- 1Platform of Biopharmacy, Faculty of Pharmacy, Université de Montréal, Montréal, QC, Canada
- 2CHU Sainte-Justine, Montréal, QC, Canada
- 3Faculty of Pharmacy, Université de Montréal, Montréal, QC, Canada
- 4Department of Pediatrics, Université de Montréal, Montréal, QC, Canada
Cannabis is one of the most widely used illicit drugs during pregnancy and lactation. With the recent legalization of cannabis in many countries, health professionals are increasingly exposed to pregnant and breastfeeding women who are consuming cannabis on a regular basis as a solution for depression, anxiety, nausea, and pain. Cannabis consumption during pregnancy can induce negative birth outcomes such as reduced birth weight and increased risk of prematurity and admission to the neonatal intensive care unit. Yet, limited information is available regarding the pharmacokinetics of cannabis in the fetus and newborn exposed during pregnancy and lactation. Indeed, the official recommendations regarding the use of cannabis during these two critical development periods lack robust pharmacokinetics data and make it difficult for health professionals to guide their patients. Many clinical studies are currently evaluating the effects of cannabis on the brain development and base their groups mostly on questionnaires. These studies should be associated with pharmacokinetics studies to assess correlations between the infant brain development and the exposure to cannabis during pregnancy and breastfeeding. Our project aims to review the available data on the pharmacokinetics of cannabinoids in adults, neonates, and animals. If the available literature is abundant in adult humans and animals, there is still a lack of published data on the exposure of pregnant and lactating women and neonates. However, some of the published information causes concerns on the exposure and the potential effects of cannabis on fetuses and neonates. The safety of cannabis use for non-medical purpose during pregnancy and breastfeeding needs to be further characterized with proper pharmacokinetic studies in humans feasible in regions where cannabis has been legalized. Given the available data, significant transfer occurs to the fetus and the breastfed newborn with a theoretical risk of accumulation of products known to be biologically active.
1 Introduction
1.1 Prevalence of Cannabis Use in General and Vulnerable Populations
Cannabis is the most commonly used illicit drug and its popularity is increasing with the years (Centers for Disease Control and Prevention, 2017; Statistics Canada, 2018; Statista, 2020). In Canada, 20% of people over 15—(nearly 6.2 million)– reported having used cannabis in 2020. This number represents a 6% increase in comparison to 2018 (Rotermann, 2021). In the United States and Europe, 9.6 % and 7.6% of the population over 12 years of age used it in 2017 and 2019, respectively (Dai and Richter, 2019; European Monitoring Centre for Drugs and Drug Addiction, 2022). Legalization in many countries or states and the development of new formulations (e.g., edibles and vaporizers) can explain this increase. In Canada in 2018, the number of cannabis users has increased by 5% after its legalization (Rotermann, 2021). Similarly in the United States, states where cannabis is legal exhibited the highest percentage of cannabis users between 2018 and 2019 while states where cannabis is either illegal or decriminalized showed the lowest percentage of cannabis users (Substance of Abuse and Mental Health Service Administration, Center for Behavioral Health Statistics and Quality, 2020).
Limited information is available on cannabis use during pregnancy and lactation especially since it remains illegal in many countries. However, it is well established that cannabis is the most widely used illicit drug during pregnancy. In the United States of America, a study reported that among 14,400 pregnant women, 3.8% have used cannabis during pregnancy, 6.5% were in their first trimester, 3.3% in their second trimester and 1.8% in their third trimester, while another reported an increase in use as 2.4% and 3.9% of pregnant women used cannabis in 2002 and 2014, respectively (Brown et al., 2017; Volkow et al., 2017). In Canada, Corsi et al. observed that among 732,818 pregnant women, 10,731 (1.5%) used cannabis during pregnancy with an increase from 1.2% in 2012 to 1.8% in 2017, based on interviews and medical records (Corsi et al., 2019). One study, carried out in Colorado, where cannabis is legal since 2012, showed that 5% of women used cannabis in the first weeks post-partum while breastfeeding (Crume et al., 2018). In addition, a questionnaire to clients of the Women, Infants and Children (WIC) program, a federal program in the USA for the health and nutrition of low-income pregnant and lactating women and children under 5 years old, revealed that 18% of women used cannabis at least once while breastfeeding (Wang, 2017). These values could be higher and underreported due to fear of losing their child’s custody. In Canada, no information is available to date on cannabis use during breastfeeding.
With its recent legalization, health professionals are increasingly exposed to pregnant and breastfeeding mothers who are consuming cannabis on a regular basis (Wang, 2017), as some sources on the Internet are touting cannabis as a solution for depression, anxiety, stress, pain, and nausea (Pertwee, 2012). However, when used during pregnancy, cannabis can induce negative birth outcomes such as reduced birth weight, and increased risk of prematurity and admission to the neonatal intensive care unit (Hayatbakhsh et al., 2012; Conner et al., 2016; Gunn et al., 2016). Longitudinal studies have reported behavioral and neurocognitive impairment in children exposed to cannabis in utero or during breastfeeding, after controlling for confounding variables such as sex of the child, ethnicity, socioeconomic status, prenatal exposure to tobacco and alcohol, or maternal substance use (Fried and Watkinson, 1990; Day et al., 1994; Goldschmidt et al., 2004; Paul et al., 2021). Moreover, cognitive deficits (e.g., executive functions, attention, memory), impulsivity and aggressive behavior were the most frequently observed effects, with long-lasting consequences up to young adulthood (Smith et al., 2006; Smith et al., 2010; Smith et al., 2016; Porath, 2018). However, most of these studies were performed in the 1980’s when THC levels in cannabis and the frequency of use were lower (Thompson et al., 2019). Therefore, it is likely that in 2022 these effects may be accentuated or that new effects may be observed. Unfortunately, there is no study available, to date, that evaluated the relationship between cannabinoid concentrations and the effects in mothers and their infants. In adults, pharmacokinetics-pharmacodynamics studies have shown that the intensity of THC effects depends on THC concentrations in the effect compartment (Grotenhermen, 2003). This relationship is likely to be similar in mothers and infants exposed to cannabis. In that regard, the Society of Obstetricians and Gynecologists of Canada has recommended in its 2022 guideline to design future studies exploring a dose-response relationship between cannabis and outcomes (Graves et al., 2022a).
1.2 Metabolism and Effects of Cannabinoids on Humans
Cannabis is mostly composed of cannabinoids that activate the endocannabinoid receptors (CB1 and CB2) found in the human body (Huestis, 2007). They can be classified in three types: vegetal (phytocannabinoids), endogenous (endocannabinoids) and synthetic cannabinoids (NPS). The most familiar and most studied cannabinoids are delta-9-tetrahydrocannabinol (THC), cannabidiol (CBD), cannabinol (CBN), cannabigerol (CBG), cannabichromene (CBC) and tetrahydrocannabivarin (THCV) (Health Canada and Branch, 2018).
THC is by far the most abundant and the most active cannabinoid in cannabis plants. This cannabinoid is a partial agonist of both CB1 and CB2 receptors, but has greater activity at CB1 subtypes, which is thought to account for its psychoactive effects producing changes in cognition, mood, or emotions (Mannekote Thippaiah et al., 2021). Thereby, THC is used for its psychoactive effects, causing a feeling of euphoria and well-being. Unfortunately, it is also known for its adverse effects including memory loss, tachycardia, nervousness, anxiety, paranoia and spatiotemporal disorientation. The hemp plant, also called Cannabis Sativa, is the most widely used for producing psychoactive products. It can contain up to 20% of THC depending on the part of the plant used. Manufactured products can contain from 5 to 25% of THC for marijuana joints, up to 35% for hashish, up to 60% for hashish oil and up to 90% for dabs (wax, shatter) (Gourvernement du Québec, 2021).
THC is widely distributed in adipose tissues due to its high lipophilicity. It is first metabolized in the liver by cytochromes P450 2C9, 2C19 and 3A4 to the active 11-hydroxy-delta 9-tetrahydrocannabinol (11-OH-THC), then oxidized to the inactive 11-nor-9-carboxy-delta 9-tetrahydrocannabinol (THC-COOH). Finally, THC-COOH undergoes glucuronidation by glucuronosyltransferases (UGT1A1, 1A3, 1A9 and 1A10) to become the inactive 11-nor-9-carboxy-delta 9-tetrahydrocannabinol glucuronide (THC-COOH glucuronide). THC can also be metabolized by the UDP-glucosyltransferase (UGT) in inactive delta 9-tetrahydrocannabinol glucuronide (THC glucuronide). 8-hydroxy-THC (8-OH-THC) and 8ß,11-dihydroxy-THC (8ß,11-diOH-THC), both active metabolites of THC, can also be used as biomarkers of cannabis use (Dinis-Oliveira, 2016). THC metabolism is altered during pregnancy as cytochromes P450 and UGT’s activity is modified during this period. Indeed, the activity of CYP2C19, 3A4 and UGTs is increased whereas that of CYP2C9 is decreased, suggesting a different exposure to cannabinoids during pregnancy (Ward and Varner, 2019). Moreover, it is well known that both THC and CBD can be measured in breast milk, cross the placental and cross the blood-brain barrier where they can activate endogenous cannabinoid receptors given their lipophilic properties.
Cannabidiol (CBD) is the second most abundant cannabinoid in hemp plants. In contrast to THC, CBD does not have psychotropic effects and acts as a THC modulator, i.e., it attenuates the psychoactive effects of THC. This cannabinoid has lower affinity for both receptors and is a non-competitive negative allosteric modulator of the CB1 receptor. Furthermore, CBD has been shown to exhibit agonist properties on 5-HT1A receptors, potentially explaining its possible antidepressant or cognition enhancement effects (Celada et al., 2004; Russo et al., 2005). Numerous clinical studies are currently studying the benefits of CBD such as relaxing, sedative, anti-inflammatory, antispasmodic, antiepileptic, antipsychotic, antiemetic, and anti-addictive effects (Health Canada and Branch, 2018). CBD can be used with THC or alone. Indeed, many CBD oils (5%–20% CBD), pastes (30%–50% CBD), creams, lotions, patches, vaporizers and capsules do not contain any THC (Johnson, 2020). The metabolism of CBD is similar to THC, as it is first metabolized by the cytochrome P450 (CYP) to the active 7-hydroxy-cannabidiol (7-OH-CBD), then oxidized to the inactive 7-carboxy-cannabidiol (7-CBD-COOH). CBD and its metabolites are also excreted as inactive glucuronide conjugates. In the same way as THC, CBD metabolism will also be altered during pregnancy.
Cannabinol (CBN) comes from the oxidation of THC in the presence of UV rays or oxygen. It has 10% of the psychoactive effects of THC (Health Canada and Branch, 2018). Additionally, CBN could have analgesic, anti-inflammatory, antispasmodic, relaxing and anxiolytic effects. It also has adverse effects associated with a high consumption such as fatigue, weariness, and reduced heart rate (Health Canada and Branch, 2018).
Little is known concerning the minor cannabinoids CBG, CBC and THCV. CBG and CBC are present at less than 1% in Cannabis Sativa. Currently, they are being studied to determine their potential benefits and are not yet commercialized (Health Canada and Branch, 2018).
1.3 Objectives
The objectives of the current review are fourfold: 1. to review the literature on the main pharmacokinetics parameters of cannabinoids in animals, general population, pregnant and lactating women and neonates; 2. to identify the cannabinoids used as biomarkers of recent and past exposure to cannabis; 3. to identify the matrices that are used to assess recent and past exposure to cannabis and to assess exposure during pregnancy and lactation and; 4. to identify missing data on the pharmacokinetics of cannabinoids that should be identified as a priority in future studies aimed at monitoring fetal and neonatal toxicity.
2 Methods
A literature search was conducted on PubMed and on the Drugs and Lactation Database (LactMed) to identify the literature on the pharmacokinetics of cannabinoids in animals, adults, pregnant and lactating women and neonates. The scientific literature was reviewed on the pharmacokinetics of cannabinoids in animals and humans from their inception until July 2021. The following terms were used to search the literature: cannabinoids, cannabis, THC, delta-9-THC, tetrahydrocannabinol, delta-9-tetrahydrocannabinol, cannabidiol, dronabinol, marijuana, pharmacokinetics, exposure, quantification, analysis, detection, animals, humans, adults, newborns, meconium, oral fluid, umbilical cord, hair, blood, breast milk, lactation, breastfeeding, pregnancy, and gestation. Additionally, a screening of the reference list of selected articles was performed to collect further relevant papers. Only English written articles were included in this review. Figure 1 represents the literature review flow chart.
2.1 Pharmacokinetics of Cannabinoids in the General Population
To assess human exposure to cannabinoids, several matrices have been characterized. Recent exposure to cannabinoids can be evaluated by quantifying cannabinoids in sweat, oral fluid, plasma, serum, and whole blood, while past exposure can be assessed by quantifying cannabinoids in hair and urine. Recent exposure is useful to determine main pharmacokinetics parameters of cannabinoids, i.e., the maximal concentration (Cmax), the time required to reach the maximal concentration (Tmax), and to understand the pathway of cannabinoids in the body. In contrast, assessing past exposure is useful to estimate the mean residence time of cannabinoids in the body and to assess the frequency of use of cannabis. Collecting all this information could be useful to estimate the exposure of pregnant and lactating women and their infants to cannabinoids. Different routes of administration are used in humans, each with their own Cmax, Tmax and T1/2. The pharmacokinetics of cannabinoids in general population is presented in Table 1.
2.1.1 Recent exposure to Cannabis
2.1.1.1 Pharmacokinetics of Cannabinoids in Plasma and Serum
Plasma and serum matrices have been characterized in many clinical studies as the drug’s pharmacodynamic and toxicity is related to concentrations in these matrices (Ohlsson et al., 1980; Lindgren et al., 1981; Cami et al., 1991; Perez-Reyes et al., 1991; Huestis et al., 1992a; Huestis et al., 1992b; Kelly and Jones, 1992; Manno et al., 2001; Huestis and Cone, 2004; Naef et al., 2004; Nadulski et al., 2005; Goodwin et al., 2006; Abrams et al., 2007; Kauert et al., 2007; Hunault et al., 2008; Skopp and Potsch, 2008; Toennes et al., 2008; Schwilke et al., 2009; Karschner et al., 2011; Milman et al., 2011; Schwope et al., 2011; Eichler et al., 2012; Klumpers et al., 2012; Stott et al., 2013a; Stott et al., 2013b; Lile et al., 2013; Desrosiers et al., 2014a; Ahmed et al., 2014; Eisenberg et al., 2014; Milman et al., 2014; Ahmed et al., 2015; Hartman et al., 2015; Lee et al., 2015; Manini et al., 2015; de Vries et al., 2016; Marsot et al., 2016; Cherniakov et al., 2017a; de Vries et al., 2017; Atsmon et al., 2018a; Atsmon et al., 2018b; Birnbaum et al., 2019; Knaub et al., 2019; Patrician et al., 2019; Taylor et al., 2019; Wolowich et al., 2019; Pichini et al., 2020a; Pichini et al., 2020b; Crockett et al., 2020; Hobbs et al., 2020). Cannabinoids tested, routes of administration, times of detection and pharmacokinetics parameters (Cmax, Tmax, T1/2) are all reported in Table 1. The variation in concentrations after pulmonary administration can be explained by multiple factors including first the difference in dosages but also, the difference in formulations, the fasting/fed stage, the addition of alcohol, tobacco, or other drugs and the interindividual variability regarding the age, the smoking topography, and the history of cannabis use. Variation of Tmax between studies is mostly caused by the variation in sampling times. Skopp observed that THC-COOH was detectable up to 48 h and THC-COOH glucuronide for more than 48 h in plasma samples of light, moderate and heavy smokers (Skopp and Pötsch, 2008).
