- Student Research Committee, Faculty of Medicine, Shahed University, Tehran, Iran
Breast cancer has recently been known as the first lethal malignancy in women worldwide. Despite the existing treatments that have improved the patients’ prognosis, some types of breast cancer are serious challenges to treat. Therefore, efforts are underway to provide more efficient therapy. Cryptotanshinone (CPT) is a liposoluble diterpenoid derivation of a traditional Chinese herbal medicine called Salvia miltiorrhiza Bunge. It has been considered in the past decades due to its vast therapeutic properties, including anti-tumor, anti-inflammatory, and anti-fibrosis. Recently, studies have found that CPT showed a significant anti-breast cancer effect in vivo and in vitro through different physiological and immunological mechanisms. This study summarized the latest research findings on the antitumor effect of CPT in breast cancer. Further, the main molecular mechanisms based on breast cancer types and combination with other drugs were reviewed to provide essential evidence for future longitudinal research and its clinical application in breast cancer treatment.
Introduction
Breast cancer (BRCA) is one of women’s most commonly diagnosed malignancies worldwide. The incidence of BRCA has increased gradually in recent years, becoming the top rank in 2021. (Ma and Jemal, 2013; DeSantis et al., 2014; Harbeck and Gnant, 2017; Sung et al., 2021). Based on evaluation of different biomarkers, including presence of hormone receptors (HR) [such as estrogen receptor (ER)] and overexpression of human epidermal growth factor receptor 2 (HER2), BRCA is divided into four main molecular subtypes: HR+/HER2-, HR+/HER2+, HER2+ and triple negative (TNBC) (Loibl et al., 2021). HR positive BRCA is less malignant than other subtypes. Although there are various BRCA risk factors related to lifestyle (Brody et al., 2007; Kaiser, 2013), medical condition (Anothaisintawee et al., 2013), carcinogenic genes (Gage et al., 2012), etc., it is well known that estrogen and ERs play a pivotal role in the initiation, development, and progression of breast cancer (Platet et al., 2004; Yager and Davidson, 2006). Three types of ER have been identified in BRCA cells, ERα and ERβ, and a G-protein coupled estrogen receptor (GPER) (Girgert et al., 2019).
The successful medical treatments for breast cancer, including surgery, chemotherapy, and radiation therapy, have been associated with better prognosis, causing a dramatic increase in the survival rate of BRCA patients (Abderrahman and Jordan, 2018). However, in some subtypes of BRCA the prognosis is poor (Tong et al., 2018). Success in controlling the progression and treatment of breast cancer with chemotherapy drugs such as tamoxifen and anti-estrogens depends on the presence of ERs, especially ERα. Thus, the treatment of ERα-negative BRCA, accounting for 40% of BRCAs, is challenging. A subgroup of ERα- breast cancer overexpress the HER2, and there are few drugs for its treatment. Another group is TNBC which is so malignant to be treated, resulting in a poor prognosis (Tong et al., 2018; Girgert et al., 2019). Also, treatment obstacles, such as multidrug resistance (MDR), decrease the clinical efficacy of treatment in BRCA patients (Merikhian et al., 2017).
Therefore, more studies have been conducted to explore an effective therapeutic agent to improve the prognosis of different subtypes of BRCA, especially by targeting estrogen signaling (Li et al., 2015). Herbal products are a treasure for pharmaceutical development, providing novel biological and natural compounds to develop new medications (Cragg and Newman, 2009). These drugs are known as valuable and safe resources in the treatment of various diseases due to their low price, low adverse effects, and high public availability (Balaña-Fouce et al., 1998).
Cryptotanshinone (CPT) is a liposoluble diterpenoid derivation, that mainly exists in plants of the genus Salvia, including Salvia przewalskii Maxim, Salvia tebesana Bunge., S. miltiorrhiza Bunge., among which S. miltiorrhiza Bunge, well-known as Danshen, has rich contents of diterpenes (Wu et al., 2020). Recently, CPT has been considered due to its vast range of therapeutic properties, including anti-tumor effects (Jiang et al., 2017), anti-inflammatory (Tang et al., 2011), anti-fibrosis, etc. (Wu et al., 2020). For anti-tumor activity, CPT inhibited the growth of various kinds of tumor cells, including lung cancer (Lee et al., 2008), prostate cancer (Shin et al., 2009), cervical cancer (Ye et al., 2010), leukemia (Kim et al., 2011), and breast cancer (Park et al., 2012). CPT, besides its cytotoxic effect, could prevent cancer cell proliferation and increase anti-tumor immunity simultaneously (Han et al., 2019). Therefore, this study summarized the latest research findings on the anti-breast cancer activity of CPT. Furthermore, the main molecular mechanisms based on breast cancer subtypes and combination with other drugs were reviewed to provide essential evidence for future longitudinal research and possible CPT clinical application in breast cancer treatment.
Results
The crucial characteristic of an ideal anti-tumor drug is the fewest cytotoxic effect on normal cells while the most cytotoxicity on cancer cells. Studies have demonstrated that CPT has such an anti-tumor effect (Zhang et al., 2018). Regarding anti-breast cancer treatment, CPT could affect different breast cancer cell lines through various mechanisms (Table 1). Zhou et al. (2020) showed that CPT has dose-dependent cytotoxicity on ERα-positive BRCA cells (MCF-7 cells) and ERα-negative BRCA cells (MDA-MB-231), decreasing the survival and proliferation of cancerous cells. Li et al. (2021) showed that CPT in any concentration inhibits the rate of proliferation time/concentration-dependent in MCF-7 and MDA-MB-231 BRCA cells. Also, their experiments on transwell invasion and cell migration demonstrated that MCF-7 cells are more sensitive to CPT than MDA-MB-231 cells. CPT inhibited the invasive ability of BRCA cells in a dose-dependent manner. At the same concentration of CPT, the migration distance of MCF-7 cells is lesser than MDA-MB-231 cells; additionally, increasing the concentration of CPT results in more potent inhibition in the cells migration.
