- 1Co-Innovation Center for Sustainable Forestry in Southern China and Key Laboratory of National Forestry and Grassland Administration on Subtropical Forest Biodiversity Conservation, College of Biology and the Environment, Nanjing Forestry University, Nanjing, China
- 2International Cultivar Registration Center for Osmanthus, College of Biology and the Environment, Nanjing Forestry University, Nanjing, China
- 3Department of Chinese Medicine, The University of Hong Kong-Shenzhen Hospital, Shenzhen, China
- 4RCI Research Institute Limited, Hong Kong, China
- 5Department of Pharmacology, University of Cambridge, Cambridge, United Kingdom
Osmanthus fragrans (scientific name: Osmanthus fragrans (Thunb.) Lour.) is a species of the Osmanthus genus in the family Oleaceae, and it has a long history of cultivation in China. O. fragrans is edible and is well known for conferring a natural fragrance to desserts. This flowering plant has long been cultivated for ornamental purposes. Most contemporary literature related to O. fragrans focuses on its edible value and new species discovery, but the functional use of O. fragrans is often neglected. O, fragrans has many properties that are beneficial to human health, and its roots, stems, leaves, flowers and fruits have medicinal value. These characteristics are recorded in the classics of traditional Chinese medicine. Studies on the metabolites and medicinal value of O. fragrans published in recent years were used in this study to evaluate the medicinal value of O. fragrans. Using keywords such as metabolites and Osmanthus fragrans, a systematic and nonexhaustive search of articles, papers and books related to the medicinal use of Osmanthus fragrans metabolites was conducted. Fifteen metabolites were identified through this literature search and classified into three categories according to their properties and structure: flavonoids, terpenes and phenolic acids. It was found that the pharmacological activities of these secondary metabolites mainly include antioxidant, anticancer, anti-inflammatory and antibacterial activities and that these metabolites can be used to treat many human diseases, such as cancer, skin diseases, cardiovascular diseases, and neurological diseases. Most of the reports that are currently available and concern the secondary metabolites of Osmanthus fragrans have limitations. Some reports introduce only the general classification of compounds in Osmanthus fragrans, and some reports introduce only a single compound. In contrast, the introduction section of this paper includes both the category and the functional value of each compound. While reviewing the data for this study, the authors found that the specific action sites of these compounds and their mechanisms of action in plants are relatively weak, and in the future, additional research should be conducted to investigate this topic further.
Introduction
Introduction to Osmanthus fragrans
O. fragrans is widely cultivated in China and was documented in the ancient pharmacopeia. During the Ming Dynasty (AD 1368–1644), the medicinal functions of O. fragrans were documented in Li Shizhen’s monumental pharmaceutical encyclopaedia Compendium of Materia Medica. O. fragrans is classified as a pungent, warm and nonpoisonous Chinese herb. It is used to promote saliva secretion, deodorize and clear phlegm. It is believed to be effective in the treatment of toothaches. Descriptions of the uses of O. fragrans, such as “O. fragrans root can be used to treat toothache due to virtual fire,” “O. fragrans flower can be used to treat bad breath” and “O. fragrans fresh bark or root bark is applied to the injured area to treat sprains” are found in the Compendium of Materia Medica. In recent years, modern scientific and technological strategies have been used to further study O. fragrans. For example, it has been found that the essential oil of O. fragrans, which is a volatile component, mostly contains terpenoids, as well as flavonoids and other glycosides, pigments, etc., which have anticancer, antioxidant, anti-inflammatory, analgesic, and cardiovascular effects (Li and Huang, 2020; Cheng, 2021). There are currently approximately one hundred varieties of O. fragrans, which can be divided into four categories, namely, the Luteus group, Albus group, Aurantiacus group, and Asiatiacus group, according to their traits and characteristics. O. fragrans is an evergreen shrub in the Oleaceae family. It is a deciduous tree with flowers that grow in small panicles. O. fragrans flowers can be produced in the spring, summer and autumn, but its peak flowering time is usually approximately September to early October. O. fragrans has the nickname “Jiulixiang,” which literally means “nine Chinese miles fragrant” because of its far-reaching scent. This property makes O. fragrans a common choice for achieving a strong and long-lasting fragrance in foods (dessert, pastry, tea and wine) as well as in producing perfumes and cosmetics. It has been reported in the literature that the terpenoids in the leaves of O. fragrans can inhibit the production of melanin (Yang, et al., 2021). Excessive deposition of melanin in the human body can lead to various skin diseases; thus, O. fragrans is used in the cosmetic industry to whiten the skin and alleviate skin diseases (Le, et al., 2022).
The medicinal uses of O. fragrans vary depending on the metabolite content of the plant. The flavonoid synthesis and carotenoid synthesis pathways are similar in the four categories of O. fragrans. However, due to variations in floral fragrance, flower colour, and genetic characteristics, the varieties in each category exhibit slight differences in metabolic pathways. Therefore, the types and quantities of anabolic metabolites in these different varieties are not the same. In terms of floral fragrance, α-ionone and β-ionone are the most important aroma-producing substances in O. fragrans. As the most aromatic varieties, varieties in the Albus group possesses the highest levels of α- and β-ionone, followed by those in the Asiatiacus group, those in the Aurantiacus group and those in the Luteus group. The varieties in the Luteus and Asiatiacus groups are sweet and greasy, and those in the Aurantiacus group have the weakest aroma among those in all the groups.
α-Carotene and β-carotene play a major role in determining the flower colour of O. fragrans. Varieties in the Aurantiacus group contain both α-carotene and β-carotene, making them orange–red. Varieties in the Luteus group conta only one (α- or β-carotene). Varieties in the Asiatiacus group may contain α-carotene or β-carotene, but those in the Albus group do not. Therefore, varieties in the Luteus group are mostly yellow, those in the Albus group are mostly light yellow, those in the Aurantiacus group are mostly orange–red, and those in the Asiatiacus group are between the colour of varieties in the Luteus and those in the Albus group, with a slightly greater similarity towards the colour of those in the Luteus group (Wang, 2009; Dan, 2014). Therefore, in most cases, we can distinguish O. fragrans varieties by flower colour.
