- 1Human Immunology Research and Education Group-GEPIH, Federal University of Paraiba, João Pessoa, Brazil
- 2Postgraduate Program of Pharmaceutical Sciences, State University of Paraíba, Campina Grande, Brazil
- 3Postgraduate Program of Science and Technology in Health, State University of Paraíba, Campina Grande, Brazil
- 4Postgraduate Program of Therapeutic Innovation, Federal University of Pernambuco, Recife, Brazil
- 5Postgraduate Program in Dentistry, Federal University of Paraíba, João Pessoa, Brazil
- 6Department of Otolaryngology and Head and Neck Surgery, McGill University, Montreal, QC, Canada
- 7Segal Cancer Centre and Lady Davis Institute for Medical Research, Departments of Medicine and Oncology, Faculty of Medicine, McGill University, Montreal, QC, Canada
- 8Postgraduate Course in Health Sciences, Federal University of Triangulo Mineiro, UFTM, Uberaba, Brazil
Gastric cancer is the fifth most common and fourth type to cause the highest mortality rates worldwide. The leading cause is related to Helicobacter pylori (H. pylori) infection. Unfortunately, current treatments have low success rates, highlighting the need for alternative treatments against carcinogenic agents, specifically H. pylori. Noteworthy, natural origin products contain pharmacologically active metabolites such as flavonoids, with potential antimicrobial applications.
Objective: This article overviews flavonoid-rich extracts’ biological and pharmacological activities. It focuses on using these substances against Helicobacter pylori infection to prevent gastric cancer. For this, PubMed and Science Direct databases were searched for studies that reported the activity of flavonoids against H. pylori, published within a 10-year time frame (2010 to August 2020). It resulted in 1,773 publications, of which 44 were selected according to the search criteria. The plant family primarily found in publications was Fabaceae (9.61%). Among the flavonoids identified after extraction, the most prevalent were quercetin (19.61%), catechin (13.72), epicatechin (11.76), and rutin (11.76). The potential mechanisms associated with anti-H. pylori activity to the extracts were: inhibition of urease, damage to genetic material, inhibition of protein synthesis, and adhesion of the microorganism to host cells.
Conclusion: Plant extracts rich in flavonoids with anti-H. pylori potential proved to be a promising alternative therapy source, reinforcing the relevance of studies with natural products.
Introduction
Gastric cancer represents the fifth most common type of cancer (1.08 million cases in 2020). It is the fourth most common cause of death among cancers worldwide (768.000 deaths in 2020) due to the advanced stage at diagnosis (Smyth et al., 2020; Sung et al., 2021). Incidence and mortality vary considerably between regions, although it is more prevalent in developing countries, in which the percentage of cases is equivalent to 70%, especially in East Asia. In addition, the incidence of gastric cancer is proportional to population age, with an average of 68 years, and is more common in men (1 in each 96) than in women (1 in each 152) (Herrero et al., 2014; medical and editorial content Team, 2018).
According to the topology, gastric cancer can be classified into cardia, usually associated with gastroesophageal reflux, and non-cardia or distal gastric cancer, caused by the interaction with different factors (Nardone, 2006; de Martel et al., 2013). The latter is histologically subdivided according to Laurén’s classification in diffuse and intestinal (Lauren, 1965). The diffuse type consists of individually infiltrated neoplastic cells without glandular structures. In contrast, the intestinal type mimics the intestinal glands. It progresses through a series of histological changes that begin with transitioning from normal mucosa to chronic superficial gastritis, followed by atrophic gastritis, intestinal metaplasia, dysplasia, and adenocarcinoma (Nardone, 2006; Polk and Peek, 2010). For both types of non-cardiac gastric cancer, the main factor associated with developing approximately 90% of adenocarcinomas is the bacterium Helicobacter pylori (H. pylori) (Peek, 2005; Plummer et al., 2015, 2016; Moss, 2017; Thrift and El-Serag, 2020).
H. pylori is a gram-negative, flagellated, microaerophilic bacteria that infects the epithelial lining of the stomach (Tsukamoto and Tatematsu, 2014; Hooi et al., 2017). The infection is prevalent in approximately 50% of the world population, varying according to geographic region, age, socioeconomic status, education level, environment, and occupation. It is usually contracted in the first years of life and tends to persist until the completion of the appropriate treatment (McColl, 2010; Cui et al., 2013; Wang et al., 2014; Schaalan et al., 2020).
The inflammatory process developed by H. pylori infection involves a variety of pathways induced in both gastric epithelial cells and circulating immune cells recruited to the infection site. Activated pathways involve mitogen-activated protein kinase (MAPK), nuclear factor-κB (NF-kB), activating protein (AP)-1, Wnt/β-catenin, PI3K pathways, signal transducers and transcription activators 3 (STAT3). These alterations cause an increase in the production of inflammatory cytokines, such as interleukin 1 (IL-1), IL-6, IL-8, and tumor necrosis factor-alpha (TNF-α). Also, they alter the apoptosis rate and proliferation and differentiation of epithelial cells. These phenomena result in the oncogenic transformation of epithelial cells and gastric cancer formation (Ding et al., 2010; Lamb and Chen, 2013; Schaalan et al., 2020). In addition, virulence factors contribute to determining the pattern of immune defense performed in response to the infection. It includes the factors named vacuolating cytotoxin (VacA), cytotoxin-associated antigen A (CagA), the Cag pathogenicity island (PAI), HP-NAP, oipA, and dupA (Ding et al., 2010; Sepulveda, 2013; Wang et al., 2014; Yamaoka, 2010, 2012).
The clinical condition produced by the microorganism in question is usually asymptomatic. However, the infection caused is associated with gastrointestinal diseases, such as chronic gastritis, peptic ulcer disease, gastric B-cell mucosa-associated lymphoid tissue lymphoma, and, as aforementioned, gastric adenocarcinoma. Thereby, H. pylori was recognized and classified as a definite (group 1) carcinogen by the World Health Organization’s International Agency for Research on Cancer in 1994 (WHO, 2010; de Martel et al., 2013; Plummer et al., 2016; Moss, 2017). Additionally, studies demonstrate that eradicating H. pylori decreases the risk of developing cancer in individuals without pre-malignant lesions. It reinforces that this infection influences the early stages of gastric carcinogenesis (Polk and Peek, 2010).
Currently, the treatment for gastric cancer consists of surgical intervention associated with chemotherapy using 5-fluorouracil (5-FU), platinum, taxane, irinotecan, and anthracycline (Ma et al., 2016). The treatment options for tumors associated with H. pylori are antibiotics (clarithromycin and amoxicillin or metronidazole), proton pump inhibitors, and bismuth (Lamb and Chen, 2013). However, surgery remains the only curative therapy. At the same time, chemotherapy can improve the outcome, thus emphasizing the importance of employing preventive recourses (Orditura et al., 2014).
Alternatively, there is an increase in the use of natural products. One of the most investigated sources is the plants, representing 25% of the medical industry (Ji et al., 2009; Lahlou, 2013; Calixto, 2019). Botanical drugs contain active metabolites with pharmacological activities capable of relieving symptoms or curing diseases (Trojan-Rodrigues et al., 2012; Ferreira et al., 2014). Thus, the application of ethnopharmacology has collaborated in discovering new chemical entities, mainly through the bioprospecting of secondary metabolites (Albino et al., 2020).
Among the most known and studied ethnobotanical constituents are flavonoids. They possess a polyphenolic benzo-
The antimicrobial potential of flavonoids against H. pylori has been described in the literature by several studies (Loo, 1997; Bae et al., 1999; Shin et al., 2005; Lee et al., 2006; Cushnie and Lamb, 2011; Pandey and Kumar, 2013; González et al., 2019). In addition to demonstrating an in vitro action against this bacterium, these compounds could also promote synergistic interactions with antibiotics commonly used in treatments against H. pylori infections (Krzyżek et al., 2021). The pathways of action of flavonoids can be diverse. Some mechanisms in H. pylori have already been described, such as inhibition of the essential function of HsrA (González et al., 2019), mediation of the response to oxidative stress (Olekhnovich et al., 2013, 2014; Pelliciari et al., 2017), interactions with virulence factors (Kim et al., 2021), recognition of molecular targets including secretion systems (Yeon et al., 2019) and enzymes (Wu et al., 2008; Zhang et al., 2008) acting on pathways that lead to changes in cell morphology of H. pylori (Krzyżek et al., 2021). In turn, the broad pharmacotherapeutic and biochemical spectrum of flavonoids and the possible contribution of these compounds to improving human health make such substances increasingly explored (Tungmunnithum et al., 2018). Still, these findings support the valuable potential of flavonoids as candidate botanical drugs for novel antibacterial and anticancer strategies. The most promising flavonoid compounds are Catechin, Epicatechin, Kaempferol, Luteolin, Morin, Myricetin, Naringenin, Naringin, Quercetin, Hyperoside, and Rutin, In this way, this article provides an overview of the biological and pharmacological activities of flavonoid-rich plant extracts with a focus on the use of these substances against H. pylori infection in the prevention of gastric cancer.
Materials and Methods
The Question Under Analysis
This review was guided by the question: “Are flavonoids-rich plant extracts a promising alternative in treatments against Helicobacter pylori infection and preventing gastric cancer?”
Search Strategy and Articles Selection
PRISMA guidelines were followed (Liberati et al., 2009; Moher et al., 2009). In addition, an electronic search was performed in the PubMed and Science Direct databases from studies published between 2010 to August 2020, with the keywords “flavonoids” and “Helicobacter pylori.”
Studies Selection
Eligible studies followed the criteria: 1) pre-clinical in vitro and in vivo; 2) studies with rodents and cells; 3) any type of treatment that used plant extracts containing flavonoids in its composition; 4) studies with positive or negative control; 5) no language restriction. Clinical research, studies with other than flavonoids that did not determine the value of the tested dose, with flavonoids in their isolated form, and studies that used flavonoids as control compounds, were excluded
Two independent reviewers selected the studies. In the first screening, titles and abstracts were evaluated, and studies considered irrelevant were excluded. The two reviewers read the articles for each potential manuscript and evaluated them based on the inclusion criteria. Duplicate studies between the bases were excluded. A third reviewer was contacted in the presence of inconsistency between the two examiners. Thus, 44 articles were selected for this review that reports the activity of plant species extracts containing flavonoids against H. pylori.
Results
The initial search of the databases (with the strategies presented in Table 1) allowed the identification of 1,773 publications. Review studies, meta-analyzes, encyclopedias, book chapters, abstracts, conference proceedings, editorials/letters, and case reports were excluded. The 567 articles left were screened based on titles and abstracts for the inclusion criteria mentioned above. At this stage, 128 articles remained, following the exclusion of 439 articles. Subsequently to the removal of 25 repeated articles, 103 remained. These studies were afterward entirely read. Finally, 44 articles were selected, as 59 articles did not meet all inclusion criteria (Figure 1). The selected studies were concentrated between 2010 and 2020 and are considered current.
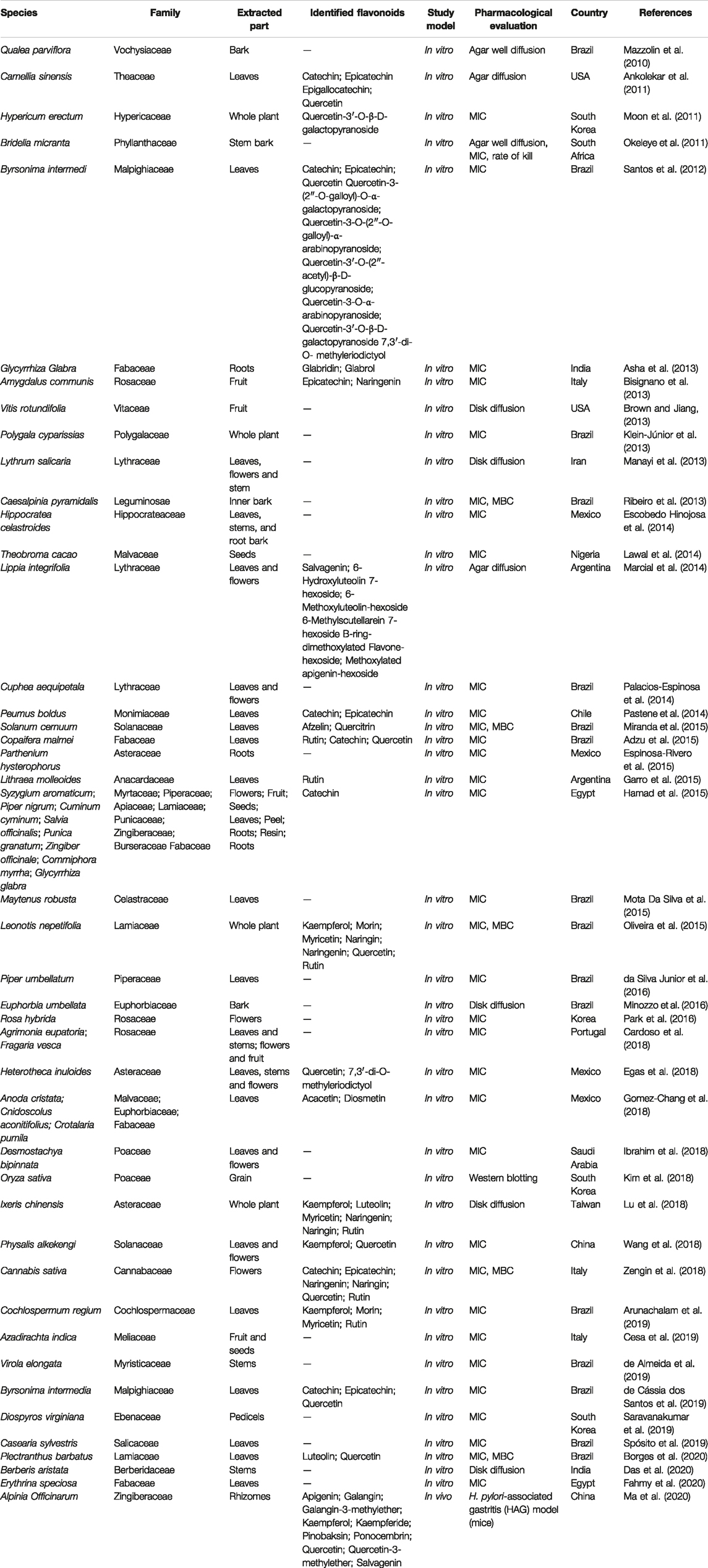
TABLE 1. Pharmacobotanical information, extracts and tests involving activity against H. pylori of flavonoids contained in plant species.
