is very low (≤0.1 μg min−1), with a short plasma half-life of 12 h. Our results show that DPOC can slow the growth of urinary RBP postoperatively within 24 h. These findings suggest that DPOC conserves the function of the proximal tubules and thereby reduces AKI.
At 1 month and 6 months after LPN, no differences in total renal function—as reflected by serum creatinine, eGFR, and total GFR—were found between the two groups. This may be due to the compensatory GFR activity of the contralateral kidney, which proportionally masked the reduced GFR in the affected kidney. To resolve this issue, radionuclide imaging with 99mTc-DTPA was used to assess whether DPOC can protect the affected kidney from injury. 99mTc-DTPA GFR is widely used in clinical work due to its high accuracy and convenience. As a result, The 99mTc-DTPA GFR in DPOC group is higher than control group at 1 month after surgery as 2.55 ml/min/1.73 m2 which is roughly equivalent to 7.6% of the whole 99mTc-DTPA GFR. This dramatic improvement in 99mTc-DTPA GFR for injury kidney may indicate a decrease in the risk for chronic kidney disease (CKD) and hemodialysis after discharge. However, no significant difference between the two groups was observed at 6 months after LPN. While gradual recovery of the kidney over the long-term may partially explain this result, there are also multiple factors that can influence long-term renal function, such as intraoperative blood loss (Simmons et al., 2012; Li and Liu, 2021). Nevertheless, DPOC caused a non-significant renoprotection trend, and the GFR value essentially returned to baseline at 6 months post-surgery. Furthermore, when assessing the contribution of the affected kidney to total renal function, the results showed that the contribution in the DPOC group was significantly higher than that in the control group. Overall, these findings indicate obvious renoprotection provided by DPOC, at least as assessed by short-term observations; a large-scale trial is required to evaluate the long-term effect.
While the overall mechanism by which DPOC conserves renal function is complex and unclear, the PI3K-Akt signaling pathway seems to play a critical role in DPOC against IRI (Dahmani et al., 2010; Gu et al., 2011; Cheng et al., 2018; Kim et al., 2018). PI3K-Akt is one of the survival cascades activated by alpha-2 adrenoceptors, which can decrease the expression of pro-apoptotic factors such as caspase-3 and Bax while up-regulating the expression of anti-apoptotic Bcl-2. Anti-inflammatory action is another possible mechanism by which DPOC conserves renal function during IRI. Gu et al. (2011) found that post-reperfusion treatment with dexmedetomidine can reduced plasma high-mobility group protein B1 (HMGB-1) levels after kidney ischemia/reperfusion. HMGB-1, a damage-associated molecular pattern released by dying cells during tissue ischemia, binds to toll-like receptor 4 (TLR-4), initiating a downstream NF-κB signaling cascade that promotes the synthesis of pro-inflammatory cytokine (IL-6, IL-1β, and TNF-α). Kim et al. (2018) demonstrated that dexmedetomidine reduced the elevation of the inflammasome components NLRP3, caspase-1 and IL-1β in a diabetic renal IRI model. In summary, the PI3K-Akt signaling pathway and anti-inflammatory effect may play key roles in DPOC, although the precise mechanism of DPOC needs to be further investigated.
The present study has a number of limitations. First, large-scale, multicenter clinical trials are required in order to further evaluate whether DPOC has long-term effects on renoprotection. Second, dexmedetomidine was administered as a single bolus dose over 10 min; larger doses of dexmedetomidine and longer infusion times should be tested in future to determine whether they can further improve renal function. Expanding follow-up studies to include more indicators (such as albuminuria or microalbuminuria) and additional timepoints (such as 2 to 3 months after LPN) would facilitate the construction of a more precise timeline of DPOC renoprotection. Finally, other isotopes such as 99mTc-mercaptoacetyltriglycine-3 may enhance the accuracy in quantification of the GFR (Shirasaki et al., 2005).
Conclusion
The findings of this study provide support for the theory that DPOC can alleviate AKI and provide short-term therapeutic benefits in reducing renal injury caused by LPN.
Data availability statement
The raw data supporting the conclusions of this article will be made available by the authors, without undue reservation.
Ethics statement
The studies involving human participants were reviewed and approved by This controlled trial was approved by the Ethics Committee of the second hospital of Anhui Medical University (No.LW201405). The patients/participants provided their written informed consent to participate in this study.
