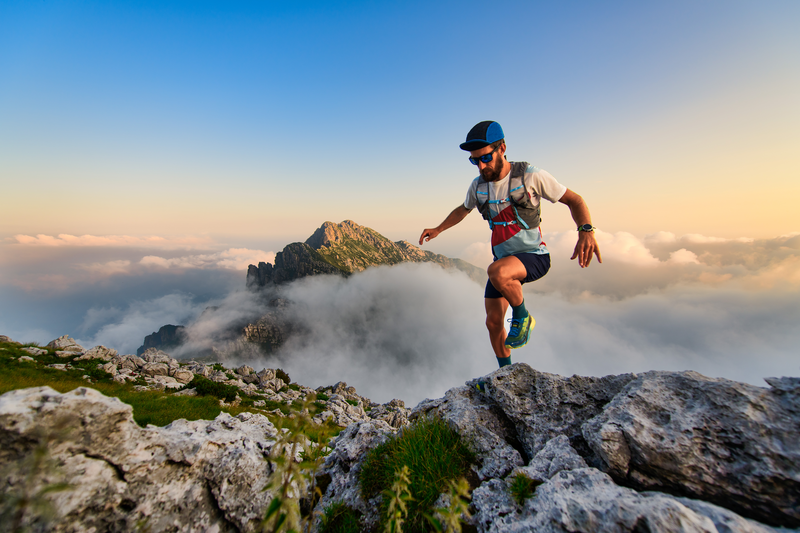
94% of researchers rate our articles as excellent or good
Learn more about the work of our research integrity team to safeguard the quality of each article we publish.
Find out more
ORIGINAL RESEARCH article
Front. Pharmacol. , 26 August 2022
Sec. Predictive Toxicology
Volume 13 - 2022 | https://doi.org/10.3389/fphar.2022.958204
This article is part of the Research Topic Toxicity Profiling of Natural Products and Druggable Compounds: Where Are We Now? View all 20 articles
Iodoacetic acid (IAA) is one of the most common water disinfection byproducts (DBPs). Humans and animals are widely and continuously exposed to it. Many species of water DBPs are harmful to the reproductive system of organisms. Nevertheless, the potential effects of IAA exposure on testosterone and spermatogenesis in vivo remain ambiguous. Spermatogenous cells are the site of spermatogenesis, Leydig cells are the site of testosterone synthesis, and Sertoli cells build the blood–testis barrier (BTB), providing a stable environment for the aforementioned important physiological functions in testicular tissue. Therefore, we observed the effects of IAA on spermatogenic cells, Leydig cells, and Sertoli cells in the testis. In this study, we found that oral administration of IAA (35 mg/kg body weight per day for 28 days) in male mice increased serum LH levels and reduced sperm motility, affecting average path velocity and straight line velocity of sperm. In addition, IAA promoted the expression of γH2AX, a marker for DNA double-strand breaks. Moreover, IAA downregulated the protein expression of the scavenger receptor class B type 1 (SRB1), and decreased lipid droplet transport into Leydig cells, which reduced the storage of testosterone synthesis raw materials and might cause a drop in testosterone production. Furthermore, IAA did not affect the function of BTB. Thus, our results indicated that IAA exposure affected spermatogenesis and testosterone synthesis by inducing DNA damage and reducing lipid droplet transport.
In recent years, male fertility has generally shown a downward trend (Agarwal et al., 2021), and the effect of endocrine disruptors is one of the reasons (Kahn et al., 2020). There are many types of endocrine disruptors, and water disinfection by-products (DBPs) are one of them. DBPs are chemical contaminants formed by the reaction between organic matter and disinfectants. Research proves that water DBPs disrupt ovarian function and spermatogenesis, and produce adverse reproductive outcomes (Gonsioroski et al., 2020a) because many species of these are cytotoxic, neurotoxic, genotoxic, carcinogenic, and teratogenic (Kali et al., 2021).
Haloacetic acid disinfection by-products (HAA-DBPs) are a class of water DBPs. Iodoacetic acid (IAA) is one type of HAA-DBPs. It is also used in the research of organic synthesis, dye industry, and plant resources, where people are exposed to a large dose of poisoning environment. Research has shown that IAA is the most genotoxic of all HAA-DBPs studied to date (Dong et al., 2019), which was more cytotoxic and genotoxic than their chlorinated and brominated analogues in primary human lymphocytes (Escobar-Hoyos et al., 2013). After inoculation into BALB/C nude mice, IAA transformed NIH3T3 cells into tumorigenic lines and formed aggressive fibro sarcomas, which demonstrated that it had a biological activity consistent with a carcinogen (Wei et al., 2013). IAA potentially disrupted the thyroid endocrine system by down-regulating the mRNA and protein expression levels of the thyrotropin receptor (TSHR) and the sodium/iodide symporter (NIS) (Xia et al., 2018). Moreover, IAA reduced cell viability significantly in the mouse primary hepatocytes (Wang et al., 2018).
For the adult female mice, IAA, as a hypothalamic–pituitary–gonadal axis toxicant, affected the pituitary directly and increased mRNA levels of kisspeptin (Kiss1) significantly in the arcuate nucleus (Gonzalez et al., 2021). Research demonstrated that IAA had reproductive and developmental toxicity (Long et al., 2021). Another study reported that IAA exhibited the most potent estrogenic activity (Kim et al., 2020). In vivo, IAA altered estrous cyclicity, ovarian gene expression, and estradiol levels in mice (Gonsioroski et al., 2021). Meanwhile, IAA inhibited follicle growth, decreased cell proliferation, and altered steroidogenesis in vitro (Jeong et al., 2016; Gonsioroski et al., 2020b). In the Comet assay, IAA resulted in DNA damage in sperm (Ali et al., 2014). However, the effect of IAA on the testis in vivo is not yet fully understood. Particularly, its effect on spermatogenesis and testosterone synthesis has not been elucidated.