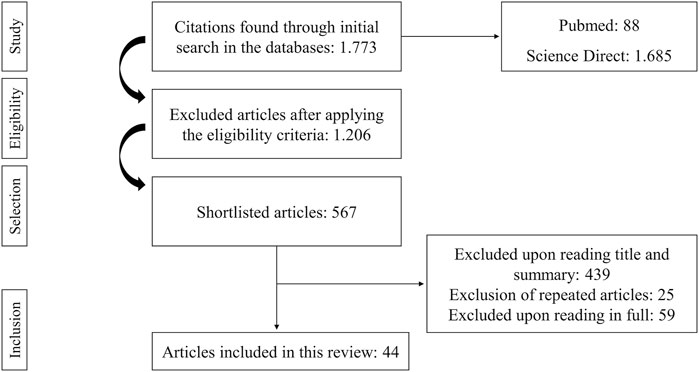
FIGURE 1. Flowchart of article selection for the systematic review. The bibliographic study started with 1.773 articles. After applying the eligibility criteria, 567 remained. Four hundred thirty-nine were excluded after reading the title, 25 excluded by repetition, and 59 excluded after full reading. Forty-four articles fit the purpose and were selected for this review.
There was variability in the study regions for the selected manuscripts, with 25% of the papers coming from Asia (Moon et al., 2011; Asha et al., 2013; Manayi et al., 2013; Wang et al., 2014; Park et al., 2016; Ibrahim et al., 2018; Kim et al., 2018; Lu et al., 2018; Saravanakumar et al., 2019; Ma X. et al., 2020; Das et al., 2020), 56.82% from America, of which 40.91% were from South America (Adzu et al., 2015; Almeida et al., 2019; Arunachalam et al., 2019; Borges et al., 2020; da Silva Junior et al., 2016; de Cássia dos Santos et al., 2019; Garro et al., 2015; Klein-Júnior et al., 2013; Marcial et al., 2014; Mazzolin et al., 2010; Minozzo et al., 2016; Miranda et al., 2015; Mota Da Silva et al., 2015; Oliveira et al., 2015; Pastene et al., 2014; Ribeiro et al., 2013; Santos et al., 2012; Spósito et al., 2019) and 15.91% from North America (Ankolekar et al., 2011; Brown and Jiang, 2013; Escobedo Hinojosa et al., 2014; Palacios-Espinosa et al., 2014; Espinosa-Rivero et al., 2015; Egas et al., 2018; Gomez-Chang et al., 2018), 9.09% of the articles originated from the Africa (Okeleye et al., 2011; Lawal et al., 2014; Hamad et al., 2015; Fahmy et al., 2020), and 9,09% from the Europe (Bisignano et al., 2013; Cardoso et al., 2018; Zengin et al., 2018; Cesa et al., 2019).
Among the Asian countries, South Korea represented 9.09% of the publications (Moon et al., 2011; Park et al., 2016; Kim et al., 2018; Saravanakumar et al., 2019), India (Asha et al., 2013; Das et al., 2020) and China (Wang et al., 2018; Ma X. et al., 2020) both 4.55%, Iran (Manayi et al., 2013), Saudi Arabia (Ibrahim et al., 2018), and Taiwan (Lu et al., 2018) 2.27%. In South America, Brazil (Adzu et al., 2015; Almeida et al., 2019; Arunachalam et al., 2019; Borges et al., 2020; da Silva Junior et al., 2016; de Cássia dos Santos et al., 2019; Klein-Júnior et al., 2013; Mazzolin et al., 2010; Minozzo et al., 2016; Miranda et al., 2015; Mota Da Silva et al., 2015; Oliveira et al., 2015; Ribeiro et al., 2013; Santos et al., 2012; Spósito et al., 2019) represented 34.09%, Argentina (Marcial et al., 2014; Garro et al., 2015) 4.55%, and Chile (Pastene et al., 2014) 2.27%. In North America, Mexico (Escobedo Hinojosa et al., 2014; Palacios-Espinosa et al., 2014; Espinosa-Rivero et al., 2015; Egas et al., 2018; Gomez-Chang et al., 2018) represented 11.36% and the United States (Ankolekar et al., 2011; Brown and Jiang, 2013) 4.55%. In the Africa, Egypt (Hamad et al., 2015; Fahmy et al., 2020) represented 4.55%, Nigeria (Lawal et al., 2014) and South Africa (Okeleye et al., 2011) both 2.27%. In the Europe, Italy (Bisignano et al., 2013; Zengin et al., 2018; Cesa et al., 2019) represented 6.82% and Portugal (Cardoso et al., 2018) 2.27% of publications.
The plant families used in the studies were Anacardaceae (Garro et al., 2015), Apiaceae (Hamad et al., 2015), Asteraceae (Espinosa-Rivero et al., 2015; Egas et al., 2018; Lu et al., 2018), Berberidaceae (Das et al., 2020), Burseraceae (Hamad et al., 2015), Cannabaceae (Zengin et al., 2018), Celastraceae (Mota Da Silva et al., 2015), Cochlospermaceae (Arunachalam et al., 2019), Ebenaceae (Saravanakumar et al., 2019), Euphorbiaceae (Minozzo et al., 2016; Gomez-Chang et al., 2018), Fabaceae (Asha et al., 2013; Adzu et al., 2015; Hamad et al., 2015; Gomez-Chang et al., 2018; Fahmy et al., 2020), Hippocrateaceae (Escobedo Hinojosa et al., 2014), Hypericaceae (Moon et al., 2011), Lamiaceae (Oliveira et al., 2015), Leguminosae (Ribeiro et al., 2013), Lythraceae (Manayi et al., 2013; Marcial et al., 2014; Palacios-Espinosa et al., 2014), Malpighiaceae (Santos et al., 2012; de Cássia dos Santos et al., 2019), Malvaceae (Lawal et al., 2014; Gomez-Chang et al., 2018), Meliaceae (Cesa et al., 2019), Monimiaceae (Pastene et al., 2014), Myristicaceae (de Almeida et al., 2019), Myrtaceae (Hamad et al., 2015), Phyllanthaceae (Okeleye et al., 2011), Piperaceae (da Silva Junior et al., 2016; Hamad et al., 2015), Poaceae (Ibrahim et al., 2018; Kim et al., 2018), Polygalaceae (Klein-Júnior et al., 2013), Rosaceae (Bisignano et al., 2013; Park et al., 2016; Cardoso et al., 2018), Salicaceae (Spósito et al., 2019), Solanaceae (Abreu Miranda et al., 2015; Wang et al., 2018), Theaceae (Ankolekar et al., 2011), Vitaceae (Brown and Jiang, 2013), Vochysiaceae (Mazzolin et al., 2010) and Zingiberaceae (Hamad et al., 2015; Ma X. et al., 2020). The most prominent family in these studies was the Fabaceae.
Between the flavonoids identified in the extracts (Table 2), quercetin (Ankolekar et al., 2011; Santos et al., 2012; Adzu et al., 2015; Oliveira et al., 2015; Egas et al., 2018; Wang et al., 2018; Zengin et al., 2018; de Cássia dos Santos et al., 2019; Ma X. et al., 2020; Borges et al., 2020), catechin (Ankolekar et al., 2011; Santos et al., 2012; Pastene et al., 2014; Adzu et al., 2015; Hamad et al., 2015; Zengin et al., 2018; de Cássia dos Santos et al., 2019), epicatechin (Ankolekar et al., 2011; Santos et al., 2012; Bisignano et al., 2013; Pastene et al., 2014; Zengin et al., 2018; de Cássia dos Santos et al., 2019), rutin (Adzu et al., 2015; Garro et al., 2015; Oliveira et al., 2015; Lu et al., 2018; Zengin et al., 2018; Arunachalam et al., 2019), kaempferol (Oliveira et al., 2015; Lu et al., 2018; Wang et al., 2018; Arunachalam et al., 2019; Ma X. et al., 2020), naringenin (Bisignano et al., 2013; Oliveira et al., 2015; Lu et al., 2018; Zengin et al., 2018), naringin (Oliveira et al., 2015; Lu et al., 2018; Zengin et al., 2018), luteolin (Lu et al., 2018; Borges et al., 2020; Fahmy et al., 2020), myricetin (Oliveira et al., 2015; Lu et al., 2018; Arunachalam et al., 2019), morin (Oliveira et al., 2015; Arunachalam et al., 2019), and quercetin-3′-O-β-D-galactopyranoside (Moon et al., 2011; Santos et al., 2012) stood out.
The tests for determination of antimicrobial activity included the evaluation of minimum inhibitory concentration (MIC) (Adzu et al., 2015; Arunachalam et al., 2019; Asha et al., 2013; Bisignano et al., 2013; Borges et al., 2020; Cardoso et al., 2018; Cesa et al., 2019; da Silva Junior et al., 2016; de Almeida et al., 2019; de Cássia dos Santos et al., 2019; Egas et al., 2018; Escobedo Hinojosa et al., 2014; Espinosa-Rivero et al., 2015; Fahmy et al., 2020; Garro et al., 2015; Gomez-Chang et al., 2018; Hamad et al., 2015; Ibrahim et al., 2018; Klein-Júnior et al., 2013; Lawal et al., 2014; Moon et al., 2011; Mota Da Silva et al., 2015; Okeleye et al., 2011; Oliveira et al., 2015; Palacios-Espinosa et al., 2014; Park et al., 2016; Pastene et al., 2014; Ribeiro et al., 2013; Santos et al., 2012; Saravanakumar et al., 2019; Spósito et al., 2019; Wang et al., 2018; Zengin et al., 2018), minimum bactericidal concentration (MBC) (Ribeiro et al., 2013; Abreu Miranda et al., 2015; Oliveira et al., 2015; Zengin et al., 2018; Borges et al., 2020), disk diffusion (Brown and Jiang, 2013; Manayi et al., 2013; Minozzo et al., 2016; Lu et al., 2018; Das et al., 2020), well agar diffusion (Mazzolin et al., 2010; Okeleye et al., 2011), agar diffusion (Ankolekar et al., 2011; Marcial et al., 2014), rate of kill (Okeleye et al., 2011), and H. pylori-associated gastritis (HAG) in vivo model (mice) (Ma X. et al., 2020).
Discussion
The use of natural products and synthetic variations of their structures is the primary source of novel chemical entities approved as drugs by federal regulatory agencies. Despite the significant advance in combinatorial chemistry, discovering new active compounds through exclusively synthetic routes does not fulfill the role of presenting itself as a primary source of therapeutic innovation. In silico analysis has been used as an optimization tool to identify natural compounds as a valuable alternative to the pharmaceutical industry (Newman and Cragg, 2020).
Natural products and their derivatives represent more than a third of all newly discovered molecular entities approved by the FDA (Food and Drugs Administration). Notably, about 25% are of plant origin (Patridge et al., 2016). Sixty-four percent of these compounds have been used to treat neoplastic diseases. For example, of the 126 drugs discovered between 1981 and 2019, 78 (48%) are natural products for antibacterials.
Flavonoids
The pharmacological potential of medicinal plants is given by the chemical structures produced by secondary plant metabolism. It presents several biosynthesis mechanisms capable of supplying substances with complex chemical structures. In addition, these structures are responsible for specialized intrinsic functions, favoring the activity in biological environments. They generally possess pharmacophoric regions, which are intricate to create or reproduce through organic synthesis (Mendes et al., 2012).
Flavonoids are among the main classes of secondary metabolites with pharmacological relevance (Wang et al., 2019). These phenolic compounds act on plants as adaptive agents, playing a crucial role in the survival of species against environmental stresses and in response to invasions by microorganisms. This natural function of flavonoids explains the growing interest in studying these compounds in searching for new drugs with antimicrobial activity, mainly due to the lack of effective therapies in the current clinical scenario (Biharee et al., 2020).
Moreover, flavonoids represent one of the most important and diversified phenolic groups among natural metabolites. Its occurrence is often associated with the color of plants, and it is frequently found in flowers, fruits, leaves, stems, and seeds (Zuanazzi and Montanha, 2007). The word “Flavonoid” derives from the Latin “Flavus,” which means light yellow. However, the color of the flavonoid often varies according to the species. The basic skeleton of the flavonoids (Figure 2) has a tricyclic structure with 15 carbon atoms, with a chromatic ring (A) fused to a pinane ring (C) connected to an aromatic ring (B), leading to the subcategories of flavonoids (Zuanazzi and Montanha, 2007; Batra and Sharma, 2013; Biharee et al., 2020).
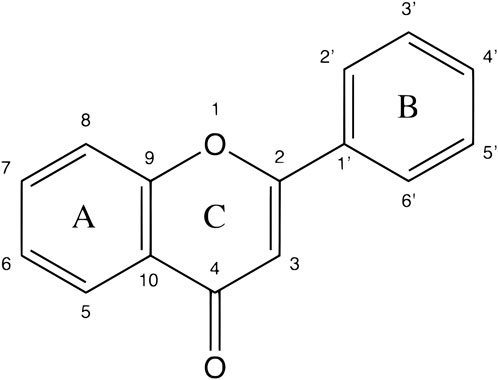
FIGURE 2. A basic chemical skeleton of flavonoids. General structural representation of a flavonoid, characteristic of 15 carbon atoms in its basic skeleton, corresponding to two benzene rings (A and B) linked by a pyran ring (C).
Literature reports have shown that flavonoids play a role in plant survival by preventing the spread of fungal and bacterial pathogens (Cho and Lee, 2015; Piasecka et al., 2015). Furthermore, flavonoid biosynthesis is closely related to defense responses in plant tissues. Significantly, it interferes in the vascular strands of leaves, which are most exposed and susceptible to contamination (Beck and Stengel, 2016). These findings support the hypothesis that flavonoids are potential antimicrobial agents that may be effective against human pathogens.
Due to the great diversity of compounds within the flavonoid group, the highly concentrated extracts exhibit more diverse mechanisms of antimicrobial activity. In other words, they target more components and functions of bacterial cells than other plant secondary metabolites (Cushnie and Lamb, 2005; Górniak et al., 2019). Other advantages of flavonoid-rich extracts with antimicrobial action compared to others are summarized in Table 3.
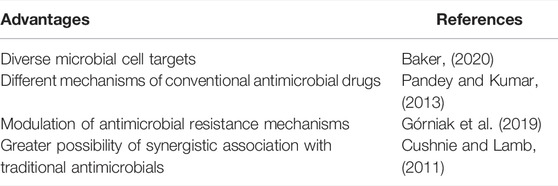
TABLE 3. Advantages of flavonoid-rich extracts with antimicrobial activities compared to other extracts.
Catechin and Epicatechin
Catechins, such as catechin and epicatechin, are flavonoids in plants, fruits (e.g., apple, strawberry, kiwi), black and green tea, red wine, beer, chocolate, and cocoa, among others (Grzesik et al., 2018). For example, green tea has a high catechin concentration (Botten et al., 2015), about 1 g/ml in a teacup (Rahardiyan, 2019). Approximately 5–7% of this concentration is epicatechin (Braicu et al., 2013).
Catechins’ antimicrobial activity is related to the interaction of this compound with the cell wall and inner membrane of bacteria and hydrogen peroxide production. One of the proposed mechanisms of action is related to the formation of high molecular mass complexes between this compound and proteins on the surface of the bacterial cell wall. It interrupts substrate transit between the intra and extracellular environment, inhibiting bacterial cell activity (Nakayama et al., 2013).
