- 1Center for DMPK Research of Herbal Medicines, Institute of Chinese Materia Medica, China Academy of Chinese Medical Sciences, Beijing, China
- 2Department of Oncology, Beijing Hospital, National Center of Gerontology, Institute of Geriatric Medicine, Chinese Academy of Medical Sciences, Beijing, China
- 3School of Traditional Chinese Medicine, Shandong University of Traditional Chinese Medicine, Jinan, China
- 4School of Chinese Pharmacy, Beijing University of Chinese Medicine, Beijing, China
- 5Department of Hepatobiliary Pancreatic Surgery, The Affiliated Hospital of Qingdao University, Qingdao, China
- 6The Key Laboratory of Geriatrics, Beijing Institute of Geriatrics, Institute of Geriatric Medicine, Chinese Academy of Medical Sciences, Beijing Hospital/National Center of Gerontology of National Health Commission, Beijing, China
Osimertinib is a third-generation epidermal growth factor receptor tyrosine kinase inhibitor (EGFR-TKI) and a star medication used to treat non-small-cell lung carcinomas (NSCLCs). It has caused broad public concern that osimertinib has relatively low stability in plasma. We explored why osimertinib and its primary metabolites AZ-5104 and AZ-7550 are unstable in rat plasma. Our results suggested that it is the main reason inducing their unstable phenomenon that the Michael addition reaction was putatively produced between the Michael acceptor of osimertinib and the cysteine in the plasma matrix. Consequently, we identified a method to stabilize osimertinib and its metabolite contents in plasma. The assay was observed to enhance the stability of osimertinib, AZ-5104, and AZ-7550 significantly. The validated method was subsequently applied to perform the pharmacokinetic study for osimertinib in rats with the newly established, elegant, and optimized ultra-performance liquid chromatography–tandem mass spectrometer (UPLC-MS/MS) strategy. The assay was assessed for accuracy, precision, matrix effects, recovery, and stability. This study can help understand the pharmacological effects of osimertinib and promote a solution for the similar problem of other Michael acceptor-contained third-generation EGFR-TKI.
1 Introduction
Non-small-cell lung cancer (NSCLC) is the largest pulmonary carcinoma subgroup of lung cancer and belongs to the most frequent malignant diseases (Xue et al., 2011; Gridelli et al., 2015). Also, the popular tyrosine kinase inhibitor (TKI) application has been well testified to benefit NSCLC patients with epidermal growth factor receptor (EGFR) mutations (Ke and Wu, 2016; Zhou et al., 2020). Osimertinib is a third-generation EGFR-TKI, selectively inhibits mutated EGFR (e.g., EGFR with exon 19 deletion, L858R, T790M, G719X, L861Q, and S768I), and has recently become the prominent star medicine for treating EGFR-mutated patients reaching a median progression-free survival (PFS) of 18.9 months (Remon et al., 2018; Soria et al., 2018; Cho et al., 2020; Floc’h et al., 2020). In addition, apart from the role of osimertinib as an anticancer drug, it has been found as a promising adjuvant agent to sensitize drug-resistant cancer cells to chemotherapy (Barbuti et al., 2019). Therefore, the analysis of osimertinib and its metabolites may promise better profiling of the distribution of components and enhance the understanding of the pharmacodynamics effects and toxicity of drugs in pre-clinic and clinic trials, and as a result in promoting osimertinib-related new drug research and development.
The osimertinib analysis in the matrix such as human serum, human plasma, human urine, rat plasma, and mouse brain has been constructed through liquid chromatography–tandem mass spectrometric (LC-MS) assay (Ballard et al., 2016; Rood et al., 2016; Irie et al., 2019; Mitchell et al., 2019; Fresnais et al., 2020; Xiong et al., 2022). However, the instability of osimertinib in plasma and whole blood at an ambient temperature drew attention recently. Rood et al. (2016) measured osimertinib in human plasma (either lithium heparin- or sodium EDTA-treated) stored at −30°C by salting-out assisted liquid–liquid extraction (LLE) using acetonitrile and magnesium sulfate (Rood et al., 2016). However, Veerman et al. (2019) found that the mass response of osimertinib decreased after incubation of plasma with osimertinib, whether at an ambient or higher temperature, thus pointing that both blood and plasma samples should be kept and processed solely on ice. This harsh environment may be uncomfortable for laboratory operators. In addition, Veelen et al. (2020) validated the sample preparation of human plasma containing osimertinib. Even if they pointed out that acidification of serum samples enhanced the stability of osimertinib, a lower temperature under 2°C was needed whether for storing or preparing the samples. A method is severely needed for storing and operating plasma containing osimertinib. Hence, a practical, convenient, and universal approach is urgently required for plasma preparation to keep the stability of osimertinib.
In the perspective of organic chemistry, whether osimertinib or its circulating metabolites, AZ-5104 and AZ-7550, contain the same Michael acceptor responsible for the covalent reaction with the cysteine-797 residue in the ATP-binding site of the EGFR kinase in vivo (Figure 1A) (Wu and Shih, 2018; Lategahn et al., 2019; Gao et al., 2022). Coincidentally, Dickinson et al. (2016) found that the remaining osimertinib decreased in the plasma of mice, rats, dogs or humans at 37°C for 6 h. Significantly, they found that human serum albumin (HSA) contributes to decreasing osimertinib during incubation. To the best of our understanding, serum albumin is the major protein component in mammal plasma (Minchiotti et al., 2008). In addition, we also noticed that the total cysteine concentration in human plasma is as high as 38,650 ng/ml (Wang et al., 2018). The evidence reviewed here suggests that a pertinent role of free or non-free cysteine in plasma may induce the reduction of osimertinib during the related investigation.
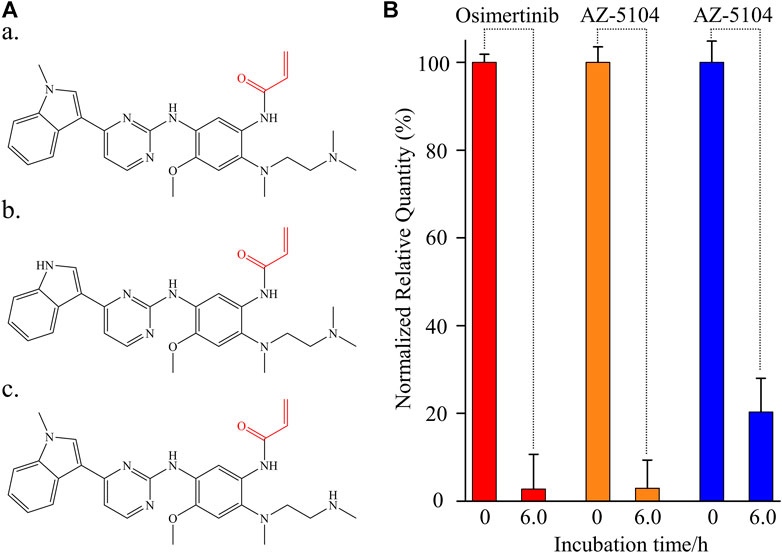
FIGURE 1. Instability of osimertinib, AZ-5104, and AZ-7550 in rat plasma. (A) Chemical structure of (a) osimertinib, (b) AZ-5104, and (c) AZ-7550. The Michael acceptor functional groups are labelled red. (B) Histogram of the normalized relative quantity of osimertinib (red), AZ-5104 (orange), and AZ-7550 (blue) after the incubation in rat plasma at 39°C for 0 and 6 h. The concentrations of analytes including propranolol in rat plasma were 10 ng/ml. The normalized relative quantity (NRQ) was calculated as the analyte/propranolol area ratio corresponding to the proposed incubation time of 0 h or 6 h over the area ratio for 0 h (n = 6). If not specified, the conditions listed upon adapted to the follow-up experiments.
