- 1Shandong Collaborative Innovation Center for Diagnosis, Treatment and Behavioral Interventions of Mental Disorders, Institute of Mental Health, Jining Medical University, Jining, China
- 2Shandong Key Laboratory of Behavioral Medicine, School of Mental Health, Jining Medical University, Jining, China
Taraxasterol is one of the bioactive triterpenoids found in dandelion, a member of the family Asteraceae. In the animal or cellular models of several ailments, including liver damage, gastritis, colitis, arthritis, pneumonia, tumors, and immune system diseases, taraxasterol has been shown to have significant preventive and therapeutic effects. This review aims to evaluate the current state of research and provide an overview of the possible applications of taraxasterol in various diseases. The reported phytochemical properties and pharmacological actions of taraxasterol, including anti-inflammatory, anti-oxidative, and anti-carcinogenic properties, and its potential molecular mechanisms in developing these diseases are highlighted. Finally, we further explored whether taraxasterol has protective effects on neuronal death in neurodegenerative diseases. In addition, more animal and clinical studies are also required on the metabolism, bioavailability, and safety of taraxasterol to support its applications in pharmaceuticals and medicine.
Introduction
Dandelion is a member of the family Asteraceae and is widely distributed in the warmer temperate zones of the Northern Hemisphere (Gonzalez-Castejon et al., 2012). The plant dandelion has long been used as a medicinal herb. Its therapeutic role was mentioned as early as the 10th and 11th centuries by Arabian physicians for the treatment of liver and spleen diseases (Faber, 1958). In traditional Chinese medicine, dandelion is used in combination with other herbs to treat hepatitis and enhance the immune response to upper respiratory tract infections, bronchitis, and pneumonia (Sweeney et al., 2005).
Medicinal plants typically contain several different chemical compounds that may act individually or synergistically to improve health (Gurib-Fakim, 2006). As one of the bioactive triterpenoids found in dandelion, taraxasterol has become a focus of pharmacological studies. Power and Browning were the first to report the isolation of taraxasterol from the non-saponifiable matter of Taraxacum officinale root (Power and Browning, 1912). They first described taraxasterol as a phytosterol. Recently, taraxasterol has received increased attention for its anti-inflammatory, anti-oxidative, and anti-carcinogenic activity and its possible beneficial effects against the development of liver damage, cancer, and numerous immune system diseases. This review aims to evaluate the properties of taraxasterol and investigate its phytochemical properties, focusing on the most recent literature analyzing the pharmacological effects of taraxasterol on several diseases.
Phytochemical properties of taraxasterol
Taraxasterol, also known as (3β, 18α, 19α)-Urs-20 (30)-en-3-ol, is a pentacyclic triterpene with a 1,2-cyclopentene phenanthrene structure. The molecular formula of taraxasterol is C30H50O, and its molecular weight and melting point are 426.72 g/mol and 221–222°C, respectively. In the 1950s, the structure and configuration of taraxasterol were reported by (Figure 1) Ames et al. (1954). Oxidosqualene cyclases (OSCs) catalyzed 2,3-oxidosqualene cyclization, which produced triterpene scaffolds (Thimmappa et al., 2014). Recently, it was found that transgenic yeast expressing LsOSC1, one putative lettuce OSC gene, can produce taraxasterol in lettuce (Lactuca sativa) (Choi et al., 2020).
Power and Browning discovered taraxasterol and first reported it from the non-saponifiable matter of Taraxacum officinale or Wiggers root (Power and Browning, 1912). The highest levels of taraxasterol were observed in the latex of Taraxacum officinale (Burrows and Simpson, 1938; Furuno et al., 1993; Akashi et al., 1994). Taraxasterol was also isolated from wild plants and regenerated organs of Taraxacum officinale using a reversed-phase HPLC with CH3CN/H2O (Furuno et al., 1993). In addition, Akash et al. found that the radioactivity of taraxasterol was mainly observed in differentiated organs of Taraxacum officinale with accumulation patterns by HPLC combined with liquid scintillation analysis (Akashi et al., 1994). In this study, the biosynthesis of taraxasterol was revealed by detecting the incorporation time course of radioactivity from [2–14 C] mevalonic acid into individual triterpenols in the shoot segments. It was discovered that taraxasterol was synthesized during the first 24 h of the experiment, with the (pseudo)laticifer cells being the probable site of its biosynthesis (Akashi et al., 1994). According to the HPLC analysis, Sharma et al. reported that the quantity of taraxasterol in the natural root extract of T. officinale was 2.96 μg/ml, whereas the quantity of taraxasterol was 3.013 μg/ml in the root callus cultures (Sharma and Zafar, 2014). However, the absolute quantitation of taraxasterol in any plant material is unavailable and needs to be further studied. In addition, taraxasterol is present in esculent plants such as legumes, cereals, nuts, and seeds and in plant oils (Xu et al., 2004). Taraxasterol is obtained from various medicinal plants in addition to esculent ones. The distribution of taraxasterol in plants is summarized in Table 1.
Pharmacological profiles of taraxasterol
The use of taraxasterol has been linked to many health advantages. The following sections review investigations that support the pharmacological properties ascribed to taraxasterol. The pharmacological activities of taraxasterol in the fight against various diseases in vitro and in vivo are summarized in Table 2. The mechanism of action of taraxasterol is summarized in Figure 2.
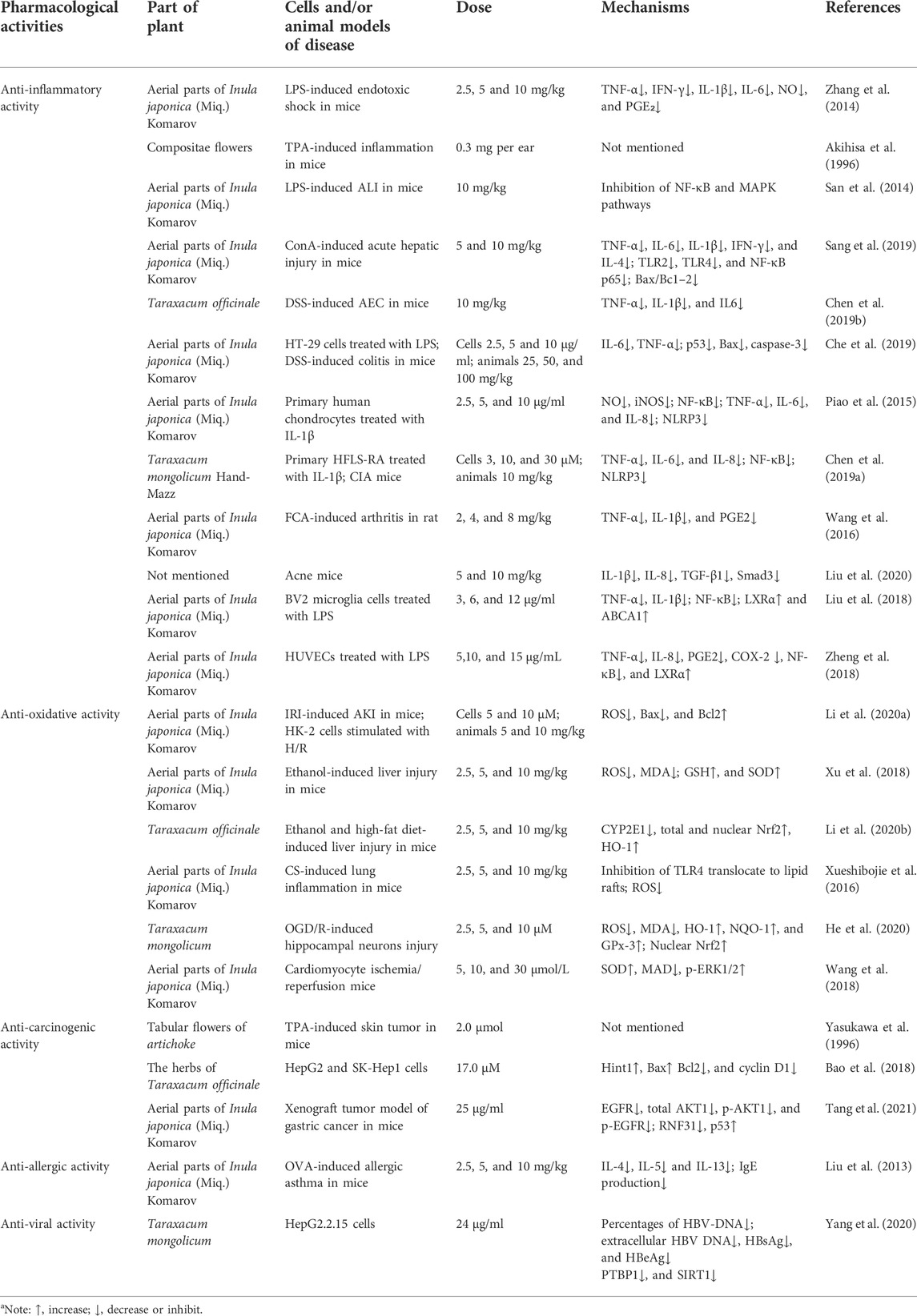
TABLE 2. Pharmacological activities of taraxasterol against diseases (in vitro and in vivo studies)a.