Some studies report catechin’s antimicrobial activity against gram-positive and gram-negative bacteria. It includes species like Escherichia coli (E. coli) (Bernal-Mercado et al., 2018), H. pylori (Díaz-Gómez et al., 2013), Staphylococcus aureus (S. aureus) (Martins et al., 2011; Sinsinwar and Vadivel, 2020), and Bacillus subtilis (B. subtilis) (Fathima and Rao, 2016).
Kaempferol
Kaempferol is a natural flavonol present in many edible plants (e.g., broccoli, cabbage, beans, tomatoes, and strawberries) as well as in traditional medicine (e.g., Ginkgo biloba L. (Ginkgoaceae); Moringa oleífera Lamarck (Moringaceae) (Saldanha et al., 2019). This compound and its glycosides possess several pharmacological properties, such as antioxidant, anti-inflammatory, antidiabetic, anticancer, cardioprotective, neuroprotective, anti-steroidal, anxiolytic, estrogenic/anti-estrogenic, analgesic, anti-allergic and antimicrobial (Mbaveng et al., 2014; Shields, 2017).
Kaempferol’s antimicrobial activity may be associated with its ability to form complexes with the bacterial cell wall, which causes the inhibition of microbial growth (Tatsimo et al., 2012). In addition, the compound blocked the formation of S. aureus biofilm in the initial adhesion stage (Ming et al., 2017). This inhibition probably occurs due to the inhibition of enzymes responsible for the beginning of biofilm formation and promoting suppression of the expression of genes of some surface proteins involved in adhesion.
In addition to S. aureus, kaempferol and its glycosides have reported activity against H. pylori (Escandón et al., 2016), E. coli (Wu et al., 2013), Pseudomonas aeruginosa (P. aeruginosa) (Tatsimo et al., 2012), Vibrio parahaemolyticus (V. parahaemolyticus), Bacillus cereus (B. cereus), Bacillus licheniformis (B. licheniformis) (Sivasothy et al., 2013), and Enterococcus faecalis (E. faecalis) (del Valle et al., 2016).
Luteolin
Luteolin is a flavone naturally found in its glycosylated structure in many edible plant species (e.g., carrot, pepper, peppermint, and oregano) (Omar, 2017). Its pharmacological activities include antioxidant, anti-inflammatory, neuroprotective, anticancer, antidiabetic, and antimicrobial (Dong et al., 2017; Shukla et al., 2019).
Luteolin demonstrates antimicrobial activity against the uropathogenic E. coli (UPEC) strain (fei Shen et al., 2014). The compound reduces UPEC adhesion to urinary epithelium cells by decreasing the expression of adhesion proteins in the microorganism’s fimbriae. Furthermore, Luteolin reduced the expression of adhesion-related genes and increased the hydrophilicity, inhibiting biofilm formation.
Other studies also describe the activities of luteolin against H. pylori (Tran Trung et al., 2020), S. aureus (Qiu et al., 2011; Joung et al., 2016; Liu et al., 2020), Listeria monocytogenes (L. monocytogenes) (Qian et al., 2020), P. aeruginosa, B. cereus, and Salmonella typhimurium (S. typhimurium) (Rashed et al., 2014).
Morin
Morin is a flavone in many fruits and plants of the Moraceae and Myrtaceae families, such as Maclura pomifera (Rafinesque) C. K. Schneider (Moraceae); Maclura tinctoria L. D. Don ex Steudel. (Moraceae); Psidium guajava L. (Myrtaceae); and Morus alba L. (Moraceae) (Mbaveng et al., 2014; Shivashankara et al., 2015; Baliga et al., 2019). This flavonoid is attributed to antioxidant, anti-inflammatory, antidiabetic, antihistamine, antitumor, antihypertensive, antiuricemic, neuroprotective, antiviral, and antimicrobial activities (Al-Numair et al., 2014; Caselli et al., 2016).
The antimicrobial activity of morin was demonstrated against Listeria monocytogenes can be explained by two mechanisms (Sivaranjani et al., 2016). Firstly, it reduces biofilm formation by inhibiting microbial motility and adhesion and compromising cell-surface and cell-cell interactions. The second mechanism is the interruption of listeriolysin O secretion. It reduces the pathogenicity of L. monocytogenes in epithelial cells and macrophages.
Morin acts against S. aureus (Amin et al., 2015), B. cereus, Salmonella enteritidis (S. enteritidis) (Arima and Danno, 2002), E. coli (Kopacz et al., 2016), and H. pylori (Tombola et al., 2003).
Myricetin
Myricetin is a flavone encountered in many fruits, vegetables, teas, berries, and red wine (Omar, 2017). Biological activities attributed to this flavone include hypoglycemic (Eddouks et al., 2014), anti-inflammatory (Shukla et al., 2019), anticarcinogenic, and antiviral (Dormán et al., 2016), and antimicrobial (Puupponen-Pimiä et al., 2001).
This compound reduces the expression of genes that encode some virulence factors responsible for bacterial colonization. Moreover, it inactivates host defenses, tissue damage, and nutrient uptake genes in pathogenic strains of Porphyromonas gingivalis (P. gingivalis) (Grenier et al., 2015). Moreover, myricetin activity was demonstrated against E. coli (Puupponen-Pimiä et al., 2001), S. aureus, and Proteus vulgaris (P. vulgaris) (Mori et al., 1987), and H. pylori (Tran Trung et al., 2020).
Naringenin and Naringin
The flavanone naringenin and its glycoside (naringin) are abundant in the peels of citrus fruits, mainly grapefruit and orange (Jadeja and Devkar, 2013). They present antioxidant, antidiabetic (Srinivasan et al., 2019), anti-inflammatory (Shukla et al., 2019), hypolipemic, antihypertensive, and antifibrotic (Casas-Grajales and Muriel, 2017), and antimicrobial (Céliz et al., 2011) activities.
Naringenin and naringin act against Salmonella enteritidis (Yin et al., 2012). The study reported the synergism of these grapefruit juice components with the acidic pH generated. In combination, these factors reduced the adhesion of S. enteritidis by inhibiting the bacterium’s acid tolerating response mechanism.
Moreover, other studies reported the activity of naringenin and naringin against E. coli, P. aeruginosa (Adamczak et al., 2019), Proteus mirabilis (P. mirabilis), Acinetobacter baumannii (A. baumannii), Klebsiella pneumonia (K. pneumoniae), S. aureus, B. subtilis, E. faecalis (Özçelik et al., 2011), and H. pylori (Tran Trung et al., 2020).
Quercetin
Quercetin is a bioflavonoid obtained from various plant sources (e.g., apple, onion, citrus fruits, and vegetables) (Shankar et al., 2015; Horwitz, 2018). It has several biological activities, such as antioxidant, anti-inflammatory, anticancer (Ay et al., 2016), antiviral (Sathya and Devi, 2017), and antimicrobial (Jaisinghani, 2017).
Feasible antimicrobial mechanisms of action of quercetin have been described (Adeyemi et al., 2020) in S. aureus and E. coli. Quercetin can initiate the peroxidation of the outer lipid membrane in gram-negative bacteria, such as E. coli. It compromises the integrity of the bacterial cell barrier, leading to cell lysis. Additionally, in gram-positive bacteria, quercetin causes oxidative stress and activates the kynurenine pathway. It depletes L-tryptophan reserves, leading to a reduction in bacterial growth.
Studies also report quercetin activity against P. aeruginosa, P. vulgaris (Jaisinghani, 2017), P. mirabilis, A. baumannii, K. pneumoniae, E. faecalis, B. subtilis (Özçelik et al., 2011) and H. pylori (Brown and Jiang, 2013).
Hyperoside and Rutin
Hyperoside (quercetin-3′-O-β-D-galactopyranoside) and rutin (quercetin-3-O-rutinoside) are quercetin glycosides found in vegetables, citrus fruits, and berries. They exhibit several biological activities, such as anti-inflammatory, antithrombotic, antidiabetic, hepatoprotective, antioxidant, antihistamine, antitumor, antiplatelet, antihypertensive, antispasmodic, antiprotozoal, and antimicrobial (Patel and Patel, 2019; Shukla et al., 2019).
Rutin can inhibit biofilm formation in E. coli and S. aureus (Al-Shabib et al., 2017). The probable mechanism of this activity is related to reducing the production of exopolysaccharides. These molecules are responsible for biofilms’ higher resistance to antimicrobials than planktonic cultures and protect the biofilm by forming multiple layers on its surface that aid in adhesion.
Additionally, hyperoside and rutin activities against P. aeruginosa (Sun et al., 2017; Adamczak et al., 2019), Serratia marcescens (S. marcescens) (Vaquero et al., 2007), E. faecalis (Jesus et al., 2018), Actinomyces viscosus (A. viscosus), Actinomyces naeslundii (A. naeslundii) (Gutiérrez-Venegas et al., 2019), S. pyogenes (Van Der Watt and Pretorius, 2001) and H. pylori (Jeong, 2009) are also described.
Use of Extracts Containing Flavonoids Against H. pylori and Its Advantages
Defined as a group I carcinogen since 1994 by the International Agency for Research on Cancer, H. pylori represents about 5% of the total burden of all cancers worldwide (WHO, 2010; Moss, 2017). In addition, H. pylori is associated with diseases such as chronic gastritis, peptic ulcer, and gastric mucosa-associated lymphoid tissue lymphoma. This bacterium has a urease enzyme capable of converting the urea present in gastric acid into ammonia, increasing the stomach pH, thus allowing its colonization. Its silent permanence results in chronic inflammation and, consequently, in the appearance of gastritis and ulcers that can lead to gastric perforation. Thereupon, the epithelial tissue begins to undergo metaplasia. The metaplastic cells start the process of uncontrolled division, which undergoes gene mutation and culminate in the formation of malignant neoplastic tissue (Figure 3) (Ladeira et al., 2003; Minozzo et al., 2016).
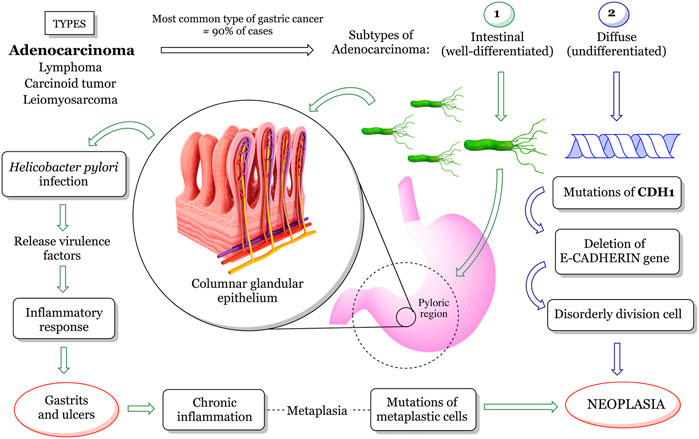
FIGURE 3. General overview of H. pylori-associated gastric cancer development. According to its topology, gastric cancer can be classified into adenocarcinoma, lymphoma, carcinoid tumor, and leiomyosarcoma. Adenocarcinoma is the type of gastric cancer most affecting the population, subdivided into (1) intestinal and (2) diffuse. Type (1) is mainly elicited by an acute immune response induced by H. pylori infection. The silent persistence of the bacteria provides a picture of chronic inflammation, consequently inducing gastritis and ulcers that can lead to gastric perforation. As a result, epithelial tissue undergoes metaplasia (cell differentiation) and behaves like intestinal cells, losing function. Metaplastic cells begin a process of disordered division by undergoing gene mutation that ends up in the formation of malignant neoplastic tissue. Type (2) adenocarcinoma is caused by genetic factors that affect the expression of the intercellular adhesion proteins. For example, E-cadherin is responsible for keeping gastric epithelial cells interconnected and controls the cell cycle.
Among the current treatment strategies for patients with H. pylori-associated gastritis and peptic ulcer disease, triple therapy is based on combinations of multiple agents, including bismuth subsalicylate, proton pump inhibitors, H2 blockers, and antibiotics, mainly clarithromycin. Additionally, the eradication of H. pylori is indicated for treating lymphoma. Also, other regimens used as adjunctive therapy include probiotics, bovine lactoferrin, and curcumin (Asha et al., 2013).
As expected, the application of multiple drugs is associated with several side effects, making it impossible for the patient to adhere to and abandon the course of treatment. In addition, these interferents can lead to the emergence of resistant strains (Ustün et al., 2006).
Therefore, the search for new anti-H. pylori therapy alternatives promoted the exploration in the field of medicinal plants. As a result, several studies have been conducted, and many natural products have anti-H. pylori mechanisms of action proven, such as urease inhibition, DNA damage, protein synthesis inhibition, and anti-inflammatory effects. Moreover, they inhibit some enzymes, such as dihydrofolate reductase and myeloperoxidase N-acetyltransferase (Figure 4) (Baker, 2020).
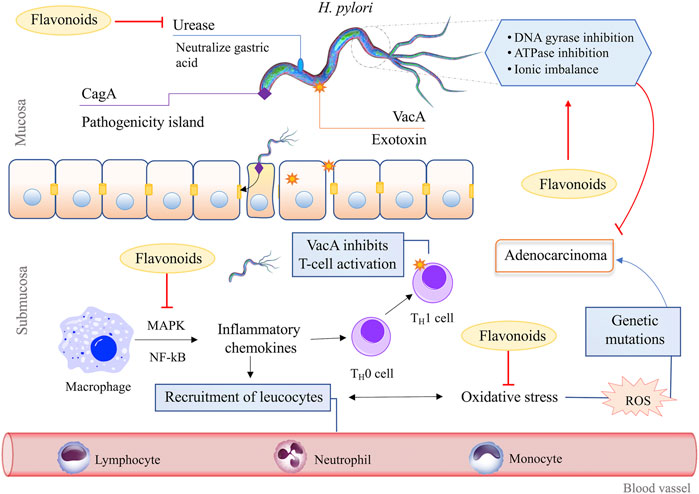
FIGURE 4. Mechanisms of action of flavonoids against H. pylori. The pathogenesis of H. pylori depends on several virulence factors, including urease. This enzyme neutralizes the acidic pH of the gastric medium and facilitates bacteria adhesion to the epithelium. Flavonoids can block this process once they inhibit urease activity. Flavonoids also act by inhibiting enzymes crucial for the reproduction and survival of H. pylori, such as DNA gyrase and ATPase. In addition, it induces ionic and metabolic imbalances at the cytoplasmic level, causing bacterial cell wall disruption, which leads to cell death or latency. Once adhered to the gastric epithelium, H. pylori induces the release of proinflammatory cytokines and the generation of specific genetic mutations which promote cell apoptosis. Flavonoids have anti-inflammatory activities. They inhibit MAPK and NF-kB pathways and regulate the oxidative stress response in phagocytes and other cells. In addition, some flavonoids act as antioxidant agents, scavenging free radicals and reestablishing the ionic balance. The Establishment of adenocarcinoma from successive gene mutations caused by chronic inflammation and the inhibition of any stage of this process can attenuate, delay or prevent the onset of gastric cancer.