This study seeks to understand and explain that cysteine’s potential role in plasma may affect the stability of osimertinib, AZ-5104, and AZ-7550 during the storage of plasma samples at room or higher temperatures. A strategy was applied to keep osimertinib stability by directly adding acetonitrile during preparation in plasma. In addition, we studied the oral pharmacokinetic study in five Sprague–Dawley (SD) rats using our newly validated UPLC-MS/MS coupling stability strategy. In general, we successfully set up an available and reliable method to increase osimertinib stability and apply it to the osimertinib pharmacokinetics study in rats.
2 Materials and Methods
2.1 Chemicals, Drugs, and Equipment
Osimertinib (purity 99.9%), AZ-5104 (purity 99.7%), and AZ-7550 hydrochloride (purity 99%) standards were purchased from MedChemExpress (Monmouth Junction, NJ, United States). Propranolol was used as inner standard (IS) during UPLC-MS/MS analysis and was bought from Sigma-Aldrich (Dorset, United Kingdom). Acetonitrile (ACN), formic acid (FA), methanol (MeOH), isopropanol (IPA), and dimethyl sulfoxide (DMSO) were all analytical reagents (ARs) provided by Merck (Darmstadt, Germany). L-Cysteine (Cys, 99%) was purchased from Bailingke (Haidian, Beijing, China). A TAGRISSO® (osimertinib) tablet (80 mg) produced by AstraZeneca (United States) was obtained from Beijing Hospital. Sodium carboxymethyl cellulose (CMC·Na) was supplied by Tianjin Chemical Reagent Company (Tianjin, China). 1 × PBS (0.01 M, pH 7.2–7.4, cell culture) was obtained from Solarbio (Beijing, China). Ethylenediaminetetraacetic acid disodium salt (EDTA·2Na) was purchased from National Pharmaceutical Group Chemical Reagent Company (Beijing, China). Male Sprague–Dawley (SD) rats (weighed 180–200 g) were provided by Beijing Vital River Laboratory Animal Technology Co., Ltd. (Beijing, China). All solutions were prepared using a Milli-Q system (Millipore, Massachusetts, United States) with an electrical resistivity of 18.2 MΩ except for L-cysteine using 1 × PBS instead. All solutions were filtered through a 0.45-mm filter. Two kinds of centrifugal filter units with a molecular weight cut-off (MWCO) of 3.0 kDa and 30 kDa were also bought from Millipore. An electronic thermostatic mixing water bath pot (DFD-700) was provided by Zhongxing (Beijing, China).
2.2 UPLC-MS/MS Instrumentation Setting and Operating Conditions
An AB Sciex API 5500 QTrap mass spectrometer (Toronto, Canada) interfaced with a Waters Acquity UPLC separation module was used to detect and quantify osimertinib, AZ-5104, AZ-7550, and propranolol. Empower 3.0 was used in UPLC and Analyst 1.6.2 software in the mass spectrometer. Other settings for mass spectrometry parameters of the four compounds are shown in Table 1.
Chromatographic separation was achieved on a Waters Acquity UPLC HSS T3 1.8 μm column (2.1 mm × 50 mm) at 40°C using a mobile phase containing 0.1% FA that consisted of solvent A (water) and solvent B (ACN). The mobile phase was delivered at 0.3 ml/min, and an elegant gradient program was used as follows: 0–0.5 min, at 10% solvent B; 0.5–2.0 min, from 10 to 90% solvent B; 2.0–2.5 min, at 100% solvent B; and 2.5–3.0 min, at 10% solvent B. To eliminate carryover as much as possible, the injection needle was washed with 2.5 ml strong needle wash solution [ACN–MeOH–IPA–water (25:25:25:25, v/v) and 2.5 ml weak needle wash solution (FA–ACN–water (0.5:50:50, v/v)] before each injection.
2.3 Sample Preparation
For researching drug–plasma interaction and validating the UPLC-MS/MS method, enough blank SD rat plasma must be prepared before relative experiments. First, about 200 ml of rat blood was extracted with 5.0 mM EDTA·2Na from the abdominal aorta. Second, the blood was centrifuged at 4.0°C at 12,000 rpm for 5.0 min. The plasma (upper layer) was then aliquoted in a 1.0-ml/tube and stored at −70°C until use.
About 10 ml of rat plasma was added into one centrifugal filter unit as molecular weight cut-off in need and filtered by centrifuging for 40 min at 5,000 rpm at 4°C. The filtered-out liquid turned transparent and was stored at −70°C until use.
Osimertinib, AZ-5104, AZ-7550, and propranolol powders were dissolved to 1.0 mg/ml by an appropriate amount of DMSO as a stock solution, aliquoted in a 100-μl/tube and stored at −70°C until use. Working solutions were prepared extemporaneously by diluting stock solutions with 50% acetonitrile.
The L-cysteine powder was fast dissolved to 1.0 mg/ml by 1 × PBS as storage solution and quickly stored at −70°C as soon as possible. To inhibit the spontaneous dimerization of cysteine to cystine in PBS, the cysteine solution was freshly prepared before each experiment.
An osimertinib tablet was ground into a powder using a mortar and pestle. Then, a certain mass of powder samples converted into 8.0 mg osimertinib was weighed and added into 10 ml 12.5 mM CMC·Na. The sample was subjected to vortex mixing (3.0 min), sonicated (3.0 min), and vortex mixing again. The vortex–sonication cycle was repeated three times.
2.4 Plasma/Cysteine–Drug Incubation
Generally, each 45 μl L-cysteine solution with a certain concentration (diluted by 1 × PBS) or rat plasma was added with 5.0 μl working solution and mixed using vortex apparatus. Then, 5.0 μl propranolol with a 100 ng/ml concentration was added and mixed as described previously. The mixture was incubated at 37°C in a thermostatic mixing water bath pot for a certain period of time. Afterward, the tube was added with 350 μl ACN, vortexed (room temperature, 2000 rpm, 5.0 min), sonicated (4°C, 5.0 min), and centrifuged (4°C, 12,000 rpm, 5.0 min). Then, 200 μl upper liquid was taken into sample tubes for UPLC-MS/MS analysis.