Author contributions
Conception and design of the research: LJ, TZ, YeZ, and DY Acquisition of data: LJ and YaZ Analysis and interpretation of the data: LJ and TZ Statistical analysis: LJ and YeZ Obtaining financing: LJ Writing of the manuscript: LJ, YaZ, and TZ Critical revision of the manuscript for intellectual content: YeZ and DY All authors read and approved the final draft.
Funding
This study was funded by the key discipline foundation of The Second Hospital of Anhui Medical University and the National Natural Science Foundation of China (No. 81200089).
Conflict of interest
The authors declare that the research was conducted in the absence of any commercial or financial relationships that could be construed as a potential conflict of interest.
Publisher’s note
All claims expressed in this article are solely those of the authors and do not necessarily represent those of their affiliated organizations, or those of the publisher, the editors and the reviewers. Any product that may be evaluated in this article, or claim that may be made by its manufacturer, is not guaranteed or endorsed by the publisher.
References
Adiyanti, S. S., and Loho, T. (2012). Acute kidney injury (AKI) biomarker. Acta Med. Indones. 44 (3), 246–255.
Balci, C., Akan, M., Boztas, N., Ozkardesler, S., Ergur, B. U., Guneli, M. E., et al. (2017). Protective effects of dexmedetomidine and remote ischemic preconditioning on renal ischemia reperfusion injury in rats. Ulus. Travma Acil Cerrahi Derg. 23 (4), 279–286.
Bunte, S., Behmenburg, F., Majewski, N., Stroethoff, M., Raupach, A., Mathes, A., et al. (2020). Characteristics of dexmedetomidine postconditioning in the field of myocardial ischemia-reperfusion injury. Anesth. Analg. 130 (1), 90–98. doi:10.1213/ANE.0000000000004417
Cheng, X., Hu, J., Wang, Y., Ye, H., Li, X., Gao, Q., et al. (2018). Effects of dexmedetomidine postconditioning on myocardial ischemia/reperfusion injury in diabetic rats: Role of the PI3K/Akt-Dependent signaling pathway. J. Diabetes Res. 2018, 3071959. doi:10.1155/2018/3071959
Cruz, D. N., de Cal, M., Garzotto, F., Perazella, M. A., Lentini, P., Corradi, V., et al. (2010). Plasma neutrophil gelatinase-associated lipocalin is an early biomarker for acute kidney injury in an adult ICU population. Intensive Care Med. 36 (3), 444–451. doi:10.1007/s00134-009-1711-1
Dahmani, S., Rouelle, D., Gressens, P., and Mantz, J. (2010). Characterization of the postconditioning effect of dexmedetomidine in mouse organotypic hippocampal slice cultures exposed to oxygen and glucose deprivation. Anesthesiology 112 (2), 373–383. doi:10.1097/ALN.0b013e3181ca6982
Esposito, E., Hayakawa, K., Maki, T., Arai, K., and Lo, E. H. (2015). Effects of postconditioning on neurogenesis and angiogenesis during the recovery phase After focal cerebral ischemia. Stroke 46 (9), 2691–2694. doi:10.1161/STROKEAHA.115.009070
Ficarra, V., Novara, G., Secco, S., Macchi, V., Porzionato, A., De Caro, R., et al. (2009). Preoperative aspects and dimensions used for an anatomical (PADUA) classification of renal tumours in patients who are candidates for nephron-sparing surgery. Eur. Urol. 56 (5), 786–793. doi:10.1016/j.eururo.2009.07.040
Gill, I. S., Kavoussi, L. R., Lane, B. R., Blute, M. L., Babineau, D., Colombo, J. R., et al. (2007). Comparison of 1,800 laparoscopic and open partial nephrectomies for single renal tumors. J. Urol. 178 (1), 41–46. doi:10.1016/j.juro.2007.03.038
