- 1State Key Laboratory of Quality Research in Chinese Medicine, Macau University of Science and Technology, Macau, China
- 2Department of Pediatrics, The Affiliated Hospital of Southwest Medical University, Sichuan Clinical Research Center for Birth Defects, Luzhou, China
- 3Department of Respiratory and Critical Care Medicine, Affiliated Hospital of Southwest Medical University, Luzhou, China
Background: It has been demonstrated that scutellarin, a natural flavone compound from Scutellaria lateriflora and Scutellaria barbata, exerts selective cytotoxicity against a range of cancer cells. However, the underlining mechanism of scutellarin on acute myeloid leukemia (AML) remains elusive.
Methods: In this study, the combination of network pharmacology and experimental verification was performed to identify the pharmacological mechanisms of scutellarin for AML therapy. The public databases, such as PharmMapper, UniProt, OMIM, GeneCards, DrugBank and PharmGkb database, were used to sceen the potential targets of scutellarin and AML. The protein-protein interaction (PPI), gene ontology (GO) and Kyoto Encyclopedia of Genes and Genomes (KEGG) pathway enrichment analysis were conducted to uncover the mechanism of scutellarin in the treatment of AML. Finally, the network pharmacological results were further confirmed by in vitro and in vivo experiments.
Results: First and foremost, we totally obtained 289 target genes for scutellarin and 10998 disease targets for AML. 253 overlapping genes were preliminarily considered the potential targets of scutellarin for AML treatment. The results of PPI network analysis, GO analysis and KEGG pathway enrichment demonstrated that the anti-AML effect of scutellarin may focused on MAPK signaling pathway. Furthermore, the cytologic tests suggested that scutellarin can inhibit AML cells proliferation through the mediation of JNK/Caspase-3 pathway. Meanwhile, pretreatment with the JNK inhibitor SP600125 rescued scutellarin-induced apoptosis. Similarly, scutellarin obviously suppressed subcutaneous xenograft growth in nude mice via regulating the JNK/Caspase-3 signaling pathway.
Conclusion: In this study, we integrated network pharmacology-based prediction and experimental validation and revealed the importance of the JNK pathway in scutellarin-mediated AML treatment.
Introduction
Acute myeloid leukemia (AML), a typical blood cancer, is featured by changes in hematopoietic cells, such as blocked differentiation, increased proliferation, or accumulation of blasts (Dohner et al., 2017). As the most common acute leukemia in adults, AML is diagnosed with a median age of 68 years and increasing with age (Reedijk et al., 2019; Ari Pelcovits 2020). Because the incidence trend is not optimistic, AML has high morbidity and mortality, which has become the focus of current research.
The ″3 + 7″ regimen, 3 days of daunorubicin followed by 7 days of cytarabine, is the standard treatment for AML. The overall survival rate in patients with AML under this standard regimen is only 40%, and the 5-years survival rate (5y-SR) of patients (≥60 years) is even lower, less than 10–15% (M.Heuser, 2020). In the past 30 years, with the rapid development of genomics, treatment methods for AML have gradually diversified. Hematopoietic stem cell transplantation, molecular targeted therapy and, more recently, CAR-T cell immunotherapy have been used in the clinic (Sallman et al., 2018; Takami, 2018; Daver et al., 2019; Yilmaz & Daver, 2019; Becker & Fathi, 2020; Roberts, 2020). Despite significant improvement in the treatment of AML, the 5y-SR has not improved significantly (Dohner et al., 2015; Thomas & Majeti, 2017). Therefore, safer and more effective treatments are urgently needed.
Traditional Chinese Medicine (TCM) has been widely used in clinical for thousands of years and is one of the most abundant resources for the discovery of anticancer drugs. As is well known, TCM treated diseases in a holistic concept and possessed the characteristics of multi-target and multi-approach, which coincides with the prevention and treatment strategy of cancer (Xiang et al., 2019). For a long time, TCM has played a unique advantage in tumor treatment. A number of clinical trials have confirmed that the combination of active ingredients of TCM and chemotherapies can prolong the survival period, improve the survival status, and can also enhance the detoxification effect (Huang X. M et al., 2020). Many Western countries also use TCM as complementary and alternative therapy (Luo et al., 2014). Scutellarin is a natural flavone compound from Scutellaria barbata and Scutellaria lateriflora, herbs that have a long history in TCM (Wang & Ma, 2018). Research on scutellarin over the past 3 decades has accumulated a wealth of evidence confirming its effectiveness in the treatment of cerebrovascular and cardiovascular diseases, especially coronary heart disease and ischemic stroke (Chen et al., 2020; Xu et al., 2021). In the past 10 years, scutellarin has been reported to have selective cytotoxicity against cancer cells from different tissues, such as glioblastoma, gastric, colorectal, and lung cancer (Fu Li and niu, 2021; Liu et al., 2019; Zeng et al., 2021; Zhang et al., 2021). Various mechanisms of action have been reported for the anticancer activities of scutellarin. Scutellarin can downregulate the hedgehog signaling pathway and thus achieve the inhibition of the proliferation and migration in human colorectal cancer cells (Tan et al., 2020). It has been reported that scutellarin could induce apoptosis through the regulatuion of TGF-β1/smad2/ROS/Caspase-3 pathway in A549 cells (Zhang et al., 2021), and via STAT3 pathway in HepG2 cells (Xu & Zhang, 2013). Scutellarin can also suppress metastasis and chemoresistance in glioma cells (Tang et al., 2019). However, the anticancer effect of scutellarin on AML has not been reported so far.
Accompanying with the quick development of system biology and bioinformatics, network pharmacology provides a new method for revealing the complex mechanism of TCM and the interrelationships among drugs, targets and diseases (Huang et al., 2020). In line with TCM theory which emphasizes the synergistic effect of ingredients, network pharmacology closely observes the effects of drugs on disease.
In our study, network pharmacology was employed to elucidate the molecular mechanisms of scutellarin for AML therapy, which was confirmed by in vitro and in vivo experimental validation. The workflow is shown in Figure 1.
Materials and methods
Cell culture
Human AML cell lines (HL-60, KG-1) were obtained from the US Model Culture Collection (ATCC, US). THP-1 human AML cells were purchased from the Chinese Academy of Sciences Cell Bank. THP-1, HL-60, KG-1 cells were cultured in RPMI-1640 medium (Gibco) supplemented with 10% fetal bovine serum (FBS, Gibco), 1% penicillin (pen) and streptomycin (strep).
