- 1CAS Key Laboratory of Tropical Marine Bio-resources and Ecology/Guangdong Key Laboratory of Marine Materia Medica, South China Sea Institute of Oceanology, Chinese Academy of Sciences, Guangzhou, China
- 2Institute of Marine Drugs, Guangxi University of Chinese Medicine, Nanning, China
- 3Guangxi Zhuang Yao Medicine Center of Engineering and Technology, Guangxi University of Chinese Medicine, Nanning, China
- 4Guangxi Key Laboratory of Chinese Medicine Foundation Research, Nanning, China
Advanced prostate cancer has a poor prognosis, and it is urgent to develop new effective drugs. 5′-Epiequisetin is a tetramic acid derivative which was isolated from a marine sponge-derived fungus Fusarium equiseti in our previous study. In this study, 5′-epiequisetin showed cytotoxicity against four prostate cancer cell lines, namely, LNCaP, 22Rv1, DU145, and PC-3 cells, with the lowest IC50 value of 4.43 ± 0.24 μM in PC-3 cells. Further studies showed that it could dramatically regulate the clonal colony formation, apoptosis, and migration of PC-3 cells. In addition, flow cytometry data showed that 5′-epiequisetin could block the cell cycle at the G1 phase. Proteome profiler array and Western blot revealed that 5′-epiequisetin could regulate the expression of proteins responsible for cell proliferation, apoptosis, and migration. 5′-Epiequisetin regulated the expression of PI3K, Akt, phosphorylated Akt, and proteins which control the cell cycle. Meanwhile, 5′-epiequisetin upregulated expression of DR5 and cleave-caspase 3, which play important roles in the process of apoptosis. Moreover, when DR5 was silenced by small interfering RNA, the proportion of apoptotic cells induced by 5′-epiequisetin remarkably declined. In addition, 5′-epiequisetin downregulated the expression of survivin which plays a key role in the process of survival and apoptosis. 5′-Epiequisetin also impacted beta-catenin and cadherins, which were associated with cell migration. In addition, 5′-Epiequisetin significantly inhibited the progression of prostate cancer in mice, accompanied by regulating the protein expression of DR5, caspase 8, survivin, and cadherins in vivo. Taken together, these findings indicated that 5′-epiequisetin showed an anti–prostate cancer effect by inducing apoptosis and inhibiting cell proliferation and migration both in vitro and in vivo, suggesting a promising lead compound for the pharmacotherapy of prostate cancer.
Introduction
Global cancer statistics 2020 showed that prostate cancer (PCa) ranked the second highest incidence rate in men, and the American cancer society predicted that PCa would be the most common cancer among men in the United States in 2021 (Siegel et al., 2021; Sung et al., 2021). In China, PCa is the sixth most common cancer in males (Zhang S. et al., 2021). Patients with localized prostate cancer could get an optimistic prognosis after taking a standard therapeutic regimen, while patients with locally advanced or extensive metastasis will eventually develop castration-resistant prostate cancer (CRPC) (Ge et al., 2020). In the past decades, remarkable progress has been made in the treatment of CRPC, and the 5-years survival rate of patients with CRPC has been improved by therapies such as immunotherapy, hormonal therapy, cytotoxic therapy, targeted therapy, and bone-directed therapy (Galsky et al., 2012). However, there is still an urgent need to find more advanced therapies to overcome insurmountable problems, such as drug resistance and adverse reactions.
Marine natural products (MNP) have been evidenced as an important source of lead compounds with novel chemical structures (Newman and Cragg, 2020; Carroll et al., 2021). An increasing number of marine-derived anti-tumor drugs have been introduced into the market; meanwhile, there are also many marine-derived agents in various stages of clinical trials (Mayer, 2020). It is worth noting that dozens of MNPs have been found with an anti-PCa effect based on unique mechanisms of action (Fan et al., 2018; Dyshlovoy and Honecker, 2020). For instance, elaiophylin and Ilicicolin A are two MNPs with anti-prostate cancer we reported in our previous studies. Elaiophylin showed potent antitumor activity against CRPC in vitro and in vivo by acting as a novel RORγ antagonist (Zheng et al., 2020). Ilicicolin A exerted antiproliferative activity in human PCa cells via inhibiting the EZH2 pathway; moreover, it could enhance the anticancer activity of enzalutamide in CRPC cancer models (Guo et al., 2021).
5′-Epiequisetin (Eeq) (Figure 1A) was identified as a tetramic acid antibiotic from a marine sponge-derived fungus, Fusarium equiseti SCSIO 41019, in our previous study (Chen et al., 2019). Relatively few studies have been reported on Eeq; however, more studies have been reported on its isomer equisetin, and it has been much studied for its antibacterial activity (Zhang et al., 2018; Chen et al., 2021; Zhang Q. et al., 2021). A recent study found that equisetin could produce anti-obesity effects by targeting 11 β-HSD1 (Xu et al., 2022). These studies suggest that this chemical structure has the potential for multiple biological activities. In screening experiments for anti-prostate cancer candidate compounds, we found that Eeq could inhibit the cell viability of four PCa cell lines (LNCaP, 22Rv1, DU145, and PC-3) to varying degrees, with the most dramatic effect on PC-3 cells. This finding inspired us to initiate a series of more in-depth studies on this compound in the field of anti-prostate cancer.
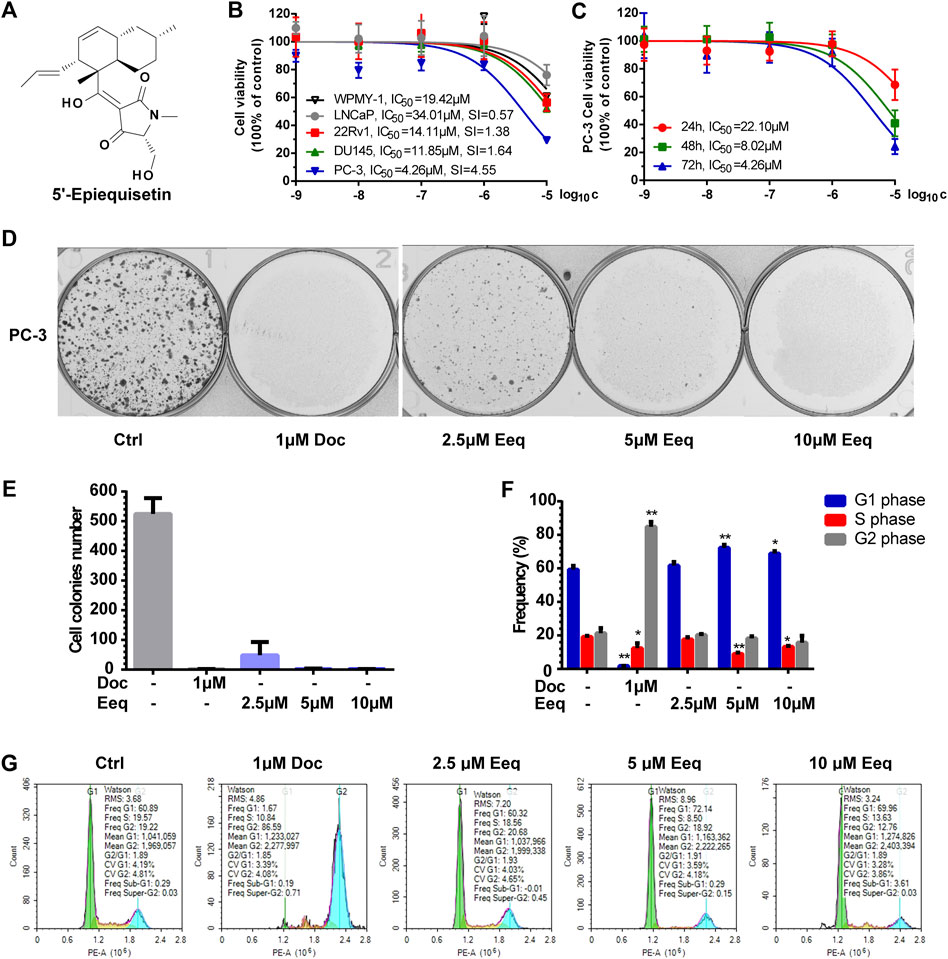
FIGURE 1. 5′-Epiequisetin (Eeq) inhibited the proliferation of prostate cancer cells. (A) Chemical structure of Eeq. (B) Eeq inhibited prostate cancer cell viability to varying degrees. (C) Eeq inhibited PC-3 cells in a time-dependent manner. (D,E) Eeq reduced PC-3 cell colony formation. (F,G) Eeq altered the distribution of PC-3 cell cycle and arrested the cell cycle in the G1 phase. *p < 0.5, **p < 0.01 vs. control.
