- Department of Orthopedics, The Fourth Affiliated Hospital of China Medical University, Shenyang, China
In many organisms, antimicrobial peptides (AMPs) display wide activities in innate host defense against microbial pathogens. Mammalian AMPs include the cathelicidin and defensin families. LL37 is the only one member of the cathelicidin family of host defense peptides expressed in humans. Since its discovery, it has become clear that they have pleiotropic effects. In addition to its antibacterial properties, many studies have shown that LL37 is also involved in a wide variety of biological activities, including tissue repair, inflammatory responses, hemotaxis, and chemokine induction. Moreover, recent studies suggest that LL37 exhibits the intricate and contradictory effects in promoting or inhibiting tumor growth. Indeed, an increasing amount of evidence suggests that human LL37 including its fragments and analogs shows anticancer effects on many kinds of cancer cell lines, although LL37 is also involved in cancer progression. Focusing on recent information, in this review, we explore and summarize how LL37 contributes to anticancer effect as well as discuss the strategies to enhance delivery of this peptide and selectivity for cancer cells.
Introduction
As the key components of the innate host immune system, antimicrobial peptides (AMPs) have been discovered in almost all life forms, ranging from bacteria to higher mammals, and act as primary defense against a broad spectrum of pathogens (Jafari et al., 2022). Mammalian AMPs include the cathelicidin and defensin families. Cathelicidins possess a highly conserved cathelin-like prosequence and variable carboxyl-terminal sequences that are consistent with the mature AMPs (Johansson et al., 1998). The only member of cathelicidin identified in humans is hCAP18, which is a positively charged antibacterial protein, with a molecular weight of 18 kDa. LL-37 is released as an active domain of hCAP18 through extracellular cleavage mediated by proteinase-3 enzyme (Kuroda et al., 2015a).
A number of studies have reported that LL-37 exerts a diverse range of pleiotropic attributes including antimicrobial activities, immunity, angiogenesis, wound repair, and bone tissue engineering (Tjabringa et al., 2003; Elssner et al., 2004; Bucki et al., 2010; Pfosser et al., 2010; Ramos et al., 2011; Liu et al., 2018; Mitchell et al., 2022). However, different from its traditional roles, emerging evidence from cancer biology studies suggests that LL-37 might promote or inhibit tumor progression (Ren et al., 2012; Piktel et al., 2016; Chen et al., 2018; Jiang et al., 2020; Chen et al., 2021; Vitale et al., 2021; Kiatsurayanon et al., 2022; Zhang et al., 2022). LL-37 plays an important and complex role in the regulation of different human cancers. These data are beginning to reveal the complex and contradictory functions of LL-37.
In this review, we first introduce the characteristic features of LL-37, focusing on its anticancer effects on various human cancers and the underlying mechanisms involved. Based on the recent studies, we also discuss the therapeutic implications of LL-37 as a potential anticancer drug. We believe that this important peptide will eventually be developed into a new anticancer drug suitable for clinical use in the future.
Characteristics and structure of LL-37
Characteristics of LL-37
Human AMPs include the cathelicidin and defensin families. Different from other animals, there is only one cathelicidin gene in humans (Frohm et al., 1997; Zanetti, 2005). As shown in Figure 1, the single cathelicidin gene called CAMP located on the human chromosome 3p21.3 encodes the human cationic antimicrobial peptide-18 (hCAP18) which is composed of 170 amino acids (Zanetti, 2004; Dürr et al., 2006). Like most antimicrobial peptides, hCAP18 is also produced as inactive preproproteins. It is a major component of the azurophilic granules of the neutrophils (Cowland et al., 1995; Sørensen et al., 2001) and is primarily produced by bone marrow, keratinocytes of inflamed sites, and cells of the mucosal epithelium (Agerberth et al., 1995; Chen and Fang, 2004; Tjabringa et al., 2005; Wolk et al., 2006). Once cell injury or infection occurs, it can provide a trigger to activate the cell degranulation by stimulating toll-like receptors (TLRs) and/or altering the cytokine (Vandamme et al., 2012). Thereafter, the inactive hCAP18 precursor protein is released from the intracellular environment and then processed by the proteolytic cleavage into the active LL-37 peptide (Zaiou et al., 2003; Fahy and Wewers, 2005; Pazgier et al., 2013).
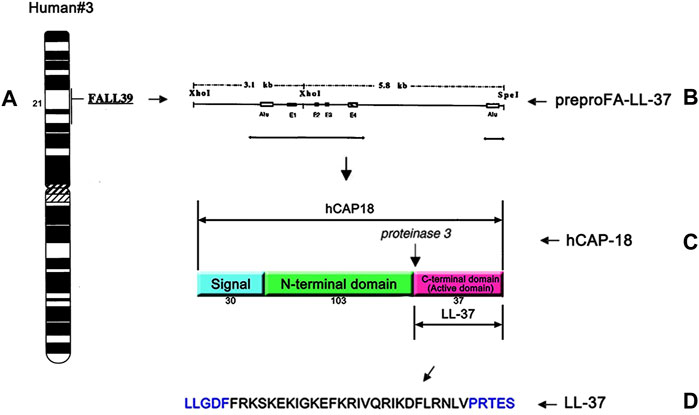
FIGURE 1. Single cathelicidin gene called CAMP located on human chromosome 3p21.3 encodes hCAP18 (A), a schematic drawing of cDNA for the complete prepro-LL-37 (B), structure and cleavage sites of hCAP18 (C), and the amino acid sequence of the antibacterial peptide LL-37 (D). The human cathelicidin hCAP18 consists of a signal peptide (30 amino acids), N-terminal domain (103 amino acids), and C-terminal domain (37 amino acids). The C-terminal domain shows various activities as an active domain and is called LL-37.
LL-37 (4.5 kDa) is an active 37-amino acid peptide. The precursor protein pre-hCAP18 (18 kDa) is converted into propeptide hCAP18 (16 kDa) via processing of the signaling peptide, and then the active LL-37 peptide is produced from the C-terminus of hCAP18 via specific serine proteases, for instance, proteinase 3 (PR3) (Vandamme et al., 2012; Gudmundsson et al., 1996). Its primary sequence is LLGDFFRKSKEKIGKEFKRIVQRIKDFLRNLVPRTES (Gudmundsson et al., 1996). LL-37 is commonly found in mucosal secretion, sweat, semen, urine, breast milk, and plasma (Malm et al., 2000; Murakami et al., 2002; Armogida et al., 2004; Rieg et al., 2005; Berkestedt et al., 2010; Fábián et al., 2012; Babikir et al., 2018).
Structure of LL-37
According to previous circular dichroism (CD), Fourier transform infrared (FT-IR) (Tossi et al., 1994; Oren et al., 1999) spectroscopy, and NMR spectroscopy studies (Porcelli et al., 2008; Wang, 2008; Wang et al., 2014), LL-37 possesses a linear cationic α-helical structure which might aid to exert its function. As shown in Figures 2A,B, the α-helical structure spanning residues 2 to 31 with unstructured C-terminal residues 32 to 37 consists of three parts, namely, an N-terminal α-helix with a pair of leucine residues (LL), a C-terminal α-helix, and a disordered C-terminal tail (residues 32–37) (Porcelli et al., 2008; Wang, 2008; Wang et al., 2019). The C-terminal tail is mobile, while the helical region is rigid. LL-37 is bent with a series of hydrophobic side chains, whereas its hydrophobic surface bordered by the positively charged residues is composed of four distinct aromatic phenylalanine side chains that all point in the same direction (Wang, 2008; Vandamme et al., 2012). The amphiphilic peptide with a positive charge and net charge of +6 can facilitate an interaction with the negatively charged molecules or structures, such as bacterial cell walls (Wang, 2008). Similarly, LL-37 also targets and binds to the cancer cells as the anionic phosphoryl serine is exposed on their surface (Wu et al., 2010a). Interestingly, not only antimicrobial but also the anticancer effect is primarily exerted by the C-terminal helix (Li et al., 2006; Falcao et al., 2015). Moreover, the N-terminal helix has been related to hemolytic activity, proteolytic resistance, and chemotaxis, whereas the disordered C-terminal tail is essential for tetramerization (Wang, 2014). The two helices are separated by a bend or break. Furthermore, it has been found that the discontinuation is found on the hydrophobic surface at S9, rather than the helix (Figures 2B,C) (Wang, 2008; Zhang et al., 2021).
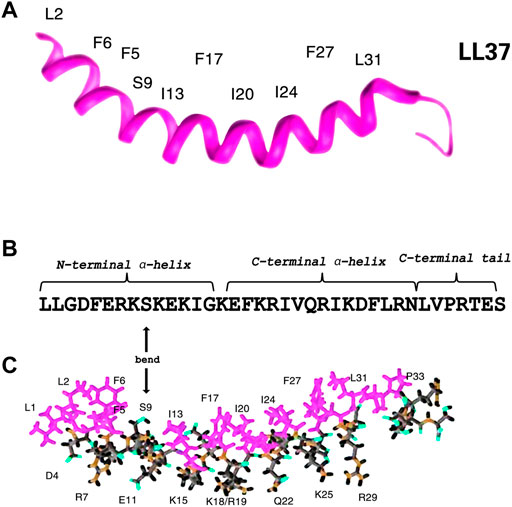
FIGURE 2. Three-dimensional structure of human cathelicidin reveals a helix followed by a C-terminal tail. Note the four phenylalanine side chains lying on the concave surface of the peptide (F6, F5, F17, and F27) (A). Sequence of LL-37. S9 is marked (B). Stick view of the structure of LL-37 with hydrophobic and hydrophilic residues selectively labeled. In both views, hydrophobic amino acids are in purplish red (C). Therefore, it is evident that there is a discontinuation of the hydrophobic surface at S9 rather than the helices (Wang, 2008).
How LL-37 can eradicate/affect cancer?
The cytotoxic effects of numerous AMPs on different tumor cell lines have been reported previously (Cheng et al., 2020; Lee et al., 2021; Athira et al., 2022; Jafari et al., 2022; Patil and Kunda, 2022). These AMPs contain several cationic and hydrophobic amino acids and were found to be involved in various anticancer activities. They were thus termed as anticancer peptides (ACPs) (Hoskin and Ramamoorthy, 2008). ACPs can bind and kill the cancer cells through direct or indirect mechanisms (Dennison et al., 2006; Huang et al., 2015).
