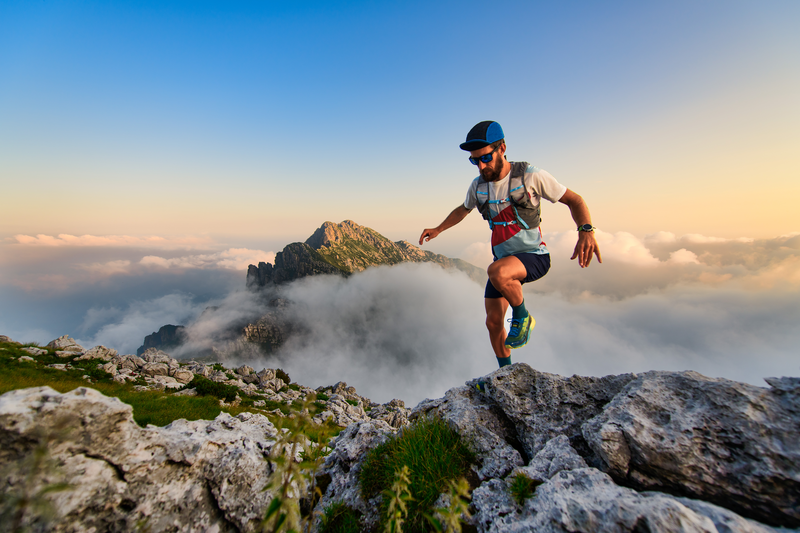
94% of researchers rate our articles as excellent or good
Learn more about the work of our research integrity team to safeguard the quality of each article we publish.
Find out more
ORIGINAL RESEARCH article
Front. Pharmacol. , 22 July 2022
Sec. Respiratory Pharmacology
Volume 13 - 2022 | https://doi.org/10.3389/fphar.2022.960267
This article is part of the Research Topic Coronavirus Disease (COVID-19): Mechanistic Basic, Translational, and Clinical Research Aimed at Identification of Effective Preventive and Therapeutic Strategies View all 5 articles
Background: Similar pathogenesis makes Corona Virus Disease 2019 (COVID-19) associated with rheumatoid arthritis (RA), ankylosing spondylitis (AS) and gouty arthritis (GA), and it is possible to introduce common drugs for the treatment of RA, AS and GA into the treatment of COVID-19. That is, “homotherapy for heteropathy”, especially cytokine inhibitors. But little is known about the specific link between the diseases. In addition, “new use of old drugs” is an important short-term strategy for the treatment of COVID-19. Cepharanthine (CEP), a monomer component of traditional Chinese medicine (TCM), is mainly used in the treatment of leukopenia and has recently been proved to have a good therapeutic effect on COVID-19, but its specific molecular mechanism has not been clearly explained. The purpose of this work is to explore the common targets and signaling pathways among COVID-19, RA, AS, and GA by means of network pharmacology (NP), and to infer the potential mechanism of CEP in the treatment of COVID-19.
Methods: Firstly, SwissTargetPrediction was used to predict the targets of CEP, and the pathogenic targets of COVID-19, RA, AS and GA were searched in GeneCards, OMIM, TTD, PharmGKB database and literature, respectively. Then, the protein interaction network of CEP and COVID-19 cross targets and the common targets of COVID-19, RA, AS and GA was constructed. Cytosscape 3.7.2 software was used to construct CEP-common targets-signaling pathways-COVID-19 network, module function analysis, gene ontology (GO) and kyoto encyclopedia of genes and genomes (KEGG). Finally, the molecular docking of hub targets and CEP was carried out by AutoDock software.
Results: The results showed that the common targets of the four diseases were tumor necrosis factor (TNF), interleukin (IL)-6 and IL-1β, and involved Coronavirus disease, IL-17 signaling pathway and TNF signaling pathway. CEP has a good binding force with AKT Serine/Threonine Kinase 1 (AKT1), phosphatidylinositol 3-kinase (PIK3) CA, PIK3CD and Angiotensin-converting enzyme 2 (ACE2), and plays a role in the treatment of COVID-19 by regulating PI3K-Akt signaling pathway, Relaxin signaling pathway, VEGF signaling pathway and HIF-1 signaling pathway.
Conclusion: Therefore, this study not only confirmed the potential mechanism of CEP in the treatment of COVID-19 at the molecular level, but also found that TNF and IL-17 inhibitors, which are commonly used in the treatment of RA, AS and GA, may also affect the treatment of COVID-19, which provides new clues and theoretical basis for the rapid discovery of effective therapeutic drugs for COVID-19.
“COVID-19” named “Corona Virus Disease 2019” by the World Health Organization (World Health Organization, 2020). Since December 2019, COVID-19 has been prevalent all over the world and has become one of the main causes of death in the world, posing a serious threat to human life and health. It is an infectious disease caused by severe acute respiratory syndrome coronavirus 2 (SARS-CoV-2) and this virus is highly contagious and spreads quickly (Chang et al., 2020; Liu Y.-C. et al., 2020). At present, although the vaccine and drug research for COVID-19 has made continuous progress, it is still a challenging process to effectively manage the development of new drugs for COVID-19. Therefore, the strategy of “new use of old drugs” is put forward to encourage the re-exploration of the use of existing drugs. The literature related to COVID-19 reported that ACE2 is the surface receptor of SARS-CoV-2 prickle glycoprotein and expressed in alveolar epithelial cells, which can promote SARS-CoV-2 to enter and infect host cells (Hoffmann et al., 2020). The interaction between ACE2 and SARS-CoV-2 down-regulates the expression of ACE2 and activates AngⅡ receptor, resulting in increased pulmonary vascular permeability and lung injury (Zhao et al., 2020). Other studies have found that ACE insertion-deletion (I/D) gene polymorphism is also associated with RA and AS. In the study of DD, ID and II alleles, the frequency of D allele in RA patients was higher than that in healthy controls, while DD gene may increase the susceptibility to RA and cause sacral and eye involvement in AS patients (Inanır et al., 2012; Ahmed et al., 2013; Elshazli et al., 2022). Therefore, ACE2 may be related to the pathological process and inflammatory reaction of RA and AS. In addition, COVID-19 is associated with a high inflammatory process. SARS-CoV-2 infection can induce the human body to produce a large number of cytokines, including IL-6, TNF-α and IL-1β, which gather inflammatory cells to the infected site, thereby destroying tissues and organs (Lai et al., 2020). A variety of cytokines and chemokines play a role in the pathogenesis of RA, AS and GA by regulating inflammation, autoimmunity and joint destruction, including IL-1β, IL-6, IL-17, TNF-α, and CXCL8. In arthritis, RA, AS and GA are three common arthritis diseases, which have a high prevalence rate in the population, and they are closely related and can be combined, so they have some similar pathogenesis and treatment. The clinical research on the three diseases is very rich, and the related therapeutic drugs are also being studied and developed continuously. Interestingly, through the analysis of recent related studies, it is not difficult to find that there are some similarities between COVID-19 and RA, AS, GA in pathogenesis, clinical manifestations and drug treatment. Accordingly, the similarity of pathogenesis introduces drugs commonly used in the treatment of RA, AS and GA into COVID-19. At present, a number of clinical studies have been carried out to down-regulate the levels of inflammatory factors in patients with COVID-19, including monoclonal antibodies against IL-6 and IL-1β, and inhibitors of JAK-STAT signaling pathway. For example, IL-6 receptor blocker tocilizumab is mainly used in RA, and it has been proved to be effective in relieving the symptoms of COVID-19 (Favalli et al., 2020). Tofacitinib is a kind of Janus Kinase (JAK) inhibitor, which can block the signal transduction of a variety of inflammatory cytokines. It has a good effect on the treatment of RA, AS and other inflammation-related diseases (Burmester et al., 2013). A tofacitinib study in Brazil of hospitalized patients with COVID-19 showed (Guimarães et al., 2021) that the tofacitinib group (18.1%) had a lower cumulative incidence of death or respiratory failure on day 28 than the placebo group (29.0%). In addition, TNF and IL-17 inhibitors, which have been used clinically for many years, have been widely used in the treatment of RA, AS, GA and other diseases. If new indications of TNF and IL-17 inhibitors can be found, the time period of drug development will be greatly shortened and the success rate of drug discovery for COVID-19 will be improved. In addition, TCM has accumulated more experience in the treatment of epidemic diseases. The treatment characteristics of multi-components, multi-targets and multi-pathways of TCM show the advantages of prevention and treatment of COVID-19, and can complement with western medicine to improve the therapeutic effect. CEP, a monomer component of TCM, is a natural alkaloid extracted from Stephania japonica, which has the effects of anti-inflammation, antioxidation, immune regulation and antivirus (Wang and Yang, 2020). It is used in the treatment of leukopenia. As early as 2020, Tong et al. (Fan et al., 2020) has reported the research results of anti-COVID-19 drugs, and found that CEP is a potential drug for the treatment of SARS-CoV-2 infection. The RNA sequencing revealed the response and antiviral activity of cells to viruses, and confirmed that CEP could reverse most of the dysfunctional genes and pathways in virus-infected cells through HSF1-mediated heat shock reaction, which provided evidence for CEP as a promising therapeutic drug (Li et al., 2021). Since then, a number of research teams in the world have published articles on CEP anti-SARS-CoV-2 in international journals, which shows that CEP has a good anti-novel coronavirus activity (Drayman et al., 2021; Ohashi et al., 2021). Therefore, as an antiviral drug, it has important value in anti-SARS-CoV-2 (Rogosnitzky et al., 2020).