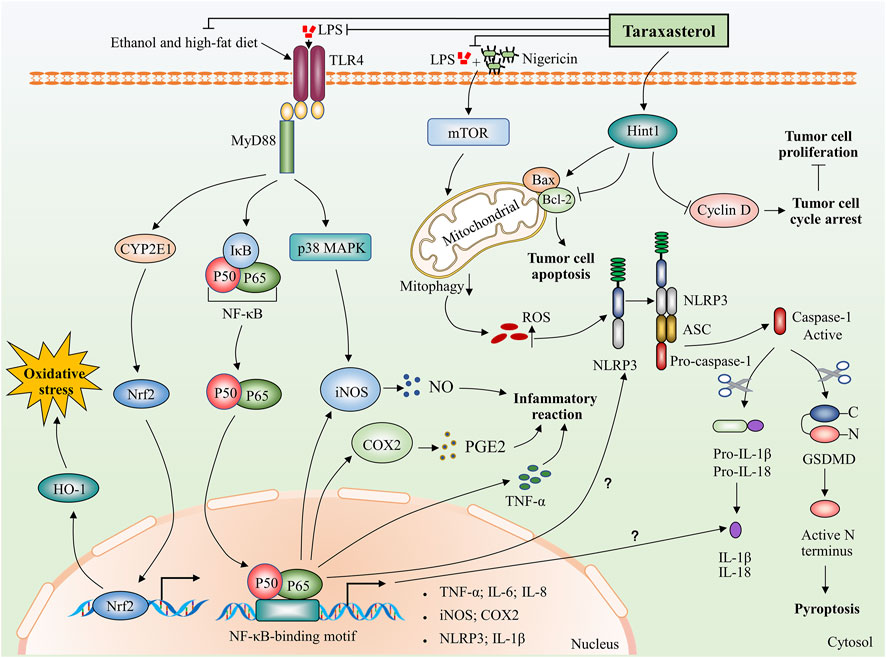
FIGURE 2. Potential biological mechanisms of taraxasterol: anti-inflammatory, anti-oxidative, and anti-carcinogenic. Taraxasterol impacts several aspects of inflammatory action. On the one hand, taraxasterol reduces the levels of inflammatory cytokines, including TNF-α and IL-6 and reduces serum levels of inflammatory mediators NO and PGE₂ through inhibiting NF-κB and MAPK signaling pathways. On the other hand, taraxasterol reduces ROS-mediated NLRP3 inflammation activation by suppressing mTOR and mitophagy, which contributes to the reduction of the inflammation reaction. Interestingly, NF-κB also regulates the expression of NLRP3 and IL-1β at transcriptional levels, yet the underlying mechanisms of whether the taraxasterol alleviates NLRP3 inflammation activation and reduces the levels of IL-1β by inhibiting the NF-κB signaling pathway are unclear. In addition, taraxasterol reduces oxidative stress induced by ethanol and a high-fat diet by inhibiting the expression of transcription factor Nrf2. Taraxasterol can promote tumor cell apoptosis and inhibit tumor cell proliferation by upregulating Hint1 expression in human liver cancer.
Anti-inflammatory activity
Inflammation describes various physiological and pathological processes triggered by noxious stimuli and conditions, such as infection and tissue injury (Medzhitov, 2008). Akihisa et al. demonstrated that the ID50 of taraxasterol extracted from the Compositae flowers was 0.3 mg/ear on 12-O-tetradecanoylphorbol-13-acetate- (TPA-) induced inflammation in mice (Akihisa et al., 1996). After 36 h of treatment with taraxasterol at doses of 2.5, 5, and 10 mg/kg, survival rates in LPS-induced endotoxic shock mouse models were up to 30, 40, and 70%, respectively. Moreover, no toxic effects of taraxasterol were observed in mice that received doses as high as 10 mg/kg. In addition, taraxasterol (10 mg/kg per day) significantly reduced levels of inflammatory cytokines, including tumor necrosis factor-α (TNF-α), interferon-γ (IFN-γ), interleukin-1β (IL-1β), and interleukin-6 (IL-6), and significantly reduced serum levels of inflammatory mediators such nitric oxide (NO) and prostaglandin E₂ (PGE₂) (Zhang et al., 2014). Intraperitoneal injection of taraxasterol (10 mg/kg per day) can significantly reduce the expression of pro-inflammatory factors, myeloperoxidase activity, and lung wet/dry ratio in a mouse model of LPS-induced acute lung injury (ALI). Mechanistically, the anti-inflammatory effects of taraxasterol may be due to the inhibition of the NF-κB and MAPK signaling pathways (San et al., 2014). The nuclear factor NF-κB is considered necessary for the expression of pro-inflammatory genes, and the NF-κB pathway is considered a prototypical pro-inflammatory signaling pathway (Lawrence, 2009). Treatment with taraxasterol at doses of 5 and 10 mg/kg significantly reduced the inflammatory response in a liver injury model induced by concanavalin A (Con A) by inhibiting the toll-like receptor-NF-κB signaling axis. In addition, taraxasterol prevented Con A-induced acute hepatic injury via the Bax/Bc1-2 anti-apoptotic signaling pathway (Sang et al., 2019). Furthermore, the increased serum alanine aminotransferase (ALT), aspartate aminotransferase (AST), and hepatic malondialdehyde (MDA) levels induced by Con A were significantly reduced by taraxasterol treatment (Sang et al., 2019). The potent anti-inflammatory properties of taraxasterol (orally with 10 mg/kg per day) have also been demonstrated in mice of an acute experimental colitis (AEC) model induced by oral administration of dextran sulfate sodium (DSS) (Chen W. et al., 2019). Peroxisome proliferator-activated receptor γ (PPARγ) plays a central role in the regulation of inflammatory signaling pathways by acting on kinases and transcription factors, such as NF-κB, c-Jun, c-Fos, and nuclear factor of activated T cell (NFAT), and by inhibiting the production of IL-1β and TNF-α (Su et al., 1999; Yang et al., 2000; Desreumaux et al., 2001). In the DSS-induced AEC animal models, taraxasterol (10 mg/kg) reversed DSS-induced PPARγ downregulation in AEC colon tissues and improved DSS-induced colitis, offering a novel insight into potential therapeutic strategies for acute colitis (Chen W. et al., 2019). In addition, Che et al. reported that taraxasterol significantly reduced the expression levels of IL-6 and TNF-α in a dose-dependent manner at doses between 2.5 and 10 μg/ml in vitro and 25 and 100 mg/kg in vivo (Che et al., 2019). Although there is evidence that taraxasterol has anti-inflammatory effects in various diseases, the precise mechanism by which it regulates inflammatory responses is still unclear. For example, both upstream multiple proteins and some non-encoded RNAs, such as RACK1 (Yao et al., 2014), tripartite motif-containing proteins (TRIMs) (Roy and Singh, 2021), microRNA-144 (Yang et al., 2022), and AMPK (Zhai et al., 2018), can regulate NF-κB expression. It is unclear whether taraxasterol inhibits NF-κB expression by regulating the expression of these proteins. Thus, further discussion is required in vitro and in vivo.