ACPs exert their biological functions in a diverse manner. These ACPs generally contain positively charged amino acids like lysine and arginine and possess a net positive charge ranging from +1 to +9 at neutral pH (Habes et al., 2019; Chiangjong et al., 2020). Moreover, as AMPs bind with bacterial membranes, ACPs can bind directly with the cancer cell walls due to their cationic and amphipathic nature (Ma et al., 2019). It has been established that different from normal eukaryotic cell membranes which are made of uncharged neutral phospholipids, sphingomyelins, and cholesterol and are neutral in charge (Zachowski, 1993; Doktorova et al., 2020), the surface of the cancer cells is net negatively charged because of increased proportions of anionic phosphatidylserine, heparan, and chondroitin sulfate proteoglycans, O-glycosylated mucins, and sialylated glycoproteins (Warren, 1974; Warren et al., 1979; Utsugi et al., 1991; Zwaal et al., 2005; Calianese and Birge, 2020; Brockhausen and Melamed, 2021; Hassan et al., 2021; Hugonnet et al., 2021). ACPs can selectively recognize cancer cells by electrostatic interactions with the negatively charged phospholipids on the surface. Some ACPs tend to kill cancer cells by causing membrane perturbation; however, some ACPs can penetrate the target cell and disrupt the mitochondrial membrane, thereby resulting in apoptosis (Deslouches and Di, 2017). ACPs bind to the membranes in different models, including carpet model, surface binding non-inserted, and perpendicular to the surface (Quemé-Peña et al., 2021). ACPs can enter the cells through two distinct mechanisms: direct or indirect. The former causes irreparable membrane damage, followed by the cell lysis, which is non-energy dependent, and the latter can modulate the integrity of the cancer cell membrane by altering some intracellular pathways, thereby resulting in cell death by apoptosis, which is energy dependent (Kumar et al., 2018; Hilchie et al., 2019; Jafari et al., 2022).
One of the best-studied ACPs is LL-37. However, contradictory results have been shown for LL-37 linked to cancers in different models. The existing data indicate that LL-37 can exert a tumorigenic effect in some cancers, including lung cancer, breast cancer, ovarian cancer, melanoma, prostate cancer, liver cancer, and skin squamous cell carcinoma (Coffelt et al., 2009; Cha et al., 2016; Muñoz et al., 2016; Wang et al., 2017; Habes et al., 2019; Jiang et al., 2020; Ding et al., 2021; Zhang et al., 2022). Mechanistically, LL-37 activated Wnt/β-catenin signaling by inducing the phosphorylation of protein kinase B and subsequent phosphorylation of glycogen synthase kinase 3β mediated by the toll-like receptor-4 expressed in lung tumor cells (Ji et al., 2019). Furthermore, LL-37 cooperated with IL-33 to increase the phosphorylation of p38 MAPK and NF-κB p65 pathways and augmented IL-6 and IL-1β secretion, which resulted in the proliferation of lung cancer cells. Sulfated glycoaminoglycans and proteoglycan syndecan-4 increase the binding of LL-37 to the cell surface, which promotes the migration of breast cancer cells. In addition, via activating TRPV2 and PI3/Akt signaling, and then inducing recruitment of TRPV2 from intracellular vesicles to the plasma membrane of pseudopodia, LL-37 promotes proliferation and growth of breast cancer cells (Farabaugh et al., 2016). On the contrary, it has also been shown that LL-37 can exert anticancer effects on other cancers, including colon cancer, glioblastoma, hematologic malignancy, gastric cancer, and oral squamous cell carcinoma (Aarbiou et al., 2006; Wu et al., 2010b; Bruns et al., 2015; Prevete et al., 2015; Chen et al., 2020; Porter et al., 2021; Chernov et al., 2022). There is no smoking gun to explain the reported opposite effects on different cancer types. Whether and how LL-37 can affect cancer and metastasis deserves further studies. In the next section, our principal discussion focusses on the potential anticancer mechanisms of LL-37.
The membranolytic mechanisms
LL-37 could directly bind and perturb efficiently zwitterionic PC (phosphatidylcholine) and negatively charged PC/PS (phosphatidylcholine/phosphatidylserine) phospholipid membranes (Juba et al., 2015). The initial interaction with the membrane is primarily brought about by various electrostatic forces, and the correlation between the cationic charge and biological activity is strengthened with the increasing charge until the optimum charge for activity has been reached (Fillion et al., 2015; Juba et al., 2015). The presence of the negatively charged lipids such as anionic phosphatidylserine (PS) in membranes of the cancer cells can also mediate an electrostatic interaction with the cationic peptides (Alvares et al., 2017; Vasquez-Montes et al., 2019). In addition to its high net positive charge (+6) (Figure 3) that can markedly reduce the repulsive forces via neutralization by the negative charges, the high affinity of LL-37 for the negatively charged membranes in light of its hydrophobic interactions between the peptide and the membranes has been reported (Oren et al., 1999; Shai, 2002).
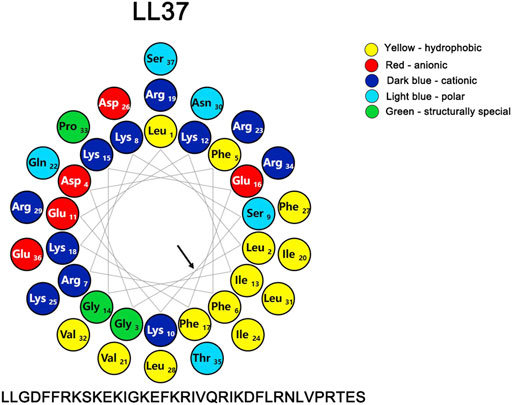
FIGURE 3. Helical wheel representation of LL-37, illustrating the amphipathic and cationic nature of LL-37. The residues are color coded: potentially negatively charged as red, potentially positively charged as dark blue, the hydrophobic residue is in yellow, the polar residues are coded as light blue, and the structurally special residues are coded as green. The helix diagram of the polypeptide was drawn with a Protein ORIGAMI (Reißer et al., 2018) software package. The arrow indicates the hydrophobic surface of the peptide.
A number of studies (Pouny and Shai, 1992; Oren et al., 1999; Ding et al., 2013; Wang, 2015; Lee et al., 2016; Zhao et al., 2018) have shown that, different from other ACPs, the model of action of LL-37 with negatively charged membranes such as the membranes of the cancer cells is a detergent-like effect exhibited through a “carpet-like” mechanism rather than a channel-forming model. In contrast to the channel formation mechanism, when bound to either zwitterionic PC or negatively charged PC/PS, LL-37 can effectively dissociate into monomers, and the hydrophobic N-terminus of LL-37 is buried only slightly in the membrane.
Specifically (Oren et al., 1999; Shai, 2002; Lee et al., 2016; Quemé-Peña et al., 2021), as shown in Figure 4, LL-37 reaches and remains on the negatively charged membranes such as the membranes of cancer cells as oligomers of different sizes; thereafter, a change in the membrane energetics and fluidity causes several local perturbations followed by dissociation into the monomers. Afterward, it is bound to the surface of the membrane, with the hydrophobic surface facing the membrane and the hydrophilic surface facing the solvent. When the threshold concentration is reached, the peptide monomers can easily diffuse into the membrane, cover, and disintegrate it in a detergent-like manner through a “carpet-like” mechanism. The overall outcome can lead to cancer cell death, such as that reported in acute myeloid leukemia cells (Xhindoli et al., 2014), bronchial epithelial cancer cells (Tzitzilis et al., 2020), and human osteosarcoma cells (Bankell et al., 2021a).
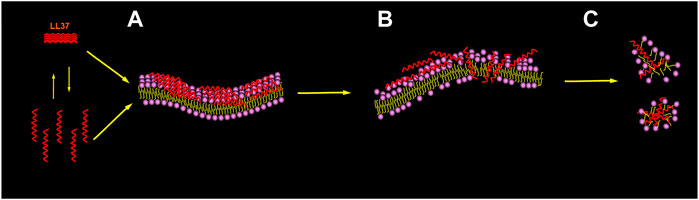
FIGURE 4. Membrane-associated mechanism for the peptide. Picture illustrating the carpet model recommended for membrane permeation. The initial binding to the membrane interface is mediated by the electrostatic interaction. The peptide reaches the membrane in the form of a monomer or oligomer and then binds to the membrane surface (A). When the threshold concentration of peptide monomer is reached, the membrane is penetrated and forms instantaneous pores (B), which also leads to membrane disintegration (C).
The non-membranolytic mechanisms
It was originally thought that membranolytic mechanisms were the only mechanism of action, but there is increasing evidence now to suggest that there may be also additional or complementary non-membranolytic mechanisms (Figure 5), such as a receptor-mediated mechanism.
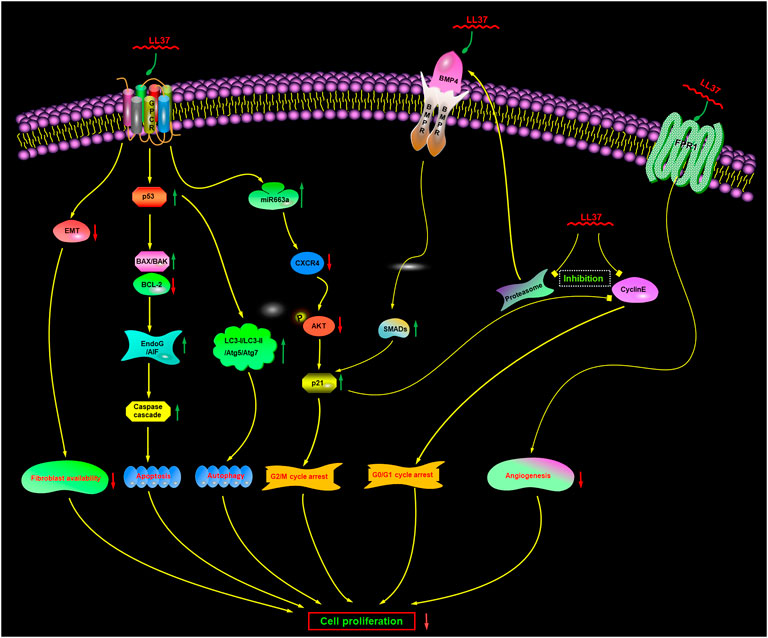
FIGURE 5. Proposed non-membranolytic anticancer mechanism of human cathelicidin LL-37. Inhibition of proteasome activity induces the upregulation of BMP4, which subsequently activates BMP signaling. GPCR, G protein-coupled receptor; CXCR4, CXC chemokine receptor type 4; EndoG, endonuclease G; AIF, apoptosis-inducing factor; FPR1, formyl peptide receptor 1. BMP4, bone morphogenetic protein 4; BMPR, bone morphogenetic protein receptor.
