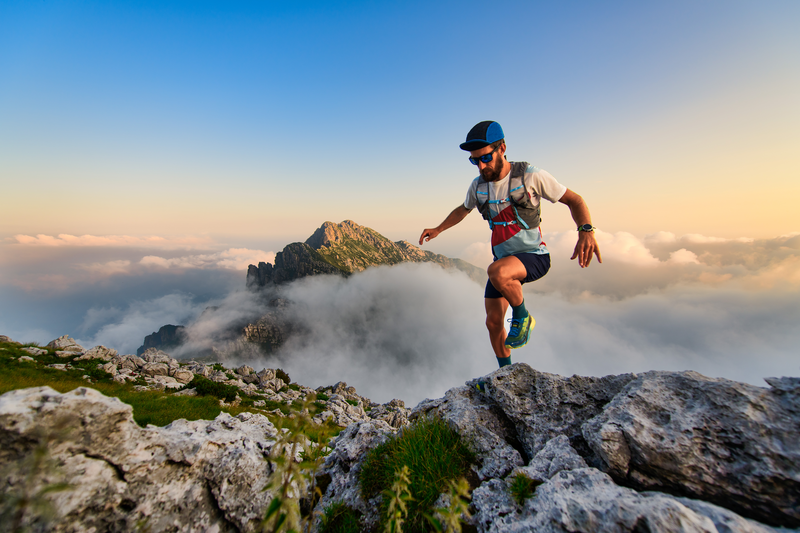
94% of researchers rate our articles as excellent or good
Learn more about the work of our research integrity team to safeguard the quality of each article we publish.
Find out more
ORIGINAL RESEARCH article
Front. Pharmacol. , 02 September 2022
Sec. Neuropharmacology
Volume 13 - 2022 | https://doi.org/10.3389/fphar.2022.974949
This article is part of the Research Topic Innovative Treatments for Neuro-Psychiatric Diseases View all 5 articles
Neferine (Nef) might possess anti-depressive properties; however, its therapeutic effects are yet to be elucidated. Therefore, in this study, we aimed to explore the anti-depressant property of Nef using a mouse model of chronic stress-induced depression. Fifteen depression-prone mice were randomly selected and divided into three groups, namely, the model, Nef, and fluoxetine (Flu) groups. We observed that in tail suspension and forced swimming tests, the Nef and Flu treatments significantly decreased the immobility time of the depressed mice, and increased their sucrose preference indices. Moreover, both Nef and Flu treatments induced significant increases in the levels of anti-depressant neurotransmitters, including dopamine (DA), serotonin (5-HT), and norepinephrine (NE), and also reduced pathological damage to the hippocampus of the depressed mice. Incidentally, Illumina MiSeq sequencing analysis demonstrated that the relative abundance of Lactobacillus in the intestinal microbiota of depressed mice was restored after Nef/Flu treatment. Moreover, colonic Lactobacillus abundance was positively correlated with the levels of DA, 5-HT, and NE in the hippocampus of the mice. In conclusion, Nef improved monoamine neurotransmitter secretion and modulated the intestinal flora structure, particularly the abundance of Lactobacillus. Hence, it showed considerable anti-depressant potential, and might be a prospective anti-depressant therapeutic agent.
In recent years, the incidence of depression, especially among children and adolescents, has been on the rise. In fact, depression has become the second most common cause of death among young (Patton et al., 2009; Johnson et al., 2018; Christ et al., 2020; Arzola et al., 2022). Therefore, conducting research to determine effective treatment options for depression is of great social significance.
Based on the “monoamine hypothesis,” a decrease in monoamine neurotransmitter levels in the synaptic cleft is recognized as the major factor related to the pathogenesis of depression in clinical settings (Zhang et al., 2019). Most classical antidepressants, such as tricyclic antidepressants (TCAS), tetracyclic antidepressants (HCA), monoamine oxidase inhibitors (MAOI), and selective serotonin (5-HT) reuptake inhibitors (SSRIs), which are all commercially available, are based on this theory (Zhang et al., 2019). However, despite using adequate doses of these drugs as well as maintenance therapy, 30%–40% of patients remain unresponsive, leading to significant treatment resistance and unsatisfactory outcomes (Cipriani et al., 2018).
In both human and animal experimental models, stress has been widely recognized as an independent risk factor for the occurrence of major depressive disorder (MDD) (Yirmiya et al., 2015; Cruz-Pereira et al., 2020). Additionally, stress-related factors, such as infection, inflammation, hypoxia, or psychological stress can lead to depression via the activation of the hypothalamic-pituitary-adrenal axis or the autonomic nervous system, the inhibition of afferent vagus nerve fibers, and leading to consequently, inflammation and tryptophan metabolism dysregulation (Herselman et al., 2022). Stress may also lead to functional deficits in certain brain regions, three of which, including the prefrontal cortex, hippocampus, and amygdala, have received the most attention in depression-related studies (Arzola et al., 2022). The notable hallmarks of MDD include apparent structural and functional deficits in the hippocampus, a brain region that is linked to mood regulation and memory (Yun et al., 2018). Depressed humans and chronically-stressed animals have reduced hippocampal activity and volumes, and show decreased expression of activity-dependent genes and processes, including reduced adult neurogenesis in the hippocampus (Kronmüller et al., 2008; Rosa and Lisanby, 2012; Chevalier et al., 2020; Liu et al., 2021). Therefore, these hippocampal deficits have long been the target of depression treatments in an effort to ameliorate depression symptoms (Miller and Hen, 2015; Yun et al., 2016; Chevalier et al., 2020; Liu et al., 2021).
Gut microbiota play an important role in regulating host brain development and behavior (Mcguinness et al., 2022; Tian et al., 2022). Several studies have demonstrated that gut microbiota dysbiosis is closely related to host’s depression symptoms (Jiang et al., 2015; Petra et al., 2015; Feng et al., 2022; Ortega et al., 2022). Interestingly, a previous study revealed that regulating the composition of intestinal flora might be effective for preventing as well as treating depression (Yarandi et al., 2016). This may be because intestinal flora can influence the absorption and bioavailability of oral drugs (Klünemann et al., 2021; Shang et al., 2021). Other recent studies have suggested that stress can also contribute to MDD by affecting gut microbiota (Herselman et al., 2022). This is because stress can lead to impaired gut barrier integrity and reduced gut mucus, as well as gut microbial dysbiosis, which in turn increases neuroinflammation-induced depression- and anxiety-like behaviors (Guolan et al., 2018; Amini-Khoei et al., 2019; Oh et al., 2020; Deng et al., 2021; Gao et al., 2022). Therefore, improving gut dysbiosis may become a new strategy for the treatment of stress-induced MMD.