Network Pharmacology (NP) uses database information of drugs, compounds, genes and diseases to construct drug targets, disease targets and signaling pathways interaction network, in order to reveal the complex mechanism of multi-component and multi-target characteristics of TCM, and provides a basis for the transition of TCM from empirical medicine to evidence-based medicine (Hopkins, 2008; Li and Zhang, 2013).
Based on the above research background, this study will explore the common targets and signaling pathways among COVID-19, RA, AS and GA diseases and the potential mechanism of CEP in COVID-19 through the methods of NP (Figure 1), so as to provide a theoretical basis for the exploration of the treatment strategy of “homotherapy for heteropathy” and “new use of old drugs”.
The Canonical SMILES structural formula of CEP is obtained by using PubChem (https://pubchem.ncbi.nlm.nih.gov/) website, and then the structural formula is imported into SwissTargetPrediction (http://www.swisstargetprediction.ch/) website (Daina et al., 2019) to predict and obtain the action targets of CEP, and consult the literature to supplement targets.
The pathogenic genes related to COVID-19 were obtained by using GeneCards (https://www.genecards.org/) (Stelzer et al., 2016), OMIM (https://omim.org/) (Amberger et al., 2015), TTD (https://db.idrblab.org/ttd/) (Wang et al., 2020), PharmGKB (https://www.pharmgkb.org/) (Whirl-Carrillo et al., 2021) database. The key words “COVID-2019” and “Corona Virus Disease 2019” were utilized to screen the COVID-19-associated targets. We established a COVID-19–related gene set by taking a union of the search results. RA, AS and GA related genes were obtained respectively by the same method, and then a data set for COVID-19, RA, AS and GA target genes was established.
By using the online tool of Venny 2.1.0, the targets of four diseases in the combination of multiple diseases (COVID-19, RA, AS, GA) were intersected, and the targets of active compound were cross-located with the targets of COVID-19. Finally, the common targets of the four diseases and the potential targets of CEP in the treatment of COVID-19 were obtained.
A network of interactions between common targets is constructed and analyzed through the STRING (https://string-db.org) database (Szklarczyk et al., 2021), which provides information about predicting and experimenting protein-protein interactions. The results of interaction analysis are imported into Cytoscape 3.7.2 software and the CytoHubba plug-in is used to analyze the connectivity (Degree) of the data in protein-protein interactions (PPI) network to select the hub targets of ranking TOP10.
GO and KEGG enrichment analysis of common targets using Cytoscape 3.7.2 software ClueGO plug-in. The minimum gene overlap was set to three and the p value was less than 0.01. GO enrichment analysis includes three modules: biological process, molecular function and cellular composition. KEGG provides pathways’ functional annotation and enrichment analysis of a given gene. Finally, we get the relevant GO and KEGG data, and sort by p value, showing the results of ranking TOP10 or TOP20.
Module function analysis is based on a complex algorithm for clustering objects with similar attributes. The Analyze Module Functions of Reactome FI (Croft et al., 2011) plug-in of Cytoscape3.7.2 software was used to cluster and analyze the common targets of multi-disease combinations (COVID-19, RA, AS, GA). The results were visualized and output in graphics.
The RCSB PDB (https://www1.rcsb.org/) database is used to retrieve and download the PDB ID of the potential target protein, while the MOL2 structure format of the active compound is downloaded in the TCMSP (http://tcmspw.com/tcmsp.php) (Ru et al., 2014). Then CEP and the hub targets were analyzed by AutoDock Tools software, and CEP ligand and target protein were converted into “PDBQT” format, and the box of appropriate size and position was set. Finally, the molecular docking was carried out by using AutoDock Vina software, the molecular binding activity between target protein and CEP was calculated, and the results of strong binding activity were visually displayed by Pymol software.
Through database search, after removing duplication and combining the search results, a total of 1,118 genes related to single disease (COVID-19) were obtained. After calculation and analysis, the hub targets of TOP10 were CD4, AKT1, INS, ACTB, GAPDH, ALB, IL-1β, IL-6, TP53 and TNF (Figures 2A,B). In the same way, the action targets of RA, AS and GA were obtained, and combined with 1,118 targets of COVID-19, 99 common targets of the four diseases were found (Figure 2A). The hub targets of TOP10 were TNF, IL-6, CD4, IL-1β, IL-10, TLR4, CXCL8, ALB, IFNG, CCL2 (Figure 2B). Compared with the hub targets of single disease (COVID-19), it is found that TNF, IL-6, IL-1β, CD4 and Albumin (ALB) are common (Figure 2B), indicating that these five targets not only play an important role in the common pathogenesis of COVID-19, RA, AS and GA, but also play a core role in the pathogenesis of single disease (COVID-19) and are potential targets for the treatment of COVID-19. After that, GO enrichment analysis was performed. The results showed that the common GO processes of COVID-19, RA, AS and GA mainly includes response to lipopolysaccharide, regulation of inflammatory response and cytokine receptor binding (Figure 2C) and the pathogenesis of COVID-19 was mainly involved in the processes of positive regulation of cytokine production, response to lipopolysaccharide and cytokine receptor binding (Figure 2D). Based on the above results, the common biological processes involved in COVID-19, RA, AS and GA were combined with the results of single disease (COVID-19) analysis. It was found that they jointly regulate the biological processes such as response to lipopolysaccharide, positive regulation of cytokine production and cytokine receptor binding (Figure 2E). Then, 99 common targets were analyzed by module function. The results showed that 99 targets could be aggregated into eight modules, such as Coronavirus disease-COVID-19, Toll-like Receptor Cascades, Toll-like receptor signaling pathway, Rheumatoid arthritis, NF-kappa B signaling pathway, MAPK signaling pathway, TNF signaling pathway, IL-17 signaling pathway, Th17 cell differentiation, which were mainly involved in immune response, inflammatory progression and bacterial and viral expression (Figure 3). Finally, KEGG analysis is carried out. The results showed that the common KEGG pathways of COVID-19, RA, AS and GA mainly includes Coronavirus disease, IL-17 signaling pathway, Th17 cell differentiation, TNF signaling pathway and Toll-like receptor signaling pathway (Figures 4A,B). The pathogenesis of COVID-19 was mainly involved and regulation of multiple pathways such as NOD-like receptor signaling pathway, Toll-like receptor signaling pathway, IL-17 signaling pathway, Coronavirus disease, TNF signaling pathway and HIF-1 signaling pathway (Figures 4C,D). Based on the above results, the common signaling pathways involved in COVID-19, RA, AS and GA were combined with the results of single disease (COVID-19) analysis. It was found that they jointly regulate the Coronavirus disease, TNF signaling pathway and IL-17 signaling pathway (Figure 4E; Table 1). The above results may indicate that these biological processes and signaling pathways are closely related to the pathogenesis of COVID-19, which is a potential way to regulate the progression of COVID-19 and implement the strategy of “new use of old drugs”.