G protein-coupled receptors
G protein-coupled receptors (GPCRs) are membrane-embedded receptors that can regulate several important biological functions. In some cancer cells (Mader et al., 2009; Ren et al., 2012; Piktel et al., 2016), LL-37 induces characteristic apoptotic cell death in a caspase-independent manner, such as phosphatidylserine externalization and DNA fragmentation, without activation of caspases. One requirement for caspase-independent apoptosis of cancer cells is the altered activity of Bcl-2 and p53. LL-37 has been reported to reduce the level of antiapoptotic Bcl-2 and increase the level of pro-apoptotic Bax/Bak (Mader et al., 2009; Ren et al., 2012; Ren et al., 2013; Chen et al., 2020; Yang et al., 2021). LL-37 can also increase the expression of p53 and p53-upregulated modulator of apoptosis (PUMA) (Ren et al., 2012; Piktel et al., 2016; Chen et al., 2020). PUMA, a direct transcriptional target of p53, is a highly efficient pro-apoptotic protein and acts as a modulator of apoptosis in several cancer cell lines (Han et al., 2001; Yu et al., 2001; Jeffers et al., 2003; Yu et al., 2003; Yu and Zhang, 2003; Roufayel et al., 2022). Another requirement for the caspase-independent apoptosis of cancer cells is the upregulated expression and translocation of apoptosis-inducing factor (AIF) and endonuclease G (EndoG). After treatment with LL-37, the nuclear levels of both AIF and EndoG are prominently increased and translocated from the mitochondria into the nucleus, resulting in cancer cell apoptosis that is caspase-independent but calpain- and AIF-dependent apoptosis and mediated via BAX activation (Mader et al., 2009; Ren et al., 2012; Açil et al., 2018; Bankell et al., 2021b).
Nevertheless, interestingly, recent studies have suggested that except in a caspase-independent manner, the cell apoptosis induced by LL-37 can also occur through a caspase-dependent manner (Açil et al., 2018; Chen et al., 2020) via the p53-Bcl-2/BAX signaling pathway.
So, a mechanism was inferred that LL-37 can potentially exert its apoptogenic action in a caspase-independent or caspase-dependent manner via activating a GPCR-p53-Bax/Bak/Bcl-2 signaling cascade to trigger AIF/EndoG-mediated apoptosis.
Regulation of the proteasome activation via bone morphogenetic protein signaling
The bone morphogenetic protein (BMP) signal is an important tumor suppressive pathway involved in the process of tumorigenesis. It is initiated via the binding of BMP ligands to BMP receptors, which can then recruit and phosphorylate the downstream Smad1/5/8. Thereafter, the heterodimers are formed by phosphorylated Smads with Smad4, which can translocate into the nucleus as transcription factors to induce the transcription of various genes mediating the biological effects of BMPs (Varga and Wrana, 2005). The proteasome is a multimeric protein complex with proteolytic activity, which can effectively upregulate the level of BMP ligands and stimulate the phosphorylation of Smad1/5/8 (Wu et al., 2008a; Wu et al., 2008b; Zhang et al., 2014).
The anticancer effect of LL-37 has been reported to involve regulation of the proteasome activation via modulation of BMP signaling (Rajkumar et al., 2005; Wu et al., 2010b; Wu et al., 2010c). The chymotrypsin-like and caspase-like activities of 20S proteasome have been reported to be significantly inhibited by LL-37. The expression of BMP4 and the phosphorylation of Smad1/5 are upregulated, and then the expression of p21Waf1 is subsequently induced at both the protein and mRNA levels (Rajkumar et al., 2005; Wu et al., 2010b). Furthermore, RNA interference which can target BMP receptor II was found to partially block the activation of the BMP signal and the inhibition of cell proliferation induced by LL-37. Moreover, LL-37 can also downregulate the expression level of cyclin E2 (Wu et al., 2010b). Both p21WAF1 and cyclin E2 can regulate the cell cycle progression by affecting the late G1 phase (Bartek and Lukas, 2001). As shown in Figure 5, the alteration of p21 and cyclin E2 expression levels can trigger G0/G1 phase cell cycle arrest and contribute to the antitumor effects of LL-37 (Wu et al., 2010a; Wu et al., 2010b). Furthermore, MG-132, the proteasome inhibitor, can produce similar effects to those of LL-37. It can induce the BMP/p21 cascade to inhibit cell proliferation in the gastric cancer cells. However, the inhibition of cancer cell proliferation could not be blocked by pertussis toxin. These findings clearly suggested that LL-37 could exert its anticancer effects through the activation of BMP signaling via a proteasome-dependent mechanism (Wu et al., 2010b).
LL-37 can act as an antitumor immunostimulatory agent on the host immune system
Immune modulation and anticancer activity are the two different faces of the same coin. A recent study has conclusively demonstrated that LL-37 can significantly influence immune responses as an essential component of innate immunity (Yang et al., 2020). Aside from the anticancer activity of LL-37, the immunostimulatory or adjuvant effect has also been used. CpG-oligodeoxynucleotides (CpG-ODNs), a toll-like receptor TLR9 ligand, are employed to enhance the tumor suppressive activity of the host immune cells in immunotherapy (Wu et al., 2010a). It has been shown that LL-37 can markedly enhance the perception of CpG-ODN and then induce the proliferation and activation of the host immune cells, such as natural killer (NK) cells, plasmacytoid dendritic cells, and B lymphocytes. These cells can thereafter induct and maintain antitumor immune responses and mediate tumor destruction (Chuang et al., 2009; Büchau et al., 2010; Hurtado and Peh, 2010).
Furthermore, it has been shown that LL-37 can act and expand OVA-antigen-specific CD8+ T cells in draining the lymph nodes and the tumor microenvironment (Mader et al., 2011a; Singh et al., 2012), which could potentially delay tumor growth. LL-37 can also promote an anticancer immune response via inhibiting CD25+CD4+FOXP3+T regulatory cells (Mader et al., 2011b). Moreover, some studies have demonstrated that intra-tumoral injections of LL-37 stimulate the innate immune system by acting plasmacytoid dendritic cells, which can in turn mediate tumor destruction (Dolkar et al., 2018). In fact, LL-37 has been utilized in a phase 1 clinical trial for melanoma patients with cutaneous metastases via intra-tumoral injections. These findings suggested that LL-37 could be employed as an antitumor immunostimulatory agent and could provide a promising strategy for antitumor immunotherapy.
Strategies to enhance LL-37 delivery and selectivity for cancer cells
Since both anticancer and cytotoxic activities of LL-37 are inhibited in human plasma, the delivery platform and modification strategies might be needed to ensure that LL-37 can reach the tumor microenvironment and promote tumor cell targeting, such as the use of nanoparticles and fusogenic liposomes and the design of peptides (Wang et al., 1998; Hilchie et al., 2019; Wang et al., 2019).
Use of nano-sized drug delivery systems
Application of nano-sized drug delivery systems can serve as a potential strategy to improve the delivery of peptides into host cells (Radaic et al., 2020). Nanoparticles with different structures and materials have been examined previously to facilitate the optimal delivery of anticancer peptides (Marverti et al., 2020; Akkın et al., 2021; Zielińska et al., 2021). In addition to being stable and non-toxic, the nanoparticles must be targetable in order to facilitate directed delivery of drugs to the exact tissues or cells (Hilchie et al., 2019). For instance, it has been reported that LL-37 loaded onto zinc oxide nanoparticles (ZnO NP) significantly suppressed the growth of the human lung cancer model cell line (BEAS-2B) (DeLong et al., 2019). Moreover, LL-37-loaded thermosensitive hydrogel nanoparticles displayed improved antiangiogenesis and antitumor activity (Fan et al., 2015). Moreover, it has been shown that CaP nanoparticles also can protect LL-37 from proteolysis (Tsikourkitoudi et al., 2020). Moreover, as reported in the literature, the anticancer activity of LL-37 improved when loaded onto the magnetic nanoparticles (Niemirowicz et al., 2015; Niemirowicz et al., 2017; Wnorowska et al., 2020).
Liposomes are lipid-based nanoparticles. Hydrophobic or hydrophilic drugs can be directly delivered into the target cancer cells via using fusogenic liposomes without the risk of degradation by the endocytic pathway (Malam et al., 2009; Kube et al., 2017). The drawbacks associated with use of liposomes include spontaneous fusion of the liposome membranes, which can cause decreased drug payload concentration and increase off-target toxicity (Monteiro et al., 2018; Akbarian et al., 2020). In order to solve these problems, nanoassemblies have been designed as an effective drug delivery vehicle. The lipid-coated targeted nanoassembly composed of Col@MSN@LL-(LL-37) has proved to be a successful delivery platform (Rathnayake et al., 2020).
These findings suggested that the formulation of LL-37 with nanoparticles could be successfully used as a potential therapeutic strategy to enhance the delivery of LL-37 against cancers.
Modification and alteration of the peptide
Another potential problem associated with LL-37 peptide is that it can be easily degraded by proteolytic enzymes present in the digestive system and blood plasma (Vlieghe et al., 2010). Susceptibility to degradation is primarily dependent on the peptide sequence. However, modification of the peptide and alteration of the sequence, such as the use of d-amino acid, sequence truncation, and modifications of C- and N-terminal, can render it unrecognizable by the various proteolytic enzymes and even influence the selectivity of the cancer cells as a basis for developing alternative cancer treatment approaches (Wang et al., 2019; Tornesello et al., 2020; Trinidad-Calderón et al., 2021). For instance, part of the LL-37 C-terminal domain, peptide sequence: FRKSKEKIGKEFKRIVQRIKDFLRNLV was found to display antiproliferative effects on human squamous cell carcinoma (Okumura et al., 2004). Moreover, a part of LL-37, KR12C: N-KRIVKLIKKWLR-C, could promote apoptosis in human breast cancer cells (Sengupta et al., 2018). The LL-37 fragments and analog peptides, such as FF/CAP18: FRKSKEKIGKFFKRIVQRIFDFLRNLV, with replacements of a glutamic acid residue and a phenylalanine at position 20, exhibited the functions of both inhibiting proliferation and promoting apoptosis in colon cancer (Kuroda et al., 2012; Kuroda et al., 2015b; Kuroda et al., 2017; Hayashi et al., 2018). Interestingly, the residues 17–32 of LL-37, abbreviated as FK-16 (FKRIVQRIKDFLRNLV) were found to induce apoptotic cell death and autophagy in the cancer cells, and these effects were even superior to that of LL-37 (Li et al., 2006; Ren et al., 2013; Zhang et al., 2019). It was observed that these peptides containing amino acid substitutions induce apoptosis in some specific types of cancer cells that have more negatively charged cell membranes than those in the normal cells, largely as compared to the original peptide. Furthermore, the variant of LL-37, obtained by cutting out both the C-terminus coil part and the N-terminus heparan sulfate binding region and replacing some positively charged amino acids with histidines, was found to display higher affinity and generic tumor selectivity than the original peptide (Capozzi et al., 2018). Specific positional Q and K mutants of LL-37 were observed to have lower hemolytic toxicities and preserved the cell-penetrating ability of human breast cancer cells (Kim et al., 2016).