Oral formulations were composed of THC alone, THC + CBD or CBD alone (Ohlsson et al., 1980; Nadulski et al., 2005; Goodwin et al., 2006; Schwilke et al., 2009; Karschner et al., 2011; Milman et al., 2011; Eichler et al., 2012; Klumpers et al., 2012; Stott et al., 2013b; Lile et al., 2013; Ahmed et al., 2014; Milman et al., 2014; Ahmed et al., 2015; Manini et al., 2015; de Vries et al., 2016; de Vries et al., 2017; Atsmon et al., 2018a; Atsmon et al., 2018b; Birnbaum et al., 2019; Knaub et al., 2019; Patrician et al., 2019; Taylor et al., 2019; Wolowich et al., 2019; Pichini et al., 2020b; Crockett et al., 2020; Hobbs et al., 2020; Pérez-Acevedo et al., 2020; Pérez-Acevedo et al., 2021). A high interindividual variability was observed across studies, explaining the large intervals of concentration. The fasting/fed stage, the formulation, the use of alcohol, the age and the dosage also influenced cannabinoid concentrations. As expected, reported Tmax were longer after oral than after pulmonary consumption. The high variation between half-lives is explained by the difference in sampling times between studies. Indeed, sampling periods vary between 2 and 130 h.
THC, CBD and THC metabolites (11-OH-THC and THC-COOH) are well characterized in plasma and serum and have demonstrated to be crucial in understanding the effects of cannabis in humans. Interestingly, THC-COOH known to be inactive, is eliminated more slowly and has higher plasma concentrations than THC. Thus, this metabolite is useful for assessing cannabis exposure over a longer period. In addition, ratios of THC on its metabolites or on CBD is promising to assess recent exposure to cannabis (Pichini et al., 2020b). THC-COOH glucuronide has an even longer half-life than THC-COOH and is detectable in most of the plasma samples, 48 h after the last consumption of cannabis (Skopp and Pötsch, 2008). Therefore, it could be used to determine someone’s last cannabis consumption. CBD metabolites, 7-OH-CBD and 7-CBD-COOH are measured at higher concentrations than CBD. Thus, they should be included in pharmacokinetics studies after CBD consumption. Studies evaluating the effects of cannabis on humans should also quantify CBN as it can evaluate recent cannabis use. In plasma and serum, sampling should be performed for at least 24 h with more samples during the first hour after pulmonary administration controlling for THC, 11-OH-THC, THC-COOH, THC-COOH glucuronide, CBD, 7-OH-CBD, 7-CBD-COOH and CBN. Finally, attention should be paid to the use of food, alcohol, tobacco, or drugs before the beginning of the study as it could influence the pharmacokinetics of cannabinoids in plasma.
2.1.1.2 Pharmacokinetics of Cannabinoids in Whole Blood
Whole blood gives similar information than plasma and serum. This matrix is advantageous as it does not need to be centrifuged immediately after extraction. However, it is less stable than plasma or serum and samples should be analyzed quickly after their collection.
THC, 11-OH-THC, THC-COOH, THC-COOH glucuronide, THCVCOOH, THC glucuronide, THCV, CBD, CBN and CBG were measured in whole blood (Table 1) (Schwope et al., 2011; Karschner et al., 2012; Schwope et al., 2012; Fabritius et al., 2013; Desrosiers et al., 2014a; Hartman et al., 2015; Newmeyer et al., 2016; Newmeyer et al., 2017; Pellesi et al., 2018; Hartley et al., 2019; Spindle et al., 2019; Spindle et al., 2020a). Pharmacokinetics of THC, 11-OH-THC, THC-COOH, THC-COOH glucuronide, CBD and CBN seem to be similar in plasma, serum, and whole blood. Therefore, the same considerations should be applied to pharmacokinetics studies in whole blood.
2.1.1.3 Pharmacokinetics of Cannabinoids in Oral Fluid (Saliva)
Oral fluid has many advantages for cannabinoid quantification: its collection is simple and non-invasive. Moreover, it gives a good idea of someone’s recent exposure to cannabis. Indeed, oral fluid is often used to detect drivers under the influence of cannabis. However, one should be careful of contamination of the oral cavity after smoking cannabis. As THC-COOH concentrations is the result of THC hepatic metabolism and is not present in cannabis smoke, its quantification is highly important in oral fluid. THC, 11-OH-THC, THC-COOH, THC-COOH glucuronide, THC glucuronide, CBD, CBN, CBG, THCV, THCA-A and CBDA were quantified in oral fluid (Huestis and Cone, 2004; Moore et al., 2006; Kauert et al., 2007; Milman et al., 2010; Toennes et al., 2010; Milman et al., 2011; Lee et al., 2012; Fabritius et al., 2013; Toennes et al., 2013; Marsot et al., 2016; Newmeyer et al., 2017; Pacifici et al., 2018; Spindle et al., 2019; Pichini et al., 2020a; Spindle et al., 2020a; Pérez-Acevedo et al., 2021). Pharmacokinetics parameters of cannabinoids in oral fluid are presented in Table 1. High variability in concentrations after pulmonary administration can be explained by the smoking topography (puff volume, duration, flow), cannabis use history, and the contamination of the oral cavity, in addition to the difference in doses. THC, THC-COOH, CBD, CBD-COOH and CBN should be quantified in oral fluid for pharmacokinetics studies or to assess the short-term exposure to cannabis. Finally, pharmacokinetics studies using oral fluid should collect samples for at least 24 h starting at baseline.
2.1.1.4 Pharmacokinetics of Cannabinoids in Sweat
Pichini, Perez-Acevedo, Gambelunghe, Huestis and Kintz have assessed the exposure to cannabis by quantifying cannabinoids in sweat as a replacement of oral fluid (Kintz et al., 2000; Huestis et al., 2008; Gambelunghe et al., 2016; Pichini et al., 2020b; Pérez-Acevedo et al., 2021). THC, 11-OH-THC, THC-COOH, THC-COOH glucuronide, THC glucuronide, CBD, CBN, THCA-A and CBDA were the cannabinoids targeted in these studies. Cmax, Tmax and T1/2 are presented in Table 1. After oral doses of cannabis, only THC, CBD and CBN were detected in sweat. Sweat can be employed for monitoring drug users in the workplace, treatment, and judicial program (Huestis et al., 2008). If sweat is chosen to assess the exposure to cannabis, THC, CBD and CBN should be monitored. Further studies are needed to better understand the pharmacokinetics of these cannabinoids in sweat.
2.1.2 Past Exposure
2.1.2.1 Pharmacokinetics of Cannabinoids in Urine
To assess the past exposure to cannabis, urine is the ideal matrix as cannabinoids are detectable for many weeks. Multiple studies have measured THC and its metabolites, THCV, CBD and its metabolites, CBN, THCA-A and CBDA concentrations in urine (Table 1) (Kelly and Jones, 1992; Huestis and Cone, 1998; Manno et al., 2001; Westin et al., 2009; Desrosiers et al., 2014b; Manini et al., 2015; Marsot et al., 2016; Pacifici et al., 2018; Patrician et al., 2019; Pichini et al., 2020b; Spindle et al., 2020b; Pérez-Acevedo et al., 2020; Pérez-Acevedo et al., 2021). Only THC, 11-OH-THC, THC-COOH, THC-COOH glucuronide, THC glucuronide and CBD were detected after pulmonary administration (Huestis and Cone, 1998; Manno et al., 2001; Desrosiers et al., 2014b; Marsot et al., 2016; Pacifici et al., 2018; Spindle et al., 2020b). THC-COOH was detected in urine up to 14 weeks after the last consumption. If urine is used, THC, 11-OH-THC, THC-COOH, THC-COOH, THC glucuronide, CBD, 7-OH-CBD and 7-CBD-COOH should be tested.
2.1.2.2 Pharmacokinetics of Cannabinoids in Hair
Past exposure to cannabis can also be detected in hair samples. Hartley, Gambelunghe, Taylor and Tzatzarakis have quantified THC, 11-OH-THC, THC-COOH, CBD and CBN in hair samples (Table 1) (Gambelunghe et al., 2016; Taylor et al., 2017; Tzatzarakis et al., 2017; Hartley et al., 2019). This matrix enables the detection of cannabis consumption up to 7 months prior sampling. THC-COOH quantification in hair samples is helpful to distinguish cannabis consumption from passive exposure (Gambelunghe et al., 2016). Thus, THC, CBD, CBN and THC-COOH should be tested to assess cannabis exposure in hair samples. However, more studies are needed to understand the pharmacokinetics of cannabinoids in hair. Indeed, it is, yet, not possible to make correlations between the presence of any cannabinoid, their concentration, and the last time of cannabis use.
2.2 Pharmacokinetics of Cannabinoids in Pregnant or Lactating Women and Newborns
Assessing the exposure of newborns to cannabis during pregnancy and lactation is essential to understand the effects of cannabis on the newborn’s development. Unfortunately, only a few studies have attempted to evaluate cannabis exposure during these two periods. Indeed, most of published studies aimed to assess whether the newborn was exposed to cannabis during pregnancy and, thus, used qualitative analysis rather than quantitative analysis. Moreover, no study has assessed the pharmacokinetics of cannabinoids in pregnant women, even though the pharmacokinetics will be altered during pregnancy and could influence the effects of cannabis on women. Therefore, pharmacokinetics studies in pregnant women could bring crucial information for the mother and her newborn, as no non-invasive method exists to quantify cannabinoids in fetuses. Indeed, exposition during pregnancy was only evaluated in one study testing urine samples of a mother and shortly after birth in meconium, hair, urine, cord blood and umbilical cord samples of newborns. Newborn’s exposure during breastfeeding has been assessed in some studies by quantifying cannabinoids in breast milk samples. Cannabinoid concentrations in pregnant and lactating women and neonates are summarized in Table 2.
2.2.1 Pharmacokinetics of Cannabinoids in Pregnant Women
Measuring cannabinoid concentrations in pregnant women is the first step in understanding the exposure of newborns during this period. Indeed, a drug pharmacokinetics can be altered due to major physiological changes in women during this period. Unfortunately, no study has measured plasmatic concentrations of cannabinoids. Westin et al. (2009) measured the concentration of THC-COOH in the urine of one pregnant woman during 14 weeks after the last consumption of cannabis reported by the woman. Concentration at week 2 was 346 ng/ml, and concentration at week 14 was 3.9 ng/ml. Assuming that the mother’s report is accurate, THC-COOH could be detected in urine up to 14 weeks after last consumption. As the THC dose taken by the pregnant mother in this study is unknown, it is difficult to compare THC-COOH concentrations to the ones obtained in the general population. For cannabis, it is expected that THC clearance will be increased due to a higher activity of the CYP2C19 and UGTs, enzymes responsible of THC metabolism (Grant et al., 2018). Moreover, an increase of the body fat could increase the volume of distribution of THC. Thus, THC concentrations might be decreased, and THC metabolite concentrations might be increased. However, free cannabinoid concentrations could increase, as THC and THC metabolites are highly bound to serum proteins and albumin which are decreased during pregnancy. During the third trimester, these three cannabinoids could accumulate in the fetus due to a higher concentration of fetal serum proteins than maternal serum proteins and a lower pH of fetal blood than maternal blood (Ward and Varner, 2019).
This could be confirmed in future studies in pregnant women by measuring THC, 11-OH-THC, THC-COOH, CBD, OH-CBD, CBD-COOH and CBN concentrations in blood for at least 24 h at each trimester while controlling the amount of cannabis ingested by the mother.
2.2.2 Pharmacokinetics of Cannabinoids in Breastfeeding Women
Breast milk can be tested in adults to assess short-term exposure to cannabis. It has the advantage of assessing both the exposure of the mother and the breastfed child to cannabis. Unfortunately, only a few studies have measured cannabinoids in breast milk and assessed their pharmacokinetics in this matrix (Presence of Δ9, 1982; Marchei et al., 2011; Baker et al., 2018; Bertrand et al., 2018; Moss et al., 2021; Wymore et al., 2021; Sempio et al., 2020). Indeed, such studies are difficult to conduct because many mothers refuse to enroll by fear of being judged or underreport their cannabis consumption making it difficult to correlate measured concentrations and the dose taken or simply stop their consumption during the study. Table 1 summarizes the pharmacokinetics parameters of cannabinoids in breast milk. Baker was the only one to assess the transfer of cannabis into breast milk by measuring cannabinoids at specific time points (Baker et al., 2018). If 11-OH-THC and THC-COOH were not quantifiable in any of the breast milk samples, THC was still quantifiable 4 hours after the cannabis administration suggesting that pharmacokinetic studies in breast milk should be performed over a longer period. Indeed, THC metabolites were probably not detected in breast milk because of the short sampling period. Bertrand et al. measured concentrations for THC, 11-OH-THC and CBD but did not detect cannabinol in any of the samples (Bertrand et al., 2018). Most concentrations were measured within 48 h after the last consumption, but one sample had THC concentrations up to 6 days after the last cannabis intake. Moss et al. (Moss et al., 2021) measured similar cannabinoid concentrations (THC, 11-OH-THC, THC-COOH and CBD) in breast milk after inhalation of cannabis or after edible use within 40 h after last consumption. In both cases, THC and CBD concentrations were at least two times higher in breast milk than in plasma. Inversely, THC metabolite concentrations were at least two times higher in plasma than in breast milk. These results are expected knowing the lipophilicity of THC and CBD. THC was detectable up to 53 h after the last cannabis use. Finally, Sempio et al. found THC, 11-OH-THC, THC-COOH, CBD and CBG detectable concentrations in 36 samples collected at an unknown time after the last cannabis consumption (Sempio et al., 2020). Other cannabinoids were not detected in any of the samples.
Overall, cannabinoid concentrations in breast milk are consistent from one study to another. THC, 11-OH-THC, THC-COOH, CBD and CBG will transfer into breast milk; therefore, their pharmacokinetics should be assessed in this matrix for at least 24 h to assess neonates’ exposure to these cannabinoids.