Gu, J., Sun, P., Zhao, H., Watts, H. R., Sanders, R. D., Terrando, N., et al. (2011). Dexmedetomidine provides renoprotection against ischemia-reperfusion injury in mice. Crit. Care 15 (3), R153. doi:10.1186/cc10283
Haber, G. P., and Gill, I. S. (2006). Laparoscopic partial nephrectomy: Contemporary technique and outcomes. Eur. Urol. 49 (4), 660–665. doi:10.1016/j.eururo.2006.02.001
Huang, J., Chen, Y., Dong, B., Kong, W., Zhang, J., Xue, W., et al. (2013). Effect of remote ischaemic preconditioning on renal protection in patients undergoing laparoscopic partial nephrectomy: A 'blinded' randomised controlled trial. BJU Int. 112 (1), 74–80. doi:10.1111/bju.12004
Huang, W. C., Levey, A. S., Serio, A. M., Snyder, M., Vickers, A. J., Raj, G. V., et al. (2006). Chronic kidney disease after nephrectomy in patients with renal cortical tumours: A retrospective cohort study. Lancet Oncol. 7 (9), 735–740. doi:10.1016/S1470-2045(06)70803-8
Ibrahim, E., Sultan, W., Helal, S., Abo-Elwafa, H., and Abdelaziz, A. (2019). Pregabalin and dexmedetomidine conscious sedation for flexible bronchoscopy: A randomized double-blind controlled study. Minerva Anestesiol. 85 (5), 487–493. doi:10.23736/S0375-9393.18.12685-X
Jiang, B., Liu, X., Chen, H., Liu, D., Kuang, Y., Xing, B., et al. (2010). Ischemic postconditioning attenuates renal ischemic/reperfusion injury in mongrel dogs. Urology 76 (6), 1519.e1–7. doi:10.1016/j.urology.2010.06.055
Kiliç, K., Hanci, V., Selek, S., Sözmen, M., Kiliç, N., Citil, M., et al. (2012). The effects of dexmedetomidine on mesenteric arterial occlusion-associated gut ischemia and reperfusion-induced gut and kidney injury in rabbits. J. Surg. Res. 178 (1), 223–232. doi:10.1016/j.jss.2012.03.073
Kim, S. H., Jun, J. H., Oh, J. E., Shin, E. J., Oh, Y. J., and Choi, Y. S. (2018). Renoprotective effects of dexmedetomidine against ischemia-reperfusion injury in streptozotocin-induced diabetic rats. PLoS One 13 (8), e0198307. doi:10.1371/journal.pone.0198307
Kim, S. H., Kim, D. H., Shin, S., Kim, S. J., Kim, T. L., and Choi, Y. S. (2019). Effects of dexmedetomidine on inflammatory mediators after tourniquet-induced ischemia-reperfusion injury: A randomized, double-blinded, controlled study. Minerva Anestesiol. 85 (3), 279–287. doi:10.23736/S0375-9393.18.13015-X
Kocoglu, H., Ozturk, H., Ozturk, H., Yilmaz, F., and Gulcu, N. (2009). Effect of dexmedetomidine on ischemia-reperfusion injury in rat kidney: A histopathologic study. Ren. Fail 31 (1), 70–74. doi:10.1080/08860220802546487
Lane, B. R., Novick, A. C., Babineau, D., Fergany, A. F., Kaouk, J. H., and Gill, I. S. (2008). Comparison of laparoscopic and open partial nephrectomy for tumor in a solitary kidney. J. Urol. 179 (3), 847–852. doi:10.1016/j.juro.2007.10.050
Lempiäinen, J., Finckenberg, P., Mervaala, E. E., Storvik, M., Kaivola, J., Lindstedt, K., et al. (2014). Dexmedetomidine preconditioning ameliorates kidney ischemia-reperfusion injury. Pharmacol. Res. Perspect. 2 (3), e00045. doi:10.1002/prp2.45
Levey, A. S., Stevens, L. A., Schmid, C. H., Zhang, Y. L., Castro, A. F., Feldman, H. I., et al. (2009). A new equation to estimate glomerular filtration rate. Ann. Intern Med. 150 (9), 604–612. doi:10.7326/0003-4819-150-9-200905050-00006
Li, S., and Liu, Q. Q. (2021). A study on the mechanism of the protective effect of GuangeFang on sepsis-associated acute kidney injury. World J. Tradit. Chin. Med. 7, 414–418.