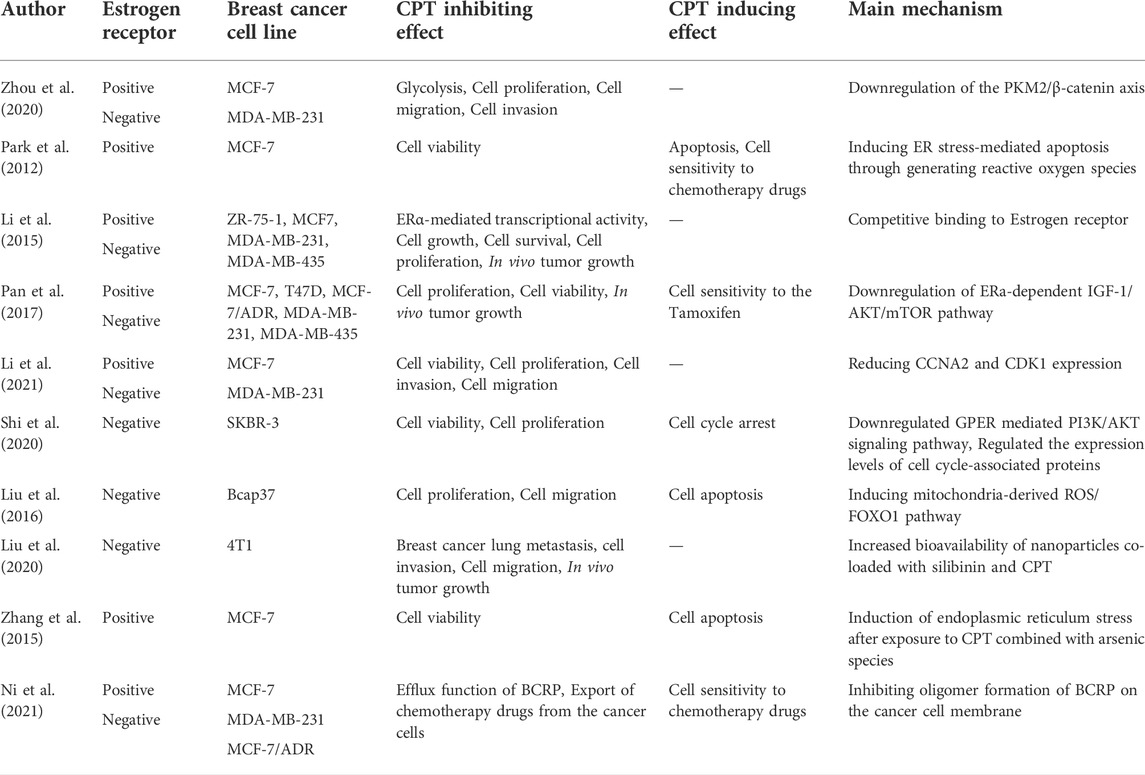
TABLE 1. The anti-breast cancer effects of CPT in estrogen-receptor dependent or independent manner.
Cryptotanshinone against estrogen receptor alpha-positive breast cancer cells (MCF-7 cell line)
ERα is a ligand-regulated transcription factor that binds to the estrogen hormone and activates a pathway, which triggers the transcription of ER target genes via binding to the estrogen-responsive elements (EREs) on their gene promoters (Klinge, 2001; Osborne et al., 2001; Deroo and Korach, 2006). Around 70%–75% of BRCAs express ERα in their cells, known as estrogen receptor alpha-positive breast cancer (ERα-positive BRCA) (Cleator et al., 2009; Johnston, 2010) Cryptotanshinone, a homogeneous chemical structure with estrogen, inhibited cell viability and proliferation in ERα-positive cells more effectively than in ERα-negative cells in a dose-dependent manner (Gong et al., 2012; Li et al., 2015; Pan et al., 2017; Li et al., 2021).
Pan et al. (2017) demonstrated that the CPT-ERα binding affinity is close to estrogen and roughly half of the Tamoxifen, presenting the anti-estrogen potential of CPT. Therefore, CPT could inhibit cell survival, growth, invasion, and migration of ERα-positive BRCA (MCF-7) cells via different mechanisms through competitive binding to ERα. CPT inhibited the ERα-mediated IGF-1/AKT/mTOR signaling and suppressed the IRS-1/AKT cascade. Thus, CPT inhibited the AKT-mTOR cascade in MCF-7 BRCA cells. Figure 1 demonstrates the regulatory effect of CPT on ERα-positive BRCA cell survival and proliferation.
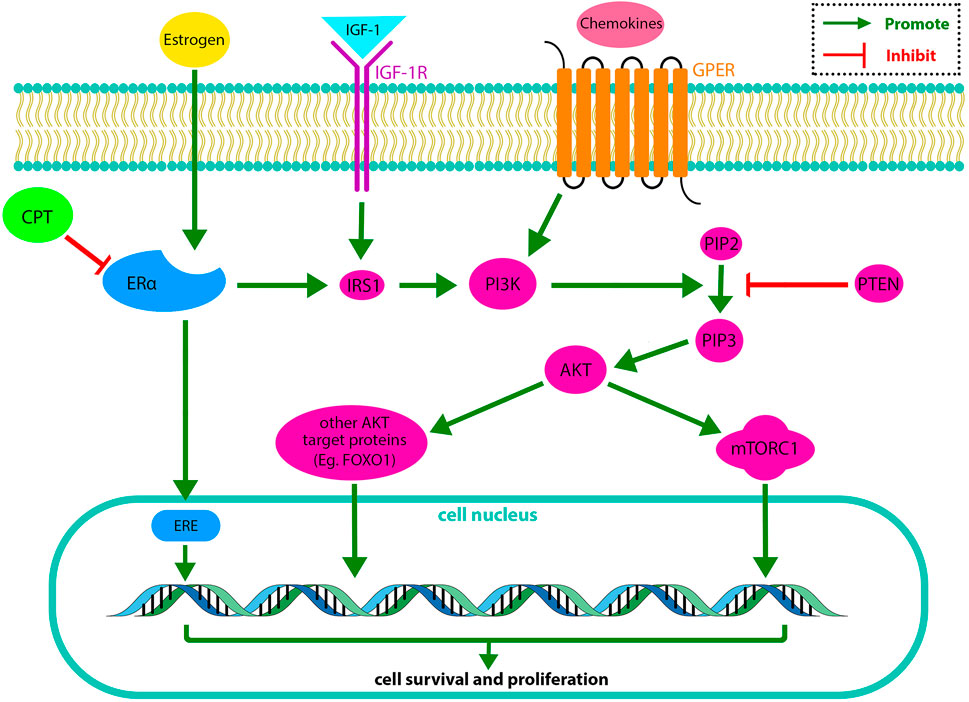
FIGURE 1. In the PI3K/AKT/mTOR pathway, IGF-1 activates IGF-1R, and some cytokines activate GPER in the cell membrane. IGF-1R and GPER activate PI3K. The activated PI3K has a catalytic effect on the phosphorylation of PIP2 to produce PIP3. PIP3 drives AKT. The activated AKT phosphorylates target proteins in the cytoplasmic fluid and cell nucleus. Finally, phosphorylation of AKT target proteins regulates cell survival and replication. On the other hand, PTEN (a PIP3 phosphatase) changes PIP3 to PIP2, suppressing the PI3K/AKT/mTOR pathway. The ERα is another receptor, activating PI3K through stimulating IRS1. CPT exerts its inhibitory effect on the PI3K/AKT/mTOR pathway by inhibiting ERα (Miricescu et al., 2020). IGF-1, insulin-like growth factor 1; IGF-1R, insulin-like growth factor receptor 1; GPER, G protein-coupled receptor; IRS1, Insulin receptor substrate 1; PI3K, Phosphatidylinositol 3-Kinase; PIP2, Phosphatidylinositol (4,5)-bisphosphate; PIP3, phosphatidylinositol (3,4,5)-trisphosphate; PTEN, phosphatase and tensin homolog; AKT, serine/threonine protein kinase; mTORC, mammalian Target of Rapamycin complex; FOXO1, Forkhead box other 1; ERE, estrogen-responsive element.