FIGURE 2. The common hub targets and GO process of single disease (COVID-19) and multiple disease combinations (COVID-19, RA, AS, GA). (A) Multi-disease combination (COVID-19, RA, AS, GA) 99 common targets. (B) Single disease (COVID-19) targets ranked TOP10 targets (left), multi-disease combination (COVID-19, RA, AS, GA) common targets ranked TOP10 targets (right), the intersection of the two, five common targets were obtained: TNF, IL-6, IL-1β, CD4 and ALB, these five targets not only play a central role in the pathogenesis and progression of single disease (COVID-19), but also are potential targets for the treatment of RA, AS and GA. (C) The common GO process involved in multiple disease combinations (COVID-19, RA, AS, GA). (D) The GO process involved in single disease (COVID-19). (E) The common GO process obtained by the intersection analysis of single disease (COVID-19) and multiple disease combinations (COVID-19, RA, AS, GA), and mainly involves response to lipopolysaccharide, positive regulation of cytokine production, cytokine receptor binding.
FIGURE 3. Module function analysis of common targets of multiple disease combinations (COVID-19, RA, AS, GA). After the functional analysis of the module, the 99 targets can be aggregated into eight modules, and the circle of each color represents one module, which is mainly related to immune response, inflammatory progression, bacteria and virus expression and other functions. And there is a close relationship between the enriched targets on each module, and also has a certain correlation with the targets on other modules.
FIGURE 4. Common signaling pathways of single disease (COVID-19) and multiple disease combinations (COVID-19, RA, AS, GA). (A,B) The common signaling pathways involved in multiple disease combinations (COVID-19, RA, AS, GA) and the pathways that rank TOP20 among them. (C,D) The signaling pathways regulated by single disease (COVID-19) and the pathways of ranking TOP20. (E) Based on the intersection analysis of TOP20 pathways in single disease (COVID-19) and multiple disease combinations (COVID-19, RA, AS, GA), 12 common regulated signaling pathways were obtained, including Coronavirus disease, TNF signaling pathway, IL-17 signaling pathway, and showed the enriched targets on each pathway. (F–H) Specific signaling transduction pathways of Coronavirus disease, TNF signaling pathway and IL-17 signaling pathway.
CEP is a bisbenzyl isowaline alkaloid, its molecular structure is shown in the figure (Figure 5A). After intersecting with COVID-19-related targets, 22 common targets (Figure 5B) were obtained. These 22 targets may be potential targets of CEP in the treatment of COVID-19. Then 22 targets were analyzed by GO and KEGG, and sorted by p value to show the GO process and signaling pathways of TOP10. The results showed that cell proliferation, cell biological regulation, nucleic acid metabolism and protein transcription and translation were mainly involved in GO processes (Figure 5C), and CEP mainly played a role in the treatment of COVID-19 by regulating PI3K-Akt signaling pathway, Relaxin signaling pathway, VEGF signaling pathway and HIF-1 signaling pathway (Figure 5D; Table 1). Finally, the CEP-targets-signaling pathways-COVID-19 network diagram is drawn by Cytoscape3.7.2 software (Figure 5E), and the hub targets such as AKT1, PIK3CA and PIK3CD are selected according to the network connectivity (Degree). Because SARS-CoV-2 can only enter cells that can express ACE2 and bind to ACE2 through S-protein, and then invade the body to cause disease. ACE2 plays a very important role in the pathogenesis of COVID-19, subsequent molecular docking will increase the analysis between ACE2 and CEP. When CEP is docked with AKT1, PIK3CA, PIK3CD and ACE2, it is generally believed that the binding energy less than −4.25 kcal/mol indicates that there is a certain binding activity between the receptor and the ligand, the binding energy less than −5.0 kcal/mol indicates that the receptor and the ligand have a better binding activity, and the binding energy less than −7.0 kcal/mol indicates a strong binding activity between the receptor and the ligand. The results show that CEP has strong binding activity (Figures 5F–I; Table 2) to these four key proteins, indicating that CEP can effectively act on these targets and indirectly regulate the pathways enriched by the targets to play a corresponding therapeutic role.
FIGURE 5. Potential molecular mechanism of CEP in the treatment of COVID-19. (A) The molecular structure of CEP is a double segment isovaline alkaloid with a molecular formula of C37H38N2O6. (B) Which is the common targets of CEP and COVID-19. (C) Results of GO analysis shows the process of ranking TOP10, which mainly involves cell proliferation, cell biological regulation and protein transcription and translation. (D) Results of KEGG analysis mainly regulate signaling pathways such as PI3K-Akt signaling pathway, Relaxin signaling pathway, VEGF signaling pathway and HIF-1 signaling pathway. (E) CEP-action targets-signaling pathways-COVID-19 association network, purple represents the TCM component CEP, blue represents the action targets, the larger the shape, the more important the target, yellow is the signaling pathways, orange represents the disease COVID-19, showing that CEP has the characteristics of multi-targets and multi-pathways therapy. (F–I) Molecular docking result of CEP and AKT1, PIK3CA, PIK3CD, ACE2.
Syndrome differentiation and treatment is the quintessence of TCM and the basic rule to guide clinical diagnosis and treatment of diseases. It is under the guidance of this principle that “homotherapy for heteropathy” comes into being. “homotherapy for heteropathy” refers to different diseases. When the disease location is the same, the etiology is the same, and the pathogenesis coincides with each other, the same method can be used to treat different diseases. As one of the most basic treatment principles of TCM, it has a very important guiding significance for improving clinical efficacy. At present, symptomatic treatment is mostly used for COVID-19, and there is no specific treatment drug, so “homotherapy for heteropathy” combined with “new use of old drugs” can be used as a potential research and development strategy in theory.
