- 1Liaoning Key Laboratory of Diabetic Cognitive and Perceptive Dysfunction, Jinzhou Medical University, Jinzhou, China
- 2Department of Neurosurgery, West China Hospital, Sichuan University, Chengdu, China
- 3Department of Encephalopathy, Affiliated Hospital of Traditional Chinese Medicine, Southwest Medical University, Luzhou, China
Objective: To investigate the effect of Zhilong Huoxue Tongyu capsule (ZLH) in the treatment of cerebral ischemia–reperfusion injury and determine the underlying molecular network mechanism.
Methods: The treatment effect of Zhilong Huoxue Tongyu capsule (ZLH) was evaluated for cerebral ischemia–reperfusion injury in middle cerebral artery occlusion (MACO) rat, and the underlying molecular network mechanism was explored by using molecular network analysis based on network pharmacology, bioinformatics including protein–protein interaction (PPI) network, Gene Ontology (GO), and Kyoto Encyclopedia of Genes and Genomes (KEGG), as well as molecular docking.
Results: The neurological function of rats in the ZLH group was significantly improved compared to those in the NS group (p = 0.000), confirming the positive effect of ZLH for the treatment of brain ischemia. There were 126 intersecting genes screened in ischemia–reperfusion cerebrum that are associated with several important biological processes, such as lipopolysaccharide, and the most important cell component, such as raft, as well as the most important molecular function pointed as cytokine receptor binding. The most important KEGG signaling pathway was the AGE-RAGE signaling pathway in diabetic complications. Moreover, according to the STRING interaction in the PPI network, 10 hub genes including MAPK14, FOS, MAPK1, JUN, MYC, RELA, ESR1, STAT1, AKT1, and IL6 were selected and exhibited in Cytoscape and molecular docking. Lastly, the relation between PPI, GO, and KEGG was analyzed. These findings indicated that multiple hub network genes have been involved in behavior improvement in cerebral ischemia–reperfusion rats subjected to ZLH treatment.
Conclusion: Zhilong Huoxue Tongyu capsule improves cerebral ischemia–reperfusion and is associated with multiple network gene expressions.
Introduction
Stroke, one of the three most common causes of death and disability, is increasing with the high-pressure life. About 70% of strokes are derived from ischemic, and the rest are intracerebral or subarachnoid hemorrhages (Sveinsson et al., 2014a). After stroke, patients suffered from various neurological disorders that prevent them from taking care of themselves, resulting in a serious impact on their quality of life, psychologically, as well as socially and financially, for both the patients and their families (Sveinsson et al., 2014b). Cell death may occur within minutes after cerebral ischemia attack, and thrombolytic therapy can be used to restore blood flow and reduce the morbidity and mortality of patients. It has been known that microcirculatory dysfunction and organ damage after cerebral ischemia–reperfusion, as a complex pathological process, play a crucial role in the process of cerebral ischemia–reperfusion, and the blood supply in the organ is first restricted, while the perfusion could restore and reoxygenate (Han et al., 2017). Cerebral ischemia–reperfusion causing the ischemic penumbra cell death may lead to additional injury in the bordering locations of the infarct core, which needs to develop several new methods so as to improve treatment effect.
Zhilong Huoxue Tongyu Capsule (ZLH) (Sichuan Medicine Z20070528) is an available traditional Chinese medicine (TCM) that could be utilized for the treatment of cerebral stroke (Liang et al., 2021; Liu et al., 2022). As a complex preparation, ZLH, consisting of Astragalus, Cassia twig, leech, Panax notoginseng, and chuanxiong, is effective in dispelling wind and dampness and relieving pain for vascular headache and migraine. Among of them, Astragalus can hold the surface for qi and fold sweat; cassia twig can generate muscle, warm the meridians, and pass through Yang Qi; leech can precipitate and break blood clots (Liu et al., 2021). However, the molecular network mechanism of ZLH in improving brain damage is largely unknown.
Network pharmacology is based on core target analysis, network interaction relationship analysis, and description of key biological processes and signaling pathways to explain the disease–target-drug co-association module, which could exhibit the network regulatory relationship between drugs and diseases, so as to uncover the mechanism of pharmaceutical agents (Li and Zhang, 2013; Zhao et al., 2019; Luo et al., 2020), especially molecular docking that reveals the relationship between the molecular structural formula and drug, and it may help us to find the hub gene to predict ligand–target interactions with drug components (Pinzi and Rastelli 2019; Song et al., 2020). All of these techniques could be used to explain the molecular network mechanism in diseases and drug addition.
In this study, we investigated the protective effect and network mechanism of ZLH against cerebral ischemia–reperfusion, by gene network analysis, molecular docking, and animal experiment.
Materials and Methods
Animals and Grouping
A total of 33 SD rats were provided by the Department of Experimental Animals, Kunming Medical University (No. SCXK (Yunnan) K2020-0004); the animal ethics code is KMMU20220854, and the rats were randomly grouped into the sham group (n = 12), NS group (n = 10), and ZLH group (n = 11). After 8 h of fasting, the rats were deeply anesthetized with 3% sodium pentobarbital and performed the right common carotid artery occlusion in rats, by inserting a wire into the internal carotid artery. Then, the wound was sutured till the embolization was removed after 1 h (Liu Haixiao et al., 2019).
Drug Therapy
A total of 40 capsules of ZLH were dissolved in 100 ml of saline (concentration of 16 g/ml), and 2 ml/day was intragastrically administered to each rat in the ZLH group. The rats in the NS group were given 2 ml/day of saline, and the sham group was fed and watered as usual. All rats in each group were observed until sacrificed at day 5.
Zea-Longa Scores
All rats were assessed 24 h after awakening from anesthesia by using Zea-Longa scores. The scoring criteria were as follows: 1) no signs of nerve damage, 0 points; 2) inability to fully extend the contralateral front paw, 1 point; 3) rotate to the opposite side while crawling, 2 points; 4) falling to the opposite side while standing or crawling, 3 points; 5) inability to walk on their own and loss of consciousness, 4 points. The higher the score indicates the more severe the behavioral disorder of the animal.
