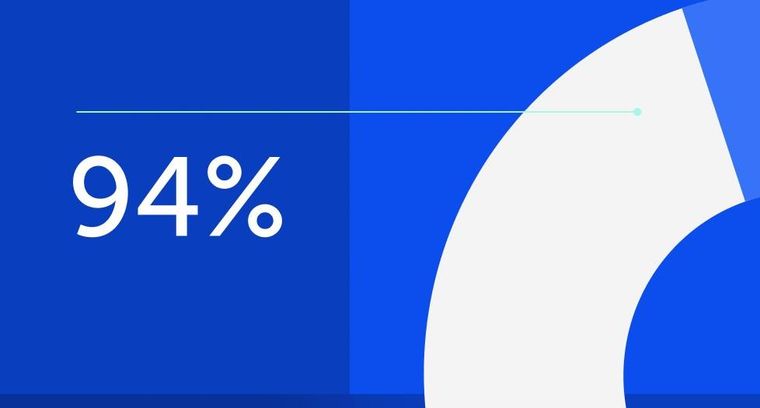
94% of researchers rate our articles as excellent or good
Learn more about the work of our research integrity team to safeguard the quality of each article we publish.
Find out more
ORIGINAL RESEARCH article
Front. Pharmacol., 08 August 2022
Sec. Ethnopharmacology
Volume 13 - 2022 | https://doi.org/10.3389/fphar.2022.917544
This article is part of the Research TopicThe Application of Network Analysis in EthnopharmacologyView all 13 articles
The Wenshenyang recipe (WSYR) has the effect of treating infertility, but the mechanisms underlying this activity have not been fully elucidated. In this study, network pharmacology and RNA sequencing were combined, with database-based “dry” experiments and transcriptome analysis-based “wet” experiments used conjointly to analyse the mechanism of WSYR in the treatment of infertility. In the dry analysis, 43 active compounds in WSYR and 44 therapeutic targets were obtained through a database search, 15 infertility pathways were significantly enriched, and key targets, such as ESR1, TP53, AKT1, IL-6, and IL-10 were identified. Then the wet experiments were performed to detect the expression changes of the 412 genes from 15 infertility pathways identified by dry analysis. HK-2 cells were treated with the three herbs of WSYR and subjected to targeted RNA sequencing. Based on the results, 92 of the 412 genes in 15 infertility pathways were identified as DEGs. Additionally, key targets, such as ESR2, STAT1, STAT3, and IL6, were also identified in the wet experiments. RT-qPCR experiments further verified that WSYR played an anti-inflammatory role by upregulating IL-4 and IL-10 and Epimedium brevicornu Maxim (Yinyanghuo) showed broader effect than Drynaria fortunei (Kunze) J. Sm (Gusuibu) and Cistanche deserticola Y.C.Ma (Roucongrong). By screening compounds of WSYR using molecular docking models of ESR1 and ESR2, it was further found that xanthogalenol in Gusuibu, arachidonate in Roucongrong, and anhydroicaritin in Yinyanghuo had good affinity for estrogen receptors. These findings provide evidence for an estrogen-regulating role of the three herbs in WSYR.
According to WHO analyses, 186 million individuals (including 48 million couples) worldwide suffer from infertility (Rutstein and Shah, 2004; Boivin et al., 2007; Mascarenhas et al., 2012). Studies have shown annual increases in the incidence of infertility in recent years (Petraglia et al., 2013), and it has become an important factor affecting human health and family stability. Factors affecting both male and female fertility include hyperprolactinism, hypogonadism, cystic fibrosis, systemic diseases, infections and lifestyle-related factors (Vander Borght and Wyns, 2018). The causes of female infertility mainly include ovulation disorders, blocked fallopian tubes, and cervical factors (Vander Borght and Wyns, 2018). The most common cause of female infertility is ovulation failure, which occurs in 30%–40% of infertile women (Nahid and Sirous, 2012). Specifically, it includes anovulation caused by pituitary secretion disorders and ovulation disorders caused by endocrine disorders. Causes of male infertility include abnormal sperm, blocked sperm delivery, and immune factors (Vander Borght and Wyns, 2018). Sperm abnormalities lead to impaired spermatogenesis, impaired maturation, blocked sperm transport ducts, and abnormal gonads, which are major causes of male infertility (Wang et al., 2020). Additional causes of male infertility mainly include abnormal semen, blocked sperm delivery and immune factors.
Assisted reproductive technology (ART) has been used in the treatment of infertility. More than 5 million children worldwide have been born through in vitro fertilization and other ART interventions (Messerlian and Gaskins, 2017). However, ART is technologically sophisticated and expensive, such that it is unaffordable or even unavailable in many countries and regions, especially low- and middle-income areas.
As an important category of complementary and alternative medicine, traditional Chinese medicine (TCM) has been widely used in treating infertility in recent decades. Compared with ART, TCM has the advantages of greater accessibility, lower cost, fewer adverse reactions, and higher safety (Huang and Chen, 2008). A study reported that the Wenshenyang recipe can promote the proliferation and differentiation of shoot stem cells and promote the synthesis of cartilage and cartilage matrix during the early limb development of the embryo, which is closely related to the formation and development of the foetus (Xue et al., 2021). The Wenshenyang Recipe consists of three Chinese medicines: Cistanche deserticola Y.C.Ma (Roucongrong), Epimedium brevicornu Maxim (Yinyanghuo) and Drynaria fortunei (Kunze) J. Sm (Gusuibu). All three herbs have similar effects, i.e., warming the kidney and strengthening yang.
Modern pharmacological studies have proven that Yinyanghuo has a wide range of hormone-like effects, regulates the function of the hypothalamus-pituitary-gonad axis, and has the effect of “stimulating yang” in animal models of yang deficiency (An et al., 2015). Yinyanghuo can improve sperm motility in rats and protect against the epididymal damage caused by chemotherapy (Cao et al., 2008). Yinyanghuo also plays a role in reducing the levels of proinflammatory mediators and cytokines (Saba et al., 2020). Roucongrong and its active ingredient echinacoside (ECH) can enhance the biosynthesis of testosterone by upregulating the expression of a variety of steroid-generating enzymes, improving poor sperm quality and reducing testicular toxicity in rats (Jiang et al., 2016). ECH can block hypothalamic androgen receptor (AR) activity and increase the secretion of luteinizing hormone and testosterone, thereby increasing sperm number, to treat oligospermia (Jiang et al., 2018). Gusuibu strengthens muscles and bones and has immunomodulatory activity (Jeong et al., 2005). However, due to the complex components of herbs and the complicated process of infertility development, the mechanism of WSYR for treating infertility is still unclear.