Recently, attempts have been made at using traditional Chinese medicine to treat MDD by altering gut microbiota structure (Li et al., 2022; Song et al., 2022; Tan et al., 2022). For example, the antidepressant, Shuganjieyu Capsules can alter gut microbiota structure and function in stress-induced depressed rats, and improve depressive symptoms (Tan et al., 2022). Interestingly, in addition to its use as treatment for nervous disorders, high fever, agitation, and insomnia, the seed embryo of lotus, a common traditional Chinese medicine, has anti-hypertensive and sedative properties (Kumarihamy et al., 2015). Specifically, neferine (Nef), derived from the seed embryo of lotus, is a unique bisbenzylisoquinoline alkaloid (Marthandam Asokan et al., 2018). A previous study on diabetic mice revealed that Nef exerts neuroprotective effects as well as ability to improve memory and overcome cognitive impairment (Wu et al., 2020). It has also been reported that Nef can be used to reverse cognitive impairment in Alzheimer’s disease in rats (Yin et al., 2020). Additionally, Nef has affinity for δ- and μ-opioid receptors (Kumarihamy et al., 2015). Moreover, it has also been demonstrated that opioid peptides and their receptors are potential candidates for developing novel antidepressant therapies; their effects are mediated by three receptor subtypes, namely δ-, μ-, and κ-receptors (Berrocoso et al., 2009). Previous studies have also shown that Nef has sedative and anxiolytic effects and can significantly reduce locomotor activity in mice in the forced swimming test (Sugimoto et al., 2008; Sugimoto et al., 2010); thus, it has been speculated that it may have antidepressant effects. Studies have shown that Nef shows anti-inflammatory activity (Jung et al., 2010; Deng et al., 2021), and inflammatory response is an important mechanism for the pathogenesis of depression (Liu et al., 2017). Other studies have demonstrated that it exerts antioxidant stress effects to reduce inflammatory response (Bharathi Priya et al., 2021), and the regulatory pathways in this regard include the ROS/NLRP3/Caspase-1 (Takahashi et al., 2019; Tang et al., 2019), NF-κB (Zhong et al., 2020; Chiu et al., 2021), and PI3K/AKT/mTOR (Qi et al., 2021) signaling pathways. Further, given that several studies on the use of Nef to regulate oxidative stress and inflammatory response in other models have been reported, in this study, our desire was to investigate the therapeutic effect of Nef on depression from another perspective, such as the monoamine hypothesis and intestinal flora structure.
Therefore, our aim in this study was to explore the anti-depressive effects of Nef via behavioral analyses, hippocampal neurotransmitter level assessment, and the investigation of the structural changes in the intestinal flora of mice. We also performed a preliminary exploration of the intestinal flora targets for the anti-depressive functions of Nef. Thus, we demonstrated the regulatory mechanism of the “microbiota-gut-brain” axis in depression.
We purchased 30 C57BL/6J mice (6-week-old, male) from Chengdu Dasuo Biotechnology Co., Ltd. (Sichuan, China), of which 25 were randomly selected for the establishment of the depression model, while the remaining five served as the control group (control). All the mice were housed and maintained under a 12-h/12-h light-dark cycle at 20–24°C. The depression model was established using a protocol in which the mice were raised alone and exposed to chronic unknown mild stress (CUMS) daily for 8 weeks, as described in a previous study (Kim et al., 2020). The specific methods are shown in Tables 1, 2. After 8 weeks, the mice were then subjected to behavioral tests, and the ones with significant depressive symptoms were considered successful depression models. Subsequently, 15 depression-prone mice were randomly selected and divided into three groups, namely the model, Nef, and fluoxetine (Flu) groups (n = 5 per group). The mice in the Nef group were intraperitoneally administered Nef injections at 20 mg/kg/d for 4 weeks, while those in the Flu group were intraperitoneally administered Flu injections at 20 mg/kg/d for 4 weeks. Nef (CAS. 2292-16-2, purity ≥98%) was purchased from Sichuan Weikeqi Biological Technology, Co., Ltd. (Sichuan, China), while Flu was purchased from Sigma Aldrich (St. Louis, MO, United States). Moreover, the mice in the control and model groups were intraperitoneally administered equal amounts of normal saline for 4 weeks. All the procedures involving the animals were approved by the ethics committee of West China Hospital (WCH) of Sichuan University (approval number 20211707A).
A day before sampling, SPTs were performed. As previously described (Liu et al., 2020), the SPTs were performed in two phases, i.e., the adaptation training phase, followed by the test phase. During the first phase, all the mice were trained to become adapted to drinking sucrose water. Thereafter, the mice were deprived of this water as well as food for 24 h. Then in the test phase, the mice were allowed to choose between two bottles, one containing 1% (W/V) sucrose solution and the other containing pure water. Both bottles were weighed in advance. After 12 h, both bottles were removed from the experimental set-up and reweighed. The total liquid, sucrose solution, and pure water consumptions of the mice were then recorded. The SPT results thus obtained were then used to measure anhedonic responses.
The formula for calculating sugar preference (SP) index was as follows:
On the second day after the SPT, the TST, a behavioral despair-based test, was performed. As previously described (Steru et al., 1985; Rosa et al., 2019), the mice were suspended 15 cm above the floor using an adhesive tape, which was placed approximately 1 cm from the tip of the tail. Thereafter, the duration of immobility of the mice in the 4 min test was recorded.
Three hours after the TST, the FST, another behavior despair-based test, was performed. As previously described (Liu et al., 2020), the mice were placed in a cylindrical container with clean water. The water depth was 30 cm, and the temperature was 25 ± 1°C. The mice were forced to swim, and the durations of their immobile states within the last 4 min of an 8 min test period were recorded.
After behavior assessment, the mice were intraperitoneally anesthetized with 30 mg/kg sodium pentobarbital and sacrificed. Hippocampal tissue samples and colon contents were then collected for subsequent analyses. A portion of the hippocampal tissue was fixed in 4% paraformaldehyde for pathological examination, while the rest was preserved in liquid nitrogen for enzyme-linked immunosorbent assay (ELISA). The colon contents were stored in liquid nitrogen and sent to Shanghai Personal Biotechnology Co., Ltd. (Shanghai, China) for 16S rRNA high-throughput sequencing.