Chemicals
Scutellarin (CAS#27740-01-8) was provided by Weiqi Biological Technology Co. Ltd. Breviscapine was provided by Yunnan Plant Pharmaceutical Co. Ltd. (National drug approval Z53020121). Z-VAD (OMe)-FMK (Z-VAD, HY-16658, MCE), SP600125 (S1876, Beyotime), CCK-8 kit (Japan Tongren Chemical Research Institute), Annexin V-FITC Apoptosis Detection Kit I (556547, BD Bioscience).
Antibodies
Western blot immunoassay was performed with antibodies against p-JNK(Cat.#9251S), JNK (Cat.#9252S), cleaved caspase-3(Cat.#9664), cleaved PARP (Cat.#5625), anti-rabbit secondary antibody (Cat.#7074) and anti-mouse secondary antibody (Cat.#7076). The above antibodies were all provided by Cell Signaling Technology. Αnti-β-actin (Cat.#A5441) was provided by Sigma Aldrich.
Proliferation assay in vitro
Cell proliferation assay was assessed with the CCK-8 kit (Japan Tongren Chemical Research Institute) on leukemia cell lines. HL-60 (2×104 cells/well), THP-1 (1.5×104 cells/well) and KG-1 (1.5×104 cells/well) cells were inoculated in 96-well plates at a volume of 90 μL/well. Then 10 μl medium containing various doses of scutellarin was added and incubated for 72 h at 37°C. Next, 5 μl/well CCK-8 solution was added and incubated for 4 h at 37°C. The SpectraMax 190 microplate reader (Molecular Devices) was used to measure the absorbency at 450 nm. The relative cell growth rate was calculated with the following equation: Relative Growth (%) = [OD (treated) -OD (blank)]/[OD (control)-OD (blank)] ×100%. The IC50 of scutellarin was counted by Graph Pad Prism 8.0 software.
The targets of scutellarin in AML
The PubChem database (https://pubchem.ncbi.nlm.nih.gov/) was applied to obtained chemical structure of scutellarin. The PharmMapper database (http://www.lilab-ecust.cn/pharmmapper/) was utilized to generate targets with high scores. Then, the protein target names were converted to corresponding gene symbols in UniProt database (http://www.uniprot.org/). The disease targets of AML were retrieved from OMIM, GeneCards, DrugBank and PharmGkb databases. The targets associated with AMLwere obtained by merging the targets of the four databases. Finally, the predicted targets of scutellarin against AML were collected by intersection of the drug and disease targets.
Construction of a “drug-disease-target” network
Based on the above analyses, the potential target genes of scutellarin were matched with the disease targets of AML to obtain the common targets. The network diagram between the scutellarin and AML-related targets was established from Cytoscape 3.8.0 (http://www.cytoscape.org/).
PPI network
The PPI network was constructed by importing the targets of scutellarin against AML to the STRING database (https://string-db.org/). Then, the interaction information was further visually analysed by Cytoscape3.8.0. In the PPI network, the key genes based on degree scores were screened using the Cytohubba plug-in.
GO and KEGG pathway analysis
GO and KEGG enrichment pathway analysis were used to search the key targets of scutellarin against AML by using the Bioconductor package in R (4.1.1) language. Statistical significance was set at q value ≤ 0.05.
Apoptosis assay
HL-60 cells were seeded in 6-well plates at 5×105 cells/well and treated with scutellarin of indicated concentrations. Then cells were centrifuged in the tube after 24 h, rinsed twice with pre-cold PBS buffer, then incubated with 5 µl PI and 5 µl FITC Annexin V in 100 µl 1×Binding Buffer at room temperature in the dark for 20 min. Next, the cells were resuspended in 400 μl 1×Binding Buffer. Cellular apoptosis was assessed with Annexin V-FITC Apoptosis Detection Kit I (BD Bioscience). The cells were analyzed by a flow cytometer (BD Bioscience) after passing through a filter. The data were analyzed by FlowJo 7.6 software.
Western blotting
HL-60 cells were inoculated in 6-well plates and incubated in a medium containing scutellarin for indicated time point. Cell lysates were prepared with RIPA buffer supplemented with phosphatase inhibitor (Roche) and protease inhibitor cocktail (Roche). Protein concentrations were quantified by the BCA protein assay kit (P0012, Beyotime). Protein samples were heated at 95°C for 10 min following diluted in 5 x loading buffer. Then, 30 µg of each sample was fractionated on SDS-PAGE gels, transferred to PVDF membrane (Millipore) and incubated with different primary antibodies overnight at 4 C. Next, HRP-conjugated secondary antibodies (1:10000) were added at room temperature and the membranes were washed with 1×TBST. Proteins were visualized with SuperSignal West Pico Chemiluminescent Substrate or SuperSignal West Dura Extended Duration Substrate (Thermo Scientific).
Xenograft assay
All mice used in the animal experiments were 4-6 weeks old female nude mice. 5×106 HL-60 cells were inoculated subcutaneously into both hind limbs of nude mice. we randomly divided the mice into two groups: vehicle (normal saline, po), Breviscapine (60 mg/kg/d, po) when tumor volumes reached 100 mm3. The therapy lasted for 21 days and the tumor size and body weight were monitored every other day. The tumor volumes were calculated as
Volume = (width2 × length)/2 (Huang et al., 2021).
All animal studies were approved by the Animal Ethical Committee of Affiliated Hospital of Southwest Medical University.
Statistical analyses
All data were shown as mean ± standard deviation (SD). And statistical analyses were performed through Graph Pad Prism 8.0 software. The data were analyzed by one-way analysis of variance (ANOVA) if comparing between more than two groups. The student’s t-test was selected to compare two groups. * represents p < 0.05, ** represents p < 0.01, *** represents p < 0.001, **** represents p < 0.0001.
Results
The precidiction of anti-AML targets of scutellarin
A total of 289 potential targets for scutellarin were retrieved from the PharmMapper database by querying the structure of scutellarin (Figure 2A; Supplementary Table S1). Similarly, 10998 targets related to AML were found in OMIM, GeneCards, DrugBank, and PharmGkb databases (Figure 2B; Supplementary Table S2). Subsequently, 253 genes were enriched after intersecting the targets of scutellarin with AML-related targets (Figure 2C; Supplementary Table S3). Finally, to elucidate the mechanism of scutellarin in AML treatment, a “drug-disease-target” network was constructed applying Cytoscape 3.8.0 software (Figure 2D).