Combinatorial applications of LL-37
Interestingly, some evidence suggests that combined treatment using LL-37 and chemotherapy drugs can yield better results. For example, combinatorial application of LL-37 and etoposide exhibited significantly better antitumor effects on C6 glioma cells (Chernov et al., 2022). Compared with CpG ODN or LL-37 alone, the combination of LL-37 and CpG-ODN in the treatment of ovarian cancer can produce better antitumor effects and improve survival rates (Chuang et al., 2009). The mechanism can be expressed as the combinational use of LL-37, and CpG-ODN enhances the ability of human B lymphocytes and plasma-like dendritic cells to recognize and bind to CpG oligonucleotide and then leading to the activation of TLR-9 (Hurtado and Peh, 2010). Furthermore, the anticancer efficacy of the LL-37 fragment peptide analog was enhanced via linking PLGA conjugate (Mori et al., 2021). Compared with the peptide alone, the conjugate micelles were shown to effectively inhibit tumor cells and increase cell permeability in colon cancer, gastric cancer, hematologic malignancy, and oral squamous cell carcinoma. In addition, when LL37 was genetically fused with M-CSFRJ6-1 in the murine model, the antitumor immune response of the M-CSFRJ6-1 DNA vaccine was also enhanced (An et al., 2005). It suggests a possible use of LL-37 as an immune adjuvant in the gene therapy of some types of diseases, such as leukemia, Hodgkin’s disease, and many solid tumors. This practical approach not only enhances the effect of traditional anticancer drugs but also markedly reduces the dosage of peptide and potential cytotoxicity.
Conclusion and future perspectives
Human cathelicidin LL-37 is an interesting peptide, which can display multiple functional roles and has been implicated in numerous diseases. The extensive functions of the peptide provide a scientific basis for analyzing its potential applications. The high interest in the therapeutic potential of this peptide originates from its potency against targeting bacteria. However, there is an increasing amount of evidence about the anticancer effects of LL-37. To date, the poor bioavailability, high production cost, and potential cytotoxicity have effectively limited the therapeutic use of LL-37.
Although a large number of studies have shown that the anticancer effects of LL-37 have potential applications in novel cancer treatment strategies, there remain some major challenges that need to be overcome. Particularly, as described in this review, the sensitivity of LL-37 varies among different cancer types. For instance, in colon cancer, glioblastoma, hematologic malignancy, gastric cancer, and oral squamous cell carcinoma, LL-37 can suppress proliferation and induce autophagy as well as apoptotic cell death via both non-membrane-based and membrane-based mechanisms. However, in other types of cancer, such as lung cancer, breast cancer, ovarian cancer, melanoma, prostate cancer, liver cancer, and skin squamous cell carcinoma, it can promote proliferation, migration, and tumorigenesis. To date, there is still no conclusive proof to explain the opposite effects of LL-37 on various cancer types. Furthermore, its selectivity and toxicity are complex. It will be very important to consider the different strategies to enhance both delivery and selectivity of LL-37 for cancer cells.
As a milestone, a phase 1 clinical trial (NCT02225366) with intra-tumoral injections of LL-37 for melanoma patients with cutaneous metastases has been completed and shown significant potency against cancer. We anticipate that research interest in the therapeutic potential of LL-37 will continue to expand, and there will be new discoveries in the near future. These achievements will reignite the hope to develop this important peptide into a novel anticancer drug suitable for clinical use.
Author contributions
Conceptualization: ZL and FL. Data curation: FL and YZ. Formal analysis: YZ. Investigation: FL and YZ. Methodology: ZL and FL. Project administration: FL and YZ. Resources: ZL. Software: FL and YZ. Supervision: ZL. Validation: ZL. Visualization: FL, YZ, and GZ. Writing—original draft: FL and YZ. Writing—review editing: ZL. ZL proposed the concept. FL and YZ wrote the main manuscript text. GZ prepared figures 1–5. All authors reviewed the manuscript.
Conflict of interest
The authors declare that the research was conducted in the absence of any commercial or financial relationships that could be construed as a potential conflict of interest.
The handling editor ZH declared a shared parent affiliation with the authors at the time of review.
Publisher’s note
All claims expressed in this article are solely those of the authors and do not necessarily represent those of their affiliated organizations, or those of the publisher, the editors, and the reviewers. Any product that may be evaluated in this article, or claim that may be made by its manufacturer, is not guaranteed or endorsed by the publisher.
References
Aarbiou, J., Tjabringa, G. S., Verhoosel, R. M., Ninaber, D. K., White, S. R., Peltenburg, L. T., et al. (2006). Mechanisms of cell death induced by the neutrophil antimicrobial peptides alpha-defensins and LL-37. Inflamm. Res. 55 (3), 119–127. doi:10.1007/s00011-005-0062-9
Açil, Y., Torz, K., Gülses, A., Wieker, H., Gerle, M., Purcz, N., et al. (2018). An experimental study on antitumoral effects of KI-21-3, a synthetic fragment of antimicrobial peptide LL-37, on oral squamous cell carcinoma. J. Craniomaxillofac. Surg. 46 (9), 1586–1592. doi:10.1016/j.jcms.2018.05.048
Agerberth, B., Gunne, H., Odeberg, J., Kogner, P., Boman, H. G., and Gudmundsson, G. H. (1995). FALL-39, a putative human peptide antibiotic, is cysteine-free and expressed in bone marrow and testis. Proc. Natl. Acad. Sci. U. S. A. 92 (1), 195–199. doi:10.1073/pnas.92.1.195
Akbarian, A., Ebtekar, M., Pakravan, N., and Hassan, Z. M. (2020). Folate receptor alpha targeted delivery of artemether to breast cancer cells with folate-decorated human serum albumin nanoparticles. Int. J. Biol. Macromol. 152, 90–101. doi:10.1016/j.ijbiomac.2020.02.106
Akkın, S., Varan, G., and Bilensoy, E. (2021). A review on cancer immunotherapy and applications of nanotechnology to chemoimmunotherapy of different cancers. Molecules 26 (11), 3382. doi:10.3390/molecules26113382
Alvares, D. S., Wilke, N., Ruggiero Neto, J., and Fanani, M. L. (2017). The insertion of Polybia-MP1 peptide into phospholipid monolayers is regulated by its anionic nature and phase state. Chem. Phys. Lipids 207, 38–48. doi:10.1016/j.chemphyslip.2017.08.001
An, L. L., Yang, Y. H., Ma, X. T., Lin, Y. M., Li, G., Song, Y. H., et al. (2005). LL-37 enhances adaptive antitumor immune response in a murine model when genetically fused with M-CSFR (J6-1) DNA vaccine. Leuk. Res. 29 (5), 535–543. doi:10.1016/j.leukres.2004.11.009
Armogida, S. A., Yannaras, N. M., Melton, A. L., and Srivastava, M. D. (2004). Identification and quantification of innate immune system mediators in human breast milk. Allergy Asthma Proc. 25 (5), 297–304.
Athira, P. P., Anooja, V. V., Anju, M. V., Neelima, S., Archana, K., Muhammed Musthafa, S., et al. (2022). A hepatic antimicrobial peptide, hepcidin from Indian major carp, catla catla: Molecular identification and functional characterization. J. Genet. Eng. Biotechnol. 20 (1), 49. doi:10.1186/s43141-022-00330-7
Babikir, I. H., Abugroun, E. A., Bilal, N. E., Alghasham, A. A., Abdalla, E. E., and Adam, I. (2018). The impact of cathelicidin, the human antimicrobial peptide LL-37 in urinary tract infections. BMC Infect. Dis. 18 (1), 17. doi:10.1186/s12879-017-2901-z
Bankell, E., Dahl, S., Gidlöf, O., Svensson, D., and Nilsson, B. O. (2021a). LL-37-induced caspase-independent apoptosis is associated with plasma membrane permeabilization in human osteoblast-like cells. Peptides 135, 170432. doi:10.1016/j.peptides.2020.170432
Bankell, E., Liu, X., Lundqvist, M., Svensson, D., Swärd, K., Sparr, E., et al. (2021b). The antimicrobial peptide LL-37 triggers release of apoptosis-inducing factor and shows direct effects on mitochondria. Biochem. Biophys. Rep. 29, 101192. doi:10.1016/j.bbrep.2021.101192
Bartek, J., and Lukas, J. (2001). Pathways governing G1/S transition and their response to DNA damage. FEBS Lett. 490 (3), 117–122. doi:10.1016/s0014-5793(01)02114-7
Berkestedt, I., Herwald, H., Ljunggren, L., Nelson, A., and Bodelsson, M. (2010). Elevated plasma levels of antimicrobial polypeptides in patients with severe sepsis. J. Innate Immun. 2 (5), 478–482. doi:10.1159/000317036
Brockhausen, I., and Melamed, J. (2021). Mucins as anti-cancer targets: Perspectives of the glycobiologist. Glycoconj. J. 38 (4), 459–474. doi:10.1007/s10719-021-09986-8
Bruns, H., Büttner, M., Fabri, M., Mougiakakos, D., Bittenbring, J. T., Hoffmann, M. H., et al. (2015). Vitamin D-dependent induction of cathelicidin in human macrophages results in cytotoxicity against high-grade B cell lymphoma. Sci. Transl. Med. 7 (282), 282ra47. doi:10.1126/scitranslmed.aaa3230