We first assessed its effects on PC-3 cell proliferation, apoptosis, and migration in vitro. Second, we sought to elucidate how it acts. Finally, we examined its anti-prostate cancer effects in vivo by establishing a xenograft tumor mouse model. Our work provided evidence for an MNP possessing an anti-PCa effect both in vitro and in vivo, suggesting a potential anti-PCa agent. More immediately, our results should aid researchers interested in the pursuit of compounds with similar structures for the development of anti-PCa drugs, identifying candidate molecules that have potential for further development. In this way, we hope this work serves in part as a catalyst to move towards the production of more robust anti-PCa molecules.
Materials and methods
Reagents and antibodies
Docetaxel was purchased from Selleck Chemicals (Cat# RP56976). RPMI 1640 medium was purchased from Gibco (Cat# 8120018). Fetal bovine serum was purchased from Gemini (Cat# 900-108). Charcoal stripped FBS was purchased from Biological Industries (Cat# 04-201-1A). Thiazolyl blue tetrazolium bromide (Cat# M8180) and crystal violet stain solution (Cat# G1063) were purchased from Solarbio life sciences. Human apoptosis array kit was from R&D Systems (Cat# ARY009). NuPAGETM 10% Bis-Tris Gel (Cat# NP0302BOX), eBioscience™ Annexin V-FITC apoptosis detection kit (Cat# BMS500FI-300), FxCycle™ PI/RNase staining solution (Cat# F10797) and Vybrant cell-labeling solutions (V22885) were purchased from Invitrogen. All antibodies used in this study were purchased from Proteintech Group (CDK4, Cat# 66950-1-lg; CDK6, Cat# 66278-1-lg; Cyclin D1, Cat# 26939-1-AP; RB1, Cat# 10048-1-lg; E2F1, Cat# 66515-1-lg; Survivin, Cat# 66495-1-lg; p21, Cat# 10355-1-AP). Eeq was isolated and identified from the marine sponge-derived fungus Fusarium equiseti SCSIO 41019 by various chromatographic and comprehensive spectroscopic methods in our previous study (Chen et al., 2019), respectively. It was determined to have ≥95% purity by analytical HPLC.
Cell culture
LNCaP, 22Rv1, DU145, PC-3, and WPMY-1 cells were obtained from the National Collection of Authenticated Cell Cultures (Shanghai, China). All cell lines were identified by STR, and the cells used in the experiment were within 15 passages. LNCaP and 22Rv1 cells were maintained in RPMI 1640 supplemented with 10% (v/v) fetal bovine serum (FBS), 100 units/mL penicillin, and 100 μg/μL streptomycin; DU145 cells were cultured in MEM plus with the same additives; PC-3 cells were cultured in DMEM F12 plus with the same additives; WPMY-1 cell line was maintained in DMEM (Gibco, China) supplemented with 5% (v/v) fetal bovine serum (FBS) (Biological Industries, Israel), 100 units/mL penicillin, and 100 μg/μL streptomycin.
MTT assay
Cell viability was analyzed using MTT assay as previously described (Wang et al., 2021). In brief, cells were seeded overnight in a 96-well plate at a density of 5*103 per well and then treated with a range of concentrations of Eeq for the intended time. After the cells were exposed to Eeq for a certain time, the MTT solution was added to the cell culture wells for 4 h. Then, OD570 values were detected by using a Hybrid Multi-Mode Reader (Synergy H1, BioTek). The experiment was repeated three times independently. IC50 was calculated by using GraphPad 9.0 software. The degree of selective cytotoxicity index was expressed as SI = IC50 in normal cells/IC50 in tumor cells.
Plate clone formation assay
PC-3 cells were seeded in a 6-well plate at a density of 1000 per well overnight, and then they were treated with DMSO (0.1%, v/v) and docetaxel (1 μM), Eeq (2.5, 5, 10 μM) for demanded time, respectively. The culture medium was changed every 72 h until the obvious cell cloning colonies were formed about 15 days later, and then the cells were fixed with 4% formaldehyde for 30 min, discarded 4% formaldehyde and washed cells with PBS buffer, and stained the colonies with crystal violet stain solution. After the cells had been stained for 30 min, we discarded the staining solution, washed the cells with PBS buffer, and then took colonies’ photos with a colony counter (GelCount, Oxford Optronix). The experiment was repeated three times independently.
Apoptosis and cell cycle assay
PC-3 cells were seeded in a 6-well plate at a density of 2.0*105 per well overnight and treated with DMSO (0.1%, v/v), docetaxel (1 μM), Eeq (2.5, 5, and 10 μM), respectively, for 48 h. The cells were collected and stained according to the instructions of eBioscience™ Annexin V-FITC apoptosis detection kit and FxCycle™ PI/RNase staining solution, respectively. Then, cell apoptosis and cell cycle distribution were detected using flow cytometry (NovoCyte, Agilent). This experiment was repeated three times independently.
Cell migration assay
A gradient concentration of Eeq (1.25, 2.5, 5, 10, 20, and 40 μM) solution was prepared with 10% FBS DMEM F12 medium in a 96 well U-shaped plate, and then these solutions were transferred to the lower chamber in CIM-plate 16 (5665817001, Agilent) with 2 wells for each concentration. Next, the upper chamber of the CIM-plate 16 was mounted directly above the lower chamber to form a complete plate. PC-3 cells were seeded in the upper chamber of the CIM-plate 16 at a density of 2.5*105. Eeq was then added to the upper chamber so that the concentration of Eeq matched that of the lower chamber. After that, the CIM-plate 16 was installed on a real-time cell analyzer (xCELLigence RTCA DP Instrument, Agilent) to detect the effect of Eeq on the migration of PC-3 cells.
Human apoptosis array
PC-3 cells were seeded in T75 Flask at a density of 5.0*106 for 48 h and treated with DMSO (0.1%, v/v) and Eeq (10 μM) for 48 h, respectively. Then, cells were collected, and proteins were extracted and analyzed according to the instructions of the human apoptosis array kit.
qPCR detection of endogenous gene expression
qPCR was performed as described previously (Wang et al., 2021). In brief, PC3 cells were seeded into 6-well plates and grown in DMEM F12 medium containing 10% FBS. After attachment, cells were treated with Eeq for 24 h. Then RNA was extracted and purified using the TRIzol reagent (15596018, Life technologies). cDNA was prepared from 1 μg of RNA with RevertAid Master Mix (M1632, Thermo Fisher Scientific). Diluted cDNA was used to perform quantitative RT-PCR (LightCycler96, Roche) using PowerUp SYBR Green master mix (A25742, applied biosystems) with GAPDH as the internal standard. Primers for quantitative RT-PCR were listed in Table 1. Experiments were performed in triplicate.