Recent studies have shown that taraxasterol exerts an anti-arthritic effect. These studies showed that taraxasterol reduced IL-1β-stimulated inflammatory responses in vitro and in vivo by suppressing the expression of COX-2 and iNOS and reducing NF-κB activation (Piao et al., 2015; Chen J. et al., 2019). Chen et al. reported that taraxasterol suppressed the NOD-like receptor protein 3 (NLRP3) inflammasome through inhibition of the expression of NLRP3, apoptosis-associated speck-like protein containing (ASC), and caspase-1 within a dose range of 0.3 to 0 μm in HFLS-RA cells and with 10 mg/kg in collagen-induced arthritis (CIA) mice (Chen J. et al., 2019). In another investigation, Wang et al. studied the protective effect of taraxasterol against Freund’s complete adjuvant- (FCA-) induced arthritis in rats. They found that taraxasterol (at doses of 2, 4, and 8 mg/kg) inhibited bone destruction by increasing serum OPG production and inhibiting the overproduction of serum inflammatory cytokines (Wang et al., 2016). In addition, Liu et al. showed that taraxasterol (10 mg/kg) improved propionibacterium acnes-induced inflammatory responses in a mouse ear edema model and suppressed pro-inflammatory chemokine production via the TGF-β/Smad pathway (Liu et al., 2020). The liver X receptors (LXRs) are members of the nuclear hormone receptor superfamily that bind and are activated by oxysterols (Lehmann et al., 1997). Liu et al. showed that taraxasterol (0 to 12 μg/ml) was a ligand of LXRα and inhibited the expression of TNF-α and IL-1ß via the activation of LXRα in LPS-stimulated BV2 microglia (Liu et al., 2018). These results suggested that taraxasterol may exert anti-inflammatory effects via activation of LXRα in the central nervous system. Similarly, another study on LPS-stimulated human umbilical vein endothelial cells also showed that taraxasterol (5–15 μg/ml) exerted anti-inflammatory effects by activating LXRα (Zheng et al., 2018).
Anti-oxidative activity
Oxidative stress is caused by exposure to reactive oxygen intermediates, which can damage proteins, nucleic acids, lipids, and cell membranes (Storz and Imlay, 1999). Studies have shown that cumulative damage caused by reactive oxygen species (ROS) contributes to numerous diseases (Apel and Hirt, 2004; Jakubczyk et al., 2020). Several studies have characterized the anti-oxidative effects of taraxasterol. In mice with acute kidney injury (AKI) induced by ischemia/reperfusion injury (IRI), Li et al. showed that taraxasterol (5 and 10 mg/kg) inhibited mitochondrial ROS production and ameliorated apoptosis in the kidney by decreasing Bax expression and increasing Bcl2 expression (Li C. et al., 2020). The transcription factor Nrf2 regulates the expression of phase II detoxification enzymes and a series of antioxidant enzymes (Chen et al., 2015). Heme oxygenase (HO-1), a phase II detoxification enzyme regulated by Nrf2, also plays an important antioxidant role (Suh et al., 2006). Many studies have shown that taraxasterol (2.5–10 mg/kg) inhibited oxidative stress by increasing the activity of the CYP2E1/Nrf2/HO-1 pathway in animal models of ethanol and high-fat diet-induced liver injury (Xu et al., 2018; Li Z. et al., 2020). Moreover, taraxasterol (2.5–10 mg/kg) also reduced the production of ROS, malondialdehyde (MDA), and increased glutathione (GSH) levels and superoxide dismutase (SOD) activity in ethanol-induced liver injury (Xu et al., 2018). An in vivo study showed that taraxasterol inhibited cigarette smoke-induced lung inflammation by inhibiting ROS production and ROS-mediated recruitment of TLR4 into lipid rafts within a dose range of 2.5–10 mg/kg. Moreover, taraxasterol also upregulated GSH production (Xueshibojie et al., 2016). In addition, taraxasterol has been shown to exert protective effects against neurological diseases. In oxygen-glucose deprivation/reperfusion- (OGD/R-) induced hippocampal neurons, taraxasterol (2.5–10 μm) significantly suppressed ROS production and MDA generation. Furthermore, taraxasterol induced nuclear Nrf2 accumulation and promoted increased expression of HO-1, NQO-1, and GPx-3 (He et al., 2020). Wang et al. found that taraxasterol increased the phosphorylation level of ERK1/2 in a cardiomyocyte ischemia/reperfusion (I/R) model, which indicated that taraxasterol exerted protective effects against oxidative stress by upregulating the ERK pathway at a dose of 30 μm (Wang et al., 2018). These results demonstrated that taraxasterol protected cardiomyocytes against hypoxia, suggesting that it may be significant for treating heart diseases (Wang et al., 2018).