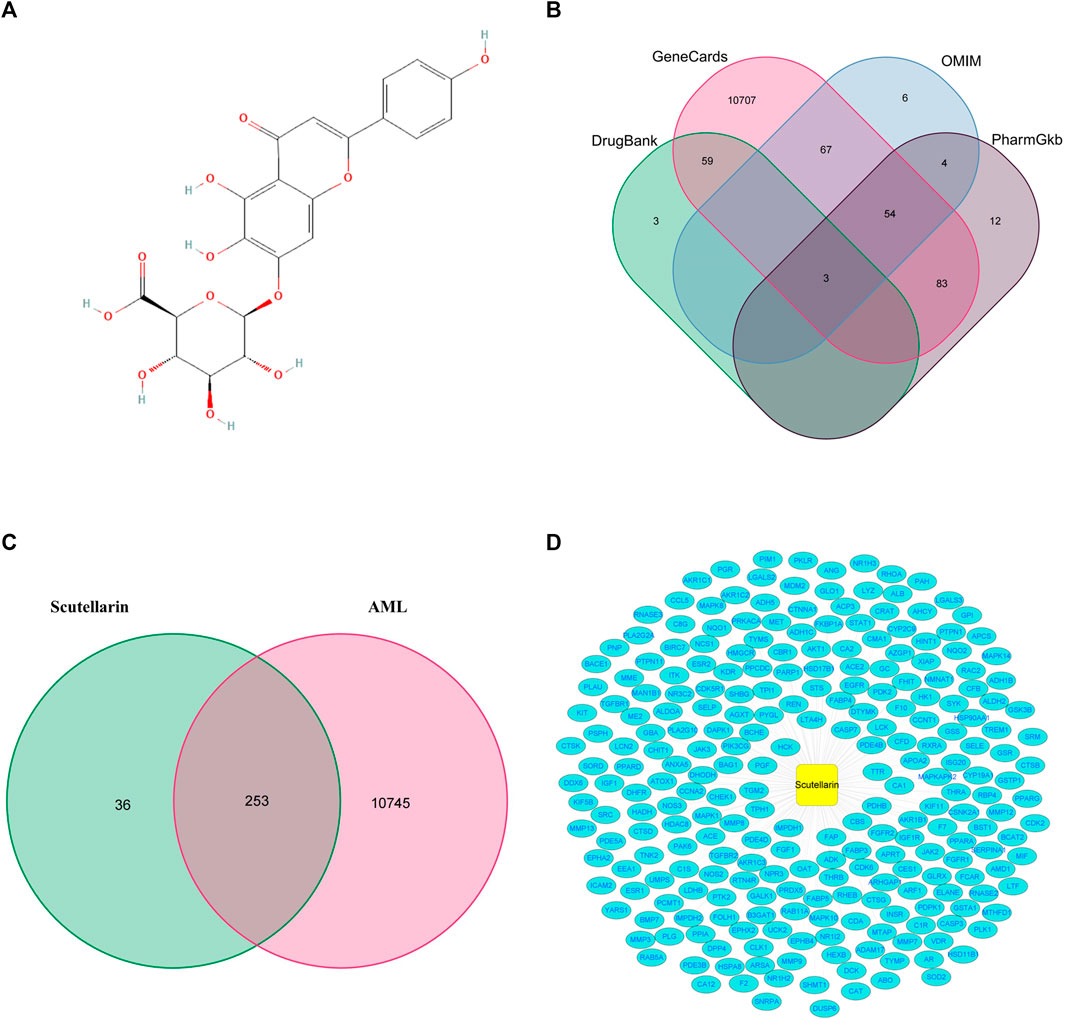
FIGURE 2. The precidiction of anti-AML targets of scutellarin. (A) Chemical structure of scutellarin. (B) The collection of AML-related genes obtained from four databases. (C) The intersection of AML-related genes and target genes of scutellarin. (D) The network map of “drug-disease-target”.
Analysis on AML-related PPI
To analyze the protein-protein interaction network, we interrogated the 253 target genes, with high confidence set to 0.7, in the STRING database (Figure 3A; Supplementary Table S4). The core parts of the network were then analyzed by CytoNCA and finally three core topological networks were obtained, which contained 17 core genes, including MAPK8, CASP3, PRKACA, MAPK1, AKT1, HSP90AA1, MET, ESR1, SRC, EGFR, JAK2, IGF1, PTK2, STAT1, RHOA, MDM2, PTPN11 (Figures 3B–D; Supplementary Table S5).
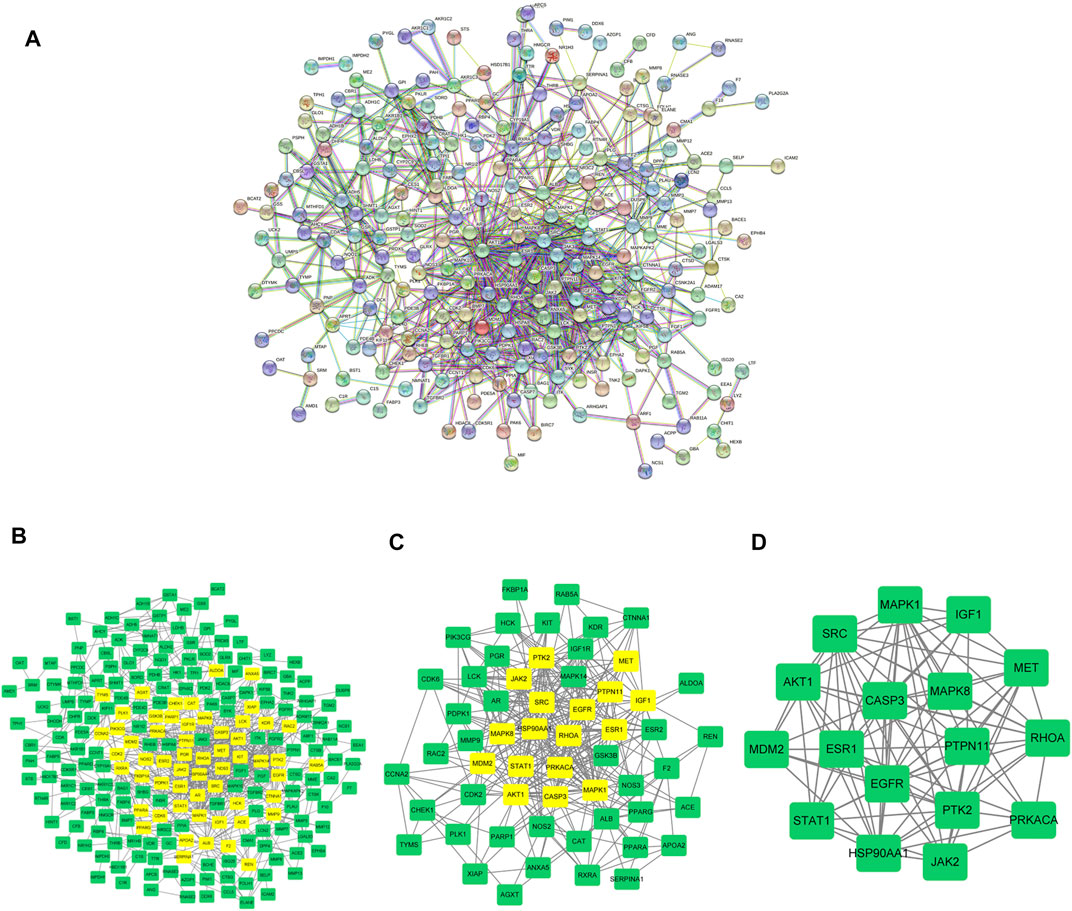
FIGURE 3. Analysis on AML-Related PPI. (A) The construction of protein interaction network of AML target genes induced by scutellarin. (B) The interactive PPI network of AML-related targets and scutellarin targets. (C) Further screening of AML target genes acted by scutellarin. (D) Seventeen core candidate target genes of scutellarin for AML treatment.