To observe the effects of IAA on the male reproductive system and to test the mechanism, we constructed a model of IAA exposure by gavage and observed several common indicators of Leydig cells, Sertoli cells, and spermatogenic cells from the gene expression and protein levels. For spermatogenic cells, γH2AX, DAZL, and VASA were observed; for Leydig cells, scavenger receptor class B type 1 (SRB1), steroidogenic acute regulatory protein (STAR), P450 side-chain cleavage enzyme (CYP11A1), and cytochrome P450 17A1 (CYP17A1) were detected; and for Sertoli cells, WT-1, N-cadherin, and β-catenin were recorded. In addition, the levels of FSH, LH, and testosterone in the mouse serum were also determined.
IAA was purchased from Sigma-Aldrich (Shanghai Warehouse, China). Antibodies for STAR (8449s) and CYP11A1 (14217s) were procured from Cell Signaling Technology, Inc. Antibody for CYP17A1 (14447-1-AP) was purchased from Wuhan SANYING Proteintech (Wuhan Warehouse, China). The other antibodies were obtained from Abcam (Shanghai, China) including DAZL (ab34139), VASA (ab13840), γH2AX (ab81299), SRB1 (ab217318), Wilms tumor-1 (WT-1) (ab89901), N-cadherin (ab18203), β-catenin (ab16051), and tubulin (ab7291).
All animals used in the experiment were male 8 -week-old C57BL/6 mice, purchased from Vital River Laboratory Animal Technology Co., Ltd. (Beijing, China). They were randomly divided into two groups, with free access to water and food. After 1 week of acclimatization, the control group (8 mice) received treatment via gavage with a matching volume of double distilled H2O, and the experiment group (10 mice) was administered IAA at 35 mg/kg via oral gavage daily. The dosage and duration of IAA were referred to the previous literature (Xia et al., 2018). All mice were adapted to a 12-h light/dark cycle at 22–25°C. They were weighed twice a week, and doses were adjusted according to their bodyweight. After treatment for 28 days, all the mice were anesthetized, the blood was collected, and the caudal part of the epididymis was used for the evaluation of sperm parameters. Then the testis samples were removed quickly for the subsequent analyses. One testis was fixed in Bouin’s solution for the histopathological examination and immunofluorescent staining, and the other testis was frozen in liquid nitrogen and stored at −80°C until used for the assessment of mRNA and protein expression.
All animal experiments were approved by the Animal Ethics Committee of Shandong Provincial Hospital and performed according to the Shandong Provincial Hospital Animal Care and Use Committee.
The level of luteinizing hormone (LH) in the serum was measured by the ELISA kit from CLOUD-CLONE CORP. (Wuhan, China). Testosterone and follicle stimulating hormone (FSH) in the serum were measured by the ELISA kit from Cusabio (Wuhan, China). The operating procedure was strictly in accordance with the kit instructions.
The cauda part of the right epididymis of each mouse was collected and cut into pieces with scissors to release sperms into an M199 culture medium (containing 1% BSA). The cultures were maintained in a humidified chamber (37°C, 5% CO2 incubator) for 5 min. A total of one hundred microliters of each sample was taken and diluted four times. Then thirty microliters of the sample were used for the following assay. Five pictures were taken per sample. Then the data were quantified by computer-aided sperm analysis (CASA, Hamilton-Thorne, Shanghai, China), and the appurtenant software was used to assess sperm concentration, motility, progressive, quantity, average path velocity, straight line velocity, and curvilinear velocity.
The testis was immersed in Bouin’s fluid fixative for 24 h, transferred to 70% ethanol, and placed into an automated tissue processor for gradient ethanol dehydration. The samples were embedded in paraffin wax and cut into 3 μm sections. Then the testicular specimens were deparaffinized with xylene and ethanol, stained with hematoxylin and eosin, dehydrated, and mounted. Afterward, the morphological changes of the testis were observed under a microscope to complete the image collection and analysis.
Total RNA was extracted from testicular tissue (10 mg) with TRIzol reagent (Takara, Tokyo, Japan) according to the manufacturer’s instructions. A Prime-Script RT Reagent Kit (Takara, Japan) was used to reverse-transcribe RNA into cDNA, and SYBR Premix Ex Taq (Takara, Japan) was used to perform a quantitative real-time polymerase chain reaction (PCR) utilizing a Thermal Cycler Dice Real-Time System (Takara, Japan). The program used to analyze the abundance of different genes was 95°C for 5 min, 40 cycles of 95°C for 10 s, 60°C for 10 s, and 72°C for 10 s, followed by a melting curve from 95 to 60°C, and cool to 37°C for 10 s. β-actin was employed as an endogenous control to normalize the data, and the 2-ΔΔCt calculation method was employed to analyze them. The qPCR primers used are listed in Supplementary Table S1.