Network pharmacology is a discipline that predicts the active ingredients in TCM prescriptions and explains the potential mechanism of action of TCM prescriptions from a systematic perspective. The concept of holism and focus on systems conforms to the characteristics of TCM and is a more suitable method for studying the multicomponent, multitarget, and multipath mechanisms of traditional Chinese medicine (Li and Zhang, 2013). However, the results predicted by network pharmacology are only speculative results based on available data, not validated effects. Therefore, we aimed to combine network pharmacology with gene expression profiling of TCM-treated cells. Transcriptome analysis was performed by RASL-seq, which combines the RNA annealing, selection, ligation (RASL) strategy and next-generation sequencing (Li et al., 2012). This technology can simultaneously detect the expression of thousands of genes after drug treatment (Shao et al., 2019) and effectively verify the large-scale gene data set predicted by network pharmacology.
In this study, the potential anti-infertility targets of WSYR were obtained through network pharmacology analysis. Pathway enrichment analysis was performed to identify the key pathways of WSYR in the treatment of infertility, and a “TCMs-components-targets-pathways” network map was constructed. Afterwards, transcriptome analysis was utilized to examine the expression of genes in the predicted pathways after drug intervention. Altogether, the key targets of WSYR in the treatment of infertility were identified, and its mechanism of action was explored.
The TCM System Pharmacology Database (TCMSP, https://tcmspw.com/tcmsp. php) (Ru et al., 2014) was used to collect the chemical components of WSYR with the keywords “roucongrong”, “yinyanghuo” and “gusuibu” respectively. Compounds with oral bioavailability (OB) ≥ 30% and drug-like properties (DL) ≥ 0.18 were selected as active components.
The potential targets of each compound were obtained through the TCMSP database. The Entrez ID, and gene symbol of each target were collected.
ClueGO (Bindea et al., 2009) was used to analyse the GO biological processes and enriched Reactome pathways of different gene sets. The bubble diagram and bar chart were drawn by the Bioinformatics Online Visualization Tool (http://www.bioinformatics.com.cn).
HK-2 cells were obtained from the National Experimental Cell Resource Sharing Platform (Wuhan, China). SK-OV-3 cells were obtained from Procell Life Science and Technology Co.,Ltd (Wuhan, China). All cells were maintained in DMEM (Gibco, Grand Island, NY) containing 10% foetal bovine serum (Gemini, Woodland, CA) and 100 U/mL penicillin–streptomycin (Gibco, Grand Island, NY) at 37°C.
Roucongrong (origin: Neimenggu), Yinyanghuo (origin: Guangdong) and Gusuibu (origin: Jilin) were purchased from Anguo Changda Chinese Herbal Medicine Co., Ltd. All three TCMs were powdered and extracted by a Soxhlet extractor (Extraction Unit B-811, Buchi, Switzerland) with 90% ethanol. Then, the solvent was concentrated in an electrically heated blast drying oven (GZX-9070MBE, Boxun, China) at 45°C. Subsequently, the concentrate was lyophilized with a freeze dryer (ALPHA1-2Dplus, Christ, Germany), weighed and stored at -80°C for later use.
Transcriptome sequencing were performed as previously described (Shao et al., 2019). Briefly, HK-2 cells were cultured in a 384-well plate for 24 h and treated with the three herbal extracts for 24 h. Then, the cells were lysed, incubated at room temperature for 10 min, and stored at -80°C. A total of 412 genes were detected by RNA annealing, selection and ligation with a high-throughput pipetting platform. The ligated products were amplified by PCR and sequenced by a gene sequencer (HiSeq X Ten, Illumina, United States). The data discussed in this publication have been deposited in NCBI’s Gene Expression Omnibus and are accessible through GEO Series accession number GSE202626 (https://www.ncbi.nlm.nih.gov/geo/query/acc.cgi?acc=GSE202626).
First, the high-throughput sequencing platform data files were converted into raw data for base identification analysis. Subsequently, the original data were filtered by more than 3 base sequence mismatches. Finally, the DESeq software package was used to identify differentially expressed genes (DEGs) with fold change (FC) > 1.5 or <0.67 and p value < 0.05. The R package ggplot2 was used to construct a volcano plot of the DEGs (Anders and Huber, 2010).
All DEGs were analysed by the STRING database to obtain protein–protein interactions. Protein–protein interaction networks were constructed by Cytoscape (Shannon et al., 2003). The cytoHubba plug-in included in Cytoscape was used for target topology analysis to obtain the main topological parameters of the PPI network. The sum of degree, betweenness and closeness after the standardization of deviation was used as the indicator to determine the key targets.
Total RNA was extracted with all in-one mini spin columns (Epoch Life Science, Fort Bend, TX). Reverse transcription into cDNA was performed using a high Capacity RNA-to-cdna kit (Invitrogen) and qPCR was carried out using the SYBR FAST qPCR Kit (Kapa Biosystems, Wilmington, MA). Finally, the 2−ΔΔCT method (Nolan et al., 2006) was used to calculate the data. The primer sequences are provided in Supplementary Table S1.
The protein crystal structure of ESR1 and ESR2 were downloaded from the Protein Data Bank (PDB, https://www.rcsb.org/) for molecular docking. The protein crystal structure was imported into Discovery Studio, and the Prepare Protein module was used to remove water, add hydrogen atom and complement incomplete residues. Active pocket was defined based on the original ligand in the complex, and molecular docking was conducted by CDOCKER algorithm. The root-mean-square-deviation (RMSD) of the initial and re-docked conformations of the original ligand were calculated. RMSD <2 Å indicated that the conformation obtained by docking could reproduce the binding mode of the ligand and receptor, reflecting the reliability of the docking model. Finally, potential antagonists were screened by the constructed docking model. Scoring function of –CDOCKER interaction energy was used to evaluate the binding ability of ligand and receptor.
Figure 1 shows the technical roadmap of this study. This study combines a database-based dry experiment and a transcriptome analysis-based wet experiment to jointly analyse the mechanism of action of WSYR in the treatment of infertility.
A total of 75 chemical components for Roucongrong, 130 for Yinyanghuo and 71 for Gusuibu were collected from TCMSP. With a screening criterion of OB ≥ 30% and DL ≥ 0.18, 6 compounds were identified as active compounds for Roucongrong, 23 for Yinyanghuo, 18 for Gusuibu, and 43 for all (Table 1). The 43 WSYR compounds predicted as active compounds for WSYR based on database were used for molecular docking, which were screened by OB ≥ 30% and DL ≥ 0.18 (as listed in Table 1). In addition, 4 compounds were shared by two herbs. For example, luteolin and kaempferol were shared by Yinyanghuo and Gusuibu, β-sitosterol was shared by Roucongrong and Gusuibu, and quercetin was shared by Roucongrong and Yinyanghuo.
TABLE 1. Gusuibu, Roucongrong and Yinyanghuo compounds with oral bioavailability (OB) ≥ 30% and drug-likeness (DL) ≥ 0.18 from TCMSP.
