- 1Razi Drug Research Center, Iran University of Medical Sciences, Tehran, Iran
- 2Neuroscience Research Center, Iran University of Medical Science, Tehran, Iran
- 3Toxicology and Diseases Group (TDG), Pharmaceutical Sciences Research Center (PSRC), Tehran University of Medical Sciences (TUMS), Tehran, Iran
- 4Pharmaceutical Sciences Research Center, Ardabil University of Medical Sciences, Ardabil, Iran
Backgrounds: Diabetes can cause diabetic neuropathy (DN), a nerve injury. High blood sugar (glucose) levels can harm nerves all over your body. The nerves in your legs and feet are the most commonly affected by DN. The purpose of this study was to conduct a review of melatonin’s potential neuroprotective properties against DN.
Method: A full systematic search was conducted in several electronic databases (Scopus, PubMed, and Web of Science) up to March 2022 under the PRISMA guidelines. Forty-seven studies were screened using predefined inclusion and exclusion criteria. Finally, the current systematic review included nine publications that met the inclusion criteria.
Result: According to in vivo findings, melatonin treatment reduces DN via inhibition of oxidative stress and inflammatory pathways. However, compared to the diabetes groups alone, melatonin treatment exhibited an anti-oxidant trend. According to other research, DN also significantly produces biochemical alterations in neuron cells/tissues. Additionally, histological alterations in neuron tissue following DN were detected.
Conclusion: Nonetheless, in the majority of cases, these diabetes-induced biochemical and histological alterations were reversed when melatonin was administered. It is worth noting that the administration of melatonin ameliorates the neuropathy caused by diabetes. Melatonin exerts these neuroprotective effects via various anti-oxidant, anti-inflammatory, and other mechanisms.
1 Introduction
Diabetic neuropathy (DN) is one of the most prevalent and debilitating complications of diabetes mellitus, severely limiting the patient’s quality of life and imposing a significant burden on the healthcare system (Leinninger et al., 2006). The global prevalence of diabetes mellitus has been steadily increasing, with an estimated 9.3% (463 million people) in 2019, expected to increase to 10.9% (700 million people) by 2045 (Saeedi et al., 2019). The DN prevalence in diabetic patients varies from 9.6 to 88.7% worldwide. This variation may be due to age, diabetes types, glucose control, disease duration, and available health facilities (Amour et al., 2019).
Despite DN’s widespread prevalence and devastating effects, there is still no treatment to prevent its progression. The current management of DN is based on three principles: intensive blood sugar control, pathogenetic treatments, symptomatic therapy, which does not possess sufficient efficacy (Hosseini and Abdollahi, 2013). Therefore, designing effective drugs against DN based on novel therapeutic strategy and targets is essential. Current experimental strategies for treating or preventing DN inhibit several metabolic pathways involved in its development, such as controlling oxidative stress and reducing chronic inflammation (Saberi Firouzi et al., 2018; Sarvestani et al., 2018; Galeshkalami et al., 2019). Therefore, administering anti-oxidants seems to be one of the most sensible treatment approaches for DN, and some studies have confirmed this hypothesis (Hosseini et al., 2010; Hosseini et al., 2011; Ghaznavi et al., 2015; Najafi et al., 2015).
Melatonin (N-acetyl-5-methoxytryptamine) is an endogenous neurohormone derived from tryptophan and produced by the pineal gland. Melatonin is a well-known powerful anti-oxidant (Nishida, 2005). It is an effective scavenger of various free radicals, and also it up-regulates the expression of anti-oxidant enzymes and down-regulates inflammatory protein expression in all cells (Rodriguez et al., 2004; Jung et al., 2010). Eliminating free radicals by melatonin is the primary mechanism for counteracting neurotoxicity caused by oxidative stress, making it a potent neuroprotective (Nishida, 2005). As reported in several literature reviews, melatonin reduces diabetic complications by neutralizing oxidative damage (Afifi, 2013; Zephy et al., 2015; Mok et al., 2019). In addition, several documents from in vitro and in vivo studies demonstrate potent neuroprotective effects of melatonin against DN (Srinivasan et al., 2006; Arreola-Espino et al., 2007; Negi et al., 2010; Zencirci et al., 2010).
In the present systematic review, we aimed to evaluate and discuss the possible protective role of melatonin against DN in vivo. In addition, it was tried to answer the following questions: (i) the underlying mechanisms of DN, (ii) the role of melatonin against DN-induced neurotoxicity, (iii) and the underlying mechanisms of the preventive role of melatonin in DN. To ultimately achieve the above aims, we conducted a comprehensive literature review to analyze the protective role of melatonin in DN.
2 Methods
A systematic search was conducted in this investigation, adhering to the Preferred Reporting Items for Systematic Reviews and Meta-Analyses (PRISMA) guideline (Moher et al., 2009). Additionally, a PICO framework was used (Moher et al., 2009). This framework includes participants (P): DN (in vitro studies) and/or patients/animals with diabetes-induced neuropathy adverse effects (clinical/in vivo studies); intervention (I): patients/animals/cells with DN; comparison (C): patients/animals/cells treated with melatonin; and outcomes (O): there were two critical outcomes: 1) changes in the neuropathy following diabetes treatment compared to control/untreated groups and 2) changes in the DN.
2.1 Search strategy
We conducted a thorough systematic search to identify all relevant works on “the role of melatonin in diabetic-induced neuropathy” in both medical subject heading (MeSH) and advance in the electronic databases Scopus, PubMed, and Web of Science up to March 2022, using the keywords of melatonin AND “Diabetic Neuropathy” OR “Diabetic Autonomic Neuropathy” OR “Diabetic Neuropathies” OR “Diabetic Autonomic Neuropathies” OR “Diabetic Neuralgias” OR “Diabetic Neuralgia” OR “Painful Diabetic Neuropathy” OR “Painful Diabetic Neuropathies” OR “Symmetric Diabetic Proximal Motor Neuropathy” OR “Asymmetric Diabetic Proximal Motor Neuropathy” OR “Diabetic Asymmetric Polyneuropathies” OR “Diabetic Asymmetric Polyneuropathy” OR “Diabetic Mononeuropathy” OR “Diabetic Mononeuropathies” OR “Diabetic Mononeuropathy Simplices” OR “Diabetic Mononeuropathy Simplex” OR “Diabetic Amyotrophy” OR “Diabetic Polyneuropathy” OR “Diabetic Amyotrophies” OR “Diabetic Polyneuropathies” in title, abstract or keywords.
2.2 Process of study selection
The inclusion criteria for this systematic review were full-text scientific papers that met the following criteria: 1) they were written in English; 2) they focused on the role of melatonin in diabetes-induced neuropathy (as defined by the keywords mentioned above); 3) they contained adequate findings; 4) there were no restrictions on publications that included clinical, in-vitro, or in-vivo studies; and 5) there were no restrictions on publication year. Additionally, this study excluded 1) hemodynamic data, 2) unrelated publications, 3) review articles, 4) case reports, 5) book chapters, 6) oral presentations, 7) letters to the editors, 8) posters, and 9) editorials.