Searching for Drug Components and Targets
The components and related target proteins of Astragalus, Cassia twig, leech, Panax notoginseng, and chuanxiong in ZLH were extracted by TCMSP (https://old.tcmsp-e.com/tcmsp.php), according to the condition of oral bioavailability (OB) that was set at > 20 and drug similarity (DL) was at >0.15. After retrieving the active ingredient of the drug, the name of the target protein corresponding to the active ingredient is checked in “related targets” according to the molarity of the active ingredient, and the name of the gene corresponding to the target protein is correspondingly searched in UniProt.
Searching for Gene Clusters of Cerebral Ischemia–Reperfusion
After entering the keyword “cerebral ischemia-reperfusion” in GeneCards (https://www.genecards.org/), we downloaded a list of genes related to cerebral ischemia–reperfusion.
Venny Intersection Diagram
The genes involved in cerebral ischemia–reperfusion were screened, and the Venny intersection map with ZLH was constructed (Venny 2.1.0, https://bioinfogp.cnb.csic.es/tools/venny/index.html). We input the gene name using “BI” in List1 and “ZL” in List2 and then constructed the Venny intersection map.
Gene Ontology Enrichment and Kyoto Encyclopedia of Genes and Genomes Pathway Analysis
The R software (version 4.1.0) was used to perform the GO enrichment and KEGG pathway analysis of the intersection obtained in Venny. In the Java environment, after the computer was first installed with the activePerl.exe file, org.Hs.eg.db, DOSE, clusterProfiler and pathView Enrichplot, ” we then used the scripts of SymolID, GO, and KEGG file packages to analyze the gene network, which includes the biological processes (BP), cellular components (CC), and molecular functions (MF), and the KEGG signaling pathway that can be obtained.
Protein–Protein Interaction Network
PPI network analysis (https://cn.string-db.org/) was performed using the intersection derived from the analysis in Venny 2.1.0. The results were then obtained by selecting multiple proteins and entering the intersecting genes in the “list of name” and selecting “Homo sapiens” for analysis.
Screening of Hub Genes
The interaction result obtained from PPI analysis was imported into Cytoscape 3.8.2 to screen hub genes, and 10 hub genes were screened based on degree values.
Molecular Docking
The screened hub genes were input into PDB, and the corresponding protein structures were downloaded; then, the corresponding gene drugs were entered into PubChem, and their 2D structures were downloaded; then, the SDF format of the receptors was converted to the MOL2 format using Open Babel. Subsequently, the ligand and receptor are then separated by dehydration using PyMOL software, with the target protein of the gene as the receptor and the corresponding drug as the ligand. Next, molecular docking was performed in AutoDock Vina to complete the docking and analysis, and the docking results were exported in the PDBQT format and converted from the PDBQT format to PDB format using Open Babel. Finally, PyMOL software was used to adjust the color of the large protein, process the ligands and residues, and calculate the length of hydrogen bonds. Lastly, the ligand and the overall picture are exported.
Cytoscape Diagram of Disease–Drug Active Component–Key Target–KEGG Pathway
All tables were imported into Cytoscape and divided into five types, including 1) type 1, drug mole number and corresponding genes; 2) type 2, disease name and disease gene; 3) type 3, KEGG signaling pathways and corresponding genes; 4) type 4, drug name and mole number; 5) type 5, KEGG signaling pathways. The disease–drug active component–key target–KEGG pathway diagram was constructed rationally by changing its shape, color, and position.
Results
Gene Cluster for ZLH and Cerebral Ischemia–Reperfusion
A total of 199 genes of components and related target proteins for ZLH were examined (Table 1), and 1,493 genes for cerebral ischemia–reperfusion were screened out (Table 2).
Intersection Genes Between ZLH and Cerebral Ischemia–Reperfusion
A total of 199 genes for ZLH and 1,493 genes for cerebral ischemia–reperfusion were crossed in Venny 2.1.0, and 126 intersecting genes were obtained (Figure 1; Table 3).
GO Analysis
The results of enrichment analysis showed that the first 20 pathways of biological process (BP), cellular component (CC), and molecular function (MF) were analyzed. The most important biological process was response to lipopolysaccharide; the main component of lipopolysaccharide was the outer membrane of Gram-negative bacteria, leading to the immune reaction. It plays an early warning role in inflammatory response after cerebral ischemia–reperfusion. The most important cell group is raft, which plays a regulatory role in the area around cerebral infarction. The most important molecular function is cytokine receptor binding, which activates intracellular enzymes to regulate gene expression (Figure 2).
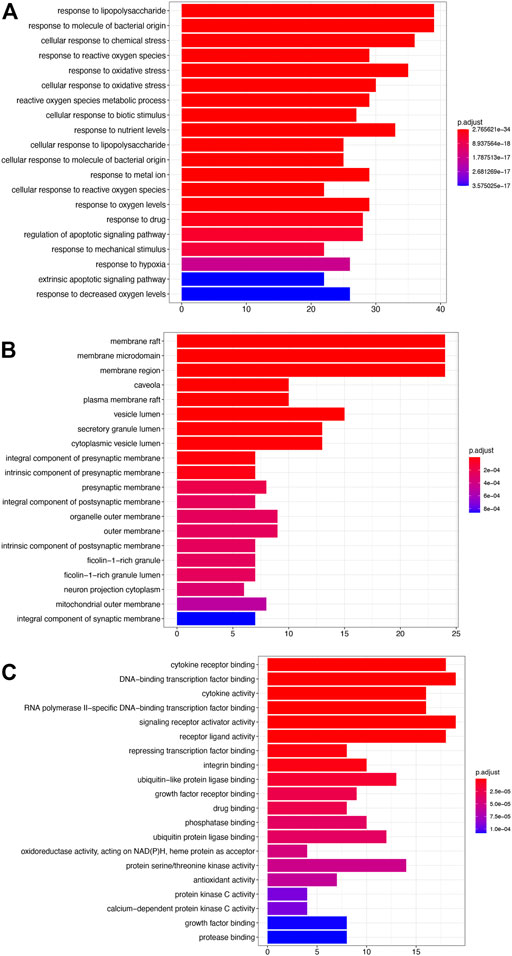
FIGURE 2. GO analysis. (A) Biological processes (BP). (B) Cellular components (CC). (C) Molecular functions (MF).