Hippocampal tissue samples from the mice were subjected to H&E staining according to the standard procedure. In brief, the hippocampal tissue samples were fixed with 4% paraformaldehyde, embedded in paraffin blocks, dehydrated, and sliced. Subsequently, the sections were stained with hematoxylin for 10–20 min, followed by eosin for 3–5 min. The staining results were then observed (at ×100 and ×400 magnification) using the Motic BA210 digital tri camera microscope (Xiamen, China).
Hippocampal tissue samples were also analyzed via Nissl staining according to the standard procedure. In brief, the hippocampal tissue sections were placed in 1% toluidine blue solution (50°C) at 56°C for 20 min. After differentiating and dehydrating in different concentrations of alcohol, staining was then observed (at ×400 magnification) using the Motic BA210 digital tri camera microscope (Xiamen, China). The average optical density of five sections for each region was recorded as the final value for that region.
The levels of hippocampal dopamine (DA), serotonin (5-HT), and norepinephrine (NE) were determined using ELISA kits according to the manufacturer’s instructions. These ELISA kits for DA (ml002024), 5-HT (ml001891), and NE (ml063805) were purchased from Shanghai Enzyme-linked Biotechnology Co., Ltd. (Shanghai, China).
The colonic contents, which were stored in liquid nitrogen and sent to the Shanghai Personal Biotechnology Co. Ltd., were subjected to paired-end sequencing using the 16S rRNA Illumina MiSeq platform for gene sequencing (Illumina, San Diego, CA, United States). After denoising, QIIME2 (2019.4) software was used for taxonomic annotation. The “Qiime taxa Barplot” command was launched, and the feature table was generated, after singleton data removal, to visualize the distribution of the microbiota composition for each sample at both the phylum and genus levels. Further, the analysis results were presented using histograms.
Data were analyzed using GraphPAD software version 8 (GraphPad Software Inc., San Diego, CA, United States), and the results were expressed as the mean ± standard deviation (SD). Statistical differences among the control, model, Nef, and Flu groups were determined by performing one-way analysis of variance (ANOVA). If the data fitted the homogeneity of variance, then the least significant difference (LSD) analysis was performed; otherwise, Tamhane’s T2 analysis was performed. Additionally, Pearson correlation analysis were performed using SPSS software version 19.0 (IBM, Armonk, NY, United States). Statistical significance was set at p < 0.05.
As shown in Figure 1, in both the TST (p < 0.0001) and FST (p < 0.001), the model mice showed significantly increased immobility durations, while their SP indices decreased significantly (p < 0.01) relative to those of the control mice. These observations indicated the successful establishment of the mouse model of depression. Additionally, the immobility durations of the Nef- and Flu-treated depressed mice in the TST (Nef, p < 0.01; Flu, p < 0.001) and FST (Nef, p < 0.05; Flu, p < 0.01) were significantly decreased, while their SP indices were significantly increased (Nef, p > 0.05; Flu, p < 0.01) relative to those of the model mice.
FIGURE 1. Nef improves behavioral depression symptoms in mice models of depression. TST, tail suspension test; FST, forced swimming test; SPT, sucrose preference test; Nef, neferine; Flu, fluoxetine. Nef and Flu both improved the depressed behavior of depressed mice. Data are presented as mean ± standard deviation (SD; n = 5 per group). **p < 0.01, ***p < 0.001, ****p < 0.0001 vs control; &p < 0.05, &&p < 0.01, &&&p < 0.001 vs model.
As shown in Figure 2, the hippocampal levels of anti-depression factors, namely DA (p < 0.01), 5-HT (p < 0.001), and NE (p < 0.0001), were significantly reduced in the model mice compared with their levels in the hippocampus of the control mice. Incidentally, the Nef and Flu treatments led to a significant increase in the hippocampal levels of DA (Nef, p < 0.05; Flu, p < 0.05), 5-HT (Nef, p < 0.05; Flu, p < 0.05), and NE (Nef, p < 0.05; Flu, p < 0.05) in the depressed mice, compared with their corresponding levels in the model mice.
FIGURE 2. Hippocampal expression of anti-depression factors in depressed mice restored by Nef treatment. DA, dopamine; 5-HT, serotonin; NE, norepinephrine; Nef, neferine; Flu, fluoxetine. Nef and Flu both increased the levels of DA, 5-HT, and NE in the hippocampus of depressed mice. Data are presented as mean ± standard deviation (SD; n = 5 per group). **p < 0.01, ***p < 0.001, ****p < 0.0001 vs control; &p < 0.05 vs model.
As shown in Figure 3, relative to the control mice, the hippocampus of depressed mice showed significantly increased pyramidal cell necrosis, while the number of Nissl bodies (p < 0.0001) decreased significantly. However, both the Nef and Flu treatments significantly reduced pyramidal cell necrosis and increased the number of Nissl bodies in the depressed mice (Nef, p < 0.01; Flu, p < 0.001) relative to the model mice.
FIGURE 3. Nef alleviates pathological damage in the hippocampus of depressed mice. H&E, hematoxylin and eosin; Green arrow, necrotic pyramidal cells; Nef, neferine; Flu, fluoxetine. Both Nef and Flu both reduced pyramidal cell necrosis and increased Nissl body count in the hippocampus of depressed mice. Data are presented as mean ± standard deviation (SD; n = 5 per group). ****p < 0.0001 vs control; &&p < 0.01, &&&p < 0.001 vs model.
The results of Illumina MiSeq sequencing of the colonic content of the mice are presented in Figure 4. At the phylum level, compared with the control group, the model group showed a decrease in the relative abundance of Firmicutes and an increase in that of Bacteroidetes. Furthermore, both Nef and Flu treatments reverted these changes in the relative abundances of Firmicutes and Bacteroidetes in the depressed mice. Additionally, at the genus level, Lactobacillus was the most dominant microflora in all the groups; however, compared with the control group, the model group showed a decrease in the relative abundance of this genus. Incidentally, both the Nef and Flu treatments restored the relative abundance of Lactobacillus in the depressed mice.
FIGURE 4. Nef treatment improves gut microbiota in depressed mice. The figure depicts gut microbiota composition in mice at phylum and genus levels (n = 5 per group). Nef, neferine; Flu, fluoxetine. At the phylum level, both Nef and Flu treatments reverted the changes in the relative abundances of Firmicutes and Bacteroidetes in the depressed mice. At the genus level, Nef as well as Flu treatments also restored the relative abundance of Lactobacillus in depressed mice.
To further confirm the correlation between gut microbiota and the improvement of depression symptoms, we performed Pearson correlation analysis involving the top 20 dominant microflora at the genus level and the hippocampal levels of anti-depressant factors. As shown in Figure 5, the relative abundance of Lactobacillus was significantly positively correlated with hippocampal levels of the anti-depressant factors, DA, 5-HT, and NE, while that of Oscillospira showed significantly negative correlations in this regard.