Preparations containing physiologically active constituents in folk medicine support the therapeutic use of flavonoids. Several pharmacological actions include antimicrobial, antitumor, anti-inflammatory, and antioxidant (Asha et al., 2013). For example, flavonoids are effective against different microorganisms by different mechanisms of action. They cause membrane disruption, antibiofilm action, cell envelope synthesis, nucleic acid synthesis, electron transport chain and ATP synthesis, formation of flavonoid-metal complexes, inhibition of bacterial toxins, and others (Górniak et al., 2019).
As mentioned above, pathogenic bacteria may resist antibiotic drugs through different mechanisms. For example, mechanisms might be the prevention of interaction of the drug with the target, efflux of the antibiotic from the cell, and direct destruction or modification of the drug compound. Moreover, bacteria can interchange resistance genes with surrounding colonies. For example, the β-lactamase gene encodes an enzyme that hydrolyzes the amide bond in the β-lactam ring through transformation (incorporation of naked DNA), transduction (phage-mediated), and conjugation. Gram-negative bacteria interfere with β-lactam ring hydrolysis, whereas Gram-positive bacteria modify the target site of antibiotics (Bush and Fisher, 2011; Bush, 2013).
Occasionally the resistance to antimicrobial agents can be obtained via combined mechanisms. For instance, although gentamicin resistance does not rely on antibiotic modification, it is executed by altering the membrane potential and efflux and 16S rRNA methylation (Waglechner and Wright, 2017).
Cistus laurifolius L. (Cistaceae) extract has proved anti-H. pylori, including against resistant strains (Ustün et al., 2006). Moreover, the extract of Glycyrrhiza glabra L. (Fabaceae) maintained its action against H. pylori even after being clinically used without any drug resistance (Fukai et al., 2002). Such findings infer how flavonoids may be helpful as lead compounds in the development of a new class of anti-H. pylori regimens.
Possible Mechanisms of Action of Flavonoids Against H. pylori
The urease enzyme is considered a potent H. pylori virulence factor. It comprises 6% of the synthesized proteins, representing a significant energy investment in colonization. Moreover, its inhibition hinders the survival of H. pylori in the gastric environment, which becomes hostile due to high acidity (Ladeira et al., 2003). In vitro experiments demonstrated that the flavonoid quercetin, present in Heterotheca inuloides Cassini (Asteraceae) extract, promotes high enzyme inhibition (IC50 = 132.4 μg/ml) (Egas et al., 2018). Additionally, a complimentary in silico study of molecular docking has identified that quercetin interacts with the catalytic site, forming ionic bonds with the zinc cation. Ionic bonds are among the strongest, supporting and inducing high enzyme inhibition activity. Catechin and epicatechin isolated from the Peumus boldus extract inhibit urease activity, with IC50 values of 66 and 112 µg GAE/ml, respectively (Pastene et al., 2014). However, due to molecules’ sizes and high polarity, they seem to neutralize only the most external pool of urease. It means they could not inhibit the urease cytoplasmic pool, suggesting a more preventive application.
Glabridin and glabrol present in the extract of Glycyrrhiza glabra L. (GutGard®) inhibit the dihydrofolate reductase (DHFR) (Asha et al., 2013). DHFR consists of a ubiquitous enzyme in every eukaryotic and prokaryotic cell that plays a crucial role in the synthesis of thymidine. It catalyzes the reduction of 7,8-dihydrofolate to 5,6,7,8-tetrahydrofolate, using NADPH as a cofactor. This reaction is an essential step in the biosynthesis of DNA nucleotide bases and, therefore, plays an essential role in bacteria survival, such as H. pylori in the human body. It is interesting to note that in addition to the anti-H. pylori activity, GutGard® also has anti-inflammatory (Chandrasekaran et al., 2011) and antioxidant (Mukherjee et al., 2010).
The potential activity of the luteolin-rich extract from Plectranthus barbatus (Andrews) Benth. ex G.Don (Lamiaceae) has been proved (Borges et al., 2020). The subinhibitory dose of ethyl acetate (EAF) fraction (128 μg/ml) produced similar morphological changes as did the subinhibitory dose of amoxicillin (0.25 μg/ml). The filamentous cells found and the production of protrusions indicate a possible action on the bacterial cell wall. These data suggest a possible action on PBPs (Penicillin-Binding Proteins), which are involved in the cell septation process, especially the 63 kDa PBP (PBP63). Besides, observations in producing protrusions and blebs suggest a possible action on other PBPs involved in the peptidoglycan wall.
When assessing the intracellular accumulation of quercetin from Vitis rotundifolia Michaux (Vitaceae), it was observed a behavior possibly interspersed in the hydrophobic region of the lipid bilayers of the cell envelope, passively diffused through the cell membrane into the cytosol (Brown and Jiang, 2013). It may be an interaction with cell membrane proteins or active import into the cytosol, leading to metabolic imbalance, followed by cell death.
Catechin, epicatechin, epigallocatechin, and quercetin flavonoids in white, green, oolong, and black teas deplete membrane electrons responsible for the transport chain (Ankolekar et al., 2011). They disrupt oxidative phosphorylation and inhibit the proton efflux linked to dehydrogenase. Thereby, they interfere with the flow of electrons at the cytochrome level. However, low hydrophobic and simple soluble phenolic compounds may not be effective. The outer lipopolysaccharide layer of H. pylori avoids oxidative phosphorylation. As a result, the membrane creates a hydrophobic microenvironment along the bacterial surface. Also, soluble phenols interrupt the H+-adenosine triphosphatase required to synthesize adenosine triphosphate. It causes hyperacidification via proton donation in the plasma membrane or in the intracellular cytosolic pathway. Another explanation is that the hydrophobic portion of polyphenols adheres to the cell wall. It would cause destabilization and rupture of the membrane and inhibition of transmembrane transport. These mechanisms can act synergistically: the disturbing and destabilizing effect of polyphenols can make it easier for simple soluble phenols to exercise their hyperacidification.
Alpinia officinarum Hance (Zingiberaceae) extracts possess the flavonoids apigenin, galangin, galangin-3-methyl ether, kaempferol, kaempferide, pinobaksin, pinocembrin, quercetin, quercetin-3-methyl ether, and salvagenin (Ma X. et al., 2020). To such metabolites has been attributed the anti-H. pylori activity by inhibiting the synthesis of the proinflammatory cytokine interleukin-8 (IL-8) via the MAPK pathway, whose gene shows a significant increase in expression in the entire genome of gastric epithelial cells after infection by H. pylori. This reduction would result in decreased inflammation and adhesion of the bacteria to the epithelium.
Moreover, the Lippia integrifolia (Griseb.) Hieronymus (Verbenaceae) extract demonstrated the flavonoids salvagenin, 6-Hydroxyluteolin 7-hexoside, 6-Methoxyluteolin-hexoside, 6-Methylscutellarein 7-hexoside, B-ring-dimethoxylated Flavone-hexoside and Methoxylated apigenin-hexoside (Marcial et al., 2014). As a result, it exhibited strong antioxidant capacity in vitro. Furthermore, it inhibited H. pylori adhesion to stomach cells by up to 40%. In comparison, the ethanol-soluble fraction showed up to 60% inhibition rates. Furthermore, the decoction significantly increased the gastric adenocarcinoma cell line (AGS) cell viability at> 10 μg/ml without influencing the proliferation rate. Besides, H. pylori-induced IL-8 secretion was significantly reduced by the coincubation of AGS cells with extracts.
Study Relevance
Here, the activity of flavonoid extracts was investigated against H. pylori for gastric cancer prevention and treatment. These studies provided a basis for further investigations that may lead to new clinical trials for proper drug administration. In this perspective, the use of botanical drugs might present as a viable and safe alternative.
Administration of botanic products is a complementary treatment for acute and chronic diseases and preventive care. GutGard® (Asha et al., 2013) is an example currently used to help control indigestion and heartburn and manage H. pylori infection at a dosage of 150 mg/day (Ribeiro, 2019). So, using extracts containing flavonoid compounds might be highly relevant to eradicating H. pylori as a curative and preventive therapeutic strategy.
Furthermore, botanical drugs are cost-effective compared to synthetic medicines and processed plant derivates (Maqbool et al., 2019). The focus on the herbal products research encourages the investigation of alternative therapeutic modalities for long-standing and persistent health problems.
Limitations
Studies using pure isolated flavonoids were rare, which required the inclusion of additional extracts in our protocol. Few publications mentioned the mechanism of action of flavonoids on H. pylori. Additionally, many articles identified their flavonoids but not their concentration. Furthermore, some studies did not use full botanical taxonomic names, limiting comparative analysis and data translation. Finally, only one in vivo study was included in this review, limiting the analytical power of the effectiveness of the flavonoid in the live organism.
Future Directions
This review on flavonoids’ antimicrobial and anticancer action is in line with recent literature (Al-Ishaq et al., 2021; González et al., 2021; Li et al., 2022). Altogether, data demonstrate the great potential of these compounds in combating H. pylori infection and gastric cancer protection. The immense variety of plants that serve as a source of flavonoids can ensure the sustainable production of drugs containing the compounds. In addition, the great potential of these compounds for various health applications is observed. New flavonoids’ extraction methods might allow for better profitability and sustainability. Moreover, dosage control and compound stability offered by smart drug delivery systems can further expand the understanding of the activities of flavonoid-rich extracts against various pathogens (de Lima Nascimento et al., 2019; Gondim et al., 2019).
Furthermore, a broader understanding of the interactions of flavonoids with drugs of choice for the treatment of H. pylori may help determine the use of the compounds as adjuvant therapy in the fight against the development of gastric cancer. Also, it is imperative to evaluate the effect of flavonoids on the human microbiome and how the metabolic process of the colonizing microbes might interfere with the activities of the compounds against pathogenic strains. Clinical trials testing the action of these compounds in patients with H. pylori infection deserve attention.
Conclusion
There is no single therapy for H. pylori eradication. Instead, an association of an antiulcerogenic drug and two antibiotics with 70–85% success rates. Our data demonstrated the relevance of new studies involving natural products, specifically extracts of plants rich in flavonoids. These compounds have shown promising results in anti-H. pylori targeting different mechanisms of action.
Author Contributions
All authors contributed to the development of the article. RI, DF, GG, and WR held the bibliographic search and selection of articles. MP and DF performed the methodology of the article. RI, DF, SA, and GG discussed the articles. RI and GG were in charge of generating the tables and figures. BG, SC, SW, and LC were responsible for the general review of the content.
Funding
Funds supported this study from the Brazilian Federal Foundation for Support and Evaluation of Graduate Education (CAPES) - Finance Code 001 (fellowships to SC and LC).
Conflict of Interest
The authors declare that the research was conducted in the absence of any commercial or financial relationships that could be construed as a potential conflict of interest.
Publisher’s Note
All claims expressed in this article are solely those of the authors and do not necessarily represent those of their affiliated organizations, or those of the publisher, the editors and the reviewers. Any product that may be evaluated in this article, or claim that may be made by its manufacturer, is not guaranteed or endorsed by the publisher.
Acknowledgments
Thanks to CAPES, UFPB, and UEPB for the most valuable support given to this research.
References
Abreu Miranda, M., Lemos, M., Alves Cowart, K., Rodenburg, D., D McChesney, J., Radwan, M. M., et al. (2015). Gastroprotective Activity of the Hydroethanolic Extract and Isolated Compounds from the Leaves of Solanum Cernuum Vell. J. Ethnopharmacol. 172, 421–429. doi:10.1016/j.jep.2015.06.047
Abreu Miranda, M., Lemos, M., Alves Cowart, K., Rodenburg, D., D. McChesney, J., Radwan, M. M., et al. (2015). Gastroprotective Activity of the Hydroethanolic Extract and Isolated Compounds from the Leaves of Solanum Cernuum Vell. J. Ethnopharmacol. 172, 421–429. doi:10.1016/j.jep.2015.06.047
Adamczak, A., Ożarowski, M., and Karpiński, T. M. (2019). Antibacterial Activity of Some Flavonoids and Organic Acids Widely Distributed in Plants. J. Clin. Med. 9, 109. doi:10.3390/jcm9010109
Adeyemi, O. S., Ebugosi, C., Akpor, O. B., Hetta, H. F., Al-Rashed, S., Otohinoyi, D. A., et al. (2020). Quercetin Caused Redox Homeostasis Imbalance and Activated the Kynurenine Pathway (Running Title: Quercetin Caused Oxidative Stress). Biol. (Basel) 9, 1–9. doi:10.3390/biology9080219
Adzu, B., Balogun, S. O., Pavan, E., Ascêncio, S. D., Soares, I. M., Aguiar, R. W., et al. (2015). Evaluation of the Safety, Gastroprotective Activity and Mechanism of Action of Standardised Leaves Infusion Extract of Copaifera Malmei Harms. J. Ethnopharmacol. 175, 378–389. doi:10.1016/j.jep.2015.09.027
Ahmad, A., Kaleem, M., Ahmed, Z., and Shafiq, H. (2015). Therapeutic Potential of Flavonoids and Their Mechanism of Action against Microbial and Viral Infections-A Review. Food Res. Int. 77, 221–235. doi:10.1016/j.foodres.2015.06.021
Al-Ishaq, R. K., Liskova, A., Kubatka, P., and Büsselberg, D. (2021). Enzymatic Metabolism of Flavonoids by Gut Microbiota and its Impact on Gastrointestinal Cancer. Cancers (Basel) 13. doi:10.3390/cancers13163934
Al-Numair, K. S., Chandramohan, G., Alsaif, M. A., Veeramani, C., and El Newehy, A. S. (2014). Morin, a Flavonoid, on Lipid Peroxidation and Antioxidant Status in Experimental Myocardial Ischemic Rats. Afr. J. Tradit. Complement. Altern. Med. 11, 14–20. doi:10.4314/ajtcam.v11i3.3
Al-Shabib, N. A., Husain, F. M., Ahmad, I., Khan, M. S., Khan, R. A., and Khan, J. M. (2017). Rutin Inhibits Mono and Multi-Species Biofilm Formation by Foodborne Drug Resistant Escherichia coli and Staphylococcus aureus. Food control. 79, 325–332. doi:10.1016/j.foodcont.2017.03.004
Albino, S. L., da Silva, J. M., de C NobreNobre, M. S. M. S., de M E Silvae Silva, Y. M. S. Y. M. S., Santos, M. B., de Araújo, R. S. A., et al. (2020). Bioprospecting of Nitrogenous Heterocyclic Scaffolds with Potential Action for Neglected Parasitosis: A Review. Curr. Pharm. Des. 26, 4112–4150. doi:10.2174/1381612826666200701160904
Almeida, G. V. B., Arunachalam, K., Balogun, S. O., Pavan, E., Ascêncio, S. D., Soares, I. M., et al. (2019). Chemical Characterization and Evaluation of Gastric Antiulcer Properties of the Hydroethanolic Extract of the Stem Bark of Virola Elongata (Benth.) Warb. J. Ethnopharmacol. 231, 113–124. doi:10.1016/j.jep.2018.11.011
Almeida, G. V. B., Arunachalam, K., Balogun, S. O., Pavan, E., Ascêncio, S. D., Soares, I. M., et al. (2019). Chemical Characterization and Evaluation of Gastric Antiulcer Properties of the Hydroethanolic Extract of the Stem Bark of Virola Elongata (Benth.) Warb. J. Ethnopharmacol. 231, 113–124. doi:10.1016/j.jep.2018.11.011
Amin, M. U., Khurram, M., Khattak, B., and Khan, J. (2015). Antibiotic Additive and Synergistic Action of Rutin, Morin and Quercetin against Methicillin Resistant Staphylococcus aureus. BMC Complement. Altern. Med. 15, 59–12. doi:10.1186/s12906-015-0580-0
Ankolekar, C., Johnson, D., Pinto, Mda S., Johnson, K., Labbe, R., and Shetty, K. (2011). Inhibitory Potential of Tea Polyphenolics and Influence of Extraction Time against helicobacter Pylori and Lack of Inhibition of Beneficial Lactic Acid Bacteria. J. Med. Food 14, 1321–1329. doi:10.1089/jmf.2010.0237
Arima, H., and Danno, G. (2002). Isolation of Antimicrobial Compounds from Guava (Psidium Guajava l.) and Their Structural Elucidation. Biosci. Biotechnol. Biochem. 66, 1727–1730. doi:10.1271/bbb.66.1727
Arunachalam, K., Damazo, A. S., Pavan, E., Oliveira, D. M., Figueiredo, F. F., Machado, M. T. M., et al. (2019). Cochlospermum Regium (Mart. Ex Schrank) Pilg.: Evaluation of Chemical Profile, Gastroprotective Activity and Mechanism of Action of Hydroethanolic Extract of its Xylopodium in Acute and Chronic Experimental Models. J. Ethnopharmacol. 233, 101–114. doi:10.1016/j.jep.2019.01.002
Asha, M. K., Debraj, D., Prashanth, D., Edwin, J. R., Srikanth, H. S., Muruganantham, N., et al. (2013). In Vitro anti-Helicobacter pylori Activity of a Flavonoid Rich Extract of Glycyrrhiza Glabra and its Probable Mechanisms of Action. J. Ethnopharmacol. 145, 581–586. doi:10.1016/j.jep.2012.11.033
Ay, M., Charli, A., Jin, H., Kanthasamy, A., and Kanthasamy, A. G. (2016). Muhammet Ay, Adhithiya Charli, Huajun Jin, Vellareddy Anantharam, Arthi Kanthasamy and Anumantha G. Kanthasamy. 447–452.