2.5 UPLC-MS/MS Validation
The linearity, accuracy, precision, selectivity, specificity, matrix effect, recovery, and stability were all experimentally validated. The detailed validation process descriptions are as follows:
2.5.1 Linearity
Seven calibration standards samples with sequential concentrations of 1.0 (lower limit of quantitation, LLOQ), 3.0, 9.0, 27, 81, 243, and 729 ng/ml for osimertinib, AZ-5104, and AZ-7550 in rat plasma with additional 10 ng/ml propranolol (inner standard, IS) were made up in three successive batches. Calibration curves were obtained by plotting each analyte’s peak area ratio to IS versus the nominal concentration of calibration standards. The weighting factor was set at 1/x2. The deviations from the mean for each calibration standard should be within ± 15%. The LLOQ should not exceed ± 20%.
2.5.2 Specificity and Selection
The specificity of the method was assessed by analyzing six batches of blank SD rat plasma to validate the interference induced by chromatographic conditions at each analyte’s retention time. The criteria of acceptance for the method specificity and selectivity are that the peak areas in the double blank samples should be less than 20% of the peak areas of LLOQ samples in each batch.
2.5.3 Accuracy and Precision
Three standards samples with sequential concentrations of 2.0, 27, and 583 ng/ml for osimertinib, AZ-5104, and AZ-7550 in rat plasma with additional 10 ng/ml propranolol (inner standard, IS) were set as the low-quality control (LQC), middle-quality control (MQC), and high-quality control (HQC), respectively. The intra- and inter-batch precision was indicated as the percent relative standard deviation (RSD%). The intra- and inter-batch accuracy was indicated as the nominal concentration’s relative error (RE). Both precision and accuracy were determined by analyzing six replicates of QC in three consecutive batches. The acceptance criteria of precision for the intra- and inter-batch of QC should be within ± 15%, except ± 20% for LLOQ. The acceptance criteria for the intra- and inter-batch accuracy are that the RE of QC should be within 85–115% of their nominal concentration at each QC concentration except 80–120% for LLOQ.
2.5.4 Matrix Effect and Recovery
The degree of the matrix influencing each analyte’s tandem mass spectrum signal was assessed by comparing the peak area of the analyte spiked after extraction and the peak area of standard in neat solution at each QC concentration using six batches of the blank matrix from different sources. The matrix effect’s acceptance criteria are that the coefficient of variation of the IS-normalized matrix effect calculated from the six batches of the matrix should be within 85–115%. The relative recovery was determined by comparing each extracted sample’s obtained value with the extracts of the spiked blank matrix with the analyte post-extraction at three QC concentration levels.
2.5.5 Stability
Each QC analyte’s long-term and freeze-thaw stability in the SD rat plasma matrix with the addition of ACN (350 μl) for osimertinib was evaluated. To assess the analyte’s long-term stability, all QC samples were preserved at −70 C for 2 months before sample processing and analyzing whether ACN was pre-added or not. For assessing the analyte’s freeze–thaw stability, all QC samples were processed three cycles of freezing (−70 C) and thawing at room temperature for 1.0 h before sample processing and analyzing, ignoring the addition of ACN. The acceptance criteria were less than 15% of the nominal values for accuracy and within 15% for precision.
2.6 Pharmacokinetics Study
Five male SD rats (180–200 g) were fed autoclaved standard laboratory food and free to access sterile water and kept in an environmentally controlled breeding room (temperature, 20 ± 2°C; humidity, 60 ± 5%; 12-h dark/light cycle) for at least 6 days before experimentation. All rats were fasted for 12 h before the experiment but were allowed free access to water. The rats were given osimertinib dissolved by CMC·Na orally at 8.0 mg/kg. The rats were anesthetized by isoflurane at 0.08, 0.25, 0.5, 1.0, 2.0, 4.0, 6.0, 8.0, 10, and 24 h after oral administration. Blood samples (about 200 μl) were immediately collected from an eye socket vein and preserved in EDTA tubes. Then, the blood sample was centrifuged in an Eppendorf laptop centrifuge 5417C (Hamburg, Germany) for 5.0 min at 12,000 rpm to obtain plasma. Plasma with a volume of 50 μl was transferred into a tube added with 350 μl ACN beforehand. The mixture was quickly vortexed by an MS3 basic vortex mixer (IKA GmbH, Germany) at 2000 rpm for 2.0 min and stored at −70°C until further processed.
Animal welfare and experimental procedures strictly followed the Guide for the Care and Use of Laboratory Animals (The Ministry of Science and Technology of China, 2006) and the related ethical regulations of the China Academy of Chinese Medical Sciences (CACMS).
3 Results and Discussion
3.1 The Instability of Osimertinib/AZ-5104/AZ-7550 in Rat Plasma
The stability of osimertinib, AZ-5104, and AZ-7550 was investigated by observing the relative quantitative change after incubating each 10 ng/ml chemical with 50 μl rat plasma (n = 6) for 0 and 6.0 h. For a better description, the three negative control experiments (incubation time = 0) were used for normalization (Figure 1B). After 6.0 h of incubation, the detected relative quantity of osimertinib, AZ-5104, and AZ-7550 fell sharply to 2.76%, 2.96%, and 20.3%, respectively, strongly showing severe instability of the three chemicals in rat plasma. These phenomena are consistent with a previous study using human plasma as a warm bath matrix (Dickinson et al., 2016), thus excluding the possibility induced by species differences. Then, the instability of all three chemicals should be attributed to the typical chemical structure of all three drugs and the material basis of animal plasma.
Similar to other third-generation anti-tumor-targeted drugs, osimertinib contains two primary functional groups. One is the skeleton which can recognize the ATP-binding site of the EGFR kinase (To et al., 2019), and the other is the Michael acceptor (red part of Figures 1A, a), which can react with the cysteine-797 residue of the EGFR kinase to form irreversible covalent bond formation. AZ-5104 and AZ-7550 are the primary demethylated metabolites of osimertinib (Jiang et al., 2018), sharing the same active Michael acceptor with osimertinib (red part of Figures 1A–C). The instability of all three chemicals is likely due to the Michael addition reaction deriving from the Michael acceptor and the cysteine in the plasma matrix. Considering that the high concentration of total cysteine in plasma is elevated to 10 μM and the pharmacology of osimertinib, we propose that the protein-containing cysteine residue and free cysteine in plasma are significant factors causing the decline of osimertinib, AZ-5104, and AZ-7550 in plasma.
3.2 Protein and Free Cysteine Reduce the Stability of Osimertinib/AZ-5104/AZ-7550
To test the contribution of plasma protein in the instability of osimertinib, AZ-5104, and AZ-7550, rat plasma filtered by a centrifugal filter unit with a specific molecular weight cut-off (MWCO) was used to incubate with the three chemicals, respectively, instead of untreated plasma. The centrifugal filter unit was used to cut off macromolecule proteins above 30 kDa or 3.0 kDa from plasma. The normalized relative quantity of osimertinib was 49.8% and 62.2% (Figure 2A, a) after incubating with plasma treated by the centrifugal filter with MWCO 30 kDa or 3.0 kDa for 6.0 h, respectively. Furthermore, the values were 85.1% and 84.5% for AZ-5104 (Figures 2A,B) and 71.0% and 73.3% for AZ-7550 (Figures 2A,C). Through comparing with the detected osimertinib, AZ-5104, and AZ7550 using untreated plasma as substrates (Figure 1B), protein-filtered plasma enhanced the stability of osimertinib, AZ5104, and AZ7550. Therefore, macromolecular protein in plasma is one of the main factors inducing the instability of osimertinib, AZ-5104, and AZ-7550 in plasma.
