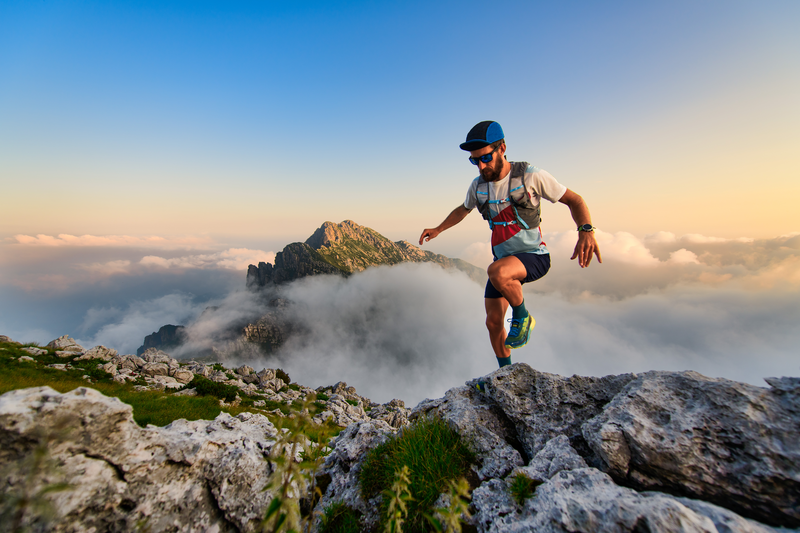
95% of researchers rate our articles as excellent or good
Learn more about the work of our research integrity team to safeguard the quality of each article we publish.
Find out more
REVIEW article
Front. Pharmacol. , 11 October 2022
Sec. Experimental Pharmacology and Drug Discovery
Volume 13 - 2022 | https://doi.org/10.3389/fphar.2022.914710
This article is part of the Research Topic Recent Pharmacological Innovation for the Opioid Crisis View all 9 articles
Perioperative multimodal analgesia can reduce the side effects of a high concentration of opioids, improving the comfort of the patient. However, insufficient analgesia of this model has prompted researchers to explore new adjuvant analgesics. Recently, an increasing number of studies have found a low-grade analgesic effect in the clinical application of ultra-short-acting β-adrenergic receptor antagonists, which are conventionally used as pharmacologic agents in the cardiovascular system. The mechanism by which ultra-short-acting β-antagonists exert antinociceptive effects has not been clarified yet. In this review, we intend to address its potential reasons from the side of neurotransmitters, inflammatory cytokines, and signaling pathways, providing theoretical proof for the application of β-adrenergic receptor antagonists in analgesia.
The International Association for the Study of Pain revised the definition of pain as “an unpleasant sensory and emotional experience associated with, or resembling that associated with, actual or potential tissue damage” (Raja et al., 2020). Surgery is a type of actual tissue injury, and postoperative pain is an important factor affecting patient recovery. Opioids can produce powerful analgesic effects, making them widely used in clinics for the treatment of perioperative and chronic pain (Tamara King, 2005). However, repeated administration of opioids can result in intraoperative acute, opioid-induced hyperalgesia, nausea, dizziness, and respiratory depression, significantly limiting extensive clinical use (Zhang et al., 2015; Ueda, 2004; Yu et al., 2016). Thus, multimodal analgesia, which can reduce the side effects of opioids and improve patient comfort, has developed as the main perioperative analgesia model (Gelman et al., 2018). Typical perioperative multimodal analgesics include opioids and nonsteroidal anti-inflammatory drugs (NSAIDs). However, NSAIDs are often associated with an increased risk of gastrointestinal, renal, and cardiovascular toxicities, thus limiting their application in patients with gastrointestinal and cardiovascular problems. The need for a new multimodal analgesic strategy that minimizes the side effects of opioids and improves the prognosis of the patient has become a serious clinical issue.
Ultra-short-acting β-blockers, including esmolol, landiolol, and flestolol, mainly act on myocardial β1 receptors but also block β2 receptors at high doses. These drugs are often used to treat angina pectoris, myocardial infarction, arrhythmia, hypertension, and other cardiovascular diseases (Gorczynski, 1985). Recently, many studies found that β-blockers administered intraoperatively may possess analgesic properties (Supplementary Table S1). Perioperative use of these drugs reduces the inhalation anesthetic and opioid dose requirement (Chia et al., 2004; Tamara King, 2005). Perioperative β-blockade in elderly surgical patients has some advantages, including maintained hemodynamic stability, improved physical sense of well-being, and decreased analgesic requirements (Zaugg et al., 1999). Studies investigating the antinociceptive effect of β-receptor antagonists have increased in number, but the role of β-receptor antagonists in the modulation of postoperative pain remains unclear. In this review, we summarize previous findings and review several possible mechanisms that may explain the clinical value of β-antagonists as analgesics.