2.2.3 Pharmacokinetics of Cannabinoids in Newborns
2.2.3.1 Pharmacokinetics of Cannabinoids in Meconium
Meconium is by far the most tested matrix to assess the exposure of newborns to cannabis, as it is easy to collect and non-invasive. Meconium samples are collected in diapers during the first 48 h after delivery, until the formation of a milk stool. Seventeen studies have measured cannabinoids in meconium (Moore et al., 1996; ElSohly and Feng, 1998; Feng et al., 2000; Boskovic et al., 2001; Ostrea et al., 2001; Vinner et al., 2003; Coles et al., 2005; Marchei et al., 2006; Montgomery et al., 2006; Gray et al., 2009; Gray et al., 2010a; Gray et al., 2010b; Tynon et al., 2015; Colby, 2017; Lamy et al., 2017; Palmer et al., 2017; Prego-Meleiro et al., 2017; Kim et al., 2018; Mantovani et al., 2018). All studies lacked information on the dose taken by the mother and only two studies reported the last trimester of cannabis use (Gray et al., 2009; Gray et al., 2010a). Cannabinoids were mainly quantifiable when the mother used cannabis during the third trimester. Gray et al. observed that cannabinoids were detectable in 7% of samples when the mother stopped her consumption in the first trimester, in 11% of samples when the mother stopped in the second trimester and in 51% of samples when the mother stopped during the third trimester (Gray et al., 2009; Gray et al., 2010a). No correlation was established between the presence of certain cannabinoids and the trimester at which cannabis use was stopped. However, according to Gray’s results, diOH-THC and 11-OH-THC were only detected when cannabis was last used during the third trimester, CBN was detected if the last use was during the second and third trimesters and THC-COOH was detected regardless of when it was used during pregnancy. (Gray et al., 2010a). THC-COOH concentrations appear to be lower when the mother has stopped using cannabis in the 1st trimester of pregnancy rather than in the 3rd trimester. Gray and Ostrea observed that maternal self-report was more sensitive than meconium in detecting neonatal exposure to cannabis (Gray et al., 2009; Gray et al., 2010b). However, in both studies, only THC-COOH was quantified in meconium, suggesting that other cannabinoids should be used to assess the newborn’s exposure to cannabis. Indeed, Marchei, Feng and Coles observed that THC, 11-OH-THC, and diOH-THC were present in meconium samples without THC-COOH, increasing the number of positive meconium samples (ElSohly and Feng, 1998; Feng et al., 2000; Coles et al., 2005; Marchei et al., 2006).
All these results suggest that pharmacokinetics studies including meconium samples should monitor at least THC, 11-OH-THC, THC-COOH, diOH-THC, CBD, 7-OH-CBD, 7-CBD-COOH, CBN and glucuronide cannabinoids and control for the type of consumption of the mother during pregnancy as well as the last trimester of cannabis use.
2.2.3.2 Pharmacokinetics of Cannabinoids in the Umbilical Cord and Cord Blood
The umbilical cord is the second most used matrix to assess cannabis exposure during pregnancy as it gives similar results than meconium (Montgomery et al., 2006; Colby, 2017; Kim et al., 2018; Wu et al., 2018). It has the advantages over meconium that 1- it can be tested immediately after delivery, 2- it is easier to collect and 3- it is always available. Colby and Montgomery observed 76% and 90.7% of agreement between umbilical cord and meconium cannabis concentrations suggesting that umbilical cord can be used as a replacement of meconium for assessing exposure during the third trimester (Montgomery et al., 2006; Colby, 2017). However, results are very scarce, and few cannabinoids have been tested in the umbilical cord. Further studies should be conducted for the same reasons as meconium. Cord blood can also be used at delivery to quantify THC and THC-COOH up to 26 h after smoking (Blackard and Tennes, 1984).
2.2.3.3 Pharmacokinetics of Cannabinoids in Neonatal Hair
Neonatal hair was tested by Boskovic, Vinner, Kintz and Bar-Oz (Boskovic et al., 2001; Bar-Oz et al., 2003; Vinner et al., 2003; Kintz, 2015). It can be used to assess the newborn exposure during the third trimester. Bar-Oz observed a sensitivity of 98% in meconium and 71% in neonatal hair for cannabinoids suggesting a better performance using meconium samples (Bar-Oz et al., 2003). Information is very scarce on the quantification of cannabinoids in neonatal hair. Therefore, it is not yet determined if the use of neonatal hair would be adequate to assess cannabis exposure during pregnancy.
2.2.3.4 Pharmacokinetics of Cannabinoids in Urine
Urine was tested once by Vinner, 48 h after delivery (Vinner et al., 2003). Urine samples can be used at delivery to assess cannabis consumption by the mother 1–8 days before delivery.
2.2.3.5 Pharmacokinetics of Cannabinoids in Newborns Exposed During Breastfeeding
Cannabinoids were only quantified once in child urine and feces after cannabis exposure during breastfeeding. Perez-Reyes measured THC, 11-OH-THC and THC-COOH concentrations in child feces while no cannabinoids were detected in child urine (Presence of Δ9, 1982). The results obtained in this study suggest that the THC present in breast milk was absorbed and metabolized by the infant because the proportion of 11-OH-THC and THC-COOH to the parent was much higher in the feces than in breast milk.
2.2.4 Summary of the Knowledge on the Cannabinoid's Pharmacokinetics in Pregnant and Lactating Women and Neonates
There is a lack of studies that assessed the exposure of women and neonates to cannabis during pregnancy and lactation. Yet, to understand the effect of cannabis on the newborn’s development, it is first important to assess their exposure during these two periods. Quantifying cannabinoids in pregnant women’s blood will first help to understand how the pharmacokinetic parameters such as the Cmax, the Tmax or the clearance are altered during pregnancy and then to assess the fetus exposure to cannabis. Infant blood would be the best matrix to assess the short-term exposure of neonates to cannabinoids during the last days of pregnancy or during lactation. Meconium and umbilical cord can be used as non-invasive matrices to assess the exposure during pregnancy, but meconium cannot be used for the first weeks of gestation and umbilical cord can only be used for the third trimester. For cannabis exposure during lactation, breast milk, urine and feces would be the best non-invasive indicator for short-term and long-term exposure to cannabis.
Based on available literature in humans we believe that THC, 11-OH-THC, THC-COOH, diOH-THC, THC-COOH glucuronide, CBD, 7-OH-CBD, 7-CBD-COOH, and CBN are of importance as they cross the placenta, can be found in breast milk or will be produced by the newborn’s metabolism and should be monitored to assess the exposure of fetuses and neonates to cannabis during pregnancy and lactation.
2.3 Pharmacokinetics of Cannabinoids in Animals
Preclinical studies on animals are used to assess the efficacy and toxicity of new drugs or formulations before the administration in humans. As all cannabis commercial formulations were first tested in preclinical studies, missing data on the pharmacokinetics of cannabinoids in humans may be available in animal models. Indeed, pharmacokinetics studies in pregnant and lactating women, in fetuses and in neonates are lacking; thus, animal data could be used to extrapolate the pharmacokinetics in these vulnerable populations. Therefore, it is important to assess which cannabinoids were tested, their concentration, their time to reach the maximal concentration (Tmax), their half-life (T1/2) and the sampling period for these types of population. Table 3 summarizes the pharmacokinetics parameters of cannabinoids in animals.
2.3.1 Pharmacokinetics of Cannabinoids in Non-Pregnant or Lactating Animals
Multiple preclinical models were used to assess the pharmacokinetics of cannabinoids: rats, mice, dogs, rhesus monkeys, guinea pigs, rabbits, and pigs (Pryor et al., 1977; Chiang et al., 1983; Perlin et al., 1985; Leuschner et al., 1986; Samara et al., 1988; Samara et al., 1990; Bornheim et al., 1995; Mannila et al., 2004; Valiveti and Stinchcomb, 2004; Brunet et al., 2006; Mannila et al., 2006; Valiveti et al., 2007; Paudel et al., 2010; Al-Ghananeem et al., 2011; Hasiwa et al., 2011; Deiana et al., 2012; Ginsburg et al., 2014; Zgair et al., 2015; Cherniakov et al., 2017b; Hlozek et al., 2017; Bartner et al., 2018; Ravula et al., 2018; Elmes et al., 2019; Fuchs et al., 2019; Xu et al., 2019; Łebkowska-Wieruszewska et al., 2019; Izgelov et al., 2020a; Izgelov et al., 2020b; Izgelov et al., 2020c; Fernández-Trapero et al., 2020; Itin et al., 2020; Torrens et al., 2020; Uziel et al., 2020; Michael, 2021). Pharmacokinetics parameters of THC, 11-OH-THC, THC-COOH, THCV, CBD, CBDV and CBG are presented in Table 3 after different modes of administration.
Torrens observed differences in the pharmacokinetics of THC, 11-OH-THC and THC-COOH between adolescent and adult mice suggesting an age-dependent pharmacokinetics of cannabinoids (Torrens et al., 2020). These results highlight the importance of studying the pharmacokinetics of cannabinoids in fetuses and newborns as their metabolism and their elimination could be significantly different. Also, variations in THC concentrations and Tmax after oral consumption were observed depending on the fed/fasting statute of dogs suggesting that this parameter should be controlled in pharmacokinetics studies to avoid interindividual variability (Fernández-Trapero et al., 2020). Finally, Perlin and Ginsburg concluded that the pharmacokinetics of THC in rhesus monkeys is similar to humans. Therefore, data in this animal, especially in the pregnant and lactating model, should be used in priority to predict the pharmacokinetics of cannabinoids in humans (Perlin et al., 1985; Ginsburg et al., 2014).
Overall, pharmacokinetics profiles of cannabinoids in animals are consistent with that of humans and could be used to predict the transfer of cannabinoids to fetuses and neonates. In all animal models, except guinea pigs, THC, CBD, 11-OH-THC and THC-COOH were detected in plasma for at least 24 h. Therefore, these four cannabinoids should be considered for pharmacokinetics studies in animals.
2.3.2 Pharmacokinetics of Cannabinoids in Pregnant and Lactating Animals
Fetal THC concentrations in rats seem to be around 10% of the maternal concentrations 60 min after the administration of THC by gastric gavage, according to Hutchings et al. (Hutchings et al., 1989). Ochiai et al. assessed the pharmacokinetics of CBD in maternal plasma, fetuses (whole body) and amniotic fluid of mice over a 24-hour period after an intravenous injection of 10 mg/kg of CBD (Ochiai et al., 2021). The authors observed that CBD transfer to the fetus (whole body) began 15 min after CBD administration at a rate of 66.9%. THC and THC-COOH were measured in maternal and fetal plasma of rhesus monkeys after the intravenous administration of 0.3 mg/kg of THC by Bailey et al. (Bailey et al., 1987). If the THC-COOH metabolite was not detected in appreciable levels in fetal plasma after 3 h, THC concentration was found to peak at 3 min in maternal plasma and 15 min in fetal plasma suggesting a rapid transfer to the placenta. Ratios of THC concentrations in fetal plasma to THC concentrations in maternal plasma can be calculated over 3 h based on the data obtained by Bailey et al. and are shown in Figure 2. Three hours after THC administration, maternal and fetal THC plasma were equal, supposing a slower elimination of THC from fetal plasma than from maternal plasma showing worrisome data when compared to the study in rats aforementioned (Hutchings et al., 1989). Abrams assessed the transfer of THC in fetuses of sheep after the inhalation of marijuana cigarettes containing 3.19% of THC (Abrams et al., 1985). THC was first detected in maternal plasma at 3 min and peaked at 10 min whereas THC was first detected in fetal plasma at 10 min and peaked at 90 min. THC concentrations were always lower in fetal plasma than in maternal plasma (0–24 h). Data on THC concentrations in fetuses from different animal models are consistent. THC rapidly crosses the placenta barrier with a lag time of 10–15 min and has a slow elimination from the fetal compartment during the 3 h following peak concentrations.
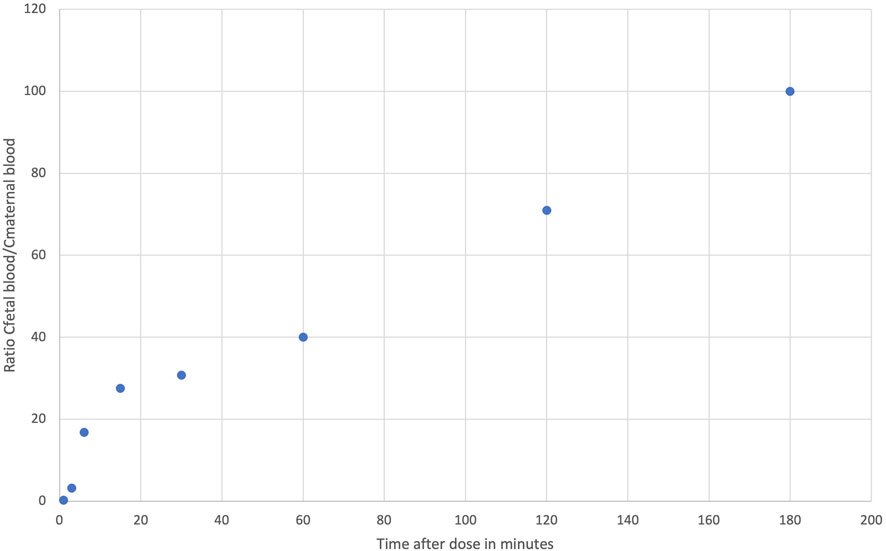
FIGURE 2. Ratios of fetal concentrations over maternal concentrations over 3 h in rhesus monkeys based on data obtained by Bailey et al. (1987).
THC and THC-COOH concentrations were also measured in breast milk of buffalos and squirrel monkeys (Chao et al., 1976; Ahmad and Ahmad, 1990). In buffalos, whose marijuana consumption accounts for approximately 5%–10% of their total vegetation consumption, THC-COOH was detectable in milk and urine specimens of both mother and offspring with concentrations in breast milk and offspring urine about 76 and 30% of maternal urine concentrations, respectively (Ahmad and Ahmad, 1990). Finally, Chao observed in breastfeeding infants of squirrel monkeys that 0.01% and 0.12% of the THC maternal dose were excreted in urine and feces, respectively, during the 18 h after breastfeeding (Chao et al., 1976). Moreover, approximately 0.2% of the labelled THC appeared in the milk. Limited interpretations can be made from these studies as neither the dose nor THC concentrations in breast milk over time are known. THC concentrations seems to be lower in breast milk than in maternal blood, and the exposition of offspring to cannabis appeared to be limited. No study measured THC concentrations in the blood of offspring.
3 Discussion
3.1 Lack of Knowledge on Cannabinoid's Pharmacokinetics
Preceding sections summarize research characterizing the exposure of animals and humans to cannabinoids. Extensive research has been published on the pharmacokinetics of THC and CBD in plasma of animals (rats and mice) and humans. However, only a handful of studies have looked at other cannabinoids of interests (CBD metabolites, THC-COOH glucuronide), either because of their activity or their long half-life, in vulnerable populations such as pregnant and lactating women and neonates. Table 4 summarizes the lack of data on the pharmacokinetics of cannabinoids identified by this review.
Data on the pharmacokinetics of cannabinoids during pregnancy and lactation are lacking. Recently the journal of Obstetrics and Gynecology has published guidelines on the use of cannabis during pregnancy and breastfeeding (Graves et al., 2022b). They concluded that more data are needed to guide clinical cares. Moreover, they pointed the urgency to make correlations between the pharmacokinetics and the pharmacodynamics of cannabis and to assess the pharmacokinetics and effects of CBD alone. The first step to understand the exposure of fetuses to cannabis during pregnancy will be to assess how the physiological changes during pregnancy will change the pharmacokinetics of cannabinoids in humans as it was never evaluated to date. Meconium has been the most tested matrix to assess the transfer of cannabinoids to fetuses. However, published studies using meconium samples were more qualitative than quantitative. Therefore, limited information can be obtained from meconium samples. Further studies should assess the ability of cannabinoids quantification in meconium to determine the last trimester of cannabis use and the frequency of consumption of women during pregnancy. Blood both in the umbilical cord and in the child will have the advantages of establishing the real exposure of the fetus to cannabis and to make correlation between the dose taken by the mother and this exposure. Future studies could use Dry Blood Spot (DBS) or Volumetric Absorptive Microsampling (VAMS) to collect infant blood as it is less invasive and more acceptable by parents (Lei and Prow, 2019). Finally, breast milk is the best matrix to assess the exposure of newborns to cannabis during breastfeeding. Unfortunately, data on the transfer of cannabinoids into breast milk is limited due to difficulties in recruiting lactating women using cannabis in clinical studies. New studies controlling the mode of administration of cannabis, the time of breast milk sampling and including CBD metabolites are critical.