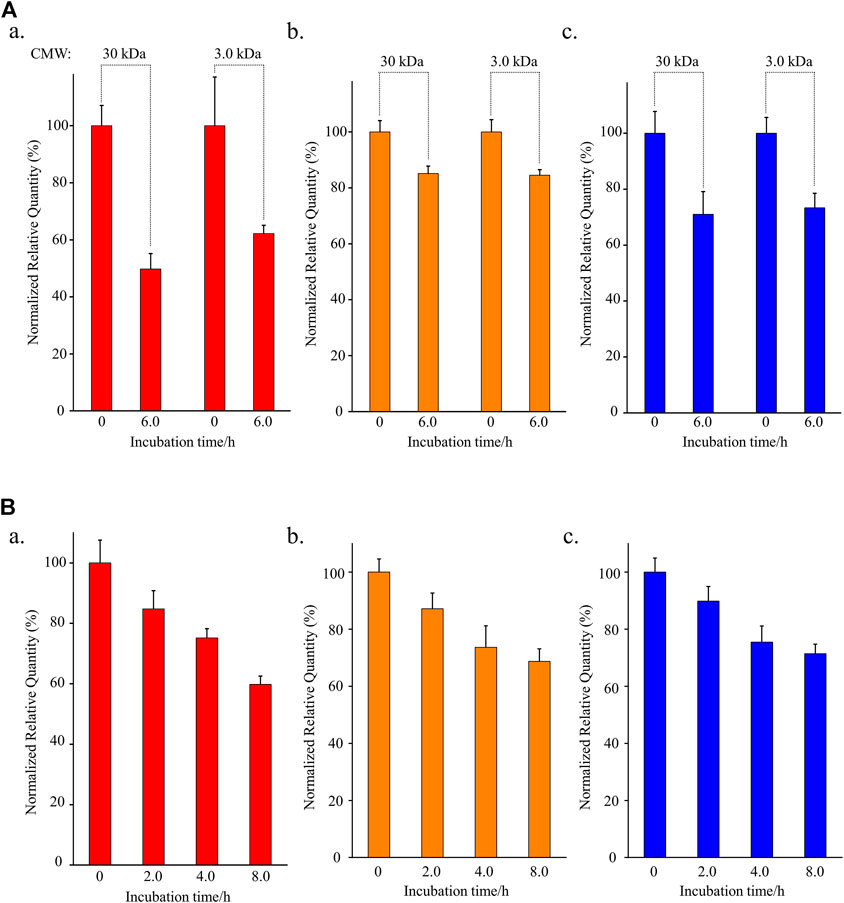
FIGURE 2. Investigation of the stabilities of (a) osimertinib (red), (b) AZ-5104 (orange), and (c) AZ-7550 (blue) in (A) filtered rat plasma and (B) L-cysteine. In (A), the cut-off molecular weight (CMW) was 30 kDa or 3.0 kDa. In (B), L-cysteine concentration was 10.0 μg/ml. Note: the working solutions except for L-cysteine were kept at 39°C for the longest incubation time to eliminate all four chemicals’ temperature instability.
To further identify the influence of free cysteine on the stability of osimertinib, AZ-5104, and AZ-7550, another experiment that incubates 10 μg/ml cysteine aqueous solution with 10 ng/ml chemical substrate for 2.0, 4, 0, and 8.0 h was designed. In addition, PBS with a pH of 7.2–7.4 was used as a solvent to mimic the rat plasma’s pH environment. The detected osimertinib, AZ-5104, and AZ-7550 present a highly negative relationship with the incubation duration (Figures 2A,B,C), and the normalized relative quantity was 59.8%, 68.7% and 71.4%, respectively, after 8.0 hours of incubation.
It is well known that only 20 amino acids are involved in protein synthesis in mammals, in which there is only one amino acid containing biological thiol, cysteine. However, osimertinib, carrying α, β-unsaturated carbonyls, was designed to be able to react with biological thiols, resulting in irreversible small-molecule-thiol adducts. No amino acid can react with osimertinib, AZ-5104, and AZ-7550 except for cysteine. However, homocysteine, an intermediate product of methionine metabolism in mammals (Kumar et al., 2017), contains one sulfhydryl group, can react with the Michael acceptor of osimertinib, AZ-5104, and AZ-7550. The average homocysteine concentration in mammals such as human is 10 μM (Brouwer et al., 1998), much lower than that of cysteine (319 μM) (Wang et al., 2018). Overall, these results indicate that the protein and free cysteine in plasma are the main factors inducing the instability of osimertinib in plasma.
3.3 Acetonitrile Maintains the Stability of Osimertinib in Plasma
In general, adding an appropriate additive is an efficient strategy to promise the stability of chemicals in a matrix. For example, adding enough complexing agent such as EDTA can enhance the stability of drugs and foods in plasma (Keowmaneechai and McClements, 2002; Song et al., 2014). This strategy is based on the fact that a complexing agent can decrease the quantity of free metal ions, which is the cofactor to the protein that can degrade the chemical. In addition, the incubation of proteinase K can also enhance the stability of several chemicals in plasma. Such a method is based on proteinase K that can disrupt the three-dimensional structure of proteins in plasma which degrades the target drug (Kim et al., 2019). However, based on our findings, the instability of osimertinib in plasma is due to protein and the free small organic molecule cysteine. As a result, both strategies targeting reducing protein activity were limited in overcoming the effect of free cysteine or cysteine residue in the plasma matrix. In addition, phenylmethylsulfonyl fluoride (PMSF), sodium fluoride (NaF), and diisopropyl fluorophosphate (DFP) were also applied to improve the stability of osimertinib, AZ-5104, and AZ-7550 in rat plasma based on the previous experiment. However, we did not achieve the desired results (data not shown). Therefore, a new additive is urgently needed to breach such barrier based on the chemical or physical theory.
Organic solvents such as acetonitrile, methanol, isopropanol, and acetone are primary solvents used to precipitate protein in the plasma sample (Bruce et al., 2009). Interestingly, the solubility of cysteine in acetonitrile is the lowest among other solvents (Han et al., 2020), indicating that acetonitrile is an appropriate additive for precipitating both protein and cysteine in plasma. To test this hypothesis, the stability of osimertinib in plasma while adding acetonitrile at 39 C (rat temperature in vivo) was tested (Figure 3A). Excitingly, no substantial changes were noticed in osimertinib levels even under incubation for 8.0 h (Figure 3, line in blue). Moreover, the same phenomenon was also observed using AZ-5104 and AZ-7550 as chemical substrates (Figure 3, lines in orange and red, respectively). Apparently, acetonitrile is an effective stabilizer for osimertinib in plasma (Figure 3B).