Mouse testicular fragments were lysed with ice-cold radioimmunoprecipitation assay buffer (RIPA buffer) supplemented with protease and phosphatase inhibitors (Shenergy Biocolor Bioscience & Technology Company, Shanghai, China), subjected to ultrasound pyrolysis, and centrifuged at 15,000 g for 15 min. Total protein was quantified using the BCA protein assay. After denaturation, proteins were separated by sodium dodecyl sulphate-polyacrylamide gel electrophoresis (SDS-PAGE) on 10%–12% polyacrylamide gels under reducing conditions. Separated proteins were electro transferred onto a polyvinylidene difluoride membrane (Millipore, Billerica, MA, United States) and blocked-in with 5% non-fat powdered milk containing 0.1% Tween 20 for 1 h. Next, to detect γH2AX, VASA, DAZL, SRB1, STAR, CYP17A1, CYP11A1, WT-1, N-cadherin, and β-catenin proteins (antibodies listed in Supplementary Table S2), the membrane was incubated with the respective primary antibodies at 4°C overnight. Thereafter, the membranes were washed briefly with TBST, incubated in the anti-rabbit or anti-mouse IgG secondary antibody conjugated to horseradish peroxidase (1:5000) for 1 h at room temperature, and visualized by a HyGLO HRP detection kit (Denville, NJ, United States). Protein expression levels were quantified with Fluor Chem Q SA software.
For fluorescent microscopy, paraffin sections of the testis were deparaffinized with xylene, hydrated with a series of alcohol solutions, and washed three times in phosphate-buffered saline (PBS). The specimens were immersed in EDTA antigen repair buffer and heated in a microwave oven for 25 min. Then they were cooled for 60 min, washed three times with PBS, and incubated with 5% donkey serum for 60 min at room temperature. Afterward, the slides were incubated with their respective primary antibodies overnight. The next day, the sections were washed three times with PBST and then incubated with FITC-conjugated donkey anti-rabbit IgG (1:1000; Thermo Fisher) for 1 h at room temperature. After a final wash in PBST, nuclei were stained with DAPI, the slides were visualized under a fluorescence microscope, and the photos were taken and analyzed.
All data were analyzed with SPSS 25.0 and were expressed as the mean ± standard error of the mean (SEM). Outliers were removed by the ROUT’s test using GraphPad outlier calculator software. Means were compared using unpaired Student’s t-test for comparisons between two groups, and a two‐tailed value of p < 0.05 was considered statistically significant.
Compared with the control group, a slight decrease in body weight was observed after the IAA exposure for 28 days (Figures 1A,B). The relative weight of testis was calculated by testes weight (mg)/body weight (g), but there were no significant reductions in the testis weight and its relative weight between two groups (Figures 1C,D). As shown in Figure 1E, no evidence of atrophy and vacuoles was seen in the experimental group. The testis tissue presented a normal testicular morphology and complete seminiferous tubules, and also possessed different stages of spermatogonium, spermatocyte, and spermatozoa in the seminiferous tubules. IAA did not alter the pathology and morphology of the testicular tissues. To clarify the effect of IAA exposure on sex hormones, serum FSH, LH, and testosterone levels were detected. There was no significant difference in the FSH level between the two groups (Figure 1F). However, a significant increase in the LH level was observed versus control (p = 0.020, Figure 1G). Although there was no significant reduction in the testosterone level, a descending trend was observed compared with the control group (p = 0.166, Figure 1H).
FIGURE 1. Effects of IAA exposure on general condition and serum hormone levels in mice. During the IAA exposure, the body weights of the mice were recorded twice a week. Only weekly values are shown in the graph (A). Pictures of mice and testis (B), testicular weight (C), and the relative weight of testis (D) between the two groups were recorded after sacrifice. Hematoxylin-eosin (H&E) sections of testicular tissue of mice were also recorded and the pathological morphology was observed (E). After IAA exposure, serum FSH, LH, and testosterone levels of mice were detected by ELISA (F–H). Data are presented as the mean ± SEM. * Significant differences compared to control (p < 0.05).
To observe the effect of IAA exposure on sperm motility, various indicators of sperm motility and concentration were tested (as listed in Table 1). As observed in our results, there were no significant changes in the following sperm parameters, such as sperm concentration, motile, progressive, and curvilinear velocity (VCL). But the average path velocity (VAP) and straight line velocity (VSL) of sperm decreased significantly compared with the control group. We also tested the sperm ratios as rapid, medium, slow, and static. There were no statistical differences in these indicators between the two groups; however, there was a clear increasing trend in the proportion of slow motile sperm.
Spermatogenesis is an important physiological function of testicular tissue. To observe the effects of IAA exposure on spermatogenic cells, the gene expression and protein levels of relevant indicators during spermatogenesis were examined. As shown in Figure 2A, no significant changes were observed in the spermatogenesis indicators Dazl, Vasa, Plzf, c-Kit, Stra8, Sycp1, Sycp3, Tnp2, and Piwil1 at the mRNA level between the two groups. But IAA treatment significantly increased γH2AX expression compared to no treatment. All seminiferous tubules contain γH2AX spermatocytes at respective steps in development. Meanwhile, γH2AX is a marker of the DNA damage response and replication stress. Although IAA exposure did not change the protein levels of VASA and DAZL compared to the control (Figures 2B,C,F,G), the protein level of γH2AX was observed increasingly by Western Blot (WB) and immunofluorescence staining experiments (Figures 2B–E).
FIGURE 2. Changes of spermatogenesis-related indexes were observed from gene expression and protein level, respectively. The expression of the following genes, such as Dazl, Vasa, Plzf, c-Kit, H2ax, Stra8, Sycp1, Sycp3, Tnp2, and Piwil1, in the testis tissue of the two groups were detected by real-time PCR (qRT-PCR) technology (A). Also, the changes of protein levels such as VASA, DAZL, and γH2AX between the two groups of mice were observed by Western Blot (B,C) and immunofluorescence staining experiments (D–G). * Significant differences compared to the control (p < 0.05).