Based on the TCMSP database, 201 potential targets were identified for WSYR, including 110 for Gusuibu, 90 for Roucongrong and 111 for Yinyanghuo. Detailed information about these targets is listed in Supplementary Table S2.
Three databases, OMIM, NCBI-Gene and DisGeNET, were used to search for infertility-related genes. A total of 642 genes were identified, including 246 from OMIM, 448 from NCBI-Gene and 124 from DisGeNET (Supplementary Table S3).
Of those 642 genes, 22 genes were identified as potential target genes in treating infertility for Roucongrong, 25 for Yinyanghuo and 25 for Gusuibu, 44 in total, as shown in Figure 2A and Table 2.
FIGURE 2. Functional analysis of infertility-related targets of WSYR identified in dry experiments (A) Venn diagram of the potential targets in WSYR and the therapeutic targets for infertility (B) Bubble chart of GO biological process enrichment analysis of 44 infertility treatment targets in WSYR (C) Bubble chart of pathway enrichment analysis of 44 infertility treatment targets in WSYR (p value ≤ 10−3) (D) Pathway enrichment analysis of infertility treatment-related targets of Roucongrong, Yinyanghuo and Gusuibu (p value ≤ 10−3) (E) Comparison of pathways regulated by Roucongrong, Yinyanghuo and Gusuibu.
There were 44 nonredundant infertility-related target genes in WSYR. To further explore the biological mechanisms of these 44 infertility targets of WSYR, GO biological process (BP) enrichment analysis was carried out. The targets were found to be involved in a variety of biological processes. The top 15 GO BPs are shown in Figure 2B and include the regulation of reactive oxygen species (ROS), the synthesis and metabolism of nitric oxide (NO), cellular response to oxidative stress, nuclear receptor activity, ligand-activated transcription factor activity, negative regulation of apoptosis signaling pathways, female pregnancy, heat generation, and cellular response to lipopolysaccharide.
At low concentrations, ROS participate in physiological regulation as a normal product of aerobic metabolism. However, excessive ROS can cause oxidative stress, which is regarded as related to oocyte maturation, embryonic development, and endometrial translocation (Wan and Qi, 2017). NO is an important free radical species that is important in embryonic development. The activity of NO and NOS is necessary for embryos to initiate the formation of the vasculature, and inhibition of NOS can lead to developmental defects or death of the embryo (Wu et al., 2020; Hitchler and Domann, 2021). Nuclear receptors play a very important role in the regulation of the final stage of ovarian follicle growth. Under the influence of follicle stimulating hormone, nuclear receptors promote the proliferation and differentiation of granulosa cells and the synthesis of steroids (Hughes and Murphy, 2021). WSYR may promote oocyte maturation and embryo development through these biological processes.
Pathway enrichment analysis was performed on the 44 infertility-related target genes of WSYR by ClueGO, and 15 pathways were enriched with a p value ≤ 10−3, as shown in Figure 2C. Among them, extra-nuclear estrogen signaling, the nuclear receptor transcription pathway, and endogenous sterols are all estrogen-related signaling pathways; regulation of TP53 degradation, regulation of TP53 expression and degradation, and the VEGFA-VEGFR2 pathway are all growth and apoptosis related pathways; interleukin-4 (IL-4) and interleukin-13 (IL-13) signaling, interleukin-10 (IL-10) signaling, and arachidonic acid metabolism are inflammatory signaling pathways; FOXO-mediated transcription, FOXO-mediated transcription of oxidative stress, metabolic and neuronal genes are oxidative stress signaling pathways. Those pathways are closely related to infertility, and involve mechanisms such as sex hormone synthesis and secretion, growth and apoptosis, inflammatory responses, and oxidative stress.
Pathway enrichment analysis was also performed on 22 infertility-related target genes for Roucongrong, 25 for Yinyanghuo and 25 for Gusuibu. As shown in Figure 2D, 2 pathways were enriched for Roucongrong, 7 pathways for Yinyanghuo and 9 pathways for Gusuibu. Therefore, we concluded that WSYR acts on multiple signaling pathways to regulate infertility and that all three constitutive herbs have both common and unique pathways, as shown in Figure 2E. All three herbs regulate inflammatory pathways. Yinyanghuo and Gusuibu both act on hormone regulation pathways. Yinyanghuo acts specially on growth and apoptosis signaling. The unique pathways of Gusuibu include FOXO-mediated transcription, arachidonic acid metabolism and endogenous sterols.
As shown in Figure 3A, a “Herbs-Compounds-Targets-Pathways” network was constructed to show the overall function of WSYR and its constitutive herbs.
FIGURE 3. Interactive networks of infertility treatment targets based on databases (A)“Herbs-Compounds-Targets-Pathways” network. Orange diamond: TCM; dark yellow triangle: active compound; green circle: gene; blue hexagon: pathway (B) Protein–protein interaction network (PPIN) of 44 WSYR infertility therapeutic targets. Dark pink circle: key targets; blue circle: other targets (C) Hormone-related PPIN based on database targets. Dark pink circle: key targets; blue circle: other targets (D) Inflammatory immune-regulated PPIN based on database targets. Dark pink circle: key targets; blue circle: other targets.
Yinyanghuo A, Yinyanghuo B and Yinyanghuo C, representative compounds of Yinyanghuo, play immune inflammation regulatory and sex hormone regulatory roles in the treatment of infertility. The above three compounds all act on prostaglandin G/H synthase 2 (PTGS2) and androgen receptor (AR). Yinyanghuo A acts on ESR1, and Yinyanghuo B acts on ESR2. PTGS2 exerts inflammatory immune regulation by affecting IL-4 and IL-13 signaling, IL-10 signaling, and arachidonic acid metabolism. It has been reported that PTGS2 is closely associated with ovulation failure and implantation disorders in infertility (Szczuko et al., 2020). AR, ESR1 and ESR2 regulate the nuclear receptor transcription pathway, and ESR1 and ESR2 regulate extra-nuclear estrogen signaling to play a hormonal regulatory role.
As components of Gusuibu (+)-catechin and naringenin play a role in regulating oxidative stress in the treatment of infertility. Both (+)-catechin and naringenin can act on catalase (CAT). CAT is an enzyme involved in oxidative stress detoxification. It protects the human body from oxidative stress by regulating FOXO-mediated transcription and FOXO-mediated transcription of oxidative stress.
The Roucongrong component quercetin plays a role in regulating inflammation immunity in infertility. Quercetin acts on IL-10, IL-1α, IL-1β, IL-6, TNF, CCL2 and other inflammatory factors and chemokines and regulates IL-4 and IL-13 signaling and IL-10 signaling.