Another study by Li et al. (2015) indicated that though CPT slightly downregulated ERα expression levels, CPT-ERα competitive binding is more pivotal than downregulating ERα protein expression. CPT could significantly inhibit the viability and proliferation of breast cancer cells due to the reduction of ERα target genes transcription via competitive binding to ERα protein. Thus, it has more efficacy in the treatment of ERα-positive rather than ERα-negative BRCA.
To find out other anti-cancer mechanisms of CPT, the differentially expressed genes (DEGs) were identified on both ERα-positive and -negative BRCA cell lines (Li et al., 2021). The results suggested three main DEGs. The Estrogen Receptor Gene (ESR1), the Cyclin-Dependent Kinase 1 (CDK1), and CCNA2. CPT intervention decreased the expression of CCNA2 and CDK1 in both cell lines, predominantly in ERα-positive BRCA cells, while no changes were observed in the ESR1 gene expression in either of the two cell lines (Pagano et al., 1992; Stein and Yang, 1995; Wang et al., 2011; Li et al., 2021).
Pharmacological interventions, which can induce prolonged endoplasmic reticulum stress (ER-stress), has been recently suggested as a possible method for tumor therapy (Healy et al., 2009). Park et al. (2012) realized that CPT induces ER-stress markers by generating reactive oxygen species (ROS). Further, the apoptosis biochemical markers (the phosphorylation level of eIF2a and protein levels of CHOP, GRP94, and GRP78), increase of sub-G1 DNA, and induction of DNA fragmentation were found in the MCF-7 cells exposed to CPT. All suggested that CPT as a natural compound induces ER-stress -mediated apoptosis in MCF-7 breast cancer cells.
Zhou et al. (2014) investigated the novel anti-tumor therapeutic role of CPT. They showed that CPT could perform its anticancer effect by stimulating the immune system, through CD4+ T cells by promoting secretion of IFN-γ or perforin. CPT acts like IL-12 and causes the release of perforin from CD4+ T cells through the phosphorylation of the JAK2/STAT4 pathway, mainly inhibited the growth of breast cancer cells.
Cryptotanshinone against estrogen receptor alpha-negative breast cancer cells (MDA-MB 231, SKBR-3, Bcap37 cell lines)
ERα-negative breast cancer treatment is a big challenge due to its poor prognosis. Previous studies demonstrated the ERα-negative breast cancer resistance against anticancer drugs (Lappano et al., 2014; Bhat et al., 2015). Thus, finding an effective treatment for ERα-negative BRCA is crucial. Some subgroups of ERα-negative BRCA cells, such as SKBR-3 cells, are membrane G protein-coupled estrogen receptor (GPER) positive (Steiman et al., 2013). In vitro research claimed that GPER might function as a tumor suppressor in BRCA cells (Ariazi et al., 2010; Weißenborn et al., 2014). Recent studies revealed that GPER and its mediated signaling pathway [phosphatidylinositide 3-kinase (PI3K)/AKT] have a vital role in the proliferation of BRCA cells (Molina et al., 2017; Hsu et al., 2019).
It has been demonstrated that CPT treatment significantly downregulated the GPER-mediated PI3K/AKT signaling pathway of the ERα-negative human breast cancer cells, SKBR-3, in a dose and time-dependent manner. CPT might arrest the cell cycle associated with GPER-mediated G1-phase block. In addition, the expression of cyclin and CDK, which modulate the cell cycle regulation, obviously decreased after CPT treatment in a dose-dependent manner (Shi et al., 2020).
Bcap37 cells, as an ERα-negative BRCA cell line, have more migration and invasion than ERα-positive BRCA cells. CPT can potentially be an apoptosis inducer, anti-proliferative, and tumor-migration inhibitor drug in the ERα-negative BRCA cell lines. Liu et al. (2016) indicated that CPT could inhibit the proliferation and migration of Bcap37 cells and could induce apoptotic pathways in a dose- and time-dependent manner by arresting the cell cycle at the S phase during interphase. The main responsible for the cytotoxic effects of CPT in the ERα-negative BRCA cells is the inhibitory effect on FOXO1 (Thannickal and Fanburg, 2000; Akasaki et al., 2014).
Cryptotanshinone in combination with other drugs
Breast cancer metastasis is a complex condition in which the tumor microenvironment plays an important role. Therefore, modulation of the tumor microenvironment through various biochemical pathways can have an anti-metastatic effect (Gao et al., 2009). Silibinin (SLB) is an herbal product that constrains tumor angiogenesis and reduces epithelial-mesenchymal transition (Deep and Agarwal, 2010; Deep and Agarwal, 2013). By modulating the tumor microenvironment via different pathways, SLB, as well as CPT, are known as anti-metastatic natural products.
Liu et al. (2020) assessed the bioavailability and anti-metastatic efficacy of oral nanoparticles for administrating the SLB and CPT lung metastasis in a 4T1 breast cancer tumor-bearing nude mouse model. They demonstrated that Silibinin- and cryptotanshinone-co-loaded nanoparticles (S/C-W-LPNs) significantly induced cell toxicity compared to SLB-co-loaded nanoparticles (S-W-LPNs) or CPT-co-loaded nanoparticles (C-W-LPNs) alone. Further, in vitro anti-metastasis study showed that S/C-W-LPNs markedly inhibited cell invasion and migration; with a relative cellular migration rate of 8.6% ± 1.38% which was less than those for C-W-LPNs and S-W-LPNs (15.5% ± 3.58%, 19.9% ± 3.35%, respectively).