In animals, THC, THC metabolites and CBD were well characterized in plasma of several models. As the popularity of CBD formulations increases, CBD metabolites should be included in animal studies that assess the safety of new CBD formulations. Other matrices than plasma have rarely been used in animal pharmacokinetics studies. Urine, feces, breast milk, amniotic fluid or even cord blood analysis could provide additional information on animal exposure to cannabinoids such as the elimination of cannabinoids, the length of exposure to cannabinoids and the transfer of cannabinoids to newborns during pregnancy and lactation. Indeed, only limited data is available on the exposure of newborns to cannabis during pregnancy and lactation. As cannabis is increasingly popular in these two populations, it is crucial to assess the exposure of newborns to cannabis during pregnancy and lactation and to assess the effects of cannabinoids on their development. As it is difficult to conduct this type of study in humans, animals could be a good alternative.
3.2 Prediction of Fetus and Neonate Exposure During Pregnancy and Lactation
As mentioned previously, data on cannabinoid pharmacokinetics in pregnant and lactating women and neonates are scarce. While waiting for robust controlled studies, it is first important to understand and interpret the data obtained in the general population and in animals. These data could also be used to estimate, by extrapolation, the exposure of pregnant and lactating women and newborns to cannabinoids. We based our extrapolations on the concentrations obtained by Huestis et al., in 1992 (Huestis et al., 1992b). They measured THC, 11-OH-THC and THC-COOH concentrations in plasma samples of 6 volunteers with a history of marijuana use after the inhalation of cannabis in a single 3.55% THC marijuana cigarette (Figure 3). As a reminder, THC and 11-OH-THC are psychoactive while THC-COOH is a non-active metabolite.
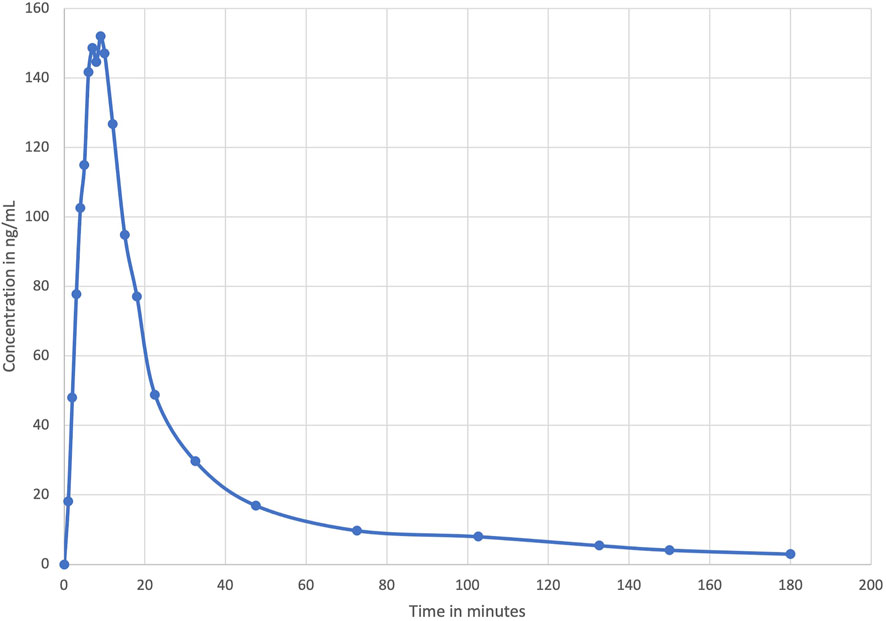
FIGURE 3. Mean plasma levels of THC during and after smoking a single 3.55% THC marijuana cigarette obtained by Huestis et al. (1992).
We previously stated that cannabinoids concentrations, volume of distribution and clearance can be altered during pregnancy. This alteration will be even more important as the pregnancy progresses. Grant et al. (2018) suggested that THC clearance could be increased by a factor of 2. Regarding the THC volume of distribution, no statement has been made, yet. However, studies that have assessed changes of this parameter for methadone, a drug with similar physicochemical properties as THC, observed no significant changes between pregnancy and post-partum period (Shiu and Ensom, 2012). Therefore, we decided to estimate cannabinoids concentrations in plasma of pregnant women considering the possibilities that either the THC volume of distribution and clearance are not affected by pregnancy or that the THC volume of distribution is unchanged, and clearance is affected by a factor of 2. The theoretical Figure 4 shows an area of calculated THC concentrations in pregnant women.
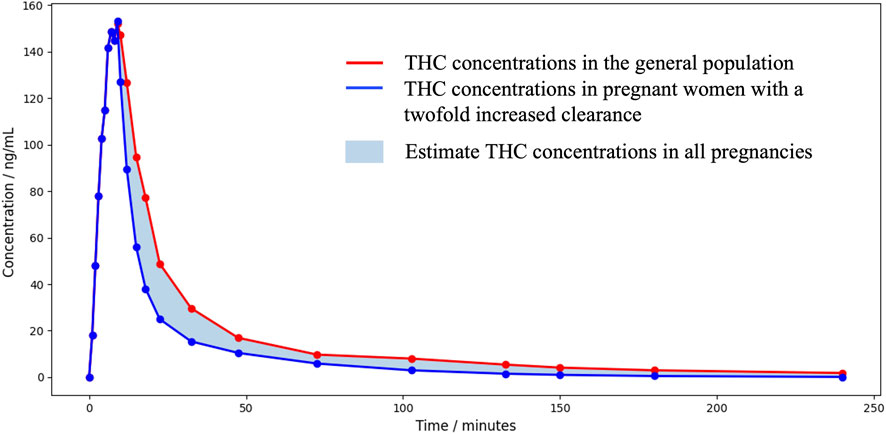
FIGURE 4. Area of theoretical THC concentrations in pregnant women over 3 h after a single 3.55% THC marijuana cigarette.
Based on this information, a pregnant woman could be exposed to similar or lower THC concentrations and similar or higher metabolites concentrations. The psychoactive effect might be similar or shorter. In fetuses, it is already well known that THC, CBD and CBN cross the placental barrier as they were detected in meconium. Metabolites concentrations measured in meconium could be the result of their passage or the transformation by the fetus metabolism. To predict the exposure of fetuses to THC, we multiplied the ratio of THC concentrations in rhesus monkey fetal plasma to THC concentrations in maternal plasma presented in Figure 2 by the calculated THC concentrations in pregnant women from Figure 4, as the pharmacokinetics of THC in rhesus monkeys was proven to be similar to that of humans. The theoretical Figure 5 represents the area of calculated THC concentrations in fetal plasma. Bailey et al. (1987) observed no appreciable THC-COOH concentrations in fetal plasma of rhesus monkeys when maternal THC-COOH concentrations were similar to that of Huestis et al. suggesting both that THC-COOH do not cross the placenta and that the monkey fetus does not metabolize THC at an appreciable level at this stage of development (around 90% of the pregnancy). These results suggest that human fetuses will be exposed to significant THC concentrations that will be eliminated much more slowly than in adults. Indeed, according to Figure 2, ratios of THC concentrations in rhesus monkey fetal plasma to THC concentrations in maternal plasma are increasing with time suggesting a slower elimination of THC by the fetus. This can be explained by the limited fetal elimination mechanisms (Morgan, 1997). Therefore, depending on the frequency of cannabis use by the mother, a fetus could be continually exposed to appreciable levels of THC during the pregnancy as it is taking several days for an adult to eliminate THC. Moreover, as a lipophilic drug, THC could easily transfer to the fetus brain and accumulate. THC-COOH was detected in human meconium and umbilical cord suggesting that this metabolite crosses the placental barrier and/or is produced by the metabolism of the fetus. However, THC-COOH is a non-active metabolite. Even though 11-OH-THC was never tested in pregnant animal models, it is unlikely that fetuses will be exposed to significant levels because of its higher polarity and its low levels in adults. Finally, it is possible that other active cannabinoids cross the placental barrier and the blood brain barrier and accumulate similarly to THC. Caution should be taken with these predictions as it is based on cannabinoid concentrations after a single dose of THC. Indeed, it is likely that after multiple cannabis doses, THC concentrations will be higher and eliminated more slowly.
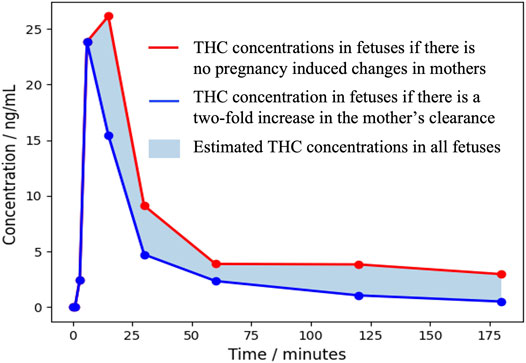
FIGURE 5. Area of theoretical THC concentrations in fetuses over 3 h after a single 3.55% THC marijuana cigarette.
From the studies published on LactMed regarding the transfer of cannabinoids in breast milk, we can conclude that THC, 11-OH-THC, THC-COOH, CBD and CBG are present in breast milk for at least 40 h (Baker et al., 2018; Bertrand et al., 2018; Moss et al., 2021; Wymore et al., 2021). THC could be detectable in breast milk for up to 6 days with higher concentrations than in plasma. The authors estimated that an infant would be exposed to a daily dose between 1.4 and 8 mcg/kg. Although these values appeared to be low, it was demonstrated by Perez-Reyes that THC from breast milk is absorbed and metabolized by the infant (Presence of Δ9, 1982). Indeed, the absorption of a drug like THC in neonates is likely to be higher than in adults due to the immaturity of the enzymatic systems. Moreover, it is possible that the quantity of THC absorbed by the neonates will be eliminated more slowly due to the low expression of CYP450 during the first months after delivery and will transfer to its brain due to its high lipophilicity (O'Hara et al., 2015).
As stated in the introduction, the use of cannabis during pregnancy and breastfeeding can induce negative birth outcomes such as reduced birth weight and increased risk of prematurity, behavioral and neurocognitive impairment, and cognitive deficits in children (Fried and Watkinson, 1990; Day et al., 1994; Goldschmidt et al., 2004; Smith et al., 2006; Smith et al., 2010; Hayatbakhsh et al., 2012; Conner et al., 2016; Gunn et al., 2016; Smith et al., 2016; Porath, 2018; Paul et al., 2021). Lately, Paul et al. observed in 11,489 children that prenatal exposure to cannabis was associated with a greater risk of psychopathologies than no exposure (Paul et al., 2021). Additionally, they found a higher risk of psychopathologies in children from mothers who continued their cannabis consumption throughout pregnancy suggesting that prolonged cannabis exposure has a direct impact on brain development.
4 Conclusion
Data on the pharmacokinetics of cannabinoids are still limited for many cannabinoids and especially for vulnerable populations such as pregnant and lactating women and neonates. With worrisome data on the exposure and the potential effects of cannabis on fetuses and neonates, scientific research should focus on filling this knowledge gap as it is a growing societal matter. Indeed, cannabis use during pregnancy and lactation has risen over the years, and with the recent legalization, it is more than ever important to assess cannabis transfer in the fetus and the breastfed child and to update official recommendations in that regard. This review will help to guide future clinical studies aimed at monitoring fetal and neonatal toxicity and assessing correlation between the pharmacokinetics and the pharmacodynamics of cannabis.
Author Contributions
AM, GLo, GLe, and EF contributed to conception and design of the study. AM created and organized the database. GLo, GLe, and EF revised the database. AM wrote the first draft of the manuscript. All authors contributed to manuscript revision, read, and approved the submitted version.
Funding
AM received funding from the Fonds de Recherche du Québec en Nature et Technologie (FRQNT).
Conflict of Interest
The authors declare that the research was conducted in the absence of any commercial or financial relationships that could be construed as a potential conflict of interest.
Publisher’s Note
All claims expressed in this article are solely those of the authors and do not necessarily represent those of their affiliated organizations, or those of the publisher, the editors and the reviewers. Any product that may be evaluated in this article, or claim that may be made by its manufacturer, is not guaranteed or endorsed by the publisher.
References
Abrams, D. I., Vizoso, H. P., Shade, S. B., Jay, C., Kelly, M. E., and Benowitz, N. L. (2007). Vaporization as a Smokeless Cannabis Delivery System: a Pilot Study. Clin. Pharmacol. Ther. 82 (5), 572–578. doi:10.1038/sj.clpt.6100200
Abrams, R. M., Cook, C. E., Davis, K. H., Niederreither, K., Jaeger, M. J., and Szeto, H. H. (1985). Plasma Delta-9-Tetrahydrocannabinol in Pregnant Sheep and Fetus after Inhalation of Smoke from a Marijuana Cigarette. Alcohol Drug Res. 6 (5), 361–369.
Ahmad, G. R., and Ahmad, N. (1990). Passive Consumption of Marijuana through Milk: A Low Level Chronic Exposure to delta-9-Tetrahydrocannabinol(THC). J. Toxicol. Clin. Toxicol. 28 (2), 255–260. doi:10.3109/15563659008993497
Ahmed, A. I., van den Elsen, G. A., Colbers, A., Kramers, C., Burger, D. M., van der Marck, M. A., et al. (2015). Safety, Pharmacodynamics, and Pharmacokinetics of Multiple Oral Doses of Delta-9-Tetrahydrocannabinol in Older Persons with Dementia. Psychopharmacol. Berl. 232 (14), 2587–2595. doi:10.1007/s00213-015-3889-y
Ahmed, A. I., van den Elsen, G. A., Colbers, A., van der Marck, M. A., Burger, D. M., Feuth, T. B., et al. (2014). Safety and Pharmacokinetics of Oral Delta-9-Tetrahydrocannabinol in Healthy Older Subjects: a Randomized Controlled Trial. Eur. Neuropsychopharmacol. 24 (9), 1475–1482. doi:10.1016/j.euroneuro.2014.06.007
Al-Ghananeem, A. M., Malkawi, A. H., and Crooks, P. A. (2011). Bioavailability of Δ⁹-tetrahydrocannabinol Following Intranasal Administration of a Mucoadhesive Gel Spray Delivery System in Conscious Rabbits. Drug Dev. Ind. Pharm. 37 (3), 329–334. doi:10.3109/03639045.2010.513009
Atsmon, J., Cherniakov, I., Izgelov, D., Hoffman, A., Domb, A. J., Deutsch, L., et al. (2018). PTL401, a New Formulation Based on Pro-nano Dispersion Technology, Improves Oral Cannabinoids Bioavailability in Healthy Volunteers. J. Pharm. Sci. 107 (5), 1423–1429. doi:10.1016/j.xphs.2017.12.020
Atsmon, J., Heffetz, D., Deutsch, L., Deutsch, F., and Sacks, H. (2018). Single-Dose Pharmacokinetics of Oral Cannabidiol Following Administration of PTL101: A New Formulation Based on Gelatin Matrix Pellets Technology. Clin. Pharmacol. Drug Dev. 7 (7), 751–758. doi:10.1002/cpdd.408
Bailey, J. R., Cunny, H. C., Paule, M. G., and Slikker, W. (1987). Fetal Disposition of Delta 9-tetrahydrocannabinol (THC) during Late Pregnancy in the Rhesus Monkey. Toxicol. Appl. Pharmacol. 90 (2), 315–321. doi:10.1016/0041-008x(87)90338-3
Baker, T., Datta, P., Rewers-Felkins, K., Thompson, H., Kallem, R. R., and Hale, T. W. (2018). Transfer of Inhaled Cannabis into Human Breast Milk. Obstet. Gynecol. 131 (5), 783–788. doi:10.1097/AOG.0000000000002575
Bar-Oz, B., Klein, J., Karaskov, T., and Koren, G. (2003). Comparison of Meconium and Neonatal Hair Analysis for Detection of Gestational Exposure to Drugs of Abuse. Arch. Dis. Child. Fetal Neonatal Ed. 88 (2), F98–F100. doi:10.1136/fn.88.2.f98
Bartner, L. R., McGrath, S., Rao, S., Hyatt, L. K., and Wittenburg, L. A. (2018). Pharmacokinetics of Cannabidiol Administered by 3 Delivery Methods at 2 Different Dosages to Healthy Dogs. Can. J. Vet. Res. 82 (3), 178–183.