KEGG Signaling Pathways
The results showed that the top 10 KEGG signaling pathways were as follows: AGE-RAGE signaling pathway in diabetic complications, lipid and atherosclerosis, fluid shear stress and atherosclerosis, IL-17 signaling pathway, TNF signaling pathway, Kaposi sarcoma-associated herpesvirus infection, hepatitis B, HIF-1 signaling pathway, human cytomegalovirus infection, and Chagas disease (Figure 3).
PPI Network Analysis and Hub Genes Screening
PPI Network Analysis
In total, 126 intersection genes were obtained from Venny 2.1.0, shown in Figure 4A and Table 4). The 10 pairs of genes that interacted most closely according to COMBINed_score in descending order were: FLT1: VEGFA, AKT1: NOS3, CAV1: EGFR, CAV1: NOS3, CCND1: CDKN1A, CCND1: ESR1, CHUK: NFKBIA, EGF: EGFR, ESR1: JUN, and FOS: JUN.
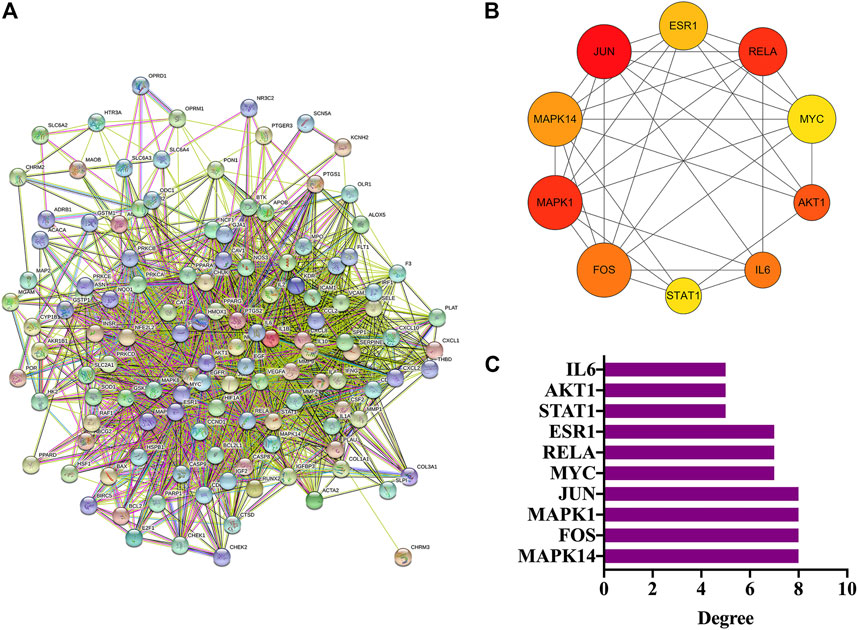
FIGURE 4. PPI network analysis and hub genes. (A) PPI network analysis. (B) Screening of hub genes. (C) Degree value of hub genes.
Screening of Hub Genes
The top 10 genes were sorted by the degree value, and the circle from the largest to smallest represented the decreasing degree value. As shown, the top 10 hub genes were MAPK14, FOS, MAPK1, JUN, MYC, RELA, ESR1, STAT1, AKT1, and IL6 (Figures 4B,C).
Results of Molecular Docking
Based on the 10 target proteins screened in Cytoscape, molecular docking was carried out with ZLH. The crystal structures of the target proteins were downloaded from the PDB database, formatted by Open Babel, and molecular docking of the target proteins to the ZLH was performed in AutoDock Vina. Finally, PyMOL software was used to process and adjust the color of the large protein, process the ligands and residues, calculate the length of hydrogen bonds, and export the ligands and the overall picture (Figure 5).
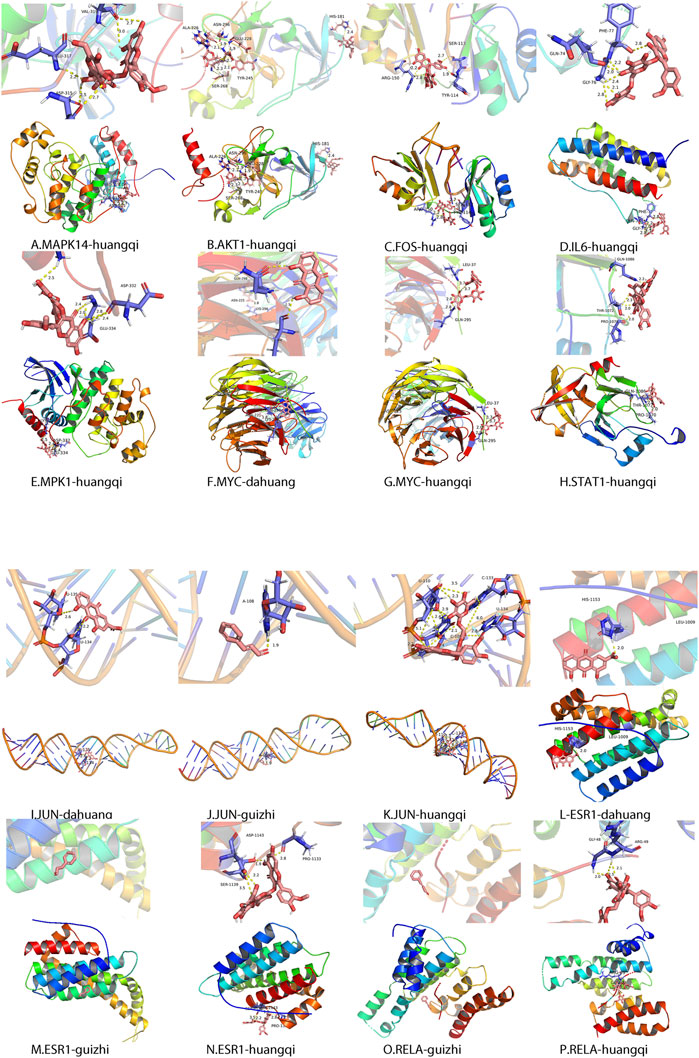
FIGURE 5. Molecular docking of related protein molecules of ZLH. (A) MAPK14–Huangqi. (B) AKT1–Huangqi. (C) FOS–Huangqi. (D) IL-6–Huangqi. (E) MAPK1–Huangqi. (F) MYC–Dahuang. (G) MYC–Huangqi. (H) STAT1–Huangqi. (I) JUN–Dahuang. (J) JUN–Guizhi. (K) Jun–Huangqi. (L) ESR1–Dahuang. (M) ESR1–Guizhi. (N)ESR1–Huangqi. (O) RELA–Guizhi. (P) RELA–Huangqi.