FIGURE 5. Pearson correlation analysis of the correlation between the top 20 dominant microflora at the genus level and the hippocampal levels of anti-depressant factors in mice (n = 5 per group). Nef, neferine; Flu, fluoxetine; DA, dopamine; 5-HT, serotonin; NE, norepinephrine. The relative abundance of Lactobacillus was significantly positively correlated with the hippocampal levels of DA, 5-HT, and NE, while that of Oscillospira showed significantly negatively correlations in this regard.
In view of the current situation that existing treatments for MDD still lack satisfactory efficacy, it is of great practical significance to continuously explore new and better treatment options. In this regard, studies on the antidepressant effects of traditional Chinese medicine represent one of the possible research directions in this regard.
In this study, Nef treatment reduced the immobility durations of depressed mice in TST and FST and increased their SP indices, suggesting that Nef played an anti-depressant role in a state of depression. In fact, Nef has been studied for its anxiolytic and antidepressant effects. In an elevated plus maze test, Sugimoto et al. (2008) observed that Nef exerts anxiolytic effects in mice. Based on FST results, they also indicated that Nef exhibits antidepressant-like antidepressant effects in mice, similar to typical antidepressants, and these effects were found to be mediated by 5-HT1A receptors (Sugimoto et al., 2010; Shi et al., 2019). Similar to these previous studies, our findings reconfirmed the antidepressant effect of Nef.
Our results also revealed that Nef improved depression by reducing hippocampal pyramidal cell necrosis and alleviating hippocampal lesions. Reportedly, pyramidal cell damage is associated with depression and memory decline (Salim, 2017). Several treatment approaches like Tuina, which affect the activation and functional connectivity of the hippocampus are thought to improve depressive symptoms (Tao et al., 2022). Therefore, the reason why Nef can protect hippocampal cells against lesions may be that it exerts anti-oxidative stress effects or inhibits the excessive release of the neurotransmitter glutamate, thereby exerts a protective effect on nerve cells (Marthandam Asokan et al., 2018; Yeh et al., 2020). However, given that related studies are limited, the specific mechanism by which Nef exerts its protective effect on nerve cells needs to be further studied.
The results of this study also demonstrated that Nef treatment restored the hippocampal levels of 5-HT, NE, and DA, thereby indicating that Nef exerts an anti-depressive effect via 5-HT/NE/DA triple reuptake. Given the critical roles of 5-HT, NE, and DA in the pathogenesis of MDD (Maletic et al., 2007; Liu et al., 2020; Rominger et al., 2015; Zhong et al., 2020), it is reasonable to think that this should be an important mechanism for the antidepressant efficacy of Nef. Zhao et al. (2022) also reported that another herbal medicine, Xiebai glycosides, can significantly improve the levels of NE and DA in brain homogenate from depressive model rats. Studies have also confirmed that the antidepressant effect of Nef is mediated by the 5-HT1A receptor. Specifically, Nef may enhance the activity of 5-HT neurons by inhibiting 5-HT reuptake or activating 5-HT metabolism (Sugimoto et al., 2010; Sugimoto et al., 2015). However, the mechanisms by which it affects DA and NE function are still unclear, hence require further in-depth studies.
In recent years, a large number of studies have confirmed that the gut microbiota composition and the associated metabolites are related to the pathogenesis, clinical phenotype, and treatment effect of depression (Mayneris-Perxachs et al., 2022), and even the structure of the brain (Lee et al., 2022). Colonization by gut microbiota from patients with depression can lead to depression-like behaviors in mice (Kelly et al., 2016; Zheng et al., 2016). However, probiotics, exercise, and diet can affect gut microbiota structure and also show antidepressant potential (Donoso et al., 2022). Additionally, both animal and human studies have shown that alterations in gut microbial composition and metabolic function may be associated with differential responses to antidepressants in depression (Duan et al., 2021; Zhang et al., 2021; Dong et al., 2022). In a particular study, it was observed that polyphenols in an edible herbal medicine can alter the abundance of flora associated with neuroinflammation by reversing intestinal microbiota dysbiosis and that intestinal flora-mediated chemical modification of polyphenols can result in their conversion into active secondary metabolites that improve depression (Hao et al., 2022). Another study showed that resveratrol markedly increases brain derived neurotrophic factor (BDNF) expression in the hippocampus, and this can help to improve depression and anxiety symptoms (Yu et al., 2019). Our study demonstrated that both Nef and Flu treatments increased the relative abundances of species belonging to phylum Firmicutes, but decreased those of species belonging to phylum Bacteroidetes in the depression mouse model. Additionally, they increased the relative abundance of Lactobacillus at the genus level. These results suggested that Nef and Flu treatments might improve depression via intestinal flora. Further, Pearson correlation analysis indicated that the relative abundance of Lactobacillus was significantly positively correlated with the levels of DA, 5-HT, and NE in the hippocampus, while that of Oscillospira showed significantly negatively correlations in this regard, suggesting that Nef improved depression via the brain-gut-microbial axis. Hence, Lactobacillus and Oscillospira might be the key microbial species associated with anti-depression.
Reportedly, Lactobacillus can mediate anti-depressant effects by promoting the functions of various neurotransmitters, such as 5-HT, DA, NE, and gamma-aminobutyric acid (GABA) (Yong et al., 2019; Yunes et al., 2020). The hypothalamic–pituitary–adrenal (HPA) axis is another target of Lactobacillus for treating depression symptoms (Johnson et al., 2021). Specifically, Lactobacillus rhamnosus can alter the expression of central GABA receptors, increase GABA expression level, and downregulate the HPA axis through the vagus nerve pathway, thereby functioning as an anti-depressant (Bravo et al., 2011; Janik et al., 2016). Therefore, the anti-depressant effects of Nef and Flu might be mediated through Lactobacillus, which is part of the intestinal flora of depressed mice; however, this requires further investigation.
In conclusion, this study revealed that Nef exerts therapeutic effects on depressed mice owing to its ability to improve hippocampal nerve damage, alleviate anti-depressant neurotransmitter secretion, and enrich the gut microbiota structure. Specifically, Lactobacillus might be the gut microbial target of Nef in treating the symptoms of depression.
The datasets presented in this study can be found in online repositories. The names of the repository/repositories and accession number(s) can be found below: https://www.ncbi.nlm.nih.gov/bioproject/PRJNA857835.
The animal study was reviewed and approved by ethics committee of West China Hospital (WCH) of Sichuan University.