Bertrand, K. A., Hanan, N. J., Honerkamp-Smith, G., Best, B. M., and Chambers, C. D. (2018). Marijuana Use by Breastfeeding Mothers and Cannabinoid Concentrations in Breast Milk. Pediatrics 142 (3). doi:10.1542/peds.2018-1076
Birnbaum, A. K., Karanam, A., Marino, S. E., Barkley, C. M., Remmel, R. P., Roslawski, M., et al. (2019). Food Effect on Pharmacokinetics of Cannabidiol Oral Capsules in Adult Patients with Refractory Epilepsy. Epilepsia 60 (8), 1586–1592. doi:10.1111/epi.16093
Blackard, C., and Tennes, K. (1984). Human Placental Transfer of Cannabinoids. N. Engl. J. Med. 311 (12), 797. doi:10.1056/NEJM198409203111213
Bornheim, L. M., Kim, K. Y., Li, J., Perotti, B. Y., and Benet, L. Z. (1995). Effect of Cannabidiol Pretreatment on the Kinetics of Tetrahydrocannabinol Metabolites in Mouse Brain. Drug Metab. Dispos. 23 (8), 825–831.
Boskovic, R., Klein, J., Woodland, C., Karaskov, T., and Koren, G. (2001). The Role of the Placenta in Variability of Fetal Exposure to Cocaine and Cannabinoids: a Twin Study. Can. J. Physiol. Pharmacol. 79 (11), 942–945. doi:10.1139/y01-080
Brown, Q. L., Sarvet, A. L., Shmulewitz, D., Martins, S. S., Wall, M. M., and Hasin, D. S. (2017). Trends in Marijuana Use Among Pregnant and Nonpregnant Reproductive-Aged Women, 2002-2014. JAMA 317 (2), 207–209. doi:10.1001/jama.2016.17383
Brunet, B., Doucet, C., Venisse, N., Hauet, T., Hébrard, W., Papet, Y., et al. (2006). Validation of Large White Pig as an Animal Model for the Study of Cannabinoids Metabolism: Application to the Study of THC Distribution in Tissues. Forensic Sci. Int. 161 (2-3), 169–174. doi:10.1016/j.forsciint.2006.04.018
Camí, J., Guerra, D., Ugena, B., Segura, J., and de la Torre, R. (1991). Effect of Subject Expectancy on the THC Intoxication and Disposition from Smoked Hashish Cigarettes. Pharmacol. Biochem. Behav. 40 (1), 115–119. doi:10.1016/0091-3057(91)90330-5
Celada, P., Puig, M., Amargós-Bosch, M., Adell, A., and Artigas, F. (2004). The Therapeutic Role of 5-HT1A and 5-HT2A Receptors in Depression. J. Psychiatry Neurosci. 29 (4), 252–265.
Centers for Disease Control and Prevention (2017). Marijuana Fast Facts and Fact Sheets. Available from: https://www.cdc.gov/marijuana/fact-sheets.htm.
Chao, F. C., Green, D. E., Forrest, I. S., Kaplan, J. N., Winship-Ball, A., and Braude, M. (1976). The Passage of 14C-Delta-9-Tetrahydrocannabinol into the Milk of Lactating Squirrel Monkeys. Res. Commun. Chem. Pathol. Pharmacol. 15 (2), 303–317.
Cherniakov, I., Izgelov, D., Barasch, D., Davidson, E., Domb, A. J., and Hoffman, A. (2017). Piperine-pro-nanolipospheres as a Novel Oral Delivery System of Cannabinoids: Pharmacokinetic Evaluation in Healthy Volunteers in Comparison to Buccal Spray Administration. J. Control Release 266, 1–7. doi:10.1016/j.jconrel.2017.09.011
Cherniakov, I., Izgelov, D., Domb, A. J., and Hoffman, A. (2017). The Effect of Pro NanoLipospheres (PNL) Formulation Containing Natural Absorption Enhancers on the Oral Bioavailability of Delta-9-Tetrahydrocannabinol (THC) and Cannabidiol (CBD) in a Rat Model. Eur. J. Pharm. Sci. 109, 21–30. doi:10.1016/j.ejps.2017.07.003
Chiang, C. W., Barnett, G., and Brine, D. (1983). Systemic Absorption of Delta 9-tetrahydrocannabinol after Ophthalmic Administration to the Rabbit. J. Pharm. Sci. 72 (2), 136–138. doi:10.1002/jps.2600720210
Colby, J. M. (2017). Comparison of Umbilical Cord Tissue and Meconium for the Confirmation of In Utero Drug Exposure. Clin. Biochem. 50 (13-14), 784–790. doi:10.1016/j.clinbiochem.2017.03.006
Coles, R., Clements, T. T., Nelson, G. J., McMillin, G. A., and Urry, F. M. (2005). Simultaneous Analysis of the Delta9-THC Metabolites 11-nor-9-carboxy-Delta9-THC and 11-hydroxy-Delta9-THC in Meconium by GC-MS. J. Anal. Toxicol. 29 (6), 522–527. doi:10.1093/jat/29.6.522
Conner, S. N., Bedell, V., Lipsey, K., Macones, G. A., Cahill, A. G., and Tuuli, M. G. (2016). Maternal Marijuana Use and Adverse Neonatal Outcomes: A Systematic Review and Meta-Analysis. Obstet. Gynecol. 128 (4), 713–723. doi:10.1097/AOG.0000000000001649
Corsi, D. J., Hsu, H., Weiss, D., Fell, D. B., and Walker, M. (2019). Trends and Correlates of Cannabis Use in Pregnancy: a Population-Based Study in Ontario, Canada from 2012 to 2017. Can. J. Public Health 110 (1), 76–84. doi:10.17269/s41997-018-0148-0
Crockett, J., Critchley, D., Tayo, B., Berwaerts, J., and Morrison, G. (2020). A Phase 1, Randomized, Pharmacokinetic Trial of the Effect of Different Meal Compositions, Whole Milk, and Alcohol on Cannabidiol Exposure and Safety in Healthy Subjects. Epilepsia 61 (2), 267–277. doi:10.1111/epi.16419
Crume, T. L., Juhl, A. L., Brooks-Russell, A., Hall, K. E., Wymore, E., and Borgelt, L. M. (2018). Cannabis Use during the Perinatal Period in a State with Legalized Recreational and Medical Marijuana: The Association between Maternal Characteristics, Breastfeeding Patterns, and Neonatal Outcomes. J. Pediatr. 197, 90–96. doi:10.1016/j.jpeds.2018.02.005
Dai, H., and Richter, K. P. (2019). A National Survey of Marijuana Use Among US Adults with Medical Conditions, 2016-2017. JAMA Netw. Open 2 (9), e1911936. doi:10.1001/jamanetworkopen.2019.11936
Day, N. L., Richardson, G. A., Goldschmidt, L., Robles, N., Taylor, P. M., Stoffer, D. S., et al. (1994). Effect of Prenatal Marijuana Exposure on the Cognitive Development of Offspring at Age Three. Neurotoxicol Teratol. 16 (2), 169–175. doi:10.1016/0892-0362(94)90114-7
de Vries, M., Van Rijckevorsel, D. C., Vissers, K. C., Wilder-Smith, O. H., and Van Goor, H. (2016). Single Dose Delta-9-Tetrahydrocannabinol in Chronic Pancreatitis Patients: Analgesic Efficacy, Pharmacokinetics and Tolerability. Br. J. Clin. Pharmacol. 81 (3), 525–537. doi:10.1111/bcp.12811
de Vries, M., van Rijckevorsel, D. C. M., Vissers, K. C. P., Wilder-Smith, O. H. G., and van Goor, H. Pain and Nociception Neuroscience Research Group (2017). Tetrahydrocannabinol Does Not Reduce Pain in Patients with Chronic Abdominal Pain in a Phase 2 Placebo-Controlled Study. Clin. Gastroenterol. Hepatol. 15 (7), 1079–e4. doi:10.1016/j.cgh.2016.09.147
Deiana, S., Watanabe, A., Yamasaki, Y., Amada, N., Arthur, M., Fleming, S., et al. (2012). Plasma and Brain Pharmacokinetic Profile of Cannabidiol (CBD), Cannabidivarine (CBDV), Δ⁹-tetrahydrocannabivarin (THCV) and Cannabigerol (CBG) in Rats and Mice Following Oral and Intraperitoneal Administration and CBD Action on Obsessive-Compulsive Behaviour. Psychopharmacol. Berl. 219 (3), 859–873. doi:10.1007/s00213-011-2415-0
Desrosiers, N. A., Himes, S. K., Scheidweiler, K. B., Concheiro-Guisan, M., Gorelick, D. A., and Huestis, M. A. (2014). Phase I and II Cannabinoid Disposition in Blood and Plasma of Occasional and Frequent Smokers Following Controlled Smoked Cannabis. Clin. Chem. 60 (4), 631–643. doi:10.1373/clinchem.2013.216507
Desrosiers, N. A., Lee, D., Concheiro-Guisan, M., Scheidweiler, K. B., Gorelick, D. A., and Huestis, M. A. (2014). Urinary Cannabinoid Disposition in Occasional and Frequent Smokers: Is THC-Glucuronide in Sequential Urine Samples a Marker of Recent Use in Frequent Smokers? Clin. Chem. 60 (2), 361–372. doi:10.1373/clinchem.2013.214106
Dinis-Oliveira, R. J. (2016). Metabolomics of Δ9-tetrahydrocannabinol: Implications in Toxicity. Drug Metab. Rev. 48 (1), 80–87. doi:10.3109/03602532.2015.1137307
Gourvernement du Québec (2021). Cannabis. Available from: https://encadrementcannabis.gouv.qc.ca/en/le-cannabis/.
Eichler, M., Spinedi, L., Unfer-Grauwiler, S., Bodmer, M., Surber, C., Luedi, M., et al. (2012). Heat Exposure of Cannabis Sativa Extracts Affects the Pharmacokinetic and Metabolic Profile in Healthy Male Subjects. Planta Med. 78 (7), 686–691. doi:10.1055/s-0031-1298334
Eisenberg, E., Ogintz, M., and Almog, S. (2014). The Pharmacokinetics, Efficacy, Safety, and Ease of Use of a Novel Portable Metered-Dose Cannabis Inhaler in Patients with Chronic Neuropathic Pain: a Phase 1a Study. J. Pain Palliat. Care Pharmacother. 28 (3), 216–225. doi:10.3109/15360288.2014.941130
Elmes, M. W., Prentis, L. E., McGoldrick, L. L., Giuliano, C. J., Sweeney, J. M., Joseph, O. M., et al. (2019). FABP1 Controls Hepatic Transport and Biotransformation of Δ9-THC. Sci. Rep. 9 (1), 7588. doi:10.1038/s41598-019-44108-3
ElSohly, M. A., and Feng, S. (1998). Delta 9-THC Metabolites in Meconium: Identification of 11-OH-delta 9-THC, 8 Beta,11-diOH-Delta 9-THC, and 11-Nor-Delta 9-THC-9-COOH as Major Metabolites of Delta 9-THC. J. Anal. Toxicol. 22 (4), 329–335. doi:10.1093/jat/22.4.329
European Monitoring Centre for Drugs and Drug Addiction (2022). Statistical Bulletin 2022-Prevalence of Drug Use. Available from: https://www.emcdda.europa.eu/data/stats2022/gps_en.
Fabritius, M., Chtioui, H., Battistella, G., Annoni, J. M., Dao, K., Favrat, B., et al. (2013). Comparison of Cannabinoid Concentrations in Oral Fluid and Whole Blood between Occasional and Regular Cannabis Smokers Prior to and after Smoking a Cannabis Joint. Anal. Bioanal. Chem. 405 (30), 9791–9803. doi:10.1007/s00216-013-7412-1
Feng, S., ElSohly, M. A., Salamone, S., and Salem, M. Y. (2000). Simultaneous Analysis of Delta9-THC and its Major Metabolites in Urine, Plasma, and Meconium by GC-MS Using an Immunoaffinity Extraction Procedure. J. Anal. Toxicol. 24 (6), 395–402. doi:10.1093/jat/24.6.395
Fernández-Trapero, M., Pérez-Díaz, C., Espejo-Porras, F., de Lago, E., and Fernández-Ruiz, J. (2020). Pharmacokinetics of Sativex® in Dogs: Towards a Potential Cannabinoid-Based Therapy for Canine Disorders. Biomolecules 10 (2). doi:10.3390/biom10020279
Fried, P. A., and Watkinson, B. (1990). 36- and 48-month Neurobehavioral Follow-Up of Children Prenatally Exposed to Marijuana, Cigarettes, and Alcohol. J. Dev. Behav. Pediatr. 11 (2), 49–58. doi:10.1097/00004703-199004000-00003
Fuchs, N., Miljanić, A., Katić, A., Brajenović, N., Micek, V., Fuchs, R., et al. (2019). Optimisation of a Gas Chromatography-Mass Spectrometry Method for the Simultaneous Determination of Tetrahydrocannabinol and its Metabolites in Rat Urine. Arh. Hig. Rada Toksikol. 70 (4), 325–331. doi:10.2478/aiht-2019-70-3352
Gambelunghe, C., Fucci, N., Aroni, K., Bacci, M., Marcelli, A., and Rossi, R. (2016). Cannabis Use Surveillance by Sweat Analysis. Ther. Drug Monit. 38 (5), 634–639. doi:10.1097/FTD.0000000000000327
Ginsburg, B. C., Hruba, L., Zaki, A., Javors, M. A., and McMahon, L. R. (2014). Blood Levels Do Not Predict Behavioral or Physiological Effects of Δ⁹-tetrahydrocannabinol in Rhesus Monkeys with Different Patterns of Exposure. Drug Alcohol Depend. 139, 1–8. doi:10.1016/j.drugalcdep.2014.02.696
Goldschmidt, L., Richardson, G. A., Cornelius, M. D., and Day, N. L. (2004). Prenatal Marijuana and Alcohol Exposure and Academic Achievement at Age 10. Neurotoxicol Teratol. 26 (4), 521–532. doi:10.1016/j.ntt.2004.04.003
Goodwin, R. S., Gustafson, R. A., Barnes, A., Nebro, W., Moolchan, E. T., and Huestis, M. A. (2006). Delta(9)-tetrahydrocannabinol, 11-Hydroxy-Delta(9)-Tetrahydrocannabinol and 11-Nor-9-Carboxy-Delta(9)-Tetrahydrocannabinol in Human Plasma after Controlled Oral Administration of Cannabinoids. Ther. Drug Monit. 28 (4), 545–551. doi:10.1097/00007691-200608000-00010
Grant, K. S., Petroff, R., Isoherranen, N., Stella, N., and Burbacher, T. M. (2018). Cannabis Use during Pregnancy: Pharmacokinetics and Effects on Child Development. Pharmacol. Ther. 182, 133–151. doi:10.1016/j.pharmthera.2017.08.014
Graves, L. E., Robert, M., Allen, V. M., Dama, S., Gabrys, R. L., Tanguay, R. L., et al. (2022). Guideline No. 425b: Cannabis Use throughout Women’s Lifespans – Part 2: Pregnancy, the Postnatal Period, and Breastfeeding. J. Obstetrics Gynaecol. Can. 44 (4), 436–444.e1.
