- 1Department of Pathology, Key Laboratory of Clinical Molecular Pathology, The First Affiliated Hospital of Xinxiang Medical University, Xinxiang, China
- 2Department of Pathology, Xinxiang Medical University, Xinxiang, China
- 3School of Public Health, Xinxiang Medical University, Xinxiang, China
- 4The Second Affiliated Hospital of Xinxiang Medical University, Xinxiang, China
N6-methyladenosine (m6A) RNA methylation is identified as the most common, abundant and reversible RNA epigenetic modification in messenger RNA (mRNA) and non-coding RNA, especially within eukaryotic messenger RNAs (mRNAs), which post-transcriptionally directs many important processes of RNA. It has also been demonstrated that m6A modification plays a pivotal role in the occurrence and development of tumors by regulating RNA splicing, localization, translation, stabilization and decay. Growing number of studies have indicated that natural products have outstanding anti-cancer effects of their unique advantages of high efficiency and minimal side effects. However, at present, there are very few research articles to study and explore the relationship between natural products and m6A RNA modification in tumorigenesis. m6A is dynamically deposited, removed, and recognized by m6A methyltransferases (METTL3/14, METTL16, WTAP, RBM15/15B, VIRMA, CBLL1, and ZC3H13, called as “writers”), demethylases (FTO and ALKBH5, called as “erasers”), and m6A-specific binding proteins (YTHDF1/2/3, YTHDC1/2, IGH2BP1/2/3, hnRNPs, eIF3, and FMR1, called as “readers”), respectively. In this review, we summarize the biological function of m6A modification, the role of m6A and the related signaling pathway in cancer, such as AKT, NF-kB, MAPK, ERK, Wnt/β-catenin, STAT, p53, Notch signaling pathway, and so on. Furthermore, we reviewed the current research on nature products in anti-tumor, and further to get a better understanding of the anti-tumor mechanism, thus provide an implication for nature products with anti-cancer research by regulating m6A modification in the future.
Introduction
In recent years, N6-methyladenosine (m6A) RNA modification, as a new regulatory mechanism for controlling gene expression in eukaryotes, has attracted more and more attention and gradually become a hot topic in biological research. Reversible epigenetic modification of m6A RNA was found not only in messenger RNA (mRNA) but also in non-coding RNA (ncRNA). m6A modification affects the biological fate of target RNA molecules and plays an important role in almost biological activities, including the occurrence, development and outcome of cancer (Chen et al., 2019a; He et al., 2019; Sun et al., 2019; Wang et al., 2020e; Huang et al., 2020). Among the more than 100 types of post-transcriptional epitranscriptomic modifications identified in RNAs thus far, m6A is the most abundant internal modification of eukaryotic RNAs, including mRNA, tRNA, rRNA, snRNA, microRNA (miRNA), and long non-coding RNA (lncRNA), contributing to RNA splicing, localization, translation, stabilization, and decay (Gokhale et al., 2016; Wang et al., 2018; Koh et al., 2019; Meyer, 2019; Zhang et al., 2019; Gong et al., 2020). In recent years, it has been found that the abnormal modification of m6A RNA is closely related to the occurrence and development of a variety of tumors (Chen et al., 2018; Jin et al., 2019; Shi et al., 2020; Wang et al., 2020a; Zhang et al., 2021a). Therefore, it is necessary to conduct in-depth studies on the relationship between m6A and tumor, especially to harness the key mechanisms involved in m6A modification for anti-cancer research (Niu et al., 2018; Gao et al., 2021).
Natural products are essential to modern medicine, such as curcumin, chrysin, cucurbitacin B, cinnamaldehyde, bruceine D, baicalein, epigallocatechin gallate, fucoidan, resveratrol, rhein, and so on. Many of which have been shown to effectively regulate the progression of cancer and enhance the anticancer effects of various anticancer drugs (Li et al., 2020a; Chen et al., 2020b; Chen et al., 2021b; Zhang et al., 2021b; Wang et al., 2021). In addition, 73 percent of antibiotics, 49 percent of anticancer compounds, and 32 percent of all new drugs approved by the U.S. Food and Drug Administration (FDA) between 1980 and 2012 were natural products or derivatives of them (Newman and Cragg, 2016; Harvey et al., 2018). These natural products, including compounds with antibiotic, antifungal, immunosuppressant, and anticancer activity, are a rich source of anticancer agents and provide new and more effective anticancer agents for therapeutic use. However, there are few reports that natural products inhibit tumor genesis and development by regulating m6A RNA modification (Qian et al., 2021b; Feng et al., 2022; Jiang et al., 2022).
In this review, we summarize the biological functions of m6A modification, the role of m6A in tumors, and the related signal pathways of m6A in tumors, such as Akt, NF-kB, MAPK, ERK, and Wnt/β-Catenin, STAT, p53, Notch signaling pathway, etc. Moreover, we also review the research progress of natural products in antitumor, and further understands its antitumor mechanism, so as to provide an implication for the anti-cancer research of natural products via regulating m6A modification in the future.
Part 1: Overview of m6A RNA Methylation
m6A RNA Methyltransferases—Writers
The RNA m6A modification is deposited by the m6A methyltransferase complex (called as “writer”), which mainly consist of a methyltransferases like 3 and 14 (METTL3 and METTL14) heterodimeric enzymatic core, and other cofactor protein, such as Wilm’s tumor-1-associated protein (WTAP), RNA binding motif protein 15 (RBM15) and its paralogue RBM15B, vir like m6A methyltransferase associated (VIRMA, originally known as KIAA1429, Virilizer), zinc finger CCCH type containing 13 (ZC3H13), and CBLL1 (Patil et al., 2016; Roundtree et al., 2017a; Table 1).
METTL3 and METTL14 are essential components of methyltransferase complex (MTC), in which METTL3 is catalytically active while METTL14 has critical structural scaffold functions, isolated METTL3 without METTL14 is not bioactive (Roundtree et al., 2017a; Hsu et al., 2017). WTAP, as the key METTL3 adaptor, directly interacts with METTL3 and recruits METTL3-METTL14 heterodimeric complex to nuclear speckles for m6A modification (Ping et al., 2014). Meanwhile, WTAP also binds to the m6A consensus RRACH (R = A/G, H = A/C/U) motif of RNA and recruits catalytic subunits METTL3 and METTL14 to the vicinity of the target RNA sequence (Ping et al., 2014). In addition, studies have shown that knockdown of WTAP reduces METTL3 and METTL14, while knockdown of METTL3 or METTL14 does not reduce or even increase WTAP, although a single knockdown of METTL3 or METTL14 reduces each other (Ping et al., 2014; Kobayashi et al., 2018). Moreover, WTAP also may scaffold the MTC and RBM15/RBM15B recruits the MTC to target sites of modification (Kan et al., 2017). METTL16 is another independent m6A methyltransferase that can directly methylate UACAGAGAA motifs. In addition, METTL16 can also regulate the activity of all cellular methyltransferases including METTL3/METTL14, so the deletion of METTLl6 can reduce the methylation level of UACAGAGAA and/or RRACH motif regions (Fazi and Fatica, 2019; Koh et al., 2019; Aoyama et al., 2020). Recent studies have shown that METTL5 as the enzyme responsible for 18s rRNA m6A modification in site of 1831A, which must form a METTL5-TRMT112 (TRMT112, a methyltransferase activator) heterodimeric complex to maintain its biological stability (Van Tran et al., 2019; Rong et al., 2020).
RNA m6A modification is enriched in the 3′untranslated region (3′UTR) and near termination codon of mature polyadenylate mRNA in mammalian system, and plays a regulatory role in the transcription of eukaryotic mRNA. VIRMA, an important WTAP interactor, is essential for the deposition of m6A to the 3′UTR of mRNA and near stop codons and recruiting the methyltransferase core components METTL3/METTL14/WTAP (Horiuchi et al., 2013; Yue et al., 2018; Shi et al., 2019; UniProt, 2019); Knockdown of VIRMA resulted in reduced m6A methylation near the 3′UTR of the stop codon. In addition, VIRMA binds to the polyadenylation cleavage factors CPSF5 and CPSF6 in an RNA-dependent manner, and knockdown of CPSF5 also resulted in a significant shortening of the 3′UTR of the mRNA (Yue et al., 2018).
ZC3H13 could promote nuclear localization of the writer complex for RNA m6A modification, ZCCHC4 as the 28s rRNA modification enzyme for m6A modification at the site of 4220A (Ren et al., 2019). RBM15/15B binds to U-rich regions and cooperates with MTC for certain RNAs methylation (Shi et al., 2019). CBLL1 (Cbl proto-oncogene like 1), also known as Hakai, was originally found to encode the E3 ubiquitin ligase for the E-cadherin complex and mediate its ubiquitination, endocytosis, and degradation in lysosomes. Recent studies have shown that CBLL1 is required for stabilization of core components METTL3/14 of the m6A RNA methylation and plays a role in the efficiency of mRNA splicing and RNA processing (Yue et al., 2018; Bawankar et al., 2021).
m6A RNA Demethylases—Erasers
The intracellular RNA m6A demethylation is mainly accomplished by fat mass and obesity-associated protein (FTO) and the alkylation repair homolog protein 5 (ALKBH5), referred to as erasers, which cooperates with m6A methylases to maintain the dynamic balance of m6A modification in the cells (Jia et al., 2011; Zheng et al., 2013; Xue et al., 2019).
As the first m6A mRNA demethylase, FTO established the concept of reversible RNA modification (Jiang et al., 2021b). The whole transcriptome RNA demethylation analysis clarified that FTO is a potent nuclear mRNA processing regulator, which preferentially binds to the pre-mRNA in the intron region, in the proximity of alternative splicing exons and poly(A) sites, and participates in alternative splicing and 3′ end mRNA processing (Bartosovic et al., 2017). In addition, multiple evidences indicate that FTO does play a key role in regulating fat mass, fat production and body weight (Merritt et al., 2018). Epidemiological studies also show that FTO SNPs is strongly associated with increased risk of various cancers (Chen and Du, 2019). FTO has recently been shown to play an m6A-dependent role in multiple biological processes, such as cancer cell apoptosis, proliferation, migration, invasion, metastasis, cell-cycle, differentiation, stem cell self-renewal and so on (Deng et al., 2018; Wang et al., 2020d). In addition, studies have shown that FTO binds multiple RNA species, including mRNA, snRNA, and tRNA, and can demethylate internal m6A and cap m6Am in mRNA, internal and cap m6Am in snRNA, internal m6A in U6 RNA, and N1-methyladenosine (m1A) in tRNA (Wei et al., 2018).
ALKBH5 is another major m6A demethylase which plays a critical biological and pharmacological role in human cancer. ALKBH5 is an independent prognostic indicator in a variety of cancers. ALKBH5 can regulate various tumor biological processes, such as the cell proliferation, invasion, migration, metastasis, cancer stem cell self-renewal and tumor microenvironment (Li et al., 2020b; Shen et al., 2020). In addition, ALKBH5 also plays an important role in human non-cancer diseases, such as reproductive system diseases (Nettersheim et al., 2019). The potential regulatory mechanism of ALKBH5 relies on m6A-dependent modifications (Wang et al., 2020c). Moreover, it was found that ALKBH5 knockout resulted in increased mRNA m6A modification level in the nucleus, but a decrease in the amount of mRNA in the cytoplasm, suggesting that ALKBH5 may be involved in mRNA transport (Bartosovic et al., 2017). In addition, neither FTO nor ALKBH5 display a preference for RRACH m6A motif demethylation (Zou et al., 2016).
m6A RNA Binding Proteins—Readers
The m6A modification plays an important role in the production, processing, splicing and translation of mRNA (Huang et al., 2021a). m6A binding protein (also called: reader) is essential for the recognition of m6A RNA, including m6A direct binding proteins (such as: YTH domain family, IGF2BPs, eIF3, etc) and m6A indirect binding proteins (hnRNP family, etc).