Medicinal Value of O. fragrans Metabolites
There are many metabolism pathways that occur throughout the lifespan in O. fragrans, and these pathways lead to the generation of many secondary metabolites. These secondary metabolites have some degree of medicinal value; however, there have been few studies on their pharmacological mechanisms. In recent years, metabolomics has become an increasingly popular technique for performing more vigorous research on medicinal plants. Metabolomic studies have shown that the roots, stems, leaves, flowers, and fruits of O. fragrans have medicinal properties. These characteristics were recorded in the classics of traditional Chinese medicine. O. fragrans roots can be used to treat rheumatic numbness and low back pain. O. fragrans flower extract can also be used to treat bad breath (Wu, et al., 2017; Li et al., 2020c). The extraction methods used for different parts of O. fragrans are also different. An ultrasonic-assisted extraction method, during which the seeds are peeled, dried in a drying oven, and finally pulverized into powder for use, is used for extraction of O. fragrans seeds. Fresh O. fragrans is often extracted by the ethanol reflux method. Like seeds, fresh O. fragrans is first dried, crushed, and finally stored (Li et al., 2020b; Ning, et al., 2020). Liao et al. used HPLC–MS to analyse the biological activity of O. fragrans root and identified dozens of compounds in O. fragrans root, including the first reported compound. The results of network pharmacology showed that O. fragrans root is effective in the treatment of inflammation, cardiovascular disease, cancer and rheumatoid arthritis, which is consistent with traditional claims of the medicinal value of O. fragrans root (Liao, et al., 2021). In the latest reports on O. fragrans, many new compounds have been reported, and O. fragrans extracts have been shown to have a variety of biological activities in vitro and in vivo. O. fragrans extract is rich in flavonoids [dihydroquercetin (DHQ), luteolin, naringenin, etc.), phenolic acids (tyrosol (Ty), rosmarinic acid (RA), protocatechualdehyde (PCA), etc.], terpenoids (loganic acid, oleanolic acid, maslinic acid, etc.), glycosides (salidroside, salidroside, etc.), fatty acids (oleic acid, linoleic acid, palmitic acid, etc.), pigments (melanin, red pigment, etc.) and many other active ingredients, and O. fragrans is the plant that contains the most flavonoids (Song et al., 2021a; Wang, et al., 2022). To evaluate the medicinal value of O. fragrans, 15 natural metabolites with biological activity were identified according to the metabolic pathways in O. fragrans through a search of studies on the synthetic pathways and chemical structures of the metabolites (Figure 1). These 15 O. fragrans metabolites can be classified into three categories based on their structure: 1) flavonoids (diosmetin (DIO), DHQ, astilbin, luteolin, and naringenin), 2) terpenoids (loganic acid, oleoside, secoxyloganin, oleanolic acid, and maslinic acid), and 3) phenolic acids (Ty, p-hydroxyphenylacetic acid, RA, salicylic acid (SA), and PCA) (Wu, et al., 2017; Yang, et al., 2021). Although some of these metabolites are not unique to O. fragrans, their medicinal effects are still of research value and may shed light on their therapeutic value. Recently, a review article published by Wang et al. introduces O. fragrans in several aspects, including botanical description, geographic distribution, traditional uses, phytochemical compositions, and pharmacological properties. However, the molecular mechanisms of these active metabolites remain unclassified. To better understand the medicinal value of secondary metabolites in O. fragrans, this article selected 15 representative secondary metabolites and introduced them from the molecular level: 1) to study their potential synthetic pathways in O. fragrans and regulatory mechanisms, 2) to understand their related physicochemical properties and synthetic pathways, 3) to study how they can treat related diseases through signal transduction (Table 1).
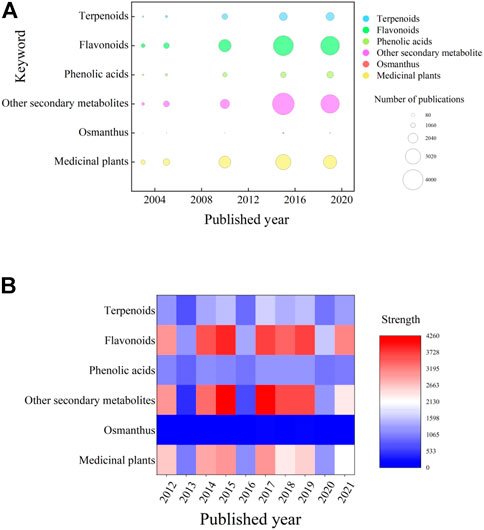
FIGURE 1. Summary of the literature according to keywork and the year published over the last 10 years. (A) Bubble chart showing the number of publications in 5 years from the past 10 years. The horizontal axis is the publication year, and the vertical axis is the relevant keyword used to search the literature. Correlative studies are relatively weak, and studies of various secondary metabolites are clearly differentiated. (B) Heatmap showing the prevalence of keywords in publications over the past 10 years. The figure shows that the research statuses of the three major categories of secondary metabolites selected in this paper are very different, with research on flavonoids being the most extensive and research on phenolic acids being the least extensive. (The basic principle of the author’s preliminary background investigation before writing this manuscript is to first conduct a general search on the research progress of O. fragrans, select compounds extracted from O. fragrans, and then screen out relatively important secondary metabolites of O. fragrans).
Classification and Overview of Secondary Metabolites
Flavonoids
Flavonoids are an important type of secondary metabolite in O. fragrans. By assessing the iron ion reduction ability of the flavonoids in O. fragrans and by using the two most commonly used antioxidant assays (the DPPH and ABTS assays), Bao and others showed that these flavonoids show good antioxidant activity (Bao, et al., 2018). Flavonoids are a class of natural compounds with low toxicity and few side effects, which make them more preferable drug candidates than other secondary metabolites of O. fragrans. Therefore, research on flavonoids may help determine the potential side effects of drugs containing these metabolites (Figure 2A).
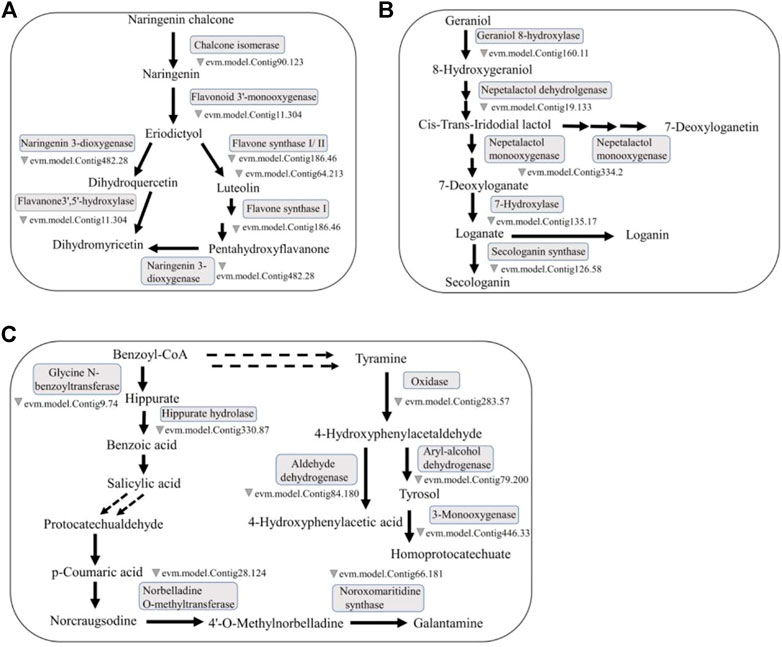
FIGURE 2. Representative medicinal compounds in O. fragrans and their biosynthetic pathways. (A) The main synthesis pathway containing naringenin, luteolin and DHQ in the flavonoid biosynthesis pathway is presented. Naringenin can produce luteolin and DHQ through reactions catalysed by different enzymes. (B) Loganic acid is a terpenoid. The synthetic pathway of loganic acid in the monoterpene biosynthesis pathway. Loganic acid can be produced from geraniol through reactions catalysed by a series of enzymes. (C) SA, PCA and Ty are synthesized via three different phenolic acid biosynthesis pathways, but the synthetic pathway of SA can be connected to the synthetic pathways of PCA and Ty. The double dotted arrow in the figure represents the connection between the pathways. (The relevant O. fragrans gene information in this biosynthetic pathway map comes from an article about O. fragrans published by) (Yang, et al., 2018).
Diosmetin
Dio is a metabolite found in the petals of O. fragrans. It can be obtained through a series of chemical reactions using hesperidin as the precursor compound. Dio is an aglycone of the flavonoid glycoside geraniol (Tang and Zhao, 2014; Ma, et al., 2018). Dio, a natural compound with a molecular formula of C16H12O6, mainly exists in plants in the free form or as a glycoside and is normally a yellow powder at room temperature. Its mechanisms of action in the treatment of different diseases are distinct and need to be analysed according to the specific situation. Studies have found that geraniol has anti-inflammatory, antibacterial, anticancer, and antioxidant properties (Si, et al., 2020). Animal studies have shown that Dio can stimulate MH7A cells and increase the apoptosis rate to affect the activation of the PI3K/Akt and NF-κB signalling pathways, thereby inhibiting the development of myocardial infarction (Chen, et al., 2020c) (Figure 3A). On this basis, Wang et al. further found that geraniol can alleviate isoproterenol-induced symptoms of cardiac hypertrophy in mice, potentially reducing the risk of heart disease (Dong, et al., 2020).