2.3 Process of data extraction
Two researchers examined each eligible study and then extracted the following data: a) author name and year of publication, b) models (tissue) and duration, c) diabetes-induced agent (dosage) and route of administration, d) outcomes of DN impairment, e) melatonin dosage & route of administration/duration of administration, and f) melatonin administration outcomes.
3 Results
3.1 Literature search and screening
A comprehensive search of electronic databases up to March 2022 yielded 47 articles. After excluding duplicated articles (n = 11) in the first screening (based on our keywords in the title and abstract), 18 articles were discarded and 18 articles were reviewed in the second screening using our full-text criteria. Finally, nine articles were determined to be eligible for the current investigation. The approach for doing the literature search and screening is depicted in Figure 1.
The information of articles, including the name of the first author and the year of publication, models (tissue) and duration, diabetes induced agent (dosage) and route of administration, outcomes of DN impairment, melatonin dosage, and route of administration/duration of administration, melatonin administration outcomes, were extracted and collected by AH and rechecked by HHA demonstrated in Table 1.
3.2 The role of melatonin against biochemical, molecular, histological, and behavioral changes induced by diabetic neuropathy
3.2.1 Biochemical changes
In the present study, biochemical changes are markers for identifying impairments produced by DN. According to the results of this study, DN induces a significant elevation in MDA and peroxynitrite levels as oxidative stress markers and TNF-α and IL-6 levels as inflammation markers compared to the normal group. Meanwhile, melatonin reduced and normalized oxidative stress (Baydas et al., 2003a; Negi et al., 2010; Metwally et al., 2018) and inflammation markers (Negi et al., 2011) compared to the diabetic neuropathic group.
The result of the present study indicated that DN significantly reduced CAT, SOD, and GPx levels as anti-oxidant markers compared to the control group. These anti-oxidant markers were restored to normal levels with melatonin administration (Baydas et al., 2003a; Babaei et al., 2010; Metwally et al., 2018).
3.2.2 Molecular changes
Molecular changes are markers for identifying cell damages produced by DN. Based on the results of this study, DN induces a significant elevation in the expression of NF-KB, phosphorylated IKB, iNOS, and COX-2 proteins as inflammation markers compared to the standard group. Melatonin reduced the expression of NF-KB, phosphorylated IKB, iNOS, and COX-2 proteins compared to the diabetic neuropathic group (Negi et al., 2011).
The result of the present study indicated that DN significantly reduced the expression of Nrf2 and HO-1 proteins as anti-oxidant markers compared to the control group. Melatonin administration restored these anti-oxidant markers to normal levels (Negi et al., 2011).
3.2.3 Histological changes
Histological changes are markers for identifying tissue damages induced by DN. DN induced an increase in GFAP and S100B levels, IHC score, DNA damage, liver damage, neurodegeneration, degeneration of the sciatic nerve, number of apparently degenerated fibers, axonal degeneration, demyelination, abnormal myelinated fibers, as compared to the control group, while treatment with melatonin reversed back these histological changes to normal (Baydas et al., 2003a; Negi et al., 2010; Negi et al., 2011; Zangiabadi et al., 2011; Afifi, 2013; Metwally et al., 2018; Magar et al., 2020).
Moreover, the results of the research indicated that DN decreased nerve blood flow, the number of total and standard fibers, hepatic mRNA expression of PGC-1α and TFAM, versus the control group. Whereas melatonin administration increased nerve blood flow, several standard fibers, hepatic mRNA expression of PGC-1α and TFAM compared with DN group (Negi et al., 2010; Afifi, 2013; Magar et al., 2020).
3.2.4 Behavioral changes
Behavioral changes are markers for identifying behavioral disorders induced by DN. The evaluation of studies indicated that DN reduced MNCV, hyperalgesia, allodynia, escape latency, and elevated pain indices compared to the normal group. These behavioral changes were restored to normal levels with melatonin administration (Babaei et al., 2010; Negi et al., 2010; Zangiabadi et al., 2011; Seyit et al., 2016; Magar et al., 2020).
4 Discussion
This study aimed to review the harmful effects of diabetes on neuron cells/tissue. Additionally, the effects of melatonin administration on these detrimental effects were examined. It is reported melatonin is safe even in extremely high doses, tests on both humans and animals have shown that short-term usage of melatonin is quite safe. There have only been reported relatively minor adverse effects, such as lightheadedness, headaches, nausea, and tiredness. Exogenous melatonin has not been shown in any study to have the potential to cause any major adverse effects (Andersen et al., 2016; Oliveira-Abreu et al., 2021). Table 1 summarizes the data on the effects of DN alone or in combination with melatonin on neuron cells/tissue. Additionally, Figure 2 depicts some of the significant alterations in the neuron cell following diabetes and the effects of melatonin administration on these changes.
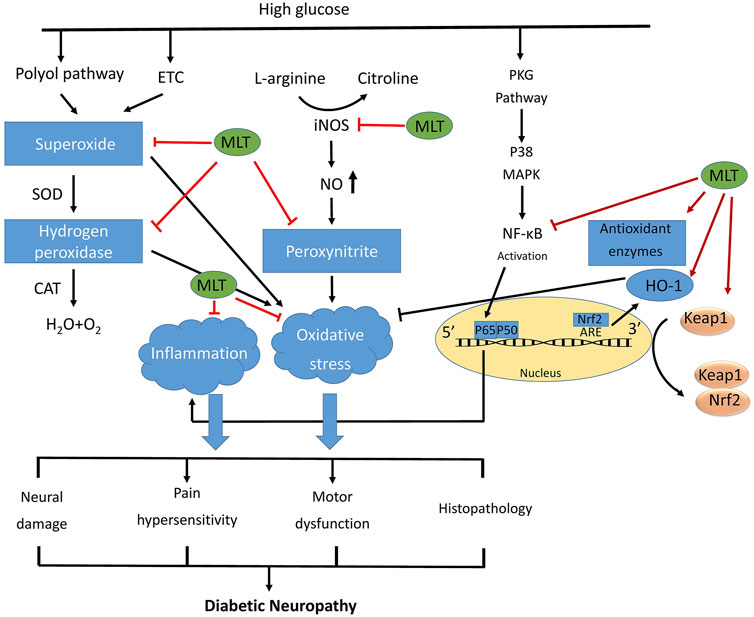
FIGURE 2. The general mechanisms of diabetic nephropathy.Schematic diagram of the protective function of melatonin against diabetic neuropathy by improving oxidative stress and inflammatory responses. Hyperglycemia activates many metabolic pathways like polyol pathway, and turnover of the mitochondrial energy-production complexes, leading to release of reactive intermediates. High glucose condition activates also PKC pathway, which lead to NF-kB activation. Melatonin inhibits reactive intermediates and NF-kB, and activates Nrf2, and as well as up regulates proteins expression of antioxidant enzymes, resulting in inhibits the oxidative stress and inflammation, and ultimately alleviating the development of diabetic neuropathy. ETC: electron transport chain; PKC: protein kinase c.