Cytoscape Map
The disease–drug active component–key target–KEGG signaling pathway diagram was constructed by importing the 44 active ingredients corresponding to ZLH, 126 common cross-targets of cerebral ischemia–reperfusion and ZLH, and the first 10 pathways of KEGG into Cytoscape software. As a result, we constructed the network interaction among all related targets, in which, the closely related pathways were linked in the figure to illustrate the regulatory role of ZLH on cerebral ischemia–reperfusion (Figure 6).
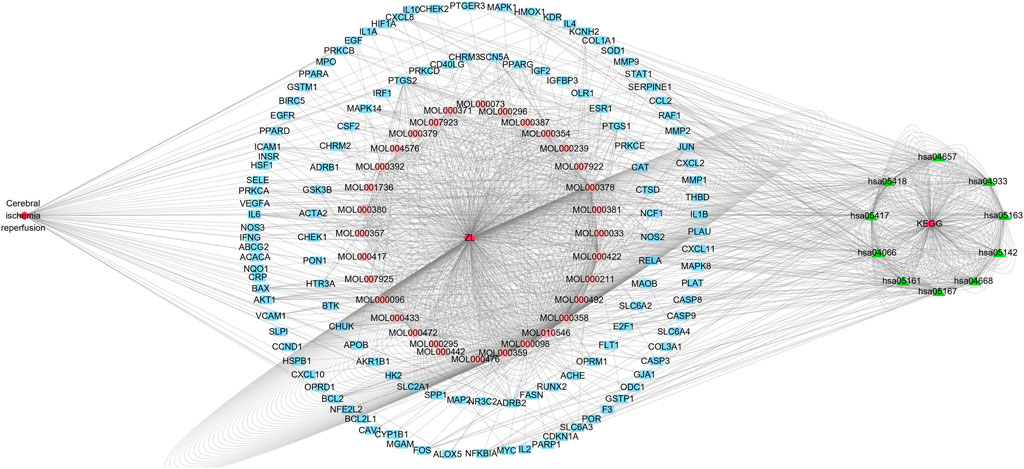
FIGURE 6. Cytoscape diagram for cerebral ischemia–reperfusion, ZLH administration, and KEGG signaling pathways. The left exhibited cerebral ischemia–reperfusion disease and the middle was the MOL number from ZLH and associated 44 active components, whereas the outer blue circle was the common gene of cerebral ischemia–reperfusion and ZLH. Lastly, the first 10 KEGG signaling pathways were put on the right side.
The Relation of Hub Genes With PPI, GO, and KEGG Pathway
To know the relation and link of hub genes with PPI, GO, and KEGG pathway, we compared the position of hub genes in the GO and KEGG pathway. We found that almost all hub gens were involved in BP and selected signal pathways, but only MAPK1 or Mapk14 were involved in CC. These pointed out that 10 hub genes selected from PPI are most importantly related to the findings of GO analysis and signal pathway. In addition, the molecular hub genes like MAPK14, FOS, MAPK1, and JUN may simultaneously locate in different signaling pathways involved in the AGE-RAGE signaling pathway in diabetic complications, lipid and atherosclerosis, fluid shear stress and atherosclerosis, IL-17 signaling pathway, TNF signaling pathway, Kaposi sarcoma-associated herpesvirus infection, hepatitis B, HIF-1 signaling pathway, human cytomegalovirus infection, and Chagas disease, which means that some co-expression genes scattered in different signaling pathways. In turn, the same signaling pathway like the IL-17 signaling pathway includes different genes including MAPK14, FOS, MAPK1, JUN, RELA, and AKT1, simultaneously (Table 5).
Zea-Longa Scores
Zea-Longa scores were performed on the sham group, NS group, and ZLH group at 1, 3, and 5 days after surgery. The results showed a significant increase in neurological dysfunction in the NS group compared to the sham group at 5 days after surgery, whereas it reversed in the ZLH-treated group, compared to the NS group (p = 0.000, Figure 7).