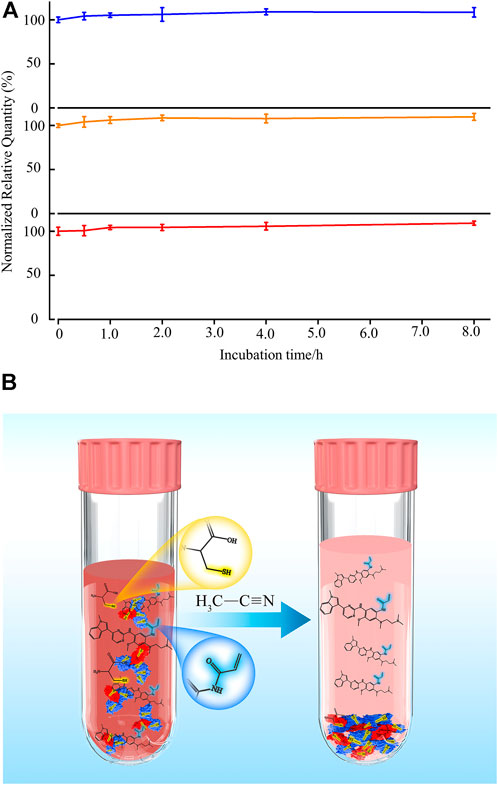
FIGURE 3. (A) Addition of acetonitrile keeps the stability of osimertinib (blue), AZ-5104 (orange), and AZ-7550 (red) in rat plasma. Each 5.0 μl chemical substrate (100 ng/ml) was incubated with 45 μl rat plasma and vortexed for 1.0 min in the experiment. Then, 350 μl acetonitrile was added, and 400 μl solution was vortexed at 1,600 rpm for 5.0 min and incubated at 39°C for 0.5, 1.0, 2.0, 4.0, and 8.0 h before the mass spectrometry sample preparation and measurement. (B) Schematic representation of adding acetonitrile in plasma protects third-generation EGFR-TKI osimertinib and its main metabolites from Michael addition with free cysteine and protein.
3.4 Method Validation
On the basis of aforementioned results, a systemic method validation for measuring osimertinib, AZ-5104, and AZ-7550 assisted by adding acetonitrile was performed according to guidelines set by Pharmacopoeia of the People’s Republic of China (2020) and the US Food and Drug Administration (2013). The UPLC-MS/MS condition was first optimized and four samples including blank, LLOQ, and plasma, were separated and detected successfully (Figure 4), showing no significant interference from SD rat plasma observed at the retention time of analytes and IS. In addition, the representative calibration curve equation was y = 0.00941x + 0.00158 (r = 0.9987) to osimertinib, y = 0.00758x + 0.000415 (r = 0.9983) to AZ-5104, and y = 0.0102x + 0.00235 (r = 0.9978) to AZ-7550. The accuracy and intra- and inter-day precision of osimertinib, AZ-5104, and AZ-7550 in SD rat plasma were calculated and summarized as given in Tables 2A–C, respectively. The data of extraction recovery and matrix effect obtained are presented in Table 3. In particular, the long-term stability and freeze–thaw stability in the SD rat plasma matrix with the addition of ACN were studied, and the results are summarized in Table 4, indicating that acetonitrile keeps the stability of osimertinib in plasma efficiently.
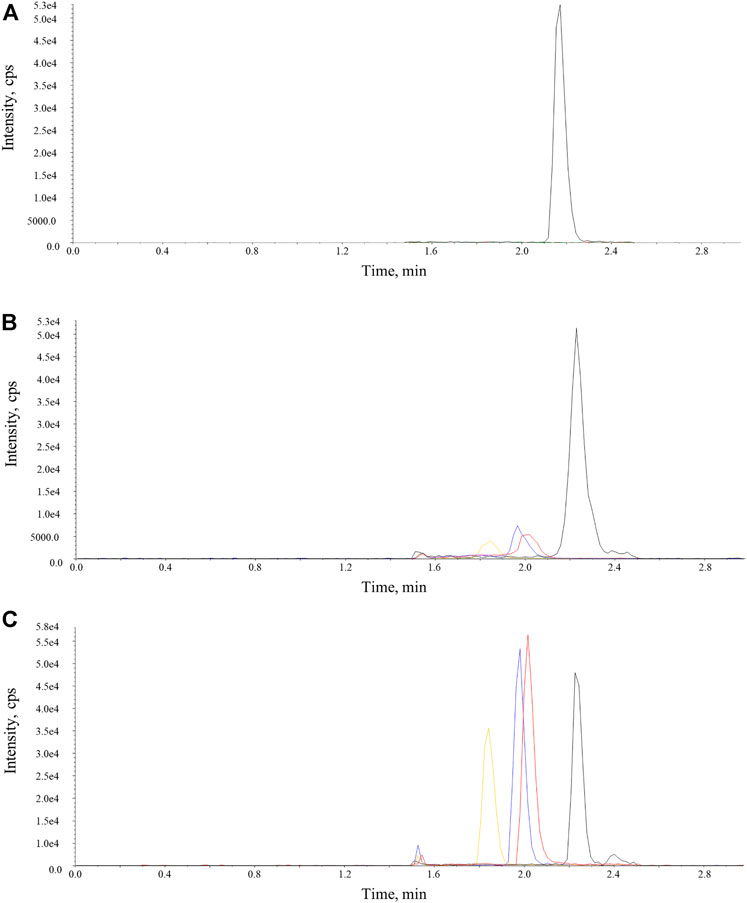
FIGURE 4. Representative chromatograms of osimertinib, AZ-5104, AZ-7550, and IS in SD rat plasma samples. The red, orange, and blue lines represent osimertinib, AZ-5104, and AZ-7550. The black line represents IS. (A) Blank, (B) LLOQ, and (C) real SD rat plasma sample.