Usually, free radicals are created in cells, and their defensive mechanisms neutralize them (Mortezaee et al., 2021). Notably, free radicals increase under oxidative stress due to an imbalance between free radicals and these defensive systems. According to literatures, diabetes raises reactive oxygen species (ROS) in neuron cells/tissue. The ROS assault the cell’s macromolecules, causing the neuron cells/tissue to malfunction (Shiri et al., 2016; Rahimifard et al., 2020).
Oxidative stress is initiated by an increase in free radicals such as superoxide (O2−), hydroxyl (OH−), singlet oxygen (1O2), and extra secondary ROS and a reduction in anti-oxidant agent defense (Tipoe et al., 2007). Increased free oxygen radicals could damage cellular carbohydrates, proteins, lipids, and nucleic acids, ultimately resulting in illness (Mathew et al., 2011). It has been demonstrated that diabetes-induced oxidative stress contributes to neuron dysfunction on a molecular level (Chung et al., 2003). Some ROS with a short half-life combines to form more strong free radicals with a long half-life that are more hazardous, including Hydrogen peroxide (H2O2), HOCl, and OCl− (Boukhenouna et al., 2018). Additionally, H2O2 can create OH− by the Fenton reaction, which is a highly reactive and poisonous compound (Lyngsie et al., 2018). Anti-oxidant enzymes (SOD, catalase, and GPx) protect tissues and cells from oxidative stress-induced damage by neutralizing free radicals (Rao et al., 2011). Thus, more ROS is produced when anti-oxidant enzymes are depleted. These reactions occasionally result in other hazardous reactive species such as OH− radicals (Beneš et al., 1999; Baeeri et al., 2018). ROS is the rate-limiting step in creating LPO, and so its creation is shown by an increase in the quantities of MDA and other thiobarbituric acid reactive compounds (TBARs) (Princ et al., 1998). Thus, ROS reacts with cell components, impairing cell function (Newsholme et al., 2016). Melatonin is a well-known powerful anti-oxidant with multiple properties that block oxygen-free radicals. Melatonin both directly and indirectly decreases oxidative tissue damage. Melatonin has been demonstrated to act as a direct anti-oxidant, scavenging oxygen free radicals (Najafi et al., 2020a). As a result, it may help to reduce LPO and MDA. Additionally, melatonin has been shown to boost the activity of anti-oxidant enzymes such as SOD, catalase, and GPx by elevation anti-oxidant defenses (Tomás‐Zapico and AJJopr, 2005).
The superoxide dismutase (SOD) enzyme is the body’s first line of defense against superoxide (Ighodaro and OJAjom, 2018). SOD converts O2− free radicals to H2O2, and oxygen molecule, which is then eliminated by catalase, and GPx (Kurutas, 2016).
Catalase is the enzyme that converts H2O2 to H2O and O2 (Aksoy et al., 2005). GSH is a tripeptide critical for the cell’s protection against free radicals (Forman et al., 2009). GSH reacts with oxygen free radicals, decreasing their concentrations; in this case, neuronal GSH levels drop during oxidative stress due to its oxidation to glutathione disulfide (GSSG) (Dringen et al., 2000). Additionally, the glutathione reductase (GR) enzyme reduces GSSG to GSH by the oxidation of NADPH to NADP+ (Yang et al., 2006). Moreover, diabetes induced DNA damage in a neuron is ameliorated by melatonin (Negi et al., 2011).
The importance of oxidative stress in the pathogenesis of several neurodegenerative illnesses has been well recognized. It has been proved that increasing HO-1 via genetic or pharmacological modification confers stress tolerance and neuroprotection (Calabrese et al., 2008).
The protective function has been demonstrated to be due to the manufacture of HO-1 activity’s end products, as shown by the use of ferulic acid, which increases bilirubin and CO production in response to -amyloid-induced oxidative stress (Joshi et al., 2006). According to other research, CO suppresses AMP-activated protein kinase (AMPK) activity, which has been associated with -amyloid-induced damage. Experiments on animal models have revealed that HO-1 protects neurons against oxidative damage (Wen et al., 2020; Engin, 2021).
In eukaryotic organisms, the nuclear factor erythroid 2-related factor 2 (Nrf2) is a transcription factor that regulates cellular redox equilibrium (Dai et al., 2020). It plays a serious function in phase II detoxification enzymes and stress proteins, via modifying the expression of genes, containing anti-oxidant/electrophile response elements (Kim et al., 2010). Given this crucial cytoprotective regulatory role for genes and proteins, the Nrf2/HO-1 axis in neuroprotection is considered as the focus research in recent years under normal and pathological conditions (Espada et al., 2010). It is also involved in neural resistance to oxidative stress and glutamate-induced excitotoxicity (Brasil et al., 2021). It is known that astrocytes protect neurons from damage by regulating Nrf2 signaling, but little is known about how astrocytes regulate neuronal Nrf2 signaling (Habas et al., 2013). This study shows that increased neuronal activity triggers the release of soluble substances that activate the astrocytic Nrf2 pathway via group I metabotropic glutamate receptors and intracellular Ca2+ (Ishii et al., 2019). The neuron–astrocyte tripartite synapse regulates endogenous anti-oxidant signaling by matching astrocyte neuroprotective capacity to neighboring neuronal synaptic activity (Sorrentino et al., 2019). The results show that DN reduces HO-1 and NRF2 expression, which melatonin reverses (Negi et al., 2011). The possible mechanism is mediated through the anti-oxidant role of melatonin.
GFAP and S100B are either trophic or poisonous in high quantities (Rothermundt et al., 2003). Overproduction of neuronal and glial markers results in neuronal and glial death, while low levels operate as neurotrophic factors, which may be significant during growth and nerve redevelopment (Mirsky et al., 2008). When untreated diabetes with extremely high glucose levels persists, tissue damage is noticed, ascribed to oxidative injury caused by forming free radicals (Giacco and Brownlee, 2010). One of the effects of this type of damage is a depleted supply of the cellular anti-oxidant GSH. Diabetes may result in glial sensitivity due to a weakened defense system against free radicals and increased glutamatergic activity (Uttara et al., 2009). Astrocytes’ increased susceptibility to oxidative stress due to diabetes increases their vulnerability to harm. Injuries to neurons and glia increase GFAP and S100B production (Baydas et al., 2005). Consistent with the current findings, we recently proposed that glial reactivity was intimately tied to the oxidative environment (Andersen, 2004). These free radicals damage macromolecules within the neuron and glial cells, resulting in protein structural and functional alterations (Kumar et al., 2012). GFAP and S100B are produced by glial cells in response to oxidative stress (Baydas et al., 2003b). The present study demonstrated that GFAP, and S100B levels elevated in DN and melatonin through its polytrophic effect reduced GFAP and S100B levels (Baydas et al., 2003b).