Cardiac output (CO) can affect liver blood flow, which in turn affects the metabolism of drugs with a high liver extraction rate (Weiss et al., 1996). Kurita et al. (2013) studied the effect of changes in CO on the plasma concentration of remifentanil under pseudo-steady-state conditions. The results showed that the plasma concentration of remifentanil was inversely proportional to cardiac output. CO is negatively correlated with the initial targeted concentration of intravenously administered drugs (Fagiolino, 2002). Birkholz et al. (2018) found that CO can influence the pharmacokinetics of sufentanil. They suggested that the dosage of opioids should be adjusted according to the case of changed CO to avoid inadequate drug effects or prolonged recovery. In addition, the decrease in CO can reduce the dosage of intraoperative fentanyl and remifentanil (Henthorn et al., 1992; Kurita et al., 2013). β-Adrenergic antagonists can slow down the heart rate and reduce the contractility of the heart muscle by inhibiting the β1 receptor. Afify and Andijani, (2017) demonstrated that the use of β-blockers enhanced the analgesic effect of morphine in a mechanism that involved decreased CO. This phenomenon has been confirmed in clinical studies: one report suggested that perioperative esmolol administration can reduce the intraoperative use of inhalation anesthetic and fentanyl and morphine consumption for the first three postoperative days (Chia et al., 2004). In addition, Morais et al. (2020) found that intraoperative esmolol can promote the reduction in pain intensity and the need for analgesic supplementation. In septoplasty surgery, the amount of remifentanil could be reduced when the heart rate was significantly decreased by esmolol (Gökçe et al., 2009). Thus, clinical research suggests that β-blockers can reduce the dose of perioperative opioids and intraoperatively inhaled anesthetics by reducing CO.
Tissue damage, such as that from surgical incisions, can lead to inflammation and exacerbate the pain state of patients with pain (Brennan et al., 1996). This pain state results from the production and release of inflammatory mediators, such as prostaglandin E2 (PGE2) and pro-inflammatory cytokines. Pro-inflammatory cytokines are small molecular polypeptides synthesized and secreted by both immune and non-immune cells in the body. They regulate a variety of cellular physiological functions and play an important role under stressful conditions such as trauma, pain, and infection (de Oliveira et al., 2011). Examples of pro-inflammatory cytokines include interleukin (IL)-6, IL-8, and IL-13. These pro-inflammatory factors are released by glial cells or neurons and play important roles in the nervous system, participating in the development of acute or chronic pain (Cunha et al., 2005; Cunha et al., 2010). IL-1β and IL-6 have higher activity among the pro-inflammatory cytokines (Shyong et al., 2003) (Figure 1). Injecting IL-1β in the mouse abdominal region, ventricles, or plantar surface can cause hyperalgesia (Perkins and Kelly, 1994; Watkins et al., 1994). IL-1β can regulate pain by directly binding to transient receptor potential vanilloid type 1 (TRPV1) (Zhou et al., 2016). Phosphorylation of TRPV1 increases action potential firing, heightening the sensitivity to thermal or mechanical stimulation (Pinho-Ribeiro et al., 2017). In in vivo, the level of IL-6 is positively correlated with pain intensity and is related to the formation and development of neuropathic pain (Ramer et al., 1998; Yan et al., 2012). Malsch et al. (2014) suspected that IL-6 directly affects nociceptors in sensory neurons. The researchers decreased the pain threshold through the knockout of IL-6 receptors in sensory neurons. Intraplantar TNF-α was also reported to produce mechanical and thermal hyperalgesia induced by inflammation (Perkins and Kelly, 1994). TNF-α activates the p38 MAPK pathway in dorsal root ganglion (DRG) neurons to promote Na+ influx and reduce the excitability threshold (Jin and Gereau, 2006).
FIGURE 1. IL-6 and IL-1β produce pain sensitization. IL-1β binds to IL-1R, and IL-6 activates IL-6R. They can regulate pain by increasing the expression of TRPV1. The activation of TRPV1 increases action potential firing, heightening the sensitivity to pain. IL-1R: IL-1 receptor. IL-6R: IL-6 receptor. TRPV1: transient receptor potential vanilloid type 1.
Activated β-receptors can increase the release of pro-inflammatory cytokines, such as IL-1β, IL-6, and TNF-α, and inhibiting these receptors can reduce the systemic and local inflammatory responses (Tan et al., 2021). Perioperative serum levels of IL-4 and IL-6 in patients undergoing laparoscopic gastrectomy were increased, and intravenous injection of esmolol reduced the increase of these inflammatory cytokines (Kim et al., 2015). Landiolol has also been shown to play a protective role in systemic inflammation by reducing serum levels of TNF (Hagiwara et al., 2009). Horikoshi et al. (2017) demonstrated that the application of landiolol can inhibit the increase of perioperative plasma IL-6 levels in patients undergoing esophageal cancer surgery. The pro-inflammatory cytokines such as IL-1β, IL-6, and TNF-α can increase the transmission of pain signals by reducing the action potential threshold. The inhibitory effect of β-blockers on the inflammatory response may alleviate their stimulating effect on perioperative pain.