To evaluate the effect of IAA exposure on testosterone synthesis, changes in gene expression, protein levels of these enzymes and LDs were examined. The results demonstrated that IAA exposure decreased LD storage in Leydig cells (Figures 3A,B). SRB1, as a high-density lipoprotein receptor, is responsible for the transport of LDs. In our study, although the gene expression did not change between the two groups (Figure 3C), there was a decrease in the protein level (Figures 3D–G). Compared with the control group, a mild decrease in Cyp11a1 mRNA expression was observed, as shown in Figure 3C. However, its protein level showed no significant difference between the two groups (Figures 3D,E,H,I). The steroidogenic acute regulatory (STAR), as a key enzyme in testosterone synthesis, tended to decrease in the experiment group, but the differences failed to reach statistical significance (Figures 3D,E). In the immunofluorescence staining experiment, it showed no difference versus control (Supplement Figures 1A,B). Also, no changes were observed in the gene expression of Cyp17a1, Cyp19a1, 3β-hsd, and Lhcgr between the two groups (Figure 3C).
FIGURE 3. Lipid droplet storage in Leydig cells and changes in testosterone synthase from gene expression and protein levels were examined. Cholesterol storage in Leydig cells between the two groups was visualized by BODIPY staining (A,B). The relative mRNA levels of the following indicators, Lhcgr, Srb1, Star, Cyp17a1, Cyp11a1, 3β-hsd, and Cyp19a1, were detected by qRT-PCR (C). Also, the protein levels of cholesterol transporter SRB1 and testosterone synthase STAR, CYP11A1, and CYP17A1 were observed by Western Blot (D,E) and immunofluorescence staining experiments (F–I). * Significant differences compared to the control (p < 0.05).
To assess the effect of IAA exposure on Sertoli cells, changes in genes and proteins of WT-1 and some indicators of tight junctions were examined. As shown in Figure 4A, there were no differences at the genetic level between the two groups on Wt-1, Claudin-11, Nectin-2, Zo-2, β-catenin, Jam-A, N-cadherin, and Connexin-43. The protein levels of WT-1, N-cadherin, and β-catenin also did not perform a statistical difference compared with control (Figures 4B–E).
FIGURE 4. Changes of BTB-related indexes were detected from gene expression and protein level. Relative mRNA levels between the two groups, Wt-1, Claudin-11, Nectin-2, Zo-2, β-catenin, Jam-A, N-cadherin and Connexin-43, were observed (A). The protein levels of WT-1, N-cadherin, and β-catenin were also observed by Western Blot (B,C) and immunofluorescence staining experiments (D,E). * Significant differences compared to the control (p < 0.05).
It is well known that endocrine disruptors have a direct impact on gonads and long-term reproductive health (Delbes et al., 2022). IAA has a negative influence on the female reproductive system, while the effects on the male reproductive system have not been systematically studied. From the perspective of the physiological structure of the testis, the male reproductive system mainly includes spermatogenic cells, Leydig cells, and Sertoli cells (Mäkelä et al., 2019). Therefore, we mainly observed the effect of IAA on its function from these three aspects. In our study, IAA exposure had no apparent effect on Sertoli cell function, but it exacerbated DNA damage in spermatogenic cells and reduced cholesterol storage in Leydig cells by reducing the protein expression of SRB1. In addition, IAA also affected sperm motility, slowing down VAP and VSL. Furthermore, it caused dysfunction of the hypothalamic–pituitary–gonadal axis, especially the increase in LH in serum.
We found that the body weight in the experimental group showed a slight decrease. This may be mainly due to the effect of IAA on gastric acid secretion (Cheng et al., 2001), which finally caused the mice to lose weight. To observe the effect of IAA on sex hormones in vivo, we measured the levels of FSH, LH, and testosterone in the serum. In our study, FSH did not change compared to the control group, but LH showed a significant increase versus control. Research showed that in adult female mice, IAA did not alter LHβ expression, while it reduced FSHβ-positive cell number and FSHβ mRNA expression at a dose of 10 mg/kg in vivo (Gonzalez et al., 2021). The inconsistent results might be due to the difference in dosage and time of medication. Given that testosterone varies widely between individuals, we did not remove outliers. Although there was no difference in testosterone between the two groups, there was a descending trend in the experimental group. We inferred that IAA exposure caused a decrease in testosterone but increased LH through negative feedback, thus maintaining normal levels of testosterone.
To observe the effect of IAA on sperm, we examined changes in relevant sperm parameters, such as sperm concentration, motility, progressiveness, VAP, VSL, VCL, ALH, and BCF. Among them, VAP and VSL showed a significant decrease compared to the control group. We deduced that was because IAA inhibited the glyceraldehyde-3-phosphate dehydrogenase (GAPDH), which was the key target of IAA (Hall et al., 2020) and one of the key enzymes in glycolysis, reduced cellular ATP levels (Dad et al., 2018), and ultimately slowed down sperm motility.
Sperm production is one of the most important functions of the testis. Spermatogenesis is a complex developmental process that consists of three stages: mitosis, meiosis, and spermiogenesis (Zhao J. et al., 2021). To observe the effect of IAA on spermatogenesis, protein levels of DAZL, VASA, γH2AX, and related gene expression changes were detected. Phosphorylated histone H2AX (γH2AX) is a hallmark of chromatin remodeling in male meiosis (Abe et al., 2020). Meanwhile, it is a sensitive marker for DNA double-strand breaks (DSBs) (Arnould et al., 2021), and a number of studies have regarded it as a marker of DNA damage (Guo et al., 2021; Zhang et al., 2021; Wang et al., 2022). Increased expression of γH2AX levels at the gene expression and protein level are detected, indicating that DNA damage is exacerbated after IAA exposure. DAZL, which is crucial for normal spermatogenesis, plays an important role in primordial germ cell formation, and genetic loss of DAZL causes infertility in both sexes of mice (Li et al., 2019). In our study, changes in DAZL gene and protein levels were not observed. VASA (DDX4/MVH), as the hallmark of meiotic cells at the stage from pachytene spermatocytes to round spermatids, is essential for male gametogenesis (Lin et al., 2017). It also showed no difference between the two groups in our study. Plzf and Kit are essential in maintaining spermatogonial stem cell proliferation (Mao et al., 2017; Yu et al., 2022). Stra8 (Sun et al., 2022), Sycp1 and Sycp3 (Dunce et al., 2018), and Tnp2 (Gustafson et al., 2020) play important roles in different stages of meiosis. Also, knockout of the Piwi gene in mice and human causes sterility (Gou et al., 2017). Fortunately, with the exception of H2AX, IAA exposure did not cause changes in the other genes described previously.