Western blot
PC-3 cells were seeded in 60 mm dishes at a density of 1.0*106 for 48 h. Then, cells received a fresh medium containing the indicated compounds, docetaxel (1 μM), and the indicated concentrations of Eeq (2.5, 5, and 10 μM). The whole cell extracts were prepared after 48 h by using the mixture of RIPA (R0020, Solarbio life science) and PMSF (P0100, Solarbio life science). Proteins were analyzed on 10% SDS-PAGE gel sand transferred to nitrocellulose membranes. In this study, 30 μg of total protein was used to detect all proteins except DR5, which was measured by using 60–80 μg of total protein. All information of antibodies is listed in the section on reagents and antibodies. Images were captured using the Chemidoc CD Touch (Bio-Rad, United States), and images were analyzed and processed using the Image Lab 6.0 (Bio-Rad, Chinese edition).
Small interfering RNA silence DR5 expression
PC-3 cells were seeded in a 6-well plate at a density of 2.0*105 for 48 h, and then cells were transfected with si-RNA (small interfering RNA sequences are shown in Table 2) by using Lipofectamine 2000 (11668019, Thermo Fisher Scientific) according to the manufacturer’s manual. After 48 h of transfection, Eeq was added to the 6-well plate, and the cells were exposed to the compound for another 48 h. Then, apoptosis was detected using flow cytometry.
Xenograft tumor model
Animal experiments were performed according to procedures approved by the Guangxi University of Chinese Medicine Institutional Animal Ethical and Welfare Committee (Approval code: 20210603-071). Male BALB/c nude mice (Hunan SJA laboratory animal Co., Ltd., China) of 5–6 weeks old were acclimated to a sterilized normal diet and water for seven days. Mice were inoculated subcutaneously with 5*105 androgen-independent PC-3 human prostate cancer cells. The PC-3 cell line was purchased from Cell Bank/Stem Cell Bank, The Committee of Type Culture Collection of the Chinese Academy of Sciences (Shanghai, China), where the cells were authenticated by STR DNA profiling and tested as mycoplasma free. The intervention started when tumors reached a volume of 50–100 mm3 about one week after inoculation. Mice were randomly assigned into three groups (n = 6-7): vehicle, 10 mg/kg docetaxel, 20 mg/kg, and 40 mg/kg Eeq. Docetaxel and Eeq were dissolved in a solvent containing 5%DMSO, 30% PEG300, 5% Tween 80 and dd H2O. Docetaxel was intraperitoneally administered twice a week for four weeks, and Eeq was administered once a day throughout the study. Tumor size was measured twice a week with calipers. Tumor volume was calculated by using the formula: π/6*length*width*width (Wang et al., 2016). Mouse body weight was measured twice a week. Mice were sacrificed when they had received 4-week intervention treatment in observance of the institutional guideline on tumor size. Xenograft tumors were harvested, weighed, and fixed with 4% paraformaldehyde and/or snap frozen and stored in liquid nitrogen.
Statistical analysis
All analyses were conducted using GraphPad Prism 9 (GraphPad Software, United States). All results were presented as mean ± standard deviation (SD) and analyzed using one-way ANOVA. Comparisons between the groups were conducted using Dunnett’s t-test. Level for statistical significance was set at *p < 0.05, **p < 0.01.
Results
5′-epiequisetin suppressed prostate cancer cells proliferation via inhibiting the PI3K/Akt signaling pathway
Eeq inhibited the cell viability of prostate cancer cells (LNCaP, 22Rv1, DU145, and PC-3) and normal prostatic cells (WPMY-1) in varying degrees in a dose-dependent manner by MTT assay, among which, it showed the most significant effect on PC-3 cells (IC50 = 4.43 ± 0.24 μM). The selective cytotoxicity index (SI) of Eeq for PC-3, DU145, 22Rv1 and LNCaP cells were 4.55, 1.64, 1.38, and 0.57, respectively, suggesting that Eeq has the best selectivity for PC-3 cells (Figure 1B). Then, we used the MTT assay to further investigate the effect of Eeq on the viability of PC-3 cells. We found that the cytotoxicity of Eeq on PC-3 cells was time-dependent (Figure 1C). Next, we performed a plate clone formation assay to investigate the effect of Eeq on the proliferation of PC-3 cells. The results showed that it could significantly inhibit the clone formation of PC-3 cells in a dose-dependent manner (Figures 1D and E). Notably, the PC-3 cell line is a prostate cancer cell that does not express the androgen receptor, and these findings suggest that Eeq does not exert its anti-prostate cancer effects through modulation of the androgen receptor signaling pathway. It must then exert its effects through other pathways.
In order to reveal how it inhibited the proliferation of prostate cancer cells, we detected the cell cycle distribution of PC-3 cells. As shown in Figures 1F and G, when being treated with docetaxel, which is an M-phase blocker, a large number of PC-3 cells were arrested at the M phase, and the proportion of G2/M phase cells increased sharply. However, unlike in the case of docetaxel, the percentage of the G1 phase increased significantly in the PC-3 cells which were treated with Eeq. These findings point to the fact that Eeq blocks the cell cycle at the G1 phase, resulting in the inability of cells to proliferate.
Cyclin D, CDK4, and CDK6 are fundamental drivers of the cell cycle in driving G1 to S phase transition (Goel et al., 2017). In order to clarify the mechanism that Eeq blocked the cell cycle in the G1 phase, we performed Western blot to detect the expression of the proteins above. As shown in Figure 2, Eeq did reduce the expression of Cyclin D, CDK4, and CDK6 proteins while upregulating the expression of p21 and p27 proteins (Figures 2D–H), which are also key roles in regulating the cell cycle from G1 phase to S phase (Coqueret, 2003). Furthermore, PI3K/Akt/p21 signaling axis is a key pathway to regulate the cell cycle (Chang et al., 2003). In the present study, Eeq inhibited PI3K and Akt protein expression by reducing Akt phosphorylation at Ser473 (Figures 2A–C). Finally, it declined the expression of survivin protein, leading to cell death (Figure 2I). Based on the findings above, it can be concluded that Eeq inhibited the function of the PI3K/Akt/p21 signaling pathway, which, in turn, blocked the G1 phase of the cell cycle, resulting in the inability of cells to divide and the eventual loss of proliferative capacity.
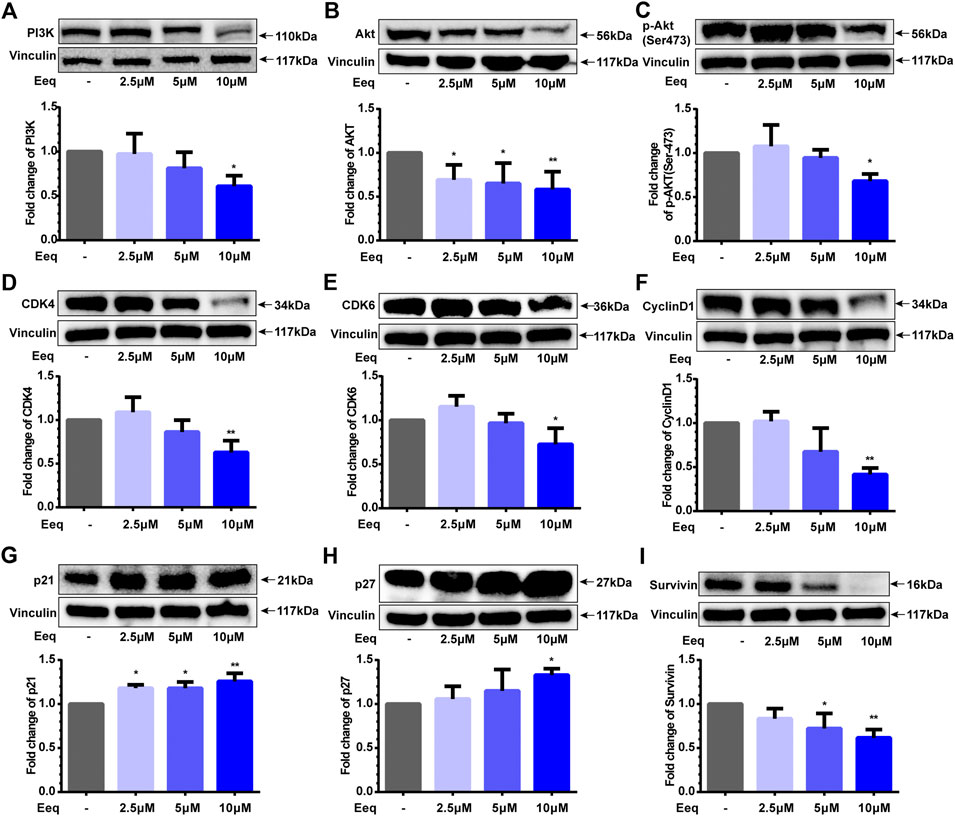
FIGURE 2. 5′-Epiequisetin (Eeq) regulated the expression of proliferation-related proteins. (A) PI3K, (B) Akt, (C) phosphorylated Akt, (D) CDK4, (E) CDK6, (F) cyclin D1, (G) p21, (H) p27, and (I) survivin. *p < 0.5, **p < 0.01 vs. control.