ZD: Conceptualization, Funding acquisition, Methodology, Data analysis, Writing - Reviewing and Editing. QX: Conceptualization, Funding acquisition, Writing - Reviewing and Editing. FX and XS: Methodology. YH: Methodology, Data analysis. JL: Supervision, Validation. HX: Project administration. QP: Conceptualization, Methodology, Writing - Reviewing and Editing. WK: Conceptualization, Methodology, Funding acquisition, Validation, Writing - Reviewing and Editing.
This work was supported by the National Natural Science Foundation of China (Grant numbers 81621003 and 81801350) and the Key R&D Projects of the Science and Technology Department of Sichuan Province (Grant Numbers 2019YFS0217 and 2022YFS0350).
We thank Asbios (Tianjin) Biotechnology Co., LTD. for their assistance with the microbiological analysis. We would also like to thank Editage for English language editing.
The authors declare that the research was conducted in the absence of any commercial or financial relationships that could be construed as a potential conflict of interest.
All claims expressed in this article are solely those of the authors and do not necessarily represent those of their affiliated organizations, or those of the publisher, the editors and the reviewers. Any product that may be evaluated in this article, or claim that may be made by its manufacturer, is not guaranteed or endorsed by the publisher.
Amini-Khoei, H., Haghani-Samani, E., Beigi, M., Soltani, A., Mobini, G. R., Balali-Dehkordi, S., et al. (2019). On the role of corticosterone in behavioral disorders, microbiota composition alteration and neuroimmune response in adult male mice subjected to maternal separation stress. Int. Immunopharmacol. 66, 242–250. doi:10.1016/j.intimp.2018.11.037
Arzola, E., Xiong, W. C., and Mei, L. (2022). Stress reduces extracellular ATP in the prefrontal cortex and activates the prefrontal cortex-lateral habenula pathway for depressive-like behavior. Biol. Psychiatry 92, 172–174. doi:10.1016/j.biopsych.2022.05.016
Berrocoso, E., Sánchez-Blázquez, P., Garzón, J., and Mico, J. A. (2009). Opiates as antidepressants. Curr. Pharm. Des. 15, 1612–1622. doi:10.2174/138161209788168100
Bharathi Priya, L., Huang, C. Y., Hu, R. M., Balasubramanian, B., and Baskaran, R. (2021). An updated review on pharmacological properties of neferine-A bisbenzylisoquinoline alkaloid from Nelumbo nucifera. J. Food Biochem. 45, e13986. doi:10.1111/jfbc.13986
Bravo, J. A., Forsythe, P., Chew, M. V., Escaravage, E., Savignac, H. M., Dinan, T. G., et al. (2011). Ingestion of Lactobacillus strain regulates emotional behavior and central GABA receptor expression in a mouse via the vagus nerve. Proc. Natl. Acad. Sci. U. S. A. 108, 16050–16055. doi:10.1073/pnas.1102999108
Chevalier, G., Siopi, E., Guenin-Macé, L., Pascal, M., Laval, T., Rifflet, A., et al. (2020). Effect of gut microbiota on depressive-like behaviors in mice is mediated by the endocannabinoid system. Nat. Commun. 11, 6363. doi:10.1038/s41467-020-19931-2
Chiu, K. M., Hung, Y. L., Wang, S. J., Tsai, Y. J., Wu, N. L., Liang, C. W., et al. (2021). Anti-allergic and anti-inflammatory effects of neferine on RBL-2H3 cells. Int. J. Mol. Sci. 22, 10994. doi:10.3390/ijms222010994
Christ, C., Schouten, M. J., Blankers, M., Van Schaik, D. J., Beekman, A. T., Wisman, M. A., et al. (2020). Internet and computer-based cognitive behavioral therapy for anxiety and depression in adolescents and young adults: Systematic review and meta-analysis. J. Med. Internet Res. 22, e17831. doi:10.2196/17831
Cipriani, A., Furukawa, T. A., Salanti, G., Chaimani, A., Atkinson, L. Z., Ogawa, Y., et al. (2018). Comparative efficacy and acceptability of 21 antidepressant drugs for the acute treatment of adults with major depressive disorder: A systematic review and network meta-analysis. Lancet 391, 1357–1366. doi:10.1016/S0140-6736(17)32802-7
Cruz-Pereira, J. S., Rea, K., Nolan, Y. M., O'leary, O. F., Dinan, T. G., and Cryan, J. F. (2020). Depression's unholy trinity: Dysregulated stress, immunity, and the microbiome. Annu. Rev. Psychol. 71, 49–78. doi:10.1146/annurev-psych-122216-011613
Deng, Y., Zhou, M., Wang, J., Yao, J., Yu, J., Liu, W., et al. (2021). Involvement of the microbiota-gut-brain axis in chronic restraint stress: Disturbances of the kynurenine metabolic pathway in both the gut and brain. Gut Microbes 13, 1–16. doi:10.1080/19490976.2020.1869501
Dong, Z., Shen, X., Hao, Y., Li, J., Xu, H., Yin, L., et al. (2022). Gut microbiome: A potential indicator for predicting treatment outcomes in major depressive disorder. Front. Neurosci. 16, 813075. doi:10.3389/fnins.2022.813075
Donoso, F., Cryan, J. F., Olavarría-Ramírez, L., Nolan, Y. M., and Clarke, G. (2022). Inflammation, lifestyle factors, and the microbiome-gut-brain Axis: Relevance to depression and antidepressant action. Clin. Pharmacol. Ther. [Epub ahead of print]. doi:10.1002/cpt.2581
Duan, J., Huang, Y., Tan, X., Chai, T., Wu, J., Zhang, H., et al. (2021). Characterization of gut microbiome in mice model of depression with divergent response to escitalopram treatment. Transl. Psychiatry 11, 303. doi:10.1038/s41398-021-01428-1
Feng, L., Xing, H., and Zhang, K. (2022). The therapeutic potential of traditional Chinese medicine in depression: Targeting adult hippocampal neurogenesis. Phytomedicine. 98, 153980. doi:10.1016/j.phymed.2022.153980