At present, it is considered that the YT521-B homology (YTH) domain family consists of YTH domain containing protein 1-2 (YTHDC1-2) and YTH domain family protein 1-3 (YTHDF1-3). YTHDC1 mediates the splicing of m6A pre-mRNA via recruiting splicing factor and nuclear export adaptor SRSF3 while blocking SRSF10 (Xiao et al., 2016), and NXF1 also involved the nuclear exporting of m6A-containing mRNAs (Roundtree et al., 2017b); ELAVL1 (ELAV like RNA binding protein 1, also known as HuR) is known to be an RNA stabilizing protein that interacts with mRNAs containing m6A modifications and cooperates with YTHDC1 to enhance the stability and subsequent translation of target gene mRNAs (Liang et al., 2021). The m6A reader YTHDC2 functions in the cytoplasm, affecting the m6A mRNA translation efficiency and abundance of its targets. It has been proposed that YTHDC2 interacts with m6A-containing mRNAs, ribosomes, and XRN1 to regulate mRNA stability and translation. In addition, MEIOC also can be bound to YTHDC2 in an RNA-independent manner, stabilize the binding of YTHDC2 to mRNA (Abby et al., 2016; Inada, 2020). YTHDF3 has been shown to promote the functions of YTHDF1 and YTHDF2, which promote m6A mRNA translation by binding to YTHDF1 and enhance RNA degradation by interacting with YTHDF2, respectively (Shi et al., 2017).
The insulin-like growth factor-2 (IGF2) mRNA-binding proteins 1, 2, and 3 (IGF2BP1/2/3) as a distinct family of m6A readers that regulate mRNA stability, transport, and translation (Uyar et al., 2017). Recent research shows that the association between IGF2BPs and target mRNAs, such as myc mRNA, is enhanced by m6A modification of target transcripts, indicating that IGF2BPs is an important m6A reader (Muller et al., 2019). In addition, IGF2BPs can stabilize the mRNA of target genes by recruiting and interacting with RNA stabilizing proteins, such as: ELAVL1/HuR, MATK3, PABPC1, etc (He et al., 2019; Zhu et al., 2020a), and also IGF2BPs can protect the target mRNA from stress condition (Huang et al., 2018). Eukaryotic initiation factor 3 (eIF3) can be regarded as a reader of 5′ UTR m6A, which can interact with m6A modified RNA, enhance ribosomal loading and promote the translation of target gene mRNA (Shah et al., 2017).
Heterogeneous ribonucleoproteins (hnRNPs) refer to a large family of RNA-binding proteins (RBPs) that are involved in multiple aspects of nucleic acid metabolism, including alternative splicing, processing, mRNA stabilization, and subsequent transcription and translation. Among them, hnRNPA2B1 can directly bind to m6A RNA to regulate RNA splicing and processing. In addition, hnRNPA2B1 can also cooperate with proteins, such as: DGCR8, etc., to promote the processing and formation of miRNA (Alarcon et al., 2015). The m6A reader protein hnRNPC or hnRNPG binds m6A RNAs through an “m6A switch” mechanism, in which m6A-mediated RNA hairpin destabilization exposes a single-stranded hnRNPC or hnRNPG-binding motif, and binds to m6A RNA and facilitates processing (Liu et al., 2017; Figure 1).
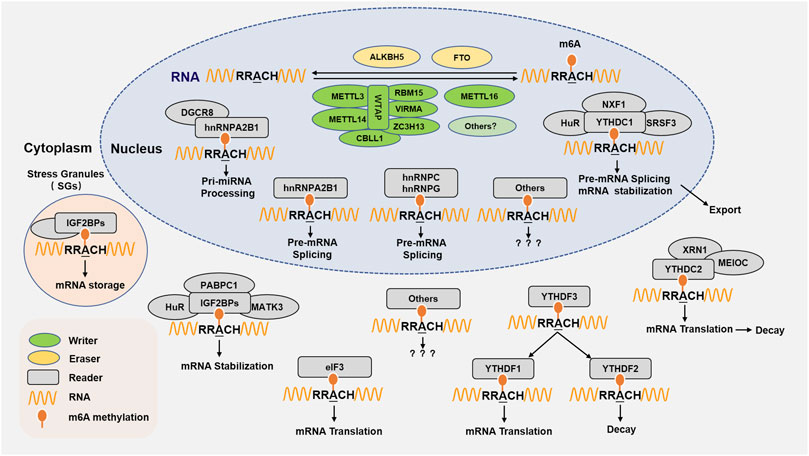
FIGURE 1. m6A RNA methylation regulating proteins and molecular functions. m6A RNA methylation is performed by its writer, eraser, and reader to add, delete, recognize m6A, respectively. The writer refers to m6A methyltransferases including METTL3, METTL14, WTAP, RBM15/15B, VIRMA/KIAA1429/Virilizer, ZC3H13, CBLL1/Hakai, and METTL16. The m6A eraser is the demethylase FTO and ALKBH5. The m6A readers are proteins that recognize m6A sites and perform a variety of functions in the nucleus or cytoplasm, the currently identified m6A reader proteins including YTHDC1, YTHDC2, YTHDF1, YTHDF2, YTHDF3, IGF2BPs, eIF3, and hnRNPA2B1, HNRNPC, HNRNPG. m6A methylation is involved multiple functions in RNA metabolism, including RNA stabilization and splicing, miRNA processing, nuclear export, translation, RNA storage and decay, and so on.
Part 2: The Role of m6A Modification in Cancer
More and more research evidences show that m6A modification is related to the proliferation, differentiation, tumorigenesis, invasion and metastasis of tumors, and plays a key role as an oncogene or tumor suppressor gene in the occurrence and development of malignant tumors (Table 2).
Acute Myeloid Leukemia
The link between m6A and tumorigenesis was first demonstrated in AML, where overexpression of methyltransferases leads to increased RNA m6A modification, leading to abnormal hematopoietic stem cell differentiation and promoting leukemogenesis (Vu et al., 2017).
The abnormally high expression of METTL3 in AML can promote m6A modification and promote the occurrence and development of AML by enhancing the translation of c-MYC, BCL2, and PTEN mRNAs. Moreover, knockdown of METTL3 can lead to increased p-AKT levels and promote cell differentiation (Vu et al., 2017). In addition, METTL3 and METTL14 exert oncogenic roles in AML by increasing m6A levels in MDM2 mRNA and targeting the MDM2/p53 signaling pathway (Sang et al., 2022). However, the low expression of METTL3 in AML-MSCs (mesenchymal stem cells from AML patients) promotes the expression of AKT protein and upregulate the PI3K-AKT signaling pathway, which ultimately leads to the enhanced adipogenesis ability of AML-MSCs and facilitates the chemoresistance of AML (Pan et al., 2021b).
ALKBH5 is aberrantly overexpressed in AML and associated with poor prognosis. ALKBH5 exerts tumor-promoting roles in the development and maintenance of AML and self-renewal of leukemia stem/initiating cells (LSCs/LICs) through post-transcriptional regulation of its key target TACC3, AXL (Wang et al., 2020b; Shen et al., 2020). FTO is highly expressed in AML containing t (11q23)/MLL rearrangements, t (15;17)/PML-RARA, FLT3-ITD and/or NPM1 mutations. FTO inhibits the expression of ASB2 and RARA by reducing m6A levels of the target mRNA, which in turn enhances leukemic oncogene-mediated cell transformation and leukemogenesis, and inhibits all-trans retinoic acid (ATRA)-induced AML cell differentiation (Li et al., 2017).
YTHDC1 is overexpressed in AML and is required for proliferation, differentiation and development, maintenance of self-renewal, leukemogenesis, and survival (Sheng et al., 2021). Moreover, YTHDC1 can also promote the development of AML by promoting phase separation. In recent years, studies have shown that abnormal phase separation is closely related to the occurrence of tumors. YTHDC1 binds to m6A and forms nuclear condensates (nYACs) which are essential for maintaining targets mRNA stability and AML cell survival and the undifferentiated state (Cheng et al., 2021).
Non-Small-Cell Lung Cancer
In non-small-cell lung cancer (NSCLC), METTL3 promotes the translation of mRNAs of certain genes by increasing m6A levels, including c-Myc, BCL-2, epidermal growth factor receptor (EGFR), hippo pathway effector TAZ and YAP, MAPKAPK2 (MK2), and DNMT3A, thereby promoting lung adenocarcinoma (LUAD) cell proliferation, survival, invasion and metastasis (Lin et al., 2016; Jin et al., 2019; Wu et al., 2021a; Zhang et al., 2021c). High expression of METTL3 can improve the stability of JUNB mRNA and increase its expression. JUNB is an important transcriptional regulator of epithelial-mesenchymal transition (EMT), including CDH1/E-cadherin, FN1/Fibronectin and VIM/Vimentin (Wanna-Udom et al., 2020). Long non-coding RNAs (lncRNAs) play key roles in a variety of physiological and pathological processes. METTL3 enhances the stability of LCAT3 lncRNA through m6A modification, and then promotes the proliferation, invasion and metastasis of LUAD cells through the LCAT3-FUBP1-MYC axis (Qian et al., 2021a). Moreover, METTL3 also upregulates the level of miR-1246 which reduces the expression of the tumor suppressor PEG3 to promote the development of lung adenocarcinoma (Huang et al., 2021b); In addition, METTL3 can also promote tumor proliferation, EMT, invasion, and migration by down-regulating miRNAs, such as: miR-1915-3p (Pan et al., 2021a). Cell ferroptosis, a novel form of programmed cell death. The highly expressed METTL3 in LUAD can promote LUAD cell proliferation and inhibit ferroptosis, and its molecular mechanism is that METTL3-mediated m6A modification enhances the stability of SLC7A11 mRNA and promotes its translation (Xu et al., 2022). Metabolic reprogramming, known as the Warburg effect, is considered a key hallmark of cancer, including lung cancer. Studies have shown that METTL3 can enhance the stability of the lncRNA ABH11-AS1 transcript, thereby enhancing the Warburg effect of lung adenocarcinoma, and ultimately promoting the occurrence and development of tumors (Xue et al., 2021).
The m6A reader YTHDC2 is frequently repressed in LUAD, indicating a poor prognosis. Downregulation of YTHDC2 expression leads to enhanced SLC7A11 and SLC3A2 mRNA stability and promotes tumorigenesis (Ma et al., 2021a; Ma et al., 2021b). But there is an interesting study showing that METTL3 has an anti-tumor effect, downregulation of METTL3 decreased protein levels of apoptotic molecules and increased protein levels of the FBXW7 gene and its target genes Mcl-1 and c-Myc, and promotes tumorigenesis in LUAD which may be caused by the heterogeneity of tumor cells (Wu et al., 2021b). METTL3, YTHDF1/3 is highly expressed in LUAD, METTL3 promotes YAP mRNA translation by recruiting YTHDF1/3 and eIF3b to the translation start site and increased YAP mRNA stability by modulating the MALAT1-miR-1914-3p-YAP axis, ultimately resulting in increased YAP expression and activity that induces NSCLC drug resistance and metastasis (Jin et al., 2019).
Hepatocellular Carcinoma
METTL3 is highly expressed in HCC and is closely associated with poor prognosis of HCC patients, and promotes HCC cell proliferation, migration, metastasis and tumorgenicity via YTHDF2-dependent post-transcriptional silencing of SOCS2, which is a member of the suppressor of cytokine signaling (SOCS) family, and is a negative regulator of the JAK/STAT pathway (Chen et al., 2018). WTAP-guided m6A modification plays a key role in HCC oncogenesis through the HuR1-ETS1-P21/P27 axis (Chen et al., 2019b). High expression of VIRMA in HCC patients is associated with poor prognosis. VIRMA induces m6A methylation of the 3′ UTR of GATA3 pre-mRNA, leading to dissociation of the RNA-binding protein HuR and degradation of GATA3 pre-mRNA, promoting tumor growth and metastasis in vivo (Lan et al., 2019).
However, METTL14, as a tumor suppressor in HCC, is down-regulated. The stability of USP48 mRNA is reduced due to decreased m6A modification of METTL14 and leads to the degradation of its downstream target gene SIRT6, which ultimately enhances aerobic glycolysis in tumor cells (Du et al., 2021). In addition, METTL14, as an anti-metastatic factor, acts as a favorable factor for HCC by regulating m6A-dependent miRNA processing (Ma et al., 2017).