Graves, L. E., Robert, M., Allen, V. M., Dama, S., Gabrys, R. L., Tanguay, R. L., et al. (2022). Guideline No. 425b: Cannabis Use throughout Women's Lifespans - Part 2: Pregnancy, the Postnatal Period, and Breastfeeding. J. Obstet. Gynaecol. Can. 44 (4), 436–e1. doi:10.1016/j.jogc.2022.01.013
Gray, T. R., Barnes, A. J., and Huestis, M. A. (2010). Effect of Hydrolysis on Identifying Prenatal Cannabis Exposure. Anal. Bioanal. Chem. 397 (6), 2335–2347. doi:10.1007/s00216-010-3772-y
Gray, T. R., Eiden, R. D., Leonard, K. E., Connors, G. J., Shisler, S., and Huestis, M. A. (2010). Identifying Prenatal Cannabis Exposure and Effects of Concurrent Tobacco Exposure on Neonatal Growth. Clin. Chem. 56 (9), 1442–1450. doi:10.1373/clinchem.2010.147876
Gray, T. R., LaGasse, L. L., Smith, L. M., Derauf, C., Grant, P., Shah, R., et al. (2009). Identification of Prenatal Amphetamines Exposure by Maternal Interview and Meconium Toxicology in the Infant Development, Environment and Lifestyle (IDEAL) Study. Ther. Drug Monit. 31 (6), 769–775. doi:10.1097/FTD.0b013e3181bb438e
Grotenhermen, F. (2003). Pharmacokinetics and Pharmacodynamics of Cannabinoids. Clin. Pharmacokinet. 42 (4), 327–360. doi:10.2165/00003088-200342040-00003
Gunn, J. K., Rosales, C. B., Center, K. E., Nuñez, A., Gibson, S. J., Christ, C., et al. (2016). Prenatal Exposure to Cannabis and Maternal and Child Health Outcomes: a Systematic Review and Meta-Analysis. BMJ Open 6 (4), e009986. doi:10.1136/bmjopen-2015-009986
Hartley, S., Simon, N., Larabi, A., Vaugier, I., Barbot, F., Quera-Salva, M. A., et al. (2019). Effect of Smoked Cannabis on Vigilance and Accident Risk Using Simulated Driving in Occasional and Chronic Users and the Pharmacokinetic-Pharmacodynamic Relationship. Clin. Chem. 65 (5), 684–693. doi:10.1373/clinchem.2018.299727
Hartman, R. L., Brown, T. L., Milavetz, G., Spurgin, A., Gorelick, D. A., Gaffney, G., et al. (2015). Controlled Cannabis Vaporizer Administration: Blood and Plasma Cannabinoids with and without Alcohol. Clin. Chem. 61 (6), 850–869. doi:10.1373/clinchem.2015.238287
Hasiwa, N., Bailey, J., Clausing, P., Daneshian, M., Eileraas, M., Farkas, S., et al. (2011). Critical Evaluation of the Use of Dogs in Biomedical Research and Testing in Europe. ALTEX 28 (4), 326–340. doi:10.14573/altex.2011.4.326
Hayatbakhsh, M. R., Flenady, V. J., Gibbons, K. S., Kingsbury, A. M., Hurrion, E., Mamun, A. A., et al. (2012). Birth Outcomes Associated with Cannabis Use before and during Pregnancy. Pediatr. Res. 71 (2), 215–219. doi:10.1038/pr.2011.25
Health Canada (2018). in Information for Health Care Professionals: Cannabis (Marihuana, Marijuana) and the Cannabinoids. Editor C. La. R. Branch.
Hlozek, T., Uttl, L., Kaderabek, L., Balikova, M., Lhotkova, E., Horsley, R. R., et al. (2017). Pharmacokinetic and Behavioural Profile of THC, CBD, and THC+CBD Combination after Pulmonary, Oral, and Subcutaneous Administration in Rats and Confirmation of Conversion In Vivo of CBD to THC. Eur. Neuropsychopharmacol. 27 (12), 1223–1237.
Hobbs, J. M., Vazquez, A. R., Remijan, N. D., Trotter, R. E., McMillan, T. V., Freedman, K. E., et al. (2020). Evaluation of Pharmacokinetics and Acute Anti-inflammatory Potential of Two Oral Cannabidiol Preparations in Healthy Adults. Phytother. Res. 34 (7), 1696–1703. doi:10.1002/ptr.6651
Huestis, M. A., and Cone, E. J. (2004). Relationship of Delta 9-tetrahydrocannabinol Concentrations in Oral Fluid and Plasma after Controlled Administration of Smoked Cannabis. J. Anal. Toxicol. 28 (6), 394–399. doi:10.1093/jat/28.6.394
Huestis, M. A., and Cone, E. J. (1998). Urinary Excretion Half-Life of 11-Nor-9-Carboxy-Delta9-Tetrahydrocannabinol in Humans. Ther. Drug Monit. 20 (5), 570–576. doi:10.1097/00007691-199810000-00021
Huestis, M. A., Henningfield, J. E., and Cone, E. J. (1992). Blood Cannabinoids. I. Absorption of THC and Formation of 11-OH-THC and THCCOOH during and after Smoking Marijuana. J. Anal. Toxicol. 16 (5), 276–282. doi:10.1093/jat/16.5.276
Huestis, M. A. (2007). Human Cannabinoid Pharmacokinetics. Chem. Biodivers. 4 (8), 1770–1804. doi:10.1002/cbdv.200790152
Huestis, M. A., Sampson, A. H., Holicky, B. J., Henningfield, J. E., and Cone, E. J. (1992). Characterization of the Absorption Phase of Marijuana Smoking. Clin. Pharmacol. Ther. 52 (1), 31–41. doi:10.1038/clpt.1992.100
Huestis, M. A., Scheidweiler, K. B., Saito, T., Fortner, N., Abraham, T., Gustafson, R. A., et al. (2008). Excretion of Delta9-tetrahydrocannabinol in Sweat. Forensic Sci. Int. 174 (2-3), 173–177. doi:10.1016/j.forsciint.2007.04.002
Hunault, C. C., Mensinga, T. T., de Vries, I., Kelholt-Dijkman, H. H., Hoek, J., Kruidenier, M., et al. (2008). Delta-9-tetrahydrocannabinol (THC) Serum Concentrations and Pharmacological Effects in Males after Smoking a Combination of Tobacco and Cannabis Containing up to 69 Mg THC. Psychopharmacol. Berl. 201 (2), 171–181. doi:10.1007/s00213-008-1260-2
Hutchings, D. E., Martin, B. R., Gamagaris, Z., Miller, N., and Fico, T. (1989). Plasma Concentrations of Delta-9-Tetrahydrocannabinol in Dams and Fetuses Following Acute or Multiple Prenatal Dosing in Rats. Life Sci. 44 (11), 697–701. doi:10.1016/0024-3205(89)90380-9
Itin, C., Barasch, D., Domb, A. J., and Hoffman, A. (2020). Prolonged Oral Transmucosal Delivery of Highly Lipophilic Drug Cannabidiol. Int. J. Pharm. 581, 119276. doi:10.1016/j.ijpharm.2020.119276
Izgelov, D., Domb, A. J., and Hoffman, A. (2020). The Effect of Piperine on Oral Absorption of Cannabidiol Following Acute vs. Chronic Administration. Eur. J. Pharm. Sci. 148, 105313. doi:10.1016/j.ejps.2020.105313
Izgelov, D., Freidman, M., and Hoffman, A. (2020). Investigation of Cannabidiol Gastro Retentive Tablets Based on Regional Absorption of Cannabinoids in Rats. Eur. J. Pharm. Biopharm. 152, 229–235. doi:10.1016/j.ejpb.2020.05.010
Izgelov, D., Shmoeli, E., Domb, A. J., and Hoffman, A. (2020). The Effect of Medium Chain and Long Chain Triglycerides Incorporated in Self-Nano Emulsifying Drug Delivery Systems on Oral Absorption of Cannabinoids in Rats. Int. J. Pharm. 580, 119201. doi:10.1016/j.ijpharm.2020.119201
Karschner, E. L., Darwin, W. D., Goodwin, R. S., Wright, S., and Huestis, M. A. (2011). Plasma Cannabinoid Pharmacokinetics Following Controlled Oral Delta9-Tetrahydrocannabinol and Oromucosal Cannabis Extract Administration. Clin. Chem. 57 (1), 66–75. doi:10.1373/clinchem.2010.152439
Karschner, E. L., Schwope, D. M., Schwilke, E. W., Goodwin, R. S., Kelly, D. L., Gorelick, D. A., et al. (2012). Predictive Model Accuracy in Estimating Last Δ9-tetrahydrocannabinol (THC) Intake from Plasma and Whole Blood Cannabinoid Concentrations in Chronic, Daily Cannabis Smokers Administered Subchronic Oral THC. Drug Alcohol Depend. 125 (3), 313–319. doi:10.1016/j.drugalcdep.2012.03.005
Kauert, G. F., Ramaekers, J. G., Schneider, E., Moeller, M. R., and Toennes, S. W. (2007). Pharmacokinetic Properties of Delta9-Tetrahydrocannabinol in Serum and Oral Fluid. J. Anal. Toxicol. 31 (5), 288–293. doi:10.1093/jat/31.5.288
Kelly, P., and Jones, R. T. (1992). Metabolism of Tetrahydrocannabinol in Frequent and Infrequent Marijuana Users. J. Anal. Toxicol. 16 (4), 228–235. doi:10.1093/jat/16.4.228
Kim, J., de Castro, A., Lendoiro, E., Cruz-Landeira, A., López-Rivadulla, M., and Concheiro, M. (2018). Detection of In Utero Cannabis Exposure by Umbilical Cord Analysis. Drug Test. Anal. 10 (4), 636–643. doi:10.1002/dta.2307
Kintz, P., Cirimele, V., and Ludes, B. (2000). Detection of Cannabis in Oral Fluid (Saliva) and Forehead Wipes (Sweat) from Impaired Drivers. J. Anal. Toxicol. 24 (7), 557–561. doi:10.1093/jat/24.7.557
Kintz, P. (2015). Contribution of In Utero Drug Exposure when Interpreting Hair Results in Young Children. Forensic Sci. Int. 249, 314–317. doi:10.1016/j.forsciint.2014.09.014
Klumpers, L. E., Beumer, T. L., van Hasselt, J. G., Lipplaa, A., Karger, L. B., Kleinloog, H. D., et al. (2012). Novel Δ(9) -tetrahydrocannabinol Formulation Namisol® Has Beneficial Pharmacokinetics and Promising Pharmacodynamic Effects. Br. J. Clin. Pharmacol. 74 (1), 42–53. doi:10.1111/j.1365-2125.2012.04164.x
Knaub, K., Sartorius, T., Dharsono, T., Wacker, R., Wilhelm, M., and Schön, C. (2019). A Novel Self-Emulsifying Drug Delivery System (SEDDS) Based on VESIsorb® Formulation Technology Improving the Oral Bioavailability of Cannabidiol in Healthy Subjects. Molecules 24 (16). doi:10.3390/molecules24162967
Lamy, S., Hennart, B., Houivet, E., Dulaurent, S., Delavenne, H., Benichou, J., et al. (2017). Assessment of Tobacco, Alcohol and Cannabinoid Metabolites in 645 Meconium Samples of Newborns Compared to Maternal Self-Reports. J. Psychiatr. Res. 90, 86–93. doi:10.1016/j.jpsychires.2017.02.012
Łebkowska-Wieruszewska, B., Stefanelli, F., Chericoni, S., Owen, H., Poapolathep, A., Lisowski, A., et al. (2019). Pharmacokinetics of Bedrocan®, a Cannabis Oil Extract, in Fasting and Fed Dogs: An Explorative Study. Res. Vet. Sci. 123, 26–28. doi:10.1016/j.rvsc.2018.12.003
Lee, D., Bergamaschi, M. M., Milman, G., Barnes, A. J., Queiroz, R. H., Vandrey, R., et al. (2015). Plasma Cannabinoid Pharmacokinetics after Controlled Smoking and Ad Libitum Cannabis Smoking in Chronic Frequent Users. J. Anal. Toxicol. 39 (8), 580–587. doi:10.1093/jat/bkv082
Lee, D., Schwope, D. M., Milman, G., Barnes, A. J., Gorelick, D. A., and Huestis, M. A. (2012). Cannabinoid Disposition in Oral Fluid after Controlled Smoked Cannabis. Clin. Chem. 58 (4), 748–756. doi:10.1373/clinchem.2011.177881
Lei, B. U. W., and Prow, T. W. (2019). A Review of Microsampling Techniques and Their Social Impact. Biomed. Microdevices 21 (4), 81. doi:10.1007/s10544-019-0412-y
Leuschner, J. T., Harvey, D. J., Bullingham, R. E., and Paton, W. D. (1986). Pharmacokinetics of Delta 9-tetrahydrocannabinol in Rabbits Following Single or Multiple Intravenous Doses. Drug Metab. Dispos. 14 (2), 230–238.