The common targets, module function, biological processes and signaling pathways of multi-disease combinations (COVID-19, RA, AS, GA) were analyzed by the method of NP. In the functional analysis of the module, it can be found that mainly involves Coronavirus disease-COVID-19 and Rheumatoid arthritis disease modules and Toll-like Receptor Cascades, Toll-like receptor signaling pathway, NF-kappa B signaling pathway, MAPK signaling pathway, TNF signaling pathway, IL-17 signaling pathway, Th17 cell differentiation signaling pathway modules (Figure 3). These modules were mainly involved in immune response, inflammatory progression and bacterial and viral expression. Like Toll-like receptor 4 (TLR4) in Toll-like receptor signaling pathway, it is an innate immune receptor on the cell surface that recognizes pathogen-associated molecular pattern (PAMP), including viral proteins, and triggers the production of type I interferon and pro-inflammatory cytokines to fight infection (de Kleijn and Pasterkamp, 2003). TLR4 may play an important role in the pathogenesis of SARS-CoV-2, its overactivation may lead to persistent or excessive innate immune response, and innate immune response is the key to identify and control infection by releasing cytokines and chemokines. It was found that the expression of TLR2 and MyD88 was related to the severity of COVID-19. In terms of mechanism, TLR2 and MyD88 are necessary for inflammatory response induced by β-coronavirus. TLR2 takes SARS-CoV-2 envelope protein as ligand and relies on TLR2 signal to induce the production of inflammatory cytokines during coronavirus infection. In addition, blocking TLR2 signal in vivo can protect the pathogenesis of SARS-CoV-2 infection (Zheng et al., 2021). In addition, the enrichment results showed that the pathogenic processes of RA, AS and GA were all related to the targets of TNF, IL-6, IL-1β and part of the Coronavirus disease signaling pathway (Figure 4G), and there were some common pathogenesis, targets and biological processes with COVID-19. Such as immune disorder, inflammatory storm and response to lipopolysaccharide. Among them, IL-6 targets can not only induce B lymphocytes to proliferate, differentiate and secrete corresponding antibodies, but also promote the proliferation and activation of T lymphocytes, thus participating in anti-infective immunity and autoimmunity (Choy et al., 2020; McConnell et al., 2021). IL-1β is a typical pro-inflammatory cytokine. Inflammatory signals can stimulate the secretion of IL-1β by activating inflammatory bodies. A small amount of IL-1β can produce an appropriate inflammatory response to activate specific immune response and play an immune surveillance role (Apte and Voronov, 2008). Moreover, it involves many regulatory pathways related to immunity and inflammation, such as IL-17 signaling pathway, TNF signaling pathway, Toll-like receptor signaling pathway, and indicates that the etiology and pathogenesis of the four diseases are partly the same, which is the premise of “homotherapy for heteropathy”. After independent analysis of COVID-19, it was found that the pathological process was mainly related to response to lipopolysaccharide process and NOD-like receptor signaling pathway, Toll-like receptor signaling pathway, IL-17 signaling pathway, TNF signaling pathway, HIF-1 signaling pathway and so on. It was confirmed that some of the signaling pathways regulated by multiple disease combinations (COVID-19, RA, AS, GA) could theoretically be used as therapeutic pathways for COVID-19, such as TNF signaling pathway and IL-17 signaling pathway are closely related to viral infection and inflammatory response (Figures 4F,H). Studies have confirmed that TNF is secreted by mononuclear macrophages after activation and transformed into TNF-α after maturation, while TNF-α can inhibit viral replication, block viral protein synthesis and virion production, and has an antiviral effect similar to that of interferon (IFN) (Wong and Goeddel, 1986; Spratte et al., 2018). IL-17A is also a pro-inflammatory cytokine, mainly produced by Th17 cells, but also by innate immune cells and other acquired immune cells (Iwakura et al., 2011). It has been reported that IL-17 is associated with a variety of inflammatory respiratory diseases. The autocrine effect of IL-17 can stimulate human bronchial epithelial and venous endothelial cells to produce chemokines, thus promoting the inflow of neutrophils and aggravating respiratory tract inflammation (Mebratu and Tesfaigzi, 2018; Wang et al., 2019; Casillo et al., 2020). This “unique” factor, highly associated with chronic inflammatory disease, also plays a role in the cardiovascular system (Maione, 2016). The pathological feature of COVID-19 is that active viral replication activates a variety of immune cells and produces a large number of inflammatory cytokines such as TNF, IL-6, IL-1β, IL-17 and IFN-γ, resulting in cytokine storm (Zhou et al., 2020a; Del Valle et al., 2020). The levels of these cytokines are positively correlated with the severity of the disease [Liu, Y. et al. 2019-novel coronavirus (2019-nCoV) infections trigger an exaggerated cytokine response aggravating lung injury ChinaXiv http://www.chinaxiv.org/abs/202002.00018 (2020)] and lead to lung injury by further aggravating clinical features, and the core mediator of this cytokine storm and its destructive consequences may be TNF (Ablamunits and Lepsy, 2022). At present, in the absence of specific antiviral therapy to neutralize COVID-19 pathogens, controlling cytokine storm may be an effective treatment. Interestingly, serum TNF levels in patients with COVID-19 were significantly higher, which was positively correlated with disease severity and death (Del Valle et al., 2020), and the hospitalization rate of COVID-19 patients treated with TNF inhibitors was lower (Gianfrancesco et al., 2020), suggesting that TNF inhibitors have potential for the treatment of COVID-19. In addition, in the process of SARS-CoV-2 infection, Karki et al. (Karki et al., 2021) found that only the combination of TNF-α and IFN-γ can induce inflammatory cell death. Treatment with neutralizing antibodies against TNF-α and IFN-γ can protect mice from SARS-CoV-2 infection and block the signaling pathway of inflammatory cell death mediated by cytokines. While Xu et al. found an increased expression of ACE2 in the skin of patients with COVID-19, and the results of virus detection suggested that the skin was the potential host of SARS-CoV-2. After treatment with IL-17 antibody, the expression of ACE2 was down-regulated, indicating that IL-17 antibody may reduce the risk of virus infection by reducing the number of cells interacting with SARS-CoV-2 (Xu et al., 2021). Therefore, can some TNF-α inhibitors (adalimumab, infliximab and golimumab) and IL-17 inhibitors (secukinumab and ixekizumab) which can be effectively used in the treatment of RA, AS and other diseases also treat COVID-19 and produce new indications? At present, some drugs have been selected for clinical trials to try to be used as therapeutic drugs for COVID-19 (such as NCT04425538, NCT04593940, NCT04403243andetal). Through the method of NP, the above part of the study analyzed the common action targets and regulatory signaling pathways of four diseases: COVID-19, RA, AS and GA, and discussed IL-17 signaling pathway and TNF signaling pathway, which are closely related to viral infection and inflammatory response, indicating that the regulation of these two signaling pathways commonly used in the treatment of RA, AS and other diseases can also affect the pathogenesis of COVID-19. Corresponding factor inhibitors (TNF-α inhibitors and IL-17 inhibitors) may also play a role in the treatment of COVID-19, achieving the treatment strategy of “new use of old drugs” and “homotherapy for heteropathy”. With the continuous report of the results of clinical trials, the effectiveness of this treatment strategy will be gradually confirmed.