GO and KEGG pathway analysis
To gain more insights into the cellular function of scutellarin in AML cells, we performed a GO term enrichment analysis of the 253 targets. A total of 2201 GO terms were obtained. Among them, there are 1935 entries for biological process (BP), 52 entries for cellular composition (CC), and 214 entries for molecular function (MF). The top-10 significantly enriched terms in each category are shown in Figure 4A. Major terms in the BP category included neutrophil mediated immunity, response to extracellular stimulus, response to nutrient levels, cellular response to chemical stress, response to oxidative stress, and protein kinase B signaling. Major CC terms involved vesicle lumen, cytoplasmic vesicle lumen, secretory granule lumen, and membrane raft. Major MF terms covered protein serine/threonine kinase activity, protein tyrosine kinase activity, and serine-type peptidase activity. Regulation of the execution phase of apoptosis and cellular component disassembly in the execution phase of apoptosis were both enriched (Supplementary Table S6).
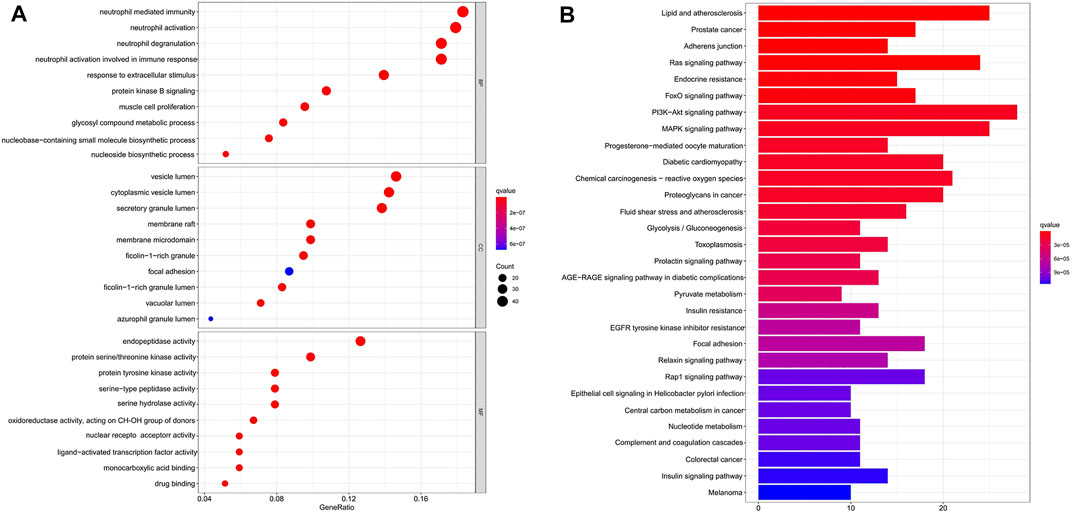
FIGURE 4. GO and KEGG pathway enrichment analysis. (A) GO analysis enrichment of candidate target genes of scutellarin for AML treatment. (B) KEGG pathways enrichment of candidate target genes of scutellarin against AML. (p < 0.05).
KEGG pathway enrichment analysis of the 253 targets enriched in 150 signaling pathways. It verified AML-related pathways involving the PI3K-Akt signaling pathway (hsa04151), MAPK signaling pathway (hsa04010), and Ras signaling pathway (hsa04014) (Supplementary Table S7). The top 30 KEGG pathways with high counts were shown in Figure 4B. The MAPK signaling pathway, which enriched a large count among AML-related pathways (Torres et al., 2010; Bomfim et al., 2019; Tian et al., 2019), plays a key role in the treatment of AML. Hence, a crucial branch of MAPK signaling, JNK pathway, was tested in vitro and in vivo to confirm whether it contributed to the anti-AML effects of scutellarin by inducing apoptosis.
Scutellarin inhibits the growth of AML cells
To investigate the cytotoxic effects of scutellarin, we treated AML cell lines for 72 h with various concentrations of scutellarin, and CCK-8 assay was employed to test the cell viability. As illutrated in Figure 5A, scutellarin dose-dependently reduced the viability of HL-60, THP-1, and KG-1 cells. The IC50 values of scutellarin on three AML cell lines were 35.99, 45.26, and 62.61 µM, respectively.