Li, Y., Wang, B., Zhang, L. L., He, S. F., Hu, X. W., Wong, G. T., et al. (2016). Dexmedetomidine combined with general anesthesia provides similar intraoperative stress response reduction when compared with a combined general and epidural anesthetic technique. Anesth. Analg. 122 (4), 1202–1210. doi:10.1213/ANE.0000000000001165
Liu, X., Chen, H., Zhan, B., Xing, B., Zhou, J., Zhu, H., et al. (2007). Attenuation of reperfusion injury by renal ischemic postconditioning: The role of NO. Biochem. Biophys. Res. Commun. 359 (3), 628–634. doi:10.1016/j.bbrc.2007.05.129
Obermüller, N., Geiger, H., Weipert, C., and Urbschat, A. (2014). Current developments in early diagnosis of acute kidney injury. Int. Urol. Nephrol. 46 (1), 1–7. doi:10.1007/s11255-013-0448-5
Ricca, L., Lemoine, A., Cauchy, F., Hamelin, J., Sebagh, M., Esposti, D. D., et al. (2015). Ischemic postconditioning of the liver graft in adult liver transplantation. Transplantation 99 (8), 1633–1643. doi:10.1097/TP.0000000000000685
Shirasaki, Y., Saika, T., Tsushima, T., Nasu, Y., Arata, R., and Kumon, H. (2005). Predicting postoperative renal insufficiency in patients undergoing nephrectomy for renal malignancy: Assessment by renal scintigraphy using 99mtechnetium-mercaptoacetyltriglycine. J. Urol. 173 (2), 388–390. doi:10.1097/01.ju.0000149980.61593.5f
Simmons, M. N., Hillyer, S. P., Lee, B. H., Fergany, A. F., Kaouk, J., and Campbell, S. C. (2012). Functional recovery after partial nephrectomy: Effects of volume loss and ischemic injury. J. Urol. 187 (5), 1667–1673. doi:10.1016/j.juro.2011.12.068
Tan, X., Yin, R., Chen, Y., Gao, D., and Zhang, X. (2015). Postconditioning attenuates renal ischemia-reperfusion injury by mobilization of stem cells. J. Nephrol. 28 (3), 289–298. doi:10.1007/s40620-015-0171-7
Thompson, R. H., Lane, B. R., Lohse, C. M., Leibovich, B. C., Fergany, A., Frank, I., et al. (2010). Every minute counts when the renal hilum is clamped during partial nephrectomy. Eur. Urol. 58 (3), 340–345. doi:10.1016/j.eururo.2010.05.047
Tufanogullari, B., White, P. F., Peixoto, M. P., Kianpour, D., Lacour, T., Griffin, J., et al. (2008). Dexmedetomidine infusion during laparoscopic bariatric surgery: The effect on recovery outcome variables. Anesth. Analg. 106 (6), 1741–1748. doi:10.1213/ane.0b013e318172c47c
Xue, J., Qin, Z., Li, X., Cao, P., and Jia, R. (2017). Protective effects of ischemic preconditioning-mediated homing of endothelial progenitor cells on renal acute ischemia and reperfusion injury in male rats. Ann. Transpl. 22, 66–74. doi:10.12659/aot.901738
Zhang, X., Li, Y., Wang, Y., Zhuang, Y., Ren, X., Yang, K., et al. (2020). Dexmedetomidine postconditioning suppresses myocardial ischemia/reperfusion injury by activating the SIRT1/mTOR axis. Biosci. Rep. 40 (5). doi:10.1042/BSR20194030
Zhao, Z. Q., Corvera, J. S., Halkos, M. E., Kerendi, F., Wang, N. P., Guyton, R. A., et al. (2003). Inhibition of myocardial injury by ischemic postconditioning during reperfusion: Comparison with ischemic preconditioning. Am. J. Physiol. Heart Circ. Physiol. 285 (2), H579–H588. doi:10.1152/ajpheart.01064.2002
Keywords: dexmedetomidine, ischemia postconditioning, laparoscopic partial nephrectomy, renal ischemia/reperfusion injury, renoprotection
Citation: Jiang L, Zhang T, Zhang Y, Yu D and Zhang Y (2022) Dexmedetomidine postconditioning provides renal protection in patients undergoing laparoscopic partial nephrectomy: A randomized controlled trial. Front. Pharmacol. 13:988254. doi: 10.3389/fphar.2022.988254
Received: 07 July 2022; Accepted: 12 September 2022;
Published: 04 October 2022.
Edited by:
Xue-Jun Sun, Second Military Medical University, ChinaReviewed by:
Zhou Hua, Shengjing Hospital of China Medical University, ChinaDi Xie, Southern Medical University, China
Copyright © 2022 Jiang, Zhang, Zhang, Yu and Zhang. This is an open-access article distributed under the terms of the Creative Commons Attribution License (CC BY). The use, distribution or reproduction in other forums is permitted, provided the original author(s) and the copyright owner(s) are credited and that the original publication in this journal is cited, in accordance with accepted academic practice. No use, distribution or reproduction is permitted which does not comply with these terms.
*Correspondence: Ye Zhang, emhhbmd5ZV9oYXNzYW5Ac2luYS5jb20=