Downregulation of ALKBH5 is associated with poor prognosis in HCC. Decreased expression of ALKBH5 leads to increased 3′UTR m6A modification of LYPD1 mRNA, which in turn results in the increased expression of LYPD1, which promotes tumor cell growth, migration, invasion and metastasis (Chen et al., 2020c). FTO can increase the proportion of stem-like cells in HCC by enhancing the expression of SOX2, KLF4, and NANOG (Bian et al., 2021).
YTHDF1 is highly expressed in HCC and associated with poor survival. YTHDF1 participates in the progression of HCC by activating the PI3K/AKT/mTOR signaling pathway and inducing EMT (Luo et al., 2021). Overexpressed IGF2BP2 can directly recognize and bind to the m6A site of FEN1 mRNA, enhancing the stability of FEN1 mRNA and promoting the proliferation of liver cancer cells in vitro and in vivo (Pu et al., 2020).
Breast Cancer
m6A regulatory factor is an important participant in the malignant progression of breast cancer and may be a prognostic and therapeutic target for BCa. METTL3 is highly up-regulated in BCa and predicts a poor prognosis. High expression of METTL3 can increase the modification level of downstream target mRNA 3′UTR m6A and enhance the stability and translation of mRNA, such as BCL-2 (Wang et al., 2020a), SOX2 (Xie et al., 2021), KRT7 (Chen et al., 2021a), MALAT1 (Zhao et al., 2021), etc., which can promote BCa cell proliferation, EMT, invasion, metastasis, tumorigenicity and stem cell. Tumor immune deficiency is an important cause of tumorigenesis. PD-L1, the ligand of PD-1, is highly expressed in many cancers and is closely associated with mortality in cancer patients, establishing the role of PD-1 in cancer-induced immunosuppression. METTL3 enhances the stability of PD-L1 mRNA, promotes the expression of PD-L1 and induces tumor immunosuppression by relying on m6A modification (Wan et al., 2022). However, METTL3 is low expression in triple negative breast cancer (TNBC) and strongly associated with short-distance-metastasis-free survival. METTL3 knockout can enhance cell migration, invasion and adhesion by reducing m6A level (Shi et al., 2020). Downregulation of METTL14 and ZC3H13 in BCa, act as tumor suppressor, has been found in breast cancer and poor prognosis is predicted (Gong et al., 2020).
High levels of FTO were significantly associated with lower survival in BCa patients. FTO promotes BCa cell proliferation, colony formation and metastasis in vitro and in vivo (Niu et al., 2019; Xu et al., 2020).
YTHDF1 and YTHDF3 are highly expressed in BCa patients and with poor prognosis, which can enhance the expression of related genes, such as FOXM1, ST6GALNAC5, GJA1, EGFR, VEGFRA, etc., and promote the metastasis, invasion and angiogenesis of BCa cells (Chang et al., 2020; Chen et al., 2022).
The Diverse Role of m6A in Cancer
More recently, extensive efforts have been made to investigate the biological effects of dysregulated m6A modifications and related mechanisms (i.e., m6A writers, erasers, and reader proteins) in various cancers, such as prostate cancer (Wang et al., 2022), colorectal cancer (Tanabe et al., 2016; Zhu et al., 2020b), gastric cancer (Xu et al., 2021), esophageal cancer (Guo et al., 2020), cervical cancer and endometrial cancer (Zhang and Yang, 2021; Huang et al., 2022), pancreatic cancer (Li et al., 2021), skin cancer (Yang et al., 2021), etc. Moreover, METTL16, as an independent regulator, plays an important role in RNA m6A modification. In the nucleus, METTL16 functions as a writer for the deposition of m6A in its specific mRNA; And in the in the cytoplasm, METTL16 could promote the translation of over 4000 mRNA transcripts via interacting with eIF3a and 3b (Su et al., 2022). In addition, m6A regulator also involved the immune response. There is evidence that the m6A eraser FTO can decrease the response of PD-1 inhibitor immunotherapy and promote tumorigenesis. Knockdown of FTO enhances the sensitivity to interferon gamma (IFNγ) and anti-PD-1 therapy (Yang et al., 2019). Furthermore, FTO could promote the malignant transformation and tumorigenesis via up-regulated the target gene NEDD4L in keratinocytes. Upon further investigation, it was found that the FTO expression was elevated after treated with low-level arsenic which is a human carcinogen (Cui et al., 2021).
Above all, different m6A regulators have different biological effects in various tumors. Even if the same regulator is in the same tumor but is of different molecular subtypes, the biological effects are also not the same, which may be related to tumor heterogeneity and tumor microenvironment. In general, m6A plays an important role in the regulation of tumor cell proliferation, cell cycle, EMT, invasion, metastasis, stemness maintenance, apoptosis, immune effect, and other aspects, which is of great significance for the occurrence and development of tumors.
Part 3: The Anti-Cancer Effect of Nature Products by Regulating m6A Modification
Natural products and compounds derived from natural products account for about 40% of all drugs approved for clinical use, among which ∼70% of anticancer drugs are derived from natural products. (Butler et al., 2014; Newman and Cragg, 2016; Bahman et al., 2018; Sahm et al., 2020). After years of efforts, natural products have also made great progress in anti-cancer, and many natural small molecules have been successfully marketed and become star therapeutic drugs in the corresponding field. For example, paclitaxel, camptothecin, homoharringtonine, ginsenoside Rg3, etc. In recent years, with the deepening of understanding and attention to natural products, many new opportunities have been brought to the development of natural anti-tumor molecules (Singla et al., 2021a; Singla et al., 2021b; Tan et al., 2021; Wang et al., 2021; Singla et al., 2022).
β-Elemene
Elemene, a sesquiterpenoid natural compound, is the extraction of traditional Chinese medicinal material herb Curcuma wenyujin, and which is a mixture of β-, γ-, δ-elemene with β-elemene as the main component. β-elemene, the most important component of elemene for pharmaceutical activity, has been shown to be effective in vitro and in vivo against a variety of cancers, such as lung, leukemia, liver cancer, gastric, cervical cancer, colorectal, ovarian, glioblastoma, and melanoma, and so on (Li et al., 2013; Bayala et al., 2014; Gong et al., 2015; Liu et al., 2016; Jiang et al., 2017; Fu et al., 2018; Chen et al., 2020a).
Gefitinib, a classic epidermal growth factor receptor (EGFR) tyrosine kinase receptor inhibitor, has been a bottleneck in the treatment of NSCLC due to the problem of drug resistance (Onitsuka et al., 2010; Sun et al., 2020). Studies have shown that METTL3 is highly expressed in NSCLC and promotes the occurrence and development of NSCLC by promoting tumor cell proliferation, EMT, invasion, metastasis, angiogenesis, and anti-apoptosis. Moreover, highly expressed METTL3 can increase autophagy in NSCLC by upregulating autophagy-related proteins ATG5 and ATG7, and eventually lead to resistance to gefitinib and other EGFR TKI drugs. β-elemene can directly inhibit the expression of METTL3, but not VIRMA and METTL14, causing the down-regulation of autophagy-related proteins LC3B, ATG5, and ATG7, thereby inhibiting autophagy, tumor cell proliferation and promoting cell apoptosis, and finally reversing the effect of gefitinib resistance in NSCLC (Liu et al., 2020). In addition, PTEN is a well-known tumor suppressor in various cancers and acts as a negative regulator of PI3K-Akt pathway. β-elemene can reduce the level of m6A modification of PTEN mRNA by downregulating the expression of METTL3, resulting in an increase in the expression of PTEN protein, which ultimately inhibits the growth of tumor cells and promotes apoptosis (Feng et al., 2022).
Soy Isoflavones Genistein
Genistein is a major active factor in soy isoflavones, the most effective functional component in soy isoflavone products, and has a variety of physiological functions. Genistein is similar in structure to mammalian estrogen-estradiol, and has the diphenolic hydroxyl active group of estrogen. So genistein has various physiological activities such as estrogen-like activity.
Studies have shown that the antioxidant and anti-proliferative properties of soy isoflavones are the main reasons for their anti-cancer effects. Soy isoflavones have obvious therapeutic effects on breast cancer, colon cancer, lung cancer, prostate cancer, skin cancer, and leukemia. Soy isoflavones can also prevent the occurrence of ovarian cancer, colon cancer, stomach cancer, and prostate cancer (Suthar et al., 2001; Sarkar et al., 2006; Kaushik et al., 2018; Park et al., 2018; Imai-Sumida et al., 2020; Aboushanab et al., 2021). In addition, down-regulation of ALKBH5 expression enhanced the expression of mesenchymal phenotype markers α-smooth muscle actin and snail, which are factors that promote EMT. Genistein can increase the expression of ALKBH5, reduce the level of RNA m6A and inhibit EMT (Ning et al., 2020).
Resveratrol
Resveratrol, a non-flavonoid polyphenolic organic compound, is a phytoalexin with the chemical formula C14H12O3. It can be synthesized in grape leaves and grape skins, and is a biologically active ingredient in wine and grape juice. Resveratrol has antioxidant, anti-inflammatory, anti-cancer and cardiovascular protection effects (Rauf et al., 2018a). The heavy metal cadmium (Cd) can promote the migration and invasion of colorectal cancer cells, and Cd could upregulate the expressions of N-cadherin, vimentin, and ZEB1 and downregulate the expression of E-cadherin in colorectal cancer cells. Resveratrol could reverse Cd-promoted migration, invasion, and EMT processes by modulating ZEB1 expression (Qian et al., 2021b). Moreover, resveratrol can reduce aflatoxin B1-induced ROS accumulation, and can also cause changes in m6A modification-related proteins, including: METTL3, FTO, YTHDF2 (Wu et al., 2020).
Rhein
Extracted from the rhizome of Rheum palmatum, Rhein is the main bioactive component of Dahuang (rhubarb) therapeutic laxatives. In tumors, Rhein mainly inhibits cell proliferation, promotes cell apoptosis, inhibits tumor cell invasion, and metastasis, and enhances hypoxia tolerance, and so on. Its target signaling pathways include: PI3K-Akt, MAPK (ERKs, JNKs, P38), Wnt, NF-kB, et al. (Henamayee et al., 2020). Rhein was the first FTO-competitive inhibitor discovered, which can increase the level of m6A modification on intracellular target mRNA. In addition, Rhein can reversibly bind to FTO or ALKBH5 to form a complex and prevent recognition of intracellular m6A substrates (Chen et al., 2012; Zannella et al., 2021).
Baicalin
Baicalin is a flavonoid compound extracted and isolated from the dry root of Scutellaria baicalensis Georgi (a dicotyledonous Lamiaceae plant), which has a variety of biological activities, including bacteriostatic, diuretic, anti-inflammatory, cholesterol-lowering, anti-thrombotic, anti-asthmatic, hemostatic, anti-allergic, and antispasmodic effects, and also has a strong anti-cancer biological effect (Wang et al., 2015; Gong et al., 2017; Lai et al., 2018).
Type 2 diabetes (T2D) is defined as a metabolic disorder characterized by hyperglycemia that can lead to abnormal organ metabolism or hormones, which in turn lead to a variety of diseases, including cancer. High glucose environment can induce hepatoma cells to up-regulate the expression of HKDC, which can promote the proliferation, invasion and metastasis of tumor cells by downregulating the HKDC/JAK2/STAT1/caspase-3 signaling pathway. Baicalin can reduce the m6A modification level of HKDC mRNA by inhibiting METTL3, which leads to the decrease of HKDC expression and the upregulation of HKDC/JAK2/STAT1/caspase-3 signaling pathway, and finally inhibits the invasion and metastasis of tumor cells (Jiang et al., 2022). In addition, Baicalein inhibits pancreatic cancer cell proliferation and invasion by inhibiting NEDD9 and its downstream Akt and ERK signaling pathways (Zhou et al., 2017).
Others Natural Product
Humantenine, an indole alkaloid compound isolated from Gelsemium elegans, is a traditional medical herb with a drug use. Wu et al (2022) studies indicated that the humantenine leads to the colon cancer cell injury via affecting the gene transcriptome and m6A regulators, including RBM15, METTL3, YTHDF3, and ALKBH5 are up-regulated, and RBM15B, ZC3H13, hnRNPA2BA, YTHDC1, YTHDC2, YTHDF2, and IGF2BP3 are down-regulated.