Gao, K., Farzi, A., Ke, X., Yu, Y., Chen, C., Chen, S., et al. (2022). Oral administration of Lactococcus lactis WHH2078 alleviates depressive and anxiety symptoms in mice with induced chronic stress. Food Funct. 13, 957–969. doi:10.1039/d1fo03723d
Guolan, D., Lingli, W., Wenyi, H., Wei, Z., Baowei, C., and Sen, B. (2018). Anti-inflammatory effects of neferine on LPS-induced human endothelium via MAPK, and NF-κβ pathways. Pharmazie 73, 541–544. doi:10.1691/ph.2018.8443
Hao, W., Gan, H., Wang, L., Huang, J., and Chen, J. (2022). Polyphenols in edible herbal medicine: Targeting gut-brain interactions in depression-associated neuroinflammation. Crit. Rev. Food Sci. Nutr. 2022, 1–17. doi:10.1080/10408398.2022.2099808
Herselman, M. F., Bailey, S., and Bobrovskaya, L. (2022). The effects of stress and diet on the "Brain-Gut" and "Gut-Brain" pathways in animal models of stress and depression. Int. J. Mol. Sci. 23, 2013. doi:10.3390/ijms23042013
Janik, R., Thomason, L. a. M., Stanisz, A. M., Forsythe, P., Bienenstock, J., and Stanisz, G. J. (2016). Magnetic resonance spectroscopy reveals oral Lactobacillus promotion of increases in brain GABA, N-acetyl aspartate and glutamate. Neuroimage 125, 988–995. doi:10.1016/j.neuroimage.2015.11.018
Jiang, H., Ling, Z., Zhang, Y., Mao, H., Ma, Z., Yin, Y., et al. (2015). Altered fecal microbiota composition in patients with major depressive disorder. Brain Behav. Immun. 48, 186–194. doi:10.1016/j.bbi.2015.03.016
Johnson, D., Dupuis, G., Piche, J., Clayborne, Z., and Colman, I. (2018). Adult mental health outcomes of adolescent depression: A systematic review. Depress. Anxiety 35, 700–716. doi:10.1002/da.22777
Johnson, D., Thurairajasingam, S., Letchumanan, V., Chan, K.-G., and Lee, L.-H. (2021). Exploring the role and potential of probiotics in the field of mental health: Major depressive disorder. Nutrients 13, 1728. doi:10.3390/nu13051728
Jung, H. A., Jin, S. E., Choi, R. J., Kim, D. H., Kim, Y. S., Ryu, J. H., et al. (2010). Anti-amnesic activity of neferine with antioxidant and anti-inflammatory capacities, as well as inhibition of ChEs and BACE1. Life Sci. 87, 420–430. doi:10.1016/j.lfs.2010.08.005
Kelly, J. R., Borre, Y., C, O. B., Patterson, E., El Aidy, S., Deane, J., et al. (2016). Transferring the blues: Depression-associated gut microbiota induces neurobehavioural changes in the rat. J. Psychiatr. Res. 82, 109–118. doi:10.1016/j.jpsychires.2016.07.019
Kim, H. R., Lee, Y.-J., Kim, T.-W., Lim, R.-N., Hwang, D. Y., Moffat, J. J., et al. (2020). Asparagus cochinchinensis extract ameliorates menopausal depression in ovariectomized rats under chronic unpredictable mild stress. BMC Complement. Med. Ther. 20, 325. doi:10.1186/s12906-020-03121-0
Klünemann, M., Andrejev, S., Blasche, S., Mateus, A., Phapale, P., Devendran, S., et al. (2021). Bioaccumulation of therapeutic drugs by human gut bacteria. Nature 597, 533–538. doi:10.1038/s41586-021-03891-8
Kronmüller, K. T., Pantel, J., Köhler, S., Victor, D., Giesel, F., Magnotta, V. A., et al. (2008). Hippocampal volume and 2-year outcome in depression. Br. J. Psychiatry 192, 472–473. doi:10.1192/bjp.bp.107.040378
Kumarihamy, M., León, F., Pettaway, S., Wilson, L., Lambert, J. A., Wang, M., et al. (2015). In vitro opioid receptor affinity and in vivo behavioral studies of Nelumbo nucifera flower. J. Ethnopharmacol. 174, 57–65. doi:10.1016/j.jep.2015.08.006
Lee, S. M., Milillo, M. M., Krause-Sorio, B., Siddarth, P., Kilpatrick, L., Narr, K. L., et al. (2022). Gut microbiome diversity and abundance correlate with gray matter volume (GMV) in older adults with depression. Int. J. Environ. Res. Public Health 19, 2405. doi:10.3390/ijerph19042405
Li, J., Li, Y., Duan, W., Zhao, Z., Yang, L., Wei, W., et al. (2022). Shugan granule contributes to the improvement of depression-like behaviors in chronic restraint stress-stimulated rats by altering gut microbiota. CNS Neurosci. Ther. 28, 1409–1424. doi:10.1111/cns.13881
Liu, C. S., Adibfar, A., Herrmann, N., Gallagher, D., and Lanctot, K. L. (2017). Evidence for inflammation-associated depression. Curr. Top. Behav. Neurosci. 31, 3–30. doi:10.1007/7854_2016_2
Liu, S., Guo, R., Liu, F., Yuan, Q., Yu, Y., and Ren, F. (2020). Gut microbiota regulates depression-like behavior in rats through the neuroendocrine-immune-mitochondrial pathway. Neuropsychiatr. Dis. Treat. 16, 859–869. doi:10.2147/NDT.S243551
Liu, S., Xiu, J., Zhu, C., Meng, K., Li, C., Han, R., et al. (2021). Fat mass and obesity-associated protein regulates RNA methylation associated with depression-like behavior in mice. Nat. Commun. 12, 6937. doi:10.1038/s41467-021-27044-7
Maletic, V., Robinson, M., Oakes, T., Iyengar, S., Ball, S. G., and Russell, J. (2007). Neurobiology of depression: An integrated view of key findings. Int. J. Clin. Pract. 61, 2030–2040. doi:10.1111/j.1742-1241.2007.01602.x
Marthandam Asokan, S., Mariappan, R., Muthusamy, S., and Velmurugan, B. K. (2018). Pharmacological benefits of neferine - a comprehensive review. Life Sci. 199, 60–70. doi:10.1016/j.lfs.2018.02.032
Mayneris-Perxachs, J., Castells-Nobau, A., Arnoriaga-Rodríguez, M., Martin, M., De La Vega-Correa, L., Zapata, C., et al. (2022). Microbiota alterations in proline metabolism impact depression. Cell Metab. 34, 681–701. e610. doi:10.1016/j.cmet.2022.04.001
Mcguinness, A. J., Davis, J. A., Dawson, S. L., Loughman, A., Collier, F., O'hely, M., et al. (2022). A systematic review of gut microbiota composition in observational studies of major depressive disorder, bipolar disorder and schizophrenia. Mol. Psychiatry 27, 1920–1935. doi:10.1038/s41380-022-01456-3