The inflammation pathway is a biological reaction to potentially damaging motivations involving various signaling pathways (Chen et al., 2017). Neutrophil cells are the primary inflammatory. Increased pro-inflammatory molecules such as chemokines, cytokines, and others worsen this reaction (Shanmugam et al., 2010). Previous research has established that neuron damage caused by diabetes is associated with inflammation (Muriach et al., 2014). NF-κB is a critical component of the inflammatory response (Liu et al., 2017). Stimulating the transcription factor IκB, NF-κB activates the genes encoding pro-inflammatory cytokines, including TNF-α, and improves the deadly effects of DN (Negi et al., 2011; Najafi et al., 2020b). Additionally, these DN promote the expression of chemokines and pro-inflammatory cytokines, for example, IL-1, IL-6, and TNF-α (Negi et al., 2011). These variables influence the extent to which neutrophils infiltrate the damaged location (Puhl and Steffens, 2019). Melatonin may have anti-inflammatory properties, as evidenced by numerous research (Farez et al., 2016). Possible anti-inflammatory processes include decreasing free radicals, activating NF-κB, down-regulation of chemotaxis, and pro-inflammatory cytokines (IL-1, IL-6, and TNF-α) of inflammation cells (Sarkar and Fisher, 2006).
Sleep patterns are linked to diabetes through metabolic syndrome and insulin resistance, and the link between disrupted sleep and diabetes is likely driven by a lack in melatonin (Wajid et al., 2020). Insulin resistance and metabolic syndrome are both associated to obesity (Anderson et al., 2001). In point of fact, people with type 2 diabetes who slept less had higher 24-h urine protein and albumin excretion levels, which are indications of more severe DN (Xiang et al., 2016). In addition, diabetes-related hyperglycemia causes a decrease in the production of melatonin, which makes it more difficult to sleep and exacerbates metabolic medical disorders (Espino et al., 2011).
The expression of the MT1 and MT2 receptors has been found in the pancreatic tissue of both humans and rodents (Nagorny et al., 2011). According to some publications, the MT1 receptor is more prevalent in alpha cells, whilst the MT2 receptor is only seen in beta cells. Even though an identical level of transcript for each was reported in alpha and beta cells, high-throughput investigation of mRNA abundance using RNA sequencing has established the presence of MT1 and MT2 in human islets (Ramracheya et al., 2008; Forrestel et al., 2017). Melatonin is responsible for regulating a wide variety of cellular processes and gene expression within the islets of the pancreas (Mulder et al., 2009). Nearly simultaneously with the finding that this hormone had “insulin-like” activity came the realization that melatonin has the ability to induce sleep through its sedative properties. In subsequent research, the effects of melatonin on glucose homeostasis were investigated and characterized (Garaulet et al., 2020; Abdulwahab et al., 2021).
In general, based on the non-clinical findings of this study, melatonin, through various mechanisms such as oxidative stress and inflammation by affecting different molecules, including MDA, GPx, CAT, SOD, TNF-α, IL-6, NF-kB, IkB, iNOS, COX-2, Nrf-2, and HO-1 leads to the reduction of neuropathy damage induced by diabetes and the pathological damage. It should be noted that these results are from non-clinical studies and for clinical studies, more studies on different models and more paths are needed.
5 Conclusion
In conclusion, this study demonstrates that neuropathy is a type of harm caused by diabetes. Diabetes causes neuropathy by generating toxic free reactive species, initiating inflammatory pathways, and causing histological injury. Melatonin administration during DN reduces the risk of neuropathy by reducing oxidative stress, inhibiting the inflammatory process, and reducing histological damage. As a result of the current study’s findings, treatment with melatonin may result in a more tolerable neuropathy for patients.
Data availability statement
The original contributions presented in the study are included in the article/Supplementary Material; further inquiries can be directed to the corresponding authors.
Author contributions
AH: Conceptualization; Data curation; Roles/Writing—original draft; Writing—review and editing. MS: Roles/Writing—original draft; Writing—review and editing. MB: Roles/Writing—original draft; Writing—review and editing. MR: Supervision Roles/Writing—original draft; Writing—review and editing. HH-A: Conceptualization; Investigation; Methodology; Supervision Roles/Writing—original draft; Writing—review and editing.
Acknowledgments
Authors wish to thank IUMS, TUMS, and ARUMS for providing the full text of the articles needed.
Conflict of interest
The authors declare that the research was conducted in the absence of any commercial or financial relationships that could be construed as a potential conflict of interest.
Publisher’s note
All claims expressed in this article are solely those of the authors and do not necessarily represent those of their affiliated organizations, or those of the publisher, the editors and the reviewers. Any product that may be evaluated in this article, or claim that may be made by its manufacturer, is not guaranteed or endorsed by the publisher.
References
Abdulwahab, D. A., El-Missiry, M. A., Shabana, S., Othman, A. I., and Amer, M. E. J. H. (2021). Melatonin protects the heart and pancreas by improving glucose homeostasis, oxidative stress, inflammation and apoptosis in T2DM-induced rats. Heliyon 7 (3), e06474. doi:10.1016/j.heliyon.2021.e06474
Afifi, N. M (2013). Neuroprotective effect of melatonin in a rat model of streptozotocin-induced diabetic neuropathy: Light and electr*on microscopic study. Egypt. J. Histology 36 (2), 321–335. doi:10.1097/01.ehx.0000428963.44300.63
Aksoy, Y., Balk, M., and Öğüş, H. (2005). The mechanism of inhibition of human erythrocyte catalase by azide. Turkish J. Biol. 28 (2-4), 65–70.