Anti-carcinogenic activity
Previous studies have shown that extracts of Taraxacum officinale inhibited proliferation and induced apoptosis in hepatocellular carcinoma (HCC), HepG2, and Huh7 cells (Koo et al., 2004; Guo J. B. et al., 2015; Yoon et al., 2016). It has also been demonstrated that taraxasterol inhibits the development and progression of tumors. Taraxasterol extracted from the aerial parts of the Chrysanthemum genus significantly inhibited cell proliferation of both PC3 (human prostate cancer) and HT-29 (human colon cancer) cells. The IC50 values of the taraxasterol compound were determined as 37.1 and 89.7 µm in the PC3 and HT-29 cells at 48 h, respectively (Boutaghane et al., 2013). However, among other tumor cells, including MCF-7 (human breast carcinoma), HeLa (human cervix carcinoma), SK-MEL-5 (human melanoma), KB (human nasopharyngeal carcinoma), P388 (murine leukemia), MOLT-4 (human acute lymphoblastic leukemia), and SK-OV-3 (human ovary carcinoma) cells, taraxasterol did not exhibit significant inhibitory activity with IC50 values equal to or higher than 49 mm, suggesting that the anti-carcinogenic activity of taraxasterol may have cellular specificity (Villarreal et al., 1994; Lee et al., 2010; Jassbi et al., 2016). In an in vivo two-stage test, administration with 2 μmol/mouse of taraxasterol markedly inhibited the tumor-promoting effect of TPA on skin tumor formation following initiation with 7,12-dimethylbenz[α]anthracene. In this study, taraxasterol caused an 86% reduction in the average number of tumors per mouse at week 20 (Yasukawa et al., 1996). However, a subsequent study showed that taraxasterol (850 nmol/ml) also exhibited about 60% inhibition of the average number of papillomas per mouse at 20 weeks in terms of the two-stage carcinogenesis test (Takasaki et al., 1999). Furthermore, in the C3H/OuJ female mice treated with taraxasterol (2.5 mg in 100 ml of drinking water), the survival ratio of the mice was 80% even at 70 weeks of breeding, suggesting that taraxasterol can remarkably suppress the spontaneous mammary carcinogenesis in the C3H/OuJ female mice (Takasaki et al., 1999). Histidine triad nucleotide-binding protein 1 (Hint1) is a tumor suppressor often downregulated in association with the development of cancer (Wang et al., 2007; Wang et al., 2009). Bao et al. found that taraxasterol (IC50: 17.0 μm) selectively inhibited the proliferation of HepG2 cells by inducing cell cycle arrest at G0/G1 and inhibited apoptosis by upregulating Hint1 transcription to regulate the expression of Bax, Bcl2, and cyclin D1 (Bao et al., 2018). In addition, oral administration of 25 μg/ml of taraxasterol in drinking water for 30 days can effectively inhibit the growth of the implanted SK-Hep1 tumor in vivo (Bao et al., 2018). In a gastric cancer subcutaneous xenograft model, taraxasterol (25 μg/ml) inhibited the growth of xenograft tumors by inhibiting EGFR/AKT1 signaling (Chen et al., 2020). The E3 ubiquitin ligase RNF31 is overexpressed in many tumors and is associated with tumorigenesis (Guo J. et al., 2015; Zhu et al., 2016; Qiu et al., 2018). Tang et al. found that taraxasterol (50 μg/ml) promoted the degradation of RNF31 by activating autophagy, thereby inhibiting the p53 degradation and colorectal cancer (CRC) cell proliferation (Tang et al., 2021). In addition, taraxasterol can inhibit cell growth of breast, cervical, and melanoma in vitro (Dai et al., 2001; Lee et al., 2010). These findings indicated that taraxasterol may be a promising candidate for treating tumors.
Others
Liu et al. found that taraxasterol (5 and 10 mg/kg) significantly decreased the production of the Th2 cytokines IL-4, IL-5, and IL-13 in bronchoalveolar lavage fluid (BALF) and reduced the levels of ovalbumin- (OVA-) specific IgE in serum (Liu et al., 2013). In addition, taraxasterol (2.5–10 mg/kg) suppressed airway hyperresponsiveness (AHR) in a dose-dependent manner. Histological studies showed that taraxasterol substantially suppressed OVA-induced inflammatory cell infiltration into lung tissues and goblet cell hyperplasia in airways, which suggested that taraxasterol may protect against allergic asthma (Liu et al., 2013). In an in vitro study, taraxasterol (7.5 and 12.5 μg/ml) reduced the number of CaOx crystals in a dose-dependent manner and reduced the diameter of CaC2O4 dihydrate crystals. In addition, the inhibition of nucleation was increased by taraxasterol in the range of 26%–64% (Yousefi Ghale-Salimi et al., 2018). A recent study found that taraxasterol also exerted anti-viral effects. Taraxasterol (24 μg/ml) significantly reduced the secretion of HBsAg, HBeAg, HBV DNA, and intracellular HBsAg. Moreover, the treatment of taraxasterol (24 μg/ml for 48 h) also decreased the protein expression levels of the host factors polypyrimidine tract binding protein 1 (PTBP1) and sirtuin 1 (SIRT1) in HepG2.2.15 cells (Yang et al., 2020). In addition, pretreatment with different concentrations of taraxasterol (30, 60, 90, 120, 150, 180, or 210 µm) markedly prevented cell injury and inflammation in H2O2-induced HUVECs by reducing the expression of vascular cell adhesion molecule 1 (VCAM-1) and the cluster of differentiation 80 (CD80) (Yang et al., 2015). In addition, taraxasterol has also been shown to exert significantly antimicrobial effects. Taraxasterol obtained from the Mexican plants of the Asteraceae elicited a significant minimum inhibitory concentration (MIC) value (12.5 μg/ml) against Staphylococcus aureus (Villarreal et al., 1994). However, Boutaghane et al. observed weak inhibition of taraxasterol against the growth of Gram-negative (E. coli, P. aeruginosa, K. pneumoniae) bacteria (Boutaghane et al., 2013). Akihisa group described the anti-tubercular activity of taraxasterol against Mycobacterium tuberculosis with the MIC values of 64 μg/ml (Akihisa et al., 2005).
Conclusion
Taraxasterol is a natural pentacyclic triterpene primarily extracted from dandelion. This article gives a general overview of the pharmacological activities of taraxasterol for treating various illnesses, such as respiratory, gastrointestinal, and urinary disorders. Taraxasterol was discovered to have excellent potential for preventing the above disorders. Anti-inflammatory, anti-oxidative, and anti-carcinogenic mechanisms may be responsible for its protective effects.
Neurodegenerative diseases, including Alzheimer’s disease (AD), Parkinson’s disease (PD), and Huntington’s disease (HD), cause progressive damage to the nervous system. The abnormal aggregation of some proteins in particular brain regions is a pathological hallmark of neurodegenerative diseases mainly caused by impaired protein-degradation systems, such as the autophagy-lysosome pathway (ALP) and the ubiquitin-proteasome system (UPS) (Zheng et al., 2014; Finkbeiner, 2020). Recently, it was found that taraxasterol promoted the degradation of RNF31 protein by enhancing autophagy and further alleviating the degradation of p53 through proteasome, indicating that taraxasterol may regulate the protein degradation pathways in cells (Tang et al., 2021). It is necessary to conduct more in vivo and in vitro studies to determine whether taraxasterol prompts the degradation of aggregate proteins by regulating ALP and UPS pathways in neurodegenerative diseases. Previous studies have shown that inflammatory stimulation can be active by genetic mutation and protein aggregation in neurodegenerative diseases (Glass et al., 2010; Stephenson et al., 2018). Microglia and astrocytes are mainly responsible for persistent inflammatory responses (Xu et al., 2016; Stephenson et al., 2018). Taraxasterol may have effects on inhibiting the inflammatory response induced by glial cells and potentially protective effect on neuronal death caused by abnormal activation of glial cells in neurodegenerative diseases, according to recent research conducted in vitro. Taraxasterol inhibited the expression of proinflammatory factors via the activation of LXR in LPS-stimulated BV2 microglia (Liu et al., 2018). However, taraxasterol can directly act on glial cells through the blood–brain barrier (BBB), and its concentration in the cerebrospinal fluid requires further investigation.
Additional research is also necessary to identify the effective concentration of taraxasterol in plasma. Zhang et al. reported that the amount of taraxasterol in the plasma of rats following oral administration could be accurately detected through a highly selective and sensitive liquid chromatography/tandem mass spectrometry (Zhang et al., 2015). This finding may aid in the pharmacokinetic study of taraxasterol in humans and other animals. Furthermore, more clinical studies are necessary on the metabolism, bioavailability, and safety of taraxasterol to support its applications in pharmaceuticals and medicine.
Author contributions
FJ contributed to the study design and wrote the manuscript. ZT, ZY, BZ, XS, and LM revised and approved the manuscript. All authors reviewed and approved the manuscript.