Discussion
In the present study, we confirmed the effect of ZLH on neurological function repair in brain ischemic rats and determined the gene network in the cerebral ischemia–reperfusion model (Sun et al., 2015). We screened the relevant components and targets of ZLH in TCMSP and found the cerebral ischemia–reperfusion genes in GeneCards (Huang et al., 2020; Li et al., 2021) and then conducted a Venny intersection diagram to show cross genes, in which, 126 genes were selected in the intersection between ZLH and cerebral ischemia–reperfusion. The GO enrichment and KEGG signaling pathways are linked to lipopolysaccharide, membrane raft, cytokine receptor binding, and AGE-RAGE signaling pathway in diabetic complications, respectively (Ding and Zhang, 2017). Then, the top 10 gene pairs with the most close relationship in PPI network analysis reported were FLT1: VEGFA, AKT1: NOS3, CAV1: EGFR, CAV1: NOS3, CCND1: CDKN1A, CCND1: ESR1, CHUK: NFKBIA, EGF: EGFR, ESR1: JUN, and FOS: JUN, whereas the top 10 hub genes were screened out according to the degree value (Martino et al., 2021). Moreover, molecular docking confirmed that ZLH could form a stable molecular binding pattern, which targets directly with MAPK14, AKT1, FOS, IL6, MPK1 MYC, ESR1, JUN, RELA, and STAT1, but not with ESR1–Guizhi and Rela–Guizhi (Pinzi and Rastelli, 2019). Our results confirmed that multiple genes regulate and interact in ischemia–reperfusion cerebrum after ZLH treatment (Cui et al., 2020), which added new evidence to explain the mechanism of ZLH improving neurological function in cerebral ischemia–reperfusion rats.
Protective Effect of ZLH in Brain Ischemic Rats
After the brain ischemia model was successfully established and the high-dose treatment of ZLH was used, we found that the score of neurological impairment was higher in brain ischemia, and it is lower in the ZLH group at 5 days. This suggested that the neurological function of cerebral ischemia–reperfusion rats was remarkably improved after high-dose administration of ZLH. Previously, it has been reported that ZLH exhibited a positive effect for the treatment of brain damage (Han et al., 2014), but the protective mechanism of ZLH keeps to be known.
Pharmacological Analysis of Network and Its Significance
Network pharmacology can explain the relationship between drugs and gene network of diseases. Through network analysis, different genes regulating the disease and interacting genes on diseases could be found. Network pharmacology also analyzes the mechanism of action of TCM prescriptions on disease, therefore providing a new way to explain the molecular mechanism of TCM efficacy (Liu et al., 2020). Despite ZLH being used to dispel wind and dehumidify, relieve pain, and treat vascular headache and migraine, the molecular mechanism is largely unknown (She et al., 2019). Previously, it was only reported that ZLH has a therapeutic effect on acute cerebral infarction, and its safety and effectiveness were systematically evaluated (Liu et al., 2021); however, the gene network of ZLH is waiting to be explored. In this study, the gene network analysis and molecular docking between ZLH and cerebral ischemia disease were conducted (Liang et al., 2021), which laid the foundation for studying the mechanism of ZLH in treating cerebral ischemia–reperfusion.
GO Enrichment and KEGG Signaling Pathway Analysis Were Performed on the Core Intersection Genes
The genes of ZLH were intersected with the genes of cerebral ischemia–reperfusion, and 126 intersection genes were obtained. The GO enrichment and KEGG signaling pathway analysis of the intersection genes showed that these genes were located in lipopolysaccharide as the most important BP. The main component of lipopolysaccharide is the outer membrane of Gram-negative bacteria, which causes immune response and plays an early warning role in the inflammatory reaction after cerebral ischemia–reperfusion (Park and Lee, 2013). The most important CC includes membrane raft, which is produced by the interaction between lipids (Quinn, 2010) and plays a regulatory role in the area around cerebral infarction (Ruscher et al., 2011). The most important MF was cytokine receptor binding, which activates intracellular enzymes and thus regulates gene expression (Spangler et al., 2015). The AGE-RAGE signaling pathway in diabetic complications is the top one signaling pathway, which indicates that patients with cerebral ischemia may develop hyperglycemia, and AGE-RAGE has harmful effects on neuron injury and inflammation in diabetic patients (Weil, 2012). The second pathway, lipid and atherosclerosis, is associated with the formation of atherosclerotic plaques (Bäck, 2009). Fluid shear stress and atherosclerosis as the third pathway plays an important role in atherosclerotic plaque vulnerability (Chen et al., 2019). The fourth pathway, IL-17 signaling pathway, is an effective pro-inflammatory cytokine that plays a role in inflammatory response after cerebral infarction (Liu Ting et al., 2019). Moreover, the TNF signaling pathway, the fifth signaling pathway, retracted inflammatory response after cerebral ischemia–reperfusion injury (Patel et al., 2014). The sixth pathway, Kaposi sarcoma-associated herpesvirus infection, which is the pathogen of malignant tumors, should be protected from viral infection after defective reperfusion (Fröhlich and Grundhoff, 2020). The seventh pathway, hepatitis B, or hepatitis virus, and the eighth pathway, HIF-1 signaling pathway, may play an important regulatory role in reducing oxidative stress response and inflammatory response after stroke (Zhang et al., 2021). The ninth pathway involved is human cytomegalovirus infection that may occur after cerebral ischemia–reperfusion. Lastly, the tenth pathway, Chagas disease, can lead to chronic diseases such as stroke when the acute infection subsides (Pérez-Molina and Molina, 2018). The summary of these pathways provided the crucial evidence to understand the molecular events in brain ischemic rats after ZLH treatment.
PPI Interaction and Hub Gene Screening
The top 10 genes with the closest relationship were obtained, and we found that CCND1, ESR1, and JUN were connected with multiple genes in the top 10 pairs (Chakraborty et al., 2014). These closely related genes may play an important role in the treatment of cerebral ischemia–reperfusion with ZLH. The top 10 hub genes screened by the degree value in Cytoscape may be involved in a variety of cellular processes as the core regulatory genes in drugs and diseases (Deng et al., 2019).
Molecular Docking Validation
As key nodes, the 10 hub genes were speculated as core genes in cerebral ischemia–reperfusion with ZLH treatment (Wei et al., 2020). Each of these 10 genes is linked to the corresponding drug ligand, which was revealed by molecular docking (Xia et al., 2020). From molecular docking analysis, it was found that Astragalus was docked with MAPK14, AKT1, FOS, IL6, MPK1 MYC, ESR1, JUN, RELA, and STAT1; Dahuang was directly docked with JUN, ESR1, and MYC; Cassia twig was docked with JUN, ESR1, and RELA. However, ESR1 and RELA had no direct interaction with Cassia twig. Therefore, through molecular docking, we provided the vital explanation for the drug directly targeting the molecule (Jiao et al., 2021).