Miller, B. R., and Hen, R. (2015). The current state of the neurogenic theory of depression and anxiety. Curr. Opin. Neurobiol. 30, 51–58. doi:10.1016/j.conb.2014.08.012
Oh, N. S., Joung, J. Y., Lee, J. Y., Song, J. G., Oh, S., Kim, Y., et al. (2020). Glycated milk protein fermented with Lactobacillus rhamnosus ameliorates the cognitive health of mice under mild-stress condition. Gut Microbes 11, 1643–1661. doi:10.1080/19490976.2020.1756690
Ortega, M. A., Alvarez-Mon, M. A., García-Montero, C., Fraile-Martinez, O., Guijarro, L. G., Lahera, G., et al. (2022). Gut microbiota metabolites in major depressive disorder-deep insights into their pathophysiological role and potential translational applications. Metabolites 12, 50. doi:10.3390/metabo12010050
Patton, G. C., Coffey, C., Sawyer, S. M., Viner, R. M., Haller, D. M., Bose, K., et al. (2009). Global patterns of mortality in young people: A systematic analysis of population health data. Lancet 374, 881–892. doi:10.1016/S0140-6736(09)60741-8
Petra, A. I., Panagiotidou, S., Hatziagelaki, E., Stewart, J. M., Conti, P., and Theoharides, T. C. (2015). Gut-microbiota-brain Axis and its effect on neuropsychiatric disorders with suspected immune dysregulation. Clin. Ther. 37, 984–995. doi:10.1016/j.clinthera.2015.04.002
Qi, Z., Wang, R., Liao, R., Xue, S., and Wang, Y. (2021). Neferine ameliorates sepsis-induced myocardial dysfunction through anti-apoptotic and antioxidative effects by regulating the PI3K/AKT/mTOR signaling pathway. Front. Pharmacol. 12, 706251. doi:10.3389/fphar.2021.706251
Rominger, A., Cumming, P., Brendel, M., Xiong, G., Zach, C., Karch, S., et al. (2015). Altered serotonin and dopamine transporter availabilities in brain of depressed patients upon treatment with escitalopram: A [123I]β-CIT SPECT study. Eur. Neuropsychopharmacol. 25, 873–881. doi:10.1016/j.euroneuro.2014.12.010
Rosa, M. A., and Lisanby, S. H. (2012). Somatic treatments for mood disorders. Neuropsychopharmacology 37, 102–116. doi:10.1038/npp.2011.225
Rosa, P. B., Bettio, L. E. B., Neis, V. B., Moretti, M., Werle, I., Leal, R. B., et al. (2019). The antidepressant-like effect of guanosine is dependent on GSK-3β inhibition and activation of MAPK/ERK and Nrf2/heme oxygenase-1 signaling pathways. Purinergic Signal. 15, 491–504. doi:10.1007/s11302-019-09681-2
Salim, S. (2017). Oxidative stress and the central nervous system. J. Pharmacol. Exp. Ther. 360, 201–205. doi:10.1124/jpet.116.237503
Shang, J., Ma, S., Zang, C., Bao, X., Wang, Y., and Zhang, D. (2021). Gut microbiota mediates the absorption of FLZ, a new drug for Parkinson's disease treatment. Acta Pharm. Sin. B 11, 1213–1226. doi:10.1016/j.apsb.2021.01.009
Shi, B., Luo, J., Fang, Y., Liu, X., Rao, Z., Liu, R., et al. (2019). Xiaoyao pills prevent lipopolysaccharide-induced depression by inhibiting inflammation and protecting nerves. Front. Pharmacol. 10, 1324. doi:10.3389/fphar.2019.01324
Song, X., Wang, W., Ding, S., Wang, Y., Ye, L., Chen, X., et al. (2022). Exploring the potential antidepressant mechanisms of puerarin: Anti-inflammatory response via the gut-brain axis. J. Affect. Disord. 310, 459–471. doi:10.1016/j.jad.2022.05.044
Steru, L., Chermat, R., Thierry, B., and Simon, P. (1985). The tail suspension test: A new method for screening antidepressants in mice. Psychopharmacol. Berl. 85, 367–370. doi:10.1007/BF00428203
Sugimoto, Y., Furutani, S., Itoh, A., Tanahashi, T., Nakajima, H., Oshiro, H., et al. (2008). Effects of extracts and neferine from the embryo of Nelumbo nucifera seeds on the central nervous system. Phytomedicine 15, 1117–1124. doi:10.1016/j.phymed.2008.09.005
Sugimoto, Y., Furutani, S., Nishimura, K., Itoh, A., Tanahashi, T., Nakajima, H., et al. (2010). Antidepressant-like effects of neferine in the forced swimming test involve the serotonin1A (5-HT1A) receptor in mice. Eur. J. Pharmacol. 634, 62–67. doi:10.1016/j.ejphar.2010.02.016
Sugimoto, Y., Nishimura, K., Itoh, A., Tanahashi, T., Nakajima, H., Oshiro, H., et al. (2015). Serotonergic mechanisms are involved in antidepressant-like effects of bisbenzylisoquinolines liensinine and its analogs isolated from the embryo of Nelumbo nucifera Gaertner seeds in mice. J. Pharm. Pharmacol. 67, 1716–1722. doi:10.1111/jphp.12473
Takahashi, K., Nakagawasai, O., Nemoto, W., Odaira, T., Sakuma, W., Onogi, H., et al. (2019). Effect of Enterococcus faecalis 2001 on colitis and depressive-like behavior in dextran sulfate sodium-treated mice: Involvement of the brain-gut axis. J. Neuroinflammation 16, 201. doi:10.1186/s12974-019-1580-7
Tan, J., Li, X., Zhu, Y., Sullivan, M. A., Deng, B., Zhai, X., et al. (2022). Antidepressant shugan jieyu capsule alters gut microbiota and intestinal microbiome function in rats with chronic unpredictable mild stress -induced depression. Front. Pharmacol. 13, 828595. doi:10.3389/fphar.2022.828595
Tang, Y. S., Zhao, Y. H., Zhong, Y., Li, X. Z., Pu, J. X., Luo, Y. C., et al. (2019). Neferine inhibits LPS-ATP-induced endothelial cell pyroptosis via regulation of ROS/NLRP3/Caspase-1 signaling pathway. Inflamm. Res. 68, 727–738. doi:10.1007/s00011-019-01256-6
Tao, J., Zhang, S., Kong, L., Zhu, Q., Yao, C., Guo, Q., et al. (2022). Effectiveness and functional magnetic resonance imaging outcomes of Tuina therapy in patients with post-stroke depression: A randomized controlled trial. Front. Psychiatry 13, 923721. doi:10.3389/fpsyt.2022.923721