Funding
This work was supported by the Shandong Traditional Chinese Medicine Science and Technology Development Plan (Grant number: 2019-0446 to FJ), the Research Fund for Academician Lin He New Medicine (Grant number: JYHL2019ZD04 to FJ), and Undergraduate Innovation Training Project (Grant number: S202010443008 to BZ).
Conflict of interest
The authors declare that the research was conducted in the absence of any commercial or financial relationships that could be construed as a potential conflict of interest.
Publisher’s note
All claims expressed in this article are solely those of the authors and do not necessarily represent those of their affiliated organizations or those of the publisher, the editors, and the reviewers. Any product that may be evaluated in this article, or claim that may be made by its manufacturer, is not guaranteed or endorsed by the publisher.
References
Akashi, T., Furuno, T., Takahashi, T., and Ayabe, S.-I. (1994). Biosynthesis of triterpenoids in cultured cells, and regenerated and wild plant organs of Taraxacum officinale. Phytochemistry 36 (2), 303–308. doi:10.1016/S0031-9422(00)97065-1
Akihisa, T., Franzblau, S. G., Ukiya, M., Okuda, H., Zhang, F., Yasukawa, K., et al. (2005). Antitubercular activity of triterpenoids from Asteraceae flowers. Biol. Pharm. Bull. 28 (1), 158–160. doi:10.1248/bpb.28.158
Akihisa, T., Yasukawa, K., Oinuma, H., Kasahara, Y., Yamanouchi, S., Takido, M., et al. (1996). Triterpene alcohols from the flowers of compositae and their anti-inflammatory effects. Phytochemistry 43 (6), 1255–1260. doi:10.1016/s0031-9422(96)00343-3
Ames, T. R., Beton, J. L., Bowers, A., Halsall, T. G., and Jones, E. (1954). The chemistry of the triterpenes and related compounds Part XXIII the structure of taraxasterol ψ-taraxasterol (heterolupeol) and lupenol-I. J. Chem. Soc. 1954 (25), 307–318. doi:10.1039/jr9540001902
Apel, K., and Hirt, H. (2004). Reactive oxygen species: metabolism, oxidative stress, and signal transduction. Annu. Rev. Plant Biol. 55, 373–399. doi:10.1146/annurev.arplant.55.031903.141701
Bajaj, Y. (1994). Medicinal and aromatic plants. Biotechnol. Agric. For. 51, 1232. doi:10.1007/978-3-642-73617-9
Bao, T., Ke, Y., Wang, Y., Wang, W., Li, Y., Wang, Y., et al. (2018). Taraxasterol suppresses the growth of human liver cancer by upregulating Hint1 expression. J. Mol. Med. 96 (7), 661–672. doi:10.1007/s00109-018-1652-7
Boutaghane, N., Voutquenne-Nazabadioko, L., Simon, A., Harakat, D., Benlabed, K., Kabouche, Z., et al. (2013). A new triterpenic diester from the aerial parts of Chrysanthemum macrocarpum. Phytochem. Lett. 6 (4), 519–525. doi:10.1016/j.phytol.2013.06.009
Burrows, S., and Simpson, J. C. E. (1938). 389 the triterpene group. Part IV. The triterpene alcohols of Taraxacum root. J. Chem. Soc., 2042–2047. doi:10.1039/JR9380002042
Chauhan, R., Ruby, K., and Dwivedi, J. (2012). Golden herbs used in piles treatment: A concise report. Int. J. Drug Dev. Res. 4 (4), 50–68.
Che, L., Li, Y., Song, R., Qin, C., Hao, W., Wang, B., et al. (2019). Anti-inflammatory and anti-apoptosis activity of taraxasterol in ulcerative colitis in vitro and in vivo. Exp. Ther. Med. 18 (3), 1745–1751. doi:10.3892/etm.2019.7736
Chen, H., Xie, K., Han, H., Li, Y., Liu, L., Yang, T., et al. (2015). Molecular hydrogen protects mice against polymicrobial sepsis by ameliorating endothelial dysfunction via an Nrf2/HO-1 signaling pathway. Int. Immunopharmacol. 28 (1), 643–654. doi:10.1016/j.intimp.2015.07.034
Chen, J., Wu, W., Zhang, M., and Chen, C. (2019a). Taraxasterol suppresses inflammation in IL-1β-induced rheumatoid arthritis fibroblast-like synoviocytes and rheumatoid arthritis progression in mice.. Int. Immunopharmacol. 70, 274–283. doi:10.1016/j.intimp.2019.02.029
Chen, W., Da, W., Li, C., Fan, H., Liang, R., Yuan, J., et al. (2019b). Network pharmacology-based identification of the protective mechanisms of taraxasterol in experimental colitis. Int. Immunopharmacol. 71, 259–266. doi:10.1016/j.intimp.2019.03.042
Chen, W., Li, J., Li, C., Fan, H. N., Zhang, J., Zhu, J. S., et al. (2020). Network pharmacology-based identification of the antitumor effects of taraxasterol in gastric cancer. Int. J. Immunopathol. Pharmacol. 34, 2058738420933107. doi:10.1177/2058738420933107
Choi, H. S., Han, J. Y., and Choi, Y. E. (2020). Identification of triterpenes and functional characterization of oxidosqualene cyclases involved in triterpene biosynthesis in lettuce (Lactuca sativa). Plant Sci. 301, 110656. doi:10.1016/j.plantsci.2020.110656
Dai, J., Zhao, C., Zhang, Q., Liu, Z. L., Zheng, R., Yang, L., et al. (2001). Taraxastane-type triterpenoids from Saussurea petrovii. Phytochemistry 58 (7), 1107–1111. doi:10.1016/s0031-9422(01)00397-1
De Amorim, M., Godinho, W., Archanjo, F., and Grael, C. (2016). Chemical constituents of pseudobrickellia brasiliensis leaves (spreng.) RM king & H. Rob (asteraceae). Rev. bras. plantas med. 18, 408–414. doi:10.1590/1983-084X/15_185
Della Loggia, R., Tubaro, A., Sosa, S., Becker, H., Saar, S., and Isaac, O. (1994). The role of triterpenoids in the topical anti-inflammatory activity of Calendula officinalis flowers. Planta Med. 60 (6), 516–520. doi:10.1055/s-2006-959562
Desreumaux, P., Dubuquoy, L., Nutten, S., Peuchmaur, M., Englaro, W., Schoonjans, K., et al. (2001). Attenuation of colon inflammation through activators of the retinoid X receptor (RXR)/peroxisome proliferator-activated receptor gamma (PPARgamma) heterodimer. A basis for new therapeutic strategies. J. Exp. Med. 193 (7), 827–838. doi:10.1084/jem.193.7.827
Finkbeiner, S. (2020). The autophagy lysosomal pathway and neurodegeneration. Cold Spring Harb. Perspect. Biol. 12 (3), a033993. doi:10.1101/cshperspect.a033993
Furuno, T., Kamiyama, A., Akashi, T., Usui, M., Takahashi, T., Ayabe, S.-i., et al. (1993). Triterpenoid constituents of tissue cultures and regenerated organs of Taraxacum officinale. Plant tissue Cult. Lett. 10 (3), 275–280. doi:10.5511/plantbiotechnology1984.10.275
Ganeva, Y., Chanev, C., Dentchev, T., and Vitanova, D. (2003). Triterpenoids and sterols from hypericwm perforatum. Comptes Rendus l'Academie Bulg. Sci. 56 (4), 437.