In addition to factor inhibitors, TCM also plays an important role in the prevention and treatment of global COVID-19, such as Lian Hua Qing Wen (Zheng et al., 2020; Shi et al., 2021), which is providing experience and wisdom for the international community to prevent and control the epidemic, highlighting the advantages of TCM. Recently, the scientific research team in China has successfully obtained the national invention patent authorization of CEP, the active ingredient of TCM. The patent specification shows that CEP 10uM inhibits SARS-CoV-2 replication by 15,393 times, showing a strong ability to inhibit viruses. In terms of resistance to COVID-19 research, CEP also belongs to the “new use of old drugs”. CEP tablets have been used clinically for several decades, mainly for leukopenia caused by radiotherapy and chemotherapy in tumor patients (Wu et al., 2001). The beneficial effect of CEP on leukopenia was first noted in 1965 and then confirmed in the early 1980s (Oyaizu et al., 2001). This drug can prevent leukopenia, especially neutropenia, in patients treated with anti-cancer drugs. For the clinical treatment of leukopenia, the current general treatment dose is 20 mg each time, three times a day. The possible adverse reactions during application are mild gastrointestinal discomfort, itchy skin, and local skin and nail bed pigmentation in a few cases. Moreover, a large multicenter clinical study has shown that CEP 30 mg is safe and significantly effective in the treatment of radiation-induced leukopenia, protecting white blood cells from radiation damage and having no effect on red blood cell and platelet counts (Kanamori et al., 2016). Judging from the current progress, the research of CEP still stays in the in vitro cell experiment, and whether it is expected to become a therapeutic drug for COVID-19 still needs to go through animal experiments, human clinical trials, market approval and other links. In this study, through the methods of NP and molecular docking, starting from the common action targets of CEP and COVID-19, the enrichment analysis of GO and KEGG was carried out. It was found that CEP interfered the pathogenesis of COVID-19 mainly through the regulation of PI3K-Akt signaling pathway, Relaxin signaling pathway, VEGF signaling pathway and HIF-1 signaling pathway. And the key targets of these important pathways, such as AKT1, PIK3CA, PIK3CD and CEP, all have good binding energy (Figures 5F–H and Table 2), which reveals that CEP treats COVID-19 mainly by acting on these key targets and regulating related pathways. It has been found that SARS-CoV-2 infection can activate PI3K/Akt/mTOR signaling pathway and its downstream targets, resulting in rapid activation of translation mechanisms involved in viral protein synthesis and intracellular phosphorylation (Kindrachuk et al., 2015). In particular, CD147, which is involved in the entry of SARS-CoV-2 into cells, can induce the activation of PI3K/Akt signaling pathway, while the closure of this pathway will inhibit the entry of some viruses (Khezri, 2021). Pulmonary fibrosis is a pathological feature of pulmonary infection in patients with COVID-19. It has been confirmed that Relaxin has the effect of anti-fibrosis and can improve pulmonary symptoms by controlling inflammation and fibrosis (Chow et al., 2012). Simultaneously, Relaxin can also effectively prevent and reverse the ischemia-reperfusion injury in cardiovascular animal model, increase the blood flow of coronary artery and prevent the possible injury of cardiomyocytes caused by COVID-19 (Masini et al., 1996; Bani et al., 1998). In addition, VEGF signaling pathway and HIF-1 signaling pathway are also rich in key targets such as AKT1, PIK3CA and PIK3CD, which may be related to the effect of CEP on COVID-19. In SARS-CoV-2 infection, the increased level of VEGF leads to high permeability, edema and tissue injury, which triggers the pathogenesis of acute lung injury in patients with COVID-19 (Teuwen et al., 2020; Wu and McGoogan, 2020). On the other hand, VEGF signaling pathway increases the level of angiotensin II (Ang II) to promote inflammation, whereas Ang II can increase vascular endothelial growth factor (VEGF), thus forming a vicious circle in the release of inflammatory cytokines (Shang et al., 2019). Finally, it causes the immune cells in the body to secrete a large number of inflammatory factors, form a cytokine storm, attack the immune organs in the human body, seriously affect the respiratory and circulatory systems, and even lead to the death of patients. The inflammatory response of lung can lead to respiratory distress and hypoxia. HIF-1 signaling pathway participates in the regulation of hypoxia in the human body and can activate the transcription of multiple genes under hypoxia conditions. Hypoxia-inducible factor-1 (HIF-1), as one of the hypoxia signal transcription factors (Zhang et al., 2017; Tian et al., 2021), regulates macrophages and T cells by regulating the expression of metabolism-related genes, thereby promoting inflammatory response (Ferraro et al., 2021). Therefore, CEP may play an anti-hypoxia effect by regulating HIF-1 signaling pathway and alleviate the clinical hypoxia symptoms of patients with severe COVID-19. Finally, CEP was docked with ACE2, a key protein in the pathogenesis of COVID-19. It is reported that ACE2 is the receptor protein (Harrison et al., 2020) of respiratory tract, alveolar and vascular endothelial cells. SARS-CoV-2 induces the internalization and exfoliation of ACE2 through the binding of S-protein to ACE2, and then invades the body, leading to the occurrence and development of acute respiratory distress syndrome (Li et al., 2005; Zhou P. et al., 2020). The combination of SARS-CoV-2 and ACE2 is the main cause of COVID-19, and SARS-CoV-2 can only enter cells that can express ACE2. Therefore, ACE2 plays an important role in SARS-CoV-2 infection (Zhang et al., 2020). The results show that CEP and ACE2 have strong binding activity, reaching -12.44 kcal/mol (Figure 5I; Table 2), which provides some data reference and support for deeply analyzing the blood components of CEP to prevent COVID-19. In this part, we explore the action mechanism of CEP through the methods of NP and molecular docking technology, and find that CEP acts on the key targets such as AKT1, PIK3CA, PIK3CD, and ACE2 through PI3K-Akt signaling pathway, Relaxin signaling pathway, VEGF signaling pathway and HIF-1 signaling pathway to achieve the purpose of anti-COVID-19, which lays a foundation for the further study of CEP and drug screening for prevention and treatment of COVID-19.
To sum up, we systematically analyzed the common action targets and signaling pathways of four diseases, including COVID-19, RA, AS and GA, based on the NP and the theory of “homotherapy for heteropathy”, and revealed some commonness in their pathogenesis. It is explained theoretically that the “new use of old drugs” of TNF and IL-17 inhibitors increases the possibility of treating COVID-19 as a new indication. Although there are nearly 100 clinical trials related to COVID-19 at home and abroad, up to now, there are no specific therapeutic drugs, and the research and development of vaccine has its lag. Therefore, this study focused on the relatively safe and commonly used TCM extract CEP, and analyzed the therapeutic target, signal transduction pathway, possible mechanism and molecular docking pathway of CEP in the treatment of COVID-19. The purpose of this study is to provide a reference basis for the clinical application of CEP and to further explore its anti-COVID-19 mechanism, but in view of the limitations of the analysis method, the results need to be further verified.
Publicly available datasets were analyzed in this study. This data can be found here: https://www.genecards.org/http://www, https://pubchem.ncbi.nlm.nih.gov/, http://www.swisstargetprediction.ch/, https://omim.org/, https://db.idrblab.org/ttd/, https://www.pharmgkb.org/.
PJ and JY were responsible for the collection, collation of data, and writing of the original manuscript. MJ and XL were accountable for collection of data. SW and NL were responsible for concept development and manuscript revision. All authors reviewed and accepted the final version of the manuscript.
This work was funded by Shandong Provincial Natural Science Fundation (ZR2019MH044), The Key Project of Shandong Geriatric Association (LKJGG 2021Z009), Xu Zhanwang Shandong Construction Project of Inheritance Studio for Famous and Old TCM Experts [(2019) No. 92].
The authors declare that the research was conducted in the absence of any commercial or financial relationships that could be construed as a potential conflict of interest.
All claims expressed in this article are solely those of the authors and do not necessarily represent those of their affiliated organizations, or those of the publisher, the editors and the reviewers. Any product that may be evaluated in this article, or claim that may be made by its manufacturer, is not guaranteed or endorsed by the publisher.