Arsenic trioxide (As2O3) is known as a successful treatment for acute promyelocytic leukemia worldwide (Zhu et al., 1997). Zhang et al. (2015) explored a new therapeutic method for the treatment of ER-positive breast cancer. They exposed the MCF-7 BRCA cell line to three arsenic species, namely inorganic arsenite (iAsIII), its intermediate metabolites monomethylarsonous acid (MMAIII), and dimethylarsinous acid (DMAIII) either alone or in combination with CPT and investigated their anti-breast cancer effects. The findings suggested that the combination of MMAIII with CPT has a remarkable synergic cytotoxic effect on cell viability. Further, they reported that MMAIII with CPT induces cellular apoptosis significantly (apoptosis rates up to 40%) compared to the combination of iAsIII or DMAIII with CPT, through changing the proapoptotic proteins Bax, Bak, and cyt c in the cytoplasm and mitochondria of BRCA cells (Zhang et al., 2015).
Cryptotanshinone and conventional therapies
Recent significant progression in the cancerous cells’ drug resistance is a prominent obstacle for clinicians during chemotherapy. One of the solutions is using compounds that can synergize with conventional chemotherapy drugs. Park et al. (2012) evaluated the alone and the synchronic cytotoxic effects of CPT and chemotherapy drugs such as 5-FU, TNFα, etoposide, and cisplatin. They observed that lonely exposure to CPT or each antitumor drug has minimal effect on MCF-7 BRCA cells’ viability and has not any noticeable induction of ER-stress or apoptotic markers. On the other hand, synchronic use of CPT and antitumor drugs showed a prominent antitumor synergism with the promotion of apoptotic markers, indicating that CPT exerts its synergistic effect through potentiation of the apoptotic activity of different antitumor drugs via the stimulation of ER-stress (Park et al., 2012).
A study of treating C57 mice with cancerous MCF-7 cells with CPT or Taxol revealed that CPT remarkably inhibited the cancerous cells’ growth from day 13 compared to non-treatment mice. However, the therapeutic effect of CPT was minimally less than Taxol. But their findings suggested that CPT, along with conventional chemotherapy drugs, could have a synergic effect on breast cancer treatment (Zhou et al., 2014).
Cryptotanshinone and multi-drug resistant breast cancer
Multi-drug resistance (MDR) in breast tumors is a condition that reduces the efficacy of chemotherapy drugs (Wang et al., 2017b). Often it occurs following long-term anti-estrogen chemotherapy and ERα-negative breast cancers. MDR has a tight association with breast cancer resistance protein (BCRP). BCRP is a membrane protein that causes efflux of chemotherapy drugs from tumor cells, therefore making cancer cells less affected by chemotherapy drugs (Mao and Unadkat, 2015; Li et al., 2016).
Thus, Ni et al. (2021) stated that BCRP might have a vital role in regulating the CPT transportation across the breast cancer cells membrane. They found that although CPT could not affect the intracellular protein and mRNA levels of BCRP/ABCG2, but inhibited the efflux function of BCRP in MCF-7 cells by reducing the BCRP expression on the cell membrane, which was ERα-dependent (Figure 2). BCRP is primarily of dimer and oligomer formation on the MCF-7 cell membrane. To find whether CPT was synergistic with BCRP-mediated efflux of anticancer drugs or not, they investigated the effect of CPT along with two of the most common BCRP drugs, MX and TOPO, compared with treatment with MX or TOPO alone. The findings showed that CPT increased the efficacy of chemotherapy drugs that can be effluxed by BCRP from tumor cells, reversing MDR (Ni et al., 2021).
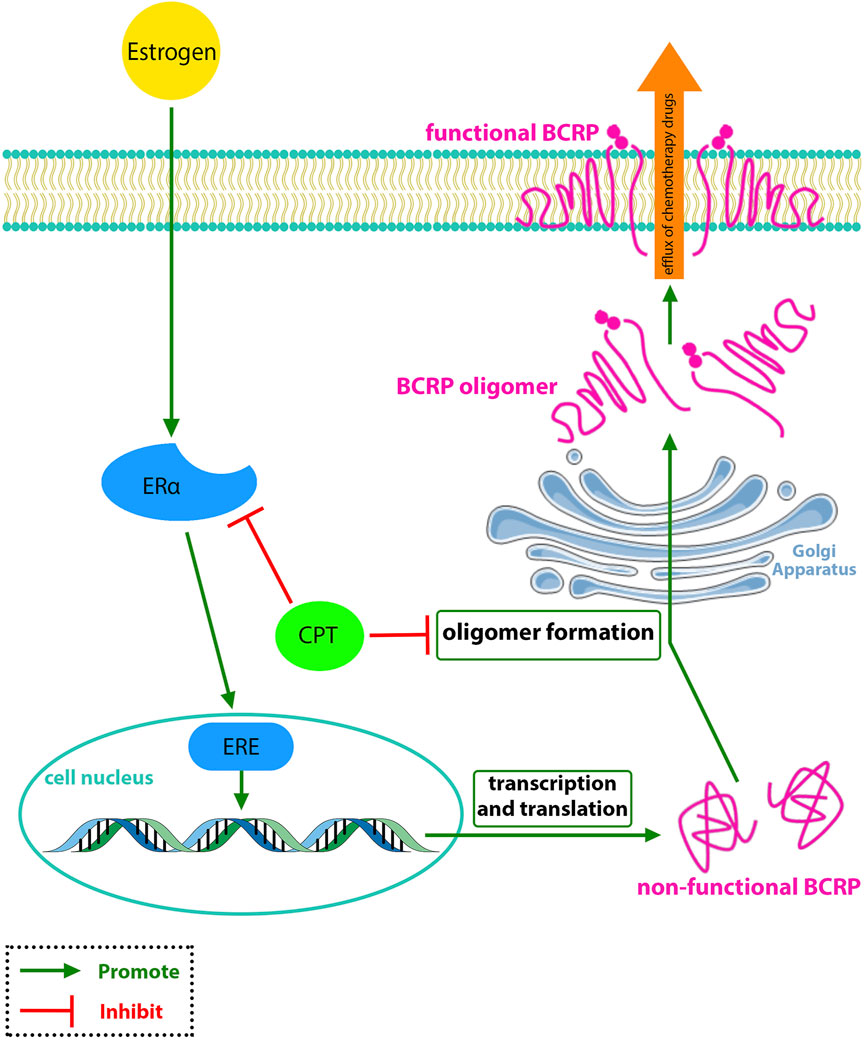
FIGURE 2. CPT interferes with the oligomer formation of BCRP and inhibits the BCRP function in the efflux of chemotherapy drugs which is associated with MDR-BRCA.