Curcumin, a polyphenol extracted from turmeric in 1815, has attracted worldwide attention due to its biological activities (such as antioxidant, anti-inflammatory, antibacterial, and antiviral), among which its anticancer potential has been described the most, and the relevant anticancer mechanism is still being investigated (Mortezaee et al., 2019; Wang et al., 2021). Curcumin inhibits the occurrence and development of tumor cells by regulating the expression of growth factors, inflammatory factors, apoptosis-related proteins, protein phosphokinases, and receptors, and cell proliferation-related genes, such as FGFs, VEGF, EGF/EGFR, TNF, IFN, ILs, Caspase-3/6/8/10, FADD, MAPK, JNK, IKK, Survivin, MCL-1, BCL-xL, cIAP-1/2, BCL-2, c-Myc, PCNA, Cyclin D1, etc (Giordano and Tommonaro, 2019). Unfortunately, there is still no relevant report on the relationship between curcumin and m6A enzyme, which also provides a broad space for us to explore the correlation between curcumin and m6A enzyme and its anti-cancer mechanism.
Chrysin (5,7-dihydroxyflavonoid) is a natural, bioactive dietary flavonoid commonly found in a variety of plant extracts, including chamomile, pleurotusostreatus, and honeycombs, as well as in honey and propolis, which has a significant medicinal function and economic value. Chrysin has a variety of biological properties, including anti-cancer, antioxidant, anti-inflammatory, antibacterial, and other effects. Several recent studies have reported that chrysin exerts its anti-cancer effects on endometrial cancer, gastric cancer, breast, lung, cervical, bladder, breast cancer, and colorectal cancer, etc. via selectively inhibiting various cell signaling pathways, such as: Akt/mTOR, JNK1/2, ERK1/2, NF-κB, etc., and promoting tumor cells apoptosis and autophagy (Xia et al., 2015a; Xia et al., 2015b; Roy et al., 2019; He et al., 2021). However, the regulatory relationship between chrysin and m6A enzyme has not been reported.
In addition, there are a number of natural products with anticancer activities, such as: cucurbitacin B, ailanthone, fucoidan, casearlucin A, Wan-Nian-Qing prescription, bruceine D, cinnamaldehyde, fisetin, quercetin, betulinic acid, astragalus polysaccharide, ginsenosides, panax notoginseng saponins, and so on (Wong et al., 2015; Syed et al., 2016; Rauf et al., 2018b; Li et al., 2020c; Jiang et al., 2021a; Huang et al., 2021c; Imran et al., 2021; Yao et al., 2021). By regulating the whole process of tumor occurrence and development, such as cell cycle, apoptosis, autophagy, invasion, and migration, and cell metabolic reprogramming, etc. (Figure 2), these natural products have broad research value and clinical treatment in the field of anti-cancer.
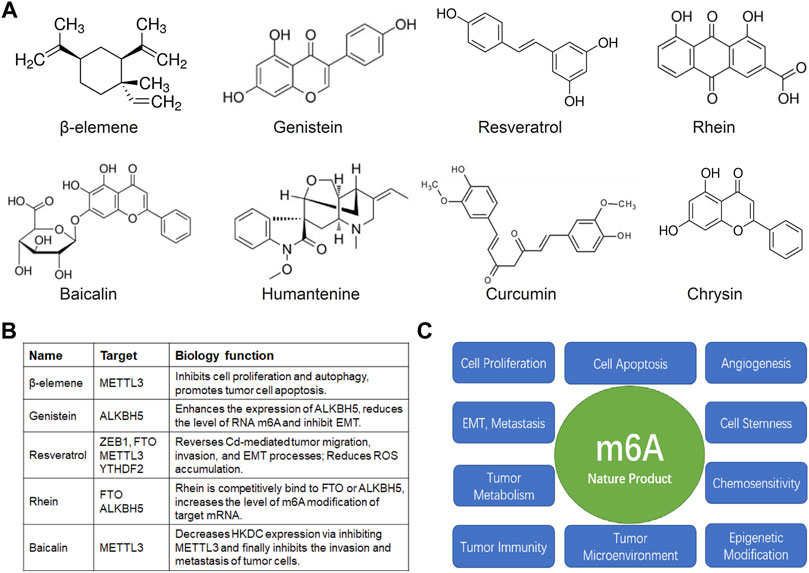
FIGURE 2. Natural products and m6A in anti-cancer research. (A) The structure of the common natural anticancer products, follow as: β-elemene, genistein, resveratrol, rhein, baicalin, humantenine, curcumin, chrysin. (B) The anti-cancer effects of β-elemene, genistein, resveratrol, rhein, baicalin depended on m6A enzyme. (C) The possible mechanism of nature products mediated m6A modification in tumor cell proliferation, apoptosis, angiogenesis, EMT, invasion, metastasis, maintenance of tumor cell stemness, tumor metabolism, chemosensitivity, tumor immunity, tumor microenvironment, and epigenetic modification.
Conclusion
More and more studies have shown that m6A modification plays a key role in the tumorigenesis and progression of various cancers, and the abnormal expression of m6A modification-related proteins is closely related to the occurrence and development of tumors. Natural products have broad prospects and applications in the field of anti-tumor research. At present, some natural products have played an important role in clinical applications. There are relatively few studies on the regulation of m6A modification by natural products, and need more extensive and in-depth research in the field of anti-tumor. The role of natural products in tumors needs further study, m6A modification in cell proliferation, apoptosis, angiogenesis, EMT, invasion, metastasis, maintenance of tumor cell stemness, tumor metabolism, chemosensitivity, tumor immunity, tumor microenvironment, and the roles and mechanisms of epigenetic modification still need to be further explored.
Author Contributions
NS, KC, JZ, KZ, JY, and HW wrote the article. JL, ZM, FZ, HM, LC, CC, MS, and YL reviewed and revised the article. All authors contributed to the article and approved the submitted version.
Funding
This work was supported by the National Natural Science Foundation of China (U1904133), Program for Young Key Teachers in Colleges and Universities in Henan Province (2020GGJS150, 2021GGJS104) and the Natural Science Foundation of Henan Province of China (212300410224, 212300410381). This work was also supported by Henan Province Training Programs of Innovation and Entrepreneurship for Undergraduates (202210472054).
Conflict of Interest
The authors declare that the research was conducted in the absence of any commercial or financial relationships that could be construed as a potential conflict of interest.
Publisher’s Note
All claims expressed in this article are solely those of the authors and do not necessarily represent those of their affiliated organizations, or those of the publisher, the editors and the reviewers. Any product that may be evaluated in this article, or claim that may be made by its manufacturer, is not guaranteed or endorsed by the publisher.
References
Abby, E., Tourpin, S., Ribeiro, J., Daniel, K., Messiaen, S., Moison, D., et al. (2016). Implementation of Meiosis Prophase I Programme Requires a Conserved Retinoid-independent Stabilizer of Meiotic Transcripts. Nat. Commun. 7, 10324. doi:10.1038/ncomms10324
Aboushanab, S. A., Khedr, S. M., Gette, I. F., Danilova, I. G., Kolberg, N. A., Ravishankar, G. A., et al. (2021). Isoflavones Derived from Plant Raw Materials: Bioavailability, Anti-cancer, Anti-aging Potentials, and Microbiome Modulation. Crit. Rev. Food Sci. Nutr. 1, 1–27. doi:10.1080/10408398.2021.1946006
Alarcón, C. R., Goodarzi, H., Lee, H., Liu, X., Tavazoie, S., and Tavazoie, S. F. (2015). HNRNPA2B1 Is a Mediator of m(6)A-dependent Nuclear RNA Processing Events. Cell 162 (6), 1299–1308. doi:10.1016/j.cell.2015.08.011
Aoyama, T., Yamashita, S., and Tomita, K. (2020). Mechanistic Insights into m6A Modification of U6 snRNA by Human METTL16. Nucleic Acids Res. 48 (9), 5157–5168. doi:10.1093/nar/gkaa227
Bahman, A. A., Abaza, M. S. I., Khoushiash, S. I., and Al-Attiyah, R. J. (2018). Sequence-dependent E-ffect of S-orafenib in C-ombination with N-atural P-henolic C-ompounds on H-epatic C-ancer C-ells and the P-ossible M-echanism of A-ction. Int. J. Mol. Med. 42 (3), 1695–1715. doi:10.3892/ijmm.2018.3725
Bartosovic, M., Molares, H. C., Gregorova, P., Hrossova, D., Kudla, G., and Vanacova, S. (2017). N6-methyladenosine Demethylase FTO Targets Pre-mRNAs and Regulates Alternative Splicing and 3'-end Processing. Nucleic Acids Res. 45 (19), 11356–11370. doi:10.1093/nar/gkx778
Bawankar, P., Lence, T., Paolantoni, C., Haussmann, I. U., Kazlauskiene, M., Jacob, D., et al. (2021). Hakai Is Required for Stabilization of Core Components of the m6A mRNA Methylation Machinery. Nat. Commun. 12 (1), 3778. doi:10.1038/s41467-021-23892-5
Bayala, B., Bassole, I. H., Scifo, R., Gnoula, C., Morel, L., Lobaccaro, J. M., et al. (2014). Anticancer Activity of Essential Oils and Their Chemical Components - a Review. Am. J. Cancer Res. 4 (6), 591–607.