Network Relationships Between the Drugs and Diseases
By constructing a disease–drug active component–key target–KEGG pathway map, it can find the network interactions between the related targets (Niu et al., 2021). In this study, it was shown that the MOL number of ZLH had a relatively close node connection with cerebral ischemia–reperfusion and KEGG signaling pathways, which illustrates the interaction between ZLH and cerebral ischemia–reperfusion (He et al., 2020).
The Functional Implication of Hub Genes From PPI in GO and KEGG Pathway
The relation of hub genes with PPI, GO, and KEGG pathway was analyzed. Several important genes were linked with the different cellular locations, biological processes, and molecular functions, meanwhile sharing the different signal pathways (Xie et al., 2018). MAPK4, Jun, Fos, Real, and IL-6 were found located in four BPs and seven signal pathways, which means that some hub genes were scattered in different pathways. In turn, the same signal pathway may include different genes, simultaneously. These showed that hub molecules from PPI take part in the core biological process or signal transduction in our observation. To our knowledge, this is the first time to reveal the relation among PPI, GO, and KEGG in brain ischemic rats subjected to ZLH treatment.
Conclusion
In summary, we confirmed the effect of ZLH in protecting the ischemic brain from injury, and by using network pharmacology, molecular docking, and bioinformatics analysis, we explained the net mechanism in cerebral ischemia–reperfusion treated with ZLH. The results revealed that the effect of ZLH in improving neurological function is associated with multiple gene expressions in cerebral ischemia–reperfusion rats. Despite determining the effect of ZLH in treating brain ischemia and explaining the associated network mechanism, we suggest that the follow-up experimental research is absolutely needed to be developed to validate the deep mechanism.
Data Availability Statement
The original contributions presented in the study are included in the article/Supplementary Material; further inquiries can be directed to the corresponding authors.
Ethics Statement
The animal study was reviewed and approved by the Animal Experiment Ethics Review Committee of Kunming Medical University, and the approval number is KMMU20220854.
Author Contributions
T-HW and XB contributed to the conception and design of the study. NL wrote the first draft of the manuscript and analyzed the data, while JS and J-LC wrote part of the manuscript. All authors participated in revising, reading, and approving the submitted version.
Funding
Translational study of microrNA-target gene regulatory network in stroke and acute brain injury. Major Science and Technology Project of Sichuan Province (in the field of social development), Project No. 2020YFS0043, fund 1 million, period 2020.1-2021.12, principal: T-HW. General Project of Joint Special Fund for Applied Basic Research of Yunnan Science and Technology Department and Kunming Medical University in 2019, Project No. 2019FE001 (-025).
Conflict of Interest
The authors declare that the research was conducted in the absence of any commercial or financial relationships that could be construed as a potential conflict of interest.
Publisher’s Note
All claims expressed in this article are solely those of the authors and do not necessarily represent those of their affiliated organizations, or those of the publisher, the editors, and the reviewers. Any product that may be evaluated in this article, or claim that may be made by its manufacturer, is not guaranteed or endorsed by the publisher.
Acknowledgments
This article was completed under the careful guidance of T-HW. The author is very grateful to T-HW for his guidance and help in writing, as well as his support and encouragement. T-HW’s rigorous and realistic, meticulous research attitude and diligent working attitude have also deeply influenced the author, giving him great inspiration and encouragement, and this spirit of inspiration will remain valuable wealth in author’s life.
References
Bäck, M. (2009). Leukotriene Signaling in Atherosclerosis and Ischemia. Cardiovasc Drugs Ther. 23 (1), 41–48. doi:10.1007/s10557-008-6140-9
Chakraborty, C., Doss C, G. P., Chen, L., and Zhu, H. (2014). Evaluating Protein-Protein Interaction (PPI) Networks for Diseases Pathway, Target Discovery, and Drug-Design Using 'In Silico Pharmacology'. Curr. Protein Pept. Sci. 15 (6), 561–571. doi:10.2174/1389203715666140724090153
Chen, Z., Qin, H., Liu, J., Wu, B., Cheng, Z., Jiang, Y., et al. (2019). Characteristics of Wall Shear Stress and Pressure of Intracranial Atherosclerosis Analyzed by a Computational Fluid Dynamics Model: A Pilot Study. Front. Neurol. 10, 1372. doi:10.3389/fneur.2019.01372
Cui, Q., Zhang, Y. L., Ma, Y. H., Yu, H. Y., Zhao, X. Z., Zhang, L. H., et al. (2020). A Network Pharmacology Approach to Investigate the Mechanism of Shuxuening Injection in the Treatment of Ischemic Stroke. J. Ethnopharmacol. 257, 112891. doi:10.1016/j.jep.2020.112891