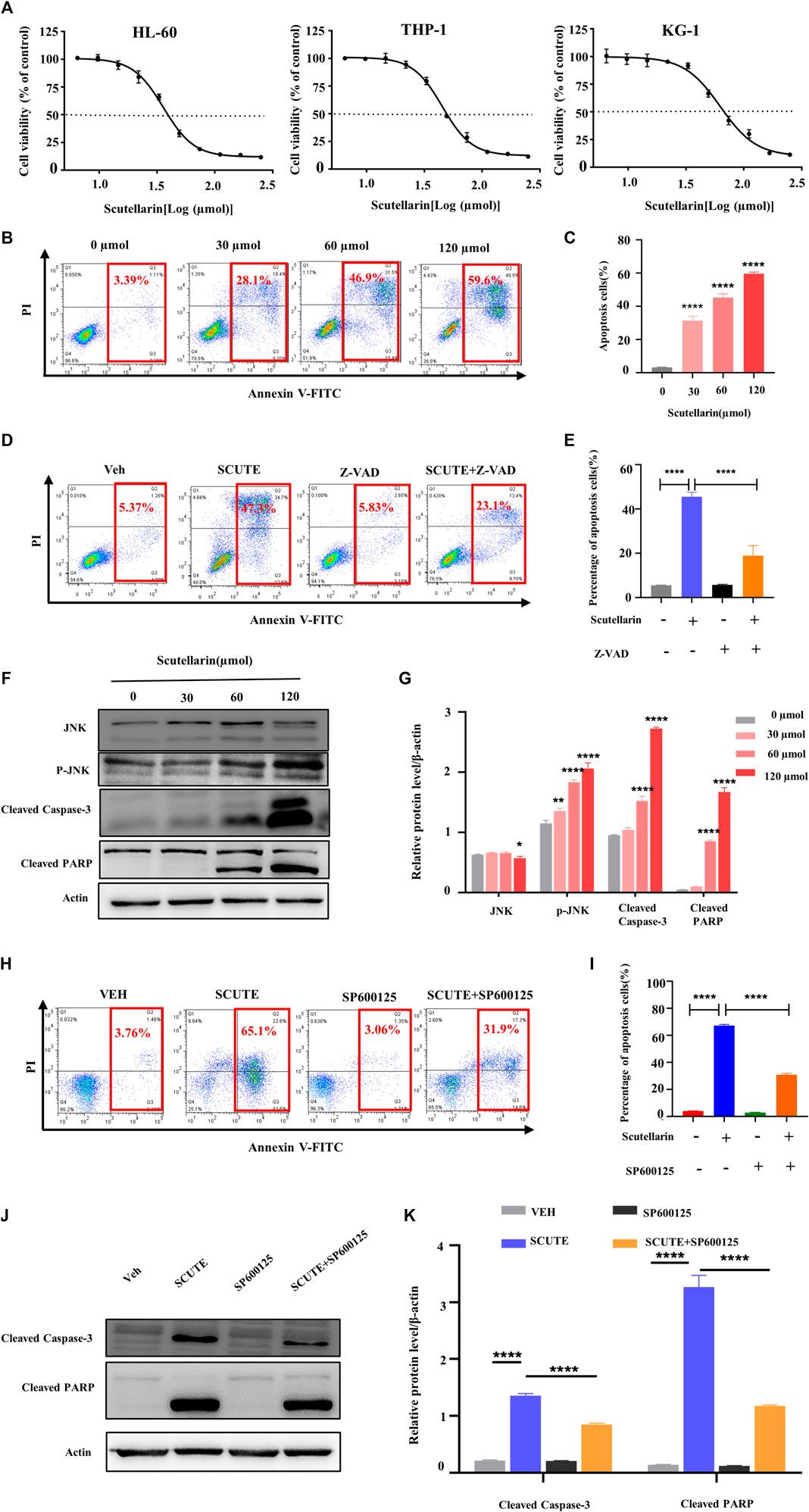
FIGURE 5. Scutellarin inhibits the growth of AML cells. (A) HL-60, THP-1 and KG-1 cells were treated with different concentrations of scutellarin for 72 h. Cell viability (% of control) was performed by CCK-8 assay. (B) Apoptosis analysis was measured by flow cytometry in HL-60 cells treated with different concentrations of scutellarin. (C) The statistical analysis result of (B). (D) Apoptosis analysis was performed by flow cytometry in HL-60 cells incubated with vehicle or scutellarin (60 µM) for 24 h following pretreated with vehicle or Z-VAD-FKM (50 µM) for 2 h. (E) The statistical analysis result of (D). (F) The levels of JNK, p-JNK, cleaved caspase-3 and cleaved PARP in HL-60 cells after scutellarin treatment by Western blot analysis. (G) The statistical analysis result of (F). (H) Apoptosis analysis was performed by flow cytometry in HL-60 cells incubated with vehicle or scutellarin (60 µM) for 24 h following pretreated with vehicle or SP600125 (10 µM) for 2 h. (I) The statistical analysis result of (H). (J) Western blot analysis of proteins in HL-60 cells incubated with vehicle or scutellarin (60 µM) for 24 h following pretreated with vehicle or SP600125 (10 µM) for 2 h. (K) The statistical analysis result of (J). All data were analyzed as mean ± SD (n = 3, **p < 0.01, ***p < 0.001, ****p < 0.0001).
To confirm the molecular mechanism of scutellarin on AML cells, we focused on HL-60 cells, which was more sensitive to scutellarin relative to other cell lines. The pro-apoptotic effect of scutellarin on HL-60 cells was determined by flow cytometric analysis. After 24 h treatment with scutellarin at indicated concentrations, the apoptosis in HL-60 cells obviously increased in a dose-dependent manner (Figures 5B,C). Furthermore, pretreatment with Z-VAD-FMK (a pan-caspase inhibitor) can partially rescue apoptosis induced by scutellarin (Figures 5D,E), strongly indicating that scutellarin induced apoptosis in HL-60 cells.
According to network pharmacology analysis, the JNK/Caspase-3 pathway is the key signaling axis associated with the effect of scutellarin against AML. Thus, we analyzed the protein levels in this pathway by Western blotting. As shown in Figures 6F,G, all proteins including cleaved Caspase-3, p-JNK, and cleaved PARP showed an dose-dependent increase after scutellarin treatment. Furthermore, we found that JNK inhibitor SP600125 partially decreased the apoptosis (Figures 6H,I), reversed the protein levels of cleaved Caspase-3 and cleaved PARP (Figures 4J,K). Therefore, these findings indicated that scutellarin induced apoptosis in AML cells through the JNK/Caspase-3 pathway.