Nociception signals can transmit from primary afferent fibers to the central nervous system. The cell bodies of the primary afferent fibers responsible for the signal transmission are located in the DRG (Comitato and Bardoni, 2021). There are many excitatory ion channels on the membranes of DRG primary afferent fibers, such as voltage-dependent Na+, K+, and Ca2+ channels and the transient receptor potential channel. Blocking these channels in the DRG can reduce the transmission of pain signals and influence analgesia (Moravcikova et al., 2018; Hermanns et al., 2019). Peripheral nociceptors are stimulated to produce action potentials, and afferent fibers of the DRG transmit these signals to the spinal cord dorsal horn. The latter contains various subtypes of excitatory or inhibitory interneurons that can interact with each other to integrate pain-associated signals from primary afferent fibers and transmit these signals to neurons in the brain.
Tetrodotoxin-resistant sodium (TTX-rNa+) channels mainly exist in the DRG (Akopian et al., 1999). They can regulate the excitability of primary afferent neurons, influencing the transmission of nociception signals (Gold, 1999). Inflammatory mediators such as PGE2 continuously activate TTX-rNa+ channels through activation of the cAMP pathway and increase neuronal excitability (Li and Schild, 2007). IL-1β increases the excitability of pain receptors by alleviating the slow resting inactivation of the TTX-rNa+ channel and enhancing the persistent near-threshold TTX-rNa+ current (Binshtok et al., 2008). When several inflammatory mediators released in response to injury sensitize subpopulations of primary afferent neurons, blocking TTX-rNa+ channels can inhibit inflammatory mediator-induced modulation of current, indicating the important role of TTX-rNa+ channels in the treatment of inflammatory pain and hyperalgesia (Gold and Levine, 1996). In in vitro, esmolol can block the TTX-rNa+ channel current in a dose-dependent manner, and high concentrations of landiolol can also inhibit TTX-rNa+ channel activity (Tanahashi et al., 2009). These results suggested that β-adrenergic receptor antagonists can exert antinociceptive effects by blocking TTX-rNa+ channels. Lidocaine can bind to the S6 segment of domain 4 on the Na+ channel α-subunit in TTX-rNa+ channels (Tanahashi et al., 2007) and inhibit signal transmission. The electrophysiological characteristics of β-adrenergic receptor antagonists are similar to those of lidocaine (Wagner et al., 1999; Kim et al., 2000), which provides basic evidence for the local application of β-receptor blockers.
A report on in vivo administration of esmolol alone explored the effect of intrathecal administration in a postoperative pain model (Ono et al., 2015). The postoperative pain model was established with the use of a plantar incision. Withdrawal latencies were assessed by applying a focused radiant heat source to explore the antinociceptive effect of esmolol. The results suggested that plantar incision produced hypersensitivity in the postoperative pain model expressed as decreased withdrawal latency to heat stimulation. The decreased latencies caused by plantar incision were significantly increased by esmolol intrathecal administration after 5 min but not after 10 or 15 min. These results indicated that intrathecal administration of esmolol alone produced antinociceptive effects of short duration in a rat postoperative pain model. In addition, an inflammatory pain model was established with intraplantar injection of formalin. The expression of c-Fos (a marker of neuronal activation, including that induced by pain) was detected after formalin administration. The researchers found that intrathecal landiolol reduced the expression of c-Fos on the injection side, suggesting that intrathecal landiolol alone produces analgesic effects on inflammatory pain (Zhao et al., 2007; Mizobuchi et al., 2012). These studies indicate that intrathecal injection of β-receptor antagonists provides a new method to control perioperative pain. However, the clinical application and safety need to be further studied.
G-protein-coupled receptors (GPCRs) comprise seven α-helical-structured transmembrane domains (TMs), an extracellular N-terminus, and an intracellular C-terminus (Gregory V Nikiforovich et al., 2007). In intracellular regions, GPCRs consist of heterotrimeric G-protein complexes, which contain Gα, Gβ, and Gγ subunits (Wess, 2022). According to their structure and function, Gα proteins are classified into four subfamilies (Gs, Gi/o, Gq/11, and G12/13) (Inoue et al., 2019). Different ligands acting on the extracellular side of GPCRs can change the TM and intracellular regions of the receptors (Wang et al., 2004). The effect on the latter may have different effects on downstream signal cascades. For example, Gs and Gi/o have opposite effects on adenylate cyclase (AC), which synthesizes cyclic adenosine monophosphate (cAMP) to activate protein kinase A (PKA) (Wess, 2022). Gs activates the process, and Gi/o inhibits the production of cAMP. The results of a human study showed that norepinephrine prolonged capsaicin-induced skin sensitivity (Drummond, 1995). In addition, Khasar et al. showed that adrenaline can cause skin mechanical hyperalgesia and sensitize DRG neurons. These effects of adrenaline are mediated by the PKA and PKC pathways.
Studies show that the cAMP pathway may produce hyperalgesia and its inhibition can increase the pain threshold (Song et al., 2006; Shao et al., 2016). This finding means that the interaction between Gs and Gi/o directly affects the transmission of pain signals. Opioid peptide receptors (OPRs) and β-adrenergic receptors (β-ARs) belong to the GPCR family and can mediate similar cellular signal transduction cascades (Mitrovic et al., 2003; Zheng et al., 2010). β-ARs are Gs-coupled receptors whose stimulation promotes the activation of AC and PKA (Pepe et al., 2004). OPRs are Gi/o-coupled receptors whose stimulation inhibits AC activity to reduce PKA activation (Law and Loh, 1999). Research shows that β-blockade prevents the stimulatory effect on the AC enzyme, reduces the number of cAMP molecules, enhances the inhibitory effect of opioids on pain transmission, and provides an antinociceptive response (Afify and Andijani, 2017). These findings may be the result of Gs stimulation weakening the impact on Gi/o (Figure 2). This provides a basis for β-receptor blockers to enhance the analgesic effect of opioids.