ACE2, angiotensin-converting enzyme 2; ALB, albumin; Ang II, angiotensin II; AS, ankylosing spondylitis; AKT1, AKT Serine/Threonine Kinase 1; CEP, Cepharanthine; COVID-19, Corona Virus Disease 2019; GA, gouty arthritis; GO, gene ontology; HIF-1, hypoxia-inducible factor-1; IFN, interferon; IL, interleukin; JAK, Janus Kinase; KEGG, kyoto encyclopedia of genes and genomes; NP, network pharmacology; OMIM, Online Mendelian Inheritance in Man; PAMP, pathogen-associated molecular pattern; PIK3, phosphatidylinositol 3-kinase; PPI, protein-protein interactions; RA, rheumatoid arthritis; SARS-CoV-2, severe acute respiratory syndrome coronavirus 2; TCM, traditional Chinese medicine; TTD, Therapeutic Target Database; TCMSP, traditional Chinese medicine systems pharmacology database and analysis platform; TLR4, Toll-like receptor 4; VEGF, vascular endothelial growth factor.
Apte, R. N., and Voronov, E. (2008). Is interleukin-1 a good or bad ‘guy’ in tumor immunobiology and immunotherapy? Immunol. Rev. 222, 222–241. doi:10.1111/j.1600-065X.2008.00615.x
Ablamunits, V., and Lepsy, C. (2022). Blocking TNF signaling may save lives in COVID-19 infection. Mol. Biol. Rep. 49, 2303–2309. doi:10.1007/s11033-022-07166-x
Ahmed, A. Z., El-Shahaly, H. A., Omar, A. S., and Ghattas, M. H. (2013). Patterns of angiotensin converting enzyme insertion/deletion gene polymorphism among an Egyptian cohort of patients with rheumatoid arthritis. Int. J. Rheum. Dis. 16, 284–290. doi:10.1111/j.1756-185X.2012.01820.x
Amberger, J. S., Bocchini, C. A., Schiettecatte, F., Scott, A. F., and Hamosh, A. (2015). OMIM.org: Online Mendelian Inheritance in Man (OMIM®), an online catalog of human genes and genetic disorders. Nucleic Acids Res. 43, D789–D798. doi:10.1093/nar/gku1205
Bani, D., Masini, E., Bello, M. G., Bigazzi, M., and Sacchi, T. B. (1998). Relaxin protects against myocardial injury caused by ischemia and reperfusion in rat heart. Am. J. Pathol. 152, 1367–1376.
Burmester, G. R., Blanco, R., Charles-Schoeman, C., Wollenhaupt, J., Zerbini, C., Benda, B., et al. (2013). Tofacitinib (CP-690, 550) in combination with methotrexate in patients with active rheumatoid arthritis with an inadequate response to tumour necrosis factor inhibitors: A randomised phase 3 trial. Lancet 381, 451–460. doi:10.1016/S0140-6736(12)61424-X
Casillo, G. M., Mansour, A. A., Raucci, F., Saviano, A., Mascolo, N., Iqbal, A. J., et al. (2020). Could IL-17 represent a new therapeutic target for the treatment and/or management of COVID-19-related respiratory syndrome? Pharmacol. Res. 156, 104791. doi:10.1016/j.phrs.2020.104791
Chang, D., Lin, M., Wei, L., Xie, L., Zhu, G., Dela Cruz, C. S., et al. (2020). Epidemiologic and clinical characteristics of novel coronavirus infections involving 13 patients outside wuhan, China. JAMA 323, 1092–1093. doi:10.1001/jama.2020.1623
Chow, B. S. M., Chew, E. G. Y., Zhao, C., Bathgate, R. A. D., Hewitson, T. D., Samuel, C. S., et al. (2012). Relaxin signals through a RXFP1-pERK-nNOS-NO-cGMP-dependent pathway to up-regulate matrix metalloproteinases: The additional involvement of iNOS. PLoS ONE 7, e42714. doi:10.1371/journal.pone.0042714
Choy, E. H., De Benedetti, F., Takeuchi, T., Hashizume, M., John, M. R., Kishimoto, T., et al. (2020). Translating IL-6 biology into effective treatments. Nat. Rev. Rheumatol. 16, 335–345. doi:10.1038/s41584-020-0419-z
Croft, D., O’Kelly, G., Wu, G., Haw, R., Gillespie, M., Matthews, L., et al. (2011). Reactome: A database of reactions, pathways and biological processes. Nucleic Acids Res. 39, D691–D697. doi:10.1093/nar/gkq1018
Daina, A., Michielin, O., and Zoete, V. (2019). SwissTargetPrediction: Updated data and new features for efficient prediction of protein targets of small molecules. Nucleic Acids Res. 47, W357–W364. doi:10.1093/nar/gkz382
de Kleijn, D., and Pasterkamp, G. (2003). Toll-like receptors in cardiovascular diseases. Cardiovasc. Res. 60, 58–67. doi:10.1016/s0008-6363(03)00348-1
Del Valle, D. M., Kim-Schulze, S., Huang, H.-H., Beckmann, N. D., Nirenberg, S., Wang, B., et al. (2020). An inflammatory cytokine signature predicts COVID-19 severity and survival. Nat. Med. 26, 1636–1643. doi:10.1038/s41591-020-1051-9
Drayman, N., DeMarco, J. K., Jones, K. A., Azizi, S.-A., Froggatt, H. M., Tan, K., et al. (2021). Masitinib is a broad coronavirus 3CL inhibitor that blocks replication of SARS-CoV-2. Science 373, 931–936. doi:10.1126/science.abg5827
Elshazli, R. M., Elsaid, A. M., Shawky, D. M., and Barakat, L. A. A. (2022). Genetic polymorphisms of ACE I/D, IL-1β G > A and IL-4 VNTR among Egyptian subjects with rheumatoid arthritis. Arch. Physiol. Biochem. 128, 576–585. doi:10.1080/13813455.2019.1703005
Fan, H.-H., Wang, L.-Q., Liu, W.-L., An, X.-P., Liu, Z.-D., He, X.-Q., et al. (2020). Repurposing of clinically approved drugs for treatment of coronavirus disease 2019 in a 2019-novel coronavirus-related coronavirus model. Chin. Med. J. 133, 1051–1056. doi:10.1097/CM9.0000000000000797
Favalli, E. G., Ingegnoli, F., De Lucia, O., Cincinelli, G., Cimaz, R., Caporali, R., et al. (2020). COVID-19 infection and rheumatoid arthritis: Faraway, so close. Autoimmun. Rev. 19, 102523. doi:10.1016/j.autrev.2020.102523
Ferraro, E., Germanò, M., Mollace, R., Mollace, V., and Malara, N. (2021). HIF-1, the warburg effect, and macrophage/microglia polarization potential role in COVID-19 pathogenesis. Oxid. Med. Cell. Longev. 2021, 8841911. doi:10.1155/2021/8841911
Gianfrancesco, M., Hyrich, K. L., Al-Adely, S., Carmona, L., Danila, M. I., Gossec, L., et al. (2020). Characteristics associated with hospitalisation for COVID-19 in people with rheumatic disease: Data from the COVID-19 global rheumatology alliance physician-reported registry. Ann. Rheum. Dis. 79, 859–866. doi:10.1136/annrheumdis-2020-217871
Guimarães, P. O., Quirk, D., Furtado, R. H., Maia, L. N., Saraiva, J. F., Antunes, M. O., et al. (2021). Tofacitinib in patients hospitalized with covid-19 pneumonia. N. Engl. J. Med. 385, 406–415. doi:10.1056/NEJMoa2101643
Harrison, A. G., Lin, T., and Wang, P. (2020). Mechanisms of SARS-CoV-2 transmission and pathogenesis. Trends Immunol. 41, 1100–1115. doi:10.1016/j.it.2020.10.004
Hoffmann, M., Kleine-Weber, H., Schroeder, S., Krüger, N., Herrler, T., Erichsen, S., et al. (2020). SARS-CoV-2 cell entry depends on ACE2 and TMPRSS2 and is blocked by a clinically proven protease inhibitor. Cell. 181, 271–280. e8. doi:10.1016/j.cell.2020.02.052
Hopkins, A. L. (2008). Network pharmacology: The next paradigm in drug discovery. Nat. Chem. Biol. 4, 682–690. doi:10.1038/nchembio.118
Inanır, A., Yigit, S., Tural, S., Ozturk, S. D., Akkanet, S., Habiboğlu, A., et al. (2012). Significant association between insertion/deletion polymorphism of the angiotensin-convertig enzyme gene and ankylosing spondylitis. Mol. Vis. 18, 2107–2113.