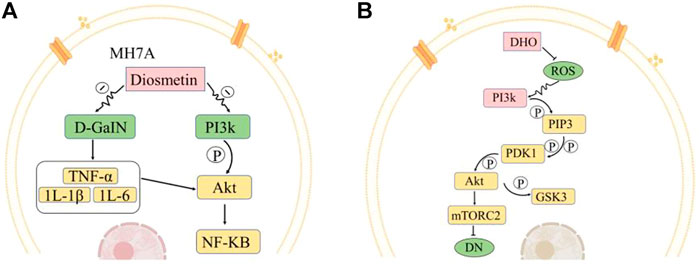
FIGURE 3. Representative medicinal compounds in O. fragrans and their pharmacological mechanisms of action. (A) Pathways by which Dio affects MH7A cell apoptosis. Dio inhibits the production of inflammatory mediators and cytokines (TNF-α, IL-1β, IL-6, etc.) induced by D-GalN and can also regulate the Akt/NF-kB pathway. Dio can also inhibit the PI3K/Akt pathway. PI3k is phosphorylated to generate Akt, and Akt is a key molecule in the activation of the NF-κB signalling pathway. Inhibition of this pathway reduces cell proliferation and inflammatory cytokine production in MH7A cells and promotes apoptosis. (B) Effects of DHQ on the mTORC2/Akt signalling pathway under high-glucose conditions. Reactive oxygen species (ROS) can activate the protein kinase PI3K signalling pathway. There are two downstream pathways of PI3k. In the one presented here, the PI3k-Akt signalling pathway affects mTORC2 and mTORC1. PI3k generates PIP3, PIP3 further activates PDK1, PDK1 activates Akt through phosphorylation, and Akt further activates mTORC2. DHQ has an inhibitory effect on ROS production. After the addition of DHQ, the activity of ROS is reduced, the regulatory effect of ROS on the PI3k pathway is weakened, the expression of Akt, which is downstream of PI3k, is decreased, and the expression of mTORC2, which is downstream of Akt, is inhibited. Akt phosphorylation can regulate GSK3 and reduce cell survival to ameliorate diabetic nephropathy in rats. (Red indicates increase/promotion, green indicates decrease/inhibition, and yellow indicates promotion of the original pathway but inhibition of this pathway.)
Dihydroquercetin
DHQ is an important flavonoid found in nature; Its molecular formula is C15H12O7, and it is generally pale yellow or colourless needle-like crystals, its melting point is 240°C, it is easily soluble in ethanol, acetic acid, boiling water and other solvents. DHQ is slightly soluble in cold water, it is almost insoluble in benzene, and it has no odour. The synthesis of DHQ involves two pathways: the phenylpropane pathway and the flavonoid pathway. Cinnamic acid is generated from phenylalanine by phenylalanine ammonia lyase, and then the generation of coumaric acid from cinnamic acid is catalysed by cinnamic acid hydroxylase. The production of naringenin chalcone from coumaric acid is catalysed by 4-coumarin-CoA ligase and chalcone synthase, and then the production of DHQ from naringenin chalcone is catalysed by chalcone isomerase and flavanone 3-hydroxylase dihydroflavonols. DHQ is found in the petals of O. fragrans, and scholars at home and abroad have found that DHQ has antioxidant, antiviral, anti-inflammatory, anti-allergic, anti-apoptotic, and antitumour properties along with other medicinal activities and protects the liver (Wang et al., 2011a; Xue, et al., 2017; Lin, et al., 2022). Oxidation and hyperglycaemia can also be affected by DHQ. Moreover, DHQ lowers blood pressure, alleviates cardiovascular diseases and can also repair brain tissue. and ameliorate brain injury and promote the differentiation of osteoblasts (Wang et al., 2011b). In addition, Ying et al. found that DHQ may reduce the symptoms of diabetic nephropathy in rats by affecting the mTORC2/Akt signalling pathway (Qiao, et al., 2020) (Figure 3B).
Astilbin
Astilbin is primarily found in the petals of O. fragrans. The molecular formula of astilbin, which exists as a white crystalline powder at room temperature that is easily soluble in methanol and ethanol and slightly soluble in water is C21H22O11. Recent studies have found that astilbin may have antidepressant, antioxidant, antidiabetic, analgesic and antibacterial properties, inhibit oedema, protect the liver and kidney, promote chondrocyte proliferation, and exert anti-inflammatory and immunosuppressive effects (Dan, 2014; Wei, et al., 2021). Li studied the mechanism underlying the protect effect of astilbin against cerebral ischaemia–reperfusion injury and found that it can activate the PI3K-Akt pathway and regulate the MAPK pathway (Yu, 2020). Xu studied the role and mechanism of astilbin in regulating Breg cells in inflammatory bowel disease and found that it can induce human PBMCs and mouse lymphocytes to secrete factors with regulatory effects, thereby suppressing the immune response, inhibiting cell apoptosis and preventing inflammation to alleviate ischaemia–reperfusion injury (Xu, 2020).
Luteolin
Luteolin is mostly found in the leaves of O. fragrans (Tang and Zhao, 2014). Luteolin is a natural flavonoid, and its molecular formula is C15H10O6. Luteolin usually exists as yellow needle-like crystals, has a melting point of approximately 330°C, and is weakly acidic, soluble in alkaline solution, slightly soluble in water, and stable under normal conditions. It is obtained by demethylation of geraniol via pyridine hydrochloride. It can also be produced by saccharol via flavonoid synthase I and flavonoid synthase II. Studies have found that luteolin has medicinal properties, such as antioxidant and antitumor properties, and that it protects the nervous system and enhances memory (Song, et al., 2015; Fu, et al., 2019). While screening for flavonoids with anti-gout effects, Hao found that luteolin can ameliorate hyperuricaemia and acute gouty arthritis by reducing the levels of inflammatory factors (TNF-α, IL-1β) in rats (Yue, 2019). Yang studied the effect of luteolin on acute liver injury induced by inorganic mercury in mice and found that luteolin can alleviate the symptoms of acute liver injury and anaemia caused by mercury (Yang, 2018).
Naringenin
Naringenin is a natural organic compound with a molecular formula of C15H12O5. It exists as a yellow powder and is soluble in ethanol, ether and benzene and almost insoluble in water. It should be stored in a cool, dry place away from light and high temperatures. Naringenin is produced from naringenin chalcone through chalcone isomerase. Naringenin is found not only in O. fragrans but also in Citrus sinensis, Pteris cretica, Lycopersicon esculentum Miller peel, Citrus aurantium Linnaeus, Allium fistulosum L., Dendrobium nobile Lindl. and other plants (Wu, et al., 2017; Lin, et al., 2022). According to the literature, naringenin has anti-inflammatory, antibacterial, antioxidant, antitumor, anti-lung damage, antiaging, and anticancer (anti-gastric cancer, anti-liver cancer, anti-breast cancer, anti-colon cancer, etc.) properties and can treat metabolic diseases such as diabetes, hypertension, and hyperlipidaemia (Zeng, et al., 2016; Jing, et al., 2019). Studies have also shown that naringenin can reduce apoptosis by activating the ER and the P13K/Akt signalling pathway (Qi, et al., 2020). β-Amyloid (Aβ25-35) alters the expression of phosphorylated Tau protein/total Tau protein in PC12 cells, thereby enhancing their proliferation. By studying the pharmacological mechanism of naringenin in the treatment of coronary heart disease, Wang and others found that naringenin inhibits coronary atherosclerosis and protects cardiomyocytes (Wang and Wang, 2021). Zeng and others found that naringenin has a similar structure as oestrogen- and can regulate the secondary messengers of downstream signalling pathways (the PKC pathway, AKT pathway, MAPK pathway, etc.) (Zeng, et al., 2018). Thus, naringenin plays a role in immune regulation. It has been used in formulas for the treatment of various diseases, such as Fuzheng Huayu tablets/capsules, Songhuafen Ganoderma lucidum tablets, Liujunzi decoction for the treatment of cervical cancer, and Shi Da Gonglao decoction for the treatment of hepatitis (Li et al., 2020b; Li et al., 2021b; Yang and Yang, 2021).