FIGURE 2. Scheme for crosstalk between opioid and β-adrenergic receptors. Stimulatory effects are indicated in green lines and inhibitory effects in red lines. Agonist acting on β-AR activates AC and stimulates PKA. Opioids combined with OPR inhibit AC and reduce the level of cAMP, which decreases the recruitment of PKA. β-Blockade prevents the stimulatory effect on AC enzyme and reduces the number of cAMPs. β-AR: β-adrenergic receptors. AC: adenylate cyclase. PKA: protein kinase A. OPRs: opioid peptide receptors. cAMP: cyclic adenosine monophosphate. Gs: stimulatory G protein. Gi: inhibitory G protein.
Facial somatosensory nerves project to multiple regions of the brain stem trigeminal nucleus complex (Fagiolino, 2002). This complex is divided into three regions that are, respectively, responsible for pain, touch, and temperature sensation. The spinal trigeminal nucleus (Sp5c), one part of the complex, is the primary site of reception and modulation of thermosensitive information. Its nociceptive signals arise from the cranio-orofacial regions. Sp5c has an important function in the modulation of nociceptive information (Takemura et al., 2006; Davies and North, 2009). In the resting state, neurotransmitters released by spontaneous exocytosis of single synaptic vesicles from the presynaptic membrane change the postsynaptic membrane current. Postsynaptic currents can be divided into miniature inhibitory postsynaptic currents (mIPSCs) and miniature excitatory postsynaptic currents (mEPSCs) according to the nature of the neurotransmitters that induced them (Chiang et al., 2021). Changes in these micro-currents also affect synaptic plasticity (Gonzalez-Islas et al., 2018). One report relayed the effect of esmolol’s role in Sp5c in vitro (Yasui et al., 2011). Brain stem slices containing Sp5c were placed in artificial cerebrospinal fluid with different Ca2+ concentrations. The role of Ca2+ in synaptic signal transmission was confirmed using esmolol intervention through recording mIPSCs and mEPSCs in synapses. The results showed that esmolol selectively increased the frequency of mIPSCs without affecting mEPSCs. It was suspected that esmolol produced analgesia in vitro by promoting the transmission of inhibitory signaling and reducing the transmission of nociceptive information to the nervous system. Esmolol increased the frequency of mIPSCs through a mechanism involving Ca2+ entry in a β-adrenoceptor-independent manner. The effect of β-receptor antagonists on pain signal transmission in Sp5c provides a new therapeutic strategy for the treatment of trigeminal neuralgia.
At present, opioids still play an important role in clinical analgesia programs. However, there is an urgent clinical need to seek an ideal multimodal analgesia program that reduces the side effects of opioids and adjuvants while maintaining the ideal analgesic effects. Some clinical studies found that intravenous injection β-receptor blockers can reduce the consumption of opioids during the perioperative period and improve patient prognoses. This review discusses some potential analgesic mechanisms of β-blockers: 1) reduction of CO affects pharmacokinetics; 2) inhibition of the release of pro-inflammatory cytokines; 3) effects on the excitation transmission of the nervous system; 4) activation of GPCR cascades to produce analgesic effects; and 5) modulation of inhibitory transmitter release in the spinal trigeminal nucleus. Until now, only a few studies have found an effect of β-receptor blockers on analgesia. Landiolol and esmolol, two of the most studied ultra-short-acting β-receptor blocker drugs, have not been fully confirmed to act through the aforementioned possible mechanisms. In addition, animal experiments revealed that the analgesic effect lasted only a few minutes because the half-life of β-receptor blockers is short and the time for them to exert their analgesic effect is limited. However, the patient-controlled analgesia scheme will not limit their long-term clinical application. Although intravenously injected β-receptor blockers produce analgesic effects, clinical studies have also found that oral β-receptor blockers can reduce the dosage of opioid analgesics after the operation and reduce the adverse reactions caused by the latter. In addition, intrathecal injection of β-receptor blockers can also play an analgesic role by interfering with spinal cord neurotransmission. Perioperative multimodal analgesia is the basis for ensuring the safety of each combination of drugs and maximizing analgesic effects. Although preclinical and clinical studies have confirmed that β-receptor blockers can produce analgesia alone and assist other drugs in inducing analgesia, the mechanism of their specific participation in analgesia and the effectiveness of the route of administration still need to be further verified. In summary, this review provides evidence for the application of feasible alternative analgesics in perioperative multimodal analgesia.
LF wrote the first draft of the manuscript. BY assessed the data. ZL and ZHL contributed to the manuscript revision. All authors contributed to the conception and design of the study, contributed to the manuscript, and approved the submitted version.
This study was supported by the National Natural Science Foundation of China (No. 82101308), Shenzhen High-level Hospital Construction Fund and the Sanming Project of Medicine in Shenzhen (SZSM201612026).