Deng, J. L., Xu, Y. H., and Wang, G. (2019). Identification of Potential Crucial Genes and Key Pathways in Breast Cancer Using Bioinformatic Analysis. Front. Genet. 10, 695. doi:10.3389/fgene.2019.00695
Ding, J., and Zhang, Y. (2018). Analysis of Key GO Terms and KEGG Pathways Associated with Carcinogenic Chemicals. Comb. Chem. High. Throughput. Screen. 20, 861–871. doi:10.2174/1386207321666171218120133
Fröhlich, J., and Grundhoff, A. (2020). Epigenetic Control in Kaposi Sarcoma-Associated Herpesvirus Infection and Associated Disease. Semin. Immunopathol. 42 (2), 143–157. doi:10.1007/s00281-020-00787-z
Han, D., Zhang, S., Fan, B., Wen, L. L., Sun, M., Zhang, H., et al. (2014). Ischemic Postconditioning Protects the Neurovascular Unit after Focal Cerebral Ischemia/reperfusion Injury. J. Mol. Neurosci. 53 (1), 50–58. doi:10.1007/s12031-013-0196-0
Han, J. Y., Li, Q., Ma, Z. Z., and Fan, J. Y. (2017). Effects and Mechanisms of Compound Chinese Medicine and Major Ingredients on Microcirculatory Dysfunction and Organ Injury Induced by Ischemia/reperfusion. Pharmacol. Ther. 177, 146–173. doi:10.1016/j.pharmthera.2017.03.005
He, R., Ou, S., Chen, S., and Ding, S. (2020). Network Pharmacology-Based Study on the Molecular Biological Mechanism of Action for Compound Kushen Injection in Anti-cancer Effect. Med. Sci. Monit. 26, e918520. doi:10.12659/msm.918520
Huang, Z., Shi, X., Li, X., Zhang, L., Wu, P., Mao, J., et al. (2020). Network Pharmacology Approach to Uncover the Mechanism Governing the Effect of Simiao Powder on Knee Osteoarthritis. Biomed. Res. Int. 2020, 6971503. doi:10.1155/2020/6971503
Jiao, X., Jin, X., Ma, Y., Yang, Y., Li, J., Liang, L., et al. (2021). A Comprehensive Application: Molecular Docking and Network Pharmacology for the Prediction of Bioactive Constituents and Elucidation of Mechanisms of Action in Component-Based Chinese Medicine. Comput. Biol. Chem. 90, 107402. doi:10.1016/j.compbiolchem.2020.107402
Li, B., Lin, H., Tang, Z. Q., Wang, C. F., Su, H. L., Wang, Y. X., et al. (2021). Study on Mechanism of Phytolaccae Radix and its Split Components Based on Network Pharmacology. Zhongguo Zhong Yao Za Zhi 46 (10), 2434–2442. doi:10.19540/j.cnki.cjcmm.20210120.401
Li, S., and Zhang, B. (2013). Traditional Chinese Medicine Network Pharmacology: Theory, Methodology and Application. Chin. J. Nat. Med. 11 (2), 110–120. doi:10.1016/s1875-5364(13)60037-0
Liang, P., Mao, L., Ma, Y., Ren, W., and Yang, S. (2021). A Systematic Review on Zhilong Huoxue Tongyu Capsule in Treating Cardiovascular and Cerebrovascular Diseases: Pharmacological Actions, Molecular Mechanisms and Clinical Outcomes. J. Ethnopharmacol. 277, 114234. doi:10.1016/j.jep.2021.114234
Liu, H., Wu, X., Luo, J., Wang, X., Guo, H., Feng, D., et al. (2019). Pterostilbene Attenuates Astrocytic Inflammation and Neuronal Oxidative Injury after Ischemia-Reperfusion by Inhibiting NF-Κb Phosphorylation. Front. Immunol. 10, 2408. doi:10.3389/fimmu.2019.02408
Liu, M., Luo, G., Liu, T., Yang, T., Wang, R., Ren, W., et al. (2022). Zhilong Huoxue Tongyu Capsule Alleviated the Pyroptosis of Vascular Endothelial Cells Induced by Ox-LDL through miR-30b-5p/NLRP3. Evid. Based Complement. Altern. Med. 2022, 3981350. doi:10.1155/2022/3981350
Liu, M., Pu, Y., Gu, J., He, Q., Liu, Y., Zeng, Y., et al. (2021). Evaluation of Zhilong Huoxue Tongyu Capsule in the Treatment of Acute Cerebral Infarction: A Systematic Review and Meta-Analysis of Randomized Controlled Trials. Phytomedicine 86, 153566. doi:10.1016/j.phymed.2021.153566
Liu, T., Han, S., Dai, Q., Zheng, J., Liu, C., Li, S., et al. (2019). IL-17A-Mediated Excessive Autophagy Aggravated Neuronal Ischemic Injuries via Src-Pp2b-mTOR Pathway. Front. Immunol. 10, 2952. doi:10.3389/fimmu.2019.02952
Liu, T. H., Chen, W. H., Chen, X. D., Liang, Q. E., Tao, W. C., Jin, Z., et al. (2020). Network Pharmacology Identifies the Mechanisms of Action of TaohongSiwu Decoction against Essential Hypertension. Med. Sci. Monit. 26, e920682. doi:10.12659/msm.920682
Luo, T. T., Lu, Y., Yan, S. K., Xiao, X., Rong, X. L., and Guo, J. (2020). Network Pharmacology in Research of Chinese Medicine Formula: Methodology, Application and Prospective. Chin. J. Integr. Med. 26 (1), 72–80. doi:10.1007/s11655-019-3064-0
Martino, E., Chiarugi, S., Margheriti, F., and Garau, G. (2021). Mapping, Structure and Modulation of PPI. Front. Chem. 9, 718405. doi:10.3389/fchem.2021.718405
Niu, W. H., Wu, F., Cao, W. Y., Wu, Z. G., Chao, Y. C., and Liang, C. (2021). Network Pharmacology for the Identification of Phytochemicals in Traditional Chinese Medicine for COVID-19 that May Regulate Interleukin-6. Biosci. Rep. 41 (1), BSR20202583. doi:10.1042/bsr20202583
Park, B. S., and Lee, J. O. (2013). Recognition of Lipopolysaccharide Pattern by TLR4 Complexes. Exp. Mol. Med. 45 (12), e66. doi:10.1038/emm.2013.97