Terpenoids
O. fragrans has a unique fragrance, largely due to the presence of volatile components called terpenoids. O. fragrans flowers are used for the production of essential oils and raw materials for various spices. Recently, the medicinal effects of terpenoids in O. fragrans, e.g., their antioxidant and lipid-lowering properties, have attracted the attention of researchers (Yue, et al., 2013) (Figure 2B).
Loganic Acid
Loganic acid, which has a molecular formula of C16H24O10, is an iridoid glycoside compound that normally exists as a white crystalline powder. It should be stored at a temperature of 2–8°C and protected from light. If it is exposed to air for a long time, its physical and chemical properties are altered. Loganic acid is produced from 7-deoxypolysaccharide, and this reaction is catalysed by 7-deoxyxylan hydroxylase. It is present in the petals of O. fragrans (Tang and Zhao, 2014; Lv, 2021). and its medicinal properties primarily include anti-lipogenesis and anti-osteoporosis properties. Park et al. studied the effect of loganic acid on the adipogenesis of 3T3-L1 preadipocytes and cells in ovariectomy-induced obesity model mice and found that loganic acid can prevent the adipocyte differentiation of 3T3-L1 preadipocytes and cells in ovariectomized mice by reducing the expression of adipogenesis-inducing genes to exert an antiadipogenic effect (Park, et al., 2018). Park et al. found that loganic acid can increase osteoblast production and upregulated the expression of related genes to induce the differentiation of osteoblasts and prevent the differentiation of primary cultured osteoclasts, indicating that loganic acid may be a potential antiosteoporosis agent (Park, et al., 2020).
Oleoside
Oleoside is a natural iridoid glycoside compound with a molecular formula of C16H22O11. It normally exists as a powder and is mostly found in plants in the Oleaceae family. It has also been found in the flowers of O. fragrans (Yue, et al., 2013; Tang and Zhao, 2014; Lv, 2021). Oleoside promotes the activity of detoxification enzymes (such as SOD, CAT, GSR, GST, etc.), competes with coenzyme Q as the electron carrier in the mitochondrial electron transport chain, acts as a free radical scavenging antioxidant and inhibits lipid peroxidation to exerts antioxidation effects. Currently, there is little research on oleoside. Most oleosides currently available on the market are oleoside-11-methyl esters. Oleoside-11-methyl esters are used as reference substances in traditional Chinese medicine research and development. Because of the low output and good effect of oleoside-11-methyl esters, they are expensive. In addition, the better the packaging specifications are, the higher the purity and price are, with the price being up to 1400 yuan/5 mg. Oleosides have a very large potential use value, so future research on oleoside compounds is needed (Fei, et al., 2010; Castejón, et al., 2020; Araújo, et al., 2021).
Secoxyloganin
Secoxyloganin is found not only in the flowers of O. fragrans but also in Lonicera japonica Thunb. It is an iridoid compound (Lv, 2021) and has a molecular formula of C17H24O11. It normally exists as a solid and needs to be stored at low temperatures. Secoxyloganin has anti-inflammatory, vasoconstriction, antiviral, antitumour and antibacterial activities (Camargo, et al., 2020). While identifying the sensitizing components in reducing injection by ultrafiltration and high-performance liquid chromatography. Wang et al. found that chlorogenic acid can cause allergic reactions but that it has no obvious sensitizing effect (Wang, et al., 2016). There are few research materials for studying secoxyloganin, and related reagents are mostly used by research institutes and university laboratories. Although the market price of secoxyloganin is lower than that of oleoside-11-methyl, it is still more expensive than other compounds. Therefore, secoxyloganin has potential medicinal value and should be studied in the future scientific.
Oleanolic Acid
Oleanolic acid is a pentacyclic triterpenoid compound with a molecular formula of C30H48O3. It mainly exists in plants in the free form, normally exists as white needle-like crystals, and is odourless and tasteless. The melting point of oleanolic acid is approximately 310°C, and it is almost insoluble in water and slightly soluble in ethanol and chloroform. It is unstable in acids and bases. Oleanolic acid is produced from β-aromatic resin by oleanolic acid synthase and is mostly found in the flowers and fruits of O. fragrans (Wu, et al., 2017). Studies have found that oleanolic acid has antitumor effects, protects the liver, lowers enzyme activity, strengthens the heart, promotes liver cell regeneration, exerts anti-inflammatory effects, promotes lymphocyte proliferation, lowers blood lipid and blood sugar levels, inhibits platelet aggregation, and protects chromosomes from damage. In addition, studies have found that free oleanolic acid can scavenge DPPH free radicals and protect human umbilical vein endothelial cells from oxidative damage. Moreover, oleanolic acid has anticancer effects; for example, it can cause apoptosis of oesophageal cancer cells through the regulation of signalling pathways, and it has few adverse reactions and low toxicity. Therefore, this compound is a good pharmaceutical agent (Shen, et al., 2019; Li et al., 2021a; Zhang, et al., 2021).
Maslinic Acid
Maslinic acid is mostly found in the flowers and fruits of O. fragrans (Yang, et al., 2021). Maslinic acid is also a pentacyclic triterpenoid with a molecular formula of C30H48O4, and it usually exists as a light yellow powder. This compound lowers blood sugar levels, has anti-inflammatory and antifibrotic effects, and inhibits parasitic infections. It is effective in treating colon cancer, colorectal cancer, pancreatic cancer, lung cancer, etc. (Li, et al., 2017; Xuan, et al., 2021; Yang, et al., 2021). By studying the effects of maslinic acid on H2O2-induced oxidative damage to BRL-3A cells and the p38 and Nrf2/HO-1 pathways, Li et al. found that maslinic acid can alleviate oxidative damage induced by hydrogen peroxide (Li et al., 2020a). Hu et al. found that maslinic acid has a dose-dependent effect on the proliferation of HeLa cervical cancer cells; that is, the higher the dose of maslinic acid is, the poorer the proliferation ability of HeLa cells (Hu, et al., 2021).
Phenolic Acid Compounds
Many literature reviews have indicated that phenolic acid compounds, which are slightly less researched than terpenoids and flavonoids but are still important, are also present in O. fragrans. Most phenolic acid compounds have unstable structures and are easily affected by external biological, physical and chemical factors (Omar, 2010; Lin, et al., 2017; Ju, et al., 2019). They are also allelochemicals that interact with crops and are widely distributed in nature. The nature and concentration of phenolic acid compounds in different plant tissues are very different, and they have high medicinal value. For example, they can inhibit the proliferation, invasion and metastasis of liver cancer cells; promote the autophagy of liver cancer cells; exert anti-inflammatory, antioxidant, anti-allergic, antitumour, and antibacterial effects; and protect blood vessels (Hao, et al., 2017) (Figure 2C).