A total of 44 WSYR infertility therapeutic targets were used to construct a PPIN (confidence level >0.9) and to calculate the degree, betweenness and closeness of each node in the network. The larger the three parameter values, the more critical are the targets in the network. The top 5 nodes with the highest sum of degree, betweenness and closeness were proposed as key targets of WSYR, including ESR1, TP53, AKT1, IL-6, and IL-10 (Figure 3B and Supplementary Table S4-A).
We constructed a hormone regulation-related PPIN (confidence level >0.4) involving the three pathways of extra-nuclear estrogen signaling, nuclear receptor transcription pathway, and endogenous sterols and calculated the degree, betweenness and closeness of each node in the network. ESR1 was the most critical target (Figure 3C and Supplementary Table S4-B).
In addition, we constructed an immune inflammation-related PPIN (confidence level >0.4) involving interleukin-4 and interleukin-13 signaling, interleukin-10 signaling, and arachidonic acid metabolism, and calculated the degree, betweenness and closeness of each node in the network. The key targets included TP53, IL-6, and PTGS2 (Figure 3D and Supplementary Table S4-C).
Due to the incomplete and unsystematic nature of network pharmacology, the predicted results may not fully reflect the actual mechanism of WSYR. The application of transcriptome analysis can identify differentially expressed genes in response to TCM treatment and complement the results from network pharmacology.
There is an old saying in Chinese medicine: the kidney stores the essence, which is the foundation of reproduction. Deficiency of kidney qi and inadequacy of Tiangui will result in insufficient production of fertilization-competent sperm, leading to infertility. Deficiency of kidney yang, inability to warm semen and warm uterus, can cause cold semen and cold uterus, thereby causing infertility. Based on this, transcriptome analysis was performed on HK-2 human renal epithelial cells to examine the transcriptional regulation of WSYR in infertility-related pathways.
According to the results above, 15 pathways with p values less than 10−3 are the most important in the treatment of infertility by WSYR. Therefore, the 412 genes in these 15 pathways were subjected to transcriptome analysis in HK-2 cells treated with Roucongrong, Yinyanghuo and Gusuibu.
As shown in the volcano map (Figure 4A), 26 DEGs were identified in Roucongrong, including 15 upregulated genes and 11 downregulated genes; 54 DEGs were identified in Yinyanghuo, including 30 upregulated genes and 24 downregulated genes; and 22 DEGs were identified in Gusuibu, including 19 upregulated genes and 3 downregulated genes (FC > 1.5 or <0.67 and p < 0.05). In conclusion, a total of 92 DEGs (Table 3) were identified for WSYR based on transcriptome analysis.
FIGURE 4. Functional analysis of WSYR DEGs based on transcriptome analysis (A) Volcano map of DEGs of Roucongrong, Yinyanghuo and Gusuibu. Red dots: upregulated genes; green dots: downregulated genes; black dots: unchanged genes (B) Bubble chart of GO biological processes enriched among the 92 DEGs related to WSYR (top 15) (C) Bubble chart of pathways enriched among the 92 DEGs related to WSYR (top 10) (D) Pathway enrichment analysis of DEGs in Roucongrong, Yinyanghuo and Gusuibu (p value ≤ 10−5) (E) Comparison of pathways regulated by Roucongrong, Yinyanghuo and Gusuibu.
To further explore the biological mechanism of these 92 DEGs in WSYR, GO BP enrichment analysis was carried out. As shown in Figure 4B, the DEGs were involved in a variety of biological processes, including regulation of cytokine-mediated signaling pathways, cell movement and migration, steroid hormone response, JAK-STAT receptor signaling pathways, neuronal death, intrinsic apoptosis signaling pathways, peptidyl-tyrosine phosphorylation modification, and proliferation of muscle and epithelial cells.
When inflammation occurs, cells such as leukocytes, fibroblasts, endothelial cells, and haematopoietic cells are induced to produce chemokines by IL-1, TNF and other cytokines and are guided by chemokines to migrate to specific tissues (Borrelli et al., 2013). Studies have found that the chemokines CXCL1 and CXCL13 are abnormally expressed in female infertility patients with chronic endometritis (Kitaya and Yasuo, 2010). Sex steroid hormones are essential elements that affect reproduction. They affect follicle formation, endometrial development, ovulation, implantation and other developmental events (Reichman and Rosenwaks, 2017) that are critical for female pregnancy. In addition, members of the JAK-STAT pathway, TYK2, STAT1 and STAT4, have been shown to be active in human sperm, indicating that defects in the JAK-STAT pathway in sperm may be related to male infertility (D'Cruz et al., 2001).
Similarly, many pathways were enriched by the 92 DEGs of WSYR; the top 10 pathways are shown in Figure 4C. Among them, extra-nuclear estrogen signaling and ESR-mediated signaling are both estrogen-related signaling pathways; the VEGFA-VEGFR2 pathway and signaling by VEGF are both growth- and apoptosis-related pathways; Interleukin-4 and interleukin-13 signaling, signaling by interleukins and cytokine signaling in immune system are inflammatory immune signaling pathways; and FOXO-mediated transcription is an oxidative stress signaling pathway. These results are consistent with those of database-based network pharmacology.
Pathway enrichment analysis of the DEGs of the three herbs was also carried out. The total numbers of enriched pathways with p value ≤ 10−5 were 21 for Roucongrong, 29 for Yinyanghuo, and 8 for Gusuibu (Figure 4D). WSYR acts on a variety of signaling pathways to regulate infertility, and the three herbs have both common and unique pathways (Figure 4E). For example, Roucongrong plays a role in inflammatory immune and hormone regulation, and Gusuibu plays a role in angiogenesis and hormone regulation. In addition to regulating inflammatory responses, angiogenesis and hormone levels, Yinyanghuo plays a unique role in the regulation of oxidative stress and receptor tyrosine kinases.
Based on the results of transcriptome analysis, we drew a “Herbs-DEGs-Pathways” diagram to show the overall function of WSYR and its constituent herbs, as shown in Figure 5A.
FIGURE 5. Interactive networks of WSYR based on DEGs (A) “Herbs-DEGs-Pathways” network. Orange diamond: TCM; green circle: gene; blue circle: pathway (B) Protein—protein interaction network (PPIN) of all DEGs from transcriptome sequencing. Dark pink circle: key targets; blue circle: other targets (C) Hormone-related PPIN based on DEGs.Dark pink circle: key targets; blue circle: other targets (D) Inflammatory immune-regulated PPIN based on DEGs.Dark pink circle: key targets; blue circle: other targets.