Babiaka, S. B., Nia, R., Abuga, K. O., Mbah, J. A., Nziko, V. d. P. N., Paper, D. H., et al. (2020). Antioxidant Potential of Flavonoid Glycosides from Manniophyton Fulvum Müll. (Euphorbiaceae): Identification and Molecular Modeling. Sci. Afr. 8, e00423. doi:10.1016/j.sciaf.2020.e00423
Bae, E. A., Han, M. J., and Kim, D. H. (1999). In Vitro Anti-Helicobacter pylori Activity of Some Flavonoids and Their Metabolites. Planta Med. 65, 442–443. doi:10.1055/s-2006-960805
Bailly, C. (2020). Molecular and Cellular Basis of the Anticancer Activity of the Prenylated Flavonoid Icaritin in Hepatocellular Carcinoma. Chem. Biol. Interact. 325, 109124. doi:10.1016/j.cbi.2020.109124
Baker, D. A. (2020). Plants against Helicobacter pylori to Combat Resistance: An Ethnopharmacological Review. Biotechnol. Rep. (Amst) 26, e00470. doi:10.1016/j.btre.2020.e00470
Baliga, M. S., Shivashankara, A. R., Venkatesh, S., Bhat, H. P., Palatty, P. L., Bhandari, G., et al. (2019). Phytochemicals in the Prevention of Ethanol-Induced Hepatotoxicity. Diet. Interv. Liver Dis. Foods, Nutr. Diet. Suppl., 79–89. doi:10.1016/B978-0-12-814466-4.00007-0
Batra, P., and Sharma, A. K. (2013). Anti-cancer Potential of Flavonoids: Recent Trends and Future Perspectives. 3 Biotech. 3, 439–459. doi:10.1007/s13205-013-0117-5
Beck, S., and Stengel, J. (2016). Mass Spectrometric Imaging of Flavonoid Glycosides and Biflavonoids in Ginkgo Biloba L. Phytochemistry 130, 201–206. doi:10.1016/j.phytochem.2016.05.005
Bernal-Mercado, A. T., Vazquez-Armenta, F. J., Tapia-Rodriguez, M. R., Islas-Osuna, M. A., Mata-Haro, V., Gonzalez-Aguilar, G. A., et al. (2018). Comparison of Single and Combined Use of Catechin, Protocatechuic, and Vanillic Acids as Antioxidant and Antibacterial Agents against Uropathogenic Escherichia coli at Planktonic and Biofilm Levels. Molecules 23. doi:10.3390/molecules23112813
Biharee, A., Sharma, A., Kumar, A., and Jaitak, V. (2020). Antimicrobial Flavonoids as a Potential Substitute for Overcoming Antimicrobial Resistance. Fitoterapia 146, 104720. doi:10.1016/j.fitote.2020.104720
Bisignano, C., Filocamo, A., La Camera, E., Zummo, S., Fera, M. T., and Mandalari, G. (2013). Antibacterial Activities of Almond Skins on cagA-Positive And-Negative Clinical Isolates of Helicobacter pylori. BMC Microbiol. 13, 103. doi:10.1186/1471-2180-13-103
Borges, A. S., Minozzo, B. R., Santos, H., Ardisson, J. S., Rodrigues, R. P., Romão, W., et al. (2020). Plectranthus Barbatus Andrews as Anti-Helicobacter pylori Agent with Activity against Adenocarcinoma Gastric Cells. Industrial Crops Prod. 146, 112207. doi:10.1016/j.indcrop.2020.112207
Botten, D., Fugallo, G., Fraternali, F., and Molteni, C. (2015). Structural Properties of Green Tea Catechins. J. Phys. Chem. B 119, 12860–12867. doi:10.1021/acs.jpcb.5b08737
Braicu, C., Ladomery, M. R., Chedea, V. S., Irimie, A., and Berindan-Neagoe, I. (2013). The Relationship between the Structure and Biological Actions of Green Tea Catechins. Food Chem. 141, 3282–3289. doi:10.1016/j.foodchem.2013.05.122
Brown, J. C., and Jiang, X. (2013). Activities of Muscadine Grape Skin and Polyphenolic Constituents against Helicobacter pylori. J. Appl. Microbiol. 114, 982–991. doi:10.1111/jam.12129
Bush, K., and Fisher, J. F. (2011). Epidemiological Expansion, Structural Studies, and Clinical Challenges of New β-lactamases from Gram-Negative Bacteria. Annu. Rev. Microbiol. 65, 455–478. doi:10.1146/annurev-micro-090110-102911
Bush, K. (2013). The ABCD's of β-lactamase Nomenclature. J. Infect. Chemother. 19, 549–559. doi:10.1007/s10156-013-0640-7
Calixto, J. B. (2019). The Role of Natural Products in Modern Drug Discovery. An. Acad. Bras. Cienc. 91 Suppl 3, e20190105–7. doi:10.1590/0001-3765201920190105
Cardoso, O., Donato, M. M., Luxo, C., Almeida, N., Liberal, J., Figueirinha, A., et al. (2018). Anti- Helicobacter pylori potential of Agrimonia eupatoria L. and Fragaria vesca. J. Funct. Foods 44, 299–303. doi:10.1016/j.jff.2018.03.027
Casas-Grajales, S., and Muriel, P. (2017). The Liver, Oxidative Stress, and Antioxidants. Liver Pathophysiol. Ther. Antioxidants, 583–604. doi:10.1016/B978-0-12-804274-8.00043-6
Caselli, A., Cirri, P., Santi, A., and Paoli, P. (2016). Morin: A Promising Natural Drug. Curr. Med. Chem. 23, 774–791. doi:10.2174/0929867323666160106150821
Céliz, G., Daz, M., and Audisio, M. C. (2011). Antibacterial activity of naringin derivatives against pathogenic strains. J. Appl. Microbiol. 111, 731–738. doi:10.1111/j.1365-2672.2011.05070.x
Cesa, S., Sisto, F., Zengin, G., Scaccabarozzi, D., Kokolakis, A. K., Scaltrito, M. M., et al. (2019). Phytochemical analyses and pharmacological screening of Neem oil. South Afr. J. Bot. 120, 331–337. doi:10.1016/j.sajb.2018.10.019
Chandrasekaran, C. V., Deepak, H. B., Thiyagarajan, P., Kathiresan, S., Sangli, G. K., Deepak, M., et al. (2011). Dual inhibitory effect of Glycyrrhiza glabra (GutGard™) on COX and LOX products. Phytomedicine 18, 278–284. doi:10.1016/j.phymed.2010.08.001
Cho, M. H., and Lee, S. W. (2015). Phenolic phytoalexins in rice: Biological functions and Biosynthesis. Int. J. Mol. Sci. 16, 29120–29133. doi:10.3390/ijms161226152
Cui, Y., Chen, J., He, Z., and Xiao, Y. (2013). SUZ12 depletion suppresses the proliferation of gastric cancer cells. Cell. Physiol. biochem. 31, 778–784. doi:10.1159/000350095
Cushnie, T. P., and Lamb, A. J. (2005). Antimicrobial activity of flavonoids. Int. J. Antimicrob. Agents 26, 343–356. doi:10.1016/j.ijantimicag.2005.09.002
Cushnie, T. P., and Lamb, A. J. (2011). Recent advances in understanding the antibacterial properties of flavonoids. Int. J. Antimicrob. Agents 38, 99–107. doi:10.1016/j.ijantimicag.2011.02.014
da Silva Junior, I. F., Balogun, S. O., de Oliveira, R. G., Damazo, A. S., and Martins, D. T. O. (2016). Piper umbellatum L.: A medicinal plant with gastric-ulcer protective and ulcer healing effects in experimental rodent models. J. Ethnopharmacol. 192, 123–131. doi:10.1016/j.jep.2016.07.011
da Silva, L. M., Boeing, T., Somensi, L. B., Cury, B. J., Steimbach, V. M., Silveria, A. C., et al. (2015). Evidence of gastric ulcer healing activity of Maytenus robusta Reissek: In Vitro and In Vivo studies. J. Ethnopharmacol. 175, 75–85. doi:10.1016/j.jep.2015.09.006
Das, S., Das, M. K., Das, R., Gehlot, V., Mahant, S., Mazumder, P. M., et al. (2020). Isolation, characterization of Berberine from Berberis aristata DC for eradication of resistant Helicobacter pylori. Biocatal. Agric. Biotechnol. 26, 101622. doi:10.1016/j.bcab.2020.101622
de Cássia dos Santos, R., Bonamin, F., Périco, L. L., Rodrigues, V. P., Zanatta, A. C., Rodrigues, C. M., et al. (2019). Byrsonima intermedia A. Juss partitions promote gastroprotection against peptic ulcers and improve healing through antioxidant and anti-inflammatory activities. Biomed. Pharmacother. 111, 1112–1123. doi:10.1016/j.biopha.2018.12.132
de Lima Nascimento, T. R., de Amoêdo Campos Velo, M. M., Silva, C. F., Costa Cruz, S. B. S., Gondim, B. L. C., Mondelli, R. F. L., et al. (2019). Current Applications of Biopolymer-based Scaffolds and Nanofibers as Drug Delivery Systems. Curr. Pharm. Des. 25, 3997–4012. doi:10.2174/1381612825666191108162948
de Martel, C., Forman, D., and Plummer, M. (2013). Gastric cancer: epidemiology and risk factors. Gastroenterol. Clin. North Am. 42, 219–240. doi:10.1016/j.gtc.2013.01.003
del Valle, P., García-Armesto, M. R., de Arriaga, D., González-Donquiles, C., Rodríguez-Fernández, P., and Rúa, J. (2016). Antimicrobial activity of kaempferol and resveratrol in binary combinations with parabens or propyl gallate against Enterococcus faecalis. Food control. 61, 213–220. doi:10.1016/j.foodcont.2015.10.001
Díaz-Gómez, R., López-Solís, R., Obreque-Slier, E., and Toledo-Araya, H. (2013). Comparative antibacterial effect of gallic acid and catechin against Helicobacter pylori. LWT - Food Sci. Technol. 54, 331–335. doi:10.1016/j.lwt.2013.07.012
Ding, S. Z., Goldberg, J. B., and Hatakeyama, M. (2010). Helicobacter pylori infection, oncogenic pathways and epigenetic mechanisms in gastric carcinogenesis. Future Oncol. 6, 851–862. doi:10.2217/fon.10.37
Dong, H., Yang, X., He, J., Cai, S., Xiao, K., and Zhu, L. (2017). Enhanced antioxidant activity, antibacterial activity and hypoglycemic effect of luteolin by complexation with Manganese(II) and its inhibition kinetics on xanthine oxidase. RSC Adv. 7, 53385–53395. doi:10.1039/c7ra11036g
Dormán, G., Flachner, B., Hajdú, I., and András, C. D. (2016). Target identification and polypharmacology of nutraceuticals. Nutraceuticals Effic. Saf. Toxic., 263–286. doi:10.1016/B978-0-12-802147-7.00021-8
Eddouks, M., Bidi, A., Bouhali, B. E. L., and Zeggwagh, N. A. (2014). Insulin resistance as a target of some plant-derived phytocompounds. Stud. Nat. Prod. Chem. 43, 351–373. doi:10.1016/B978-0-444-63430-6.00011-4
Egas, V., Salazar-Cervantes, G., Romero, I., Méndez-Cuesta, C. A., Rodríguez-Chávez, J. L., and Delgado, G. (2018). Anti-Helicobacter pylori metabolites from Heterotheca inuloides (Mexican arnica). Fitoterapia 127, 314–321. doi:10.1016/j.fitote.2018.03.001
Escandón, R. A., del Campo, M., López-Solis, R., Obreque-Slier, E., and Toledo, H. (2016). Antibacterial effect of kaempferol and (−)-epicatechin on Helicobacter pylori. Eur. Food Res. Technol. 242, 1495–1502. doi:10.1007/s00217-016-2650-z
Espinosa-Rivero, J., Rendón-Huerta, E., and Romero, I. (2015). Inhibition of Helicobacter pylori growth and its colonization factors by Parthenium hysterophorus extracts. J. Ethnopharmacol. 174, 253–260. doi:10.1016/j.jep.2015.08.021
Fahmy, N. M., Al-Sayed, E., Michel, H. E., El-Shazly, M., and Singab, A. N. B. (2020). Gastroprotective effects of Erythrina speciosa (Fabaceae) leaves cultivated in Egypt against ethanol-induced gastric ulcer in rats. J. Ethnopharmacol. 248, 112297. doi:10.1016/j.jep.2019.112297
Fathima, A., and Rao, J. R. (2016). Selective toxicity of Catechin-a natural flavonoid towards bacteria. Appl. Microbiol. Biotechnol. 100, 6395–6402. doi:10.1007/s00253-016-7492-x
Ferreira, T. S., Moreira, C. Z., Cária, N. Z., Victoriano, G., Silva Jr, W. F., and Magalhães, J. C. (2014). Phytotherapy: an introduction to its history, use and application. Rev. Bras. Plantas Med. 16, 290–298. doi:10.1590/S1516-05722014000200019
Fukai, T., Marumo, A., Kaitou, K., Kanda, T., Terada, S., and Nomura, T. (2002). Anti-Helicobacter pylori flavonoids from licorice extract. Life Sci. 71, 1449–1463. doi:10.1016/S0024-3205(02)01864-7
Garro, M. F., Salinas Ibáñez, A. G., Vega, A. E., Arismendi Sosa, A. C., Pelzer, L., Saad, J. R., et al. (2015). Gastroprotective effects and antimicrobial activity of Lithraea molleoides and isolated compounds against Helicobacter pylori. J. Ethnopharmacol. 176, 469–474. doi:10.1016/j.jep.2015.11.009
Ge, L., Li, J., Wan, H., Zhang, K., Wu, W., Zou, X., et al. (2018). NMR data for novel flavonoids from Lonicera japonica flower buds. Data Brief. 21, 2192–2207. doi:10.1016/j.indcrop.2018.08.07310.1016/j.dib.2018.11.021
Gomez-Chang, E., Uribe-Estanislao, G. V., Martinez-Martinez, M., Gálvez-Mariscal, A., and Romero, I. (2018). Anti-helicobacter pylori potential of three edible plants known as quelites in Mexico. J. Med. Food 21, 1150–1157. doi:10.1089/jmf.2017.0137
Gondim, B. L. C., Oshiro-Júnior, J. A., Fernanandes, F. H. A., Nóbrega, F. P., Castellano, L. R. C., and Medeiros, A. C. D. (2019). Plant Extracts Loaded in Nanostructured Drug Delivery Systems for Treating Parasitic and Antimicrobial Diseases. Curr. Pharm. Des. 25, 1604–1615. doi:10.2174/1381612825666190628153755
González, A., Casado, J., and Lanas, Á. (2021). Fighting the Antibiotic Crisis: Flavonoids as Promising Antibacterial Drugs Against Helicobacter pylori Infection. Front. Cell. Infect. Microbiol. 11, 709749. doi:10.3389/fcimb.2021.709749
González, A., Salillas, S., Velázquez-Campoy, A., Espinosa Angarica, V., Fillat, M. F., Sancho, J., et al. (2019). Identifying potential novel drugs against Helicobacter pylori by targeting the essential response regulator HsrA. Sci. Rep. 9, 11294. doi:10.1038/s41598-019-47746-9
Górniak, I., Bartoszewski, R., and Króliczewski, J. (2019). Comprehensive Review of Antimicrobial Activities of Plant Flavonoids, 18, 241–272. doi:10.1007/s11101-018-9591-zComprehensive review of antimicrobial activities of plant flavonoidsPhytochem. Rev.