Gawronska-Grzywacz, M., and Krzaczek, T. (2007). Identification and determination of triterpenoids in Hieracium pilosella L. J. Sep. Sci. 30 (5), 746–750. doi:10.1002/jssc.200600253
Glass, C. K., Saijo, K., Winner, B., Marchetto, M. C., and Gage, F. H. (2010). Mechanisms underlying inflammation in neurodegeneration. Cell 140 (6), 918–934. doi:10.1016/j.cell.2010.02.016
Gonzalez-Castejon, M., Visioli, F., and Rodriguez-Casado, A. (2012). Diverse biological activities of dandelion. Nutr. Rev. 70 (9), 534–547. doi:10.1111/j.1753-4887.2012.00509.x
Gudaitytė, O., and Venskutonis, P. R. (2007). Chemotypes of Achillea millefolium transferred from 14 different locations in Lithuania to the controlled environment. Biochem. Syst. Ecol. 35 (9), 582–592. doi:10.1016/j.bse.2007.03.016
Guo, J. B., Ye, H. H., and Chen, J. F. (2015b). Anti-proliferation effect of Taraxacum mongolicum extract in HepG2 cells and its mechanism. Zhong Yao Cai 38 (10), 2129–2133. doi:10.13863/j.issn1001-4454.2015.10.028
Guo, J., Liu, X., and Wang, M. (2015a). miR-503 suppresses tumor cell proliferation and metastasis by directly targeting RNF31 in prostate cancer. Biochem. Biophys. Res. Commun. 464 (4), 1302–1308. doi:10.1016/j.bbrc.2015.07.127
Gurib-Fakim, A. (2006). Medicinal plants: traditions of yesterday and drugs of tomorrow. Mol. Asp. Med. 27 (1), 1–93. doi:10.1016/j.mam.2005.07.008
Haladova, M., Eisenreichova, E., Budesinsky, M., Ubik, K., and Grancai, D. (2001). Constituents of the leaves of holodiscus discolor (pursh) maxim. Ceska Slov. Farm. 50 (6), 280–282.
He, Y., Jiang, K., and Zhao, X. (2020). Taraxasterol protects hippocampal neurons from oxygen-glucose deprivation-induced injury through activation of Nrf2 signalling pathway. Artif. Cells Nanomed. Biotechnol. 48 (1), 252–258. doi:10.1080/21691401.2019.1699831
Itoh, T., Uetsuki, T., Tamura, T., and Matsumoto, T. (1980). Characterization of triterpene alcohols of seed oils from some species of theaceae, phytolaccaceae and sapotaceae. Lipids 15 (6), 407–411. doi:10.1007/BF02534064
Jakubczyk, K., Dec, K., Kaldunska, J., Kawczuga, D., Kochman, J., Janda, K., et al. (2020). Reactive oxygen species - sources, functions, oxidative damage. Pol. Merkur. Lek. 48 (284), 124–127.
Jassbi, A. R., Firuzi, O., Miri, R., Salhei, S., Zare, S., Zare, M., et al. (2016). Cytotoxic activity and chemical constituents of Anthemis mirheydari. Pharm. Biol. 54 (10), 2044–2049. doi:10.3109/13880209.2016.1141220
Kamboj, A., and Saluja, A. (2009). Bryophyllum pinnatum (lam.) kurz.: Phytochemical and pharmacological profile: A review. Pharmacogn. Rev. 3 (6), 364.
Koo, H. N., Hong, S. H., Song, B. K., Kim, C. H., Yoo, Y. H., Kim, H. M., et al. (2004). Taraxacum officinale induces cytotoxicity through TNF-alpha and IL-1alpha secretion in Hep G2 cells. Life Sci. 74 (9), 1149–1157. doi:10.1016/j.lfs.2003.07.030
Lawrence, T. (2009). The nuclear factor NF-kappaB pathway in inflammation. Cold Spring Harb. Perspect. Biol. 1 (6), a001651. doi:10.1101/cshperspect.a001651
Lee, D. Y., Jung, L., Park, J. H., Yoo, K. H., Chung, I. S., Baek, N. I., et al. (2010). CYTOTOXIC TRITERPENOIDS FROM Cornus kousa FRUITS. Chem. Nat. Compd. 46 (1), 142–145. doi:10.1007/s10600-010-9550-4
Lehmann, J. M., Kliewer, S. A., Moore, L. B., Smith-Oliver, T. A., Oliver, B. B., Su, J. L., et al. (1997). Activation of the nuclear receptor LXR by oxysterols defines a new hormone response pathway. J. Biol. Chem. 272 (6), 3137–3140. doi:10.1074/jbc.272.6.3137
Li, C., Zheng, Z., Xie, Y., Zhu, N., Bao, J., Yu, Q., et al. (2020a). Protective effect of taraxasterol on ischemia/reperfusion-induced acute kidney injury via inhibition of oxidative stress, inflammation, and apoptosis. Int. Immunopharmacol. 89 (Pt A), 107169. doi:10.1016/j.intimp.2020.107169
Li, Z., Lian, Y., Wei, R., Jin, L., Cao, H., Zhao, T., et al. (2020b). Effects of taraxasterol against ethanol and high-fat diet-induced liver injury by regulating TLR4/MyD88/NF-κB and Nrf2/HO-1 signaling pathways.. Life Sci. 262, 118546. doi:10.1016/j.lfs.2020.118546
Liu, B., He, Z., Wang, J., Xin, Z., Wang, J., Li, F., et al. (2018). Taraxasterol inhibits LPS-induced inflammatory response in BV2 microglia cells by activating LXRα.. Front. Pharmacol. 9, 278. doi:10.3389/fphar.2018.00278
Liu, H., Sun, X., Sun, M., and Jin, C. (2020). Effects of taraxasterol on inflammatory factor levels and morphology of thymus in acne model of mice through TGF-β/Smad pathway. J. Hebei Med. Univ. 41, 810–814. doi:10.3969/ji.ssn.1007-3205.2020.07.015
Liu, J., Xiong, H., Cheng, Y., Cui, C., Zhang, X., Xu, L., et al. (2013). Effects of taraxasterol on ovalbumin-induced allergic asthma in mice. J. Ethnopharmacol. 148 (3), 787–793. doi:10.1016/j.jep.2013.05.006
Medzhitov, R. (2008). Origin and physiological roles of inflammation. Nature 454 (7203), 428–435. doi:10.1038/nature07201
Ngo, S. T., and Li, M. S. (2013). Top-leads from natural products for treatment of alzheimer's disease: docking and molecular dynamics study. Mol. Simul. 39 (4), 279–291. doi:10.1080/08927022.2012.718769
Oliveira, P. A. d., Turatti, I. C. C., and Oliveira, D. C. R. d. (2006). Comparative analysis of triterpenoids from Mikania cordifolia collected from four different locations. Rev. Bras. Cienc. Farm. 42, 547–552. doi:10.1590/S1516-93322006000400010
Piao, T., Ma, Z., Li, X., and Liu, J. (2015). Taraxasterol inhibits IL-1β-induced inflammatory response in human osteoarthritic chondrocytes.. Eur. J. Pharmacol. 756, 38–42. doi:10.1016/j.ejphar.2015.03.012
Power, F. B., and Browning, H. (1912). CCLII.—the constituents of taraxacum root. J. Chem. Soc. Trans. 101, 2411–2429. doi:10.1039/ct9120102411
Qiu, P., Xu, T. J., Lu, X. D., Yang, W., Zhang, Y. B., Xu, G. M., et al. (2018). MicroRNA-378 regulates cell proliferation and migration by repressing RNF31 in pituitary adenoma. Oncol. Lett. 15 (1), 789–794. doi:10.3892/ol.2017.7431
Ren, Y. L., and Yang, J. S. (2001). Studies on chemical constituents from Chinese medicinal plant Hemistepta lyrata Bunge. Zhongguo Zhong Yao Za Zhi 26 (6), 405–406. doi:10.3321/j.issn:0513-4870.2001.10.007
Roy, M., and Singh, R. (2021). TRIMs: selective recruitment at different steps of the NF-κB pathway-determinant of activation or resolution of inflammation.. Cell. Mol. Life Sci. 78 (17-18), 6069–6086. doi:10.1007/s00018-021-03900-z
Ryu, S. B., and Lee, H.-Y. (2006). Methods for Agrobacterium-mediated transformation of dandelion. US patent application.