Proepiregulin (EREG) is coregulated by Roucongrong, Yinyanghuo and Gusuibu and acts on three signaling pathways: extra-nuclear estrogen signaling, ESR-mediated signaling and signaling by receptor tyrosine kinases. EREG is a member of the epidermal growth factor family, which can regulate angiogenesis and remodelling to promote tissue repair, wound healing and oocyte maturation (Riese and Cullum, 2014). Haem oxygenase 1 (HMOX1) is jointly regulated by Roucongrong and Yinyanghuo and acts on three signaling pathways: IL-4 and IL-13 signaling, signaling by interleukins and cytokine signaling in the immune system. HMOX1 catalyses the degradation of haem to produce carbon monoxide (CO), iron and biliverdin-IXα. The HMOX1/CO system has been found to exert cytoprotective effects on damaged organs and in animal models by regulating inflammation and apoptosis (Ryter, 2019). MMP7 is jointly regulated by Roucongrong and Yinyanghuo and acts on three signaling pathways: extranuclear estrogen signaling, ESR-mediated signaling and signal transduction. MMPs can degrade the extracellular matrix (ECM), affect the migration of germ cells, affect the spermatogenesis pathway, and play important roles in spermatogenesis and semen quality (Warinrak et al., 2015).
To identify the key targets of WSYR, a PPIN was constructed based on the 92 DEGs obtained by transcriptome analysis (confidence level >0.9), and 86 nodes are illustrated in Figure 5B and Supplementary Table S5-A. The degree, betweenness and closeness of each node in the network were calculated. The results showed that the key targets included STAT3, TP53, PIK3R1, EGFR, HRAS, PTK2, IL6, STAT1, SDC2 and EGF.
To further identify the key targets of WSYR in hormones, 19 DEGs (confidence level >0.4) corresponding to the two hormone-related pathways of extra-nuclear estrogen signaling and ESR-mediated signaling in Figure 4E were selected to construct a PPIN. Nineteen nodes are shown in Figure 5C and Supplementary Table S5-B. Additionally, the degree, betweenness and closeness of each node in the network was calculated. The results showed that the most critical target of hormone regulation is ESR2.
To further identify the key targets of WSYR in immune inflammation, 35 DEGs (confidence level >0.4) corresponding to the three inflammatory immune-related pathways interleukin-4 and interleukin-13 signaling, signaling by interleukins, and cytokine signaling in the immune system, as shown in Figure 4E, were selected to construct a PPIN. Thirty-five nodes are shown in Figure 5D and Supplementary Table S5-C. The degree, betweenness and closeness of each node in the network are calculated. The results showed that the key targets of WSYR involved in inflammatory responses included STAT3, IL6 and STAT1.
Based on the dry experiments and wet experiments, we have found that WSYR mainly plays a role in hormone regulation and inflammatory responses. In the next step, we plan to use RT-qPCR to verify the regulatory effect of WSYR on key genes involved in inflammation and to perform molecular docking to further identify the active compounds present in WSYR and validate the interaction between compounds and potential targets of WSYR.
To verify the anti-inflammatory effect of WSYR, we examined target genes in inflammatory responses by RT-qPCR. In this study, IL-4 and IL-10 enriched by the inflammatory pathway were selected for RT-qPCR validation in SK-OV-3 cells. IL-4 and IL-10 are classic anti-inflammatory cytokines that limit excessive tissue destruction caused by inflammation.
The experimental results showed that WSYR could play an anti-inflammatory role by upregulating the expression of IL-4 and IL-10 (Figure 6A and Figure 6C). Specifically, Gusuibu and Roucongrong can upregulate the expression of IL-4, and Yinyanghuo can upregulate the expression of IL-4 and IL10 to exert anti-inflammatory effects (Figure 6B and Figure 6D).
FIGURE 6. Action of WSYR and its herbs Gusuibu, Roucongrong, Yinyanghuo on SK-OV-3 cells. SK-OV-3 cells were treated with WSYR (100, 200, 300 μg/ml), Gusuibu (200 μg/ml), Roucongrong (200 μg/ml), Yinyanghuo (200 μg/ml) for 24 h. The mRNA expression of IL-4 (A,B), IL-10 (C,D) was analyzed by qPCR. *p < 0.05 and **p < 0.01 indicate statistical significance compared to control.
Studies have reported that estrogen receptors are important for maintaining follicle, oocyte growth and ovulation function (Tang et al., 2019). Our results suggested that WSYR treats infertility by regulating estrogen receptors, but the specific mechanism of action is still unclear. The molecular docking model of ESR1 and ESR2 was constructed, and the compounds of WSYR were evaluated by docking.
The protein crystal complex (pdb id: 5FQP) was selected as the docking target of ESR1. The original ligand ESR1 antagonist (Scott et al., 2015) was used as the positive control. Its -cdocker interaction energy (-CIE) score is 65.414, RMSD is 0.3557, pocket radius is 8.522, and pocket site is (14.230, 22.133, 65.663). The positive drug score (65.414) was set as the threshold, and the drug score value > threshold×75% as the standard for the drug to have a good affinity with ESR1. The molecular docking results of 43 WSYR compounds with ESR1 are shown in Table 4. Finally, the following components have strong affinity with ESR1: marckine (54.5303), xanthogalenol (53.776), arachidonate (51.688), yinyanghuo A (51.0526), linoleyl acetate (50.8283), suchilactone (50.5918), and davallioside A_qt (49.7764), and the result is shown in Figure 7.
FIGURE 7. The 3D interaction of the original ligand GQD (A), marckine (B), xanthogalenol (C), arachidonate (D) and yinyanghuo A (E), linoleyl acetate (F), suchilactone (G) and davallioside A_qt (H) with ESR1.
The protein crystal complex 1L2J (Shiau et al., 2002) was selected as the docking target of ESR2. The original ligand ESR2 antagonist was used as the positive control. Its -cdocker interaction energy score is 52.8724, RMSD is 0.6922, pocket radius is 7.574, and pocket site is (32.212, 82.073, -11.407). The positive drug score (52.8724) was set as the threshold, and the drug score value > threshold×90% was set as the standard for the drug to have a good affinity with ESR2. The molecular docking results of 43 WSYR compounds with ESR2 are shown in Table 5. Finally, the following components have strong affinity with ESR2: anhydroicaritin (50.9932), 8-isopentenyl-kaempferol (50.8610), davalliosideA_qt (50.4315), xanthogalenol (49.4134), and linoleyl acetate (49.0539) and the results are shown in Figure 8.
FIGURE 8. The 3D interaction of the original ligand THC (A), anhydroicaritin (B), 8-isopentenyl-kaempferol (C), davallioside A_qt (D), xanthogalenol (E) and linoleyl acetate (F) with ESR2.
In summary, the results showed that xanthogalenol in Gusuibu, arachidonate in Roucongrong, and anhydroicaritin in Yinyanghuo have good affinity for estrogen receptors. The proposed mechanism of WSYR in treating infertility was summarized in Supplementary Table S6, including the constituent herbs, compounds and corresponding functions.