Grenier, D., Chen, H., Ben Lagha, A., and Fournier-Larente, M. P. (2015). Dual action of myricetin on Porphyromonas gingivalis and the inflammatory response of host cells: A promising therapeutic molecule for periodontal diseases. PLoS One 10, e0131758–15. doi:10.1371/journal.pone.0131758
Grzesik, M., Naparło, K., Bartosz, G., and Sadowska-Bartosz, I. (2018). Antioxidant properties of catechins: Comparison with other antioxidants. Food Chem. 241, 480–492. doi:10.1016/j.foodchem.2017.08.117
Gutiérrez-Venegas, G., Gómez-Mora, J. A., Meraz-Rodríguez, M. A., Flores-Sánchez, M. A., and Ortiz-Miranda, L. F. (2019). Effect of flavonoids on antimicrobial activity of microorganisms present in dental plaque. Heliyon 5, e03013. doi:10.1016/j.heliyon.2019.e03013
Hamad, G. M., Taha, T. H., El-Deeb, N. M., and Alshehri, A. M. (2015). Advanced trends in controlling Helicobacter pylori infections using functional and therapeutically supplements in baby milk. J. Food Sci. Technol. 52, 8156–8163. doi:10.1007/s13197-015-1875-3
Herrero, R., Park, J. Y., and Forman, D. (2014). The fight against gastric cancer - The IARC Working Group report. Best. Pract. Res. Clin. Gastroenterol. 28, 1107–1114. doi:10.1016/j.bpg.2014.10.003
Hinojosa, W. I., Quiróz, M. A., Álvarez, I. R., Castañeda, P. E., Villarreal, M. L., and Taketa, A. C. (2014). Anti-Helicobacter pylori, gastroprotective, anti-inflammatory, and cytotoxic activities of methanolic extracts of five different populations of Hippocratea celastroides collected in Mexico. J. Ethnopharmacol. 155, 1156–1163. doi:10.1016/j.jep.2014.06.044
Hooi, J. K. Y., Lai, W. Y., Ng, W. K., Suen, M. M. Y., Underwood, F. E., Tanyingoh, D., et al. (2017). Global Prevalence of Helicobacter pylori Infection: Systematic Review and Meta-Analysis. Gastroenterology 153, 420–429. doi:10.1053/j.gastro.2017.04.022
Horwitz, R. J. (2018). “Chapter 30 - The Allergic Patient,” in Integrative Medicine (Fourth Edition). Editor D. Rakel (Amsterdam, Netherlands: Elsevier), 300–300.e2.
Ibrahim, N. H., Awaad, A. S., Alnafisah, R. A., Alqasoumi, S. I., El-Meligy, R. M., and Mahmoud, A. Z. (2018). In - Vitro activity of Desmostachya bipinnata (L.) Stapf successive extracts against Helicobacter pylori clinical isolates. Saudi Pharm. J. 26, 535–540. doi:10.1016/j.jsps.2018.02.002
Imran, M., Rauf, A., Abu-Izneid, T., Nadeem, M., Shariati, M. A., Khan, I. A., et al. (2019). Luteolin, a flavonoid, as an anticancer agent: A review. Biomed. Pharmacother. 112, 108612. doi:10.1016/j.biopha.2019.108612
Jadeja, R. N., and Devkar, R. V. (2014). Polyphenols and Flavonoids in Controlling Non-Alcoholic Steatohepatitis. Polyphenols Hum. Heal. Dis. 1, 615–623. doi:10.1016/B978-0-12-398456-2.00047-5
Jaisinghani, R. N. (2017). Antibacterial properties of quercetin. Microbiol. Res. (Pavia) 8. doi:10.4081/mr.2017.6877
Jeong, C.-S. (2009). Evaluation for protective effect of rutin, a natural flavonoid, against Hcl/ethanol-induced gastric lesions. Biomol. Ther. 17, 199–204. doi:10.4062/biomolther.2009.17.2.199
Jesus, R. S., Piana, M., Freitas, R. B., Brum, T. F., Alves, C. F. S., Belke, B. V., et al. (2018). In Vitro antimicrobial and antimycobacterial activity and HPLC-DAD screening of phenolics from Chenopodium ambrosioides L. Braz J. Microbiol. 49, 296–302. doi:10.1016/j.bjm.2017.02.012
Ji, H. F., Li, X. J., and Zhang, H. Y. (2009). Natural products and drug discovery. Can thousands of years of ancient medical knowledge lead us to new and powerful drug combinations in the fight against cancer and dementia? EMBO Rep. 10, 194–200. doi:10.1038/embor.2009.12
Joseph Sahayarayan, J., Udayakumar, R., Arun, M., Ganapathi, A., Alwahibi, M. S., Aldosari, N. S., et al. (2020). Effect of different Agrobacterium rhizogenes strains for in-vitro hairy root induction, total phenolic, flavonoids contents, antibacterial and antioxidant activity of (Cucumis anguria L.). Saudi J. Biol. Sci. 27, 2972–2979. doi:10.1016/j.sjbs.2020.08.050
Joung, D. K., Lee, Y. S., Han, S. H., Lee, S. W., Cha, S. W., Mun, S. H., et al. (2016). Potentiating activity of luteolin on membrane permeabilizing agent and ATPase inhibitor against methicillin-resistant Staphylococcus aureus. Asian pac. J. Trop. Med. 9, 19–22. doi:10.1016/j.apjtm.2015.12.004
Jucá, M. M., Cysne Filho, F. M. S., de Almeida, J. C., Mesquita, D. D. S., Barriga, J. R. M., Dias, K. C. F., et al. (2020). Flavonoids: biological activities and therapeutic potential. Nat. Prod. Res. 34, 692–705. doi:10.1080/14786419.2018.1493588
Kim, H. W., Woo, H. J., Yang, J. Y., Kim, J.-B., and Kim, S.-H. (2021). Hesperetin Inhibits Expression of Virulence Factors and Growth of Helicobacter pylori. Ijms 22, 10035. doi:10.3390/ijms221810035
Kim, S.-H., Lee, M. H., Park, M., Woo, H. J., Kim, Y. S., Tharmalingam, N., et al. (2018). Regulatory Effects of Black Rice Extract on Helicobacter pylori Infection-Induced Apoptosis. Mol. Nutr. Food Res. 62, 1700586. doi:10.1002/mnfr.201700586
Klein-Júnior, L. C., Santin, J. R., Lemos, M., Silveira, A. C., Rocha, J. A., Beber, A. P., et al. (2013). Role of gastric mucus secretion, oxinitrergic system and sulfhydryl groups on the gastroprotection elicited by Polygala cyparissias (Polygalaceae) in mice. J. Pharm. Pharmacol. 65, 767–776. doi:10.1111/jphp.12038
Kopacz, M., Woźnicka, E., and Gruszecka, J. (2016). Antibacterial activity of morin and its complexes with La(III), Gd(III) and Lu(III) ions. Acta Pol. Pharm. 62, 65–67.
Krzyżek, P., Migdał, P., Paluch, E., Karwańska, M., Wieliczko, A., and Gościniak, G. (2021). Myricetin as an Antivirulence Compound Interfering with a Morphological Transformation into Coccoid Forms and Potentiating Activity of Antibiotics against Helicobacter pylori. Int. J. Mol. Sci. 22, 2695. doi:10.3390/ijms22052695
Kumar, S., and Pandey, A. K. (2013). Chemistry and biological activities of flavonoids: An overview. ScientificWorldJournal 2013, 162750. doi:10.1155/2013/162750
Ladeira, M. S. P., Salvadori, D. M. F., and Rodrigues, M. A. M. (2003). Biopatologia Do Helicobacter pylori. J. Bras. Patol. Med. Lab. 39, 335–342. doi:10.1590/s1676-24442003000400011
Lahlou, M. (2013). The Success of Natural Products in Drug Discovery. Pp 04, 17–31. doi:10.4236/pp.2013.43a003
Lamb, A., and Chen, L. F. (2013). Role of the Helicobacter pylori-Induced inflammatory response in the development of gastric cancer. J. Cell.Biochem. 114, 491–497. doi:10.1002/jcb.24389
Lauren, P. (1965). THE TWO HISTOLOGICAL MAIN TYPES OF GASTRIC CARCINOMA: DIFFUSE AND SO-CALLED INTESTINAL-TYPE CARCINOMA. AN ATTEMPT AT A HISTO-CLINICAL CLASSIFICATION. Acta Pathol. Microbiol. Scand. 64, 31–49. doi:10.1111/apm.1965.64.1.31
Lawal, T. O., Olorunnipa, T. A., and Adeniyi, B. A. (2014). Susceptibility testing and bactericidal activities of Theobroma cacao Linn. (cocoa) on Helicobacter pylori in an In Vitro study. J. Herb. Med. 4, 201–207. doi:10.1016/j.hermed.2014.09.004
Lee, H. M., Hong, E., Jeon, B. Y., Kim, D. U., Byun, J. S., Lee, W., et al. (2006). Crystallization and preliminary X-ray crystallographic study of HP1043, a Helicobacter pylori orphan response regulator. Biochim. Biophys. Acta 1764, 989–991. doi:10.1016/j.bbapap.2005.10.024
Li, C., Li, X., Jiang, Z., Wang, D., Sun, L., Li, J., et al. (2022). Flavonoids Inhibit Cancer by Regulating the Competing Endogenous RNA Network. Front. Oncol. 12, 842790. doi:10.3389/fonc.2022.842790
Liberati, A., Altman, D. G., Tetzlaff, J., Mulrow, C., Gøtzsche, P. C., Ioannidis, J. P., et al. (2009). The PRISMA statement for reporting systematic reviews and meta-analyses of studies that evaluate health care interventions: Explanation and elaboration. PLoS Med. 6, e1000100. doi:10.1371/journal.pmed.1000100
Liu, C., Huang, H., Zhou, Q., Liu, B., Wang, Y., Li, P., et al. (2020). Pithecellobium clypearia extract enriched in gallic acid and luteolin has antibacterial activity against MRSA and reduces resistance to erythromycin, ceftriaxone sodium and levofloxacin. J. Appl. Microbiol. 129, 848–859. doi:10.1111/jam.14668
Loo, V. G., Fallone, C. A., De Souza, E., Lavallée, J., and Barkun, A. N. (1997). In-vitro susceptibility of Helicobacter pylori to ampicillin, clarithromycin, metronidazole and omeprazole. J. Antimicrob. Chemother. 40, 881–883. doi:10.1093/jac/40.6.881
Lu, M.-C., Chiu, H.-F., Lin, C.-P., Shen, Y.-C., Venkatakrishnan, K., and Wang, C.-K. (2018). Anti- Helicobacter pylori effect of various extracts of ixeris chinensis on inflammatory markers in human gastric epithelial AGS cells. J. Herb. Med. 11, 60–70. doi:10.1016/j.hermed.2017.08.002
Ma, J., Shen, H., Kapesa, L., and Zeng, S. (2016). Lauren classification and individualized chemotherapy in gastric cancer. Oncol. Lett. 11, 2959–2964. doi:10.3892/ol.2016.4337
Ma, Q., Wei, R., Sang, Z., and Dong, J. (2020a). Structural characterization, neuroprotective and hepatoprotective activities of flavonoids from the bulbs of Heleocharis dulcis. Bioorg. Chem. 96, 103630. doi:10.1016/j.bioorg.2020.103630
Ma, X., You, P., Xu, Y., Ye, X., Tu, Y., Liu, Y., et al. (2020b). Anti-Helicobacter pylori-associated gastritis effect of the ethyl acetate extract of Alpinia officinarum Hance through MAPK signaling pathway. J. Ethnopharmacol. 260, 113100. doi:10.1016/j.jep.2020.113100
Maleki, S. J., Crespo, J. F., and Cabanillas, B. (2019). Anti-inflammatory effects of flavonoids. Food Chem. 299, 125124. doi:10.1016/j.foodchem.2019.125124
Manayi, A., Khanavi, M., Saeidnia, S., Saiednia, S., Azizi, E., Mahmoodpour, M. R., et al. (2013). Biological activity and microscopic characterization of Lythrum salicaria L. Daru 21, 61–67. doi:10.1186/2008-2231-21-61
Maqbool, M., Amin Dar, M., Gani, I., Ahmad Mir, S., and Khan, M. (2019). Herbal Medicines As An Alternative Source of Therapy: A Review. World J. Pharm. Pharm. Sci. 8, 374–380. doi:10.20959/wjpps20192-13108
Marcial, G., Sendker, J., Brandt, S., De Lampasona, M. P., Catalán, C. A., De Valdez, G. F., et al. (2014). Gastroprotection as an example: Antiadhesion against Helicobacter pylori, anti-inflammatory and antioxidant activities of aqueous extracts from the aerial parts of Lippia integrifolia Hieron. J. Ethnopharmacol. 155, 1125–1133. doi:10.1016/j.jep.2014.06.039
Martins, A., Vasas, A., Viveiros, M., Molnár, J., Hohmann, J., and Amaral, L. (2011). Antibacterial properties of compounds isolated from Carpobrotus edulis. Int. J. Antimicrob. Agents 37, 438–444. doi:10.1016/j.ijantimicag.2011.01.016
Mazzolin, L. P., Nasser, A. L., Moraes, T. M., Santos, R. C., Nishijima, C. M., Santos, F. V., et al. (2010). Qualea parviflora Mart.: An integrative study to validate the gastroprotective, antidiarrheal, antihemorragic and mutagenic action. J. Ethnopharmacol. 127, 508–514. doi:10.1016/j.jep.2009.10.005
Mbaveng, A. T., Hamm, R., and Kuete, V. (2014). Harmful and Protective Effects of Terpenoids from African Medicinal Plants, 557–576. doi:10.1016/B978-0-12-800018-2.00019-4
McColl, K. E. (2010). Clinical practice. Helicobacter pylori infection. N. Engl. J. Med. 362, 1597–1604. doi:10.1056/NEJMcp1001110
medical, and editorial content Team (2018). About Stomach Cancer. Am. Cancer Soc., 1–14. Available at: https://www.cancer.org/content/dam/CRC/PDF/Public/8838.00.pdf.