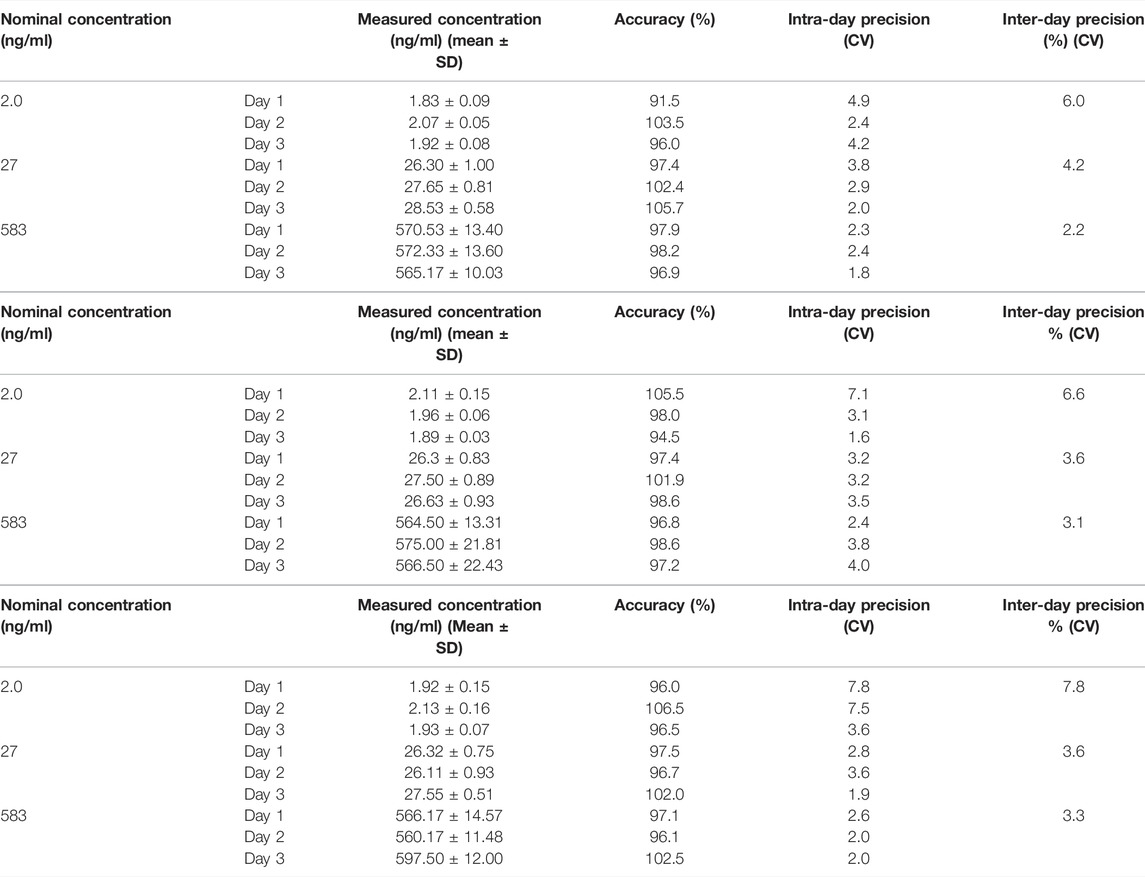
TABLE 2. Accuracy and intra- and inter-day precision of (A) osimertinib, (B) AZ-5104, and (C) AZ-7550 in SD rat plasma (n = 6). (A)
3.5 Pharmacokinetics Study of Osimertinib in Rat Plasma
To further apply such an assay in a pre-clinical pharmacokinetics study, we performed pharmacokinetics study of osimertinib in rat plasma using five male SD rats. Unlike the conventional pre-clinical pharmacokinetics experimental scheme, the preparation of rat plasma was online. The rat blood was taken from the eye socket vein at the appropriate time point and immediately followed by high-speed centrifugation for plasma preparation. Then, 50 μl of freshly made rat plasma was mixed with a seven-order volume of acetonitrile for maintaining the stability of osimertinib. Each concentration of osimertinib in rat plasma was obtained by the validated UPLC-MS/MS assay, and all data are described statistically as a concentration–time curve (Figure 5), displaying the concentration of osimertinib in rat plasma peaks (65.5 ng/ml) after 2.5 h of oral administration which slowly reduced in the following 20 h. Also after 24 h of oral administration, osimertinib was not detectable in rat plasma. Such a pre-clinical experiment indicates the applicability of the strategy that stabilizes osimertinib in plasma by adding ACN and the methodology in processing rat plasma online.
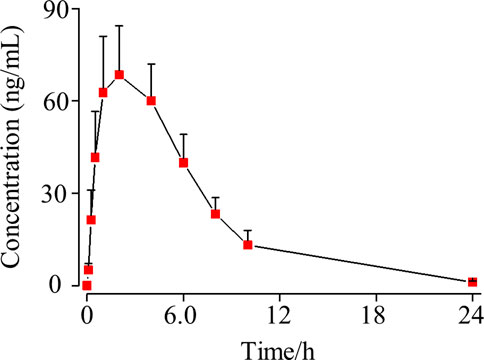
FIGURE 5. SD rat plasma concentration–time curve of osimertinib (mean ± standard deviation, n = 5) after oral administration.
One should note that acetonitrile treatment should be concomitant with the whole animal experiment before sample storage, thus adding tension and complexity to a certain extent. Therefore, an optimal and feasible protocol is needed to make the proposed strategy convenient for operation in future research applications.
4 Conclusion
In summary, we confirmed that both the protein and cysteine in plasma are the main factors that induce the instability of osimertinib and its primary metabolites AZ-5104 and AZ-7550 through a series of experiments. A new strategy stabilizing osimertinib by adding enough acetonitrile was proposed and validated based on the physicochemical properties of plasma and cysteine. In addition, coupled with the online biological sample processing method, the strategy was successfully applied in a pre-clinical pharmacokinetics study. The proposed strategy adapts to human subjects, and a relative work is underway. It is notable that most third-generation EGFR-TKIs (such as naquotinib and olmutinib) contain at least one Michael acceptor targeting cysteine whether free in plasma or imported into the protein (Attwa et al., 2018; Alrabiah et al., 2019). Therefore, the organic additive-assisted method coupled with the online biological sample processing methodology may promote the research and development of other third-generation EGFR-TKI in the future study.
Data Availability Statement
The original contributions presented in the study are included in the article/Supplementary Material. Further inquiries can be directed to the corresponding authors.
Ethics Statement
The animal study was reviewed and approved by the Experimental Animal Welfare Ethics Committee, Institute of Chinese Materia Medica, China Academy of Chinese Medical Sciences.
Author Contributions
ZY: methodology, investigation, writing—original draft, and funding acquisition. XY, SW, and WC: supervision and validation. XW: resources and supervision. XY, QW, WH, CK, and WY: participating in experiments. YL: writing—review and editing, conceptualization, supervision, funding acquisition, and validation. X-YZ: resources, supervision, subject design, editing and revisions, and funding acquisition.
Funding
This work was supported by the National Key R&D Program of China (2020YFC2008106, 2017ZX09304026), the Scientific and Technological Innovation Project of China Academy of Chinese Medical Sciences (CI2021B015 and CI2021A04909), the National Science and Technology Major Project (2017ZX09304026), the Fundamental Research Funds for Central Scientific Research Institutes of the Institute of China Academy of Chinese Medical Sciences, Chinese Materia Medica (L2020031), the Fundamental Research Funds for the Central Public Welfare Research Institutes (ZXKT20031), and the grant from the Special Fund for Outstanding Young Scientific and Technological Talents (ZZ14-YQ-030).
Conflict of Interest
The authors declare that the research was conducted in the absence of any commercial or financial relationships that could be construed as a potential conflict of interest.
Publisher’s Note
All claims expressed in this article are solely those of the authors and do not necessarily represent those of their affiliated organizations, or those of the publisher, the editors, and the reviewers. Any product that may be evaluated in this article, or claim that may be made by its manufacturer, is not guaranteed or endorsed by the publisher.