The authors declare that the research was conducted in the absence of any commercial or financial relationships that could be construed as a potential conflict of interest.
All claims expressed in this article are solely those of the authors and do not necessarily represent those of their affiliated organizations, or those of the publisher, the editors, and the reviewers. Any product that may be evaluated in this article, or claim that may be made by its manufacturer, is not guaranteed or endorsed by the publisher.
The Supplementary Material for this article can be found online at: https://www.frontiersin.org/articles/10.3389/fphar.2022.914710/full#supplementary-material
Afify, E. A., and Andijani, N. M. (2017). Potentiation of morphine-induced antinociception by propranolol: The involvement of dopamine and GABA systems. Front. Pharmacol. 8, 794. doi:10.3389/fphar.2017.00794
Akopian, A. N., Souslova, V., England, S., Okuse, K., Ogata, N., Ure, J., et al. (1999). The tetrodotoxin-resistant sodium channel SNS has a specialized function in pain pathways. Nat. Neurosci. 2, 541–548. doi:10.1038/9195
Binshtok, A. M., Wang, H., Zimmermann, K., Amaya, F., Vardeh, D., Shi, L., et al. (2008). Nociceptors are interleukin-1beta sensors. J. Neurosci. 28, 14062–14073. doi:10.1523/JNEUROSCI.3795-08.2008
Birkholz, T., Leuthold, C., Schmidt, J., Ihmsen, H., Schuttler, J., and Jeleazcov, C. (2018). Influence of cardiac output on the pharmacokinetics of sufentanil in anesthetized pigs. Anesthesiology 128, 912–920. doi:10.1097/ALN.0000000000002160
Brennan, T. J., Vandermeulen, E. P., and Gebhart, G. F. (1996). Characterization of a rat model of incisional pain. Pain 64, 493–501. doi:10.1016/0304-3959(95)01441-1
Chia, Y. Y., Chan, M. H., Ko, N. H., and Liu, K. (2004). Role of beta-blockade in anaesthesia and postoperative pain management after hysterectomy. Br. J. Anaesth. 93, 799–805. doi:10.1093/bja/aeh268
Chiang, C. W., Shu, W. C., Wan, J., Weaver, B. A., and Jackson, M. B. (2021). Recordings from neuron-HEK cell cocultures reveal the determinants of miniature excitatory postsynaptic currents. J. Gen. Physiol. 153, e202012849. doi:10.1085/jgp.202012849
Comitato, A., and Bardoni, R. (2021). Presynaptic inhibition of pain and touch in the spinal cord: From receptors to circuits. Int. J. Mol. Sci. 22, E414. doi:10.3390/ijms22010414
Cunha, T. M., Talbot, J., Pint, L. G., Vieira, S. M., Souza, G. R., Guerrero, A. T., et al. (2010). Caspase-1 is involved in the Genesis of inflammatory hypernociception by contributing to peripheral IL-1β maturation. Mol. Pain 4, 63. doi:10.1186/1744-8069-6-63
Cunha, T. M., Verri, W. A., Silva, J. S., Poole, S., Cunha, F. Q., and Ferreira, S. H. (2005). A cascade of cytokines mediates mechanical inflammatory hypernociception in mice. Proc. Natl. Acad. Sci. U. S. A. 102, 1755–1760. doi:10.1073/pnas.0409225102
Davies, A. J., and North, R. A. (2009). Electrophysiological and morphological properties of neurons in the substantia gelatinosa of the mouse trigeminal subnucleus caudalis. Pain 146, 214–221. doi:10.1016/j.pain.2009.07.038
De oliveira, C. M. B., Sakata, R. K., Issy, A. M., Gerola, L. R., and Salomao, r. (2011). Cytokines and pain. Braz. J. Anesthesiol. 61, 255–265. doi:10.1016/s0034-7094(11)70029-0
Drummond, P. D. (1995). Noradrenaline increases hyperalgesia to heat in skin sensitized by capsaicin. Pain 60, 311–315. doi:10.1016/0304-3959(94)00130-7
Fagiolino, P. (2002). The influence of cardiac output distribution on the tissue/plasma drug concentration ratio. Eur. J. Drug Metab. Pharmacokinet. 27, 79–81. doi:10.1007/BF03190420
Gelman, D., Gelmanas, A., Urbanaite, D., Tamosiunas, R., Sadauskas, S., Bilskiene, D., et al. (2018). Role of Multimodal Analgesia in the Evolving Enhanced Recovery after Surgery Pathways, Med. Kaunas., 54.20, doi:10.3390/medicina54020020
Gokçe, B. M., Karabiyik, L., and Karadenizli, Y. (2009). Hypotensive anesthesia with esmolol. Assessment of hemodynamics, consumption of anesthetic drugs, and recovery. Saudi Med. J. 30, 771–777.