Tyrosol
The molecular formula of Ty is C8H10O2, and Ty normally exists as a yellow–green fine powder. Ty, which is a derivative of phenyl ethyl alcohol, is present in the flowers of O. fragrans. The precursor of Ty is generated from tyrosine by aromatic aldehyde synthase, and the Ty precursor is further synthesized into Ty by other enzymes (Wu, et al., 2017; Yang, et al., 2021). Ty is a mild compound that has a strong ability to scavenge highly toxic hydroxyl free radicals and can prevent the occurrence of coronary heart disease and tumours (García-Villalba, et al., 2015). Ty also has antioxidative, anti-inflammatory and antibacterial effects; lowers blood sugar levels; and increases longevity. It also has a therapeutic on common neurological diseases, such as Alzheimer’s disease and Parkinson’s disease. Studies have shown that the Ty content in the plasma reaches the peak level 2 h after ingestion, indicating that Ty conjugates are a major metabolite in the plasma (Shi, et al., 2020). Furthermore, Ty can promote the proliferation of endothelial cells (He, et al., 2017).
p-Hydroxyphenylacetic Acid
The molecular formula of p-hydroxyphenylacetic acid is C8H8O3, and it is normally exists as a white crystalline powder. It is slightly soluble in water and soluble in ether, ethanol and ethyl acetate. It should be stored in a cool dry place, and direct contact with the skin and eyes should be avoided during use. P-Hydroxyphenylacetic acid can be obtained through a series of chemical reactions between phenol and glyoxylic acid under alkaline conditions. It is an important rejuvenating herb. This compound is also present in many medicinal plants, such as Forsythia suspensa, Taraxacum mongolicum Hand.-Mazz, Rhodiola rosea L., Cedrus deodara (Roxb.) G. Don, and Pistacia chinensis Bunge, and a previous study reported that O. fragrans flowers contain this compound (Wei, et al., 2015; Wu, et al., 2017). P-Hydroxyphenyl acetic acid is also a raw material used to synthesize the anti-Parkinsonian drug pimavanserin (Hu, et al., 2020). Furthermore, it can also be used to synthesize the cardiovascular drug atenolol, which is used to treat various side effects caused by hypertension, and the analgesic and anti-inflammatory drug ibuprofen. It can even be used to synthesize the antibiotic drug laoxycephalosporin, which is used to alleviate the symptoms of antibiotic-sensitive bacterial infections, such as sepsis, bronchitis, cholecystitis and other types of inflammation (Peng, et al., 2014; Bai, 2016).
Rosmarinic Acid
RA is a water-soluble natural phenolic acid compound with a molecular formula of C18H16O8. It is a natural antioxidant with strong antioxidant activity. At low concentrations, it exists as a light yellow to brown powder, and at high concentrations, it exists as a white powder. It is more suitable for storage under acidic and low-temperature conditions. Since light has a strong impact on RA, it should be protected from light as much as possible during use. RA is produced from phenylalanine through a reaction catalysed by a series of enzymes, such as phenylalanine lyase and RA synthase, and is mostly found in the flowers of O. fragrans (Xue, et al., 2018; Lv, 2021). The currently recognized pharmacological activities of RA and its derivatives include antimicrobial (bacteria, fungi, viruses), anti-inflammatory (dermatitis, pneumonia, nephritis, periodontitis, arthritis, etc.), antitumour, antidepressant, and antioxidant properties. It can also prevent and treat allergic rhinitis induced by PM2.5 and inhibit the formation of kidney stones (Ke and Min, 2019; Zhou, et al., 2021). Lucia Cattaneo et al. found that rosemary extract inhibits the proliferation of A375 human melanoma cells and may induce apoptosis of colorectal cancer cells by interfering with the MAPK/ERK pathway (Cattaneo, et al., 2015).
Salicylic Acid
SA is a fat-soluble compound with a chemical formula of C7H6O3. It generally exists as a white needle-like crystal or hairy crystalline powder and has a melting point of approximately 159°C. It is stable at room temperature and as it has a corrosive effect, it can irritate the skin and mucous membranes. The main biosynthetic pathway of SA is the isochorismate synthase pathway, which is catalysed by chorismate synthase and isochorismate lyase, and SA is present in the flowers of O. fragrans (Zhang, 2008; Wang, et al., 2014). SA is widely used in many industries, specifically the pharmaceutical industry for the preparation of various drugs, such as aspirin, Zhitongling, sodium salicylate, and salicylamide. It is also used in the diagnosis and treatment of papules and pustular rosacea and can improve skin texture, and high concentrations of SA can exfoliate keratinocytes to achieve whitening; thus, it is often used to treat hair follicle sebaceous gland diseases (Cockayne, et al., 2011; Dayal, et al., 2020). SA can also prevent thrombosis, reduce blood viscosity, inhibit platelet aggregation and adhesion, reverse the hardening of blood vessels, alter vascular permeability, prevent arteriosclerosis, etc. (Cockayne, et al., 2011; Xin, et al., 2018; Ting, et al., 2021).
Protocatechualdehyde
The molecular formula of PCA is C7H6O3, and PCA exists as a light beige needle crystal or off-white powder. PCA has a melting point of 153°C and is soluble in cold water; easily soluble in ethanol, acetone, ethyl acetate, ether and hot water; and insoluble in benzene and chloroform. PCA can be prepared from p-hydroxybenzaldehyde through bromination, methoxylation, hydrolysis and other reactions, and it is mostly found in O. fragrans (Zhang, 2008; Xue, et al., 2018). Its pharmacological activity is relatively extensive, but studies have shown that it has some degree of toxicity. Thus, it is important to be careful when using this compound. PCA is known to inhibit inflammation, cell apoptosis, platelet aggregation, leukocyte chemotaxis and migration; exert antioxidative effects and effects against liver fibrosis, hepatitis B virus infection, and septicemia; promote microcirculation; reduces morphological abnormalities in acanthocytes; exert neuroprotection; hinder the formation of melanin; promote bone healing; and protect the myocardium by reducing calcium overload. It can also protect against cerebral ischaemia in rats by downregulating GFAP and AQP-4 protein expression in brain tissue when reperfusion injury does not disrupt neurovascular unit homeostasis (Zhang, et al., 2013; Jin, et al., 2021).
Conclusion and Prospects
O. fragrans is widely cultivated in China because it is beautiful and easy to grow. In addition, it is a relatively common tree species. It is mostly used as a street tree for ornamental purposes, and research on its use as a medicinal material is relatively limited. Many years ago, the pharmacological effects of O. fragrans was investigated, and this plant was used to treat diseases. At that time, people did not know what metabolites were, and metabolomics techniques were not available; however, researchers used observation and experimentation to identify the medicinal properties of various plants to eradicate disease sources. With advances in science and technology, the therapeutic efficacy of O. fragrans in clinical trials should be studied. If more human and financial resources are invested in scientific research in the future, this plant may also be used as a raw material for medicinal agents. The secondary metabolites mentioned in this article mainly have antioxidative, anticancer, anti-inflammatory and antibacterial activities (Table 2). However, more research is needed on the other pharmacological activities of these metabolites. While consulting the relevant literature on representative metabolites of O. fragrans, we found that the understanding of the synthetic pathways and mechanisms of these plants metabolites is incomplete, and more research on this topic is also needed. Therefore, we need to spend more time researching the metabolites of O. fragrans. At present, advanced molecular biotechnology methods, such as variable splicing and SWATH-MS technology, have been applied to study woody plants (Chen, et al., 2020a; Chen, et al., 2021b). Alternative splicing is an important transcriptional regulation mechanism through which we can control plant growth and development. For example, the colour of O. fragrans can be altered by artificially moving the start sites of certain genes (Chen, et al., 2020b; Zhang, et al., 2020; Song et al., 2021b). Proteomic analysis based on SWATH-MS can help better determine the mechanism of action of plants (Chen, et al., 2021a; Shen, et al., 2021), and we can apply these advanced technologies in future research on O. fragrans. Whole O. fragrans can be used as a medicine and is a traditional Chinese medicinal material that has been used for many years. Metabolomics, which does not require much effort, has been developed as a novel technique in recent years. However, we need to find a way to better combine metabolomics data with data on the pharmacological effects of O. fragrans. We hope that in the future, research will focus on the development of O. fragrans as a medicinal agent for treating different diseases.
Author Contributions
C-CF, M-XC, and F-YZ: conceptualization. C-CF, M-XC, and F-YZ: writing of the original draft. F-YX, Y-CQ, H-LK, Y-FD, G-MW, and T-PF: writing of the review and editing. F-YZ, and M-XC: funding. The version of the manuscript was approved by all authors.
Funding
This work was supported by the Natural Science Foundation of Jiangsu Province (SBK2020042924) and Jiangsu Agricultural Science and Technology Innovation Fund [CX(21)2023].
Conflict of Interest
Author KH-L was employed by RCI Research Institute Limited.