Amour, A. A., Chamba, N., Kayandabila, J., Lyaruu, I. A., Marieke, D., Shao, E. R., et al. (2019). Prevalence, patterns, and factors associated with peripheral neuropathies among diabetic patients at tertiary hospital in the kilimanjaro region: Descriptive cross-sectional study from north-eastern Tanzania. Int. J. Endocrinol. 2019, 5404781. doi:10.1155/2019/5404781
Andersen, L. P. H., Gögenur, I., and Rosenberg, J. (2016). The safety of melatonin in humans. Clin. Drug Investig. 36 (3), 169–175. doi:10.1007/s40261-015-0368-5
Andersen, J. K. J. (2004). Oxidative stress in neurodegeneration: Cause or consequence? Nat. Med. 10 (7), S18–S25. doi:10.1038/nrn1434
Anderson, P., Critchley, J., Chan, J., Cockram, C., Lee, Z., Thomas, G., et al. (2001). Factor analysis of the metabolic syndrome: Obesity vs insulin resistance as the central abnormality. Int. J. Obes. Relat. Metab. Disord. 25 (12), 1782–1788. doi:10.1038/sj.ijo.0801837
Arreola-Espino, R., Urquiza-Marín, H., Ambriz-Tututi, M., Araiza-Saldaña, C. I., Caram-Salas, N. L., Rocha-González, H. I., et al. (2007). Melatonin reduces formalin-induced nociception and tactile allodynia in diabetic rats. Eur. J. Pharmacol. 577 (1-3), 203–210. doi:10.1016/j.ejphar.2007.09.006
Babaei, B. F., Zare, S., Heydari, R., and Farokhi, F. (2010). Effects of melatonin and vitamin E on peripheral neuropathic pain in streptozotocin-induced diabetic rats. Iran. J. Basic Med. Sci.
Baeeri, M., Mohammadi-Nejad, S., Rahimifard, M., Navaei-Nigjeh, M., Moeini-Nodeh, S., Khorasani, R., et al. (2018). Molecular and biochemical evidence on the protective role of ellagic acid and silybin against oxidative stress-induced cellular aging. Mol. Cell. Biochem. 441 (1-2), 21–33. doi:10.1007/s11010-017-3172-0
Baydas, G., Reiter, R. J., Yasar, A., Tuzcu, M., Akdemir, I., and Nedzvetskii, V. S. (2003). Melatonin reduces glial reactivity in the hippocampus, cortex, and cerebellum of streptozotocin-induced diabetic rats. Free Radic. Biol. Med. 35 (7), 797–804. doi:10.1016/s0891-5849(03)00408-8
Baydas, G., Nedzvetskii, V. S., Tuzcu, M., and Yasar, A. (2003). Increase of glial fibrillary acidic protein and S-100B in hippocampus and cortex of diabetic rats: Effects of vitamin E. Eur. J. Pharmacol. 462 (1-3), 67–71. doi:10.1016/s0014-2999(03)01294-9
Baydas, G., Sonkaya, E., Tuzcu, M., Yasar, A., and Donder, E. J. A. P. S. (2005). Novel role for gabapentin in neuroprotection of central nervous system in streptozotocine-induced diabetic rats. Acta Pharmacol. Sin. 26 (4), 417–422. doi:10.1111/j.1745-7254.2005.00072.x
Beneš, L., Ďuračková, Z., and MJLs, F. (1999). Chemistry, physiology and pathology of free radicals. Life Sci. 65 (18-19), 1865–1874. doi:10.1016/s0024-3205(99)00439-7
Boukhenouna, S., Wilson, M. A., Bahmed, K., and Kosmider, B. (2018). Reactive oxygen species in chronic obstructive pulmonary disease. Oxid. Med. Cell. Longev. 2018, 5730395. doi:10.1155/2018/5730395
Brasil, F. B., de Almeida, F. J. S., Luckachaki, M. D., Dall'Oglio, E. L., and de Oliveira MRJEJoP, (2021). Astaxanthin prevents mitochondrial impairment in the dopaminergic SH-SY5Y cell line exposed to glutamate-mediated excitotoxicity: Role for the Nrf2/HO-1/CO-BR axis. Eur. J. Pharmacol. 908, 174336. doi:10.1016/j.ejphar.2021.174336
Calabrese, V., Cornelius, C., Mancuso, C., Pennisi, G., Calafato, S., Bellia, F., et al. (2008). Cellular stress response: A novel target for chemoprevention and nutritional neuroprotection in aging, neurodegenerative disorders and longevity. Neurochem. Res. 33 (12), 2444–2471. doi:10.1007/s11064-008-9775-9
Chen, L., Deng, H., Cui, H., Fang, J., Zuo, Z., Deng, J., et al. (2017). Inflammatory responses and inflammation-associated diseases in organs. Oncotarget 9 (6), 7204–7218. doi:10.18632/oncotarget.23208
Chung, S. S., Ho, E. C., Lam, K. S., and Chung, S. K. J. (2003). Contribution of polyol pathway to diabetes-induced oxidative stress. J. Am. Soc. Nephrol. 14, S233–S236. doi:10.1097/01.asn.0000077408.15865.06
Dai, X., Yan, X., Wintergerst, K. A., Cai, L., Keller, B. B., and Tan, Y. J. T. (2020). Nrf2: Redox and metabolic regulator of stem cell state and function. Trends Mol. Med. 26 (2), 185–200. doi:10.1016/j.molmed.2019.09.007
Dringen, R., Gutterer, J. M., and JJEjob, Hirrlinger (2000). Glutathione metabolism in brain: Metabolic interaction between astrocytes and neurons in the defense against reactive oxygen species. Eur. J. Biochem. 267 (16), 4912–4916. doi:10.1046/j.1432-1327.2000.01597.x
Engin, A. (2021). Protein kinase-mediated decision between the life and death. Protein kinase-mediated decisions between life and death. Springer, 1–33.
Espada, S., Ortega, F., Molina-Jijón, E., Rojo, A. I., Pérez-Sen, R., Pedraza-Chaverri, J., et al. (2010). The purinergic P2Y13 receptor activates the Nrf2/HO-1 axis and protects against oxidative stress-induced neuronal death. Free Radic. Biol. Med. 49 (3), 416–426. doi:10.1016/j.freeradbiomed.2010.04.031
Espino, J., Pariente, J. A., and ABJWjod, Rodríguez (2011). Role of melatonin on diabetes-related metabolic disorders. World J. Diabetes 2 (6), 82–91. doi:10.4239/wjd.v2.i6.82
Farez, M. F., Calandri, I. L., Correale, J., and Quintana, F. J. J. B. (2016). Anti‐inflammatory effects of melatonin in multiple sclerosis. BioEssays 38 (10), 1016–1026. doi:10.1002/bies.201600018
Forman, H. J., Zhang, H., and Rinna, A. (2009). Glutathione: Overview of its protective roles, measurement, and biosynthesis. Mol. Asp. Med. 30 (1-2), 1–12. doi:10.1016/j.mam.2008.08.006
Forrestel, A. C., Miedlich, S. U., Yurcheshen, M., Wittlin, S. D., and Sellix, M. T. J. D. (2017). Chronomedicine and type 2 diabetes: Shining some light on melatonin. Diabetologia 60 (5), 808–822. doi:10.1007/s00125-016-4175-1
Galeshkalami, N. S., Abdollahi, M., Najafi, R., Baeeri, M., Jamshidzade, A., Falak, R., et al. (2019). Alpha-lipoic acid and coenzyme Q10 combination ameliorates experimental diabetic neuropathy by modulating oxidative stress and apoptosis. Life Sci. 216, 101–110. doi:10.1016/j.lfs.2018.10.055
Garaulet, M., Qian, J., Florez, J. C., Arendt, J., Saxena, R., FAJTiE, Scheer, et al. (2020). Melatonin effects on glucose metabolism: Time to unlock the controversy. Trends Endocrinol. Metab. 31 (3), 192–204. doi:10.1016/j.tem.2019.11.011
Ghaznavi, H., Najafi, R., Mehrzadi, S., Hosseini, A., Tekyemaroof, N., Shakeri-Zadeh, A., et al. (2015). Neuro-protective effects of cerium and yttrium oxide nanoparticles on high glucose-induced oxidative stress and apoptosis in undifferentiated PC12 cells. Neurol. Res. 37 (7), 624–632. doi:10.1179/1743132815Y.0000000037
Giacco, F., and Brownlee, M. (2010). Oxidative stress and diabetic complications. Circ. Res. 107 (9), 1058–1070. doi:10.1161/CIRCRESAHA.110.223545
Habas, A., Hahn, J., Wang, X., and MJPotNAoS, Margeta (2013). Neuronal activity regulates astrocytic Nrf2 signaling. Proc. Natl. Acad. Sci. U. S. A. 110 (45), 18291–18296. doi:10.1073/pnas.1208764110
Hosseini, A., and Abdollahi, M. J. Om (2013). Longevity c. Diabetic neuropathy and oxidative stress: therapeutic perspectives. in Oxidative medicine and cellular longevity, 2013.