The second important function of the testis is testosterone biosynthesis. In order to observe the effect of IAA on testosterone, we focused on the changes in cholesterol and key enzymes in cholesterol transport and testosterone synthesis. SRB1 is a cell surface HDL receptor that mediates HDL-cholesteryl ester (CE) uptake, and it promotes the transport of CEs to Leydig cells and stores them as lipid droplets (LDs), which are used for the synthesis of testosterone in testis tissue (Shen et al., 2018). In our study, the content of cholesterol in Leydig cells decreased in the IAA-exposed group. This was because IAA affected LD transport by reducing the protein expression of SRB1, although IAA did not alter the gene expression of it. We infer that IAA causes changes in SRB1 protein levels through post-transcriptional translation or epigenetic modification, which, of course, requires further experimental validation. At the same time, we also detected a series of key enzymes in testosterone synthesis, such as STAR, CYP11A1, CYP17A1, and 3β-HSD. Except for the decrease of Cyp11a1 at the gene level, other indicators did not show significant differences both in gene expression and at the protein level. However, STAR, one of the most critical enzymes regulating testosterone synthesis (Zhao L. et al., 2021), showed a significant downward trend at the protein level, which further corroborated our conclusion that testosterone had declined during the medication. In Leydig cells, LHCGR acts as an LH receptor to sense the control of testosterone synthesis by the hypothalamic–pituitary–testicular axis (Holota et al., 2020). Gene expression of Lhcgr did not change between the two groups. In addition, the gene level of Cyp19a1, a key enzyme that converts testosterone to estrogen, was examined, and did not change compared to control. This means IAA does not influence the conversion of testosterone to estrogen in male mice.
An important function of Sertoli cells is to form the BTB, which forms the microenvironment for spermatogenesis and protects spermatogenic cells from autoimmune reactions. To assess the function of the BTB, we examined the expression of WT-1, N-cadherin, and β-catenin. WT-1, expressed in all Sertoli cells, is essential for germ cell survival and spermatogenesis (Gupta et al., 2021). N-Cadherin is a protein with greater connection flexibility, which is highly expressed in testis tissue (Verón et al., 2021). At the same time, in other tissues, it is often regarded as highly correlated with tissue migration, and is even used as one of the indicators of tumor aggressiveness (He et al., 2021). β-Catenin is also a characteristic protein of the BTB, and its abnormal accumulation can lead to impaired testicular junction integrity, which can lead to abnormal structures and functions of the BTB (Lei et al., 2021). In our study, the gene expression and protein levels of WT-1, N-cadherin, and β-catenin were not different between the two groups. As important components of tight junctions, such as claudin11 (She et al., 2021), Nectin-2 (Bronson et al., 2017), Zo-2 (Otani et al., 2019), Jam-A (Ebnet, 2017), and Connexin 43 (Liang et al., 2019), they all play a key role in maintaining the integrity of the BTB. Fortunately, there were no statistically significant differences in their gene levels.
In conclusion, in male mice, IAA reduced sperm motility, exacerbated DNA damage, and decreased storage of LDs, the raw material for testosterone synthesis. Of course, a deeper mechanism is needed in further research. At present, the adverse health consequences of endocrine disruptors have been known to all sectors of society (La Merrill et al., 2020), and various countries are also formulating some corresponding regulatory measures (Kassotis et al., 2020). However, little attention has been paid to water DBPs such as IAA. It not only affects the health of frontline workers producing such materials but also has long-term human contact in the form of water DBPs. Given that these endocrine disruptors have toxic effects on humans and even transmit their toxic effects to offspring in a genetic or epigenetic manner (Lombó and Herráez, 2021), laws and regulations on the control of them should be formulated as soon as possible. Also, we should try to find other healthier disinfection methods to replace chlorine-containing disinfectants to reduce their harm to the reproductive system and even human health.
The original contributions presented in the study are included in the article/Supplementary Material; further inquiries can be directed to the corresponding authors.
The animal study was reviewed and approved by the Animal Ethics Committee of Shandong Provincial Hospital.
YL was responsible for the completion of molecular biology experiments and the draft of the article. XH completed the collection and arrangement of the experimental data. LF and MW completed the production of the chart. CY was responsible for the design, guidance of the experimental techniques, and manuscript correction. QG completed the overall thinking and supervision of the project.
This work was supported by grants from the National Natural Science Foundation (81770860 and 81641030) and the Key Research and Development Plan of Shandong Province (2017CXGC1214).
The authors declare that the research was conducted in the absence of any commercial or financial relationships that could be construed as a potential conflict of interest.
All claims expressed in this article are solely those of the authors and do not necessarily represent those of their affiliated organizations, or those of the publisher, the editors, and the reviewers. Any product that may be evaluated in this article, or claim that may be made by its manufacturer, is not guaranteed or endorsed by the publisher.