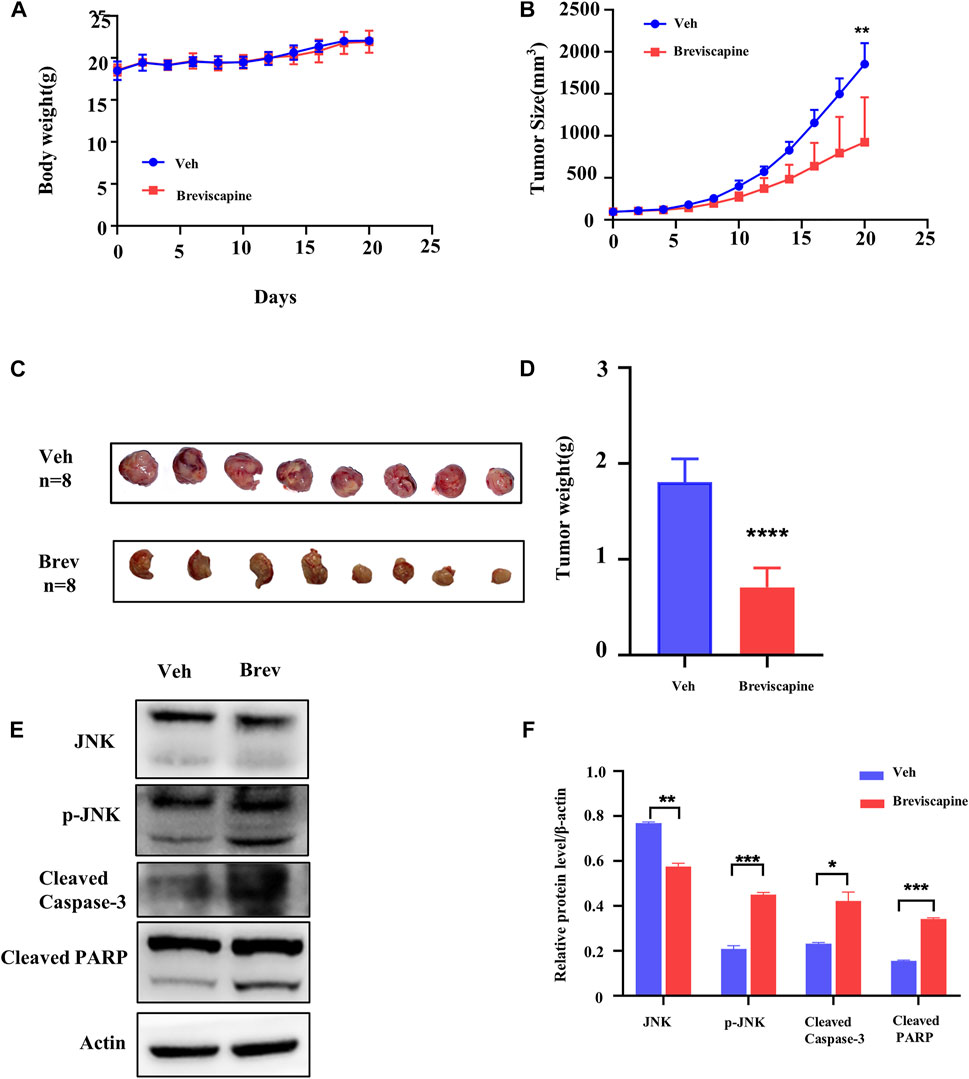
FIGURE 6. Scutellarin suppresses xenograft growth in vivo model. (A) The average body weight in breviscapine (60 mg/kg/d, po) and vehicle (normal saline, po) groups. (B) Xenograft size from nude mice in breviscapine and vehicle groups. (C) Xenograft excised from nude mice in each group. (D) The average weight of each xenograft. (E) The levels of JNK, p-JNK, cleaved Caspase-3 and cleaved PARP in the tumors with breviscapine treatment were evaluated by Western blotting. (F) The statistical analysis result of (E). Veh n = 8, Brev n = 8. The data were shown as the mean ± SD, **p < 0.01, ****p < 0.0001.
Scutellarin suppresses xenograft growth in vivo model
Next, we explored whether scutellarin was still effective in the animal models of AML. HL-60 cells were inoculated subcutaneously into both hind limbs of nude mice, and breviscapine, containing scutellarin ≥90%, was administered at 60 mg/kg/d orally, which was corresponding to the maximum dose in humans per the guide for dose conversion between humans and mice (Nair & Jacob, 2016). Breviscapine did not affect the body weight of the mice (Figure 6A). But it inhibited the xenograft growth significantly in nude mice (Figures 6B–D). Subsequently, proteins from tumor tissues were extracted and subjected to Western blot analysis. Consistent with the in vitro findings, p-JNK, cleaved Caspase-3 and cleaved PARP were significantly increased (Figures 6E,F).
Discussion
AML is a rapidly progressing hematopoietic malignancy whose global prevalence is rising. Although TCM has been proved to be a good choice for AML treatment, inferring the mechanism of action is challenging due to the complex chemical composition. The coincidence of the concept of network pharmacology and the theory of TCM makes the former suitable for the study of the mechanism of action of the latter (Luo et al., 2020).
This study employed a network pharmacology-based approach to infer the underlying molecular mechanism of scutellarin for treating AML, and also performed in vitro and in vivo experimental validation. First, we screened 289 potential targets for scutellarin from the PharmMapper database and retrieved 10998 AML-related genes from the OMIM, GeneCards, DrugBank, and PharmGkb databases. By merging targets of scutellarin with those of AML, a total of 253 overlapping genes were identified. Next, The results of PPI network analysis, GO and KEGG pathway analysis, revealed MAPK signaling pathway was critical for scutellarin-induced apoptosis. Thus, we chose the key proteins of MAPK and Caspase-3, a typical apoptosis-related protein for subsequent experimental validation.
The JNK signaling pathway regulates multiple cellular functions, such as apoptosis, survival, aging, cell proliferation, differentiation, and inflammation (Xu et al., 2015; Hammouda et al., 2020). It promotes apoptosis via the transcriptional regulation or phosphorylation of the key mitochondrial pro- and anti-apoptotic proteins (Dhanasekaran & Reddy, 2008). Modulation of the JNK pathway has been recognized as an effective avenue to treat cancers, including oral tumors, osteosarcoma, and colorectal cancer (Fu et al., 2020; Kon-Young Ji 2021; Liu et al., 2021), especially AML (Torres et al., 2010; Bomfim et al., 2019; Tian et al., 2019).
To verify the analysis of network pharmacology, we did in vitro and in vivo experiments. We found that scutellarin significantly induces apoptosis in AML cells. Additionally, Z-VAD-FMK, a specific inhibitor of apoptosis, significantly attenuated the pro-apoptotic effects of scutellarin. Accordingly, well-known apoptosis markers (Giuseppa Pistritto and claudia, 2016), such as cleaved PARP and cleaved Caspase-3, were induced by scutellarin dose-dependently. In addition, p-JNK was increased significantly with scutellarin treatment in HL-60 cells. Meanwhile, pretreatment with the JNK inhibitor SP600125 rescued scutellarin-induced apoptosis. Consistent with the in vitro data, scutellarin suppressed subcutaneous xenograft growth in nude mice, and the change of the protein levels in the JNK/Caspase-3 pathway was also recapitulated.
This study integrated network pharmacology-based prediction and experimental validation to uncover the importance of the JNK pathway in scutellarin-mediated AML treatment.
Data availability statement
The original contributions presented in the study are included in the article/Supplementary Material, further inquiries can be directed to the corresponding authors.
Ethics statement
The animal study was reviewed and approved by Ethics Committee of Experimental Animals, Southwest Medical University.
Author contributions
All authors listed have made a substantial, direct, and intellectual contribution to the work and approved it for publication.
Funding
This study was funded by the Science and Technology Project of Health Commission of Sichuan. (Project codes:19PJ288), the Basic Application Research of Sichuan Science and Technology Department (Project codes:2019YJ0690), the Key r&d projects of Sichuan Science and Technology Department (Project codes: 2019YFS0531), and the Science and Technology Development Fund, Macau SAR (File no. 0013/2019/A1 and 0036/2020/A1).