Saeecd, M. T., Agarwal, R., Khan, M., Ahmad, F., Matsumoto, T., Akihisa, T., et al. (1991). Unsaponifiable lipid constituents of ten indian seed oils. J. Am. Oil Chem. Soc. 68 (3), 193–197. doi:10.1007/bf02657768
San, Z., Fu, Y., Li, W., Zhou, E., Li, Y., Song, X., et al. (2014). Protective effect of taraxasterol on acute lung injury induced by lipopolysaccharide in mice. Int. Immunopharmacol. 19 (2), 342–350. doi:10.1016/j.intimp.2014.01.031
Sang, R., Yu, Y., Ge, B., Xu, L., Wang, Z., Zhang, X., et al. (2019). Taraxasterol from Taraxacum prevents concanavalin A-induced acute hepatic injury in mice via modulating TLRs/NF-κB and Bax/Bc1-2 signalling pathways.. Artif. Cells Nanomed. Biotechnol. 47 (1), 3929–3937. doi:10.1080/21691401.2019.1671433
Sharma, K., and Zafar, R. (2014). Simultaneous estimation of taraxerol and taraxasterol in root callus cultures of Taraxacum officinale Weber. Int. J. Pharmacogn. Phytochem. Res. 6 (3), 540–546.
Singh, B., Sahu, P. M., and Sharma, M. K. (2002). Anti-inflammatory and antimicrobial activities of triterpenoids from Strobilanthes callosus nees. Phytomedicine 9 (4), 355–359. doi:10.1078/0944-7113-00143
Stephenson, J., Nutma, E., van der Valk, P., and Amor, S. (2018). Inflammation in CNS neurodegenerative diseases. Immunology 154 (2), 204–219. doi:10.1111/imm.12922
Stiti, N., and Hartmann, M. A. (2012). Nonsterol triterpenoids as major constituents of Olea europaea. J. Lipids 2012, 476595. doi:10.1155/2012/476595
Storz, G., and Imlay, J. A. (1999). Oxidative stress. Curr. Opin. Microbiol. 2 (2), 188–194. doi:10.1016/s1369-5274(99)80033-2
Su, C. G., Wen, X., Bailey, S. T., Jiang, W., Rangwala, S. M., Keilbaugh, S. A., et al. (1999). A novel therapy for colitis utilizing PPAR-gamma ligands to inhibit the epithelial inflammatory response. J. Clin. Invest. 104 (4), 383–389. doi:10.1172/JCI7145
Suh, G. Y., Jin, Y., Yi, A. K., Wang, X. M., and Choi, A. M. (2006). CCAAT/enhancer-binding protein mediates carbon monoxide-induced suppression of cyclooxygenase-2. Am. J. Respir. Cell Mol. Biol. 35 (2), 220–226. doi:10.1165/rcmb.2005-0154OC
Sweeney, B., Vora, M., Ulbricht, C., and Basch, E. (2005). Evidence-based systematic review of dandelion (Taraxacum officinale) by natural standard research collaboration. J. Herb. Pharmacother. 5 (1), 79–93. doi:10.1080/j157v05n01_09
Takasaki, M., Konoshima, T., Tokuda, H., Masuda, K., Arai, Y., Shiojima, K., et al. (1999). Anti-carcinogenic activity of Taraxacum plant. II. Biol. Pharm. Bull. 22 (6), 606–610. doi:10.1248/bpb.22.606
Tang, C. T., Yang, J., Liu, Z. D., Chen, Y., and Zeng, C. (2021). Taraxasterol acetate targets RNF31 to inhibit RNF31/p53 axis-driven cell proliferation in colorectal cancer. Cell Death Discov. 7 (1), 66. doi:10.1038/s41420-021-00449-5
Thimmappa, R., Geisler, K., Louveau, T., O'Maille, P., and Osbourn, A. (2014). Triterpene biosynthesis in plants. Annu. Rev. Plant Biol. 65, 225–257. doi:10.1146/annurev-arplant-050312-120229
Thongkhao, K., Pongkittiphan, V., Phadungcharoen, T., Tungphatthong, C., Urumarudappa, S. K. J., Pengsuparp, T., et al. (2020). Differentiation of Cyanthillium cinereum, a smoking cessation herb, from its adulterant Emilia sonchifolia using macroscopic and microscopic examination, HPTLC profiles and DNA barcodes. Sci. Rep. 10 (1), 14753. doi:10.1038/s41598-020-71702-7
Valko, V., Fickova, M., Pravdova, E., Nagy, M., Grancai, D., Czigle, S., et al. (2006). Cytotoxicity of water extracts from leaves and branches of Philadelphus coronarius L. Biomed. Pap. Med. Fac. Univ. Palacky. Olomouc Czech. Repub. 150 (1), 71–73. doi:10.5507/bp.2006.007
Villarreal, M. L., Alvarez, L., Alonso, D., Navarro, V., Garcia, P., Delgado, G., et al. (1994). Cytotoxic and antimicrobial screening of selected terpenoids from Asteraceae species. J. Ethnopharmacol. 42 (1), 25–29. doi:10.1016/0378-8741(94)90019-1
Wang, L., Li, H., Zhang, Y., Santella, R. M., and Weinstein, I. B. (2009). HINT1 inhibits beta-catenin/TCF4, USF2 and NFkappaB activity in human hepatoma cells. Int. J. Cancer 124 (7), 1526–1534. doi:10.1002/ijc.24072
Wang, L., Zhang, Y., Li, H., Xu, Z., Santella, R. M., Weinstein, I. B., et al. (2007). Hint1 inhibits growth and activator protein-1 activity in human colon cancer cells. Cancer Res. 67 (10), 4700–4708. doi:10.1158/0008-5472.CAN-06-4645
Wang, S., Wang, Y., Liu, X., Guan, L., Yu, L., Zhang, X., et al. (2016). Anti-inflammatory and anti-arthritic effects of taraxasterol on adjuvant-induced arthritis in rats. J. Ethnopharmacol. 187, 42–48. doi:10.1016/j.jep.2016.04.031
Wang, W., Zhang, H., Wang, L., and Bao, T. (2018). Protctive effects of taraxasterol on oxidatively injured cardiomyocytes. Chongqing Yixue 47, 1572–1579.