Gold, M. S., and Levine, J. D. (1996). DAMGO inhibits prostaglandin E2-induced potentiation of a TTX-resistant Na+ current in rat sensory neurons in vitro Neurosci. Lett. 212, 83–86. doi:10.1016/0304-3940(96)12791-9
Gold, M. S. (1999). Tetrodotoxin-resistant Na+ currents and inflammatory hyperalgesia. Proc. Natl. Acad. Sci. U. S. A. 96, 7645–7649. doi:10.1073/pnas.96.14.7645
Gonzalez-islas, C., Bulow, P., and Wenner, P. (2018). Regulation of synaptic scaling by action potential-independent miniature neurotransmission. J. Neurosci. Res. 96, 348–353. doi:10.1002/jnr.24138
Gorczynski, R. J. (1985). Basic pharmacology of esmolol. Am. J. Cardiol. 56, 3F–13F. doi:10.1016/0002-9149(85)90910-5
Hagiwara, S., Iwasaka, H., Maeda, H., and Noguchi, T. (2009). Landiolol, an ultrashort-acting beta1-adrenoceptor antagonist, has protective effects in an LPS-induced systemic inflammation model. Shock 31, 515–520. doi:10.1097/SHK.0b013e3181863689
Henthorn, T. K., Krejcie, T. C., and Avram, M. J. (1992). The relationship between alfentanil distribution kinetics and cardiac output. Clin. Pharmacol. Ther. 52, 190–196. doi:10.1038/clpt.1992.129
Hermanns, H., Hollmann, M. W., Stevens, M. F., Lirk, P., Brandenburger, T., Piegeler, T., et al. (2019). Molecular mechanisms of action of systemic lidocaine in acute and chronic pain: A narrative review. Br. J. Anaesth. 123, 335–349. doi:10.1016/j.bja.2019.06.014
Horikoshi, Y., Goyagi, T., Kudo, R., Kodama, S., Horiguchi, T., and Nishikawa, T. (2017). The suppressive effects of landiolol administration on the occurrence of postoperative atrial fibrillation and tachycardia, and plasma IL-6 elevation in patients undergoing esophageal surgery: A randomized controlled clinical trial. J. Clin. Anesth. 38, 111–116. doi:10.1016/j.jclinane.2017.01.036
Inoue, A., Raimondi, F., Kadji, F. M. N., Singh, G., Kishi, T., Uwamizu, A., et al. (2019). Illuminating G-protein-coupling selectivity of GPCRs. Cell 177, 1933–1947. doi:10.1016/j.cell.2019.04.044
Jin, X., and Gereau, R. W. T. (2006). Acute p38-mediated modulation of tetrodotoxin-resistant sodium channels in mouse sensory neurons by tumor necrosis factor-alpha. J. Neurosci. 26, 246–255. doi:10.1523/JNEUROSCI.3858-05.2006
Khasar, S. G., Mccarter, G., and Levine, J. D. (1999). Epinephrine produces a beta-adrenergic receptor-mediated mechanical hyperalgesia and in vitro sensitization of rat nociceptors. J. Neurophysiol. 81 (3), 1104–1112. doi:10.1152/jn.1999.81.3.1104
Kim, Y., Hwang, W., Cho, M. L., Her, Y. M., Ahn, S., and Lee, J. (2015). The effects of intraoperative esmolol administration on perioperative inflammatory responses in patients undergoing laparoscopic gastrectomy: A dose-response study. Surg. Innov. 22, 177–182. doi:10.1177/1553350614532534
Kim, Y. S., shin, Y. K., Lee, C., and Song, J. (2000). Block of sodium currents in rat dorsal root ganglion neurons by diphenhydramine. Brain Res. 881, 190–198. doi:10.1016/s0006-8993(00)02860-2
Kurita, T., Uraoka, M., Jiang, Q., Suzuki, M., Morishima, Y., Morita, K., et al. (2013). Influence of cardiac output on the pseudo-steady state remifentanil and propofol concentrations in swine. Acta Anaesthesiol. Scand. 57, 754–760. doi:10.1111/aas.12076
Law, P. Y., and Loh, H. H. (1999). Regulation of opioid receptor activities. J. Pharmacol. Exp. Ther. 289, 607–624.