5′-epiequisetin restrained prostate cancer cells migration via suppressing the beta-catenin/cadherin signaling pathway
On the other hand, we found that Eeq inhibited PC-3 cell migration with an IC50 value of 6.80 ± 0.31 μM by real-time cell analysis (Figure 3A). In addition to directly corresponding to the cell cycle, the PI3K/Akt signaling pathway also plays a key role in cell invasion and migration (Hamidi et al., 2017). It is well known that beta-catenin is involved in WNT and/or PI3K/Akt signaling pathways which regulate cancer cell migration, thereby it is considered a target for the treatment of cancer (Tang et al., 2019; Zhang et al., 2020). We found that Eeq decreased the protein expression of β-catenin and N-cadherin and increased the expression of E-cadherin (Figures 3B–D), while it showed no impact on WNT5A/5B (Supplementary Figure S2F). These findings may provide evidence that Eeq attenuated epithelial–mesenchymal transitions through PI3K/Akt/beta-catenin/cadherin in prostate cancer cells, thereby inhibiting the migration of PC-3 cells.
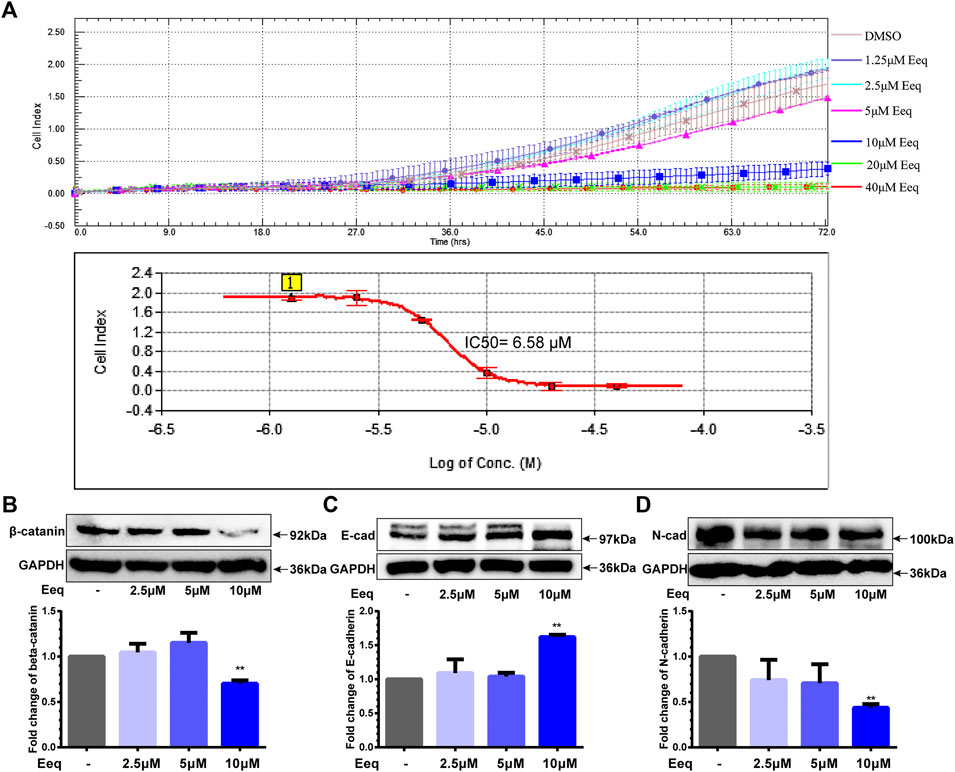
FIGURE 3. 5′-Epiequisetin (Eeq) inhibited the migration of PC-3 cells. (A) IC50 of Eeq inhibited PC-3 cell migration. (B) Impact of Eeq on protein expression of beta-catenin. (C) Impact of Eeq on protein expression of E-cadherin. (D) Impact of Eeq on protein expression of N-Cadherin. *p < 0.5, **p < 0.01 vs. control.
5′-epiequisetin induced PC-3 cells apoptosis through the DR5 signaling pathway
To further identify how Eeq produced cytotoxicity, we used flow cytometry to detect the apoptosis of PC-3 cells. As shown in Figures 4A and B, Eeq induced cell apoptosis in a dose-dependent manner with docetaxel acting as a positive control. In order to clarify how Eeq induced apoptosis, we performed a human apoptosis array. As shown in Figures 4C and D, Bcl-x, cleaved caspase-3, DR5, p21, and p27, were upregulated after Eeq had been treated for 48 h. However, the survivin protein that promotes cell survival was downregulated. Among the apoptosis proteins encapsulated in the human apoptosis array, DR4, DR5, TNFRSF6, and TNFRSF1A are proteins involved in the TNF signal transduction pathway, which is an important pathway that mediates apoptosis. DR5 was upregulated after Eeq had been treated for 48 h, while DR4, TNFRSF6, and TNFRSF1A were not upregulated by Eeq (Figure 4C). Therefore, we studied DR5 in the follow-up work. We noticed that DR5 was a cell membrane receptor that mediated cell apoptosis. These findings inspired us to speculate that Eeq mediated apoptosis by driving the DR5 signaling pathway. Then, in order to confirm this hypothesis, we conducted a series of experiments.