Mendes, D. B., Lemes, L. S., Carlos, R., Cruz, S., Lúcia, M., Castro, L. De, et al. (2012). Metabólitos secundários e hipertireodismo. J. Biotechnol. Biodivers. 3, 1–69. Available at: https://www.infoteca.cnptia.embrapa.br/infoteca/handle/doc/951393.
Ming, D., Wang, D., Cao, F., Xiang, H., Mu, D., Cao, J., et al. (2017). Kaempferol inhibits the primary attachment phase of biofilm formation in Staphylococcus aureus. Front. Microbiol. 8, 1–11. doi:10.3389/fmicb.2017.02263
Minozzo, B. R., Lemes, B. M., Justo, A. D. S., Lara, J. E., Petry, V. E. K., Fernandes, D., et al. (2016). Anti-ulcer mechanisms of polyphenols extract of Euphorbia umbellata (Pax) Bruyns (Euphorbiaceae). J. Ethnopharmacol. 191, 29–40. doi:10.1016/j.jep.2016.06.032
Moher, D., Liberati, A., Tetzlaff, J., Altman, D. G., Altman, D., Antes, G., et al. (2009). Preferred reporting items for systematic reviews and meta-analyses: The PRISMA statement. PLoS Med. 6, e1000097. doi:10.1371/journal.pmed.1000097
Moon, H. I., Lee, Y. C., and Lee, J. H. (2011). Phenol glycosides with In Vitro anti-helicobacter pylori activity from hypericum erectum Thunb. Phytother. Res. 25, 1389–1391. doi:10.1002/ptr.3453
Mori, A., Nishino, C., Enoki, N., and Tawata, S. (1987). Antibacterial activity and mode of action of plant flavonoids against Proteus vulgaris and Staphylococcus aureus. Phytochemistry 26, 2231–2234. doi:10.1016/S0031-9422(00)84689-0
Moss, S. F. (2017). The Clinical Evidence Linking Helicobacter pylori to Gastric Cancer. Cell. Mol. Gastroenterol. Hepatol. 3, 183–191. doi:10.1016/j.jcmgh.2016.12.001
Mukherjee, M., Bhaskaran, N., Srinath, R., Shivaprasad, H. N., Allan, J. J., Shekhar, D., et al. (2010). Anti-ulcer and antioxidant activity of GutGard. Indian J. Exp. Biol. 48, 269–274.
Nakayama, M., Shimatani, K., Ozawa, T., Shigemune, N., Tsugukuni, T., Tomiyama, D., et al. (2013). A study of the antibacterial mechanism of catechins: Isolation and identification of Escherichia coli cell surface proteins that interact with epigallocatechin gallate. Food control. 33, 433–439. doi:10.1016/j.foodcont.2013.03.016
Nardone, G. (2006). “16 Role of Helicobacter pylori in Gastric Cancer,” in Handbook of Immunohistochemistry and in Situ Hybridization of Human Carcinomas, 205–220. doi:10.1016/S1874-5784(05)80078-9
Newman, D. J., and Cragg, G. M. (2020). Natural Products as Sources of New Drugs over the Nearly Four Decades from 01/1981 to 09/2019. J. Nat. Prod. 83, 770–803. doi:10.1021/acs.jnatprod.9b01285
Okeleye, B. I., Bessong, P. O., and Ndip, R. N. (2011). Preliminary phytochemical screening and In Vitro anti-helicobacter pylori activity of extracts of the stem bark of bridelia micrantha (Hochst., Baill., Euphorbiaceae). Molecules 16, 6193–6205. doi:10.3390/molecules16086193
Olekhnovich, I. N., Vitko, S., Chertihin, O., Hontecillas, R., Viladomiu, M., Bassaganya-Riera, J., et al. (2013). Mutations to Essential Orphan Response Regulator HP1043 of Helicobacter pylori Result in Growth-Stage Regulatory Defects. Infect. Immun. 81, 1439–1449. doi:10.1128/IAI.01193-12
Olekhnovich, I. N., Vitko, S., Valliere, M., and Hoffman, P. S. (2014). Response to Metronidazole and Oxidative Stress Is Mediated through Homeostatic Regulator HsrA (HP1043) in Helicobacter pylori. J. Bacteriol. 196, 729–739. doi:10.1128/JB.01047-13
Oliveira, D. M., Melo, F. G., Balogun, S. O., Flach, A., De Souza, E. C., De Souza, G. P., et al. (2015). Antibacterial mode of action of the hydroethanolic extract of Leonotis nepetifolia (L.) R. Br. involves bacterial membrane perturbations. J. Ethnopharmacol. 172, 356–363. doi:10.1016/j.jep.2015.06.027
Omar, S. H. (2018). Biophenols. Biophenols Impacts Prospects Anti-Alzheimer Drug Discov., 103–148. doi:10.1016/B978-0-12-809593-5.00004-5
Orditura, M., Galizia, G., Sforza, V., Gambardella, V., Fabozzi, A., Laterza, M. M., et al. (2014). Treatment of gastric cancer. World J. Gastroenterol. 20, 1635–1649. doi:10.3748/wjg.v20.i7.1635
Özçelik, B., Kartal, M., and Orhan, I. (2011). Cytotoxicity, antiviral and antimicrobial activities of alkaloids, flavonoids, and phenolic acids. Pharm. Biol. 49, 396–402. doi:10.3109/13880209.2010.519390
Palacios-Espinosa, J. F., Arroyo-García, O., García-Valencia, G., Linares, E., Bye, R., and Romero, I. (2014). Evidence of the anti-Helicobacter pylori, gastroprotective and anti-inflammatory activities of Cuphea aequipetala infusion. J. Ethnopharmacol. 151, 990–998. doi:10.1016/j.jep.2013.12.012
Pandey, A. K., and Kumar, S. (2013). Perspective on Plant Products as Antimicrobials Agents: A Review. Pharmacologia 4, 469–480. doi:10.5567/pharmacologia.2013.469.480
Park, D., Shin, K., Choi, Y., Guo, H., Cha, Y., Kim, S. H., et al. (2016). Antimicrobial activities of ethanol and butanol fractions of white rose petal extract. Regul. Toxicol. Pharmacol. 76, 57–62. doi:10.1016/j.yrtph.2016.01.011
Pastene, E., Parada, V., Avello, M., Ruiz, A., and García, A. (2014). Catechin-based Procyanidins from peumus boldus mol. aqueous extract inhibit helicobacter pylori urease and adherence to adenocarcinoma gastric cells. Phytother. Res. 28, 1637–1645. doi:10.1002/ptr.5176
Patel, K., and Patel, D. K. (2019). The Beneficial Role of Rutin, A Naturally Occurring Flavonoid in Health Promotion and Disease Prevention: A Systematic Review and Update. Bioact. Food as Diet. Interv. Arthritis Relat. Inflamm. Dis., 457–479. doi:10.1016/b978-0-12-813820-5.00026-x
Patridge, E., Gareiss, P., Kinch, M. S., and Hoyer, D. (2016). An analysis of FDA-approved drugs: Natural products and their derivatives. Drug Discov. Today 21, 204–207. doi:10.1016/j.drudis.2015.01.009
Peek, R. M. (20052005). Orchestration of aberrant epithelial signaling by Helicobacter pylori CagA. Sci. STKE 2005, pe14–280. doi:10.1126/stke.2772005pe14
Pelliciari, S., Pinatel, E., Vannini, A., Peano, C., Puccio, S., De Bellis, G., et al. (2017). Insight into the essential role of the Helicobacter pylori HP1043 orphan response regulator: genome-wide identification and characterization of the DNA-binding sites. Sci. Rep. 7, 41063. doi:10.1038/srep41063
Piasecka, A., Jedrzejczak-Rey, N., and Bednarek, P. (2015). Secondary metabolites in plant innate immunity: Conserved function of divergent chemicals. New Phytol. 206, 948–964. doi:10.1111/nph.13325
Plummer, M., de Martel, C., Vignat, J., Ferlay, J., Bray, F., and Franceschi, S. (2016). Global burden of cancers attributable to infections in 2012: a synthetic analysis. Lancet Glob. Health 4, e609–16. doi:10.1016/S2214-109X(16)30143-7
Plummer, M., Franceschi, S., Vignat, J., Forman, D., and De Martel, C. (2015). Global burden of gastric cancer attributable to Helicobacter pylori. Int. J. Cancer 136, 487–490. doi:10.1002/ijc.28999
Polk, D. B., and Peek, R. M. (2010). Helicobacter pylori: Gastric cancer and beyond. Nat. Rev. Cancer 10, 403–414. doi:10.1038/nrc2857
Puupponen-Pimia, R., Nohynek, L., Meier, C., Kahkonen, M., Heinonen, M., Hopia, A., et al. (2001). Antimicrobial properties of phenolic compounds from berries. J. Appl. Microbiol. 90, 494–507. doi:10.1046/j.1365-2672.2001.01271.x
Qian, W., Liu, M., Fu, Y., Zhang, J., Liu, W., Li, J., et al. (2020). Antimicrobial mechanism of luteolin against Staphylococcus aureus and Listeria monocytogenes and its antibiofilm properties. Microb. Pathog. 142, 104056. doi:10.1016/j.micpath.2020.104056
Qiu, J., Li, H., Meng, H., Hu, C., Li, J., Luo, M., et al. (2011). Impact of luteolin on the production of alpha-toxin by Staphylococcus aureus. Lett. Appl. Microbiol. 53, 238–243. doi:10.1111/j.1472-765X.2011.03098.x
Rahardiyan, D. (2019). Antibacterial potential of catechin of tea (Camellia sinensis) and its applications. Food Res. 3, 1–6. doi:10.26656/fr.2017.3(1).097
Rashed, K., Ćirić, A., Glamočlija, J., and Soković, M. (2014). Antibacterial and antifungal activities of methanol extract and phenolic compounds from Diospyros virginiana L. Industrial Crops Prod. 59, 210–215. doi:10.1016/j.indcrop.2014.05.021
Ribeiro, A. R., Diniz, P. B., Estevam, C. S., Pinheiro, M. S., Albuquerque-Júnior, R. L., and Thomazzi, S. M. (2013). Gastroprotective activity of the ethanol extract from the inner bark of Caesalpinia pyramidalis in rats. J. Ethnopharmacol. 147, 383–388. doi:10.1016/j.jep.2013.03.023
Ribeiro, L. H. L. (2019). Análise dos programas de plantas medicinais e fitoterápicos no Sistema Único de Saúde (SUS) sob a perspectiva territorial. Ciênc. saúde coletiva 24, 1733–1742. doi:10.1590/1413-81232018245.15842017
Russo, M., Moccia, S., Spagnuolo, C., Tedesco, I., and Russo, G. L. (2020). Roles of flavonoids against coronavirus infection. Chem. Biol. Interact. 328, 109211. doi:10.1016/j.cbi.2020.109211
Saldanha, E., Saxena, A., Kaur, K., Kalekhan, F., Venkatesh, P., Fayad, R., et al. (2019). Polyphenols in the Prevention of Ulcerative Colitis. Diet. Interv. Gastrointest. Dis. Foods, Nutr. Diet. 2, 277–287. doi:10.1016/B978-0-12-814468-8.00023-5
Santos, R. C., Kushima, H., Rodrigues, C. M., Sannomiya, M., Rocha, L. R., Bauab, T. M., et al. (2012). Byrsonima intermedia A. Juss.: Gastric and duodenal anti-ulcer, antimicrobial and antidiarrheal effects in experimental rodent models. J. Ethnopharmacol. 140, 203–212. doi:10.1016/j.jep.2011.12.008
Saravanakumar, K., Chellia, R., Hu, X., Kathiresan, K., Oh, D. H., and Wang, M. H. (2019). Eradication of Helicobacter pylori through the inhibition of urease and peptide deformylase: Computational and biological studies. Microb. Pathog. 128, 236–244. doi:10.1016/j.micpath.2019.01.001
Sathya, S., and Pandima Devi, K. (2018). The Use of Polyphenols for the Treatment of Alzheimer's Disease. Role Mediterr. Diet. Brain Neurodegener. Dis., 239–252. doi:10.1016/B978-0-12-811959-4.00015-8
Schaalan, M., Mohamed, W., and Fathy, S. (2020). MiRNA-200c, MiRNA-139 and ln RNA H19; new predictors of treatment response in H-pylori- induced gastric ulcer or progression to gastric cancer. Microb. Pathog. 149, 104442. doi:10.1016/j.micpath.2020.104442
Sepulveda, A. R. (2013). Helicobacter, Inflammation, and Gastric Cancer. Curr. Pathobiol. Rep. 1, 9–18. doi:10.1007/s40139-013-0009-8
Shankar, G. M., Antony, J., and Anto, R. J. (2015). Quercetin and Tryptanthrin: Two Broad Spectrum Anticancer Agents for Future Chemotherapeutic Interventions. Enzymes 37, 43–72. doi:10.1016/bs.enz.2015.05.001
Shen, X. F., Ren, L. B., Teng, Y., Zheng, S., Yang, X. L., Guo, X. J., et al. (2014). Luteolin decreases the attachment, invasion and cytotoxicity of UPEC in bladder epithelial cells and inhibits UPEC biofilm formation. Food Chem. Toxicol. 72, 204–211. doi:10.1016/j.fct.2014.07.019
Shields, M. (2017). Chemotherapeutics. Chemotherapeutics, 295–313. doi:10.1016/b978-0-12-802104-0.00014-7
Shin, J. E., Kim, J. M., Bae, E. A., Hyun, Y. J., and Kim, D. H. (2005). In Vitro Inhibitory Effect of Flavonoids on Growth, Infection and Vacuolation of Helicobacter pylori. Planta Med. 71, 197–201. doi:10.1055/s-2005-837816