Bian, X., Shi, D., Xing, K., Zhou, H., Lu, L., Yu, D., et al. (2021). AMD1 Upregulates Hepatocellular Carcinoma Cells Stemness by FTO Mediated mRNA Demethylation. Clin. Transl. Med. 11 (3), e352. doi:10.1002/ctm2.352
Butler, M. S., Robertson, A. A., and Cooper, M. A. (2014). Natural Product and Natural Product Derived Drugs in Clinical Trials. Nat. Prod. Rep. 31 (11), 1612–1661. doi:10.1039/c4np00064a
Chang, G., Shi, L., Ye, Y., Shi, H., Zeng, L., Tiwary, S., et al. (2020). YTHDF3 Induces the Translation of m6A-Enriched Gene Transcripts to Promote Breast Cancer Brain Metastasis. Cancer Cell 38 (6), 857–871. e857. doi:10.1016/j.ccell.2020.10.004
Chen, B., Ye, F., Yu, L., Jia, G., Huang, X., Zhang, X., et al. (2012). Development of Cell-Active N6-Methyladenosine RNA Demethylase FTO Inhibitor. J. Am. Chem. Soc. 134 (43), 17963–17971. doi:10.1021/ja3064149
Chen, F., Chen, Z., Guan, T., Zhou, Y., Ge, L., Zhang, H., et al. (2021a). N6 -Methyladenosine Regulates mRNA Stability and Translation Efficiency of KRT7 to Promote Breast Cancer Lung Metastasis. Cancer Res. 81 (11), 2847–2860. doi:10.1158/0008-5472.CAN-20-3779
Chen, H., Yu, Y., Yang, M., Huang, H., Ma, S., Hu, J., et al. (2022). YTHDF1 Promotes Breast Cancer Progression by Facilitating FOXM1 Translation in an m6A-dependent Manner. Cell Biosci. 12 (1), 19. doi:10.1186/s13578-022-00759-w
Chen, J., and Du, B. (2019). Novel Positioning from Obesity to Cancer: FTO, an m6A RNA Demethylase, Regulates Tumour Progression. J. Cancer Res. Clin. Oncol. 145 (1), 19–29. doi:10.1007/s00432-018-2796-0
Chen, L., Li, Q., Jiang, Z., Li, C., Hu, H., Wang, T., et al. (2021b). Chrysin Induced Cell Apoptosis through H19/let-7a/COPB2 Axis in Gastric Cancer Cells and Inhibited Tumor Growth. Front. Oncol. 11, 651644. doi:10.3389/fonc.2021.651644
Chen, M., Wei, L., Law, C. T., Tsang, F. H., Shen, J., Cheng, C. L., et al. (2018). RNA N6-Methyladenosine Methyltransferase-like 3 Promotes Liver Cancer Progression through YTHDF2-dependent Posttranscriptional Silencing of SOCS2. Hepatology 67 (6), 2254–2270. doi:10.1002/hep.29683
Chen, P., Li, X., Zhang, R., Liu, S., Xiang, Y., Zhang, M., et al. (2020a). Combinative Treatment of β-elemene and Cetuximab Is Sensitive to KRAS Mutant Colorectal Cancer Cells by Inducing Ferroptosis and Inhibiting Epithelial-Mesenchymal Transformation. Theranostics 10 (11), 5107–5119. doi:10.7150/thno.44705
Chen, R., Wu, J., Lu, C., Yan, T., Qian, Y., Shen, H., et al. (2020b). Systematic Transcriptome Analysis Reveals the Inhibitory Function of Cinnamaldehyde in Non-small Cell Lung Cancer. Front. Pharmacol. 11, 611060. doi:10.3389/fphar.2020.611060
Chen, X. Y., Zhang, J., and Zhu, J. S. (2019a). The Role of m6A RNA Methylation in Human Cancer. Mol. Cancer 18 (1), 103. doi:10.1186/s12943-019-1033-z
Chen, Y., Peng, C., Chen, J., Chen, D., Yang, B., He, B., et al. (2019b). WTAP Facilitates Progression of Hepatocellular Carcinoma via m6A-HuR-dependent Epigenetic Silencing of ETS1. Mol. Cancer 18 (1), 127. doi:10.1186/s12943-019-1053-8
Chen, Y., Zhao, Y., Chen, J., Peng, C., Zhang, Y., Tong, R., et al. (2020c). ALKBH5 Suppresses Malignancy of Hepatocellular Carcinoma via m6A-Guided Epigenetic Inhibition of LYPD1. Mol. Cancer 19 (1), 123. doi:10.1186/s12943-020-01239-w
Cheng, Y., Xie, W., Pickering, B. F., Chu, K. L., Savino, A. M., Yang, X., et al. (2021). N6-Methyladenosine on mRNA Facilitates a Phase-Separated Nuclear Body that Suppresses Myeloid Leukemic Differentiation. Cancer Cell 39 (7), 958–e8. doi:10.1016/j.ccell.2021.04.017
Cui, Y. H., Yang, S., Wei, J., Shea, C. R., Zhong, W., Wang, F., et al. (2021). Autophagy of the m6A mRNA Demethylase FTO Is Impaired by Low-Level Arsenic Exposure to Promote Tumorigenesis. Nat. Commun. 12 (1), 2183. doi:10.1038/s41467-021-22469-6
Deng, X., Su, R., Stanford, S., and Chen, J. (2018). Critical Enzymatic Functions of FTO in Obesity and Cancer. Front. Endocrinol. (Lausanne) 9, 396. doi:10.3389/fendo.2018.00396
Du, L., Li, Y., Kang, M., Feng, M., Ren, Y., Dai, H., et al. (2021). USP48 Is Upregulated by Mettl14 to Attenuate Hepatocellular Carcinoma via Regulating SIRT6 Stabilization. Cancer Res. 81 (14), 3822–3834. doi:10.1158/0008-5472.CAN-20-4163
Fazi, F., and Fatica, A. (2019). Interplay between N 6-Methyladenosine (m6A) and Non-coding RNAs in Cell Development and Cancer. Front. Cell Dev. Biol. 7, 116. doi:10.3389/fcell.2019.00116
Feng, Y., Li, C., Liu, S., Yan, F., Teng, Y., Li, X., et al. (2022). β-Elemene Restrains PTEN mRNA Degradation to Restrain the Growth of Lung Cancer Cells via METTL3-Mediated N6 Methyladenosine Modification. J. Oncol. 2022, 1–11. doi:10.1155/2022/3472745
Fu, B., Wang, N., Tan, H. Y., Li, S., Cheung, F., and Feng, Y. (2018). Multi-Component Herbal Products in the Prevention and Treatment of Chemotherapy-Associated Toxicity and Side Effects: A Review on Experimental and Clinical Evidences. Front. Pharmacol. 9, 1394. doi:10.3389/fphar.2018.01394
Gao, R., Ye, M., Liu, B., Wei, M., Ma, D., and Dong, K. (2021). m6A Modification: A Double-Edged Sword in Tumor Development. Front. Oncol. 11, 679367. doi:10.3389/fonc.2021.679367
Giordano, A., and Tommonaro, G. (2019). Curcumin and Cancer. Nutrients 11 (10), 2376. doi:10.3390/nu11102376
Gokhale, N. S., McIntyre, A. B. R., McFadden, M. J., Roder, A. E., Kennedy, E. M., Gandara, J. A., et al. (2016). N6-Methyladenosine in Flaviviridae Viral RNA Genomes Regulates Infection. Cell Host Microbe 20 (5), 654–665. doi:10.1016/j.chom.2016.09.015
Gong, M., Liu, Y., Zhang, J., Gao, Y.-j., Zhai, P.-p., Su, X., et al. (2015). β-Elemene Inhibits Cell Proliferation by Regulating the Expression and Activity of Topoisomerases I and IIαin Human Hepatocarcinoma HepG-2 Cells. BioMed Res. Int. 2015, 1–10. doi:10.1155/2015/153987
Gong, P. J., Shao, Y. C., Yang, Y., Song, W. J., He, X., Zeng, Y. F., et al. (2020). Analysis of N6-Methyladenosine Methyltransferase Reveals METTL14 and ZC3H13 as Tumor Suppressor Genes in Breast Cancer. Front. Oncol. 10, 578963. doi:10.3389/fonc.2020.578963
Gong, W. Y., Zhao, Z. X., Liu, B. J., Lu, L. W., and Dong, J. C. (2017). Exploring the Chemopreventive Properties and Perspectives of Baicalin and its Aglycone Baicalein in Solid Tumors. Eur. J. Med. Chem. 126, 844–852. doi:10.1016/j.ejmech.2016.11.058
Guo, H., Wang, B., Xu, K., Nie, L., Fu, Y., Wang, Z., et al. (2020). m6A Reader HNRNPA2B1 Promotes Esophageal Cancer Progression via Up-Regulation of ACLY and ACC1. Front. Oncol. 10, 553045. doi:10.3389/fonc.2020.553045
Harvey, C. J. B., Tang, M., Schlecht, U., Horecka, J., Fischer, C. R., Lin, H. C., et al. (2018). HEx: A Heterologous Expression Platform for the Discovery of Fungal Natural Products. Sci. Adv. 4 (4), eaar5459. doi:10.1126/sciadv.aar5459
He, L., Li, H., Wu, A., Peng, Y., Shu, G., and Yin, G. (2019). Functions of N6-Methyladenosine and its Role in Cancer. Mol. Cancer 18 (1), 176. doi:10.1186/s12943-019-1109-9
He, Y., Shi, Y., Huang, H., Feng, Y., Wang, Y., Zhan, L., et al. (2021). Chrysin Induces Autophagy through the Inactivation of the ROS-mediated Akt/mTOR S-ignaling P-athway in E-ndometrial C-ancer. Int. J. Mol. Med. 48 (3), 172. doi:10.3892/ijmm.2021.5005
Henamayee, S., Banik, K., Sailo, B. L., Shabnam, B., Harsha, C., Srilakshmi, S., et al. (2020). Therapeutic Emergence of Rhein as a Potential Anticancer Drug: A Review of its Molecular Targets and Anticancer Properties. Molecules 25 (10), 2278. doi:10.3390/molecules25102278
Horiuchi, K., Kawamura, T., Iwanari, H., Ohashi, R., Naito, M., Kodama, T., et al. (2013). Identification of Wilms' Tumor 1-associating Protein Complex and its Role in Alternative Splicing and the Cell Cycle. J. Biol. Chem. 288 (46), 33292–33302. doi:10.1074/jbc.M113.500397
Hsu, P. J., Shi, H., and He, C. (2017). Epitranscriptomic Influences on Development and Disease. Genome Biol. 18 (1), 197. doi:10.1186/s13059-017-1336-6
Huang, H., Weng, H., and Chen, J. (2020). m6A Modification in Coding and Non-coding RNAs: Roles and Therapeutic Implications in Cancer. Cancer Cell 37 (3), 270–288. doi:10.1016/j.ccell.2020.02.004
Huang, H., Weng, H., Sun, W., Qin, X., Shi, H., Wu, H., et al. (2018). Recognition of RNA N6-Methyladenosine by IGF2BP Proteins Enhances mRNA Stability and Translation. Nat. Cell Biol. 20 (3), 285–295. doi:10.1038/s41556-018-0045-z
Huang, J., Shao, Y., and Gu, W. (2021a). Function and Clinical Significance of N6-Methyladenosine in Digestive System Tumours. Exp. Hematol. Oncol. 10 (1), 40. doi:10.1186/s40164-021-00234-1
Huang, S., Luo, S., Gong, C., Liang, L., Xiao, Y., Li, M., et al. (2021b). MTTL3 Upregulates microRNA-1246 to Promote Occurrence and Progression of NSCLC via Targeting Paternally Expressed Gene 3. Mol. Ther. Nucleic Acids 24, 542–553. doi:10.1016/j.omtn.2021.02.020
Huang, W., Kong, F., Li, R., Chen, X., and Wang, K. (2022). Emerging Roles of m6A RNA Methylation Regulators in Gynecological Cancer. Front. Oncol. 12, 827956. doi:10.3389/fonc.2022.827956
Huang, Y., Hou, Y., Qu, P., and Cai, Y. (2021c). Editorial: Combating Cancer with Natural Products: What Would Non-coding RNAs Bring? Front. Oncol. 11, 747586. doi:10.3389/fonc.2021.747586
Imai-Sumida, M., Dasgupta, P., Kulkarni, P., Shiina, M., Hashimoto, Y., Shahryari, V., et al. (2020). Genistein Represses HOTAIR/Chromatin Remodeling Pathways to Suppress Kidney Cancer. Cell Physiol. Biochem. 54 (1), 53–70. doi:10.33594/000000205
Imran, M., Saeed, F., Gilani, S. A., Shariati, M. A., Imran, A., Afzaal, M., et al. (2021). Fisetin: An Anticancer Perspective. Food Sci. Nutr. 9 (1), 3–16. doi:10.1002/fsn3.1872