Lile, J. A., Kelly, T. H., Charnigo, R. J., Stinchcomb, A. L., and Hays, L. R. (2013). Pharmacokinetic and Pharmacodynamic Profile of Supratherapeutic Oral Doses of Δ(9) -THC in Cannabis Users. J. Clin. Pharmacol. 53 (7), 680–690. doi:10.1002/jcph.90
Lindgren, J. E., Ohlsson, A., Agurell, S., Hollister, L., and Gillespie, H. (1981). Clinical Effects and Plasma Levels of Delta 9-tetrahydrocannabinol (Delta 9-THC) in Heavy and Light Users of Cannabis. Psychopharmacol. Berl. 74 (3), 208–212. doi:10.1007/BF00427095
Manini, A. F., Yiannoulos, G., Bergamaschi, M. M., Hernandez, S., Olmedo, R., Barnes, A. J., et al. (2015). Safety and Pharmacokinetics of Oral Cannabidiol when Administered Concomitantly with Intravenous Fentanyl in Humans. J. Addict. Med. 9 (3), 204–210. doi:10.1097/ADM.0000000000000118
Mannekote Thippaiah, S., Iyengar, S. S., and Vinod, K. Y. (2021). Exo- and Endo-Cannabinoids in Depressive and Suicidal Behaviors. Front. Psychiatry 12, 636228. doi:10.3389/fpsyt.2021.636228
Mannila, J., Järvinen, T., Järvinen, K., Tervonen, J., and Jarho, P. (2006). Sublingual Administration of Delta9-tetrahydrocannabinol/beta-cyclodextrin Complex Increases the Bioavailability of Delta9-tetrahydrocannabinol in Rabbits. Life Sci. 78 (17), 1911–1914. doi:10.1016/j.lfs.2005.08.025
Mannila, J., Lehtonen, M., Järvinen, T., and Jarho, P. (2004). Determination of Delta9-tetrahydrocannabinol from Rabbit Plasma by Gas Chromatography-Mass Spectrometry Using Two Ionization Techniques. J. Chromatogr. B Anal. Technol. Biomed. Life Sci. 810 (2), 283–290. doi:10.1016/j.jchromb.2004.08.014
Manno, J. E., Manno, B. R., Kemp, P. M., Alford, D. D., Abukhalaf, I. K., McWilliams, M. E., et al. (2001). Temporal Indication of Marijuana Use Can Be Estimated from Plasma and Urine Concentrations of Delta9-Tetrahydrocannabinol, 11-Hydroxy-Delta9-Tetrahydrocannabinol, and 11-Nor-Delta9-Tetrahydrocannabinol-9-Carboxylic Acid. J. Anal. Toxicol. 25 (7), 538–549. doi:10.1093/jat/25.7.538
Mantovani, C. C., Silva, J. P. E., Forster, G., Almeida, R. M., Diniz, E. M. A., and Yonamine, M. (2018). Simultaneous Accelerated Solvent Extraction and Hydrolysis of 11-Nor-Δ9-Tetrahydrocannabinol-9-Carboxylic Acid Glucuronide in Meconium Samples for Gas Chromatography-Mass Spectrometry Analysis. J. Chromatogr. B Anal. Technol. Biomed. Life Sci. 1074-1075, 1–7. doi:10.1016/j.jchromb.2018.01.009
Marchei, E., Escuder, D., Pallas, C. R., Garcia-Algar, O., Gómez, A., Friguls, B., et al. (2011). Simultaneous Analysis of Frequently Used Licit and Illicit Psychoactive Drugs in Breast Milk by Liquid Chromatography Tandem Mass Spectrometry. J. Pharm. Biomed. Anal. 55 (2), 309–316. doi:10.1016/j.jpba.2011.01.028
Marchei, E., Pellegrini, M., Pacifici, R., Palmi, I., Lozano, J., García-Algar, O., et al. (2006). Quantification of Delta9-tetrahydrocannabinol and its Major Metabolites in Meconium by Gas Chromatographic-Mass Spectrometric Assay: Assay Validation and Preliminary Results of the "meconium Project". Ther. Drug Monit. 28 (5), 700–706. doi:10.1097/01.ftd.0000245380.95186.13
Marsot, A., Audebert, C., Attolini, L., Lacarelle, B., Micallef, J., and Blin, O. (2016). Comparison of Cannabinoid Concentrations in Plasma, Oral Fluid and Urine in Occasional Cannabis Smokers after Smoking Cannabis Cigarette. J. Pharm. Pharm. Sci. 19 (3), 411–422. doi:10.18433/J3F31D
Michael, J. (2021). Huerkamp. Animals Used in Research: MSD MANUAL Veterinary Manual. Available from: https://www.msdvetmanual.com/exotic-and-laboratory-animals/laboratory-animals/animals-used-in-research.
Milman, G., Barnes, A. J., Schwope, D. M., Schwilke, E. W., Darwin, W. D., Goodwin, R. S., et al. (2010). Disposition of Cannabinoids in Oral Fluid after Controlled Around-The-Clock Oral THC Administration. Clin. Chem. 56 (8), 1261–1269. doi:10.1373/clinchem.2009.141853
Milman, G., Bergamaschi, M. M., Lee, D., Mendu, D. R., Barnes, A. J., Vandrey, R., et al. (2014). Plasma Cannabinoid Concentrations during Dronabinol Pharmacotherapy for Cannabis Dependence. Ther. Drug Monit. 36 (2), 218–224. doi:10.1097/FTD.0b013e3182a5c446
Milman, G., Schwope, D. M., Schwilke, E. W., Darwin, W. D., Kelly, D. L., Goodwin, R. S., et al. (2011). Oral Fluid and Plasma Cannabinoid Ratios after Around-The-Clock Controlled Oral Δ(9)-tetrahydrocannabinol Administration. Clin. Chem. 57 (11), 1597–1606. doi:10.1373/clinchem.2011.169490
Montgomery, D., Plate, C., Alder, S. C., Jones, M., Jones, J., and Christensen, R. D. (2006). Testing for Fetal Exposure to Illicit Drugs Using Umbilical Cord Tissue vs Meconium. J. Perinatol. 26 (1), 11–14. doi:10.1038/sj.jp.7211416
Moore, C., Coulter, C., Rana, S., Vincent, M., and Soares, J. (2006). Analytical Procedure for the Determination of the Marijuana Metabolite 11-nor-Delta9-tetrahydrocannabinol-9-carboxylic Acid in Oral Fluid Specimens. J. Anal. Toxicol. 30 (7), 409–412. doi:10.1093/jat/30.7.409
Moore, C., Lewis, D., Becker, J., and Leikin, J. (1996). The Determination of 11-Nor-Delta 9-Tetrahydrocannabinol-9-Carboxylic Acid (THCCOOH) in Meconium. J. Anal. Toxicol. 20 (1), 50–51. doi:10.1093/jat/20.1.50
Morgan, D. J. (1997). Drug Disposition in Mother and Foetus. Clin. Exp. Pharmacol. Physiol. 24 (11), 869–873. doi:10.1111/j.1440-1681.1997.tb02707.x
Moss, M. J., Bushlin, I., Kazmierczak, S., Koop, D., Hendrickson, R. G., Zuckerman, K. E., et al. (2021). Cannabis Use and Measurement of Cannabinoids in Plasma and Breast Milk of Breastfeeding Mothers. Pediatr. Res. doi:10.1038/s41390-020-01332-2
Nadulski, T., Pragst, F., Weinberg, G., Roser, P., Schnelle, M., Fronk, E. M., et al. (2005). Randomized, Double-Blind, Placebo-Controlled Study about the Effects of Cannabidiol (CBD) on the Pharmacokinetics of Delta9-tetrahydrocannabinol (THC) after Oral Application of THC Verses Standardized Cannabis Extract. Ther. Drug Monit. 27 (6), 799–810. doi:10.1097/01.ftd.0000177223.19294.5c
Naef, M., Russmann, S., Petersen-Felix, S., and Brenneisen, R. (2004). Development and Pharmacokinetic Characterization of Pulmonal and Intravenous Delta-9-Tetrahydrocannabinol (THC) in Humans. J. Pharm. Sci. 93 (5), 1176–1184. doi:10.1002/jps.20037
Newmeyer, M. N., Swortwood, M. J., Andersson, M., Abulseoud, O. A., Scheidweiler, K. B., and Huestis, M. A. (2017). Cannabis Edibles: Blood and Oral Fluid Cannabinoid Pharmacokinetics and Evaluation of Oral Fluid Screening Devices for Predicting Δ9-Tetrahydrocannabinol in Blood and Oral Fluid Following Cannabis Brownie Administration. Clin. Chem. 63 (3), 647–662. doi:10.1373/clinchem.2016.265371
Newmeyer, M. N., Swortwood, M. J., Barnes, A. J., Abulseoud, O. A., Scheidweiler, K. B., and Huestis, M. A. (2016). Free and Glucuronide Whole Blood Cannabinoids' Pharmacokinetics after Controlled Smoked, Vaporized, and Oral Cannabis Administration in Frequent and Occasional Cannabis Users: Identification of Recent Cannabis Intake. Clin. Chem. 62 (12), 1579–1592. doi:10.1373/clinchem.2016.263475
O'Hara, K., Wright, I. M., Schneider, J. J., Jones, A. L., and Martin, J. H. (2015). Pharmacokinetics in Neonatal Prescribing: Evidence Base, Paradigms and the Future. Br. J. Clin. Pharmacol. 80 (6), 1281–1288. doi:10.1111/bcp.12741
Ochiai, W., Kitaoka, S., Kawamura, T., Hatogai, J., Harada, S., Iizuka, M., et al. (2021). Maternal and Fetal Pharmacokinetic Analysis of Cannabidiol during Pregnancy in Mice. Drug Metab. Dispos. 49 (4), 337–343. doi:10.1124/dmd.120.000270
Ohlsson, A., Lindgren, J. E., Wahlen, A., Agurell, S., Hollister, L. E., and Gillespie, H. K. (1980). Plasma Delta-9 Tetrahydrocannabinol Concentrations and Clinical Effects after Oral and Intravenous Administration and Smoking. Clin. Pharmacol. Ther. 28 (3), 409–416. doi:10.1038/clpt.1980.181
Ostrea, E. M., Knapp, D. K., Tannenbaum, L., Ostrea, A. R., Romero, A., Salari, V., et al. (2001). Estimates of Illicit Drug Use during Pregnancy by Maternal Interview, Hair Analysis, and Meconium Analysis. J. Pediatr. 138 (3), 344–348. doi:10.1067/mpd.2001.111429
Pacifici, R., Pichini, S., Pellegrini, M., Tittarelli, R., Pantano, F., Mannocchi, G., et al. (2018). Determination of Cannabinoids in Oral Fluid and Urine of "light Cannabis" Consumers: a Pilot Study. Clin. Chem. Lab. Med. 57 (2), 238–243. doi:10.1515/cclm-2018-0566
Palmer, K. L., Wood, K. E., and Krasowski, M. D. (2017). Evaluating a Switch from Meconium to Umbilical Cord Tissue for Newborn Drug Testing: A Retrospective Study at an Academic Medical Center. Clin. Biochem. 50 (6), 255–261. doi:10.1016/j.clinbiochem.2016.11.026
Patrician, A., Versic-Bratincevic, M., Mijacika, T., Banic, I., Marendic, M., Sutlović, D., et al. (2019). Examination of a New Delivery Approach for Oral Cannabidiol in Healthy Subjects: A Randomized, Double-Blinded, Placebo-Controlled Pharmacokinetics Study. Adv. Ther. 36 (11), 3196–3210. doi:10.1007/s12325-019-01074-6
Paudel, K. S., Hammell, D. C., Agu, R. U., Valiveti, S., and Stinchcomb, A. L. (2010). Cannabidiol Bioavailability after Nasal and Transdermal Application: Effect of Permeation Enhancers. Drug Dev. Ind. Pharm. 36 (9), 1088–1097. doi:10.3109/03639041003657295
Paul, S. E., Hatoum, A. S., Fine, J. D., Johnson, E. C., Hansen, I., Karcher, N. R., et al. (2021). Associations between Prenatal Cannabis Exposure and Childhood Outcomes: Results from the ABCD Study. JAMA Psychiatry 78 (1), 64–76. doi:10.1001/jamapsychiatry.2020.2902
Pellesi, L., Licata, M., Verri, P., Vandelli, D., Palazzoli, F., Marchesi, F., et al. (2018). Pharmacokinetics and Tolerability of Oral Cannabis Preparations in Patients with Medication Overuse Headache (MOH)-a Pilot Study. Eur. J. Clin. Pharmacol. 74 (11), 1427–1436. doi:10.1007/s00228-018-2516-3
Pérez-Acevedo, A. P., Busardò, F. P., Pacifici, R., Mannocchi, G., Gottardi, M., Poyatos, L., et al. (2020). Disposition of Cannabidiol Metabolites in Serum and Urine from Healthy Individuals Treated with Pharmaceutical Preparations of Medical Cannabis. Pharm. (Basel) 13 (12). doi:10.3390/ph13120459
Pérez-Acevedo, A. P., Pacifici, R., Mannocchi, G., Gottardi, M., Poyatos, L., Papaseit, E., et al. (2021). Disposition of Cannabinoids and Their Metabolites in Serum, Oral Fluid, Sweat Patch and Urine from Healthy Individuals Treated with Pharmaceutical Preparations of Medical Cannabis. Phytother. Res. 35 (3), 1646–1657. doi:10.1002/ptr.6931
Perez-Reyes, M., White, W. R., McDonald, S. A., Hicks, R. E., Jeffcoat, A. R., and Cook, C. E. (1991). The Pharmacologic Effects of Daily Marijuana Smoking in Humans. Pharmacol. Biochem. Behav. 40 (3), 691–694. doi:10.1016/0091-3057(91)90384-e
Perlin, E., Smith, C. G., Nichols, A. I., Almirez, R., Flora, K. P., Cradock, J. C., et al. (1985). Disposition and Bioavailability of Various Formulations of Tetrahydrocannabinol in the Rhesus Monkey. J. Pharm. Sci. 74 (2), 171–174. doi:10.1002/jps.2600740213
Pertwee, R. G. (2012). Targeting the Endocannabinoid System with Cannabinoid Receptor Agonists: Pharmacological Strategies and Therapeutic Possibilities. Philos. Trans. R. Soc. Lond B Biol. Sci. 367 (1607), 3353–3363. doi:10.1098/rstb.2011.0381
Pichini, S., Mannocchi, G., Berretta, P., Zaami, S., Pirani, F., Pacifici, R., et al. (2020). Δ9-Tetrahydrocannabinol and Cannabidiol Time Courses in the Sera of "Light Cannabis" Smokers: Discriminating Light Cannabis Use from Illegal and Medical Cannabis Use. Ther. Drug Monit. 42 (1), 151–156. doi:10.1097/FTD.0000000000000683
Pichini, S., Mannocchi, G., Gottardi, M., Pérez-Acevedo, A. P., Poyatos, L., Papaseit, E., et al. (2020). Fast and Sensitive UHPLC-MS/MS Analysis of Cannabinoids and Their Acid Precursors in Pharmaceutical Preparations of Medical Cannabis and Their Metabolites in Conventional and Non-conventional Biological Matrices of Treated Individual. Talanta 209, 120537. doi:10.1016/j.talanta.2019.120537
Prego-Meleiro, P., Lendoiro, E., Concheiro, M., Cruz, A., López-Rivadulla, M., and de Castro, A. (2017). Development and Validation of a Liquid Chromatography Tandem Mass Spectrometry Method for the Determination of Cannabinoids and Phase I and II Metabolites in Meconium. J. Chromatogr. A 1497, 118–126. doi:10.1016/j.chroma.2017.03.066
Pryor, G. T., Husain, S., and Mitoma, C. (1977). Influence of Fasting on the Absorption and Effects of Delta9-Tetrahydrocannabinol after Oral Administration in Sesame Oil. Pharmacol. Biochem. Behav. 6 (3), 331–341. doi:10.1016/0091-3057(77)90033-8
Ravula, A., Chandasana, H., Setlow, B., Febo, M., Bruijnzeel, A. W., and Derendorf, H. (2018). Simultaneous Quantification of Cannabinoids Tetrahydrocannabinol, Cannabidiol and CB1 Receptor Antagonist in Rat Plasma: An Application to Characterize Pharmacokinetics after Passive Cannabis Smoke Inhalation and Co-administration of Rimonabant. J. Pharm. Biomed. Anal. 160, 119–125. doi:10.1016/j.jpba.2018.07.004
Rotermann, M. (2021). Looking Back from 2020, How Cannabis Use and Related Behaviours Changed. Canada: Statistics Canada.