The Supplementary Material for this article can be found online at: https://www.frontiersin.org/articles/10.3389/fphar.2022.958204/full#supplementary-material
Abe, H., Alavattam, K. G., Hu, Y. C., Pang, Q., Andreassen, P. R., Hegde, R. S., et al. (2020). The initiation of meiotic sex chromosome inactivation sequesters DNA damage signaling from autosomes in mouse spermatogenesis. Curr. Biol. 30 (3), 408–420. e5. doi:10.1016/j.cub.2019.11.064
Agarwal, A., Baskaran, S., Parekh, N., Cho, C. L., Henkel, R., Vij, S., et al. (2021). Male infertility. Lancet 397 (10271), 319–333. doi:10.1016/s0140-6736(20)32667-2
Ali, A., Kurzawa-Zegota, M., Najafzadeh, M., Gopalan, R. C., Plewa, M. J., and Anderson, D. (2014). Effect of drinking water disinfection by-products in human peripheral blood lymphocytes and sperm. Mutat. Res. 770, 136–143. doi:10.1016/j.mrfmmm.2014.08.003
Arnould, C., Rocher, V., Finoux, A. L., Clouaire, T., Li, K., Zhou, F., et al. (2021). Loop extrusion as a mechanism for formation of DNA damage repair foci. Nature 590 (7847), 660–665. doi:10.1038/s41586-021-03193-z
Bronson, R., Mikhailik, A., Schwedes, J., Gnatenko, D., and Hatchwell, E. (2017). Detection of candidate nectin gene mutations in infertile men with severe teratospermia. J. Assist. Reprod. Genet. 34 (10), 1295–1302. doi:10.1007/s10815-017-0985-4
Cheng, A. M., Morrison, S. W., Yang, D. X., and Hagen, S. J. (2001). Energy dependence of restitution in the gastric mucosa. Am. J. Physiol. Cell Physiol. 281 (2), C430–C438. doi:10.1152/ajpcell.2001.281.2.C430
Dad, A., Jeong, C. H., Wagner, E. D., and Plewa, M. J. (2018). Haloacetic acid water disinfection byproducts affect pyruvate dehydrogenase activity and disrupt cellular metabolism. Environ. Sci. Technol. 52 (3), 1525–1532. doi:10.1021/acs.est.7b04290
Delbes, G., Blázquez, M., Fernandino, J. I., Grigorova, P., Hales, B. F., Metcalfe, C., et al. (2022). Effects of endocrine disrupting chemicals on gonad development: Mechanistic insights from fish and mammals. Environ. Res. 204, 112040. doi:10.1016/j.envres.2021.112040
Dong, H., Qiang, Z., and Richardson, S. D. (2019). Formation of iodinated disinfection byproducts (I-DBPs) in drinking water: emerging concerns and current issues. Acc. Chem. Res. 52 (4), 896–905. doi:10.1021/acs.accounts.8b00641
Dunce, J. M., Dunne, O. M., Ratcliff, M., Millán, C., Madgwick, S., Usón, I., et al. (2018). Structural basis of meiotic chromosome synapsis through SYCP1 self-assembly. Nat. Struct. Mol. Biol. 25 (7), 557–569. doi:10.1038/s41594-018-0078-9
Ebnet, K. (2017). Junctional adhesion molecules (JAMs): cell adhesion receptors with pleiotropic functions in cell physiology and development. Physiol. Rev. 97 (4), 1529–1554. doi:10.1152/physrev.00004.2017
Escobar-Hoyos, L. F., Hoyos-Giraldo, L. S., Londoño-Velasco, E., Reyes-Carvajal, I., Saavedra-Trujillo, D., Carvajal-Varona, S., et al. (2013). Genotoxic and clastogenic effects of monohaloacetic acid drinking water disinfection by-products in primary human lymphocytes. Water Res. 47 (10), 3282–3290. doi:10.1016/j.watres.2013.02.052
Gonsioroski, A., Meling, D. D., Gao, L., Plewa, M. J., and Flaws, J. A. (2021). Iodoacetic acid affects estrous cyclicity, ovarian gene expression, and hormone levels in mice. Biol. Reprod. 105 (4), 1030–1042. doi:10.1093/biolre/ioab108
Gonsioroski, A., Meling, D. D., Gao, L., Plewa, M. J., and Flaws, J. A. (2020). Iodoacetic acid inhibits follicle growth and alters expression of genes that regulate apoptosis, the cell cycle, estrogen receptors, and ovarian steroidogenesis in mouse ovarian follicles. Reprod. Toxicol. 91, 101–108. doi:10.1016/j.reprotox.2019.10.005
Gonsioroski, A., Mourikes, V. E., and Flaws, J. A. (2020). Endocrine disruptors in water and their effects on the reproductive system. Int. J. Mol. Sci. 21 (6), 1929. doi:10.3390/ijms21061929
Gonzalez, R. V. L., Weis, K. E., Gonsioroski, A. V., Flaws, J. A., and Raetzman, L. T. (2021). Iodoacetic acid, a water disinfection byproduct, disrupts hypothalamic, and pituitary reproductive regulatory factors and induces toxicity in the female pituitary. Toxicol. Sci. 184 (1), 46–56. doi:10.1093/toxsci/kfab106
Gou, L. T., Kang, J. Y., Dai, P., Wang, X., Li, F., Zhao, S., et al. (2017). Ubiquitination-deficient mutations in human Piwi cause male infertility by impairing histone-to-protamine exchange during spermiogenesis. Cell 169 (6), 1090–1104. doi:10.1016/j.cell.2017.04.034