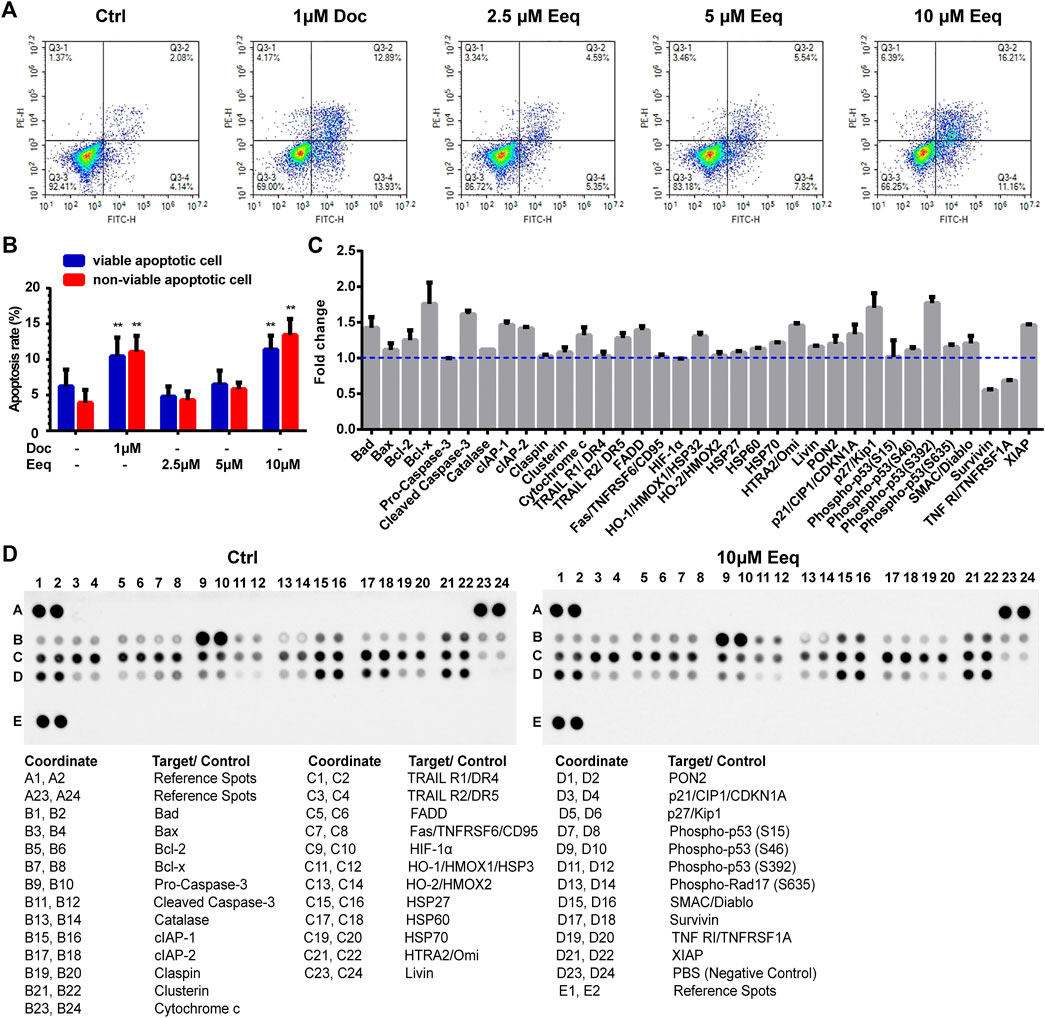
FIGURE 4. 5′-Epiequisetin (Eeq) induced PC-3 cell apoptosis. (A,B) Eeq induced PC-3 cell apoptosis in a dose-dependent manner. (C,D) Eeq regulated the expression of apoptosis-regulated proteins. *p < 0.5, **p < 0.01 vs. control.
Activation of caspase-8 and caspase-3 is the key link in the induction of apoptosis by DR5 (Muñoz-Pinedo and López-Rivas, 2018). In this study, caspase-8 and caspase-3 were activated, as evidenced by a decrease in the full length of caspase-8/3 and an increase in cleaved caspase-8 (Figures 5A and B). Furthermore, we found Eeq increased expression of DR5 on both mRNA and protein levels (Figures 5C and D). Most importantly, the apoptotic cells induced by Eeq were significantly reduced (Figure 5H) when DR5 expression was silenced by small interfering RNA (Figures 5E–G). Taken together, Eeq induced PC-3 cell apoptosis through the DR5 signaling pathway.
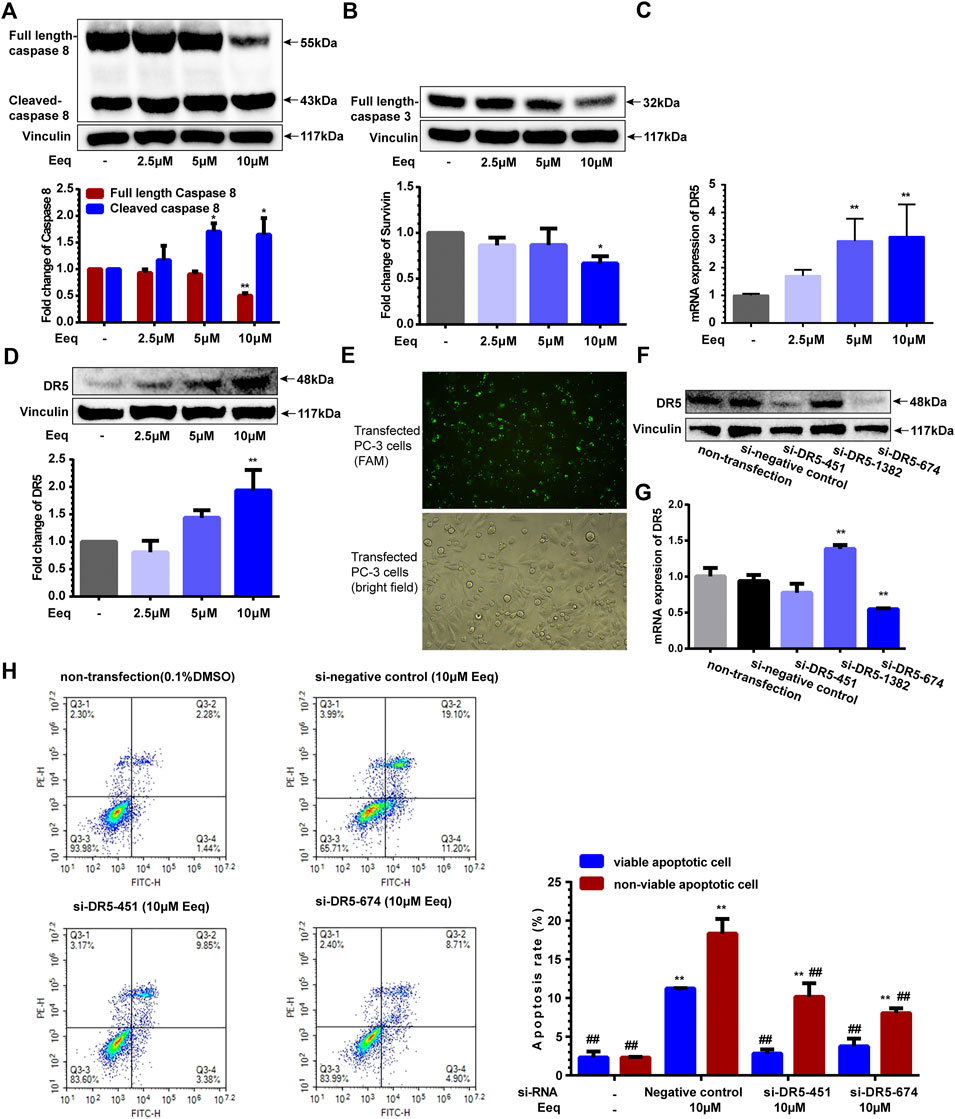
FIGURE 5. 5′-Epiequisetin (Eeq) induced PC-3 cell apoptosis by activating the DR5 signaling pathway. (A) Eeq regulated caspase 8 protein expression. (B) Eeq reduced caspase 3 protein expression. (C) Eeq increased mRNA expression of DR5. (D) Eeq increased protein expression of DR5. (E) PC-3 cells were transfected with small interfering RNA. (F) Small interfering RNA knocked down DR5 protein expression. (G) Small interfering RNA knocked down DR5 mRNA expression. (H) Eeq induced a decrease in apoptosis of PC-3 cells after DR5 was knocked down by small interfering RNA. *p < 0.5, **p < 0.01 vs. control; #p < 0.5, ##p < 0.01 vs. negative control (si-RNA).