The remaining authors declare that the research was conducted in the absence of any commercial or financial relationships that could be construed as a potential conflict of interest.
Publisher’s Note
All claims expressed in this article are solely those of the authors and do not necessarily represent those of their affiliated organizations, or those of the publisher, the editors and the reviewers. Any product that may be evaluated in this article, or claim that may be made by its manufacturer, is not guaranteed or endorsed by the publisher.
Abbreviations
D-GalN, D-galactosamine; DHQ, dihydroquercetin; Dio, diosmetin; DN, diabetic nephropathy; O. fragrans, Osmanthus fragrans; PCA, protocatechualdehyde; RA, rosmarinic acid; ROS, reactive oxygen species; SA, salicylic acid; Ty, tyrosol.
References
Araújo, M., Prada, J., Mariz-Ponte, N., Santos, C., Pereira, J. A., Pinto, D. C. G. A., et al. (2021). Antioxidant Adjustments of Olive Trees (Olea Europaea) under Field Stress Conditions. Plants 10, 684. doi:10.3390/plants10040684
Bai, X. (2016). Study on the Chemical Constituents of Chinese Medicine Forsythia. Heilongjiang Med. 29, 601–602. doi:10.14035/j.cnki.hljyy.2016.04.002
Bao, Q., Dan, W., Tsing, L. K., Swift, S., Mingming, Z., and Yun, L. (2018). Antioxidant Activity of Osmanthus Flavonoids In Vitro and In Vivo. Food Res. Dev. 39, 14–20.
Camargo, L. R. P., de Carvalho, V. M., Díaz, I. E. C., Paciencia, M. L. B., Frana, S. A., Younes, R. N., et al. (2020). Susceptibility of Virulent and Resistant Escherichia coli Strains to Non-polar and Polar Compounds Identified in Microplumeria Anomala. Vet. World 13, 1376–1387. doi:10.14202/vetworld.2020.1376-1387
Castejón, M. L., Montoya, T., Alarcón-de-la-Lastra, C., and Sánchez-Hidalgo, M. (2020). Potential Protective Role Exerted by Secoiridoids from Olea europaea L. in Cancer, Cardiovascular, Neurodegenerative, Aging-Related, and Immunoinflammatory Diseases. Antioxidants 9, 149. doi:10.3390/antiox9020149
Cattaneo, L., Cicconi, R., Mignogna, G., Giorgi, A., Mattei, M., Graziani, G., et al. (2015). Anti-Proliferative Effect of Rosmarinus officinalis L. Extract on Human Melanoma A375 Cells. PloS one 10, e0132439. doi:10.1371/journal.pone.0132439
Chen, M. X., Zhang, K. L., Zhang, M., Das, D., Fang, Y. M., Dai, L., et al. (2020b). Alternative splicing and its regulatory role in woody plants. Tree Physiol. 40, 1475–1486. doi:10.1093/treephys/tpaa076
Chen, M. X., Zhang, Y., Fernie, A. R., Liu, Y. G., and Zhu, F. Y. (2021a). SWATH-MS-Based Proteomics: Strategies and Applications in Plants. Trends Biotechnol. 39, 433–437. doi:10.1016/j.tibtech.2020.09.002
Chen, M. X., Mei, L. C., Wang, F., Boyagane Dewayalage, I. K. W., Yang, J. F., Dai, L., et al. (2021b). PlantSPEAD: a web resource towards comparatively analysing stress‐responsive expression of splicing‐related proteins in plant. Plant Biotechnol. J. 19, 227–229. doi:10.1111/pbi.13486
Chen, M. X., Zhang, K. L., Gao, B., Yang, J. F., Tian, Y., Das, D., et al. (2020a). Phylogenetic comparison of 5′ splice site determination in central spliceosomal proteins of the U1‐70K gene family, in response to developmental cues and stress conditions. Plant J. 103, 357–378. doi:10.1111/tpj.14735
Chen, Y., Wang, Y., Liu, M., Zhou, B., and Yang, G. (2020c). Diosmetin exhibits anti-proliferative and anti-inflammatory effects on TNF-α-stimulated human rheumatoid arthritis fibroblast-like synoviocytes through regulating the Akt and NF-κB signaling pathways. Phytother. Res. 34, 1310–1319. doi:10.1002/ptr.6596
Cheng, Z. (2021). Research progress on extraction technology of osmanthus essential oil. Light Ind. Technol. 37, 16–17.
Cockayne, S., Hewitt, C., Hicks, K., Jayakody, S., Kang'ombe, A. R., Stamuli, E., et al. (2011). Cryotherapy versus salicylic acid for the treatment of plantar warts (verrucae): a randomised controlled trial. BMJ 342, d3271. doi:10.1136/bmj.d3271
Dan, H. (2014). Floral Aroma and Color of Main Osmanthus Cultivars in and Their Responses to Temperature Changes. China, Zhejiang: Zhejiang Agriculture and Forestry University.
Dayal, S., Kalra, K. D., and Sahu, P. (2020). Comparative study of efficacy and safety of 45% mandelic acid versus 30% salicylic acid peels in mild-to-moderate acne vulgaris. J. Cosmet. Dermatol 19, 393–399. doi:10.1111/jocd.13168
Dong, L., Hao, C., Xinyu, Z., and Haisheng, W. (2020). Research progress of dihydroquercetin. Adv. Biotechnol. 10, 226–233. doi:10.19586/j.2095-2341.2020.0008
Fei, J., Nan, Y., Shihui, Q., Peng, C., and Hui, H. (2010). Effects of 8 compounds in Ligustrum lucidum on glucose consumption in insulin-resistant HepG2 cells. Strait Pharm. 22, 164–167.
Fu, L., Shaokang, W., and Guiju, S. (2019). Research Progress on the Activity and Function of Luteolin, 434. doi:10.26914/c.cnkihy.2019.010680
García-Villalba, R., Tomás-Barberán, F., Fança-Berthon, P., Roller, M., Zafrilla, P., Issaly, N., et al. (2015). Targeted and Untargeted Metabolomics to Explore the Bioavailability of the Secoiridoids from a Seed/Fruit Extract (Fraxinus angustifolia Vahl) in Human Healthy Volunteers: A Preliminary Study. Molecules 20, 22202–22219. doi:10.3390/molecules201219845
Hao, Z., Qian, X., Luyun, Z., Xiaoyu, G., and Hui, Z. (2017). New progress in the study of pharmacological effects of tyrosol. J. Tonghua Normal Univ. 38, 48–53. doi:10.13877/j.cnki.cn22-1284.2017.12.013
He, Z., Baoli, X., Lan, Y., and Xiaobo, W. (2017). Star Point Design-Effect Surface Method to Optimize the Extraction Process of Soul Resurrection. Chin. J. Pharm. 33, 413–417.
Hu, K., Meiju, J., Dongdong, W., Yuxuan, X., Shuqi, J., and Jie, R. (2020). Synthesis of Antiparkinsonian Psychotic Drug Pimaserin. Synth. Chem. 28, 330–335. doi:10.15952/j.cnki.cjsc.1005-1511.18342
Hu, W., Xia, M., and Ronghua, G. (2021). In Vitro study of maslinic acid inhibiting the occurrence and development of cervical cancer HeLa cells by promoting mitochondrial apoptosis. China Maternal Child Health 36, 1881–1885. doi:10.19829/j.zgfybj.issn.1001-4411.2021.08.056
Jin, F., Yaling, X., Qingting, M., Hanwen, Y., and Fangyan, H. (2021). Protective effect of protocatechualdehyde on neurovascular unit homeostasis disruption after cerebral ischemia-reperfusion injury in rats. China Pharm. 32, 1811–1817.