By integrating the results of dry and wet experiments, it was found that the WSYR treats infertility by regulating hormone levels and inflammatory responses. Estrogen, estrogen receptors and various enzymes are closely related to clinical polycystic ovary syndrome, endometriosis and other reproductive endocrine diseases (Tang et al., 2019). Estrogen receptor is a ligand-activated transcription factor, which has several functional domains such as DNA binding domain, protein binding domain and transcriptional regulatory domain (Kuiper et al., 1996). Estrogen functions through ESR1 and ESR2 in follicle formation and ovulation. During the growth of follicles, ESR1 is mainly expressed on the follicle theca to regulate the proliferative effect of estrogen; while ESR2 is mainly expressed in the granulosa cells of the growing follicles at various stages to promote cell differentiation and retard proliferation (Pelletier and El-Alfy, 2000).
Various drugs by targeting estrogen receptors have been developed. For example, Clomiphene citrate (CC) is a clinically used estrogen receptor antagonistic drug to treat female and male infertility for 50 years (Roth et al., 2013). CC is an artificially synthetic estrogen derivative that can bind with estrogen receptor. CC antagonizes the hypothalamus-pituitary ER, leading to inhibition of the negative feedback effect of estradiol in circulation and increasing the pulse frequency of hypothalamus gonadotropin-releasing hormone. This leads to an increase in luteinizing hormone and follicle-stimulating hormone generated by the pituitary. Eventually, follicle growth and sperm generation are promoted (Revelli et al., 2011; Scovell and Khera, 2018; Quaas and Legro, 2019).
In our study, crystal complexes of ESR1 and ESR2 and their antagonists were selected to construct a molecular docking model to screen potential estrogen receptor antagonists in WSYR. The results revealed several compounds with high affinity to ESR1 or ESR2 and some of them have already been reported as related to estrogen regulation. For example, xanthogalenol in Gusuibu showed high affinity for both ESR1 and ESR2. Xanthogalenol may play a role in bone protection by activating the estrogen signaling pathway and promoting the differentiation and mineralization of osteoblasts in vitro (Wang et al., 2011). The arachidonate in Roucongrong showed high affinity for ESR1. It has been reported that arachidonate has pervasive modulatory effects on estrogen receptors in central and peripheral tissues (Kato, 1989). Anhydroicaritin in Yinyanghuo, which is the aglycone of Yinyanghuo’s indicator component icariin, showed high affinity to ESR2. As reported, anhydroicaritin has selective estrogen receptor regulatory activity (Tong et al., 2011). In addition, compounds such as marckine, yinyanghuo A, linoleyl acetate, suchilactone, davallioside A_qt, and 8-isopentenyl-kaempferol were also predicted by molecular docking. They may have potential estrogen-regulating effects and need further study.
In addition, WSYR was found with an anti-inflammatory role by our study. IL-4 and IL-10 are multipotent anti-inflammatory cytokines that mainly inhibit the proinflammatory processes. IL-4 signaling plays a part in not only Th2 cell function but also the regulation of regulatory T cells, which is essential in successful pregnancies. IL-10 mainly exerts anti-inflammatory effects by inhibiting proinflammatory cytokines such as IL-1, IL-12 and TNF. During normal pregnancy, IL-4 and IL-10 perform multiple functions, promoting placental formation and regulating trophoblast invasion and differentiation. Thus, the precise regulation of IL-4 and IL-10 is important for reducing maternal inflammation during different stages of pregnancy (Chatterjee et al., 2014). In this study, it was found that WSYR can exert an anti-inflammatory effect by upregulating the expression of IL-4 and IL-10. Among them, Gusuibu, Roucongrong and Yinyanhuo upregulate the expression of IL-4, while Yinyanghuo upregulates the expression of IL-4 and IL10. The role of the inflammatory markers was illustrated in Supplementary Figure S1.
This study integrates the methodical nature of dry experiments and the authenticity of wet experiments, systematically analyses the mechanism of action of WSYR in the treatment of infertility, and provides theoretical support and evidence for the clinical application of WSYR. However, the interaction of active compounds in WSYR and therapeutic targets needs to be further validated by in vitro experiments. Besides, since infertility is a complex disease, animal experiments are necessary to verify the regulatory role of WSYR on hormonal regulation and inflammatory responses in vivo.
The datasets presented in this study can be found in online repositories. The names of the repository/repositories and accession number(s) can be found below: NCBI GEO, GSE202626.
Conceptualization: LX and SZ. Transcriptome experiment: WH and LQ. Data analysis and visualization: XZ and SZ. Validation: CM and WG. Project administration: HD and HL. Writing original draft: LX, SZ and DZ.
This work was supported by National Key Research and Development Plan (2018YFC1704300) and National Natural Science Foundation of China (61927819).
The authors declare that the research was conducted in the absence of any commercial or financial relationships that could be construed as a potential conflict of interest.
All claims expressed in this article are solely those of the authors and do not necessarily represent those of their affiliated organizations, or those of the publisher, the editors and the reviewers. Any product that may be evaluated in this article, or claim that may be made by its manufacturer, is not guaranteed or endorsed by the publisher.
The Supplementary Material for this article can be found online at: https://www.frontiersin.org/articles/10.3389/fphar.2022.917544/full#supplementary-material
An, R., Li, B., You, L. S., and Wang, X. H. (2015). Improvement of kidney yang syndrome by icariin through regulating hypothalamus-pituitary-adrenal Axis. Chin. J. Integr. Med. 21 (10), 765–771. doi:10.1007/s11655-015-2063-z
Anders, S., and Huber, W. (2010). Differential expression analysis for sequence count data. Genome Biol. 11 (10), R106. doi:10.1186/gb-2010-11-10-r106
Bindea, G., Mlecnik, B., Hackl, H., Charoentong, P., Tosolini, M., Kirilovsky, A., et al. (2009). Cluego: A cytoscape plug-in to decipher functionally grouped gene ontology and pathway annotation networks. Bioinformatics 25 (8), 1091–1093. doi:10.1093/bioinformatics/btp101
Boivin, J., Bunting, L., Collins, J. A., and Nygren, K. G. (2007). International estimates of infertility prevalence and treatment-seeking: Potential need and demand for infertility medical care. Hum. Reprod. (Oxf) 22 (6), 1506–1512. doi:10.1093/humrep/dem046
Borrelli, G. M., Carvalho, K. I., Kallas, E. G., Mechsner, S., Baracat, E. C., Abrão, M. S., et al. (2013). Chemokines in the pathogenesis of endometriosis and infertility. J. Reprod. Immunol. 98 (1-2), 1–9. doi:10.1016/j.jri.2013.03.003
Cao, Y. Q., Ma, S. M., Fan, X. L., Lin, Y. X., Chen, M. X., Song, X. A., et al. (2008). Epimedium alleviates chemotherapy-induced damage to the ultrastructure and function of rat epididymides. Natl. J. Androl. 14 (2), 184–188. Chinese.