Büchau, A. S., Morizane, S., Trowbridge, J., Schauber, J., Kotol, P., Bui, J. D., et al. (2010). The host defense peptide cathelicidin is required for NK cell-mediated suppression of tumor growth. J. Immunol. 184 (1), 369–378. doi:10.4049/jimmunol.0902110
Bucki, R., Leszczyńska, K., Namiot, A., and Sokołowski, W. (2010). Cathelicidin LL-37: A multitask antimicrobial peptide. Arch. Immunol. Ther. Exp. 58 (1), 15–25. doi:10.1007/s00005-009-0057-2
Calianese, D. C., and Birge, R. B. (2020). Biology of phosphatidylserine (PS): Basic physiology and implications in immunology, infectious disease, and cancer. Cell Commun. Signal. 18 (1), 41. doi:10.1186/s12964-020-00543-8
Capozzi, E., Aureli, S., Minicozzi, V., Rossi, G. C., Stellato, F., and Morante, S. (2018). Designing effective anticancer-radiopeptides. A Molecular Dynamics study of their interaction with model tumor and healthy cell membranes. Biochim. Biophys. Acta. Biomembr. 1860 (11), 2348–2355. doi:10.1016/j.bbamem.2018.05.021
Cha, H. R., Lee, J. H., Hensel, J. A., Sawant, A. B., Davis, B. H., Lee, C. M., et al. (2016). Prostate cancer-derived cathelicidin-related antimicrobial peptide facilitates macrophage differentiation and polarization of immature myeloid progenitors to protumorigenic macrophages. Prostate 76 (7), 624–636. doi:10.1002/pros.23155
Chen, K., Gong, W., Huang, J., Yoshimura, T., and Wang, J. M. (2021). The potentials of short fragments of human anti-microbial peptide LL-37 as a novel therapeutic modality for diseases. Front. Biosci. 26 (11), 1362–1372. doi:10.52586/5029
Chen, P. H., and Fang, S. Y. (2004). The expression of human antimicrobial peptide LL-37 in the human nasal mucosa. Am. J. Rhinol. 18 (6), 381–385. doi:10.1177/194589240401800608
Chen, X., Ji, S., Si, J., Zhang, X., Wang, X., Guo, Y., et al. (2020). Human cathelicidin antimicrobial peptide suppresses proliferation, migration and invasion of oral carcinoma HSC-3 cells via a novel mechanism involving caspase-3 mediated apoptosis. Mol. Med. Rep. 22 (6), 5243–5250. doi:10.3892/mmr.2020.11629
Chen, X., Zou, X., Qi, G., Tang, Y., Guo, Y., Si, J., et al. (2018). Roles and mechanisms of human cathelicidin LL-37 in cancer. Cell. Physiol. biochem. 47 (3), 1060–1073. doi:10.1159/000490183
Cheng, M. H., Pan, C. Y., Chen, N. F., Yang, S. N., Hsieh, S., Wen, Z. H., et al. (2020). Piscidin-1 induces apoptosis via mitochondrial reactive oxygen species-regulated mitochondrial dysfunction in human osteosarcoma cells. Sci. Rep. 10 (1), 5045. doi:10.1038/s41598-020-61876-5
Chernov, A. N., Tsapieva, A., Alaverdian, D. A., Filatenkova, T. A., Galimova, E. S., Suvorova, M., et al. (2022). In vitro evaluation of the cytotoxic effect of Streptococcus pyogenes strains, protegrin PG-1, cathelicidin LL-37, nerve growth factor and chemotherapy on the C6 glioma cell line. Molecules 27 (2), 569. doi:10.3390/molecules27020569
Chiangjong, W., Chutipongtanate, S., and Hongeng, S. (2020). Anticancer peptide: Physicochemical property, functional aspect and trend in clinical application (Review). Int. J. Oncol. 57 (3), 678–696. doi:10.3892/ijo.2020.5099
Chuang, C. M., Monie, A., Wu, A., Mao, C. P., and Hung, C. F. (2009). Treatment with LL-37 peptide enhances antitumor effects induced by CpG oligodeoxynucleotides against ovarian cancer. Hum. Gene Ther. 20 (4), 303–313. doi:10.1089/hum.2008.124
Coffelt, S. B., Marini, F. C., Watson, K., Zwezdaryk, K. J., Dembinski, J. L., LaMarca, H. L., et al. (2009). The pro-inflammatory peptide LL-37 promotes ovarian tumor progression through recruitment of multipotent mesenchymal stromal cells. Proc. Natl. Acad. Sci. U. S. A. 106 (10), 3806–3811. doi:10.1073/pnas.0900244106
Cowland, J. B., Johnsen, A. H., and Borregaard, N. (1995). hCAP-18, a cathelin/pro-bactenecin-like protein of human neutrophil specific granules. FEBS Lett. 368 (1), 173–176. doi:10.1016/0014-5793(95)00634-l
DeLong, R. K., Comer, J., Mathew, E. N., and Jaberi-Douraki, M. (2019). Comparative molecular immunological activity of physiological metal oxide nanoparticle and its anticancer peptide and RNA complexes. Nanomater. (Basel) 9 (12), 1670. doi:10.3390/nano9121670
Dennison, S. R., Whittaker, M., Harris, F., and Phoenix, D. A. (2006). Anticancer alpha-helical peptides and structure/function relationships underpinning their interactions with tumour cell membranes. Curr. Protein Pept. Sci. 7 (6), 487–499. doi:10.2174/138920306779025611
Deslouches, B., and Di, Y. P. (2017). Antimicrobial peptides with selective antitumor mechanisms: Prospect for anticancer applications. Oncotarget 8 (28), 46635–46651. doi:10.18632/oncotarget.16743
Ding, B., Soblosky, L., Nguyen, K., Geng, J., Yu, X., Ramamoorthy, A., et al. (2013). Physiologically-relevant modes of membrane interactions by the human antimicrobial peptide, LL-37, revealed by SFG experiments. Sci. Rep. 3, 1854. doi:10.1038/srep01854
Ding, X., Bian, D., Li, W., Xie, Y., Li, X., Lv, J., et al. (2021). Host defense peptide LL-37 is involved in the regulation of cell proliferation and production of pro-inflammatory cytokines in hepatocellular carcinoma cells. Amino Acids 53 (3), 471–484. doi:10.1007/s00726-021-02966-0
Doktorova, M., Symons, J. L., and Levental, I. (2020). Structural and functional consequences of reversible lipid asymmetry in living membranes. Nat. Chem. Biol. 16 (12), 1321–1330. doi:10.1038/s41589-020-00688-0
Dolkar, T., Trinidad, C. M., Nelson, K. C., Amaria, R. N., Nagarajan, P., Torres-Cabala, C. A., et al. (2018). Dermatologic toxicity from novel therapy using antimicrobial peptide LL-37 in melanoma: A detailed examination of the clinicopathologic features. J. Cutan. Pathol. 45 (7), 539–544. doi:10.1111/cup.13262
Dürr, U. H., Sudheendra, U. S., and Ramamoorthy, A. (2006). LL-37, the only human member of the cathelicidin family of antimicrobial peptides. Biochim. Biophys. Acta 1758 (9), 1408–1425. doi:10.1016/j.bbamem.2006.03.030
Elssner, A., Duncan, M., Gavrilin, M., and Wewers, M. D. (2004). A novel P2X7 receptor activator, the human cathelicidin-derived peptide LL37, induces IL-1 beta processing and release. J. Immunol. 172 (8), 4987–4994. doi:10.4049/jimmunol.172.8.4987
Fábián, T. K., Hermann, P., Beck, A., Fejérdy, P., and Fábián, G. (2012). Salivary defense proteins: Their network and role in innate and acquired oral immunity. Int. J. Mol. Sci. 13 (4), 4295–4320. doi:10.3390/ijms13044295
Fahy, R. J., and Wewers, M. D. (2005). Pulmonary defense and the human cathelicidin hCAP-18/LL-37. Immunol. Res. 31 (2), 75–89. doi:10.1385/IR:31:2:075
Falcao, C. B., Pérez-Peinado, C., de la Torre, B. G., Mayol, X., Zamora-Carreras, H., Jiménez, M. Á., et al. (2015). Structural dissection of crotalicidin, a rattlesnake venom cathelicidin, retrieves a fragment with antimicrobial and antitumor activity. J. Med. Chem. 58 (21), 8553–8563. doi:10.1021/acs.jmedchem.5b01142
Fan, R., Tong, A., Li, X., Gao, X., Mei, L., Zhou, L., et al. (2015). Enhanced antitumor effects by docetaxel/LL37-loaded thermosensitive hydrogel nanoparticles in peritoneal carcinomatosis of colorectal cancer. Int. J. Nanomedicine 10, 7291–7305. doi:10.2147/IJN.S89066
Farabaugh, S. M., Chan, B. T., Cui, X., Dearth, R. K., and Lee, A. V. (2016). Lack of interaction between ErbB2 and insulin receptor substrate signaling in breast cancer. Cell Commun. Signal. 14 (1), 25. doi:10.1186/s12964-016-0148-8
Fillion, M., Valois-Paillard, G., Lorin, A., Noël, M., Voyer, N., and Auger, M. (2015). Membrane interactions of synthetic peptides with antimicrobial potential: Effect of electrostatic interactions and amphiphilicity. Probiotics Antimicrob. Proteins 7 (1), 66–74. doi:10.1007/s12602-014-9177-z
Frohm, M., Agerberth, B., Ahangari, G., Stâhle-Bäckdahl, M., Lidén, S., Wigzell, H., et al. (1997). The expression of the gene coding for the antibacterial peptide LL-37 is induced in human keratinocytes during inflammatory disorders. J. Biol. Chem. 272 (24), 15258–15263. doi:10.1074/jbc.272.24.15258
Gudmundsson, G. H., Agerberth, B., Odeberg, J., Bergman, T., Olsson, B., and Salcedo, R. (1996). The human gene FALL39 and processing of the cathelin precursor to the antibacterial peptide LL-37 in granulocytes. Eur. J. Biochem. 238 (2), 325–332. doi:10.1111/j.1432-1033.1996.0325z.x
Habes, C., Weber, G., and Goupille, C. (2019). Sulfated glycoaminoglycans and proteoglycan syndecan-4 are involved in membrane fixation of LL-37 and its pro-migratory effect in breast cancer cells. Biomolecules 9 (9), 481. doi:10.3390/biom9090481
Han, J., Flemington, C., Houghton, A. B., Gu, Z., Zambetti, G. P., Lutz, R. J., et al. (2001). Expression of Bbc3, a pro-apoptotic BH3-only gene, is regulated by diverse cell death and survival signals. Proc. Natl. Acad. Sci. U. S. A. 98, 11318–11323. doi:10.1073/pnas.201208798