In a similar study, Pan et al. (2017) used cancer cells with the acquired multidrug resistance (MCF-7/ADR). The results showed an undetected Tamoxifen cytotoxic effect on the MCF-7/ADR cancer cells, while CPT had a significant inhibitory effect. Moreover, CPT conjoined with Tamoxifen plies a synergic effect on the MCF-7/ADR cells. These findings indicated that CPT suppresses cell viability and proliferation in the tamoxifen-resistant BRCA cells especially combined with Tamoxifen.
Cryptotanshinone inhibits the metabolism of breast cancer cells
Cancerous cells have a higher metabolic rate to promote tumor proliferation and progression (Fanciulli et al., 2000; Lu et al., 2008). Expression of glycolysis-related proteins, like HK2, LDHA, and PKM2, increases in breast cancer cells. PKM2, a poor prognostic marker, is an enzyme that induces glycolysis in breast cancer cells. CPT reduces the expression of PKM2 in both ERα-positive and ERα-negative breast cancer cells, inhibiting glycolysis. Glycolysis inhibition reduces metabolic rate and increases the sensitivity of cancerous cells to chemotherapy drugs (Zhou et al., 2020).
It is worth noting that some articles clarified that PKM2 also could be translocated into the nucleus of breast cancer cells, functioning as a transcription factor that transactivates β-catenin. β-Catenin is one of the most important mediators of angiogenesis, invasion, and cell migration in breast cancer (Yang et al., 2011; Wang et al., 2017a). Therefore CPT inhibits the invasion and migration of breast cancer cells by inhibiting the PKM2/β-catenin axis in both ERα-positive and -negative breast cancer cell lines (Zhou et al., 2020).
Conclusion
Breast cancer incidence is dramatically increasing year by year in women worldwide. It has recently passed the other malignancies and has become the most life-threatening female cancer with the first rank (Sung et al., 2021). Despite various treatments available for different severity of breast cancer, including surgical resection, chemotherapy, and radiotherapy, there are types of BRCA with poor prognosis, particularly triple-negative breast cancer. While surgical resection is prescribed for only a minority of BRCA patients, most patients undergo chemotherapy which has increased the patients’ lifelong. A beneficial drug causes minimal damage to healthy body cells while having the most cytotoxicity to cancer cells. Therefore, suggesting new anticancer medicine with greater efficacy and fewer side effects is one of the hotspots for cancer treatment in recent years.
Cryptothanshinone, a derivation of the plant S. miltiorrhiza Bunge, has been recently considered by researchers due to its numerous anti-inflammatory and antitumor activity in vivo and in vitro. Notably, this Chinese herbal medicine is efficient in cancer treatment through different mechanisms, including targeting various molecular signaling pathways. In this study, we focused on the anti-cancer activity of CPT against breast cancer and summarized the various biological mechanisms through which CPT affects different types of BRCA. Most studies were conducted using ERα-positive and -negative BRCA. But no study considered the TNBC type. Therefore, evaluating the effects of CPT on the most challenging type of breast cancer, TNBC, is recommended for future studies. For ERα-Positive BRCA cells, studies have shown that CPT inhibits proliferation, migration, invasion, and cell viability of tumor cells in vivo or in vitro. Effects on estrogen receptor function, regulation of gene expression, endoplasmic reticulum stress-induced apoptosis, induction of the immune response, and inhibition of glycolysis are mechanisms that are affected by CPT.
In the case of ERα-negative BRCA cells, in vivo and in vitro studies have demonstrated that CPT provided its anti-cancer effect through GPER-mediated pathways, apoptosis via reactive oxygen species, and to a lesser extent through regulation of gene expression. Also, CPT has shown a synergic effect, more bioavailability, and more induced sensitivity to chemotherapy when combined with other drugs such as Silibinin, arsenic species, and conventional chemotherapy drugs. In summary, cryptotanshinone should be recognized as herbal medicine that offers many antitumor mechanisms and has considerable potential for treating female breast cancer.
Author contributions
DD designed the study, searched databases, and consulted literature. DD and SI participated in manuscript sorting. DD, SI, and SK drafted the manuscript. All authors carefully revised and edited the manuscript.
Conflict of interest
The authors declare that the research was conducted in the absence of any commercial or financial relationships that could be construed as a potential conflict of interest.
Publisher’s note
All claims expressed in this article are solely those of the authors and do not necessarily represent those of their affiliated organizations, or those of the publisher, the editors and the reviewers. Any product that may be evaluated in this article, or claim that may be made by its manufacturer, is not guaranteed or endorsed by the publisher.