Chatterjee, P., Chiasson, V. L., Bounds, K. R., and Mitchell, B. M. (2014). Regulation of the anti-inflammatory cytokines interleukin-4 and interleukin-10 during pregnancy. Front. Immunol. 5, 253. doi:10.3389/fimmu.2014.00253
D'Cruz, O. J., Vassilev, A. O., and Uckun, F. M. (2001). Members of the janus kinase/signal transducers and activators of transcription (JAK/STAT) pathway are present and active in human sperm. Fertil. Steril. 76 (2), 258–266. doi:10.1016/s0015-0282(01)01896-9
Hitchler, M. J., and Domann, F. E. (2021). The epigenetic and morphogenetic effects of molecular oxygen and its derived reactive species in development. Free Radic. Biol. Med. 170, 70–84. doi:10.1016/j.freeradbiomed.2021.01.008
Huang, S. T., and Chen, A. P. (2008). Traditional Chinese medicine and infertility. Curr. Opin. Obstet. Gynecol. 20 (3), 211–215. doi:10.1097/GCO.0b013e3282f88e22
Hughes, C., and Murphy, B. D. (2021). Nuclear receptors: Key regulators of somatic cell functions in the ovulatory process. Mol. Asp. Med. 78, 100937. doi:10.1016/j.mam.2020.100937
Jeong, J. C., Lee, B. T., Yoon, C. H., Kim, H. M., and Kim, C. H. (2005). Effects of drynariae rhizoma on the proliferation of human bone cells and the immunomodulatory activity. Pharmacol. Res. 51 (2), 125–136. doi:10.1016/j.phrs.2004.06.005
Jiang, Z., Wang, J., Li, X., and Zhang, X. (2016). Echinacoside and Cistanche tubulosa (schenk) R. Wight ameliorate bisphenol A-induced testicular and sperm damage in rats through gonad Axis regulated steroidogenic enzymes. J. Ethnopharmacol. 193, 321–328. doi:10.1016/j.jep.2016.07.033
Jiang, Z., Zhou, B., Li, X., Kirby, G. M., and Zhang, X. (2018). Echinacoside increases sperm quantity in rats by targeting the hypothalamic androgen receptor. Sci. Rep. 8 (1), 3839. doi:10.1038/s41598-018-22211-1
Kato, J. (1989). Arachidonic acid as a possible modulator of estrogen, progestin, androgen, and glucocorticoid receptors in the central and peripheral tissues. J. Steroid Biochem. 34 (1-6), 219–227. doi:10.1016/0022-4731(89)90085-x
Kitaya, K., and Yasuo, T. (2010). Aberrant expression of selectin E, CXCL1, and CXCL13 in chronic endometritis. Mod. Pathol. 23 (8), 1136–1146. doi:10.1038/modpathol.2010.98
Kuiper, G. G., Enmark, E., Pelto-Huikko, M., Nilsson, S., and Gustafsson, J. A. (1996). Cloning of a novel receptor expressed in rat prostate and ovary. Proc. Natl. Acad. Sci. U. S. A. 93 (12), 5925–5930. doi:10.1073/pnas.93.12.5925
Li, H., Zhou, H., Wang, D., Qiu, J., Zhou, Y., Li, X., et al. (2012). Versatile pathway-centric approach based on high-throughput sequencing to anticancer drug Discovery. Proc. Natl. Acad. Sci. U. S. A. 109 (12), 4609–4614. doi:10.1073/pnas.1200305109
Li, S., and Zhang, B. (2013). Traditional Chinese medicine network pharmacology: Theory, methodology and application. Chin. J. Nat. Med. 11 (2), 110–120. doi:10.1016/S1875-5364(13)60037-0
Mascarenhas, M. N., Flaxman, S. R., Boerma, T., Vanderpoel, S., and Stevens, G. A. (2012). National, regional, and global trends in infertility prevalence since 1990: A systematic analysis of 277 health surveys. PLoS Med. 9 (12), e1001356. doi:10.1371/journal.pmed.1001356
Messerlian, C., and Gaskins, A. J. (2017). Epidemiologic approaches for studying assisted reproductive technologies: Design, methods, analysis, and interpretation. Curr. Epidemiol. Rep. 4 (2), 124–132. doi:10.1007/s40471-017-0105-0
Nahid, L., and Sirous, K. (2012). Comparison of the effects of letrozole and Clomiphene citrate for ovulation induction in infertile women with polycystic ovary syndrome. Minerva Ginecol. 64 (3), 253–258.
Nolan, T., Hands, R. E., and Bustin, S. A. (2006). Quantification of mRNA using real-time RT-PCR. Nat. Protoc. 1 (3), 1559–1582. doi:10.1038/nprot.2006.236
Pelletier, G., and El-Alfy, M. (2000). Immunocytochemical localization of estrogen receptors alpha and beta in the human reproductive organs. J. Clin. Endocrinol. Metab. 85 (12), 4835–4840. doi:10.1210/jcem.85.12.7029
Petraglia, F., Serour, G. I., and Chapron, C. (2013). The changing prevalence of infertility. Int. J. Gynaecol. Obstet. 123, S4–S8. doi:10.1016/j.ijgo.2013.09.005
Quaas, A. M., and Legro, R. S. (2019). Pharmacology of medications used for ovarian stimulation. Best. Pract. Res. Clin. Endocrinol. Metab. 33 (1), 21–33. doi:10.1016/j.beem.2018.10.002
Reichman, D., and Rosenwaks, Z. (2017). The impact of genetic steroid disorders on human fertility. J. Steroid Biochem. Mol. Biol. 165, 131–136. doi:10.1016/j.jsbmb.2016.04.014
Revelli, A., Casano, S., Salvagno, F., and Delle Piane, L. (2011). Milder is better? Advantages and disadvantages of "mild" ovarian stimulation for human in vitro fertilization. Reprod. Biol. Endocrinol. 9, 25. doi:10.1186/1477-7827-9-25
Riese, D. J., and Cullum, R. L. (2014). Epiregulin: Roles in normal physiology and cancer. Semin. Cell Dev. Biol. 28, 49–56. doi:10.1016/j.semcdb.2014.03.005
Roth, L. W., Ryan, A. R., and Meacham, R. B. (2013). Clomiphene citrate in the management of male infertility. Semin. Reprod. Med. 31 (4), 245–250. doi:10.1055/s-0033-1345271
Ru, J., Li, P., Wang, J., Zhou, W., Li, B., Huang, C., et al. (2014). Tcmsp: A database of systems pharmacology for drug Discovery from herbal medicines. J. Cheminform. 6, 13. doi:10.1186/1758-2946-6-13
Rutstein, S. O., and Shah, I. H. (2004). “Infecundity, infertility, and childlessness in developing countries,” in DHS comparative reports No. 9 (Calverton, Maryland, USA: ORC Macro and the World Health Organization).