Inada, T. (2020). Quality Controls Induced by Aberrant Translation. Nucleic Acids Res. 48 (3), 1084–1096. doi:10.1093/nar/gkz1201
Jia, G., Fu, Y., Zhao, X., Dai, Q., Zheng, G., Yang, Y., et al. (2011). N6-methyladenosine in Nuclear RNA Is a Major Substrate of the Obesity-Associated FTO. Nat. Chem. Biol. 7 (12), 885–887. doi:10.1038/nchembio.687
Jiang, H., Yao, Q., An, Y., Fan, L., Wang, J., and Li, H. (2022). Baicalin Suppresses the Progression of Type 2 Diabetes-Induced Liver Tumor through Regulating METTL3/m6A/HKDC1 axis and Downstream P-JAK2/STAT1/clevaged Capase3 Pathway. Phytomedicine 94, 153823. doi:10.1016/j.phymed.2021.153823
Jiang, W., Li, X., Dong, S., and Zhou, W. (2021a). Betulinic Acid in the Treatment of Tumour Diseases: Application and Research Progress. Biomed. Pharmacother. 142, 111990. doi:10.1016/j.biopha.2021.111990
Jiang, X., Liu, B., Nie, Z., Duan, L., Xiong, Q., Jin, Z., et al. (2021b). The Role of m6A Modification in the Biological Functions and Diseases. Sig Transduct. Target Ther. 6 (1), 74. doi:10.1038/s41392-020-00450-x
Jiang, Z., Jacob, J. A., Loganathachetti, D. S., Nainangu, P., and Chen, B. (2017). β-Elemene: Mechanistic Studies on Cancer Cell Interaction and its Chemosensitization Effect. Front. Pharmacol. 8, 105. doi:10.3389/fphar.2017.00105
Jin, D., Guo, J., Wu, Y., Du, J., Yang, L., Wang, X., et al. (2019). m6A mRNA Methylation Initiated by METTL3 Directly Promotes YAP Translation and Increases YAP Activity by Regulating the MALAT1-miR-1914-3p-YAP axis to Induce NSCLC Drug Resistance and Metastasis. J. Hematol. Oncol. 12 (1), 135. doi:10.1186/s13045-019-0830-6
Kan, L., Grozhik, A. V., Vedanayagam, J., Patil, D. P., Pang, N., Lim, K. S., et al. (2017). The m6A Pathway Facilitates Sex Determination in Drosophila. Nat. Commun. 8, 15737. doi:10.1038/ncomms15737
Kaushik, S., Shyam, H., Sharma, R., and Balapure, A. K. (2018). Dietary Isoflavone Daidzein Synergizes Centchroman Action via Induction of Apoptosis and Inhibition of PI3K/Akt Pathway in MCF-7/MDA MB-231 Human Breast Cancer Cells. Phytomedicine 40, 116–124. doi:10.1016/j.phymed.2018.01.007
Kobayashi, M., Ohsugi, M., Sasako, T., Awazawa, M., Umehara, T., Iwane, A., et al. (2018). The RNA Methyltransferase Complex of WTAP, METTL3, and METTL14 Regulates Mitotic Clonal Expansion in Adipogenesis. Mol. Cell Biol. 38 (16), e00116–18. doi:10.1128/MCB.00116-18
Koh, C. W. Q., Goh, Y. T., and Goh, W. S. S. (2019). Atlas of Quantitative single-Base-Resolution N6-Methyl-Adenine Methylomes. Nat. Commun. 10 (1), 5636. doi:10.1038/s41467-019-13561-z
Lai, W., Jia, J., Yan, B., Jiang, Y., Shi, Y., Chen, L., et al. (2018). Baicalin Hydrate Inhibits Cancer Progression in Nasopharyngeal Carcinoma by Affecting Genome Instability and Splicing. Oncotarget 9 (1), 901–914. doi:10.18632/oncotarget.22868
Lan, T., Li, H., Zhang, D., Xu, L., Liu, H., Hao, X., et al. (2019). KIAA1429 Contributes to Liver Cancer Progression through N6-methyladenosine-dependent Post-transcriptional Modification of GATA3. Mol. Cancer 18 (1), 186. doi:10.1186/s12943-019-1106-z
Li, J., Wang, F., Liu, Y., Wang, H., and Ni, B. (2021). N6-methyladenosine (m6A) in Pancreatic Cancer: Regulatory Mechanisms and Future Direction. Int. J. Biol. Sci. 17 (9), 2323–2335. doi:10.7150/ijbs.60115
Li, L., Dong, Z., Shi, P., Tan, L., Xu, J., Huang, P., et al. (2020a). Bruceine D Inhibits Cell Proliferation through Downregulating LINC01667/MicroRNA-138-5p/Cyclin E1 Axis in Gastric Cancer. Front. Pharmacol. 11, 584960. doi:10.3389/fphar.2020.584960
Li, N., Kang, Y., Wang, L., Huff, S., Tang, R., Hui, H., et al. (2020b). ALKBH5 Regulates Anti-PD-1 Therapy Response by Modulating Lactate and Suppressive Immune Cell Accumulation in Tumor Microenvironment. Proc. Natl. Acad. Sci. U. S. A. 117 (33), 20159–20170. doi:10.1073/pnas.1918986117
Li, Q. Q., Lee, R. X., Liang, H., Wang, G., Li, J. M., Zhong, Y., et al. (2013). β-Elemene Enhances Susceptibility to Cisplatin in Resistant Ovarian Carcinoma Cells via Downregulation of ERCC-1 and XIAP and Inactivation of JNK. Int. J. Oncol. 43 (3), 721–728. doi:10.3892/ijo.2013.1996
Li, W., Hu, X., Wang, S., Jiao, Z., Sun, T., Liu, T., et al. (2020c). Characterization and Anti-tumor Bioactivity of astragalus Polysaccharides by Immunomodulation. Int. J. Biol. Macromol. 145, 985–997. doi:10.1016/j.ijbiomac.2019.09.189
Li, Z., Weng, H., Su, R., Weng, X., Zuo, Z., Li, C., et al. (2017). FTO Plays an Oncogenic Role in Acute Myeloid Leukemia as a N6-Methyladenosine RNA Demethylase. Cancer Cell 31 (1), 127–141. doi:10.1016/j.ccell.2016.11.017
Liang, D., Lin, W. J., Ren, M., Qiu, J., Yang, C., Wang, X., et al. (2021). m6A Reader YTHDC1 Modulates Autophagy by Targeting SQSTM1 in Diabetic Skin. Autophagy 1, 1–20. doi:10.1080/15548627.2021.1974175
Lin, S., Choe, J., Du, P., Triboulet, R., and Gregory, R. I. (2016). The M(6)A Methyltransferase METTL3 Promotes Translation in Human Cancer Cells. Mol. Cell 62 (3), 335–345. doi:10.1016/j.molcel.2016.03.021
Liu, M., Zhao, G., Cao, S., Zhang, Y., Li, X., and Lin, X. (2016). Development of Certain Protein Kinase Inhibitors with the Components from Traditional Chinese Medicine. Front. Pharmacol. 7, 523. doi:10.3389/fphar.2016.00523
Liu, N., Zhou, K. I., Parisien, M., Dai, Q., Diatchenko, L., and Pan, T. (2017). N6-methyladenosine Alters RNA Structure to Regulate Binding of a Low-Complexity Protein. Nucleic Acids Res. 45 (10), 6051–6063. doi:10.1093/nar/gkx141
Liu, S., Li, Q., Li, G., Zhang, Q., Zhuo, L., Han, X., et al. (2020). The Mechanism of m6A Methyltransferase METTL3-Mediated Autophagy in Reversing Gefitinib Resistance in NSCLC Cells by β-elemene. Cell Death Dis. 11 (11), 969. doi:10.1038/s41419-020-03148-8
Luo, X., Cao, M., Gao, F., and He, X. (2021). YTHDF1 Promotes Hepatocellular Carcinoma Progression via Activating PI3K/AKT/mTOR Signaling Pathway and Inducing Epithelial-Mesenchymal Transition. Exp. Hematol. Oncol. 10 (1), 35. doi:10.1186/s40164-021-00227-0
Ma, J. Z., Yang, F., Zhou, C. C., Liu, F., Yuan, J. H., Wang, F., et al. (2017). METTL14 Suppresses the Metastatic Potential of Hepatocellular Carcinoma by Modulating N6 -methyladenosine-dependent Primary MicroRNA Processing. Hepatology 65 (2), 529–543. doi:10.1002/hep.28885
Ma, L., Chen, T., Zhang, X., Miao, Y., Tian, X., Yu, K., et al. (2021a). The m6A Reader YTHDC2 Inhibits Lung Adenocarcinoma Tumorigenesis by Suppressing SLC7A11-dependent Antioxidant Function. Redox Biol. 38, 101801. doi:10.1016/j.redox.2020.101801
Ma, L., Zhang, X., Yu, K., Xu, X., Chen, T., Shi, Y., et al. (2021b). Targeting SLC3A2 Subunit of System XC- Is Essential for m6A Reader YTHDC2 to Be an Endogenous Ferroptosis Inducer in Lung Adenocarcinoma. Free Radic. Biol. Med. 168, 25–43. doi:10.1016/j.freeradbiomed.2021.03.023
Merritt, D. C., Jamnik, J., and El-Sohemy, A. (2018). FTO Genotype, Dietary Protein Intake, and Body Weight in a Multiethnic Population of Young Adults: a Cross-Sectional Study. Genes Nutr. 13, 4. doi:10.1186/s12263-018-0593-7
Meyer, K. D. (2019). DART-seq: an Antibody-free Method for Global m6A Detection. Nat. Methods 16 (12), 1275–1280. doi:10.1038/s41592-019-0570-0
Mortezaee, K., Salehi, E., Mirtavoos-Mahyari, H., Motevaseli, E., Najafi, M., Farhood, B., et al. (2019). Mechanisms of Apoptosis Modulation by Curcumin: Implications for Cancer Therapy. J. Cell Physiol. 234 (8), 12537–12550. doi:10.1002/jcp.28122
Müller, S., Glass, M., Singh, A. K., Haase, J., Bley, N., Fuchs, T., et al. (2019). IGF2BP1 Promotes SRF-dependent Transcription in Cancer in a m6A- and miRNA-dependent Manner. Nucleic Acids Res. 47 (1), 375–390. doi:10.1093/nar/gky1012
Nettersheim, D., Berger, D., Jostes, S., Kristiansen, G., Lochnit, G., and Schorle, H. (2019). N6-Methyladenosine Detected in RNA of Testicular Germ Cell Tumors Is Controlled by METTL3, ALKBH5, YTHDC1/F1/F2, and HNRNPC as Writers, Erasers, and Readers. Andrology 7 (4), 498–506. doi:10.1111/andr.12612
Newman, D. J., and Cragg, G. M. (2016). Natural Products as Sources of New Drugs from 1981 to 2014. J. Nat. Prod. 79 (3), 629–661. doi:10.1021/acs.jnatprod.5b01055
Ning, Y., Chen, J., Shi, Y., Song, N., Yu, X., Fang, Y., et al. (2020). Genistein Ameliorates Renal Fibrosis through Regulation Snail via m6A RNA Demethylase ALKBH5. Front. Pharmacol. 11, 579265. doi:10.3389/fphar.2020.579265
Niu, Y., Lin, Z., Wan, A., Chen, H., Liang, H., Sun, L., et al. (2019). RNA N6-Methyladenosine Demethylase FTO Promotes Breast Tumor Progression through Inhibiting BNIP3. Mol. Cancer 18 (1), 46. doi:10.1186/s12943-019-1004-4
Niu, Y., Wan, A., Lin, Z., Lu, X., and Wan, G. (2018). N 6-Methyladenosine Modification: a Novel Pharmacological Target for Anti-cancer Drug Development. Acta Pharm. Sin. B 8 (6), 833–843. doi:10.1016/j.apsb.2018.06.001
Onitsuka, T., Uramoto, H., Nose, N., Takenoyama, M., Hanagiri, T., Sugio, K., et al. (2010). Acquired Resistance to Gefitinib: the Contribution of Mechanisms Other Than the T790M, MET, and HGF Status. Lung Cancer 68 (2), 198–203. doi:10.1016/j.lungcan.2009.05.022
Pan, H., Pan, Z., Guo, F., Meng, F., Zu, L., Fan, Y., et al. (2021a). MicroRNA-1915-3p Inhibits Cell Migration and Invasion by Targeting SET in Non-small-cell Lung Cancer. BMC Cancer 21 (1), 1218. doi:10.1186/s12885-021-08961-8
Pan, Z. P., Wang, B., Hou, D. Y., You, R. L., Wang, X. T., Xie, W. H., et al. (2021b). METTL3 Mediates Bone Marrow Mesenchymal Stem Cell Adipogenesis to Promote Chemoresistance in Acute Myeloid Leukaemia. FEBS Open Bio 11 (6), 1659–1672. doi:10.1002/2211-5463.13165