Guo, H., Ouyang, Y., Wang, J., Cui, H., Deng, H., Zhong, X., et al. (2021). Cu-induced spermatogenesis disease is related to oxidative stress-mediated germ cell apoptosis and DNA damage. J. Hazard. Mat. 416, 125903. doi:10.1016/j.jhazmat.2021.125903
Gupta, A., Mandal, K., Singh, P., Sarkar, R., and Majumdar, S. S. (2021). Declining levels of miR-382-3p at puberty trigger the onset of spermatogenesis. Mol. Ther. Nucleic Acids 26, 192–207. doi:10.1016/j.omtn.2021.07.001
Gustafson, E. A., Seymour, K. A., Sigrist, K., Rooij, D. G. D. E., and Freiman, R. N. (2020). ZFP628 is a TAF4b-interacting transcription factor required for mouse spermiogenesis. Mol. Cell. Biol. 40 (7), e00228–19. doi:10.1128/MCB.00228-19
Hall, D. R., Yeung, K., and Peng, H. (2020). Monohaloacetic acids and monohaloacetamides attack distinct cellular proteome thiols. Environ. Sci. Technol. 54 (23), 15191–15201. doi:10.1021/acs.est.0c03144
He, J., Chu, Z., Lai, W., Lan, Q., Zeng, Y., Lu, D., et al. (2021). Circular RNA circHERC4 as a novel oncogenic driver to promote tumor metastasis via the miR-556-5p/CTBP2/E-cadherin axis in colorectal cancer. J. Hematol. Oncol. 14 (1), 194. doi:10.1186/s13045-021-01210-2
Holota, H., Thirouard, L., Monrose, M., Garcia, M., De Haze, A., Saru, J. P., et al. (2020). FXRα modulates leydig cell endocrine function in mouse. Mol. Cell. Endocrinol. 518, 110995. doi:10.1016/j.mce.2020.110995
Jeong, C. H., Gao, L., Dettro, T., Wagner, E. D., Ricke, W. A., Plewa, M. J., et al. (2016). Monohaloacetic acid drinking water disinfection by-products inhibit follicle growth and steroidogenesis in mouse ovarian antral follicles in vitro. Reprod. Toxicol. 62, 71–76. doi:10.1016/j.reprotox.2016.04.028
Kahn, L. G., Philippat, C., Nakayama, S. F., Slama, R., and Trasande, L. (2020). Endocrine-disrupting chemicals: Implications for human health. Lancet Diabetes Endocrinol. 8 (8), 703–718. doi:10.1016/S2213-8587(20)30129-7
Kali, S., Khan, M., Ghaffar, M. S., Rasheed, S., Waseem, A., Iqbal, M. M., et al. (2021). Occurrence, influencing factors, toxicity, regulations, and abatement approaches for disinfection by-products in chlorinated drinking water: a comprehensive review. Environ. Pollut. 281, 116950. doi:10.1016/j.envpol.2021.116950
Kassotis, C. D., Vandenberg, L. N., Demeneix, B. A., Porta, M., Slama, R., and Trasande, L. (2020). Endocrine-disrupting chemicals: economic, regulatory, and policy implications. Lancet. Diabetes Endocrinol. 8 (8), 719–730. doi:10.1016/S2213-8587(20)30128-5
Kim, D. H., Park, C. G., and Kim, Y. J. (2020). Characterizing the potential estrogenic and androgenic activities of two disinfection byproducts, mono-haloacetic acids and haloacetamides, using in vitro bioassays. Chemosphere 242, 125198. doi:10.1016/j.chemosphere.2019.125198
La Merrill, M. A., Vandenberg, L. N., Smith, M. T., Goodson, W., Browne, P., Patisaul, H. B., et al. (2020). Consensus on the key characteristics of endocrine-disrupting chemicals as a basis for hazard identification. Nat. Rev. Endocrinol. 16 (1), 45–57. doi:10.1038/s41574-019-0273-8
Lei, W. L., Li, Y. Y., Meng, T. G., Ning, Y., Sun, S. M., Zhang, C. H., et al. (2021). Specific deletion of protein phosphatase 6 catalytic subunit in Sertoli cells leads to disruption of spermatogenesis. Cell Death Dis. 12 (10), 883. doi:10.1038/s41419-021-04172-y
Li, H., Liang, Z., Yang, J., Wang, D., Wang, H., Zhu, M., et al. (2019). DAZL is a master translational regulator of murine spermatogenesis. Natl. Sci. Rev. 6 (3), 455–468. doi:10.1093/nsr/nwy163
Liang, J., Wang, N., He, J., Du, J., Guo, Y., Li, L., et al. (2019). Induction of Sertoli-like cells from human fibroblasts by NR5A1 and GATA4. Elife 8, e48767. doi:10.7554/eLife.48767
Lin, Z., Hsu, P. J., Xing, X., Fang, J., Lu, Z., Zou, Q., et al. (2017). Mettl3-/Mettl14-mediated mRNA N6-methyladenosine modulates murine spermatogenesis. Cell Res. 27 (10), 1216–1230. doi:10.1038/cr.2017.117
Lombó, M., and Herráez, P. (2021). The effects of endocrine disruptors on the male germline: an intergenerational health risk. Biol. Rev. Camb. Philos. Soc. 96 (4), 1243–1262. doi:10.1111/brv.12701
Long, K., Sha, Y., Mo, Y., Wei, S., Wu, H., Lu, D., et al. (2021). Androgenic and teratogenic effects of iodoacetic acid drinking water disinfection byproduct in vitro and in vivo. Environ. Sci. Technol. 55 (6), 3827–3835. doi:10.1021/acs.est.0c06620
Mäkelä, J. A., Koskenniemi, J. J., Virtanen, H. E., and Toppari, J. (2019). Testis development. Endocr. Rev. 40 (4), 857–905. doi:10.1210/er.2018-00140