References
Abderrahman, B., and Jordan, V. C. (2018). Successful targeted therapies for breast cancer: The worcester foundation and future opportunities in women’s health. Endocrinology 159 (8), 2980–2990. doi:10.1210/en.2018-00263 | |
Akasaki, Y., Alvarez‐Garcia, O., Saito, M., Caramés, B., Iwamoto, Y., and Lotz, M. K. (2014). FoxO transcription factors support oxidative stress resistance in human chondrocytes. Arthritis Rheumatol. 66 (12), 3349–3358. doi:10.1002/art.38868 | |
Anothaisintawee, T., Wiratkapun, C., Lerdsitthichai, P., Kasamesup, V., Wongwaisayawan, S., Srinakarin, J., et al. (2013). Risk factors of breast cancer: A systematic review and meta-analysis. Asia. Pac. J. Public Health 25 (5), 368–387. doi:10.1177/1010539513488795 | |
Ariazi, E. A., Brailoiu, E., Yerrum, S., Shupp, H. A., Slifker, M. J., Cunliffe, H. E., et al. (2010). The G protein–coupled receptor GPR30 inhibits proliferation of estrogen receptor–positive breast cancer cells. Cancer Res. 70 (3), 1184–1194. doi:10.1158/0008-5472.CAN-09-3068 | |
Balaña-Fouce, R., Reguera, R., Cubrıa, J., and Ordóñez, D. (1998). The pharmacology of leishmaniasis. Gen. Pharmacol. 30 (4), 435–443. doi:10.1016/s0306-3623(97)00268-1 | |
Bhat, M. A., Al-Dhfyan, A., Naglah, A. M., Khan, A. A., and Al-Omar, M. A. (2015). Lead optimization of 2-Cyclohexyl-N-[(Z)-(3-methoxyphenyl/3-hydroxyphenyl) methylidene] hydrazinecarbothioamides for targeting the HER-2 overexpressed breast cancer cell line SKBr-3. Molecules 20 (10), 18246–18263. doi:10.3390/molecules201018246 | |
Brody, J. G., Rudel, R. A., Michels, K. B., Moysich, K. B., Bernstein, L., Attfield, K. R., et al. (2007). Environmental pollutants, diet, physical activity, body size, and breast cancer: Where do we stand in research to identify opportunities for prevention? Cancer 109, 2627–2634. doi:10.1002/cncr.22656 | |
Cleator, S. J., Ahamed, E., Coombes, R. C., and Palmieri, C. (2009). A 2009 update on the treatment of patients with hormone receptor—Positive breast cancer. Clin. Breast Cancer 9, S6–S17. doi:10.3816/CBC.2009.s.001 | |
Cragg, G. M., and Newman, D. J. (2009). Nature: A vital source of leads for anticancer drug development. Phytochem. Rev. 8 (2), 313–331. doi:10.1007/s11101-009-9123-y |
Deep, G., and Agarwal, R. (2010). Antimetastatic efficacy of silibinin: Molecular mechanisms and therapeutic potential against cancer. Cancer Metastasis Rev. 29 (3), 447–463. doi:10.1007/s10555-010-9237-0 | |
Deep, G., and Agarwal, R. (2013). Targeting tumor microenvironment with silibinin: Promise and potential for a translational cancer chemopreventive strategy. Curr. Cancer Drug Targets 13 (5), 486–499. doi:10.2174/15680096113139990041 | |
Deroo, B. J., and Korach, K. S. (2006). Estrogen receptors and human disease. J. Clin. Invest. 116 (3), 561–570. doi:10.1172/JCI27987 | |
DeSantis, C., Ma, J., Bryan, L., and Jemal, A. (2014). Breast cancer statistics, 2013. Ca. Cancer J. Clin. 64 (1), 52–62. doi:10.3322/caac.21203 | |
Fanciulli, M., Bruno, T., Giovannelli, A., Gentile, F. P., Di Padova, M., Rubiu, O., et al. (2000). Energy metabolism of human LoVo colon carcinoma cells: Correlation to drug resistance and influence of lonidamine. Clin. Cancer Res. 6 (4), 1590–1597. |
Gage, M., Wattendorf, D., and Henry, L. (2012). Translational advances regarding hereditary breast cancer syndromes. J. Surg. Oncol. 105 (5), 444–451. doi:10.1002/jso.21856 | |
Gao, D., Du, J., Cong, L., and Liu, Q. (2009). Risk factors for initial lung metastasis from breast invasive ductal carcinoma in stages I–III of operable patients. Jpn. J. Clin. Oncol. 39 (2), 97–104. doi:10.1093/jjco/hyn133 | |
Girgert, R., Emons, G., and Gründker, C. (2019). Estrogen signaling in erα-negative breast cancer: ERβ and GPER. Front. Endocrinol. 9, 781. doi:10.3389/fendo.2018.00781 |
Gong, Y., Li, Y., Abdolmaleky, H. M., Li, L., and Zhou, J.-R. (2012). Tanshinones inhibit the growth of breast cancer cells through epigenetic modification of Aurora A expression and function. PloS one 7 (4), e33656. doi:10.1371/journal.pone.0033656 | |
Han, Z., Liu, S., Lin, H., Trivett, A. L., Hannifin, S., Yang, D., et al. (2019). Inhibition of murine hepatoma tumor growth by cryptotanshinone involves TLR7-dependent activation of macrophages and induction of adaptive antitumor immune defenses. Cancer Immunol. Immunother. 68 (7), 1073–1085. doi:10.1007/s00262-019-02338-4 | |
Harbeck, N., and Gnant, M. (2017). Breast cancer. Lancet 389 (10074), 1134–1150. doi:10.1016/S0140-6736(16)31891-8 | |
Healy, S. J., Gorman, A. M., Mousavi-Shafaei, P., Gupta, S., and Samali, A. (2009). Targeting the endoplasmic reticulum-stress response as an anticancer strategy. Eur. J. Pharmacol. 625 (1-3), 234–246. doi:10.1016/j.ejphar.2009.06.064 | |
Hsu, L.-H., Chu, N.-M., Lin, Y.-F., and Kao, S.-H. (2019). G-protein coupled estrogen receptor in breast cancer. Int. J. Mol. Sci. 20 (2), 306. doi:10.3390/ijms20020306 |
Jiang, G., Liu, J., Ren, B., Zhang, L., Owusu, L., Liu, L., et al. (2017). Anti-tumor and chemosensitization effects of Cryptotanshinone extracted from Salvia miltiorrhiza Bge. on ovarian cancer cells in vitro. J. Ethnopharmacol. 205, 33–40. doi:10.1016/j.jep.2017.04.026 | |
Johnston, S. R. (2010). New strategies in estrogen receptor–positive breast cancer. Clin. Cancer Res. 16 (7), 1979–1987. doi:10.1158/1078-0432.CCR-09-1823 | |
Kaiser, J. (2013). Cholesterol forges link between obesity and breast cancer. US: American Association for the Advancement of Science.