Iwakura, Y., Ishigame, H., Saijo, S., and Nakae, S. (2011). Functional specialization of interleukin-17 family members. Immunity 34, 149–162. doi:10.1016/j.immuni.2011.02.012
Kanamori, S., Hiraoka, M., Fukuhara, N., Oizumi, Y., Danjo, A., Nakata, K., et al. (2016). Clinical efficacy of cepharanthin(r)for radiotherapy-induced leukopenia - a nationwide, multicenter, and observational study. Gan Kagaku Ryoho. 43, 1075–1079.
Karki, R., Sharma, B. R., Tuladhar, S., Williams, E. P., Zalduondo, L., Samir, P., et al. (2021). Synergism of TNF-α and IFN-γ triggers inflammatory cell death, tissue damage, and mortality in SARS-CoV-2 infection and cytokine shock syndromes. Cell. 184, 149–168.e17. e17. doi:10.1016/j.cell.2020.11.025
Khezri, M. R. (2021). PI3K/AKT signaling pathway: A possible target for adjuvant therapy in COVID-19. Hum. Cell. 34, 700–701. doi:10.1007/s13577-021-00484-5
Kindrachuk, J., Ork, B., Hart, B. J., Mazur, S., Holbrook, M. R., Frieman, M. B., et al. (2015). Antiviral potential of ERK/MAPK and PI3K/AKT/mTOR signaling modulation for Middle East respiratory syndrome coronavirus infection as identified by temporal kinome analysis. Antimicrob. Agents Chemother. 59, 1088–1099. doi:10.1128/AAC.03659-14
Lai, Q., Spoletini, G., Bianco, G., Graceffa, D., Agnes, S., Rossi, M., et al. (2020). SARS‐CoV2 and immunosuppression: A double‐edged sword. Transpl. Infect. Dis. 22, e13404. doi:10.1111/tid.13404
Li, F., Li, W., Farzan, M., and Harrison, S. C. (2005). Structure of SARS coronavirus spike receptor-binding domain complexed with receptor. Science 309, 1864–1868. doi:10.1126/science.1116480
Li, S., Liu, W., Chen, Y., Wang, L., An, W., An, X., et al. (2021). Transcriptome analysis of cepharanthine against a SARS-CoV-2-related coronavirus. Brief. Bioinform. 22, 1378–1386. doi:10.1093/bib/bbaa387
Li, S., and Zhang, B. (2013). Traditional Chinese medicine network pharmacology: Theory, methodology and application. Chin. J. Nat. Med. 11, 110–120. doi:10.1016/S1875-5364(13)60037-0
Liu, Y.-C., Kuo, R.-L., and Shih, S.-R. (2020). COVID-19: The first documented coronavirus pandemic in history. Biomed. J. 43, 328–333. doi:10.1016/j.bj.2020.04.007
Liu, Y., Zhang, C., Huang, F., Yang, Y., Wang, F., Yuan, J., et al. (2020). 2019-novel coronavirus (2019-nCoV) infections trigger an exaggerated cytokine response aggravating lung injury. ChinaXiv Available at: http://www.chinaxiv.org/abs/202002.00018 (Accessed February 12, 2020). doi:10.12074/202002.00018
Maione, F. (2016). Commentary: IL-17 in chronic inflammation: From discovery to targeting. Front. Pharmacol. 7, 250. doi:10.3389/fphar.2016.00250
Masini, E., Salvemini, D., Mugnai, L., Bello, M. G., Bani, D., Mannaioni, P. F., et al. (1996). The effect of relaxin on myocardial ischaemia-reperfusion injury and histamine release in vitro and in vivo. Inflamm. Res. 45 (Suppl. 1), S27–S28. doi:10.1007/BF03354073
McConnell, M. J., Kawaguchi, N., Kondo, R., Sonzogni, A., Licini, L., Valle, C., et al. (2021). Liver injury in COVID-19 and IL-6 trans-signaling-induced endotheliopathy. J. Hepatol. 75, 647–658. doi:10.1016/j.jhep.2021.04.050
Mebratu, Y. A., and Tesfaigzi, Y. (2018). IL-17 plays a role in respiratory syncytial virus–induced lung inflammation and emphysema in elastase and LPS-injured mice. Am. J. Respir. Cell. Mol. Biol. 58, 717–726. doi:10.1165/rcmb.2017-0265OC
Ohashi, H., Watashi, K., Saso, W., Shionoya, K., Iwanami, S., Hirokawa, T., et al. (2021). Potential anti-COVID-19 agents, cepharanthine and nelfinavir, and their usage for combination treatment. iScience 24, 102367. doi:10.1016/j.isci.2021.102367
Oyaizu, H., Adachi, Y., Yasumizu, R., Ono, M., Ikebukuro, K., Fukuhara, S., et al. (2001). Protection of T cells from radiation-induced apoptosis by Cepharanthin®. Int. Immunopharmacol. 1, 2091–2099. doi:10.1016/S1567-5769(01)00127-8
Rogosnitzky, M., Okediji, P., and Koman, I. (2020). Cepharanthine: A review of the antiviral potential of a Japanese-approved alopecia drug in COVID-19. Pharmacol. Rep. 72, 1509–1516. doi:10.1007/s43440-020-00132-z
Ru, J., Li, P., Wang, J., Zhou, W., Li, B., Huang, C., et al. (2014). TCMSP: A database of systems pharmacology for drug discovery from herbal medicines. J. Cheminform. 6, 13. doi:10.1186/1758-2946-6-13
Shang, J., He, Q., Chen, Y., Yu, D., Sun, L., Cheng, G., et al. (2019). miR‐15a‐5p suppresses inflammation and fibrosis of peritoneal mesothelial cells induced by peritoneal dialysis via targeting VEGFA. J. Cell. Physiol. 234, 9746–9755. doi:10.1002/jcp.27660
Shi, M., Peng, B., Li, A., Li, Z., Song, P., Li, J., et al. (2021). Broad anti-viral capacities of lian-hua-qing-wen capsule and jin-hua-qing-Gan granule and rational use against COVID-19 based on literature mining. Front. Pharmacol. 12, 640782. doi:10.3389/fphar.2021.640782
Spratte, J., Princk, H., Schütz, F., Rom, J., Zygmunt, M., Fluhr, H., et al. (2018). Stimulation of chemokines in human endometrial stromal cells by tumor necrosis factor-α and interferon-γ is similar under apoptotic and non-apoptotic conditions. Arch. Gynecol. Obstet. 297, 505–512. doi:10.1007/s00404-017-4586-3
Stelzer, G., Rosen, N., Plaschkes, I., Zimmerman, S., Twik, M., Fishilevich, S., et al. (2016). The GeneCards suite: From gene data mining to disease genome sequence analyses. Curr. Protoc. Bioinforma. 54, 1.30.1–3. doi:10.1002/cpbi.5
Szklarczyk, D., Gable, A. L., Nastou, K. C., Lyon, D., Kirsch, R., Pyysalo, S., et al. (2021). The STRING database in 2021: Customizable protein–protein networks, and functional characterization of user-uploaded gene/measurement sets. Nucleic Acids Res. 49, D605–D612. doi:10.1093/nar/gkaa1074