Mao, A. P., Ishizuka, I. E., Kasal, D. N., Mandal, M., and Bendelac, A. (2017). A shared Runx1-bound Zbtb16 enhancer directs innate and innate-like lymphoid lineage development. Nat. Commun. 8 (1), 863. doi:10.1038/s41467-017-01780-1
Otani, T., Nguyen, T. P., Tokuda, S., Sugihara, K., Sugawara, T., Furuse, K., et al. (2019). Claudins and JAM-A coordinately regulate tight junction formation and epithelial polarity. J. Cell Biol. 218 (10), 3372–3396. doi:10.1083/jcb.201812157
She, J., Feng, N., Zheng, W., Zheng, H., Cai, P., Zou, H., et al. (2021). Zearalenone exposure disrupts blood-testis barrier integrity through excessive Ca2+-mediated autophagy. Toxins 13 (12), 875. doi:10.3390/toxins13120875
Shen, W. J., Azhar, S., and Kraemer, F. B. (2018). SR-B1: a unique multifunctional receptor for cholesterol influx and efflux. Annu. Rev. Physiol. 80, 95–116. doi:10.1146/annurev-physiol-021317-121550
Sun, S., Jiang, Y., Zhang, Q., Pan, H., Li, X., Yang, L., et al. (2022). Znhit1 controls meiotic initiation in male germ cells by coordinating with Stra8 to activate meiotic gene expression. Dev. Cell 57 (7), 901–913. e4. doi:10.1016/j.devcel.2022.03.006
Verón, G. L., Veiga, M. F., Cameo, M., Marín-Briggiler, C. I., and Vazquez-Levin, M. H. (2021). Epithelial and neural cadherin in mammalian fertilization: studies in the mouse model. Cells 11 (1), 102. doi:10.3390/cells11010102
Wang, J., Jia, R., Zheng, X., Sun, Z., Liu, R., and Zong, W. (2018). Drinking water disinfection byproduct iodoacetic acid interacts with catalase and induces cytotoxicity in mouse primary hepatocytes. Chemosphere 210, 824–830. doi:10.1016/j.chemosphere.2018.07.061
Wang, W., Peng, M., Yuan, H., Liu, C., Zhang, Y., Fang, Y., et al. (2022). Studying the mechanism of sperm DNA damage caused by folate deficiency. J. Cell. Mol. Med. 26 (3), 776–788. doi:10.1111/jcmm.17119
Wei, X., Wang, S., Zheng, W., Wang, X., Liu, X., Jiang, S., et al. (2013). Drinking water disinfection byproduct iodoacetic acid induces tumorigenic transformation of NIH3T3 cells. Environ. Sci. Technol. 47 (11), 5913–5920. doi:10.1021/es304786b
Xia, Y., Mo, Y., Yang, Q., Yu, Y., Jiang, M., Wei, S., et al. (2018). Iodoacetic acid disrupting the thyroid endocrine system in vitro and in vivo. Environ. Sci. Technol. 52 (13), 7545–7552. doi:10.1021/acs.est.8b01802
Yu, S., Zhou, C., He, J., Yao, Z., Huang, X., Rong, B., et al. (2022). BMP4 drives primed to naïve transition through PGC-like state. Nat. Commun. 13 (1), 2756. doi:10.1038/s41467-022-30325-4
Zhang, X. Y., Wang, B., Xu, S., Wang, J., Gao, L., Song, Y. P., et al. (2021). Reactive oxygen species-evoked genotoxic stress mediates arsenic-induced suppression of male germ cell proliferation and decline in sperm quality. J. Hazard. Mat. 406, 124768. doi:10.1016/j.jhazmat.2020.124768
Zhao, J., Lu, P., Wan, C., Huang, Y., Cui, M., Yang, X., et al. (2021). Cell-fate transition and determination analysis of mouse male germ cells throughout development. Nat. Commun. 12 (1), 6839. doi:10.1038/s41467-021-27172-0
Keywords: iodoacetic acid, testis, sperm, testosterone, γH2AX, SRB1
Citation: Liang Y, Huang X, Fang L, Wang M, Yu C and Guan Q (2022) Effect of iodoacetic acid on the reproductive system of male mice. Front. Pharmacol. 13:958204. doi: 10.3389/fphar.2022.958204
Received: 31 May 2022; Accepted: 28 July 2022;
Published: 26 August 2022.
Edited by:
Tariq Ismail, COMSATS University Islamabad, Abbottabad Campus, PakistanReviewed by:
Irum Shahzadi, COMSATS University Islamabad, PakistanCopyright © 2022 Liang, Huang, Fang, Wang, Yu and Guan. This is an open-access article distributed under the terms of the Creative Commons Attribution License (CC BY). The use, distribution or reproduction in other forums is permitted, provided the original author(s) and the copyright owner(s) are credited and that the original publication in this journal is cited, in accordance with accepted academic practice. No use, distribution or reproduction is permitted which does not comply with these terms.
*Correspondence: Chunxiao Yu, eXVjaHgwOEAxNjMuY29t; Qingbo Guan, ZG9jdG9yZ3VhbnFpbmdib0AxNjMuY29t
Disclaimer: All claims expressed in this article are solely those of the authors and do not necessarily represent those of their affiliated organizations, or those of the publisher, the editors and the reviewers. Any product that may be evaluated in this article or claim that may be made by its manufacturer is not guaranteed or endorsed by the publisher.
Research integrity at Frontiers
Learn more about the work of our research integrity team to safeguard the quality of each article we publish.