Kim, J.-H., Jeong, S.-J., Kwon, T.-R., Yun, S.-M., Jung, J. H., Kim, M., et al. (2011). Cryptotanshinone enhances TNF-α-induced apoptosis in chronic myeloid leukemia KBM-5 cells. Apoptosis 16 (7), 696–707. doi:10.1007/s10495-011-0605-1 | |
Klinge, C. M. (2001). Estrogen receptor interaction with estrogen response elements. Nucleic Acids Res. 29 (14), 2905–2919. doi:10.1093/nar/29.14.2905 | |
Lappano, R., Pisano, A., and Maggiolini, M. (2014). GPER function in breast cancer: An overview. Front. Endocrinol. 5, 66. doi:10.3389/fendo.2014.00066 | |
Lee, C.-Y., Sher, H.-F., Chen, H.-W., Liu, C.-C., Chen, C.-H., Lin, C.-S., et al. (2008). Anticancer effects of tanshinone I in human non-small cell lung cancer. Mol. Cancer Ther. 7 (11), 3527–3538. doi:10.1158/1535-7163.MCT-07-2288 | |
Li, H., Gao, C., Liang, Q., Liu, C., Liu, L., Zhuang, J., et al. (2021). Cryptotanshinone is a intervention for er-positive breast cancer: An integrated approach to the study of natural product intervention mechanisms. Front. Pharmacol. 11, 2223. doi:10.3389/fphar.2020.592109 |
Li, S., Wang, H., Hong, L., Liu, W., Huang, F., Wang, J., et al. (2015). Cryptotanshinone inhibits breast cancer cell growth by suppressing estrogen receptor signaling. Cancer Biol. Ther. 16 (1), 176–184. doi:10.4161/15384047.2014.962960 | |
Li, W., Zhang, H., Assaraf, Y. G., Zhao, K., Xu, X., Xie, J., et al. (2016). Overcoming ABC transporter-mediated multidrug resistance: Molecular mechanisms and novel therapeutic drug strategies. Drug resist. updat. 27, 14–29. doi:10.1016/j.drup.2016.05.001 | |
Liu, X., Pan, L., Liang, J., Li, J., and Wu, S. (2016). Cryptotanshinone inhibits proliferation and induces apoptosis via mitochondria-derived reactive oxygen species involving FOXO1 in estrogen receptor-negative breast cancer Bcap37 cells. RSC Adv. 6 (27), 22232–22243. doi:10.1039/c5ra22523j |
Liu, Y., Xie, X., Hou, X., Shen, J., Shi, J., Chen, H., et al. (2020). Functional oral nanoparticles for delivering silibinin and cryptotanshinone against breast cancer lung metastasis. J. Nanobiotechnology 18 (1), 83–15. doi:10.1186/s12951-020-00638-x | |
Loibl, S., Poortmans, P., Morrow, M., Denkert, C., and Curigliano, G. (2021). Breast cancer. Lancet 397 (10286), 1750–1769. doi:10.1016/s0140-6736(20)32381-3 | |
Lu, C.-W., Lin, S.-C., Chen, K.-F., Lai, Y.-Y., and Tsai, S.-J. (2008). Induction of pyruvate dehydrogenase kinase-3 by hypoxia-inducible factor-1 promotes metabolic switch and drug resistance. J. Biol. Chem. 283 (42), 28106–28114. doi:10.1074/jbc.M803508200 | |
Ma, J., and Jemal, A. (2013). “Breast cancer statistics,” in Breast cancer metastasis and drug resistance, 1–18. |
Mao, Q., and Unadkat, J. D. (2015). Role of the breast cancer resistance protein (BCRP/ABCG2) in drug transport—An update. AAPS J. 17 (1), 65–82. doi:10.1208/s12248-014-9668-6 | |
Merikhian, P., Ghadirian, R., Farahmand, L., Mansouri, S., and Majidzadeh-A, K. (2017). MUC1 induces tamoxifen resistance in estrogen receptor-positive breast cancer. Expert Rev. Anticancer Ther. 17 (7), 607–613. doi:10.1080/14737140.2017.1340837 | |
Miricescu, D., Totan, A., Stanescu-Spinu, I.-I., Badoiu, S. C., Stefani, C., and Greabu, M. (2020). PI3K/AKT/mTOR signaling pathway in breast cancer: From molecular landscape to clinical aspects. Int. J. Mol. Sci. 22 (1), 173. doi:10.3390/ijms22010173 |
Molina, L., Figueroa, C. D., Bhoola, K. D., and Ehrenfeld, P. (2017). GPER-1/GPR30 a novel estrogen receptor sited in the cell membrane: Therapeutic coupling to breast cancer. Expert Opin. Ther. Targets 21 (8), 755–766. doi:10.1080/14728222.2017.1350264 | |
Ni, W., Fan, H., Zheng, X., Xu, F., Wu, Y., Li, X., et al. (2021). Cryptotanshinone inhibits erα-dependent and -independent BCRP oligomer formation to reverse multidrug resistance in breast cancer. Front. Oncol. 11 (688), 624811. doi:10.3389/fonc.2021.624811 | |
Osborne, C. K., Schiff, R., Fuqua, S. A., and Shou, J. (2001). Estrogen receptor: Current understanding of its activation and modulation. Clin. Cancer Res. 7 (12), 4338s–4342s. |
Pagano, M., Pepperkok, R., Verde, F., Ansorge, W., and Draetta, G. (1992). Cyclin A is required at two points in the human cell cycle. EMBO J. 11 (3), 961–971. doi:10.1002/j.1460-2075.1992.tb05135.x | |
Pan, Y., Shi, J., Ni, W., Liu, Y., Wang, S., Wang, X., et al. (2017). Cryptotanshinone inhibition of mammalian target of rapamycin pathway is dependent on oestrogen receptor alpha in breast cancer. J. Cell. Mol. Med. 21 (9), 2129–2139. doi:10.1111/jcmm.13135 | |
Park, I.-J., Kim, M.-J., Park, O. J., Choe, W., Kang, I., Kim, S.-S., et al. (2012). Cryptotanshinone induces ER stress-mediated apoptosis in HepG2 and MCF7 cells. Apoptosis 17 (3), 248–257. doi:10.1007/s10495-011-0680-3 | |
Platet, N., Cathiard, A. M., Gleizes, M., and Garcia, M. (2004). Estrogens and their receptors in breast cancer progression: A dual role in cancer proliferation and invasion. Crit. Rev. Oncol. Hematol. 51 (1), 55–67. doi:10.1016/j.critrevonc.2004.02.001 | |
Shi, D., Zhao, P., Cui, L., Li, H., Sun, L., Niu, J., et al. (2020). Inhibition of PI3K/AKT molecular pathway mediated by membrane estrogen receptor GPER accounts for cryptotanshinone induced antiproliferative effect on breast cancer SKBR-3 cells. BMC Pharmacol. Toxicol. 21 (1), 32–10. doi:10.1186/s40360-020-00410-9 | |
Shin, D.-S., Kim, H.-N., Shin, K. D., Yoon, Y. J., Kim, S.-J., Han, D. C., et al. (2009). Cryptotanshinone inhibits constitutive signal transducer and activator of transcription 3 function through blocking the dimerization in DU145 prostate cancer cells. Cancer Res. 69 (1), 193–202. doi:10.1158/0008-5472.CAN-08-2575 | |