Li, B., and Schild, J. H. (2007). Persistent tetrodotoxin-resistant Na+ currents are activated by prostaglandin E2 via cyclic AMP-dependent pathway in C-type nodose neurons of adult rats. Biochem. Biophys. Res. Commun. 355, 1064–1068. doi:10.1016/j.bbrc.2007.02.086
Malsch, P., Andratsch, M., Vogl, C., Link, A. S., Alzheimer, C., Brierley, S. M., et al. (2014). Deletion of interleukin-6 signal transducer gp130 in small sensory neurons attenuates mechanonociception and down-regulates TRPA1 expression. J. Neurosci. 34, 9845–9856. doi:10.1523/JNEUROSCI.5161-13.2014
Mitrovic, I., Margeta-Mitrovic, M., Bader, S., Stoffel, M., Jan, L. Y., and Basbaum, A. I. (2003). Contribution of GIRK2-mediated postsynaptic signaling to opiate and alpha 2-adrenergic analgesia and analgesic sex differences. Proc. Natl. Acad. Sci. U. S. A. 100, 271–276. doi:10.1073/pnas.0136822100
Mizobuchi, S., Matsuoka, Y., Obata, N., Kaku, R., Itano, Y., Tomotsuka, N., et al. (2012). Antinociceptive effects of intrathecal landiolol injection in a rat formalin pain model. Acta Med. Okayama 66, 285–289. doi:10.18926/AMO/48569
Morais, V. B. D., Sakata, R. K., Huang, A. P. S., and Ferraro, L. (2020). Randomized, double-blind, placebo-controlled study of the analgesic effect of intraoperative esmolol for laparoscopic gastroplasty. Acta Cir. Bras. 35, e202000408. doi:10.1590/s0102-865020200040000008
Moravcikova, L., Kralovicova, J., and Lacinova, L. (2018). SNC80 and naltrindole modulate voltage-dependent sodium, potassium and calcium channels via a putatively delta opioid receptor-independent mechanism. Gen. Physiol. Biophys. 37, 299–307. doi:10.4149/gpb_2018009
Ono, H., Ohtani, N., Matoba, A., Kido, K., Yasui, Y., and Masaki, E. (2015). Efficacy of intrathecal esmolol on heat-evoked responses in a postoperative pain model. Am. J. Ther. 22, 111–116. doi:10.1097/MJT.0b013e318274e0a1
Pepe, S., Van den brink, O. W., Lakatta, E. G., and Xiao, R. P. (2004). Cross-talk of opioid peptide receptor and beta-adrenergic receptor signalling in the heart. Cardiovasc. Res. 63, 414–422. doi:10.1016/j.cardiores.2004.04.022
Perkins, M. N., and Kelly, D. (1994). Interleukin-1 beta induced-desArg9bradykinin-mediated thermal hyperalgesia in the rat. Neuropharmacology 33, 657–660. doi:10.1016/0028-3908(94)90171-6
Pinho-ribeiro, F. A., Verri, W. A., and Chiu, I. M. (2017). Nociceptor sensory neuron-immune interactions in pain and inflammation. Trends Immunol. 38, 5–19. doi:10.1016/j.it.2016.10.001
Raja, S. N., Carr, D. B., Cohen, M., Finnerup, N. B., Flor, H., Gibson, S., et al. (2020). The revised international association for the study of pain definition of pain: Concepts, challenges, and compromises. Pain 161, 1976–1982. doi:10.1097/j.pain.0000000000001939
Ramer, M. S., Murphy, P. G., Richardson, P. M., and Bisby, M. A. (1998). Spinal nerve lesion-induced mechanoallodynia and adrenergic sprouting in sensory ganglia are attenuated in interleukin-6 knockout mice. Pain 78, 115–121. doi:10.1016/s0304-3959(98)00121-3
Shao, X. M., Sun, J., Jiang, Y. L., Liu, B. Y., Shen, Z., Fang, F., et al. (2016). Inhibition of the cAMP/PKA/CREB pathway contributes to the analgesic effects of electroacupuncture in the anterior cingulate cortex in a rat pain memory model. Neural Plast., 2016, 5320641. doi:10.1155/2016/5320641
Shyong, E. Q., Lucchinetti, E., Tagliente, T. M., Hossain, S., Silverstein, J. H., and Zaugg, M. (2003). Interleukin balance and early recovery from anesthesia in elderly surgical patients exposed to β-adrenergic antagonism. J. Clin. Anesth. 15, 170–178. doi:10.1016/s0952-8180(03)00033-3
Song, X. J., Wang, Z. B., Gan, Q., and Walters, E. T. (2006). cAMP and cGMP contribute to sensory neuron hyperexcitability and hyperalgesia in rats with dorsal root ganglia compression. J. Neurophysiol. 95, 479–492. doi:10.1152/jn.00503.2005,
Takemura, M., Sugiyo, S., Moritani, M., Kobayashi, M., and Yonehara, N. (2006). Mechanisms of orofacial pain control in the central nervous system. Arch. Histol. Cytol. 69, 79–100. doi:10.1679/aohc.69.79
Tamara king, M. H. O., Toddvanderah, W., Frank, P., Lai, J., and Lai, J. (2005). Is paradoxical pain induced by sustained opioid exposure an underlying mechanism of opioid antinociceptive tolerance? Neurosignals. 14, 194–205. doi:10.1159/000087658
Tan, S., Zhou, F., Zhang, Z., Wang, J., Xu, J., Zhuang, Q., et al. (2021). Beta-1 blocker reduces inflammation and preserves intestinal barrier function after open abdominal surgery. Surgery 169, 885–893. doi:10.1016/j.surg.2020.11.004
Tanahashi, S., Iida, H., Dohi, S., Oda, A., Osawa, Y., and Yamaguchi, S. (2009). Comparative effects of ultra-short-acting beta1-blockers on voltage-gated tetrodotoxin-resistant Na+ channels in rat sensory neurons. Eur. J. Anaesthesiol. 26, 196–200. doi:10.1097/EJA.0b013e32831ac268