Hassan, N., Greve, B., Espinoza-Sánchez, N. A., and Götte, M. (2021). Cell-surface heparan sulfate proteoglycans as multifunctional integrators of signaling in cancer. Cell. Signal. 77, 109822. doi:10.1016/j.cellsig.2020.109822
Hayashi, M., Kuroda, K., Ihara, K., Iwaya, T., and Isogai, E. (2018). Suppressive effect of an analog of the antimicrobial peptide of LL-37 on colon cancer cells via exosome-encapsulated miRNAs. Int. J. Mol. Med. 42 (6), 3009–3016. doi:10.3892/ijmm.2018.3875
Hilchie, A. L., Hoskin, D. W., and Power Coombs, M. R. (2019). Anticancer activities of natural and synthetic peptides. Adv. Exp. Med. Biol. 1117, 131–147. doi:10.1007/978-981-13-3588-4_9
Hoskin, D. W., and Ramamoorthy, A. (2008). Studies on anticancer activities of antimicrobial peptides. Biochim. Biophys. Acta 1778 (2), 357–375. doi:10.1016/j.bbamem.2007.11.008
Huang, Y., Feng, Q., Yan, Q., Hao, X., and Chen, Y. (2015). Alpha-helical cationic anticancer peptides: A promising candidate for novel anticancer drugs. Mini Rev. Med. Chem. 15 (1), 73–81. doi:10.2174/1389557514666141107120954
Hugonnet, M., Singh, P., Haas, Q., and von Gunten, S. (2021). The distinct roles of sialyltransferases in cancer biology and onco-immunology. Front. Immunol. 12, 799861. doi:10.3389/fimmu.2021.799861
Hurtado, P., and Peh, C. A. (2010). LL-37 promotes rapid sensing of CpG oligodeoxynucleotides by B lymphocytes and plasmacytoid dendritic cells. J. Immunol. 184 (3), 1425–1435. doi:10.4049/jimmunol.0902305
Jafari, A., Babajani, A., Sarrami Forooshani, R., Yazdani, M., and Rezaei-Tavirani, M. (2022). Clinical applications and anticancer effects of antimicrobial peptides: From bench to bedside. Front. Oncol. 12, 819563. doi:10.3389/fonc.2022.819563
Jeffers, J. R., Parganas, E., Lee, Y., Yang, C., Wang, J., Brennan, J., et al. (2003). Puma is an essential mediator of P53-dependent and-independent apoptotic pathways. Cancer Cell 4, 321–328. doi:10.1016/s1535-6108(03)00244-7
Ji, P., Zhou, Y., Yang, Y., Wu, J., Zhou, H., Quan, W., et al. (2019). Myeloid cell-derived LL-37 promotes lung cancer growth by activating Wnt/β-catenin signaling. Theranostics 9 (8), 2209–2223. doi:10.7150/thno.30726
Jiang, Y., Liao, H., Zhang, X., Cao, S., Hu, X., Yang, Z., et al. (2020). IL-33 synergistically promotes the proliferation of lung cancer cells in vitro by inducing antibacterial peptide LL-37 and proinflammatory cytokines in macrophages. Immunobiology 225 (6), 152025. doi:10.1016/j.imbio.2020.152025
Johansson, J., Gudmundsson, G. H., Rottenberg, M. E., Berndt, K. D., and Agerberth, B. (1998). Conformation-dependent antibacterial activity of the naturally occurring human peptide LL-37. J. Biol. Chem. 273 (6), 3718–3724. doi:10.1074/jbc.273.6.3718
Juba, M. L., Porter, D. K., Williams, E. H., Rodriguez, C. A., Barksdale, S. M., and Bishop, B. M. (2015). Helical cationic antimicrobial peptide length and its impact on membrane disruption. Biochim. Biophys. Acta 1848 (5), 1081–1091. doi:10.1016/j.bbamem.2015.01.007
Kiatsurayanon, C., Peng, G., and Niyonsaba, F. (2022). Opposing roles of antimicrobial peptides in skin cancers. Curr. Pharm. Des. 28 (3), 248–258. doi:10.2174/1381612827666211021163318
Kim, S., Hyun, S., Lee, Y., Lee, Y., and Yu, J. (2016). Nonhemolytic cell-penetrating peptides: Site specific introduction of glutamine and lysine residues into the α-helical peptide causes deletion of its direct membrane disrupting ability but retention of its cell penetrating ability. Biomacromolecules 17 (9), 3007–3015. doi:10.1021/acs.biomac.6b00874
Kube, S., Hersch, N., Naumovska, E., Gensch, T., Hendriks, J., Franzen, A., et al. (2017). Fusogenic liposomes as nanocarriers for the delivery of intracellular proteins. Langmuir 33 (4), 1051–1059. doi:10.1021/acs.langmuir.6b04304
Kumar, P., Kizhakkedathu, J. N., and Straus, S. K. (2018). Antimicrobial peptides: Diversity, mechanism of action and strategies to improve the activity and biocompatibility in vivo. Biomolecules 8 (1), 4. doi:10.3390/biom8010004
Kuroda, K., Fukuda, T., Isogai, H., Okumura, K., Krstic-Demonacos, M., and Isogai, E. (2015b). Antimicrobial peptide FF/CAP18 induces apoptotic cell death in HCT116 colon cancer cells via changes in the metabolic profile. Int. J. Oncol. 46 (4), 1516–1526. doi:10.3892/ijo.2015.2887
Kuroda, K., Fukuda, T., Krstic-Demonacos, M., Demonacos, C., Okumura, K., Isogai, H., et al. (2017). miR-663a regulates growth of colon cancer cells, after administration of antimicrobial peptides, by targeting CXCR4-p21 pathway. BMC Cancer 17 (1), 33. doi:10.1186/s12885-016-3003-9
Kuroda, K., Fukuda, T., Yoneyama, H., Katayama, M., Isogai, H., Okumura, K., et al. (2012). Anti-proliferative effect of an analogue of the LL-37 peptide in the colon cancer derived cell line HCT116 p53+/+ and p53-/-. Oncol. Rep. 28 (3), 829–834. doi:10.3892/or.2012.1876
Kuroda, K., Okumura, K., Isogai, H., and Isogai, E. (2015a). The human cathelicidin antimicrobial peptide LL-37 and mimics are potential anticancer drugs. Front. Oncol. 5, 144. doi:10.3389/fonc.2015.00144
Lee, R. H., Oh, J. D., Hwang, J. S., Lee, H. K., and Shin, D. (2021). Antitumorigenic effect of insect-derived peptide poecilocorisin-1 in human skin cancer cells through regulation of Sp1 transcription factor. Sci. Rep. 11 (1), 18445. doi:10.1038/s41598-021-97581-0
Lee, T. H., Hall, K. N., and Aguilar, M. I. (2016). Antimicrobial peptide structure and mechanism of action: A focus on the role of membrane structure. Curr. Top. Med. Chem. 16 (1), 25–39. doi:10.2174/1568026615666150703121700
Li, X., Li, Y., Han, H., Miller, D. W., and Wang, G. (2006). Solution structures of human LL-37 fragments and NMR-based identification of a minimal membrane-targeting antimicrobial and anticancer region. J. Am. Chem. Soc. 128 (17), 5776–5785. doi:10.1021/ja0584875
Liu, Z., Yuan, X., Liu, M., Fernandes, G., Zhang, Y., Yang, S., et al. (2018). Antimicrobial peptide combined with BMP2-modified mesenchymal stem cells promotes calvarial repair in an osteolytic model. Mol. Ther. 26 (1), 199–207. doi:10.1016/j.ymthe.2017.09.011
Ma, R., Wong, S. W., Ge, L., Shaw, C., Siu, S. W. I., and Kwok, H. F. (2019). In vitro and MD simulation study to explore physicochemical parameters for antibacterial peptide to become potent anticancer peptide. Mol. Ther. Oncolytics 16, 7–19. doi:10.1016/j.omto.2019.12.001
Mader, J. S., Ewen, C., Hancock, R. E., and Bleackley, R. C. (2011b). The human cathelicidin, LL-37, induces granzyme-mediated apoptosis in regulatory T cells. J. Immunother. 34 (3), 229–235. doi:10.1097/CJI.0b013e318207ecdf
Mader, J. S., Marcet-Palacios, M., Hancock, R. E., and Bleackley, R. C. (2011a). The human cathelicidin, LL-37, induces granzyme-mediated apoptosis in cytotoxic T lymphocytes. Exp. Cell Res. 317 (4), 531–538. doi:10.1016/j.yexcr.2010.11.015
Mader, J. S., Mookherjee, N., Hancock, R. E., and Bleackley, R. C. (2009). The human host defense peptide LL-37 induces apoptosis in a calpain- and apoptosis-inducing factor-dependent manner involving Bax activity. Mol. Cancer Res. 7 (5), 689–702. doi:10.1158/1541-7786.MCR-08-0274
Malam, Y., Loizidou, M., and Seifalian, A. M. (2009). Liposomes and nanoparticles: Nanosized vehicles for drug delivery in cancer. Trends Pharmacol. Sci. 30 (11), 592–599. doi:10.1016/j.tips.2009.08.004
Malm, J., Sørensen, O., Persson, T., Frohm-Nilsson, M., Johansson, B., Bjartell, A., et al. (2000). The human cationic antimicrobial protein (hCAP-18) is expressed in the epithelium of human epididymis, is present in seminal plasma at high concentrations, and is attached to spermatozoa. Infect. Immun. 68 (7), 4297–4302. doi:10.1128/iai.68.7.4297-4302.2000
Marverti, G., Gozzi, G., Maretti, E., Lauriola, A., Severi, L., Sacchetti, F., et al. (2020). A peptidic thymidylate-synthase inhibitor loaded on pegylated liposomes enhances the antitumour effect of chemotherapy drugs in human ovarian cancer cells. Int. J. Mol. Sci. 21 (12), 4452. doi:10.3390/ijms21124452
Mitchell, G., Silvis, M. R., Talkington, K. C., Budzik, J. M., Dodd, C. E., Paluba, J. M., et al. (2022). Ceragenins and antimicrobial peptides kill bacteria through distinct mechanisms. mBio 13 (1), e0272621. doi:10.1128/mbio.02726-21
Monteiro, L. O. F., Fernandes, R. S., Oda, C. M. R., Lopes, S. C., Townsend, D. M., Cardoso, V. N., et al. (2018). Paclitaxel-loaded folate-coated long circulating and pH-sensitive liposomes as a potential drug delivery system: A biodistribution study. Biomed. Pharmacother. 97, 489–495. doi:10.1016/j.biopha.2017.10.135
Mori, T., Hazekawa, M., Yoshida, M., Nishinakagawa, T., Uchida, T., and Ishibashi, D. (2021). Enhancing the anticancer efficacy of a LL-37 peptide fragment analog using peptide-linked PLGA conjugate micelles in tumor cells. Int. J. Pharm. 606, 120891. doi:10.1016/j.ijpharm.2021.120891
Muñoz, M., Craske, M., Severino, P., de Lima, T. M., Labhart, P., Chammas, R., et al. (2016). Antimicrobial peptide LL-37 participates in the transcriptional regulation of melanoma cells. J. Cancer 7 (15), 2341–2345. doi:10.7150/jca.16947