Wang, Z., Guhling, O., Yao, R., Li, F., Yeats, T. H., Rose, J. K., et al. (2011). Two oxidosqualene cyclases responsible for biosynthesis of tomato fruit cuticular triterpenoids. Plant Physiol. 155 (1), 540–552. doi:10.1104/pp.110.162883
Wu, H., Xin, X., Su, Z., and Aisa, H. (2011). 2-Isopropyl-6-methylpyrimidin-4 (3H)-one and taraxasterol from the stems of Cichorium glandulosum. Chem. Nat. Compd. 47 (4), 664–666. doi:10.1007/s10600-011-0027-x
Xu, L., He, D., and Bai, Y. (2016). Microglia-mediated inflammation and neurodegenerative disease. Mol. Neurobiol. 53 (10), 6709–6715. doi:10.1007/s12035-015-9593-4
Xu, L., Yu, Y., Sang, R., Li, J., Ge, B., Zhang, X., et al. (2018). Protective effects of taraxasterol against ethanol-induced liver injury by regulating CYP2E1/nrf2/HO-1 and NF-κB signaling pathways in mice.. Oxid. Med. Cell. Longev. 2018, 8284107. doi:10.1155/2018/8284107
Xu, R., Fazio, G. C., and Matsuda, S. P. (2004). On the origins of triterpenoid skeletal diversity. Phytochemistry 65 (3), 261–291. doi:10.1016/j.phytochem.2003.11.014
Xueshibojie, L., Duo, Y., and Tiejun, W. (2016). Taraxasterol inhibits cigarette smoke-induced lung inflammation by inhibiting reactive oxygen species-induced TLR4 trafficking to lipid rafts. Eur. J. Pharmacol. 789, 301–307. doi:10.1016/j.ejphar.2016.07.047
Yang, D., Liu, X., Liu, M., Chi, H., Liu, J., Han, H., et al. (2015). Protective effects of quercetin and taraxasterol against H2O2-induced human umbilical vein endothelial cell injury in vitro. Exp. Ther. Med. 10 (4), 1253–1260. doi:10.3892/etm.2015.2713
Yang, L., Zheng, W., Lv, X., Xin, S., Sun, Y., Xu, T., et al. (2022). microRNA-144 modulates the NF-κB pathway in miiuy croaker (Miichthys miiuy) by targeting IκBα gene.. Dev. Comp. Immunol. 130, 104359. doi:10.1016/j.dci.2022.104359
Yang, X. Y., Wang, L. H., Chen, T., Hodge, D. R., Resau, J. H., DaSilva, L., et al. (2000). Activation of human T lymphocytes is inhibited by peroxisome proliferator-activated receptor gamma (PPARgamma) agonists. PPARgamma co-association with transcription factor NFAT. J. Biol. Chem. 275 (7), 4541–4544. doi:10.1074/jbc.275.7.4541
Yang, Y., Ying, G., Wu, S., Wu, F., and Chen, Z. (2020). In vitro inhibition effects of Hepatitis B virus by dandelion and taraxasterol. Infect. Agent. Cancer 15, 44. doi:10.1186/s13027-020-00309-4
Yao, F., Long, L. Y., Deng, Y. Z., Feng, Y. Y., Ying, G. Y., Bao, W. D., et al. (2014). RACK1 modulates NF-κB activation by interfering with the interaction between TRAF2 and the IKK complex.. Cell Res. 24 (3), 359–371. doi:10.1038/cr.2013.162
Yarnell, E., and Abascal, K. (2009). Dandelion (Taraxacum officinale and T. mongolicum). Integr. Med. 8 (2), 35–38.
Yasukawa, K., Akihisa, T., Oinuma, H., Kaminaga, T., Kanno, H., Kasahara, Y., et al. (1996). Inhibitory effect of taraxastane-type triterpenes on tumor promotion by 12-O-tetradecanoylphorbol-13-acetate in two-stage carcinogenesis in mouse skin. Oncology 53 (4), 341–344. doi:10.1159/000227584
Yasukawa, K., Matsubara, H., and Sano, Y. (2010). Inhibitory effect of the flowers of artichoke (Cynara cardunculus) on TPA-induced inflammation and tumor promotion in two-stage carcinogenesis in mouse skin. J. Nat. Med. 64 (3), 388–391. doi:10.1007/s11418-010-0403-z
Yoon, J. Y., Cho, H. S., Lee, J. J., Lee, H. J., Jun, S. Y., Lee, J. H., et al. (2016). Novel TRAIL sensitizer Taraxacum officinale F.H. Wigg enhances TRAIL-induced apoptosis in Huh7 cells. Mol. Carcinog. 55 (4), 387–396. doi:10.1002/mc.22288
Yousefi Ghale-Salimi, M., Eidi, M., Ghaemi, N., and Khavari-Nejad, R. A. (2018). Inhibitory effects of taraxasterol and aqueous extract of Taraxacum officinale on calcium oxalate crystallization: in vitro study. Ren. Fail. 40 (1), 298–305. doi:10.1080/0886022X.2018.1455595
Zhai, C., Shi, W., Feng, W., Zhu, Y., Wang, J., Li, S., et al. (2018). Activation of AMPK prevents monocrotaline-induced pulmonary arterial hypertension by suppression of NF-κB-mediated autophagy activation.. Life Sci. 208, 87–95. doi:10.1016/j.lfs.2018.07.018
Zhang, N., Pang, L., Dong, N., Xu, D., and Xu, H. (2015). Quantification of taraxasterol in rat plasma by LC/MS/MS: Application to a pharmacokinetic study. Biomed. Chromatogr. 29 (11), 1643–1649. doi:10.1002/bmc.3473
Zhang, X., Xiong, H., Li, H., and Cheng, Y. (2014). Protective effect of taraxasterol against LPS-induced endotoxic shock by modulating inflammatory responses in mice. Immunopharmacol. Immunotoxicol. 36 (1), 11–16. doi:10.3109/08923973.2013.861482
Zhao, J., Evangelopoulos, D., Bhakta, S., Gray, A. I., and Seidel, V. (2014). Antitubercular activity of Arctium lappa and Tussilago farfara extracts and constituents. J. Ethnopharmacol. 155 (1), 796–800. doi:10.1016/j.jep.2014.06.034
Zheng, C., Geetha, T., and Babu, J. R. (2014). Failure of ubiquitin proteasome system: risk for neurodegenerative diseases. Neurodegener. Dis. 14 (4), 161–175. doi:10.1159/000367694
Zheng, F., Dong, X., and Meng, X. (2018). Anti-inflammatory effects of taraxasterol on LPS-stimulated human umbilical vein endothelial cells. Inflammation 41 (5), 1755–1761. doi:10.1007/s10753-018-0818-3
Keywords: taraxasterol, dandelion, botany, pharmacological profile, phytochemistry
Citation: Jiao F, Tan Z, Yu Z, Zhou B, Meng L and Shi X (2022) The phytochemical and pharmacological profile of taraxasterol. Front. Pharmacol. 13:927365. doi: 10.3389/fphar.2022.927365
Received: 24 April 2022; Accepted: 29 June 2022;
Published: 04 August 2022.
Edited by:
Helen Michelle McGettrick, University of Birmingham, United KingdomReviewed by:
Pooja Sharma, Punjabi University, IndiaDariusz Jedrejek, Institute of Soil Science and Plant Cultivation, Poland
Copyright © 2022 Jiao, Tan, Yu, Zhou, Meng and Shi. This is an open-access article distributed under the terms of the Creative Commons Attribution License (CC BY). The use, distribution or reproduction in other forums is permitted, provided the original author(s) and the copyright owner(s) are credited and that the original publication in this journal is cited, in accordance with accepted academic practice. No use, distribution or reproduction is permitted which does not comply with these terms.
*Correspondence: Fengjuan Jiao, amlhb2ZlbmdqdWFuODUxNkAxNjMuY29t