Tables 5′-Epiequisetin suppressed the growth of prostate cancer in vivo
In order to further evaluate the anti-PCa effect of Eeq in vivo, we constructed a PCa xenograft model by subcutaneously injecting PC-3 cells into the right flank of the mice. In the first experiment, we divided the model mice into vehicle group, docetaxel group (10 mg/kg), and Eeq group (10–20 mg/kg). The Eeq concentrations tested in mice refer to one of our previous studies. Ilicicolin A is also a marine compound which was isolated from the Beibu Gulf coral–derived fungus Acremonium sclerotigenum GXIMD 02501. It showed antiproliferative activity in human prostate cancer at 2.5–10 μM in vitro and at 10 mg/kg in vivo (Guo et al., 2021). The inhibitory activity of Eeq on prostate cancer proliferation in vitro is similar to that of ilicicolin A, so we set the 10 mg/kg dose as the initial dose of Eeq in vivo. We found that Eeq showed tumor growth inhibition in only half the number of mice at a dose of 10–20 mg/kg. In general, the tumor growth inhibition of 10–20 mg/kg Eeq was less effective than that of 10 mg/kg docetaxel in terms of tumor size and weight (Supplementary Figure S1). Then, we carried out the second experiment and changed the dose of Eeq to 20–40 mg/kg. Herein, 20–40 mg/kg Eeq dramatically inhibited tumor growth in mice, evidenced by a sharp decline in tumor size and weight (Figures 6A–C). Moreover, all mice showed good tolerance to 20–40 mg/kg Eeq, and no mice died during the experiment. There were no significant changes in body weight and organ index (Figure 6D; Supplementary Figures S2A–E). Furthermore, we also examined mice tumor tissues and found that Eeq also promoted DR5 and E-cadherin while inhibiting expression of caspase 8 (full length), surviving, and N-cadherin in vivo (Figures 6E–G). However, the expression of PI3K and Akt proteins in mice tumor tissue was not inhibited as in vitro (Figure 6H), suggesting that Eeq could not affect this signaling pathway in mice. Based on the findings above, we confirm that Eeq played an anti-PCa role through DR5 and beta-catenin/E-cadherin signaling pathways.
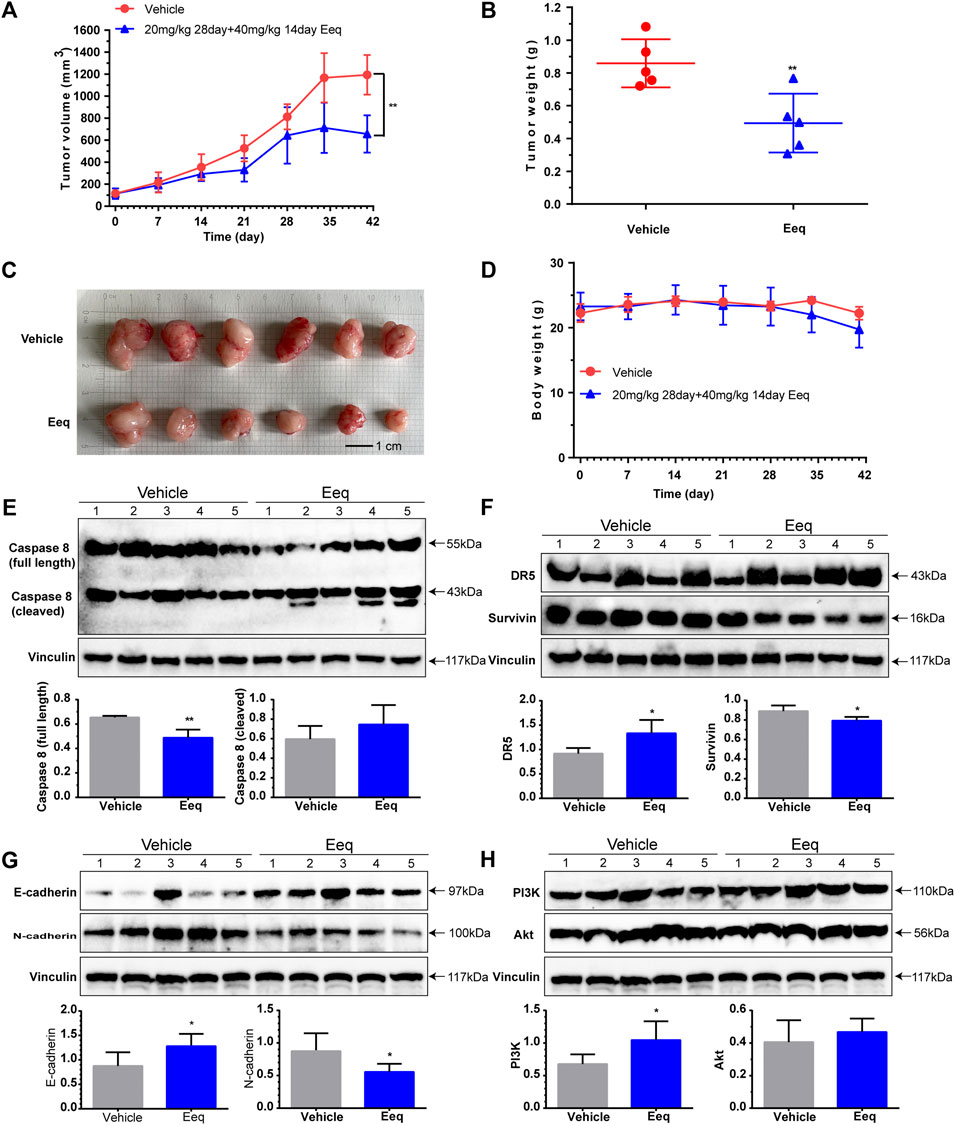
FIGURE 6. 5′-Epiequisetin (Eeq) suppressed prostate cancer development in vivo. (A) Eeq reduced tumor volume in mice. (B) Eeq decreased tumor weight in mice. (C) Tumor size and appearance in mice. (D) Body weight of mice during administration. (E) Eeq regulated the expression of caspase 8 protein in mice tumor tissue. (F) Eeq increased DR5 protein and decreased survivin protein expression in mice tumor tissue. (G) Eeq increased E-cadherin protein and decreased N-cadherin protein expression in mice tumor tissue. (H) Eeq had no significant effect on PI3K and Akt protein expression in tumor tissue of mice. *p < 0.5, **p < 0.01 vs. vehicle.
Discussion
PI3K/Akt signaling pathway is involved in the regulation of cell survival, cell cycle progression, and cellular growth, which has been widely recognized. It was found to be commonly activated in human cancer, thereby suppressing the function of this signaling pathway has become a strategy for treating cancer (Alzahrani, 2019). In the present study, we found that Eeq induced cell cycle to be arrested at the G1 phase terminated cell division, resulting in inhibition of PCa cell proliferation. We traced the PI3K/Akt signaling pathway that regulates the cell cycle, and we found that Eeq reduced PI3K and Akt protein expression and inhibited Akt phosphorylation at Ser473 in vitro. These findings evidenced that Eeq inhibited cell proliferation through negative regulation of PI3K/Akt signaling in PC-3 cells in vitro. However, assays on mice tumor tissue showed that Eeq did not reduce the expression of PI3K and Akt proteins as it did at the cellular level. These data showed that the results of PI3K and Akt proteins in vitro and in vivo are not consistent. These findings suggested that the role of Eeq on PI3K and Akt protein at the cellular level may not be shown at the integrated animal level.