Patel, F. J., Volkmann, D. T., Taylor, G. W., Hansson, M. A., Anderson, J. F., Zhou, Y., et al. (2014). Retracted: IL-37 Reduces Inflammatory Response after Cerebral Ischemia and Reperfusion Injury through Down-Regulation of Pro-inflammatory Cytokines. Cytokine 69 (2), 234–239. doi:10.1016/j.cyto.2014.06.011
Pérez-Molina, J. A., and Molina, I. (2018). Chagas Disease. Lancet 391 (10115), 82–94. doi:10.1016/s0140-6736(17)31612-4
Pinzi, L., and Rastelli, G. (2019). Molecular Docking: Shifting Paradigms in Drug Discovery. Int. J. Mol. Sci. 20 (18), 4331. doi:10.3390/ijms20184331
Quinn, P. J. (2010). A Lipid Matrix Model of Membrane Raft Structure. Prog. Lipid Res. 49 (4), 390–406. doi:10.1016/j.plipres.2010.05.002
Ruscher, K., Shamloo, M., Rickhag, M., Ladunga, I., Soriano, L., Gisselsson, L., et al. (2011). The Sigma-1 Receptor Enhances Brain Plasticity and Functional Recovery after Experimental Stroke. Brain 134 (Pt 3), 732–746. doi:10.1093/brain/awq367
She, Y., Shao, L., Zhang, Y., Hao, Y., Cai, Y., Cheng, Z., et al. (2019). Neuroprotective Effect of Glycosides in Buyang Huanwu Decoction on Pyroptosis Following Cerebral Ischemia-Reperfusion Injury in Rats. J. Ethnopharmacol. 242, 112051. doi:10.1016/j.jep.2019.112051
Song, X., Zhang, Y., Dai, E., Wang, L., and Du, H. (2020). Prediction of Triptolide Targets in Rheumatoid Arthritis Using Network Pharmacology and Molecular Docking. Int. Immunopharmacol. 80, 106179. doi:10.1016/j.intimp.2019.106179
Spangler, J. B., Moraga, I., Mendoza, J. L., and Garcia, K. C. (2015). Insights into Cytokine-Receptor Interactions from Cytokine Engineering. Annu. Rev. Immunol. 33, 139–167. doi:10.1146/annurev-immunol-032713-120211
Sun, K., Fan, J., and Han, J. (2015). Ameliorating Effects of Traditional Chinese Medicine Preparation, Chinese Materia Medica and Active Compounds on Ischemia/reperfusion-Induced Cerebral Microcirculatory Disturbances and Neuron Damage. Acta Pharm. Sin. B 5 (1), 8–24. doi:10.1016/j.apsb.2014.11.002
Sveinsson, O. A., Kjartansson, O., and Valdimarsson, E. M. (2014a). Cerebral Ischemia/infarction - Epidemiology, Causes and Symptoms. Laeknabladid 100 (5), 271–279. doi:10.17992/lbl.2014.05.543
Sveinsson, Ó. Á., Kjartansson, Ó., and Valdimarsson, E. M. (2014b). Cerebral Ischemia/infarction - Diagnosis and Treatment. Laeknabladid 100 (7-8), 393–401. doi:10.17992/lbl.2014.0708.553
Wei, M., Li, H., Li, Q., Qiao, Y., Ma, Q., Xie, R., et al. (2020). Based on Network Pharmacology to Explore the Molecular Targets and Mechanisms of Gegen Qinlian Decoction for the Treatment of Ulcerative Colitis. Biomed. Res. Int. 2020, 5217405. doi:10.1155/2020/5217405
Weil, Z. M. (2012). Ischemia-induced Hyperglycemia: Consequences, Neuroendocrine Regulation, and a Role for RAGE. Horm. Behav. 62 (3), 280–285. doi:10.1016/j.yhbeh.2012.04.001
Xia, Q. D., Xun, Y., Lu, J. L., Lu, Y. C., Yang, Y. Y., Zhou, P., et al. (2020). Network Pharmacology and Molecular Docking Analyses on Lianhua Qingwen Capsule Indicate Akt1 Is a Potential Target to Treat and Prevent COVID-19. Cell Prolif. 53 (12), e12949. doi:10.1111/cpr.12949
Xie, W., Zhou, P., Sun, Y., Meng, X., Dai, Z., Sun, G., et al. (2018). Protective Effects and Target Network Analysis of Ginsenoside Rg1 in Cerebral Ischemia and Reperfusion Injury: A Comprehensive Overview of Experimental Studies. Cells 7 (12), 270. doi:10.3390/cells7120270
Zhang, C., Zhen, L., Fang, Z., Yu, L., Zhang, Y., Wei, H., et al. (2021). Adiponectin Treatment Attenuates Cerebral Ischemia-Reperfusion Injury through HIF-1α-Mediated Antioxidation in Mice. Oxid. Med. Cell Longev. 2021, 5531048. doi:10.1155/2021/5531048
Keywords: ZhiLong HuoXue TongYu capsule, cerebral ischemia–reperfusion, network pharmacology, molecular docking, gene network analysis
Citation: Li N, Sun J, Chen J-L, Bai X and Wang T-H (2022) Gene Network Mechanism of Zhilong Huoxue Tongyu Capsule in Treating Cerebral Ischemia–Reperfusion. Front. Pharmacol. 13:912392. doi: 10.3389/fphar.2022.912392
Received: 05 April 2022; Accepted: 16 May 2022;
Published: 07 July 2022.
Edited by:
Yu-Sheng Wu, National Pingtung University of Science and Technology, TaiwanReviewed by:
Tai-Tu Lin, Pacific Northwest National Laboratory (DOE), United StatesNahid Aboutaleb, Iran University of Medical Sciences, Iran
Copyright © 2022 Li, Sun, Chen, Bai and Wang. This is an open-access article distributed under the terms of the Creative Commons Attribution License (CC BY). The use, distribution or reproduction in other forums is permitted, provided the original author(s) and the copyright owner(s) are credited and that the original publication in this journal is cited, in accordance with accepted academic practice. No use, distribution or reproduction is permitted which does not comply with these terms.
*Correspondence: Ting-Hua Wang, d2FuZ3RpbmdodWFAdmlwLjE2My5jb20=; Xue Bai, Yng3MjQ2QDE2My5jb20=
†These authors have contributed equally to this work and share first authorship