Hosseini, A., Sharifzadeh, M., Rezayat, S. M., Hassanzadeh, G., Hassani, S., Baeeri, M., et al. (2010). Benefit of magnesium-25 carrying porphyrin-fullerene nanoparticles in experimental diabetic neuropathy. Int. J. Nanomedicine 5, 517–523. doi:10.2147/ijn.s11643
Hosseini, A., Abdollahi, M., Hassanzadeh, G., Rezayat, M., Hassani, S., Pourkhalili, N., et al. (2011). Protective effect of magnesium‐25 carrying porphyrin‐fullerene nanoparticles on degeneration of dorsal root ganglion neurons and motor function in experimental diabetic neuropathy. Basic Clin. Pharmacol. Toxicol. 109 (5), 381–386. doi:10.1111/j.1742-7843.2011.00741.x
Ighodaro, O., and OJAjom, Akinloye (2018). First line defence antioxidants-superoxide dismutase (SOD), catalase (CAT) and glutathione peroxidase (GPX): Their fundamental role in the entire antioxidant defence grid. Alexandria J. Med. 54 (4), 287–293. doi:10.1016/j.ajme.2017.09.001
Ishii, T., Warabi, E., and Mann, G. E. J. F. R. B. (2019). Circadian control of BDNF-mediated Nrf2 activation in astrocytes protects dopaminergic neurons from ferroptosis. Free Radic. Biol. Med. 133, 169–178. doi:10.1016/j.freeradbiomed.2018.09.002
Joshi, G., Perluigi, M., Sultana, R., Agrippino, R., Calabrese, V., and DAJNi, Butterfield (2006). In vivo protection of synaptosomes by ferulic acid ethyl ester (FAEE) from oxidative stress mediated by 2, 2-azobis (2-amidino-propane) dihydrochloride (AAPH) or Fe2+/H2O2: Insight into mechanisms of neuroprotection and relevance to oxidative stress-related neurodegenerative disorders. Neurochem. Int. 48 (4), 318–327. doi:10.1016/j.neuint.2005.11.006
Jung, K. H., Hong, S. W., Zheng, H. M., Lee, H. S., Lee, H., Lee, D. H., et al. (2010). Melatonin ameliorates cerulein‐induced pancreatitis by the modulation of nuclear erythroid 2‐related factor 2 and nuclear factor‐kappaB in rats. J. Pineal Res. 48 (3), 239–250. doi:10.1111/j.1600-079X.2010.00748.x
Kim, J., Cha, Y-N., Surh, Y-J. J. M. R. F., and MMo, Mutagenesis (2010). A protective role of nuclear factor-erythroid 2-related factor-2 (Nrf2) in inflammatory disorders. Mutat. Res. 690 (1-2), 12–23. doi:10.1016/j.mrfmmm.2009.09.007
Kumar, H., Lim, H-W., More, S. V., Kim, B-W., Koppula, S., Kim, I. S., et al. (2012). The role of free radicals in the aging brain and Parkinson's disease: Convergence and parallelism. Int. J. Mol. Sci. 13 (8), 10478–10504. doi:10.3390/ijms130810478
Kurutas, E. B. (2016). The importance of antioxidants which play the role in cellular response against oxidative/nitrosative stress: Current state. Nutr. J. 15 (1), 71. doi:10.1186/s12937-016-0186-5
Leinninger, G. M., Edwards, J. L., Lipshaw, M. J., and Feldman, E. (2006). Mechanisms of disease: Mitochondria as new therapeutic targets in diabetic neuropathy. Nat. Clin. Pract. Neurol. 2 (11), 620–628. doi:10.1038/ncpneuro0320
Liu, T., Zhang, L., Joo, D., and Sun, S-C. (2017). NF-κB signaling in inflammation. Signal Transduct. Target. Ther. 2, 17023. doi:10.1038/sigtrans.2017.23
Lyngsie, G., Krumina, L., Tunlid, A., and Persson, P. J. Sr (2018). Generation of hydroxyl radicals from reactions between a dimethoxyhydroquinone and iron oxide nanoparticles. Sci. Rep. 8 (1), 10834–10839. doi:10.1038/s41598-018-29075-5
Magar, A., Devasani, K., Majumdar, A. J. E., and Science, M. (2020). Melatonin ameliorates neuropathy in diabetic rats by abating mitochondrial dysfunction and metabolic derangements. Endocr. Metabolic Sci. 1 (3-4), 100067. doi:10.1016/j.endmts.2020.100067
Mathew, B. B., Tiwari, A., and Jatawa, S. K. J. JoP. R. (2011). Free radicals and antioxidants: A review. J. Pharm. Res. 4 (12), 4340.