Russo, E. B., Burnett, A., Hall, B., and Parker, K. K. (2005). Agonistic Properties of Cannabidiol at 5-HT1a Receptors. Neurochem. Res. 30 (8), 1037–1043. doi:10.1007/s11064-005-6978-1
Samara, E., Bialer, M., and Harvey, D. J. (1990). Pharmacokinetics of Urinary Metabolites of Cannabidiol in the Dog. Biopharm. Drug Dispos. 11 (9), 785–795. doi:10.1002/bdd.2510110906
Samara, E., Bialer, M., and Mechoulam, R. (1988). Pharmacokinetics of Cannabidiol in Dogs. Drug Metab. Dispos. 16 (3), 469–472.
Schwilke, E. W., Schwope, D. M., Karschner, E. L., Lowe, R. H., Darwin, W. D., Kelly, D. L., et al. (2009). Delta9-tetrahydrocannabinol (THC), 11-Hydroxy-THC, and 11-Nor-9-Carboxy-THC Plasma Pharmacokinetics during and after Continuous High-Dose Oral THC. Clin. Chem. 55 (12), 2180–2189. doi:10.1373/clinchem.2008.122119
Schwope, D. M., Bosker, W. M., Ramaekers, J. G., Gorelick, D. A., and Huestis, M. A. (2012). Psychomotor Performance, Subjective and Physiological Effects and Whole Blood Δ⁹-tetrahydrocannabinol Concentrations in Heavy, Chronic Cannabis Smokers Following Acute Smoked Cannabis. J. Anal. Toxicol. 36 (6), 405–412. doi:10.1093/jat/bks044
Schwope, D. M., Karschner, E. L., Gorelick, D. A., and Huestis, M. A. (2011). Identification of Recent Cannabis Use: Whole-Blood and Plasma Free and Glucuronidated Cannabinoid Pharmacokinetics Following Controlled Smoked Cannabis Administration. Clin. Chem. 57 (10), 1406–1414. doi:10.1373/clinchem.2011.171777
Sempio, C., Wymore, E., Palmer, C., Bunik, M., Henthorn, T. K., Christians, U., et al. (2020). Detection of Cannabinoids by LC-MS-MS and ELISA in Breast Milk. J. Anal. Toxicol. doi:10.1093/jat/bkaa142
Shiu, J. R., and Ensom, M. H. (2012). Dosing and Monitoring of Methadone in Pregnancy: Literature Review. Can. J. Hosp. Pharm. 65 (5), 380–386. doi:10.4212/cjhp.v65i5.1176
Skopp, G., and Pötsch, L. (2008). Cannabinoid Concentrations in Spot Serum Samples 24-48 Hours after Discontinuation of Cannabis Smoking. J. Anal. Toxicol. 32 (2), 160–164. doi:10.1093/jat/32.2.160
Smith, A. M., Fried, P. A., Hogan, M. J., and Cameron, I. (2006). Effects of Prenatal Marijuana on Visuospatial Working Memory: an fMRI Study in Young Adults. Neurotoxicol Teratol. 28 (2), 286–295. doi:10.1016/j.ntt.2005.12.008
Smith, A. M., Longo, C. A., Fried, P. A., Hogan, M. J., and Cameron, I. (2010). Effects of Marijuana on Visuospatial Working Memory: an fMRI Study in Young Adults. Psychopharmacol. Berl. 210 (3), 429–438. doi:10.1007/s00213-010-1841-8
Smith, A. M., Mioduszewski, O., Hatchard, T., Byron-Alhassan, A., Fall, C., and Fried, P. A. (2016). Prenatal Marijuana Exposure Impacts Executive Functioning into Young Adulthood: An fMRI Study. Neurotoxicol Teratol. 58, 53–59. doi:10.1016/j.ntt.2016.05.010
Spindle, T. R., Cone, E. J., Herrmann, E. S., Mitchell, J. M., Flegel, R., LoDico, C., et al. (2020). Pharmacokinetics of Cannabis Brownies: A Controlled Examination of Δ9-Tetrahydrocannabinol and Metabolites in Blood and Oral Fluid of Healthy Adult Males and Females. J. Anal. Toxicol. 44 (7), 661–671. doi:10.1093/jat/bkaa067
Spindle, T. R., Cone, E. J., Kuntz, D., Mitchell, J. M., Bigelow, G. E., Flegel, R., et al. (2020). Urinary Pharmacokinetic Profile of Cannabinoids Following Administration of Vaporized and Oral Cannabidiol and Vaporized CBD-Dominant Cannabis. J. Anal. Toxicol. 44 (2), 109–125. doi:10.1093/jat/bkz080
Spindle, T. R., Cone, E. J., Schlienz, N. J., Mitchell, J. M., Bigelow, G. E., Flegel, R., et al. (2019). Acute Pharmacokinetic Profile of Smoked and Vaporized Cannabis in Human Blood and Oral Fluid. J. Anal. Toxicol. 43 (4), 233–258. doi:10.1093/jat/bky104
Statistics Canada (2018). Canadian Tobacco, Alcohol and Drugs Survey. Available from: https://www150.statcan.gc.ca/n1/daily-quotidien/181030/dq181030b-eng.htm.
Stott, C. G., White, L., Wright, S., Wilbraham, D., and Guy, G. W. (2013). A Phase I Study to Assess the Effect of Food on the Single Dose Bioavailability of the THC/CBD Oromucosal Spray. Eur. J. Clin. Pharmacol. 69 (4), 825–834. doi:10.1007/s00228-012-1393-4
Stott, C. G., White, L., Wright, S., Wilbraham, D., and Guy, G. W. (2013). A Phase I Study to Assess the Single and Multiple Dose Pharmacokinetics of THC/CBD Oromucosal Spray. Eur. J. Clin. Pharmacol. 69 (5), 1135–1147. doi:10.1007/s00228-012-1441-0
Substance of Abuse and Mental Health Service Administration, Center for Behavioral Health Statistics and Quality (2020). National Survey on Drugs Use and Health, 2019 and Quarters 1 and 4, 2020. Available from: https://www.samhsa.gov/data/sites/default/files/reports/rpt35339/2020NSDUHsaePercents012422/NSDUHsaePercents2020.pdf.
Taylor, L., Crockett, J., Tayo, B., and Morrison, G. (2019). A Phase 1, Open-Label, Parallel-Group, Single-Dose Trial of the Pharmacokinetics and Safety of Cannabidiol (CBD) in Subjects with Mild to Severe Hepatic Impairment. J. Clin. Pharmacol. 59 (8), 1110–1119. doi:10.1002/jcph.1412
Taylor, M., Lees, R., Henderson, G., Lingford-Hughes, A., Macleod, J., Sullivan, J., et al. (2017). Comparison of Cannabinoids in Hair with Self-Reported Cannabis Consumption in Heavy, Light and Non-cannabis Users. Drug Alcohol Rev. 36 (2), 220–226. doi:10.1111/dar.12412
Thompson, R., DeJong, K., and Lo, J. (2019). Marijuana Use in Pregnancy: A Review. Obstet. Gynecol. Surv. 74 (7), 415–428. doi:10.1097/OGX.0000000000000685
Toennes, S. W., Ramaekers, J. G., Theunissen, E. L., Moeller, M. R., and Kauert, G. F. (2008). Comparison of Cannabinoid Pharmacokinetic Properties in Occasional and Heavy Users Smoking a Marijuana or Placebo Joint. J. Anal. Toxicol. 32 (7), 470–477. doi:10.1093/jat/32.7.470
Toennes, S. W., Ramaekers, J. G., Theunissen, E. L., Moeller, M. R., and Kauert, G. F. (2010). Pharmacokinetic Properties of Delta9-Tetrahydrocannabinol in Oral Fluid of Occasional and Chronic Users. J. Anal. Toxicol. 34 (4), 216–221. doi:10.1093/jat/34.4.216
Toennes, S. W., Schneider, K., Wunder, C., Kauert, G. F., Moeller, M. R., Theunissen, E. L., et al. (2013). Influence of Ethanol on the Pharmacokinetic Properties of Δ9-tetrahydrocannabinol in Oral Fluid. J. Anal. Toxicol. 37 (3), 152–158. doi:10.1093/jat/bkt002
Torrens, A., Vozella, V., Huff, H., McNeil, B., Ahmed, F., Ghidini, A., et al. (2020). Comparative Pharmacokinetics of Δ9-Tetrahydrocannabinol in Adolescent and Adult Male Mice. J. Pharmacol. Exp. Ther. 374 (1), 151–160. doi:10.1124/jpet.120.265892
Tynon, M., Porto, M., and Logan, B. K. (2015). Simplified Analysis of 11-Hydroxy-Delta-9-Tetrahydrocannabinol and 11-Carboxy-Delta-9-Tetrahydrocannabinol in Human Meconium: Method Development and Validation. J. Anal. Toxicol. 39 (1), 35–40. doi:10.1093/jat/bku107
Tzatzarakis, M. N., Alegakis, A. K., Kavvalakis, M. P., Vakonaki, E., Stivaktakis, P. D., Kanaki, K., et al. (2017). Comparative Evaluation of Drug Deposition in Hair Samples Collected from Different Anatomical Body Sites. J. Anal. Toxicol. 41 (3), 214–223. doi:10.1093/jat/bkw127
Uziel, A., Gelfand, A., Amsalem, K., Berman, P., Lewitus, G. M., Meiri, D., et al. (2020). Full-Spectrum Cannabis Extract Microdepots Support Controlled Release of Multiple Phytocannabinoids for Extended Therapeutic Effect. ACS Appl. Mater Interfaces 12 (21), 23707–23716. doi:10.1021/acsami.0c04435
Valiveti, S., Agu, R. U., Hammell, D. C., Paudel, K. S., Earles, D. C., Wermeling, D. P., et al. (2007). Intranasal Absorption of Delta(9)-tetrahydrocannabinol and WIN55,212-2 Mesylate in Rats. Eur. J. Pharm. Biopharm. 65 (2), 247–252. doi:10.1016/j.ejpb.2006.08.009
Valiveti, S., and Stinchcomb, A. L. (2004). Liquid Chromatographic-Mass Spectrometric Quantitation of Delta9-tetrahydrocannabinol and Two Metabolites in Pharmacokinetic Study Plasma Samples. J. Chromatogr. B Anal. Technol. Biomed. Life Sci. 803 (2), 243–248. doi:10.1016/j.jchromb.2003.12.024
Vinner, E., Vignau, J., Thibault, D., Codaccioni, X., Brassart, C., Humbert, L., et al. (2003). Neonatal Hair Analysis Contribution to Establishing a Gestational Drug Exposure Profile and Predicting a Withdrawal Syndrome. Ther. Drug Monit. 25 (4), 421–432. doi:10.1097/00007691-200308000-00002
Volkow, N. D., Han, B., Compton, W. M., and Blanco, C. (2017). Marijuana Use during Stages of Pregnancy in the United States. Ann. Intern Med. 166 (10), 763–764. doi:10.7326/L17-0067
Wang, G. S. (2017). Pediatric Concerns Due to Expanded Cannabis Use: Unintended Consequences of Legalization. J. Med. Toxicol. 13 (1), 99–105. doi:10.1007/s13181-016-0552-x
Ward, R. M., and Varner, M. W. (2019). Principles of Pharmacokinetics in the Pregnant Woman and Fetus. Clin. Perinatol. 46 (2), 383–398. doi:10.1016/j.clp.2019.02.014
Westin, A. A., Huestis, M. A., Aarstad, K., and Spigset, O. (2009). Short Communication: Urinary Excretion of 11-nor-9-carboxy-Delta(9)-tetrahydrocannabinol in a Pregnant Woman Following Heavy, Chronic Cannabis Use. J. Anal. Toxicol. 33 (9), 610–614. doi:10.1093/jat/33.9.610
Wolowich, W. R., Greif, R., Kleine-Brueggeney, M., Bernhard, W., and Theiler, L. (2019). Minimal Physiologically Based Pharmacokinetic Model of Intravenously and Orally Administered Delta-9-Tetrahydrocannabinol in Healthy Volunteers. Eur. J. Drug Metab. Pharmacokinet. 44 (5), 691–711. doi:10.1007/s13318-019-00559-7
Wu, F., Scroggin, T. L., Metz, T. D., and McMillin, G. A. (2018). Development of a Liquid Chromatography-Tandem Mass Spectrometry Method for the Simultaneous Determination of Four Cannabinoids in Umbilical Cord Tissue. J. Anal. Toxicol. 42 (1), 42–48. doi:10.1093/jat/bkx078
Wymore, E. M., Palmer, C., Wang, G. S., Metz, T. D., Bourne, D. W. A., Sempio, C., et al. (2021). Persistence of Δ-9-Tetrahydrocannabinol in Human Breast Milk. JAMA Pediatr. 175 (6), 632–634. doi:10.1001/jamapediatrics.2020.6098
Xu, C., Chang, T., Du, Y., Yu, C., Tan, X., and Li, X. (2019). Pharmacokinetics of Oral and Intravenous Cannabidiol and its Antidepressant-like Effects in Chronic Mild Stress Mouse Model. Environ. Toxicol. Pharmacol. 70, 103202. doi:10.1016/j.etap.2019.103202
Zgair, A., Wong, J. C., Sabri, A., Fischer, P. M., Barrett, D. A., Constantinescu, C. S., et al. (2015). Development of a Simple and Sensitive HPLC-UV Method for the Simultaneous Determination of Cannabidiol and Δ(9)-tetrahydrocannabinol in Rat Plasma. J. Pharm. Biomed. Anal. 114, 145–151. doi:10.1016/j.jpba.2015.05.019
Keywords: pregnancy, breastfeeding, pharmacokinetics, cannabis, neonates, exposure
Citation: Monfort A, Ferreira E, Leclair G and Lodygensky GA (2022) Pharmacokinetics of Cannabis and Its Derivatives in Animals and Humans During Pregnancy and Breastfeeding. Front. Pharmacol. 13:919630. doi: 10.3389/fphar.2022.919630
Received: 13 April 2022; Accepted: 27 May 2022;
Published: 12 July 2022.
Edited by:
Elena Y. Enioutina, The University of Utah, United StatesReviewed by:
Valentina Bassareo, University of Cagliari, ItalyAna Martin-Sanchez, Pompeu Fabra University, Spain
Copyright © 2022 Monfort, Ferreira, Leclair and Lodygensky. This is an open-access article distributed under the terms of the Creative Commons Attribution License (CC BY). The use, distribution or reproduction in other forums is permitted, provided the original author(s) and the copyright owner(s) are credited and that the original publication in this journal is cited, in accordance with accepted academic practice. No use, distribution or reproduction is permitted which does not comply with these terms.
*Correspondence: Gregory Anton Lodygensky, Z2EubG9keWdlbnNreUB1bW9udHJlYWwuY2E=