Epithelial–mesenchymal transition (EMT) was found to enhance tumor cell metastasis, chemoresistance, and tumor stemness (Dongre and Weinberg, 2019; Lu and Kang, 2019). The increased expression of N-cadherin and the decreased expression of E-cadherin were associated with the development of EMT (Loh et al., 2019). Drug therapies that target these proteins have thus been developed (Mariotti et al., 2007; Wong et al., 2018). In this study, we found Eeq decreased expression of N-cadherin and increased expression of E-cadherin. This finding indicated that Eeq might alleviate and/or reverse the EMT process. The EMT process is regulated by a complex network of signaling pathways and transcription factors, including the PI3K/Akt/beta-catenin and Wnt/beta-catenin signaling pathways (Gonzalez and Medici, 2014). It is well known that the interaction between E-cadherin and β-catenin occurs during the EMT process (Schmalhofer et al., 2009), so we tracked the protein expression of beta-catenin and Wnt. We found Eeq indeed down-regulated the beta-catenin while it showed no impact on Wnt protein expression. Furthermore, we detected E-cadherin and N-Cadherin in mice tumor tissue and obtained consistent results at the cellular level. Conclusively, Eeq inhibited cell migration by attenuating the EMT process, which was related to the beta-catenin/cadherins signaling pathway.
DR5 is a member of the tumor necrosis factor receptor superfamily, which can initiate the apoptosis pathway by binding to its associated ligand, including synthetic and natural agents (Yuan et al., 2018). Therefore, it is considered to be a cancer treatment strategy. Bioymifi was the first DR5 activator reported in 2013, and it could bind directly to DR5 to activate the caspase 8-induced apoptosis signaling pathway, leading to human glioblastoma (T98G) cells apoptosis (Wang et al., 2013). In the present study, we found that Eeq also activated the caspase 8-induced apoptosis signaling pathway in vitro. We further confirmed that Eeq increased DR5 expression at both mRNA and protein levels. More intriguingly, when DR5 was silenced by small interfering RNAs, Eeq-induced apoptosis was drastically reduced. We also detected DR5 and Caspase 8 protein expression in mice tumor tissue and obtained consistent results at the cellular level.
These data suggest that Eeq induced apoptosis through DR5 both in vivo and in vitro, thus, exerting anti-PCa effects. To our knowledge, there are few reports on the biological activity of Eeq except the antibacterial effects hitherto. In this study, we first proved its anti-PCa effect from three phenotypes of cell proliferation, migration, and apoptosis and then revealed how it produced these effects. According to our current data, we concluded that Eeq could play anti-PCa roles through PI3K/Akt and DR5 signaling pathways. We also realize that these findings may not be the whole picture of the anticancer effect of Eeq due to the limitations of detection methods. However, we confirmed that Eeq indeed had an anti-PCa effect in vitro and in vivo. In addition, it may also have effects to overcome other tumors with aberrant PI3K/Akt and DR5 signaling pathways. We believe that the present study shed the first light on the anti-prostate cancer capacity of Eeq. However, whether Eeq can be developed into an agent for the treatment of prostate cancer and other tumors needs more extensive and in-depth research.
Data availability statement
The original contributions presented in the study are included in the article/Supplementary Material; further inquiries can be directed to the corresponding author.
Ethics statement
The animal study was reviewed and approved by the Guangxi University of Chinese Medicine Institutional Animal Ethical and Welfare Committee.
Author contributions
XW and YL initiated and designed the study; XL provided the tested compound; XW, XG, CC, ZY, JW, WF, HH, CG, XZ, and XF completed the experimental research under the guidance and supervision of YL; XW wrote the manuscript and drew the figures; XL and YL revised the manuscript.
Funding
This research was supported by the Specific Research Project of Guangxi for Research Bases and Talents (AD20159033 and AD20297003), the National Natural Science Foundation of China (21772210 and U20A20101), the Special Fund for Bagui Scholars of Guangxi (YL), and the Natural Science Foundation of Guangxi Zhuang Autonomous Region (2018GXNSFBA281043 and 2020GXNSFGA297002).
Acknowledgments
The authors would like to thank Prof. Pang (Yuzhou Pang, Guangxi University of Chinese Medicine) for kindly providing us with a laboratory and equipment.
Conflict of interest
The authors declare that the research was conducted in the absence of any commercial or financial relationships that could be construed as a potential conflict of interest.
Publisher’s note
All claims expressed in this article are solely those of the authors and do not necessarily represent those of their affiliated organizations, or those of the publisher, the editors, and the reviewers. Any product that may be evaluated in this article, or claim that may be made by its manufacturer, is not guaranteed or endorsed by the publisher.
Supplementary material
The Supplementary Material for this article can be found online at: https://www.frontiersin.org/articles/10.3389/fphar.2022.920554/full#supplementary-material
References
Alzahrani, A. S. (2019). PI3K/Akt/mTOR inhibitors in cancer: at the bench and bedside. Semin. Cancer Biol. 59, 125–132. doi:10.1016/j.semcancer.2019.07.009
Carroll, A. R., Copp, B. R., Davis, R. A., Keyzers, R. A., and Prinsep, M. R. (2021). Marine natural products. Nat. Prod. Rep. 2, 362–413. doi:10.1039/d0np00089b
Chang, F., Lee, J. T., Navolanic, P. M., Steelman, L. S., Shelton, J. G., Blalock, W. L., et al. (2003). Involvement of PI3K/Akt pathway in cell cycle progression, apoptosis, and neoplastic transformation: a target for cancer chemotherapy. Leukemia 3, 590–603. doi:10.1038/sj.leu.2402824
Chen, C., Luo, X., Li, K., Guo, C., Li, J., Lin, X., et al. (2019). Antibacterial secondary metabolites from a marine sponge-derived fungus Fusarium equiseti SCSIO 41019. Chin. J. Antibiotics 44, 1035–1040. doi:10.13461/j.cnki.cja.006629
Chen, S., Liu, D., Zhang, Q., Guo, P., Ding, S. Y., Shen, J. Z., et al. (2021). A marine antibiotic kills multidrug-resistant bacteria without detectable high-level resistance. ACS Infect. Dis. 7 (4), 884–893. doi:10.1021/acsinfecdis.0c00913
Coqueret, O. (2003). New roles for p21 and p27 cell-cycle inhibitors: a function for each cell compartment? Trends Cell Biol. 2, 65–70. doi:10.1016/s0962-8924(02)00043-0
Dongre, A., and Weinberg, R. A. (2019). New insights into the mechanisms of epithelial-mesenchymal transition and implications for cancer. Nat. Rev. Mol. Cell Biol. 2, 69–84. doi:10.1038/s41580-018-0080-4
Dyshlovoy, S. A., and Honecker, F. (2020). Marine compounds and cancer: updates 2020. Mar. Drugs 12, 643. doi:10.3390/md18120643
Fan, M., Nath, A. K., Tang, Y., Choi, Y. J., Debnath, T., Choi, E. J., et al. (2018). Investigation of the anti-prostate cancer properties of marine-derived compounds. Mar. Drugs 5, 160. doi:10.3390/md16050160
Galsky, M. D., Small, A. C., Tsao, C-k., and Oh, W. K. (2012). Clinical development of novel therapeutics for castration-resistant prostate cancer: historic challenges and recent successes. Ca. Cancer J. Clin. 5, 299–308. doi:10.3322/caac.21141
Ge, R., Wang, Z., Montironi, R., Jiang, Z., Cheng, M., Santoni, M., et al. (2020). Epigenetic modulations and lineage plasticity in advanced prostate cancer. Ann. Oncol. 4, 470–479. doi:10.1016/j.annonc.2020.02.002
Goel, S., DeCristo, M. J., Watt, A. C., BrinJones, H., Sceneay, J., Li, B. B., et al. (2017). CDK4/6 inhibition triggers anti-tumour immunity. Nature 7668, 471–475. doi:10.1038/nature23465
Gonzalez, D. M., and Medici, D. (2014). Signaling mechanisms of the epithelial-mesenchymal transition. Sci. Signal. 344, re8. doi:10.1126/scisignal.2005189
Guo, L., Luo, X., Yang, P., Zhang, Y., Huang, J., Wang, H., et al. (2021). Ilicicolin A exerts antitumor effect in castration-resistant prostate cancer via suppressing EZH2 signaling pathway. Front. Pharmacol. 12, 723729. doi:10.3389/fphar.2021.723729
Hamidi, A., Song, J., Thakur, N., Itoh, S., Marcusson, A., Bergh, A., et al. (2017). TGF-β promotes PI3K-AKT signaling and prostate cancer cell migration through the TRAF6-mediated ubiquitylation of p85α. Sci. Signal. 486, eaal4186. doi:10.1126/scisignal.aal4186
Loh, C. Y., Chai, J. Y., Tang, T. F., Wong, W. F., Sethi, G., Shanmugam, M. K., et al. (2019). The E-cadherin and N-cadherin switch in epithelial-to-mesenchymal transition: signaling, therapeutic implications, and challenges. Cells 10, 1118. doi:10.3390/cells8101118
Lu, W., and Kang, Y. (2019). Epithelial-mesenchymal plasticity in cancer progression and metastasis. Dev. Cell 3, 361–374. doi:10.1016/j.devcel.2019.04.010
Mariotti, A., Perotti, A., Sessa, C., and Rüegg, C. (2007). N-cadherin as a therapeutic target in cancer. Expert Opin. Investig. Drugs 4, 451–465. doi:10.1517/13543784.16.4.451
Mayer, A. (2020). Marine pharmaceutical: The clinical pipeline. Available at: https://www.midwestern.edu/departments/marinepharmacology/clinical-pipeline (Accessed Dec 14, 2021).