Metwally, M. M. M., Ebraheim, L. L. M., and Galal, A. A. A. (2018). Potential therapeutic role of melatonin on STZ-induced diabetic central neuropathy: A biochemical, histopathological, immunohistochemical and ultrastructural study. Acta Histochem. 120 (8), 828–836. doi:10.1016/j.acthis.2018.09.008
Mirsky, R., Woodhoo, A., Parkinson, D. B., Arthur‐Farraj, P., Bhaskaran, A., and Jessen, K. R. J. (2008). Novel signals controlling embryonic Schwann cell development, myelination and dedifferentiation. J. Peripher. Nerv. Syst. 13 (2), 122–135. doi:10.1111/j.1529-8027.2008.00168.x
Moher, D., Liberati, A., Tetzlaff, J., and Altman, D. G. (2009). Preferred reporting items for systematic reviews and meta-analyses: The PRISMA statement. Ann. Intern. Med. 151 (4), 264w64–9. doi:10.7326/0003-4819-151-4-200908180-00135
Mok, J. X., Ooi, J. H., Ng, K. Y., Koh, R. Y., SMJHmb, Chye, and investigation, c. (2019). A new prospective on the role of melatonin in diabetes and its complications. Horm. Mol. Biol. Clin. Investig. 40 (1). doi:10.1515/hmbci-2019-0036
Mortezaee, K., Narmani, A., Salehi, M., Bagheri, H., Farhood, B., Haghi-Aminjan, H., et al. (2021). Synergic effects of nanoparticles-mediated hyperthermia in radiotherapy/chemotherapy of cancer. Life Sci. 269, 119020. doi:10.1016/j.lfs.2021.119020
Mulder, H., Nagorny, C., Lyssenko, V., and Groop, L. J. D. (2009). Melatonin receptors in pancreatic islets: Good morning to a novel type 2 diabetes gene. Diabetologia 52 (7), 1240–1249. doi:10.1007/s00125-009-1359-y
Muriach, M., Flores-Bellver, M., Romero, F. J., and Barcia, J. M. (2014). Diabetes and the brain: Oxidative stress, inflammation, and autophagy. Oxid. Med. Cell. Longev. 2014, 102158. doi:10.1155/2014/102158
Nagorny, C. L., Sathanoori, R., Voss, U., Mulder, H., and NJJopr, Wierup (2011). Distribution of melatonin receptors in murine pancreatic islets. J. Pineal Res. 50 (4), 412–417. doi:10.1111/j.1600-079X.2011.00859.x
Najafi, R., Sharifi, A. M., and Hosseini, A. J. Mbd (2015). Protective effects of alpha lipoic acid on high glucose-induced neurotoxicity in PC12 cells. Metab. Brain Dis. 30 (3), 731–738. doi:10.1007/s11011-014-9625-1
Najafi, M., Hooshangi Shayesteh, M. R., Mortezaee, K., Farhood, B., and Haghi-Aminjan, H. (2020). The role of melatonin on doxorubicin-induced cardiotoxicity: A systematic review. Life Sci. 241, 117173. doi:10.1016/j.lfs.2019.117173
Najafi, M., Mortezaee, K., Rahimifard, M., Farhood, B., and Haghi-Aminjan, H. (2020). The role of curcumin/curcuminoids during gastric cancer chemotherapy: A systematic review of non-clinical study. Life Sci. 257, 118051. doi:10.1016/j.lfs.2020.118051
Negi, G., Kumar, A., Kaundal, R. K., Gulati, A., and Sharma, S. S. (2010). Functional and biochemical evidence indicating beneficial effect of Melatonin and Nicotinamide alone and in combination in experimental diabetic neuropathy. Neuropharmacology 58 (3), 585–592. doi:10.1016/j.neuropharm.2009.11.018
Negi, G., Kumar, A., and Sharma, S. S. J. Jopr (2011). Melatonin modulates neuroinflammation and oxidative stress in experimental diabetic neuropathy: Effects on NF‐κB and Nrf2 cascades. J. Pineal Res. 50 (2), 124–131. doi:10.1111/j.1600-079X.2010.00821.x
Newsholme, P., Cruzat, V. F., Keane, K. N., Carlessi, R., and de Bittencourt, (2016). Molecular mechanisms of ROS production and oxidative stress in diabetes. Biochem. J. 473 (24), 4527–4550. doi:10.1042/BCJ20160503C
Nishida, S. (2005). Metabolic effects of melatonin on oxidative stress and diabetes mellitus. Endocrine 27 (2), 131–136. doi:10.1385/endo:27:2:131
Oliveira-Abreu, K., Cipolla-Neto, J., and Leal-Cardoso, J. H. J. I. JoM. S. (2021). Effects of melatonin on diabetic neuropathy and retinopathy. Int. J. Mol. Sci. 23 (1), 100. doi:10.3390/ijms23010100
Princ, F. G., Juknat, A. A., Amitrano, A. A., and Batlle, A. J. G. P. T. V. S. (1998). Effect of reactive oxygen species promoted by δ-aminolevulinic acid on porphyrin biosynthesis and glucose uptake in rat cerebellum. Gen. Pharmacol. 31 (1), 143–148. doi:10.1016/s0306-3623(97)00388-1
Puhl, S-L., and Steffens, S. (2019). Neutrophils in post-myocardial infarction inflammation: Damage vs. Resolution? Front. Cardiovasc. Med. 6, 25. doi:10.3389/fcvm.2019.00025
Rahimifard, M., Baeeri, M., Bahadar, H., Moini-Nodeh, S., Khalid, M., Haghi-Aminjan, H., et al. (2020). Therapeutic effects of gallic acid in regulating senescence and diabetes; an in vitro study. Molecules 25 (24), 5875. doi:10.3390/molecules25245875
Ramracheya, R. D., Muller, D. S., Squires, P. E., Brereton, H., Sugden, D., Huang, G. C., et al. (2008). Function and expression of melatonin receptors on human pancreatic islets. J. Pineal Res. 44 (3), 273–279. doi:10.1111/j.1600-079X.2007.00523.x
Rao, P. S., Kalva, S., Yerramilli, A., and SJFr, Mamidi (2011). Free radicals and tissue damage: Role of antioxidants. Free radicals antioxidants 1 (4), 2–7. doi:10.5530/ax.2011.4.2
Rodriguez, C., Mayo, J. C., Sainz, R. M., Antolín, I., Herrera, F., Martín, V., et al. (2004). Regulation of antioxidant enzymes: A significant role for melatonin. J. Pineal Res. 36 (1), 1–9. doi:10.1046/j.1600-079x.2003.00092.x
Rothermundt, M., Peters, M., Prehn, J. H., and VJMr, Arolt (2003). S100B in brain damage and neurodegeneration. Microsc. Res. Tech. 60 (6), 614–632. doi:10.1002/jemt.10303
Saberi Firouzi, S., Namazi Sarvestani, N., Bakhtiarian, A., Ghazi Khansari, M., Karimi, M. Y., Ranjbar, A., et al. (2018). Sildenafil protective effects on high glucose-induced neurotoxicity in PC12 cells: The role of oxidative stress, apoptosis, and inflammation pathways in an in vitro cellular model for diabetic neuropathy. Neurol. Res. 40 (8), 624–636. doi:10.1080/01616412.2018.1458813