Conflict of interest
The authors declare that the research was conducted in the absence of any commercial or financial relationships that could be construed as a potential conflict of interest.
Publisher’s note
All claims expressed in this article are solely those of the authors and do not necessarily represent those of their affiliated organizations, or those of the publisher, the editors and the reviewers. Any product that may be evaluated in this article, or claim that may be made by its manufacturer, is not guaranteed or endorsed by the publisher.
Supplementary material
The Supplementary Material for this article can be found online at: https://www.frontiersin.org/articles/10.3389/fphar.2022.952677/full#supplementary-material
The target of Scutellarin predicted by PhamMapper.
The targets of AML predicted by OMIM, GeneCards, PharmGkb, rugBank.
Drug-Disease targets.
String-interactions.
Core genes.
GO.
KEGG.
References
Ari Pelcovits, R. N., and Niroula, R. (2020). Acute myeloid leukemia: A review. R. I. Med. J. 103 (3), 38–40.
Becker, J. S., and Fathi, A. T. (2020). Targeting IDH mutations in AML: Wielding the double-edged sword of differentiation. Curr. Cancer Drug Targets 20 (7), 490–500. doi:10.2174/1568009620666200424145622
Bomfim, L. M., de Araujo, F. A., Dias, R. B., Sales, C. B. S., Rocha, C. A. G., Correa, R. S., et al. (2019). Ruthenium(II) complexes with 6-methyl-2-thiouracil selectively reduce cell proliferation, cause DNA double-strand break and trigger caspase-mediated apoptosis through JNK/p38 pathways in human acute promyelocytic leukemia cells. Sci. Rep. 9 (1), 11483. doi:10.1038/s41598-019-47914-x
Chen, H. L., Jia, W. J., Li, H. E., Han, H., Li, F., Zhang, X. L., et al. (2020). Scutellarin exerts anti-inflammatory effects in activated microglia/brain macrophage in cerebral ischemia and in activated BV-2 microglia through regulation of MAPKs signaling pathway. Neuromolecular Med. 22 (2), 264–277. doi:10.1007/s12017-019-08582-2
Daver, N., Schlenk, R. F., Russell, N. H., and Levis, M. J. (2019). Targeting FLT3 mutations in AML: Review of current knowledge and evidence. Leukemia 33 (2), 299–312. doi:10.1038/s41375-018-0357-9
Dhanasekaran, D. N., and Reddy, E. P. (2008). JNK signaling in apoptosis. Oncogene 27 (48), 6245–6251. doi:10.1038/onc.2008.301
Dohner, H., Estey, E., Grimwade, D., Amadori, S., Appelbaum, F. R., Buchner, T., et al. (2017). Diagnosis and management of AML in adults: 2017 ELN recommendations from an international expert panel. Blood 129 (4), 424–447. doi:10.1182/blood-2016-08-733196
Dohner, H., Weisdorf, D. J., and Bloomfield, C. D. (2015). Acute myeloid leukemia. N. Engl. J. Med. 373 (12), 1136–1152. doi:10.1056/NEJMra1406184
Fu, C. Y., Lay, I. S., Shibu, M. A., Tseng, Y. S., Kuo, W. W., Yang, J. J., et al. (2020). Selective activation of ZAK beta expression by 3-hydroxy-2-phenylchromone inhibits human osteosarcoma cells and triggers apoptosis via JNK activation. Int. J. Mol. Sci. 21 (9), E3366. doi:10.3390/ijms21093366
Fu Li, S. W., Niu., M., and Niu, M. (2021). Scutellarin inhibits the growth and EMT of gastric cancer cells through regulating PTEN/PI3K pathway. Biol. Pharm. Bull. 44 (6), 780–788. doi:10.1248/bpb.b20-00822
Giuseppa Pistritto, D. T., Claudia, Ceci., Ceci, C., Garufi, A., and D'Orazi, G. (2016). Apoptosis as anticancer mechanism: Function and dysfunction of its modulators and targeted therapeutic strategies. AGING 8, 603–619. doi:10.18632/aging.100934<
Hammouda, M. B., Ford, A. E., Liu, Y., and Zhang, J. Y. (2020). The JNK signaling pathway in inflammatory skin disorders and cancer. Cells 9 (4), E857. doi:10.3390/cells9040857
Heuser, M. Y. O., Boissel, N., Mauri, B., Craddock, C., Janssen, J., Wierzbowska, A., et al. (2020). Acute myeloid leukaemia in adult patients: ESMO clinical practice guidelines for diagnosis, treatment and follow-up. Ann. Oncol. 31 (6), 697–712. doi:10.1016/j.annonc.2020.02.018
Huang, S., Zhang, Z., Li, W., Kong, F., Yi, P., Huang, J., et al. (2020). Network pharmacology-based prediction and verification of the active ingredients and potential targets of zuojinwan for treating colorectal cancer. Drug Des. devel. Ther. 14, 2725–2740. doi:10.2147/DDDT.S250991
Huang, X.-M., Huang, J.-j., Du, J.-j., Zhang, N., Long, Z., Yang, Y., et al. (2021). Autophagy inhibitors increase the susceptibility of KRAS-mutant human colorectal cancer cells to a combined treatment of 2-deoxy-D-glucose and lovastatin. Acta Pharmacol. Sin. 42, 1875–1887. doi:10.1038/s41401-021-00612-9
Huang, X. M., Wang, J., Lin, W. J., Zhang, N., Du, J. J., Long, Z., et al. (2020). Kanglaite injection plus platinum-based chemotherapy for stage III/IV non-small cell lung cancer: A meta-analysis of 27 RCTs. Phytomedicine. 67, 153154. doi:10.1016/j.phymed.2019.153154
Kon-Young Ji, K. M. K., Yun Hee, K., Ki-Shuk, S., Joo Young, L., Taesoo, K., Sungwook, C., et al. (2021). Serum starvation sensitizes anticancer effect of anemarrhena asphodeloides via p38/JNK-induced cell cycle arrest and apoptosis in colorectal cancer cells. Am. J. Chin. Med. 49, 1001–1016. doi:10.1142/S0192415X21500488
Liu, K., Tian, T., Zheng, Y., Zhou, L., Dai, C., Wang, M., et al. (2019). Scutellarin inhibits proliferation and invasion of hepatocellular carcinoma cells via down-regulation of JAK2/STAT3 pathway. J. Cell. Mol. Med. 23 (4), 3040–3044. doi:10.1111/jcmm.14169
Liu, Y. T., Ho, H. Y., Lin, C. C., Chuang, Y. C., Lo, Y. S., Hsieh, M. J., et al. (2021). Platyphyllenone induces autophagy and apoptosis by modulating the AKT and JNK mitogen-activated protein kinase pathways in oral cancer cells. Int. J. Mol. Sci. 22 (8), 4211. doi:10.3390/ijms22084211