Shivashankara, A. R., Venkatesh, S., Bhat, H. P., Palatty, P. L., and Baliga, M. S. (2015). Can Phytochemicals be Effective in Preventing Ethanol-Induced Hepatotoxicity in the Geriatric Population? An Evidence-Based Revisit. Foods Diet. Suppl. Prev. Treat. Dis. Older Adults, 163–170. doi:10.1016/B978-0-12-418680-4.00017-8
Shukla, R., Pandey, V., Vadnere, G. P., and Lodhi, S. (2019). Role of Flavonoids in Management of Inflammatory Disorders. Bioact. Food as Diet. Interv. Arthritis Relat. Inflamm. Dis., 293–322. –322. doi:10.1016/b978-0-12-813820-5.00018-0
Sinsinwar, S., and Vadivel, V. (2020). Catechin isolated from cashew nut shell exhibits antibacterial activity against clinical isolates of MRSA through ROS-mediated oxidative stress. Appl. Microbiol. Biotechnol. 104, 8279–8297. doi:10.1007/s00253-020-10853-z
Sivaranjani, M., Gowrishankar, S., Kamaladevi, A., Pandian, S. K., Balamurugan, K., and Ravi, A. V. (2016). Morin inhibits biofilm production and reduces the virulence of Listeria monocytogenes - An In Vitro and In Vivo approach. Int. J. Food Microbiol. 237, 73–82. doi:10.1016/j.ijfoodmicro.2016.08.021
Sivasothy, Y., Sulaiman, S. F., Ooi, K. L., Ibrahim, H., and Awang, K. (2013). Antioxidant and antibacterial activities of flavonoids and curcuminoids from Zingiber spectabile Griff. Food control. 30, 714–720. doi:10.1016/j.foodcont.2012.09.012
Smyth, E. C., Nilsson, M., Grabsch, H. I., van Grieken, N. C., and Lordick, F. (2020). Gastric cancer. Lancet 396, 635–648. doi:10.1016/S0140-6736(20)31288-5
Spósito, L., Oda, F. B., Vieira, J. H., Carvalho, F. A., dos Santos Ramos, M. A., de Castro, R. C., et al. (2019). In Vitro and In Vivo anti-Helicobacter pylori activity of Casearia sylvestris leaf derivatives. J. Ethnopharmacol. 233, 1–12. doi:10.1016/j.jep.2018.12.032
Srinivasan, S., Vinothkumar, V., and Murali, R. (2019). Antidiabetic Efficacy of Citrus Fruits With Special Allusion to Flavone Glycosides. Bioact. Food as Diet. Interv. Diabetes, 335–346. doi:10.1016/b978-0-12-813822-9.00022-9
Sun, Y., Sun, F., Feng, W., Qiu, X., Liu, Y., Yang, B., et al. (2017). Hyperoside inhibits biofilm formation of Pseudomonas aeruginosa. Exp. Ther. Med. 14, 1647–1652. doi:10.3892/etm.2017.4641
Sung, H., Ferlay, J., Siegel, R. L., Laversanne, M., Soerjomataram, I., Jemal, A., et al. (2021). Global Cancer Statistics 2020: GLOBOCAN Estimates of Incidence and Mortality Worldwide for 36 Cancers in 185 Countries. Ca. Cancer J. Clin. 71, 209–249. doi:10.3322/caac.21660
Tatsimo, S. J., Tamokou, Jde D., Havyarimana, L., Csupor, D., Forgo, P., Hohmann, J., et al. (2012). Antimicrobial and antioxidant activity of kaempferol rhamnoside derivatives from Bryophyllum pinnatum. BMC Res. Notes 5, 1–6. doi:10.1186/1756-0500-5-158
Thrift, A. P., and El-Serag, H. B. (2020). Burden of Gastric Cancer. Clin. Gastroenterol. Hepatol. 18, 534–542. doi:10.1016/j.cgh.2019.07.045
Tian, C., Chang, Y., Zhang, Z., Wang, H., Xiao, S., Cui, C., et al. (2019). Extraction technology, component analysis, antioxidant, antibacterial, analgesic and anti-inflammatory activities of flavonoids fraction from Tribulus terrestris L. leaves. Heliyon 5, e02234. doi:10.1016/j.heliyon.2019.e02234
Tombola, F., Campello, S., De Luca, L., Ruggiero, P., Del Giudice, G., Papini, E., et al. (2003). Plant polyphenols inhibit VacA, a toxin secreted by the gastric pathogen Helicobacter pylori. FEBS Lett. 543, 184–189. doi:10.1016/S0014-5793(03)00443-5
Tran Trung, H., Truong Thi Huynh, H., Nguyen Thi Thuy, L., Nguyen Van Minh, H., Thi Nguyen, M. N., and Luong Thi, M. N. (202020080–20089). Growth-inhibiting, bactericidal, antibiofilm, and urease inhibitory activities of hibiscus rosa sinensis L. flower constituents toward antibiotic sensitive- And resistant-strains of helicobacter pylori. ACS Omega 5, 20080–20089. doi:10.1021/acsomega.0c01640
Trojan-Rodrigues, M., Alves, T. L., Soares, G. L., and Ritter, M. R. (2012). Plants used as antidiabetics in popular medicine in Rio Grande Do Sul, southern Brazil. J. Ethnopharmacol. 139, 155–163. doi:10.1016/j.jep.2011.10.034
Tsukamoto, T., and Tatematsu, M. (2014). Role of Helicobacter pylori in gastric neoplasia. Curr. Infect. Dis. Rep. 16, 402–406. doi:10.1007/s11908-014-0402-4
Tungmunnithum, D., Thongboonyou, A., Pholboon, A., and Yangsabai, A. (2018). Flavonoids and Other Phenolic Compounds from Medicinal Plants for Pharmaceutical and Medical Aspects: An Overview. Med. (Basel) 5, 93. doi:10.3390/medicines5030093
Ustün, O., Ozçelik, B., Akyön, Y., Abbasoglu, U., and Yesilada, E. (2006). Flavonoids with anti-Helicobacter pylori activity from Cistus laurifolius leaves. J. Ethnopharmacol. 108, 457–461. doi:10.1016/j.jep.2006.06.001
Van Der Watt, E., and Pretorius, J. C. (2001). Purification and identification of active antibacterial components in Carpobrotus edulis L. J. Ethnopharmacol. 76, 87–91. doi:10.1016/S0378-8741(01)00197-0
Vaquero, M. J. R., Alberto, M. R., and de Nadra, M. C. M. (2007). Antibacterial effect of phenolic compounds from different wines. Food control. 18, 93–101. doi:10.1016/j.foodcont.2005.08.010
Waglechner, N., and Wright, G. D. (2017). Antibiotic resistance: it's bad, but why isn't it worse? BMC Biol. 15, 84–88. doi:10.1186/s12915-017-0423-1
Wang, F., Meng, W., Wang, B., and Qiao, L. (2014). Helicobacter pylori-induced gastric inflammation and gastric cancer. Cancer Lett. 345, 196–202. doi:10.1016/j.canlet.2013.08.016
Wang, S., Alseekh, S., Fernie, A. R., and Luo, J. (2019). The Structure and Function of Major Plant Metabolite Modifications. Mol. Plant 12, 899–919. doi:10.1016/j.molp.2019.06.001
Wang, Y., Zhang, J. Y., Song, X. N., Zhang, Z. Y., Li, J. F., and Li, S. (2018). Anti-ulcer and anti-Helicobacter pylori potentials of the ethyl acetate fraction of Physalis alkekengi L. var. franchetii (Solanaceae) in rodent. J. Ethnopharmacol. 211, 197–206. doi:10.1016/j.jep.2017.09.004
Wei, R., Ma, Q., Zhong, G., He, J., and Sang, Z. (2020). Isolation and characterization of flavonoid derivatives with anti-prostate cancer and hepatoprotective activities from the flowers of Hosta plantaginea (Lam.) Aschers. J. Ethnopharmacol. 253, 112685. doi:10.1016/j.jep.2020.112685
Wu, B.-L., Wu, Z.-W., Yang, F., Shen, X.-F., Wang, L., Chen, B., et al. (2019). Flavonoids from the seeds of Oroxylum indicum and their anti-inflammatory and cytotoxic activities. Phytochem. Lett. 32, 66–69. doi:10.1016/j.phytol.2019.05.003
Wu, D., Kong, Y., Han, C., Chen, J., Hu, L., Jiang, H., et al. (2008). d-Alanine:d-alanine ligase as a new target for the flavonoids quercetin and apigenin. Int. J. Antimicrob. Agents 32, 421–426. doi:10.1016/j.ijantimicag.2008.06.010
Wu, T., Zang, X., He, M., Pan, S., and Xu, X. (2013). Structure-activity relationship of flavonoids on their anti-Escherichia coli activity and inhibition of DNA gyrase. J. Agric. Food Chem. 61, 8185–8190. doi:10.1021/jf402222v
Xie, Y., Yang, W., Tang, F., Chen, X., and Ren, L. (2014). Antibacterial Activities of Flavonoids: Structure-Activity Relationship and Mechanism. Curr. Med. Chem. 22, 132–149. doi:10.2174/0929867321666140916113443
Yamaoka, Y. (2010). Mechanisms of disease: Helicobacter pylori virulence factors. Nat. Rev. Gastroenterol. Hepatol. 7, 629–641. doi:10.1038/nrgastro.2010.154
Yamaoka, Y. (2012). Pathogenesis ofHelicobacter pylori-Related Gastroduodenal Diseases from Molecular Epidemiological Studies. Gastroenterology Res. Pract. 2012, 1–9. doi:10.1155/2012/371503
Yeon, M. J., Lee, M. H., Kim, D. H., Yang, J. Y., Woo, H. J., Kwon, H. J., et al. (2019). Anti-inflammatory effects of Kaempferol on Helicobacter pylori-induced inflammation. Biosci. Biotechnol. Biochem. 83, 166–173. doi:10.1080/09168451.2018.1528140
Yin, X., Gyles, C. L., and Gong, J. (2012). Grapefruit juice and its constituents augment the effect of low pH on inhibition of survival and adherence to intestinal epithelial cells of Salmonella enterica serovar Typhimurium PT193. Int. J. Food Microbiol. 158, 232–238. doi:10.1016/j.ijfoodmicro.2012.07.022
Zengin, G., Menghini, L., Di Sotto, A., Mancinelli, F., Carradori, S., Cesa, S., et al. (2018). Chromatographic analyses, In Vitro biological activities, and cytotoxicity of cannabis sativa l. Essential oil: A multidisciplinary study. Molecules 23. doi:10.3390/molecules23123266
Zhang, L., Kong, Y., Wu, D., Zhang, H., Wu, J., Chen, J., et al. (2008). Three flavonoids targeting the beta-hydroxyacyl-acyl carrier protein dehydratase from Helicobacter pylori: crystal structure characterization with enzymatic inhibition assay. Protein Sci. 17, 1971–1978. doi:10.1110/ps.036186.108
Zuanazzi, J. A. S., and Montanha, J. A. (2007). “Flavonóides,” in Farmacognosia - Da Planta Ao Medicamento (Porto Alegre: Editora da UFRGS), 577–614.
Keywords: phytotherapy, stomach neoplasms, anti-infective agents, ethnopharmacology, preventive medicine
Citation: Ivyna de Araújo Rêgo R, Guedes Silvestre GF, Ferreira de Melo D, Albino SL, Pimentel MM, Silva Costa Cruz SB, Silva Wurzba SD, Rodrigues WF, Goulart de Lima Damasceno BP and Cançado Castellano LR (2022) Flavonoids-Rich Plant Extracts Against Helicobacter pylori Infection as Prevention to Gastric Cancer. Front. Pharmacol. 13:951125. doi: 10.3389/fphar.2022.951125
Received: 23 May 2022; Accepted: 20 June 2022;
Published: 31 August 2022.
Edited by:
Muhammad Hasnat, University of Veterinary and Animal Sciences, PakistanReviewed by:
Hafiz Ishfaq Ahmad, University of Veterinary and Animal Sciences, PakistanFaisal Raza, Shanghai Jiao Tong University, China
Copyright © 2022 Ivyna de Araújo Rêgo, Guedes Silvestre, Ferreira de Melo, Albino, Pimentel, Silva Costa Cruz, Silva Wurzba, Rodrigues, Goulart de Lima Damasceno and Cançado Castellano. This is an open-access article distributed under the terms of the Creative Commons Attribution License (CC BY). The use, distribution or reproduction in other forums is permitted, provided the original author(s) and the copyright owner(s) are credited and that the original publication in this journal is cited, in accordance with accepted academic practice. No use, distribution or reproduction is permitted which does not comply with these terms.
*Correspondence: Lúcio Roberto Cançado Castellano, bHVjaW9jYXN0ZWxsYW5vMkBnbWFpbC5jb20=
†ORCID: Renaly Ivyna de Araújo Rêgo, orcid.org/0000-0002-1085-0127; Geovana Ferreira Guedes Silvestre, orcid.org/0000-0002-5783-0449; Demis Ferreira de Melo, orcid.org/0000-0001-8486-5578; Sonaly Lima Albino, orcid.org/0000-0002-2904-036X; Marcela Monteiro Pimentel, orcid.org/0000-0001-9059-1120; Sara Brito Silva Costa Cruz, orcid.org/0000-0003-1509-900X; Sabrina Daniela Silva Wurzba, orcid.org/0000-0002-3742-2819; Wellington Francisco Rodrigues, orcid.org/0000-0002-3426-2186; Bolívar Ponciano Goulart de Lima Damasceno, orcid.org/0000-0002-0747-0297; Lúcio Roberto Cançado Castellano, orcid.org/0000-0003-0851-5298