Steiman, J., Peralta, E. A., Louis, S., and Kamel, O. (2013). Biology of the estrogen receptor, GPR30, in triple negative breast cancer. Am. J. Surg. 206 (5), 698–703. doi:10.1016/j.amjsurg.2013.07.014 | |
Stein, B., and Yang, M. X. (1995). Repression of the interleukin-6 promoter by estrogen receptor is mediated by NF-kappa B and C/EBP beta. Mol. Cell. Biol. 15 (9), 4971–4979. doi:10.1128/mcb.15.9.4971 | |
Sung, H., Ferlay, J., Siegel, R. L., Laversanne, M., Soerjomataram, I., Jemal, A., et al. (2021). Global cancer statistics 2020: GLOBOCAN estimates of incidence and mortality worldwide for 36 cancers in 185 countries. Ca. Cancer J. Clin. 71 (3), 209–249. doi:10.3322/caac.21660 | |
Tang, S., Shen, X.-Y., Huang, H.-Q., Xu, S.-W., Yu, Y., Zhou, C.-H., et al. (2011). Cryptotanshinone suppressed inflammatory cytokines secretion in RAW264. 7 macrophages through inhibition of the NF-κB and MAPK signaling pathways. Inflammation 34 (2), 111–118. doi:10.1007/s10753-010-9214-3 | |
Thannickal, V. J., and Fanburg, B. L. (2000). Reactive oxygen species in cell signaling. Am. J. Physiol. Lung Cell. Mol. Physiol. 279 (6), L1005–L1028. doi:10.1152/ajplung.2000.279.6.L1005 | |
Tong, C. W., Wu, M., Cho, W., and To, K. K. (2018). Recent advances in the treatment of breast cancer. Front. Oncol. 8, 227. doi:10.3389/fonc.2018.00227 | |
Wang, J., Li, M., Chen, D., Nie, J., Xi, Y., Yang, X., et al. (2017a). Expression of C-myc and β-catenin and their correlation in triple negative breast cancer. Minerva Med. 108 (6), 513–517. doi:10.23736/S0026-4806.17.05213-2 | |
Wang, J., Seebacher, N., Shi, H., Kan, Q., and Duan, Z. (2017b). Novel strategies to prevent the development of multidrug resistance (MDR) in cancer. Oncotarget 8 (48), 84559–84571. doi:10.18632/oncotarget.19187 | |
Wang, Q., Su, L., Liu, N., Zhang, L., Xu, W., and Fang, H. (2011). Cyclin dependent kinase 1 inhibitors: A review of recent progress. Curr. Med. Chem. 18 (13), 2025–2043. doi:10.2174/092986711795590110 | |
Weißenborn, C., Ignatov, T., Poehlmann, A., Wege, A. K., Costa, S. D., Zenclussen, A. C., et al. (2014). GPER functions as a tumor suppressor in MCF-7 and SK-BR-3 breast cancer cells. J. Cancer Res. Clin. Oncol. 140 (4), 663–671. doi:10.1007/s00432-014-1598-2 | |
Wu, Y.-H., Wu, Y.-R., Li, B., and Yan, Z.-Y. (2020). Cryptotanshinone: A review of its pharmacology activities and molecular mechanisms. Fitoterapia 145, 104633. doi:10.1016/j.fitote.2020.104633 | |
Yager, J. D., and Davidson, N. E. (2006). Estrogen carcinogenesis in breast cancer. N. Engl. J. Med. 354 (3), 270–282. doi:10.1056/NEJMra050776 | |
Yang, W., Xia, Y., Ji, H., Zheng, Y., Liang, J., Huang, W., et al. (2011). Nuclear PKM2 regulates β-catenin transactivation upon EGFR activation. Nature 480 (7375), 118–122. doi:10.1038/nature10598 | |
Ye, Y., Xu, W., and Zhong, W. (2010). Effects of cryptotanshinone on proliferation and apoptosis of Hela cell line of cervical cancer. Zhongguo Zhong yao za zhi= Zhongguo zhongyao zazhi= China J. Chin. materia medica 35 (1), 118–121. doi:10.4268/cjcmm20100125 | |
Zhang, W., Yu, W., Cai, G., Zhu, J., Zhang, C., Li, S., et al. (2018). Retracted article: A new synthetic derivative of cryptotanshinone KYZ3 as STAT3 inhibitor for triple-negative breast cancer therapy. Cell Death Dis. 9 (11), 1098–1111. doi:10.1038/s41419-018-1139-z | |
Zhang, Y. F., Zhang, M., Huang, X. L., Fu, Y. J., Jiang, Y. H., Bao, L. L., et al. (2015). The combination of arsenic and cryptotanshinone induces apoptosis through induction of endoplasmic reticulum stress-reactive oxygen species in breast cancer cells. Metallomics 7 (1), 165–173. doi:10.1039/c4mt00263f | |
Zhou, J., Su, C.-M., Chen, H.-A., Du, S., Li, C.-W., Wu, H., et al. (2020). Cryptanshinone inhibits the glycolysis and inhibits cell migration through PKM2/β-catenin axis in breast cancer. Onco. Targets. Ther. 13, 8629–8639. doi:10.2147/OTT.S239134 | |
Zhou, J., Xu, X.-Z., Hu, Y.-R., Hu, A.-R., Zhu, C.-L., and Gao, G.-S. (2014). Cryptotanshinone induces inhibition of breast tumor growth by cytotoxic CD4+ T cells through the JAK2/STAT4/perforin pathway. Asian pac. J. Cancer Prev. 15 (6), 2439–2445. doi:10.7314/apjcp.2014.15.6.2439 | |
Keywords: salvia miltiorrhiza, cryptotanshinon, tanshinone C, breast cancer, molecular mechanism, drug combination, estrogen receptor
Citation: Dalil D, Iranzadeh S and Kohansal S (2022) Anticancer potential of cryptotanshinone on breast cancer treatment; A narrative review. Front. Pharmacol. 13:979634. doi: 10.3389/fphar.2022.979634
Received: 27 June 2022; Accepted: 31 August 2022;
Published: 16 September 2022.
Edited by:
Dietrich Büsselberg, Weill Cornell Medicine- Qatar, QatarReviewed by:
Changping Deng, East China University of Science and Technology, ChinaChao Zhang, China Pharmaceutical University, China
Copyright © 2022 Dalil, Iranzadeh and Kohansal. This is an open-access article distributed under the terms of the Creative Commons Attribution License (CC BY). The use, distribution or reproduction in other forums is permitted, provided the original author(s) and the copyright owner(s) are credited and that the original publication in this journal is cited, in accordance with accepted academic practice. No use, distribution or reproduction is permitted which does not comply with these terms.
*Correspondence: Davood Dalil, Davood.dalil@shahed.ac.ir