Park, S., Bazer, F. W., Lim, W., and Song, G. (2018). The O-Methylated Isoflavone, Formononetin, Inhibits Human Ovarian Cancer Cell Proliferation by Sub G0/G1 Cell Phase Arrest through PI3K/AKT and ERK1/2 Inactivation. J. Cell Biochem. 119 (9), 7377–7387. doi:10.1002/jcb.27041
Patil, D. P., Chen, C. K., Pickering, B. F., Chow, A., Jackson, C., Guttman, M., et al. (2016). m(6)A RNA Methylation Promotes XIST-Mediated Transcriptional Repression. Nature 537 (7620), 369–373. doi:10.1038/nature19342
Ping, X. L., Sun, B. F., Wang, L., Xiao, W., Yang, X., Wang, W. J., et al. (2014). Mammalian WTAP Is a Regulatory Subunit of the RNA N6-Methyladenosine Methyltransferase. Cell Res. 24 (2), 177–189. doi:10.1038/cr.2014.3
Pu, J., Wang, J., Qin, Z., Wang, A., Zhang, Y., Wu, X., et al. (2020). IGF2BP2 Promotes Liver Cancer Growth through an m6A-FEN1-dependent Mechanism. Front. Oncol. 10, 578816. doi:10.3389/fonc.2020.578816
Qian, X., Yang, J., Qiu, Q., Li, X., Jiang, C., Li, J., et al. (2021a). LCAT3, a Novel m6A-Regulated Long Non-coding RNA, Plays an Oncogenic Role in Lung Cancer via Binding with FUBP1 to Activate C-MYC. J. Hematol. Oncol. 14 (1), 112. doi:10.1186/s13045-021-01123-0
Qian, Y., Wang, R., Wei, W., Wang, M., and Wang, S. (2021b). Resveratrol Reverses the Cadmium-Promoted Migration, Invasion, and Epithelial-Mesenchymal Transition Procession by Regulating the Expression of ZEB1. Hum. Exp. Toxicol. 40 (12_Suppl. l), S331–S338. doi:10.1177/09603271211041678
Rauf, A., Imran, M., Butt, M. S., Nadeem, M., Peters, D. G., and Mubarak, M. S. (2018a). Resveratrol as an Anti-cancer Agent: A Review. Crit. Rev. Food Sci. Nutr. 58 (9), 1428–1447. doi:10.1080/10408398.2016.1263597
Rauf, A., Imran, M., Khan, I. A., Ur-Rehman, M., Gilani, S. A., Mehmood, Z., et al. (2018b). Anticancer Potential of Quercetin: A Comprehensive Review. Phytother. Res. 32 (11), 2109–2130. doi:10.1002/ptr.6155
Ren, W., Lu, J., Huang, M., Gao, L., Li, D., Wang, G. G., et al. (2019). Structure and Regulation of ZCCHC4 in m6A-Methylation of 28S rRNA. Nat. Commun. 10 (1), 5042. doi:10.1038/s41467-019-12923-x
Rong, B., Zhang, Q., Wan, J., Xing, S., Dai, R., Li, Y., et al. (2020). Ribosome 18S m6A Methyltransferase METTL5 Promotes Translation Initiation and Breast Cancer Cell Growth. Cell Rep. 33 (12), 108544. doi:10.1016/j.celrep.2020.108544
Roundtree, I. A., Evans, M. E., Pan, T., and He, C. (2017a). Dynamic RNA Modifications in Gene Expression Regulation. Cell 169 (7), 1187–1200. doi:10.1016/j.cell.2017.05.045
Roundtree, I. A., Luo, G. Z., Zhang, Z., Wang, X., Zhou, T., Cui, Y., et al. (2017b). YTHDC1 Mediates Nuclear Export of N6-Methyladenosine Methylated mRNAs. Elife 6, e31311. doi:10.7554/eLife.31311
Roy, S., Sil, A., and Chakraborty, T. (2019). Potentiating Apoptosis and Modulation of P53, Bcl2, and Bax by a Novel Chrysin Ruthenium Complex for Effective Chemotherapeutic Efficacy against Breast Cancer. J. Cell Physiol. 234 (4), 4888–4909. doi:10.1002/jcp.27287
Sahm, B. D. B., Peres, J., Rezende-Teixeira, P., Santos, E. A., Branco, P. C., Bauermeister, A., et al. (2020). Targeting the Oncogenic TBX2 Transcription Factor with Chromomycins. Front. Chem. 8, 110. doi:10.3389/fchem.2020.00110
Sang, L., Wu, X., Yan, T., Naren, D., Liu, X., Zheng, X., et al. (2022). The m6A RNA Methyltransferase METTL3/METTL14 Promotes Leukemogenesis through the Mdm2/p53 Pathway in Acute Myeloid Leukemia. J. Cancer 13 (3), 1019–1030. doi:10.7150/jca.60381
Sarkar, F. H., Adsule, S., Padhye, S., Kulkarni, S., and Li, Y. (2006). The Role of Genistein and Synthetic Derivatives of Isoflavone in Cancer Prevention and Therapy. Mini Rev. Med. Chem. 6 (4), 401–407. doi:10.2174/138955706776361439
Shah, A., Rashid, F., Awan, H. M., Hu, S., Wang, X., Chen, L., et al. (2017). The DEAD-Box RNA Helicase DDX3 Interacts with m6A RNA Demethylase ALKBH5. Stem Cells Int. 2017, 8596135. doi:10.1155/2017/8596135
Shen, C., Sheng, Y., Zhu, A. C., Robinson, S., Jiang, X., Dong, L., et al. (2020). RNA Demethylase ALKBH5 Selectively Promotes Tumorigenesis and Cancer Stem Cell Self-Renewal in Acute Myeloid Leukemia. Cell Stem Cell 27 (1), 64–e9. e69. doi:10.1016/j.stem.2020.04.009
Sheng, Y., Wei, J., Yu, F., Xu, H., Yu, C., Wu, Q., et al. (2021). A Critical Role of Nuclear m6A Reader YTHDC1 in Leukemogenesis by Regulating MCM Complex-Mediated DNA Replication. Blood 138 (26), 2838–2852. doi:10.1182/blood.2021011707
Shi, H., Wang, X., Lu, Z., Zhao, B. S., Ma, H., Hsu, P. J., et al. (2017). YTHDF3 Facilitates Translation and Decay of N6-Methyladenosine-Modified RNA. Cell Res. 27 (3), 315–328. doi:10.1038/cr.2017.15
Shi, H., Wei, J., and He, C. (2019). Where, when, and How: Context-dependent Functions of RNA Methylation Writers, Readers, and Erasers. Mol. Cell 74 (4), 640–650. doi:10.1016/j.molcel.2019.04.025
Shi, Y., Zheng, C., Jin, Y., Bao, B., Wang, D., Hou, K., et al. (2020). Reduced Expression of METTL3 Promotes Metastasis of Triple-Negative Breast Cancer by m6A Methylation-Mediated COL3A1 Up-Regulation. Front. Oncol. 10, 1126. doi:10.3389/fonc.2020.01126
Singla, R. K., Behzad, S., Khan, J., Tsagkaris, C., Gautam, R. K., Goyal, R., et al. (2022). Natural Kinase Inhibitors for the Treatment and Management of Endometrial/Uterine Cancer: Preclinical to Clinical Studies. Front. Pharmacol. 13, 801733. doi:10.3389/fphar.2022.801733
Singla, R. K., Sai, C. S., Chopra, H., Behzad, S., Bansal, H., Goyal, R., et al. (2021a). Natural Products for the Management of Castration-Resistant Prostate Cancer: Special Focus on Nanoparticles Based Studies. Front. Cell Dev. Biol. 9, 745177. doi:10.3389/fcell.2021.745177
Singla, R. K., Sharma, P., Dubey, A. K., Gundamaraju, R., Kumar, D., Kumar, S., et al. (2021b). Natural Product-Based Studies for the Management of Castration-Resistant Prostate Cancer: Computational to Clinical Studies. Front. Pharmacol. 12, 732266. doi:10.3389/fphar.2021.732266
Su, R., Dong, L., Li, Y., Gao, M., He, P. C., Liu, W., et al. (2022). METTL16 Exerts an m6A-independent Function to Facilitate Translation and Tumorigenesis. Nat. Cell Biol. 24 (2), 205–216. doi:10.1038/s41556-021-00835-2
Sun, C., Gao, W., Liu, J., Cheng, H., and Hao, J. (2020). FGL1 Regulates Acquired Resistance to Gefitinib by Inhibiting Apoptosis in Non-small Cell Lung Cancer. Respir. Res. 21 (1), 210. doi:10.1186/s12931-020-01477-y
Sun, T., Wu, R., and Ming, L. (2019). The Role of m6A RNA Methylation in Cancer. Biomed. Pharmacother. 112, 108613. doi:10.1016/j.biopha.2019.108613
Suthar, A. C., Banavalikar, M. M., and Biyani, M. K. (2001). Pharmacological Activities of Genistein, an Isoflavone from Soy (Glycine Max): Part I-AantiCcancer Activity. Indian J. Exp. Biol. 39 (6), 511–519.
Syed, D. N., Adhami, V. M., Khan, N., Khan, M. I., and Mukhtar, H. (2016). Exploring the Molecular Targets of Dietary Flavonoid Fisetin in Cancer. Semin. Cancer Biol. 40-41, 130–140. doi:10.1016/j.semcancer.2016.04.003
Tan, X., Fu, J., Yuan, Z., Zhu, L., and Fu, L. (2021). ACNPD: The Database for Elucidating the Relationships between Natural Products, Compounds, Molecular Mechanisms, and Cancer Types. Front. Pharmacol. 12, 746067. doi:10.3389/fphar.2021.746067
Tanabe, A., Tanikawa, K., Tsunetomi, M., Takai, K., Ikeda, H., Konno, J., et al. (2016). RNA Helicase YTHDC2 Promotes Cancer Metastasis via the Enhancement of the Efficiency by Which HIF-1α mRNA Is Translated. Cancer Lett. 376 (1), 34–42. doi:10.1016/j.canlet.2016.02.022
UniProt, C. (2019). UniProt: a Worldwide Hub of Protein Knowledge. Nucleic Acids Res. 47 (D1), D506–D515. doi:10.1093/nar/gky1049
Uyar, B., Yusuf, D., Wurmus, R., Rajewsky, N., Ohler, U., and Akalin, A. (2017). RCAS: an RNA Centric Annotation System for Transcriptome-wide Regions of Interest. Nucleic Acids Res. 45 (10), e91. doi:10.1093/nar/gkx120
van Tran, N., Ernst, F. G. M., Hawley, B. R., Zorbas, C., Ulryck, N., Hackert, P., et al. (2019). The Human 18S rRNA m6A Methyltransferase METTL5 Is Stabilized by TRMT112. Nucleic Acids Res. 47 (15), 7719–7733. doi:10.1093/nar/gkz619
Vu, L. P., Pickering, B. F., Cheng, Y., Zaccara, S., Nguyen, D., Minuesa, G., et al. (2017). The N6-Methyladenosine (m6A)-Forming Enzyme METTL3 Controls Myeloid Differentiation of Normal Hematopoietic and Leukemia Cells. Nat. Med. 23 (11), 1369–1376. doi:10.1038/nm.4416
Wan, W., Ao, X., Chen, Q., Yu, Y., Ao, L., Xing, W., et al. (2022). METTL3/IGF2BP3 axis Inhibits Tumor Immune Surveillance by Upregulating N6-Methyladenosine Modification of PD-L1 mRNA in Breast Cancer. Mol. Cancer 21 (1), 60. doi:10.1186/s12943-021-01447-y
Wang, C. Z., Zhang, C. F., Chen, L., Anderson, S., Lu, F., and Yuan, C. S. (2015). Colon Cancer Chemopreventive Effects of Baicalein, an Active Enteric Microbiome Metabolite from Baicalin. Int. J. Oncol. 47 (5), 1749–1758. doi:10.3892/ijo.2015.3173
Wang, H., Xu, B., and Shi, J. (2020a). N6-methyladenosine METTL3 Promotes the Breast Cancer Progression via Targeting Bcl-2. Gene 722, 144076. doi:10.1016/j.gene.2019.144076
Wang, H., Zhang, K., Liu, J., Yang, J., Tian, Y., Yang, C., et al. (2021). Curcumin Regulates Cancer Progression: Focus on ncRNAs and Molecular Signaling Pathways. Front. Oncol. 11, 660712. doi:10.3389/fonc.2021.660712
Wang, J., Li, Y., Wang, P., Han, G., Zhang, T., Chang, J., et al. (2020b). Leukemogenic Chromatin Alterations Promote AML Leukemia Stem Cells via a KDM4C-ALKBH5-AXL Signaling Axis. Cell Stem Cell 27 (1), 81–e8. e88. doi:10.1016/j.stem.2020.04.001
Wang, J., Wang, J., Gu, Q., Ma, Y., Yang, Y., Zhu, J., et al. (2020c). The Biological Function of m6A Demethylase ALKBH5 and its Role in Human Disease. Cancer Cell Int. 20, 347. doi:10.1186/s12935-020-01450-1