Jing, T., Ruolan, T., Zhongjian, L., Bin, T., and Jianmin, G. (2019). Research progress on extraction of naringenin and its anticancer effect. Green Technol. 10, 240–244. doi:10.16663/j.cnki.lskj.2019.08.086
Ju, L., Yumei, L., Yani, G., Tongxia, Z., Jianlong, Z., and Xuemei, X. (2019). Research progress on metabolism and allelopathic effects of phenolic acids. Heilongjiang Agric. Sci. 42, 175–182.
Ke, L., and Min, H. C. (2019). Research progress on biological effects of rosmarinic acid. Int. J. Laboratory Med. 40, 1032–1036.
Le, D. D., Lee, Y. E., and Lee, M. (2022). Triterpenoids from the leaves of Osmanthus fragrans var. aurantiacus with their anti-melanogenesis and anti-tyrosinase activities. Nat. Prod. Res., 1–7. doi:10.1080/14786419.2022.2035384
Li, B., Qiaoyun, W., and Zhiwu, H. (2021a). Protective effect of oleanolic acid on oxidative damage of human umbilical vein endothelial cells. Chin. J. Clin. Pharmacol. 37, 1548–1550+1561. doi:10.13699/j.cnki.1001-6821.2021.12.019
Li, G., Kaifeng, Z., Shaogang, Q., Lei, C., Caixia, Y., and Yongqi, G. (2020a). Effects of maslinic acid on H_2O_2-induced oxidative damage and p38 and Nrf2/HO-1 pathways in BRL-3A cells. Chin. J. Cell. Biol. 42, 453–460.
Li, H., Yao, W., Liu, Q., Xu, J., Bao, B., Shan, M., et al. (2017). Application of UHPLC-ESI-Q-TOF-MS to Identify Multiple Constituents in Processed Products of the Herbal Medicine Ligustri Lucidi Fructus. Molecules 22, 689. doi:10.3390/molecules22050689
Li, M., Ting, L., Jianyun, Z., Junyan, Z., Likuan, L., and Jinping, M. (2020b). Research progress on determination methods and pharmacological effects of oleanolic acid and ursolic acid. Qinghai Grass Ind. 29, 58–63.
Li, R., Yujie, Z., Chaoying, L., and Yifeng, P. (2021b). Study on HPLC method for determination of naringenin in pine pollen. Shanghai Pharm. 42, 64–66+70.
Li, Y., and Huang, G. (2020). Research progress on chemical constituents and pharmacological activities of different parts of osmanthus fragrans fruit. Technol. Innovation Prod. 41, 67–69+73.
Liao, X., Hong, Y., and Chen, Z. (2021). Identification and quantification of the bioactive components in Osmanthus fragrans roots by HPLC-MS/MS. J. Pharm. Anal. 11, 299–307. doi:10.1016/j.jpha.2020.06.010
Lin, C., Dawei, C., and Jungui, D. (2022). Research progress in synthetic biology of flavonoids. J. Pharm. 57, 1322–1335. doi:10.16438/j.0513-4870.2022-0008
Lin, P., Xiaozhou, J., Yan, Q., and Shili, L. (2017). Research progress of phenolic compounds. Guangdong Chem. 44, 50–52.
LiY, ., Jianhong, Z., Dan, J., and Hongmei, L. (2020). Research progress of plant secondary metabolite transporter. Chin. Herb. Med. 51, 5065–5076.
Lv, C. (2021). Process Optimization and Biological Activity Study of Sweet-Scented Osmanthus Extract. Shanghai, China: Shanghai University of Applied Sciences.
Ma, N., Yajing, L., and Jiping, F. (2018). Research progress on pharmacological effects of geranialignin. J. Liaoning Univ. Traditional Chin. Med. 20, 214–217. doi:10.13194/j.issn.1673-842x.2018.09.062
Ning, N., Jianjun, H., Guangan, Y., and Jiansheng, Y. (2020). Research progress on extraction technology of total flavonoids from osmanthus fragrans. Shandong Chem. 49, 55+58. doi:10.19319/j.cnki.issn.1008-021x.2020.19.022
Omar, S. H. (2010). Cardioprotective and neuroprotective roles of oleuropein in olive. Saudi Pharm. J. 18, 111–121. doi:10.1016/j.jsps.2010.05.005
Park, E., Kim, J., Yeo, S., Kim, G., Ko, E. H., Lee, S. W., et al. (2018). Antiadipogenic Effects of Loganic Acid in 3T3-L1 Preadipocytes and Ovariectomized Mice. Molecules 23, 1663. doi:10.3390/molecules23071663
Park, E., Lee, C. G., Lim, E., Hwang, S., Yun, S. H., Kim, J., et al. (2020). Osteoprotective Effects of Loganic Acid on Osteoblastic and Osteoclastic Cells and Osteoporosis-Induced Mice. Int. J. Mol. Sci. 22, 233. doi:10.3390/ijms22010233
Peng, D., Juan, G., Xiumei, G., Jinlan, W., and Shujun, Z. (2014). Study on the chemical constituents of Mongolian dandelion root. Propr. Chin. Med. 36, 1462–1466.
Qi, Q., Zhong-hua, H., Hong-dan, X., Huai-yu, X., Hui-feng, S., Ning, Z., et al. (2020). Effects of naringenin on oxidative stress and Tau protein phosphorylation in Aβ_(25-35)-injured PC12 cells. Chin. J. Exp. Formulas 26, 92–99. doi:10.13422/j.cnki.syfjx.20202140
Qiao, Y., Wei, Z., Panyingying, , , Linxiong, D., Xiaxia, Z., and Fei, H. (2020). Renal protective effect of dihydroquercetin on diabetic nephropathy rats and the effect of mTORC2/Akt signaling pathway. Chin. J. Nephrol. Integr. Traditional Chin. West. Med. 21, 581–584+660.
Shen, C. C., Chen, M. X., Xiao, T., Zhang, C., Shang, J., Zhang, K. L., et al. (2021). Global proteome response to Pb(II) toxicity in poplar using SWATH-MS-based quantitative proteomics investigation. Ecotoxicol. Environ. Saf. 220, 112410. doi:10.1016/j.ecoenv.2021.112410
Shen, X., Hongmei, Z., Lei, Z., and Weiwei, J. (2019). Research progress of oleanolic acid. Guangzhou Chem. 47, 16–19.
Shi, X., Weiwei, C., Bao, H., Zhongjie, L., and Liying, Y. (2020). Extraction and separation of effective substances from radish and its effect on cell proliferation activity. Strait Pharm. 32, 27–29.
Si, Q., Shi, Y., Huang, D., and Zhang, N. (2020). Diosmetin alleviates hypoxia-induced myocardial apoptosis by inducing autophagy through AMPK activation. Mol. Med. Rep. 22, 1335–1341. doi:10.3892/mmr.2020.11241
Song, H.-Y., Jeong, D.-E., and Lee, M. (2021a). Bioactivity-Guided Extract Optimization of Osmanthus fragrans var. aurantiacus Leaves and Anti-Inflammatory Activities of Phillyrin. Plants 10, 1545. doi:10.3390/plants10081545
Song, T., Das, D., Ye, N. H., Wang, G. Q., Zhu, F. Y., Chen, M. X., et al. (2021b). Comparative transcriptome analysis of coleorhiza development in japonica and Indica rice. BMC Plant Biol. 21, 514. doi:10.1186/s12870-021-03276-z
Song, X., Xiaolin, L., Anyon, K., Caixia, Y., Grand, , , Dexian, Z., et al. (2015). Research progress on antibacterial and anti-resistance effects of luteolin. Chin. Poult. 37, 44–46. doi:10.16372/j.issn.1004-6364.2015.17.010
Tang, W., and Zhao, Y. (2014). Research progress on chemical constituents and pharmacological effects of Oleracea. Chin. Herb. Med. 45, 590–602.
Ting, J. T., Jianfang, L., and Xiaodong, L. (2021). Research progress on extraction method and application of salicylic acid. Tianjin Chem. 35, 7–9.