Murakami, M., Ohtake, T., Dorschner, R. A., Schittek, B., Garbe, C., and Gallo, R. L. (2002). Cathelicidin anti-microbial peptide expression in sweat, an innate defense system for the skin. J. Invest. Dermatol. 119 (5), 1090–1095. doi:10.1046/j.1523-1747.2002.19507.x
Niemirowicz, K., Durnaś, B., Tokajuk, G., Piktel, E., Michalak, G., Gu, X., et al. (2017). Formulation and candidacidal activity of magnetic nanoparticles coated with cathelicidin LL-37 and ceragenin CSA-13. Sci. Rep. 7 (1), 4610. doi:10.1038/s41598-017-04653-1
Niemirowicz, K., Prokop, I., Wilczewska, A. Z., Wnorowska, U., Piktel, E., Wątek, M., et al. (2015). Magnetic nanoparticles enhance the anticancer activity of cathelicidin LL-37 peptide against colon cancer cells. Int. J. Nanomedicine 10, 3843–3853. doi:10.2147/IJN.S76104
Okumura, K., Itoh, A., Isogai, E., Hirose, K., Hosokawa, Y., Abiko, Y., et al. (2004). C-terminal domain of human CAP18 antimicrobial peptide induces apoptosis in oral squamous cell carcinoma SAS-H1 cells. Cancer Lett. 212 (2), 185–194. doi:10.1016/j.canlet.2004.04.006
Oren, Z., Lerman, J. C., Gudmundsson, G. H., Agerberth, B., and Shai, Y. (1999). Structure and organization of the human antimicrobial peptide LL-37 in phospholipid membranes: Relevance to the molecular basis for its non-cell-selective activity. Biochem. J. 341 (3), 501–513. doi:10.1042/bj3410501
Patil, S. M., and Kunda, N. K. (2022). Nisin ZP, an antimicrobial peptide, induces cell death and inhibits non-small cell lung cancer (NSCLC) progression in vitro in 2D and 3D cell culture. Pharm. Res. doi:10.1007/s11095-022-03220-2
Pazgier, M., Ericksen, B., Ling, M., Toth, E., Shi, J., Li, X., et al. (2013). Structural and functional analysis of the pro-domain of human cathelicidin, LL-37. Biochemistry 52 (9), 1547–1558. doi:10.1021/bi301008r
Pfosser, A., El-Aouni, C., Pfisterer, I., Dietz, M., Globisch, F., Stachel, G., et al. (2010). NF kappaB activation in embryonic endothelial progenitor cells enhances neovascularization via PSGL-1 mediated recruitment: Novel role for LL37. Stem Cells 28 (2), 376–385. doi:10.1002/stem.280
Piktel, E., Niemirowicz, K., Wnorowska, U., Wątek, M., Wollny, T., Głuszek, K., et al. (2016). The role of cathelicidin LL-37 in cancer development. Arch. Immunol. Ther. Exp. 64 (1), 33–46. doi:10.1007/s00005-015-0359-5
Porcelli, F., Verardi, R., Shi, L., Henzler-Wildman, K. A., Ramamoorthy, A., and Veglia, G. (2008). NMR structure of the cathelicidin-derived human antimicrobial peptide LL-37 in dodecylphosphocholine micelles. Biochemistry 47 (20), 5565–5572. doi:10.1021/bi702036s
Porter, R. J., Murray, G. I., Alnabulsi, A., Humphries, M. P., James, J. A., Salto-Tellez, M., et al. (2021). Colonic epithelial cathelicidin (LL-37) expression intensity is associated with progression of colorectal cancer and presence of CD8+ T cell infiltrate. J. Pathol. Clin. Res. 7 (5), 495–506. doi:10.1002/cjp2.222
Pouny, Y., and Shai, Y. (1992). Interaction of D-amino acid incorporated analogues of pardaxin with membranes. Biochemistry 31 (39), 9482–9490. doi:10.1021/bi00154a022
Prevete, N., Liotti, F., Visciano, C., Marone, G., Melillo, R. M., de Paulis, A., et al. (2015). The formyl peptide receptor 1 exerts a tumor suppressor function in human gastric cancer by inhibiting angiogenesis. Oncogene 34 (29), 3826–3838. doi:10.1038/onc.2014.309
Quemé-Peña, M., Juhász, T., Kohut, G., Ricci, M., Singh, P., Szigyártó, I. C., et al. (2021). Membrane association modes of natural anticancer peptides: Mechanistic details on helicity, orientation, and surface coverage. Int. J. Mol. Sci. 22 (16), 8613. doi:10.3390/ijms22168613
Radaic, A., de Jesus, M. B., and Kapila, Y. L. (2020). Bacterial anti-microbial peptides and nano-sized drug delivery systems: The state of the art toward improved bacteriocins. J. Control. Release 321, 100–118. doi:10.1016/j.jconrel.2020.02.001
Rajkumar, S. V., Richardson, P. G., Hideshima, T., and Anderson, K. C. (2005). Proteasome inhibition as a novel therapeutic target in human cancer. J. Clin. Oncol. 23 (3), 630–639. doi:10.1200/JCO.2005.11.030
Ramos, R., Silva, J. P., Rodrigues, A. C., Costa, R., Guardão, L., Schmitt, F., et al. (2011). Wound healing activity of the human antimicrobial peptide LL37. Peptides 32, 1469–1476. doi:10.1016/j.peptides.2011.06.005
Rathnayake, K., Patel, U., Pham, C., McAlpin, A., Budisalich, T., and Jayawardena, S. N. (2020). Targeted delivery of antibiotic therapy to inhibit Pseudomonas aeruginosa using lipid-coated mesoporous silica core-shell nanoassembly. ACS Appl. Bio Mat. 3 (10), 6708–6721. doi:10.1021/acsabm.0c00622
Reißer, S., Prock, S., Heinzmann, H., and Ulrich, A. S. (2018). Protein origami: A program for the creation of 3D paper models of folded peptides. Biochem. Mol. Biol. Educ. 46 (4), 403–409. doi:10.1002/bmb.21132
Ren, S. X., Cheng, A. S., To, K. F., Tong, J. H., Li, M. S., Shen, J., et al. (2012). Host immune defense peptide LL-37 activates caspase-independent apoptosis and suppresses colon cancer. Cancer Res. 72 (24), 6512–6523. doi:10.1158/0008-5472.CAN-12-2359
Ren, S. X., Shen, J., Cheng, A. S., Lu, L., Chan, R. L., Li, Z. J., et al. (2013). FK-16 derived from the anticancer peptide LL-37 induces caspase-independent apoptosis and autophagic cell death in colon cancer cells. PLoS One 8 (5), e63641. doi:10.1371/journal.pone.0063641
Rieg, S., Steffen, H., Seeber, S., Humeny, A., Kalbacher, H., Dietz, K., et al. (2005). Deficiency of dermcidin-derived antimicrobial peptides in sweat of patients with atopic dermatitis correlates with an impaired innate defense of human skin in vivo. J. Immunol. 174 (12), 8003–8010. doi:10.4049/jimmunol.174.12.8003
Roufayel, R., Younes, K., Al-Sabi, A., and Murshid, N. (2022). BH3-Only proteins noxa and puma are key regulators of induced apoptosis. Life (Basel) 12 (2), 256. doi:10.3390/life12020256
Sengupta, P., Banerjee, N., Roychowdhury, T., Dutta, A., Chattopadhyay, S., and Chatterjee, S. (2018). Site-specific amino acid substitution in dodecameric peptides determines the stability and unfolding of c-MYC quadruplex promoting apoptosis in cancer cells. Nucleic Acids Res. 46 (19), 9932–9950. doi:10.1093/nar/gky824
Shai, Y. (2002). Mode of action of membrane active antimicrobial peptides. Biopolymers 66 (4), 236–248. doi:10.1002/bip.10260
Singh, M., Liu, C., Lou, Y., Gregorio, J., Conrad, C., Hwu, P., et al. (2012). Abstract 1580: LL-37- mediates tumor antigen-specific-T cell expansion and tumor regression. Cancer Res. 72, 1580. doi:10.1158/1538-7445.am2012-1580
Sørensen, O. E., Follin, P., Johnsen, A. H., Calafat, J., Tjabringa, G. S., Hiemstra, P. S., et al. (2001). Human cathelicidin, hCAP-18, is processed to the antimicrobial peptide LL-37 by extracellular cleavage with proteinase 3. Blood 97 (12), 3951–3959. doi:10.1182/blood.v97.12.3951
Tjabringa, G. S., Aarbiou, J., Ninaber, D. K., Drijfhout, J. W., Sørensen, O. E., Borregaard, N., et al. (2003). The antimicrobial peptide LL-37 activates innate immunity at the airway epithelial surface by transactivation of the epidermal growth factor receptor. J. Immunol. 171 (12), 6690–6696. doi:10.4049/jimmunol.171.12.6690
Tjabringa, G. S., Rabe, K. F., and Hiemstra, P. S. (2005). The human cathelicidin LL-37: A multifunctional peptide involved in infection and inflammation in the lung. Pulm. Pharmacol. Ther. 18 (5), 321–327. doi:10.1016/j.pupt.2005.01.001
Tornesello, A. L., Borrelli, A., Buonaguro, L., Buonaguro, F. M., and Tornesello, M. L. (2020). Antimicrobial peptides as anticancer agents: Functional properties and biological activities. Molecules 25 (12), 2850. doi:10.3390/molecules25122850
Tossi, A., Scocchi, M., Skerlavaj, B., and Gennaro, R. (1994). Identification and characterization of a primary antibacterial domain in CAP18, a lipopolysaccharide binding protein from rabbit leukocytes. FEBS Lett. 339 (1-2), 108–112. doi:10.1016/0014-5793(94)80395-1
Trinidad-Calderón, P. A., Varela-Chinchilla, C. D., and García-Lara, S. (2021). Natural peptides inducing cancer cell death: Mechanisms and properties of specific candidates for cancer therapeutics. Molecules 26 (24), 7453. doi:10.3390/molecules26247453
Tsikourkitoudi, V., Karlsson, J., Merkl, P., Loh, E., Henriques-Normark, B., and Sotiriou, G. A. (2020). Flame-made calcium phosphate nanoparticles with high drug loading for delivery of biologics. Molecules 25 (7), 1747. doi:10.3390/molecules25071747
Tzitzilis, A., Boura-Theodorou, A., Michail, V., Papadopoulos, S., Krikorian, D., Lekka, M. E., et al. (2020). Cationic amphipathic peptide analogs of cathelicidin LL-37 as a probe in the development of antimicrobial/anticancer agents. J. Pept. Sci. 26 (7), e3254. doi:10.1002/psc.3254
Utsugi, T., Schroit, A. J., Connor, J., Bucana, C. D., and Fidler, I. J. (1991). Elevated expression of phosphatidylserine in the outer membrane leaflet of human tumor cells and recognition by activated human blood monocytes. Cancer Res. 51 (11), 3062–3066.