Luo, J., Shang, Q. H., Han, M., Chen, K. J., and Xu, H. (2014). Traditional Chinese medicine injection for angina pectoris: An overview of systematic reviews. Am. J. Chin. Med. 42 (1), 37–59. doi:10.1142/s0192415x14500037
Luo, T. T., Lu, Y., Yan, S. K., Xiao, X., Rong, X. L., and Guo, J. (2020). Network pharmacology in research of Chinese medicine formula: Methodology, application and prospective. Chin. J. Integr. Med. 26 (1), 72–80. doi:10.1007/s11655-019-3064-0
Nair, A. B., and Jacob, S. (2016). A simple practice guide for dose conversion between animals and human. J. Basic Clin. Pharm. 7 (2), 27–31. doi:10.4103/0976-0105.177703
Reedijk, A. M. J., Klein, K., Coebergh, J. W. W., Kremer, L. C., Dinmohamed, A. G., de Haas, V., et al. (2019). Improved survival for children and young adolescents with acute myeloid leukemia: A Dutch study on incidence, survival and mortality. Leukemia 33 (6), 1349–1359. doi:10.1038/s41375-018-0314-7
Roberts, A. W. (2020). Therapeutic development and current uses of BCL-2 inhibition. Hematol. Am. Soc. Hematol. Educ. Program 1, 1–9. doi:10.1182/hematology.2020000154
Sallman, D. A. K. T., Poire, X., Havelange, V., Lewalle, P., Davila, M. L., Wang, E. S., et al. (2018). Remissions in relapse/refractory acute myeloid leukemia patients following treatment with NKG2D CAR-T therapy without a prior preconditioning chemotherapy. Blood 132, 902. doi:10.1182/blood-2018-99-111326
Takami, A. (2018). Hematopoietic stem cell transplantation for acute myeloid leukemia. Int. J. Hematol. 107 (5), 513–518. doi:10.1007/s12185-018-2412-8
Tan, L., Lei, N., He, M., Zhang, M., Sun, Q., Zeng, S., et al. (2020). Scutellarin protects against human colorectal cancer in vitro by down regulation of hedgehog signaling pathway activity. Int. J. Pharmacol. 16, 53–62. doi:10.3923/ijp.2020.53.62
Tang, S. L., Gao, Y. L., and Hu, W. Z. (2019). Scutellarin inhibits the metastasis and cisplatin resistance in glioma cells. Onco. Targets. Ther. 12, 587–598. doi:10.2147/OTT.S187426
Thomas, D., and Majeti, R. (2017). Biology and relevance of human acute myeloid leukemia stem cells. Blood 129 (12), 1577–1585. doi:10.1182/blood-2016-10-696054
Tian, Y., Jia, S. X., Shi, J., Gong, G. Y., Yu, J. W., Niu, Y., et al. (2019). Polyphyllin I induces apoptosis and autophagy via modulating JNK and mTOR pathways in human acute myeloid leukemia cells. Chem. Biol. Interact. 311, 108793. doi:10.1016/j.cbi.2019.108793
Torres, F., Quintana, J., and Estevez, F. (2010). 5, 7, 3'-trihydroxy-3, 4'-dimethoxyflavone-induced cell death in human leukemia cells is dependent on caspases and activates the MAPK pathway. Mol. Carcinog. 49 (5), 464–475. doi:10.1002/mc.20619
Wang, L., and Ma, Q. (2018). Clinical benefits and pharmacology of scutellarin: A comprehensive review. Pharmacol. Ther. 190, 105–127. doi:10.1016/j.pharmthera.2018.05.006
Xiang, Y., Guo, Z., Zhu, P., Chen, J., and Huang, Y. (2019). Traditional Chinese medicine as a cancer treatment: Modern perspectives of ancient but advanced science. Cancer Med. 8 (5), 1958–1975. doi:10.1002/cam4.2108
Xu, G., Zhang, L., Ma, A., Qian, Y., Ding, Q., Liu, Y., et al. (2015). SIP1 is a downstream effector of GADD45G in senescence induction and growth inhibition of liver tumor cells. Oncotarget 6, 33636–33647. doi:10.18632/oncotarget.5602
Xu, H., and Zhang, S. (2013). Scutellarin-induced apoptosis in HepG2 hepatocellular carcinoma cells via a STAT3 pathway. Phytother. Res. 27 (10), 1524–1528. doi:10.1002/ptr.4892
Xu, L., Chen, R., Zhang, X., Zhu, Y., Ma, X., Sun, G., et al. (2021). Scutellarin protects against diabetic cardiomyopathy via inhibiting oxidative stress and inflammatory response in mice. Ann. Palliat. Med. 10 (3), 2481–2493. doi:10.21037/apm-19-516
Yilmaz, M., and Daver, N. (2019). From DNA sequencing to clinical trials: Finding new targeted drugs for acute myeloid leukemia. Drugs 79 (11), 1177–1186. doi:10.1007/s40265-019-01144-7
Zeng, S., Chen, L., Sun, Q., Zhao, H., Yang, H., Ren, S., et al. (2021). Scutellarin ameliorates colitis-associated colorectal cancer by suppressing Wnt/β-catenin signaling cascade. Eur. J. Pharmacol. 906, 174253. doi:10.1016/j.ejphar.2021.174253
Keywords: Scutellarin, AML, network pharmacology, JNK/Caspase-3 signal pathway, apoptosis
Citation: Huang Z, Yang Y, Fan X and Ma W (2022) Network pharmacology-based investigation and experimental validation of the mechanism of scutellarin in the treatment of acute myeloid leukemia. Front. Pharmacol. 13:952677. doi: 10.3389/fphar.2022.952677
Received: 25 May 2022; Accepted: 08 August 2022;
Published: 07 September 2022.
Edited by:
Mounir Tilaoui, Waterford Institute of Technology, IrelandReviewed by:
Eswar Shankar, The Ohio State University, United StatesGaurav Kandoi, Invaio Sciences, United States
Copyright © 2022 Huang, Yang, Fan and Ma. This is an open-access article distributed under the terms of the Creative Commons Attribution License (CC BY). The use, distribution or reproduction in other forums is permitted, provided the original author(s) and the copyright owner(s) are credited and that the original publication in this journal is cited, in accordance with accepted academic practice. No use, distribution or reproduction is permitted which does not comply with these terms.
*Correspondence: Xianming Fan, ZnhtMTI5MTIwQHNpbmEuY29t; Wenzhe Ma, d3ptYUBtdXN0LmVkdS5tbw==
†These authors have contributed equally to this work