Abbreviations
ACN, Acetonitrile; AR, analytical reagent; DMSO, dimethyl sulfoxide; EGFR-TKI, epidermal growth factor receptor tyrosine kinase inhibitor; EDTA·2Na, ethylenediaminetetraacetic acid disodium salt; FA, formic acid; HQC, high quality control; HS, human serum albumin; IS, inner standard; IPA, isopropanol; Cys, L-cysteine; LLE, liquid–liquid extraction; LLOQ, lower limit of quantitation; LQC, low quality control; MeOH, methanol; MQC, middle quality control; MWCO, molecular weight cut-off; NSCLCs, non-small-cell lung carcinomas; PFS, progression-free survival; RE, relative error; RSD, relative standard deviation; CMC·Na, sodium carboxymethyl cellulose; SD rats, Sprague–Dawley rats; UPLC-MS/MS, ultra-performance liquid chromatography–tandem mass spectrometer.
References
-Ke, E. E., and Wu, Y. L. (2016). EGFR as a Pharmacological Target in EGFR-Mutant Non-small-cell Lung Cancer: Where Do We Stand Now? Trends Pharmacol. Sci. 37 (11), 887–903. doi:10.1016/j.tips.2016.09.003
Alrabiah, H., Kadi, A. A., Attwa, M. W., and Abdelhameed, A. S. (2019). A Simple Liquid Chromatography-Tandem Mass Spectrometry Method to Accurately Determine the Novel Third-Generation EGFR-TKI Naquotinib with its Applicability to Metabolic Stability Assessment. RSC Adv. 9, 4862–4869. doi:10.1039/C8RA09812C
Attwa, M. W., Kadi, A. A., Darwish, H. W., and Abdelhameed, A. S. (2018). Investigation of the Metabolic Stability of Olmutinib by Validated LC-MS/MS: Quantification in Human Plasma. RSC Adv. 8, 40387–40394. doi:10.1039/C8RA08161A
Ballard, P., Yates, J. W., Yang, Z., Kim, D. W., Yang, J. C., Cantarini, M., et al. (2016). Preclinical Comparison of Osimertinib with Other EGFR-TKIs in EGFR-Mutant NSCLC Brain Metastases Models, and Early Evidence of Clinical Brain Metastases Activity. Clin. Cancer Res. 22 (20), 5130–5140. doi:10.1158/1078-0432.CCR-16-0399
Barbuti, A. M., Zhang, G.-N., Gupta, P., Narayanan, S., and Chen, Z.-S. (2019). “EGFR and HER2 Inhibitors as Sensitizing Agents for Cancer Chemotherapy,” in Protein Kinase Inhibitors as Sensitizing Agents for Chemotherapy. Editors Z.-S. Chen,, and D.-H. Yang (United States: Academic Press), 1–11. doi:10.1016/B978-0-12-816435-8.00001-8
Brouwer, D. A., Welten, H. T., Reijngoud, D. J., van Doormaal, J. J., and Muskiet, F. A. (1998). Plasma folic acid cutoff value, derived from its relationship with homocyst(e)ine. Clin. Chem. 44, 1545–1550. doi:10.1093/clinchem/44.7.1545
Bruce, S. J., Tavazzi, I., Parisod, V., Rezzi, S., Kochhar, S., and Guy, P. A. (2009). Investigation of Human Blood Plasma Sample Preparation for Performing Metabolomics Using Ultrahigh Performance Liquid Chromatography/Mass Spectrometry. Anal. Chem. 81 (9), 3285–3296. doi:10.1021/ac8024569
Cho, J. H., Lim, S. H., An, H. J., Kim, K. H., Park, K. U., Kang, E. J., et al. (2020). Osimertinib for Patients with Non-small-cell Lung Cancer Harboring Uncommon EGFR Mutations: A Multicenter, Open-Label, Phase II Trial (KCSG-LU15-09). J. Clin. Oncol. 38 (5), 488–495. doi:10.1200/JCO.19.00931
Dickinson, P. A., Cantarini, M. V., Collier, J., Frewer, P., Martin, S., Pickup, K., et al. (2016). Metabolic Disposition of Osimertinib in Rats, Dogs, and Humans: Insights into a Drug Designed to Bind Covalently to a Cysteine Residue of Epidermal Growth Factor Receptor. Drug Metab. Dispos. 44 (8), 1201–1212. doi:10.1124/dmd.115.069203
Floc'h, N., Lim, S., Bickerton, S., Ahmed, A., Orme, J., Urosevic, J., et al. (2020). Osimertinib, an Irreversible Next-Generation EGFR Tyrosine Kinase Inhibitor, Exerts Antitumor Activity in Various Preclinical NSCLC Models Harboring the Uncommon EGFR Mutations G719X or L861Q or S768I. Mol. Cancer Ther. 19 (11), 2298–2307. doi:10.1158/1535-7163.MCT-20-0103
Fresnais, M., Roth, A., Foerster, K. I., Jäger, D., Pfister, S. M., Haefeli, W. E., et al. (2020). Rapid and Sensitive Quantification of Osimertinib in Human Plasma Using a Fully Validated MALDI-IM-MS/MS Assay. Cancers (Basel) 12 (7). doi:10.3390/cancers12071897
Gao, N., Zhang, X., Hu, X., Kong, Q., Cai, J., Hu, G., et al. (2022). The Influence of CYP3A4 Genetic Polymorphism and Proton Pump Inhibitors on Osimertinib Metabolism. Front. Pharmacol. 13, 794931. Available at: https://www.frontiersin.org/article/10.3389/fphar.2022.794931. doi:10.3389/fphar.2022.794931
Gridelli, C., Rossi, A., Carbone, D. P., Guarize, J., Karachaliou, N., Mok, T., et al. (2015). Non-small-cell Lung Cancer. Nat. Rev. Dis. Prim. 1, 15009. doi:10.1038/nrdp.2015.9
Han, J., Liu, H., Hu, S., Qiu, J., Yi, D., An, M., et al. (2020). Determination and Correlation of the Solubility of L-Cysteine in Several Pure and Binary Solvent Systems. J. Chem. Eng. Data 65 (5), 2649–2658. doi:10.1021/acs.jced.0c00031
Irie, K., Nanjo, S., Hata, A., Yamasaki, Y., Okada, Y., Katakami, N., et al. (2019). Development of an LC-MS/MS-based Method for Quantitation of Osimertinib in Human Plasma and Cerebrospinal Fluid. Bioanalysis 11 (9), 847–854. doi:10.4155/bio-2018-0292
Jiang, T., Su, C., Ren, S., Cappuzzo, F., Rocco, G., Palmer, J. D., et al. (2018). A Consensus on the Role of Osimertinib in Non-small Cell Lung Cancer from the AME Lung Cancer Collaborative Group. J. Thorac. Dis. 10 (7), 3909–3921. doi:10.21037/jtd.2018.07.61
Keowmaneechai, E., and McClements, D. J. (2002). Influence of EDTA and Citrate on Physicochemical Properties of Whey Protein-Stabilized Oil-In-Water Emulsions Containing CaCl2. J. Agric. Food Chem. 50 (24), 7145–7153. doi:10.1021/jf020489a