Vandamme, D., Landuyt, B., Luyten, W., and Schoofs, L. (2012). A comprehensive summary of LL-37, the factotum human cathelicidin peptide. Cell. Immunol. 280 (1), 22–35. doi:10.1016/j.cellimm.2012.11.009
Varga, A. C., and Wrana, J. L. (2005). The disparate role of BMP in stem cell biology. Oncogene 24 (37), 5713–5721. doi:10.1038/sj.onc.1208919
Vasquez-Montes, V., Gerhart, J., Thévenin, D., and Ladokhin, A. S. (2019). Divalent cations and lipid composition modulate membrane insertion and cancer-targeting action of pHLIP. J. Mol. Biol. 431 (24), 5004–5018. doi:10.1016/j.jmb.2019.10.016
Vitale, I., Yamazaki, T., Wennerberg, E., Sveinbjørnsson, B., Rekdal, Ø., Demaria, S., et al. (2021). Targeting cancer heterogeneity with immune responses driven by oncolytic peptides. Trends Cancer 7 (6), 557–572. doi:10.1016/j.trecan.2020.12.012
Vlieghe, P., Lisowski, V., Martinez, J., and Khrestchatisky, M. (2010). Synthetic therapeutic peptides: Science and market. Drug Discov. Today 15 (1-2), 40–56. doi:10.1016/j.drudis.2009.10.009
Wang, G. (2014). Human antimicrobial peptides and proteins. Pharm. (Basel) 7 (5), 545–594. doi:10.3390/ph7050545
Wang, G. (2015). Improved methods for classification, prediction, and design of antimicrobial peptides. Methods Mol. Biol. 1268, 43–66. doi:10.1007/978-1-4939-2285-7_3
Wang, G., Mishra, B., Epand, R. F., and Epand, R. M. (2014). High-quality 3D structures shine light on antibacterial, anti-biofilm and antiviral activities of human cathelicidin LL-37 and its fragments. Biochim. Biophys. Acta 1838 (9), 2160–2172. doi:10.1016/j.bbamem.2014.01.016
Wang, G., Narayana, J. L., Mishra, B., Zhang, Y., Wang, F., Wang, C., et al. (2019). Design of antimicrobial peptides: Progress made with human cathelicidin LL-37. Adv. Exp. Med. Biol. 1117, 215–240. doi:10.1007/978-981-13-3588-4_12
Wang, G. (2008). Structures of human host defense cathelicidin LL-37 and its smallest antimicrobial peptide KR-12 in lipid micelles. J. Biol. Chem. 283 (47), 32637–32643. doi:10.1074/jbc.M805533200
Wang, W., Zheng, Y., Jia, J., Li, C., Duan, Q., Li, R., et al. (2017). Antimicrobial peptide LL-37 promotes the viability and invasion of skin squamous cell carcinoma by upregulating YB-1. Exp. Ther. Med. 14 (1), 499–506. doi:10.3892/etm.2017.4546
Wang, Y., Agerberth, B., Löthgren, A., Almstedt, A., Johansson, J., and Lothgren, A. (1998). Apolipoprotein A-I binds and inhibits the human antibacterial/cytotoxic peptide LL-37. J. Biol. Chem. 273 (50), 33115–33118. doi:10.1074/jbc.273.50.33115
Warren, L., Buck, C. A., and Tuszynski, G. P. (1979). Alterations of membrane glycoproteins in malignant cells. Dev. Biol. Stand. 42, 177–182.
Wnorowska, U., Fiedoruk, K., Piktel, E., Prasad, S. V., Sulik, M., Janion, M., et al. (2020). Nanoantibiotics containing membrane-active human cathelicidin LL-37 or synthetic ceragenins attached to the surface of magnetic nanoparticles as novel and innovative therapeutic tools: Current status and potential future applications. J. Nanobiotechnology 18 (1), 3. doi:10.1186/s12951-019-0566-z
Wolk, K., Witte, E., Wallace, E., Döcke, W. D., Kunz, S., Asadullah, K., et al. (2006). IL-22 regulates the expression of genes responsible for antimicrobial defense, cellular differentiation, and mobility in keratinocytes: A potential role in psoriasis. Eur. J. Immunol. 36 (5), 1309–1323. doi:10.1002/eji.200535503
Wu, W. K., Cho, C. H., Lee, C. W., Wu, K., Fan, D., Yu, J., et al. (2010c). Proteasome inhibition: A new therapeutic strategy to cancer treatment. Cancer Lett. 293 (1), 15–22. doi:10.1016/j.canlet.2009.12.002
Wu, W. K., Sung, J. J., To, K. F., Yu, L., Li, H. T., Li, Z. J., et al. (2010b). The host defense peptide LL-37 activates the tumor-suppressing bone morphogenetic protein signaling via inhibition of proteasome in gastric cancer cells. J. Cell. Physiol. 223 (1), 178–186. doi:10.1002/jcp.22026
Wu, W. K., Sung, J. J., Wu, Y. C., Li, Z. J., Yu, L., and Cho, C. H. (2008a). Bone morphogenetic protein signalling is required for the anti-mitogenic effect of the proteasome inhibitor MG-132 on colon cancer cells. Br. J. Pharmacol. 154 (3), 632–638. doi:10.1038/bjp.2008.115
Wu, W. K., Sung, J. J., Yu, L., and Cho, C. H. (2008b). Proteasome inhibitor MG-132 lowers gastric adenocarcinoma TMK1 cell proliferation via bone morphogenetic protein signaling. Biochem. Biophys. Res. Commun. 371 (2), 209–214. doi:10.1016/j.bbrc.2008.04.059
Wu, W. K., Wang, G., Coffelt, S. B., Betancourt, A. M., Lee, C. W., Fan, D., et al. (2010a). Emerging roles of the host defense peptide LL-37 in human cancer and its potential therapeutic applications. Int. J. Cancer 127 (8), 1741–1747. doi:10.1002/ijc.25489
Xhindoli, D., Pacor, S., Guida, F., Antcheva, N., and Tossi, A. (2014). Native oligomerization determines the mode of action and biological activities of human cathelicidin LL-37. Biochem. J. 457 (2), 263–275. doi:10.1042/BJ20131048
Yang, B., Good, D., Mosaiab, T., Liu, W., Ni, G., Kaur, J., et al. (2020). Significance of LL-37 on immunomodulation and disease outcome. Biomed. Res. Int. 2020, 8349712. doi:10.1155/2020/8349712
Yang, T., Li, J., Jia, Q., Zhan, S., Zhang, Q., Wang, Y., et al. (2021). Antimicrobial peptide 17BIPHE2 inhibits the proliferation of lung cancer cells in vitro and in vivo by regulating the ERK signaling pathway. Oncol. Lett. 22 (1), 501. doi:10.3892/ol.2021.12762
Yu, J., and Zhang, L. (2003). No PUMA, no death: Implications for p53-dependent apoptosis. Cancer Cell 4 (4), 248–249. doi:10.1016/s1535-6108(03)00249-6
Yu, J., Wang, Z., Kinzler, K. W., Vogelstein, B., and Zhang, L. (2003). PUMA mediates the apoptotic response to P53 in colorectal cancer cells. Proc. Natl. Acad. Sci. U. S. A. 100, 1931–1936. doi:10.1073/pnas.2627984100
Yu, J., Zhang, L., Hwang, P. M., Kinzler, K. W., and Vogelstein, B. (2001). PUMA induces the rapid apoptosis of colorectal cancer cells. Mol. Cell 7, 673–682. doi:10.1016/s1097-2765(01)00213-1
Zachowski, A. (1993). Phospholipids in animal eukaryotic membranes: Transverse asymmetry and movement. Biochem. J. 294 (1), 1–14. doi:10.1042/bj2940001
Zaiou, M., Nizet, V., and Gallo, R. L. (2003). Antimicrobial and protease inhibitory functions of the human cathelicidin (hCAP18/LL-37) prosequence. J. Invest. Dermatol. 120 (5), 810–816. doi:10.1046/j.1523-1747.2003.12132.x
Zanetti, M. (2004). Cathelicidins, multifunctional peptides of the innate immunity. J. Leukoc. Biol. 75 (1), 39–48. doi:10.1189/jlb.0403147
Zanetti, M. (2005). The role of cathelicidins in the innate host defenses of mammals. Curr. Issues Mol. Biol. 7 (2), 179–196.
Zhang, H., Zhen, J., Zhang, R., Wanyan, Y., Liu, K., Yuan, X., et al. (2022). Cathelicidin hCAP18/LL-37 promotes cell proliferation and suppresses antitumor activity of 1, 25(OH)2D3 in hepatocellular carcinoma. Cell Death Discov. 8 (1), 27. doi:10.1038/s41420-022-00816-w
Zhang, N., Yang, Y., Wang, Z., Yang, J., Chu, X., Liu, J., et al. (2019). Polypeptide-engineered DNA tetrahedrons for targeting treatment of colorectal cancer via apoptosis and autophagy. J. Control. Release 309, 48–58. doi:10.1016/j.jconrel.2019.07.012
Zhang, Y., Chen, X., Qiao, M., Zhang, B. Q., Wang, N., Zhang, Z., et al. (2014). Bone morphogenetic protein 2 inhibits the proliferation and growth of human colorectal cancer cells. Oncol. Rep. 32 (3), 1013–1020. doi:10.3892/or.2014.3308
Zhang, Y., Lakshmaiah Narayana, J., Wu, Q., Dang, X., and Wang, G. (2021). Structure and activity of a selective antibiofilm peptide SK-24 derived from the NMR structure of human cathelicidin LL-37. Pharm. (Basel) 14 (12), 1245. doi:10.3390/ph14121245
Zhao, L., Cao, Z., Bian, Y., Hu, G., Wang, J., and Zhou, Y. (2018). Molecular dynamics simulations of human antimicrobial peptide LL-37 in model POPC and POPG lipid bilayers. Int. J. Mol. Sci. 19 (4), 1186. doi:10.3390/ijms19041186
Zielińska, A., Szalata, M., Gorczyński, A., Karczewski, J., Eder, P., Severino, P., et al. (2021). Cancer nanopharmaceuticals: Physicochemical characterization and in vitro/in vivo applications. Cancers (Basel) 13 (8), 1896. doi:10.3390/cancers13081896
Keywords: antimicrobial peptides, cathelicidin (LL37), hCAP18, LL37, cancer, anticancer
Citation: Lu F, Zhu Y, Zhang G and Liu Z (2022) Renovation as innovation: Repurposing human antibacterial peptide LL-37 for cancer therapy. Front. Pharmacol. 13:944147. doi: 10.3389/fphar.2022.944147
Received: 14 May 2022; Accepted: 22 July 2022;
Published: 23 August 2022.
Edited by:
Zhi Li, The First Affiliated Hospital of China Medical University, ChinaCopyright © 2022 Lu, Zhu, Zhang and Liu. This is an open-access article distributed under the terms of the Creative Commons Attribution License (CC BY). The use, distribution or reproduction in other forums is permitted, provided the original author(s) and the copyright owner(s) are credited and that the original publication in this journal is cited, in accordance with accepted academic practice. No use, distribution or reproduction is permitted which does not comply with these terms.
*Correspondence: Zunpeng Liu, enBsaXVAY211LmVkdS5jbg==