Wang, B., Luan, F., Bao, Y., Peng, X., Rao, Z., Tang, Q., et al. (2022). Traditional uses, phytochemical constituents and pharmacological properties of Osmanthus fragrans: A review. J. Ethnopharmacol. 293, 115273. doi:10.1016/j.jep.2022.115273
Wang, F., Li, C.-y., Zheng, Y.-f., Li, H.-y., Xiao, W., and Peng, G.-p. (20162016). Identification of the Allergenic Ingredients in Reduning Injection by Ultrafiltration and High-Performance Liquid Chromatography. J. Immunol. Res. 2016, 1–7. doi:10.1155/2016/4895672
Wang, L. (2009). Synthesis and Transformation Law and Pharmaceutical Research of the Active Ingredients of Osmanthus Fragrans. China, Hubei: Huazhong University of Science and Technology.
Wang, N., Sihui, Shitao, Y., Wei, Z., and Guoxi, X. (2011a). Component analysis of sweet-scented osmanthus extract membrane separation products and its application in cigarettes. Fragr. Cosmet. 39, 22–26.
Wang, Q., Haixue, K., Lun, W., Yang, L., and Zhibin, W. (2011b). Protective effect of dihydroquercetin on isoproterenol-induced myocardial ischemia in rats. Chin. J. Exp. Formulas 17, 177–180. doi:10.13422/j.cnki.syfjx.2011.17.062
Wang, Y., LipingKaijing, G., Liyu, C., and Qing, T. (2014). Study on the chemical constituents of red-backed osmanthus. Nat. Prod. Res. Dev. 26, 47–49. doi:10.16333/j.1001-6880.2014.01.015
Wang, Z., and Wang, J. (2021). Mechanism prediction and pharmacological research progress of naringenin in the treatment of coronary heart disease. Tradit. Chin. Med. Inf. 38, 77–81. doi:10.19656/j.cnki.1002-2406.210416
Wei, S., Yumin, Z., Tao, M., and Jun, W. (2021). Based on the network pharmacology method to analyze the mechanism and experimental verification of astilbin in the intervention of osteoarthritis. Drug Eval. Stud. 44, 728–735.
Wei, Y., Zurong, S., Jinqi, L., and Guosheng, Z. (2015). Study on the Chemical Composition of Osmanthus fragrans. Chin. J. Traditional Chin. Med. 40, 679–685.
Wu, C., Xianying, F., and Wei, X. (2017). Research progress on non-volatile components and pharmacological activities of Osmanthus fragrans. Nat. Prod. Res. Dev. 29, 1439–1448. doi:10.16333/j.1001-6880.2017.8.028
Xin, X., Shifeng, C., and Naihong, C. (2018). Research progress on medicinal value of honey. J. Neuropharmacol. 8, 52.
Xu, Y. (2020). The Role and Mechanism of Astilbin Up-Regulating Breg Cells in Inflammatory Bowel Disease. Jiangsu, China: Yangzhou University.
Xuan, L., Yingchun, H., and Fangliang, Z. (2021). Research progress on pharmacological effects and mechanisms of maslinic acid. Chin. J. Mod. Med. 31, 49–53.
Xue, A., Meng, C., Huajie, Z., and Luo, L. (2018). Analysis of Osmanthus fragrans polyphenols by electrospray extraction and ionization mass spectrometry. Food Sci. 39, 221–226.
Xue, C., Hongxin, F., Zhongguang, Z., and Shuming, H. (2017). Research progress on pharmacological effects of dihydroquercetin. Chin. Med. J. 45, 90–92. doi:10.19664/j.cnki.1002-2392.2017.01.026
Yang, D., Guang, X., Yan, Z., Wanchao, Z., Luochuan , , and Caifu, D. (2021). Research progress on main chemical constituents and biological activities of Osmanthus fragrans. China's wild plant Resour. 40, 47–52.
Yang, D. (2018). Interventional Effect of Luteolin on Inorganic Mercury-Induced Acute Liver Injury in Mice. China, Heilongjiang: Northeast Agricultural University.
Yang, X., Yue, Y., Li, H., Ding, W., Chen, G., Shi, T., et al. (2018). The chromosome-level quality genome provides insights into the evolution of the biosynthesis genes for aroma compounds of Osmanthus fragrans. Hortic. Res. 5, 72. doi:10.1038/s41438-018-0108-0
Yang, X.-S., and Yang, Z. (2021). Analysis of Algorithms. Shandong Med. 61, 39–61. doi:10.1016/b978-0-12-821986-7.00010-x
Yu, L. (2020). The Protective Effect of Astilbin on Cerebral Ischemia-Reperfusion Injury and its Mechanism. Jiangsu, China: Soochow University.
Yue, H. (2019). Screening of Anti-gout Flavonoids and Study on Intestinal Absorption of Luteolin. Jilin, China: Jilin Agricultural University.
Yue, S., Jinmei, W., and Wenyi, K. (2013). Study on triterpenoids and hypolipidemic effect of Osmanthus fragrans. Chin. J. Exp. Formulas 19, 126–128.
Zeng, M., Yan, W., Wolf, L., peaked, h., and Chaoke, T. (2016). Research progress of naringenin and metabolic syndrome. Chin. J. Arteriosclerosis 24, 1055–1058.
Zeng, W., Fayun, Z., Gangjun, D., Lingtao, J., Lei, Q., Wenjuan, D., et al. (2018). Naringenin: A New Generation of Immunomodulators. Adv. Biochem. Biophysics 45, 915–925. doi:10.16476/j.pibb.2018.0189
Zhang, C., Lili, G., and Rank, K. (2013). Pharmacological research progress of protocatechualdehyde. Chin. J. Exp. Formulas 19, 338–342.
Zhang, D., Chen, M.-X., Zhu, F.-Y., Zhang, J., and Liu, Y.-G. (2020). Emerging Functions of Plant Serine/Arginine-Rich (SR) Proteins: Lessons from Animals. Crit. Rev. Plant Sci. 39, 173–194. doi:10.1080/07352689.2020.1770942
Zhang, X. (2008). Preliminary Study on the Antioxidant Activity of Polygonum Cuspidatum Rhizome and Osmanthus Fragrans Pulp. Guangxi Normal University.
Zhang, Y., Yanna, S., Ge, W., Huafang, W., and Ying, Y. (2021). To investigate the effect of oleanolic acid on apoptosis of esophageal cancer cells based on the regulation of JNK signaling pathway. Adv. Mod. Biomed. 21, 2226–2229. doi:10.13241/j.cnki.pmb.2021.12.006
Keywords: Osmanthus fragrans, secondary metabolites, medicinal compound, terpenoids, flavonoids, phenolic acids
Citation: Fu C-C, Xu F-Y, Qian Y-C, Koo H-L, Duan Y-F, Weng G-M, Fan T-P, Chen M-X and Zhu F-Y (2022) Secondary Metabolites of Osmanthus fragrans: Metabolism and Medicinal Value. Front. Pharmacol. 13:922204. doi: 10.3389/fphar.2022.922204
Received: 17 April 2022; Accepted: 20 June 2022;
Published: 18 July 2022.
Edited by:
Shahid Ul Islam, University of California, Davis, United StatesReviewed by:
Luqman Jameel Rather, Southwest University, ChinaManzoor A. Mir, University of Kashmir, India
Copyright © 2022 Fu, Xu, Qian, Koo, Duan, Weng, Fan, Chen and Zhu. This is an open-access article distributed under the terms of the Creative Commons Attribution License (CC BY). The use, distribution or reproduction in other forums is permitted, provided the original author(s) and the copyright owner(s) are credited and that the original publication in this journal is cited, in accordance with accepted academic practice. No use, distribution or reproduction is permitted which does not comply with these terms.
*Correspondence: Mo-Xian Chen, Y214MjAwOTkyMDczNEBnbWFpbC5jb20=; Fu-Yuan Zhu, Znl6aHVAbmpmdS5lZHUuY24=
†These authors have contributed equally to this work