Saeedi, P., Petersohn, I., Salpea, P., Malanda, B., Karuranga, S., Unwin, N., et al. (2019). Global and regional diabetes prevalence estimates for 2019 and projections for 2030 and 2045: Results from the international diabetes federation diabetes atlas, 9th edition. Diabetes Res. Clin. Pract. 157, 107843. doi:10.1016/j.diabres.2019.107843
Sarkar, D., and Fisher, P. B. J. Cl (2006). Molecular mechanisms of aging-associated inflammation. Cancer Lett. 236 (1), 13–23. doi:10.1016/j.canlet.2005.04.009
Sarvestani, N. N., Firouzi, S. S., Falak, R., Karimi, M. Y., Gholami, M. D., Rangbar, A., et al. (2018). Phosphodiesterase 4 and 7 inhibitors produce protective effects against high glucose-induced neurotoxicity in PC12 cells via modulation of the oxidative stress, apoptosis and inflammation pathways. Metab. Brain Dis. 33 (4), 1293–1306. doi:10.1007/s11011-018-0241-3
Seyit, D. A., Degirmenci, E., and Oguzhanoglu, A. (2016). Evaluation of electrophysiological effects of melatonin and alpha lipoic acid in rats with streptozotocine induced diabetic neuropathy. Exp. Clin. Endocrinol. Diabetes 124 (5), 300–306. doi:10.1055/s-0042-103750
Shanmugam, M. K., Bhatia, M. J. I., and Targets, A-D. (2010). The role of pro-inflammatory molecules and pharmacological agents in acute pancreatitis and sepsis. Inflamm. Allergy Drug Targets 9 (1), 20–31. doi:10.2174/187152810791292881
Shiri, M., Navaei-Nigjeh, M., Baeeri, M., Rahimifard, M., Mahboudi, H., Shahverdi, A. R., et al. (2016). Blockage of both the extrinsic and intrinsic pathways of diazinon-induced apoptosis in PaTu cells by magnesium oxide and selenium nanoparticles. Int. J. Nanomedicine 11, 6239–6250. doi:10.2147/IJN.S119680
Sorrentino, Z. A., Giasson, B. I., and PJAn, Chakrabarty (2019). α-Synuclein and astrocytes: Tracing the pathways from homeostasis to neurodegeneration in lewy body disease. Acta Neuropathol. 138 (1), 1–21. doi:10.1007/s00401-019-01977-2
Srinivasan, V., Pandi-Perumal, S., Cardinali, D., Poeggeler, B., Hardeland, R. J. B., and Functions, B. (2006). Melatonin in Alzheimer's disease and other neurodegenerative disorders. Behav. Brain Funct. 2 (1), 15–23. doi:10.1186/1744-9081-2-15
Tipoe, G. L., Leung, T-M., Hung, M-W., Fung, M-L. J. C., and Targets, H. D-D. (2007). Green tea polyphenols as an anti-oxidant and anti-inflammatory agent for cardiovascular protection. Cardiovasc. Hematol. Disord. Drug Targets 7 (2), 135–144. doi:10.2174/187152907780830905
Tomás‐Zapico, C., and AJJopr, C-M. (2005). A proposed mechanism to explain the stimulatory effect of melatonin on antioxidative enzymes. J. Pineal Res. 39 (2), 99–104. doi:10.1111/j.1600-079X.2005.00248.x
Uttara, B., Singh, A. V., Zamboni, P., and Mahajan, R. T. (2009). Oxidative stress and neurodegenerative diseases: A review of upstream and downstream antioxidant therapeutic options. Curr. Neuropharmacol. 7 (1), 65–74. doi:10.2174/157015909787602823
Wajid, F., Poolacherla, R., Mim, F. K., Bangash, A., Rutkofsky, I. H. J. JoD., and Disorders, M. (2020). Therapeutic potential of melatonin as a chronobiotic and cytoprotective agent in diabetes mellitus. J. Diabetes Metab. Disord. 19 (2), 1797–1825. doi:10.1007/s40200-020-00585-2
Wen, C., Huang, C., Yang, M., Fan, C., Li, Q., Zhao, J., et al. (2020). The secretion from bone marrow Mesenchymal stem cells pretreated with Berberine rescues neurons with oxidative damage through activation of the Keap1-Nrf2-HO-1 signaling pathway. Neurotox. Res. 38 (1), 59–73. doi:10.1007/s12640-020-00178-0
Xiang, L., Jiang, P., Zhou, L., Sun, X., Bi, J., Cui, L., et al. (2016). Additive effect of qidan dihuang grain, a traditional Chinese medicine, and angiotensin receptor blockers on albuminuria levels in patients with diabetic nephropathy: A randomized, parallel-controlled trial.
Yang, M., Chan, H., and Yu, L. J. T. (2006). Glutathione peroxidase and glutathione reductase activities are partially responsible for determining the susceptibility of cells to oxidative stress. Toxicology 226 (2-3), 126–130. doi:10.1016/j.tox.2006.06.008
Zangiabadi, N., Sheibani, V., Asadi-Shekaari, M., Shabani, M., Jafari, M., Asadi, A. R., et al. (2011). Effects of melatonin in prevention of neuropathy in STZ-induced diabetic rats. Am. J. Pharmacol. Toxicol. 6 (2), 59–67. doi:10.3844/ajptsp.2011.59.67
Zencirci, S. G., Bilgin, M. D., and HJJonm, Y. (2010). Electrophysiological and theoretical analysis of melatonin in peripheral nerve crush injury. J. Neurosci. Methods 191 (2), 277–282. doi:10.1016/j.jneumeth.2010.07.008
Keywords: diabetic neuropathy, melatonin, oxidative stress, inflammation, systemic review
Citation: Hosseini A, Samadi M, Baeeri M, Rahimifard M and Haghi-Aminjan H (2022) The neuroprotective effects of melatonin against diabetic neuropathy: A systematic review of non-clinical studies. Front. Pharmacol. 13:984499. doi: 10.3389/fphar.2022.984499
Received: 02 July 2022; Accepted: 05 August 2022;
Published: 31 August 2022.
Edited by:
Sevgi Gezici, University of Gaziantep, TurkeyReviewed by:
Oluwafemi Adeleke Ojo, Bowen University, NigeriaYaswanth Kuthati, Cathay General Hospital, Taiwan
Copyright © 2022 Hosseini, Samadi, Baeeri, Rahimifard and Haghi-Aminjan. This is an open-access article distributed under the terms of the Creative Commons Attribution License (CC BY). The use, distribution or reproduction in other forums is permitted, provided the original author(s) and the copyright owner(s) are credited and that the original publication in this journal is cited, in accordance with accepted academic practice. No use, distribution or reproduction is permitted which does not comply with these terms.
*Correspondence: Mahban Rahimifard, bWFoYmFuLnJhaGltaWZhcmRAZ21haWwuY29t; Hamed Haghi-Aminjan, aGFtZWRoYWdoaS5hQGdtYWlsLmNvbQ==