Ryter, S. W. (2019). Heme oxygenase-1/carbon monoxide as modulators of autophagy and inflammation. Arch. Biochem. Biophys. 678, 108186. doi:10.1016/j.abb.2019.108186
Saba, E., Lee, Y. Y., Kim, M., Hyun, S. H., Park, C. K., Son, E., et al. (2020). A novel herbal formulation consisting of red ginseng extract and Epimedium koreanum nakai-attenuated dextran sulfate sodium-induced colitis in mice. J. Ginseng Res. 44 (6), 833–842. doi:10.1016/j.jgr.2020.02.003
Scott, J. S., Bailey, A., Davies, R. D., Degorce, S. L., MacFaul, P. A., Gingell, H., et al. (2015). Tetrahydroisoquinoline phenols: Selective estrogen receptor downregulator antagonists with oral bioavailability in rat. ACS Med. Chem. Lett. 7 (1), 94–99. doi:10.1021/acsmedchemlett.5b00413
Scovell, J. M., and Khera, M. (2018). Testosterone replacement therapy versus Clomiphene citrate in the young hypogonadal male. Eur. Urol. Focus 4 (3), 321–323. doi:10.1016/j.euf.2018.07.033
Shannon, P., Markiel, A., Ozier, O., Baliga, N. S., Wang, J. T., Ramage, D., et al. (2003). Cytoscape: A software environment for integrated models of biomolecular interaction networks. Genome Res. 13 (11), 2498–2504. doi:10.1101/gr.1239303
Shao, W., Li, S., Li, L., Lin, K., Liu, X., Wang, H., et al. (2019). Chemical genomics reveals inhibition of breast cancer lung metastasis by ponatinib via C-jun. Protein Cell 10 (3), 161–177. doi:10.1007/s13238-018-0533-8
Shiau, A. K., Barstad, D., Radek, J. T., Meyers, M. J., Nettles, K. W., Katzenellenbogen, B. S., et al. (2002). Structural characterization of a subtype-selective ligand reveals a novel mode of estrogen receptor antagonism. Nat. Struct. Biol. 9 (5), 359–364. doi:10.1038/nsb787
Szczuko, M., Kikut, J., Komorniak, N., Bilicki, J., Celewicz, Z., Ziętek, M., et al. (2020). The role of arachidonic and linoleic acid derivatives in pathological pregnancies and the human reproduction process. Int. J. Mol. Sci. 21 (24), 9628. doi:10.3390/ijms21249628
Tang, Z. R., Zhang, R., Lian, Z. X., Deng, S. L., and Yu, K. (2019). Estrogen-receptor expression and function in female reproductive disease. Cells 8 (10), 1123. doi:10.3390/cells8101123
Tong, J. S., Zhang, Q. H., Huang, X., Fu, X. Q., Qi, S. T., Wang, Y. P., et al. (2011). Icaritin causes sustained ERK1/2 activation and induces apoptosis in human endometrial cancer cells. PloS one 6 (3), e16781. doi:10.1371/journal.pone.0016781
Vander Borght, M., and Wyns, C. (2018). Fertility and infertility: Definition and epidemiology. Clin. Biochem. 62, 2–10. doi:10.1016/j.clinbiochem.2018.03.012
Wan, Y., and Qi, C. (2017). The mechanism of oxidative stress in infertility and the research progress of Chinese and western medicine treatment. Hebei J. Traditional Chin. Med. 39 (5), 788–796. Chinese.
Wang, X., Zhen, L., Zhang, G., Wong, M. S., Qin, L., Yao, X., et al. (2011). Osteogenic effects of flavonoid aglycones from an osteoprotective fraction of Drynaria fortuneian in vitro efficacy study. Phytomedicine 18 (10), 868–872. doi:10.1016/j.phymed.2011.01.022
Wang, M., Wang, Q., Du, Y., Jiang, H., and Zhang, X. (2020). Vitamins combined with traditional Chinese medicine for male infertility: A systematic review and meta-analysis. Andrology 8 (5), 1038–1050. doi:10.1111/andr.12787
Warinrak, C., Wu, J. T., Hsu, W. L., Liao, J. W., and Cheng, F. P. (2015). Expression of matrix metalloproteinases (MMP-2, MMP-9) and their inhibitors (TIMP-1, TIMP-2) in canine testis, epididymis and semen. Reprod. Domest. Anim. 50 (1), 48–57. doi:10.1111/rda.12448
Wu, Z., Yao, H., Xu, H., Wang, Y., Hu, W., Lou, G., et al. (2020). Inhibition of enos by L-NAME resulting in rat hind limb developmental defects through PFKFB3 mediated angiogenetic pathway. Sci. Rep. 10 (1), 16754. doi:10.1038/s41598-020-74011-1
Keywords: wenshenyang recipe, infertility, network pharmacology, transcriptome analysis, GO enrichment analysis, pathway enrichment analysis, molecular docking
Citation: Xie L, Zhao S, Zhang X, Huang W, Qiao L, Zhan D, Ma C, Gong W, Dang H and Lu H (2022) Wenshenyang recipe treats infertility through hormonal regulation and inflammatory responses revealed by transcriptome analysis and network pharmacology. Front. Pharmacol. 13:917544. doi: 10.3389/fphar.2022.917544
Received: 11 April 2022; Accepted: 11 July 2022;
Published: 08 August 2022.
Edited by:
Ren-You Gan, Chinese Academy of Agricultural Sciences, ChinaReviewed by:
Jayaprakash N Kolla, Institute of Molecular Genetics (ASCR), CzechiaCopyright © 2022 Xie, Zhao, Zhang, Huang, Qiao, Zhan, Ma, Gong, Dang and Lu. This is an open-access article distributed under the terms of the Creative Commons Attribution License (CC BY). The use, distribution or reproduction in other forums is permitted, provided the original author(s) and the copyright owner(s) are credited and that the original publication in this journal is cited, in accordance with accepted academic practice. No use, distribution or reproduction is permitted which does not comply with these terms.
*Correspondence: Lan Xie, eGllbGFuQHRzaW5naHVhLmVkdS5jbg==
†These authors contributed equally to this work
Disclaimer: All claims expressed in this article are solely those of the authors and do not necessarily represent those of their affiliated organizations, or those of the publisher, the editors and the reviewers. Any product that may be evaluated in this article or claim that may be made by its manufacturer is not guaranteed or endorsed by the publisher.
Research integrity at Frontiers
Learn more about the work of our research integrity team to safeguard the quality of each article we publish.