Kim, J., Basiri, B., Hassan, C., Punt, C., van der Hage, E., den Besten, C., et al. (2019). Metabolite Profiling of the Antisense Oligonucleotide Eluforsen Using Liquid Chromatography-Mass Spectrometry. Mol. Ther. Nucleic Acids 17, 714–725. doi:10.1016/j.omtn.2019.07.006
Kumar, A., Palfrey, H. A., Pathak, R., Kadowitz, P. J., Gettys, T. W., and Murthy, S. N. (2017). The Metabolism and Significance of Homocysteine in Nutrition and Health. Nutr. Metab. (Lond) 14, 78. doi:10.1186/s12986-017-0233-z
Lategahn, J., Keul, M., Klövekorn, P., Tumbrink, H. L., Niggenaber, J., Müller, M. P., et al. (2019). Inhibition of Osimertinib-Resistant Epidermal Growth Factor Receptor EGFR-T790M/C797S. Chem. Sci. 10 (46), 10789–10801. doi:10.1039/C9SC03445E
Minchiotti, L., Galliano, M., Kragh-Hansen, U., and Peters, T. (2008). Mutations and Polymorphisms of the Gene of the Major Human Blood Protein, Serum Albumin. Hum. Mutat. 29 (8), 1007–1016. doi:10.1002/humu.20754
Mitchell, R., Bailey, C., Ewles, M., Swan, G., and Turpin, P. (2019). Determination of Osimertinib in Human Plasma, Urine and Cerebrospinal Fluid. Bioanalysis 11 (10), 987–1001. doi:10.4155/bio-2018-0262
Remon, J., Steuer, C. E., Ramalingam, S. S., and Felip, E. (2018). Osimertinib and Other Third-Generation EGFR TKI in EGFR-Mutant NSCLC Patients. Ann. Oncol. 29, i20–i27. doi:10.1093/annonc/mdx704
Rood, J. J. M., van Bussel, M. T. J., Schellens, J. H. M., Beijnen, J. H., and Sparidans, R. W. (2016). Liquid Chromatography-Tandem Mass Spectrometric Assay for the T790M Mutant EGFR Inhibitor Osimertinib (AZD9291) in Human Plasma. J. Chromatogr. B Anal. Technol. Biomed. Life Sci. 1031, 80–85. doi:10.1016/j.jchromb.2016.07.037
Song, Y., Huang, Z., Song, Y., Tian, Q., Liu, X., She, Z., et al. (2014). The Application of EDTA in Drug Delivery Systems: Doxorubicin Liposomes Loaded via NH4EDTA Gradient. Int. J. Nanomedicine 9, 3611–3621. doi:10.2147/IJN.S64602
Soria, J. C., Ohe, Y., Vansteenkiste, J., Reungwetwattana, T., Chewaskulyong, B., Lee, K. H., et al. (2018). Osimertinib in Untreated EGFR-Mutated Advanced Non-small-cell Lung Cancer. N. Engl. J. Med. 378 (2), 113–125. doi:10.1056/NEJMoa1713137
To, C., Jang, J., Chen, T., Park, E., Mushajiang, M., De Clercq, D. J. H., et al. (2019). Single and Dual Targeting of Mutant EGFR with an Allosteric Inhibitor. Cancer Discov. 9 (7), 926–943. doi:10.1158/2159-8290.CD-18-0903
van Veelen, A., van Geel, R., de Beer, Y., Dingemans, A. M., Stolk, L., Ter Heine, R., et al. (2020). Validation of an Analytical Method Using HPLC-MS/MS to Quantify Osimertinib in Human Plasma and Supplementary Stability Results. Biomed. Chromatogr. 34 (4), e4771. doi:10.1002/bmc.4771
Veerman, G. D. M., Lam, M. H., Mathijssen, R. H. J., Koolen, S. L. W., and de Bruijn, P. (2019). Quantification of Afatinib, Alectinib, Crizotinib and Osimertinib in Human Plasma by Liquid Chromatography/triple-Quadrupole Mass Spectrometry; Focusing on the Stability of Osimertinib. J. Chromatogr. B Anal. Technol. Biomed. Life Sci. 1113, 37–44. doi:10.1016/j.jchromb.2019.03.011
Wang, N., Wang, Y., Gao, J., Ji, X., He, J., Zhang, J., et al. (2018). A Ratiometric Fluorescent BODIPY-Based Probe for Rapid and Highly Sensitive Detection of Cysteine in Human Plasma. Analyst 143 (23), 5728–5735. doi:10.1039/C8AN01438H
Wu, S. G., and Shih, J. Y. (2018). Management of Acquired Resistance to EGFR TKI-Targeted Therapy in Advanced Non-small Cell Lung Cancer. Mol. Cancer 17 (1), 38. doi:10.1186/s12943-018-0777-1
Xiong, X., Zhang, Y., Wang, Z., Zhou, C., Yang, P., Du, X., et al. (2022). Simultaneous Quantitative Detection of Afatinib, Erlotinib, Gefitinib, Icotinib, Osimertinib and Their Metabolites in Plasma Samples of Patients with Non-small Cell Lung Cancer Using Liquid Chromatography-Tandem Mass Spectrometry. Clin. Chim. Acta 527, 1–10. doi:10.1016/j.cca.2021.12.028
Xue, W., Meylan, E., Oliver, T. G., Feldser, D. M., Winslow, M. M., Bronson, R., et al. (2011). Response and Resistance to NF-κB Inhibitors in Mouse Models of Lung Adenocarcinoma. Cancer Discov. 1 (3), 236–247. doi:10.1158/2159-8290.CD-11-0073
Keywords: osimertinib, plasma stability, UPLC-MS/MS, acetonitrile, cysteine, non-small-cell lung cancer
Citation: Yuan Z, Yu X, Wu S, Wu X, Wang Q, Cheng W, Hu W, Kang C, Yang W, Li Y and Zhou X-Y (2022) Instability Mechanism of Osimertinib in Plasma and a Solving Strategy in the Pharmacokinetics Study. Front. Pharmacol. 13:928983. doi: 10.3389/fphar.2022.928983
Received: 26 April 2022; Accepted: 20 June 2022;
Published: 22 July 2022.
Edited by:
Sabina Passamonti, University of Trieste, ItalyReviewed by:
Hemant Jadhav, Birla Institute of Technology and Science, IndiaUmberto Malapelle, University of Naples Federico II, Italy
Copyright © 2022 Yuan, Yu, Wu, Wu, Wang, Cheng, Hu, Kang, Yang, Li and Zhou. This is an open-access article distributed under the terms of the Creative Commons Attribution License (CC BY). The use, distribution or reproduction in other forums is permitted, provided the original author(s) and the copyright owner(s) are credited and that the original publication in this journal is cited, in accordance with accepted academic practice. No use, distribution or reproduction is permitted which does not comply with these terms.
*Correspondence: Xiao-Yang Zhou, a2VlbmFsbEBzaW5hLmNvbQ==; Yingfei Li, eWZsaUBpY21tLmFjLmNu
†These authors have contributed equally to this work