Teuwen, L.-A., Geldhof, V., Pasut, A., and Carmeliet, P. (2020). COVID-19: The vasculature unleashed. Nat. Rev. Immunol. 20, 389–391. doi:10.1038/s41577-020-0343-0
Tian, M., Liu, W., Li, X., Zhao, P., Shereen, M. A., Zhu, C., et al. (2021). HIF-1α promotes SARS-CoV-2 infection and aggravates inflammatory responses to COVID-19. Signal Transduct. Target. Ther. 6, 308. doi:10.1038/s41392-021-00726-w
Wang, L., Liu, J., Wang, W., Qi, X., Wang, Y., Tian, B., et al. (2019). Targeting IL-17 attenuates hypoxia-induced pulmonary hypertension through downregulation of β-catenin. Thorax 74, 564–578. doi:10.1136/thoraxjnl-2018-211846
Wang, Y., Zhang, S., Li, F., Zhou, Y., Zhang, Y., Wang, Z., et al. (2020). Therapeutic target database 2020: Enriched resource for facilitating research and early development of targeted therapeutics. Nucleic Acids Res. 48, D1031–D1041. doi:10.1093/nar/gkz981
Wang, Z., and Yang, L. (2020). Turning the tide: Natural products and natural-product-inspired chemicals as potential counters to SARS-CoV-2 infection. Front. Pharmacol. 11, 1013. doi:10.3389/fphar.2020.01013
Whirl-Carrillo, M., Huddart, R., Gong, L., Sangkuhl, K., Thorn, C. F., Whaley, R., et al. (2021). An evidence-based framework for evaluating pharmacogenomics knowledge for personalized medicine. Clin. Pharmacol. Ther. 110, 563–572. doi:10.1002/cpt.2350
Wong, G. H. W., and Goeddel, D. V. (1986). Tumour necrosis factors α and β inhibit virus replication and synergize with interferons. Nature 323, 819–822. doi:10.1038/323819a0
World Health Organization (2020). WHO Novel coronavirus (2019-nCoV) situation reports. Available at: https://www.who.int/emergencies/diseases/novel-coronavirus-2019/situation-reports (accessed Sep 7, 2020).
Wu, J., Suzuki, H., Zhou, Y.-W., Liu, W., Yoshihara, M., Kato, M., et al. (2001). Cepharanthine activates caspases and induces apoptosis in Jurkat and K562 human leukemia cell lines. J. Cell. Biochem. 82, 200–214. doi:10.1002/jcb.1155
Wu, Z., and McGoogan, J. M. (2020). Characteristics of and important lessons from the coronavirus disease 2019 (COVID-19) outbreak in China: Summary of a report of 72 314 cases from the Chinese center for disease control and prevention. JAMA 323, 1239–1242. doi:10.1001/jama.2020.2648
Xu, Q., Chen, L., Li, X., and Zheng, J. (2021). If skin is a potential host of SARS-CoV-2, IL-17 antibody could reduce the risk of COVID-19. J. Am. Acad. Dermatol. 84, e173. doi:10.1016/j.jaad.2020.10.084
Zhou, P., Yang, X.-L., Wang, X.-G., Hu, B., Zhang, L., Zhang, W., et al. (2020b). A pneumonia outbreak associated with a new coronavirus of probable bat origin. Nature 579, 270–273. doi:10.1038/s41586-020-2012-7
Zhang, L., Lin, D., Sun, X., Curth, U., Drosten, C., Sauerhering, L., et al. (2020). Crystal structure of SARS-CoV-2 main protease provides a basis for design of improved α-ketoamide inhibitors. Science 368, 409–412. doi:10.1126/science.abb3405
Zhang, Q., Yu, H., Qi, J., Tang, D., Chen, X., Wan, J., et al. (2017). Natural formulas and the nature of formulas: Exploring potential therapeutic targets based on traditional Chinese herbal formulas. PLoS ONE 12, e0171628. doi:10.1371/journal.pone.0171628
Zhao, Y., Zhao, Z., Wang, Y., Zhou, Y., Ma, Y., Zuo, W., et al. (2020). Single-cell RNA expression profiling of ACE2, the receptor of SARS-CoV-2. Am. J. Respir. Crit. Care Med. 202, 756–759. doi:10.1164/rccm.202001-0179LE
Zheng, M., Karki, R., Williams, E. P., Yang, D., Fitzpatrick, E., Vogel, P., et al. (2021). TLR2 senses the SARS-CoV-2 envelope protein to produce inflammatory cytokines. Nat. Immunol. 22, 829–838. doi:10.1038/s41590-021-00937-x
Zheng, S., Baak, J. P., Li, S., Xiao, W., Ren, H., Yang, H., et al. (2020). Network pharmacology analysis of the therapeutic mechanisms of the traditional Chinese herbal formula Lian Hua Qing Wen in Corona virus disease 2019 (COVID-19), gives fundamental support to the clinical use of LHQW. Phytomedicine 79, 153336. doi:10.1016/j.phymed.2020.153336
Keywords: COVID-19, cepharanthine, network pharmacology, new use of old drugs, homotherapy for heteropathy
Citation: Jiang P, Ye J, Jia M, Li X, Wei S and Li N (2022) The common regulatory pathway of COVID-19 and multiple inflammatory diseases and the molecular mechanism of cepharanthine in the treatment of COVID-19. Front. Pharmacol. 13:960267. doi: 10.3389/fphar.2022.960267
Received: 02 June 2022; Accepted: 30 June 2022;
Published: 22 July 2022.
Edited by:
Paola Patrignani, University of Studies G. d'Annunzio Chieti and Pescara, ItalyReviewed by:
Xinghua Qin, Northwestern Polytechnical University, ChinaCopyright © 2022 Jiang, Ye, Jia, Li, Wei and Li. This is an open-access article distributed under the terms of the Creative Commons Attribution License (CC BY). The use, distribution or reproduction in other forums is permitted, provided the original author(s) and the copyright owner(s) are credited and that the original publication in this journal is cited, in accordance with accepted academic practice. No use, distribution or reproduction is permitted which does not comply with these terms.
*Correspondence: Shujun Wei, MjgyNTg3ODEzQHFxLmNvbQ==; Nianhu Li, dGlnZXJsZWUwNzNAMTI2LmNvbQ==
†These authors have contributed equally to this work
Disclaimer: All claims expressed in this article are solely those of the authors and do not necessarily represent those of their affiliated organizations, or those of the publisher, the editors and the reviewers. Any product that may be evaluated in this article or claim that may be made by its manufacturer is not guaranteed or endorsed by the publisher.
Research integrity at Frontiers
Learn more about the work of our research integrity team to safeguard the quality of each article we publish.