Wang, J. Y., Chen, L. J., and Qiang, P. (2020d). The Potential Role of N6-Methyladenosine (m6A) Demethylase Fat Mass and Obesity-Associated Gene (FTO) in Human Cancers. Onco Targets Ther. 13, 12845–12856. doi:10.2147/OTT.S283417
Wang, T., Kong, S., Tao, M., and Ju, S. (2020e). The Potential Role of RNA N6-Methyladenosine in Cancer Progression. Mol. Cancer 19 (1), 88. doi:10.1186/s12943-020-01204-7
Wang, Y., Chen, J., Gao, W. Q., and Yang, R. (2022). METTL14 Promotes Prostate Tumorigenesis by Inhibiting THBS1 via an m6A-YTHDF2-dependent Mechanism. Cell Death Discov. 8 (1), 143. doi:10.1038/s41420-022-00939-0
Wang, Y., Li, Y., Yue, M., Wang, J., Kumar, S., Wechsler-Reya, R. J., et al. (2018). N6-methyladenosine RNA Modification Regulates Embryonic Neural Stem Cell Self-Renewal through Histone Modifications. Nat. Neurosci. 21 (2), 195–206. doi:10.1038/s41593-017-0057-1
Wanna-Udom, S., Terashima, M., Lyu, H., Ishimura, A., Takino, T., Sakari, M., et al. (2020). The m6A Methyltransferase METTL3 Contributes to Transforming Growth Factor-Beta-Induced Epithelial-Mesenchymal Transition of Lung Cancer Cells through the Regulation of JUNB. Biochem. Biophys. Res. Commun. 524 (1), 150–155. doi:10.1016/j.bbrc.2020.01.042
Wei, J., Liu, F., Lu, Z., Fei, Q., Ai, Y., He, P. C., et al. (2018). Differential m6A, m6Am, and m1A Demethylation Mediated by FTO in the Cell Nucleus and Cytoplasm. Mol. Cell 71 (6), 973–e5. doi:10.1016/j.molcel.2018.08.011
Wong, A. S., Che, C. M., and Leung, K. W. (2015). Recent Advances in Ginseng as Cancer Therapeutics: a Functional and Mechanistic Overview. Nat. Prod. Rep. 32 (2), 256–272. doi:10.1039/c4np00080c
Wu, H., Li, F., and Zhu, R. (2021a). miR-338-5p Inhibits Cell Growth and Migration via Inhibition of the METTL3/m6A/c-Myc Pathway in Lung Cancer. Acta Biochim. Biophys. Sin. (Shanghai) 53 (3), 304–316. doi:10.1093/abbs/gmaa170
Wu, J., Gan, Z., Zhuo, R., Zhang, L., Wang, T., and Zhong, X. (2020). Resveratrol Attenuates Aflatoxin B1-Induced ROS Formation and Increase of m6A RNA Methylation. Anim. (Basel) 10 (4), 677. doi:10.3390/ani10040677
Wu, Y., Chang, N., Zhang, Y., Zhang, X., Xu, L., Che, Y., et al. (2021b). METTL3-mediated m6A mRNA Modification of FBXW7 Suppresses Lung Adenocarcinoma. J. Exp. Clin. Cancer Res. 40 (1), 90. doi:10.1186/s13046-021-01880-3
Wu, Y., Chen, X., Bao, W., Hong, X., Li, C., Lu, J., et al. (2022). Effect of Humantenine on mRNA m6A Modification and Expression in Human Colon Cancer Cell Line HCT116. Genes 13 (5), 781. doi:10.3390/genes13050781
Xia, Y., Lian, S., Khoi, P. N., Yoon, H. J., Han, J. Y., Chay, K. O., et al. (2015a). Chrysin Inhibits Cell Invasion by Inhibition of Recepteur D'origine Nantais via Suppressing Early Growth Response-1 and NF-Κb Transcription Factor Activities in Gastric Cancer Cells. Int. J. Oncol. 46 (4), 1835–1843. doi:10.3892/ijo.2015.2847
Xia, Y., Lian, S., Khoi, P. N., Yoon, H. J., Joo, Y. E., Chay, K. O., et al. (2015b). Chrysin Inhibits Tumor Promoter-Induced MMP-9 Expression by Blocking AP-1 via Suppression of ERK and JNK Pathways in Gastric Cancer Cells. PLoS One 10 (4), e0124007. doi:10.1371/journal.pone.0124007
Xiao, W., Adhikari, S., Dahal, U., Chen, Y. S., Hao, Y. J., Sun, B. F., et al. (2016). Nuclear M(6)A Reader YTHDC1 Regulates mRNA Splicing. Mol. Cell 61 (4), 507–519. doi:10.1016/j.molcel.2016.01.012
Xie, J., Ba, J., Zhang, M., Wan, Y., Jin, Z., and Yao, Y. (2021). The m6A Methyltransferase METTL3 Promotes the Stemness and Malignant Progression of Breast Cancer by Mediating m6A Modification on SOX2. J. BUON 26 (2), 444–449.
Xu, X., Zhou, E., Zheng, J., Zhang, C., Zou, Y., Lin, J., et al. (2021). Prognostic and Predictive Value of m6A "Eraser" Related Gene Signature in Gastric Cancer. Front. Oncol. 11, 631803. doi:10.3389/fonc.2021.631803
Xu, Y., Lv, D., Yan, C., Su, H., Zhang, X., Shi, Y., et al. (2022). METTL3 Promotes Lung Adenocarcinoma Tumor Growth and Inhibits Ferroptosis by Stabilizing SLC7A11 m6A Modification. Cancer Cell Int. 22 (1), 11. doi:10.1186/s12935-021-02433-6
Xu, Y., Ye, S., Zhang, N., Zheng, S., Liu, H., Zhou, K., et al. (2020). The FTO/miR-181b-3p/ARL5B Signaling Pathway Regulates Cell Migration and Invasion in Breast Cancer. Cancer Commun. (Lond) 40 (10), 484–500. doi:10.1002/cac2.12075
Xue, L., Li, J., Lin, Y., Liu, D., Yang, Q., Jian, J., et al. (2021). m6 A Transferase METTL3-Induced lncRNA ABHD11-AS1 Promotes the Warburg Effect of Non-small-cell Lung Cancer. J. Cell Physiol. 236 (4), 2649–2658. doi:10.1002/jcp.30023
Xue, M., Zhao, B. S., Zhang, Z., Lu, M., Harder, O., Chen, P., et al. (2019). Viral N6-Methyladenosine Upregulates Replication and Pathogenesis of Human Respiratory Syncytial Virus. Nat. Commun. 10 (1), 4595. doi:10.1038/s41467-019-12504-y
Yang, S., Wei, J., Cui, Y. H., Park, G., Shah, P., Deng, Y., et al. (2019). m6A mRNA Demethylase FTO Regulates Melanoma Tumorigenicity and Response to Anti-PD-1 Blockade. Nat. Commun. 10 (1), 2782. doi:10.1038/s41467-019-10669-0
Yang, Z., Yang, S., Cui, Y.-H., Wei, J., Shah, P., Park, G., et al. (2021). METTL14 Facilitates Global Genome Repair and Suppresses Skin Tumorigenesis. Proc. Natl. Acad. Sci. U.S.A. 118 (35), e2025948118. doi:10.1073/pnas.2025948118
Yao, L. C., Wu, L., Wang, W., Zhai, L. L., Ye, L., Xiang, F., et al. (2021). Panax Notoginseng Saponins Promote Cell Death and Chemosensitivity in Pancreatic Cancer through the Apoptosis and Autophagy Pathways. Anticancer Agents Med. Chem. 21 (13), 1680–1688. doi:10.2174/1871520620999201110191459
Yue, Y., Liu, J., Cui, X., Cao, J., Luo, G., Zhang, Z., et al. (2018). VIRMA Mediates Preferential m6A mRNA Methylation in 3'UTR and Near Stop Codon and Associates with Alternative Polyadenylation. Cell Discov. 4, 10. doi:10.1038/s41421-018-0019-0
Zannella, C., Rinaldi, L., Boccia, G., Chianese, A., Sasso, F. C., De Caro, F., et al. (2021). Regulation of m6A Methylation as a New Therapeutic Option against COVID-19. Pharm. (Basel) 14 (11), 1135. doi:10.3390/ph14111135
Zhang, C., Fu, J., and Zhou, Y. (2019). A Review in Research Progress Concerning m6A Methylation and Immunoregulation. Front. Immunol. 10, 922. doi:10.3389/fimmu.2019.00922
Zhang, H., Zhao, L., Li, S., Wang, J., Feng, C., Li, T., et al. (2021a). N6-Methylandenosine-Related lncRNAs in Tumor Microenvironment Are Potential Prognostic Biomarkers in Colon Cancer. Front. Oncol. 11, 697949. doi:10.3389/fonc.2021.697949
Zhang, X., Liu, X., Zhang, Y., Yang, A., Zhang, Y., Tong, Z., et al. (2021b). Wan-Nian-Qing, a Herbal Composite Prescription, Suppresses the Progression of Liver Cancer in Mice by Regulating Immune Response. Front. Oncol. 11, 696282. doi:10.3389/fonc.2021.696282
Zhang, Y., Liu, S., Zhao, T., and Dang, C. (2021c). METTL3-mediated m6A M-odification of Bcl-2 mRNA P-romotes N-on-small C-ell L-ung C-ancer P-rogression. Oncol. Rep. 46 (2), 163. doi:10.3892/or.2021.8114
Zhang, Y., and Yang, Y. (2021). Effects of m6A RNA Methylation Regulators on Endometrial Cancer. J. Clin. Lab. Anal. 35 (9), e23942. doi:10.1002/jcla.23942
Zhao, C., Ling, X., Xia, Y., Yan, B., and Guan, Q. (2021). The m6A Methyltransferase METTL3 Controls Epithelial-Mesenchymal Transition, Migration and Invasion of Breast Cancer through the MALAT1/miR-26b/HMGA2 axis. Cancer Cell Int. 21 (1), 441. doi:10.1186/s12935-021-02113-5
Zheng, G., Dahl, J. A., Niu, Y., Fedorcsak, P., Huang, C. M., Li, C. J., et al. (2013). ALKBH5 Is a Mammalian RNA Demethylase that Impacts RNA Metabolism and Mouse Fertility. Mol. Cell 49 (1), 18–29. doi:10.1016/j.molcel.2012.10.015
Zhou, R. T., He, M., Yu, Z., Liang, Y., Nie, Y., Tai, S., et al. (2017). Baicalein Inhibits Pancreatic Cancer Cell Proliferation and Invasion via Suppression of NEDD9 Expression and its Downstream Akt and ERK Signaling Pathways. Oncotarget 8 (34), 56351–56363. doi:10.18632/oncotarget.16912
Zhu, S., Wang, J. Z., Chen, D., He, Y. T., Meng, N., Chen, M., et al. (2020a). An Oncopeptide Regulates m6A Recognition by the m6A Reader IGF2BP1 and Tumorigenesis. Nat. Commun. 11 (1), 1685. doi:10.1038/s41467-020-15403-9
Zhu, W., Si, Y., Xu, J., Lin, Y., Wang, J. Z., Cao, M., et al. (2020b). Methyltransferase like 3 Promotes Colorectal Cancer Proliferation by Stabilizing CCNE1 mRNA in an m6A-dependent Manner. J. Cell Mol. Med. 24 (6), 3521–3533. doi:10.1111/jcmm.15042
Keywords: m6A, RNA methylation, molecular function, signaling pathway, implication, nature products, anti-cancer
Citation: Song N, Cui K, Zhang K, Yang J, Liu J, Miao Z, Zhao F, Meng H, Chen L, Chen C, Li Y, Shao M, Zhang J and Wang H (2022) The Role of m6A RNA Methylation in Cancer: Implication for Nature Products Anti-Cancer Research. Front. Pharmacol. 13:933332. doi: 10.3389/fphar.2022.933332
Received: 30 April 2022; Accepted: 27 May 2022;
Published: 16 June 2022.
Edited by:
Yue Hou, Northeastern University, ChinaReviewed by:
Kunqi Chen, Fujian Medical University, ChinaWeili Miao, Stanford University, United States
Jiangbo Wei, The University of Chicago, United States
Divya Jha, Icahn School of Medicine at Mount Sinai, United States
Copyright © 2022 Song, Cui, Zhang, Yang, Liu, Miao, Zhao, Meng, Chen, Chen, Li, Shao, Zhang and Wang. This is an open-access article distributed under the terms of the Creative Commons Attribution License (CC BY). The use, distribution or reproduction in other forums is permitted, provided the original author(s) and the copyright owner(s) are credited and that the original publication in this journal is cited, in accordance with accepted academic practice. No use, distribution or reproduction is permitted which does not comply with these terms.
*Correspondence: Jinghang Zhang, amluZ2hhbmd6aGFuZ194eG11QDE2My5jb20=; Haijun Wang, d25hdnkyMDAyOTlAMTYzLmNvbQ==