Muñoz-Pinedo, C., and López-Rivas, A. (2018). A role for caspase-8 and TRAIL-R2/DR5 in ER-stress-induced apoptosis. Cell Death Differ. 1, 226. doi:10.1038/cdd.2017.155
Newman, D. J., and Cragg, G. M. (2020). Natural products as sources of new drugs over the nearly four decades from 01/1981 to 09/2019. J. Nat. Prod. 3, 770–803. doi:10.1021/acs.jnatprod.9b01285
Schmalhofer, O., Brabletz, S., and Brabletz, T. (2009). E-cadherin, beta-catenin, and ZEB1 in malignant progression of cancer. Cancer Metastasis Rev. 1-2, 151–166. doi:10.1007/s10555-008-9179-y
Siegel, R. L., Miller, K. D., Fuchs, H. E., and Jemal, A. (2021). Cancer statistics, 2021. CA. Cancer J. Clin. 1, 7–33. doi:10.3322/caac.21654
Sung, H., Ferlay, J., Siegel, R. L., Laversanne, M., Soerjomataram, I., Jemal, A., et al. (2021). Global cancer statistics 2020: GLOBOCAN estimates of incidence and mortality worldwide for 36 cancers in 185 countries. CA. Cancer J. Clin. 3, 209–249. doi:10.3322/caac.21660
Tang, W., Lv, B., Yang, B., Chen, Y., Yuan, F., Ma, L., et al. (2019). TREM2 acts as a tumor suppressor in hepatocellular carcinoma by targeting the PI3K/Akt/β-catenin pathway. Oncogenesis 2, 9. doi:10.1038/s41389-018-0115-x
Wang, G., Wang, X., Yu, H., Wei, S., Williams, N., Holmes, D. L., et al. (2013). Small-molecule activation of the TRAIL receptor DR5 in human cancer cells. Nat. Chem. Biol. 2, 84–89. doi:10.1038/nchembio.1153
Wang, P., Henning, S. M., Magyar, C. E., Elshimali, Y., Heber, D., and Vadgama, J. V. (2016). Green tea and quercetin sensitize PC-3 xenograft prostate tumors to docetaxel chemotherapy. J. Exp. Clin. Cancer Res. 35, 73. doi:10.1186/s13046-016-0351-x
Wang, X., Zhu, J., Yan, H., Shi, M., Zheng, Q., Wang, Y., et al. (2021). Kaempferol inhibits benign prostatic hyperplasia by resisting the action of androgen. Eur. J. Pharmacol. 907, 174251. doi:10.1016/j.ejphar.2021.174251
Wong, S. H. M., Fang, C. M., Chuah, L. H., Leong, C. O., and Ngai, S. C. (2018). E-cadherin: Its dysregulation in carcinogenesis and clinical implications. Crit. Rev. Oncol. Hematol. 121, 11–22. doi:10.1016/j.critrevonc.2017.11.010
Xu, Z., Liu, D. Y., Liu, D., Ren, X., Liu, H., Qi, G. H., et al. (2022). Equisetin is an anti-obesity candidate through targeting 11β-HSD1. Acta Pharm. Sin. B 12 (5), 2358–2373. doi:10.1016/j.apsb.2022.01.006
Yuan, X., Gajan, A., Chu, Q., Xiong, H., Wu, K., and Wu, G. S. (2018). Developing TRAIL/TRAIL death receptor-based cancer therapies. Cancer Metastasis Rev. 4, 733–748. doi:10.1007/s10555-018-9728-y
Zhang, M. M., Wang, M. J., Zhu, X. C., Yu, W. G., and Gong, Q. H. (2018). Equisetin as potential quorum sensing inhibitor of Pseudomonas aeruginosa. Biotechnol. Lett. 40 (5), 865–870. doi:10.1007/s10529-018-2527-2
Zhang, Q., Chen, S., Liu, X., Lin, W., and Zhu, K. (2021). Equisetin restores colistin sensitivity against multi-drug resistant gram-negative bacteria. Antibiot. (Basel) 10 (10), 1263. doi:10.3390/antibiotics10101263
Zhang, S., Sun, K., Zheng, R., Zeng, H., He, J., Chen, R., et al. (2021). Cancer incidence and mortality in China, 2015. J. Natl. Cancer Cent. 1, 2–11. doi:10.1016/j.jncc.2020.12.001
Zhang, X., Wang, L., and Qu, Y. (2020). Targeting the β-catenin signaling for cancer therapy. Pharmacol. Res. 160, 104794. doi:10.1016/j.phrs.2020.104794
Keywords: 5′-epiequisetin, prostate cancer, phosphoinositol 3-kinase, protein kinase B, death receptor 5
Citation: Wang X, Luo X, Gan X, Chen C, Yang Z, Wen J, Fang W, Huang H, Gao C, Zhou X, Feng X and Liu Y (2022) Analysis of regulating activities of 5′-epiequisetin on proliferation, apoptosis, and migration of prostate cancer cells in vitro and in vivo. Front. Pharmacol. 13:920554. doi: 10.3389/fphar.2022.920554
Received: 14 April 2022; Accepted: 11 July 2022;
Published: 10 August 2022.
Edited by:
Rosy Iara Maciel De Azambuja Ribeiro, Universidade Federal de São João del-Rei, BrazilReviewed by:
Viviane Aline Oliveira Silva, Barretos Cancer Hospital, BrazilCatherine Maria McDermott, Bond University, Australia
Copyright © 2022 Wang, Luo, Gan, Chen, Yang, Wen, Fang, Huang, Gao, Zhou, Feng and Liu. This is an open-access article distributed under the terms of the Creative Commons Attribution License (CC BY). The use, distribution or reproduction in other forums is permitted, provided the original author(s) and the copyright owner(s) are credited and that the original publication in this journal is cited, in accordance with accepted academic practice. No use, distribution or reproduction is permitted which does not comply with these terms.
*Correspondence: Yonghong Liu, yonghongliu@scsio.ac.cn
†These authors have contributed equally to this work and share first authorship