Tian, T., Mao, Q., Xie, J., Wang, Y., Shao, W. H., Zhong, Q., et al. (2022). Multi-omics data reveals the disturbance of glycerophospholipid metabolism caused by disordered gut microbiota in depressed mice. J. Adv. Res. 39, 135–145. doi:10.1016/j.jare.2021.10.002
Wu, X. L., Deng, M. Z., Gao, Z. J., Dang, Y. Y., Li, Y. C., and Li, C. W. (2020). Neferine alleviates memory and cognitive dysfunction in diabetic mice through modulation of the NLRP3 inflammasome pathway and alleviation of endoplasmic-reticulum stress. Int. Immunopharmacol. 84, 106559. doi:10.1016/j.intimp.2020.106559
Yarandi, S. S., Peterson, D. A., Treisman, G. J., Moran, T. H., and Pasricha, P. J. (2016). Modulatory effects of gut microbiota on the central nervous system: How gut could play a role in neuropsychiatric health and diseases. J. Neurogastroenterol. Motil. 22, 201–212. doi:10.5056/jnm15146
Yeh, K. C., Hung, C. F., Lin, Y. F., Chang, C., Pai, M. S., and Wang, S. J. (2020). Neferine, a bisbenzylisoquinoline alkaloid of Nelumbo nucifera, inhibits glutamate release in rat cerebrocortical nerve terminals through 5-HT(1A) receptors. Eur. J. Pharmacol. 889, 173589. doi:10.1016/j.ejphar.2020.173589
Yin, S., Ran, Q., Yang, J., Zhao, Y., and Li, C. (2020). Nootropic effect of neferine on aluminium chloride-induced Alzheimer's disease in experimental models. J. Biochem. Mol. Toxicol. 34, e22429. doi:10.1002/jbt.22429
Yirmiya, R., Rimmerman, N., and Reshef, R. (2015). Depression as a microglial disease. Trends Neurosci. 38, 637–658. doi:10.1016/j.tins.2015.08.001
Yong, S. J., Tong, T., Chew, J., and Lim, W. L. (2019). Antidepressive mechanisms of probiotics and their therapeutic potential. Front. Neurosci. 13, 1361. doi:10.3389/fnins.2019.01361
Yu, Y. C., Li, J., Zhang, M., Pan, J. C., Yu, Y., Zhang, J. B., et al. (2019). Resveratrol improves brain-gut Axis by regulation of 5-HT-dependent signaling in the rat model of irritable bowel syndrome. Front. Cell. Neurosci. 13, 30. doi:10.3389/fncel.2019.00030
Yun, S., Reynolds, R. P., Masiulis, I., and Eisch, A. J. (2016). Re-evaluating the link between neuropsychiatric disorders and dysregulated adult neurogenesis. Nat. Med. 22, 1239–1247. doi:10.1038/nm.4218
Yun, S., Reynolds, R. P., Petrof, I., White, A., Rivera, P. D., Segev, A., et al. (2018). Stimulation of entorhinal cortex-dentate gyrus circuitry is antidepressive. Nat. Med. 24, 658–666. doi:10.1038/s41591-018-0002-1
Yunes, R. A., Poluektova, E. U., Vasileva, E. V., Odorskaya, M. V., Marsova, M. V., Kovalev, G. I., et al. (2020). A multi-strain potential probiotic formulation of GABA-producing Lactobacillus plantarum 90sk and bifidobacterium adolescentis 150 with antidepressant effects. Probiotics Antimicrob. Proteins 12, 973–979. doi:10.1007/s12602-019-09601-1
Zhang, B., Wang, P. P., Hu, K. L., Li, L. N., Yu, X., Lu, Y., et al. (2019). Antidepressant-like effect and mechanism of action of honokiol on the mouse lipopolysaccharide (LPS) depression model. Molecules 24, E2035. doi:10.3390/molecules24112035
Zhang, W., Qu, W., Wang, H., and Yan, H. (2021). Antidepressants fluoxetine and amitriptyline induce alterations in intestinal microbiota and gut microbiome function in rats exposed to chronic unpredictable mild stress. Transl. Psychiatry 11, 131. doi:10.1038/s41398-021-01254-5
Zhao, H., Jiang, M., and Miao, M. (2022). Pharmacological study on total glycosides of Xiebai protecting against depression model. J. Healthc. Eng. 2022, 5498130. doi:10.1155/2022/5498130
Zheng, P., Zeng, B., Zhou, C., Liu, M., Fang, Z., Xu, X., et al. (2016). Gut microbiome remodeling induces depressive-like behaviors through a pathway mediated by the host's metabolism. Mol. Psychiatry 21, 786–796. doi:10.1038/mp.2016.44
Keywords: neferine, anti-depressant, chronic mild stress, intestinal flora, Lactobacillus, monoamine neurotransmitters
Citation: Dong Z, Xie Q, Xu F, Shen X, Hao Y, Li J, Xu H, Peng Q and Kuang W (2022) Neferine alleviates chronic stress-induced depression by regulating monoamine neurotransmitter secretion and gut microbiota structure. Front. Pharmacol. 13:974949. doi: 10.3389/fphar.2022.974949
Received: 21 June 2022; Accepted: 16 August 2022;
Published: 02 September 2022.
Edited by:
Lucia Gozzo, Gaspare Rodolico Hospital, ItalyReviewed by:
Tahmineh Mokhtari, Chinese Academy of Sciences (CAS), ChinaCopyright © 2022 Dong, Xie, Xu, Shen, Hao, Li, Xu, Peng and Kuang. This is an open-access article distributed under the terms of the Creative Commons Attribution License (CC BY). The use, distribution or reproduction in other forums is permitted, provided the original author(s) and the copyright owner(s) are credited and that the original publication in this journal is cited, in accordance with accepted academic practice. No use, distribution or reproduction is permitted which does not comply with these terms.
*Correspondence: Qiang Peng, cWlhbmdwZW5nenpAc2N1LmVkdS5jbg==; Weihong Kuang, a3doaGxqQHNjdS5lZHUuY24=
Disclaimer: All claims expressed in this article are solely those of the authors and do not necessarily represent those of their affiliated organizations, or those of the publisher, the editors and the reviewers. Any product that may be evaluated in this article or claim that may be made by its manufacturer is not guaranteed or endorsed by the publisher.
Research integrity at Frontiers
Learn more about the work of our research integrity team to safeguard the quality of each article we publish.