Tanahashi, S., Iida, H., Oda, A., Osawa, Y., Uchida, M., and Dohi, S. (2007). Effects of ifenprodil on voltage-gated tetrodotoxin-resistant Na+ channels in rat sensory neurons. Eur. J. Anaesthesiol. 24, 782–788. doi:10.1017/S0265021507000440
Ueda, H. (2004). Locus-specific involvement of anti-opioid systems in morphine tolerance and dependence. Ann. N. Y. Acad. Sci. 1025, 376–382. doi:10.1196/annals.1307.046
Nikiforovich, G. V., Christinataylor, M., and Marshall, G. R. (2007). Modeling of the complex between transducin and photoactivated rhodopsin, a prototypical G-protein-coupled receptor. Biochemistry 46, 4734–4744. doi:10.1021/bi700185p
Wagner, L. E., Eaton, M., Sabnis, S. S., and Gingrich, K. J. (1999). Meperidine and lidocaine block of recombinant voltage-dependent Na+ channels: Evidence that meperidine is a local anesthetic. Anesthesiology 91, 1481–1490. doi:10.1097/00000542-199911000-00042
Wang, X., Kim, S. H., Ablonczy, Z., Crouch, R. K., and knapp, D. R. (2004). Probing rhodopsin-transducin interactions by surface modification and mass spectrometry. Biochemistry 43, 11153–11162. doi:10.1021/bi049642f
Watkins, L. R., Wiertelak, E. P., Goehler, L. E., Smith, K. P., Martin, D., and Maier, S. F. (1994). Characterization of cytokine-induced hyperalgesia. Brain Res. 654, 15–26. doi:10.1016/0006-8993(94)91566-0
Weiss, M., Hubner, G. H., Hubner, I. G., and Teichmann, W. (1996). Effects of cardiac output on disposition kinetics of sorbitol: Recirculatory modelling. Br. J. Clin. Pharmacol. 41, 261–268. doi:10.1046/j.1365-2125.1996.03121.x
Wess, J. (2022). In vivo metabolic roles of G proteins of the Gi family studied with novel mouse models. Endocrinology 163, bqab245. doi:10.1210/endocr/bqab245
Yan, J., Melemedjian, O. K., Price, T. J., and Dussor, G. (2012). Sensitization of dural afferents underlies migraine-related behavior following meningeal application of interleukin-6 (IL-6). Mol. Pain 8, 6. doi:10.1186/1744-8069-8-6
Yasui, Y., Masaki, E., and Kato, F. (2011). Esmolol modulates inhibitory neurotransmission in the substantia gelatinosa of the spinal trigeminal nucleus of the rat. BMC Anesthesiol. 11, 15. doi:10.1186/1471-2253-11-15
Yu, E. H., Tran, D. H., Lam, S. W., and Irwin, M. G. (2016). Remifentanil tolerance and hyperalgesia: Short-term gain, long-term pain? Anaesthesia 71, 1347–1362. doi:10.1111/anae.13602
Zaugg, M., Tagliente, T., Lucchinetti, E., Jacobs, E., Krol, M., Bodian, C., et al. (1999). Beneficial effects from beta-adrenergic blockade in elderly patients undergoing noncardiac surgery. Anesthesiology 91, 1674–1686. doi:10.1097/00000542-199912000-00020
Zhang, X., Bao, L., and Li, S. (2015). Opioid receptor trafficking and interaction in nociceptors. Br. J. Pharmacol. 172, 364–374. doi:10.1111/bph.12653
Zhao, H., Sugawara, T., Miura, S., Iijima, T., and Kashimoto, s. (2007). Intrathecal landiolol inhibits nociception and spinal c-Fos expression in the mouse formalin test. Can. J. Anaesth. 54, 201–207. doi:10.1007/BF03022641
Zheng, H., Loh, H. H., and Law, P. Y. (2010). Agonist-selective signaling of G protein-coupled receptor: Mechanisms and implications. IUBMB Life 62, 112–119. doi:10.1002/iub.293
Keywords: β-receptor antagonist, antinociception, cytokines, neurotransmitters, GPCRs, Sp5c
Citation: Fu L, Yu B, Li Z and Liu Z (2022) The therapeutic potential of ultra-short-acting β-receptor antagonists in perioperative analgesic: Evidence from preclinical and clinical studies. Front. Pharmacol. 13:914710. doi: 10.3389/fphar.2022.914710
Received: 07 April 2022; Accepted: 20 September 2022;
Published: 11 October 2022.
Edited by:
Muhammad Afzal, Jouf University, Saudi ArabiaReviewed by:
Renato Leonardo De Freitas, São Paulo State University, BrazilCopyright © 2022 Fu, Yu, Li and Liu. This is an open-access article distributed under the terms of the Creative Commons Attribution License (CC BY). The use, distribution or reproduction in other forums is permitted, provided the original author(s) and the copyright owner(s) are credited and that the original publication in this journal is cited, in accordance with accepted academic practice. No use, distribution or reproduction is permitted which does not comply with these terms.
*Correspondence: Zheng Li, bHpoZW5nNTE1QDE2My5jb20=; Zhiheng Liu, emhpaGVuZ19saXVfdG9uZ2ppQDE2My5jb20=
Disclaimer: All claims expressed in this article are solely those of the authors and do not necessarily represent those of their affiliated organizations, or those of the publisher, the editors and the reviewers. Any product that may be evaluated in this article or claim that may be made by its manufacturer is not guaranteed or endorsed by the publisher.
Research integrity at Frontiers
Learn more about the work of our research integrity team to safeguard the quality of each article we publish.